- 1Jiangsu Province Key Laboratory of Soil and Water Conservation and Ecological Restoration, Nanjing Forestry University, Nanjing, Jiangsu, China
- 2Jiangxi Institute of Land Space Survey and Planning, Nanchang, Jiangxi, China
- 3Technology Innovation Center for Land Spatial Eco-protection and Restoration in Great Lakes Basin, Ministry of Natural Resources (MNR), Nanchang, Jiangxi, China
Introduction: The stability of exposed slopes is prone to natural disasters, seriously threatening socio-economic and human security. Through years of exploration and research, we proposed an active permanent greening (APG) method based on patented mineral solubilizing microorganisms (MSMs) as an improvement over the traditional greening method.
Methods: In this study, we selected two MSMs (Bacillus thuringiensis and Gongronella butleri) and a plant species (Lolium perenne L.) set up six treatments (T1, T2, T3, T4, T5, and T6) to investigate the effectiveness of the MSMs and their combinations with the plant species on the soil stability using APG method.
Results: We noted that both MSMs and the plant species significantly improved soil aggregate stability and organic matter content. Of all the treatments, the T1 treatment exhibited better results, with soil aggregate stability and organic matter content increased to 45.63% and 137.57%, respectively, compared to the control. Soil stability was significant positively correlated with macroaggregate content and negatively with microaggregates. Using structural equation modeling analysis, we further evaluated the mechanism underpinning the influence of organic matter content and fractions on the content of each graded agglomerates. The analysis showed that the macroaggregate content was influenced by the presence of the plant species, primarily realized by altering the content of organic matter and aromatic and amide compounds in the agglomerates, whereas the microaggregate content was influenced by the addition of MSMs, primarily realized by the content of organic matter and polysaccharide compounds. Overall, we observed that the effect of the co-action of MSMs and the plant species was significantly better than that of using MSMs or the plant species alone.
Discussion: The findings of this study provide reliable data and theoretical support for the development and practical application of the APG method to gradually develop and improve the new greening approach.
1 Introduction
The utilization of natural resources has greatly contributed to the social and economic progress of mankind (Shao, 2019). However, while providing human and food security, it has resulted in several negative impacts on the environment (Okello et al., 2015; Vanwalleghem et al., 2017; Kattel, 2022), including the formation of a large number of exposed slopes (Wang Y. et al., 2022). Exposed slopes can deteriorate the local microclimate, causing a series of environmental problems, such as landslides and mudslides, and seriously threatening the safety of human life and property (Dyregrov et al., 2018; Newnham et al., 2019; Rosselló et al., 2020). Relying on nature’s power to repair exposed slopes is almost difficult to realize. Therefore, an ever-increasing number of experts and scholars are committed to finding efficient and economical ways to restore the ecology of exposed slopes (Huang et al., 2017; Li et al., 2018; Zhang et al., 2018).
The common technologies for the treatment and restoration of exposed slopes worldwide include the spraying greening method, three-dimensional planting network greening method, vegetation green carpet, planting hole greening, and thick substrate technology (Li et al., 2017; Tang et al., 2018; Li et al., 2019; Liu et al., 2019; Wang Y. et al., 2022). Based on these technologies, a series of studies and engineering practices have been conducted in various countries to continuously optimize the greening substrate and improve engineering technology, and as a result, a variety of new technologies have been gradually developed (Huang et al., 2017; Li et al., 2017; Broda and Gawlowski, 2018; Li et al., 2018; Wang Y. et al., 2022). However, existing technologies still exhibit numerous issues (Tang et al., 2018; Li et al., 2019; Faiz et al., 2022), such as poor adaptability of the substrate on slopes, poor erosion resistance, and ease of spalling. Therefore, improving slope soil stability is key to realizing permanent greening of slopes.
Soil environment reconstruction is the priority of permanent greening of bare slopes (Cao et al., 2015; Han et al., 2019). Soil aggregates are the basic structural units of soil, and their stability is an important indicator of how well the soil performs its coordination mechanisms and environmental functions (Li T. et al., 2020; Guhra et al., 2022). The fragmentation of aggregates has long been recognized as the first and most critical step in the occurrence of soil erosion, which directly affects the stability of soil on slopes, making the study of soil aggregate stability particularly important (Cui et al., 2022; Li et al., 2022; Wang et al., 2023). Nonetheless, there is no unanimous conclusion on the most critical factors affecting the stability of aggregates, but it is widely accepted that the content of soil organic matter is the key to soil aggregate stability (Tisdall and Oades, 1982; Boyle et al., 1989; Obalum et al., 2017; Sarker et al., 2018; Mustafa et al., 2020; Zhou et al., 2020; Voltr et al., 2021).
Microorganisms play an important role in biogeochemical cycles and have great potential for restoring soil function and re-establishing ecological balance (Bertrand et al., 2015). They not only provide nutrients to plants by solubilizing and releasing metal ions from minerals but also change soil nutrient levels through acidolysis and complexation (Khan et al., 2007; Kamran et al., 2017). They can also improve soil conditions, promote plant growth, and increase stress resistance by utilizing microbial metabolism, hormone production, and antagonism (Giassi et al., 2016; Wang et al., 2019; Deng et al., 2020; Abulfaraj and Jalal, 2021). In addition, soil microorganisms secrete and produce organic cementing substances, such as polysaccharides or cement soil clay particles, through mycelia (Yang et al., 2015; Ogarkov et al., 2018; Barbosa et al., 2019; Ji et al., 2019; Chung et al., 2021), thereby promoting the formation of aggregates (Sandhya and Ali, 2015; Deka et al., 2018).
Given the great potential of microorganisms in the revegetation of slopes, we proposed an active permanent greening (APG) method based on selected mineral solubilizing microorganisms (MSMs) (Wang L. et al., 2023). We isolated a variety of microorganisms from the dolomite rock wall of the Mufu Mountains, Nanjing, and selected four typical strains (Jinchi et al., 2014; Guanglin et al., 2015; Jinchi et al., 2015a; Jinchi et al., 2015b) for use in slope management after preliminary experiments on cultivation and solubilization mechanisms. However, studies have been conducted on the effects of MSMs on soil nutrients and plant growth, yet soil aggregates have not been investigated. Therefore, based on the results of previous studies, we selected two superior strains and set up six treatments for controlled experiment. The objectives of the study were (1) to investigate the effects of MSMs and their combination with a plant species on the distribution and stability of soil aggregates, (2) to investigate the effects of MSMs and their combination with the plant species on the content and properties of soil organic matter, and (3) to explore the intrinsic mechanism by which MSMs and their combination with a plant species enhance slope stability by regulating soil aggregation structure.
This study explored the effect of organic matter on soil aggregate stability and its mechanism under the action of microorganisms and plants, and the results will enrich the existing literature on soil stability research. Meanwhile, it demonstrates the role of MSMs in slope management more comprehensively, and helps to promote the systematic research of APG methods, solve the problems related to the traditional greening methods that are difficult to maintain in the long term, and form an efficient, environmentally friendly and sustainable greening technology.
2 Materials and methods
2.1 Microorganism strains
The strains NL-11 and NL-15 used in this study were isolated from the surface of the weathered rock wall of the Mufu Mountains, Nanjing (rock properties: CaO, 62.34%; MgO, 27.93%; K2O, 1.75%; Fe2O3, 3.00%; Al2O3, 0.61%; SiO2, 1.35%; Na2O,0.04%; and others, 2.95%), and screened using adaptation and mineral solubilization tests. These strains were identified using 16S rRNA sequencing as Bacillus thuringiensis and Gongronella butleri [preserved in the China Center for Type Culture Collection (CCTCC) as M2012453 and M2012454] (Jinchi et al., 2014; Guanglin et al., 2015). These well-preserved strains were inoculated on nutrient agar (peptone, 10.0 g/L; beef extract powder, 3.0 g/L; NaCl, 5.0 g/L; and agar,15.0g/L) and potato sucrose (potato infusion powder, 7.0 g/L; sucrose, 20.0 g/L; and agar, 20.0 g/L) media and cultured at 30°C for 24–48 h. To achieve appropriate colony numbers, cultures of the strains were prepared by inoculating the activated strains individually in nutrient broth (peptone, 10.0 g/L; beef extract powder, 3.0 g/L; and NaCl, 5.0 g/L) and potato liquid (potato dip powder, 6.0 g/L; glucose, 20.0 g/L; and chloramphenicol, 0.1 g/L) media and incubating them at 30°C for 24 h.
2.2 Plant material and soil strategies
A pot experiment was conducted by mixing the culture thoroughly with an appropriate amount of sterilized soil (soil properties: effective nitrogen content of 101.88 mg·kg-1; effective phosphorus content of 5.44 mg·kg-1; effective potassium content of 102.35 mg·kg-1; and organic matter content of 7.38 g·kg-1). Three replicates were set for each treatment and the initial water content was set at 0.3 cm3/cm3 (V/V). After six months of routine maintenance, soil samples were collected from the pots for testing, with four sites mixed from each pot.
Lolium perenne L., a salt- and drought-tolerant engineered plant, was selected for this study, and its seeds were provided by Heyou Landscaping Engineering Co., Ltd (Jiangsu, China). Six treatment regimens were used in the study (Table 1), with sterile water for the control group and three replicates for each treatment.
2.3 Determination of the distribution and characterization of soil aggregates
Soil samples were collected from three sites for each treatment set-up and mixed. Fresh soil was gently broken along natural cracks to remove plant debris. A certain weight of soil sample was passed sequentially through sieves with pore sizes of 2 mm, 0.25 mm, and 0.053 mm; and weighed individually. Thereafter, the percentage of dry, sieved aggregates at each level was calculated as the percentage of the total amount of soil, and the aggregates were allocated to a certain amount of air-dried soil sample according to the proportion of dry, sieved aggregates. Water-stabilized aggregates of different sizes were then obtained by wet sieving the soil aggregates. Approximately 100 g of prepared soil samples were placed on the top of a set of sieves with pore sizes of 2 mm, 0.25 mm, and 0.053 mm from top to bottom, soaked in distilled water for 5 min, and then shaken vertically up and down for 20 min. The soil samples were transferred to aluminum boxes, dried, and weighed to calculate the weight proportion of each soil aggregate fraction (WSA). Based on the results of agglomerate composition determined by the wet sieving method, the content of water-stable agglomerates with diameter >0.25 mm (R > 0.25 mm), mean weight diameter (MWD), geometric mean diameter (GMD), and fractal dimension (D) were calculated. The formula for each index is as follows (Elliott, 1986; Balestrini et al., 2014):
where, is the mean weight diameter (mm), is the geometric mean diameter (mm), is the average diameter of the soil particle of any size range (mm), is the percentage content of aggregates of the i particle size (%), is the proportion of agglomerates with diameter >0.25 mm (%), is the mass of aggregates with a diameter >0.25mm (g), is the mass of water-stable aggregates (g), is the soil fractal dimension, is particle size smaller than the grain size R of the soil cumulative weight (g), is the total mass of the soil (g), R is the average diameter of the upper and lower limits of the range of particle size (mm), and is the upper limit of particle size.
2.4 Determination of soil organic matter composition and characterization of soil aggregates
The organic matter content of soil aggregates was determined using the concentrated sulfuric acid–potassium dichromate method (external heating), with a standard solution of ferrous sulfate titrated against an excess of potassium dichromate (Walkley and Black, 1934).
