- 1Crop Improvement Section, Indian Council of Agricultural Research (ICAR)-Directorate of Onion and Garlic Research, Pune, Maharashtra, India
- 2Genome Informatics Facility, Office of Biotechnology, Iowa State University, Ames, IA, United States
Cuticular wax is a characteristic feature of land plants that provides protection against both biotic and abiotic stresses. In this study, a glossy mutant lacking an epicuticular wax layer was identified in the γ-irradiated M2 mutant population of the onion cultivar Bhima Super. The inheritance of the mutant’s glossy phenotype was determined to be recessive and single locus. Scanning electron microscopy analysis showed poor accumulation of wax crystals in the glossy mutant, concentrated near the stomata. The plant height, number of leaves per plant, and stomatal parameters of the mutant were similar to the wild-type. RNA-seq was used to comprehend the expression variations of waxy cuticle-related genes in the glossy mutant and its wild-type waxy cultivars. Differential gene expression analysis of the RNA-seq data revealed that the genes involved in wax biosynthesis, such as AcCER1, AcCER26, AcMAH1, and AcWSD1, were downregulated by 2.72, 1.74, 2.59 and 2.12-fold, respectively, in the glossy mutant respectively. The expression patterns of these four unigenes were validated using semi-quantitative RT-PCR. The glossy mutant displayed a substantial 3.5-fold reduction in cuticular wax load compared to the wild-type due to the significant downregulation of these wax biosynthesis genes. These findings represent early advancements in understanding the molecular mechanisms of wax biosynthesis in onions. Furthermore, they provide a foundation for utilizing the glossy mutant trait in breeding programmes to enhance stress and pest resilience.
Introduction
Onion is an important vegetable crop and is preferred for its flavor profile. India is the second-largest contributor to onion production in the world, with a substantial output of 26,738,000 metric tons. Moreover, India ranks among the top consumers and exporters of onions on a global scale (FAOSTAT, 2021). The leaves of onions display characteristic white wax deposits on their surfaces.
Cuticular wax is an important adaptation of terrestrial plants, forming a protective outer layer on plant surfaces (Edwards et al., 1996; Yeats and Rose, 2013). This layer helps to maintain the water balance of plants by regulating non-stomatal water loss and provides a barrier against a range of biotic and abiotic stresses, including drought, cold, UV radiation, pests and pathogens. The composition of cuticular wax varies between species, as well as within a species between different organs, developmental stages and tissue types (Yeats and Rose, 2013). In addition, environmental factors such as light, drought, humidity temperature can also influence the distribution of cuticular wax within a species. The distribution and chemical composition of cuticular wax can affect a plant’s ability to tolerate biotic and abiotic stress (Yeats and Rose, 2013; Serrano et al., 2014; Singh et al., 2018), making it an important trait for breeders to consider when developing crops with improved stress tolerance. The crystal structure of cuticular wax also varies based on its composition and can impact the survival, feeding and oviposition of insects. The wax composition or wax load on the leaf surface has been shown to be important in determining plant tolerance to thrips in onion. Leaf wax composition analysis shows that Hentriacontanone-16 is a major component of cuticular wax that determines the total wax load in leek and onion leaves (Rhee et al., 1998; Munaiz et al., 2020). The wax related phenotype is mainly determined by the quantitative variation in accumulation of hentriacontanone-16 in visually different phenotypes of onion such as waxy, semi-glossy and glossy (Munaiz et al., 2020). Genetic analysis of naturally occurring mutants exhibiting a glossy phenotype in BianGan Welsh onion (Yang et al., 2017) and in the onion varieties ‘Australian Brown’ and ‘White Persian’ (Jones et al., 1944; Munaiz and Havey, 2020) has shown that this trait is controlled by a recessive allele and is determined by different loci.
Cuticular wax is composed of a complex mixture of very long chain of fatty acids (VLCFAs) and their derivatives, such as aldehydes, alkanes, ketones, fatty alcohol and even cyclic compounds (Yeats and Rose, 2013). The fatty acids are synthesized in the chloroplast and exported to the endoplasmic reticulum for elongation (Block and Jouhet, 2015). Further, the cuticular wax are synthesized in epidermal cells and deposited on the surface. The biosynthesis of cuticular wax is a complex process that involves the coordination of several genes. These cuticular wax biosynthesis genes have been identified in several plants, including maize, rapeseed, tomato and model plants such as Arabidopsis and rice. Eceriferum 6 (CER6), Eceriferum 10 (CER10), Glossy 8A (GL8A), Glossy 8B (GL8B), Formate dehydrogenase (FDH), Fatty Acid Elongation1 (FAE1), 3-ketoacyl-CoA synthase1 (KCS1) and Protein-tyrosine phosphatase 2 (PAS2) genes then elongate these C16 and C18 CoA esters to form VLCFAs precursors mediated through long-chain acyl-CoA synthetase (LACS) enzymes. KCS, KCR, HACD, and CER26, which are endoplasmic reticulum-associated fatty acid elongases, work sequentially to synthesize fully saturated long chains acyl-CoA. These acyl-CoAs then enter either the decarbonylation pathway or the acyl reduction pathway. The decarbonylation pathway involves the conversion of long chain fatty acyl-CoAs into aldehydes, alkanes, secondary alcohols and ketones through the action of FAR, CER1, CER4, and MAH1 genes. The acyl reduction pathway, which includes FAR, CER4, and WSD1 genes, converts fully saturated long chain acyl-CoAs into primary alcohols and wax esters. These waxes are transported to plasma membrane by an unknown mechanism and subsequently into the cell wall by ABC transporters (CER5 and ABCG). Further, transport of wax compounds across the cell wall is facilitated by lipid transfer proteins to the final destination at the cuticle (Li-Beisson et al., 2013).
In this study, we report a glossy mutant in onion developed through γ-irradiation mutagenesis. A comparative transcriptome analysis between the glossy mutant and its wild-type counterpart revealed significant differences in the wax biosynthesis pathway genes between the glossy mutant and the wild-type. These observations highlight the importance of comprehending the molecular mechanisms involved in wax biosynthesis in onions, as it holds great potential for enhancing crop quality and resilience to stress.
