- 1Key Laboratory of Seed Innovation, Institute of Genetics and Developmental Biology, Chinese Academy of Sciences, Beijing, China
- 2College of Advanced Agricultural Sciences, University of Chinese Academy of Sciences, Beijing, China
- 3CAS−JIC Centre of Excellence for Plant and Microbial Science (CEPAMS), Institute of Genetics and Developmental Biology, Chinese Academy of Sciences, Beijing, China
Legumes and actinorhizal plants establish symbiotic relationships with nitrogen-fixing bacteria, resulting in the formation of nodules. Nodules create an ideal environment for nitrogenase to convert atmospheric nitrogen into biological available ammonia. NODULE INCEPTION (NIN) is an indispensable transcription factor for all aspects of nodule symbiosis. Moreover, NIN is consistently lost in non-nodulating species over evolutions. Here we focus on recent advances in the signaling mechanisms of NIN during nodulation and discuss the role of NIN in the evolution of nitrogen-fixing nodule symbiosis.
Introduction
Nitrogen is an indispensable nutrient for plant growth. The earth’s atmosphere contains approximately 78% nitrogen. However, atmospheric nitrogen (N2) cannot be directly utilized by most plants. Only some plant species from Fabales, Fagales, Cucurbitales, and Rosales (FaFaCuRo) clades exhibit the ability to establish symbiotic interactions with soil nitrogen-fixing bacteria, which are referred to rhizobia or Frankia (Soltis et al., 1995; Kistner and Parniske, 2002). This mutualistic symbiosis occurs within specialized structures known as nodules. Rhizobia in nodules utilize the catalytic activity of nitrogenase to convert atmospheric dinitrogen into ammonium, which serves as a nitrogen source for the host plant. In return, the host plant reciprocates by providing rhizobia with carbon sources (Santi et al., 2013; Stambulska and Bayliak, 2019). The establishment of symbiotic interactions requires communications and signal processing between host plants and bacterial partners. These molecular dialogues have been extensively studied in legumes, with a particular focus on model plants such as Medicago truncatula and Lotus japonicus (Roy et al., 2020; Wang et al., 2022).
Initially, legumes produce and release flavonoid compounds into the rhizosphere in nitrogen-deficient soils, serving as a signal to attract and stimulate rhizobia to produce oligosaccharide, known as Nod factors, thereby initiating a dialogue with the host plant (Zipfel and Oldroyd, 2017). Subsequently, Nod factors are recognized by specific types of receptor kinases, including LjNFR1 (NOD FACTOR RECEPTOR)/MtLYK3 (LysM RECEPTOR KINASE 3), LjNFR5/MtNFP (NOD FACTOR PERCEPTION), and LjSYMRK (SYMBIOTIC RECEPTOR LIKE KINASE)/MtDMI2 (DOSE NOT MAKE INFECTION), which form homomeric and heteromeric complexes on the plasma membrane of root hair cells, triggering the nitrogen-fixing symbiotic signaling pathway (Endre et al., 2002; Stracke et al., 2002; Limpens et al., 2003; Madsen et al., 2003; Radutoiu et al., 2003; Arrighi et al., 2006; Smit et al., 2007; Broghammer et al., 2012; Moling et al., 2014). The perception of Nod factors by receptor kinases located on the membrane transmits the signal to the cell interior, leading to periodic fluctuations in the calcium concentration, referred as calcium spiking, in the nuclei of epidermal root hair cells, which depends on the collaboration of nuclear membranes-localized calcium channel proteins LjPOLLUX/MtDMI1, LjCASTOR, MtCNGC15 (CYCLIC NUCLEOTIDE GATED CHANNEL) and MCA8 (M. truncatula calcium ATPase) (Ané et al., 2004; Imaizumi-Anraku et al., 2005; Charpentier et al., 2008; Capoen et al., 2011; Charpentier et al., 2016). The occurrence of nuclear calcium spiking serves as a hallmark event for the activation of the symbiotic signaling pathway (Oldroyd and Downie, 2004). Following that, a calcium and calmodulin-dependent serine/threonine protein kinase, LjCCaMK/MtDMI3, is activated upon to decode the calcium signals, resulting in the phosphorylation of transcription factor LjCYCLOPS/MtIPD3 (INTERACTING PROTEIN OF DMI3) (Lévy et al., 2004; Yano et al., 2008; Singh and Parniske, 2012; Miller et al., 2013; Yuan et al., 2022). Activated LjCYCLOPS/MtIPD3 forms a transcriptional complex with NSP1, NSP2 (NODULATION SIGNALING PATHWAY) and DELLA proteins. Then, this complex promotes the expression of key transcription factor NIN, thus initiating NIN-regulated transcriptional network (Singh et al., 2014; Jin et al., 2016). Notably, NIN is one of the earliest-activated transcription factors downstream of common symbiotic signaling pathway (Schiessl et al., 2019).
The transcription factor NIN belongs to a plant-specific RWP-RK protein family. NIN controls all aspects of symbiotic nodulation in legumes: rhizobial infection, nodule organogenesis, transition to nitrogen fixation, and regulation of nodule number in legumes and actinorhizal plants (Figure 1) (Schauser et al., 1999; Borisov et al., 2003; Marsh et al., 2007; Soyano et al., 2013; Soyano et al., 2014; Clavijo et al., 2015; Liu C.W. et al., 2019; Liu J. et al., 2019b; Bu et al., 2020; Feng et al., 2021). Moreover, NIN gene is consistently lost or unfunctional in some non-nodulating species of FaFaCuRo clades, suggesting that the nitrogen-fixing ability of plants may associate with functional NIN protein (Griesmann et al., 2018; van Velzen et al., 2018; Zhang et al., 2023). In this review, we focus on recent advances in understanding the regulatory mechanisms of NIN in nodule symbiosis and discuss the evolution of NIN function in nitrogen-fixing nodule (NFN) symbiosis.
