- Plant Science Laboratory, Cranfield University, Cranfield, United Kingdom
Table grapes are considered non-climacteric fruit, not showing a rapid increase in respiration rate and ethylene production during ripening. Previous research has suggested that abscisic acid (ABA) may have a more crucial role in grape postharvest behaviour. This study aimed to identify biomarkers of postharvest resilience and flavour life of imported table grapes. An experiment was designed to determine i) the role of ABA and catabolites on grape berry senescence; ii) the spatial distribution of these hormones within the grape berry, and iii) the effect of 1-MCP and storage temperature on its postharvest quality. Hence, the use of an ethylene inhibitor, 1-methylcyclopropane (1-MCP), during table grape storage was investigated. Table grapes (Vitis vinifera L.) cv. ‘Krissy’ were subjected to i) control (untreated); and ii) 1-MCP (1 µL L-1; 12 hours; 15°C) and stored under two scenarios: i) 15 days at 0.5°C, followed by five days at 5.5°C to simulate shelf-life; and ii) 20 days at 5.5°C to simulate a higher storage temperature followed by shelf-life. Physiological (i.e. mould incidence, skin colour, firmness, respiration rate) and biochemical analysis (i.e. individual sugars, organic acids, abscisic acid and catabolites) were performed. Grapes subjected to 5.5°C showed significantly higher mould incidence at the end of the shelf-life compared to 0.5°C storage temperature (12.6% vs. 3.1%). Also, and for the first time, the spatial distribution of ABA during the senescence of table grapes was profiled; the distal section had three times more ABA and metabolites than the proximal. We demonstrated that senescence processes were initiated after a significant increase in respiration rate (from 1 to 2.8 mL CO2 kg-1 h-1), and that ABA could be considered a biomarker for table grapes senescence, since an ABA peak preceded the increase in respiration rate, mould incidence, organic acids, and sucrose hydrolysis during postharvest storage; and coincided with a decrease in berry firmness. These findings are of significant importance for the industry as understanding how ABA regulates both senescence processes and quality changes during postharvest cold storage of tables grapes can improve the consistency and reduce waste and consumer complaints.
1 Introduction
Table grapes follow a non-climacteric ripening pattern, and thus do not show a burst in respiration and ethylene production at the onset of ripening (Zenoni et al., 2023). However, it has been reported that ethylene is somehow involved in regulating grape ripening processes, such as the decrease in acids and the accumulation of sugars and anthocyanins during berry development (Yan et al., 2023). Nonetheless, many studies have highlighted a more influential role of ABA on these ripening processes (Sun et al., 2010; McAtee et al., 2013; Pilati et al., 2017; Coelho et al., 2019; Gao-Takai et al., 2019; Zenoni et al., 2023).
There are three ABA peaks, which occur during grape berry growth, at ripening, and at postharvest senescence, the latter being an irreversible process if a certain ABA threshold is achieved. ABA can be esterified to ABA-GE and stored in the vacuoles to respond to stresses, hydroxylated to 7’ -, 8’ -, or 9’-OH-ABA, or isomerised to PA or DPA (Jia et al., 2017). Although some research has been done on ABA and ABA metabolites during ripening and senescence in fruits like cherries (Serradilla et al., 2019) and strawberries (Tosetti et al., 2020), little is known about the role of ABA and the different ABA catabolites during grape berry postharvest senescence. Previous research has highlighted the role of phytohormones as regulators of defence mechanisms against pathogens responsible for e.g. decay (Amaro et al., 2023). In particular, ABA has been found to be associated to grapevine susceptibility towards pathogens such as Erysiphe necator (Coelho et al., 2019).
The ethylene antagonist 1-methylcyclopropene (1-MCP) has been widely used in postharvest studies to better understand the ripening process of fresh produce (Watkins, 2006). However, there is a lack of information on the effect of 1-MCP on grapes postharvest quality and senescence. Moreover, the results obtained from the few published studies are not conclusive. Wang et al. (2019) applied 1-MCP immediately after harvest and they found that 1-MCP was able to reduce decay, minimised abscission and inhibited raquis browning in table grape branches (‘Kyoho’ and ‘Yongyou NO.1’). In another study, Bellincontro et al. (2006) investigated the effect of 1-MCP on stored grapes. Their results showed that 1-MCP lowered ethylene production, whilst no change in grape berry respiration rate (RR) was observed. Moreover, 1-MCP-treated berries showed a higher incidence of grey mould and a significant loss of terpenols and esters. More recently, Silva et al. (2013) applied different doses of 1-MCP (0, 500, 1,000 and 2,000 nL L-1 for 12 hours) to stored berries and found that the treatment maintained the ascorbic acid and total anthocyanins contents without affecting berry firmness and decreased berry drop as the doses increased.
Therefore, despite the efforts made to understand the mechanisms behind the ripening and senescence of non-climacteric fruit, the role of the different ripening hormones remains unclear. The objective of this study was three-fold: i) to understand the role of ABA and ABA catabolites on grape berry senescence; ii) to study the spatial distribution of these hormones within the grape berry during senescence and its effect on berry quality, and iii) to evaluate the effect of 1-MCP and storage temperature on the postharvest quality of ‘Krissy’ table grapes.
2 Materials and methods
2.1 Plant material
The experiment was conducted on grapes (Vitis vinifera L.) from a commercial vineyard of drip irrigated 3-year-old ‘Krissy’ vines grafted onto Paulsen 1103 (3.5 m × 3 m spacing) located in Murcia (SE Spain).
The grape clusters (n = 200) were monitored during the 2018 growing season and harvested at their optimal commercial maturity according to market standards. The samples were then transported to the Plant Science Laboratory at Cranfield University (UK) by refrigerated lorry (0.5°C and 85% RH) within five days of harvest as per standard commercial practice.
2.2 Experimental design
Grape clusters showing decay or mechanical damage were discarded, and sound fruit was placed in cold storage (0.5°C and 85% RH) overnight to acclimatise. Then, two treatment groups were considered: i) control (untreated); and ii) 1-MCP, where one hundred grape clusters were placed inside 100 L hermetically sealed boxes and treated with 1-MCP (1 µL L-1) for 12 hours at 15°C, as described in Foukaraki et al. (2016).
Following the 1-MCP application, two storage scenarios were considered: i) scenario 1: 15 days at 0.5°C and 85% RH as per normal storage conditions, followed by five days at 5.5°C and 85% RH to simulate shelf-life; and ii) scenario 2: 20 days at 5.5°C and 85% RH to simulate a higher storage temperature followed by shelf-life.
Three replicates per treatment group and storage scenario were analysed every five days. Each replicate consisted of three grape clusters from which thirty random berries were analysed (n = 90).
2.3 Physiological analysis
2.3.1 Mould incidence, berry skin colour and berry firmness
Mould incidence was recorded as the percentage of mouldy berries per replicate, according to Youssef et al. (2019). Afterwards, grape skin colour was determined using a hand-held tristimulus colourimeter with a D65 illuminant and 8 mm light aperture (model CR-400 Chroma Meter, Konica Minolta Inc., Cheshire, UK). The colourimeter was calibrated with a white plate, and it provided the lightness (L*), chroma index, and Hue angle values of the samples. These parameters are widely used as a measure of ripeness (Conesa et al., 2016).
The firmness of individual berries was determined using a uniaxial testing machine calibrated with a 5 N cell (Instron, model 5542, MA, USA). Maximum compressive load in Newtons (N) was measured by compressing the berry 1 mm deep with a 4 mm diameter probe at a speed of 100 mm min-1 (Alamar et al., 2017).
2.3.2 Respiration rate
Respiration Rate (RR) was measured as described in Collings et al. (2018). Samples were placed in 3 L airtight glass jars and dry clean air (300 mL min-1) was passed through the jars into a Sable Respirometry System (model 1.3.8 Pro, Sable Systems International, Las Vegas, USA). Each sample jar (n = 3 replicates per treatment) was measured for 2 min to achieve a stable reading. An empty jar (continuous air for 1 min) was used as a baseline to avoid cross-contamination between treatment measurements. The rate of CO2 production was expressed in mL kg-1 h-1.
