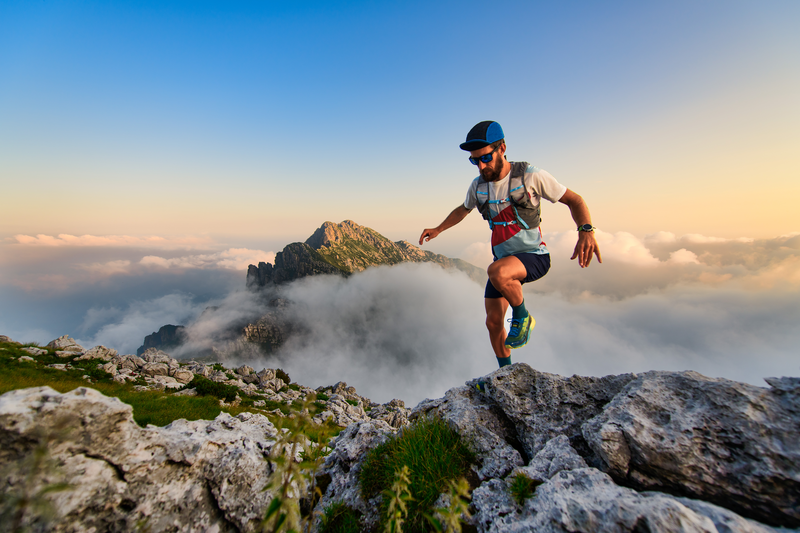
94% of researchers rate our articles as excellent or good
Learn more about the work of our research integrity team to safeguard the quality of each article we publish.
Find out more
ORIGINAL RESEARCH article
Front. Plant Sci. , 23 October 2023
Sec. Functional Plant Ecology
Volume 14 - 2023 | https://doi.org/10.3389/fpls.2023.1277013
Background and aims: Special root structures that can dissolve insoluble phosphorus locked in soil are supposed to contribute not only to the growing status of themselves but also to the neighbouring plants. However, whether dauciform roots have any effect on the neighbouring plants and how does it respond to meadow degradation had not been studied.
Methods: Alpine meadows with different degradation statuses were selected and the functional traits of Carex filispica and the co-occurring species Polygonum viviparum were measured to explore their response to degradation, as well as the response of Polygonum viviparum to the dauciform roots of Carex filispica.
Results: The results showed that 1) the number of dauciform roots decreased with the intensifying degradation, positively related to available phosphorus in the soil and negatively related to the aboveground phosphorus of Carex filispica. 2) Carex filispica and Polygonum viviparum are similar in specific leaf area and specific root area, yet different in the phosphorus content. The available phosphorus in the soil was negatively related to the aboveground phosphorus of Carex filispica and positively related to that of Polygonum viviparum. 3) When lightly degraded, the proportion of dauciform roots had positive effects on the aboveground resource-acquiring traits of Polygonum viviparum, which were no longer significant at heavy degradation. 4) Polygonum viviparum and Carex filispica without dauciform roots have similar performance: a decrease of belowground carbon with the increasing degradation, and a trend toward resource conservation with the increasing proportion of dauciform roots, which did not exist in Carex filispica with dauciform roots.
Conclusion: Our study found that dauciform roots had a beneficial effect on the resource acquisition of their neighbouring plants. However, due to the uncontrollable nature of natural habitats, whether this effect is stable and strong enough to be performed in ecological restoration requires further lab-controlled studies.
Phosphorus (P), an essential massive element required for plant growth, is abundant in natural soil, yet mostly as insoluble P and hardly available for plants (Richardson et al., 2009). P was found to be a restrictive factor for plant growth in meadows (Fridley, 2002), especially at high altitudes, which are generally characterized by severe nitrogen (N) deposition which intensifies the P limitation (Wright et al., 2004; Craine and Jackson, 2010; Penuelas et al., 2013; Fan et al., 2020). With the intensifying P limitation, traits that contribute to P uptake are increasingly associated with the performance and existence of plants (Turner, 2008; Olde Venterink, 2011). Thus, different morphological and physiological variations in roots have occurred to enhance P-acquisition (Lambers et al., 2006): most plants go for mycorrhizae, but a few plants develop specific root structures such as cluster roots and dauciform roots (Brundrett, 2009; Raven et al., 2018).
Dauciform root (DR), with a swollen axis and dense long root hairs, is mostly formed by Cyperaceae under P deficiency (Da Vies et al., 1973; Shane et al., 2006). In severely P-impoverished habitats such as south-western Australia, DR-forming plants have a high diversity (Shane et al., 2005), yet in the natural habitat of China, they are only described in degraded alpine meadows in the northwest of Yunnan Province (Gao and Yang, 2010). As we know, the available P is increasingly limited with the degradation of alpine meadows (Fan et al., 2020). So, is the formation of DRs related to the limited P in these degraded meadows? And does the number of dauciform roots increase with the increasing degradation and the decreasing available P?
Interesting fact, in these degraded alpine meadows, two dominant species stably occur together, Carex filispica, a species of Cyperaceae found to form dauciform roots here, and Polygonum viviparum (Gao and Yang, 2010). Species that can survive are often the ones with life strategies to adapt to the local environments (Grime, 2006), which can be reflected and quantified by their functional traits (McIntyre et al., 1999; Reich, 2014). These traits can be better predicted collaboratively, rather than independently (Watson and Szathmáry, 2016; Sporbert et al., 2021). The leaf economic spectrum (LES) indicates that these co-variant traits represent a trade-off between construction cost and longevity: they either have higher specific leaf area, photosynthetic capacity, respiration, leaf N, P content, and a shorter lifespan, or the other way around (Wright et al., 2004; Wright et al., 2007; Suding et al., 2008; Reich, 2014). Similarly, the root economic spectrum (RES) indicates that generally, roots with higher specific root area and N, P content tend to be more resource-acquiring yet short-lived, and vice versa, although it may be complicated by special root structure (Freschet et al., 2010; Carmona et al., 2021). So, the very existence of C. filispica and P. viviparum in alpine meadows indicates that they both have functional traits to acquire resources and survive the environments, the question is, of these traits, what do they have in common and how do they differ?
