- 1Division of Agronomy, Indian Council of Agricultural Research (ICAR)-Indian Agricultural Research Institute, New Delhi, India
- 2Division of Microbiology, Indian Council of Agricultural Research (ICAR)-Indian Agricultural Research Institute, New Delhi, India
- 3Indian Council of Agricultural Research (ICAR)-National Academy of Agricultural Research Management, Hyderabad, Telangana, India
- 4Division of Natural Resource Management, Indian Council of Agricultural Research (ICAR)-Indian Agricultural Research Institute, Dhemaji, Assam, India
During the summer and rainy seasons (April-October) of 2020 and 2021, two consecutive field experiments were conducted at the research farm of the ICAR-Indian Agricultural Research Institute, New Delhi, India. In this study, we examined the effects of summer green manuring crops (GM) and a variety of zinc fertilizers (ZnF) on Basmati rice (Oryza sativa L.) growth, physiological development, yield response, zinc nutrition and economic returns. A combination of GM residues and nano zinc fertilization helped significantly enhancing Basmati rice’s growth and its physiological development. Following the incorporation of Sesbania aculeata (Sesbania), successive Basmati rice physiological parameters were significantly improved, as well as grain, straw, biological yields, harvest index and economic returns. The highest Zn content of 15.1 mg kg -1 and the lowest of 11.8 mg kg -1 in milled rice grain were recorded in Sesbania green manuring (G2) and control i.e., in the fallow (G1), respectively. Coating onto urea with 0.2% nano zinc oxide (NZnCU) was observed to be more effective than other zinc sources in terms of growth parameters, yield attributes, zinc nutrition, grain and straw yields for succeeding Basmati rice crop; however, the effects were comparable to those of bulk zinc oxide-coated urea (BZnCU) of 1%. The highest Zn content of 15.1 mg kg -1 was recorded with the application of 1% BZnCU and the lowest of 11.96 mg kg -1 with the soil application of 5 kg Zn ha -1 through bulk ZnO in the milled rice grain. Application of 1% BZnCU led to a 26.25% increase in Zn content of milled rice grain compared to soil application of 5 kg Zn ha -1 through bulk ZnO. As a result, the combination of inclusion of Sesbania aculeata (Sesbania) residue and 0.2% NZnCU was identified as the most effective treatment, for Basmati rice growth and physiological development. A combination of nano Zn fertilization in conjunction with the incorporation of green manure can be advocated for better growth, physiological performance, zinc dense grains, and higher profitability of Basmati rice for farmers and consumers.
1 Introduction
India holds a prominent position as the world’s second–largest producer of rice, responsible for a fifth of global rice cultivation (Kumar et al., 2021). It serves as a vital nutritional source, fulfilling 15% of protein requirements and 21% of caloric needs for its populace (Prasad et al., 2017). Particularly noteworthy is the Basmati rice, which is prized for its elongation upon cooking and its fluffy texture. In the financial year 2021–22, the country garnered a staggering US $3.54 billion through Basmati rice exports alone, making it the global leader in this market (APEDA, 2023). However, the average yield of rice in India lags at 3.42 tons per hectare (Directorate of Economics and Statistics, 2020). Given the burgeoning population, the nation faces the daunting challenge of ramping up rice production to an estimated 130 million tons by 2030 to maintain food security. This task is complicated by numerous obstacles, including inadequate irrigation systems, deteriorating soil quality, and imbalances in macro– and micronutrients (Bhatt et al., 2016). For example, high temperature and moisture content in paddy soils hasten the decay of organic matter (Mohammad et al., 2005), resulting in its depletion. The use of green manures has been touted as a way to mitigate soil erosion and augment both soil structure and fertility (Mandal et al., 2003). One specific micronutrient issue that prevails, especially in Indian and South Asian paddy fields, is zinc (Zn) deficiency, observed in approximately 51.2% (Shukla et al., 2021) of Indian soils. The scarcity of zinc is exacerbated due to its transformation into insoluble compounds in waterlogged conditions and high soil pH (Prasad et al., 2014). Zinc is indispensable for various plant metabolic functions, ranging from cell wall synthesis to carbohydrate metabolism (Broadley et al., 2007). Its deficiency manifests as leaf discoloration, reduced growth, and sterile seeds (Cakmak, 2008).
Traditional solutions involve zinc fertilization (Nayak et al., 2022), the efficacy of which varies depending on the type of fertilizer (Karak et al., 2005) and the rice genotype (Nayak et al., 2023). Recently, nano zinc applications have gained traction, offering benefits such as greater efficiency (Sadhukhan et al., 2021), quicker response, and enhanced grain enrichment (Baral et al., 2019; Baral et al., 2020; Baral et al., 2023). Nano–zinc oxide (ZnO) application has been shown to bolster both rice yield and grain zinc content (Pavithra et al., 2017). Similarly, zinc oxide–coated urea has displayed a favorable impact on yield and zinc content in both rice and wheat crops (Shivay et al., 2008). According to the findings of another study (Dimkpa et al., 2020), coating urea with nano–ZnO led to improved plant performance as well as increased zinc accumulation in wheat. Notably, existing research largely concentrates on the sole effects of ZnO nanoparticles (NPs) on plant health. Very few studies explore the synergistic benefits of coupling summer green manuring with nano–ZnO–coated urea. The integrated approach of utilizing both green manures and nano–fertilizers could offer a holistic solution, promising enhanced crop growth, physiological performance, yield, and economic returns.
In light of the aforementioned factors, a two–year field studies were conducted to scrutinize the effects of summer green manures and nanoscale zinc fertilizers on various parameters, such as growth, physiological indices, and economic viability under summer green manure–Basmati rice cropping sequence.
2 Materials and methods
2.1 Experimental site
Over the duration of two successive years, field studies were carried at the ICAR–Indian Agricultural Research Institute, New Delhi, India. The experiments were undertaken during the summer–rainy seasons of 2020 (first year of field study) and 2021 (second year of field study) in sandy clay–loam soil, specifically a typic Ustochrept soil type. The research farm of the ICAR–Indian Agricultural Research Institute is positioned at 28°38′ 24′′ N, 77°10′ 26′′ E and 228.6 meters above mean sea level. The region experiences an average annual rainfall of 650 mm, with more than 80% occurring during the south–west monsoon season from July to September. The mean annual evaporation of the site is approximately 850 mm. The experimental field at the institute farm has been traditionally managed using conventional methods, following a 4–year crop rotation scheme (Table 1). However, the fertilization management varied during two years of our field study, as outlined in Table 2. The initial soil analysis revealed that the experimental field had 171 kg ha–1 of alkaline permanganate oxidizable nitrogen (N) (Subbiah and Asija, 1956), 15.9 kg ha–1 of available phosphorus (P) (Olsen et al., 1954), 309 kg ha–1 of 1 N ammonium acetate exchangeable potassium (K) (Hanway and Heidel, 1952), and 0.65% organic carbon (C) (Prasad et al., 2006). The soil pH, determined using a 1:2.5 soil and water ratio, was found to be 7.68 (Prasad et al., 2006). Additionally, the diethylene triamine penta acetic acid (DTPA)–extractable zinc (Zn) content in the soil was measured to be 0.67 mg kg–1 (Lindsay and Norvell, 1978). Based on the critical level of DTPA–extractable Zn ranging from 0.38–0.90 mg kg–1 soil, for rice cultivation in the rice–wheat belt of North India, as reported by (Takkar et al., 1997), it was hypothesized that the application of Zn would affect the growth and yield of Basmati rice in the experimental field.
2.2 Treatment details
The experiment was conducted in a split–plot design with one summer fallow and two green manure crops as the main plot and six subplots comprising diverse Zn fertilizer sources with three replication. A detailed description of the treatments is given in Table 2.
2.3 Characterization of different Zn sources used in the experiment
The Zn sources employed in our experiment i.e., both bulk ZnO and nano ZnO (manufacturer: Sigma Aldrich) were characterized using the following techniques mentioned in Table 3.
2.4 Crop establishment and management
The 45–day–old green manure crops were incorporated into the experimental field using a tractor–operated disc harrow, as per the specific treatment requirements. To ensure proper mixing, two rounds of harrowing were carried out. Before incorporating the green manure crops into the soil, a substantial irrigation of 10 cm was applied to the land. The field was then subjected to two rounds of disk ploughing, followed by three cycles of puddling using a puddler in the presence of standing water. At the final round of puddling, 26 kg P ha–1 in the form of single superphosphate and 33 kg K ha–1 in the form of muriate of potash were evenly distributed. For the application of nitrogen, prilled urea (manufacturer: KRIBHCO) and zinc–coated urea were utilized, which were divided into three equal portions. One–third of the nitrogen was applied as a basal dose, another third at the 50% tillering stage, and the remaining third at the panicle initiation stage. In both years of the study, during the first fortnight of July, the Basmati rice variety ‘Pusa Basmati 6’, aged 25 days was transplanted into the field at a spacing of 20 cm × 10 cm. Throughout the experiment, the rice crop was cultivated following the recommended package of practices. Detailed information regarding the schedule of crop establishment followed during both years of experimentation can be found in Table 4.
2.5 Determination of physiological indices, yield and economics
Plant height for Basmati rice was measured at regular 30–day intervals until harvest using a standard metre scale and recorded in centimetres (cm) from the base of the plant at the ground surface to the tip of the tallest leaf. The amount of dry matter accumulated by Basmati rice on five different hills was monitored at 30–day intervals. After being air dried, these plants went into a hot air oven set to 60 ± 2°C to continue the drying process until a constant weight was achieved. The data was collected using a dry weight scale and expressed in g hill–1. The surface area of the leaves was determined by removing them from the plant, washing them in deionized water, and drying them on paper towels. Leaf area was measured using a leaf area metre (Model LICOR 3000, USA) and results were given in square centimetres per plant unit of foliage. The leaf area index (LAI) was determined at 30, 60, and 90 DAT using (Evans, 1972). To determine the crop growth rate (CGR) in a given area and over a given time period, the following formula (Watson, 1952) was used:
Where, W1 and W2 are the dry weight (g) values at time T1 and T2, respectively. T1 and T2 are time in days after transplanting. The photosynthetic efficiency of leaves is measured by the net assimilation rate (NAR), which is the gain in assimilate per unit leaf area over time (Watson, 1952):
Where, W1 and W2 are dry weights and LA1 and LA2 are leaf area values recorded at times T1 and T2, respectively. The RGR was also computed as per the procedure of (Watson, 1952).
The seeds were washed, dried in the sun, and weighed after threshing. The yields were transformed to tonnes per hectare. Before the plants were threshed, their weight was recorded after they had been dried in the sun. The yield of straw was calculated by subtracting the weight of the seeds from the total. The yields of both grain and straw were reported in tonnes per hectare. The market price of various inputs was used to estimate the cost of cultivation for various green manuring and Zn fertilization strategies. Net returns were determined by subtracting the cost of cultivation from the gross returns, which were determined by calculating the grain and straw yield and the prevailing market prices of grain and straw in respective seasons. By dividing net returns by the cost of cultivation, the benefit–cost ratio was computed.
