- 1Department of Forest Botany, Institute of Forest Sciences, Warsaw University of Life Sciences, Warsaw, Poland
- 2Polish Academy of Sciences Botanical Garden - Centre for Biological Diversity Conservation in Powsin, Warsaw, Poland
The evolution of the vascular system has led to the formation of conducting and supporting elements and those that are involved in the mechanisms of storage and defense against the influence of biotic and abiotic factors. In the case of the latter, the general evolutionary trend was probably related to a change in their arrangement, i.e. from cells scattered throughout the tissue to cells organized into ducts or cavities. These cells, regardless of whether they occur alone or in a cellular structure, are an important defense element of trees, having the ability to synthesize, among others, natural resins. In the tracheid-based secondary xylem of gymnosperms, the resin ducts, which consist of secretory cells, are of two types: axial, interspersed between the tracheids, and radial, carried in some rays. They are interconnected and form a continuous system. On the other hand, in the tracheid-based secondary xylem of monocotyledons, the resin-producing secretory cells do not form specialized structures. This review summarizes knowledge on the morpho-anatomical features of various types of resin-releasing secretory cells in relation to their: (i) location, (ii) origin, (iii) mechanism of formation, (iv) and ecological significance.
1 Introduction
Various types of secondary growth have emerged during evolution. A special type of secondary growth has appeared in some monocotyledons, which is a manifestation of the activity of the monocot cambium producing secondary xylem along with secondary phloem in the form of vascular bundles (Carlquist, 2012; Jura-Morawiec et al., 2015; Maděra et al., 2020; Jura-Morawiec et al., 2021; Tulik et al., 2022). Another product of the monocot cambium is the parenchyma, the cells of which fill the space between the vascular bundles and constitute a large part of the secondary growth (Hubálková et al., 2017). The secondary xylem in monocotyledons is represented by tracheids (Carlquist, 2012; Jura-Morawiec, 2017). The secondary xylem in conifers is formed from the vascular cambium and includes both tracheids and parenchyma cells. In addition, the secondary xylem is spatially separated from the secondary phloem and contains only about 10% of parenchyma cells (Evert, 2006). Xylem parenchyma cells perform many functions, i.e. they participate in the transport and storage of water (Johnson et al., 2012; Klein et al., 2016; Carlquist, 2018), are a good neighbor and take part in postmortem tracheary element walls lignification (Baghdady et al., 2006; Smith et al., 2013; Blokhina et al., 2019), they re-fill cavitated tracheary elements (Spicer, 2014; Secchi et al., 2017), affect the mechanical properties of xylem (Reiterer et al., 2002; Arbellay et al., 2012), accumulate reserve substances (Tomasella et al., 2019; Słupianek et al., 2021), synthesize secondary metabolites in the process of heartwood formation (Hillis, 1987), participate in compartmentalization after tree injury (Morris et al., 2016), and secrete resin (Back, 2002; Cabrita, 2019). The latter function is performed by specialized parenchyma cells forming secretory structures (Bannan, 1936; Nagy et al., 2000). Among the monocotyledons with tracheid- based secondary xylem, there is only a small group of plants in the genus Dracaena, known as dragon trees, which have the ability to secrete resin (Maděra et al., 2020; Liu et al., 2021), which have the ability to secrete resin. Unlike conifers, in the secondary growth of dragon tree, this function is performed by single parenchyma cells (Jura-Morawiec and Tulik, 2015). However, these cells are difficult to identify among other parenchyma cells and it is not known whether this is their only function or whether they have many functions.
Although the secondary xylem of both conifers and dragon trees is based on tracheids, the parenchyma cells present in their bodies differ in terms of resin synthesize and secretion processes, therefore in this review we summarize the knowledge on the morpho-anatomical features of various types of resin-releasing secretory cells, taking into account their: (i) location, (ii) origin, (iii) mechanism of formation, (iv) and ecological significance.
2 Resin ducts in the secondary xylem of coniferous trees
In conifers such as Cathaya, Pinus, Picea, Larix, Pseudotsuga, and Keteleeria (K. davidiana, K. evelyniana) resin ducts (syn. resin canals) are a normal feature of the secondary xylem, and their formation can also be induced by external factors leading to traumatic resin duct development. In contrast, Abies, Nothotsuga, Tsuga, Cedrus or Pseudolarix are capable of producing only traumatic resin ducts in the secondary xylem (Bannan, 1936; Wu and Hu, 1997; Hudgins et al., 2005; Arbellay et al., 2014; Esteban et al., 2021).