Furthermore, the effect of neighbouring plants can complicate the response of plants to environments. Functioning similarly to cluster roots (Shane et al., 2006), dauciform roots can dissolve insoluble P with their exudates, thereby increasing available P in the soil (Shane et al., 2006). Such dissolution of P is supposed to be beneficial not only for the growing status of the plant itself but even for the neighbouring plants (Muler et al., 2014), which has already been proved on cluster roots (Gardner and Boundy, 1983; Horst and Waschkies, 1987; Cu et al., 2005), while the relationship between dauciform roots and the neighbouring plants has never been addressed. However, this beneficial effect of cluster roots on neighbouring plants is expected to be weakened in ecosystems with greater competition (Callaway, 2007), while the competition for available soil nutrients, especially P, is found to increase with the increasing meadow degradation (Niu et al., 2016). So, can P. viviparum benefit from the dauciform roots of C. filispica, and is this beneficial effect weakened with the increase of degradation?
To address the above questions, we proposed these hypotheses: (a) the number of dauciform roots increases with the increasing degradation and the decreasing available P in the soil. (b) P. viviparum and C. filispica have similar resource-acquisitive traits in response to the degradation. (c) the growth status of P. viviparum has a positive correlation with the amount of dauciform roots of C. filispica, which weakens with the increasing degradation. Eight alpine meadow plots with two stages of degradation were selected in Baima Snow Mountain and the properties of dauciform roots, as well as the LES and RES traits of C. filispica and P. viviparum, were measured. The role of dauciform roots on neighbouring species was explored to provide a reference for future studies and the potential value of ecological restoration of Cyperaceae.
The study area is in Baima Snow Mountain National Nature Reserve, Yunnan Province, with a mean annual temperature of -1.0°C and precipitation of 600-650mm (Gao and Yang, 2016). The site selected is located at Baima Snow Mountain Pass at an altitude of 4300m (28°20’9’’N, 99°4’36’’E), which is exposed to a certain degree of human disturbance due to its closeness to the road. The area is mainly an alpine scrub-meadow zone, dominated by Carex filispica and Polygonum viviparum, and contains Eleocharis yokoscensis, Gentiana scabra, and Veronica didyma, et al.
Our experiments were performed in September 2021 and 2022. In September 2021, using coverage as an initial reference for degradation, eight plots were selected and measured for their soil physical and chemical properties, based on which the plots were then classified into light degradation (n=4) and heavy degradation (n=4) by Cluster analysis. Meanwhile, the functional traits related to the economic spectrum were measured for C. filispica and P. viviparum: Specific leaf area (SLA), aboveground phosphorus (AGP), photosynthetic capacity (Pnmax), specific root area (SRA), and belowground phosphorus (BGP) were selected because they are key components of LES and RES, which reflect the trade-off between resource-acquisition and self-defense (Wright et al., 2004; Poorter et al., 2010; Reich, 2014), while leaf area (LA), aboveground carbon (AGC), root surface area (RA), and belowground carbon (BGC) were selected to reflect the accumulation and allocation of carbon gain (Hermans et al., 2006). However, C. filispica in the 2021 experiment was measured as a whole, which was then found to be divided into individuals with and without DRs. Therefore, follow-up experiments were performed in September 2022, in which the above functional traits of individuals with and without DRs were measured respectively.
Soil properties: Each plot was measured three times by an SC-900 soil compactness meter for the average value of soil compaction (SC) between the depth of 0-20 cm, and the ring knife soil samples were taken at the depth of 0-10cm and 10-20cm to calculate the bulk density (BD). Soil samples were taken to measure soil chemical properties such as organic carbon (OC), total phosphorus (TP), available phosphorus (AP), total nitrogen (TN), and available nitrogen (AN). OC was determined by colorimetry after oxidation with a mixture of K2Cr2O7 and H2SO4 (Bao, 2000), TP was determined by the vanado-molybdate method (Horwitz, 1975) after digestion with H2SO4-HClO4, AP was measured using a UV-vis spectrophotometer by the methods of Bao (Bao, 2000), TN was determined using the Kjeldahl acid-digestion method with an auto-analyzer, and AN was measured by the Alkaline diffusion methods of Bao (Bao, 2000).
Functional traits: the chosen functional traits were measured for C. filispica and P. viviparum, with three replicates for each species in each plot. All the leaves from the three individuals were removed and measured by LI-COR portable leaf area meter for the average leaf area. Their root was scattered in the water and scanned by an LA-S root analyzer for the root surface area. The SLA (leaf area/leaf dry weight) and SRA (root surface area/root dry weight) were then calculated. The Pnmax was calculated from the light carves recorded by the LAI-6400 photosynthesis system, with the temperature set at 25°C. The carbon (C) and phosphorus (P) contents were measured separately for their aboveground and belowground parts. Carbon was determined by colorimetry after oxidation with a mixture of K2Cr2O7 and H2SO4 (Bao, 2000), and phosphorus was determined by the Vanadium molybdate yellow colorimetric method after digestion with H2SO4-H2O2 (Bao, 2000). We picked random individuals of C. filispica and observed their whole root system using an XTL-3B stereoscopic microscope, until we collected 10 individuals with DRs for each plot and recorded the number of DRs (lateral roots with swollen axes) per plant. The proportions of C. filispica with DRs were recorded, and their absolute cover of each plot was calculated (C.f with DRs proportion= the total cover of C. filispica × the proportion of individuals with DRs).
