- 1College of Horticulture and Gardening, Yangtze University, Jingzhou, Hubei, China
- 2Botany and Microbiology Department, College of Science, King Saud University, Riyadh, Saudi Arabia
- 3Plant Production Department, College of Food and Agricultural Sciences, King Saud University, Riyadh, Saudi Arabia
Soil drought is detrimental to plant growth worldwide, particularly by triggering reactive oxygen species (ROS) burst. Serendipita indica (Si), a culturable root-associated endophytic fungus, can assist host plants in dealing with abiotic stresses; however, it is unknown whether and how Si impacts the drought tolerance of citrus plants. To unravel the effects and roles of Si on drought-stressed plants, trifoliate orange (Poncirus trifoliata L. Raf.; a citrus rootstock) seedlings were inoculated with Si and exposed to soil drought, and growth, gas exchange, ROS levels, antioxidant defense systems, and expression of genes encoding antioxidant enzymes and fatty acid desaturases in leaves were measured. Soil drought suppressed plant biomass, whereas Si inoculation significantly increased plant biomass (10.29%-22.47%) and shoot/root ratio (21.78%-24.68%) under ample water and drought conditions, accompanied by improved net photosynthetic rate (105.71%), water use efficiency (115.29%), chlorophyll index (55.34%), and nitrogen balance index (63.84%) by Si inoculation under soil drought. Soil drought triggered an increase in leaf hydrogen peroxide and superoxide anion levels, while Si inoculation significantly reduced these ROS levels under soil drought, resulting in lower membrane lipid peroxidation with respect to malondialdehyde changes. Furthermore, Si-inoculated seedlings under soil drought had distinctly higher levels of ascorbate and glutathione, as well as catalase, peroxidase, and glutathione peroxidase activities, compared with no-Si-inoculated seedlings. Si inoculation increased the expression of leaf PtFAD2, PtFAD6, PtΔ9, PtΔ15, PtFe-SOD, PtCu/Zn-SOD, PtPOD, and PtCAT1 genes under both ample water and soil drought conditions. Overall, Si-inoculated trifoliate orange plants maintained a low oxidative burst in leaves under drought, which was associated with stimulation of antioxidant defense systems. Therefore, Si has great potential as a biostimulant in enhancing drought tolerance in plants, particularly citrus.
Introduction
Drought stress (DS) is a frequent environmental factor that has a detrimental impact on plant physiological activities and morphological performance, such as lowering leaf gas exchange, slowing plant growth, and overproduction of reactive oxygen species (ROS) (Ahluwalia et al., 2021). ROS are highly reactive and toxic by-products of photosynthesis and photorespiration processes in plants, and the excess ROS causes oxidative damage to various macromolecules, thereby limiting plant growth and development (Ilyas et al., 2021; Tyagi et al., 2022a). Superoxide anion () and hydrogen peroxide (H2O2) are the two most prevalent ROS induced by DS in plants (Miller et al., 2010). Plants also possess antioxidant defense systems to scavenge ROS, where antioxidant enzymes include superoxide dismutase (SOD), catalase (CAT), peroxidase (POD), and others, and non-enzymatic antioxidants include ascorbic acid (AsA), glutathione (GSH), carotenoids, and tocopherols (Mukarram et al., 2021). As a result, uncovering changes in antioxidant defense systems could clarify the drought-resistant potential of plants.
Citrus is the most widely grown fruit crop in the world (Addi et al., 2022). Because of its poor root hairs, trifoliate orange (Poncirus trifoliata L. Raf.), a common citrus rootstock, relies heavily on extraradical hyphae of arbuscular mycorrhizae in roots for water and nutrient uptake from the soil (Ortas, 2012). Symbiotic associations between arbuscular mycorrhizal fungi (AMF) and plants are prevalent, with AMF providing water and mineral nutrients to the host and the host providing carbohydrates to the fungal partner (Prasad et al., 2008; Tyagi et al., 2022b). Earlier studies have demonstrated that AMF could enhance drought tolerance in citrus, and the underlying mechanism is associated with mycorrhizal improvement of root structure and physiological activities, as well as stressed gene expression activation (Marulanda et al., 2007; Yaghoubian et al., 2014; Cheng et al., 2021; Liu et al., 2022; Wang et al., 2023). However, the application of AMF in the citrus field is limited because it cannot be cultured in vitro on a large scale without host plants. As a result, selecting an effective culturable endophytic fungus with functions similar to AMF has become a pressing problem in citriculture.
Serendipita indica (formerly Piriformospora indica) (Si) is a culturable endophytic fungus that can colonize a variety of host roots, including citrus (Varma et al., 2012; Yang et al., 2021a). Si possesses AMF-like characteristics (Mensah et al., 2020) and was isolated from an Indian desert (Verma et al., 1998), suggesting that it may be drought-tolerant. Earlier studies had reported significant increases in biomass and sustained growth in barley (Hordeum vulgare) and Arabidopsis (Arabidopsis thaliana) after inoculation with Si under drought (Sherameti et al., 2008; Ghaffari et al., 2019). Proteomics demonstrated that the colonization of Si raised photosynthesis-related protein levels in drought-stressed host plants (Ghaffari et al., 2019). Si colonization in cabbage (Brassica campestris) decreased leaf malondialdehyde (MDA) levels under DS, and several antioxidant enzyme activities were upregulated within 24 h (Sun et al., 2010). After Si inoculation, wheat (Triticum aestivum), eggplant (Solanum melongena), and walnut (Juglans regia) decreased ROS levels and elevated CAT and POD activities in leaves (Yaghoubian et al., 2014; Swetha and Padmavathi, 2020; Liu et al., 2021). Si inoculation also changes the expression of stressed genes under DS. Si inoculation, for example, boosted the expression of four drought-associated genes in leaves of drought-stressed cabbage, namely, DREB2A, CBL1, ANAC072, and RD29A (Sun et al., 2010). However, Si inoculation in wheat inhibited CAT activity under drought conditions, achieving a significant level at −0.5 MPa (Hosseini et al., 2017). In maize, CAT and ascorbate peroxidase (APX) activities were also decreased under DS by Si (Hosseini et al., 2018). These conflicting results show that Si is variable in modulating antioxidant defense systems in host plants and more research needs to be investigated, especially as the molecular mechanism lags behind physiological advances.
Citrus plants, particularly trifoliate orange, have been demonstrated to be a host plant for Si, and inoculation with Si promoted their growth behavior through increasing auxin levels and nutrient acquisition (Yang et al., 2021a; Liu et al., 2023). However, it is unknown whether and how Si impacts the drought tolerance of trifoliate orange in terms of antioxidant defense systems. This study was carried out to investigate the effects of Si inoculation on growth, leaf gas exchange, ROS levels, antioxidant enzyme activities, antioxidant levels, and the expression of genes encoding antioxidant enzymes and fatty acid desaturases under DS. Such study can evaluate the potential of Si as a biostimulant for drought tolerance in citrus.
Materials and methods
Plant culture and experimental design
Four-leaf-old trifoliate orange seedlings grown in autoclaved sands were chosen. Si was inoculated at the time of transplanting. Si was provided by Prof. Z.-H. Tian (Yangtze University), which was kept in our laboratory. The proliferation of this fungus was performed in vitro as per the protocol of Yang et al. (2021a), achieving a spore suspension of 5.0 × 108 CFU/mL and a mycelial solution of 0.018 g/mL.
Three seedlings were planted in a plastic pot that had been pre-filled with an autoclaved mixture consisting of soil and river sands mixed in a 4: 1 ratio by volume to obtain a relative low Olsen-P level (9.73 mg/kg). At the time of transplanting, 12.5 mL of spore suspension and 14.5 mL of mycelial solution were inoculated around roots of potted seedlings as the inoculation treatment (+Si). In contrast, the uninoculated treatment (−Si) also received the same volume but autoclaved spore suspension and mycelium solution (Rong et al., 2022). The treated seedlings were subjected to the controlled environments described by Cao et al. (2023). The weighing method was used to keep the soil moisture of these potted plants at 75% of the maximum water holding capacity (MWHC) in the field (well-watered, WW). The condition lasted for 7 weeks. Subsequently, the soil moisture regime was altered for half of the plants to 55% of the MWHC in the field (DS) for 9 weeks, while the soil moisture regime remained unchanged for the remaining plants.
Thus, this study consisted of two factors: Si inoculation treatments (+Si and − Si) and two soil moistures (WW and DS). There were four treatments, each with six replications, with a total of 72 seedlings and 24 pots.
Determination of growth and root fungal colonization frequency
After 9 weeks of drought exposure, the treated plants were harvested and weighed promptly. The Epson Root Scanner (V700) and WinRHIZO software (2007b) were used to quantify root surface area and volume. Then, 1-cm root segments were selected and stained for Si colonization in the roots using the method of Phillips and Hayman (1970). In addition, root segments were cut into thin slices of longitudinal sections using a double-sided blade. Subsequently, a drop of 0.05% trypan blue was introduced to observe the fungal colonization. Root fungal colonization was examined under a microscope, and root fungal colonization frequency was estimated as the percentage of Si -colonized root segment number to total detected root segment number.
Determination of leaf physiological variables
On a sunny day (9:00 a.m.) before harvest, leaf gas exchange parameters, including net photosynthetic rate (Pn), transpiration rate (Tr), and stomatal conductance (Gs), were measured on the fourth leaf below the tip of trifoliate orange seedlings using a Li-6400 portable photosynthesizer (Li-COR, USA). The photosynthesizer was preheated for 20 min before used. After calibrating and zeroing the photosynthesizer, the leaf area, ambient water vapor pressure, and CO2 concentrations were set at 6.5 cm2, 1.01 kPa, and 400 µmol/m2/s, respectively. During measurement, the data were recorded after stabilization. Water use efficiency (WUE) was defined as the Pn/Tr ratio.
A portable plant polyphenol-chlorophyll meter (Dualex Scientific+, Orsay, France) was used to measure nitrogen balance index (Nbi) and chlorophyll index (Chi) in leaves.
The concentration of leaf H2O2 was determined according to the KI colorimetric method reported by Velikova et al. (2000). Leaf levels were assayed using the protocol outlined by Zou et al. (2015). Leaf MDA concentrations were measured according to the thiobarbituric acid method described by Sudhakar et al. (2001).
Leaf CAT activity was determined colorimetrically at 240 nm according to the method described by He et al. (2020). The absorbance of reaction solutions changed by 0.01 at 240 nm in 1 min as a unit (U) of CAT. Leaf POD activity was assayed using the guaiacol method described by Chance and Maehly (1955), where the absorbance of reaction solutions changed by 0.1 at 470 nm in 1 min as a U of POD. Leaf APX activity was determined as per the protocol outlined by Wu (2018), where the reaction solution consisted of 50 mM potassium phosphate buffer (the enzyme extraction solution), 6 mM AsA, and supernatants. The absorbance of reaction solutions changed by 0.01 at 290 nm in 1 min as a U of APX. Leaf glutathione reductase (GR) activity was analyzed according to the method of Chen and Wang (2002), where the reaction mixture consisted of 1 mM NADPH, 0.1 M tricine-NaOH buffer (the enzyme extraction solution), supernatants, and 5 mM oxidized GSH. The absorbance of reaction solutions changed by 0.01 at 340 nm in 1 min as a U of GR.
