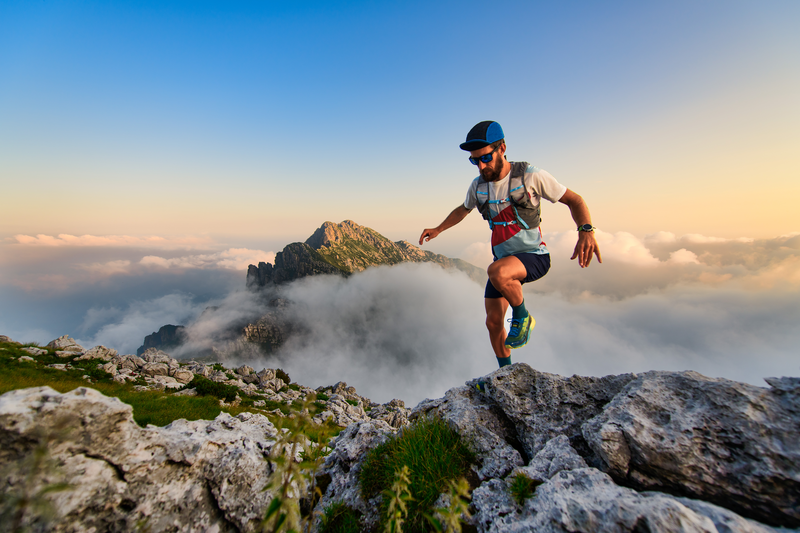
95% of researchers rate our articles as excellent or good
Learn more about the work of our research integrity team to safeguard the quality of each article we publish.
Find out more
METHODS article
Front. Plant Sci. , 04 October 2023
Sec. Photosynthesis and Photobiology
Volume 14 - 2023 | https://doi.org/10.3389/fpls.2023.1271341
This article is part of the Research Topic Quality of Ornamental Crops: Effect of Genotype, Preharvest, and Improved Production Chains on Quality Attributes of Ornamental Crops, volume II View all 11 articles
In urban areas, ornamental plants face different constraints, such as the shading of buildings and trees. Therefore, the selection of suitable species and their integration or combination with pre-existing plants is very important. Trees, shrubs, and herbaceous plant species must be distributed according to plant light requirements and shading intensity. Ornamental plants are classified into two groups based on their light intensity or shade tolerance: sun and shade species. To properly position the plants, especially in the immediate vicinity of buildings, it is necessary to study the projection of shadows during the year and the most critical periods, such as July and August. The position of ornamental species with different shading tolerances can be obtained by characterizing the leaf gas exchange for each species. Among the physiological parameters, the most important is the light compensation point, which is the light intensity corresponding to a net photosynthesis equal to zero. This means that the assimilation of carbon dioxide through photosynthesis is equal to the carbon dioxide emitted by respiration. This steady state represents the most critical condition for plants to endure the summer. The distribution of species inside a green area should be determined by considering the minimum light intensity that allows sufficient photosynthesis to compensate for the respiration rate. In this context, non-destructive leaf gas exchange, chlorophyll a fluorescence, and chlorophyll content can be useful tools for selecting suitable ornamental plants under diverse shading conditions.
The selection of ornamental plants for building a green urban or peri-urban area is based on the aesthetic value of the plants and their ability to adapt to suboptimal conditions. Together with the experimental values, the level of maintenance requirements must also be carefully considered, particularly if the maintenance is under municipal administration. Plant maintenance includes watering, fertilization, and pruning (Aronson et al., 2017). Annual, perennial, woody shrubs, and tree species were also selected, considering their tolerance to biotic and abiotic stresses (Toscano et al., 2019). Ornamental plants are resistant to pests and diseases. This characteristic is important to reduce the need for pesticides and ensure that plants remain healthy and visually appealing. Moreover, treatment in urban areas is not permitted. The plants were selected based on their seasonal interest, including the flowering period, foliage color, and unique features, such as bark or berries. Select a combination of plants that will provide visual interest throughout the year, ensuring a vibrant and dynamic urban landscape. Urban environmental tolerance is therefore an important issue. Some species are more tolerant to urban conditions such as compacted soil, air or soil pollution, low nutrient availability, and limited space. In the selection of plants, it is also important to use non-invasive species that have the potential to escape cultivation and negatively impact native ecosystems (Leotta et al., 2023). Plants must be chosen based on their interactions with the inhabitants. In particular, plants must be safe; therefore, potential hazards associated with certain plants must be appropriately evaluated, such as thorns, toxic berries or leaves, or allergies, and plants that may pose a risk to people or pets in urban areas should be avoided. Selecting ornamental plants for urban green spaces requires careful consideration of various factors to ensure that the plants thrive and to enhance the aesthetic appeal of the area. The selection of plants can consider the most important factors that can compromise the ornamental value of the area over a long period. Under low maintenance management conditions, plants must tolerate the most frequent abiotic stresses (Francini and Sebastiani, 2019).