Materials and methods
Plant material
The present study was conducted during 2019-2022 at the Indian Council of Agricultural Research - Directorate of Onion and Garlic Research (ICAR-DOGR), situated at the latitude (27°19’00.2 N) and longitude (82°25’00.1E) Pune, Maharashtra, India 553.8 meters above sea level. Onion being a biennial crop, its seed production was done during rabi season and the subsequent generations were grown in kharif during 2019-2021. The glossy mutant phenotype was observed in the seed-germinated M2 population of γ-irradiation-treated Indian short-day onion cv. Bhima Super (B. Super) mutant line (IR-300-53). Bulbs of the M2 generation γ-irradiated onion (glossy type) and its non-mutagenized counterpart [wild-type (WT)/waxy], were planted in a greenhouse under a 16/8-h light/dark photoperiod at 25°C. The phenotypic data, including plant height and number of leaves per plant were recorded at 75 days after transplanting (DAT). The glossy mutants were both selfed and outcrossed with the wild-type. Seeds from the subsequent F1 and M3 generations were sown in the greenhouse. The F1 plants were backcrossed with the glossy mutant and the number of glossy and waxy phenotypes were recorded in the M3, F1 and BC1F1 generations. All plant studies were carried out in accordance with relevant institutional, national, and international guidelines and legislation.
Scanning electron microscopy
Onion leaves are isobilateral, having equal stomatal density on both sides of the leaf. The third leaf from the center of both the WT waxy and M3 mutant glossy plants were torn into 3×3 mm2 pieces using tweezers and mounted on aluminium stubs. The leaf specimens were then sputter-coated with gold and examined for the micro-structure analysis using an Environmental Scanning Electron Microscope (ESEM) (FEI, Quanta 200 and ESEM Mode) at Sophisticated Analytical Instrument Facility (SAIF) of Indian Institute of technology (Bombay, India). Electron micrographs of leaf surfaces from three replicate plants were taken for each line and used to visually estimate the shape of the epicuticular waxes and cuticular striation. The stomatal count was measured using the IMAGEJ software v. 1.43u. Stomatal index was calculated using the formula, Stomatal index (%) = (S/S+E) × 100; where, S and E are the number of stomata and epidermal cells, respectively; in the microscopic field of view (FOV).
Epicuticular wax coverage
The epicuticular wax content was extracted using the colorimetric method as described by Ebercon et al. (1977). Intact onion leaves were immersed in 15 ml distilled chloroform for 10 s and kept in a boiling water bath until the smell of chloroform was no longer detected. A 5 ml wax reagent (K2Cr2O7 prepared in conc. H2SO4) was added to each tube and kept at 60°C in a water bath for 30 min. After cooling, 12 ml of deionized water was added to each tube, and the solution was filtered through a filter paper. The absorbance was measured at a wavelength of 590 nm using SPECTROstar Nano microplate reader. The wax content was calculated using standard Polyethylene Glycol-3000 and expressed as mg per cm2.
RNA sequencing, de novo assembly and sequence clustering
Total RNA was isolated from 6-weeks-old leaves of wild-type and M3 generation glossy mutant plants in 2 biological replicates using TRIzol reagent. The mRNA was then isolated and subjected to quality control (QC) before being used for paired-end (PE) library preparation. The libraries were sequenced on the Illumina NovaSeq 6000 platform using 2×150 bp chemistry at Eurofins India Pvt. Ltd. (Bangalore, India). The generated raw sequence datasets were deposited in the NCBI in the Short Read Archive (SRA) database with Bioproject accession number PRJNA943431. The sequenced raw paired-end reads of all samples were processed to obtain clean and high-quality concordant reads using Trimmomatic v0.38 (Bolger et al., 2014) to remove adapters, ambiguous reads (reads with unknown nucleotides “N” larger than 5%), and low-quality sequences (reads with more than 10% quality threshold (QV) < 20 phred score. The resulting high-quality (QV>20) paired-end reads were used for de novo assembly using Trinity v2.8.4 (Henschel et al., 2012) with a kmer of 25, and the mapped high quality reads of all samples were pooled together for assembly into transcripts.
CDS prediction, functional annotation and gene ontology analysis
The assembled transcripts were then further clustered together using CD-HIT-EST-4.6 to get unigenes. Only those unigenes which were found to have >90% coverage at 3X read depth were considered for downstream analysis. To identify sample-wise CDS from the pooled set of CDS, reads from each of the samples were mapped onto the final set of pooled CDS sequences using Bowtie mapper v2.4.4 (Langmead and Salzberg, 2012). TransDecoder v5.3.0 was used to predict coding sequences from the unigenes. Functional annotation of the CDS was performed using the DIAMOND program (Buchfink et al., 2015) with BLASTX alignment mode to find the homologous sequences for the genes against non-redundant protein database (NR) from NCBI. Gene ontology (GO) analyses of identified CDS of each sample were carried out using Blast2GO program (Conesa et al., 2005). GO assignments were used to classify the functions of the predicted CDS. GO mapping was used to group identified genes into three main domains: Biological Process (BP), Molecular Function (MF) and Cellular Component (CC). To identify the potential involvement of the predicted CDS in biological pathways, all the identified CDS for each of the samples were mapped to reference canonical pathways in KEGG (Eudicots database) using KEGG automated annotation server, KAAS (Moriya et al., 2007).
Differentially expressed gene analysis
The unigenes were used as the transcriptome reference for quantifying transcript abundance using Salmon version 1.8.0. A reference transcriptome index was created using the salmon “index” command. Transcript quantification was performed using the salmon “quant” command. The read counts generated was used for differential expression analysis with DESeq2 version 1.36.0 (Love et al., 2014). Genes that with absolute log fold change > 1 and adjusted p-value < 0.05 were determined to be differentially expressed geneS (Love et al., 2014; Patro et al., 2017).
