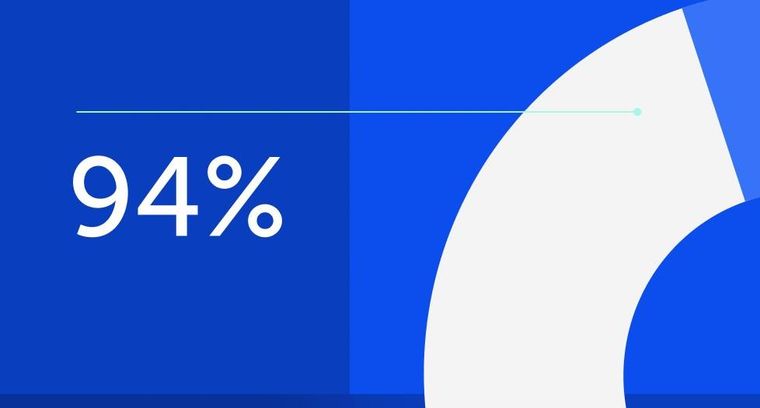
94% of researchers rate our articles as excellent or good
Learn more about the work of our research integrity team to safeguard the quality of each article we publish.
Find out more
ORIGINAL RESEARCH article
Front. Plant Sci., 22 September 2023
Sec. Functional Plant Ecology
Volume 14 - 2023 | https://doi.org/10.3389/fpls.2023.1251441
This article is part of the Research TopicEcological Management of Invasive Alien PlantsView all 9 articles
Ambrosia artemisiifolia is a highly invasive weed. Identifying the characteristics and the factors influencing its establishment and population growth may help to identify high invasion risk areas and facilitate monitoring and prevention efforts. Six typical habitats: river banks, forests, road margins, farmlands, grasslands, and wastelands, were selected from the main distribution areas of A. artemisiifolia in the Yili Valley, China. Six propagule quantities of A. artemisiifolia at 1, 5, 10, 20, 50, and 100 seeds m-2 were seeded by aggregation, and dispersion in an area without A. artemisiifolia. Using establishment probability models and Allee effect models, we determined the minimum number of seeds and plants required for the establishment and population growth of A. artemisiifolia, respectively. We also assessed the moisture threshold requirements for establishment and survival, and the influence of native species. The influence of propagule pressure on the establishment of A. artemisiifolia was significant. The minimum number of seeds required varied across habitats, with the lowest being 60 seeds m-2 for road margins and the highest being 398 seeds for forests. The minimum number of plants required for population growth in each habitat was 5 and the largest number was 43 in pasture. The aggregation distribution of A. artemisiifolia resulted in a higher establishment and survival rate. The minimum soil volumetric water content required for establishment was significantly higher than that required for survival. The presence of native dominant species significantly reduced the establishment and survival rate of A. artemisiifolia. A. artemisiifolia has significant habitat selectivity and is more likely to establish successfully in a habitat with aggregated seeding with sufficient water and few native species. Establishment requires many seeds but is less affected by the Allee effect after successful establishment, and only a few plants are needed to ensure reproductive success and population growth in the following year. Monitoring should be increased in high invasion risk habitats.
The successful establishment and reproduction of invasive alien plants after entering new habitats are essential for the continuation and rapid growth of the population (Warren and Bradford, 2011). Clarifying the regularity, characteristics, and influencing factors of the establishment and population growth of invasive plants and estimating the invasive risk in various habitats can facilitate the identification of high invasion risk areas, leading to timely monitoring, early warning, prevention, and control treatment (Gertzen et al., 2011; Zhao et al., 2020).
Invasive alien plants that spread to new habitats require sufficient seed numbers to establish and grow and survive (Colautti et al., 2006). Sexually reproducing species also need to overcome the lack of mates and effective pollinators in small populations in order to reproduce successfully (Courchamp et al., 2008). At the same time, native species and abiotic environmental factors (moisture, temperature, etc.) have a continuous impact on establishment, reproduction, and population growth (Visscher et al., 2023).
The propagule pressure hypothesis suggests that the number of propagules introduced into a new habitat and the frequency of introduction events are among the most important factors influencing the success of plant invasion (Simberloff, 2009). The number of propagators entering a new habitat directly affects the probability of survival and determines the success of establishment of invasive alien plants (Lockwood et al., 2009; Williams et al., 2021). Meanwhile, since resources are fully available during the very early phases of colonization, most propagule numbers can rapidly preempt or modify the available niche (You et al., 2016). Therefore, for successful establishment, the number of arriving individuals must be above an ‘invasion threshold’ (Lewis and Kareiva, 1993) defined as the minimum number required for establishment to occur.
After establishment, as the population is currently small, the influence of the Allee effect is more prominent (Allee, 1931). In sexually reproducing invasive alien plants, the Allee effect is primarily driven by the fewer potential mates and effective pollinators (Courchamp et al., 2008). Determining whether the Allee effect exists in an established population and the minimum number of plants that can achieve successful reproduction and facilitate population growth can provide a theoretical basis for the scientific development of efficient and low-cost prevention and control strategies (Berec et al., 2007; Tobin et al., 2011).
Propagules that spread to new habitats must also overcome environmental constraints, such as water availability and temperature. Additionally, competition from a resident community may reduce invasion capacity (Levine et al., 2004). Although there is little evidence suggesting that species interactions can completely resist invaders, some resident communities can reduce the establishment, abundance, and fitness of invaders to a certain extent (Liu et al., 2014). Therefore, identifying the effects of environmental factors on the establishment and population growth of invasive alien plants can provide an important basis for determining the invasiveness of habitats.
Predicting the establishment of exotic species is a central aim of invasion biology and depends on propagule pressure and population processes (Rojas-Botero et al., 2022). Although the relationship between the number of invasive plant propagules and invasion success has been well investigated (Warren et al., 2012; Vedder et al., 2021), data on the minimum number of propagules required for the successful establishment of invasive alien plant populations are limited. Therefore, it is very important to determine the minimum number of seeds required for successful establishment and the minimum number of plants for population growth of invasive alien plants under the influence of different environmental factors to judge their invasion risk.
It is useful to summarize the rules of invasive plant establishment and population growth to accurately predict the risk of habitat invasion by determining the minimum number of propagules required for successful establishment and the minimum number of plants required for population growth (Catford et al., 2019). In areas of invaded natural habitats where the invader is not present, the simulated invasion of artificially sown propagules could more comprehensively identify the combined influence of multiple biological and abiotic factors on establishment success (Warren et al., 2012).
The invasive annual herbaceous species, Ambrosia artemisiifolia, is a troublesome plant in many regions, including Central and Eastern Europe and China (Essl et al., 2015; Montagnani et al., 2017). A. artemisiifolia produces a large amount of pollen, costing European healthcare systems approximately 7.4 billion euros in the treatment of associated allergies every year (Schafner et al., 2020). A. artemisiifolia can forms a persistent soil seed bank as a result of complex germination strategies (Essl et al., 2015). The influence of propagule pressure and disturbances on the distribution of A. artemisiifolia is greater than that of environmental factors (Dullinger et al., 2009; Urbanowicz et al., 2018). Kröel-Dulay et al. (2019) found that the invasion probability of A. artemisiifolia increases with increasing propagule pressure in continuously disturbed habitats. However, the minimum number of seeds required for successful establishment (MNS) and the minimum number of plants required for the population growth (MNP) of A. artemisiifolia have not been studied.
A. artemisiifolia relies on seeds to propagate, most of which spread around the plant at a close distance due to the action of both wind and gravity, while a small number of seeds spread over long distances through animal feeding or attachment to the surface of animal fur, along with rivers, or mixed in cargo (Essl et al., 2015). Seed dispersal models indicate that seed aggregation or dispersal may occur when reaching new habitats via long-distance dispersal (Cousens et al., 2008).