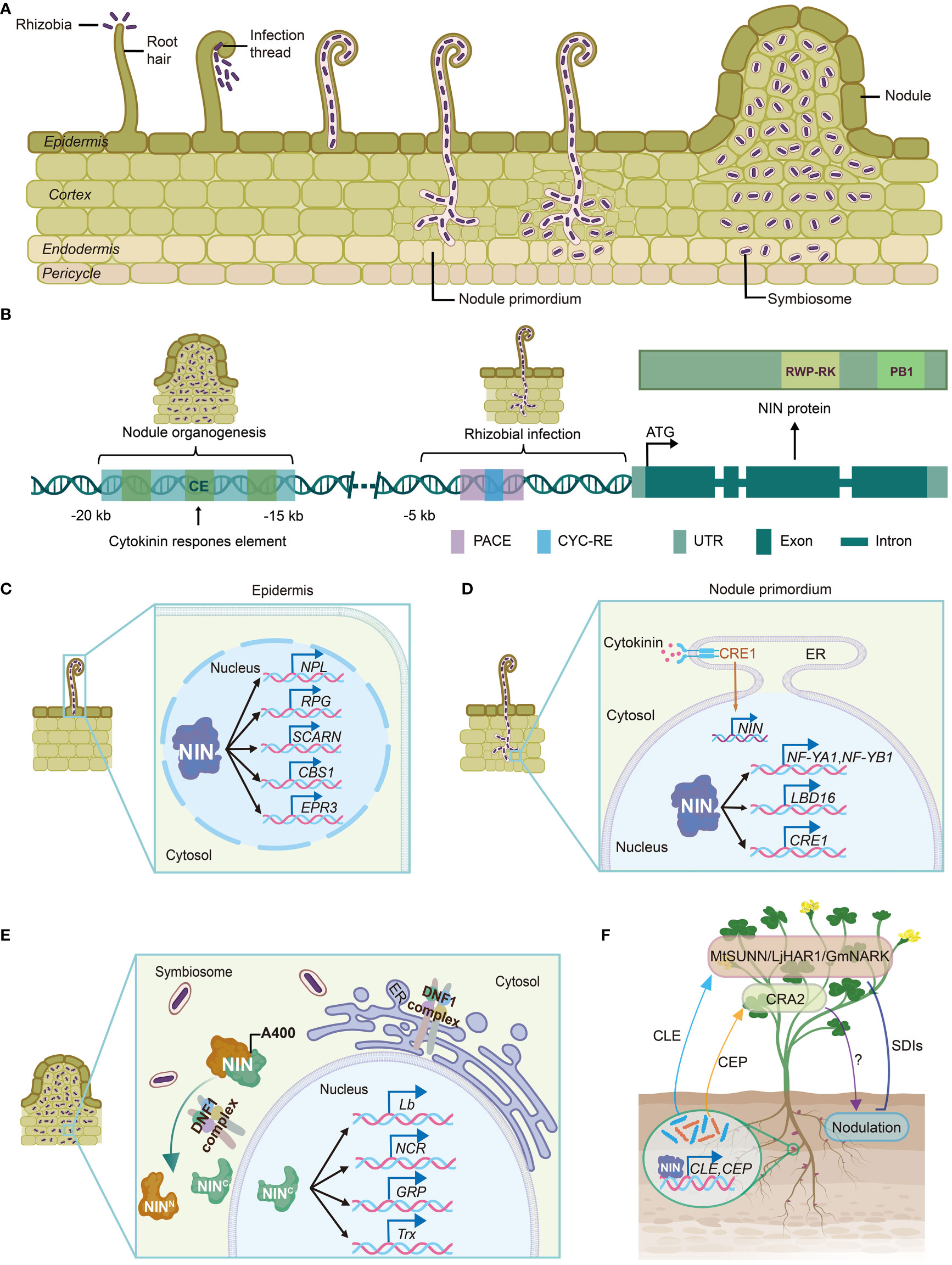
Figure 1 The transcription factor NIN plays essential roles in nitrogen-fixing nodulation. (A) Rhizobial infection and nodule organogenesis. Rhizobia enter the host plant through root hairs, which trap the bacteria inside. Afterwards, infection threads are formed and permit invasion of the rhizobia into inner root tissues. Nodule meristem initiates below the infection site in the cortex. The epidermal and cortical processes are coordinated to allow successful intracellular accommodation of rhizobia. Then infection threads then release membrane-bound rhizobia cells into nodule, where the bacteria differentiate and transit into nitrogen-fixing state. (B) NIN promoter harbors several cis-elements crucial for both rhizobial infection and nodule organogenesis. The CYC-RE and PACE elements in LjNIN promoter are required for infection thread development. The green boxes indicate three conserved regions identified in MtNIN promoter. The CE region in MtNIN promoter is essential for nodule organogenesis. (C) NIN facilitates rhizobial infection by regulating expression of infection-associated genes, such as NPL, RPG, SCARN, CBS1 and EPR3. NPL encodes a pectate lyase enzyme involved in cell wall restructuring during rhizobia invasion. RPG is a critical determinant for the formation of infectosome, which is a protein complex essential for infection thread development. SCARN encodes a nodulation-specific component of the SCAR/WAVE complex. CBS1 contains a cystathionine-β-synthase (CBS) domain and a domain of unknown function. EPR3 is a LysM receptor that recognizes exopolysaccharides on the surface of rhizobia, promoting infection thread initiation. (D) NIN is essential for nodule organogenesis. NIN drives the expression of NF-YA1 and NF-YB1, inducing cortical cell division and nodule primordium formation. NIN also controls the expression of LBD16, a key transcription factor involved in lateral root development, which has been hijacked to coordinate nodule development. Additionally, activated cytokinin receptor CRE1 promotes NIN expression in nodule primordium. Then, cortical NIN proteins activate CRE1 expression, forming a positive feedback loop. (E) NIN determines the cellular state transition to nitrogen fixation. The DNF1-complex mediates the processing of NIN protein at A400, generating a C-terminal NIN fragment, which specifically activates a suit of genes involved in symbiosome development and nitrogen fixation, such as Lb, NCR, GRP, and Trx. (F) NIN controls AON signaling. NIN activates the expression of CLE and CEP peptides in root, which are subsequently transported to shoot. After perceived by MtSUNN/LjHAR1/GmNARK receptors, the CLE peptides activate the production of SDIs, which move back to root and suppress further nodulation. CEP peptides are recognized by shoot receptor CRA2, resulting in promotion of nodulation. Created with medpeer.cn. NIN, NODULE INCEPTION; CYC-RE, CYCLOPS-responsive element; PACE, Predisposition-Associated Cis-regulatory Element; CE, cytokinin response element-containing region; NPL, NODULATION PECTATE LYASE; RPG, RHIZOBIUM-DIRECTED POLAR GROWTH; EPR3, EXOPOLYSACCHARIDE RECEPTOR 3; ER, endoplasmic reticulum; NF-Y, NUCLEAR FACTOR-Y SUBUNIT; LBD16, LATERAL ORGAN BOUNDARIES DOMAIN 16; CRE1, CYTOKININ RESPONSE 1; DNF1, Defective in Nitrogen Fixation 1; Lb, leghemoglobin; NCR, nodule specific cysteine-rich; GRP, glycine-rich peptide; Trx, thioredoxin; AON, autoregulation of nodulation; CLE, CLAVATA3/EMBRYO SURROUNDING REGION; CEP, C-terminally Encoded Peptide; SUNN, SUPERNUMERARY NODULES; HAR1, HYPERNODULATION AND ABERRANT ROOT; NARK, NODULE AUTOREGULATION RECEPTOR KINASE; SDI, shoot-derived inhibitor; CRA2, COMPACT ROOT ARCHITECTURE2.
The transcription factor NIN: structure and function
The transcription factor NIN was initially identified in L. japonicus by forward genetic screening (Schauser et al., 1999). nin mutations block the rhizobial entry at an early stage (Table 1) (Schauser et al., 1999; Borisov et al., 2003; Marsh et al., 2007; Feng et al., 2021). CYCLOPS activates NIN expression by binding to the CYCLOPS-responsive elements (CYC-RE or PACE) in the NIN promoter (Singh et al., 2014; Cathebras et al., 2022). The CYC-RE in NIN promoter is conserved in legumes (Liu C. W. et al., 2019). It was recently reported that a putative CYC-RE was also present in the promoter of one poplar NIN ortholog (Irving et al., 2022), suggesting that this element may be recruited before the origin of nodulation. Both CYC-RE and PACE are critical for NIN function during infection thread development (Figure 1B) (Liu J. et al., 2019; Akamatsu et al., 2022; Cathebras et al., 2022). Additionally, another cis-element CE (cytokinin response element-containing region) was reported to be essential for nodule organogenesis and NIN expression in the pericycle (Figure 1B) (Liu J. et al., 2019). The CE is not present outside nodulated legumes (Liu and Bisseling, 2020; Zhang et al., 2023), suggesting that original recruitment of NIN into nodulation may occur in CYC-RE, whereas CE-dependent induction of NIN in inner root cell layers may evolve later.
NIN protein is characterized by a conserved 60-amino acid-long sequence containing an RWPxRK motif, which exhibits conservation with the MID (minus dominance) protein, the first identified member possessing this motif (Ferris and Goodenough, 1997). The conserved RWPxRK motif was subsequently designated as the RWP-RK domain and categorized as a novel class of transcription factors (Schauser et al., 1999). Structure predictions and a series of protein-DNA binding assays demonstrate the DNA-binding capability of RWP-RK domain, allowing NIN to interact with specific DNA sequences located within the promoters of target genes. These NIN-regulated genes include early nodulation genes such as NF-YA1 and NF-YB1 (NUCLEAR FACTOR-Y SUBUNIT A1), NPL (NODULATION PECTATE LYASE), CRE1 (CYTOKININ RESPONSE 1), ASYMMETRIC LEAVES 2-LIKE 18/LATERAL ORGAN BOUNDARIES DOMAIN 16 (ASL18/LBD16), and late nodulation-associated genes such as leghemoglobins, thioredoxins, nodule-specific cysteine-rich (NCR) peptides and glycine-rich peptides (Figures 1C–F) (Xie et al., 2012; Soyano et al., 2013; Vernié et al., 2015; Soyano et al., 2019; Feng et al., 2021).