Among the various criteria for distinguishing the secretory structures involved in resin synthesis, secretion, and accumulation under hydrostatic pressure, one is based on their anatomical structure. In the secondary xylem of Abies, Cedrus, Tsuga, and Pseudolarix, the resin-producing cells form blisters, which are a sac-like structure. This structure is surrounded with a layer of parenchyma cells termed epithelial cells (Wu and Hu, 1997). These cells die in the short time and their walls are lignified. In turn, in Cathaya, Pinus, Picea, Larix and Pseudotsuga the tube-like resin ducts are found. In these genera, thick-walled (except Pinus, which has stretchable, thin-walled epithelial cells) and long-lived secretory epithelial cells synthesize resin (Nagy et al., 2000). Not only the thickness of the epithelial cell walls varies, but also their number depending on the conifers. i.e. in Pinus sylvestris there are usually 4-6 cells surrounding the lumen of the duct while in Picea, Larix or Pseudotsuga there are 7-12 cells around the lumen (Schweingruber, 1978). In addition, the epithelium may be surrounded by 1-3 layers of pectin-enrich subsidiary cells easily distinguishable morpho-anatomically from epithelial cells and crushed during the development of the duct (Wiedenhoeft and Miller, 2002; Esteban et al., 2005). The elongate crystals both in epithelial and subsidiary parenchyma cells may also be present (Wiedenhoeft et al., 2003).
Resin ducts are classified to their arrangement as axial and radial. Epithelial cells of the axial resin ducts originate from fusiform cambial initials, while those of the radial resin ducts originate from ray cambial initials. The lumen between the epithelial cells can be formed by cells separation (schizogenous), cell lysis (lysigenous), or through a developmental process that involves both schizogenous and lysigenous pathways, known as schizolysigenous (Turner, 1999). The most commonly described lumen duct formation is that of schizogenous formation (Nagy et al., 2000) and occurs where it forms between the initial of the epithelial cells after hydrolysis of the middle lamella that binds the cells together. Auxin, which is involved in the secondary xylem formation, may promote the differentiation of resin ducts (Aloni, 2021). Since resin ducts do not form until several weeks after auxin application, hence it is assumed that in conifers the effect of auxin on resin duct development may include auxin-ethylene crosstalk (Fahn, 1988; Hudgins et al., 2006; Arbellay et al., 2014).
Resin ducts form co-planar networks in conifers secondary xylem (Figure 1A). Axial resin ducts, with an average diameter of 200 µm, are usually found in the outer region of the earlywood and in the first-formed latewood in every annual ring. Under normal conditions there are only a few, scattered axial resin ducts in the secondary xylem (Fahn et al., 1979; Wu and Hu, 1997). In spruce they usually occur singly or in pairs, rarely in groups of three in close proximity to each other. Normally, the axial resin ducts become longer as the age of the cambium increases (LaPascha and Wheeler, 1990; Krokene et al., 2008). Radial resin ducts start with vertical ducts and appear in some rays. Since uniseriate rays are many in conifers, those that include radial resin ducts are multiseriate and named fusiform rays (IAWA Committee, 1964). The density of the radial ducts in tangential sections varies from 0.15 to 3.5 ducts per mm2 of secondary xylem (Wu and Hu, 1997). Axial and radial resin ducts occur in the secondary xylem of Cathaya, Larix, Picea, Pinus and Psudotsuga while Keteleeria has only vertical resin ducts (Wu and Hu, 1997; Esteban et al., 2021).
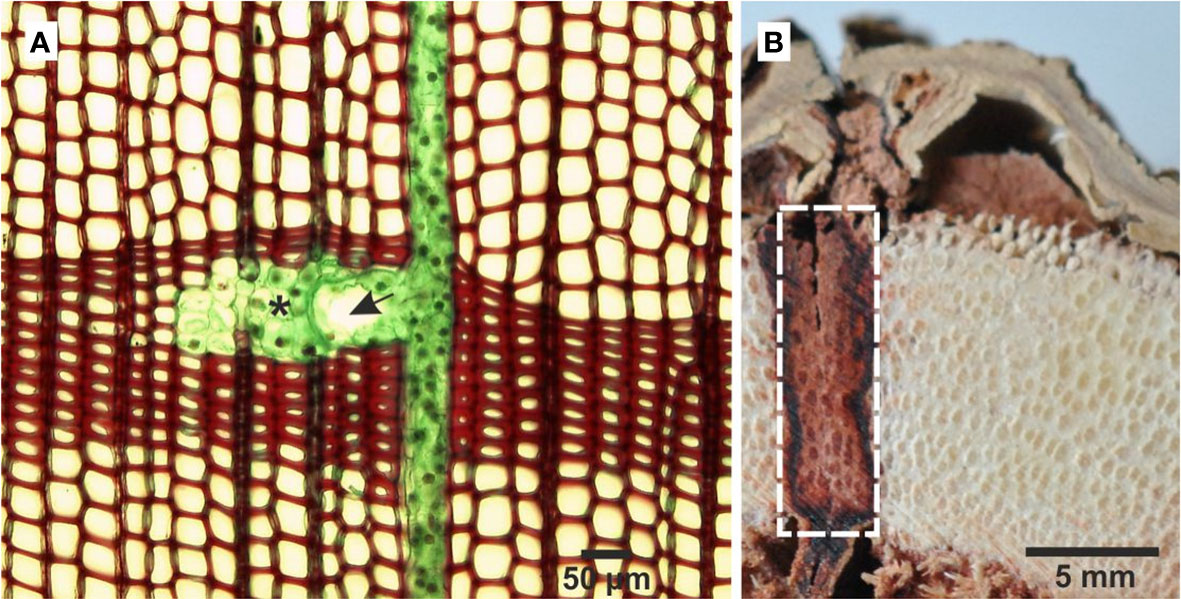
Figure 1 Cross sections through the trunk of Pinus sylvestris (A) and Dracaena draco (B). The axial resin duct and the ray carrying the radial resin duct (fusiform ray), which together form a co-planar network in the secondary xylem. The asterisk denotes subsidiary cell, and the arrow denotes epithelial cell with thin, cellulose cell walls (A). The white-sided rectangle covers the wound area with visible dragon’s blood in the tracheids of the vascular bundles and in the parenchyma cells of the secondary growth (B).