A VERTEX70 Fourier-transform infrared spectrometer (FTIR, Bruker, Hamburg, Germany) was used to analyze the structure of the organic matter in soil aggregates. Briefly, potassium bromide crystals were ground to powder and pressed into tablets after thorough grinding and mixing in the ratio of 1:100 (sample: potassium bromide). The spectra were scanned using an FTIR spectrometer, and the spectra were recorded in the range of 4000–500 cm-1, with a resolution of 4 cm-1 and 32 scans in the transmission mode (Szymański, 2017; Tafintseva et al., 2019; Lin et al., 2020; Mohamed and Janaki, 2021).
2.5 Data analyses
The FTIR spectra were automatically baseline corrected and smoothened using the Omnic 8.2 software, and the absorption peaks were regionally integrated (Lin et al., 2020; Mohamed and Janaki, 2021). The signal intensity of each absorption peak was obtained by calculating the percentage of each absorption peak area to the total peak area. Structural equation modeling (SEM) is a multivariate data analysis method that combines a variety of statistical analyses and is suitable for the study of interrelationships among multiple variables. SEM is utilized to establish, estimate and test causal relationships between indicators. The SPSS 26.0 software was used for statistical analyses. Significant differences between different treatments were determined using one-way analysis of variance (ANOVA) and least significant difference (LSD, p< 0.05) test, and the means were compared using Duncan’s test. Data were plotted using the Origin 2021 software.
3 Results
3.1 Composition and characterization of water-stable agglomerates
The effect of different treatments on agglomerate composition varied significantly (Figure 1). MSMs and plants dramatically increased the weight of agglomerates with diameters of >2 mm and 0.25–2 mm.
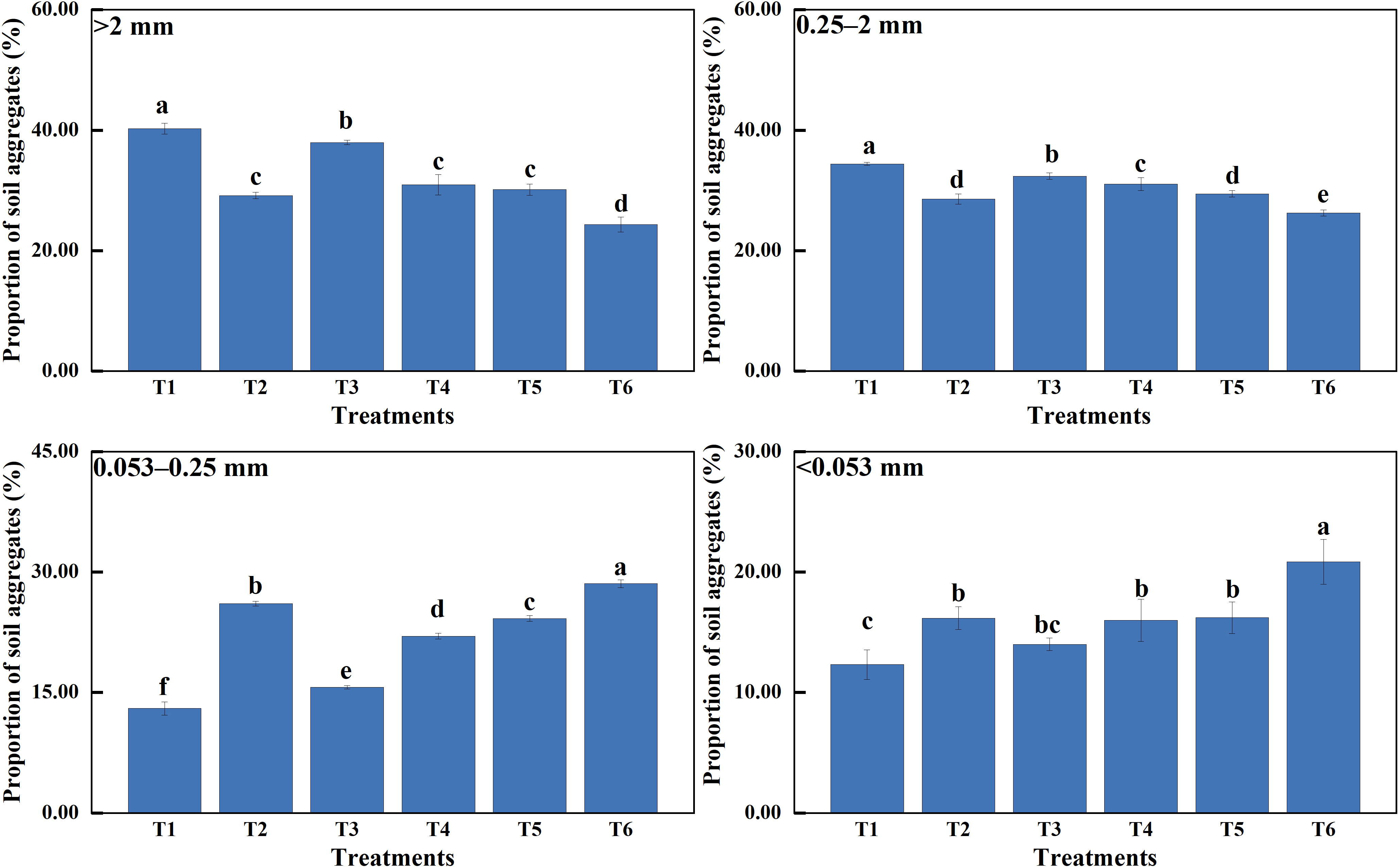
Figure 1 Composition and distribution of water-stable agglomerates. >2 mm: soil aggregates with a diameter >2 mm; 0.25–2 mm: soil aggregates with a diameter of 0.25–2 mm; 0.053–0.25 mm: soil aggregates with a diameter of 0.053–0.25 mm;<0.053 mm: soil aggregates with a diameter<0.053 mm. T1: B. thuringiensis + L. perenne; T2: B. thuringiensis; T3: G. butleri + L. perenne; T4: G. butleri; T5: L. perenne; T6: Control. Different letters denote significant differences (P< 0.05) between various treatments based on one-way analysis of variance and Duncan’s test.
The MWD and GWD of soil aggregates varied significantly among treatments (Table 2), with a consistent pattern (T1 > T3 > T4 > T5 > T2 > T6), indicating that MSMs and L. perenne exhibited a remarkable effect on the stability of soil aggregates. Meanwhile, MSMs and L. perenne showed a significant increase in the content of macroaggregates (diameter >0.25 mm) and a decrease of microaggregates (diameter<0.25 mm). The most noticeable effect was observed in the T1 treatment, where R0.25, MWD and GWD increased by 47.54%, 45.63% and 84.35%, respectively, compared to the control.
3.2 Organic matter content and characterization of agglomerates
The presence of MSMs and the plant species increased the organic matter content of soil aggregates at all grain levels (Figure 2). The organic matter content of soil aggregates at different grain levels followed the following order: SOM>2 mm (soil organic matter content of aggregates with a diameter >2 mm) > SOM2–0.25 mm > SOM0.25–0.053 mm > SOM<0.053 mm. The organic matter content and storage of T1 sample was significantly higher than the other treatments.
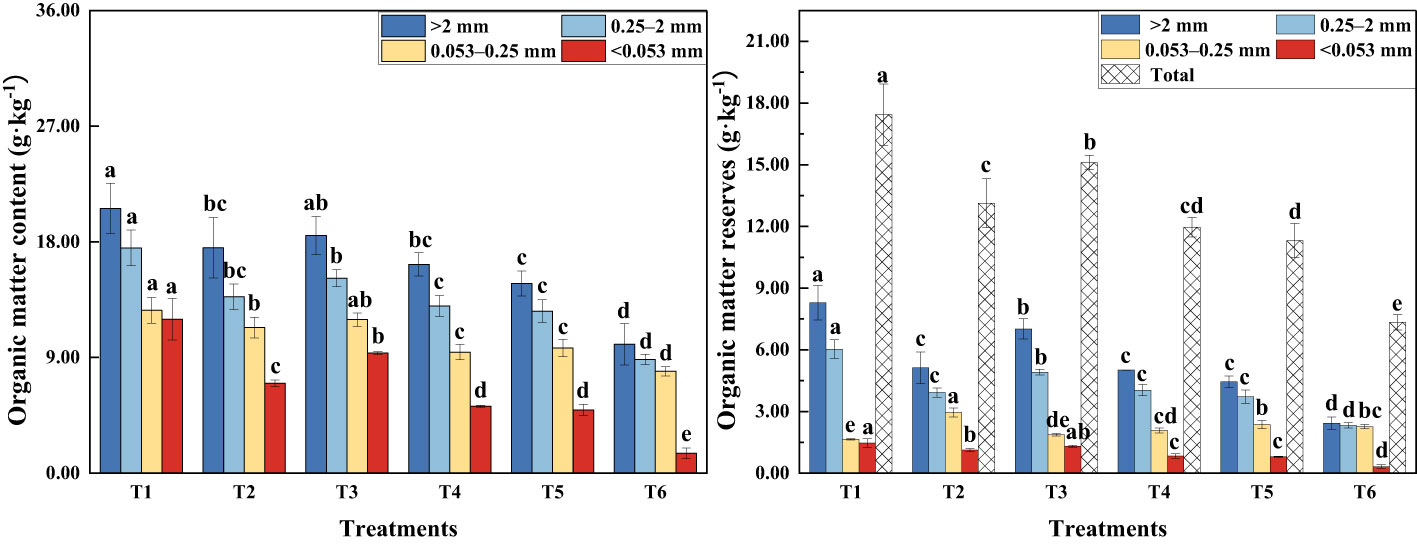
Figure 2 Organic matter content and storage of agglomerates of various grain sizes. >2 mm: soil aggregates with a diameter >2 mm; 0.25–2 mm: soil aggregates with a diameter of 0.25–2 mm; 0.053–0.25 mm: soil aggregates with a diameter of 0.053–0.25 mm;<0.053 mm: soil aggregates with a diameter<0.053 mm. T1: B. thuringiensis + L. perenne; T2: B. thuringiensis; T3: G. butleri + L. perenne; T4: G. butleri; T5: L. perenne; T6: Control. Different letters denote significant differences (P< 0.05) between various treatments based on one-way analysis of variance and Duncan’s test.
The infrared spectra of the samples exposed to different treatments and of different grain sizes exhibited similar absorption bands and peaks, revealing the characteristics of polysaccharide, aromatic, aliphatic, amide and alcohol/phenolic functional groups, respectively (Figure 3). The different absorption bands and peaks differ only in peak height and peak area, indicating that they exhibited the same functional groups but different contents.