Another remarkable characteristic of NIN protein is the presence of PB1 (Phox and Bem1) domain at its C-terminal end, which mediates protein-protein interactions, allowing NIN to form dimers or oligomers (Figure 1B) (Sumimoto et al., 2007; Feng et al., 2021). NIN-like proteins (NLPs), named after its homology to NIN protein, share the RWP-RK and PB1 domains with NIN, but not its N-terminal nitrate binding domain (Liu et al., 2022). NLPs have been characterized as key regulators of nitrate signaling in land plants (Castaings et al., 2009; Konishi and Yanagisawa, 2013; Marchive et al., 2013; Chardin et al., 2014; Liu et al., 2017; Alvarez et al., 2020; Liu et al., 2022). Interestingly, M. truncatula NLP1 and L. japonicus NLP1/4 are required for the repression of nodulation by nitrate (Lin et al., 2018; Nishida et al., 2018; Nishida et al., 2021). MtNLP1 interacts with NIN through the PB1 domain, leading to suppression of NIN-activated CRE1 expression (Lin et al., 2018). Adaptations in NIN promoter and functional changes to NIN protein enable its specific functions in NFN symbiosis.
NIN facilitates intracellular rhizobial infection
The infection thread is crucial for rhizobia invasion into host plant during nodulation. Upon recognition of Nod factors released by rhizobia, root hairs of host plant undergo curling, enclosing the rhizobia attached to the surface of root hairs (Esseling et al., 2003). Subsequently, cell wall surrounding the enclosed rhizobia is locally degraded, and the cytoskeleton in root hair undergoes rearrangement, resulting in the invagination of cell membrane and formation of a tubular structure known as infection thread. Rhizobia gain entry into plant cells through infection threads, extending to the base of the root hair and subsequently penetrating the developing nodule primordia, which arise from differentiated cells composed of cortical cells, endodermis and pericycle (Figure 1A) (Oldroyd, 2013).
NIN is among the earliest-responding genes to rhizobia inoculation, suggesting its involvement in the initiation of bacterial infection, except rhizobial crack-entry infection in peanut (Arachis hypogaea) (Schiessl et al., 2019; Mergaert et al., 2020; Bhattacharjee et al., 2022). NIN loss of function leads to widespread defects in gene expression, highlighting its pivotal role in the gene regulatory network governing rhizobia infection (Liu C.W. et al., 2019). Notably, NIN controls many early genes associated with nodulation (Figure 1C). Among them, NPL encodes a pectate lyase enzyme involved in cell wall restructuring during rhizobia invasion (Xie et al., 2012). RPG (RHIZOBIUM-DIRECTED POLAR GROWTH) is a critical determinant for the formation of an exocyst complex (termed as infectosome) during bacterial infection (Arrighi et al., 2008; Lace et al., 2023). NIN directly binds to the RPG promoter and induce its expression (Li et al., 2023). SCARN (SCAR-Nodulation), a gene responsible for actin rearrangement during rhizobia infection, is induced by rhizobia in epidermal cells and directly regulated by NIN (Qiu et al., 2015). In addition, the absence of the membrane-localized protein CBS1 (cystathionine-β-synthase-like 1) results in the formation of an elevated number of microcolonies, whose expression is dependent on NIN (Sinharoy et al., 2016). NIN also promotes the expression of EPR3 (Exopolysaccharide Receptor 3) in L. japonicus, a LysM receptor gene responsible for sensing rhizobia exopolysaccharides and facilitating the entry of rhizobia into host cells (Kawaharada et al., 2015; Kawaharada et al., 2017). These extensive regulations by NIN underscore its central role in orchestrating the early responses to rhizobia invasion in legumes.
NIN is essential for nodule organogenesis
As root hairs curl and entrap compatible rhizobia, cell divisions in the cortex, endodermis and pericycle are induced, triggering the formation of nodule primordia (Xiao et al., 2014). After successful invasion of legume plants by rhizobia, the initiation of root nodule organogenesis occurs in a coordinated manner to ensure accurate intrusion of infection thread into developing nodule primordium (Figure 1A) (Oldroyd and Downie, 2004). Mutants of NF-YA1 and NF-YB1, members of nuclear factor-Y (NF-Y) transcription factor family, show abnormal infection thread development, delayed nodule formation, and smaller nodules, demonstrating their important roles in root nodule development (Laporte et al., 2013; Soyano et al., 2013). Further studies show that NIN directly binds to the promoter regions of LjNF-YA1 and LjNF-YB1 genes, promoting their expression (Figure 1D). Overexpression of LjNIN and LjNF-YA1 genes induces cell division in the root cortex, resulting in the formation of nodule-like structures (Soyano et al., 2013). ASL18/LBD16 is a key transcription factor that regulates lateral root development by activating expression of auxin synthesis-related genes, thus promoting auxin biosynthesis and influencing lateral root growth (Shahan and Benfey, 2020). Interestingly, the developmental program controlled by ASL18/LBD16 in lateral roots appears to be involved in root nodule organogenesis as well. NIN recruits the core developmental program of lateral roots to facilitate root nodule formation by promoting the expression of ASL18/LBD16 (Figure 1D) (Schiessl et al., 2019; Soyano et al., 2019). These findings demonstrate that LBD16 and NF-Y transcription factors act downstream of NIN and work cooperatively to regulate root nodule development (Bishopp and Bennett, 2019).
Phytohormone cytokinin plays key roles in regulating various aspects of plant growth and development. Exogenous application of cytokinin has been shown to induce formation of nodule-like structures in leguminous plants (Gauthier-Coles et al., 2018). In L. japonicus, gain-of-function mutants snf2 and snf5 (spontaneous nodule formation) of the cytokinin receptor gene LHK1 (LOTUS HISTIDINE KINASE1) exhibit a spontaneous nodule phenotype in the absence of rhizobia (Tirichine et al., 2007; Liu et al., 2018). Similarly, overexpression of gain-of-function CRE1 mutant, a homolog of LHK1 in M. truncatula, also induces rhizobia-free nodule formation (Jin et al., 2016). These results demonstrate the essential role of cytokinin signaling in nodule formation. Interestingly, the NIN gene is up-regulated by cytokinin treatment or snf2 mutation. And spontaneous nodule formation in snf2 mutants appears a NIN-dependent manner (Tirichine et al., 2007). Consistently, a distal element in NIN promoter, containing putative cytokinin B-type response regulator binding sites, is responsible for cytokinin-induced NIN expression, which is necessary for nodule organogenesis (Figure 1B) (Liu J. et al., 2019b). Furthermore, NIN protein is sufficient to activate the expression of CRE1, forming a positive feedback regulatory loop that promotes nodule development (Figure 1D) (Vernié et al., 2015). Alongside this, cytokinin and NIN-overexpression induced cortical cell divisions are dependent on the GRAS proteins MtSHR (SHORTROOT) and MtSCR (SCARECROW) (Dong et al., 2021). Together, these findings reveal the crucial role of NIN-dependent regulatory network governing root nodule formation in leguminous plants.
NIN determines the transition to nitrogen fixation
Symbiotic nitrogen fixation requires a low-oxygen environment for proper activity of nitrogenase (Kondorosi et al., 2013). However, the mechanism controlling the transition to nitrogen fixation remains elusive for years. While NIN has been extensively studied for its role in various aspects of nodule initiation and development, recent research reveals that NIN also regulates the transition of nodule cells into nitrogen-fixing state. Nodulation activated signal peptidase complex (SPC) mediates the processing of NIN protein, resulting in production of a C-terminal NIN fragment containing the DNA binding domain. The processed C-terminal product of NIN specifically activates a suite of genes associated with symbiosome development and nitrogen fixation [such as genes encoding leghemoglobins, nodule specific cysteine-rich (NCR) peptides and thioredoxins], thereby controlling the cell state transition (Figure 1E) (Feng et al., 2021). In addition, NIN and its close homolog NLP2 directly promote the expression of leghemoglobins, which buffer the oxygen concentration within nodules (Jiang et al., 2021). These findings demonstrate the important roles of NIN and NLP2 in creating suitable environment for nitrogen fixation.