The analyses were performed using SPSS (ver 19.0, IBM, Armonk, NY, USA) and the figures were produced using Origin (ver 2022, OriginLab, USA). One-way ANOVAs were used to test for differences in each functional trait of C. filispica and P. viviparum at different degradation, and post-hoc tests were tested by LSD if there were differences. General linear regression models (GLM) were used to simulate the correlation between the functional traits and the environmental factors. In addition to the linear terms, models were fitted with quadratic terms for the functional traits and the proportion of dauciform roots, to test for the non-linear effects. Pearson correlation analysis was used to test the correlation among the DR properties, the functional traits, and the environmental factors. Principal component analysis (PCA) was used to downscale the standardized functional traits related to the economic spectrum of leaves and roots and to calculate the PC1 value. The structural equation models were analyzed by Amos 21.0 (Amos Development Co., Armonk, NY, USA) to figure out the effects of different factors on the values of the first axis of each species.
The soil chemical and physical properties of the light-degraded and heavy-degraded plots were shown in Table 1. Apart from the available P, all the nutrients were significantly different between the two stages of degradation, as well as the physical properties, yet the composition of the community showed no significant difference (Table 1).
As shown in Table 2, most of the differences were interspecific ones between C. filispica and P. viviparum: whether degraded or not, C. filispica had significantly lower P and higher C than P. viviparum, both aboveground and belowground. P. viviparum had significantly higher leaf area and root surface area than C. filispica, as well as the photosynthetic capacity, although, their specific leaf area and specific root area showed no significant difference.
Table 2 Comparison of functional traits of Carex filispica (C.f) and Polygonum viviparum (P.v) at different degradation.
As can be seen from Table 2, the only difference in the response to degradation between C. filispica and P. viviparum was that as the degradation increased, there was a significant decrease in carbon concentration both above- and belowground in P. viviparum, while not significant in C. filispica. Figure 1 showed that aboveground C of P. viviparum was positively related to both total and available P in soil, while C. filispica was only positively related to total P and unaffected by available P.
Figure 1 Relations of (A) aboveground carbon (C) in plants and total phosphorus (P) in soil; (B) aboveground carbon in plants and available phosphorus in soil. Regression lines are taken from phylogenetic generalized least squares models. The black dashed lines indicate Carex filispica (C.f), purple dashed lines for Polygonum viviparum (P.v). Asterisks indicate significant correlations, * p<0.05; *** p<0.001.
Further analysis (Table 3) showed that the belowground C of C. filispica without DRs decreased with the increasing degradation, just like P. viviparum, yet C. filispica with DRs showed no such trend.
Table 3 Comparison of carbon concentration of Carex filispica (C.f) with and without dauciform roots (DRs) and Polygonum viviparum (P.v) at different degradation.
Available P in soil was positively related to aboveground P in P. viviparum, yet negatively related to that in C. filispica (Figure 2). The average number of dauciform roots significantly decreased with the increase in degradation (Table 4). The average number of dauciform roots was positively related to total P and available P in the soil, yet negatively related to the aboveground P in C. filispica (Figure 3).
Figure 2 Relations of aboveground phosphorus (P) in plants and available phosphorus in soil. Regression lines are taken from phylogenetic generalized least squares models. The black dashed line indicates Carex filispica (C.f), purple dashed line for Polygonum viviparum (P.v). Asterisks indicate significant correlations, * p<0.05; *** p<0.001.
Figure 3 Relations of (A) the average number of dauciform roots (DR) and total phosphorus (P) in soil; (B) the average number of dauciform roots and available phosphorus in soil. (C) the average number of dauciform roots and aboveground phosphorus in Carex filispica. Regression lines are taken from phylogenetic generalized least squares models. Asterisks indicate significant correlations, * p<0.05; ** p<0.01.
GEM showed that the proportion of dauciform roots was positively related to the leaf area of P. viviparum and showed no such relation with C. filispica. On the other hand, the relative cover of C. filispica with dauciform roots showed negative relations with the root surface area of both C. filispica and P. viviparum (Figure 4).
Figure 4 Relations of (A) average leaf area and the proportion of dauciform roots (DR); (B) root surface area and the cover of Carex filispica with dauciform roots. The black dashed lines indicate Carex filispica (C.f), purple dashed lines for Polygonum viviparum (P.v). Asterisks indicate significant correlations, * p<0.05.
Further analysis of P. viviparum found that at light degradation, the proportion of dauciform roots had a positive relation with their photosynthetic capacity and specific leaf area, while the number of dauciform roots showed a negative relation with the root surface area and specific root area. Yet, all these correlations between DR properties and their functional traits were not significant at heavy degradation (Figure 5).
Figure 5 Relationships among dauciform root (DR) traits, and the functional traits of Carex filispica and Polygonum viviparum at different degradation, described by Pearson correlation coefficients. (A) relationships of Carex filispica at light degradation. (B) relationships of Carex filispica at heavy degradation. (C) relationships of Polygonum viviparum at light degradation. (D) relationships of Polygonum viviparum at heavy degradation. Pnmax, photosynthetic capacity; LA, leaf area; SLA, specific leaf area; RA, root surface area; SRA, specific root area. Asterisks indicate significant correlations, * p<0.05, ** p<0.01.