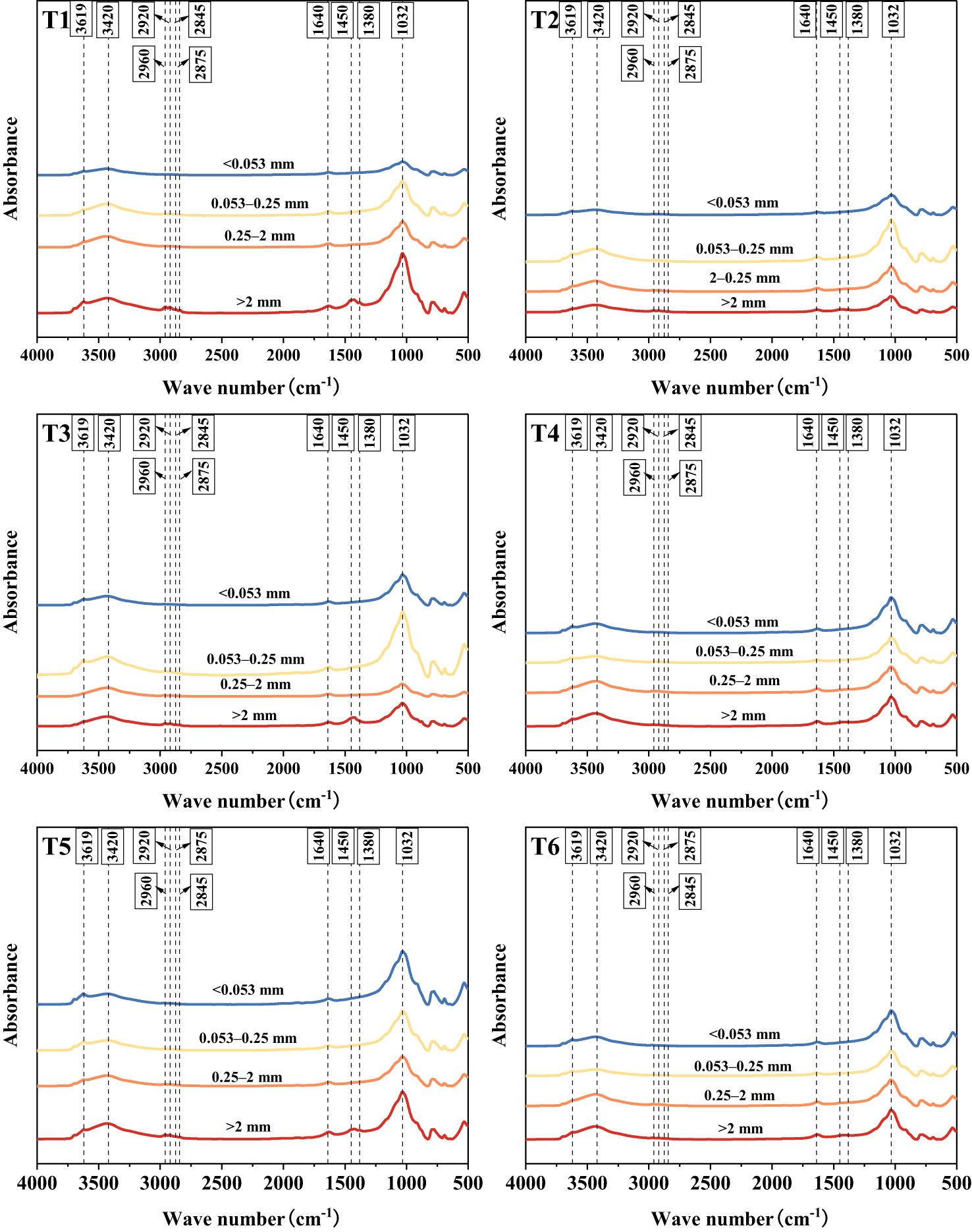
Figure 3 Infrared spectra of agglomerates at each grain level. >2 mm: soil aggregates with a diameter >2 mm; 0.25–2 mm: soil aggregates with a diameter of 0.25–2 mm; 0.053–0.25 mm: soil aggregates with a diameter of 0.053–0.25 mm;<0.053 mm: soil aggregates with a diameter<0.053 mm. T1: B. thuringiensis + L. perenne; T2: B. thuringiensis; T3: G. butleri + L. perenne; T4: G. butleri; T5: L. perenne; T6: Control.
The relative contents of major functional groups of different size aggregates in different treatments are shown in Figure 4. The highest content of organic matter components in samples exposed to different treatments and of different grain sizes were polysaccharides and amides, followed by aromatic and aliphatic compounds, and the lowest contents were those of alcohols and phenols. For agglomerates with a diameter >2 mm, the presence of MSMs and the plant species increased the content of aliphatic compounds and decreased the content of alcohols and phenols. For agglomerates with a diameter <0.053 mm, the presence of MSMs and the plant species significantly increased the content of alcohols and phenolics.
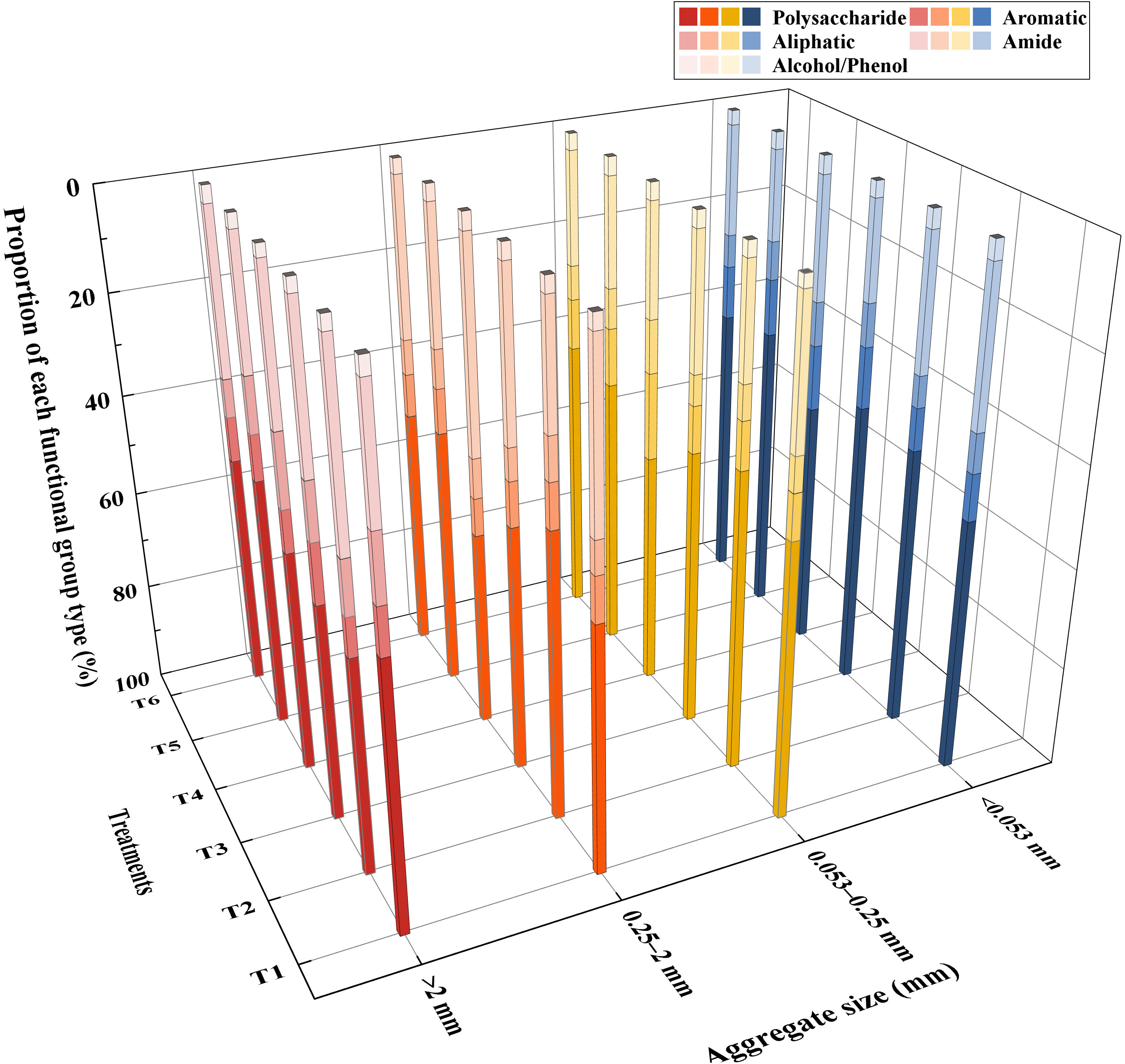
Figure 4 Functional group analysis of agglomerates based on different treatments and aggregate size. >2 mm: soil aggregates with a diameter >2 mm; 0.25–2 mm: soil aggregates with a diameter of 0.25–2 mm; 0.053–0.25 mm: soil aggregates with a diameter of 0.053–0.25 mm;<0.053 mm: soil aggregates with a diameter<0.053 mm. T1: B. thuringiensis + L. perenne; T2: B. thuringiensis; T3: G. butleri + L. perenne; T4: G. butleri; T5: L. perenne; T6: Control.
3.3 Correlation analysis of organic matter and water stability characteristics of agglomerates
Soil aggregate stability was significantly positively correlated with the weight of aggregates with diameters >2 mm and 2–0.25 mm, whereas it was significantly negatively correlated with the weight of other fractions (diameter of 0.25–0.053 mm, and <0.053 mm) (Figure 5). Additionally, soil aggregate stability was positively correlated with the organic matter content of the aggregates of all grades. The weights of aggregates with diameters >2 mm and 2–0.25 mm were positively correlated with their organic matter content, whereas the weights of other graded aggregates were significantly negatively correlated with their organic matter content.
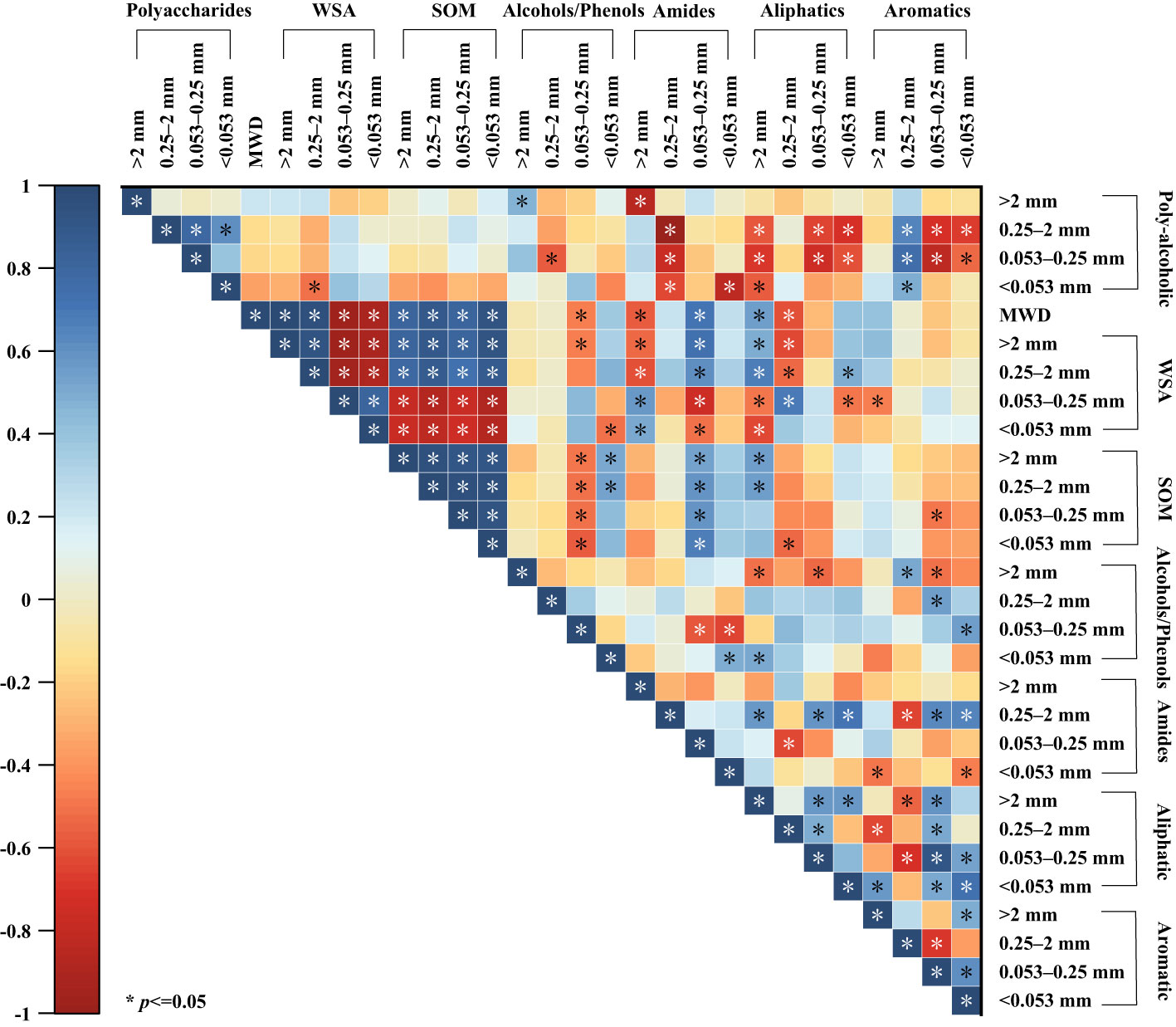
Figure 5 Correlation analysis of organic matter content and water stability characteristics of agglomerates at various grain levels. >2 mm: soil aggregates with a diameter >2 mm; 0.25–2 mm: soil aggregates with a diameter of 0.25–2 mm; 0.053–0.25 mm: soil aggregates with a diameter of 0.053–0.25 mm;<0.053 mm: soil aggregates with a diameter<0.053 mm. * indicates significant differences at P ≤ 0.05. SOM: soil organic matter content; WSA: the weight proportion of soil aggregates.