A combination of plants of diverse heights and those close to tall buildings should be carefully planned to avoid the negative effects of shading. Light intensity and duration are important parameters for photosynthesis and plant morphology (Cocetta et al., 2017). The lack of light can reduce the growth rate and ornamental quality. Accordingly, the combination and integration of plants must be conducted by considering the shading intensity created by buildings or other plants. Based on light requirements, ornamental species are classified as shade and sun (Bohning and Burnside, 1956). Shade plants do not require a large amount of light and grow underbrushes naturally. These plants have low light compensation points and are rich in chlorophylls. Sun plants require high light intensity and leaves with high light-compensation points. Light intensity must be sufficient to provide sufficient photosynthetic activity to accumulate sufficient sugar to satisfy basal metabolism.
The shadows in the green areas can be classified into three levels.
● Light or bright shade: The area of interest can be completely shaded for a few hours per day. The sun’s rays are blocked from buildings or vegetation for several hours during the day, but there are hours when the area is exposed to the full sun.
● Partial shade: The area is shaded for most of the day, but at least early in the morning or evening, the plants are reached by the sun’s rays.
● Complete or total shade: The area is shaded daily.
This classification system provides a simple and general guide for the selection and positioning of ornamental plants in the proposed green area.
Light or shade intensity and temperature directly affect photosynthesis, whereas respiration is regulated only by temperature. The difference between photosynthesis and respiration represents net photosynthesis. Because photosynthesis produces sugars and respiration uses them, growth occurs when the balance between photosynthesis and respiration on a 24 h basis is positive. These physiological processes can change during different seasons, leading to the loss of ornamental quality. Therefore, they must be carefully studied to avoid positioning unsuitable plants in terms of adaptation to shade. The compensation point of different species could be a criterion for selecting ornamental plants for use in urban and peri-urban green areas. Based on these considerations, shade projections, which are the patterns of light intensity resulting from the shading of buildings and other objects, must be identified year-round, although most importantly, during spring and summer. Furthermore, temperature must also be accounted for, as net photosynthesis is temperature-dependent; increasing temperature enhances the respiration rate and reduces net photosynthesis (Evensen and Olson, 1992). Consequently, during the hottest weeks of summer, plants with a high light compensation point may produce insufficient carbohydrates for storage or growth, leading to plant death.
The relationship between the plant respiration rate and environmental temperature is well known. As the temperature increased, the respiration rate of plants also increased. This relationship can be explained by the basic principles of biochemical reactions as well as physiological and metabolic processes in plants (Atkin and Tjoelker, 2003). Plant respiration involves the breakdown of organic molecules such as sugars and carbohydrates to release energy for cellular activities. It occurs in both leaves and roots; therefore, soil temperature in urban environments is also a critical issue because of the low water availability, especially in the summer period. Several studies have investigated the relationship between plant respiration rate and temperature in urban environments (Lambrecht et al., 2016). The most evident effect was the altered phenology. Plants in urban areas can affect the timing of life cycle events including flowering, leaf emergence, and senescence. Urban plants may exhibit earlier flowering or extended growing seasons because of higher temperatures or altered light conditions in cities.
Studies performed on Crepis sancta (L.) Babc. plants grown in urban and rural areas showed that plants in different environments differed in phenological, morphological, and physiological traits. Plants grown in urban environments exhibit delayed flowering and senescence (Lambrecht et al., 2016). Delaying the phenological stages can be a plant strategy to accumulate sufficient resources to allow seed production for survival purposes.
