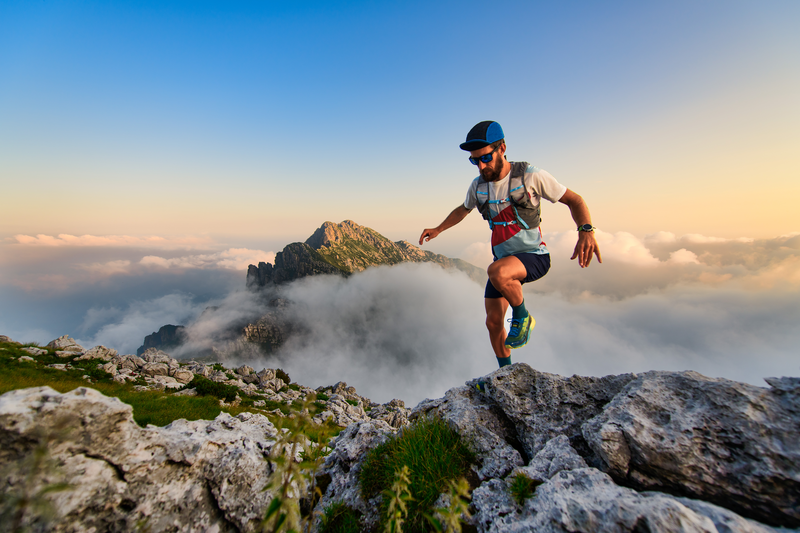
94% of researchers rate our articles as excellent or good
Learn more about the work of our research integrity team to safeguard the quality of each article we publish.
Find out more
ORIGINAL RESEARCH article
Front. Plant Sci. , 04 January 2024
Sec. Plant Metabolism and Chemodiversity
Volume 14 - 2023 | https://doi.org/10.3389/fpls.2023.1297449
Introduction: Ferula is a traditional, edible, and important medicinal plant with high economic value. The distinction between edible and non-edible Ferula remains unclear.
Methods: In this study, headspace solid-phase microextraction coupled to gas chromatography-mass spectrometry (HS-SPME/GC-MS) and ultra-high performance liquid chromatography-tandem mass spectrometry (UHPLC-MS/MS) non-targeted metabolomics techniques were used to systematically and comprehensively analyse secondary metabolites in the leaves and roots of four species of Ferula, considering their edibility.
Results: A total of 166 leaf volatile organic compounds (VOCs) and 1,079 root metabolites were identified. Additionally, 42 potential VOCs and 62 differential root metabolites were screened to distinguish between edible and non-edible Ferula. Twelve volatile metabolites were specific to F. feurlaeoides, and eight compounds were specific to the three edible Ferula species. The results showed that compounds containing sulphur, aldehydes, and ketones, which produce pungent odours, were the primary sources of the strong odour of Ferula. The root differential metabolites include 13 categories, among which the high concentration group is organic acids, amino acids, terpenoids and fatty acids. The bioactive metabolites and VOCs in the roots exhibited species-specific characteristics. VOCs with various odors were linked to the distribution of root metabolites in both edible and non-edible Ferula plants. The screened root markers may contribute to the formation of characteristic VOCs.
Discussion: This study identified the difference in flavour between edible and non-edible Ferula plants and, for the first time, demonstrated the contribution of the efficacy of Ferula root to the unique flavour of the above-ground parts of Ferula. These results provide a theoretical basis for selecting Ferula for consumption and help evaluate the quality of different species of Ferula. Our findings may facilitate food processing and the further development of Ferula.
Pharmacologically active ingredients of medicinal plants have provided rich resources for the development of new drugs (Rahman et al., 2019). Several medicinal plants are also edible and can supply the necessary nutrients to the body; such plants are known as edible and medicinal plants (EMPs). EMPs contain high amounts of vitamins, fibre, and essential amino acids, as well as other medicinally active ingredients essential for good health, and some EMPs are more popular than others owing to their unique flavour. However, their nutritional and phytochemical compositions remain to be fully elucidated (Tan et al., 2022).
In recent years, interest in edible medicinal plants as new products with beneficial properties has increased (Cheng et al., 2022). Apiaceae is one of the most studied families of angiosperms with economic importance (Wei et al., 2019). Apiaceae plants are known for their production of aromatic compounds, particularly volatile oils, which contribute to their distinctive flavours and medicinal properties. These volatile oils are often rich in compounds such as terpenes, phenylpropanoids, and flavonoids, which have been extensively studied for their pharmacological activities (Amiri and Joharchi, 2016; Bahmankar et al., 2019; Mohammadhosseini et al., 2019; Akaberi et al., 2020). Furthermore, Apiaceae plants have significant ethnobotanical importance, being traditionally used by various ethnic groups for culinary, medicinal, and ritualistic purposes, and several species are consumed as wild vegetables or spices due to their high nutritional and medicinal value (Li et al., 2018). The Chinese government has promoted the cultivation and breeding of medicinal plants of the Apiaceae family, and various medicinal plants, such as Panax ginseng C.A. Meyer (He et al., 2022), Angelica sinensis (Oliv.) Diels (Xiao et al., 2021), and Ligusticum chuanxiong Hort (Chen et al., 2018), have been domesticated as cash crops.
Ferula, the third largest genus in the family Apiaceae, is distributed worldwide, particularly in Asia (Khayat et al., 2023) (Supplementary Figure 1). Ferula is a perennial herb that bears one or more fruits. Plants of this genus have been recorded in the Compendium of Materia Medica in China for 2000 years. The whole plant of most species has a potent smell like that of onions and garlic; its roots and rhizomes have been used as medicinal materials due to their immunosuppressive, antipyretic, analgesic, and anti-inflammatory activities (Amalraj and Gopi, 2017; Zhang et al., 2021). During early spring in Central Asia, the crops are not fully grown; however, Ferula leaves grow and are collected as a vegetable due to their unique flavour of these leaves. In addition, its dried roots or leaves are used as spices; therefore, the whole plant has important economic value and is an important EMP (Radulović et al., 2013). Owing to the special flavour of its leaves and the excellent medicinal value of the roots, Ferula has been used as a special food in China. For the protection of Ferula resources and their sustainable development, research and planting have been conducted in Xinjiang, the main Ferula production area in China.
Previous studies on Ferula have focused on the analysis of its chemicals and validation of their medicinal value (Mohammadhosseini et al., 2019); for instance, the natural extract of Ferula has been reported to exhibit antibacterial, anticancer, antioxidant and melanogenesis inhibitory effects (Charmforoshan et al., 2019; Kamelan Kafi et al., 2022). Although there is a wealth of information on the biological activity of Ferula, few studies have simultaneously compared multiple types of Ferula species simultaneously. In addition, many of the parts used to study the biological activities were underground parts; therefore, little consideration has been given to the botanical characteristics of the aboveground parts, which comprise more organs. The unique odour of Ferula is attributed to the VOCs in the leaves, and research has shown that the VOCs of plants are not only aromatic components, but also exhibit various medicinal properties as natural compounds, including antibacterial effects (Ricciardi et al., 2021). Studies have also shown that VOCs are essential for defence responses and interactions between plants and microbes (Boncan et al., 2020; Feng et al., 2023). However, systematic studies on the overall VOC composition of Ferula plants are currently lacking. In addition, compared with the fast and high-throughput characteristics of omics, traditional nutrient composition detection methods only focus on the detection of a few compounds or a certain class of compounds, and cannot effectively reflect the whole picture of metabolic compounds in Ferula. Metabolomics, as a rapidly advancing omics technique, focuses on the comprehensive analysis and quantification of metabolites in distinct anatomical regions of higher plants. Its application extends to the investigation of medicinal plants and its relevance in the food industry has also been extensively explored (Mei et al., 2022; Utpott et al., 2022). Exploring the scientific connotations of traditional medicinal knowledge using phytochemistry, pharmacology, and metabolomics is the mainstream research direction for medicinal botany (Wang et al., 2023).