2.4 Biochemical analysis
For biochemical analysis, berries were cut transversely at ca. one third from the top of the berry. Before biochemical analysis, the samples were snap-frozen in liquid nitrogen and placed in a -50°C CoolSafe freeze-dryer (Scanvac, Labogene, Denmark) for seven to ten days. After this, the freeze-dried samples were ground and kept at -40°C until analysis (García-Pastor et al., 2021b).
2.4.1 Individual sugars
Extraction and analysis of individual sugars (glucose, fructose, and sucrose) were done following Davis et al. (2007). Briefly, 150 mg of freeze-dried fruit samples were extracted with 3 mL of 62.5% (v/v) aqueous High Performance Liquid Chromatography (HPLC)-grade methanol in a water bath at 55°C for 15 min, vortexing every 5 min for 30 s. The extract was then filtered through 0.2 μm PTFE filters and stored in 2 mL HPLC vials at -40°C until analysis. Before analysis, the extracts were diluted 1:9 with HPLC grade water.
Detection was performed using a Refractive Index Detector (RID) coupled to an Agilent 1200 series HPLC system (Agilent Technologies, Germany) with a Phenomenex Rezex RCM monosaccharide Ca+2 (8%) ion exclusion column (300 mm x 7.8 mm), 8 μm particle size, fitted with a Phenomenex Carbo Ca+4 mm x 3 mm guard column (Phenomenex, CA). The column oven temperature was set at 80°C and the refractive index detector at 50°C. The mobile phase used was HPLC-grade water at a flow rate of 0.6 mL min-1, and the autosampler injection volume was 20 μL. A 6-point calibration curve ranging from 0.05 to 2.5 mg mL-1 was used for quantification, and the results were expressed as mg g-1 dry weight (DW).
2.4.2 Organic acids
Analysis of non-volatile organic acids was done as described in Terry et al. (2007) with slight modifications. Briefly, freeze-dried samples were dissolved in 3% aqueous metaphosphoric acid, kept at room temperature for 10 min, filtered through 0.2 μm cellulose filters into HPLC vials, and analysed immediately after extraction.
The acids content was determined using an Agilent 1200 series HPLC system with an Alltech Prevail Organic Acid 250 mm x 4.6 mm, 5 μm particle size column fitted with an Alltech Prevail Organic Acid 7.5 mm x 4.6 mm, 5 μm particle size guard column (Alltech, CA, USA). The column oven temperature was set at 35°C, and the compounds were detected by a diode array detector (DAD) set at 210 nm. The mobile phase used was 25 mM KH2PO4 HPLC-grade water at a flow rate of 1.5 mL min-1, and the autosampler injection volume was 20 μL. A 5-point calibration curve ranging from 0.05 to 1 mg mL-1 with a mixture of tartaric and malic acids was used for quantification, and the results were expressed as mg g-1 DW.
2.4.3 Abscisic acid and catabolites
ABA and ABA catabolites concentration was determined by following Serradilla et al. (2019) with modifications. Freeze-dried samples (5 mg) were mixed with 500 μL of methanol/formic acid/water (60:5:35, v/v) and 10 μL of the internal standards mix (100 ng mL-1) in a 1.5 mL Eppendorf tube, and shaken in a Star Beater (R&L Slaughter Ltd., Essex, UK) at 30 Hz for 2 min. The internal standard mix was made of the labelled forms of the compounds: (-)-5,8'8'8'-d4-abscisic acid (d4-ABA); (-)-7',7',7'-d3-phaseic acid (d3-PA); (-)-7',7',7'-d3-dihydrophaseic acid (d3-DPA); (+)-4,5,8',8',8'd5-abscisic acid glucose ester (d5-ABA-GE); (±)-5,8',8',8'-d4-7'-dihydroxy-ABA (d4−7 -OH-ABA). The tubes were then placed in dry ice and kept in darkness for 20 min. Afterwards, the tubes were centrifuged at 4°C and 14,000 rpm for 10 min, then transferred to 15 mL falcon tubes and freeze-dried overnight. The extracts were reconstituted with 500 μL of acetonitrile/formic acid/water (10:0.1:89.9, v/v), vortexed for 1 min, sonicated for 1 min, and centrifuged at 4°C and 4,500 rpm for 1 min. The samples (20 μL) were then injected on a Phenomenex Luna C18 100 mm x 2 mm, 3 μm with guard column at 40 °C, and analysed by an Agilent 1200 series HPLC system coupled to a Q-Trap 6500 mass spectrometer (AB Sciex, MA, USA). The concentration of ABA and ABA metabolites was quantified based on Morris et al. (2018) methodology. A 6-point calibration curve ranging from 0.1 to 100 ng mL-1 was used for quantification, and the results were expressed as ng g-1 DW.
2.5 Statistical analysis
All statistical analyses were carried out using Statistica for Windows version 13 (Dell Inc., USA). Data were subjected to normality tests and outliers were removed if necessary. An analysis of variance (ANOVA) was performed to test the experimental hypotheses. When significant differences were found, the data were subjected to a Fisher’s post-hoc test. Least significant differences (LSD) were calculated for each analysis (p < 0.05) and shown on the graphs when the interaction was significant. When factors were significant, LSD values were included in the corresponding caption for comparison. The differences between scenario/temperature, treatment (± 1-MCP), storage time and berry section (for biochemical analysis) were studied. Figures were plotted in SigmaPlot 14 (Systat Software Inc., USA).
3 Results
3.1 Mould incidence, berry firmness, and berry skin colour
Mould incidence in grapes was solely affected by storage temperature, being five times more severe in grapes stored at 5.5°C than the incidence found on grapes stored at 0.5°C, which did not show any decay until the end of storage. In the case of firmness, it was observed that storage time was the key factor involved, showing a decline during storage even for berries subjected to 1-MCP treatment (Figure Captions Figure 1). Chroma index progressively decreased over storage time (Supplementary Figure 1), and Hue angle significantly increased at the end of storage, with berries acquiring a dull, dark red skin colour. These changes were linked to storage time, playing 1-MCP and temperature a non-significant role.
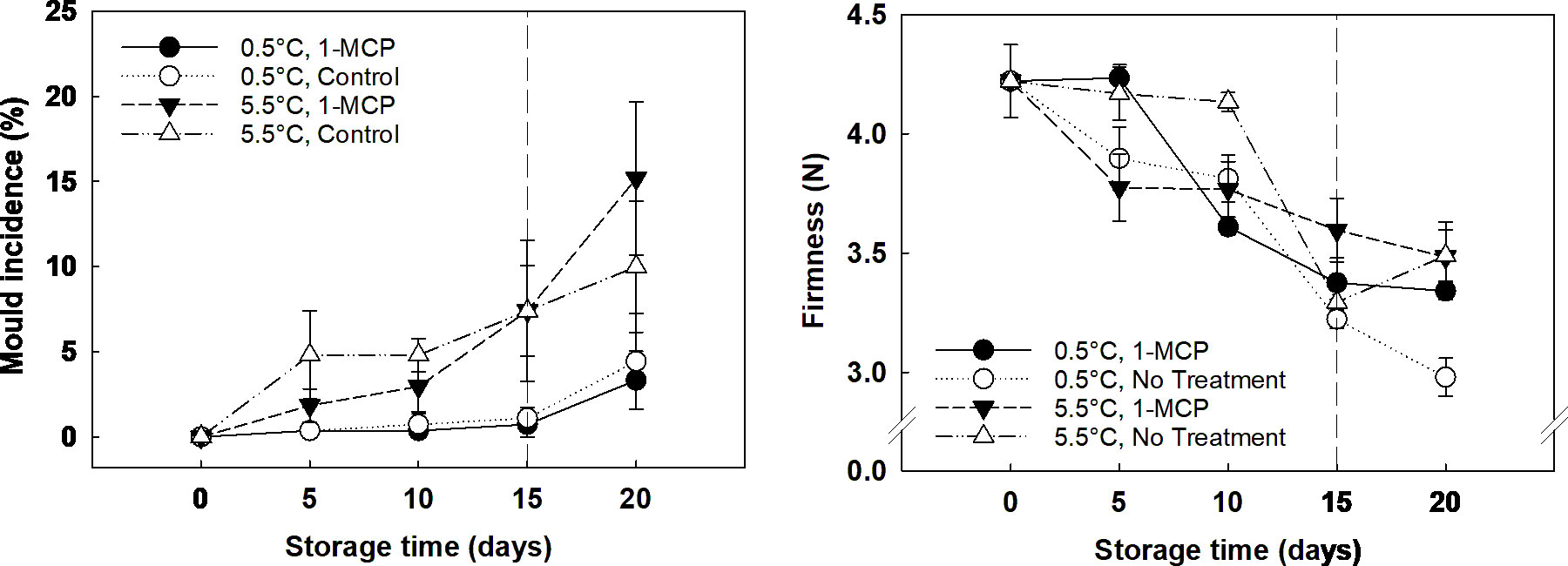
Figure 1 Mould incidence (%; left) and firmness (N; right) of ´Krissy´ grapes. Table grapes were treated on arrival (5 days postharvest) with 1-MCP (1 µL L-1 for 12 h at 15°C [1-MCP]) or without 1-MCP (air [control]) prior to storage and subjected to two postharvest storage scenarios: i) 15 days at 0.5°C and 85% RH, followed by 5 days at 5.5°C, and ii) 20 days at 5.5°C and 85% RH. The vertical stripped line shows when all samples were stored at 5.5°C. Data represents means (n = 90 berries) ± standard error. LSD0.05 [mould incidence] temperature x storage time = 3.71. LSD0.05 [firmness] storage time = 0.31.