Therefore, we asked if there are differences in the establishment of the seeds of A. artemisiifolia when they are aggregated or dispersed after reaching new habitats and if and how established plants successfully reproduce for population continuity and growth. What factors influence the establishment and growth of A. artemisiifolia? We sowed A. artemisiifolia in six typical invasived habitats, namely, river banks, forests, road margins, farmland, grassland, and wasteland, invaded by A. artemisiifolia were selected in the Yili Valley, China. Areas without A. artemisiifolia distribution were artificially seeded to simulate long-distance dispersal of A. artemisiifolia.
In this study, we aimed to (1) determine the characteristics of propagule pressure and the MNS of A. artemisiifolia in each habitat; (2) Allee effect and fruiting characteristics of A. artemisiifolia to determine the MNP needed to achieve population growth; and (3) the effects of environmental factors (native species, temperature, and moisture) on establishment and population growth of A. artemisiifolia. This study determined the degree of difficulty in the establishment and population growth of A. artemisiifolia in the main invasive habitats. Our results provide an important theoretical basis for early monitoring and effective prevention and control measures to mitigate the damage associated with the invasion of A. artemisiifolia.
The Yili Valley (42°14′–44°53′N, 80°09′–84°56′E) lies in the westernmost part of the Tianshan Mountain Range of Xinjiang. The Valley comprises 56,400 km2, and has a continental temperate arid climate. The region has an average annual temperature of 10.4°C and precipitation of 417.6 mm. In grassland habitats, which represent the wettest area in the Yili River, precipitation can reach 500 mm annually. The Yili Valley, with its rich plant diversity and extensive seed dispersal via canals, cattle, sheep, and tourists, provides favorable conditions for the invasion and rapid spread of invasive alien species (Jia et al., 2011).
The seeds used in this experiment were collected from complete plants in 8 A. artemisiifolia populations in different habitats in the invaded area of Yili Valley in September 2020 and the first batch of mature seeds were collected. Before the experiment, all immature, shriveled, worm-fed, and moldy seeds were removed; the remaining healthy mature seeds were thoroughly mixed, and several groups of 1, 5, 10, 20, 50, and 100 seeds were randomly selected for packaging. Therefore, the seeds used in the experiment were not subject to variations in germination rate and seed quality which could influence the results.
According to our previous investigation (Dong et al., 2020), there are six typical habitat types invaded by A. artemisiifolia in the Yili Valley: river banks, forests, road margins, farmland, grassland, and wasteland. There is virtually no A. artemisiifolia observed in other habitats. For this study the following habitats were used: the forest consisting of a shelterbelt on both sides of an agricultural road, the grassland on a hilly pasture, the wasteland was an abandoned farmland area (abandoned for five years), the farmland was artificial irrigated land, and the road margins were on both sides of the national road with heavy truck traffic.
In November 2020, an area with flat terrain, similar soil conditions and no intrusion and distribution of A. artemisiifolia, was selected as the experimental plot for each habitat. A 2 m high gauze was used to surround the experimental plot 2 m away from the experimental plot to avoid animal and human interference, and at the same time, the diffusion and invasion of A. artemisiifolia would not occur in the surrounding area of the experimental plot.
The entire plot was divided into 10 cells sufficiently far apart as replicates for each treatment (Figure 1A). In each cell, 10 quadrats (1 m × 1 m) were set up as pressure treatments for the propagules (Figure 1B). To ensure that the same treatments were not adjacent, quadrats were randomly selected. The space between the quadrats in each treatment was sufficiently large to ensure no interaction between quadrats.
Figure 1 Schematic diagram of the experimental setting of propagule pressure with different seeding number and methods. (A) Ten replicates in every plot. (B) Seeding number and method of Ambrosia artemisiifolia in one repeated treatment. A: Aggregated seeding, D: Dispersed seeding.
In the central area of each plot, a soil temperature and humidity recorder (WatchDog 1400 Data Logger) was installed at a position with stable terrain and no fluctuation. Temperature and humidity changes were recorded continuously at hourly intervals every day as habitat temperature and humidity data, respectively. After the experiments, the data were returned to the laboratory for analysis.
The introduction of invasive plant propagules is a random event; therefore, the number of propagules introduced into new habitats is usually not large. By observing the newly diffused ragweed population in the Yili Valley and referring to previous studies (Deng et al., 2016; Kröel-Dulay et al., 2019), we identified six reproductive number treatments: 1, 5, 10, 20, 50, and 100 seeds m-2.
Considering the aggregation and dispersal of A. artemisiifolia seeds that have spread into new habitats (Houseman, 2014), two sowing methods were adopted: (1) Aggregation: All seeds were concentrated in the central area of the quadrat to simulate a relatively concentrated distribution of seeds post mixing in goods or being swallowed by animals, collected in feces, discharged from the body, and dispersed into new habitats. (2) Dispersion: All seeds were evenly spread throughout the quadrat to simulate the relatively dispersed distribution of seeds after they were attached to animal fur or dispersed into new habitats by vehicles, rivers, or other media. Since the numbers of seeds treated with one and five were too small, a dispersed seeding treatment was not set up.
A total of 600 quadrats were used in the experiment: 6 habitats× [(6 × 10 propagule treatments) + (4 × 10 seed treatments)].
Due to continuous germination, for the maximum number of established plants in each treatment, a large number of seedling stage plants were concentrated near the end (SS; on April 25 ± 3 days, 2021). The number of A. artemisiifolia plants in the quadrat was investigated, and the establishment rate under each treatment was calculated (the number of established plants/the number of seeds sown in the treatment).During the mature stage of A. artemisiifolia (MS; September 15 ± 3 days, 2021), the number of surviving plants in the quadrat was counted and the survival rate under each treatment was calculated (the number of surviving plants in the setting stage/the number of established plants in the SS). Furthermore, the number of seeds per plant of all surviving A. artemisiifolia in each treatment was counted, and the total number of seeds in the population was calculated (sum of seeds per plant of all A. artemisiifolia in the quadrat). If some plants lost seeds, the number of pistil clusters and the location of the seeds were estimated.
To clarify the effects of native species on the establishment and survival of A. artemisiifolia, the number (total number of individuals in the quadrat for general plants and tillering number for gramineous plants), frequency, coverage, and plant height of A. artemisiifolia and the native species in each quadrat were counted during these two periods. The relative abundance (RA), relative coverage (RC), relative frequency (RF), relative height (RH) of A. artemisiifolia and native species were calculated for each habitat during SS and MS (Supplementary Table A.1).
To determine the growth capacity of the A. artemisiifolia population, the newly established A. artemisiifolia in each treatment quadrat was investigated at the SS of the following year (April 25 ± 3 days, 2022).
At the end of all experiments, all A. artemisiifolia in the plots were subjected to continuous chemical treatment (Wang et al., 2022), to ensure their removal. The surrounding areas were monitored and maintained for two consecutive years to ensure that no A. artemisiifolia had spread into the surrounding habitat and caused new invasions.
The density-dependent invasion pressure model constructed by Barney et al. (2016) is as follows:
where Y is defined as the invasion pressure or the probability that at least one propagule is established in a given area, P is the establishment probability of a single propagule in a given area, N is the number of propagules arriving in a given area in an introduction event, and D is a density dependence parameter that is expected to fall between 0 and -1.
In the present study, under different seeding treatments, the seeds of A. artemisiifolia were affected by the number and seeding method (aggregation/dispersion), and the density-dependent effect was different, which further affected the final establishment probability. Therefore, we used Equation (1) to consider the effect of density dependence on the establishment probability of A. artemisiifolia with different propagule numbers.
Through experimental observation, the establishmet rate P of A. artemisiifolia in different habitats under different treatments was obtained, and the slope D of the survival curve of A. artemisiifolia population in the SS under different N treatments (1, 5, 10, 20, 50, and 100 seeds m-2) was calculated. Then, 100 groups of data observed in each habitat in the experiment (sowing number 6× repeat 10+ sowing method 4× repeat 10) were substituted in Equation (1) to calculate the Y values under different treatments and solve each parameter to determine the relationship between the invasion and establishment probability of A. artemisiifolia and the number of propagators. The N value at Y = 1 was determined by fitting; therefore, the MNS of A. artemisiifolia invading and established in each habitat of Yili Valley was obtained.