NIN controls autoregulation of nodulation
Symbiotic nodulation is an energy-consuming process, and excessive nodule formation adversely affects regular development of host plant (Wang et al., 2021; Ke et al., 2022). To maintain energy balance between nitrogen fixation and other developmental processes, nodule number is tightly controlled by autoregulation of nodulation (AON) system. AON signaling pathway consists of root-derived signals, receptors in shoot and shoot-derived inhibitors (SDIs), which involve root-shoot-root communications determining optimal nodule numbers (Roy et al., 2020). Transcription factor NIN activates the expression of CLE (CLAVATA3/EMBRYO SURROUNDING REGION) peptides to initiate AON (Figure 1F) (Soyano et al., 2014; Laffont et al., 2020; Wang et al., 2020). The AON-related CLE peptides are widely present in legumes, including M. truncatula CLE12/13, L. japonicus CLE-RS1/2 (CLE-ROOT SIGNAL), as well as RIC1/2 (RHIZOBIUM INDUCED CLE) peptides in soybean (Glycine max) and common bean (Phaseolus vulgaris). As root-derived signals, these CLEs are transported through xylem to shoot (Okamoto et al., 2008; Mortier et al., 2010; Lim et al., 2011), where they are recognized by the leucine-rich-repeat receptor-like kinase (LRR-RLK), termed MtSUNN (SUPER NUMERIC NODULES) in M. truncatula, LjHAR1 (HYPER NODULATION ABERRANT ROOT FORMATION 1) in L. japonicus, and GmNARK (NODULE AUTOREGULATION RECEPTOR KINASE) in G. max. This perception triggers production of SDIs that move back to root suppressing further nodulation (Krusell et al., 2002; Nishimura et al., 2002; Searle et al., 2003; Schnabel et al., 2005; Tsikou et al., 2018). In contrast, M. truncatula CEP7 (C-terminally Encoded Peptide), which is induced by rhizobia and cytokinin, plays a crucial role in promoting rhizobia infections and nodule formation through the receptor MtCRA2 (COMPACT ROOT ARCHITECTURE 2) in shoots. Coordinated expression of CLE and CEP genes by NIN allows precise control of nodule number in plants (Figure 1F) (Laffont et al., 2020). In soybean, GmNINa activates the expression of miR172c, which relieves the transcriptional repression of GmRIC1/2 by NNC1 (Nodule Number Control 1), thus activating AON pathway. Conversely, NNC1 represses miR172c expression, forming a negative feedback loop. NNC1 also interacts with GmNINa to antagonistically regulate the transcriptional activation of GmRIC1/2. Thus, the GmNINa-miR172c-NNC1 signaling axis systemically regulates nodulation and AON signaling (Wang et al., 2019).
Discussion
Symbiotic associations between plants and nitrogen-fixing microbes shape the global ecosystems during the evolution of life on earth. However, plants forming NFN symbiosis are restricted to the FaFaCuRo families (Griesmann et al., 2018; van Velzen et al., 2018; Zhang et al., 2023). Possibly because NFN symbiosis needs intensive energy to produce nodules and fuel nitrogen-fixing reactions. Alternatively, reduced immune responses allowing rhizobia invasion may make plants susceptible to disease (Mathesius, 2022). Recent phylogenomic studies propose a scenario of single gain of nodulation, followed by multiple losses (van Velzen et al., 2019). The emergence of master regulator NIN from a duplication event of NLP and subsequent evolutionary changes, such as the acquisition of specific promoter elements and/or amino acid substitutions, underscores its adaptive significance in driving the evolution of NFN symbiosis in legumes (Liu J. et al., 2019b; Cathebras et al., 2022; Zhang et al., 2023). More efforts are needed to decipher the molecular changes on NIN protein to enable occurrence of NFN symbiosis. We need to better understand the underlying mechanisms of NIN and NLP in regulating different biological processes that range from NFN symbiosis and nitrate signaling. Knowledge from analysis of fossil samples with root nodules and ancient DNA studies would also provide direct evidence for how the NFN symbiosis origins.
Since this initial discovery, NIN has been identified as essential for nodulation in nitrogen-fixing land plants. Besides legumes, NIN orthologs are also required for NFN symbiosis in actinorhizal plants Parasponia andersonii and Casuarina glauca (Clavijo et al., 2015; Bu et al., 2020). P. andersonii hosts rhizobia in thread-like structure, called fixation thread, which is equivalent to symbiosome in legumes that hosts its rhizobial partners. Both structures provide proper environments for nitrogen fixation (Behm et al., 2014). It would be interesting to explore whether the regulatory mechanisms on nodulation and nitrogen fixation mediated by NIN is conserved between legumes and actinorhizal plants. Furthermore, a novel NFN between seagrass Posidonia oceanica and N2-fixing symbiont has been reported recently (Mohr et al., 2021). This finding makes it possible to test functional conservation of NIN in NFN symbiosis across land and aquatic plants. To obtain a complete picture of mechanisms that control the interactions between plants and nitrogen-fixing microorganisms, more plant-microbe systems are needed to be established. Future investigations of NFN symbiosis occurring across diverse plant species will expand our knowledge of molecular mechanisms for nitrogen-fixing signaling and will allow a strategic initiative towards the transfer of the nitrogen-fixing symbiosis to non-nodulating crops.
Author contributions
LS: Investigation, Validation, Writing – original draft, Writing – review & editing. JF: Conceptualization, Funding acquisition, Investigation, Project administration, Resources, Supervision, Validation, Visualization, Writing – review & editing.
Funding
The author(s) declare financial support was received for the research, authorship, and/or publication of this article. Research on this topic in Jian Feng’s laboratory is supported by the National Key R&D Program of China (2022YFF1003201), the National Natural Science Foundation of China (32370261), and the Key Laboratory of Seed Innovation.
Acknowledgments
The authors thank members of Jian Feng’s laboratory for continuous discussion. We apologize to our colleagues whose work could not be cited here owing to limited space.
Conflict of interest
The authors declare that the research was conducted in the absence of any commercial or financial relationships that could be construed as a potential conflict of interest.
Publisher’s note
All claims expressed in this article are solely those of the authors and do not necessarily represent those of their affiliated organizations, or those of the publisher, the editors and the reviewers. Any product that may be evaluated in this article, or claim that may be made by its manufacturer, is not guaranteed or endorsed by the publisher.