As established above, ornamental plants used in urban and peri-urban green areas should be adapted to shade and light conditions. Green shaded areas can be generated by buildings or other plants. As a general guide, shade-tolerant plants should be used in shaded areas and sun-tolerant plants in areas of full sunlight exposure. However, shade is not constant throughout the day or during the different seasons. Therefore, it is important to identify the shade intensity of trees and buildings around green areas will be planned. The correct selection of plants in the area should be carried out by matching the light compensation point of each species with the light intensity and photoperiod throughout the daily and seasonal cycles. Figure 1 illustrates the distribution of plants with different light compensation point values.
Figure 1 Chlorophyll a fluorescence induction curve in Ficus elastica Roxb. ex Hornem., Wisteria sinensis (Sims) DC., Hedera helix L., and Quercus robur L., in plants under different light conditions.
In summer, more extensive plant canopies and higher temperatures can reduce photosynthetic activity and increase respiration, resulting in a negative net photosynthesis. Under these conditions, plants used more energy than they accumulated over 24 h. Therefore, the selection of plants should account for this, ensuring that the amount of light is sufficient to compensate for the respiration rate within a 24 h interval. The problem is less acute in spring, as plants typically do not show symptoms of shading’ however, with an increase in temperature, plants start to suffer from limited light conditions.
Chlorophyll a fluorescence is a non-destructive measurement that allows the evaluation of light use efficiency, leaf functionality, and the overall leaf health status, under optimal or stress conditions. Chlorophyll a fluorescence is widely used to establish stressful conditions in plants. For example, it has been used to measure the chlorophyll a fluorescence in Ficus elastica Roxb. ex Hornem., Wisteria sinensis (Sims) DC., Hedera helix L., Quercus robur L.
The results showed that F. elastica had the highest induction curve, whereas Q. robur had the lowest. W. sinensis had a similar trend F. elastica and H. helix to Q. robur (Figure 1).
The JIP test allows the calculation of several indices from the data obtained from the data points (Tsimilli-Michael, 2020). These indices can help identify species with the highest variability. The performance index (PI), time to reach the maximal fluorescence (Tfm), and reaction centers per PSII antenna Chl a (RC/ABS) are the parameters that could greatly show the differences in plants under different light conditions (Figure 2). An expanded list of the JIP indices is provided in Supplementary Table 1.
Figure 2 The JIP test-derived parameters were obtained from the elaboration of the chlorophyll a fluorescence induction curve. All parameters of Wisteria sinensis (Sims) DC. (red line), Hedera helix L. (orange line), and Quercus robur L. (green line) were standardized to Ficus elastica Roxb. ex Hornem (light blue line).
Plants can adapt to suboptimal conditions. In light compensation points of ornamental plants under low- or high-light conditions are reported in Table 1. Plants can alter their light compensation points by adapting to various environments. For example, Pseudomonas scutellarioides R. Br. reported an increase in the light compensation points in plants grown under high-pressure sodium lamps (HPS) or light-emitting diodes (LEDs) (Domurath et al., 2012). Lower compensation points are required for plants used in indoor environments, such as Philodendron erubescens K. Koch & Augustin and Dracaena surculosa Lindl. (Tan et al., 2017), whereas an analogous experiment simulating indoor conditions by lowering the light intensity resulted in reduced light compensation points in Leea coccinea Planch and L. rubra Blume ex Spreng. (Sarracino et al., 1992). Different light compensation points can also be observed in the same plant under different light-exposure conditions (Zhao et al., 2012). Leaves of Paeonia lactiflora Pall. exposed to the sun showed double the light compensation point compared with those under shade. This information can be useful when selecting plants for use in shaded locations, as adaptation may be used to lower the light composition of a species at the nursery level by applying different shading nets.
Table 1 Light compensation points (LCPs) of different species and light intensities are recommended in the area during the day of the most critical season.