Fresh leaf samples (0.30 g) were ground into a homogenate in 5 mL of 5% trichloroacetic acid solution and centrifuged at 15,000×g for 15 min. The supernatant was used for the assay of AsA and GSH, and the procedure for the assay had been described in detail by Li et al. (2022).
Determination of the expression of genes encoding antioxidant enzymes and fatty acid desaturases
Total RNA of leaves was extracted using the TaKaRa MiniBEST Plant RNA Extraction Kit. Following detection of total RNA concentration and purity, total RNA was reverse transcribed into cDNA based on the PrimeScript™ RT reagent Kit with the gDNA Eraser kit. Each treatment’s cDNA obtained was employed as the template for RT-PCR amplification. According to the findings of Wu et al. (2019a), five antioxidant enzyme genes and four fatty acid saturase genes were chosen and thus designed for their primers in qRT-PCR (Supplementary Table S1). The internal reference gene in this investigation was β-actin. The SYBR Green PCR Master Mix and Real-time PCR Detection System (BIO-RAD, Hercules, USA) were used for real-time PCR. There were three biological replicates for each determination. The 2−ΔΔCt method (Livak and Schmittgen, 2001) was used to calculate expression of genes. Relative expression of genes was normalized with the uninoculation treatment under WW conditions.
Statistical analysis
The two-way analysis of variance under the condition of SAS software (v8.1) was used to compare the variance of the experimental data, and Duncan’s multiple-range test was performed to assess significant (p< 0.05) differences across treatments.
Results
Effects of DS on root fungal colonization frequency
Fungal colonization was found in roots of Si-inoculated seedlings, but not in no-Si-inoculated seedlings, with more transparent pear-shaped chlamydospores in Si-inoculated roots under DS (Figures 1A, B) than under WW (Figures 1C, D). Root fungal colonization frequency was 30.1% under WW conditions and 61.9% under DS conditions, respectively (Table 1). Si inoculation and DS treatment interacted (p< 0.01) to affect root fungal colonization frequency.
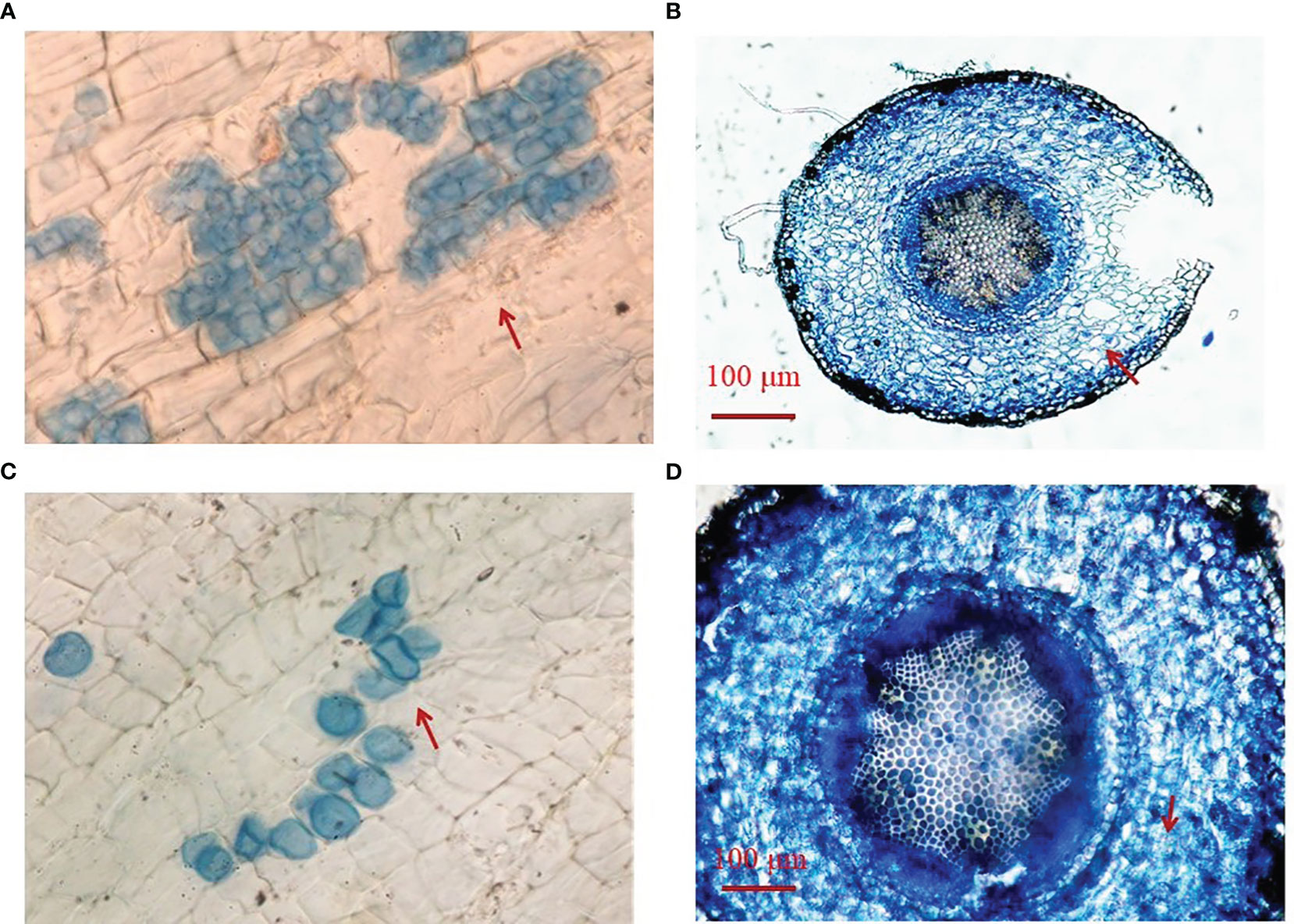
Figure 1 Root colonization of Serendipita indica (Si) in trifoliate orange seedlings under drought stress (A, B) and well-watered (C, D) conditions. The red arrow indicates transparent pear-shaped chlamydospores.
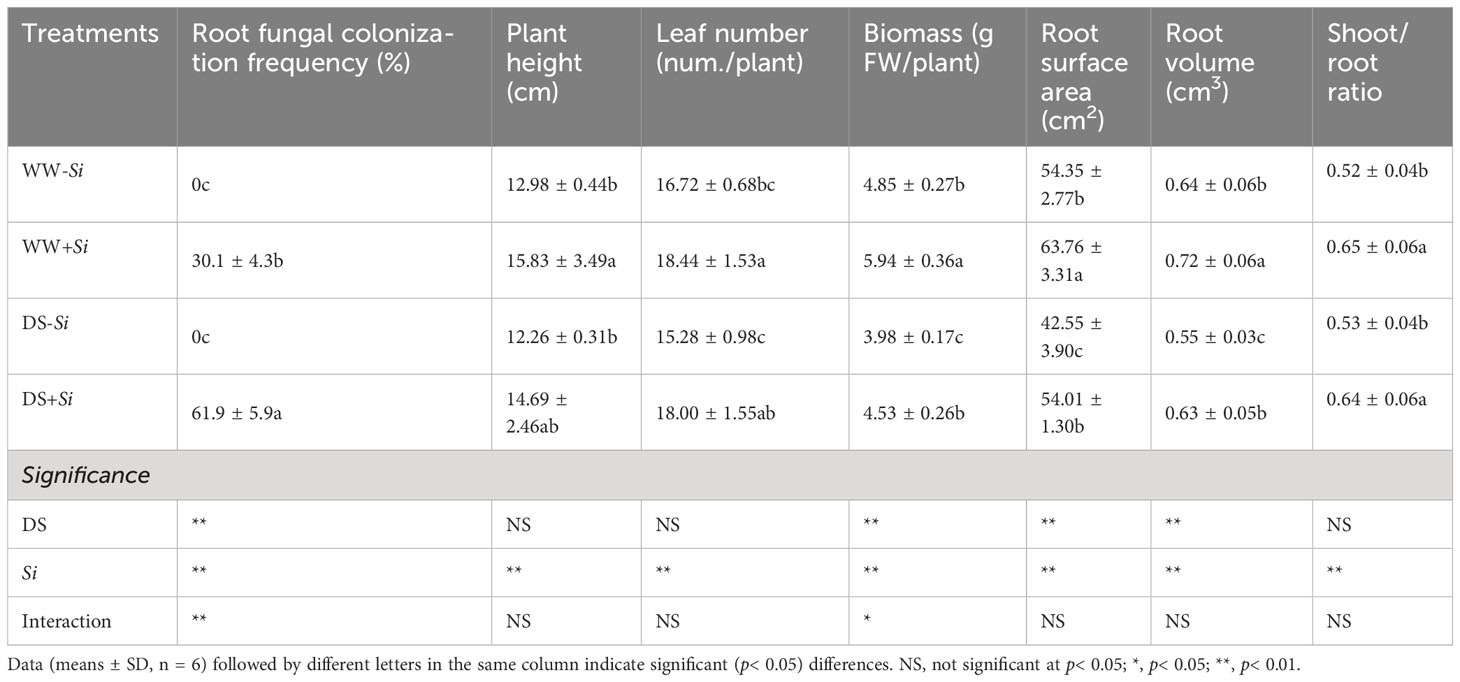
Table 1 Changes in root fungal colonization frequency and plant growth of trifoliate orange seedlings inoculated with Serendipita indica (Si) under well-watered (WW) and drought stress (DS) conditions.
Effects of Si inoculation on plant growth variables under DS
DS and Si inoculation significantly impacted plant growth behavior of trifoliate orange seedlings (Supplementary Figure S1). DS treatment inhibited plant biomass, root surface area, and root volume of Si-inoculated plants by 23.74%, 15.29%, and 12.50%, respectively, compared with WW treatment (Table 1). Leaf number, biomass, root surface, and root volume of no-Si-inoculated plants were also decreased under DS versus WW by 8.38%, 17.94%, 21.71%, and 14.06%, respectively. Si inoculation significantly increased shoot/root ratio, leaf number, biomass, root surface area, and root volume under WW conditions by 24.68%, 17.80%, 22.47%, 26.93%, and 12.50%, respectively, and under DS conditions by 21.78%, 19.82%, 10.29%, 13.82%, 17.31%, and 14.55%, respectively, compared with no-Si inoculation. The interaction of DS treatment and Si inoculation significantly affected biomass.
Effects of Si inoculation on leaf gas exchange under DS
Leaf Pn, Gs, and Tr in Si-inoculated seedlings was inhibited under DS versus WW by 42.89%, 43.63%, and 43.40%, respectively, and leaf Pn and WUE in no-Si-inoculated seedlings were also suppressed by 40.86% and 64.20%, respectively (Figures 2A–D). Compared with no-Si inoculation, Si inoculation profoundly raised leaf Pn, Tr, and Gs under WW conditions by 113.01%, 179.16%, and 165.67%, respectively, and it also raised leaf Pn and WUE by 105.71% and 115.29% under DS conditions, respectively. DS and Si inoculation interactively (p< 0.01) affected Tr, Gs, and WUE (Table 2).