References
Akamatsu, A., Nagae, M., Takeda, N. (2022). The CYCLOPS response element in the NIN promoter is important but not essential for infection thread formation during Lotus japonicus-rhizobia symbiosis. Mol. Plant Microbe Interact. 35 (8), 650–658. doi: 10.1094/MPMI-10-21-0252-R
Alvarez, J. M., Schinke, A. L., Brooks, M. D., Pasquino, A., Leonelli, L., Varala, K., et al. (2020). Transient genome-wide interactions of the master transcription factor NLP7 initiate a rapid nitrogen-response cascade. Nat. Commun. 11 (1), 1157. doi: 10.1038/s41467-020-14979-6
Ané, J. M., Kiss, G. B., Riely, B. K., Penmetsa, R. V., Oldroyd, G. E., Ayax, C., et al. (2004). Medicago truncatula DMI1 required for bacterial and fungal symbioses in legumes. Science 303 (5662), 1364–1367. doi: 10.1126/science.1092986
Arrighi, J. F., Barre, A., Ben Amor, B., Bersoult, A., Soriano, L. C., Mirabella, R., et al. (2006). The Medicago truncatula lysine motif-receptor-like kinase gene family includes NFP and new nodule-expressed genes. Plant Physiol. 142 (1), 265–279. doi: 10.1104/pp.106.084657
Arrighi, J. F., Godfroy, O., de Billy, F., Saurat, O., Jauneau, A., Gough, C. (2008). The RPG gene of Medicago truncatula controls Rhizobium-directed polar growth during infection. Proc. Natl. Acad. Sci. U.S.A. 105 (28), 9817–9822. doi: 10.1073/pnas.0710273105
Bai, M., Yuan, J., Kuang, H., Gong, P., Li, S., Zhang, Z., et al. (2020). Generation of a multiplex mutagenesis population via pooled CRISPR-Cas9 in soya bean. Plant Biotechnol. J. 18 (3), 721–731. doi: 10.1111/pbi.13239
Behm, J. E., Geurts, R., Kiers, E. T. (2014). Parasponia: a novel system for studying mutualism stability. Trends Plant Sci. 19 (12), 757–763. doi: 10.1016/j.tplants.2014.08.007
Bhattacharjee, O., Raul, B., Ghosh, A., Bhardwaj, A., Bandyopadhyay, K., Sinharoy, S. (2022). Nodule INception-independent epidermal events lead to bacterial entry during nodule development in peanut (Arachis hypogaea). New Phytol. 236 (6), 2265–2281. doi: 10.1111/nph.18483
Bishopp, A., Bennett, M. J. (2019). Turning lateral roots into nodules. Science 366 (6468), 953–954. doi: 10.1126/science.aay8620
Borisov, A. Y., Madsen, L. H., Tsyganov, V. E., Umehara, Y., Voroshilova, V. A., Batagov, A. O., et al. (2003). The Sym35 gene required for root nodule development in pea is an ortholog of Nin from Lotus japonicus. Plant Physiol. 131 (3), 1009–1017. doi: 10.1104/pp.102.016071
Broghammer, A., Krusell, L., Blaise, M., Sauer, J., Sullivan, J. T., Maolanon, N., et al. (2012). Legume receptors perceive the rhizobial lipochitin oligosaccharide signal molecules by direct binding. Proc. Natl. Acad. Sci. 109 (34), 13859–13864. doi: 10.1073/pnas.1205171109
Bu, F., Rutten, L., Roswanjaya, Y. P., Kulikova, O., Rodriguez-Franco, M., Ott, T., et al. (2020). Mutant analysis in the nonlegume Parasponia andersonii identifies NIN and NF-YA1 transcription factors as a core genetic network in nitrogen-fixing nodule symbioses. New Phytol. 226 (2), 541–554. doi: 10.1111/nph.16386
Capoen, W., Sun, J., Wysham, D., Otegui, M. S., Venkateshwaran, M., Hirsch, S., et al. (2011). Nuclear membranes control symbiotic calcium signaling of legumes. Proc. Natl. Acad. Sci. U.S.A. 108 (34), 14348–14353. doi: 10.1073/pnas.1107912108
Castaings, L., Camargo, A., Pocholle, D., Gaudon, V., Texier, Y., Boutet-Mercey, S., et al. (2009). The nodule inception-like protein 7 modulates nitrate sensing and metabolism in Arabidopsis. Plant J. 57 (3), 426–435. doi: 10.1111/j.1365-313X.2008.03695.x
Cathebras, C., Gong, X., Andrade, R. E., Vondenhoff, K., Keller, J., Delaux, P.-M., et al. (2022). A novel cis-element enabled bacterial uptake by plant cells. BioRxiv 28, 486070. doi: 10.1101/2022.03.28.486070
Chardin, C., Girin, T., Roudier, F., Meyer, C., Krapp, A. (2014). The plant RWP-RK transcription factors: key regulators of nitrogen responses and of gametophyte development. J. Exp. Bot. 65 (19), 5577–5587. doi: 10.1093/jxb/eru261
Charpentier, M., Bredemeier, R., Wanner, G., Takeda, N., Schleiff, E., Parniske, M. (2008). Lotus japonicus CASTOR and POLLUX are ion channels essential for perinuclear calcium spiking in legume root endosymbiosis. Plant Cell 20 (12), 3467–3479. doi: 10.1105/tpc.108.063255
Charpentier, M., Sun, J., Vaz Martins, T., Radhakrishnan, G. V., Findlay, K., Soumpourou, E., et al. (2016). Nuclear-localized cyclic nucleotide-gated channels mediate symbiotic calcium oscillations. Science 352 (6289), 1102–1105. doi: 10.1126/science.aae0109
Clavijo, F., Diedhiou, I., Vaissayre, V., Brottier, L., Acolatse, J., Moukouanga, D., et al. (2015). The Casuarina NIN gene is transcriptionally activated throughout Frankia root infection as well as in response to bacterial diffusible signals. New Phytol. 208 (3), 887–903. doi: 10.1111/nph.13506
Davis, T. M. (1988). Two genes that confer ineffective nodulation in chickpea (Cicer arietinum l.). J. Hered. 79 (6), 476–478. doi: 10.1093/oxfordjournals.jhered.a110555
Dong, W., Zhu, Y., Chang, H., Wang, C., Yang, J., Shi, J., et al. (2021). An SHR-SCR module specifies legume cortical cell fate to enable nodulation. Nature 589 (7843), 586–590. doi: 10.1038/s41586-020-3016-z
Endre, G., Kereszt, A., Kevei, Z., Mihacea, S., Kaló, P., Kiss, G. B. (2002). A receptor kinase gene regulating symbiotic nodule development. Nature 417 (6892), 962–966. doi: 10.1038/nature00842
Engvild, K. C. (1987). Nodulation and nitrogen fixation mutants of pea, Pisum sativum. Theor. Appl. Genet. 74 (6), 711–713. doi: 10.1007/BF00247546
Esseling, J. J., Lhuissier, F. G., Emons, A. M. (2003). Nod factor-induced root hair curling: continuous polar growth towards the point of nod factor application. Plant Physiol. 132 (4), 1982–1988. doi: 10.1104/pp.103.021634
Feng, J., Lee, T., Schiessl, K., Oldroyd, G. E. D. (2021). Processing of NODULE INCEPTION controls the transition to nitrogen fixation in root nodules. Science 374 (6567), 629–632. doi: 10.1126/science.abg2804
Ferris, P. J., Goodenough, U. W. (1997). Mating type in Chlamydomonas is specified by mid, the minus-dominance gene. Genetics 146 (3), 859–869. doi: 10.1093/genetics/146.3.859
Frailey, D. C., Zhang, Q., Wood, D. J., Davis, T. M. (2022). Defining the mutation sites in chickpea nodulation mutants PM233 and PM405. BMC Plant Biol. 22 (1), 66. doi: 10.1186/s12870-022-03446-7
Fu, M., Sun, J., Li, X., Guan, Y., Xie, F. (2022). Asymmetric redundancy of soybean nodule inception (NIN) genes in root nodule symbiosis. Plant Physiol. 188 (1), 477–489. doi: 10.1093/plphys/kiab473
Gauthier-Coles, C., White, R. G., Mathesius, U. (2018). Nodulating legumes are distinguished by a sensitivity to cytokinin in the root cortex leading to pseudonodule development. Front. Plant Sci. 9. doi: 10.3389/fpls.2018.01901
Griesmann, M., Chang, Y., Liu, X., Song, Y., Haberer, G., Crook, M. B., et al. (2018). Phylogenomics reveals multiple losses of nitrogen-fixing root nodule symbiosis. Science 361 (6398), eaat1743. doi: 10.1126/science.aat1743
Imaizumi-Anraku, H., Takeda, N., Charpentier, M., Perry, J., Miwa, H., Umehara, Y., et al. (2005). Plastid proteins crucial for symbiotic fungal and bacterial entry into plant roots. Nature 433 (7025), 527–531. doi: 10.1038/nature03237
Irving, T. B., Chakraborty, S., Maia, L. G. S., Knaack, S., Conde, D., Schmidt, H. W., et al. (2022). An LCO-responsive homolog of NODULE INCEPTION positively regulates lateral root formation in Populus sp. Plant Physiol. 190 (3), 1699–1714. doi: 10.1093/plphys/kiac356