Aeschynanthus longicaulis Wall. ex R.Br., the morphological and photosynthetic responses were measured under high or low irradiance (Li et al., 2014). Under high irradiance, the photosynthetic photon flux density (PPFD) was maintained under 650 µmol·m−2·s−1 by internal and external shading of the greenhouse. The light intensity of the low irradiance treatment was maintained under 150 µmol·m−2·s−1 by extra shading on the bench (Li et al., 2014). The LCP of Athyrium pachyphlebium C. Chr. at four different shade ranges was 813 μmol m–2 s–1 to 886 μmol m–2 s–1, 576 μmol m–2 s–1 to 633 μmol m–2 s–1, 335 μmol m–2 s–1 to 402 μmol m–2 s–1, 134 μmol m–2 s–1 to 175 μmol m–2 s–1 and 1,846 μmol m–2 s–1 to 1,914 μmol m–2 s–1. The LCP under the highest light regime was 33 μmol m–2 s–1 to 16 μmol m–2 s–1 (Huang et al., 2011). Similar study was performed on Passiflora morifolia Mast., P. suberosa subsp. litoralis (Kunth) Port.-Utl. ex M.A.M.Azevedo, Baumgratz and Gonç.-Estev., and P. palmeri var. sublanceolata Killip (Pires et al., 2011). These ornamental plants showed different LCPs ranging from 36 μmol m–2 s–1 to 21 μmol m–2 s–1 under reduced light intensities of 25%, 50%, and 75% solar radiation for evaluating the adaptation ability (Pires et al., 2011). Artificial shade was provided using different shading nylon nets, fixed in wooden frames with dimensions of 5 m × 5 m × 2 m, under field conditions, which allowed a 25% reduction (1,000 μmol m–2 s–1–1,400 μmol m–2 s–1, min–max), 50% reduction (600 μmol m–2 s–1 –900 μmol m–2 s–1), and 75% reduction (200 μmol m–2 s–1 –400 μmol m–2 s–1) of solar radiation, along with a control treatment under full sunlight (1,500 μmol m–2 s–1–1,900 μmol m–2 s–1). The reduced light intensity by 75% allowed the LCP reduction in all three species and the highest plasticity was observed in P. suberosa subsp. litoralis, and P. palmeri var. sublanceolata (Pires et al., 2011). The shading effect of LCP and plant growth were studied in Ficus benjamina L. grown under five shading conditions during two growing periods (Scuderi et al., 2012). The control (0% shading) and 80% shading levels had light intensities ranging from 880 μmol·m−2·s−1 to 142 μmol·m−2·s−1 in the first growing period and from 1,531 μmol·m−2·s−1 to 173 μmol·m−2·s−1 in the second growing period. Under these shading regimes, the LCP of F. benjamina was 17 μmol m–2 s–1 and 5.9 μmol m–2 s–1 (Scuderi et al., 2012).
Two species, Physocarpus amurensis (Maxim.) Maxim. and P. opulifolius (L.) Maxim. were exposed to low (100 μmol m–2 s–1) or high light intensity (1,000 μmol m–2 s–1 –1,500 μmol m–2 s–1), and their LCP respected the two conditions. The LCP values were 26 μmol m–2 s–1 and 28 μmol m–2 s–1 for P. amurensis and P. opulifolius, respectively, under high-irradiance conditions. At low irradiance, both species have an LCP of 20 μmol m–2 s–1 (Zhang et al., 2016).
Shade and sun exposure conditions were studied in Calophyllum inophyllum L. (Clusiaceae), Inga spectabilis (Vahl) Willd. (Fabaceae), and Ormosia macrocalyx Ducke (Fabaceae). C. inophyllum and I. spectabilis under shade showed a LCP three times lower than plants exposed to sun. O. macrocalyx only halved the LCP when exposed to shade (Slot et al., 2019).
Several plants have been adapted to indoor conditions to evaluate the ability of some species to remove carbon dioxide from the environment (Treesubsuntorn and Thiravetyan, 2018). The LCP of these species ranges from 10 µmol·m−2·s−1 to 15 µmol·m−2·s−1 (Torpy et al., 2017).
Based on experimental or literature information (Ignatieva, 2021), plants can be planted in various green areas. If we consider plants with LCP 25 µmol·m−2·s−1, 50 µmol·m−2·s−1, and 75 µmol·m−2·s−1 (Figure 3A) the possible planting position/zone in the area mapped for the shade/light intensity are reported in Figure 3B.