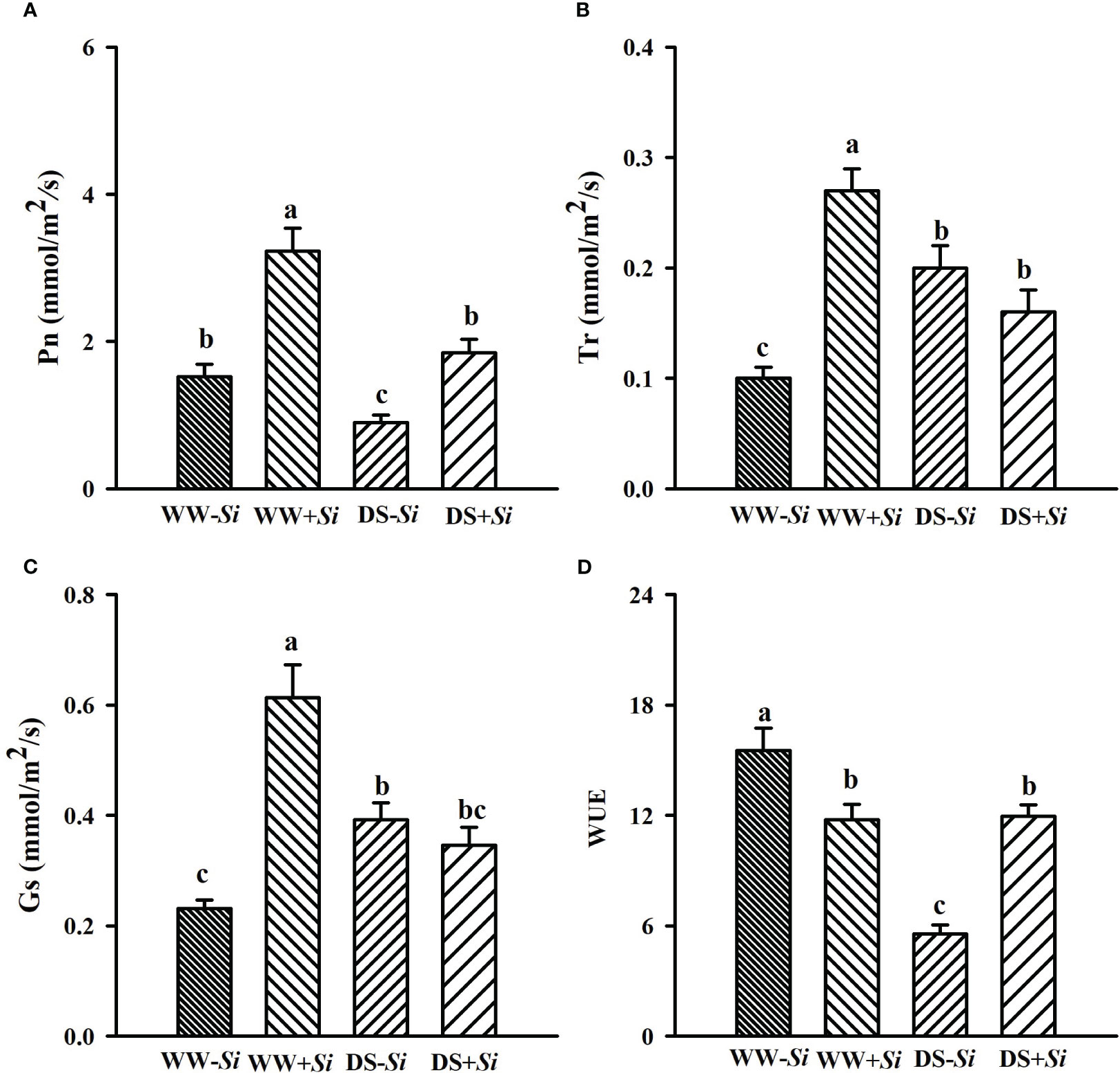
Figure 2 Effects of Serendipita indica (Si) on leaf net photosynthetic rate (Pn) (A), transpiration rate (Tr) (B), stomatal conductance (Gs) (C), and water use efficiency (WUE) (D) of trifoliate orange seedlings under well-watered (WW) and drought stress (DS) conditions. Data (means ± SD, n = 4) followed by different letters upon the bars indicate significant (p< 0.05) differences.
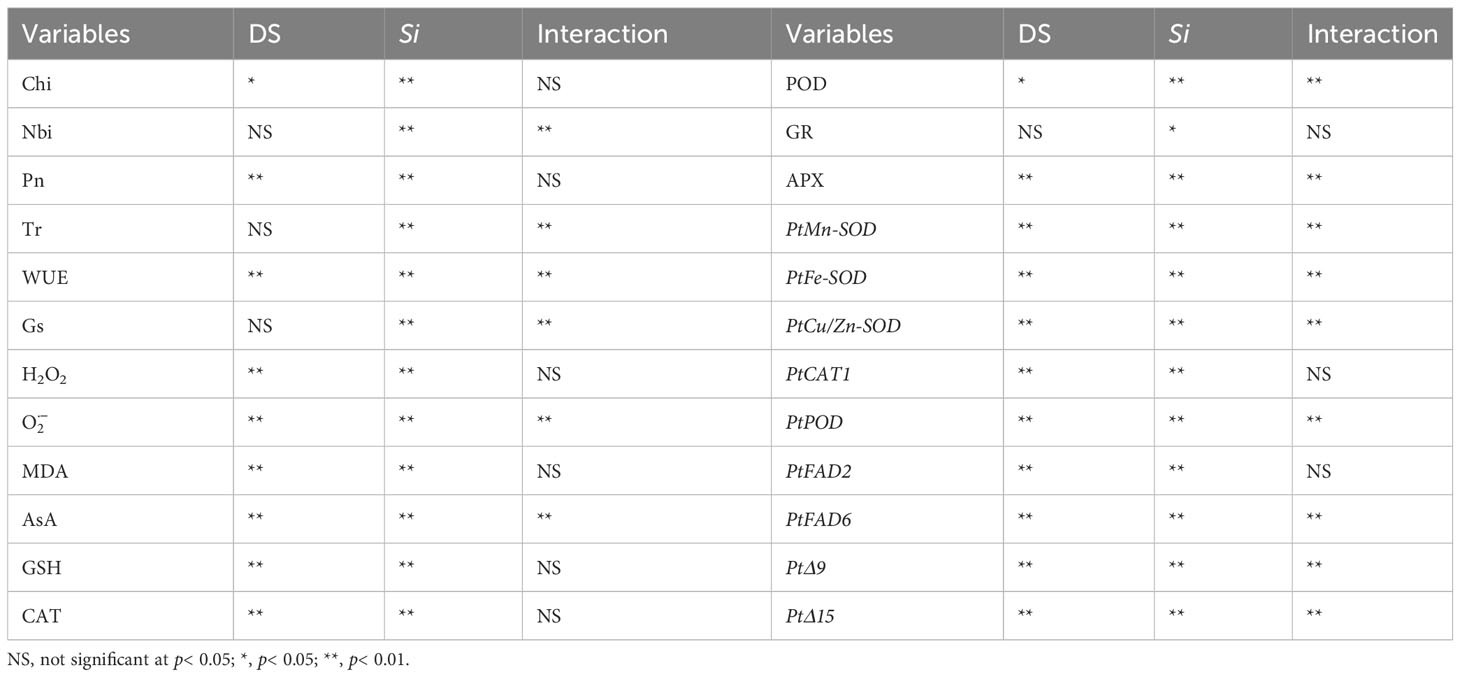
Table 2 Significance of variables in trifoliate orange seedlings inoculated with Serendipita indica (Si) under drought stress (DS) conditions.
Effects of Si inoculation on leaf chlorophyll index and nitrogen balance index under DS
Compared with WW treatment, soil drought significantly reduced leaf Chi of Si-inoculated seedlings and Nbi of no-Si-inoculated seedlings by 8.50% and 18.78%, respectively, coupled with an 8.78% significant increase in Nbi of Si-inoculated seedlings (Figures 3A, B). Si inoculation raised leaf Chi and Nbi by 58.33% and 22.34% under WW conditions and 55.34% and 63.84% under DS conditions, respectively, compared with no-Si treatment. A significant (p< 0.01) interaction appeared in Nbi (Table 2).
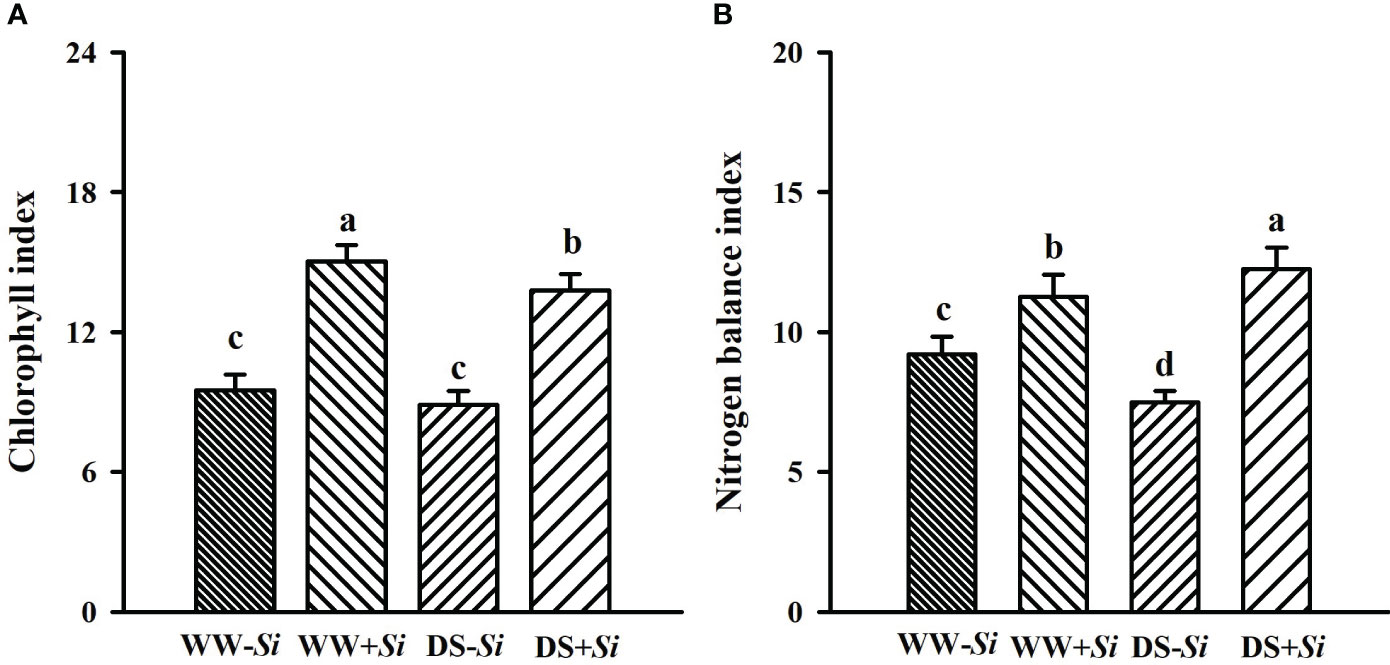
Figure 3 Effects of Serendipita indica (Si) on leaf chlorophyll index (A) and nitrogen balance index (B) in leaves of trifoliate orange seedlings under well-watered (WW) and drought stress (DS) conditions. Data (means ± SD, n = 4) followed by different letters upon the bars indicate significant (p< 0.05) differences.