The unique odour of Ferula can help distinguish between edible and nonedible Ferula species. The more pungent the smell is, the better the medicinal value and the greater the benefits to the body; however, evidence to support this is lacking, and the correlation between the unique smell of Ferula and its medicinal value has not been fully studied. Specifically, two major questions remain to be answered: (1) can the smell be used to distinguish between edible and non-edible Ferula species? (2) what is the relationship between the smell and the medicinal components? To answer these questions, the compound compositions of the main medicinal part (root) and main edible part (leaf) of four species of Ferula were analysed in this study. Changes in the VOCs of different species of Ferula were assessed using headspace solid-phase microextraction coupled to gas chromatography-mass spectrometry (HS-SPME/GC-MS), while the metabolic profiles of different species of Ferula were investigated using the non-targeted metabolomics method, ultra-high performance liquid chromatography-tandem mass spectrometry (UHPLC-MS/MS). Overall, this study aimed to determine the metabolic characteristics of four species of Ferula, identify specific markers of the leaves and roots of Ferula, and elucidate the effects of roots on leaf metabolism. The results of this study will help clarify the contribution of VOCs to the leaf flavour, elucidate the effects of roots on leaf metabolism, and highlight the correlation between the effects of metabolism in the root and leaf.
Leaves and roots of four species of Ferula were collected from farms in Shihezi City (Collection details are shown in Supplementary Table 1). The four species were identified as Ferula lehmannii Boiss. Fl. Or., F. sinkiangensis K. M. Shen., F. teterrima Kar.et Kir., and F. feurlaeoides (Steud.) Korov by Professor Yan Ping from the Herbarium of Shihezi University (SHI). Among them, F. lehmannii, F. sinkiangensis, and F. teterrima are medicinal plants with a strong odour throughout the plant and are commonly consumed as wild vegetables. Ferula feurlaeoides has a bitter smell and is not used as a vegetable or medicinal plant.
The collected leaf samples were immediately placed in liquid nitrogen and transported to the laboratory. All samples were stored at −80°C until analysis. After the fresh roots were collected, they were dried indoors and protected from direct sunlight. Three samples were collected for each species, and subsequent analysis was conducted after thorough drying.
LC-MS grade methanol (MeOH) and LC-MS grade acetonitrile (ACN) were obtained from Fisher Scientific (Loughborough, UK). 2- Amino-3-(2-chloro-phenyl)-propionic acid and ammonium formate were obtained from Aladdin Company (Shanghai, China); while formic acid was sourced from TCI (Shanghai, China). The water was purified using the Millipore system (Millipore, Bedford).
The determination was performed using the HS-SPME method. 1g leaves was placed in a 20mL headspace bottle, and then sealed with a rubber spacer-equipped bottle cap. The extraction was carried out using CTC Autosampler Syringes, extraction fibres: 50/30um DVB/CAR on PDMS (57348-U, Supelco, USA); Temperature: 50 °; He oscillation was conducted for 15 minutes followed by a 30-minute extraction period; Oscillation speed: 250 rpm; Analysis time: 5 min; GC cycle time: 50 min. After extraction, the probe was removed and inserted into the gas chromatography inlet. The extraction layer was then pushed out, and GC-MS was employed for the detection and analysis of volatile compounds.
GC conditions: The volatile matter was detected on an Agilent 7890 B gas chromatograph (Agilent Technologies, USA) coupled with an Agilent 5977B triple quadrupole mass spectrometry detector. DB-wax capillary column (30 m ×0.25 mm inner diameter ×0.25 μm film thickness) for the separation of volatile compounds. The initial column temperature was set at 40°C and held for 5 min. Then, the temperature was increased at a rate of 5°C/min until it reached 220°C. Subsequently, the temperature was further increased at a rate of 20/min to 250°C and held for 2.5 min. The injection temperature is 270°C and the shunt free mode is used with an inlet lining with an internal diameter of 0.75 mm. The flow rate of the carrier gas was set at 1.0 ml/min (99.999%).
MS condition: The ion source was operated in the EI+ mode with an electron ionization energy 70eV. The ion source temperature was set at 230 °, and the quadrupole temperature was set at 150 °. Full scan mode was used for mass spectrometry analysis with a scanning range of 20-400 m/z.
The sample was weighed and placed in a 2 mL centrifuge tube. Methanol (600 μL) containing 2-Amino-3-(2-chloro-phenyl)-propionic acid (4 ppm) was added and vortexed for 30 seconds. Glass beads (100 mg) were added and the mixture was ground for 90 seconds. Ultrasound treatment was conducted for 15 minutes at room temperature. After centrifugation at 12,000 rpm and 4°C for 10 minutes, the supernatant was filtered through a 0.22 μm membrane and transferred for subsequent analysis (Vasilev et al., 2016).
UHPLC-MS/MS analysis was performed on the Thermo Orbitrap Exploris 120 mass spectrometer (Thermo Fisher Scientific, USA) equipped with an Electrospray ion Source (ESI) and a Thermo Vanquish ultra-high performance Liquid chromatography (UHPLC) system (Thermo Fisher Scientific, USA). Chromatographic separation was conducted using ACQUITY UPLC® HSS T3(2.1×150 mm, 1.8 μm) (Waters, Milford, MA, USA) column.
The automatic sampler and column temperatures were set at 10°C and 40°C, respectively. The flow rate in gradient mode was 0.25 mL/min. In the positive ion mode, the mobile phase consisted of formic acid water (0.1% formic acid aqueous solution, v/v) (mobile phase A) and acidified acetonitrile (0.1% acetonitrile solution, v/v) (mobile phase B). The linear gradient of mobile phase B was 0-1 min, 2% B; 1-9 min, 2%-50% B; 9-12 min, 50%-98% B; 12-13.5 min, 98% B; 13.5-14 min, 98%-2% B; 14-20 min, 2% B. In the negative ion mode, the mobile phase consisted of acetonitrile (C) and 5 mM ammonium formate water (D). The gradient elution procedure was as follows 0-1 min, 2% C; 1-9 min, 2%-50% C; 9-12 min, 50%-98% C; 12-13.5 min, 98% C; 13.5-14 min, 98%-2% C; 14-17 min, 2% VC (Zelena et al., 2009; Want et al., 2013).
A sample volume of 2 uL was injected into the system and analysed in positive and negative ion ionization (ESI) mode. The positive ion spray voltage was set at 3.50 kV, and the negative ion spray voltage was set at -2.50 kV. The capillary temperature was maintained at 325 °. For the first-level analysis, a full scan was performed at a resolution of 60,000, covering a mass-to-charge ratio (m/z)range of100-1000, and the second-level fragmentation was carried out using higher-energy collisional dissociation (HCD). with a collision voltage of 30%, the second-level analysis was performed at a resolution of 15,000, and the first four ions were subjected to fragmentation before signal collection. Additionally, dynamic exclusion was employed to eliminate redundant MS/MS information.
The identification of volatile compounds from HS-SPME/GC-MS data was performed based on their mass spectrum fragments. The identification process involved manual analysis and comparison with the National Institute of Standards and Technology (NIST Mass Spectral Library 2014). In addition, the mass nucleus ratio of the isolated volatile compounds was compared with the literature values to identify the GC-MS detection results, and the peak area normalization method was used to calculate the volatile compounds content.