Figure 3 Light saturation curves and light compensation points (LCPs) of three different ornamental species (A) and the positioning of plants in different shade or light intensities that match the requirement of LCP on a 24 h basis (B).
Considering the information reported in Table 1 in the shade area with light intensity below 10 µmol·m−2·s−1 can be planted the Heuchera americana L. In areas comprised between 10 and 15 can be planted species such as Chlorophytum comosum (Thunb.) Jacques, Epipremnum aureum (Linden ex André) G.S.Bunting, Ficus benjamina L., and Gibasis Raf. spp. In areas with higher light intensities, the number of species that can be used greatly increased (Table 1). Of course, species must be selected based on the temperature of the geographical area in winter and these temperatures must be compatible with species tolerance.
Ornamental plants must be maintained under optimal nutrition and water conditions. The light saturation curve and light compensation point should be measured under specific light intensities that should be set from 1,000 µmol m−2 s−1 to 1,600 µmol m−2 s−1 for sun plants, 100 µmol m−2 s−1–200 µmol m−2 s−1 for shade plants and of 500 µmol m−2 s−1–700 µmol m−2 s−1 for intermediate conditions. The plasticity of plants to adapt to low light regimes must be evaluated by transferring plants under shading and reducing the light intensity using nets with a reduction of 25%, 50%, or 75% solar radiation. Measurements should be carried out after one week of adaptation.
The light saturation curve and light compensation point of a plant can be measured using a leaf gas exchange analyzer. This instrument allows for the identification of the light compensation point and light intensity that allows the achievement of the highest net photosynthesis. The light saturation curve can be determined by measuring the CO2 assimilation by decreasing the light intensity from 1,600 μmol m−2 s−1 to 0 μmol m−2 s−1. The CO2 concentration should be at ambient level (400 µL L−1). Readings were taken between 9:30 A.M. and 11:30 A.M. from the fully expanded leaves of the three plants.
Chlorophyll a fluorescence can be measured using a portable fluorimeter that allows the determination of plant health status, light-use efficiency, and plant adaptability to different light conditions. The chlorophyll a fluorescence induction curve was measured using a Handy Plant Efficiency Analyzer (PEA, Hansatech, UK). Chlorophyll a fluorescence can be measured in leaves that must be dark-adapted for 30 min by using a leaf clip (4 mm diameter). A rapid pulse of high light intensity of 3,300 μmol m−2 s−1 (600 W m−2) was emitted on the leaf area shaded by the leaf clip (Figure 4). The sensor recorded the leaf fluorescence. Chlorophyll a fluorescence parameters, such as Fo (minimal fluorescence), Fm (maximum fluorescence), Fv (variable fluorescence), and Fv/FM ratio (maximum quantum efficiency of photosystem II), were automatically calculated. JIP analysis can be performed to determine several indices related to light use efficiency and plant adaptation (Supplementary Table 1).
Figure 4 Leaf clip used for dark incubation of leaf area. The leaf clip should be placed on fully expanded and mature leaves. The incubation period should be at least 40 min to allow complete oxidation of PSII.
Based on the data collected regarding the light compensation point, it was possible to distribute the plants in the green areas. Light availability at the planting position must be higher than the light intensity of the light compensation point under the worst conditions, such as in summer with high temperatures.
The success of building a green area depends on many environmental factors including soil properties, temperature, relative humidity, and light or shade intensity. In particular, the low-light conditions that plants can be exposed to during the summer season must be considered. Correct ornamental plant species selection should be performed considering the light compensation point of the species and the shade intensity in the green area generated by tall trees or buildings.
The original contributions presented in the study are included in the article/Supplementary material, further inquiries can be directed to the corresponding author/s.
AlF: Writing – original draft. ST: Writing – review & editing. AnF: Conceptualization, Writing – original draft, Writing – review & editing. DR: Supervision, Writing – review & editing.
The authors declare financial support was received for the research, authorship, and/or publication of this article. This study was funded by MUR—PRIN2022—CUP G53D23003990006—Exploring edible and NATIVe Australian and South African plant species for Mediterranean ornamental industry (NATIVASA).
The authors declare that the research was conducted in the absence of any commercial or financial relationships that could be construed as a potential conflict of interest.