Semi-quantitative RT-PCR analysis
Total RNA was isolated from 100 mg shoot tissues of M3 glossy mutant and wild-type B. Super plants (with 2 biological replicates of each) using RNeasy® Plant Mini kit (Qiagen). The RNA was quantified using Nanodrop (UV/Vis Nano spectrophotometer, Nabi, Metler Toledo). Total RNA (1 µg) was treated with DNase I, RNase free kit (Thermo Scientific) to remove any remaining DNA. First-strand cDNA synthesis was prepared using SuperScript™ IV Reverse Transcriptase kit (Invitrogen) as per manufacturer’s instructions and quantified using Nanodrop. First-strand cDNA (30 ng) was used for semi-quantitative RT-PCR analysis to validate the differentially expressed genes involved in the wax-biosynthesis pathway. Gene-specific primer sets were used, and the PCR reactions were performed according to the conditions described in Table S1. PCR products were run on 3% agarose gel and the image was captured with a digital camera under a UV light (Vilber Lourmat, ECX20.W). The band area was calculated using the ImageJ analyser tool and the band area of the wild-type was compared with the glossy mutant in terms of log2 fold change.
Statistical analysis
To evaluate the variation in physical parameters between the glossy mutant and wild-type (WT) plants, a one-way analysis of variance (ANOVA) was employed using Statistical Analysis Software, Version 9.2 (Sas Institute, 2009). Mean comparisons were performed using the least significant difference (LSD) approach at a significance level of P=0.05. Chi-square analysis was performed in the segregating populations to understand the segregation pattern of the glossy mutant.
Results
Identification, phenotypic variance and segregation of glossy mutants
Four glossy green mutant phenotypes were observed in the M2 population of γ-irradiation-treated onion mutant line (B. Super-IR-300-53) (Figure S1). Bulbs from the M2 glossy mutant phenotype were harvested and planted separately under greenhouse conditions. The average plant height was 49.5 ± 2.2 cm and 57.5 ± 4.8 cm, and the number of leaves per plant was 6.5 ± 0.6 and 6.75 + 0.9 in M2 glossy mutant and WT; respectively. No significant differences were found between the glossy mutant and wild-type plants, with respect to plant height and number of leaves per plant. M2 plants were selfed and the subsequent M3 generation was found to be glossy. F1 plants derived from the cross between M2 and WT exhibited the waxy phenotype. However, when F1 plants were backcrossed with M3 glossy mutant, the subsequent BC1F1 population segregated in a 1:1 ratio (waxy: glossy).
Leaf surface microstructure and morphology