Traumatic resin ducts arise from cambial cells after trunk induction with metal pins in Tsuga sieboldii as documented by Kuroda and Shimaji (1983), and Picea abies trunk inoculated with Ceratocystis polonica (Nagy et al., 2000). They are also formed is hormone-mediated mode, it is assumed that both MJ (methyl jasmonate) and ethylene activate genes related to defense and formation of traumatic resin ducts (McKay et al., 2003; Schmidt et al., 2011). They appear relatively quickly after the injury, however, the time of year when the injury occurs is believed to be critical to the timing of traumatic resin duct onset (Gärtner and Heinrich, 2009).
Traumatic resin ducts are typically distributed in dense concentric series in the earlywood with a predominance in the vertical axis and occur singly or form an anastomosing network of cavities (Franceschi et al., 2002; Martin et al., 2002; Krokene et al., 2003). In Cedrus, are present in both vertical and radial systems in the vicinity of the wound (Fahn et al., 1979; Esteban et al., 2023). Traumatic resin ducts tend to be shorter than the resin ducts that are normal constituents of secondary xylem, however, in some cases they may be also longer and wider, scattered and found in remote areas from the injury (Krokene et al., 2008). Traumatic resin ducts are usually accompanied by small-diameter tracheids with thickened cell walls.
When considering the occurrence of resin ducts in compression wood, it should be noted that although Lee and Eom (1988) saw traumatic vertical resin ducts in Pinus koraiensis compression wood, this feature does not appear to be a consistent characteristic of compression wood. In species with resin ducts in secondary xylem, large areas with severe compression wood that fill the entire increment of secondary xylem often have no resin ducts, but in small areas of transient compression wood or in mild compression wood, resin ducts normally appear (Donaldson and Singh, 2013). In Cedrus deodara, Xu et al. (2018) found that branches with a 45° inclination and compression wood had more resin ducts than branches with a different inclination and other secondary xylem position.
Resin secretion is an important trait in the evolutionary adaptation of conifers to environmental conditions. It is chemically toxic, physically repels insects/pathogens and accounts for up to 1-5% of pine stem mass under normal growth conditions, but after treatment with chemical elicitors, an increase of resin content in the stem by 20% is observed (Stubbs et al., 1984; Wolter and Zinkel, 1984). Pinus rigida, P. merkusii, P. ponderosa, P. caribaea or P. canariensis have an extraordinary content of resin in the secondary xylem of trunk and are therefore referred to as pitch pines in commerce (Esteban et al., 2005). As pointed out by Hudgins et al. (2004), resin-producing members of the Pinaceae family are threatened by aggressive insects, while those with little or no resin have few or no aggressive pests. Despite the fact that plant defenses resulting from resin synthesis are costly and requires endogenous resource allocation and host energy input, they have been found to show trade-offs in growth, reproduction, and defensive traits (Herms and Mattson, 1992; Slack et al., 2017; Tuller et al., 2018; Watss et al., 2023). In addition, resin ducts as features of coniferous tree resistance may link dendrochronology and resin-based defense mechanisms (Slack et al., 2017; Zhao and Erbilgin, 2019; Hood et al., 2020; Vázquez-González et al., 2020; Catherwood et al., 2022).
It is also worth noting that in addition to the resin ducts in the sapwood, resin is also present in the heartwood, providing a defense against fungal decay and increasing the durability of the secondary xylem (Hillis, 1987; Piqueras et al., 2020; Bieniasz and Tulik, 2022). During formation of heartwood the resin ducts are frequently obstructed with tylosoids as a result of proliferation of the epithelial cells (Howard and Manwiller, 1969; Leggate et al., 2020) or due to the “fixation” of epithelial cells in a swollen state by events coincident with the formation of heartwood, including deposition of secondary cell walls and/or lignification as indicated by LaPascha and Wheeler (1990). Cown et al. (2011) found that a mature Pinus radiata stem contains approximately 25% heartwood with up to 10% resin content in the inner rings. Heartwood resin is stored in the lumen of the tracheids, but resinous tracheids may also be seen in sapwood which was reported in Pinus elliottii × Pinus caribaea by Leggate et al. (2019; 2020).