Effects of Si inoculation on leaf ROS levels under DS
Leaf H2O2 and levels were significantly raised under DS versus WW conditions: 17.21% and 29.26% higher in Si-inoculated seedlings and 20.21% and 69.66% higher in no-Si-inoculated seedlings, respectively (Figures 4A, B). Compared with no-Si treatment, Si inoculation had a significantly inhibitory effect on leaf H2O2 and levels, with 8.88% and 21.54% lower under WW and 11.15% and 40.22% lower under DS, respectively. A significant (p< 0.01) interaction appeared in levels (Table 2).
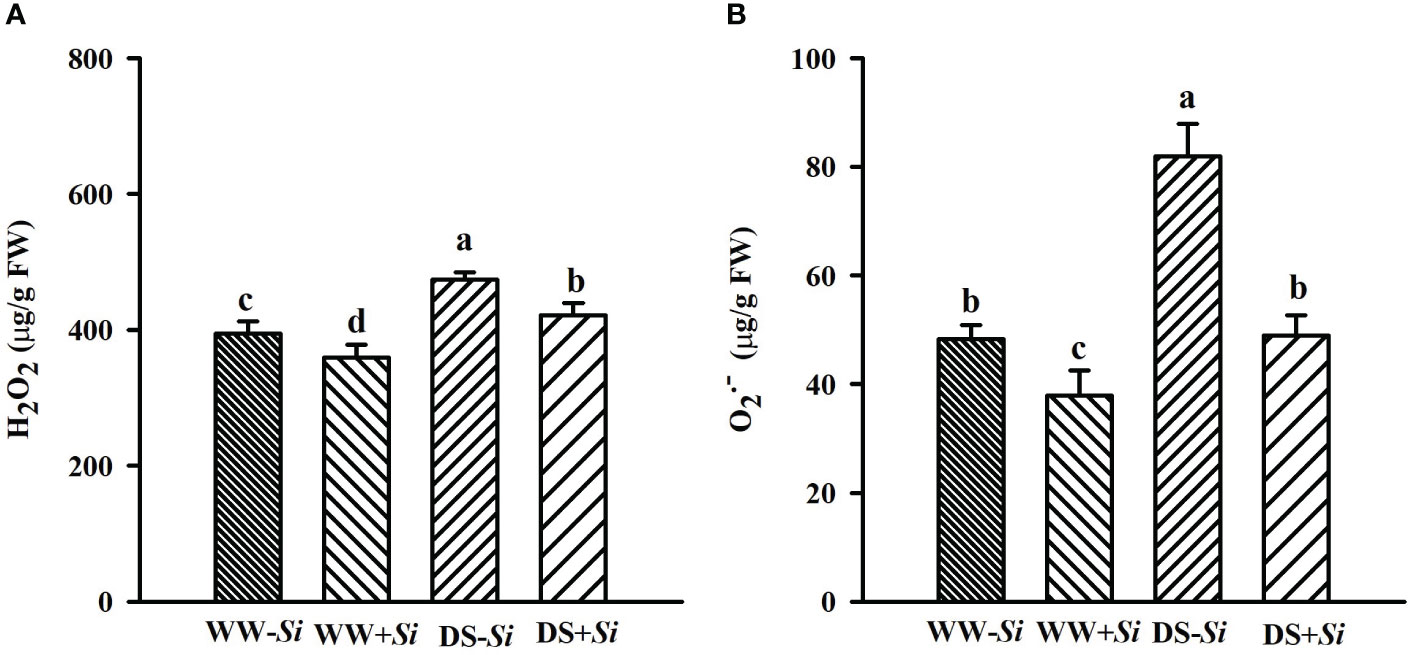
Figure 4 Effects of Serendipita indica (Si) on leaf hydrogen peroxide (H2O2) (A) and superoxide anion radical () (B) concentrations in trifoliate orange seedlings under well-watered (WW) and drought stress (DS) conditions. Data (means ± SD, n = 4) followed by different letters upon the bars indicate significant (p< 0.05) differences.
Effects of Si inoculation on leaf MDA levels under DS
Leaf MDA levels were significantly increased by 16.95% in no-Si-inoculated seedlings, but not Si-inoculated seedlings, under DS versus WW (Figure 5). Compared to no-Si treatment, Si inoculation significantly reduced leaf MDA levels by 15.13% under WW and 17.12% under DS, respectively.
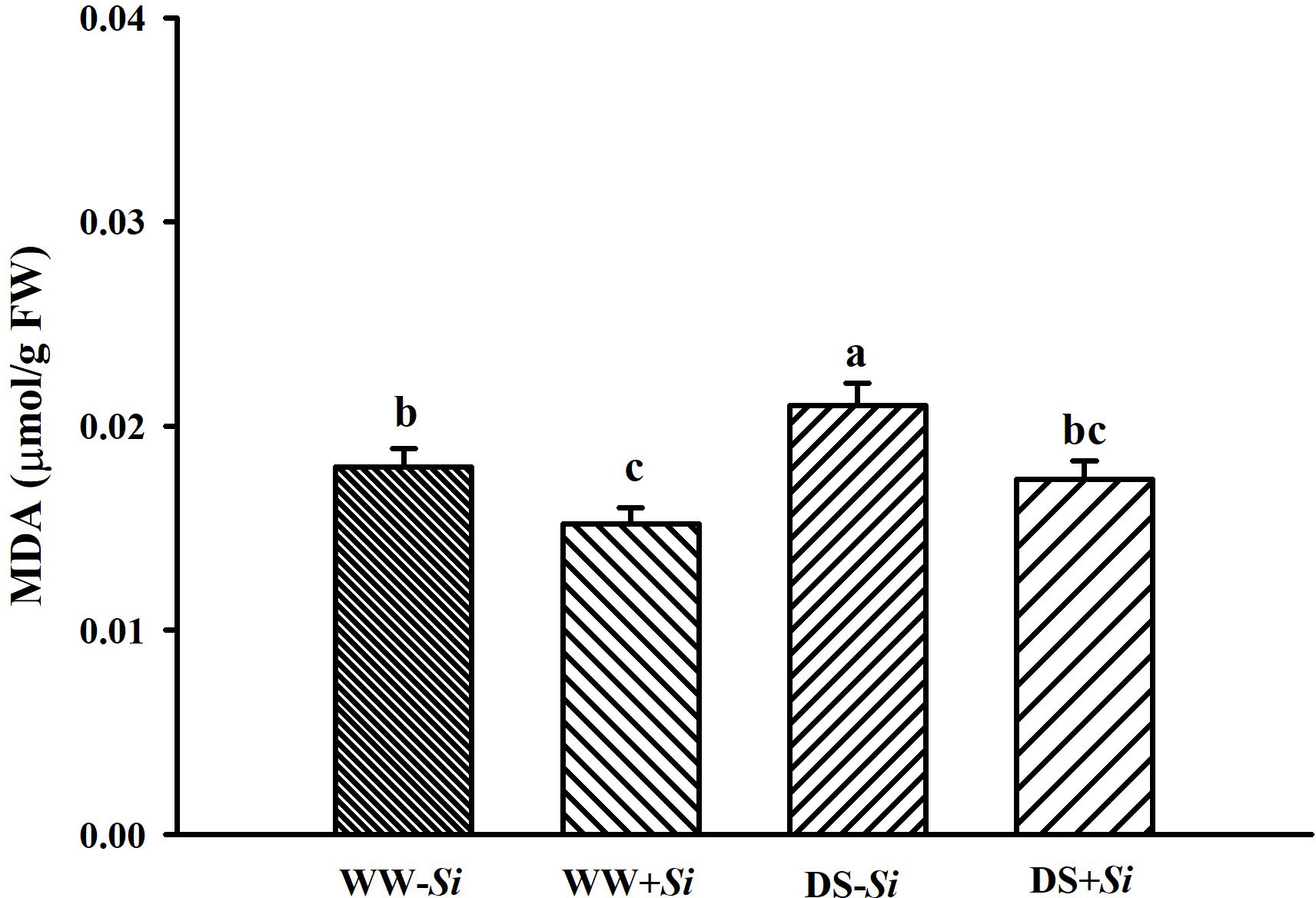
Figure 5 Effects of Serendipita indica (Si) on leaf malondialdehyde (MDA) concentrations in trifoliate orange seedlings under well-watered (WW) and drought stress (DS) conditions. Data (means ± SD, n = 4) followed by different letters upon the bars indicate significant (p< 0.05) differences.
Effects of Si inoculation on leaf antioxidant levels under DS
Leaf AsA and GSH levels were significantly decreased under DS versus WW conditions by 45.89% and 7.13% in Si-inoculated seedlings and 15.04% and 9.42% in no-Si-inoculated seedlings, respectively (Figures 6A, B). However, Si inoculation significantly raised leaf AsA and GSH levels by 85.66% and 11.50% under WW conditions and 18.24% and 14.31% under DS conditions, respectively, compared with no-Si inoculation treatment.
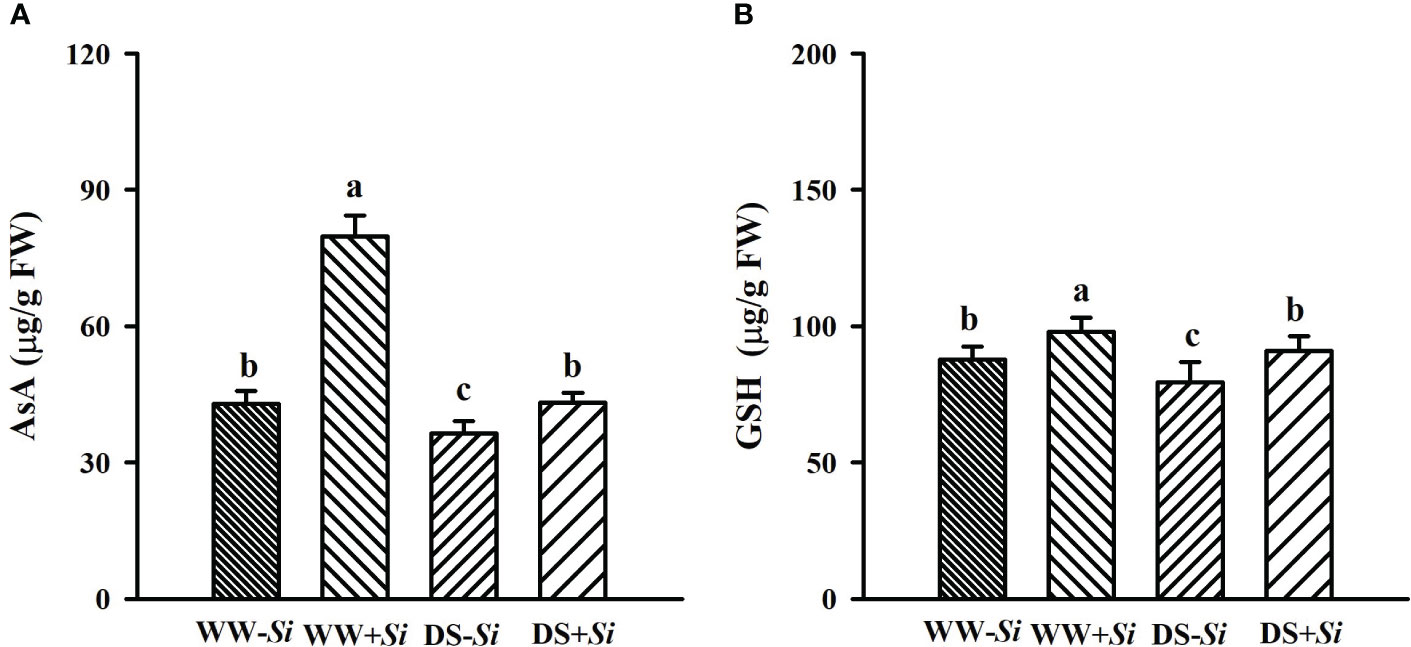
Figure 6 Effects of Serendipita indica (Si) on ascorbic acid (AsA) (A) and glutathione (GSH) (B) concentrations in leaves of trifoliate orange seedlings under well-watered (WW) and drought stress (DS) conditions. Data (means ± SD, n = 4) followed by different letters upon the bars indicate significant (p< 0.05) differences.