The bright green leaf of glossy mutant was in stark contrast to the glaucous leaf of WT in the experimental field. The color variation of the onion foliage is directly correlated with the amount and type of epicuticular wax deposits. SEM micrographs (Figure 1A) revealed large quantities of spiky, needle-like microscopic wax crystals uniformly covering the leaf surface of waxy WT onion, while in the glossy type, relatively few wax crystals were scattered around the stomatal region alone (Figure 1B). The bright green leaf of glossy mutant (Figure 1D) was distinguishable from the waxy leaf of the wild-type onion (Figure 1C). The foliage of the mutant onion appears shiny green, and water droplets easily form and adhere to leaf surfaces after the leaves are sprayed with water (Figure 1F). In contrast, the leaves of wild-type are greenish-grey, due to higher wax deposits (Figure 1E). The glossy leaves of mutant onion showed a wet phenotype (hydrophilic) as water droplets easily adhered onto the surface and coalesced. However, wild-type onion was more hydrophobic as water droplets formed beads due to a dense layer of epicuticular wax. The number of stomata and stomatal index were measured in the glossy mutant and wild-type onions. The results revealed a significant difference between the two genotypes: the mutant had 247 stomata with a stomatal index of 49, while the wild-type had 225 stomata with a stomatal index of 45 (Figure 2).
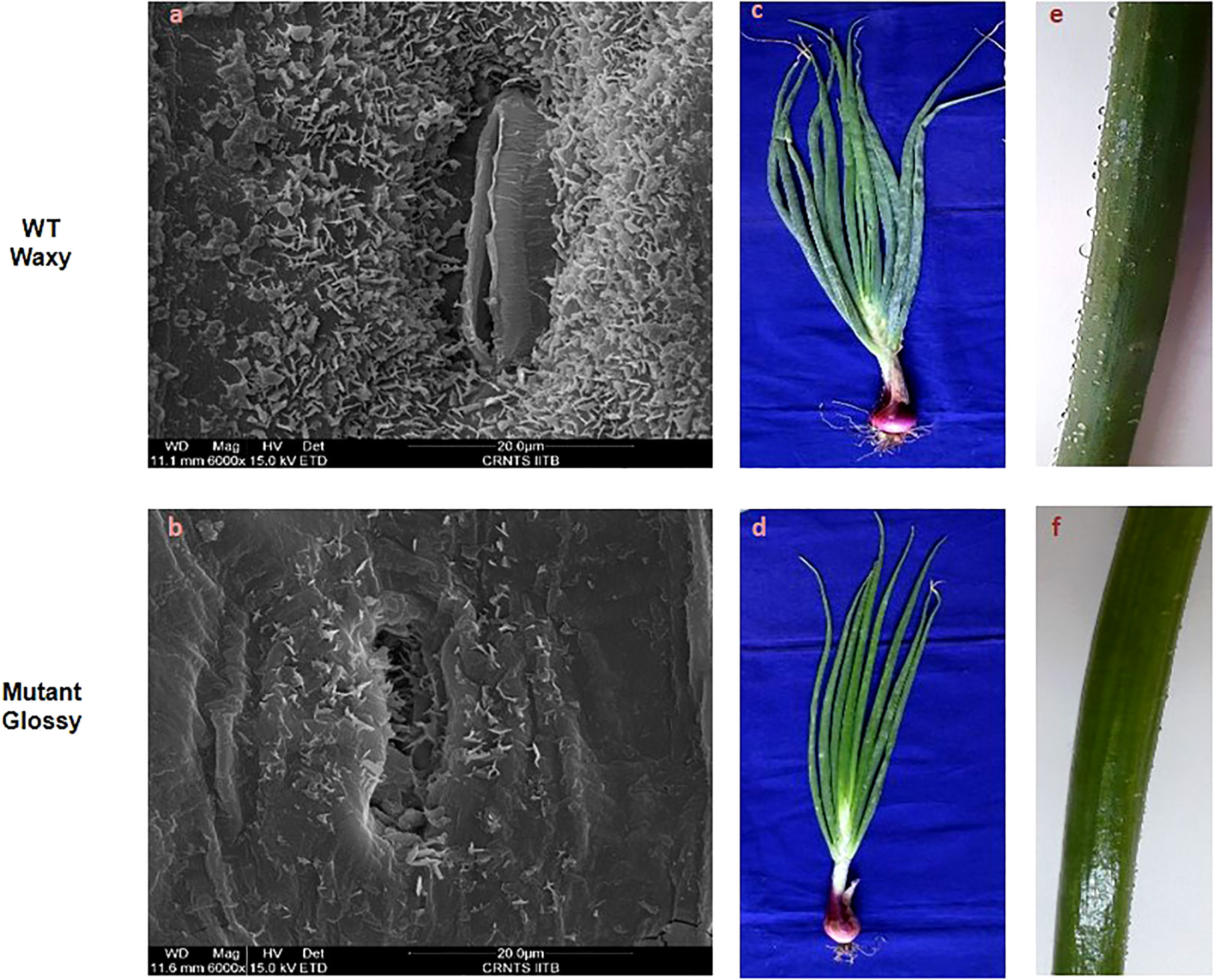
Figure 1 Leaf surface microstructure and morphology of WT and glossy mutant. Comparative scanning electron micrographs of leaf surface at magnification of 6000x of (A) WT waxy, (B) mutant glossy. Large quantities of spiky, needle-like crystals microscopic wax crystals were uniformly covered on the leaf surface of waxy WT onion, whereas in glossy type, relatively few wax crystals were scattered around the stomatal region alone. (C) Greenish gray (glaucous) phenotype of WT waxy, (D) Shiny glossy green phenotype of mutant glossy. (E) WT waxy onion leaf surface showing water droplets due to its hydrophobic nature, (F) water droplets were easily adhered and coalesced on to the leaf surface of mutant glossy.
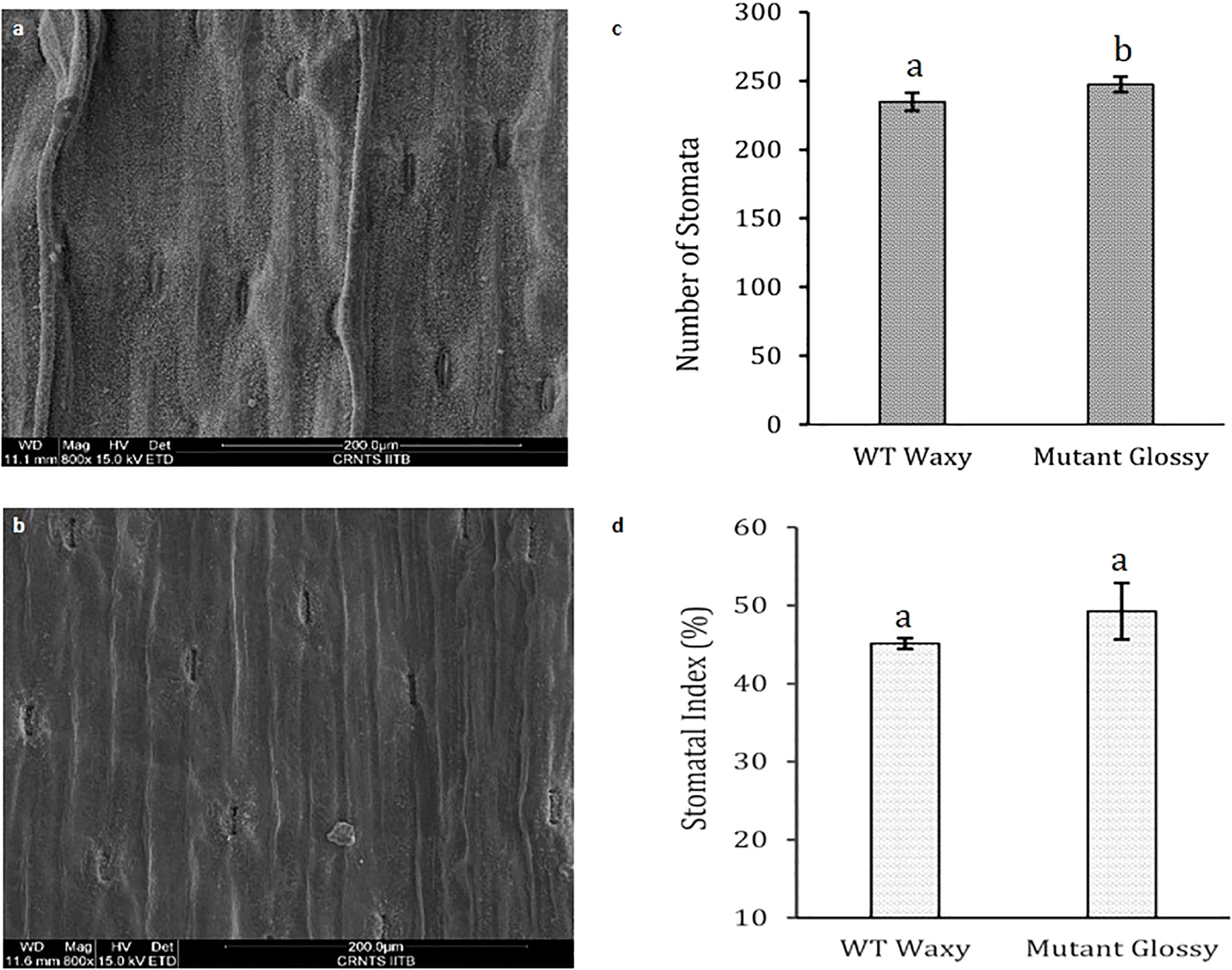
Figure 2 Leaf stomatal parameters in WT and glossy mutant. Comparative scanning electron micrographs of leaf surface at magnification of 800x of (A) WT waxy, (B) mutant glossy, (C) Number of stomata in 200 µm length in WT waxy and mutant glossy lines. (D) Stomatal index of WT waxy and mutant glossy lines. Stomatal index was calculated in percentage using formula, SI= (S/S+E) x 100 where, S and E are the number of stomata and epidermal cells respectively in 200 µm length microscopic view field. The bars indicate mean ± SE of 3 replicates. The lowercase letters "a and b" indicates the significant difference.