3.2 Respiration rate
The treatment with 1-MCP significantly decreased the RR of the grape berries stored at 5.5°C compared to control (2.3 and 2.6 and mL CO2 kg-1 h-1, respectively). However, 1-MCP did not affect grapes stored at 0.5°C. The CO2 production of grape berries significantly decreased (p = 0.000000) at the beginning of the storage, from 1.5 to 0.8 mL CO2 kg-1 h-1 at day 0 and day 10, respectively. Then, RR significantly increased to 3.0 mL CO2 kg-1 h-1 at day 15. Storage temperature was the main effect for RR: grapes stored at 5.5°C showed significantly higher RR (p = 0.000000; 2.5 mL CO2 kg-1 h-1) than grapes stored at 0.5°C (1.4 mL CO2 kg-1 h-1; Figure 2).
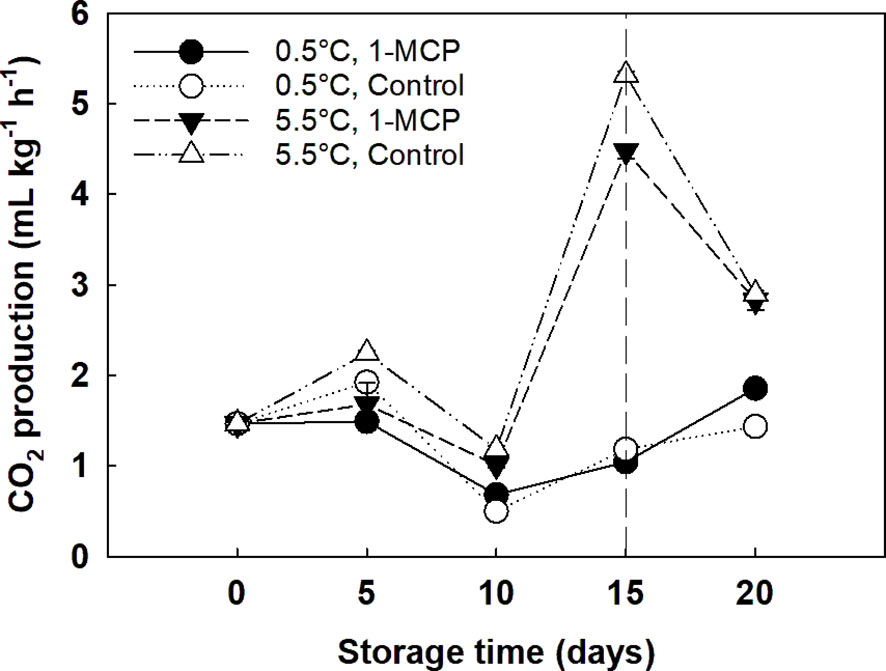
Figure 2 Respiration rate (RR) expressed as CO2 production (mL kg-1 h-1) of ´Krissy´ grapes. Table grapes were treated with 1-MCP (1 µL L-1 for 12 h at 15°C [1-MCP]) or without 1-MCP (air [control]) prior to storage and subjected to two postharvest storage scenarios: i) 15 days at 0.5°C and 85% RH, followed by 5 days at 5.5°C, and ii) 20 days at 5.5°C and 85% RH. The vertical stripped line shows when all samples were stored at 5.5°C. Data represents means (n = 90 berries) ± standard error. LSD0.05 for the interaction temperature x storage time = 0.3; LSD0.05 for the interaction treatment x storage time = 0.3.
3.3 Individual sugars
The main sugars found in the grape berry were fructose and glucose, while the concentration of sucrose was ten times lower (Figure 3). Storage time and temperature were the key factors affecting sugar content, whilst 1-MCP only had a significant effect at the end of shelf-life. At this time, the distal section of the treated berries stored at 0.5°C showed a significantly lower content of fructose and glucose than the rest of the samples. Sucrose concentration significantly increased from day 5 (20 mg g-1 DW) to day 10 (26 mg g-1 DW) of storage, and then decreased to similar values of those recorded at day 5 for both storage temperatures and sections.
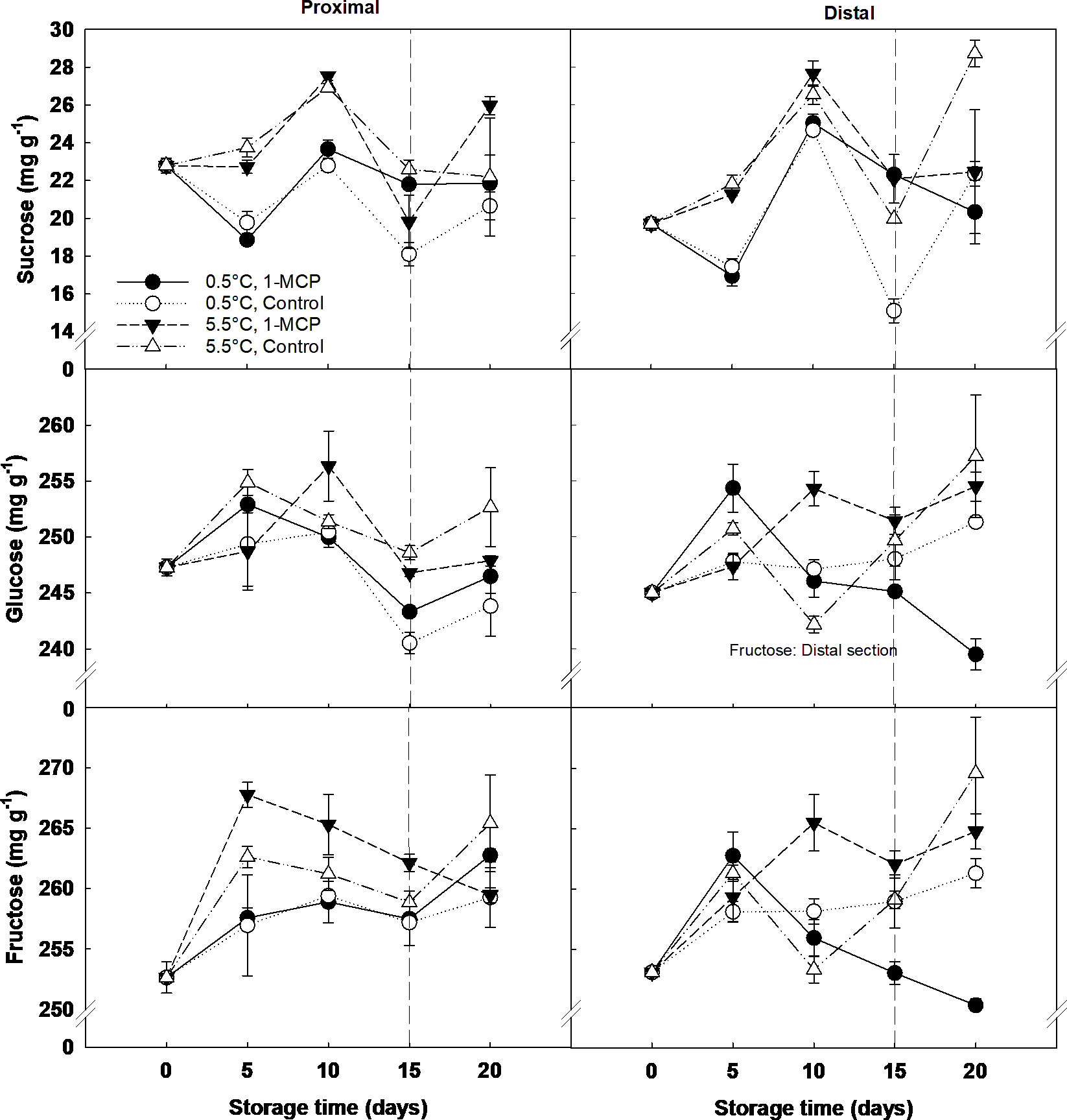
Figure 3 Individual sugars (sucrose, fructose and glucose) expressed as mg g-1 DW in the proximal (left) and distal (right) sections of ´Krissy´ grapes. Table grapes were treated with 1-MCP (1 µL L-1 for 12 h at 15°C [1-MCP]) or without 1-MCP (air [control]) prior to storage and subjected to two postharvest storage scenarios: i) 15 days at 0.5°C and 85% RH, followed by 5 days at 5.5°C and ii) 20 days at 5.5°C and 85% RH. The vertical stripped line shows when all samples were stored at 5.5°C. Data represents means (n = 90 berries) ± standard error. LSD0.05 [sucrose, glucose, fructose] for storage time =1.51, 3.26, 3.28, respectively. LSD0.05 [sucrose, glucose, fructose] for temperature =10.97, 1.98, 1.99, respectively.