2.6 Chemical analysis
Plant specimens harvested from the field were subjected to natural sun–drying, followed by oven–drying at a controlled temperature of 60 ± 2°C for a six–hour duration. Subsequently, the samples were pulverized. Analytical chemistry techniques were employed on 0.5–gram portions extracted from the rice grain and straw. The concentration of micronutrients in the dry matter was assessed utilizing di–acid digestion protocols, and quantification was performed through atomic absorption spectrophotometer (model: Elements AS AAS4141, ECIL, Hyderabad, India) (Prasad et al., 2006). Nutrient uptake metrics were then determined by multiplying these concentrations with the dry weight, with results expressed in grams per hectare (g ha–1).
2.7 Statistical analysis
A pooled analysis was performed on data collected over a span of two years, and the averages were reported. The statistical analyses were executed using R (version 4.2.2) in conjunction with R–Studio (version 2022.12.0 + 353), utilizing the “agricolae” package (de Mendiburu and de Mendiburu, 2019). To evaluate the statistical significance between different treatment groups, a 5% significance level was adopted. Further, Duncan’s Multiple Range Test (DMRT) was employed as a post–hoc analysis for categorizing treatment means (Gomez and Gomez, 1984).
3 Results
3.1 Growth and physiological indices of Basmati rice
The impact of summer green manuring (GM) and zinc fertilization (ZnF) on various growth metrics of Basmati rice was found to be statistically significant, as evidenced in Table 5 and Figures 1, 2. Distinctive responses to GM were observed in relation to key parameters such as plant height, dry matter content, leaf area index, crop growth rate (CGR), and net assimilation rate (NAR) (Table 5 and Figures 1, 2). Among the GM crops, Sesbania (G2) yielded significantly taller plants compared to the cowpea GM treatment (G3) at the time of harvest, with the control group exhibiting the minimum plant height (Figure 1). Sesbania (G2) also surpassed other GM treatments in terms of dry matter accumulation at harvest (Figure 2) and leaf area index at 60 days after transplanting (DAT) (Table 5). Regarding CGR and NAR metrics, Sesbania (G2) demonstrated superior performance during the trial period (Table 5). While zinc fertilization strategies also yielded a significant effect, particularly on plant height and leaf area index, the 1% BZnCU (ZnF4) regime yielded higher values at both flowering and harvesting stages. Remarkably, the synergistic implementation of Sesbania GM (G2) and 0.2% NZnCU (ZnF6) yielded elevated CGR and NAR values between 30–60 DAT, and this was statistically comparable to results from 1% BZnCU (ZnF4) application (Table 5). On a comparative scale, Sesbania GM (G2) elevated the dry matter content by approximately 12.2% over the control group at the point of harvest. The growth and physiological traits of Basmati rice were markedly influenced by both the integration of green manure crop residues and different zinc fertilization approaches. Amongst various GM alternatives, Sesbania (G2) consistently outperformed others in key growth indicators like plant height, leaf area index, and dry matter content at almost all growth stages, except for leaf area index at 30 DAT. Additionally, cowpea GM (G3) also contributed positively to the growth metrics of the Basmati rice plants. Conversely, the least growth was observed when Basmati rice was grown following a summer fallow regime (G1). The hierarchy of efficacy among different zinc sources was as follows: 1% BZnCU (ZnF4) = 0.2% NZnCU (ZnF6) > 0.1% NZnCU (ZnF5) > nitrogen via prilled urea + 5 kg Zn ha–1as bulk ZnO (ZnF3) > nitrogen via prilled urea (ZnF2) > 5 kg Zn ha–1 as bulk ZnO (ZnF1).
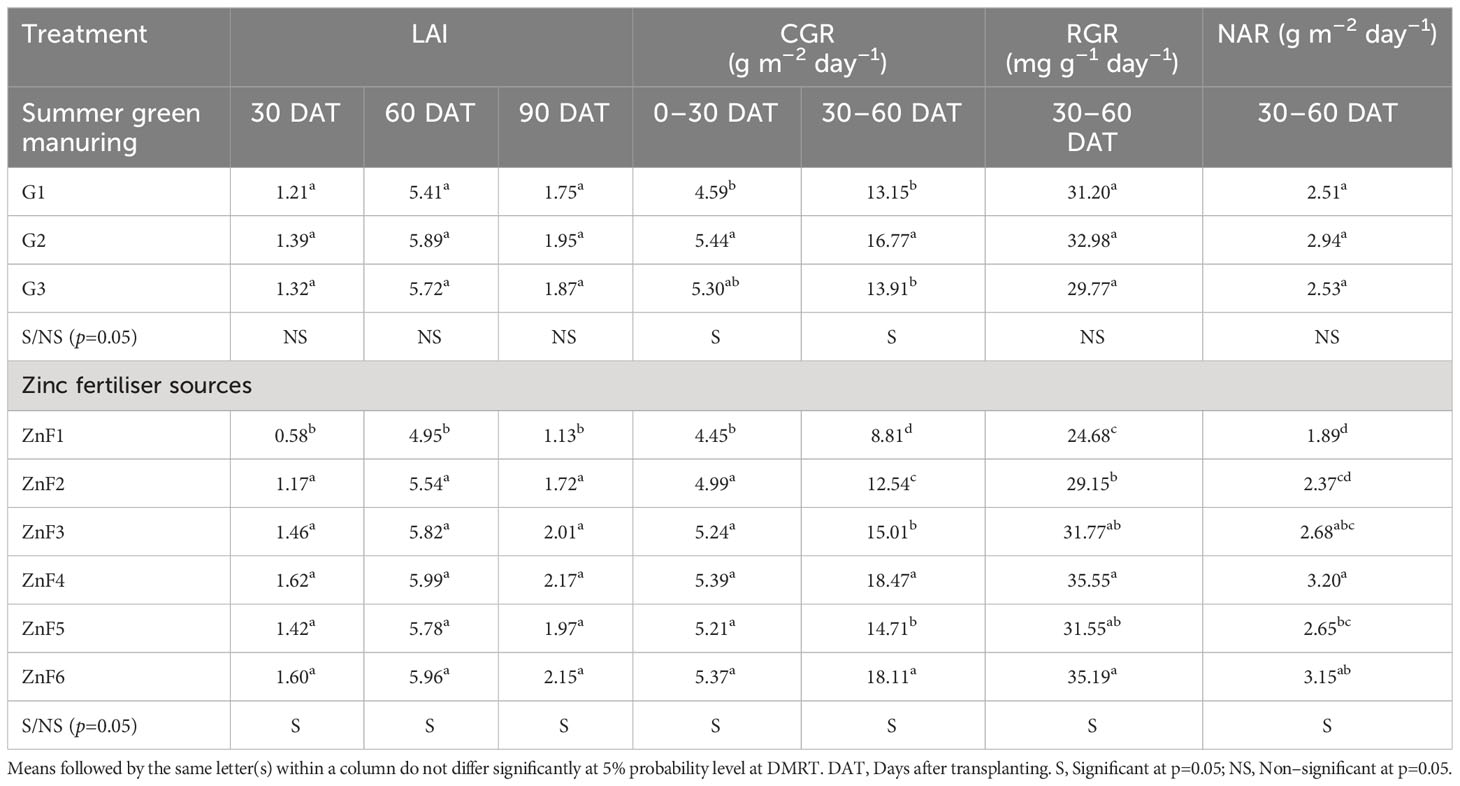
Table 5 Effect of summer green manuring and Zn fertilizer sources on various physiological indices of Basmati rice (pooled data of 2 years).
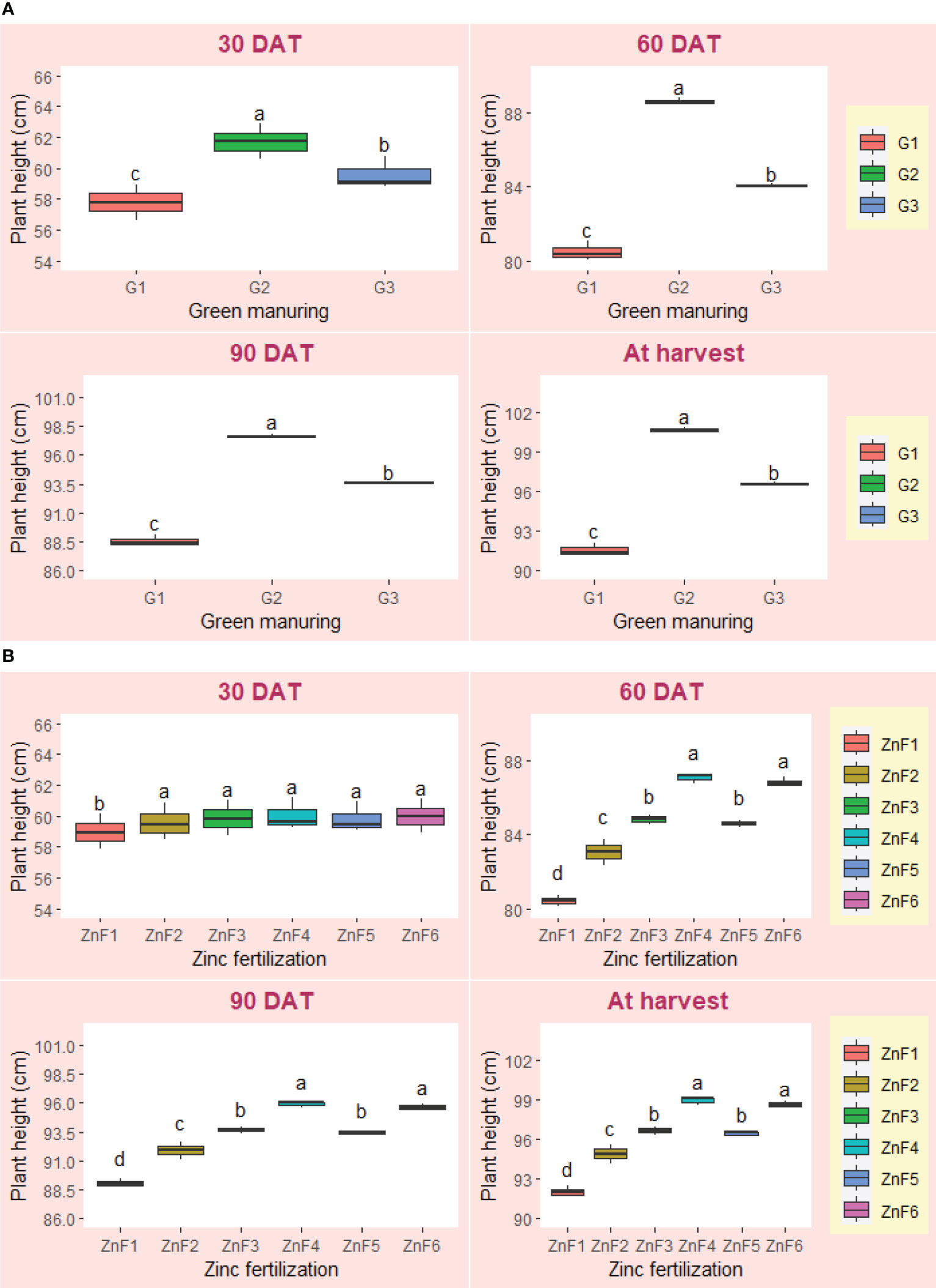
Figure 1 (A) Effect of summer green manuring on the plant height (cm) of Basmati rice (pooled data of 2 years). (B) Effect of Zn fertilizer sources on the plant height (cm) of Basmati rice (pooled data of 2 years). Boxplot of same letter(s) don’t differ significantly at 5% probability level by DMRT. DAT, Days after transplanting.