The author(s) declared that they were an editorial board member of Frontiers, at the time of submission. This had no impact on the peer review process and the final decision.
All claims expressed in this article are solely those of the authors and do not necessarily represent those of their affiliated organizations, or those of the publisher, the editors and the reviewers. Any product that may be evaluated in this article, or claim that may be made by its manufacturer, is not guaranteed or endorsed by the publisher.
The Supplementary Material for this article can be found online at: https://www.frontiersin.org/articles/10.3389/fpls.2023.1271341/full#supplementary-material
Aronson, M. F., Lepczyk, C. A., Evans, K. L., Goddard, M. A., Lerman, S. B., MacIvor, J. S., et al. (2017). Biodiversity in the city: key challenges for urban green space management. Front. Ecol. Environ. 15 (4), 189–196. doi: 10.1002/fee.1480
Atkin, O. K., Tjoelker, M. G. (2003). Thermal acclimation and the dynamic response of plant respiration to temperature. Trends Plant Sci. 8 (7), 343–351. doi: 10.1016/S1360-1385(03)00136-5
Bohning, R. H., Burnside, C. A. (1956). The effect of light intensity on rate of apparent photosynthesis in leaves of sun and shade plants. Am. J. Bot. 43 (8), 557–561. doi: 10.2307/2438868
Burnside, C. A., Böhning, R. H. (1957). The effect of prolonged shading on the light saturation curves of apparent photosynthesis in sun plants. Plant Physiol. 32 (1), 61. doi: 10.1104/pp.32.1.61
Cocetta, G., Casciani, D., Bulgari, R., Musante, F., Kołton, A., Rossi, M., et al. (2017). Light use efficiency for veges production in protected and indoor environments. Eur. Phys. J. Plus 132 (1), 1–15. doi: 10.1140/epjp/i2017-11298-x
Domurath, N., Schroeder, F. G., Glatzel, S. (2012). Light response curves of selected plants under different light conditions. Acta Hortic. 956, 291–298. doi: 10.17660/ActaHortic.2012.956.33
Evensen, K. B., Olson, K. M. (1992). Forcing temperature affects postproduction quality, dark respiration rate, and ethylene responsiveness of Pelargonium ×domesticum. J. Am. Soc Hortic. Sci. 117 (4), 596–599. doi: 10.21273/JASHS.117.4.596
Francini, A., Sebastiani, L. (2019). Abiotic stress effects on performance of horticultural crops. Horticulturae 5 (4), 67. doi: 10.3390/horticulturae5040067
Garland, K. F., Burnett, S. E., Day, M. E., van Iersel, M. W. (2012). Influence of substrate water content and daily light integral on photosynthesis, water use efficiency, and morphology of Heuchera americana. J. Am. Soc Hortic. Sci. 137 (1), 57–67. doi: 10.21273/JASHS.137.1.57
Huang, D., Wu, L., Chen, J. R., Dong, L. (2011). Morphological plasticity, photosynthesis and chlorophyll fluorescence of Athyrium pachyphlebium at different shade levels. Photosynthetica 49, 611–618. doi: 10.1007/s11099-011-0076-1
Ignatieva, M. (2021). “Evolution of the Approaches to Planting Design of Parks and Gardens as Main Greenspaces of Green Infrastructure,” in Urban Services to Ecosystems. Future City, vol. 17 . Eds. Catalano, C., Andreucci, M. B., Guarino, R., Bretzel, F., Leone, M., Pasta, S. (Cham: Springer). doi: 10.1007/978-3-030-75929-2_23
Lambrecht, S. C., Mahieu, S., Cheptou, P. O. (2016). Natural selection on plant physiological traits in an urban environment. Acta Oecol. 77, 67–74. doi: 10.1016/j.actao.2016.09.002
Leotta, L., Toscano, S., Ferrante, A., Romano, D., Francini, A. (2023). New strategies to increase the abiotic stress tolerance in woody ornamental plants in Mediterranean climate. Plants 12 (10), 2022. doi: 10.3390/plants12102022
Li, Q., Deng, M., Xiong, Y., Coombes, A., Zhao, W. (2014). Morphological and photosynthetic response to high and low irradiance of Aeschynanthus longicaulis. Sci. World J. doi: 10.1155/2014/347461