3.4 Organic acids
The concentration of both tartaric and malic acid experienced a significant decrease from day 0 to day 10, independent of the berry section sampled, and then remained constant until the end of shelf-life. Significant differences between temperatures and treatments were only observed at day 5, with treated grapes stored at 0.5°C showing a higher level of malic acid than the rest of the samples. The effect of 1-MCP on the tartaric and malic acid content was not significant at 5.5°C; however, it did affect the organic acids content at the beginning of storage when the berries were kept at 0.5°C. Thus, malic acid levels on day 5 were significantly higher for treated grapes stored at 0.5°C than control, and this difference was more pronounced in the distal section of the berries. In contrast, the application of 1-MCP resulted in a significant decrease in the overall content of tartaric acid in table grape berries during the first five days of storage at 0.5°C. Yet this differences between treatments disappeared from day 10 onwards. When looking at the spatial distribution of organic acids, a higher tartaric acid concentration was found in the proximal section at the beginning of storage - day 5 - (average ca. 40 mg g-1 DW) compared to the distal section (average ca. 28 mg g-1 DW); while malic acid showed the opposite trend - more concentrated in the distal section (ca. 18 vs. 12 mg g-1 DW, respectively) (Figure 4).
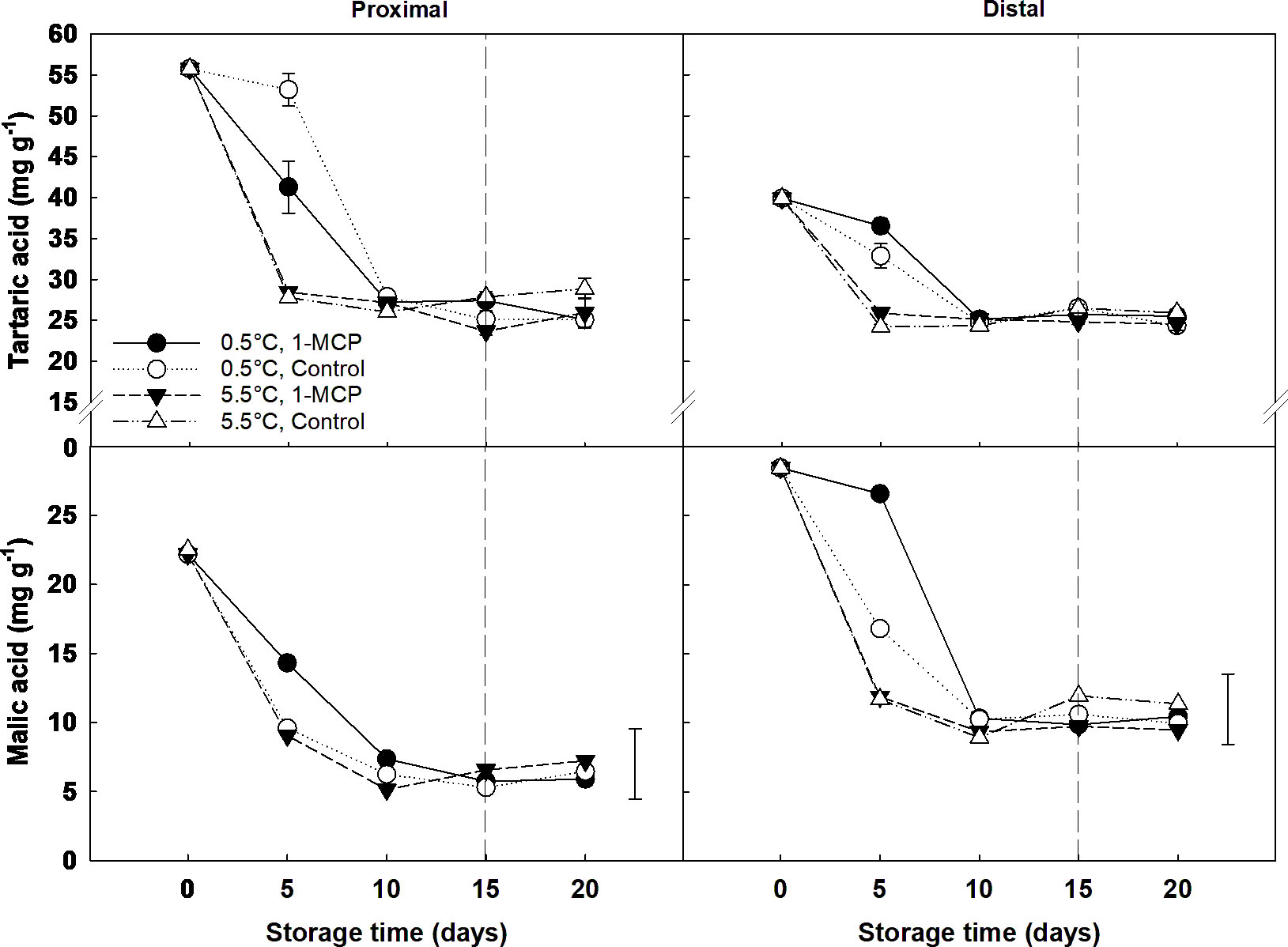
Figure 4 Organic acids (tartaric and malic acids) expressed as mg g-1 DW in the proximal (left) and distal (right) sections of ´Krissy´ grapes. Table grapes were treated with 1-MCP (1 µL L-1 for 12 h at 15°C [1-MCP]) or without 1-MCP (air [control]) prior to storage and subjected to two postharvest storage scenarios: i) 15 days at 0.5°C and 85% RH, followed by 5 days at 5.5°C and ii) 20 days at 5.5°C and 85% RH. The vertical stripped line shows when all samples were stored at 5.5°C. Data represents means (n = 90 berries) ± standard error. LSD0.05 [tartaric acid] for the interaction temperature x section x storage time = 4.35; LSD0.05 [malic acid] for the interaction temperature x treatment x section x storage time = 5.09.
3.5 Abscisic acid and catabolites
Overall, ABA and its catabolites significantly increased throughout cold storage and decreased by the end of shelf-life (Figure 5; Supplementary Figure 2). Grapes stored at 5.5°C had 20% lower ABA and 15% higher ABA-GE content than grapes stored at 0.5°C. The distal section of the berries showed a significantly higher (3-fold) ABA content than the proximal section. However, the ABA content in the distal section of 1-MCP-treated grapes was approximately half (238 ng g-1 DW) of that found in control berries (435 ng g-1 DW) at the end of storage (Figure 5). Phaseic acid was not detected in our samples.