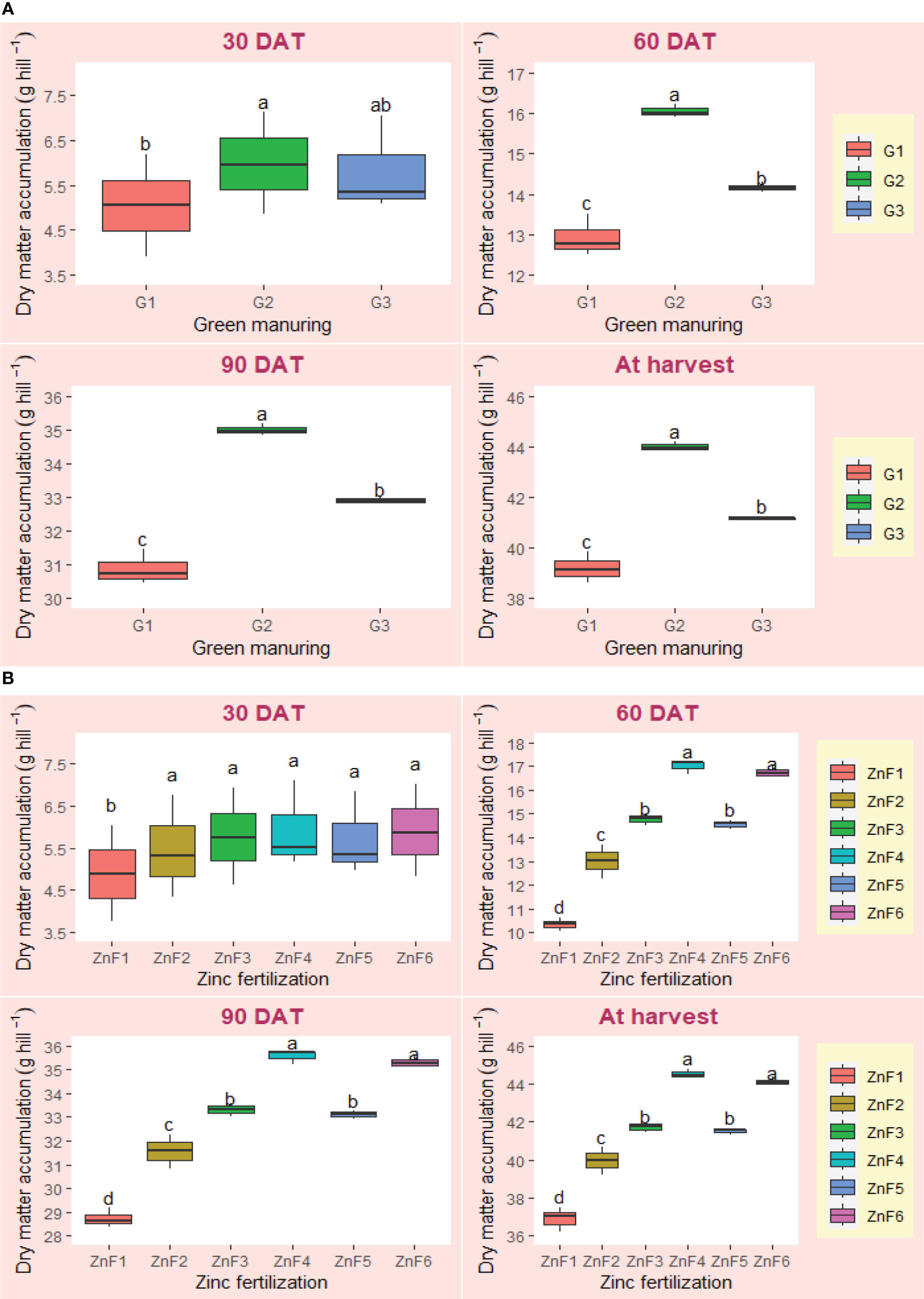
Figure 2 (A) Effect of summer green manuring on the dry matter accumulation (g hill–1) of Basmati rice (pooled data of 2 years). (B) Effect of Zn fertilizer sources on the dry matter accumulation (g hill–1) of Basmati rice (pooled data of 2 years). Boxplot of same letter(s) don’t differ significantly at 5% probability level by DMRT. DAT, Days after transplanting.
3.2 Yields and harvest index of Basmati rice
The employment of nano zinc sources along with summer green manuring (GM) exhibited a pronounced influence on the yields of Basmati rice, as depicted in Figures 3, 4. The incorporation of Sesbania (G2) as a green manure during the summer season led to significantly enhanced grain and straw yields, outperforming both cowpea (G3) and summer fallow (G1). Specifically, the 1% BZnCU (ZnF4) zinc fertilization regime delivered superior grain yield (4.47 t ha–1) and harvest index (32.1%), which were statistically on par with the outcomes from 0.2% NZnCU (ZnF6). The second most effective treatment was the 0.1% NZnCU application in terms of both grain and harvest index, as illustrated in Figure 3. In the context of performance metrics, the zinc sources manifested a hierarchical order of effectiveness: 1% BZnCU (ZnF4) = 0.2% NZnCU (ZnF6) > 0.1% NZnCU (ZnF5) > nitrogen supplied through prilled urea with 5 kg Zn ha–1 as bulk ZnO (ZnF3) > nitrogen supplied through prilled urea alone (ZnF2) > 5 kg Zn ha–1 as bulk ZnO (ZnF1), as shown in Figure 3. The application of 0.2% NZnCU (ZnF6) led to a grain yield and harvest index increase of 36.9% and 12.6%, respectively, over the control condition involving 5 kg Zn ha–1 as bulk ZnO. Correlation analyses between grain yield and various physiological indicators, as well as zinc content in the dry matter of both milled rice and straw, revealed intriguing patterns. A moderate but statistically significant linear correlation was observed between the leaf area index (LAI) at 60 days after transplanting (DAT) and grain yield (r=0.805, p=<0.001). Conversely, the correlations between grain yield and LAI at 30 DAT (r=0.078, p=< 0.05) and 90 DAT (r=0.035, p=<0.05) were not significant, as illustrated in Figure 5. Notably, there was a highly significant linear relationship between zinc content in the dry matter of straw (r=0.805, p=<0.001) and milled rice (r=0.726, p=<0.001) with grain yield (Figure 5). Subsequent regression analyses indicated that various factors accounted for significant variability in grain yield. Specifically, LAI at 60 DAT explained 99% of the variability (R²=0.99), followed by mean crop growth rate (CGR) 0–30 DAT (R²=0.89) and 30–60 DAT (R²=0.93), mean relative growth rate (RGR) 30–60 DAT (R²=0.85), mean net assimilation rate (NAR) 30–60 DAT (R²=0.89), and zinc content in the dry matter of milled rice (R²=0.76) and straw (R²=0.64), as elucidated in Figures 6–8.
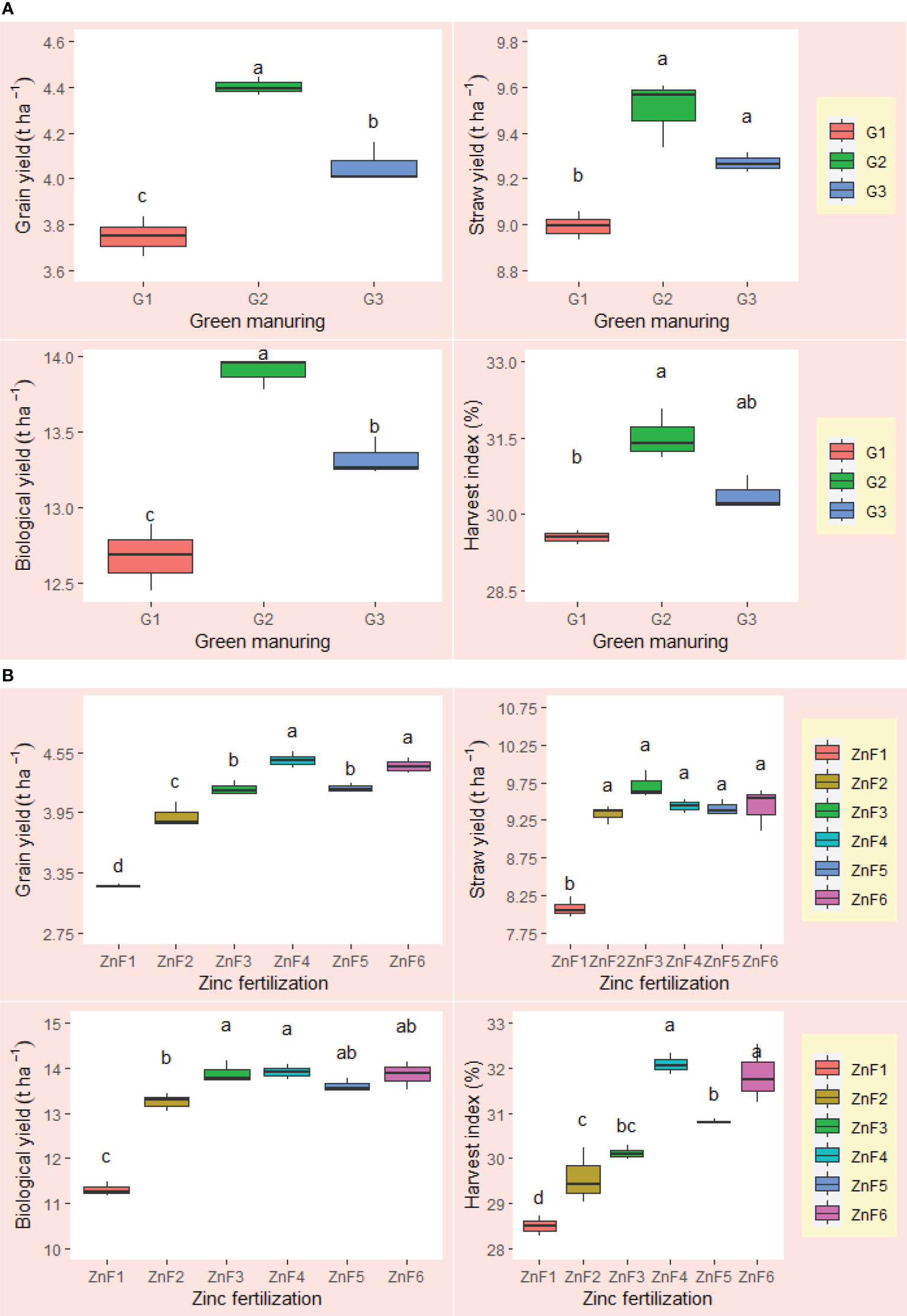
Figure 3 (A) Effect of summer green manuring on the yields and harvest index of Basmati rice (pooled data of 2 years). (B) Effect of Zn fertilizer sources on the yields and harvest index of Basmati rice (pooled data of 2 years). Boxplot of same letter(s) don’t differ significantly at 5% probability level by DMRT.