We referred to Tobin et al. (2011) to consider the effects of different treatments (removal and application of pesticides, among others) on the number of invasive populations and constructed the following model:
where the population density Nt+1 at time t+1 equals the per-capita fecundity λ multiplied by the population density Nt at time t times the fraction Nt/(Nt + θ) of individuals that succeed. In identifying mates and reproduce; θ is the Allee effect strength. It is assumed that a fraction P (0< P< 1) of individuals dies.
In this study, due to various biological and abiotic factors, the P ratio of the individual deaths of A. artemisiifolia surviving after successful establishment under different propagation pressures was also observed; this effect was continuous with the growth of A. artemisiifolia. Here, we redefined P as the proportion of A. artemisiifolia dying from establishment to fruiting but retained its expression.
Therefore, for A. artemisiifolia which survives and bears fruit after establishment, population density in the second year can be expressed using Equation (2). The Allee effect threshold for A. artemisiifolia population was
In other words, after successful establishment, the population density of A. artemisiifolia could not achieve effective population growth when it was lower than Ap, and the population growth rate Nt+1/Nt was less than 1–0.
In this study, the number of surviving A. artemisiifolia plants (1-P)Nt, the number of seed λ, and the number of newly settled A. artemisiifolia plants Nt+1 at the second SS of invasion under different treatments in each habitat were experimentally observed. A total of 100 groups of data (sowing number 6× 10 repetitions + sowing method 4× 10 repetitions) observed in each habitat in the experiment were substituted into Equations (2) and (3) to calculate the θ value and threshold Ap of the Allee effect under different treatments and solve for the parameters to determine the relationship between population growth and plant number after the establishment of A. artemisiifolia. Then, the Allee effect threshold Ap is calculated. By fitting the Nt value at an Nt+1/Nt value of 1, the MNP required for the successful reproduction and population growth of A. artemisiifolia in each habitat in the Yili Valley was obtained.
For the soil volume, water content, and temperature data obtained from each habitat, the temperature and humidity data for the initial 30 days of observation were selected for the growth period. Furthermore, the average value of the temperature and humidity values recorded every day (once every hour, 12 groups in total) were considered as the temperature and humidity of the day, for a total of 30 treatments, with an average value of the temperature and humidity of the growth period.
The coefficient of variation (CV) was calculated to reflect the degree of fluctuation in soil temperature and humidity during different growth periods (Supplementary Table A.2).
To clarify the effects of soil volumetric water content and temperature on the settlement and survival of A. artemisiifolia and determine the threshold range and optimal value of soil volumetric water content and temperature required for the settlement and survival of A. artemisiifolia, A Gaussian regression model was used to fit the soil volumetric water content and temperature with the establishment and survival rates of A. artemisiifolia at SS and MS, respectively.
The establishment and survival rates were fitted to soil volumetric water content or temperature, respectively, using a unitary quadratic function. If the unitary quadratic function is in line with these two relationships, a Gaussian relationship can be determined (Zhang, 2004). The quadratic equation of a variable with a Gaussian relation is converted into a Gaussian regression equation as follows:
where Y is the establishment or survival rate of all the seeding treatments in the plot, A is the maximum establishment or survival rate of A. artemisiifolia, μ is the soil volume water content or temperature value when the establishment rate or survival rate of A. artemisiifolia peaks, that is, the optimal value of environmental factors, and t is the tolerance of A. artemisiifolia, the higher the t value, the wider the range of A. artemisiifolia adaptation to this environmental factor. The ecological threshold interval of soil volume water content and temperature of A. artemisiifolia was[μ−2t, μ+2t], and the optimal ecological threshold interval was [μ−t, μ+t]. Therefore, the key to determining the threshold of soil volume water content and temperature for the A. artemisiifolia establishment and survival is to determine the values of μ and t.
The species distribution data of the Global Biodiversity Information Facility (GBIF) were searched using the R software to identify the water and temperature characteristics and variation in A. artemisiifolia in North America, Europe, and the Yili Valley, in native and invaded ranges.
Erroneous or duplicate records and filter data were removed, and the latitude and longitude information of the species distribution points were used to extract climate variables from the WorldClim database for analysis and mapping (Supplementary Figure A.1).
We used the SPSS software, data were tested for normality using the “QQ plot” in the “descriptive statistics”, and then distributed in a straight line for each treatment. All data followed a normal distribution. Further, data were tested for homogeneity. If the variance difference of each group was not significant (p > 0.05), the data conformed to the homogeneity of variance.
We used generalized linear mixed models (GLMM) and selected normal distribution model constant function to evaluate the fixed effects (seeding number, seeding method, and seeding number × seeding method) on establishment rate, survival rate and total seed number. We accounted for the influence of native species, by evaluating sum of the RA, RC and RH of the native species and the interaction between them (RA × RC, RA × RH, RC × RH, RA × RC × RH) as random effects in the GLMMs. The mixed models were fit using the Laplace approximation in the ‘lme4’ package (Bates and Maechler, 2009) of the R statistical programming environment (R.V4.1.1). The inclusion or exclusion of the random effects was based on Akaike’s information criterion (AIC) values (Akaike, 1973). AIC measures the distance of models to truth; that is, the information loss in the data given that the model is a simplification of reality and cannot explain full variance in the data (Burnham and Anderson, 2002). In effect, AIC is a measure of goodness-of-fit, as the more familiar R2 measure, with an added penalty for model complexity as measured by the number of fit parameters in the model.
To examine the impact of native species traits on the establishment rate and survival rate of A. artemisiifolia, Pearson correlation analyses of “sum of the RA of the native species”, “sum of the RC of the native species” and “sum of the RH of the native species” and “establishment/survival rate” data were conducted for each habitat. Therefore, some factors showed a significant correlation, while others showed no significant correlation.
ANOVA with Duncan’s method was used to compare the differences in establishment and survival rate of A. artemisiifolia under different seeding number and seeding methods, as well as the differences in the total number of seeds and the number of new plants in the next year.
The GLMM test results (Table 1) showed that the seeding number, seeding method, and their interaction had significant effects on the establishment and survival rates of A. artemisiifolia in each habitat (Figure 2). The total number of seeds in the population was mainly affected by the seeding number and seeding method (Tables 2, 3).
Table 1 Test results of GLMM on the effects of various factors on establishment rate, survival rate and total seed number of population of Ambrosia artemisiifolia.
Figure 2 Effects of sowing number and method on establishment rate (A–E) and survival rate (F–J) of Ambrosia artemisiifolia in each habitat (Mean ± SE). Different letters indicate significant difference in seeding number under the same seeding method (P< 0.05), * indicated that there were significant differences among different seeding methods under the same seeding number (P< 0.05). Since there was no A. artemisiifolia germination in the wasteland, no data was obtained, so it was not shown in the figure.
Table 2 Total seed number of Ambrosia artemisiifolia population in different habitats under different treatments (seeds m-2, Mean ± SE).
Table 3 Number of newly established Ambrosia artemisiifolia in different habitats in the second year (plants m-2, Mean ± SE).
In random effects, relative abundance (RA) in each habitat, relative coverage (RC) and its interaction with RA in forests, and relative height (RH) and its interaction and RA in grassland had significant negative effects on establishment and survival rates (Figures 3, 4). None of the random effects significantly affected the total number of seeds in a population.
Figure 3 Effects of native species in each habitat on establishment rate of Ambrosia artemisiifolia. Trendline fitting indicates a significant effect, while trendline not fitting indicates no significant effect. RA: Total relative abundance of native species. RC: Total relative coverage of native species. RH: Total relative plant height of native species. (A, D, G, J, M) Effects of RA in each habitat on the establishment rate of Ambrosia artemisiifolia. (B, E, H, K, N) Effects of RC in each habitat on the establishment rate of Ambrosia artemisiifolia. (C, F, I, L, O) Effects of RH in each habitat on the establishment rate of Ambrosia artemisiifolia. misiifolia.