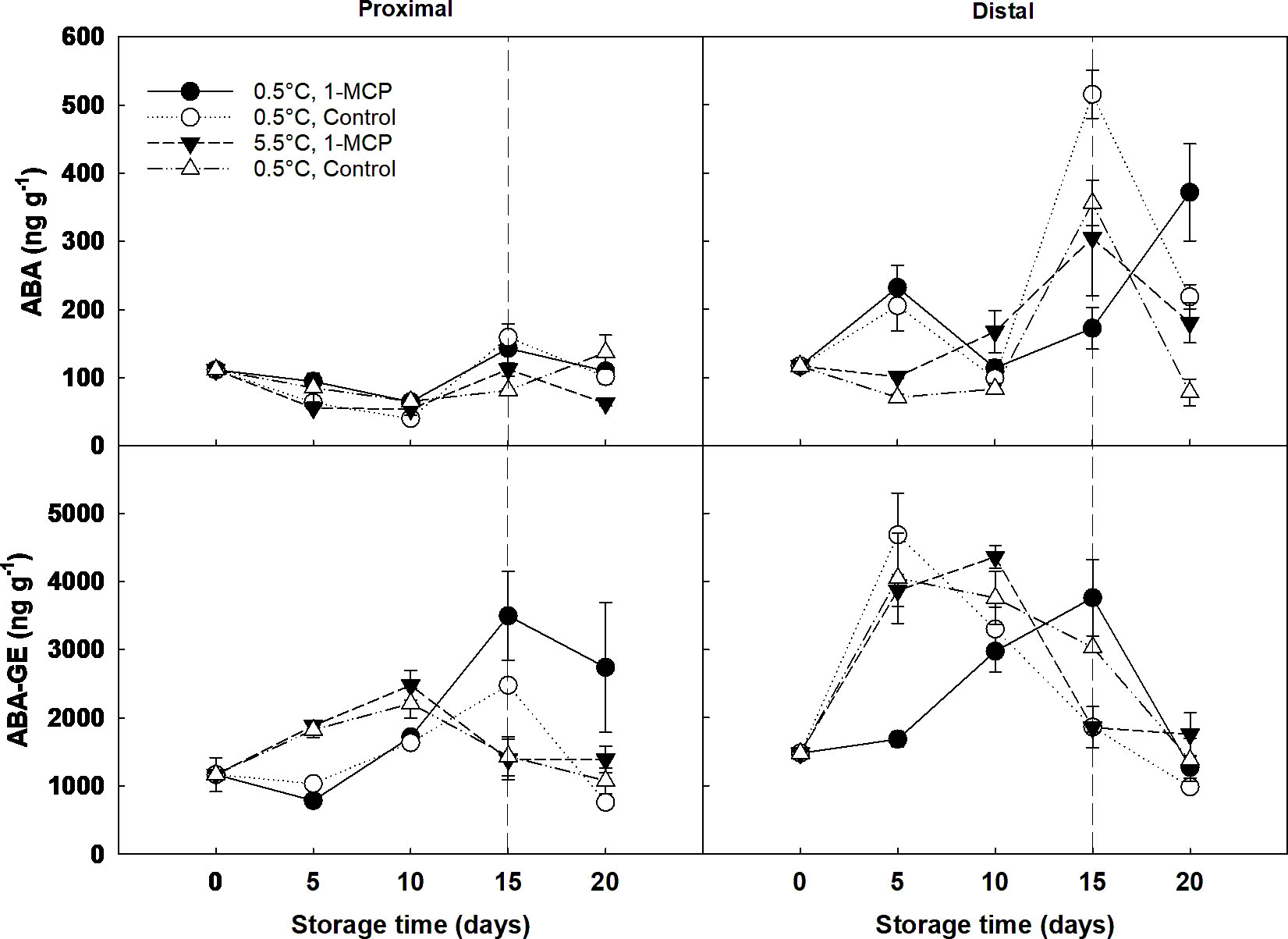
Figure 5 Abscisic acid (ABA) and the ABA metabolite ABA-glucose ester (ABA-GE) expressed as ng g-1 DW in the proximal (left) and distal (right) sections of ´Krissy´ grapes. Table grapes were treated with 1-MCP (1 µL L-1 for 12 h at 15°C [1-MCP]) or without 1-MCP (air [control]) prior to storage and subjected to two postharvest storage scenarios: i) 15 days at 0.5°C and 85% RH, followed by 5 days at 5.5°C, and ii) 20 days at 5.5°C and 85% RH. The vertical stripped line shows when all samples were stored at 5.5°C. Data represents means (n = 90 berries) ± standard error. LSD0.05 [ABA] for the interaction storage time x treatment x section = 102.22. LSD0.05 [ABA-GE] for the interaction storage time x section = 956.36; for the interaction storage time x temperature = 102.22.
4 Discussion
4.1 1-MCP application increases mould incidence in ‘Krissy’ table grapes
The ethylene antagonist 1-MCP is a compound that inhibits ethylene perception by binding to and hence inactivating the ethylene binding protein (EBP; Kyaw et al., 2023). Previous studies have shown how 1-MCP can inhibit senescence related processes in non-climacteric fruits such as grapes (Li et al., 2016a) and sweet cherries (Serradilla et al., 2019). Bellincontro et al. (2006) demonstrated that a single dose of 1-MCP (1 mg L-1) applied for 15 h at 20°C in 300 L boxes during postharvest storage reduced ethylene production yet only for two days after treatment application. In addition, there was an increase in grey mould incidence after six days of storage at 20°C (Bellincontro et al., 2006).
It has been previously reported that ethylene is required to upregulate defence-related genes against fungal attack in Arabidopsis (Ferrari et al., 2003). Therefore, blocking ethylene production with 1-MCP could result in a higher mould incidence at the end of shelf life, as the conditions for mould growth are more favourable because of berry firmness loss and degradation of organics acids and sucrose. Moreover, the higher mould incidence found at the end of the storage in 1-MCP treated grapes was mirrored by lower concentrations of fructose and glucose in the distal section of the berry. It can be hypothesised that these monosaccharides were being used as substrate by the fungi. Further research should be carried out to validate this hypothesis. Other ethylene removal methods for the storage and transportation of fruit and vegetables have been reviewed recently (Ying et al., 2021). Thus, while 1-MCP inhibits ethylene, other technologies like ozone, low-temperature catalytic oxidation, and plasma catalysis act by eliminating ethylene. For instance, Feliziani et al. (2014) demonstrated that storing ‘Crimson Seedless’ table grapes in chambers containing 0.1 μL L-1 ozone or higher at 2°C for three weeks reduced grey mould incidence by 65% after 5 to 8 weeks of storage.
In the study herein, treated grapes stored at 5.5°C were highly affected by mould incidence at the end of shelf-life. This result agrees with the above-mentioned study (Bellincontro et al., 2006) and with previously published research on non-climacteric fruit like strawberry (Bower et al., 2003). It has been demonstrated previously that local resistance to Botrytis cinerea (grey mould) in Arabidopsis requires the upregulation of defence genes that use ethylene as a secondary messenger (Ferrari et al., 2003). Therefore, blocking ethylene production with 1-MCP could be stopping the expression of these defence genes and, as a result, decreasing the crop resistance to a fungal attack.
4.2 1-MCP treatment delayed sucrose hydrolysis and the decline in the organic acids during table grape cold storage
Regarding RR, table grapes stored at 5.5°C experienced a significant increase in CO2 production from day 10 to day 15, which was lower in 1-MCP treated grapes than in control. It has been previously proposed that the increase in RR at the onset of ripening occurs due to a change in the berries’ metabolism and that sugars, and not malate, are the primary substrate used for respiration (Famiani et al., 2014). This hypothesis is confirmed by the results seen here, which showed that a lower RR due to the 1-MCP treatment delayed both the hydrolysis of sucrose into fructose and glucose and the decline in the organic acid content compared to untreated grapes. In contrast to these results, Bellincontro et al. (2006) and Li et al. (2016b) found that 1-MCP applied after harvest did not significantly affect the RR of ‘Aleatico’ wine grapes and ‘Thompson Seedless’ white table grapes, respectively. However, it is necessary to highlight that Li et al. (2016b) only measured the RR of five berries clipped off at the end of the pedicel after three and six days from applying the 1-MCP treatment, while in this study the RR of three whole bunches per condition was measured every five days for 20 days. Therefore, while 1-MCP delays the increase in RR and the degradation of organic acids and sugars in table grapes stored at 5.5°C, the treatment is not able to counteract the effect of a higher storage temperature, which results in a higher mould incidence and, hence, the unsuitability of the grapes to be commercialised and accepted by the end consumer. According to our results, the use of 1-MCP has not been proved to have a positive effect on extending the postharvest life of table grapes, therefore, it is recommended to evaluate the effect of other ethylene removal techniques like the ones mentioned above.