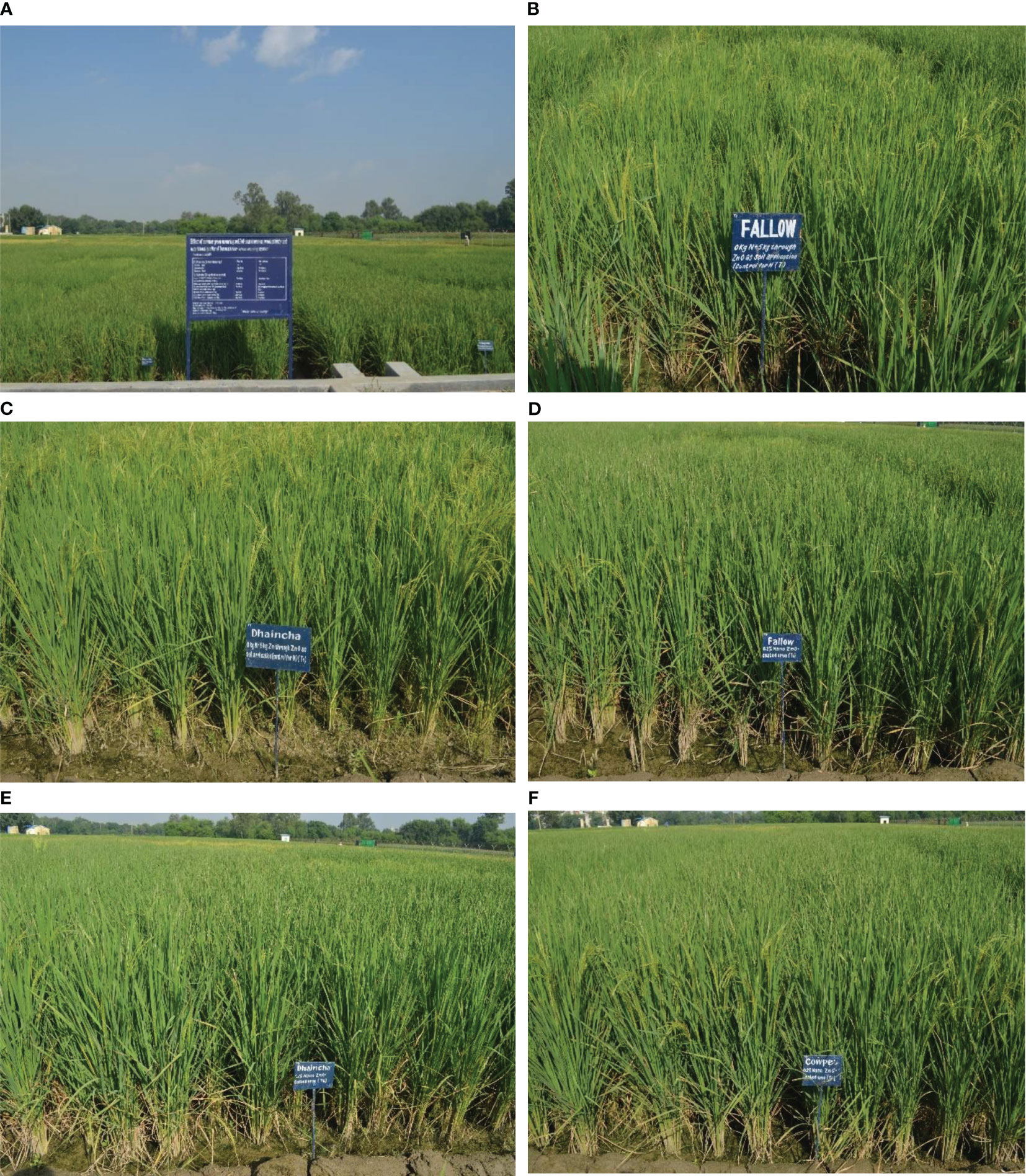
Figure 4 (A) Experimental field view. (B) Fallow+ 5 kg Zn ha–1 as bulk ZnO. (C) Sesbania + 5 kg Zn ha–1 as bulk ZnO. (D) Fallow + 0.2% NZCU. (E) Sesbania + 0.2% NZCU. (F) Cowpea + 0.2% NZCU.
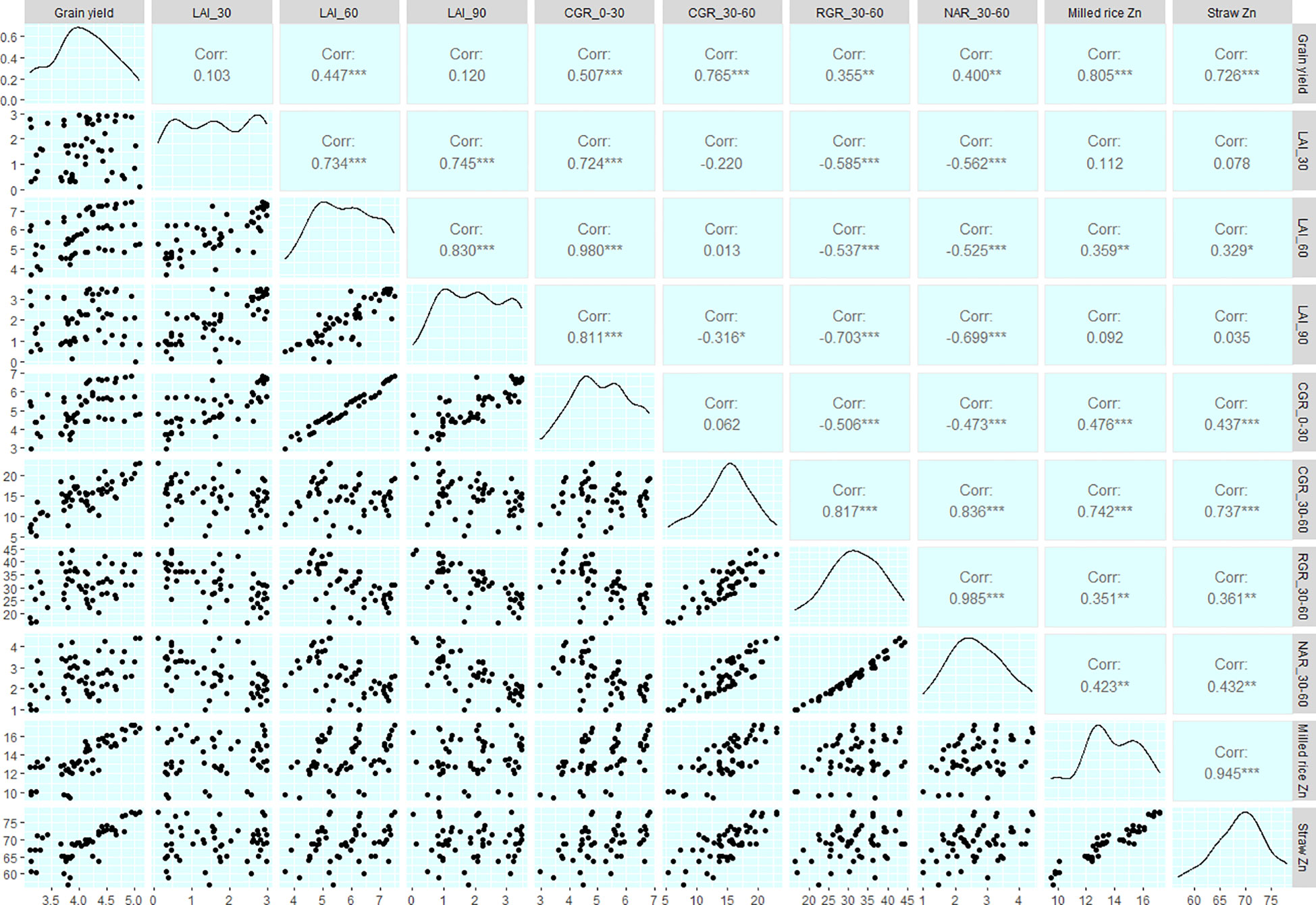
Figure 5 Correlation matrix depicting correlation co–efficient (upper half), density plot (diagonal) and scatter plot (lower half). LAI_30, Leaf area index at 30 DAT; LAI_60, Leaf area index at 60 DAT; LAI_90, Leaf area index at 90 DAT; CGR_0–30, Mean CGR 0–30 DAT; CGR_30–60, Mean CGR 30–60 DAT; RGR_30–60, Mean RGR 30–60 DAT; NAR_30–60, Mean NAR 30–60 DAT; DAT, Days after transplanting; *, Significant at p=0.05; **, Significant at p=0.01; ***, Significant at p=0.001.