Figure 4 Effects of native species in each habitat on survival rate of Ambrosia artemisiifolia. Trendline fitting indicates a significant effect, while trendline not fitting indicates no significant effect. RA: Total relative abundance of native species. RC: Total relative coverage of native species. RH: Total relative plant height of native species. (A, D, G, J, M) Effects of RA in each habitat on the survival rate of Ambrosia artemisiifolia. (B, E, H, K, N) Effects of RC in each habitat on the survival rate of Ambrosia artemisiifolia. (C, F, I, L, O) Effects of RH in each habitat on the survival rate of Ambrosia artemisiifolia.
At the seedling stage (SS), there were significant differences in the establishment rate of A. artemisiifolia among the treatments with different seeding numbers (Figures 2A-E). No plants germinated in the wasteland, established with 1 or 5 seed treatments in any habitat, or were successfully established in 10 seed treatment in forest. With an increase in the number of propagules, the establishment rate significantly increased, and the establishment rate of farmland and road margins was very high, up to 44%. Among the different sowing methods, the establishment rate of aggregated sowing was higher than that of dispersed sowing, and the difference was significantfor the 50 and 100 seed treatments.
At the mature stage (MS), there were significant differences in plant survival rates among treatments with different sowing amounts in different habitats (Figures 2F-J). The survival rate of plants in the road margins was the highest (27.5%) while the survival rate of plants in the grassland was the lowest. No plants survived in 10 seed treatment. Among the different seeding methods, only the 50 and 100 seed treatments on river banks and road margins, respectively, were significantly different.
At the MS, all the surviving A. artemisiifolia were able to successfully produce seeds. By comparing the total number of seeds in each habitat, with an increase in the number of sows, the total number of seeds in the population significantly increased with increasing seeding. The number of seeds from the aggregated sowing method was significantly higher than from the dispersed sowing method (Table 2).
At the SS of the second year, the number of newly established A. artemisiifolia plants increased in all treatments with increasing seeding. On river banks and road margins, the number of newly established plants with aggregated sowing in the 50 and 100 seed treatments were significantly higher than that with dispersed sowing (Table 3).
At the SS, the effects of the native species in each habitat were significantly different. Except for the forest, RA in the other habitats had a significant negative effect on the establishment rate of A. artemisiifolia (Figures 3A, D, G, J, M). RC had a significant negative effect on the establishment rate of A. artemisiifolia in the forest and grassland habitats (Figures 3B, E, H, K, N). For example, in forest, the RC of Phlomis umbrosa and Arctium lappa reached 29.03 ± 2.35% and 25.81 ± 3.76%, respectively, 4–5 times that of A. artemisiifolia (Supplementary Table A.1). The RH had no significant effect on the establishment rate of A. artemisiifolia (Figures 3C, F, I, L, O).
At the MS, the effects of the native species in each habitat were significantly different. RA had a significant negative effect on the survival rate of A. artemisiifolia except for the dispersed sowing treatment in the forest and farmland (Figures 4A, D, G, J, M). RC had a significant negative effect on the establishment rate of A. artemisiifolia only in forest (Figures 4B, E, H, K, N), while the RC of Phlomis umbrosa and Arctium lappa reached 29.15 ± 2.3% and 23.35 ± 1.65%, respectively, 3–4 times that of A. artemisiifolia. RH had a significant negative effect on the survival rate of A. artemisiifolia only in the grasslands (Figures 4C, F, I, L, O). For example, the RH of Chenopodium album, Artemisia annua, and Cannabis sativa was 11.79 ± 2.7%, 10.8 ± 1.45%, and 10.09 ± 2.73%, respectively, 1.5 times that of A. artemisiifolia (Supplementary Table A.1).
The MNS of A. artemisiifolia varied significantly among habitats (Figures 5A-E). The MNS required by river banks were 81 (aggregation) and 163 (dispersion), forest were 398 (aggregation) and 238 (dispersion), road margins were 60 (aggregation) and 74 (dispersion), farmland were 113 (aggregation) and 120 (dispersion), and grassland were 126 (aggregation) and 166 (dispersion).
Figure 5 Prediction of MNS (A-E) and MNP (F-J) in different habitats under different treatments. The solid blue line represents aggregated sowing, and the dashed blue line corresponds to the N value when the establishment rate (Y) is 1. The solid red line represents the dispersed sowing, and the dotted red line corresponds to the N value when the establishment success rate (Y) is 1.
The MNP of A. artemisiifolia varied significantly among the habitats (Figures 5F-J). The MNP required by river banks were 5 (aggregation) and 16 (dispersion), forest were 14 (aggregation) and 11 (dispersion), road margins were 11 (aggregation) and 15 (dispersion), farmland were 10 (aggregation) and 12 (dispersion), and grassland were 32 (aggregation) and 43 (dispersion).
Regression analysis of the measured data of soil temperature and volumetric water content showed that soil volumetric water content had a significant positive effect on the establishment rate and survival rate of A. artemisiifolia (Figures 6A, C), whereas soil temperature had no significant effect. According to the conversion relationship between the quadratic equation and the Gaussian model, the Gaussian regression equation (Equation 5) of soil volumetric water content and establishment rate at the SS, and the Gaussian regression equation (Equation 6) of soil volumetric water content and survival rate at the MS were obtained.
Figure 6 Relationship between establishment rate and survival rate of Ambrosia artemisiifolia and soil volumetric water content and Gaussian fitting curve. (A) Regression of establishment rate and soil volume moisture content at seedling stage. (B) Gaussian curves of fitted establishment rate and soil volumetric water content. (C) Regression of survival rate and soil volume moisture content at seedling stage. (D) Gaussian curves of fitted survival rate and soil volumetric water content. The black dots are showing in A and C represents the actual value.
According to Equation 5, the optimal soil volumetric water content for the establishment of A. artemisiifolia is 28.11%, the tolerance value is 1.38%, the threshold range of soil volumetric water content required for establishment is 25.77–30.45%, and the optimal range is 26.94–29.28% (Figure 6B). Conversely, according to Equation 6, the optimal soil volumetric water content for A. artemisiifolia survival at MS is 22.88%, the tolerance value is 3.67%, the threshold range of soil volumetric water content required for survival is 15.54–30.22%, and the optimal range is 19.21–26.55% (Figure 6D).
Prediction, rapid and accurate monitoring, and effective prevention and control of the invasion of A. artemisiifolia remain challenging. A. artemisiifolia can achieve rapid adaptive evolution under future climate change scenarios (Sun and Roderick, 2019) and has spread to Europe, North America, and Asia (Skjøth et al., 2019; Xian et al., 2023). Although studies have found that roadside and river banks are the most vulnerable habitats to invasion by A. artemisiifolia (Lemke et al., 2019), the degree of probability of invasion, going from individual establishment to population outbreak after A. artemisiifolia spreads to new habitats, remains unclear.
When invasive alien plants spread to new habitats, their propagules either die or become dormant owing to the restrictions of environmental factors. Therefore, numerous single introductions of propagators will increase the survival probability of invasive species and contribute to their successful establishment (Wittmann et al., 2014). A. artemisiifolia seeds exhibit a high degree of dormancy; because of secondary dormancy, A. artemisiifolia seeds can remain alive in the soil for decades. Seed dormancy can be broken by wet, dark stratification at 4°C for 2 weeks to obtain about 75% of germination (Baskin and Baskin, 1987; Fumanal et al., 2006). Thus, dispersal by A. artemisiifolia into new habitats requires many seeds to ensure establishment.
In this study we found that for the same seed treatment, the risk level of successful establishment of A. artemisiifolia in each habitat was as follows: road margins> river banks> farmland> grassland> forest. The MNS was lowest at road margins at 60 seeds and highest in forests at 398 seeds. MNS in river banks, farmland, grassland, and other habitats was in the range of 80–166 seeds. The higher the MNS of the habitat, the lower the potential risk of an outbreak upon arrival. Therefore, the successful establishment of A. artemisiifolia is the result of the accumulation of more seeds, rather than immediate invasion once it reaches the new habitat.