Effects of Si inoculation on leaf antioxidant enzyme activities under DS
Compared with WW treatment, DS treatment significantly decreased leaf GR and APX activities by 12.09% and 24.26% in Si-inoculated seedlings, while it distinctly raised leaf POD activities by 34.07% in Si-inoculated seedlings, along with a significant decrease in leaf APX and CAT levels by 16.26% and 23.90% in no-Si-inoculated seedlings (Figures 7A–D). Si inoculation significantly increased leaf GR, APX, POD, and CAT activities under WW by 28.98%, 82.34%, 26.84%, and 11.88%, respectively, compared with no-Si inoculation. Under DS, Si inoculation significantly raised leaf POD, APX, and CAT activities by 87.36%, 64.92%, and 38.43%, respectively, compared with no-Si inoculation. A significant (p< 0.01) interaction appeared in POD and APX activities (Table 2).
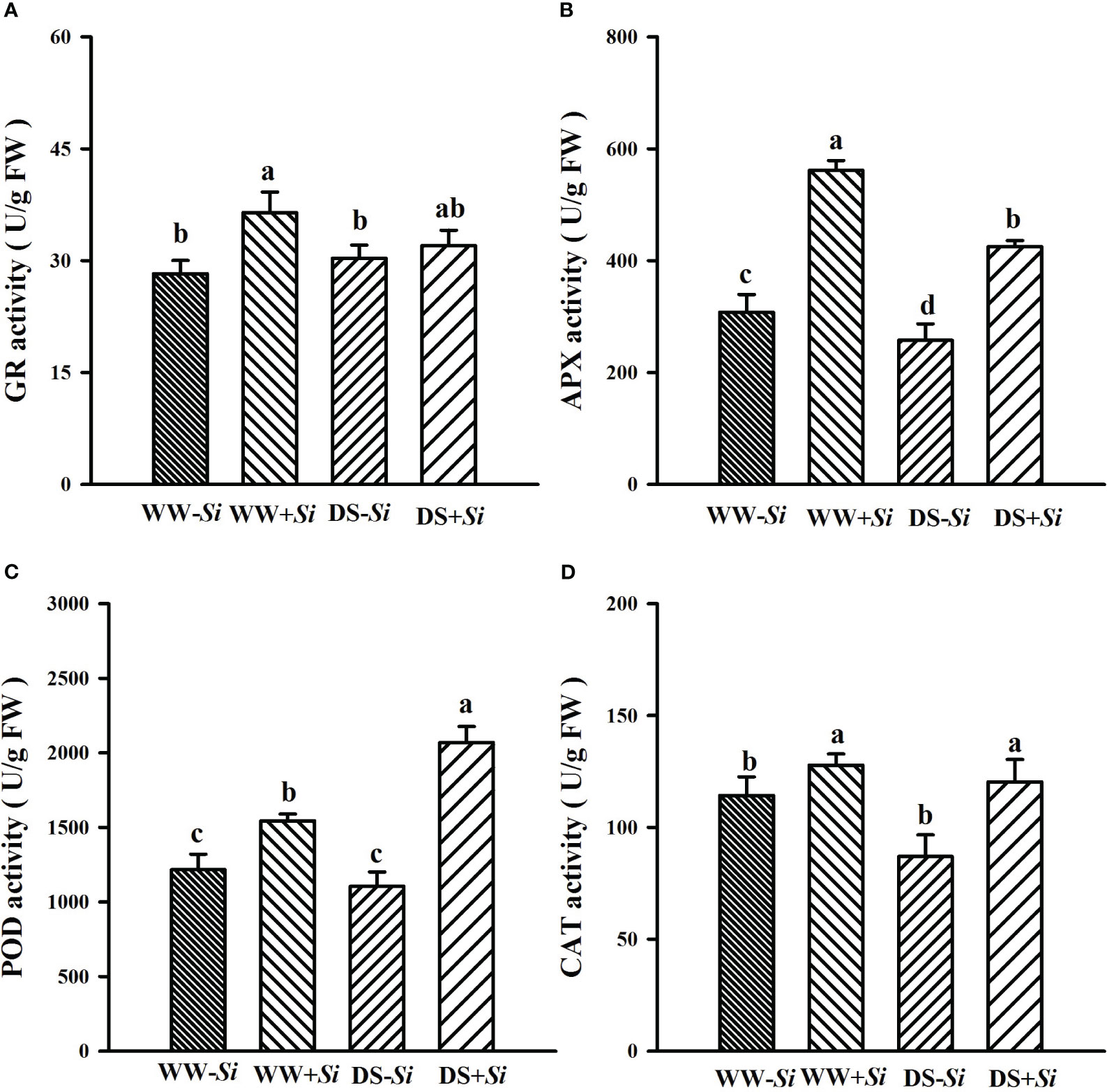
Figure 7 Effects of Serendipita indica (Si) on glutathione reductase (GR) (A), ascorbate peroxidase (APX) (B), peroxidase (POD) (C), and catalase (CAT) (D) in leaves of trifoliate orange seedlings under well-watered (WW) and drought stress (DS) conditions. Data (means ± SD, n = 4) followed by different letters upon the bars indicate significant (p< 0.05) differences.
Effects of Si inoculation on leaf antioxidant enzyme genes expression under DS
Compared with WW treatment, DS treatment triggered upregulation of PtCu/Zn-SOD and PtCAT1 gene expression in leaves of Si-inoculated seedlings by 1.07- and 0.94-fold, respectively, but it also suppressed the expression of PtMn-SOD, PtFe-SOD, and PtPOD genes in leaves of no-Si-inoculated seedlings by 0.77-, 0.53-, and 0.07-fold, respectively (Figure 8). The expression of PtMn-SOD, PtCu/Zn-SOD, PtPOD, and PtCAT1 genes in leaves of no-Si inoculated seedlings was upregulated under DS versus WW conditions by 0.79-, 0.25-, 3.24-, and 1.65-fold, respectively, accompanied by the downregulated expression of PtFe-SOD genes. Si inoculation induced the upregulated expression of PtMn-SOD, PtFe-SOD, PtCu/Zn-SOD, PtPOD, and PtCAT1 genes under WW conditions by 8.38-, 5.46-, 2.12-, 5.28-, and 1.56-fold, respectively, compared with no-Si inoculation. Under DS conditions, Si inoculation upregulated the expression of PtMn-SOD, PtFe-SOD, PtCu/Zn-SOD, PtPOD, and PtCAT1 genes by 0.19-, 3.43-, 4.19-, 0.38-, 0.87-fold, respectively. There was a significant interaction between DS treatment and Si inoculation on the expression of leaf PtMn-SOD, PtFe-SOD, PtCu/Zn-SOD, and PtPOD genes (Table 2).
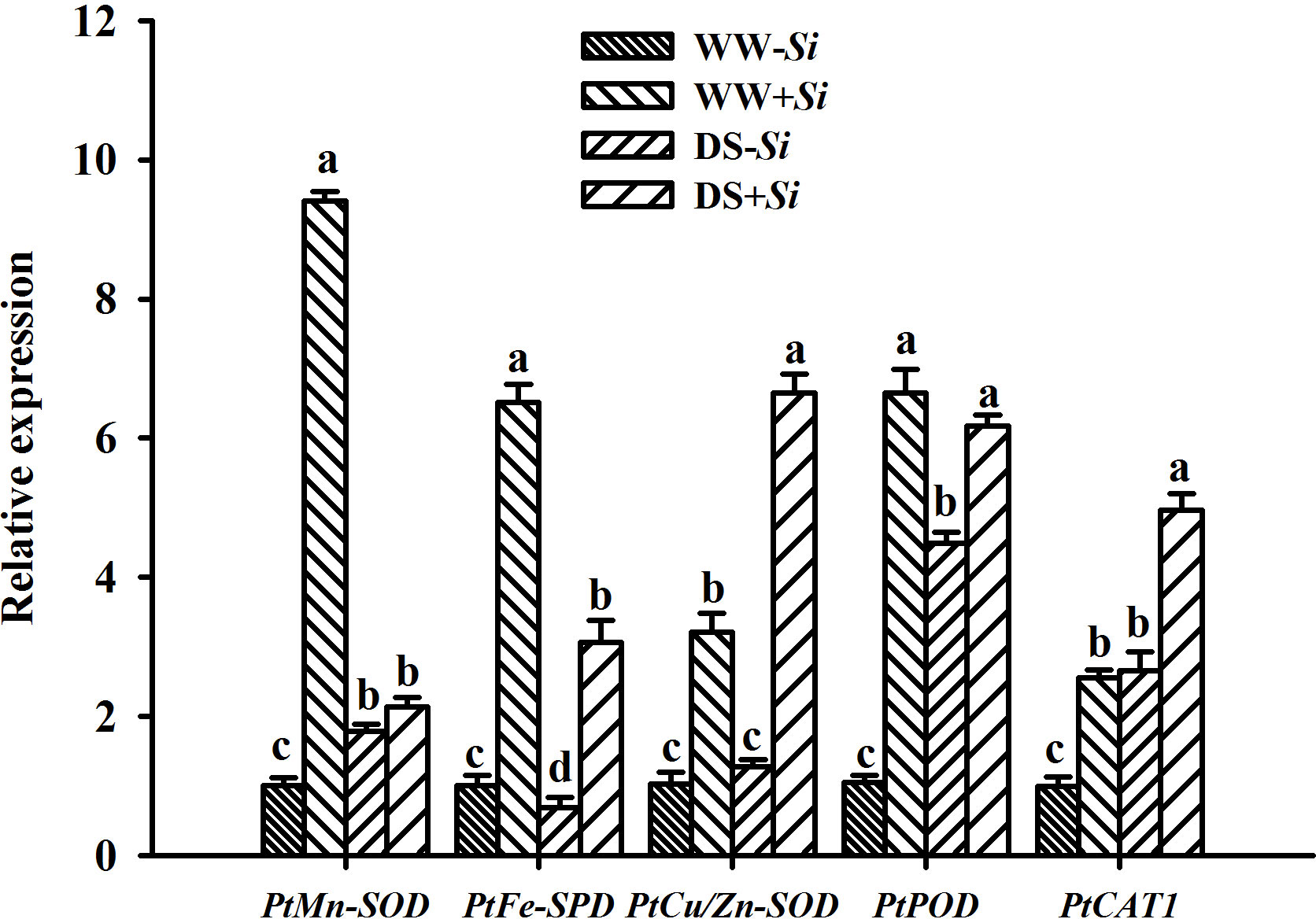
Figure 8 Effects of Serendipita indica (Si) on relative expression of five antioxidant enzyme genes in leaves of trifoliate orange seedlings under well-watered (WW) and drought stress (DS) conditions. Data (means ± SD, n = 3) followed by different letters upon the bars indicate significant (p< 0.05) differences.