3.4 Effects of organic matter composition on the agglomerates of different grain sizes
Amides (−0.283), aromatic compounds (0.266), and organic matter content (0.652) directly affected the WSA of diameter >2 mm (Figure 6). The presence of the plant species indirectly affected the WSA of diameter >2 mm by altering the content of amide compounds (−0.673), aromatic compounds (0.592), and organic matter content (0.400). The presence of the plant species exhibited a significant positive effect (0.609) on WSA of diameter >2 mm.
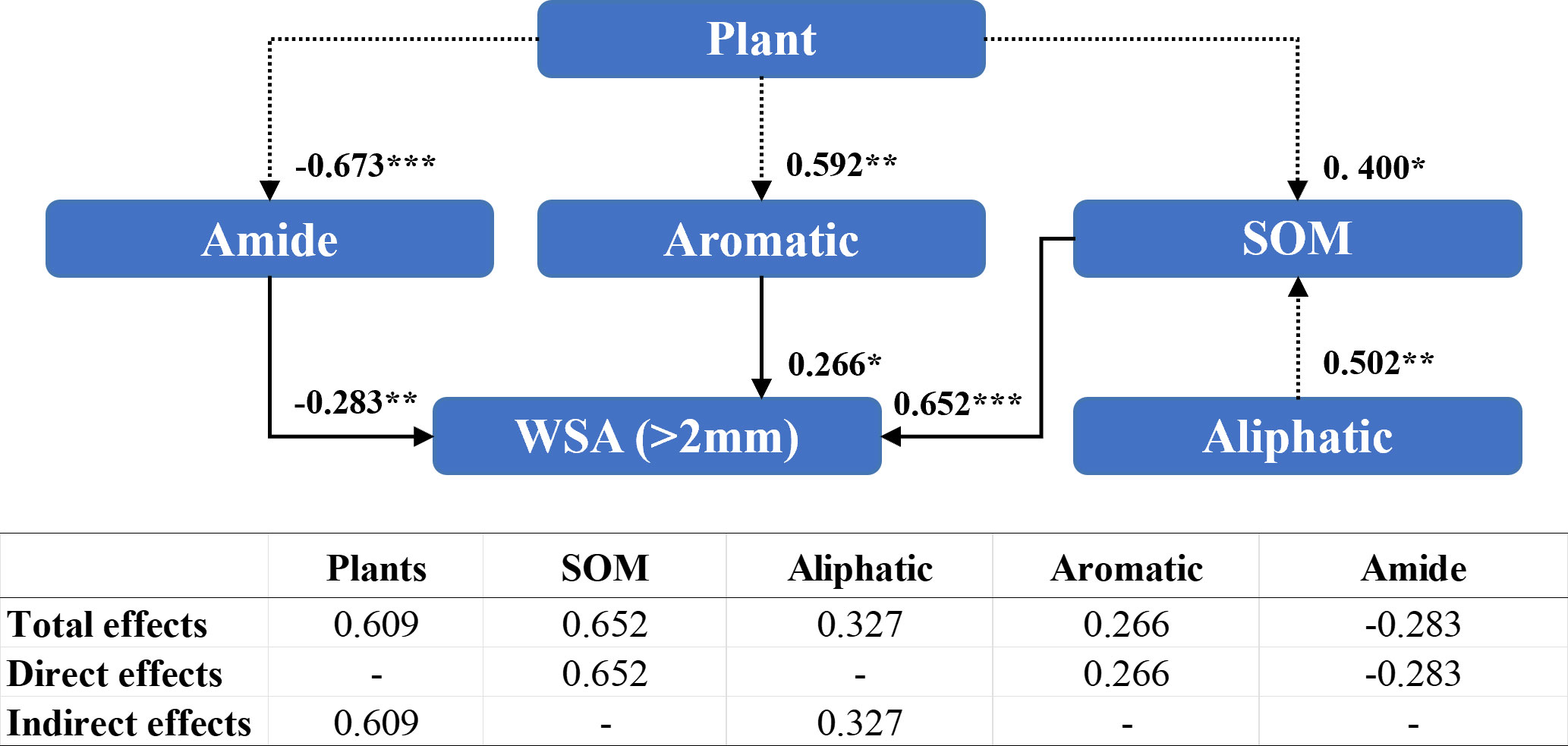
Figure 6 Structural equation modeling of organic matter characteristics affecting the stability of soil aggregates with a diameter >2 mm. CMIN/DF = 1.059; GFI = 0.989; RMSEA< 0.08. Standardized path coefficients are shown as numbers on arrows. ***, **, and * indicate significant differences at P< 0.001, P< 0.01, and P< 0.05, respectively. SOM: soil organic matter content; WSA (>2 mm): the weight proportion of soil aggregates with a diameter >2 mm.
Amide compounds (0.336), organic matter content (0.653) and the plant species (0.253) directly influenced the WSA of diameter between 2 mm and 0.25 mm (Figure 7), while the plant species indirectly regulated the WSA of diameter 2–0.25 mm by affecting aromatic compound (0.390) and organic matter contents (0.758). Additionally, the presence of the plant species exhibited a significant positive effect (0.649) on WSA of diameter 2–0.25 mm.
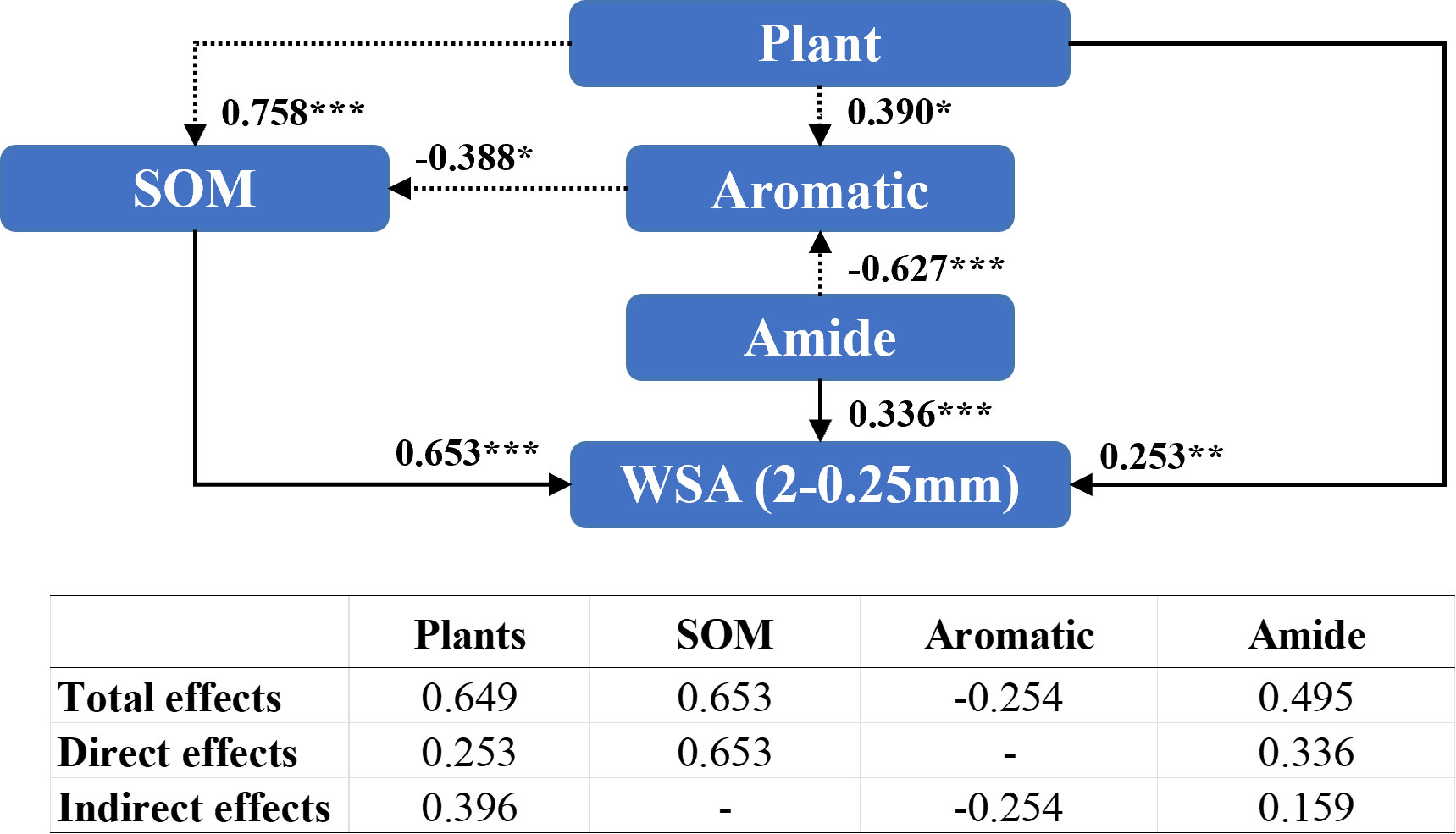
Figure 7 Structural equation modeling of organic matter characteristics affecting the stability of soil aggregates with a diameter between 2 mm and 0.25 mm. CMIN/DF = 1.324; CFI = 0.982; RMSEA< 0.08. Standardized path coefficients are shown as numbers on arrows. ***, **, and * indicate significant differences at P< 0.001, P< 0.01, and P< 0.05, respectively. WSA (2–0.25 mm): the weight proportion of soil aggregates with a diameter between 2 mm and 0.25 mm.
Polysaccharides (0.360) and organic matter content (–0.857) directly affected the WSA of diameter between 0.25 mm and 0.053 mm (Figure 8). In contrast, MSMs were able to regulate WSA of diameter 0.05–0.053 mm indirectly by affecting organic matter content (0.678). Nonetheless, the addition of MSMs exhibited a markedly negative influence (–0.581) on WSA of diameter 2–0.25 mm.