3 Resin-secreting cells in the secondary growth of monocotyledonous dragon trees
In the stem of the monocotyledonous dragon trees, secretion of red resin, called dragon’s blood, does not involve forming specialized structures (Fan et al., 2008; Jura-Morawiec and Tulik, 2015; Jura-Morawiec and Tulik, 2016). Resin-secreting parenchyma cells have no morphological features to distinguish them. It has been suggested that all of the living parenchyma cells in the secondary body of stem have the potential for resin secretion (Jura-Morawiec and Tulik, 2015), the only limitation is their lifespan. To date, the lifespan of these cells has not been investigated, but based on observations of lignin autofluorescence in the stem of D. draco, it has been concluded that it is related to the distance from the monocot cambium (Jura-Morawiec and Wiland-Szymańska, 2014).With increasing radial distance from the meristem, the cell walls of the parenchyma gradually become lignified, followed by cell death, which excludes them from resin production.
It is not clear how the resin is formed in parenchyma cells and transported along the tissue. Ding et al. (2020) have suggested that the major chemical constituents of dragon’s blood are transported out of the intracellular space in response to various stimuli by three potential transport mechanisms i.e., vesicle trafficking mediated transport, GST (glutathione S-transferase) transport or membrane transport. Recent studies of the leaf shedding of dragon trees have shown that dragon’s blood is in the form of vesicles, which have a tendency to aggregate and fill the cells or intercellular spaces (Jura-Morawiec et al., 2023). After the injury of the secondary tissues, resin typically fills the parenchyma cells and enters tracheids through the pits occluding their lumen (Cui et al., 2013; Jura-Morawiec and Tulik, 2015; Xu et al., 2022; Figure 1B). Resin secretion can be additionally enhanced by high humidity and fungal infection (Wang et al., 2010, 2011), and its accumulation increases after acid and sodium salt treatment (reviewed by Ding et al., 2020).
The mass of living parenchyma cells with the ability to secrete resin has a role in the dragon tree defense mechanism (Wang et al., 2010; Jura-Morawiec and Tulik, 2016). After the injury, the resin-filled parenchyma cells, together with the resin occluded lumen of the tracheids, limit the spread of infection/pathogen in all directions. In turn, the immediate solidification of the resin and its red aposematic (warning) color prevent access to living tissue, acting as a physical and chemical barrier. However, it should be noted that the appearance of the red resin color is a gradual process and is not visible immediately after the wound. In D. draco, it was observed two weeks after stem cutting (Jura-Morawiec and Tulik, 2015), while in D. cochinchinensis it was visible 3-4 days after wounding or fungal infection (Wang et al., 2011), with a clear red layer covering the wound site after 90 days (Xu et al., 2022).
4 Perspectives
In the course of evolution of the secondary growth, parenchyma cells have acquired various functions including resin synthesis and secretion. It seems that knowledge about the resin-based defense mechanisms of coniferous species may be useful in predicting the possibility of introducing alien coniferous species to new areas under the conditions of ongoing global warming. Undoubtedly, Cedrus libani or P. canariensis seem to be such species due to their high wound-healing capacity. In the case of monocotyledonous dragon trees, research efforts should focus on an in-depth understanding of their biology. Although this study has been going on since the 19th century, there is still a large gap in our understanding of dragon’s blood secretion, including the relationship between the lifespan of the parenchyma cells, the age of the dragon tree, and resin production. Analysis of the biological aging of parenchyma cells by measuring their metabolic activity in combination with histochemical techniques can help fill this knowledge gap.
Author contributions
MT: Funding acquisition, Writing – original draft. JJ-M:Writing – review & editing and also prepared the microphotograph.
Funding
The authors declare that no financial support was received for the research, authorship, and/or publication of this article. This article was partly founded by the Institute of Forest Sciences of the Warsaw University of Life Sciences.
Conflict of interest
The authors declare that the study was conducted in the absence of any commercial or financial relationships that could be construed as a potential conflict of interest.
Publisher's note
All claims expressed in this article are solely those of the authors and do not necessarily represent those of their affiliated organizations, or those of the publisher, the editors and the reviewers. Any product that may be evaluated in this article, or claim that may be made by its manufacturer, is not guaranteed or endorsed by the publisher.