Effects of Si inoculation on leaf fatty acid desaturase genes expression under DS
The expression of PtFAD2 gene in leaves of Si-inoculated plants was downregulated by 0.10-fold under DS versus WW conditions, accompanied by 0.35- and 0.86-fold upregulation of PtFAD6 and PtΔ15, respectively (Figure 9). In leaves of no-Si-inoculated plants, the expression of PtΔ9 gene was upregulated by 1.94- fold under DS versus WW conditions. Compared with no-Si-inoculated treatment, Si inoculation significantly raised the expression of leaf PtFAD2, PtFAD6, PtΔ9, and PtΔ15 genes by 3.70-, 3.65-, 3.30-, and 1.18-fold under WW conditions, respectively, and by 4.94-, 8.52-, 0.63-, and 1.86-fold under DS conditions, respectively. DS treatment and Si inoculation interacted significantly to affect the expression of PtFAD6, PtΔ9, and PtΔ15 genes (Table 2).
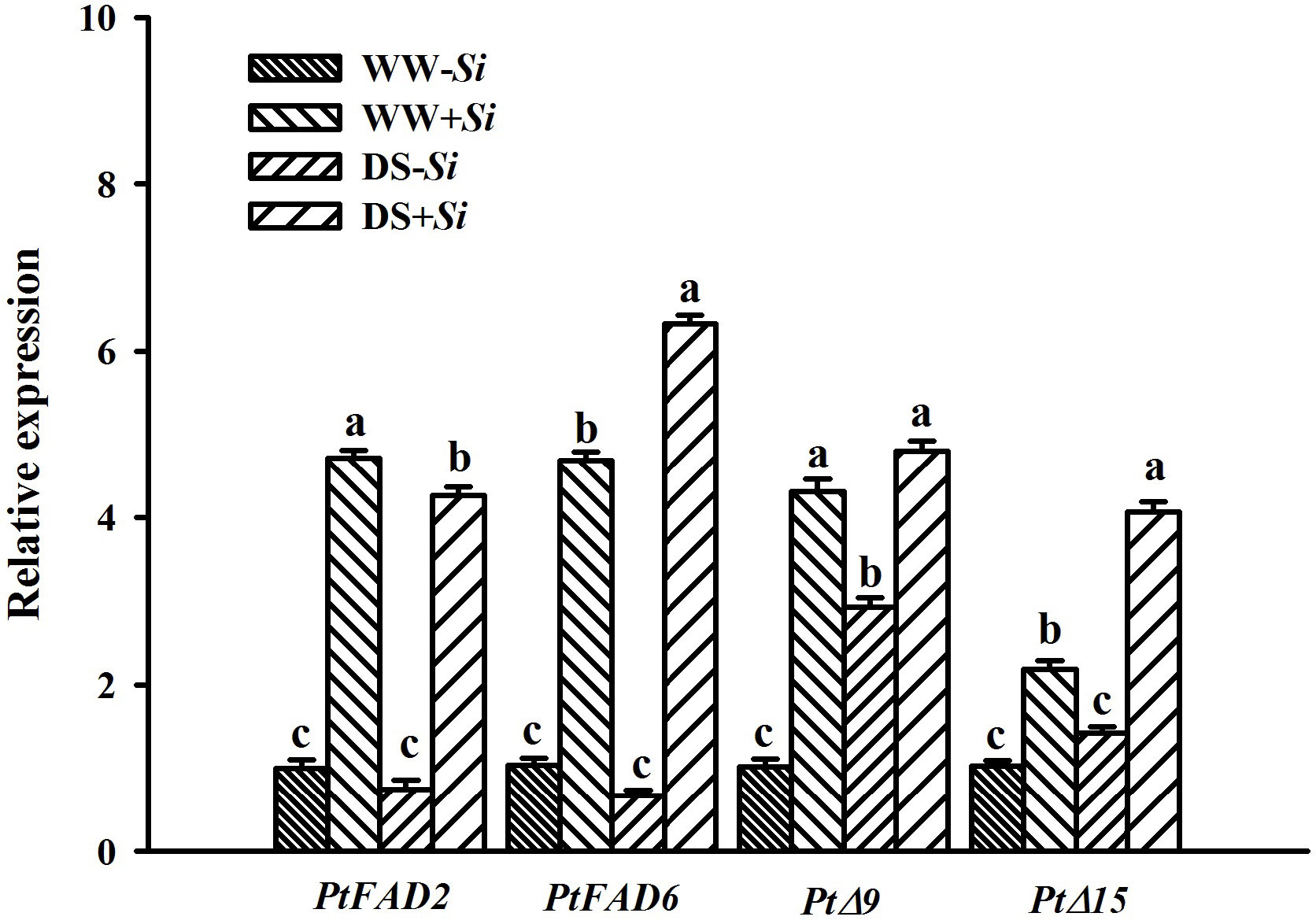
Figure 9 Effects of Serendipita indica (Si) on relative expression of four fatty acid desaturase genes in leaves of trifoliate orange seedlings under well-watered (WW) and drought stress (DS) conditions. Data (means ± SD, n = 3) followed by different letters upon the bars indicate significant (p< 0.05) differences.
Discussion
In this study, root colonization frequency of Si in trifoliate orange seedlings was significantly increased under DS versus WW conditions, which is consistent with Si-colonized white clover under DS (Rong et al., 2022). Si was isolated from arid zones and is therefore well adapted to drought (Boorboori and Zhang, 2022). It has been shown that Si preferentially colonized the root-hair zone, and the colonization frequency of Si increased with root senescence (e.g., under drought conditions) (Schäfer and Kogel, 2009). Nevertheless, a decrease in root Si colonization was observed in wheat plants under DS versus WW conditions (Yaghoubian et al., 2014). In Eleusin coracana plants, DS also induced the decrease in root Si colonization (Tyagi et al., 2017). In maize, root Si colonization was not distinctly affected by DS (Xu et al., 2017). This suggests that the response of root Si colonization to DS is variable.
Plants change root architecture in response to DS, with reduced lateral root density and allocating more nutrients to old roots (Lynch, 2018). Soil drought can strongly inhibit crop growth (Wahab et al., 2022). The present study also observed a decrease in plant growth variables under DS versus WW conditions, regardless of Si inoculation or not. However, Si-inoculated trifoliate orange seedlings represented greater plant growth performance and root surface area and volume, regardless of WW and DS. Similar results were reported in barley and wheat inoculated with Si under DS conditions (Hosseini et al., 2017; Ghaffari et al., 2019). Such changes may be linked to the fact that Si could promote the auxin and cytokinin synthesis of host plants (Liu et al., 2023; Rong et al., 2023).
Leaf gas exchange is closely linked to growth responses (Wu et al., 2019b). In the present study, DS treatment significantly reduced Pn, WUE, and Nbi in leaves of no-Si-inoculated seedlings, and Pn, Tr, Gs, Chi, and Nbi in Si-inoculated seedlings, compared with WW treatment. Interestingly, DS significantly raised Tr and Gs in no-Si-inoculated seedlings compared with WW treatment. This may be explained by the fact that prolonged DS irreversibly damages leaf tissues of no-Si-inoculated plants, thus accelerating Tr and Gs and leaving them in a more drought state (Yang et al., 2021b). In addition, Si application considerably raised Pn, Gs, Tr, Chi, and Nbi in WW-treated seedlings and Pn, Chi, Nbi, and WUE in DS-treated seedlings, compared with no-Si-inoculated treatment. This showed a significant improvement of WUE in Si-inoculated seedlings only under DS conditions, which was related to the involvement of mycelium of Si in water uptake. On the other hand, the Si inoculation also enhanced Pn by promoting chlorophyll formation, accompanied by an enhancement of Nbi. Under DS, Si-inoculated rice plants also exhibited similar results (Saddique et al., 2018). In Eleusine coracana plants, Si inoculation distinctly raised chlorophyll levels under DS (Tyagi et al., 2017). These increases under both Si inoculation and DS conditions are associated with Si-promoted P uptake and photosystem II efficiency (Tariq et al., 2017). Li et al. (2021) also observed the raised Chi level in Ipomoea batatas plants after Si inoculation. Proteomics analysis showed that Si inoculation on barley led to significant upregulation of various photosynthesis-related protein levels under DS, including photosystem complex proteins and photorespiratory enzymes (Ghaffari et al., 2019).
In the present study, ROS levels were induced to increase, and MDA was elevated in Si- and no-Si-inoculated trifoliate orange seedlings under DS versus WW conditions, indicating that the drought triggered oxidative damage in trifoliate orange seedlings. Furthermore, inoculation with Si was able to significantly reduce leaf H2O2 and levels as well as MDA concentrations, accompanied by a higher decrease under DS conditions than under WW conditions. Similar result was reported in maize plants under DS after Si inoculation (Kaboosi et al., 2023). MDA is a by-product of membrane lipid damage under DS (Pavlović et al., 2018). Sun et al. (2010) found that MDA levels in leaves of Si-colonized B. campestris plants in response to DS were delayed, coupled with the upregulation of antioxidant enzyme activities within 24 h. Si inoculation under DS also triggered a decrease in leaf ROS and MDA contents in Triticum aestivum and Solanum melongena (Yaghoubian et al., 2014; Swetha and Padmavathi, 2020). Therefore, Si-inoculated plants recorded lower oxidative burst and oxidative damage under drought, showing their enhanced drought tolerance. Nevertheless, Si colonization in walnut plants dramatically decreased MDA levels in leaves, but not roots under DS, suggesting a tissue dependency (Liu et al., 2021).
In plants, the AsA-GSH cycle, mediated by GR and APX, is associated with H2O2 scavenging (Irshad et al., 2021). Our study indicated that drought treatment markedly reduced leaf AsA and GSH levels, whereas inoculation with Si significantly increased leaf AsA and GSH levels, regardless of soil moisture regimes. Meanwhile, Si-inoculated seedlings also maintained a high APX activity under drought and a higher APX and GR activity under WW than no-Si-inoculated seedlings. This means that Si-inoculated plants have a more efficient AsA-GSH cycle to scavenge ROS under DS, which is in agreement with the results obtained by Rong et al. (2022) inoculating Si on white clover under DS. In A. thaliana, Si inoculation responded to DS by enhancing the AsA-GSH cycle pathway in plants (Sun et al., 2010). Under salt stress conditions, Si also provided tomato plants with a superior AsA-GSH cycle to eliminate ROS (Ghorbani et al., 2018), suggesting that Si plays an important role in modulating the AsA-GSH cycle under adversity.