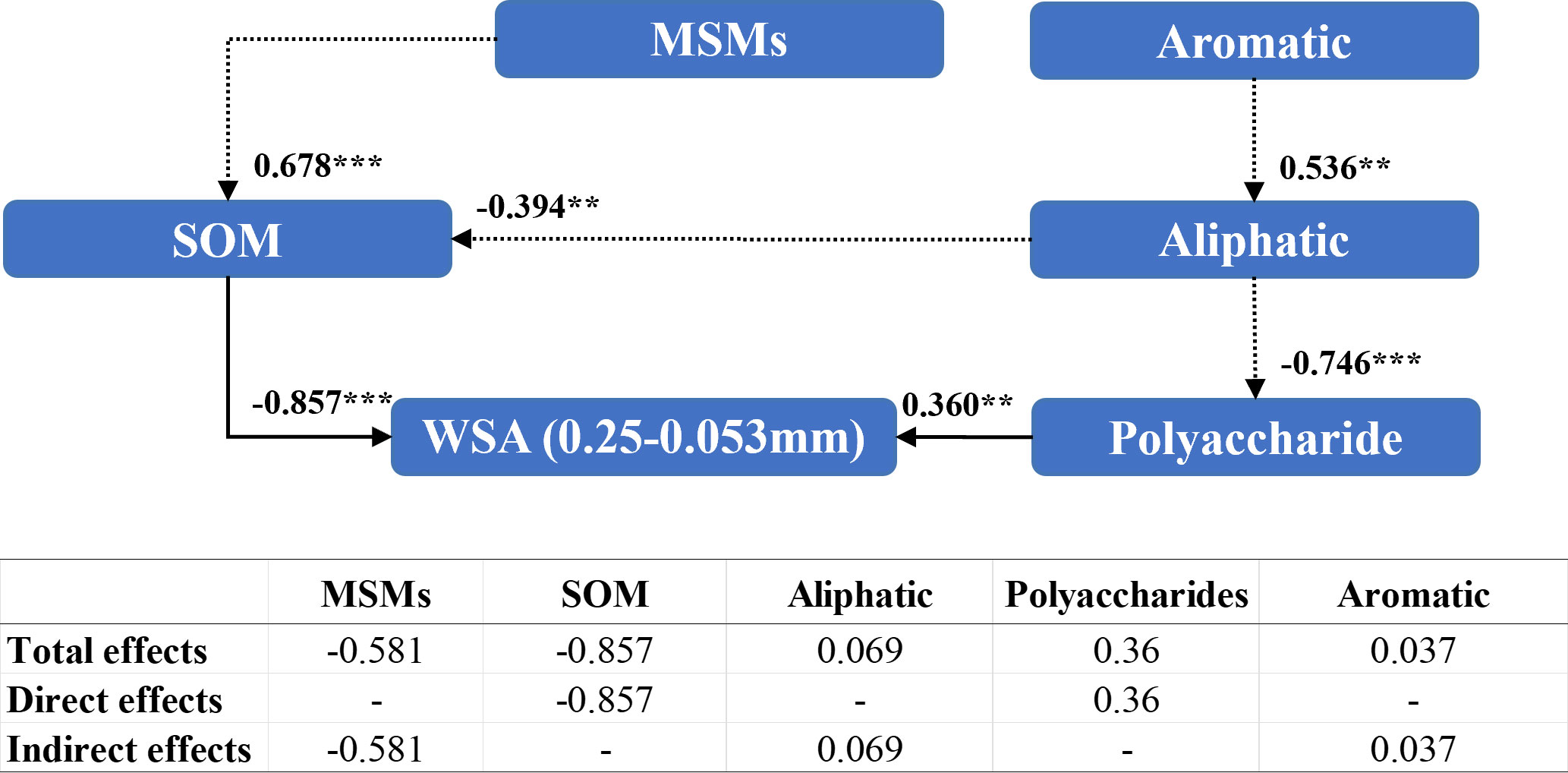
Figure 8 Structural equation modeling of organic matter characteristics affecting the stability of soil aggregates with a diameter between 0.25 mm and 0.053 mm. CMIN/DF = 1.264; CFI = 0.950; MSEA< 0.08. Standardized path coefficients are shown as numbers on arrows. *** and ** indicate significant differences at P< 0.001, P< 0.01, respectively. WSA (0.25–0.053 mm): the weight proportion of soil aggregates with a diameter between 0.25 mm and 0.053 mm
Amides (0.260), polysaccharide compounds (0.262), and organic matter content (-0.854) directly influenced the WSA of diameter<0.053 mm (Figure 9). However, MSMs indirectly regulated the WSA of diameter<0.053 mm by affecting polysaccharide (–0.530), amide (0.483), and organic matter contents (0.716). Furthermore, the addition of MSMs exhibited a considerable negative influence (-0.624) on WSA of diameter<0.053 mm.
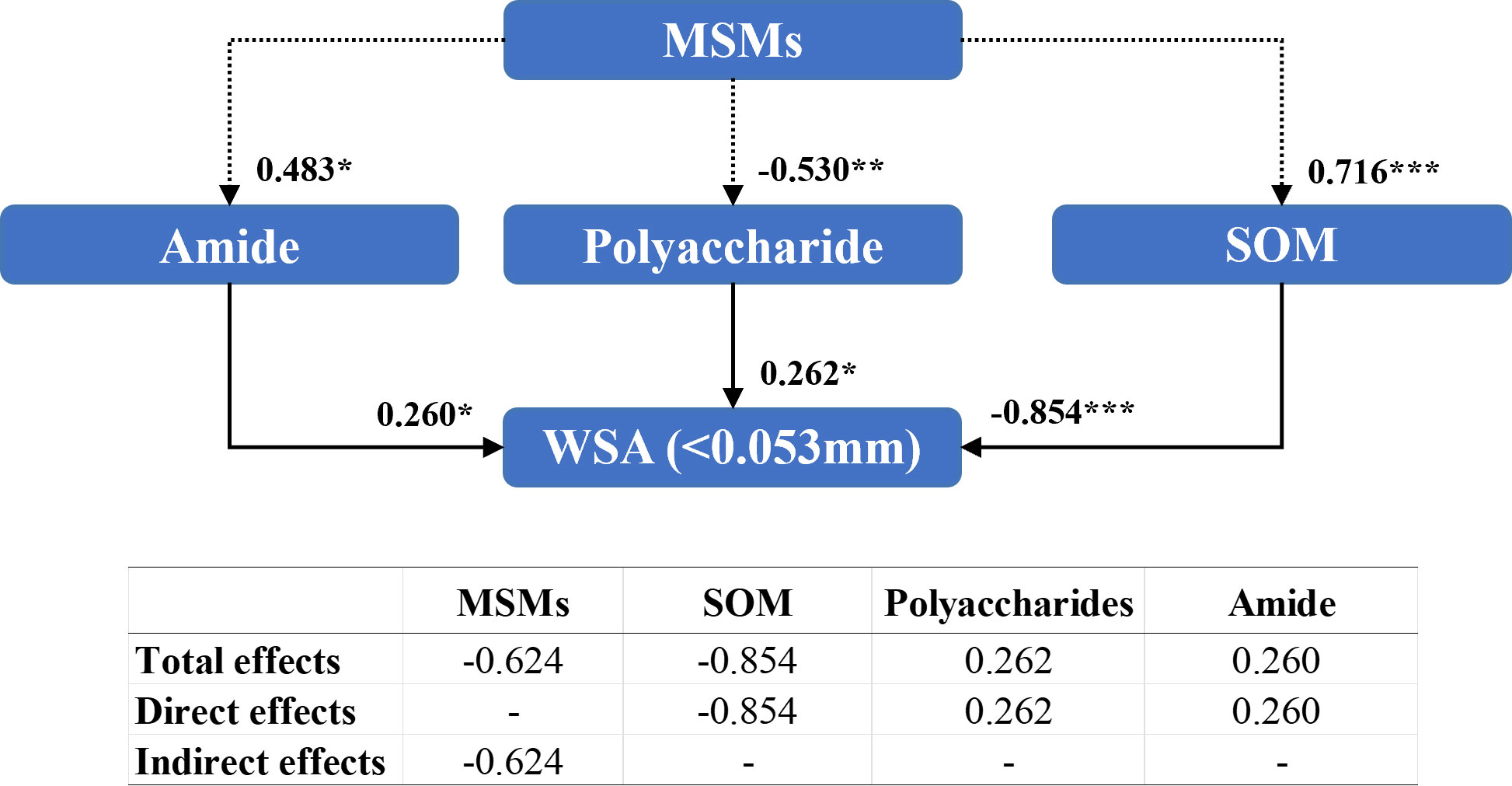
Figure 9 Structural equation modeling of organic matter characteristics affecting the stability of soil aggregates with a diameter between 0.25 mm and 0.053 mm. CMIN/DF = 1.065; CFI = 0.994; RMSEA< 0.08. Standardized path coefficients are shown as numbers on arrows. ***, **, and * indicate significant differences at P< 0.001, P< 0.01, and P< 0.05, respectively. WSA (<0.053 mm): the weight proportion of soil aggregates with a diameter<0.053 mm.
4 Discussion
The rapid population growth in the second half of the last century has intensified the demand for natural resources and interference with nature. At the same time, engineering activities to promote economic development have caused a series of environmental problems (Okello et al., 2015; Vanwalleghem et al., 2017; Kattel, 2022), including the emergence of a large number of bare slopes, and thus ecological and national economic and social development as important constraints cannot be ignored (Rosselló et al., 2020; Wang Y. et al., 2022). Therefore, slope regreening and ecological reconstruction have been the major concerns of researchers (Zhang et al., 2018). Our group has been devoted to theoretical research and technological innovation in slope ecological management and reconstruction for several years by drawing on the experiences and results of slope management measures in various countries and utilizing a series of controlled experiments.
Wu et al. isolated and screened a few microorganisms with strong adaptability and prominent mineral solubilization from the surface of the weathered rock wall of the Mufu Mountains, Nanjing, and explored the mechanisms underlying mineral solubilization (Wu et al., 2017a; Wu et al., 2017b). Jia et al. further investigated the screened microorganisms and found that a variety of MSMs could promote root growth, increase root strength, increase the number of root nodules, and affect the number and species of inter-root microorganisms (Jia et al., 2021). Meanwhile, Li et al. discovered through research that MSMs can affect soil nutrient cycling, especially on the nitrogen cycling with extremely significant effects (Li C. et al., 2020; Li et al., 2021a; Li et al., 2021b). In addition, we conducted a series of optimization studies on slope spraying substrates, including water retention agents (Wang L. et al., 2022), comprehensively analyzed and ranked the effects of different treatments, and preliminarily applied the MSM-based APG method (Wang L. et al., 2023). The results showed that APG was an effective and feasible slope greening method, with great application value and broad application prospects. However, soil restoration and reconstruction are the key to the ecological management of slopes, but the effects of MSMs on soil aggregates using the APG method have not yet been investigated.
Soil microorganisms play an important role in the formation and stabilization of soil aggregates, with bacteria and fungi showing considerable aggregation of soil particles (Deka et al., 2018; Zhao et al., 2018; Hou et al., 2021). It has been shown that Bacillus cells and their secretions have remarkable aggregation effects on soil particles, with secretions performing particularly well (Sandhya and Ali, 2015; Ma et al., 2017). Fungi, on the other hand, were not only able to aggregate soil particles through cells and their secretions but were also able to use mycelia to aggregate soil particles and form larger agglomerates through entanglement (Lehmann and Rillig, 2015; Lehmann et al., 2017; Morris et al., 2019; Lehmann et al., 2020). This was consistent with the results of this study, where treatment with MSMs (T2 and T4) showed better structure and stability of soil aggregates compared to the control, and notably, the addition of NL-15 (T4) resulted in higher levels of macroaggregates (diameter >0.25 mm) than the treatments with addition of NL-11 (T2) (Figure 1). Plant roots not only influence soil aggregates by releasing various secretions but also rearrange soil particles by root entanglement, thus improving soil aggregate stability (Blankinship et al., 2016; Zeng et al., 2018; Dou et al., 2020; Merino-Martín et al., 2021). This was consistent with the findings of the present study (Figure 1). Moreover, MSMs together with the plant species were more effective in enhancing soil aggregate stability than MSMs or the plant species alone. This may be related to the increase in sticky substances in the secretions due to the interaction of MSMs with the plant species (Blankinship et al., 2016; Merino-Martín et al., 2021). It could also be attributed to the fact that MSMs have a significant enhancement effect on the length density of the plant root system, which in turn results in enhanced agglomeration and increased soil stability (Lehmann and Rillig, 2015).