Principal component analysis (PCA) of the economic spectrum traits of C. filispica and P. viviparum was performed. Table 5 showed that a higher PC1 value indicates a tendency of higher SRA, Pnmax, BGP, SLA, and AGP, which means that the plants are leaning toward the resource-acquisitive side on the plant economic spectrum. The PC1 value of P. viviparum was negatively correlated with the relative cover of C. filispica with dauciform roots (r=-0.749, p=0.032).
Table 5 Trait loadings and eigenvalues of the economic spectrum trait variation explained by successive principal components (PC) in the trait PCA.
Structural equation modeling showed that available P in the soil had a negative relation with the PC1 value of the economic spectrum of each group (Figure 6). However, the proportion of C. filispica with dauciform roots only had negative effects on the value of C. filispica without dauciform roots and P. viviparum, leading them towards the conservative side, while this relationship was not significant on C. filispica with dauciform roots themselves.
Figure 6 The structural equation model of effects of soil phosphorus (P) and dauciform root (DR) properties on the PC1 value of the economic spectrum of Carex filispica (C.f) with and without dauciform roots and Polygonum viviparum (P.v). Purple lines represent positive impacts while dark grey lines represent negative ones. Lines of different thickness and the numbers represent the size of the standardized path coefficients, and asterisks represent the level of significance (* p<0.05, ** p<0.01).
Alpine meadow ecosystems are generally characterized by an abundance of total nutrients and a shortage of available nutrients (Fan et al., 2020). At different stages of degradation, the functional traits of plants are limited by different soil nutrients (Li et al., 2016), and the competition for available P was proved to be enhanced with the increasing degradation (Niu et al., 2016). In our study, the aboveground P of C. filispica showed a negative relation to the available soil P while that of P. viviparum was positively related (Figure 2). This indicates that P. viviparum has a stronger capability of available P acquisition, which therefore disadvantages the P acquisition of C. filispica when there is adequate available P in the soil. In addition, a portion of C. filispica had produced dauciform roots, which could provide a physiological advantage in P acquisition in the lack of available P by dissolving and utilizing the P fixed in the undecomposed organic matter of soil (Shane et al., 2005; Playsted et al., 2006; Masuda et al., 2020), therefore explains the inverse ratio their aboveground P showed with soil available P (Figure 2).
Despite that dauciform roots are considered an adaptation to low P (Lambers et al., 2006; Oliveira et al., 2015), species with dauciform roots are widely distributed worldwide from low to high P (Shane et al., 2005; Zemunik et al., 2015; Gusewell and Schroth, 2017). Our study area is not a typical P-deficient environment, therefore, only a portion of C. filispica produced dauciform roots, and only in small amounts (Gao and Yang, 2016). Since aboveground P in C. filispica is negatively related to available soil P (Figure 2), the average number of dauciform roots, while negatively related to aboveground P in C. filispica, turned out to be positively related to available soil P (Figure 3) and decreased with the increasing degradation (Table 4). Being contrast to our hypothesis, our result is consistent with previous studies that the formation of dauciform roots is regulated by P in the aboveground parts of plants, not in the soil (Shane et al., 2006).
Species that can survive are often the ones with ‘suitable’ traits to adapt to the local environments (Grime, 2006). The variation in functional traits can be mainly explained by edaphic factors (Salazar Zarzosa et al., 2021), and the variation in root economic traits was proved to drive aboveground biodiversity effects (Bu et al., 2017). In our study, the resource-acquisitive traits of C. filispica and P. viviparum were similar while the accumulation of carbon gain was significantly different: their SLA and SRA appeared to be of no significant difference, even though P. viviparum has a significantly higher leaf area and root area than C. filispica. Interspecifically, the above- and belowground P of C. filispica was significantly lower than that of P. viviparum, and their above- and belowground C was higher, regardless of the stage of degradation (Table 2). In a previous study, a typical non-mycorrhizal carboxylate-releasing species in Cyperaceae showed relatively lower Leaf P content compared to other species (Zhou et al., 2022), which is consistent with our result. As for their response to degradation, there is only a significant difference in above- and belowground C content: P. viviparum decreased as the degradation intensified, while those of C. filispica remained stable (Table 2). As mentioned before, P. viviparum appears to have stronger competitiveness for P, while the dauciform roots of C. filispica can stabilize their P content under P-impoverished environments. It may also explain the fact that the aboveground C of C. filispica only showed a positive correlation with total soil P while P. viviparum showed relations with both total P and available P (Figure 1): C. filispica is not dependent on available P because of the presence of dauciform roots, and might even be limited under high available P environments owing to the competition from P. viviparum.
Compared to the interspecific variability of traits, intraspecific ones were generally considered negligible, however, they were recently proven to have significant effects on the community and ecosystem functioning (Fu et al., 2020). The filtering of environments is not only interspecific but also intraspecific, that is, if the environment is eliminating species with lower leaf carbon content, it is most likely that individuals with lower carbon content within the species will also be eliminated (Sandel et al., 2021). The carbon content in P. viviparum was found to decrease significantly with the increasing degradation, while that of C. filispica showed no significant difference (Table 2). After being divided into two groups according to the presence of dauciform roots, the belowground C of C. filispica without dauciform roots showed a significant decrease with degradation, just like P. viviparum, while the ones with dauciform roots remained unchanged (Table 3). Therefore, it can be speculated that the previous results are due to dauciform roots adapting to heavily degraded environments, thus affecting the carbon content of C. filispica as a whole.