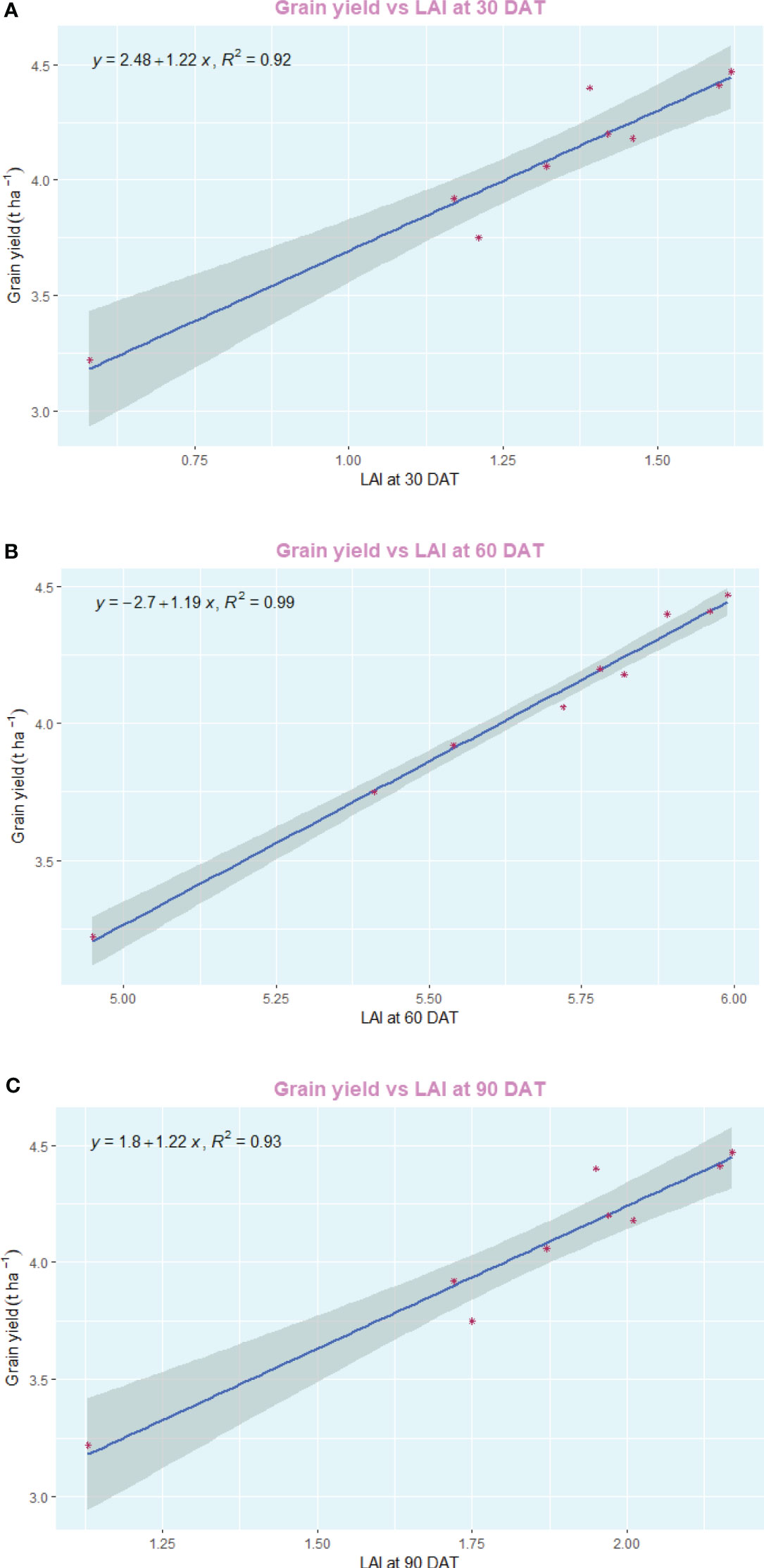
Figure 6 (A) Regression between grain yield and LAI at 30 DAT. (B) Regression between grain yield and LAI at 60 DAT. (C) Regression between grain yield and LAI at 90 DAT. DAT, Days after transplanting.
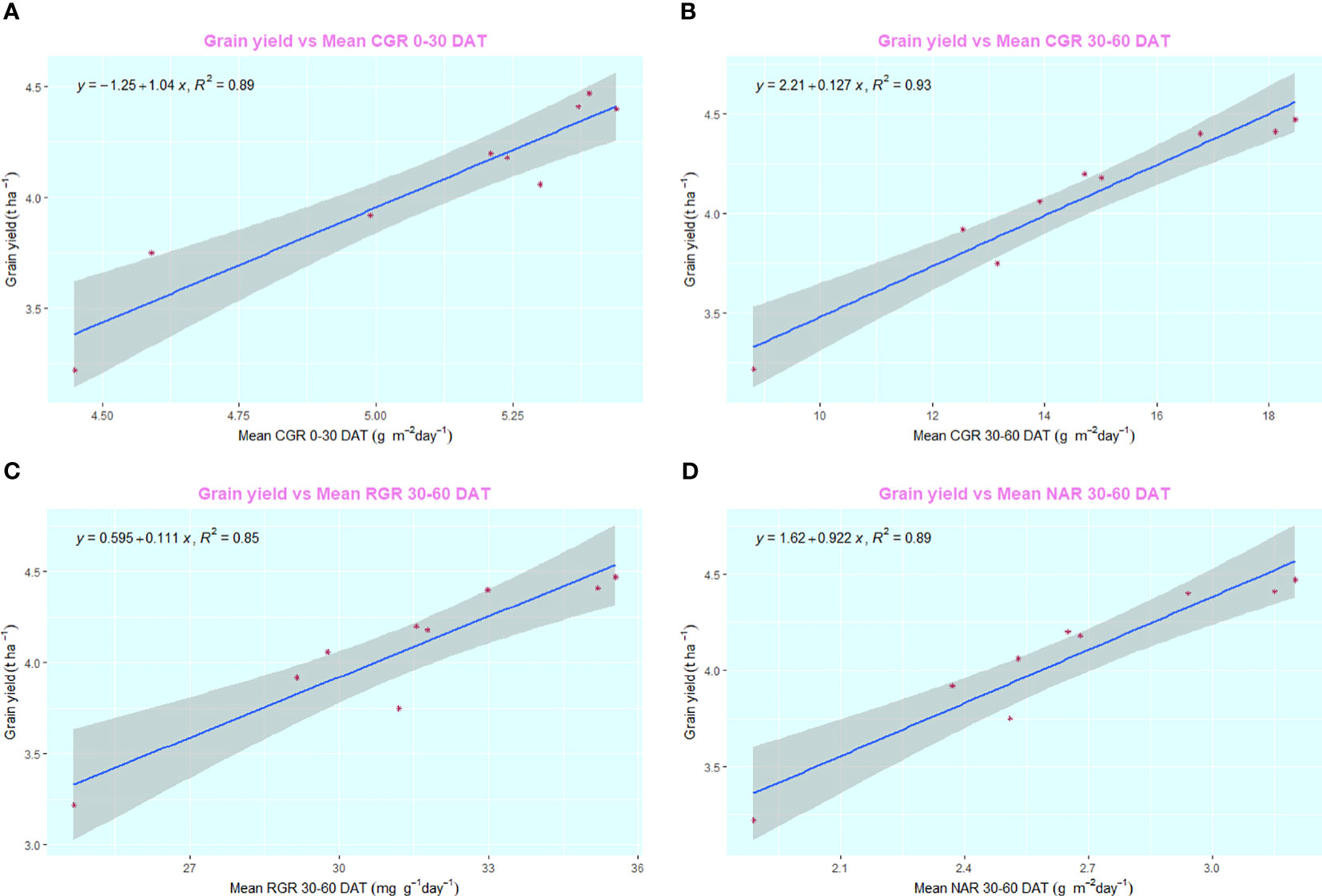
Figure 7 (A) Regression between grain yield and mean CGR 0–30 DAT. (B) Regression between grain yield and mean CGR 30–60 DAT. (C) Regression between grain yield and mean RGR 30–60 DAT. (D) Regression between grain yield and mean NAR 30–60 DAT. DAT, Days after transplanting.
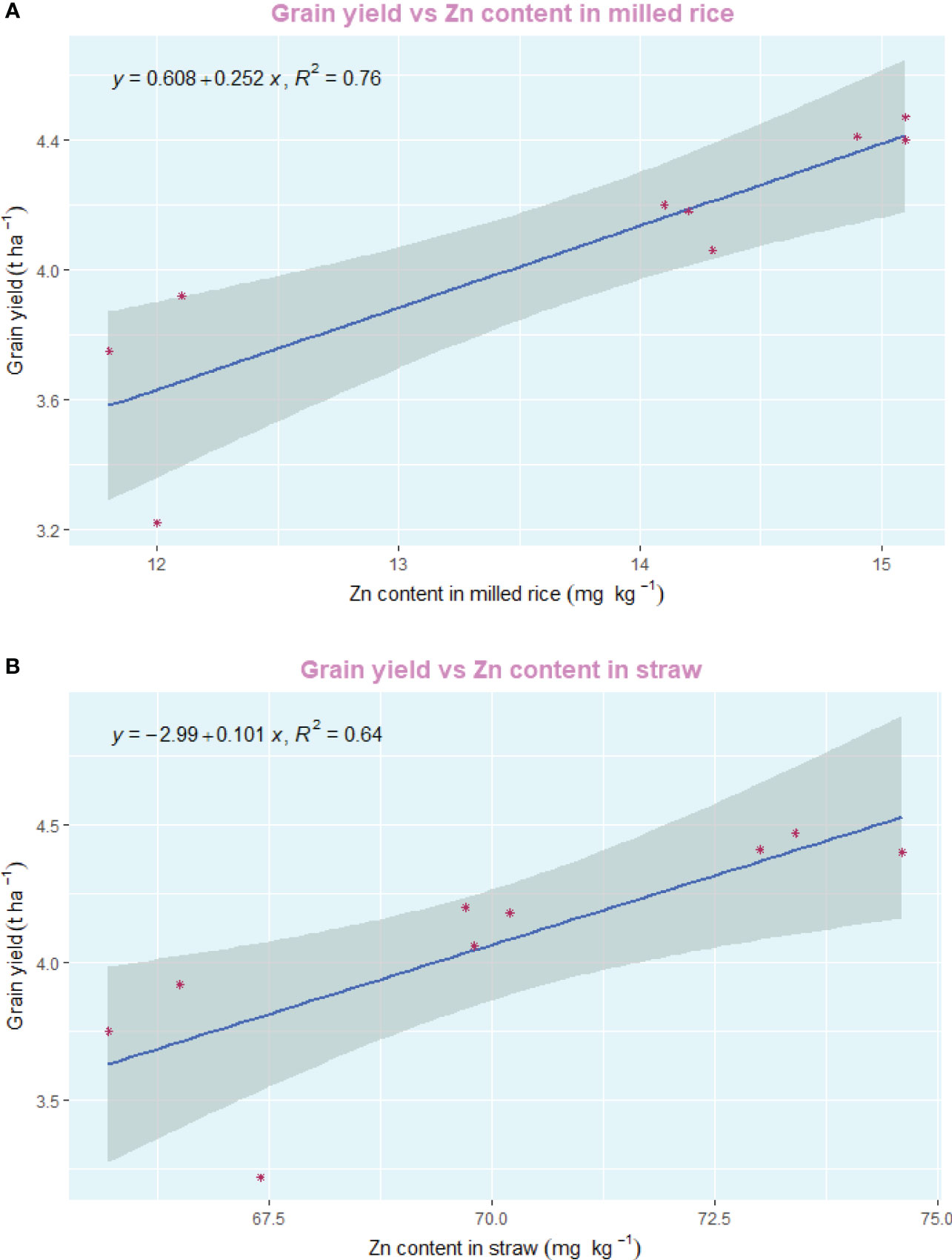
Figure 8 (A) Regression between grain yield and Zn content in milled rice. (B) Regression between grain yield and Zn content in straw.