Soil organic matter is fundamental to the soil system and influences soil formation, fertility, soil, and other properties (Murphy, 2015; Obalum et al., 2017; Jin et al., 2020; Voltr et al., 2021). Several studies have shown that the content and composition of soil organic matter depends on both plant inputs and the presence of soil microorganisms (Paul, 2016; Angst et al., 2021). Microorganisms promote the degradation of soil organic matter through decomposition and metabolism to obtain the nutrients they need. Continuous growth and reproduction of microorganisms results in an increase in biomass and the production of large quantities of active substances. They also reduce carbon loss by metabolizing available carbon sources in the soil into more stable organic matter (Kallenbach et al., 2016; Paul, 2016; Liang et al., 2019; Liang et al., 2017). The results of this study showed that the addition of MSMs significantly increased the organic matter content of the soil (Figure 2), with a remarkable increase in aliphatic organic matter in macroaggregates (diameter >0.25mm) and alcoholic and phenolic organic matter in microaggregates (diameter<0.25 mm) (Figure 5).
The plant root system continuously releases substances or exudates into the soil, including sugars, amino acids, vitamins, long-chain carbohydrates, enzymes, and lysates, during root cell rupturing (Bais et al., 2006; Wang A. et al., 2023). In this study, we noted that the presence of the plant species resulted in a significant increase in the organic matter content of the soil compared to the control (Figure 2), especially aliphatic and polysaccharide organic matter in macroaggregates and alcoholic, phenolic, and aliphatic organic matter in microaggregates (Figure 5). Simple compounds released in plant root secretions are taken up by microorganisms, attach to mineral surfaces, enter the soil, and eventually form stable organic matter. At the same time, root secretions promote the mineralization of organic matter by stimulating microbial activity and accelerating the decomposition of unprotected carbon in the soil. Total organic matter depends on the net effect of these two opposing mechanisms (Bais et al., 2006; Miltner et al., 2011; Bradford et al., 2013; Cotrufo et al., 2013). In this study, the binding of MSMs to the roots of the plant species significantly increased the organic matter content of the soil (Figure 2), especially aliphatic organic matter in macroaggregates and amide organic matter in microaggregates. Notably, the coexistence of MSMs with the plant species (T1 and T3) exhibited improved effects on soil organic matter compared to MSMs alone (T2 and T4). In addition, the promotion of organic matter by NL-11 was more pronounced than that of NL-15, which was also evident by the polysaccharide content in macroaggregates, as well as the amide organic matter content in microaggregates.
Numerous conceptual models regarding the formation and stabilization mechanisms of agglomerates, such as the aggregate hierarchy model (Tisdall and Oades, 1982), aggregate turnover model (Six et al., 2000), and tertiary structure model (Christensen, 2001), have emerged. The results of a large number of studies show that organic matter plays an important role in the process of agglomerate formation, and the higher the content of soil organic matter, the stronger the stability of the formed agglomerates (Boyle et al., 1989; Zhao et al., 2017; Li T. et al., 2020), which is also consistent with the results of the present study (Figure 5). In recent years, researchers have focused on the effects of soil organic matter chemistry on soil aggregates (Recio-Vazquez et al., 2014; Guan et al., 2018; Sarker et al., 2018). Oades et al. (Oades and Waters, 1991; Guhra et al., 2022) found that microaggregates are virtually devoid of plant debris and are stabilized primarily by microorganisms and their derivatives, including polysaccharides, microbial cells, and other components. In this study, we observed that the addition of MSMs influenced the organic matter and polysaccharides in the microaggregates and hence the percentage of microaggregate content (Figures 6, 7), whereas in macroaggregates, larger soil particles combined with microaggregates through biological interactions (microbial secretions, root systems, and mycelia) (Six et al., 2000; Rillig et al., 2017). There have been several reports of strong correlation between plant growth and the nature of macroaggregates (Poirier et al., 2017; Schomburg et al., 2018). The present study also noted that the presence of the plant species was vital for macroaggregate content, which could affect the content of organic matter and aromatic compounds and thus the percentage of macroaggregate content (Figures 8, 9).
5 Conclusions
To achieve an efficient and cost-effective method for the management of slopes, we proposed the active permanent greening (APG) method based on patented mineral solubilizing microorganisms (MSMs). We investigated the changes in soil aggregates and organic matter using the APG method in a controlled experiment, and explored the intrinsic mechanism by which MSMs and their combination with plants enhance slope stabilization by regulating soil aggregate structure. The results showed that (1) MSMs and the plant species dramatically affected the distribution of soil aggregates and improved their stability. Among them, G. butleri performed better and improved soil aggregate stability by 20.72% compared to the control. (2) MSMs and the plant species significantly increased the organic matter content of the soil, where B. thuringiensis performed better, improving organic matter content by 78.85% compared to the control. Remarkably, the synergistic effect of MSMs and the plant species on improving soil aggregate stability and organic matter content was better than using MSMs or the plant species alone, with the combination of B. thuringiensis and L. perenne yielding the best results among all treatments. (3) Macroaggregate (diameter > 2mm) content had a significant positive effect on soil stability, while the other fractions had a significant negative effect. (4) We also used structural equation modeling to investigate the relationship between organic matter and WSA (the weight proportion of soil aggregates) under different combinations of MSMs and the plant species and found that the weight proportion of macroaggregates was mainly affected by the plant species, whereas that of microaggregates was mainly affected by MSMs. The application of the APG method in slope management has great potential and deserves further more in-depth and comprehensive research.
Patents
Jinchi Zhang, Guanglin Wang, Bo Zhang, Yanwen Wu: An efficient limestone erosion bacterium Bacillus thuringiensis NL-11 and its application. CN103087954B; Guanglin Wang, Jinchi Zhang, Jie Lin, Rong Cao: An efficient limestone erosion fungus Gongronella butleri NL-15 and its application. CN103087926B.
Data availability statement
The original contributions presented in the study are included in the article/supplementary material. Further inquiries can be directed to the corresponding author.
Author contributions
LW: Conceptualization, Data curation, Formal Analysis, Investigation, Methodology, Software, Validation, Writing – original draft. XT: Investigation, Methodology, Software, Validation, Writing – review & editing. XL: Investigation, Methodology, Project administration, Software, Supervision, Validation, Writing – review & editing. RX: Data curation, Investigation, Methodology, Software, Writing – review & editing. JZ: Formal Analysis, Funding acquisition, Project administration, Resources, Writing – review & editing.
Funding
The author(s) declare financial support was received for the research, authorship, and/or publication of this article. Postgraduate Research & Practice Innovation Program of Jiangsu Province [KYLX16_0864]; Innovation and Promotion of Forestry Science and Technology Program of Jiangsu Province [LYKJ (2021) 30]; Scientific Research Project of Baishanzu National Park [2021ZDLY01]; Priority Academic Program Development of Jiangsu Higher Education Institutions [PAPD]. Jiangsu Science and Technology Plan Project [BE2022420].
Conflict of interest
The authors declare that the research was conducted in the absence of any commercial or financial relationships that could be construed as a potential conflict of interest.
Publisher’s note
All claims expressed in this article are solely those of the authors and do not necessarily represent those of their affiliated organizations, or those of the publisher, the editors and the reviewers. Any product that may be evaluated in this article, or claim that may be made by its manufacturer, is not guaranteed or endorsed by the publisher.
References
Abulfaraj, A. A., Jalal, R. S. (2021). Use of plant growth-promoting bacteria to enhance salinity stress in soybean (Glycine max L.) plants. Saudi J. Biol. Sci. 28 (7), 3823–3834. doi: 10.1016/j.sjbs.2021.03.053
Angst, G., Mueller, K. E., Nierop, K. G. J., Simpson, M. J. (2021). Plant- or microbial-derived? A review on the molecular composition of stabilized soil organic matter. Soil Biol. Biochem. 156, 108189. doi: 10.1016/j.soilbio.2021.108189
Bais, H. P., Weir, T. L., Perry, L. G., Gilroy, S., Vivanco, J. M. (2006). The role of root exudates in rhizosphere interactions with plants and other organisms. Annu. Rev. Plant Biol. 57 (1), 233–266. doi: 10.1146/annurev.arplant.57.032905.105159
Balestrini, R., Zhang, P., Wei, T., Jia, Z., Han, Q., Ren, X., et al. (2014). Effects of straw incorporation on soil organic matter and soil water-stable aggregates content in semiarid regions of northwest China. PloS One 9 (3), e92839. doi: 10.1371/journal.pone.0092839
Barbosa, M. V., Pedroso, D., Curi, N., Carneiro, M. A. C. (2019). Do different arbuscular mycorrhizal fungi affect the formation and stability of soil aggregates? Ciec. e Agrotecnologia 43. doi: 10.1590/1413-7054201943003519
Bertrand, J.-C., Bonin, P., Caumette, P., Gattuso, J.-P., Grégori, G., Guyoneaud, R., et al. (2015). “Biogeochemical Cycles,” in Environmental Microbiology: Fundamentals and Applications (Dordrecht: Springer), 511–617. doi: 10.1007/978-94-017-9118-2_14
Blankinship, J. C., Fonte, S. J., Six, J., Schimel, J. P. (2016). Plant versus microbial controls on soil aggregate stability in a seasonally dry ecosystem. Geoderma 272, 39–50. doi: 10.1016/j.geoderma.2016.03.008
Boyle, M., Frankenberger, W. T., Stolzy, L. H. (1989). The influence of organic matter on soil aggregation and water infiltration. J. Production Agric. 2 (4), 290–299. doi: 10.2134/jpa1989.0290
Bradford, M. A., Keiser, A. D., Davies, C. A., Mersmann, C. A., Strickland, M. S. (2013). Empirical evidence that soil carbon formation from plant inputs is positively related to microbial growth. Biogeochemistry 113 (1-3), 271–281. doi: 10.1007/s10533-012-9822-0
Broda, J., Gawlowski, A. (2018). Influence of sheep wool on slope greening. J. Natural Fibers 17 (6), 820–832. doi: 10.1080/15440478.2018.1534190
Cao, Y., Wang, J., Bai, Z., Zhou, W., Zhao, Z., Ding, X., et al. (2015). Differentiation and mechanisms on physical properties of reconstructed soils on open-cast mine dump of loess area. Environ. Earth Sci. 74 (8), 6367–6380. doi: 10.1007/s12665-015-4607-0
Christensen, B. T. (2001). Physical fractionation of soil and structural and functional complexity in organic matter turnover. Eur. J. Soil Sci. 52 (3), 345–353. doi: 10.1046/j.1365-2389.2001.00417.x
Chung, K. S., Shin, J. S., Lee, J. H., Park, S. E., Han, H. S., Rhee, Y. K., et al. (2021). Protective effect of exopolysaccharide fraction from Bacillus subtilis against dextran sulfate sodium-induced colitis through maintenance of intestinal barrier and suppression of inflammatory responses. Int. J. Biol. Macromolecules 178, 363–372. doi: 10.1016/j.ijbiomac.2021.02.186
Cotrufo, M. F., Wallenstein, M. D., Boot, C. M., Denef, K., Paul, E. (2013). The Microbial Efficiency-Matrix Stabilization (MEMS) framework integrates plant litter decomposition with soil organic matter stabilization: do labile plant inputs form stable soil organic matter? Global Change Biol. 19 (4), 988–995. doi: 10.1111/gcb.12113
Cui, L., Li, X., Lin, J., Guo, G., Zhang, X., Zeng, G. (2022). The mineralization and sequestration of soil organic carbon in relation to gully erosion. Catena 214. doi: 10.1016/j.catena.2022.106218
Deka, P., Goswami, G., Das, P., Gautom, T., Chowdhury, N., Boro, R. C., et al. (2018). Bacterial exopolysaccharide promotes acid tolerance in Bacillus amyloliquefaciens and improves soil aggregation. Mol. Biol. Rep. 46 (1), 1079–1091. doi: 10.1007/s11033-018-4566-0
Deng, J., Kong, S. S., Wang, F., Liu, Y., Jiao, J. Y., Lu, Y. Y., et al. (2020). Identification of a new Bacillus sonorensis strain KLBC GS-3 as a biocontrol agent for postharvest green mould in grapefruit. Biol. Control 151, 104393. doi: 10.1016/j.biocontrol.2020.104393
Dou, Y., Yang, Y., An, S., Zhu, Z. (2020). Effects of different vegetation restoration measures on soil aggregate stability and erodibility on the Loess Plateau, China. Catena 185, 104294. doi: 10.1016/j.catena.2019.104294
Dyregrov, A., Yule, W., Olff, M. (2018). Children and natural disasters. Eur. J. Psychotraumatol. 9. doi: 10.1080/20008198.2018.1500823
Elliott, E. T. (1986). Aggregate structure and carbon, nitrogen, and phosphorus in native and cultivated soils. Soil Sci. Soc. America J. 50 (3), 627–633. doi: 10.2136/sssaj1986.03615995005000030017x
Faiz, H., Ng, S., Rahman, M. (2022). A state-of-the-art review on the advancement of sustainable vegetation concrete in slope stability. Construction Building Materials 326, 126502. doi: 10.1016/j.conbuildmat.2022.126502
Giassi, V., Kiritani, C., Kupper, K. C. (2016). Bacteria as growth-promoting agents for citrus rootstocks. Microbiological Res. 190, 46–54. doi: 10.1016/j.micres.2015.12.006
Guan, S., An, N., Zong, N., He, Y., Shi, P., Zhang, J., et al. (2018). Climate warming impacts on soil organic carbon fractions and aggregate stability in a Tibetan alpine meadow. Soil Biol. Biochem. 116, 224–236. doi: 10.1016/j.soilbio.2017.10.011
Guanglin, W., Jinchi, Z., Jie, L., Rong, C. (2015). An efficient limestone erosion fungus Gongronella butleri NL-15 and its application.