RNA-seq assembly and analysis
RNA sequencing of 6-week-old leaves of wild-type onion (C1 and C2) and its glossy mutant (G1 and G2) was performed in duplicate using the Illumina platform (NovaSeq6000) with 2 × 150 bp chemistry. After stringent quality assessment and data filtering, a total of 104.33 million paired-end were generated, corresponding to 30.26 Gb of sequence data. Details of high-quality reads, transcripts generated, unigenes identified, predicted CDSs, functional annotation and classification of predicted CDSs, identification of differentially expressed genes (DEGs) and pathway enrichment analysis are summarized in Supplement S1. Before identification of DEGs, the annotated CDs from two biological replicates of each glossy M3 mutant and wild-type were pooled together independently and expression of unigenes were normalized. A total of 596 annotated unigenes were found to be differentially expressed between wild-type and glossy mutant, with 295 upregulated and 301 downregulated genes in the mutant (Supplementary Sheet S2).
Profiling cuticular wax biosynthesis pathway genes
Out of 301 downregulated unigenes, 4 critical genes related to wax biosynthesis pathway i.e. AcMAH1, AcWSD1, AcCER1 and AcCER26 were significantly downregulated in the glossy mutant (Figure 3A). There was 1.74, 2.72, 2.12 and 2.59-fold decrease in the transcripts level of ECR26, ECR1, WSD1 and MAH1 genes, respectively (Table 1). To validate the RNA-seq results, expression levels of these genes were evaluated by semi-quantitative PCR, which also showed down-regulation of these genes in the glossy mutant (Figure 3B; Figure S2). Semi-quantitative PCR analysis had shown 1.17, 2.19, 1.61 and 1.95-fold down-regulation of ECR26, ECR1, WSD1 and MAH1 genes, respectively. The results of the semi-quantitative PCR were consistent with those of the RNA-seq analysis (Table 1).
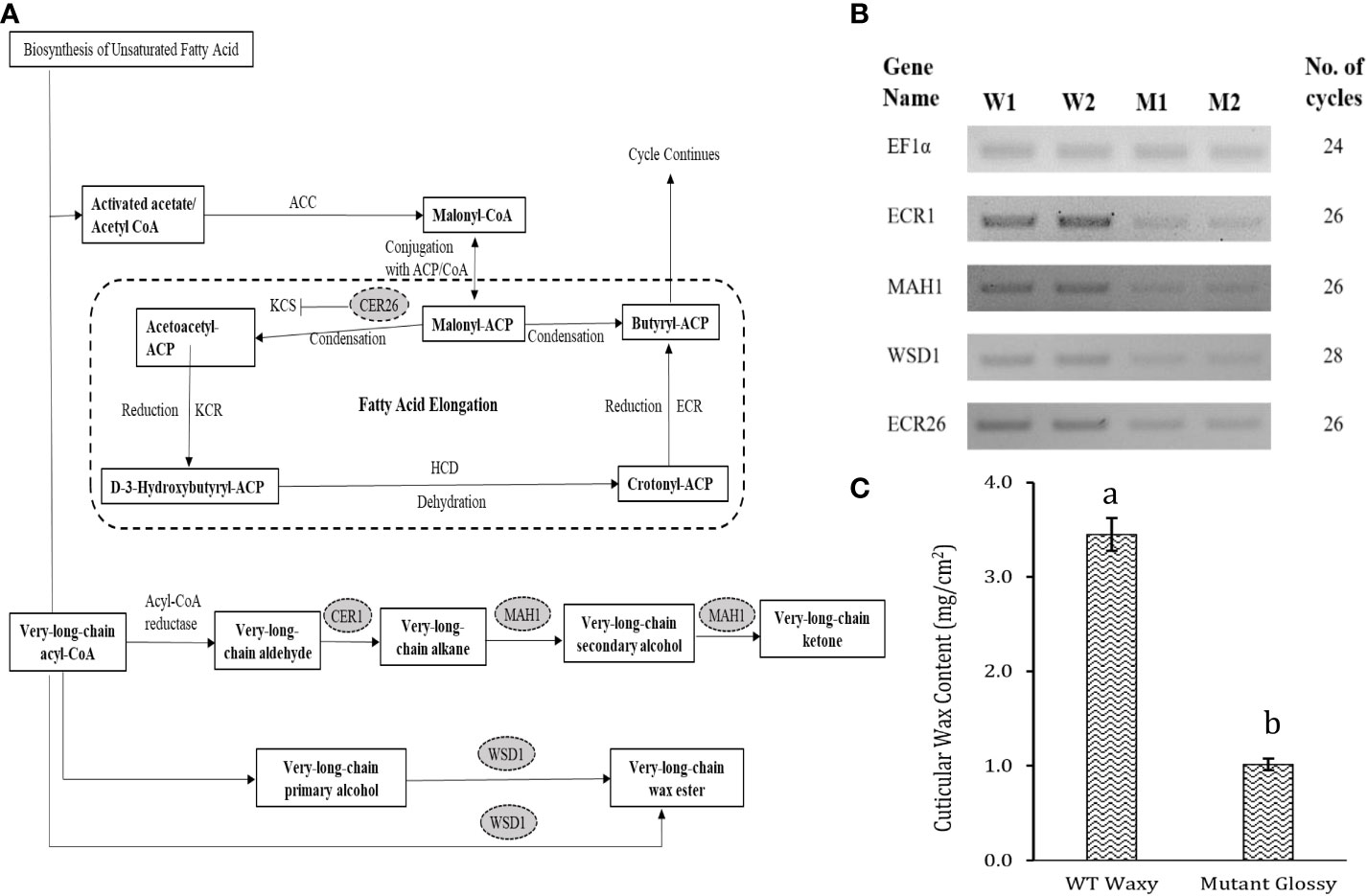
Figure 3 Downregulation of cuticular wax biosynthetic genes in the onion glossy mutant. (A) Basic cuticular wax biosynthetic pathway. (B) Oval highlighted genes were downregulated genes in glossy mutant onion lines by RNA-seq analysis. Question marks and dotted lines indicate unidentified enzymes and processes, respectively, (B) Validation of 4 downregulated genes of cuticular wax biosynthesis pathway by semi-quantitative RT-PCR. W1 and W2 indicates 2 biological replicates of WT waxy, whereas M1 and M2 indicates 2 biological replicates of mutant glossy. Total RNA was extracted from 6-week-old shoot tissues and the transcript levels were examined using semiquantitative RT-PCR. (C) Epicuticular wax content in WT waxy and mutant glossy lines. The bars indicate mean ± SE of 3 replicates. The lowercase letters "a and b" indicates the significant difference.