3.3 Zinc nutrition
The investigation into the effects of green manures (GM) and zinc formulations (ZnF) on zinc nutrition of rice revealed that, marked variations were noted in zinc (Zn) concentrations within both milled rice and straw, overall zinc uptake, and the zinc harvest index (ZnHI). Across various GM applications, straw consistently outperformed milled rice in Zn concentrations (Figure 9), showcasing a range of 65.7–74.6 mg kg–1 in straw as opposed to a narrower 11.8–15.1 mg kg–1 spectrum in milled rice. In the realm of GM, Sesbania green manuring (G2) was identified as a superior performer, eclipsing fallow management (G1) in all measured Zn–related metrics across two years research period (Figure 9). The highest Zn content of 15.1 mg kg–1 and the lowest of 11.8 mg kg–1 in straw was recorded in Sesbania green manuring (G2) and control i.e., in the fallow (G1), respectively (Figure 9). When data were harmonized over various ZnF scenarios, G2 outperformed a noteworthy upswing in Zn metrics by 27.96%, 13.54%, 23.5%, and 10.18% in milled rice, straw, total Zn uptake, and ZnHI, respectively, relative to G1. In a parallel trend, cowpea green manuring (G3) also yielded Zn enrichment, albeit at a comparatively subdued scale. Strikingly, among the ZnF regime, 1% BZnCU (ZnF4) excelled, demonstrating comparable Zn enrichment capabilities with 0.2% NZnCU (ZnF6) in both grain and straw. The latter ZnF regime notably amplified Zn concentration in milled rice, straw, total Zn uptake, and ZnHI by increments of 24.16%, 8.30%, 30.0%, and 12.26%, respectively over sole application of bulk ZnO. The study further elucidated that an integrative approach, combining zinc with urea, either as a coating or through concurrent application, produced a synergistic uptick in Zn metrics. This conjoint strategy was notably more efficacious than singular urea applications or the standalone deployment of 5 kg Zn ha–1 via bulk ZnO. Such integrated methodologies bolstered Zn levels in milled rice, straw, total Zn absorption, and ZnHI by 20.41%, 7.62%, 15.91%, and 9.52%, respectively, over sole application of bulk ZnO.
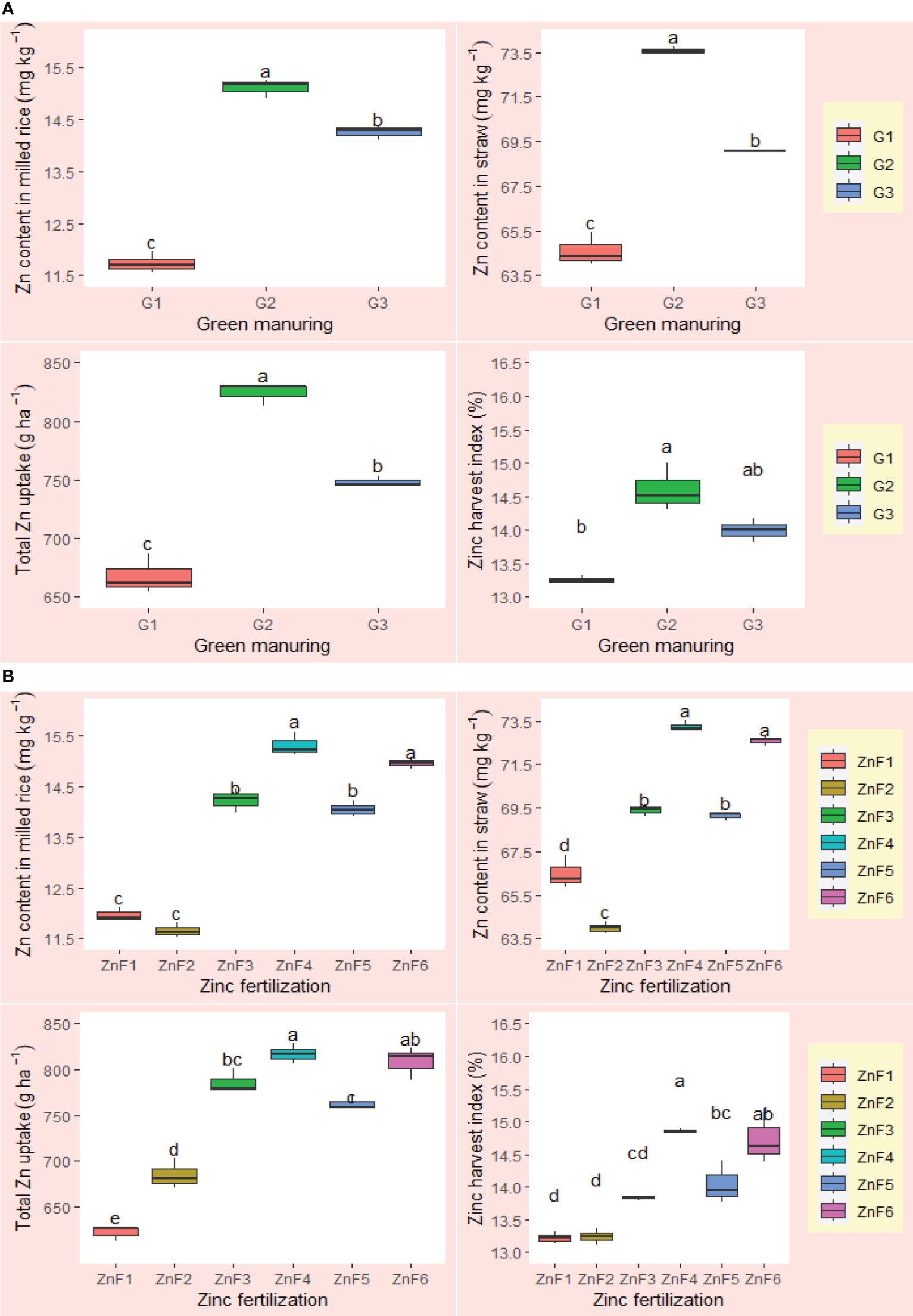
Figure 9 (A) Effect of summer green manuring on the zinc nutrition of Basmati rice (pooled data of 2 years). (B) Effect of Zn fertilizer sources on the zinc nutrition of Basmati rice (pooled data of 2 years). Boxplot of same letter(s) don’t differ significantly at 5% probability level by DMRT.
3.4 Economics
In an economic analysis of Basmati rice cultivation, the financial metrics were notably influenced by the use of green manures and nano–zinc fertilization techniques (Table 6). Despite the premium seed cost associated with Sesbania green manuring (G2), this practice demonstrated better performance in delivering superior gross and net returns as well as an improved benefit–cost Ratio (net B:C) over the evaluation period. In particular, fields conditioned with Sesbania (G2) demonstrated superior yield performances compared to those with cowpea (G3) or fallow conditions (G1), thereby explaining its higher economic efficacy. Cowpea manuring (G3), although beneficial, lagged behind Sesbania in terms of all major economic indicators. Moreover, the financial robustness of Basmati rice cultivation was significantly swayed by different nano Zn fertilization regimes (Table 6). The application of 1% BZnCU (ZnF4) conspicuously helped in achieving noteworthy gross and net returns, as well as net B:C. Intriguingly, this performance was statistically indistinguishable from that of 0.2% NZnCU (ZnF6), with 0.1% NZnCU (ZnF5) regime is the second best. On deploying 0.2% NZnCU (ZnF6), a significant uptick was observed in both gross returns and net B:C by an average of 33.33% and 0.57, respectively than fallow.Consequently, a synergistic approach incorporating 0.2% NZnCU (ZnF6) fertilization coupled with Sesbania green manuring (G2) during the summer season presents a lucrative agronomic strategy for farmers grappling with zinc–deficient soils, offering a pathway to both sustainable productivity and optimized economic returns.
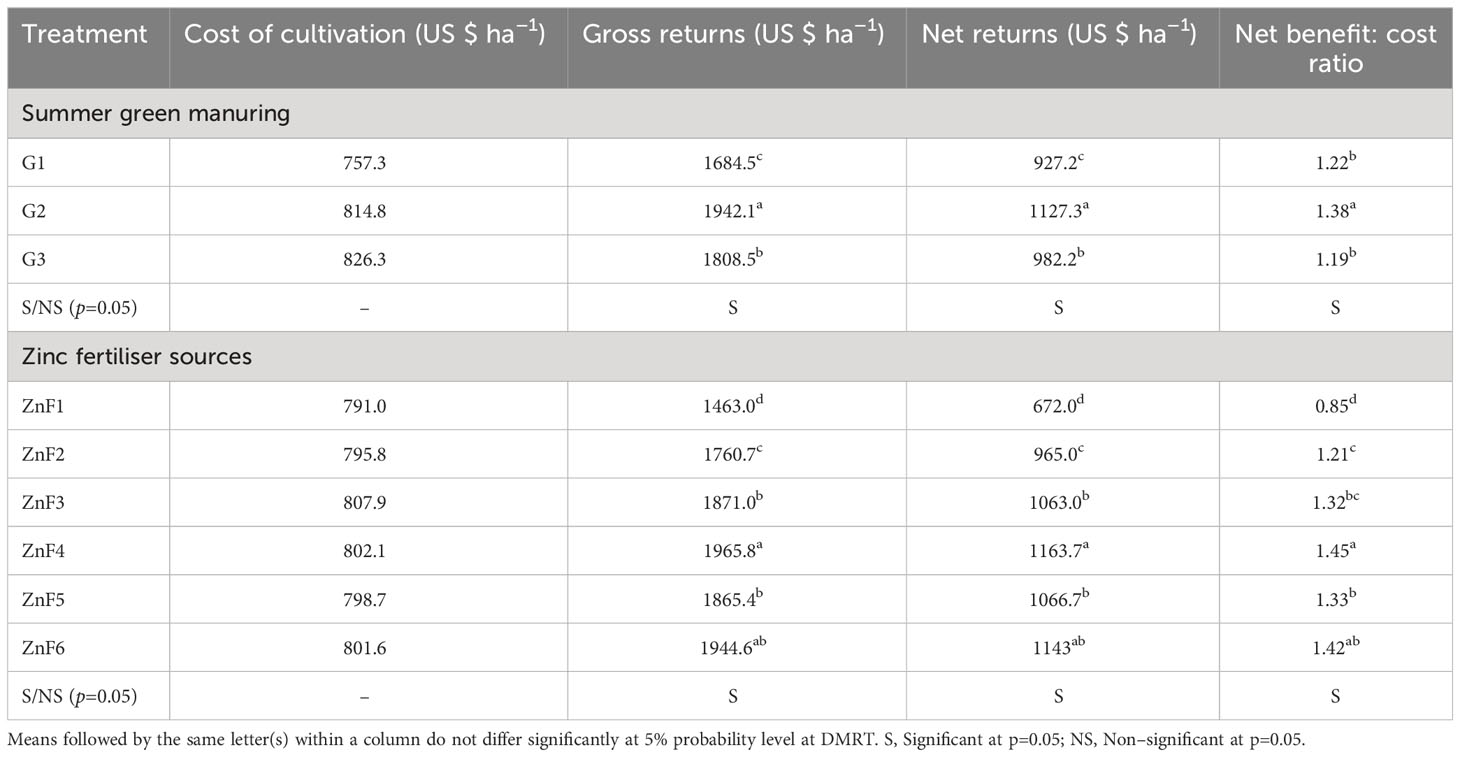
Table 6 Effect of summer green manuring and Zn fertilizer sources on economic returns of Basmati rice (pooled data of 2 years).