Jiang, S., Jardinaud, M. F., Gao, J., Pecrix, Y., Wen, J., Mysore, K., et al. (2021). NIN-like protein transcription factors regulate leghemoglobin genes in legume nodules. Science 374 (6567), 625–628. doi: 10.1126/science.abg5945
Jin, Y., Liu, H., Luo, D., Yu, N., Dong, W., Wang, C., et al. (2016). DELLA proteins are common components of symbiotic rhizobial and mycorrhizal signalling pathways. Nat. Commun. 7 (1), 12433. doi: 10.1038/ncomms12433
Kawaharada, Y., Kelly, S., Nielsen, M. W., Hjuler, C. T., Gysel, K., Muszyński, A., et al. (2015). Receptor-mediated exopolysaccharide perception controls bacterial infection. Nature 523 (7560), 308–312. doi: 10.1038/nature14611
Kawaharada, Y., Nielsen, M. W., Kelly, S., James, E. K., Andersen, K. R., Rasmussen, S. R., et al. (2017). Differential regulation of the Epr3 receptor coordinates membrane-restricted rhizobial colonization of root nodule primordia. Nat. Commun. 8, 14534. doi: 10.1038/ncomms14534
Ke, X., Xiao, H., Peng, Y., Wang, J., Lv, Q., Wang, X. (2022). Phosphoenolpyruvate reallocation links nitrogen fixation rates to root nodule energy state. Science 378 (6623), 971–977. doi: 10.1126/science.abq8591
Kistner, C., Parniske, M. (2002). Evolution of signal transduction in intracellular symbiosis. Trends Plant Sci. 7 (11), 511–518. doi: 10.1016/s1360-1385(02)02356-7
Kondorosi, E., Mergaert, P., Kereszt, A. (2013). A paradigm for endosymbiotic life: cell differentiation of Rhizobium bacteria provoked by host plant factors. Annu. Rev. Microbiol. 67, 611–628. doi: 10.1146/annurev-micro-092412-155630
Konishi, M., Yanagisawa, S. (2013). Arabidopsis NIN-like transcription factors have a central role in nitrate signalling. Nat. Commun. 4, 1617. doi: 10.1038/ncomms2621
Krusell, L., Madsen, L. H., Sato, S., Aubert, G., Genua, A., Szczyglowski, K., et al. (2002). Shoot control of root development and nodulation is mediated by a receptor-like kinase. Nature 420 (6914), 422–426. doi: 10.1038/nature01207
Lace, B., Su, C., Invernot Perez, D., Rodriguez-Franco, M., Vernié, T., Batzenschlager, M., et al. (2023). RPG acts as a central determinant for infectosome formation and cellular polarization during intracellular rhizobial infections. Elife 12, e80741. doi: 10.7554/eLife.80741
Laffont, C., Ivanovici, A., Gautrat, P., Brault, M., Djordjevic, M. A., Frugier, F. (2020). The NIN transcription factor coordinates CEP and CLE signaling peptides that regulate nodulation antagonistically. Nat. Commun. 11 (1), 3167. doi: 10.1038/s41467-020-16968-1
Laporte, P., Lepage, A., Fournier, J., Catrice, O., Moreau, S., Jardinaud, M.-F., et al. (2013). The CCAAT box-binding transcription factor NF-YA1 controls rhizobial infection. J. Exp. Bot. 65 (2), 481–494. doi: 10.1093/jxb/ert392
Lévy, J., Bres, C., Geurts, R., Chalhoub, B., Kulikova, O., Duc, G., et al. (2004). A putative Ca2+ and calmodulin-dependent protein kinase required for bacterial and fungal symbioses. Science 303 (5662), 1361–1364. doi: 10.1126/science.1093038
Li, X., Liu, M., Cai, M., Chiasson, D., Groth, M., Heckmann, A. B., et al. (2023). RPG interacts with E3-ligase CERBERUS to mediate rhizobial infection in Lotus japonicus. PloS Genet. 19 (2), e1010621. doi: 10.1371/journal.pgen.1010621
Lim, C. W., Lee, Y. W., Hwang, C. H. (2011). Soybean nodule-enhanced CLE peptides in roots act as signals in GmNARK-mediated nodulation suppression. Plant Cell Physiol. 52 (9), 1613–1627. doi: 10.1093/pcp/pcr091
Limpens, E., Franken, C., Smit, P., Willemse, J., Bisseling, T., Geurts, R. (2003). LysM domain receptor kinases regulating rhizobial Nod factor-induced infection. Science 302 (5645), 630–633. doi: 10.1126/science.1090074
Lin, J. S., Li, X., Luo, Z., Mysore, K. S., Wen, J., Xie, F. (2018). NIN interacts with NLPs to mediate nitrate inhibition of nodulation in Medicago truncatula. Nat. Plants 4 (11), 942–952. doi: 10.1038/s41477-018-0261-3
Liu, J., Bisseling, T. (2020). Evolution of NIN and NIN-like genes in relation to nodule symbiosis. Genes (Basel) 11 (7), 777. doi: 10.3390/genes11070777
Liu, C. W., Breakspear, A., Guan, D., Cerri, M. R., Jackson, K., Jiang, S., et al. (2019). NIN acts as a network hub controlling a growth module required for rhizobial infection. Plant Physiol. 179 (4), 1704–1722. doi: 10.1104/pp.18.01572
Liu, K. H., Liu, M., Lin, Z., Wang, Z. F., Chen, B., Liu, C., et al. (2022). NIN-like protein 7 transcription factor is a plant nitrate sensor. Science 377 (6613), 1419–1425. doi: 10.1126/science.add1104
Liu, K. H., Niu, Y., Konishi, M., Wu, Y., Du, H., Sun Chung, H., et al. (2017). Discovery of nitrate-CPK-NLP signalling in central nutrient-growth networks. Nature 545 (7654), 311–316. doi: 10.1038/nature22077
Liu, J., Rasing, M., Zeng, T., Klein, J., Kulikova, O., Bisseling, T. (2021). NIN is essential for development of symbiosomes, suppression of defence and premature senescence in Medicago truncatula nodules. New Phytol. 230 (1), 290–303. doi: 10.1111/nph.17215
Liu, J., Rutten, L., Limpens, E., van der Molen, T., van Velzen, R., Chen, R., et al. (2019). A remote cis-regulatory region is required for NIN expression in the pericycle to initiate nodule primordium formation in Medicago truncatula. Plant Cell 31 (1), 68–83. doi: 10.1105/tpc.18.00478
Liu, H., Sandal, N., Andersen, K. R., James, E. K., Stougaard, J., Kelly, S., et al. (2018). A genetic screen for plant mutants with altered nodulation phenotypes in response to rhizobial glycan mutants. New Phytol. 220 (2), 526–538. doi: 10.1111/nph.15293
Madsen, E. B., Madsen, L. H., Radutoiu, S., Olbryt, M., Rakwalska, M., Szczyglowski, K., et al. (2003). A receptor kinase gene of the LysM type is involved in legumeperception of rhizobial signals. Nature 425 (6958), 637–640. doi: 10.1038/nature02045
Madsen, L. H., Fukai, E., Radutoiu, S., Yost, C. K., Sandal, N., Schauser, L., et al. (2005). LORE1, an active low-copy-number TY3-gypsy retrotransposon family in the model legume Lotus japonicus. Plant J. 44 (3), 372–381. doi: 10.1111/j.1365-313X.2005.02534.x
Marchive, C., Roudier, F., Castaings, L., Bréhaut, V., Blondet, E., Colot, V., et al. (2013). Nuclear retention of the transcription factor NLP7 orchestrates the early response to nitrate in plants. Nat. Commun. 4, 1713. doi: 10.1038/ncomms2650
Marsh, J. F., Rakocevic, A., Mitra, R. M., Brocard, L., Sun, J., Eschstruth, A., et al. (2007). Medicago truncatula NIN is essential for rhizobial-independent nodule organogenesis induced by autoactive calcium/calmodulin-dependent protein kinase. Plant Physiol. 144 (1), 324–335. doi: 10.1104/pp.106.093021
Mathesius, U. (2022). Are legumes different? Origins and consequences of evolving nitrogen fixing symbioses. J. Plant Physiol. 276, 153765. doi: 10.1016/j.jplph.2022.153765
Mergaert, P., Kereszt, A., Kondorosi, E. (2020). Gene expression in nitrogen-fixing symbiotic nodule cells in Medicago truncatula and other nodulating plants. Plant Cell 32 (1), 42–68. doi: 10.1105/tpc.19.00494
Miller, J. B., Pratap, A., Miyahara, A., Zhou, L., Bornemann, S., Morris, R. J., et al. (2013). Calcium/calmodulin-dependent protein kinase is negatively and positively regulated by calcium, providing a mechanism for decoding calcium responses during symbiosis signaling. Plant Cell 25 (12), 5053–5066. doi: 10.1105/tpc.113.116921
Mohr, W., Lehnen, N., Ahmerkamp, S., Marchant, H. K., Graf, J. S., Tschitschko, B., et al. (2021). Terrestrial-type nitrogen-fixing symbiosis between seagrass and a marine bacterium. Nature 600 (7887), 105–109. doi: 10.1038/s41586-021-04063-4