This study also represented enhanced CAT and POD activities after Si inoculation under WW and DS. It has been demonstrated that Si colonization raised antioxidant enzyme activities in host plants including CAT and POD (Lin et al., 2019). Under drought, Si inoculation also enhanced the CAT activity of I. batatas (Li et al., 2021). Wheat inoculated with Si exhibited lower levels of lipid peroxidation as well as higher CAT and APX activities under DS (Yaghoubian et al., 2014). Si inoculation, on the other hand, reduced CAT activity of drought-stressed wheat and APX activity of drought-stressed wheat and maize (Hosseini et al., 2017; Hosseini et al., 2018). This indicated that Si effects on antioxidant enzyme activities are variable. Alternatively, Si inoculation activates drought-escape mechanisms in host plants, thereby doing not require enhanced antioxidant enzyme activities in response to drought (Jangir et al., 2021). In follow-up studies, we should explore how the Si activates the signaling pathway of antioxidant enzyme system in host plants subjected to DS.
Inoculation with Si also altered the expression of genes encoding antioxidant enzymes and fatty acid desaturases under DS, with increased expression in leaf PtFe-SOD, PtCu/Zn-SOD, PtPOD, PtCAT1, PtFAD2, PtFAD6, PtΔ9, and PtΔ15 genes. Similarly, inoculation of Rhizophagus irregularis upregulated leaf PpGR, PpMn-SOD, and PpCu/Zn-SOD expression of Robinia pseudoacacia plants under 200 mM NaCl conditions, but not 100 mM NaCl (Chen et al., 2020). Wu et al. (2019a) also reported that Funneliformis mosseae inoculation upregulated root PtFAD2, PtFAD6, and PtΔ9 gene expression in trifoliate orange under DS. In field citrus, Si inoculation also upregulated the expression of CsPOD, CsCAT1, and CsFAD6 in leaves (Li et al., 2022). This suggests that even in the absence of abiotic stress, Si can activate the expression of antioxidant defense genes in host plants. In leaves of B. campestris and maize, Si inoculation also upregulated the expression of stressed genes (DREB2A, CBL1, ANAC072, and RD29A) under soil drought (Sun et al., 2010; Xu et al., 2017). In Gerbera jamesonii seedlings, Si inoculation also triggered the upregulated expression of NHX2 and SOS1 under salt stress (Chen et al., 2022). Sun et al. (2010) proposed that the Ca2+ sensing regulatory protein could activate Si to induce drought-responsive gene expression. However, whether this case occurred in this study remains to be verified.
Conclusions
In summary, Si inoculation alleviated the inhibitory effect of soil drought on growth, Pn, WUE, and Chi of trifoliate orange seedlings, as well as the oxidative damage. This study firstly reported that low oxidative burst in Si-inoculated seedlings exposed to soil drought was associated with increased antioxidant enzyme activities and antioxidant levels, as well as upregulated expression of genes encoding antioxidant enzymes and fatty acid desaturases. Si has a high potential as a biostimulator for enhanced plant drought tolerance.
Data availability statement
The original contributions presented in the study are included in the article/Supplementary Material. Further inquiries can be directed to the corresponding author.
Author contributions
Conceptualization, Q-SW and J-LC; data curation, J-LC and YW; methodology, J-LC. resources, Q-SW; supervision, Q-SW; writing—original draft, YW; writing—review and editing, AH, EA, and Q-SW. All authors contributed to the article and approved the submitted version.
Funding
This study was supported by the Plan in Scientific and Technological Innovation Team of Outstanding Young Scientists, Hubei Provincial Department of Education (T201604). The authors would like to extend their sincere appreciation to the Researchers Supporting Project Number (RSP2023R356), King Saud University, Riyadh, Saudi Arabia.
Acknowledgments
The authors would like to extend their sincere appreciation to the Researchers Supporting Project Number (RSP2023R356), King Saud University, Riyadh, Saudi Arabia.
Conflict of interest
The authors declare that the research was conducted in the absence of any commercial or financial relationships that could be construed as a potential conflict of interest.
Publisher’s note
All claims expressed in this article are solely those of the authors and do not necessarily represent those of their affiliated organizations, or those of the publisher, the editors and the reviewers. Any product that may be evaluated in this article, or claim that may be made by its manufacturer, is not guaranteed or endorsed by the publisher.
Supplementary material
The Supplementary Material for this article can be found online at: https://www.frontiersin.org/articles/10.3389/fpls.2023.1247342/full#supplementary-material
References
Addi, M., Elbouzidi, A., Abid, M., Tungmunnithum, D., Elamrani, A., Hano, C. (2022). An overview of bioactive flavonoids from Citrus fruits. Appl. Sci. 12, 29. doi: 10.3390/app12010029
Ahluwalia, O., Singh, P. C., Bhatia, R. (2021). A review on drought stress in plants: Implications, mitigation and the role of plant growth promoting rhizobacteria. Res. Environ. Sustain. 5, 100032. doi: 10.1016/j.resenv.2021.100032
Boorboori, M. R., Zhang, H. Y. (2022). The role of Serendipita indica (Piriformospora indica) in improving plant resistance to drought and salinity stresses. Biology 11, 952. doi: 10.3390/biology11070952
Cao, J. L., He, W. X., Zou, Y. N., Wu, Q. S. (2023). An endophytic fungus, Piriformospora indica, enhances drought tolerance of trifoliate orange by modulating the antioxidant defense system and composition of fatty acids. Tree Physiol. 43, 452–466. doi: 10.1093/treephys/tpac126
Chance, B., Maehly, A. C. (1955). Assay of catalases and peroxidases. Method Enzymol. 2, 773–775. doi: 10.1016/S0076-6879(55)02300-8
Chen, W., Lin, F., Lin, K. H., Chen, C. M., Xia, C. S., Liao, Q. L., et al. (2022). Growth promotion and salt-tolerance improvement of Gerbera jamesonii by root colonization of Piriformospora indica. J. Plant Growth Regul. 41, 1219–1228. doi: 10.1007/s00344-021-10385-4
Chen, J. X., Wang, X. F. (2002). The Guidance of Plant Physiology Experiments (Guangzhou, China: South China University of Technology Press).
Chen, J., Zhang, H. Q., Zhang, X. L., Tang, M. (2020). Arbuscular mycorrhizal symbiosis mitigates oxidative injury in black locust under salt stress through modulating antioxidant defence of the plant. Environ. Exp. Bot. 175, 104034. doi: 10.1016/j.envexpbot.2020.104034
Cheng, H. Q., Zou, Y. N., Wu, Q. S., Kuča, K. (2021). Arbuscular mycorrhizal fungi alleviate drought stress in trifoliate orange by regulating H+-ATPase activity and gene expression. Front. Plant Sci. 12. doi: 10.3389/fpls.2021.659694
Ghaffari, M. R., Mirzaei, M., Ghabooli, M., Khatabi, B., Wu, Y. Q., Zabet-Moghaddam, M., et al. (2019). Root endophytic fungus Piriformospora indica improves drought stress adaptation in barley by metabolic and proteomic reprogramming. Environ. Exp. Bot. 157, 197–210. doi: 10.1016/j.envexpbot.2018.10.002
Ghorbani, A., Razavi, S., Omran, V. G., Pirdashti, H. (2018). Piriformospora indica alleviates salinity by boosting redox poise and antioxidative potential of tomato. Russ. J. Plant Physiol. 65, 898–907. doi: 10.1134/S1021443718060079
He, J. D., Zou, Y. N., Wu, Q. S., Kuča, K. (2020). Mycorrhizas enhance drought tolerance of trifoliate orange by enhancing activities and gene expression of antioxidant enzymes. Sci. Hortic. 262, 108745. doi: 10.1016/j.scienta.2019.108745
Hosseini, F., Mosaddeghi, M. R., Dexter, A. R. (2017). Effect of the fungus Piriformospora indica on physiological characteristics and root morphology of wheat under combined drought and mechanical stresses. Plant Physiol. Biochem. 118, 107–120. doi: 10.1016/j.plaphy.2017.06.005
Hosseini, F., Mosaddeghi, M. R., Dexter, A. R., Sepehri, M. (2018). Maize water status and physiological traits as affected by root endophytic fungus Piriformospora indica under combined drought and mechanical stresses. Planta 247, 1229–1245. doi: 10.1007/s00425-018-2861-6
Ilyas, M., Nisar, M., Khan, N., Hazrat, A., Khan, A. H., Hayat, K., et al. (2021). Drought tolerance strategies in plants: A mechanistic approach. J. Plant Growth Regul. 40, 926–944. doi: 10.1007/s00344-020-10174-5
Irshad, A., Rehman, R. N. U., Abrar, M. M., Saeed, Q., Sharif, R., Hu, T. (2021). Contribution of rhizobium–legume symbiosis in salt stress tolerance in Medicago truncatula evaluated through photosynthesis, antioxidant enzymes, and compatible solutes accumulation. Sustainability 13, 3369. doi: 10.3390/su13063369
Jangir, P., Shekhawat, P. K., Bishnoi, A., Ram, H., Soni, P. (2021). Role of Serendipita indica in enhancing drought tolerance in crops. Physiol. Mol. Plant P. 116, 101691. doi: 10.1016/j.pmpp.2021.101691
Kaboosi, E., Rahimi, A., Abdoli, M., Ghabooli, M. (2023). Comparison of Serendipita indica inoculums and a commercial biofertilizer effects on physiological characteristics and antioxidant capacity of maize under drought stress. J. Soil Sci. Plant Nutri. 23, 900–911. doi: 10.1007/s42729-022-01091-5
Li, Q., Kuo, Y. W., Lin, K. H., Huang, W., Deng, C., Yeh, K. W., et al. (2021). Piriformospora indica colonization increases the growth, development, and herbivory resistance of sweet potato (Ipomoea batatas L.). Plant Cell Rep. 40, 339–350. doi: 10.1007/s00299-020-02636-7
Li, Q. S., Xie, Y. C., Rahman, M. M., Hashem, A., Abd Allah, E. F., Wu, Q. S. (2022). Arbuscular mycorrhizal fungi and endophytic fungi activate leaf antioxidant defense system of lane late navel orange. J. Fungi 8, 282. doi: 10.3390/jof8030282
Lin, H. F., Xiong, J., Zhou, H. M., Chen, C. M., Lin, F. Z., Xu, X. M., et al. (2019). Growth promotion and disease resistance induced in Anthurium colonized by the beneficial root endophyte Piriformospora indica. BMC Plant Biol. 19, 40. doi: 10.1186/s12870-019-1649-6
Liu, X. Q., Cheng, S., Aroca, R., Zou, Y. N., Wu, Q. S. (2022). Arbuscular mycorrhizal fungi induce flavonoid synthesis for mitigating oxidative damage of trifoliate orange under water stress. Environ. Exp. Bot. 204, 105089. doi: 10.1016/j.envexpbot.2022.105089