Discussion
Segregation pattern of glossy mutant
The F1 plants derived from the cross between M2 and WT exhibited a waxy phenotype, revealing no segregation and thereby indicating the possibility of a recessive mutation. When F1 lines were back-crossed with M3 glossy mutant lines, BC1F1 lines showed an equal mixture of waxy and glossy mutants (Table 2), which confirmed the single-locus recessive gene inheritance of the glossy mutant phenotype. In consistent with our results, several studies had also shown that glossy mutant trait is controlled by single recessive gene/locus in maize (Bianchi et al., 1979), cabbage (Zeng et al., 2017; Liu et al., 2018), Welsh onion (Yang et al., 2017), Barely (Luan et al., 2017) and onion (Munaiz and Havey, 2020).
Epiculticular wax coverage and morphology of glossy mutant
The glossy mutant onion from the present study exhibited a distinct bright green phenotype compared to the WT waxy onion. The type and quantity of epicuticular wax deposits influences leaf color and leaf wettability. Damon and Havey, 2014, had visually classified onions as ‘‘glossy’’ or ‘‘semi-glossy,’’ on the basis of low to intermediate amounts of epicuticular waxes. Glossy mutant plants showed significantly fewer wax crystals, mainly concentrated around the stomatal region, giving it a greenish-grey color in contrast to WT. The shape of these wax crystals may vary based on self-assembly as sheet, filiform, tubular, and granular. According to descriptions of epicuticular wax crystals by Jeffree (1986), the spiky crystals on onion foliage are likely formed by a ketone. Studies by Gülz et al. (1992) and Gülz et al. (1993) concluded that characteristic lipid crystals can be observed on leaves if one lipid class comprises at least 40% of the total profile. Therefore, the spiky, needle-like microscopic wax crystals that predominate onion foliage can possibly be ketone(s). The leaves of the glossy mutant exhibit a hydrophilic property, facilitating the easy adhesion and coalescence of water droplets on their surface. In contrast, the wild-type onion has a dense layer of epicuticular wax, rendering it more hydrophobic and causing water droplets to form beads on its surface. These findings are consistent with previous studies conducted on rice and Welsh onion, highlighting the role of epicuticular wax in influencing leaf color, hydrophobicity, and water droplet behavior (Qin et al., 2011; Yang et al., 2017). Our results are in accordance with Yang et al. (2017), where the glossy mutant and wild-type of Welsh onion showed no significant differences in stomatal density.
Cuticular wax biosynthesis genes
The cuticular wax biosynthesis and secretion pathway affects its content and composition. Studies on plant epicuticular wax biosynthesis have revealed ketones, including hentriacontanone-16, derived from the decarbonylation pathway involving aldehydes and alkanes as intermediates (Kunst and Samuels, 2003). Previous studies have provided a strong evidence that hentriacontanone-16 is the predominant epicuticular wax on onion (Damon et al., 2014) and leek (Rhee et al., 1998) foliage. The proteins MAH1 and CER1 are involved in the decarbonylation pathway, where CER1 converts long chain aldehydes to alkanes and MAH1 converts alkanes into secondary alcohols and ketones (Aarts et al., 1995; Greer et al., 2007). According to Jeffree (1986), the spikey wax crystals deposited on onion foliage is likely to be ketone which is in accordance with our results.
Mutations in the cer1 gene result in glossy green stems with decreased wax deposition in Arabidopsis, while mutations in the mah1 gene lead to glaucous inflorescence stems without affecting epicuticular wax crystals (Aarts et al., 1995; Greer et al., 2007). WSD1 participates in the acyl reduction pathway, and involved in synthesis of wax esters, a component of wax. Arabidopsis wsd1 mutant had significantly reduced wax ester levels in the stem wax (Li et al., 2008). Leaf surface wax biosynthesis was broadly affected in glossy mutant of B. napus due to suppression of CER1 and other wax-related genes (MAH1, WSD1, CAC3, FATB, ABCG28, etc.). Previous research has shown that upregulation of CER1, WSD1 and MAH1 genes triggered biosynthesis and deposition of cuticular wax on the leaves of EMS-mutagenized Dianthus spiculifolius plants in the M2 generation (Zhou et al., 2018). CER26 is involved in the elongation of the very long chain fatty acids of 30 carbons or more, and its mutation substantially decreased the amount of these very long chain fatty acids (Pascal et al., 2013). The mutation, however, did not affect the wax load on leaf or stem. In our study, we observed a significant difference in leaf cuticular wax content between the WT waxy and M3 glossy mutant lines. The wax load in the WT waxy was 3.5 ± 0.17 mg cm-2, whereas it was 1.0 ± 0.06 mg cm-2 in the glossy mutant lines (Figure 3C). Significant suppression of the four 4 critical wax biosynthesis genes (AcCER1, AcMAH1, AcWSD1 and AcCER26) in our glossy mutant may have disrupted or slowed down the acyl reduction and decarbonylation pathways of wax biosynthesis, resulting in 3.5 times lower cuticular wax load in the glossy mutant than the WT waxy.