Guhra, T., Stolze, K., Totsche, K. U. (2022). Pathways of biogenically excreted organic matter into soil aggregates. Soil Biol. Biochem. 164, 108483. doi: 10.1016/j.soilbio.2021.108483
Han, Z., Zhong, S., Ni, J., Shi, Z., Wei, C. (2019). Estimation of soil erosion to define the slope length of newly reconstructed gentle-slope lands in hilly mountainous regions. Sci. Rep. 9 (1). doi: 10.1038/s41598-019-41405-9
Hou, J., Xu, X., Yu, H., Xi, B., Tan, W. (2021). Comparing the long-term responses of soil microbial structures and diversities to polyethylene microplastics in different aggregate fractions. Environ. Int. 149, 106398. doi: 10.1016/j.envint.2021.106398
Huang, Z., Chen, J., Ai, X., Li, R., Ai, Y., Li, W. (2017). The texture, structure and nutrient availability of artificial soil on cut slopes restored with OSSS – Influence of restoration time. J. Environ. Manage. 200, 502–510. doi: 10.1016/j.jenvman.2017.05.043
Ji, L., Tan, W., Chen, X. (2019). Arbuscular mycorrhizal mycelial networks and glomalin-related soil protein increase soil aggregation in Calcaric Regosol under well-watered and drought stress conditions. Soil Tillage Res. 185, 1–8. doi: 10.1016/j.still.2018.08.010
Jia, Z., Meng, M., Li, C., Zhang, B., Zhai, L., Liu, X., et al. (2021). Rock-solubilizing microbial inoculums have enormous potential as ecological remediation agents to promote plant growth. Forests 12 (3), 357-369. doi: 10.3390/f12030357
Jin, Z., Shah, T., Zhang, L., Liu, H., Peng, S., Nie, L. (2020). Effect of straw returning on soil organic carbon in rice–wheat rotation system: A review. Food Energy Secur. 9 (2). doi: 10.1002/fes3.200
Jinchi, Z., Guanglin, W., Bo, Z., Yanwen, W. (2014). An efficient limestone erosion bacterium Bacillus thuringiensis NL-11 and its application.
Jinchi, Z., Guanglin, W., Jiayao, Z., Qun, W. (2015a). An efficient limestone erosion bacterium Bacillus megaterium NL-7 and its application.
Jinchi, Z., Guanglin, W., Li, W., Bo, Z. (2015b). An efficient limestone erosion actinomycete Streptomyces thermophilus monoxide NL-1 from Limestone and Its Application.
Kallenbach, C. M., Frey, S. D., Grandy, A. S. (2016). Direct evidence for microbial-derived soil organic matter formation and its ecophysiological controls. Nat. Commun. 7 (1). doi: 10.1038/ncomms13630
Kamran, S., Shahid, I., Baig, D. N., Rizwan, M., Malik, K. A., Mehnaz, S. (2017). Contribution of zinc solubilizing bacteria in growth promotion and zinc content of wheat. Front. Microbiol. 8. doi: 10.3389/fmicb.2017.02593
Kattel, G. R. (2022). Climate warming in the Himalayas threatens biodiversity, ecosystem functioning and ecosystem services in the 21st century: is there a better solution? Biodiversity Conserv. 31 (8-9), 2017–2044. doi: 10.1007/s10531-022-02417-6
Khan, M. S., Zaidi, A., Wani, P. A. (2007). Role of phosphate-solubilizing microorganisms in sustainable agriculture - A review. Agron. Sustain. Dev. 27 (1), 29–43. doi: 10.1051/agro:2006011
Lehmann, A., Rillig, M. C. (2015). Understanding mechanisms of soil biota involvement in soil aggregation: A way forward with saprobic fungi? Soil Biol. Biochem. 88, 298–302. doi: 10.1016/j.soilbio.2015.06.006
Lehmann, A., Zheng, W., Rillig, M. C. (2017). Soil biota contributions to soil aggregation. Nat. Ecol. Evol. 1 (12), 1828–1835. doi: 10.1038/s41559-017-0344-y
Lehmann, A., Zheng, W., Ryo, M., Soutschek, K., Roy, J., Rongstock, R., et al. (2020). Fungal traits important for soil aggregation. Front. Microbiol. 10. doi: 10.3389/fmicb.2019.02904
Li, C., Jia, Z., Peng, X., Zhai, L., Zhang, B., Liu, X., et al. (2021a). Functions of mineral-solubilizing microbes and a water retaining agent for the remediation of abandoned mine sites. Sci. Total Environ. 761, 143215. doi: 10.1016/j.scitotenv.2020.143215
Li, C., Jia, Z., Yuan, Y., Cheng, X., Shi, J., Tang, X., et al. (2020). Effects of mineral-solubilizing microbial strains on the mechanical responses of roots and root-reinforced soil in external-soil spray seeding substrate. Sci. Total Environ. 723, 138079. doi: 10.1016/j.scitotenv.2020.138079
Li, C., Jia, Z., Zhai, L., Zhang, B., Peng, X., Liu, X., et al. (2021b). Effects of mineral-solubilizing microorganisms on root growth, soil nutrient content, and enzyme activities in the rhizosphere soil of robinia pseudoacacia. Forests 12 (1). doi: 10.3390/f12010060
Li, C., Zhao, S., Zhou, H., Liu, C., Zhang, Y. (2019). A review of research progress of vegetation concrete on coastal highway slope. J. Coast. Res. 94, 367. doi: 10.2112/SI94-075.1
Li, R., Zhang, W., Yang, S., Zhu, M., Kan, S., Chen, J., et al. (2018). Topographic aspect affects the vegetation restoration and artificial soil quality of rock-cut slopes restored by external-soil spray seeding. Sci. Rep. 8 (1). doi: 10.1038/s41598-018-30651-y
Li, S., Li, Y., Shi, J., Zhao, T., Yang, J. (2017). Optimizing the formulation of external-soil spray seeding with sludge using the orthogonal test method for slope ecological protection. Ecol. Eng. 102, 527–535. doi: 10.1016/j.ecoleng.2017.02.060
Li, T., Zhang, Y., Bei, S., Li, X., Reinsch, S., Zhang, H., et al. (2020). Contrasting impacts of manure and inorganic fertilizer applications for nine years on soil organic carbon and its labile fractions in bulk soil and soil aggregates. Catena 194. doi: 10.1016/j.catena.2020.104739
Li, X., Wang, J., Lin, J., Yin, W., Shi, Y. Y., Wang, L., et al. (2022). Hysteresis analysis reveals dissolved carbon concentration - discharge relationships during and between storm events. Water Res. 226, 119220. doi: 10.1016/j.watres.2022.119220
Liang, C., Amelung, W., Lehmann, J., Kästner, M. (2019). Quantitative assessment of microbial necromass contribution to soil organic matter. Global Change Biol. 25 (11), 3578–3590. doi: 10.1111/gcb.14781
Liang, C., Schimel, J. P., Jastrow, J. D. (2017). The importance of anabolism in microbial control over soil carbon storage. Nat. Microbiol. 2 (8). doi: 10.1038/nmicrobiol.2017.105
Lin, H., Bean, S. R., Tilley, M., Peiris, K. H. S., Brabec, D. (2020). Qualitative and quantitative analysis of sorghum grain composition including protein and tannins using ATR-FTIR spectroscopy. Food Analytical Methods 14 (2), 268–279. doi: 10.1007/s12161-020-01874-5
Liu, Q., Su, L.-j., Xiao, H., Xu, W.-n., Xia, Z.-y. (2019). Selection and configuration of plants in high- and steep-Cutting rock slope greening in a subtropical region: case study of the first-Phase urban expressway of xiazhou avenue in yichang. J. Highway Transportation Res. Dev. (English Edition) 13 (4), 102–110. doi: 10.1061/JHTRCQ.0000710
Ma, W., Peng, D., Walker, S. L., Cao, B., Gao, C.-H., Huang, Q., et al. (2017). Bacillus subtilis biofilm development in the presence of soil clay minerals and iron oxides. NPJ Biofilms Microbiomes 3 (1). doi: 10.1038/s41522-017-0013-6
Merino-Martín, L., Stokes, A., Gweon, H. S., Moragues-Saitua, L., Staunton, S., Plassard, C., et al. (2021). Interacting effects of land use type, microbes and plant traits on soil aggregate stability. Soil Biol. Biochem. 154, 108072. doi: 10.1016/j.soilbio.2020.108072
Miltner, A., Bombach, P., Schmidt-Brücken, B., Kästner, M. (2011). SOM genesis: microbial biomass as a significant source. Biogeochemistry 111 (1-3), 41–55. doi: 10.1007/s10533-011-9658-z
Mohamed, B. A., Janaki, P. (2021). Determination of active ingredients in commercial insecticides using spectral characteristics of Fourier transform infrared spectroscopy (FTIR). J. Appl. Natural Sci. 13 (SI), 110–123. doi: 10.31018/jans.v13iSI.2809
Morris, E. K., Morris, D. J. P., Vogt, S., Gleber, S. C., Bigalke, M., Wilcke, W., et al. (2019). Visualizing the dynamics of soil aggregation as affected by arbuscular mycorrhizal fungi. ISME J. 13 (7), 1639–1646. doi: 10.1038/s41396-019-0369-0
Murphy, B. W. (2015). Impact of soil organic matter on soil properties—a review with emphasis on Australian soils. Soil Res. 53 (6), 605. doi: 10.1071/SR14246
Mustafa, A., Minggang, X., Ali Shah, S. A., Abrar, M. M., Nan, S., Baoren, W., et al. (2020). Soil aggregation and soil aggregate stability regulate organic carbon and nitrogen storage in a red soil of southern China. J. Environ. Manage. 270, 110894. doi: 10.1016/j.jenvman.2020.110894
Newnham, E. A., Gao, X., Tearne, J., Guragain, B., Jiao, F., Ghimire, L., et al. (2019). Adolescents’ perspectives on the psychological effects of natural disasters in China and Nepal. Transcultural Psychiatry 57 (1), 197–211. doi: 10.1177/1363461519893135
Oades, J. M., Waters, A. G. (1991). Aggregate hierarchy in soils. Soil Res. 29 (6), 815. doi: 10.1071/SR9910815
Obalum, S. E., Chibuike, G. U., Peth, S., Ouyang, Y. (2017). Soil organic matter as sole indicator of soil degradation. Environ. Monit. Assess. 189 (4). doi: 10.1007/s10661-017-5881-y
Ogarkov, O. B., Badleeva, V., Belkova, N. L., Adelshin, R. V., Tsyrenova, T. A., Khromova, P. A., et al. (2018). The Phenomenon of the Formation of Biofilms by Brevibacillus spp. and Bacillus spp. in the Presence of Clinical Strains of Mycobacterium tuberculosis. Mol. genetics Microbiol. Virol. 32 (3), 148–154. doi: 10.1007/s11104-017-3407-6
Okello, C., Tomasello, B., Greggio, N., Wambiji, N., Antonellini, M. (2015). Impact of population growth and climate change on the freshwater resources of lamu island, Kenya. Water 7 (12), 1264–1290. doi: 10.3390/w7031264
Paul, E. A. (2016). The nature and dynamics of soil organic matter: Plant inputs, microbial transformations, and organic matter stabilization. Soil Biol. Biochem. 98, 109–126. doi: 10.1016/j.soilbio.2016.04.001
Poirier, V., Roumet, C., Angers, D. A., Munson, A. D. (2017). Species and root traits impact macroaggregation in the rhizospheric soil of a Mediterranean common garden experiment. Plant Soil 424 (1-2), 289–302.