The distribution of A. artemisiifolia in the Yili Valley has an obvious habitat bias. Except for the native areas in this study, the distribution of bias in other habitats was small or difficult to invade (Zhao et al., 2022). Although only six typical habitats of A. artemisiifolia distribution were selected as research sites in this study, they were scattered throughout the study area, and the habitats under different spatial distribution comprehensively represented the habitat types of A. artemisiifolia growth. Therefore, the monitoring of A. artemisiifolia should focus on the above habitats. A. artemisiifolia mainly relies on vehicle cargo transport and drifting with currents to achieve long-distance diffusion. Roadsides, river banks, and ditches are accessible habitats after long-distance diffusion (Joly et al., 2011; Pinke et al., 2011). MNS is relatively small (60 seeds for road margins and 81 for river banks), which should be prioritized for monitoring. In other habitats where the MNS is larger (forest, 238; farmland, 113; grassland, 126), the risk of successful outbreaks is lower. If only a small number of seeds are dispersed in such habitats over long distances through excretion by animals after feeding or via pedestrians, animals, and vehicles; monitoring is not vital in this case.
The successful reproduction of sexual plants after spreading to new habitats is an important manifestation of adaptation, in which the success of reproduction and mating is crucial. In low-density populations, the Allee effect is caused by inbreeding depression, an inability to attract or find a mate, or an inability to repel predators (Courchamp et al., 2008). There are four stages of the biological invasion process: (1) arrival, (2) establishment, (3) spread, and (4) impact on the environment and economy (Lockwood et al., 2007); Allee effects are important during establishment and spread (Taylor and Hastings, 2005). Especially for wind-pollinated invasive plants, smaller and more isolated populations limit growth and reduce invasive ability due to stronger Allee effects caused by pollen limitation (Liebhold and Tobin, 2008).
A. artemisiifolia relies mainly on wind pollination and is monoecious and cross-flowered (Essl et al., 2015). The Allee effect likely occurs in low-density A. artemisiifolia populations that spread to new habitats. In this study, at least five plants were required to reproduce successfully on river banks, and the MNP required to reproduce successfully in habitats outside grassland habitat was in the range of 10–16 plants. This suggests that A. artemisiifolia has a low demand for the number of plants needed to achieve population growth, and a high risk of outbreak hazards once successfully established.
Pollen production varies among A. artemisiifolia plants from 0.1 to 3.8 billion pollen grains per plant (Fumanal et al., 2007; Smith et al., 2013), according to plant size. The pollen viability and stigma receptive period of A. artemisiifolia are approximately 20 and 16 days, respectively, and maintain strong receptivity for approximately 7 consecutive days, with pollen viability of up to 92% (Hao et al., 2015). As the flowers of A. artemisiifolia are uniovulate, plants at lower densities capture sufficient pollen for a high seed set (Friedman and Barrett, 2008). However, there are records of A. artemisiifolia pollen in air samples hundreds of kilometers from the nearest population, indicating the long-distance travel of the A. artemisiifolia pollen (Marco et al., 2006; Stępalska et al., 2020). These characteristics give A. artemisiifolia a strong probability of successful pollination; therefore, only a small number of plants are required for successful reproduction.
It has been argued that natural selection may favor the evolution of selfing in non-native ranges, as invading species frequently have small initial population sizes (Razanajatovo et al., 2016). In North America, A. artemisiifolia is highly self-incompatible and has a high outcrossing rate (Friedman and Barrett, 2008). However, Li et al. (2012) demonstrated no shift from outcrossing to selfing during A. artemisiifolia invasion in China. The maintenance of high outcrossing rates in colonizing populations of A. artemisiifolia is likely facilitated by the production of wind-borne pollen (Friedman and Barrett, 2011). Many high-quality seeds can be obtained by a breeding mode dominated by outcrossing, which guarantees a high level of genetic diversity in A. artemisiifolia to limit genetic bottlenecks or other restrictions in the initial stage of invasion, which is conducive to stable population growth.
The aggregated distribution of plants is associated with alleviating biological and abiotic environmental stresses (such as drought, low temperatures, wind, and animal feeding) and improving their survival and reproductive ability. Examples include density-dependent hybridization (Schmitt et al., 1987), fairy circles (Getzin et al., 2016), intraspecific facilitation (Reijers et al., 2019), and responses to amplifying and alleviating the effects of environmental pressures (Hodgson et al., 2017; Tamura et al., 2020).
The success of Invasive alien plants depends on the degree of seed and plant aggregation (Moore et al., 2003). The aggregation effect of seed germination is the embodiment of the cluster advantage. When more than one species is involved, a lack of seed clustering allows a second species to invade and possibly outcompete the first species at a fine scale (Velázquez et al., 2014). Seeds of the same species sense each other through the release of allelopathic substances (Renne et al., 2014) and gain competitive advantages over the seeds of other species through strategies such as rapid germination (Byun, 2023).
The germination rate of plants with an aggregated distribution was higher (Ghazian et al., 2021). After establishment, the interspecific competitiveness of seedlings is enhanced based on the advantages of aggregation, which can alleviate competition among native species and significantly increase their survival rate (Čuda et al., 2015; El-Barougy et al., 2020). However, for sexual plants, aggregated plant distribution is more conducive to the successful reproduction of pollen adjacent to hetero-plants, thus compensating for the insufficient plant number in the population. Therefore, an increase in the degree of aggregation improves individual fitness and affects population growth (Kanarek et al., 2013).
In this study, under the same seed treatment, the establishment and survival rates of A. artemisiifolia plants with an aggregated distribution were higher than those with a dispersed distribution, especially for 50 and 100 seed treatments. A. artemisiifolia mainly relies on gravity to achieve close-range spread (Essl et al., 2015). Owing to the large number and high density of aggregated distributions of A. artemisiifolia plants, the final seed number per unit area was large, which ensured a stable seed supply and achieved stable population growth in the second year. Although the number of seeds per unit area of dispersed A. artemisiifolia was significantly lower than that of the aggregated sowing, most seeds scattered near the plant after maturity and had an aggregation effect, which resulted in higher germination and establishment rates in the second year, thus achieving rapid population growth. Therefore, aggregated seed distribution lays the foundation for the rapid formation of the cluster growth advantage of A. artemisiifolia.
Habitat conditions play a fundamental role by influencing the invasion process and the composition of native species. Water is an important factor affecting species distribution. Indeed, precipitation contributes more than 50% to the potential distribution of A. artemisiifolia (Liu et al., 2021). Simulations on the effect of different precipitation levels on the growth of A. artemisiifolia, found that A. artemisiifolia was highly adaptable to drought (Leiblein and Lösch, 2011; Leskovšek et al., 2012). However, temperature had no significant effect on the growth and distribution of A. artemisiifolia in the Yili Valley (Dong et al., 2020).
In the native range of A. artemisiifolia, precipitation in the distribution area is approximately 1,000 mm, and 800 mm in invaded ranges such as France and Austria, respectively (Essl et al., 2015). In Xinyuan County, the main distribution area of A. artemisiifolia in the Yili Valley, the average annual precipitation is 417.6 mm, with the maximum reaching 500 mm. A. artemisiifolia invaded the Yili Valley in 2010. Since 2014, the area occupied by these species has rapidly increased; by 2017, these two species occupied 1,322 km2 (Dong et al., 2020).
In this study, soil water volume had a significant positive effect on the establishment rate of A. artemisiifolia. Although the average annual precipitation in the study area was lower than that in the native and invasive areas in Europe, the water supply on river banks or ditches was sufficient and stable, and the water supply on roadsides could also meet the water demand for germination, establishment, and survival of A. artemisiifolia. The soil volumetric water content was the lowest in the wasteland, where A. artemisiifolia failed to germinate. This suggests that water availability is a prerequisite for the establishment of A. artemisiifolia seeds after dispersal into new habitats.