Our research findings indicated that within the wax biosynthesis pathway, CER1 displayed the highest significant downregulation among the four downregulated genes. These results are consistent with the earlier study by Pu et al. (2013), which showed notable downregulation of CER1 (4.13-fold) and mild downregulation of other wax biosynthesis-related genes, including MAH1, WSD1, CAC3, FATB, ABCG28, and FAR (ranging from 1.38 to 1.80-fold), in the glossy mutant of Brassica napus. Another study conducted by Liu et al. (2014) also revealed downregulation of four wax biosynthesis pathway genes, namely LACS2, ECR3 (WAX2 isoform 1), ECR3 (Fatty acid hydroxylase superfamily), and ABCG12 (1.3 to 1.55-fold), with LACS2 exhibiting the most substantial downregulation (5-fold) in glossy mutant of Welsh onion. The variation in downregulated genes and their expression levels in glossy mutants might be influenced by the specific genotype and the regulatory gene that was prabably mutated. The glossy mutant has potential applications in breeding. For instance, the male sterile parent could act as a reliable indicator for eliminating rogue plants in hybrid seed production, as well as in genetic studies focused on wax biosynthesis and export. Compared to the waxy-type, the glossy phenotype of onion has been associated with significantly less epicuticular wax and has been found resistant to thrips infestation (Damon et al., 2014). The quantity of epicuticular wax deposition is directly to insect resistance in various crops such as Sorghum bicolor (Starks and Weibel, 1981), Triticum aestivum (Lowe et al., 1985), Brassica oleracea var. capitata (Voorrips et al., 2008) and Allium cepa (Damon et al., 2014). Additionally, novel genes associated with cuticular wax biosynthesis potentially serve as valuable genetic resources for enhancing drought resistance in crops (Xue et al., 2017). Considering these factors, we anticipate that our glossy mutant phenotype could offer a potential advantage in onion breeding, contributing to the development of lines with increased resistance to biotic and abiotic stressors.
Conclusion
The wax layer on the epidermal surface provides protection to terrestrial plants from UV radiation, water loss and gas exchange, and biotic stresses. In this study, we isolated a glossy mutant from the M2 population of γ-irradiated onion mutant lines in variety B. Super. The glossy trait is controlled by a recessive locus. The mutant accumulates wax poorly on the leaf surface, and we show that there is a concomitant downregulation of four structural genes in the wax biosynthesis pathway, namely AcCER1, AcCER26, AcWSD1, and AcMAH1, which potentially explains the reduction in wax accumulation. Single gene inheritance indicates that the mutation could be within a regulatory gene that controls these structural genes. Therefore, it would be worthwhile to map this regulatory locus using markers identified around this locus. Additionally, the glossy mutant can be a valuable addition to studies on both abiotic and biotic stress in plant breeding. We will conduct further investigation to determine the influence of cuticle thickness and the epicuticular waxes in conferring resistance to onion plants against various environmental stressors and pests.
Data availability statement
All data are included in the article. RNA-seq raw data is available at the NCBI database (https://www.ncbi.nlm.nih.gov/bioproject/PRJNA943431).
Author contributions
TM and SA conceived and designed the experiments. TM, SS, and YK conducted all the experiments. TM and SS drafted the manuscript. SA and VS carried out the data analysis and manuscript editing. SA supervised the entire work.
Funding
This research work was supported by a grant from Indian Council of Agricultural Research- ICAR National Fellow project (Project code: HORTDOGRSOL201700800088).
Acknowledgments
Authors are highly thankful to Dr. Major Singh, Former Director, ICAR-DOGR, Pune for his suggestions during the entire work.
Conflict of interest
The authors declare that the research was conducted in the absence of any commercial or financial relationships that could be construed as a potential conflict of interest.
Publisher’s note
All claims expressed in this article are solely those of the authors and do not necessarily represent those of their affiliated organizations, or those of the publisher, the editors and the reviewers. Any product that may be evaluated in this article, or claim that may be made by its manufacturer, is not guaranteed or endorsed by the publisher.
Supplementary material
The Supplementary Material for this article can be found online at: https://www.frontiersin.org/articles/10.3389/fpls.2023.1245308/full#supplementary-material
References
Aarts, M. G., Keijzer, C. J., Stiekema, W. J., Pereira, A. (1995). Molecular characterization of the CER1 gene of arabidopsis involved in epicuticular wax biosynthesis and pollen fertility. Plant Cell 7 (12), 2115–2127. doi: 10.1105/tpc.7.12.2115
Bianchi, G., Avato, P., Salamini, F. (1979). Glossy mutants of maize. Hered. 42 (3), 391–395. doi: 10.1038/hdy.1979.42
Block, M. A., Jouhet, J. (2015). Lipid trafficking at endoplasmic reticulum–chloroplast membrane contact sites. Curr. Opin. Cell Biol. 35, 21–29. doi: 10.1016/j.ceb.2015.03.004
Bolger, A. M., Lohse, M., Usadel, B. (2014). Trimmomatic: a flexible trimmer for Illumina sequence data. Bioinformatics 30 (15), 2114–2120. doi: 10.1093/bioinformatics/btu170
Buchfink, B., Xie, C., Huson, D. H. (2015). Fast and sensitive protein alignment using Diamond. Nat. Methods 12 (1), 59–60. doi: 10.1038/nmeth.3176
Conesa, A., Götz, S., García-Gómez, J. M., Terol, J., Talón, M., Robles, M. (2005). Blast2GO: a universal tool for annotation, visualization and analysis in functional genomics research. Bioinformatics 21 (18), 3674–3676. doi: 10.1093/bioinformatics/bti610
Damon, S. J., Groves, R. L., Havey, M. J. (2014). Variation for epicuticular waxes on onion foliage and impacts on numbers of onion thrips. J. Am. Soc. Hortic. Sci. 139 (4), 495–501. doi: 10.21273/JASHS.139.4.495
Damon, S. J., Havey, M. J. (2014). Quantitative trait loci controlling amounts and types of epicuticular waxes in onion. J. Am. Soc. Hortic. Sci. 139 (5), 597–602. doi: 10.21273/JASHS.139.5.597
Ebercon, A., Blum, A., Jordan, W. R. (1977). A rapid colorimetric method for epicuticular wax contest of sorghum leaves 1. Crop Sci. 17 (1), 179–180. doi: 10.2135/cropsci1977.0011183X001700010047x
Edwards, D., Abbott, G. D., Raven, J. A. (1996). “Cuticles of early land plants: a palaeoecophysiological evaluation,” in Plant Cuticles: An Integrated Functional Approach. Environmental Plant Biology. Ed. Kerstiens, G. (Oxford: BIOS Scientific Publishers), 1–31.