Moling, S., Pietraszewska-Bogiel, A., Postma, M., Fedorova, E., Hink, M. A., Limpens, E., et al. (2014). Nod factor receptors form heteromeric complexes and are essential for intracellular infection in Medicago nodules. Plant Cell 26 (10), 4188–4199. doi: 10.1105/tpc.114.129502
Mortier, V., Den Herder, G., Whitford, R., Van de Velde, W., Rombauts, S., D’haeseleer, K., et al. (2010). CLE peptides control Medicago truncatula nodulation locally and systemically. Plant Physiol. 153 (1), 222–237. doi: 10.1104/pp.110.153718
Murray, J., Karas, B., Ross, L., Brachmann, A., Wagg, C., Geil, R., et al. (2006). Genetic suppressors of the Lotus japonicus har1-1 hypernodulation phenotype. Mol. Plant Microbe Interact. 19 (10), 1082–1091. doi: 10.1094/MPMI-19-1082
Nishida, H., Nosaki, S., Suzuki, T., Ito, M., Miyakawa, T., Nomoto, M., et al. (2021). Different DNA-binding specificities of NLP and NIN transcription factors underlie nitrate-induced control of root nodulation. Plant Cell 33 (7), 2340–2359. doi: 10.1093/plcell/koab103
Nishida, H., Tanaka, S., Handa, Y., Ito, M., Sakamoto, Y., Matsunaga, S., et al. (2018). A NIN-LIKE PROTEIN mediates nitrate-induced control of root nodule symbiosis in Lotus japonicus. Nat. Commun. 9 (1), 499. doi: 10.1038/s41467-018-02831-x
Nishimura, R., Hayashi, M., Wu, G.-J., Kouchi, H., Imaizumi-Anraku, H., Murakami, Y., et al. (2002). HAR1 mediates systemic regulation of symbiotic organ development. Nature 420 (6914), 426–429. doi: 10.1038/nature01231
Okamoto, S., Ohnishi, E., Sato, S., Takahashi, H., Nakazono, M., Tabata, S., et al. (2008). Nod factor/nitrate-induced CLE genes that drive HAR1-mediated systemic regulation of nodulation. Plant Cell Physiol. 50 (1), 67–77. doi: 10.1093/pcp/pcn194
Oldroyd, G. E. (2013). Speak, friend, and enter: signalling systems that promote beneficial symbiotic associations in plants. Nat. Rev. Microbiol. 11 (4), 252–263. doi: 10.1038/nrmicro2990
Oldroyd, G. E., Downie, J. A. (2004). Calcium, kinases and nodulation signalling in legumes. Nat. Rev. Mol. Cell Biol. 5 (7), 566–576. doi: 10.1038/nrm1424
Perry, J., Brachmann, A., Welham, T., Binder, A., Charpentier, M., Groth, M., et al. (2009). TILLING in Lotus japonicus identified large allelic series for symbiosis genes and revealed a bias in functionally defective ethyl methanesulfonate alleles toward glycine replacements. Plant Physiol. 151 (3), 1281–1291. doi: 10.1104/pp.109.142190
Pislariu, C. I., Murray, D., Wen, J., Cosson, V., Muni, R. R. D., Wang, M., et al. (2012). A Medicago truncatula tobacco retrotransposon insertion mutant collection with defects in nodule development and symbiotic nitrogen fixation. Plant Physiol. 159 (4), 1686–1699. doi: 10.1104/pp.112.197061
Qiu, L., Lin, J. S., Xu, J., Sato, S., Parniske, M., Wang, T. L., et al. (2015). SCARN a novel class of SCAR protein that is required for root-hair infection during legume nodulation. PloS Genet. 11 (10), e1005623. doi: 10.1371/journal.pgen.1005623
Radutoiu, S., Madsen, L. H., Madsen, E. B., Felle, H. H., Umehara, Y., Grønlund, M., et al. (2003). Plant recognition of symbiotic bacteria requires two LysM receptor-like kinases. Nature 425 (6958), 585–592. doi: 10.1038/nature02039
Roy, S., Liu, W., Nandety, R. S., Crook, A., Mysore, K. S., Pislariu, C. I., et al. (2020). Celebrating 20 years of genetic discoveries in legume nodulation and symbiotic nitrogen fixation. Plant Cell 32 (1), 15–41. doi: 10.1105/tpc.19.00279
Sandal, N., Petersen, T. R., Murray, J., Umehara, Y., Karas, B., Yano, K., et al. (2006). Genetics of symbiosis in Lotus japonicus: recombinant inbred lines, comparative genetic maps, and map position of 35 symbiotic loci. Mol. Plant Microbe Interact. 19 (1), 80–91. doi: 10.1094/MPMI-19-0080
Santi, C., Bogusz, D., Franche, C. (2013). Biological nitrogen fixation in non-legume plants. Ann. Bot. 111 (5), 743–767. doi: 10.1093/aob/mct048
Schauser, L., Roussis, A., Stiller, J., Stougaard, J. (1999). A plant regulator controlling development of symbiotic root nodules. Nature 402 (6758), 191–195. doi: 10.1038/46058
Schiessl, K., Lilley, J. L. S., Lee, T., Tamvakis, I., Kohlen, W., Bailey, P. C., et al. (2019). NODULE INCEPTION recruits the lateral root developmental program for symbiotic nodule organogenesis in Medicago truncatula. Curr. Biol. 29 (21), 3657–3668.e3655. doi: 10.1016/j.cub.2019.09.005
Schnabel, E., Journet, E.-P., de Carvalho-Niebel, F., Duc, G., Frugoli, J. (2005). The Medicago truncatula SUNN gene encodes a CLV1-like leucine-rich repeat receptor kinase that regulates nodule number and root length. Plant Mol. Biol. 58 (6), 809–822. doi: 10.1007/s11103-005-8102-y
Searle, I. R., Men, A. E., Laniya, T. S., Buzas, D. M., Iturbe-Ormaetxe, I., Carroll, B. J., et al. (2003). Long-distance signaling in nodulation directed by a CLAVATA1-like receptor kinase. Science 299 (5603), 109–112. doi: 10.1126/science.1077937
Shahan, R., Benfey, P. N. (2020). A co-opted regulator of lateral root development controls nodule organogenesis in Lotus. Dev. Cell 52 (1), 6–7. doi: 10.1016/j.devcel.2019.12.009
Singh, S., Katzer, K., Lambert, J., Cerri, M., Parniske, M. (2014). CYCLOPS, a DNA-binding transcriptional activator, orchestrates symbiotic root nodule development. Cell Host Microbe 15 (2), 139–152. doi: 10.1016/j.chom.2014.01.011
Singh, S., Parniske, M. (2012). Activation of calcium- and calmodulin-dependent protein kinase (CCaMK), the central regulator of plant root endosymbiosis. Curr. Opin. Plant Biol. 15 (4), 444–453. doi: 10.1016/j.pbi.2012.04.002
Sinharoy, S., Liu, C., Breakspear, A., Guan, D., Shailes, S., Nakashima, J., et al. (2016). A Medicago truncatula cystathionine-b-synthase-like domain-containing protein is required for rhizobial infection and symbiotic nitrogen fixation. Plant Physiol. 170 (4), 2204–2217. doi: 10.1104/pp.15.01853
Smit, P., Limpens, E., Geurts, R., Fedorova, E., Dolgikh, E., Gough, C., et al. (2007). Medicago LYK3, an entry receptor in rhizobial nodulation factor signaling. Plant Physiol. 145 (1), 183–191. doi: 10.1104/pp.107.100495
Soltis, D. E., Soltis, P. S., Morgan, D. R., Swensen, S. M., Mullin, B. C., Dowd, J. M., et al. (1995). Chloroplast gene sequence data suggest a single origin of the predisposition for symbiotic nitrogen fixation in angiosperms. Proc. Natl. Acad. Sci. U.S.A. 92 (7), 2647–2651. doi: 10.1073/pnas.92.7.2647
Soyano, T., Hirakawa, H., Sato, S., Hayashi, M., Kawaguchi, M. (2014). Nodule Inception creates a long-distance negative feedback loop involved in homeostatic regulation of nodule organ production. Proc. Natl. Acad. Sci. U.S.A. 111 (40), 14607–14612. doi: 10.1073/pnas.1412716111
Soyano, T., Kouchi, H., Hirota, A., Hayashi, M. (2013). Nodule inception directly targets NF-Y subunit genes to regulate essential processes of root nodule development in Lotus japonicus. PloS Genet. 9 (3), e1003352. doi: 10.1371/journal.pgen.1003352
Soyano, T., Shimoda, Y., Kawaguchi, M., Hayashi, M. (2019). A shared gene drives lateral root development and root nodule symbiosis pathways in Lotus. Science 366 (6468), 1021–1023. doi: 10.1126/science.aax2153
Stambulska, U. Y., Bayliak, M. M. (2019). “Legume-rhizobium symbiosis: secondary metabolites, free radical processes, and effects of heavy metals,” in Co-Evolution of Secondary Metabolites. Eds. Merillon, J.-M., Ramawat, K. G. (Cham: Springer International Publishing), 1–32.