While studies on dauciform roots are extremely limited, these special root structures with the function of P-dissolving are supposed and proved to be beneficial not only for the plant itself but even for the growing status of the neighbouring plants (Muler et al., 2014). The promoting effect of cluster roots on neighbouring plants had been proven in several studies (Gardner and Boundy, 1983; Horst and Waschkies, 1987; Cu et al., 2005), however, this beneficial effect is not always reflected in P (Muler et al., 2014): in some previous studies, the neighbouring plants had both increased P and growth when planted with cluster root forming plants (Gardner and Boundy, 1983), in others, there appeared to be no difference in P while their growth was enhanced (Muler et al., 2014). The allocation of plants is usually biased towards the organs in charge of absorbing the most limited resources (Bloom et al., 1985; Poorter et al., 2012): roots are responsible for the uptake of nutrients and water while leaves are essential for photosynthesis (Rehling et al., 2021). In this study, the proportion of dauciform roots was negatively related to the root surface area of P. viviparum and positively related to the leaf area (Figure 4), which shows a relief of additional inputs to the belowground and a bias towards aboveground resources.
Furthermore, at light degradation, P. viviparum was affected by the proportion of dauciform roots, with increasing specific leaf area and photosynthetic capacity aboveground, decreasing root surface area and specific root area belowground (Figure 5C). The functional traits shown at this point can be explained by the presence of dauciform roots contributing to P-uptake, which releases the inputs for the nutrient-taking fine roots and invests in the aboveground competition for light. On the other hand, plants lacking phosphorus tend to show a decrease in stomatal conductivity (Thomas et al., 2006) and an increase in leaf dry mass per unit area (Fyllas et al., 2009; Turnbull et al., 2016). Our experiment shows that at light degradation, the lower the proportion of dauciform roots, the lower the photosynthetic rate and specific leaf area of P. viviparum, which shows P deficiency and can be easily explained by the P-uptaking promotion of dauciform roots. Interestingly, when the degradation increased, the functional traits of P. viviparum stopped being correlated with the proportion or number of dauciform roots (Figure 5D). In previous studies, the beneficial effect of cluster roots on other plants was expected to be weakened with greater physical stress or competition (Callaway, 2007), which is consistent with our results on dauciform roots: with the increasing degradation, dauciform roots of C. filispica no longer significantly contribute to the functional traits of P. viviparum, and the C content of P. viviparum suffered a great decrease.
Other than responding to the environments, functional traits can also play important roles in determining ecosystem functioning: they can be divided into response traits and effect traits, response ones describe the response of plants to the environment while effect ones describe the effect of plants on the ecosystem (Violle et al., 2007; Liu et al., 2021). A trait may correspond to one or several ecosystem functions (Sack and Buckley, 2020), while root economic traits were considered to be correlated with soil nutrient availability (Mommer and Weemstra, 2012; Freschet et al., 2021). Therefore, an alternative theory here for our result on the first hypothesis is that the dissolution of more dauciform roots increases the available P content of the soil, causing the positive correlation between the number of dauciform roots and the available soil P, also, the growth status of P.viviparum.
In fact, while having the same response of belowground C to the intensifying degradation, P. viviparum and C. filispica without dauciform roots also showed a similar response to dauciform roots: as the proportion of dauciform roots increased, their position on the economic spectrum was both biased in a resource-conservative direction (Figure 6), while individuals with dauciform roots did not have the same tendency. Our result is consistent with an earlier study showing that individuals with dauciform roots were proven to have more resource-acquiring traits after human trampling (Fan et al., 2023). Species with resource-acquisitive strategies tend to increase as disturbance intensified (Huang et al., 2021), and owing to the resource acquisition of dauciform roots contributing to the P-dissolution to ease the disturbance, all the other neighbouring plants remained resource-conservative. While intraspecific competition was mainly for belowground resources (Rehling et al., 2021), previous research showed that Cyperaceae may acquire P without depending on carboxylate release (Zhou et al., 2022). At the cost of producing dauciform roots in some of the individuals, C. filispica enhanced the P acquisition of the whole population, even the aboveground traits of P. viviparum at light degradation.
In this study, patterns and trends were noticed: the number of dauciform roots actually had a positive correlation with the available soil P, as well as the growth status of P. viviparum, which vanished with the increase of degradation. Most importantly, dauciform roots had significant effects on the position of P. viviparum and C. filispica without dauciform roots on the economic spectrum. This study contributes to the understanding of the role of dauciform roots on the companion species: with the intensifying P limitation, Cyperaceae with dauciform roots may play an important role in ecological restoration projects. However, the study area is not a typical P-deficient environment and some of the causality is still unclear due to the uncontrollable nature of the natural habitats. Whether this effect of dauciform roots is stable and strong enough to play a role in ecological restoration and subsequently be applied to the relief of phosphorus deficiency in artificial cultivation, requires further controlled variable studies.
The raw data supporting the conclusions of this article will be made available by the authors, without undue reservation.
RF: Conceptualization, Formal Analysis, Investigation, Writing – original draft. YH: Investigation, Writing – review & editing. WL: Investigation, Writing – review & editing. SJ: Investigation, Writing – review & editing. WJ: Conceptualization, Funding acquisition, Supervision, Writing – review & editing.
The author(s) declare financial support was received for the research, authorship, and/or publication of this article. This work was supported by the Natural Science Foundation of China (32071859).
We thank the Management and Protection Bureau of Baima Snow Mountain National Nature Reserve for providing sites and the rangers who helped to sample and measure.
The authors declare that the research was conducted in the absence of any commercial or financial relationships that could be construed as a potential conflict of interest.