References
Aloni, R. (2021). Vascular Differentiation and Plant Hormones (Berlin: Springer). doi: 10.1007/978-3-030-53202-4_17
Arbellay, E., Fonti, P., Stoffel, M. (2012). Duration and extension of anatomical changes in wood structure after cambial injury. J. Exp. Bot. 63, 3271–3277. doi: 10.1093/jxb/ers050
Arbellay, E., Stoffel, M., Sutherland, E. K., Smith, K. T., Falk, D. A. (2014). Changes in tracheid and ray traits in fire scars of North American conifers and their ecophysiological implications. Ann. Bot. 114, 223–232. doi: 10.1093/aob/mcu112
Back, E. L. (2002). Pattern of parenchyma and canal resin composition in softwoods and hardwoods. J. Wood Sci. 48, 167–170. doi: 10.1007/BF00771362
Baghdady, A., Blervacq, A. S., Jouanin, L., Grima-Pettenati, J., Sivadon, P., Hawkins, S. (2006). Eucalyptus gunnii CCR and CAD2 promoters are active in lignifying cells during primary and secondary xylem formation in Arabidopsis thaliana. Plant Physiol. Biochem. 44, 674–683. doi: 10.1016/j.plaphy.2006.10.027
Bannan, M. W. (1936). Vertical resin ducts in the secondary wood of the Abietineae. New Phytol. 35, 11–46. doi: 10.1111/j.1469-8137.1936.tb06864.x
Bieniasz, A., Tulik, M. (2022). The longest living xylem cells locked in lignified cell walls - the case of xylem parenchyma in European ash (Fraxinus excelsior L.) stems. Acta Biol. Crac. Ser. Bot. 64, 65–74. doi: 10.24425/abcsb.2022.143383
Blokhina, O., Laitinen, T., Hatakeyama, Y., Delhomme, N., Paasela, T., Zhao, L., et al. (2019). Ray parenchymal cells contribute to lignification of tracheids in developing xylem of Norway Spruce. Plant Physiol. 181, 1552–1572. doi: 10.1104/pp.19.00743
Cabrita, P. (2019). “A model for resin flow,” in Plant Cell and Tissue Differentiation and Secondary Metabolites. Eds. Ramawat, K., Ekiert, H., Goyal, S. (Berlin: Springer, Cham). doi: 10.1007/978-3-030-11253-0_5-1
Carlquist, S. (2012). Monocot xylem revisited: new information, new paradigms. Bot. Rev. 78, 87–153. doi: 10.1007/s12229-012-9096-1
Carlquist, S. (2018). Living cells in wood 3. Overview; Functional anatomy of the parenchyma network. Bot. Rev. 84, 242–294. doi: 10.1007/s12229-018-9198-5
Catherwood, A., Knapp, P., Mitchell, T. J. (2022). Scientific note: Loblolly pine traumatic resin ducts serve as indicators of cool-season weather events at Nags Head, North Carolina. Castanea 86, 296–304. doi: 10.2179/0008-7475.86.2.296
Cown, D. J., Donaldson, L. A., Downes, G. M. (2011). A review of resin features in radiata pine. N. Z. J. For. Sci. 41, 41–60.
Cui, J. L., Wang, C., Guo, S. X., Xiao, P. G., Wang, M. L. (2013). Stimulation of dragon's blood accumulation in Dracaena Cambodiana via fungal inoculation. Fitoterapia 87, 31–36. doi: 10.1016/j.fitote.2013.03.012
Ding, X., Zhu, J., Wang, H., Chen, H., Mei, W. (2020). Dragon’s blood from Dracaena Cambodiana in China: applied history and induction techniques toward formation mechanism. Forests 11, 372. doi: 10.3390/f11040372
Donaldson, L. A., Singh, A. P. (2013). “Formation and structure of compression wood,” in Cellular Aspects of Wood Formation, Plant Cell Monographs. Ed. Fromm, J. (Berlin: Springer), 225–256.
Esteban, L. G., de Palacios, P., Garcia-Iruela, A., Garcia-Fernández, F. (2021). Comparative wood anatomy in Pinaceae with reference to its systematic position. Forests 12, 1706. doi: 10.3390/f12121706
Esteban, L. G., Gasson, P., Climent, J. M., de Palacios, P., Guindo, A. (2005). The wood of Pinus canariensis and its resinous heartwood. IAWA J. 36, 69–77. doi: 10.1163/22941932-90001602
Esteban, L. G., Palacios, P., Heinz, I., Gasson, P., Garcia-Iruela, A., Garcia-Fernández, F. (2023). Softwood anatomy: a review. Forest 14, 323. doi: 10.3390/f14020323
Evert, R. F. (2006). Esau's plant anatomy: meristems, cells, and tissues of the plant body: their structure, function, and development (New Jersey: John Wiley and Sons).
Fahn, A., Werker, E., Bentzur, P. (1979). Seasonal effects of wounding and growth-substances on development of traumatic resin ducts in Cedrus libani. New Phytol. 82, 537–544. doi: 10.1111/j.1469-8137.1979.tb02680.x
Fan, L. L., He, J. X., Chen, H. B., Cai, S. Q. (2008). Microscopical study of original plant of Chinese drug dragon’s blood Dracaena cochinchinensis and distribution and constituents detection of its resin. Zhongguo Zhong Yao Za Zhi 33, 1112–1117.
Franceschi, V. R., Krekling, T., Christiansen, E. (2002). Application of methyl jasmonate on Picea abies (Pinaceae) stems induces defense-related responses in phloem and xylem. Am. J. Bot. 89, 578–586. doi: 10.3732/ajb.89.4.578
Gärtner, H., Heinrich, I. (2009). The formation of traumatic rows of resin ducts in Larix decidua and Picea abies (Pinaceae) as a result of wounding experiments in the dormant season. IAWA J. 30, 199–215. doi: 10.1163/22941932-90000215
Herms, D. A., Mattson, W. J. (1992). The dilemma of plants: to grow or defends. Q. Rev. Biol. 67, 283–335. doi: 10.1086/417659
Hood, S. M., Reed, Ch., C., Kane, J. M. (2020). Axial resin ducts quantification in tree rings: A functional defense traits. MethodsX 7, 101035. doi: 10.1016/j.mex.2020.101035
Howard, E. T., Manwiller, F. G. (1969). Anatomical characteristics of southern pine stemwood. Wood Sci. 2, 77–86.