Stracke, S., Kistner, C., Yoshida, S., Mulder, L., Sato, S., Kaneko, T., et al. (2002). A plant receptor-like kinase required for both bacterial and fungal symbiosis. Nature 417 (6892), 959–962. doi: 10.1038/nature00841
Sumimoto, H., Kamakura, S., Ito, T. (2007). Structure and function of the PB1 domain, a protein interaction module conserved in animals, fungi, amoebas, and plants. Sci. STKE 2007 (401), re6. doi: 10.1126/stke.4012007re6
Suzaki, T., Yano, K., Ito, M., Umehara, Y., Suganuma, N., Kawaguchi, M. (2012). Positive and negative regulation of cortical cell division during root nodule development in Lotus japonicus is accompanied by auxin response. Development 139 (21), 3997–4006. doi: 10.1242/dev.084079
Tirichine, L., Sandal, N., Madsen, L. H., Radutoiu, S., Albrektsen, A. S., Sato, S., et al. (2007). A gain-of-function mutation in a cytokinin receptor triggers spontaneous root nodule organogenesis. Science 315 (5808), 104–107. doi: 10.1126/science.1132397
Tsikou, D., Yan, Z., Holt, D. B., Abel, N. B., Reid, D. E., Madsen, L. H., et al. (2018). Systemic control of legume susceptibility to rhizobial infection by a mobile microRNA. Science 362 (6411), 233–236. doi: 10.1126/science.aat6907
Tsyganov, V. E., Voroshilova, V. A., Kukalev, A. S., Jacobi, L. M., Azarova, T. S., Borisov, A. Y., et al. (1999). Pisum sativum L. genes Sym14 and Sym35 control infection thread growth initiation during the development of symbiotic nodules. Russ. J. Genet. 35 (3), 284–291.
van Velzen, R., Doyle, J. J., Geurts, R. (2019). A resurrected scenario: single gain and massive loss of nitrogen-fixing nodulation. Trends Plant Sci. 24 (1), 49–57. doi: 10.1016/j.tplants.2018.10.005
van Velzen, R., Holmer, R., Bu, F., Rutten, L., van Zeijl, A., Liu, W., et al. (2018). Comparative genomics of the nonlegume Parasponia reveals insights into evolution of nitrogen-fixing rhizobium symbioses. Proc. Natl. Acad. Sci. 115 (20), E4700–E4709. doi: 10.1073/pnas.1721395115
Veerappan, V., Jani, M., Kadel, K., Troiani, T., Gale, R., Mayes, T., et al. (2016). Rapid identification of causative insertions underlying Medicago truncatula Tnt1 mutants defective in symbiotic nitrogen fixation from a forward genetic screen by whole genome sequencing. BMC Genomics 17, 141. doi: 10.1186/s12864-016-2452-5
Vernié, T., Kim, J., Frances, L., Ding, Y., Sun, J., Guan, D., et al. (2015). The NIN transcription factor coordinates diverse nodulation programs in different tissues of the Medicago truncatula root. Plant Cell 27 (12), 3410–3424. doi: 10.1105/tpc.15.00461
Wang, D., Dong, W., Murray, J., Wang, E. (2022). Innovation and appropriation in mycorrhizal and rhizobial symbioses. Plant Cell 34 (5), 1573–1599. doi: 10.1093/plcell/koac039
Wang, T., Guo, J., Peng, Y., Lyu, X., Liu, B., Sun, S., et al. (2021). Light-induced mobile factors from shoots regulate rhizobium-triggered soybean root nodulation. Science 374 (6563), 65–71. doi: 10.1126/science.abh2890
Wang, L., Sun, Z., Su, C., Wang, Y., Yan, Q., Chen, J., et al. (2019). A GmNINa-miR172c-NNC1 regulatory network coordinates the nodulation and autoregulation of nodulation pathways in soybean. Mol. Plant 12 (9), 1211–1226. doi: 10.1016/j.molp.2019.06.002
Wang, Z., Wang, L., Wang, Y., Li, X. (2020). The NMN module conducts nodule number orchestra. iScience 23 (2), 100825. doi: 10.1016/j.isci.2020.100825
Xiao, T. T., Schilderink, S., Moling, S., Deinum, E. E., Kondorosi, E., Franssen, H., et al. (2014). Fate map of Medicago truncatula root nodules. Development 141 (18), 3517–3528. doi: 10.1242/dev.110775
Xie, F., Murray, J. D., Kim, J., Heckmann, A. B., Edwards, A., Oldroyd, G. E. D., et al. (2012). Legume pectate lyase required for root infection by rhizobia. Proc. Natl. Acad. Sci. 109 (2), 633–638. doi: 10.1073/pnas.1113992109
Yano, K., Yoshida, S., Muller, J., Singh, S., Banba, M., Vickers, K., et al. (2008). CYCLOPS, a mediator of symbiotic intracellular accommodation. Proc. Natl. Acad. Sci. U.S.A. 105 (51), 20540–20545. doi: 10.1073/pnas.0806858105
Yoro, E., Suzaki, T., Toyokura, K., Miyazawa, H., Fukaki, H., Kawaguchi, M. (2014). A positive regulator of nodule organogenesis, NODULE INCEPTION, acts as a negative regulator of rhizobial infection in Lotus japonicus. Plant Physiol. 165 (2), 747–758. doi: 10.1104/pp.113.233379
Yuan, P., Luo, F., Gleason, C., Poovaiah, B. W. (2022). Calcium/calmodulin-mediated microbial symbiotic interactions in plants. Front. Plant Sci. 13. doi: 10.3389/fpls.2022.984909
Zhang, Y., Fu, Y., Xian, W., Li, X., Feng, Y., Bu, F., et al. (2023). Comparative phylogenomics and phylotranscriptomics provide insights into the genetic complexity of nitrogen fixing root nodule symbiosis. Plant Commun. 100671. doi: 10.1016/j.xplc.2023.100671
Keywords: nitrogen-fixing symbiosis, NODULE INCEPTION, infection thread, nodule organogenesis, autoregulation of nodulation
Citation: Shen L and Feng J (2024) NIN—at the heart of NItrogen-fixing Nodule symbiosis. Front. Plant Sci. 14:1284720. doi: 10.3389/fpls.2023.1284720
Received: 29 August 2023; Accepted: 27 December 2023;
Published: 12 January 2024.
Edited by:
Chang Fu Tian, China Agricultural University, ChinaReviewed by:
Dong Wang, University of Massachusetts Amherst, United StatesSenjuti Sinharoy, National Institute of Plant Genome Research (NIPGR), India
Copyright © 2024 Shen and Feng. This is an open-access article distributed under the terms of the Creative Commons Attribution License (CC BY). The use, distribution or reproduction in other forums is permitted, provided the original author(s) and the copyright owner(s) are credited and that the original publication in this journal is cited, in accordance with accepted academic practice. No use, distribution or reproduction is permitted which does not comply with these terms.
*Correspondence: Jian Feng, jfeng@genetics.ac.cn