4.3 ABA metabolites: the hidden players in grape berry ripening and stress responses
4.3.1 Abscisic acid accumulation can regulate grape berry metabolism
ABA is known to regulate plant growth and developmental processes, such as cell division, seed dormancy, maturation, ripening, and abscission, as well as stress responses to drought, cold, or pathogen attack (Finkelstein, 2013; Jia et al., 2017; Coelho et al., 2019). While a few studies have investigated the profile and role of ABA and its catabolites during the preharvest stages of grape berry development and ripening, less attention has been given to the hormonal profile of berries during postharvest and senescence. According to Coombe and Hale (1973), the onset and rate of berry ripening are a function of ABA accumulation. The authors suggested that berry ripening starts once ABA accumulates to a certain level (viz. 62 ng g-1 fresh weight [FW]), below which the berry will not ripen. In the literature, there is a considerable variation in the value of this peak and the moment in berry development when it appears. For instance, Coombe and Hale (1973) observed that ‘Doradillo’ grape veraison started with an ABA concentration of 62 ng g-1 FW, which then increased to 212 ng g-1 FW 13 days after veraison. In contrast, Sun et al. (2010) reported an ABA peak of 500 ng g-1 FW two days after ‘Muscat Hamburg’ grape veraison and a second ABA peak at similar levels six days after harvest after which the berries started to senesce. In a study by Owen et al. (2009), the ABA concentration in the pulp of immature ‘Merlot’ grape berries was 4,000 ng g-1 DW, while mature berries harvested at commercial maturity had an ABA content of 400 ng g-1 DW. Moreover, the authors found that ABA was catabolised to form ABA-GE by conjugation instead of producing DPA by oxidation at the onset of veraison. However, the mentioned study did not quantify ABA or its catabolites during the postharvest berry senescence.
In this work, ABA accumulation coincided with a decrease in the organic acid content and firmness of the berries and an increase in berry respiration rate, sugar hydrolysis, and disease incidence, which are factors determining quality acceptance for the final consumer (Wolf, 2002; Jianying et al., 2014). These results suggest that the grape berries started to senesce after reaching an ABA concentration higher than 100 ng g-1 DW and that ABA played a role in regulating grape berry metabolism during storage and shelf-life. The crosstalk between ABA and sucrose in regulating fruit ripening processes has been recently reviewed (Durán-Soria et al., 2020; Alferez et al., 2021). Jia et al. (2017) found that treatments with exogenous ABA and sucrose regulate berry ripening of ‘Fujiminori’, a black table grape, and that sucrose can induce the ABA synthesis gene expression. According to Luo et al. (2020), ABA and sucrose play a synergistic role in regulating the ripening of strawberry, a non-climacteric produce. Our results were not conclusive in this area; even though we found a negative correlation between sucrose and ABA, there was not enough evidence to consider this correlation as proof for signalling the triggering of senescence processes.
In addition to regulating sugar levels, ABA is involved in the biosynthesis of secondary metabolites like anthocyanins, which are pigments that give red, purple, and blue colours to plants (Owen et al., 2009; Zhang et al., 2014). For this reason, ABA is applied to coloured grape varieties during berry maturation to improve skin colour development by enhancing the accumulation of anthocyanin biosynthetic enzyme genes (Yamamoto et al., 2015; Domingues Neto et al., 2017). In this study, an increase in the fructose and glucose content coincided with darker berry colour and a higher ABA content by the end of storage and shelf-life, confirming the role of sugars in the stimulation of pigmentation (Durán-Soria et al., 2020). Darker berry colour is related to higher consumer acceptability, and therefore, the understanding of ABA metabolism and its interaction with pigment development is key for the grape industry.
4.3.2 ABA metabolites: unravelling their role in ripening and stress response
ABA-GE concentration was ten times higher than ABA, playing an important role in grape berry ripening, especially on stressed fruit (Zarrouk et al., 2016). The highest peak in ABA-GE accumulation occurred for berries subjected to 1-MCP at 0.5 C with the jump of temperature from storage to shelf life stages, showing an abiotic stress as an adjustment to the changing environment. The general trend for ABA-GE concentration was increasing, linking to berry development (Zarrouk et al., 2016). It has been demonstrated that ABA-GE can regulate ABA accumulation; this is interesting because the increase of ABA concentrations follows a previous increase in ABA-GE concentrations, suggesting that ABA-GE can play a role in ABA synthesis through β-glucosidase in agreement with e.g., López-Carbonell et al. (2009) and Xu et al. (2014).
ABA is stored in the form of ABA-GE in the vacuoles and the apoplast during the early berry developmental stages, being released to the endoplasmic reticulum under water stress or dehydration (Finkelstein, 2013; Jia et al., 2017). Thus, it could be hypothesised that the proximal section would have a higher hormonal content due to dehydration in the abscission zone, as ripening related changes in the cell wall undergo from the formation of a dehiscence zone to the softening of the flesh tissue (McAtee et al., 2013). Contrastingly, here it was found that the concentration of ABA and its metabolites, mainly ABA-GE, was significantly higher (3-fold) in the distal section when compared to the proximal section, confirming the hypothesis of a different hormonal distribution within the grape berry during senescence. Two facts could explain this: firstly, the distal section of the berries had a darker colour than the proximal, and secondly, it was observed that this section also presented a higher mould incidence during the last stages of cold storage and shelf-life.
Conversely, DPA concentration was ten times lower than ABA in agreement with a previous study by García-Pastor et al. (2021a) on ‘Magenta’ table grape. DPA showed an increasing trend, particularly during shelf life as seen in Pogány et al. (2020).
High levels of DPA have been observed during the early developmental stages (Owen et al., 2009). Therefore, a low level of this hormone could potentially indicate an advanced level of ripening or senescence. A larger sample size and a deeper dive in molecular biology pathways could help identify the involvement of DPA in ripening and stress responses (Supplementary Figure 2).
5 Conclusions
The concomitant increase of ABA, respiration rate, and sucrose observed at the end of cold storage confirmed the crucial role of ABA in regulating processes associated with the senescence of the non-climacteric grape berry. 1-MCP delayed the degradation of sugars and organic acids, key contributors to flavour life and therefore becoming a good tool to maintain organoleptic traits. However, whether respiration or the changes in endogenous ABA concentration are the triggers of the different senescence mechanisms undergone in the grape berry needs further investigation. It is also recommended that these studies focus their attention on the distal section of the berries, as it has shown a higher hormonal content than the proximal part. The benefit of ethylene antagonists like 1-MCP on non-climacteric produces during postharvest storage is not entirely evident yet, and further research is suggested.
Data availability statement
The datasets generated and analysed in this study can be found in CORD using the following link http://doi.org/10.17862/cranfield.rd.23727189.
Author contributions
MA: Conceptualization, Data curation, Methodology, Supervision, Visualization, Writing – review & editing. NF: Writing – review & editing. LT: Conceptualization, Funding acquisition, Resources, Supervision, Writing – review & editing. ÁN: Conceptualization, Data curation, Formal Analysis, Methodology, Visualization, Writing – original draft, Writing – review & editing.
Funding
The authors declare financial support was received for the research, authorship, and/or publication of this article. We thank AMFRESH Group and Cranfield University for sponsoring this project through the Cranfield Industrial Partnership PhD Scheme.
Acknowledgments
The Authors would like to thank Sam Franklin for his technical support during the project.
Conflict of interest
The authors declare that the research was conducted in the absence of any commercial or financial relationships that could be construed as a potential conflict of interest.