The UHPLC-MS/MS data were converted into mzXML file format using the MSConvert tool in the Proteowizard software package (v3.0.8789). The quantitative list of substances was obtained by employing the R XCMS software package for peak detection, peak filtering and peak alignment, the parameters are bw = 2, ppm = 15, peakwidth = c (5, 30), mzwid = 0.015, mzdiff = 0.01, method=‘centWave’. Substance identification utilized public databases, including HMDB (http://www.hmdb.ca), massbank (http://www.massbank.jp/), LipidMaps (http://www.lipidmaps.org), mzcloud (https://www.mzcloud.org), KEGG (http://www.genome.jp/kegg/) and self-built material bank were used for substance identification, and the parameter with the parameter set to ppm < 30 ppm. The LOESS signal correction method based on QC sample, was employed for data correction and eliminates system error. After normalization, only ion peaks with relative standard deviations (RSDs) less than 30% in QC were kept to ensure proper metabolite identification. After the pretreatment of data had been submitted to the MetaboAnalyst (https://www.metaboanalyst.ca), used for principal component analysis (PCA), orthogonal partial least squares discriminant analysis (OPLS-DA)were performed. Clustering heatmap was performed based on the normalized data of the independent variables. Meanwhile, clustering heatmap analysis (HCA) was conducted using MetaboAnalyst 5.0. Screening criteria for volatile differential metabolites consisted of Fold Change ≥ 2 or Fold Change ≤ -2 and p < 0.05. The differential metabolites of the roots were screened by combining variables important in the projection variables important in the projection (VIP) > 1.0 and p < 0.05.The selected differential metabolites were enriched and analyzed according to metabolic pathways using MetaboAnalyst database. Variance analysis (ANOVA), significance analysis (p < 0.05), and mapping were performed using SPSS 19.0 (SPSS Inc., Chicago, IL, USA) and Origin 2023b software, respectively.
We assessed the volatile metabolites in leaf samples of Ferula and identified 166 VOCs (Supplementary Table 2), including 54 alkenes (Among them are 24 monoterpene hydrocarbons and 29 sesquiterpene hydrocarbons), 29 aldehydes, 21 ketones, 14 alcohols, 14 esters, 12 sulphur-containing compounds, 9 acids and 13 other compounds. Leaf flavour quality was affected by the content and proportion of aroma components. The clustering heat map (Figure 1A) showed that the leaves had abundant aroma components, all of which showed good repeatability within the group, and edible Ferula was well distinguished from nonedible Ferula. There were significant differences in the composition, content, proportion, and unique components, indicating obvious differences in aroma characteristics among the different species.
Figure 1 Heatmaps and type of measured volatiles in Ferula samples. (A) Heatmap of volatile metabolites. (B) Histogram of relative content of volatile metabolite components. FE, F. feurlaeoide; LE, F. lehmannii; SI, F. sinkiangensis; TE, F. teterrima. Different letters indicate significant species differences at the 0.05 level.
There were also significant differences in the contents of various compounds among the different species. As shown in Figure 1B, alkenes, aldehydes, and sulphur-containing compounds had the highest contents among the volatile compounds. Furthermore, alkene compounds were the most abundant (56). Ferula feurlaeoides contained significantly higher alkene compounds than the other species, while F. lehmannii contained 35.27% of the total alkene compounds identified.
Aldehydes were the second most abundant component (30); the content of aldehydes in F. feurlaeoides was significantly lower than that in the remaining species. Alcohols and ketones were less abundant than alkenes, aldehydes, and sulphur compounds, with no significant differences among the species. Although there were only 11 sulphur-containing compounds, the content was very high in the volatiles, and the content in F. feurlaeoides was very small (0.32%) in the four species of Ferula; this is consistent with the smell of the four species of Ferula. Other compounds, such as esters and acids, were present in relatively small amounts. Compounds with higher contents may have a higher contribution to the unique aroma of different flavours. The odour characteristics of 31 compounds with a high content (≥1%) in the four Ferula species are listed in Table 1. Among them, (Z)-sec-butyl propenyl disulfide, (E)-sec-butyl propenyl disulphide, and β-myrcene are the typical aroma components reported in Ferula.
Multivariate analysis was performed based on the relative content of 166 VOCs using PCA and OPLS-DA to distinguish the four species of Ferula. Unsupervised PCA was used to evaluate the clustering of the four groups of Ferula samples. As shown in Figure 2A, the first principal component (PC) 1 and PC (PC2) accounted for 48.9% and 17.2% of the variation, respectively, with a cumulative contribution rate of 66.1%. Ferula samples from the different odour groups were all within the 95% confidence interval, indicating that the results were credible. As shown in the OPLS-DA plot (Figure 2B), samples from the four species of Ferula were divided into two groups based on whether they were edible or not, indicating significant differences in the metabolites. Further analysis of the odour characteristic compounds between the three strongly aromatic edible and nonedible (F. feurlaeoides) Ferula species was conducted to explore the composition differences; a correlation analysis was also performed.
Figure 2 Multivariate statistical analysis of volatile organic compounds. (A) Principal component analysis of all samples. (B) OPLS-DA score plot of all samples. (C) Volcano plot of differential metabolites. (D) Cluster heat map of differential volatile metabolite. (E) Taxonomic donut plot of differential volatiles. (F) Correlation analysis diagram of differential volatiles. * indicates a significant correlation.
To identify potential odour markers to distinguish the four species of Ferula, VIP and fold-change results from the OPLS-DA model were used to screen for the most representative metabolites. A total of 42 potential VOCs were screened; these metabolites could distinguish between the two different odours of Ferula leaf samples (Figure 2C). Figures 2D, E show an overview of the distribution of four characteristic metabolites of Ferula, including twelve alkenes, seven aldehydes, seven ketones, six alcohols, three esters, two acids, two sulphur-containing compounds, and three other classes. Twelve volatile metabolites were specific to F. feurlaeoides, while eight were specific to the three edible Ferula species.
Sixteen compounds showed an upregulated trend, representing a total of eight compound types (Figure 2C), mainly alkenes (eight species). Among them, eight compounds were common to the three edible Ferula species with special flavours; 1-Hexanol and β-elemene showed the highest upregulation trend. Most upregulated compounds were characteristic metabolites of edible Ferula with a pungent flavour. Although there were common compounds among the four Ferula species, the content in F. feurlaeoides was much lower than that in the other three species; therefore, these upregulated compounds are characteristic markers of Ferula consumption.
Twenty-six compounds were downregulated compounds, nine of which were different and12 of which were unique to F. feurlaeoides. In contrast to the upregulated compounds, the F. feurlaeoides content was advantageous. Dimethyl sulphide was unique to F. feurlaeoides. Although the content was extremely low, it was in line with our previous results showing that sulphide is a common constituent of Ferula. These downregulated compounds, mostly having fresh grassy and floral-fruity smell, are characteristic marker compounds of F. feurlaeoides.
Correlation analysis showed that butanoic acid, a significantly downregulated compound, was significantly positively correlated with dimethyl sulphide (p < 0.05) (Figure 2F). There was a negative correlation between compounds shared by the other three edible Ferula species, which was significant for compounds such as alcohols and ketones. In addition, two sulphides, thiophene,3,4-dimethyl- and 1-(1-(methylthio) propyl)-2-propyldisulfane, were significantly positively correlated with propanoic acid,1-pentano, propanoic acid (p < 0.05). These four compounds had a pungent odour and are important flavour sources for the three edible Ferula species. Among the alkene compounds, the specific compounds of edible Ferula exhibited an opposite trend to the alkene compounds of F. feurlaeoides. In general, the correlation between the compounds with a special flavour and the characteristic compounds of F. feurlaeoides showed an opposite trend, consistent with the results of the up- and downregulated compounds; therefore, the 42 compounds can represent the differential metabolites of these four species.