After the seeds of invasive plants reach a new habitat and successfully overcome the restriction of abiotic environmental factors, competition with native species has a gradually significant effect on establishment and population growth (Blackburn et al., 2011). Native community diversity is one of the main factors affecting invasiveness (Elton, 1958). Bioimpedance occurs when native species in a local community occupy the niche of an invasive species, thereby reducing its survival and fitness (Levine et al., 2004). Higher species diversity can significantly reduce the probability of the successful establishment and survival of invasive species (Vilà et al., 2011). A. artemisiifolia is gnot a strong competitor in less disturbed habitats, especially in the early invasion stage; its abundance and coverage are not dominant, and it cannot effectively compete with many of the native species it encounters (Bazzaz, 1968; Gentili et al., 2015).
In the present study, the influence of native plants on the establishment and survival of A. artemisiifolia was mainly reflected in the abundance of native species and the dominant native species with high plant heights and high biomass. In the forest, there were abundant native species, and the plant coverage of perennial plants such as Phlomis umbrosa and Arctium lappa was high, especially funder aggregated treatment of A. artemisiifolia, where seedlings provide shelter, affecting establishment. This also explains why the MNS of forests was the highest among all habitats, and the MNS and MNP of aggregated distribution was greater than those for dispersed distribution. Grassland was similar to forest in that native species were abundant and due to the height of native C. album, A. annua and C. sativa, although A. artemisiifolia could successfully establish, it could not form an effective clustering advantage over the growth period. Therefore, all A. artemisiifolia plants treated at a low density in the grassland (10) died at MS. The proportion of viable plants in the high-density treatment (50 and 100) was the lowest in all habitats.
In other habitats, although the abundance of native species had a significant negative effect on the establishment and survival of A. artemisiifolia, due to the small number and variety of native species, no species were significantly larger than A. artemisiifolia in coverage or height. Owing to the advantages of cluster growth and tall plants, interactions such as interspecies competition could not completely exclude A. artemisiifolia from achieving successful establishment and survival.
This study has shown that the successful invasion of A. artemisiifolia is not likely achieved following dispersal by isolated propagules to new habitats, but rather when the seeds are concentrated by aggregation in habitats with sufficient water supply and few native species, such as river banks, ditches, and roadsides. Therefore, monitoring of these high invasion risk areas should be strengthened to prevent invasion. Once established, A. artemisiifolia can successfully reproduce and achieve rapid population growth in the second year of invasion. When found, A. artemisiifolia plants should be completely removed to avoid the dispersal of seeds to surrounding areas and continued invasion.
The raw data supporting the conclusions of this article will be made available by the authors, without undue reservation.
WZ conceived this study, performed data analyses, and wrote the manuscript. HW and ZH collected data of this study. TL led and coordinated the project. All authors read and approved the final manuscript. All authors contributed to the article and approved the submitted version.
This study was supported by Key Science and Technology Project of Xinjiang Production and Construction Corps (No. 2022AB010) and National Natural Science Foundation of China (No. 31770461).
All authors were employed by Xinjiang Production and Construction Corps Key Laboratory of Oasis Town and Mountain-basin System Ecology.
All claims expressed in this article are solely those of the authors and do not necessarily represent those of their affiliated organizations, or those of the publisher, the editors and the reviewers. Any product that may be evaluated in this article, or claim that may be made by its manufacturer, is not guaranteed or endorsed by the publisher.
The Supplementary Material for this article can be found online at: https://www.frontiersin.org/articles/10.3389/fpls.2023.1251441/full#supplementary-material
Akaike, H. (1973). “Information theory as an extension of the maximum likelihood principle,” in 2nd Int. Symp. on Information Theory. Eds. Petrov, B. N., Csaki, F. (BNPBF Csaki Budapest: Academiai Kiado), 267–281.
Allee, W. C. (1931). Animal aggregations, a study in general sociology (Chicago: University of Chicago Press).
Barney, J. N., Ho, M. W., Atwater, D. Z. (2016). Propagule pressure cannot always overcome biotic resistance: the role of density-dependent establishment in four invasive species. Weed. Res. 56 (3), 208–218. doi: 10.1111/wre.12204
Baskin, J. M., Baskin, C. C. (1987). Temperature requirements for after- ripening in buried seeds of four summer annual weeds. Weed. Res. 27, 385–389. doi: 10.1111/j.1365-3180.1987.tb00776
Bates, D., Maechler, M. (2009). Linear mixed-effects models using S4 classes. – R package ver. 0.999375-31.
Bazzaz, F. A. (1968). Succession on abandoned fields in the Shawnee Hills, southern Illinois. Ecology 49 (5), 924–936. doi: 10.2307/1936544
Berec, L., Angulo, E., Courchamp, F. (2007). Multiple Allee effects and population management. Trends Ecol. Evol. 22, 185–191. doi: 10.1016/j.tree.2006.12.002
Blackburn, T. M., Pyšek, P., Bacher, S., Carlton, J. T., Duncan, R. P., Jarošík, V., et al. (2011). A proposed unified framework for biological invasions. Trends Ecol. Evol. 26 (7), 333–339. doi: 10.1016/j.tree.2011.03.023
Burnham, K. P., Anderson, D. R. (2002). Model selection and multimodel inference: a practical information-theoretic approach (Springer New York).
Byun, C. (2023). Role of priority effects in invasive plant species management: early arrival of native seeds guarantees the containment of invasion by Giant ragweed. Ecol. Evol. 13 (3), e9940. doi: 10.1002/ece3.9940
Catford, J. A., Smith, A. L., Wragg, P. D., Clark, A. T., Kosmala, M., Cavender-Bares, J., et al. (2019). Traits linked with species invasiveness and community invasibility vary with time, stage and indicator of invasion in a long-term grassland experiment. Ecol. Lett. 22 (4), 593–604. doi: 10.1111/ele.13220
Colautti, R. I., Grigorovich, I. A., MacIsaac, H. J. (2006). Propagule pressure: a null model for biological invasions. Biol. Invasions 8, 1023–1037. doi: 10.1007/s10530-006-9007-7
Courchamp, F., Berec, L., Gascoigne, J. (2008). Allee Effects in Ecology and Conservation (Oxford: Oxford University Press).
Cousens, R., Dytham, C., Law, R. (2008). Dispersal in Plants: A Population Perspective (Oxford: Oxford University Press).
Čuda, J., Skálová, H., Janovský, Z., Pyšek, P. (2015). Competition among native and invasive impatiens species: the roles of environmental factors, population density and life stage. Aob Plants 7, plv033. doi: 10.1093/aobpla/plv033
Deng, Z. Z., Zhao, X. J., Zhao, C. Y., Li, J. S. (2016). The impact of propagule pressure on the successful colonization and population persistence of invasive weed Ambrosia artemisiifolia. Chin. J. Ecol. 35 (6), 1511–1515. doi: 10.13292/j.1000-4890.201606.034
Dong, H., Song, Z., Liu, T., Liu, Z., Liu, Y., Chen, B. (2020). Causes of differences in the distribution of the invasive plants Ambrosia artemisiifolia and Ambrosia trifida in the Yili Valley, China. Ecol. Evol. 10, 13122–13133. doi: 10.1002/ece3.6902
Dullinger, S., Kleinbauer, I., Peterseil, J., Smolik, M., Essl, F. (2009). Niche based distribution modelling of an invasive alien plant: effects of population status, propagule pressure and invasion history. Biol. Invasions 11, 2401–2414. doi: 10.1007/s10530-009-9424-5
El-Barougy, R., MacIvor, J. S., Arnillas, C. A., Nada, R. M., Khedr, A. H. A., Cadotte, M. W. (2020). Richness, phylogenetic diversity, and abundance all have positive effects on invader performance in an arid ecosystem. Ecosphere 11, e03045. doi: 10.1002/ecs2.3045
Essl, F., Biró, K., Brandes, D., Broennimann, O., Bullock, J. M., Chapman, D. S., et al. (2015). Biological flora of the British Isles: Ambrosia artemisiifolia. J. Ecol. 103 (4), 1069–1098. doi: 10.1111/1365-2745.12424
Friedman, J., Barrett, S. C. (2008). High outcrossing in the annual colonizing species Ambrosia artemisiifolia (Asteraceae). Ann. Bot. 101 (9), 1303–1309. doi: 10.1093/aob/mcn039
Friedman, J., Barrett, S. (2011). Genetic and environmental control of temporal and size-dependent sex allocation in a wind-pollinated plant. Evolution 65, 2061–2074. doi: 10.1111/j.1558-5646.2011.01284.x
Fumanal, B., Chauvel, B., Bretagnolle, F. (2007). Estimation of pollen and seed production of common ragweed in France. Ann. Agr. Env. Med. 14 (2), 233–236.