Recio-Vazquez, L., Almendros, G., Knicker, H., Carral, P., Álvarez, A.-M. (2014). Multivariate statistical assessment of functional relationships between soil physical descriptors and structural features of soil organic matter in Mediterranean ecosystems. Geoderma 230-231, 95–107. doi: 10.1016/j.geoderma.2014.04.002
Rillig, M. C., Muller, L. A. H., Lehmann, A. (2017). Soil aggregates as massively concurrent evolutionary incubators. ISME J. 11 (9), 1943–1948. doi: 10.1038/ismej.2017.56
Rosselló, J., Becken, S., Santana-Gallego, M. (2020). The effects of natural disasters on international tourism: A global analysis. Tourism Manage. 79, 104080. doi: 10.1016/j.tourman.2020.104080
Sandhya, V., Ali, S. Z. (2015). The production of exopolysaccharide by Pseudomonas putida GAP-P45 under various abiotic stress conditions and its role in soil aggregation. Microbiology 84 (4), 512–519. doi: 10.1134/S0026261715040153
Sarker, T. C., Incerti, G., Spaccini, R., Piccolo, A., Mazzoleni, S., Bonanomi, G. (2018). Linking organic matter chemistry with soil aggregate stability: Insight from 13C NMR spectroscopy. Soil Biol. Biochem. 117, 175–184. doi: 10.1016/j.soilbio.2017.11.011
Schomburg, A., Verrecchia, E. P., Guenat, C., Brunner, P., Sebag, D., Le Bayon, R. C. (2018). Rock-Eval pyrolysis discriminates soil macro-aggregates formed by plants and earthworms. Soil Biol. Biochem. 117, 117–124. doi: 10.1016/j.soilbio.2017.11.010
Shao, L. (2019). Geological disaster prevention and control and resource protection in mineral resource exploitation region. Int. J. Low-Carbon Technol. 14 (2), 142–146. doi: 10.1093/ijlct/ctz003
Six, J., Elliott, E. T., Paustian, K. (2000). Soil macroaggregate turnover and microaggregate formation: a mechanism for C sequestration under no-tillage agriculture. Soil Biol. Biochem. 32 (14), 2099–2103. doi: 10.1016/S0038-0717(00)00179-6
Szymański, W. (2017). Quantity and chemistry of water-extractable organic matter in surface horizons of Arctic soils under different types of tundra vegetation – A case study from the Fuglebergsletta coastal plain (SW Spitsbergen). Geoderma 305, 30–39. doi: 10.1016/j.geoderma.2017.05.038
Tafintseva, V., Shapaval, V., Smirnova, M., Kohler, A. (2019). Extended multiplicative signal correction for FTIR spectral quality test and pre-processing of infrared imaging data. J. Biophotonics 13 (3). doi: 10.1002/jbio.201960112
Tang, W., Mohseni, E., Wang, Z. (2018). Development of vegetation concrete technology for slope protection and greening. Construction Building Materials 179, 605–613. doi: 10.1016/j.conbuildmat.2018.05.207
Tisdall, J. M., Oades, J. M. (1982). Organic matter and water-stable aggregates in soils. J. Soil Sci. 33 (2), 141–163. doi: 10.1111/j.1365-2389.1982.tb01755.x
Vanwalleghem, T., Gómez, J. A., Infante Amate, J., González de Molina, M., Vanderlinden, K., Guzmán, G., et al. (2017). Impact of historical land use and soil management change on soil erosion and agricultural sustainability during the Anthropocene. Anthropocene 17, 13–29. doi: 10.1016/j.ancene.2017.01.002
Voltr, V., Menšík, L., Hlisnikovský, L., Hruška, M., Pokorný, E., Pospíšilová, L. (2021). The soil organic matter in connection with soil properties and soil inputs. Agronomy 11 (4), 779. doi: 10.3390/agronomy11040779
Walkley, A., Black, I. A. (1934). An examination of the degtjareff method for determining soil organic matter, and a proposed modification of the chromic acid titration method. Soil Sci. 37 (1), 29–38. doi: 10.1097/00010694-193401000-00003
Wang, X. H., Li, Q., Sui, J. K., Zhang, J. M., Liu, Z. Y., Du, J. F., et al. (2019). Isolation and characterization of antagonistic bacteria paenibacillus jamilae HS-26 and their effects on plant growth. BioMed. Res. Int. 2019, 3638926–3638913. doi: 10.1155/2019/3638926
Wang, Y., Liu, J., Lin, C., Ma, X.-f., Song, Z.-z., Chen, Z.-h., et al. (2022). Polyvinyl acetate-based soil stabilization for rock slope ecological restoration. J. Environ. Manage. 324, 116209. doi: 10.1016/j.jenvman.2022.116209
Wang, L., Tang, X., Liu, X., Zhang, J. (2022). Mineral-solubilizing soil bacteria permanently green rocky slopes by enhancing soil adhesion to the surface of rocky slopes. Forests 13 (11), 1820-1833. doi: 10.3390/f13111820
Wang, L., Tang, X., Liu, X., Zhang, J. (2023). Active permanent greening – a new slope greening technology based on mineral solubilizing microorganisms. Front. Plant Sci. 14. doi: 10.3389/fpls.2023.1219139
Wang, A., Zha, T., Zhang, Z. (2023). Variations in soil organic carbon storage and stability with vegetation restoration stages on the Loess Plateau of China. Catena 228. doi: 10.1016/j.catena.2023.107142
Wu, Y., Zhang, J., Guo, X. (2017a). An indigenous soil bacterium facilitates the mitigation of rocky desertification in carbonate mining areas. Land Degradation Dev. 28 (7), 2222–2233. doi: 10.1002/ldr.2749
Wu, Y., Zhang, J., Guo, X., Wang, Y., Wang, Q. (2017b). Isolation and characterisation of a rock solubilising fungus for application in mine-spoil reclamation. Eur. J. Soil Biol. 81, 76–82. doi: 10.1016/j.ejsobi.2017.06.011
Yang, H., Deng, J., Yuan, Y., Fan, D., Zhang, Y., Zhang, R., et al. (2015). Two novel exopolysaccharides from Bacillus amyloliquefaciens C-1: antioxidation and effect on oxidative stress. Curr. Microbiol. 70 (2), 298–306. doi: 10.1007/s00284-014-0717-2
Zeng, Q., Darboux, F., Man, C., Zhu, Z., An, S. (2018). Soil aggregate stability under different rain conditions for three vegetation types on the Loess Plateau (China). Catena 167, 276–283. doi: 10.1016/j.catena.2018.05.009
Zhang, W., Ruirui, L., Ai, X., Chen, J., Xu, W., Li, W., et al. (2018). Enzyme activity and microbial biomass availability in artificial soils on rock-cut slopes restored with outside soil spray seeding (OSSS): Influence of topography and season. J. Environ. Manage. 211, 287–295. doi: 10.1016/j.jenvman.2018.01.005
Zhao, J., Chen, S., Hu, R., Li, Y. (2017). Aggregate stability and size distribution of red soils under different land uses integrally regulated by soil organic matter, and iron and aluminum oxides. Soil Tillage Res. 167, 73–79. doi: 10.1016/j.still.2016.11.007
Zhao, F. Z., Fan, X. D., Ren, C. J., Zhang, L., Han, X. H., Yang, G. H., et al. (2018). Changes of the organic carbon content and stability of soil aggregates affected by soil bacterial community after afforestation. Catena 171, 622–631. doi: 10.1016/j.catena.2018.08.006
Keywords: slope stability, organic matter characteristics, infrared spectral analysis, soil aggregates, structural equation modeling
Citation: Wang L, Tang X, Liu X, Xue R and Zhang J (2023) Mineral solubilizing microorganisms and their combination with plants enhance slope stability by regulating soil aggregate structure. Front. Plant Sci. 14:1303102. doi: 10.3389/fpls.2023.1303102
Received: 27 September 2023; Accepted: 16 November 2023;
Published: 28 December 2023.
Edited by:
Sergio de los Santos Villalobos, Instituto Tecnológico de Sonora (ITSON), MexicoReviewed by:
Chun Liu, Jinan University, ChinaVasileios Tzanakakis, Hellenic Mediterranean University, Greece
Copyright © 2023 Wang, Tang, Liu, Xue and Zhang. This is an open-access article distributed under the terms of the Creative Commons Attribution License (CC BY). The use, distribution or reproduction in other forums is permitted, provided the original author(s) and the copyright owner(s) are credited and that the original publication in this journal is cited, in accordance with accepted academic practice. No use, distribution or reproduction is permitted which does not comply with these terms.
*Correspondence: Jinchi Zhang, emhhbmdqYzg4MTFAZ21haWwuY29t