The root samples of the four species of edible and nonedible Ferula were examined using non-targeted metabolomics; the overall relative content and species distribution are shown in Figure 3A. A total of 709 non-volatile metabolites of 17 classes were identified, which included 130 organic acids, 93 amino acids, 68 carbohydrates, 60 nucleotides, 50 alkaloids, 48 phenols, 42 terpenoids, 40 fatty acids, 32 flavonoids, 24 alcohols, 18 esters, 15 phenylpropanoids, 14 vitamins, 11 ketones, 10 amines, 10 aldehydes, and 87 other compounds.
Figure 3 Metabolite analysis of Ferula root. (A) Pie chart of root metabolites. (B) heatmap of root metabolites. (C) PCA score plots in the positive mode. (D) PCA score plots in the negative ion mode.
Heatmaps based on all the identified metabolites showed that their variation and accumulation differed significantly across species (Figure 3B). The metabolite accumulation in the three species of edible Ferula, i.e., F. lehmannii, F. sinkiangensis, and F. teterrima, was similar, and there was a clear distinction between them and the nonedible F. feurlaeoides. MetaboAnalyst was used for multivariate analysis of the data matrix of non-targeted metabolites from the root using Pareto correlation to differentiate between different groups of samples based on different metabolites. PCA showed that the metabolites were significantly different between the two groups. Data from the positive- and negative ion model samples confirmed their repeatability and reliability (Figures 3C, D). Further, results showed that the first two principal components contributed 76.5% of the cumulative contribution in the positive ion model (Figure 2C) (PC1 = 60.3%; PC2 = 16.2%). The total contribution in the negative ion model was 73.6% (PC1 = 46.3%, PC2 = 27.3%) (Figure 2D), and the contribution of both positive and negative ion models exceeded 70%, indicating that the model was reliable and that the good intergroup separation model was consistent with the results of the heat map.
Figure 4A shows the OPLS-DA results. Edible and nonedible Ferula species were well separated in the model. To evaluate the performance of the OPLS-DA model, the goodness of fit (R2) and goodness of prediction (Q2) were selected as the statistical validation parameters.R2 > 0.5 and Q2 value close to 1.0 indicated a high degree of fi. In the OPLS-DA mode, the accuracies of R2 and Q2 were both > 0.9,further indicating the reliability of the model (Figure 4B).
Figure 4 (A) OPLS-DA score plot of all samples. (B) Inertia histogram of model overview and 100 iterations of random permutation test of the OPLS-DA model. (C) Important features identified by OPLS-DA and VIP scores.
To further explore and screen the potential differential metabolites of the four species of Ferula, VIP score was calculated to assess the strength of the influence and explanatory power of each variable factor on the classification and differentiation of each group of samples (Figure 4C). The higher the VIP value, the greater the difference in metabolites between the groups and the more important it was to distinguish and classify the types of key bioactive components of Ferula (Scavarda et al., 2021). A total of 62 different metabolites were identified with VIP > 1, p <0.05 (t-test), and FC > 1 as thresholds (Table 2). As shown in Figure 5A, the 62 metabolites comprised 13 types, the most abundant of which were organic acids, amino acids, terpenoids, and fatty acids. Figure 5B shows the concentrations of the characteristic metabolites in the roots of the four species of Ferula. The fatty acid content was the highest; the fatty acid content of F. feurlaeoides accounted for more than half (50.48%) of all metabolites, while the organic acid content was much higher than that of the other three species of Ferula. However, the amino acid, terpene, nucleotide, alkaloid, and ester compound contents were much lower than those in the three edible Ferula species. For these characteristic metabolites, we selected those with content > 1% which included leucine, tryptophan, oleic acid, neochlorogenic acid, guanosine, and caryophyllene α oxide, for comparative analysis (Figure 5C).
Figure 5 Differential metabolite profiles of edible and nonedible Ferula. (A) Number and type of differential metabolite. (B) Percentage of differential metabolites. (C) Distribution of key metabolites substances in the four Ferula (content>1%).
To further understand the metabolic pathways enriched in the differential metabolites, MetaboAnalyst was used to perform KEGG enrichment analysis of the selected differential metabolites of edible and nonedible Ferula species. Here, the 42 and 62 significantly different metabolites identified in the volatile and bioactive characteristic metabolites of Ferula, respectively, were distributed across 30 metabolic pathways (Figures 6A, B). The eight most significant metabolic pathways were phenylalanine metabolism; butanoate metabolism of VOC differential metabolites; aminoacyl-tRNA biosynthesis; tryptophan metabolism; fatty acid biosynthesis; cutin, suberin, and wax biosynthesis; biosynthesis of unsaturated fatty acids; and biosynthesis of phenylalanine, tyrosine, and tryptophan. Fatty acid biosynthesis and phenylalanine metabolism pathways were enriched in both volatile characteristic metabolites and bioactive differential metabolites of Ferula (Figures 6C, D).
Figure 6 KEGG enrichment of the differential metabolites. (A) Volatile differential metabolites. (B) Root differential metabolites. (C) KEGG metabolic pathway classification of the differential metabolites in Leaf volatiles. (D) Root pharmacodynamic metabolites of KEGG metabolic pathway classification. (E) Correlation analysis among roots and volatile compounds in key characteristic metabolites of edible and nonedible Ferula. * indicates a significant correlation.
Subsequently, we correlated the volatile differential metabolites with six major characteristic metabolites of the bioactive components and found consistent correlation trends between the two typical amino acid metabolites (Figure 6E). The characteristic volatiles of F. feurlaeoides showed a significant negative correlation (p < 0.05), and the characteristic volatiles of the three edible Ferula species were positively correlated. Since most aroma components of Ferula are ketones, alcohols, acids, and esters, a large part of the aroma precursors synthesised by these compounds originate from amino acids. Among the bioactive components, nucleotides were significantly positively correlated with guanosine and 1-pentanol (p <0.05) and with sulphide 1-[1-(methylthio)propyl]-2-propyldisulfane. Conversely, methacrolein and α-terpinene levels showed a significant negative correlation (p < 0.05).
Oleic and neochlorogenic acids are the characteristic bioactive components of F. feurlaeoides, and their contents are higher than those in edible Ferula. This study showed that oleic and neochlorogenic acids were positively correlated with aromatic volatiles, such as alcohols, phenylacetaldehyde, alkenes, and ketones. Pungent or foul-smelling compounds, such as propanoic acid, 1-pentanol, butanal, propanal, 1-penten-3-one, 1-[1-(methylthio)propyl]-2-propyldisulfane, and thiophene,3,4-dimethyl-, showed a negative correlation. In fatty acid metabolism, unsaturated fatty acids are important precursors for the formation of volatile aroma compounds, which also explains the positive correlation between predominant fatty acid compounds in F. feurlaeoides and various aroma compounds. Terpenoid compounds, such as caryophyllene α-oxide, were significantly positively correlated with β-elemene and α-muurolene, two alkenes with fresh grass and herbal flavour (p < 0.05). There was a significant negative correlation between β-myrcene and 2,6, 6-trimethyl-2-cyclohexene-1,4-dione (p < 0.05).