Pires, M. V., Almeida, A. A. F., Figueiredo, A. L., Gomes, F. P., Souza, M. M. (2011). Photosynthetic characteristics of ornamental passion flowers grown under different light intensities. Photosynthetica 49, 593–602. doi: 10.1007/s11099-011-0075-2
Sarracino, J. M., Merritt, R., Chin, C. K. (1992). Light acclimatization potential of Leea coccinia and Leea rubra grown under low light flux. HortScience 27 (5), 404–406. doi: 10.21273/HORTSCI.27.5.404
Scuderi, D., Giuffrida, F., Toscano, S., Romano, D. (2012). Growth, physiological response, and quality characteristics of weeping fig in response to shading levels and climatic conditions. HortScience 47 (11), 1586–1592. doi: 10.21273/HORTSCI.47.11.1586
Slot, M., Krause, G. H., Krause, B., Hernández, G. G., Winter, K. (2019). Photosynthetic heat tolerance of shade and sun leaves of three tropical tree species. Photosynth. Res. 141, 119–130. doi: 10.1007/s11120-018-0563-3
Tan, C. L., Wong, N. H., Tan, P. Y., Ismail, M., Wee, L. Y. (2017). Growth light provision for indoor greenery: A case study. Energy Build. 144, 207–217. doi: 10.1016/j.enbuild.2017.03.044
Torpy, F. R., Zavattaro, M., Irga, P. J. (2017). Green wall technology for the phytoremediation of indoor air: a system for the reduction of high CO2 concentrations. Air Qual. Atmos. Health 10, 575–585. doi: 10.1007/s11869-016-0452-x
Toscano, S., Ferrante, A., Romano, D. (2019). Response of Mediterranean ornamental plants to drought stress. Horticulturae 5, 6. doi: 10.3390/horticulturae5010006
Treesubsuntorn, C., Thiravetyan, P. (2018). ). Botanical biofilter for indoor toluene removal and reduction of carbon dioxide emission under low light intensity by using mixed C3 and CAM plants. J. Clean. Prod. 194, 94–100. doi: 10.1016/j.jclepro.2018.05.141
Tsimilli-Michael, M. (2020). Special issue in honour of Prof. Reto J. Strasser–Revisiting JIP-test: An educative review on concepts, assumptions, approximations, definitions and terminology. Photosynthetica 58, 275–292. doi: 10.32615/ps.2019.150
Zhang, H., Zhong, H., Wang, J., Sui, X., Xu, N. (2016). Adaptive changes in chlorophyll content and photosynthetic features to low light in Physocarpus amurensis Maxim and Physocarpus opulifolius “Diabolo”. PeerJ 4, e2125. doi: 10.7717/peerj.2125
Keywords: light compensation point, shadow projection, shade plants, sun plants, chlorophyll a fluorescence, photosynthesis
Citation: Francini A, Toscano S, Ferrante A and Romano D (2023) Method for selecting ornamental species for different shading intensity in urban green spaces. Front. Plant Sci. 14:1271341. doi: 10.3389/fpls.2023.1271341
Received: 04 August 2023; Accepted: 15 September 2023;
Published: 04 October 2023.
Edited by:
Roland Valcke, University of Hasselt, BelgiumReviewed by:
Roberta Paradiso, University of Naples Federico II, ItalyCopyright © 2023 Francini, Toscano, Ferrante and Romano. This is an open-access article distributed under the terms of the Creative Commons Attribution License (CC BY). The use, distribution or reproduction in other forums is permitted, provided the original author(s) and the copyright owner(s) are credited and that the original publication in this journal is cited, in accordance with accepted academic practice. No use, distribution or reproduction is permitted which does not comply with these terms.
*Correspondence: Stefania Toscano, c3RlZmFuaWEudG9zY2Fub0B1bmltZS5pdA==
Disclaimer: All claims expressed in this article are solely those of the authors and do not necessarily represent those of their affiliated organizations, or those of the publisher, the editors and the reviewers. Any product that may be evaluated in this article or claim that may be made by its manufacturer is not guaranteed or endorsed by the publisher.
Research integrity at Frontiers
Learn more about the work of our research integrity team to safeguard the quality of each article we publish.