Fumanal, B., Plenchette, C., Chauvel, B., Bretagnolle, F. (2006). Which role can arbuscular mycorrhizal fungi play in the facilitation of Ambrosia artemisiifolia L. invasion in France? Mycorrhiza 17, 25–35. doi: 10.1007/s00572-006-0078-1
Gentili, R., Gilardelli, F., Ciappetta, S., Ghiani, A., Citterio, S. (2015). Inducing competition: intensive grassland seeding to control Ambrosia artemisiifolia. Weed. Res. 55 (3), 278–288. doi: 10.1111/wre.12143
Gertzen, E. L., Leung, B., Yan, N. D. (2011). Propagule pressure, Allee effects and the probability of establishment of an invasive species (Bythotrephes longimanus). Ecosphere 2 (3), 1–17. doi: 10.1890/ES10-00170.1
Getzin, S., Yizhaq, H., Bell, B., Erickson, T. E., Postle, A. C., Katra, I., et al. (2016). Discovery of fairy circles in Australia supports self-organization theory. P. Natl. Acad. Sci. U.S.A. 113 (13), 3551–3556. doi: 10.1073/pnas.1522130113
Ghazian, N., Braun, J., Owen, M., Lortie, C. J., Cho, C. (2021). Seed aggregation tips the scale in plant competition. Community Ecol. 22 (3), 403–412. doi: 10.1007/s42974-021-00064-5
Hao, J. H., Jin, J. J., Chen, G. Q., Wang, L. X. (2015). Breeding system of a noxious invasive alien plant, Ambrosia artemisiifolia L. Acta Ecol. Sin. 35 (8), 2516–2520. doi: 10.5846/stxb201306101590
Hodgson, E. E., Essington, T. E., Halpern, B. S. (2017). Density dependence governs when population responses to multiple stressors are magnified or mitigated. Ecology 98 (10), 2673–2683. doi: 10.1002/ecy.1961
Houseman, G. R. (2014). Aggregated seed arrival alters plant diversity in grassland communities. J. Plant Ecol. 7 (1), 51–58. doi: 10.1093/jpe/rtt044
Jia, F. Q., Han, N. E., Zhang, X. F., Zhang, W., Zhao, Y. (2011). Age structures of components of Leymus chinensis population in different habitats in the Yili River Valley Area, China. Chin. J. Grassl. 33 (2), 95–99.
Joly, M., Bertrand, P., Gbangou, R. Y., White, M. C., Dubé, J., Lavoie, C. (2011). Paving the way for invasive species: road type and the spread of common ragweed (Ambrosia artemisiifolia). Environ. Manage. 48, 514–522. doi: 10.1007/s00267-011-9711-7
Kanarek, A. R., Webb, C. T., Barfield, M., Holt, R. D. (2013). Allee effects, aggregation, and invasion success. Theor. Ecol. 6, 153–164. doi: 10.1007/s12080-012-0167-z
Kröel-Dulay, G., Csecserits, A., Szitár, K., Molnár, E., Szabó, R., Ónodi, G., et al. (2019). The potential of common ragweed for further spread: invasibility of different habitats and the role of disturbances and propagule pressure. Biol. Invasions 21, 137–149. doi: 10.1007/s10530-018-1811-3
Leiblein, M. C., Lösch, R. (2011). Biomass development and CO2 gas exchange of Ambrosia artemisiifolia L. under different soil moisture conditions. Flora 206, 511–516. doi: 10.1016/j.flora.2010.09.011
Lemke, A., Kowarik, I., von der Lippe, M. (2019). How traffic facilitates population expansion of invasive species along roads: the case of common ragweed in Germany. J. Appl. Ecol. 56 (2), 413–422. doi: 10.1111/1365-2664.13287
Leskovšek, R., Eler, K., Batič, F., Simončič, A. (2012). The influence of nitrogen, water and competition on the vegetative and reproductive growth of common ragweed (Ambrosia artemisiifolia L.). Plant Ecol. 213, 769–781. doi: 10.1007/s11258-012-0040-6
Levine, J. M., Adler, P. B., Yelenik, S. G. (2004). A meta-analysis of biotic resistance to exotic plant invasions. Ecol. Lett. 7 (10), 975–989. doi: 10.1111/j.1461-0248.2004.00657.x
Lewis, M. A., Kareiva, P. (1993). Allee dynamics and the spread of invading organisms. Theor. Popul. Biol. 43 (2), 141–158. doi: 10.1006/tpdi.1993.1007
Li, X. M., Liao, W. J., Wolfe, L. M., Zhang, D. Y. (2012). No evolutionary shift in the mating system of North American Ambrosia artemisiifolia (Asteraceae) following its introduction to China. PloS One 7 (2), e31935. doi: 10.1371/journal.pone.0031935
Liebhold, A. M., Tobin, P. C. (2008). Population ecology of insect invasions and their management. Annu. Rev. Entomol. 53, 387–408. doi: 10.1146/annurev.ento.52.11
Liu, R. H., Chen, Q. W., Dong, B. C., Yu, F. H. (2014). Effffects of vegetative propagule pressure on the establishment of an introduced clonal plant, Hydrocotyle vulgaris. Sci. Rep. 4, 5507. doi: 10.1038/srep05507
Liu, X. L., Li, H. Q., Wang, J. H., Sun, X. P., Xing, L. G. (2021). The current and future potential geographical distribution of common ragweed, Ambrosia artemisiifolia in China. Pakistan J. Bot. 53, 167–172. doi: 10.30848/PJB2021-1(18
Lockwood, J. L., Cassey, P., Blackburn, T. M. (2009). The more you introduce the more you get: the role of colonization pressure and propagule pressure in invasion ecology. Divers. Distrib. 15 (5), 904–910. doi: 10.1111/j.1472-4642.2009.00594.x
Lockwood, J. L., Hoopes, M., Marchetti, M. (2007). Invasion Ecology (Malden: Blackwell Publishing Ltd.).