Changes in aroma composition influence aroma quality (Zheng et al., 2022). The flavour of Ferula is derived from the odour produced by the various volatile compounds. Therefore, volatile compound content influences the aroma characteristics of different species of Ferula. Differences in flavour among different types of Ferula were initially investigated. The combination of solid phase microextraction and SPME-GC-MS has the characteristics of high sensitivity, high accuracy and rapid operation, and is a common means to study volatile aroma compounds in food. In this study, HS-SPME/GC-MS was used to detect the volatile components of four species of Ferula. The ketone compound 1-penten-3-one, which is common among the three edible Ferula species, has a pungent onion-like taste (Li et al., 2017). Sulphur-containing substances generally have a low threshold value and strong odour, presenting a pungent taste similar to that of garlic, onion, and leek. According to the National Standards of the People’s Republic of China, Classification of natural spices (GB/T 21725-2017), Ferula belongs to the spice-type of plants, which is supported by this study. The compounds (Z)-sec-Butyl propenyl disulphide and (E)-sec-butyl propenyl disulfide are present in both F. teterrima and F. sinkiangensis, with concentrations exceeding 1%. They are also detected in F. lehmannii, but not in the non-edible F. feurlaeoides. Previous studies have shown that these sulphur compounds are representative compounds of Ferula (Muturi et al., 2018; Boghrati and Iranshahi, 2019; Sonigra and Meena, 2021) as also been shown that volatile sulphur compounds can be used to determine the type and origin of Ferula (Volatile sulphur compounds: The possible metabolite pattern to identify the sources and types of asafoetida by headspace GC/MS analysis). However, although F. feurlaeoides contains sulphide, the amount is too low to be a major contributor to the flavour. The presence of sulphur imparts a unique flavor to Ferula, thus making it one of the significant distinguishing factors between edible and nonedible Ferula in this study.
A number of studies have shown that terpene compounds are an important part of the volatile oils and volatiles of Ferula (Salehi et al., 2019). Among the 42 potential VOCs screened, monoterpene hydrocarbons and sesquiterpene hydrocarbons have the highest content and the most abundant species. β-pinene, o-Cymene, Sabinene and β-Myrcene are all compounds found in Ferula, accounting for more than 80% of the total alkene compounds. Among them, β-pinene had the highest content among the monoterpene hydrocarbons in the three edible Ferula species, while the other three monoterpene hydrocarbons had the highest content in F. feurlaeoides. These compounds are also highly reported representative compounds of Ferula. Studies have shown that terpene compounds mostly have floral, citrus, and woody aroma (Quan et al., 2023), which contributes to the aroma of Ferula. Alcohols and aldehydes are mostly fresh and grassy compounds, while compounds unique to F. feurlaeoides mostly have floral and fruity aroma. Compounds, such as 1-hexanol and 5-hepten-2-one, 6-methyl, have a sweet citrus odour; these fresh-smelling compounds are significantly more abundant in F. feurlaeoides than in the remaining three species. Therefore, compared with the non-edible F. feurlaeoides, which has a higher proportion of alkene, the many sulphide and ketone compounds in edible species contribute to the rich flavor of Ferula.
As a traditional medicinal part, the roots of Ferula contain significant chemical composition groups. UHPLC-MS/MS offers faster analysis, improved resolution, and higher sensitivity compared to HPLC-MS/MS when used in conjunction with tandem mass spectrometric detection (Rathod et al., 2019). Therefore, we performed metabolite profiling of the roots of edible and non-edible Ferula species by untargeted UHPLC-MS/MS. After multivariate analysis, we identified 62 metabolites that contributed the most to the flavour of the four species main constituents of Ferula. Subsequently, we selected six metabolites as primary characteristic components, including fatty acids, amino acids, organic acids, nucleotides, and terpenoids. These metabolites contribute significantly to the overall flavor and medicinal value of Ferula. Relevant research indicates that Ferula, among numerous spice species within the Apiaceae family, exhibits a higher content of fatty acids. Oleic acid, in particular, emerges as a significant constituent of the fatty acid composition in Ferula (Daga et al., 2022). The oleic acid content was high in all four species of Ferula; however, the oleic acid content of F. feurlaeoides was significantly higher than that of the other three species of Ferula. As a typical monounsaturated fatty acid, oleic acid is easily digested and absorbed by the human body (Zhou et al., 2019). The four species of Ferula included in this study were medicinal plants, and the high fatty acid content reflects the value of Ferula as a traditional medicinal plant. Neochlorogenic acid, a phenolic acid, is an isomer of chlorogenic acids with various pharmacological activities, such as antioxidant, anti-spasmodic, and antiviral effects; it promotes liver glucose utilisation and inhibits carcinogenic mutagenesis. Neochlorogenic acid is used in the pharmaceutical and healthcare industries, chemical industries, and functional food industries (Mehmood et al., 2022). Neochlorogenic acid and oleic acid are important characteristic compounds of F. feurlaeoides and the most abundant fatty acids and organic acids.
Free amino acids are important flavour-forming water-soluble compounds (Pei et al., 2014). The four Ferula species were rich in free amino acid compounds. L-leucine and L-tryptophan were the two major amino acid metabolites. Among them, L-tryptophan was bitter, and the amino acid content of edible Ferula was significantly higher than that of F. feurlaeoides (p < 0.05), indicating that tryptophan significantly contributes to the overall flavour. Furthermore, amino acids, which serve as the cornerstone of a healthy diet, have also been found in abundance in F. assa-foetida L. (Singh et al., 2023). Guanosine is also an important characteristic metabolite in the four species of Ferula. Nucleosides are important metabolites involved in regulatory processes, protection against myocardial damage, and antiviral activity (Du et al., 2015). Natural nucleosides are not only used as biomarkers for the diagnosis of human diseases but also as important markers for the detection of bioactive ingredients during the quality evaluation of Chinese medicinal materials. In addition, nucleotides can have a synergistic effect with amino acids to enhance food flavour (Zhao et al., 2020).
In this study, the roots of the four species of Ferula contained large amounts of olefins such as caryophyllene α-oxide. Moreover, olefins were observed to be abundant in various Ferula species such as F. tunetana Pomel ex Batt (Baccari et al., 2023). Caryophyllene α-oxide, a well-known oxygenated sesquiterpene, exhibits a wide range of protective effects in pharmaceutical, nutritional, and cosmetic applications. Additionally, it possesses various pharmacological and biological activities, including anti-inflammatory, antifungal, analgesic, and anticholinesterase properties (Jassal et al., 2021).These terpenoids exhibit strong antibacterial and anticarcinogenic activities along with antioxidant, anti-asthma, anti-anxiety, and analgesic activities (Chen et al., 2021). Ferula lehmannii, F. sinkiangensis, and F. teterrima not only have edible value but are also more valuable than F. feurlaeoides. Indeed, their medicinal composition is much higher than that of F. feurlaeoides, consistent with traditional cognition. Therefore, the large number of rich amino acids, nucleotides, and alkenes contribute to the good nutritional value and unique flavour of Ferula. These results indicate that the six key metabolites selected in this study have good medicinal value and are representative, consistent with our previous findings (Jiang et al., 2022; Jiang et al., 2023).