Publisher’s note
All claims expressed in this article are solely those of the authors and do not necessarily represent those of their affiliated organizations, or those of the publisher, the editors and the reviewers. Any product that may be evaluated in this article, or claim that may be made by its manufacturer, is not guaranteed or endorsed by the publisher.
Supplementary material
The Supplementary Material for this article can be found online at: https://www.frontiersin.org/articles/10.3389/fpls.2023.1266807/full#supplementary-material
References
Alamar, M. C., Collings, E., Cools, K., Terry, L. A. (2017). Impact of controlled atmosphere scheduling on strawberry and imported avocado fruit. Postharvest Biol. Physiol. 134, 76–86. doi: 10.1016/j.postharvbio.2017.08.003
Alferez, F., de Carvalho, D. U., Boakye, D. (2021). Interplay between abscisic acid and gibberellins, as related to ethylene and sugars, in regulating maturation of non-climacteric fruit. Int. J. Mol. Sci. 22 (669), 1–10. doi: 10.3390/ijms22020669
Amaro, R., Diniz, I., Santos, H., Pimentel, D., Rego, C., Mithöfer, A., et al. (2023). Hormone changes in tolerant and susceptible grapevine leaves under powdery mildew infection. J. Plant Growth Regul. 42, 3606–3614. doi: 10.1007/s00344-022-10823-x
Bellincontro, A., Fardelli, A., De Santis, D., Botondi, R., Mencarelli, F. (2006). Postharvest ethylene and 1-MCP treatments both affect phenols, anthocyanins, and aromatic quality of Aleatico grapes and wine. Aust. J. Grape Wine Res. 12 (2), 141–149. doi: 10.1111/j.1755-0238.2006.tb00054.x
Bower, J. H., Biasi, W. V., Mitcham, E. J. (2003). Effects of ethylene and 1-MCP on the quality and storage life of strawberries. Postharvest Biol. Technol. 28 (3), 417–423. doi: 10.1016/S0925-5214(02)00208-9
Coelho, J., Almeida-Trapp, M., Pimentel, D., Soares, F., Reis, P., Rego, C., et al. (2019). The study of hormonal metabolism of Trincadeira and Syrah cultivars indicates new roles of salicylic acid, jasmonates, ABA and IAA during grape ripening and upon infection with Botrytis cinerea. Plant Sci. 283, 266–277. doi: 10.1016/j.plantsci.2019.01.024
Collings, E. R., Alamar Gavidia, M. C., Cools, K., Redfern, S., Terry, L. A. (2018). Effect of UV-C on the physiology and biochemical profile of fresh Piper nigrum berries. Postharvest Biol. Technol. 136, 161–165. doi: 10.1016/j.postharvbio.2017.11.007
Coombe, B. G., Hale, C. R. (1973). The hormone content of ripening grape berries and the effects of growth substance treatments. Plant Physiology 51(4), 629–634. doi: doi.org/10.1104/pp.51.4.629
Conesa, M. R., Falagán, N., de la Rosa, J. M., Aguayo, E., Domingo, R., Pérez Pastor, A. (2016). Post-veraison deficit irrigation regimes enhance berry coloration and health-promoting bioactive compounds in ´Crimson Seedless´ table grapes. Agric. Water Manage. 163, 9–18. doi: 10.1016/j.agwat.2015.08.026
Davis, F., Terry, L. A., Chope, G. A., Faul, C. F. J. (2007). Effect of extraction procedure on measured sugar concentrations in onion (Allium cepa L.) bulbs. J. Agric. Food Chem. 55 (11), 4299–4306. doi: 10.1021/jf063170p
Domingues Neto, F. J., Tecchio, M. A., Pimentel Junior, A., Ferracioli Vedoato, B. T., Pereira Lima, G. P., Roberto, S. R. (2017). Effect of ABA on colour of berries, anthocyanin accumulation and total phenolic compounds of ‘Rubi’ table grape (Vitis vinifera). Aust. J. Crop Sci. 11 (2), 199–205. doi: 10.21475/ajcs.17.11.02.p269
Durán-Soria, S., Pott, D. M., Osorio, S., Vallarino, J. G. (2020). Sugar signalling during fruit ripening. Front. Plant Sci. 11. doi: 10.3389/fpls.2020.564917
Famiani, F., Farinelli, D., Palliotti, A., Moscatello, S., Battistelli, A., Walker, R. P. (2014). Is stored malate the quantitatively most important substrate utilised by respiration and ethanolic fermentation in grape berry pericarp during ripening? Plant Physiol. Biochem. 76, 52–57. doi: 10.1016/j.plaphy.2013.12.017
Feliziani, E., Romanazzi, G., Smilanick., J. L. (2014). Application of low concentrations of ozone during the cold storage of table grapes. Postharvest Biol. Technol. 93, 38–48. doi: 10.1016/j.postharvbio.2014.02.006
Ferrari, S., Vairo, D., Ausubel, F. M., Cervone, F., De Lorenzo, G. (2003). Tandemly duplicated Arabidopsis genes that encode polygalacturonase-inhibiting proteins are regulated coordinately by different signal transduction pathways in response to fungal infection. Plant Cell 15, 93–106. doi: 10.1105/tpc.005165
Finkelstein, R. (2013). Abscisic acid synthesis and response. Arabidopsis book 11, e0166. doi: 10.1199/tab.0166
Foukaraki, S., Cools, K., Chope, G. A., Terry, L. A. (2016). Impact of ethylene and 1-MCP on sprouting and sugar accumulation in stored potatoes. Postharvest Biol. Technol. 114, 95–103. doi: 10.1016/j.postharvbio.2015.11.013
Gao-Takai, M., Katayama-Ikegami, A., Matsuda, K., Shindo, H., Uemae, S., Oyaizu, M. (2019). A low temperature promotes anthocyanin biosynthesis but does not accelerate endogenous abscisic acid accumulation in red-skinned grapes. Plant Sci. 283 (March), 165–176. doi: 10.1016/j.plantsci.2019.01.015
García-Pastor, M. E., Falagán, N., Gine-Bordonaba, J., Wojcik, D. A., Terry, L. A., Alamar, M. C. (2021b). Cultivar and tissue-specific changes of abscisic acid, its catabolites and individual sugars during postharvest handling of flat peaches (Prunus persica cv. platycarpa). Postharvest Biol. Technol. 181, 111688. doi: 10.1016/j.postharvbio.2021.111688
García-Pastor, M. E., Giménez, M. J., Serna-Escolano, V., Guillén, F., Valero, D., Serrano, M., et al. (2021a). Oxalic acid preharvest treatment improves colour and quality of seedless table grape ´Magenta´ upregulating on-vine abscisic acid metabolism, relative VvNCED1 gene expression, and the antioxidant system in berries. Front. Plant Sci. 12. doi: 10.3389/fpls.2021.740240
Jia, H., Xie, Z., Wang, C., Shangguan, L., Qian, N., Cui, M., et al. (2017). Abscisic acid, sucrose, and auxin coordinately regulate berry ripening process of the Fujiminori grape. Funct. Integr. Genomics 17 (4), 441–457. doi: 10.1007/s10142-017-0546-z
Jianying, F., Xia, W., Zetian, F., Weisong, M. (2014). Assessment of consumers’ perception and cognition toward table grape consumption in China. Br. Food J. 116 (4), 611–628. doi: 10.1108/BFJ-04-2012-0101
Kyaw, P. N., Singh, Z., Tokala, V. Y. (2023). 1 H-cyclopropa [b] naphthalene: a novel ethylene antagonist for extending storage life and maintaining quality of climacteric and suppressed climacteric plums. Plant Growth Regul. 1–15. doi: 10.1007/s10725-023-01030-z’
Li, L., Kaplunov, T., Zutahy, Y., Daus, A., Porat, R., Lichter, A. (2016b). The effects of 1-methylcyclopropane and ethylene on postharvest rachis browning in table grapes. Postharvest Biol. Technol. 107, 16–22. doi: 10.1016/j.postharvbio.2015.04.001
Li, L., Lichter, A., Chalupowicz, D., Gamrasni, D., Goldberg, T., Nerya, O., et al. (2016a). Effects of the ethylene-action inhibitor 1-methylcyclopropylene on postharvest quality of non-climacteric fruit crops. Postharvest Biol. Technol. 111, 322–329. doi: 10.1016/j.postharvbio.2015.09.031