Marco, M., Paola, D. M., Alfonso, C., Marzia, O., Simone, O. (2006). Long distance transport of ragweed pollen as a potential cause of allergy in central Italy. Ann. Allerg. Asthma. Im. 96 (1), 86–91. doi: 10.1016/S1081-1206(10)61045-9
Montagnani, C., Gentili, R., Smith, M., Guarino, M. F., Citterio, S. (2017). The worldwide spread, success, and impact of ragweed (Ambrosia spp.). Crit. Rev. Plant Sci. 36, 139–178. doi: 10.1080/07352689.2017.1360112
Moore, J. L., Mouquet, N., Lawton, J. H., Loreau, M. (2003). Coexistence, saturation and invasion in simulated plant assemblages. Oikos 94 (2), 303–314. doi: 10.1034/j.1600-0706.2001.940211.x
Pinke, G., Karacsony, P., Czucz, B., Botta-Dukat, Z. (2011). Environmental and land-use variables determining the abundance of Ambrosia artemisiifolia in arable fields in Hungary. Preslia 83 (2), 219–235. doi: 10.1111/j.1759-6831.2011.00132.x
Razanajatovo, M., Maurel, N., Dawson, W., Essl, F., Kreft, H., Pergl, J., et al. (2016). Plants capable of selfing are more likely to become naturalized. Nat. Commun. 7 (1), 13313. doi: 10.1038/ncomms13313
Reijers, V. C., van den Akker, M., Cruijsen, P. M., Lamers, L. P., van der Heide, T. (2019). Intraspecific facilitation explains the persistence of Phragmites australis in modified coastal wetlands. Ecosphere 10 (8), e02842. doi: 10.1002/ecs2.2842
Renne, I. J., Sinn, B. T., Shook, G. W., Sedlacko, D. M., Dull, J. R., Villarreal, D., et al. (2014). Eavesdropping in plants: delayed germination via biochemical recognition. J. Ecol. 102 (1), 86–94. doi: 10.1111/1365-2745.12189
Rojas-Botero, S., Kollmann, J., Teixeira, L. H. (2022). Competitive trait hierarchies of native communities and invasive propagule pressure consistently predict invasion success during grassland establishment. Biol. Invasions 24 (1), 107–122. doi: 10.1007/s10530-021-02630-4
Schafner, U., Steinbach, S., Sun, Y. (2020). Biological weed control to relieve millions from Ambrosia allergies in Europe. Nat. Commun. 11 (1), 1–7. doi: 10.1038/s41467-020-15586-1
Schmitt, J., Eccleston, J., Ehrhardt, D. W. (1987). Density-dependent flowering phenology, outcrossing, and reproduction in Impatiens capensis. Oecologia 72, 341–347. doi: 10.1007/BF00377561
Simberloff, D. (2009). The role of propagule pressure in biological invasions. Annu. Rev. Ecol. Evol. S. 40, 81–102. doi: 10.1146/annurev.ecolsys.11
Skjøth, C. A., Sun, Y., Karrer, G., Sikoparija, B., Smith, M., Schaffner, U., et al. (2019). Predicting abundances of invasive ragweed across Europe using a “top-down” approach. Sci. Total Enviro. 686, 212–222. doi: 10.1016/j.scitotenv.2019.05.215
Smith, M., Cecchi, L., Skjøth, C. A., Karrer, G., Šikoparija, B. (2013). Common ragweed: a threat to environmental health in Europe. Environ. Int. 61, 115–126. doi: 10.1016/j.envint.2013.08.005
Stępalska, D., Myszkowska, D., Piotrowicz, K., Kluska, K., Chłopek, K., Grewling, Ł., et al. (2020). High Ambrosia pollen concentrations in Poland respecting the long distance transport (LDT). Sci. Total Environ. 736, 139615. doi: 10.1016/j.scitotenv.2020.139615
Sun, Y., Roderick, G. K. (2019). Rapid evolution of invasive traits facilitates the invasion of common ragweed, Ambrosia artemisiifolia. J. Ecol. 107 (6), 2673–2687. doi: 10.1111/1365-2745.13198
Tamura, M., Ohgushi, T., Ida, T. Y. (2020). Intraspecific neighbourhood effect: population-level consequence of aggregation of highly defended plants. Funct. Ecol. 34 (3), 597–605. doi: 10.1111/1365-2435.13515
Taylor, C. M., Hastings, A. (2005). Allee effects in biological invasions. Ecol. Lett. 8, 895–908. doi: 10.1111/j.1461-0248.2005.00787.x
Tobin, P. C., Berec, L., Liebhold, A. M. (2011). Exploiting Allee effects for managing biological invasions. Ecol. Lett. 14 (6), 615–624. doi: 10.1111/j.1461-0248.2011.01614.x
Urbanowicz, C., Hutyra, L. R., Stinson, K. A. (2018). The effects of urbanization and land use on ragweed distribution. Ecosphere 9 (12), e02512. doi: 10.1002/ecs2.2512
Vedder, D., Leidinger, L., Sarmento Cabral, J. (2021). Propagule pressure and an invasion syndrome determine invasion success in a plant community model. Ecol. Evol. 11 (23), 17106–17116. doi: 10.1002/ece3.8348
Velázquez, J., Garrahan, J. P., Eichhorn, M. P. (2014). Spatial complementarity and the coexistence of species. PloS One 9 (12), e114979. doi: 10.1371/journal.pone.0114979
Vilà, M., Espinar, J. L., Hejda, M., Hulme, P. E., Jarošík, V., Maron, J. L., et al. (2011). Ecological impacts of invasive alien plants: a meta-analysis of their effects on species, communities, and ecosystems. Ecol. Lett. 14 (7), 702–708. doi: 10.1111/j.1461-0248.2011.01628.x
Visscher, A. M., Wellstein, C., Vanek, S., Bricca, A., Meza, K., Huaraca, J., et al. (2023). Drivers of growth and establishment of the invasive plant Rumex acetosella within Andean fallow systems. Agr. Ecosyst. Environ. 351, 108446. doi: 10.1016/j.agee.2023.108446
Wang, H., Liu, T., Zhao, W., Liu, X., Sun, M., Su, P., et al. (2022). Reduced invasiveness of common ragweed (Ambrosia artemisiifolia) using low-dose herbicide treatments for high-efficiency and eco-friendly control. Front. Plant Sci. 13. doi: 10.3389/fpls.2022.861806
Warren, R. J., Bahn, V., Bradford, M. A. (2012). The interaction between propagule pressure, habitat suitability and density-dependent reproduction in species invasion. Oikos 121 (6), 874–881. doi: 10.1111/j.1600-0706.2011.20174.x
Warren, R. J., Bradford, M. A. (2011). The shape of things to come: woodland herb niche contraction begins during recruitment in mesic forest microhabitat. P. R. Soc B-Biol. Sci. 278 (1710), 1390–1398. doi: 10.1098/rspb.2010.1886
Williams, H. E., Brockerhoff, E. G., Liebhold, A. M., Ward, D. F. (2021). Probing the role of propagule pressure, stochasticity, and Allee effects on invasion success using experimental introductions of a biological control agent. Ecol. Entomol. 46, 383–393. doi: 10.1111/een.12979
Wittmann, M. J., Metzler, D., Gabriel, W., Jeschke, J. M. (2014). Decomposing propagule pressure: the effects of propagule size and propagule frequency on invasion success. Oikos 123 (4), 441–450. doi: 10.1111/j.1600-0706.2013.01025.x
Xian, X., Zhao, H., Wang, R., Huang, H., Chen, B., Zhang, G., et al. (2023). Climate change has increased the global threats posed by three ragweeds (Ambrosia L.) in the Anthropocene. Sci. Total Enviro. 859, 160252. doi: 10.1016/j.scitotenv.2022.160252
You, W. H., Han, C. M., Fang, L. X., Du, D. L. (2016). Propagule pressure, habitat conditions and clonal integration influence the establishment and growth of an invasive clonal plant, Alternanthera philoxeroides. Front. Plant Sci. 7, 568. doi: 10.3389/fpls.2016.00568
Zhao, W., Liu, T., Sun, M., Wang, H., Liu, X., Su, P. (2022). Rapid monitoring of Ambrosia artemisiifolia in semi-arid regions based on ecological convergence and phylogenetic relationships. Front. Ecol. Evol. 10. doi: 10.3389/fevo.2022.926990
Keywords: propagule pressure, Allee effect, aggregation effect, invasion probability, invasion threshold
Citation: Zhao W, Xue Z, Liu T, Wang H and Han Z (2023) Factors affecting establishment and population growth of the invasive weed Ambrosia artemisiifolia. Front. Plant Sci. 14:1251441. doi: 10.3389/fpls.2023.1251441
Received: 01 July 2023; Accepted: 05 September 2023;
Published: 22 September 2023.
Edited by:
David R. Clements, Trinity Western University, CanadaReviewed by:
Satu Ramula, University of Turku, FinlandCopyright © 2023 Zhao, Xue, Liu, Wang and Han. This is an open-access article distributed under the terms of the Creative Commons Attribution License (CC BY). The use, distribution or reproduction in other forums is permitted, provided the original author(s) and the copyright owner(s) are credited and that the original publication in this journal is cited, in accordance with accepted academic practice. No use, distribution or reproduction is permitted which does not comply with these terms.
*Correspondence: Tong Liu, NDY5MDA0NTA5QHFxLmNvbQ==
†These authors share first authorship
Disclaimer: All claims expressed in this article are solely those of the authors and do not necessarily represent those of their affiliated organizations, or those of the publisher, the editors and the reviewers. Any product that may be evaluated in this article or claim that may be made by its manufacturer is not guaranteed or endorsed by the publisher.
Research integrity at Frontiers
Learn more about the work of our research integrity team to safeguard the quality of each article we publish.