Liu, B., Jing, D., Liu, F., Ma, H. L., Liu, X. H., Peng, L. (2021). Serendipita indica alleviates drought stress responses in walnut (Juglans regia L.) seedlings by stimulating osmotic adjustment and antioxidant defense system. Appl. Microbiol. Biot. 105, 8951–8968. doi: 10.1007/s00253-021-11653-9
Liu, R. C., Yang, L., Zou, Y. N., Wu, Q. S. (2023). Root-associated endophytic fungi modulate endogenous auxin and cytokinin levels to improve plant biomass and root morphology of trifoliate orange. Hortic. Plant J. 9, 463–472. doi: 10.1016/j.hpj.2022.08.009
Livak, K. J., Schmittgen, T. D. (2001). Analysis of relative gene expression data using real-time quantitative PCR and the 2–ΔΔCT method. Methods 25, 402–408. doi: 10.1006/meth.2001.1262
Lynch, J. P. (2018). Rightsizing root phenotypes for drought resistance. J. Exp. Bot. 69, 3279–3292. doi: 10.1093/jxb/ery048
Marulanda, A., Porcel, R., Barea, J. M., Azcón, R. (2007). Drought tolerance and antioxidant activities in lavender plants colonized by native drought-tolerant or drought-sensitive Glomus species. Microb. Ecol. 54, 543–552. doi: 10.1007/s00248-007-9237-y
Mensah, R. A., Li, D., Liu, F., Tian, N., Sun, X., Hao, X., et al. (2020). Versatile Piriformospora indica and its potential applications in horticultural crops. Hortic. Plant J. 6, 111–121. doi: 10.1016/j.hpj.2020.01.002
Miller, G., Suzuki, N., Ciftci-Yilmaz, S., Mittler, R. (2010). Reactive oxygen species homeostasis and signalling during drought and salinity stresses. Plant Cell Environ. 33, 453–467. doi: 10.1111/j.1365-3040.2009.02041
Mukarram, M., Choudhary, S., Kurjak, D., Petek, A., Khan, M. M. A. (2021). Drought: Sensing, signalling, effects and tolerance in higher plants. Physiol. Plant. 172, 1291–1300. doi: 10.1111/ppl.13423
Ortas, I. (2012). “Mycorrhiza in citrus: growth and nutrition,” in Advances in Citrus Nutrition. Ed. Srivastava, A. (Dordrecht: Springer), 333–351. doi: 10.1007/978-94-007-4171-3_23
Pavlović, I., Petřík, I., Tarkowská, D., Lepeduš, H., Bok, V. V., Brkanac, S. R., et al. (2018). Correlations between phytohormones and drought tolerance in selected Brassica crops: Chinese cabbage, white cabbage and kale. Int. J. Mol. Sci. 19, 2866. doi: 10.3390/ijms19102866
Phillips, J. M., Hayman, D. S. (1970). Improved procedures for clearing roots and staining parasitic and vesicular-arbuscular mycorrhizal fungi for rapid assessment of infection. Trans. Br. Mycol. Soc 55, 158–161. doi: 10.1016/s0007-1536(70)80110-3
Prasad, R., Sharma, M., Chatterjee, S., Chauhan, G., Tripathi, S., Das, A., et al. (2008). “Interactions of Piriformospora indica with medicinal plants,” in Mycorrhiza: State of the Art, Genetics and Molecular Biology, Eco-Function, Biotechnology, Eco-Physiology, Structure and Systematics. Ed. Varma, A. (Berlin, Heidelberg: Springer), 655–678. doi: 10.1007/978-3-540-78826-3_31
Rong, Z. Y., Jiang, D. J., Cao, J. L., Hashem, A., Abd Allah, E. F., Alsayed, M. F., et al. (2022). Endophytic fungus Serendipita indica accelerates ascorbate-glutathione cycle of white clover in response to water stress. Front. Microbiol. 13. doi: 10.3389/fmicb.2022.967851
Rong, Z. Y., Lei, A. Q., Wu, Q. S., Srivastava, A. K., Hashem, A., Abd Allah, E. F., et al. (2023). Serendipita indica promotes P acquisition and growth in tea seedlings under P deficit conditions by increasing cytokinins and indoleacetic acid and phosphate transporter gene expression. Front. Plant Sci. 14. doi: 10.3389/fpls.2023.1146182
Saddique, M. A. B., Ali, Z., Khan, A. S., Rana, I. A., Shamsi, I. H. (2018). Inoculation with the endophyte Piriformospora indica significantly affects mechanisms involved in osmotic stress in rice. Rice 11, 1–12. doi: 10.1186/s12284-018-0226-1
Schäfer, P., Kogel, K. H. (2009). “The sebacinoid fungus Piriformospora indica: an orchid mycorrhiza which may increase host plant reproduction and fitness,” in Plant Relationships. Ed. Deising, H. B. (Berlin Heidelberg: Springer-Verlag), 99–12. doi: 10.1007/978-3-540-87407-2
Sherameti, I., Tripathi, S., Varma, A., Oelmüller, R. (2008). The root-colonizing endophyte Pirifomospora indica confers drought tolerance in Arabidopsis by stimulating the expression of drought stress–related genes in leaves. Mol. Plant Microbe Interact. 21, 799–807. doi: 10.1094/MPMI-21-6-0799
Sudhakar, C., Lakshmi, A., Giridarakumar, S. (2001). Changes in the antioxidant enzyme efficacy in two high yielding genotypes of mulberry (Morus alba L.) under NaCl salinity. Plant Sci. 161, 613–619. doi: 10.1016/S0168-9452(01)00450-2
Sun, C., Johnson, J. M., Cai, D. G., Sherameti, I., Oelmüller, R., Lou, B. G. (2010). Piriformospora indica confers drought tolerance in Chinese cabbage leaves by stimulating antioxidant enzymes, the expression of drought-related genes and the plastid-localized CAS protein. J. Plant Physiol. 167, 1009–1017. doi: 10.1016/j.jplph.2010.02.013
Swetha, S., Padmavathi, T. (2020). Mitigation of drought stress by Piriformospora indica in Solanum melongena L. cultivars. Proc. Natl. Acad. Sci. India Sect. B Biol. Sci. 90, 585–593. doi: 10.1007/s40011-019-01128-3
Tariq, A., Pan, K., Olatunji, O. A., Graciano, C., Li, Z., Sun, F., et al. (2017). Phosphorous application improves drought tolerance of Phoebe zhennan. Front. Plant Sci. 8. doi: 10.3389/fpls.2017.01561
Tyagi, J., Chaudhary, P., Jyotsana, U. B., Bhandari, G., Chaudhary, A. (2022b). “Impact of endophytic fungi in biotic stress management,” in Plant Protection: From Chemicals to Biologicals. Eds. Soni, R., Suyal, D. C., Goel, R. (Berlin, Germany: Walter de Gruyter GmbH & Co KG.), 447–462. doi: 10.1515/9783110771558-017
Tyagi, J., Chaudhary, P., Mishra, A., Khatwani, M., Dey, S., Varma, A. (2022a). Role of endophytes in abiotic stress tolerance: with special emphasis on Serendipita indica. Int. J. Environ. Res. 16, 62. doi: 10.1007/s41742-022-00439-0
Tyagi, J., Varma, A., Pudake, R. N. (2017). Evaluation of comparative effects of arbuscular mycorrhiza (Rhizophagus intraradices) and endophyte (Piriformospora indica) association with finger millet (Eleusine coracana) under drought stress. Eur. J. Soil Biol. 81, 1–10. doi: 10.1016/j.ejsobi.2017.05.007
Varma, A., Bakshi, M., Lou, B., Hartmann, A., Oelmueller, R. (2012). Piriformospora indica: a novel plant growth-promoting mycorrhizal fungus. Agric. Res. 1, 117–131. doi: 10.1007/s40003-012-00195
Velikova, V., Yordanov, I., Edreva, A. (2000). Oxidative stress and some antioxidant systems in acid rain-treated bean plants: protective role of exogenous polyamines. Plant Sci. 151, 59–66. doi: 10.1016/S0168-9452(99)00197-1
Verma, S., Varma, A., Rexer, K. H., Hassel, A., Kost, G., Sarbhoy, A., et al. (1998). Piriformospora indica, gen. et sp. nov., a new root-colonizing fungus. Mycologia 90, 896–903. doi: 10.1080/00275514.1998.12026983
Wahab, A., Abdi, G., Saleem, M. H., Ali, B., Ullah, S., Shah, W., et al. (2022). Plants’ physio-biochemical and phyto-hormonal responses to alleviate the adverse effects of drought stress: A comprehensive review. Plants 11, 1620. doi: 10.3390/plants11131620
Wang, Y., Zou, Y. N., Shu, B., Wu, Q. S. (2023). Deciphering molecular mechanisms regarding enhanced drought tolerance in plants by arbuscular mycorrhizal fungi. Sci. Hortic. 308, 111591. doi: 10.1016/j.scienta.2022.111591
Wu, Q. S. (2018). Experimental Guidelines in Plant Physiology (Beijing, China: China Agriculture Press).
Wu, A., Hammer, G. L., Doherty, A., Caemmerer, S. V., Farquhar, G. D. (2019b). Quantifying impacts of enhancing photosynthesis on crop yield. Nat. Plants 5, 380–388. doi: 10.1038/s41477-019-0398-8
Wu, Q. S., He, J. D., Srivastava, A. K., Zou, Y. N., Kuča, K. (2019a). Mycorrhiza enhance drought tolerance of citrus by altering root fatty acid compositions and their saturation levels. Tree Physiol. 39, 1149–1158. doi: 10.1093/treephys/tpz039
Xu, L., Wang, A., Wang, J., Wei, Q., Zhang, W. (2017). Piriformospora indica confers drought tolerance on Zea mays L. through enhancement of antioxidant activity and expression of drought-related genes. Crop J. 5, 251–258. doi: 10.1016/j.cj.2016.10.002
Yaghoubian, Y., Goltapeh, E. M., Pirdashti, H., Esfandiari, E., Feiziasl, V., Dolatabadi, H. K., et al. (2014). Effect of Glomus mosseae and Piriformospora indica on growth and antioxidant defense responses of wheat plants under drought stress. Agric. Res. 3, 239–245. doi: 10.1007/s40003-014-0114-x
Yang, L., Zou, Y. N., Tian, Z. H., Wu, Q. S., Kuča, K. (2021a). Effects of beneficial endophytic fungal inoculants on plant growth and nutrient absorption of trifoliate orange seedlings. Sci. Hortic. 277, 109815. doi: 10.1016/j.scienta.2020.109815
Yang, X., Lu, M., Wang, Y., Wang, Y., Liu, Z., Chen, S. (2021b). Response mechanism of plants to drought stress. Horticulturae 7 (3), 50. doi: 10.3390/horticulturae7030050
Keywords: antioxidation, citrus, endophytic fungus, gas exchange, oxidative damage
Citation: Wang Y, Cao J-L, Hashem A, Abd_Allah EF and Wu Q-S (2023) Serendipita indica mitigates drought-triggered oxidative burst in trifoliate orange by stimulating antioxidant defense systems. Front. Plant Sci. 14:1247342. doi: 10.3389/fpls.2023.1247342
Received: 25 June 2023; Accepted: 15 September 2023;
Published: 04 October 2023.
Edited by:
Mamoona Rauf, Abdul Wali Khan University Mardan, PakistanReviewed by:
Juan B. Arellano, Spanish National Research Council (CSIC), SpainParul Chaudhary, Graphic Era Hill University, India
Copyright © 2023 Wang, Cao, Hashem, Abd_Allah and Wu. This is an open-access article distributed under the terms of the Creative Commons Attribution License (CC BY). The use, distribution or reproduction in other forums is permitted, provided the original author(s) and the copyright owner(s) are credited and that the original publication in this journal is cited, in accordance with accepted academic practice. No use, distribution or reproduction is permitted which does not comply with these terms.
*Correspondence: Qiang-Sheng Wu, d3VxaWFuZ3NoQDE2My5jb20=