All claims expressed in this article are solely those of the authors and do not necessarily represent those of their affiliated organizations, or those of the publisher, the editors and the reviewers. Any product that may be evaluated in this article, or claim that may be made by its manufacturer, is not guaranteed or endorsed by the publisher.
Bloom, A. J., Chapin, F. S., III, Mooney, H. A. (1985). Resource limitation in plants-an economic analogy. Annu. Rev. Ecol. Syst. 16, 363–392. doi: 10.1146/annurev.es.16.110185.002051
Brundrett, M. C. (2009). Mycorrhizal associations and other means of nutrition of vascular plants: understanding the global diversity of host plants by resolving conflicting information and developing reliable means of diagnosis. Plant Soil 320, 37–77. doi: 10.1073/pnas.1903178116
Bu, W., Schmid, B., Liu, X., Li, Y., Härdtle, W., von Oheimb, G., et al. (2017). Interspecific and intraspecific variation in specific root length drives aboveground biodiversity effects in young experimental forest stands. J. Plant Ecol. 10, 158–169. doi: 10.1093/jpe/rtw096
Callaway, R. M. (2007). Positive interactions and interdependence in plant communities. (Dordrecht, Netherlands: Springer).
Carmona, C. P., Bueno, C. G., Toussaint, A., Diaz, S., Moora, M., Munson, A. D., et al. (2021). Fine-root traits in the global spectrum of plant form and function. Nature 597, 683–68+. doi: 10.1038/s41586-021-03871-y
Craine, J. M., Jackson, R. D. (2010). Plant nitrogen and phosphorus limitation in 98 North American grassland soils. Plant Soil 334, 73–84. doi: 10.1007/s11104-009-0237-1
Cu, S. T. T., Hutson, J., Schuller, K. A. (2005). Mixed culture of wheat (Triticum aestivum L.) with white lupin (Lupinus albus L.) improves the growth and phosphorus nutrition of the wheat. Plant Soil 272, 143–151. doi: 10.1007/s11104-004-4336-8
Da Vies, J., Briarty, L. G., Rieley, J. O. (1973). Observations on the swollen lateral roots of the Cyperaceae. New Phytol. 72, 167–174. doi: 10.1111/j.1469-8137.1973.tb02022.x
Fan, B., Lin, L., Cao, G., Ke, X., Li, Y., Du, Y., et al. (2020). Relationship between plant roots and physical soil properties in alpine meadows at different degradation stages. Acta Ecol. Sin. 40, 2300–2309. doi: 10.5846/stxb201811262559
Fan, R., Hua, J., Huang, Y., Lin, J., Ji, W. (2023). What role do dauciform roots play? Responses of Carex filispica to trampling in alpine meadows based on functional traits. Ecol. Evol. 13. doi: 10.1002/ece3.9875
Freschet, G. T., Cornelissen, J. H. C., van Logtestijn, R. S. P., Aerts, R. (2010). Evidence of the 'plant economics spectrum' in a subarctic flora. J. Ecol. 98, 362–373. doi: 10.1111/j.1365-2745.2009.01615.x
Freschet, G. T., Roumet, C., Comas, L. H., Weemstra, M., Bengough, A. G., Rewald, B., et al. (2021). Root traits as drivers of plant and ecosystem functioning: current understanding, pitfalls and future research needs. New Phytol. 232, 1123–1158. doi: 10.1111/nph.17072
Fridley, J. D. J. O. (2002). Resource availability dominates and alters the relationship between species diversity and ecosystem productivity in experimental plant communities. Oecologia 132, 271–277. doi: 10.1007/s00442-002-0965-x
Fu, H., Yuan, G., Jeppesen, E. (2020). Trait-based community assembly of submersed macrophytes subjected to nutrient enrichment in freshwater lakes: Do traits at the individual level matter? Ecol. Indic. 110, 105895. doi: 10.1016/j.ecolind.2019.105895
Fyllas, N. M., Patiño, S., Baker, T. R., Bielefeld Nardoto, G., Martinelli, L. A., Quesada, C. A., et al. (2009). Basin-wide variations in foliar properties of Amazonian forest: phylogeny, soils and climate. Biogeosciences 6, 2677–2708. doi: 10.5194/bg-6-2677-2009
Gao, Q., Yang, Z. L. (2010). Ectomycorrhizal fungi associated with two species of Kobresia in an alpine meadow in the eastern Himalaya. Mycorrhiza 20, 281–287. doi: 10.1007/s00572-009-0287-5
Gao, Q., Yang, Z. L. (2016). Diversity and distribution patterns of root-associated fungi on herbaceous plants in alpine meadows of southwestern China. Mycologia 108, 281–291. doi: 10.3852/14-324
Gardner, W. K., Boundy, K. A. (1983). The acquisition of phosphorus byLupinus albus L. Plant Soil 70, 391–402. doi: 10.1007/BF02374894
Grime, J. P. (2006). Plant strategies, vegetation processes, and ecosystem properties. (Hobeken, NJ, USA: John Wiley & Sons).
Gusewell, S., Schroth, M. H. (2017). How functional is a trait? Phosphorus mobilization through root exudates differs little between Carex species with and without specialized dauciform roots. New Phytol. 215, 1438–1450. doi: 10.1111/nph.14674
Hermans, C., Hammond, J. P., White, P. J., Verbruggen, N. (2006). How do plants respond to nutrient shortage by biomass allocation? Trends Plant Sci. 11, 610–617. doi: 10.1016/j.tplants.2006.10.007
Horst, W. J., Waschkies, C. (1987). Phosphatversorgung von Sommerweizen (Triticum aestivum L.) in Mischkultur mit weisser Lupine (Lupinus albus L.). Zeitschrift für Pflanzenernährung und Bodenkunde 150, 1–8. doi: 10.1002/jpln.19871500102
Horwitz, W. (1975). Official methods of analysis (Washington, DC: Association of Official Analytical Chemists).