According to the characteristic difference in VOCs from the Ferula species acid and the main active components of Ferula, the pathways related to the flavor and medicinal value of different types of Ferula were further studied. We performed KEGG metabolic pathway analysis of the major metabolites of Ferula. The amino acid pathway is the main pathway for the synthesis of flavour-characteristic volatile compounds in the leaves and roots of Ferula. Previous studies have shown that changes in phenylalanine content are positively correlated with aromatic substances. Phenylalanine is a source of phenolic metabolites. In this study, the common characteristic metabolite of the bioactive components, phenylacetaldehyde, was also a key metabolite of phenylalanine metabolism through acylation, methylation, hydroxylation, and other reactions. Ultimately, phenylacetaldehyde affects the synthesis of volatile compounds, such as phenylacetaldehyde in volatiles (Aragüez and Valpuesta Fernández, 2013). Phenylacetaldehyde is a volatile compound abundant in to F. feurlaeoides. Tyrosine, tryptophan, and phenylalanine are involved in protein synthesis and are precursors of many natural products, such as alkaloids and cell wall components. Tryptophan is a precursor of several secondary metabolites such as glucosinolates and alkaloids, which enhance the biosynthesis of aromatic compounds (Miao et al., 2022). In addition to their bioactive composition, the volatiles of edible and nonedible Ferula species differ based on the type of organic acid; however, in terms of bioactive composition, nonedible F. feurlaeoides and edible Ferula have similar biosynthetic pathways and produce similar metabolites. The difference lies in the types of volatile odours and their bioactive content.
Fatty acids are important metabolites in the biosynthesis of aromatic volatile compounds (Zhou et al., 2019). In the fatty acid metabolism pathway, hydroperoxides generated from unsaturated fatty acids catalysed by lipoxygenase are converted into aromatic compounds by hydroperoxidase (Yang et al., 2022). In this study, the fatty acid content of the four species of Ferula was the highest among the various metabolites. Fatty acids were the key metabolite products of Ferula. Five fatty acids were identified in the enrichment pathway; however, 1-hexanol involved in fatty acid biosynthesis was a unique volatile of F. feurlaeoides. These differences in metabolites suggest that the difference between edible and nonedible Ferula also lies in fatty acid content and type. Previous studies have reported that there is a significant synergistic effect between umami amino acids and flavoured nucleotides and that the formation of sulphur-containing compounds is affected by enzymatic reactions with amino acids as precursors (Yu et al., 2020). Common amino acid metabolism aroma precursors, such as L-Leucine and L-Tryptophan, and flavour compounds were obtained via a two-step reaction of transamination and decarboxylation (Jia et al., 2022). Therefore, amino acids, nucleotides, and terpenoids play key roles in VOC formation from different species of Ferula.
This study found that the unique odour of edible Ferula was attributed to the presence of volatile compounds, such as acids, aldehydes, alkenes, and sulphur-containing compounds. In addition, the bioactive components of the three edible Ferula species were significantly associated with pungent odours, whereas those of the nonedible F. feurlaeoides were positively associated with fresh fruity and grassy aromas and negatively associated with pungent volatiles. This answers our second research question that the smell of Ferula is linked to the bioactive ingredients, and the stronger the odour, the higher the content of major bioactive ingredients, such as amino acids and nucleotides. Odour profiling can be used for screening and assessing Ferula quality. The understanding of odour-bioactivity relationship opens doors for novel food, cosmetic, and medicinal product development. In summary, our results demonstrate the relationship between differential metabolites and provides a valuable foundation and potential avenues for further exploration.
The raw/processed data required to reproduce these findings cannot be shared at this time as the data also forms part of an ongoing study, but are available from the corresponding author on reasonable request. Requests to access the datasets should be directed to Li Zhuang, emh1YW5nbGlzaHp1QGZveG1haWwuY29t.
MJ: Writing – original draft, Writing – review & editing, Conceptualization, Data curation, Formal analysis, Investigation, Methodology, Project administration, Software, Visualization. MP: Writing – review & editing. YL: Writing – review & editing. GL: Writing – review & editing. XL: Writing – review & editing. LZ: Funding acquisition, Project administration, Writing – review & editing, Resources.
The author(s) declare financial support was received for the research, authorship, and/or publication of this article. This research was supported by the National Natural Science Foundation of China (31360139, 433 41561010 and 31560177).
Author XL is employed by the company Xinjiang Compass Biotechnology Co., Ltd.
The remaining authors declare that the research was conducted in the absence of any commercial or financial relationships that could be construed as a potential conflict of interest.
All claims expressed in this article are solely those of the authors and do not necessarily represent those of their affiliated organizations, or those of the publisher, the editors and the reviewers. Any product that may be evaluated in this article, or claim that may be made by its manufacturer, is not guaranteed or endorsed by the publisher.
The Supplementary Material for this article can be found online at: https://www.frontiersin.org/articles/10.3389/fpls.2023.1297449/full#supplementary-material
Supplementary Figure 1 | Global distribution of Ferula species. Global distribution information for the genus Ferula was obtained from the Global Biodiversity Information Facility Database (http://www.gbif.org/), and mapped using the ArcGIS 10.8 software.
EMPS, edible and medicinal plants; FE, F. feurlaeoides; GC-MS, gas chromatography mass spectrometry; HPLC, high-performance liquid chromatography; HS-SPME, headspace solid phase microextraction; LE, F. lehmannii; OPLS-DA, orthogonal partial least squares discriminant analysis; PCA, principal component analysis; SI, F. sinkiangensis; TE, F. teterrima; UHPLC-MS/MS, ultra Performance Liquid Chromatography-tandem mass spectrometry; VIP, variable importance in the project; VOCs, volatile organic compounds.
Akaberi, M., Tayarani-Najaran, Z., Mehregan, I., Asili, J., Sahebkar, A., Hassanzadeh-Khayyat, M., et al. (2020). Review of the essential oil composition of Iranian endemic and native taxa of apiaceae (Umbelliferae). Curr. Org. Chem. 24, 909–1009. doi: 10.2174/1385272824999200513103632
Amalraj, A., Gopi, S. (2017). Biological activities and medicinal properties of Asafoetida: A review. J. Tradit. Complement. Med. 7, 347–359. doi: 10.1016/j.jtcme.2016.11.004