López-Carbonell, M., Gabasa, M., Jáuregui, O. (2009). Enhanced determination of abscisic acid (ABA) and abscisic acid glucose ester (ABA-GE) in Cistus albidus plants by liquid chromatography-mass spectrometry in tandem mode. Plant Physiol. Biochem. 47(4), 256–261. doi: 10.1016/j.plaphy.2008.12.016
Luo, Y., Ge, C., Ling, Y., Mo, F., Yang, M., Jiang, L., et al. (2020). ABA and sucrose co-regulate strawberry fruit ripening and show inhibition of glycolysis. Mol. Genet. Genomics 295 (2), 421–438. doi: 10.1007/s00438-019-01629-w
McAtee, P., Karim, S., Schaffer, R., David, K. (2013). A dynamic interplay between phytohormones is required for fruit development, maturation, and ripening. Front. Plant Sci. 4 (APR). doi: 10.3389/fpls.2013.00079
Morris, W. L., Alamar, M. C., Lopez-Cobollo, R. M., Cañete-Castillo, J., Bennett, M., Van der Kaay, J., et al. (2018). A member of the TERMINAL FLOWER 1/CENTRORADIALIS gene family controls sprout growth in potato tubers. J. Exp. Bot. 70 (3), 835–843. doi: doi.org/10.1093/jxb/ery387
Owen, S. J., Lafond, M. D., Bowen, P., Bogdanoff, C., Usher, K., Abrams, S. R. (2009). Profiles of abscisic acid and its catabolites in developing Merlot grape (Vitis vinifera) berries. Am. J. Enology Viticulture 60 (3), 277–284. doi: 10.5344/ajev.2009.60.3.277
Pilati, S., Bagagli, G., Sonego, P., Moretto, M., Brazzale, D., Castorina, G., et al. (2017). Abscisic acid is a major regulator of grape berry ripening onset: New insights into ABA signaling network. Front. Plant Sci. 8. doi: 10.3389/fpls.2017.01093
Pogány, M., Dankó, T., Hegyi-Kaló, J., Kámán-Tóth, E., Réka Szám, D., Hamow, K. A., et al. (2020). Redox and hormonal changes in the transcriptome of grape (Vitis vinifera) berries during natural noble rot development. Plants 11, 864. doi: doi.org/10.3390/plants11070864
Serradilla, M. J., Falagán, N., Bohmer, B., Terry, L. A., Alamar, M. C. (2019). The role of ethylene and 1-MCP in early-season sweet cherry ‘Burlat’ storage life. Scientia Hortic. 258, 108787. doi: 10.1016/j.scienta.2019.108787
Silva, R. S., Silva, S. M., Rocha, A., Dantas, R. L., Schunemann, A. P. P., Pereira, W. E. (2013). Influence of 1-MCP on berry drop and quality of “Isabel” grape. Acta Hortic. 1012, 509–514. doi: 10.17660/actahortic.2013.1012.67
Sun, L., Zhang, M., Ren, J., Qi, J., Zhang, G., Leng, P. (2010). Reciprocity between abscisic acid and ethylene at the onset of berry ripening and after harvest. BMC Plant Biol. 10, 1–11. doi: 10.1186/1471-2229-10-257
Terry, L. A., Chope, G. A., Giné Bordonaba, J. (2007). Effect of water deficit irrigation and inoculation with Botrytis cinerea on strawberry (Fragaria x ananassa) fruit quality. J. Agric. Food Chem. 55 (26), 10812–10819. doi: 10.1021/jf072101n
Tosetti, R., Elmi, F., Pradas, I., Cools, K., Terry, L. A. (2020). Continuous exposure to ethylene differentially affects senescence in receptacle and achene tissues in strawberry fruit. Front. Plant Sci. 11. doi: 10.3389/fpls.2020.00174
Wang, L., Luo, Z., Li, J., Yang, M., Yan, J., Lu, H., et al. (2019). Morphological and quality characterization of grape berry and rachis in response to postharvest 1-methylcyclopropene and elevated oxygen and carbon dioxide atmospheres. Postharvest Biol. Technol. 153, 110–117. doi: 10.1016/j.postharvbio.2019.04.001
Watkins, C. B. (2006). The use of 1-methylcyclopropene (1-MCP) on fruits and vegetables. Biotechnol. Adv. 24, 389–409. doi: 10.1016/j.bioteChadv.2006.01.005
Wolf, M. M. (2002). An analysis of the impact of price on consumer purchase interest in organic grapes and a profile of organic purchasers. Am. Agric. Economics Assoc. Annu. Meeting (February 2002), 1–24. doi: 10.22004/ag.econ.19663
Xu, Z., Yoo, Y., Hwang, I. (2014). “ABA conjugates and their physiological roles in plant cells,” in Abscisic acid: metabolism, transport and signaling. Ed. Zhang, D. P. (Dordrecht: Springer), 77–87. doi: 10.1007/978-94-017-9424-4_5
Yamamoto, L. Y., Koyama, R., De Assis, A. M., Borges, W. F. S., De Oliveira, I. R., Roberto, S. R. (2015). Color of berry and juice of “Isabel” grape treated with abscisic acid in different ripening stages. Pesquisa Agropecuaria Bras. 50 (12), 1160–1167. doi: 10.1590/S0100-204X2015001200005
Yan, H., Wang, R., Ji, N., Li, J., Ma, C., Lei, J., et al. (2023). Regulation of cell wall degradation and energy metabolism for maintaining shelf quality of blueberry by short-term 1-methylcyclopropene treatment. Agronomy 13 (46). doi: 10.3390/agronomy13010046
Ying, Q., Chunli, L., Hao, L., Huaming, Y., Junfeng, G. (2021). Elimination or removal of ethylene for fruit and vegetable storage via low-temperature catalytic oxidation. J. Agric. Food Chem. 69 (36), 10419–10439. doi: 10.1021/acs.jafc.1c02868
Youssef, K., de Oliveira, A. G., Tischer, C. A., Hussain, I., Roberto, S. R. (2019). Synergistic effect of a novel chitosan/silica nanocomposites-based formulation against gray mold of table grapes and its possible mode of action. Int. J. Biol. Macromolecules 141, 247–258. doi: 10.1016/j.ijbiomac.2019.08.249
Zarrouk, O., Brunetti, C., Egipto, R., Pinheiro, C., Genebra, T., Gori, A., et al. (2016). Grape ripening is regulated by deficit irrigation/elevated temperatures according to cluster position in the canopy. Front. Plant Sci. 7. doi: 10.3389/fpls.2016.01640
Zenoni, S., Savoi, S., Busatto, N., Tornielli, G. B., Costa, F. (2023). Molecular regulation of apple and grape ripening: exploring common and distinct transcriptional aspects of representative climacteric and nonclimacteric fruits. J. Exp. Bot., erad324, 1–17. doi: 10.1093/jxb/erad324
Keywords: senescence, ABA, ethylene, 1-methylcyclopropane, table grapes, postharvest
Citation: Navarro-Calderón Á, Falagán N, Terry LA and Alamar MC (2023) Biomarkers of postharvest resilience: unveiling the role of abscisic acid in table grapes during cold storage. Front. Plant Sci. 14:1266807. doi: 10.3389/fpls.2023.1266807
Received: 25 July 2023; Accepted: 12 September 2023;
Published: 29 September 2023.
Edited by:
Sergio Ruffo Roberto, State University of Londrina, BrazilReviewed by:
Khamis Youssef, Agricultural Research Center, EgyptHong Ru Liu, Shanghai Academy of Agricultural Sciences, China
Aline Priscilla Gomes Da Silva, Michigan State University, United States
Copyright © 2023 Navarro-Calderón, Falagán, Terry and Alamar. This is an open-access article distributed under the terms of the Creative Commons Attribution License (CC BY). The use, distribution or reproduction in other forums is permitted, provided the original author(s) and the copyright owner(s) are credited and that the original publication in this journal is cited, in accordance with accepted academic practice. No use, distribution or reproduction is permitted which does not comply with these terms.
*Correspondence: M. Carmen Alamar, m.d.alamargavidia@cranfield.ac.uk