4 Discussion
Peak leaf area index (LAI) values were observed at 60 days after transplantation (DAT), with value between 4.95 and 5.89. This leaf expansion is primarily due to the leaves reaching their full developmental and functional potential at this stage, eclipsing the leaf areas recorded at both 30 and 90 DAT. These observations are in harmony with previous research (Singh and Shivay, 2014). The differential treatment response can be linked to several factors: enhanced nutrient bioavailability, favorable modifications in soil physicochemical properties due to green manure infusion, and the elevated zinc bioavailability as a result of nano Zn fertilizer applications. These contributing factors likely accounted for the variations observed in plant growth metrics across different treatments. Additionally, the superior dry matter accrual observed in plants treated conjointly with urea and nano–zinc can be ascribed to the amplified LAI and crop growth rate (CGR) during the grain–filling phases. The vital role of zinc in chlorophyll biosynthesis has been corroborated by other studies (Sadak and Bakry, 2020). Evidence indicates that nano–Zn formulations enhance Basmati rice growth metrics due to their increased bioavailability compared to alternative zinc sources. Zinc, being critical for auxin synthesis, can influence plant height, as noted in research (Alloway, 2008). Furthermore, studies (Taran et al., 2017) have highlighted the stabilizing effect of ZnO nanoparticles on photosynthetic pigments, thereby boosting the plant’s photosynthetic capabilities. In the control plots, the stunted growth parameters could be attributed to soil submergence conditions, which precipitate the formation of insoluble zinc compounds, subsequently inhibiting plant zinc uptake and constraining growth. On the flip side, the superior zinc bioavailability in soils treated with 0.2% NZnCU (ZnF6) might result from limited soil interactions, thereby avoiding the common soil reactions seen with traditional zinc sources. The incorporation of Sesbania (G2) and cowpea (G3) green manures contributed dry biomass of approximately 5.2 and 3.43 t ha–1, and 4.98 and 3.24 t ha–1 in the first and second years of the study, respectively (Supplementary Figure S1). Particularly, Sesbania’s inclusion introduced readily decomposable organic matter, elevating the soil’s organic content and nutrient profile, which synergistically enhanced Basmati rice yield. The surge in grain and straw yields from the conjoint use of summer green manuring and nano–Zn fertilization can be elucidated through increased vegetative and physiological indices. For instance, the observed yield augmentation as a result of zinc fertilization (ZnF) is likely attributable to zinc–induced boosts in dry matter accumulation—evidenced by high LAI at 60 DAT—and other physiological parameters, as supported by a high R2 value in linear regression analyses. Moreover, this zinc–enhanced yield is also substantiated by the elevated zinc levels in both the straw and grain dry matter, further corroborated by high R2 values.
The current escalation in crop yield is closely linked to the zinc–induced augmentation of chlorophyll synthesis, corroborating findings from previous studies (Patel et al., 2022) that also attributed yield–enhancing attributes to improved photosynthetic efficiency. This study’s revelations, highlighting the efficacy of nano–zinc oxide in bolstering rice grain output, resonate with established literature on the subject (Kheyri et al., 2019; Adhikary et al., 2022). The amplification in both yield and harvest index in Basmati rice upon the utilization of nano–zinc–infused urea is likely driven by elevated zinc assimilation, subsequently fueling biomass expansion (Pooniya et al., 2012; Shivay and Prasad, 2012). Further, zinc’s role in facilitating the translocation of photosynthates amplifies these outcomes, aligning with earlier findings (Pavithra et al., 2017) that identified nano–zinc as a potent alternative for rice cultivation enhancement. Incorporating green manure crops prior to Basmati rice transplantation enriches the soil’s organic matter pool. This organic enrichment may have catalyzed the complexation of applied zinc with soil–bound organic compounds, thereby circumventing the formation of less bioavailable, insoluble zinc complexes. This leads to heightened zinc accessibility to the rice plants, culminating in superior growth and productivity metrics. Earlier research also substantiates the positive influence of zinc–fortified urea on plant physiology and yields (Shivay et al., 2008). The harmonious interplay between nitrogen and zinc availability likely triggered a synergistic crop response, eventually steering toward an elevated grain yield, a trend consistent with earlier research (Cahill et al., 2010). In the current analysis, zinc application was found to enrich the harvest index. Such improvements are arguably mediated by the zinc–induced surge in leaf area index, mean net assimilation rate (NAR) between 30 and 60 DAT, and overall dry matter accumulation. Comparatively, the study found ZnO NPs to be more efficacious than their bulk counterparts, with coated urea formulations also outpacing their uncoated equivalents. Typical coated fertilizers function as nutrient slow–release mechanisms, modulating the nutrient release rate from the encapsulating compound. On the other hand, non–coated urea tends to exhibit rapid nutrient release kinetics due to its higher solubility. Bulk ZnO displays quick dissolution upon aqueous contact (Shivay et al., 2008), whereas ZnO NPs, owing to their minute size and elevated surface area, adhere to the urea surface, exhibiting a gradual release. Furthermore, nano ZnO coatings comprise finely dispersed particles, while bulk ZnO tends to form particle agglomerations, adding another layer of complexity to their respective performance.
In the current investigation, the utilization of nano–sized zinc significantly outperformed its bulk ZnO counterpart in enhancing zinc assimilation within rice tissues. The nanoparticles, owing to their expansive surface area and elevated reactivity, acted as catalysts for superior zinc absorption and bioavailability (Liu et al., 2016). Applying urea coated with ZnO to the soil demonstrated an uptick in the activity levels of both urease and phosphatase enzymes, which likely facilitated a greater soil–based zinc availability, ultimately benefitting the plant’s edible parts (Abdullah et al., 2022). Interestingly, due to their high surface area and improved solubility, ZnO NPs resulted in amplified zinc concentrations within plant tissues when compared to bulk ZnO (Prasad et al., 2012). This higher zinc levels in milled rice might be attributed to the xylem–based translocation of ZnO NPs from the roots to the aerial parts and eventually to the grains (Pérez–de–Luque, 2017). Prior studies have also demonstrated the efficacy of zinc–fortified urea in enhancing zinc uptake in other cereal crops like wheat (Dimkpa et al., 2020) and maize (Pooniya et al., 2017). In this study, a noteworthy anomaly surfaced: lower zinc concentrations were observed in both milled rice and straw from lone urea–treated rice as opposed to those treated with soil–applied bulk ZnO at 5 kg Zn ha–1. This phenomenon can likely be rationalized by the dilution effect (Jarrell and Beverly, 1981). Significantly, the straw component served as the principal zinc repository within the rice plant, displaying zinc levels that were multiple times greater than those in grains. Our initial hypothesis, positing an enhanced zinc uptake from zinc–coated urea samples, was corroborated (Liu et al., 2016). Elevated grain yields were attributed to the greater zinc bioavailability from nanoparticle–treated plots compared to controls and bulk ZnO applications. In alignment with our hypothesis, urea coated with 0.2% NZnCU appeared to stimulate the functionality of various plant–based zinc transporters, thereby resulting in augmented zinc levels in both milled rice and straw. Overall, the observed differences across treatments can be credited to both the residual impact of green manuring (GM) on the rice crop, as well as the beneficial influence of zinc fertilization. Thus, the strategic incorporation of ZnO NPs in this study underscores their potential to minimize fertilizer inputs without compromising either crop yield or nutritional integrity.
5 Conclusion
Relative to alternative green manuring sources, the physiological growth and development of Basmati rice exhibited superior performance when augmented with Sesbania as a pre–transplantation green manure. Further enhancement in both grain and straw yields was achieved when this organic manuring strategy was synergistically combined with nano–zinc (NZnCU) fertilization at a concentration of 0.2%. These results suggest that coupling nano–zinc fertilization with the incorporation of summer green manures like Sesbania in Basmati rice could enhance growth trajectories and productivity and may offer a robust framework for nutrient management. Such a strategy could be advantageous in intensively–cultivated rice ecosystems, promising not only superior growth and nutritional outcomes but also enhanced economic viability.
Data availability statement
The original contributions presented in the study are included in the article/Supplementary Material. Further inquiries can be directed to the corresponding author.
Author contributions
KB, YSS, and RP led the research work, planned, supervised, and conducted field experiments, and read and edited the manuscript. KB, SM, SN, and KR collected soil, plant samples and performed chemical analysis, also wrote the initial draft of the manuscript, and prepared figures and tables. RP, YSS, DK and CS project supervision, reviewed, read, and edited the manuscript with significant contribution. All authors contributed to the article and approved the submitted version.
Funding
The author(s) declare that no financial support was received for the research, authorship, and/or publication of this article.
Acknowledgments
We acknowledge the ICAR–Indian Agricultural Research Institute and the Indian Council of Agricultural Research, New Delhi, India for the financial support and necessary facilities.
Conflict of interest
The authors declare that the research was conducted in the absence of any commercial or financial relationships that could be construed as a potential conflict of interest.
Publisher’s note
All claims expressed in this article are solely those of the authors and do not necessarily represent those of their affiliated organizations, or those of the publisher, the editors and the reviewers. Any product that may be evaluated in this article, or claim that may be made by its manufacturer, is not guaranteed or endorsed by the publisher.
Supplementary material
The Supplementary Material for this article can be found online at: https://www.frontiersin.org/articles/10.3389/fpls.2023.1283588/full#supplementary-material
Supplementary Figure 1 | Dry matter accumulation (t ha–1) by summer green manuring crops.
References
Abdullah, B., Niazi, M. B. K., Jahan, Z., Khan, O., Shahid, A., Shah, G. A., et al. (2022). Role of zinc–coated urea fertilizers in improving nitrogen use efficiency, soil nutritional status, and nutrient use efficiency of test crops. Front. Envi Sci. 10. doi: 10.3389/fenvs.2022.888865
Adhikary, S., Biswas, B., Chakraborty, D., Timsina, J., Pal, S., Tarafdar, J. C., et al. (2022). Seed priming with selenium and zinc nanoparticles modifies germination, growth, and yield of direct–seeded rice (Oryza sativa L.). Sci. Rep. 12, 7103. doi: 10.1038/s41598-022-11307-4
Alloway, B. J. (2008). Zinc in soils and crop nutrition. 2nd ed. (Brussels: International Zinc Association).
APEDA. (2023). Available at: https://apeda.gov.in/apedawebsite/SubHead_Products/Basmati_Rice.htm (Accessed 15 January, 2023).
Baral, K., Kumar, D., Shivay, Y. S. (2019). Effects of ZnO nano–particles embedded nitrogen, phosphorus and potassium fertilizer on productivity, physical grain quality and nutrient harvest indices of aromatic rice (Oryza sativa). Indian J. Agron. 64 (4), 520–523.