Amiri, M. S., Joharchi, M. R. (2016). Ethnobotanical knowledge of Apiaceae family in Iran: A review. Avicenna J. Phytomed. 6, 621–635. doi: 10.22038/ajp.2016.6696
Aragüez, I., Valpuesta Fernández, V. (2013). Metabolic engineering of aroma components in fruits. Biotechnol. J. 8, 1144–1158. doi: 10.1002/biot.201300113
Baccari, W., Saidi, I., Filali, I., Znati, M., Tounsi, M., Ascrizzi, R., et al. (2023). The root essential oil from the Tunisian endemic plant Ferula tunetana: Chemical composition, biological evaluation, molecular docking analysis and drug-likeness prediction. Arab. J. Chem. 19, 105044. doi: 10.1016/j.arabjc.2023.105044
Bahmankar, M., Mortazavian, S. M. M., Tohidfar, M., Sadat Noori, S. A., Izadi Darbandi, A., Al-fekaiki, D. F. (2019). Chemotypes and morpho-physiological characters affecting essential oil yield in Iranian cumin landraces. Ind. Crops Prod. 128, 256–269. doi: 10.1016/j.indcrop.2018.10.080
Boghrati, Z., Iranshahi, M. (2019). Ferula species: A rich source of antimicrobial compounds. J. Herb. Med. 16, 100244. doi: 10.1016/j.hermed.2018.10.009
Boncan, D. A. T., Tsang, S. S. K., Li, C., Lee, I. H. T., Lam, H. M., Chan, T. F., et al. (2020). Terpenes and terpenoids in plants: Interactions with environment and insects. Int. J. Mol. Sci. 21, 7382. doi: 10.3390/ijms21197382
Charmforoshan, E., Karimi, E., Oskoueian, E., Es-Haghi, A., Iranshahi, M. (2019). Inhibition of human breast cancer cells (MCF-7 cell line) growth via cell proliferation, migration, and angiogenesis by auraptene of Ferula szowitsiana root extract. J. Food Meas. Charact. 13, 2644–2653. doi: 10.1007/s11694-019-00185-6
Chen, F., Miao, X., Lin, Z., Xiu, Y., Shi, L., Zhang, Q., et al. (2021). Disruption of metabolic function and redox homeostasis as antibacterial mechanism of Lindera glauca fruit essential oil against Shigella flexneri. Food Control 130, 108282. doi: 10.1016/j.foodcont.2021.108282
Chen, Z., Zhang, C., Gao, F., Fu, Q., Fu, C., He, Y., et al. (2018). A systematic review on the rhizome of Ligusticum chuanxiong Hort. (Chuanxiong). Food Chem. Toxicol. 119, 309–325. doi: 10.1016/j.fct.2018.02.050
Cheng, Z., Zhang, Q., Long, C. (2022). Research status of ethnobotany, (2017–2022). Biodivers. Sci. 30, 22372. doi: 10.17520/biods.2022372
Daga, P., Vaishnav, S. R., Dalmia, A., Tumaney, A. W. (2022). Extraction, fatty acid profile, phytochemical composition and antioxidant activities of fixed oils from spices belonging to Apiaceae and Lamiaceae family. J. Food Sci. Technol. 59, 518–553. doi: 10.1007/s13197-021-05036-1
Du, L. Y., Qian, D. W., Jiang, S., Shang, E. X., Guo, J. M., Liu, P., et al. (2015). Comparative characterization of nucleotides, nucleosides and nucleobases in Abelmoschus manihot roots, stems, leaves and flowers during different growth periods by UPLC-TQ-MS/MS. J. Chromatogr. B Anal. Technol. Biomed. Life Sci. 1006, 130–137. doi: 10.1016/j.jchromb.2015.10.021
Feng, L., Lin, Y., Cai, Y., Wei, W., Yang, J., Zhan, R., et al. (2023). Terpenoid VOC profiles and functional characterization of terpene synthases in diploid and tetraploid cytotypes of Chrysanthemum indicum L. Plant Physiol. Biochem. 200, 107766. doi: 10.1016/j.plaphy.2023.107766
He, J., Wang, J., Qi, G., Yao, L., Li, X., Paek, K. Y., et al. (2022). Comparison of polysaccharides in ginseng root cultures and cultivated ginseng and establishment of high-content uronic acid plant synthesis system. Ind. Crops Prod. 186, 115155. doi: 10.1016/j.indcrop.2022.115155
Jassal, K., Kaushal, S., Rashmi, Rani, R. (2021). Antifungal potential of guava (Psidium guajava) leaves essential oil, major compounds: beta-caryophyllene and caryophyllene oxide. Arch. Phytopathol. Plant Prot. 54, 19–20. doi: 10.1080/03235408.2021.1968287
Jia, Z., Wang, Y., Wang, L., Zheng, Y., Jin, P. (2022). Amino acid metabolomic analysis involved in flavor quality and cold tolerance in peach fruit treated with exogenous glycine betaine. Food Res. Int. 157, 111204. doi: 10.1016/j.foodres.2022.111204
Jiang, M., Lan, S., Peng, M., Wang, Z., Zhuang, L. (2023). The diversity of Ferula species and environmental factors on metabolite composition using untargeted metabolomics. Food Biosci. 56, 103075. doi: 10.1016/j.fbio.2023.103075
Jiang, M., Lan, S., Wang, Z., Pu, X., Zhuang, L. (2022). Analysis of the volatile components in different parts of three Ferula species via combined DHSA-GC-MS and multivariate statistical analysis. LWT - Food Sci. Technol. 167, 113846. doi: 10.1016/j.lwt.2022.113846
Kamelan Kafi, M., Bolvari, N. E., Mohammad Pour, S., Moghadam, S. K., Shafaei, N., Karimi, E., et al. (2022). Encapsulated phenolic compounds from Ferula gummosa leaf: A potential phytobiotic against Campylobacter jejuni infection. J. Food Process. Preserv. 46, e16802. doi: 10.1111/jfpp.16802
Khayat, M. T., Alharbi, M., Ghazawi, K. F., Mohamed, G. A., Ibrahim, S. R. M. (2023). Ferula sinkiangensis (Chou-AWei, chinese ferula): traditional uses, phytoconstituents, biosynthesis, and pharmacological activities. Plants 12, 902. doi: 10.3390/plants12040902
Li, M., Hou, X., Wang, F., Tan, G., Xu, Z., Xiong, A. (2018). Advances in the research of celery, an important Apiaceae vegetable crop. Crit. Rev. Biotechnol. 38, 172–183. doi: 10.1080/07388551.2017.1312275
Li, N., Wang, H.-Y., Zhao, F., Liu, C.-J. (2017). Effects of sulfur compounds of toona sinensis seedlings on the aroma compounds. Food Res. Dev. 38, 35–39. doi: 10.3969/j.issn.1005-6521.2017.13.008
Mehmood, A., Li, J., Rehman, A. U., Kobun, R., Llah, I. U., Khan, I., et al. (2022). Xanthine oxidase inhibitory study of eight structurally diverse phenolic compounds. Front. Nutr. 9. doi: 10.3389/fnut.2022.966557
Mei, X., Wang, S., Zhang, L., Gu, S., Wei, L., Li, J., et al. (2022). Widely targeted metabolomics analysis revealed components change regularity of Salvia miltiorrhiza Bunge after post-harvest drying under different temperature. Ind. Crops Prod. 188, 115638. doi: 10.1016/j.indcrop.2022.115638
Miao, Y., Tan, C., Peng, C., Gong, J. (2022). Metabolomic analysis of the characteristic components of coffea arabica L.Based on UHPLC-QE-MS. J. Chin. Inst. Food Sci. Technol. 22, 355–367. doi: 10.16429/j.1009-7848.2022.11.037
Mohammadhosseini, M., Venditti, A., Sarker, S. D., Nahar, L., Akbarzadeh, A. (2019). The genus Ferula: Ethnobotany, phytochemistry and bioactivities - A review. Ind. Crops Prod. 129, 350–394. doi: 10.1016/j.indcrop.2018.12.012
Muturi, E. J., Ramirez, J. L., Zilkowski, B., Flor-Weiler, L. B., Rooney, A. P. (2018). Ovicidal and larvicidal effects of garlic and Asafoetida essential oils against West Nile virus vectors. J. Insect Sci. 18, 43. doi: 10.1093/jisesa/iey036
Pei, F., Shi, Y., Gao, X., Wu, F., Mariga, A. M., Yang, W., et al. (2014). Changes in non-volatile taste components of button mushroom (Agaricus bisporus) during different stages of freeze drying and freeze drying combined with microwave vacuum drying. Food Chem. 165, 547–554. doi: 10.1016/j.foodchem.2014.05.130