Huang, X., Sheng, Z., He, K., Zhang, Y., Kang, B., Ding, J., et al. (2021). Functional diversity and redundancy of subalpine meadows subjected to anthropogenic disturbances. J. Plant Ecol. 14, 870–883. doi: 10.1093/jpe/rtab039
Lambers, H., Shane, M. W., Cramer, M. D., Pearse, S. J., Veneklaas, E. J. (2006). Root structure and functioning for efficient acquisition of phosphorus: Matching morphological and physiological traits. Ann. Bot. 98, 693–713. doi: 10.1093/aob/mcl114
Li, D., Kang, S., Zhao, M.-Y., Zhang, Q., Ren, H.-J., Ren, J., et al. (2016). Relationships between soil nutrients and plant functional traits in different degradation stages of Leymus chinensis steppe in Nei Mongol, China. Chin. J. Plant Ecol. 40, 991. doi: 10.17521/cjpe.2015.0465
Liu, C., Li, Y., Yan, P., He, N. (2021). How to improve the predictions of plant functional traits on ecosystem functioning? Front. Plant Sci. 12, 622260. doi: 10.3389/fpls.2021.622260
Masuda, G., Maruyama, H., Lumbers, H., Wasaki, J. (2020). Formation of dauciform roots by Japanese native Cyperaceae and their contribution to phosphorus dynamics in soils. Plant Soil. 461, 107–118. doi: 10.1007/s11104-020-04565-6
McIntyre, S., Lavorel, S., Landsberg, J., Forbes, T. (1999). Disturbance response in vegetation–towards a global perspective on functional traits. J. Veg Sci. 10, 621–630. doi: 10.2307/3237077
Mommer, L., Weemstra, M. (2012). The role of roots in the resource economics spectrum. New Phytol. 195, 725–727. doi: 10.1111/j.1469-8137.2012.04247.x
Muler, A. L., Oliveira, R. S., Lambers, H., Veneklaas, E. J. (2014). Does cluster-root activity benefit nutrient uptake and growth of co-existing species? Oecologia 174, 23–31. doi: 10.1007/s00442-013-2747-z
Niu, K., He, J.-S., Lechowicz, M. J. (2016). Foliar phosphorus content predicts species relative abundance in P-limited Tibetan alpine meadows. Perspect. Plant Ecol. Evol. Syst. 22, 47–54. doi: 10.1016/j.ppees.2016.08.002
Olde Venterink, H. (2011). Does phosphorus limitation promote species-rich plant communities? Plant Soil 345, 1–9. doi: 10.1007/s11104-011-0796-9
Oliveira, R. S., Galvao, H. C., de Campos, M. C. R., Eller, C. B., Pearse, S. J., Lambers, H. (2015). Mineral nutrition of campos rupestres plant species on contrasting nutrient-impoverished soil types. New Phytol. 205, 1183–1194. doi: 10.1111/nph.13175
Penuelas, J., Poulter, B., Sardans, J., Ciais, P., van der Velde, M., Bopp, L., et al. (2013). Human-induced nitrogen–phosphorus imbalances alter natural and managed ecosystems across the globe. Nat. Commun. 4, 1–10. doi: 10.1038/ncomms3934
Playsted, C. W. S., Johnston, M. E., Ramage, C. M., Edwards, D. G., Cawthray, G. R., Lambers, H. (20062006). Functional significance of dauciform roots: exudation of carboxylates and acid phosphatase under phosphorus deficiency in Caustis blakei (Cyperaceae). New Phytol. 170, 491–500. doi: 10.1111/j.1469-8137.2006.01697.x
Poorter, L., McDonald, I., Alarcon, A., Fichtler, E., Licona, J. C., Pena-Claros, M., et al. (2010). The importance of wood traits and hydraulic conductance for the performance and life history strategies of 42 rainforest tree species. New Phytol. 185, 481–492. doi: 10.1111/j.1469-8137.2009.03092.x
Poorter, H., Niklas, K. J., Reich, P. B., Oleksyn, J., Poot, P., Mommer, L. (2012). Biomass allocation to leaves, stems and roots: meta-analyses of interspecific variation and environmental control. New Phytol. 193, 30–50. doi: 10.1111/j.1469-8137.2011.03952.x
Raven, J. A., Lambers, H., Smith, S. E., Westoby, M. (2018). Costs of acquiring phosphorus by vascular land plants: patterns and implications for plant coexistence. New Phytol. 217, 1420–1427. doi: 10.1111/nph.14967
Rehling, F., Sandner, T. M., Matthies, D., Hector, A. (2021). Biomass partitioning in response to intraspecific competition depends on nutrients and species characteristics: A study of 43 plant species. J. Ecol. 109, 2219–2233. doi: 10.1111/1365-2745.13635
Reich, P. B. (2014). The world-wide ‘fast–slow’plant economics spectrum: a traits manifesto. J. Ecol. 102, 275–301. doi: 10.1111/1365-2745.12211
Richardson, A. E., Barea, J.-M., McNeill, A. M., Prigent-Combaret, C. (2009). Acquisition of phosphorus and nitrogen in the rhizosphere and plant growth promotion by microorganisms. Plant Soil 321, 305–339. doi: 10.1007/s11104-009-9895-2
Sack, L., Buckley, T. N. (2020). Trait multi-functionality in plant stress response. Integr. Comp. Biol. 60, 98–112. doi: 10.1093/icb/icz152
Salazar Zarzosa, P., Diaz Herraiz, A., Olmo, M., Ruiz-Benito, P., Barron, V., Bastias, C. C., et al. (2021). Linking functional traits with tree growth and forest productivity in Quercus ilex forests along a climatic gradient. Sci. Total Environ. 786, 147468. doi: 10.1016/j.scitotenv.2021.147468