Hubálková, I., Houška, J., Kubícek, J., Mazal, P., Pavliš, J., Pohoralý, J., et al. (2017). Preliminary anatomical study on secondary thickening parts of endemic Dracaena cinnabari Balf.fil. from the Soqotra island. Wood Res. 62, 67–77.
Hudgins, J. W., Christiansen, E., Franceschi, V. R. (2004). Induction of anatomically based defense responses in stems of diverse conifers by methyl jasmonate: a phylogenetic perspective. Tree Physiol. 24, 251–264. doi: 10.1093/treephys/24.3.251
Hudgins, J. W., McDonald, G. I., Zambino, P. J., Klopfenstein, N. B., Franceschi, V. R. (2005). Anatomical and cellular responses of Pinus monticola stem tissue to invasion by Cronatrium ribicola. For. Path. 35, 423–443. doi: 10.1111/j.1439-0329.2005.00425.x
Hudgins, J. W., Ralph, S., Franceschi, V. R., Bohlmann, J. (2006). Ethylene in induced conifer defense: cDNA cloning, protein expression, and cellular and subcellular localization of 1-aminocyclopropane-1-carboxylate oxidase in resin duct and phenolic parenchyma cells. Planta 224, 865–877. doi: 10.1007/s00425-006-0274-4
IAWA Committee (1964). “Committee on nomenclature international association of wood anatomists (IAWA),” in Multilingual Glossary of Terms Used in Wood Anatomy (Switzerland: Verlagsanstalt Buchdruckerei Konkordia).
Johnson, D. M., McCulloh, K. A., Woodruff, D. R., Meinzer, F. C. (2012). Hydraulic safety margins and embolism reversal in stems and leaves: why are conifers and angiosperms so different? Plant Sci. 195, 48–53. doi: 10.1016/j.plantsci.2012.06.010
Jura-Morawiec, J. (2017). Atypical origin, structure, and arrangement of secondary tracheary elements in the stem of the monocotyledonous dragon tree, Dracaena draco. Planta 245, 93–99. doi: 10.1007/s00425-016-2593-4
Jura-Morawiec, J., Marcinkiewicz, J., Caujape-Castells, J. (2023). Unraveling the role of dragon’s blood in the undisturbed growth of dragon trees. Trees 37, 993–999. doi: 10.1007/s00468-022-02349-2
Jura-Morawiec, J., Oskolski, A., Simpson, P. (2021). Revisiting the anatomy of the monocot cambium, a novel merystem. Planta 254, 6. doi: 10.1007/s00425-021-03654-9
Jura-Morawiec, J., Tulik, M. (2015). Morpho-anatomical basis of dragon´s blood secretion in Dracaena draco stem. Flora 213, 1–5. doi: 10.1016/j.flora.2015.03.003
Jura-Morawiec, J., Tulik, M. (2016). Dragon’s blood secretion and its ecological significance. Chemoecology 26, 101–105. doi: 10.1007/s00049-016-0212-2
Jura-Morawiec, J., Tulik, M., Iqbal, M. (2015). Lateral meristems responsible for secondary growth of the monocotyledons: a survey of the state of the art. Bot. Rev. 81, 150–161. doi: 10.1007/s12229-015-9152-8
Jura-Morawiec, J., Wiland-Szymańska, J. (2014). A novel insight into the structure of amphivasal secondary bundles on the example of Dracaena draco L. stem. Trees 28, 871–877. doi: 10.1007/s00468-014-1000-7
Klein, T., Cohen, S., Paudel, I., Preisler, Y., Rotenberg, E., Yakir, D. (2016). Diurnal dynamics of water transport, storage and hydraulic conductivity in pine trees under seasonal drought. iForest Biogeosci. For. 9, 710–719. doi: 10.3832/ifor2046-009
Krokene, P., Nagy, N. E., Krekling, T. (2008). “Traumatic resin ducts and polyphenolic parenchyma cells in Conifers,” in Induced Plant Resistance to Herbivory. Ed. Schaller, A. (Berlin: Springer), 147–169.
Krokene, P., Siolheim, H., Krekling, T., Christiansen, E. (2003). Inducible anatomical defense responses in Norway spruce stems and their possible role in induced resistance. Tree Physiol. 23, 191–197. doi: 10.1093/treephys/23.3.191
Kuroda, K., Shimaji, K. (1983). Traumatic resin canal formation as a marker of xylem growth. For. Sci. 29, 653–659.