Baral, K., Kumar, D., Shivay, Y. S. (2020). Effect of zinc oxide nanoparticles embedded NPK fertilizer on growth, yield and zinc concentration and uptake of aromatic rice (Oryza sativa). Indian J. Agric. Sci. 90 (8), 1626–1630. doi: 10.56093/ijas.v90i8.105980
Baral, K., Shivay, Y. S., Prasanna, R., Kumar, D., Shrivastava, M., Chakraborty, D., et al. (2023). Interplay between nano zinc oxide–coated urea and summer green manuring in basmati rice: implications on yield response, nutrient acquisition and grain fortification. Front. Sustain Food Syst. 7. doi: 10.3389/fsufs.2023.1187717
Bhatt, R., Kukal, S. S., Busari, M. A., Arora, S., Yadav., M. (2016). Sustainability issues on rice–wheat cropping system. Int. Soil Water Cons Res. 4 (1), 64–74. doi: 10.1016/j.iswcr.2015.12.001
Broadley, M. R., White, P. J., Hammond, J. P., I. Zelko, I., Lux., A. (2007). Zinc in plants. New Phytol. 173 (4), 677–702. doi: 10.1111/j.1469-8137.2007.01996.x
Cahill, S., Osmond, D., Weisz, R., Heiniger, R. (2010). Evaluation of alternative nitrogen fertilizers for corn and winter wheat production. Agron. J. 102 (4), 1226–1236. doi: 10.2134/agronj2010.0095
Cakmak, I. (2008). Enrichment of cereal grains with zinc: agronomic or genetic biofortification? Plant Soil. 302 (1), 1–17. doi: 10.1007/s11104-007-9466-3
Dimkpa, C. O., Andrews, J., Fugice, J., Singh, U., Bindraban, P. S., Elmer, W. H., et al. (2020). Facile coating of urea with low–dose ZnO nanoparticles promotes wheat performance and enhances Zn uptake under drought stress. Front. Plant Sci. 11:168. doi: 10.3389/fpls.2020.00168
Directorate of Economics and Statistics (2020). Pocket Book of Agricultural Statistics (Chennai, India: Directorate of Economics and Statistics).
Gomez, K. A., Gomez, A. A. (1984). “Statistical procedures for agricultural research,” in An International Rice Research Institute Book, 2nd edition (New York: A Wiley–Inter–science Publication, John Wiley & Sons).
Hanway, J. J., Heidel, H. (1952). Soil analysis methods as used in Iowa State College Soil Testing Laboratory. Bull. 57 Iowa State Coll. Agric. p, 131.
Jarrell, W. M., Beverly, R. B. (1981). The dilution effect in plant nutrition studies. Adv. Agron. 34, 197–224. doi: 10.1016/S0065-2113(08)60887-1
Karak, T., Singh, U. K., Das, S., Das, D. K., Kuzyakov, Y. (2005). Comparative efficacy of ZnSO4 and Zn–EDTA application for fertilization of rice (Oryza sativa L.). Arch. Agron. Soil Sci. 51 (3), 253–264. doi: 10.1080/03650340400026701
Kheyri, N., Norouzi, H. A., Mobasser, H. R., Torabi, B. (2019). Effects of silicon and zinc nanoparticles on growth, yield, and biochemical characteristics of rice. Agron. J. 111 (6), 3084–3090. doi: 10.2134/agronj2019.04.0304
Kumar, N., Chhokar, R. S., Meena, R. P., Kharub, A. S., Gill, S. C., Tripathi, S. C., et al. (2021). Challenges and opportunities in productivity and sustainability of rice cultivation system: a critical review in Indian perspective. Cer Res. Commun. 50, 573–601. doi: 10.1007/s42976-021-00214-5
Lindsay, W. L., Norvell, W. A. (1978). Development of DTPA soil test for zinc, iron, manganese and copper. Soil Sci. Soc. Am. J. 42, 421–428. doi: 10.2136/sssaj1978.03615995004200030009x
Liu, H., Gan, W., Rengel, Z., Zhao, P. (2016). Effects of zinc fertilizer rate and application method on photosynthetic characteristics and grain yield of summer maize. Soil Sci. Plant Nutr. 16, 550–562. doi: 10.4067/S0718-95162016005000045
Mandal, U. K., Singh, G., Victor, U. S., Sharma, K. L. (2003). Green manuring: its effect on soil properties and crop growth under rice–wheat cropping system. Eur. J. Agron. 19 (2), 225–237. doi: 10.1016/S1161-0301(02)00037-0
Mohammad, A., Hamad, R. J., Khan, E. A., Mohammad, R. (2005). Comparative assessment of diverse rice varieties to green manuring (Sesbania aculeata). J. Res. (Sci). 16, 39–43.
Nayak, S., Mandi, S., Baral, K., Prasanna, R., Shivay, Y. S. (2022). “Agronomic approaches for biofortification of staple food crops,” in Biofortification of Staple Crops (Springer Singapore), 483–517. doi: 10.1007/978-981-16-3280-8_19
Nayak, S., Shivay, Y. S., Prasanna, R., Mandi, S., Kumar, D., Meena, S. L., et al. (2023). Non–biofortified rice variety responds more to zinc fertilization than biofortified variety in terms of zinc translocation and biofortification. J. Soil Sci. Plant Nutt. doi: 10.1007/s42729–023–01247–x
Olsen, R., Cole, C. V., Watanabe, F. S., Dean, L. A. (1954). Estimation of available phosphorus in soils by extraction with sodium bicarbonate. Circular, 939. United States Department of Agriculture, Washington, DC.
Patel, P. S., Singh, S. K., Patra, A., Jatav, S. S. (2022). Root dipping, foliar and soil application of zinc increase growth, yields, and grain zinc in rice (Oryza sativa L.) grown in moderate zinc soil of Inceptisol order. Comm Soil Sci. Plant Anal. 53 (15), 1917–1929. doi: 10.1080/00103624.2022.2069800
Pavithra, G. J., Rajashekar Reddy, B. H., Salimath, M., Geetha, K. N., Shankar, A. G. (2017). Zinc oxide nano particles increases Zn uptake, translocation in rice with positive effect on growth, yield and moisture stress tolerance. Indian J. Plant Phy. 22, 287–294. doi: 10.1007/s40502-017-0303-2
Pérez–de–Luque, A. (2017). Interaction of nanomaterials with plants: what do we need for real applications in agriculture? Front. Environ. Sci. 5. doi: 10.3389/fenvs.2017.00012
Pooniya, V., Choudhary, A. K., Swarnalakshmi, K. (2017). High–value crops imbedded intensive cropping systems for enhanced productivity, resource–use–efficiency, energetics and soil–health in Indo–Gangetic Plains. Proc. Natl. Acad. Sci. India Sect. B: Biol. Sci. 87, 1073–1090. doi: 10.1007/s40011-015-0679-6
Pooniya, V., Shivay, Y. S., Rana, A., Nain, L., Prasanna, R. (2012). Enhancing soil nutrient dynamics and productivity of Basmati rice through residue incorporation and zinc fertilization. Eur. J. Agron. 41, 28–37. doi: 10.1016/j.eja.2012.03.004
Prasad, R., Shivay, Y. S., Kumar, D. (2014). Agronomic biofortification of cereal grains with iron and zinc. Adv. Agron. 125, 55–91. doi: 10.1016/B978-0-12-800137-0.00002-9
Prasad, R., Shivay, Y. S., Kumar, D. (2017). Current status, challenges, and opportunities in rice production. Rice Product. Worldwide, 1–32. doi: 10.1007/978-3-319-47516-5_1
Prasad, R., Shivay, Y. S., Kumar, D., Sharma, S. N. (2006). Learning by doing exercises in soil fertility (A practical manual for soil fertility). Division Agro. Indian Agric. Res. Instit. New Delhi pp, 68.
Prasad, T. N. V., Sudhakar, K. V. P., Sreenivasulu, Y., Latha, P., Munaswamy, V., Reddyy, K. R., et al. (2012). Effect of nanoscale zinc oxide particles on the germination, growth and yield of peanut. J. Plant Nutr. 35 (6), 905–927. doi: 10.1080/01904167.2012.663443
Sadak, M. S., Bakry, B. A. (2020). Zinc–oxide and nano ZnO oxide effects on growth, some biochemical aspects, yield quantity, and quality of flax (Linum usitatissimum L.) in absence and presence of compost under sandy soil. Bull. Natl. Res. Centre. 44, 1–12. doi: 10.1186/s42269-020-00348-2
Sadhukhan, R., Sharma, L. D., Sen, S., Karmakar, S., Banerjee, K., Baral, K. (2021). Enhancing the productivity of field crops through nano–fertilizer. Agric. Dev. Asia–Potential Use Nano–Mater. Nano–Technol. doi: 10.5772/intechopen.101146
Shivay, Y. S., Kumar, D., Prasad, R., Ahlawat, I. P. S. (2008). Relative yield and zinc uptake by rice from zinc sulphate and zinc oxide coatings onto urea. Nutr. Cycl Agroeco. 80, 181–188. doi: 10.1007/s10705-007-9131-5
Shivay, Y. S., Prasad, R. (2012). Zinc–coated urea improves productivity and quality of basmati rice (Oryza sativa L.) under zinc stress condition. J. Plant Nutr. 35 (6), 928–951. doi: 10.1080/01904167.2012.663444
Shukla, A. K., Behera, S. K., Prakash, C., Patra, A. K., Rao, C. S., Chaudhari, S. K., et al. (2021). Assessing multi–micronutrients deficiency in agricultural soils of India. Sustainability 13 (16), 9136. doi: 10.3390/su13169136
Singh, A., Shivay, Y. S. (2014). Enhancement of growth parameters and productivity of basmati rice through summer green manuring and zinc fertilization. Int. J. Bio-res. Stress Manage. 5 (4), 486–494. doi: 10.5958/0976-4038.2014.00600.9
Subbiah, B. V., Asija, G. L. (1956). A rapid procedure for the estimation of available nitrogen in soils. Curr. Sci. 25 (8), 259–260.
Takkar, P. N., Singh, M. V., Ganeshmurthy, A. N. (1997). A critical review of plant nutrient supply needs, efficiency and policy issues for Indian agriculture for the year 2000: Micronutrients and trace elements. Plant nutrient supply efficiency Policy 2000–2025., 238–264.
Taran, N., Storozhenko, V., Svietlova, N., Batsmanova, L., Shvartau, V., Kovalenko, M. (2017). Effect of zinc and copper nanoparticles on drought resistance of wheat seedlings. Nanoscale Res. letters. 12, 1–6. doi: 10.1186/s11671-017-1839-9
Keywords: economics, green manuring, growth, nano Zn, yields, zinc biofortification
Citation: Baral K, Shivay YS, Prasanna R, Kumar D, Srinivasarao C, Mandi S, Nayak S and Reddy KS (2023) Enhancing physiological metrics, yield, zinc bioavailability, and economic viability of Basmati rice through nano zinc fertilization and summer green manuring in semi–arid South Asian ecosystem. Front. Plant Sci. 14:1283588. doi: 10.3389/fpls.2023.1283588
Received: 26 August 2023; Accepted: 05 October 2023;
Published: 31 October 2023.
Edited by:
Asif Naeem, Nuclear Institute for Agriculture and Biology, PakistanReviewed by:
Atique ur Rehman, Bahauddin Zakariya University, PakistanSuresh Kaushik, Independent Researcher, New Delhi, India
Copyright © 2023 Baral, Shivay, Prasanna, Kumar, Srinivasarao, Mandi, Nayak and Reddy. This is an open-access article distributed under the terms of the Creative Commons Attribution License (CC BY). The use, distribution or reproduction in other forums is permitted, provided the original author(s) and the copyright owner(s) are credited and that the original publication in this journal is cited, in accordance with accepted academic practice. No use, distribution or reproduction is permitted which does not comply with these terms.
*Correspondence: Yashbir Singh Shivay, eXNzaGl2YXlAaG90bWFpbC5jb20=