Sandel, B., Pavelka, C., Hayashi, T., Charles, L., Funk, J., Halliday, F. W., et al. (2021). Predicting intraspecific trait variation among California's grasses. J. Ecol. 109 (7), 2662–2677. doi: 10.1111/1365-2745.13673
Shane, M. W., Cawthray, G. R., Cramer, M. D., Kuo, J., Lambers, H. (2006). Specialized 'dauciform' roots of Cyperaceae are structurally distinct, but functionally analogous with 'cluster' roots. Plant Cell Environ. 29, 1989–1999. doi: 10.1111/j.1365-3040.2006.01574.x
Shane, M. W., Dixon, K. W., Lambers, H. (2005). The occurrence of dauciform roots amongst Western Australian reeds, rushes and sedges, and the impact of phosphorus supply on dauciform-root development in Schoenus unispiculatus (Cyperaceae). New Phytol. 165, 887–898. doi: 10.1111/j.1469-8137.2004.01283.x
Sporbert, M., Welk, E., Seidler, G., Jandt, U., Aćić, S., Biurrun, I., et al. (2021). Different sets of traits explain abundance and distribution patterns of European plants at different spatial scales. J. Veg Sci. 32. doi: 10.1111/jvs.13016
Suding, K. N., Lavorel, S., Chapin Iii, F., Cornelissen, J. H., Díaz, S., Garnier, E., et al. (2008). Scaling environmental change through the community-level: A trait-based response-and-effect framework for plants. Global Change Biol. 14, 1125–1140. doi: 10.1111/j.1365-2486.2008.01557.x
Thomas, D. S., Montagu, K. D., Conroy, J. P. (2006). Leaf inorganic phosphorus as a potential indicator of phosphorus status, photosynthesis and growth of Eucalyptus grandis seedlings. Forest Ecol. Manag. 223, 267–274. doi: 10.1016/j.foreco.2005.11.006
Turnbull, M. H., Griffin, K. L., Fyllas, N. M., Lloyd, J., Meir, P., Atkin, O. K. (2016). Separating species and environmental determinants of leaf functional traits in temperate rainforest plants along a soil-development chronosequence. Functional Plant Biol. 43 (8), 751–765. doi: 10.1071/FP16035
Turner, B. L. (2008). Resource partitioning for soil phosphorus: a hypothesis. J. Ecol. 96, 698–702. doi: 10.1111/j.1365-2745.2008.01384.x
Violle, C., Navas, M.-L., Vile, D., Kazakou, E., Fortunel, C., Hummel, I., et al. (2007). Let the concept of trait be functional! Oikos 116, 882–892. doi: 10.1111/j.0030-1299.2007.15559.x
Watson, R. A., Szathmáry, E. (2016). How can evolution learn? Trends Ecol. Evol. 31, 147–157. doi: 10.1016/j.tree.2015.11.009
Wright, I. J., Ackerly, D. D., Bongers, F., Harms, K. E., Ibarra-Manriquez, G., Martinez-Ramos, M., et al. (2007). Relationships among ecologically important dimensions of plant trait variation in seven Neotropical forests. Ann. Bot. 99, 1003–1015. doi: 10.1093/aob/mcl066
Wright, I. J., Reich, P. B., Westoby, M., Ackerly, D. D., Baruch, Z., Bongers, F., et al. (2004). The worldwide leaf economics spectrum. Nature 428, 821–827. doi: 10.1038/nature02403
Zemunik, G., Turner, B. L., Lambers, H., Laliberté, E. (2015). Diversity of plant nutrient-acquisition strategies increases during long-term ecosystem development. Nat. Plants 1, 1–4. doi: 10.1038/nplants.2015.50
Keywords: dauciform root, neighbouring plants, degraded meadow, economic spectrum, Cyperaceae
Citation: Fan R, Huang Y, Liu W, Jiang S and Ji W (2023) Dauciform roots affect the position of the neighboring plants on the economic spectrum in degraded alpine meadows. Front. Plant Sci. 14:1277013. doi: 10.3389/fpls.2023.1277013
Received: 13 August 2023; Accepted: 11 October 2023;
Published: 23 October 2023.
Edited by:
Xinsheng Chen, Anhui University, ChinaReviewed by:
Yingxin Huang, Chinese Academy of Sciences (CAS), ChinaCopyright © 2023 Fan, Huang, Liu, Jiang and Ji. This is an open-access article distributed under the terms of the Creative Commons Attribution License (CC BY). The use, distribution or reproduction in other forums is permitted, provided the original author(s) and the copyright owner(s) are credited and that the original publication in this journal is cited, in accordance with accepted academic practice. No use, distribution or reproduction is permitted which does not comply with these terms.
*Correspondence: Wenli Ji, aml3ZW5saUBud3N1YWYuZWR1LmNu
Disclaimer: All claims expressed in this article are solely those of the authors and do not necessarily represent those of their affiliated organizations, or those of the publisher, the editors and the reviewers. Any product that may be evaluated in this article or claim that may be made by its manufacturer is not guaranteed or endorsed by the publisher.
Research integrity at Frontiers
Learn more about the work of our research integrity team to safeguard the quality of each article we publish.