LaPascha, C. A., Wheeler, E. A. (1990). Resin canals in Pinus taeda. Longitudinal canal lengths and interconnections between longitudinal and radial canals. IAWA Bull. 11, 227–238. doi: 10.1163/22941932-90001180
Lee, P. W., Eom, Y. G. (1988). Anatomical comparison between compression wood and opposite wood in a branch of Korean pine (Pinus koraiensis). IAWA Bull. 9, 275–284. doi: 10.1163/22941932-90001077
Leggate, W., Redman, A., Wood, J., Baillers, H., Lee, D. L. (2019). Radial permeability of the hybrid pine (Pinus elliottii × Pinus caribaea) in Australia. BioRes. 14, 4358–4372. doi: 10.15376/biores.14.2.4358-4372
Leggate, W., Shirmohammadi, M., McGavin, R. L., Chandra, K., Knackstedt, M., Kneufling, L., et al. (2020). Influence of wood’s anatomical and resin traits on the radial permeability of the hybrid pone (Pinus elliottii × Pinus caribaea) wood in Australia. BioRes. 15, 6851–6873. doi: 10.15376/biores.15.3.6851-6873
Liu, Y., Zhao, X., Yao, R., Li, C., Zhang, Z., Xu, Y., et al. (2021). Dragon’s blood from Dracaena worldwide: species, traditional uses, phytochemistry and pharmacology. Am. J. Chin. Med. 49, 1–53. doi: 10.1142/S0192415X21500634
Maděra, P., Forrest, A., Hanacek, P., Vahalik, P., Gebauer, R., Plichta, R., et al. (2020). What we know and what we do not know about dragon trees? Forests 11, 236. doi: 10.3390/f11020236
Martin, D., Tholl, D., Gershenzon, J., Bohlmann, J. (2002). Methyl jasmonate induces traumatic resin ducts, terpenoid resin biosynthesis, and terpenoid accumulation in developing xylem of Norway spruce stems. Plant Physiol. 129, 1003–1018. doi: 10.1104/pp.011001
McKay, S. A. B., Hunter, W. L., Godard, K. A., Wang, S. X., Martin, D. M., Bohlmann, J., et al. (2003). Insect attack and wounding induce traumatic resin duct development and gene expression of (-)-pinene synthase in Sitka spruce. Plant Physiol. 133, 368–378. doi: 10.1104/pp.103.022723
Morris, H., Brodersen, C., Schwarze, F. W. M. R., Jansen, S. (2016). The parenchyma of secondary xylem and its critical role in tree defense against fungal decay in relation to CODIT model. Front. Plant Sci. 7, 1665. doi: 10.3389/fpls.2016.01665
Nagy, N. E., Franceschi, V. R., Solheim, H., Korkene, P., Christiansen, E. (2000). Wound-induced traumatic resin duct development in stems of Norway spruce (Pinaceae): Anatomy and cytochemical traits. Am. J. Bot. 87, 302–313. doi: 10.2307/2656626
Piqueras, S., Füchtner, S., Oliveira, R. R., Gómez-Sánchez, A., Jelavić, S., Keplinger, T., et al. (2020). Understanding the formation of heartwood in Larch using sunchrotron infrared imaging combined with multivariate analysis and atomic force microscope infrared spectroscopy. Front. Plant Sci. 10. doi: 10.3389/fpls.2019.01701
Reiterer, A., Burgert, I., Sinn, G., Tschegg, S. (2002). The radial reinforcement of the wood structure and its implication on mechanical and fracture mechanical properties - A comparison between two tree species. J. Mater. Sci. 37, 935–940. doi: 10.1023/A:1014339612423
Schmidt, A., Nagel, R., Krekling, T., Christinsen, E., Gershenzon, J., Krokene, P. (2011). Induction of isoprenyl diphosphate synthases, plant hormones and defense signaling genes correlates with traumatic resin duct formation in Norway spruce (Picea abies). Phytochemistry 69, 49–57. doi: 10.1016/j.phytochem.2007.06.022
Secchi, F., Pagliarani, C., Zwienieck, M. A. (2017). The functional role of xylem parenchyma cells and aquaporins during recovery from severe water stress. Plant Cell Environ. 40, 858–871. doi: 10.1111/pce.12831
Slack, A. W., Kane, J. M., Knapp, E. E., Rosemary, L. S. (2017). Contrasting impacts of climate and competition on large sugar pine growth and defense in a fire-excluded forest of the Central Sierra Nevada. Forests 8, 244. doi: 10.3390/f8070244
Słupianek, A., Dolzblasz, A., Sokołowska, K. (2021). Xylem parenchyma-role and relevance in wood functioning in trees. Plants 10, 1247. doi: 10.3390/plants10061247
Smith, R. A., Schuetz, M., Roach, M., Mansfield, S. D., Ellis, B., Samuels, L. (2013). Neighboring parenchyma cells contribute to Arabidopsis xylem lignification, while lignification of interfascicular fibers is cell autonomous. Plant Cell 10, 3988–3999. doi: 10.1105/tpc.113.117176
Spicer, R. (2014). Symplasmic networks in secondary vascular tissues: Parenchyma distribution and activity supporting long-distance transport. J. Exp. Bot. 65, 1829–1848. doi: 10.1093/jxb/ert459
Stubbs, J., Roberst, D. R., Outcalt, K. W. (1984). “Chemical stimulation of lightwood in southern pines,” in Southeastern Forest Experiment Station, Asheville, Technical Report (Asheville, NC, U.S.: General United States Department of Agriculture, Forest Service), SE–25. 51 p. Available at: https://www.fs.usda.gov/research/treesearch/916#.