Quan, W., Jin, J., Qian, C., Li, C., Zhou, H. (2023). Characterization of volatiles in flowers from four Rosa chinensis cultivars by HS-SPME-GC × GC-QTOFMS. Front. Plant Sci. 14. doi: 10.3389/fpls.2023.1060747
Radulović, N. S., Zlatković, D. B., Randjelović, P. J., Stojanović, N. M., Novaković, S. B., Akhlaghi, H. (2013). Chemistry of spices: Bornyl 4-methoxybenzoate from Ferula ovina (Boiss.) Boiss. (Apiaceae) induces hyperalgesia in mice. Food Funct. 4, 1751–1758. doi: 10.1039/c3fo60319a
Rahman, I. U., Afzal, A., Iqbal, Z., Ijaz, F., Ali, N., Shah, M., et al. (2019). Historical perspectives of ethnobotany. Clin. Dermatol. 37, 382–388. doi: 10.1016/j.clindermatol.2018.03.018
Rathod, R. H., Chaudhari, S. R., Patil, A. S., Shirkhedkar, A. A. (2019). Ultra-high performance liquid chromatography-MS/MS (UHPLC-MS/MS) in practice: analysis of drugs and pharmaceutical formulations. Futur. J. Pharm. Sci. 5, 6. doi: 10.1186/s43094-019-0007-8
Ricciardi, V., Marcianò, D., Sargolzaei, M., Maddalena, G., Maghradze, D., Tirelli, A., et al. (2021). From plant resistance response to the discovery of antimicrobial compounds: The role of volatile organic compounds (VOCs) in grapevine downy mildew infection. Plant Physiol. Biochem. 160, 294–305. doi: 10.1016/j.plaphy.2021.01.035
Salehi, M., Naghavi, M. R., Bahmankar, M. (2019). A review of Ferula species: Biochemical characteristics, pharmaceutical and industrial applications, and suggestions for biotechnologists. Ind. Crops Prod. 139, 111511. doi: 10.1016/j.indcrop.2019.111511
Scavarda, C., Cordero, C., Strocchi, G., Bortolini, C., Bicchi, C., Liberto, E., et al (2021). Cocoa smoky off-flavour: A MS-based analytical decision maker for routine controls. Food Chem. 336, 127691. doi: 10.1016/j.foodchem.2020.127691
Singh, P. P., Joshi, R., Kumar, R., Kumar, A., Sharma, U. (2023). Comparative phytochemical analysis of Ferula assa-foetida with Ferula jaeschkeana and commercial oleo-gum resins using GC–MS and UHPLC-PDA-QTOF-IMS. Food Res. Int. 164, 112434. doi: 10.1016/j.foodres.2022.112434
Sonigra, P., Meena, M. (2021). Metabolic profile, bioactivities, and variations in the chemical constituents of essential oils of the Ferula genus (Apiaceae). Front. Pharmacol. 11. doi: 10.3389/fphar.2020.608649
Tan, W., Pan, T., Wang, S., Li, P., Men, Y., Tan, R., et al. (2022). Immunometabolism modulation, a new trick of edible and medicinal plants in cancer treatment. Food Chem. 376, 131860. doi: 10.1016/j.foodchem.2021.131860
Utpott, M., Rodrigues, E., Rios, A., de, O., Mercali, G. D., Flôres, S. H. (2022). Metabolomics: An analytical technique for food processing evaluation. Food Chem. 366, 130685. doi: 10.1016/j.foodchem.2021.130685
Vasilev, N., Boccard, J., Lang, G., Grömping, U., Fischer, R., Goepfert, S., et al. (2016). Structured plant metabolomics for the simultaneous exploration of multiple factors. Sci. Rep. 6, 37390. doi: 10.1038/srep37390
Wang, M., Li, X., Ding, H., Chen, H., Liu, Y., Wang, F., et al. (2023). Comparison of the volatile organic compounds in Citrus reticulata ‘Chachi’ peel with different drying methods using E-nose, GC-IMS and HS-SPME-GC-MS. Front. Plant Sci. 14. doi: 10.3389/fpls.2023.1169321
Want, E. J., Masson, P., Michopoulos, F., Wilson, I. D., Theodoridis, G., Plumb, R. S., et al. (2013). Global metabolic profiling of animal and human tissues via UPLC-MS. Nat. Protoc. 8, 17–32. doi: 10.1038/nprot.2012.135
Wei, J., Gao, Y. Z., Zhou, J., Liu, Z. W. (2019). Collection and sorting of medicinal plants in Chinese Apiaceae(Umbelliferae). China Journal of Chinese Materia Medica 44, 5329–5335. doi: 10.19540/j.cnki.cjcmm.20191101.103
Xiao, W. J., Guo, F. X., Chen, Y., Liu, L. L., Chen, Y. Z., Jiao, X. S., et al. (2021). Effect of organic fertilizer application on the medicinal character, yield and disease resistance of Angelica sinensis. Acta Prataculturae Sin. 30, 189–199. doi: 10.11686/cyxb2020166
Yang, J., Li, H., Lin, Y., Liu, W., Wei, C. (2022). Transcriptome analysis of two flaxseed cultivars grown in xinjiang and mining of differentially expressed genes related to flaxseed oil aroma. Food Sci. 43, 70–76. doi: 10.7506/spkx1002-6630-20201120-213
Yu, J., Wen, R., Yan, Q., Chen, Q., Qin, L. (2020). Bioactive constituents of allium and their physiological functions: A review. Food Sci. 41, 255–265. doi: 10.7506/spkx1002-6630-20190225-170
Zelena, E., Dunn, W. B., Broadhurst, D., Francis-McIntyre, S., Carroll, K. M., Begley, P., et al. (2009). Development of a robust and repeatable UPLC - MS method for the long-term metabolomic study of human serum. Anal. Chem. 81, 1357–1364. doi: 10.1021/ac8019366
Zhang, S. S., Guo, S., Zheng, Z. J., Liu, S. J., Hou, Y F.., Ho, C. T. (2021). Characterization of volatiles in Allium tenuissimum L. flower by headspace-gas chromatography-olfactometry-mass spectrometry, odor activity values, and the omission and recombination experiments. LWT 151, 112144. doi: 10.1016/j.lwt.2021.112144
Zhao, S., Zhou, N., Yang, L., Wei, Z. (2020). Determination of 10 nucleoside compositions in Fritillaria thunbergii miq. from different Regions. Chin. Wild Plant Resources 39, 35–40. doi: 10.3969/j.issn.1006-9690.2020.10.006
Zheng, Z., Zhang, X., Fu, F., Sun, X., Pan, T., Guo, Z., et al. (2022). Study on aroma components of canarium album of different cultivars based on HS-SPME-GC-MS. Sci. Technol. Food Ind. 43, 282–290. doi: 10.13386/j.issn1002-0306.2022020244
Keywords: edible and medicinal plants (EMPs), genus Ferula, HS-SPME-GC-MS, volatile organic compounds (VOCs), bioactive component, non-targeted metabolomics
Citation: Jiang M, Peng M, Li Y, Li G, Li X and Zhuang L (2024) Quality evaluation of four Ferula plants and identification of their key volatiles based on non-targeted metabolomics. Front. Plant Sci. 14:1297449. doi: 10.3389/fpls.2023.1297449
Received: 20 September 2023; Accepted: 08 December 2023;
Published: 04 January 2024.
Edited by:
Zhentian Lei, University of Missouri, United StatesReviewed by:
Majid Mohammadhosseini, Islamic Azad University, IranCopyright © 2024 Jiang, Peng, Li, Li, Li and Zhuang. This is an open-access article distributed under the terms of the Creative Commons Attribution License (CC BY). The use, distribution or reproduction in other forums is permitted, provided the original author(s) and the copyright owner(s) are credited and that the original publication in this journal is cited, in accordance with accepted academic practice. No use, distribution or reproduction is permitted which does not comply with these terms.
*Correspondence: Li Zhuang, emh1YW5nbGlzaHp1QGZveG1haWwuY29t
Disclaimer: All claims expressed in this article are solely those of the authors and do not necessarily represent those of their affiliated organizations, or those of the publisher, the editors and the reviewers. Any product that may be evaluated in this article or claim that may be made by its manufacturer is not guaranteed or endorsed by the publisher.
Research integrity at Frontiers
Learn more about the work of our research integrity team to safeguard the quality of each article we publish.