Tomasella, M., Petrussa, E., Petruzzellis, F., Nardini, A., Casolo, V. (2019). The possible role of non-structural carbohydrates in the regulation of tree hydraulics. Int. J. Mol. Sci. 21, 144. doi: 10.3390/ijms21010144
Tulik, M., Wojtan, R., Jura-Morawiec, J. (2022). Theoretical considerations regarding the functional anatomical traits of primary and secondary xylem in dragon tree trunk using the example of. Dracaena draco. Planta 256, 52. doi: 10.1007/s00425-022-03966-4
Tuller, J., Marquis, R. J., Andrade, S. M. M., Monteiro, A. B., Faria, L. D. B. (2018). Trade-offs between growth, reproduction, and defense in response to resource availability manipulations. PloS One 13, e0201873. doi: 10.1371/journal.pone.0201873
Turner, G. (1999). A brief history of the lysigenous gland hypothesis. Bot. Rev. 65, 76–88. doi: 10.1007/BF02856558
Vázquez-González, C., Zas, R., Erbilgin, N., Ferrenberg, S., Rozas, V., Sampedro, L. (2020). Resin ducts as resistance traits in conifers: linking dendrochronology and resin-based defenses. Tree Physiol. 40, 1313–1326. doi: 10.1093/treephys/tpaa064
Wang, X. H., Zhang, C., Yang, L. L., Gomes-Laranjo, J. (2011). Production of dragon's blood in Dracaena cochinchinensis plants by inoculation of Fusarium proliferatum. Plant Sci. 180, 292–299. doi: 10.1016/j.plantsci.2010.09.007
Wang, X. H., Zhang, C., Yang, L. L., Yang, X. H., Lou, J. D., Cao, Q. E., et al. (2010). Enhanced dragon’s blood production in Dracaena cochinchinensis by elicitation of Fusarium oxysporum strains. J. Med. Plants Res. 4, 2633–2640. doi: 10.5897/JMPR09.573
Watss, S., Kaur, S., Kariyat, R. (2023). Revisiting plant defense-fitness trade-off hypotheses using Solanum as a model genus. Front. Ecol. Evol. 10. doi: 10.3389/fevo.2022.1094961
Wiedenhoeft, A., Cmiller, R. B., Theim, T. J. (2003). Analysis of three microscopic characters for separating the wood of Pinus contorta and P. ponderosa. IAWA J. 24, 257–267. doi: 10.1163/22941932-90001595
Wiedenhoeft, A. C., Miller, R. B. (2002). Brief comments on the nomenclature of softwood axial resin canals and their associated cells. IAWA J. 23, 299–3303. doi: 10.1163/22941932-90000305
Wolter, K. E., Zinkel, D. F. (1984). Observations of the physiological mechanisms and chemical constituents of induced oleoresin synthesis in Pinus resinosa. Can. J. For. Res. 14, 452–458. doi: 10.1139/x84-080
Wu, H., Hu, Z. (1997). Comparative anatomy of resin ducts of the Pinaceae. Trees 11, 135–143. doi: 10.1007/s004680050069
Xu, B., Chao, J., Yang, Z., Li, W. (2018). The occurrence of resin canals in branches of Cedrus deodara with different inclination angles. Wood Sci. Technol. 52, 505–517. doi: 10.1007/s00226-017-0964-z
Xu, Y., Zhang, K., Zhang, Z., Liu, Y., Lv, F., Sun, P., et al. (2022). A chromosomal-level genome assembly for Dracaena cochinchinensis reveals the molecular basis of its longevity and formation of dragon’s blood. Plant Commun. 3, 100456. doi: 10.1016/j.xplc.2022.100456
Keywords: resin, secretory cells, ducts, conifers, Dracaena spp., defense mechanism
Citation: Tulik M and Jura-Morawiec J (2023) An arrangement of secretory cells involved in the formation and storage of resin in tracheid-based secondary xylem of arborescent plants. Front. Plant Sci. 14:1268643. doi: 10.3389/fpls.2023.1268643
Received: 28 July 2023; Accepted: 21 August 2023;
Published: 05 September 2023.
Edited by:
Xupo Ding, Chinese Academy of Tropical Agricultural Sciences, ChinaReviewed by:
Guillermo Angeles, Instituto de Ecología (INECOL), MexicoRyogo Nakada, Forestry and Forest Products Research Institute, Japan
Copyright © 2023 Tulik and Jura-Morawiec. This is an open-access article distributed under the terms of the Creative Commons Attribution License (CC BY). The use, distribution or reproduction in other forums is permitted, provided the original author(s) and the copyright owner(s) are credited and that the original publication in this journal is cited, in accordance with accepted academic practice. No use, distribution or reproduction is permitted which does not comply with these terms.
*Correspondence: Mirela Tulik, bWlyZWxhX3R1bGlrQHNnZ3cuZWR1LnBs
†ORCID: Mirela Tulik, orcid.org/0000-0003-4021-6546
Joanna Jura-Morawiec, orcid.org/0000-0002-0143-5853