FAOSTAT (2021) Food and Agriculture Organization of the United Nations (Rome: FAO). Available at: https://www.fao.org/faostat/en/#data/QCL (Accessed 12-07-2023).
Greer, S., Wen, M., Bird, D., Wu, X., Samuels, L., Kunst, L., et al. (2007). The cytochrome P450 enzyme CYP96A15 is the midchain alkane hydroxylase responsible for formation of secondary alcohols and ketones in stem cuticular wax of Arabidopsis. Plant Physiol. 145 (3), 653–667. doi: 10.1104/pp.107.107300
Gülz, P. G., Müller, E., Herrmann, T., Lösel, P. (1993). Epicuticular leaf waxes of the hop (Humulus lupulus). chemical composition and surface structures. Z. fur Naturforsch. - Sect. C J. Biosci. 48 (9-10), 689–696. doi: 10.1515/znc-1993-9-1002
Gülz, P. G., Müller, E., Schmitz, K., Marner, F. J., Güth, S. (1992). Chemical composition and surface structures of epicuticular leaf waxes of Ginkgo biloba, Magnolia grandiflora and Liriodendron tulipifera. Z. fur Naturforsch. - Sect. C J. Biosci. 47 (7-8), 516–526. doi: 10.1515/znc-1992-7-805
Henschel, R., Nista, P. M., Lieber, M., Haas, B. J., Wu, L. S., LeDuc, R. D. (2012). “Trinity RNA-Seq assembler performance optimization,” in XSEDE'12 Proceedings of the 1st Conference of the Extreme Science and Engineering Discovery Environment: Bridging from the eXtreme to the campus and beyond. New York, United States: Association for Computing Machinery. doi: 10.1145/2335755.2335842
Jeffree, C. E. (1986). The cuticle, epicuticular waxes and trichomes of plants, with reference to their structure, functions and evolution. in Insects and the plant surface. Eds. B. E. Juniper and T. R. E. Southwood. (LondonA: Edward Arnold Publishers Ltd.), 23–64.
Jones, H. A., Clarke, A. E., Stevenson, F. J. (1944). Studies in the genetics of the onion (Allium cepa L.). Proc. Am. Soc Hortic. Sci. 44, 479–484.
Kunst, L., Samuels, A. L. (2003). Biosynthesis and secretion of plant cuticular wax. Prog. Lipid Res. 42(1), 51–80. doi: 10.1016/S0163-7827(02)00045-0
Langmead, B., Salzberg, S. L. (2012). Fast gapped-read alignment with Bowtie 2. Nat. Methods 9 (4), 357–359. doi: 10.1038/nmeth.1923
Li, F., Wu, X., Lam, P., Bird, D., Zheng, H., Samuels, L., et al. (2008). Identification of the wax ester synthase/acyl-coenzyme A: diacylglycerol acyltransferase WSD1 required for stem wax ester biosynthesis in Arabidopsis. Plant Physiol. 148 (1), 97–107. doi: 10.1104/pp.108.123471
Li-Beisson, Y., Shorrosh, B., Beisson, F., Andersson, M. X., Arondel, V., Bates, P. D., et al. (2013). Acyl-lipid metabolism. Arabidopsis book/American Soc. Plant Biologists 11, 1–70. doi: 10.1199/tab.0161
Liu, D., Dong, X., Liu, Z., Tang, J., Zhuang, M., Zhang, Y., et al. (2018). Fine mapping and candidate gene identification for wax biosynthesis locus, BoWax1 in Brassica oleracea L. var. capitata. Front. Plant Sci. 9. doi: 10.3389/fpls.2018.00309
Liu, Q., Wen, C., Zhao, H., Zhang, L., Wang, J., Wang, Y. (2014). RNA-Seq reveals leaf cuticular wax-related genes in Welsh onion. PloS One 9 (11), e113290. doi: 10.1371/journal.pone.0113290
Love, M. I., Huber, W., Anders, S. (2014). Moderated estimation of fold change and dispersion for RNA-seq data with DESeq2. Genome Biol. 15 (12), 1–21. doi: 10.1186/s13059-014-0550-8
Lowe, H. J. B., Murphy, G. J. P., Parker, M. L. (1985). Non-glaucousness, a probable aphid-resistance character of wheat. Ann. Appl. Biol. 106 (3), 555–560. doi: 10.1111/j.1744-7348.1985.tb03146.x
Luan, H., Shen, H., Zhang, Y., Zang, H., Qiao, H., Tao, H., et al. (2017). Comparative transcriptome analysis of barley (Hordeum vulgare L.) glossy mutant using RNA-Seq. Braz. J. Bot. 40 (1), 247–256. doi: 10.1007/s40415-016-0328-1
Moriya, Y., Itoh, M., Okuda, S., Yoshizawa, A. C., Kanehisa, M. (2007). KAAS: an automatic genome annotation and pathway reconstruction server. Nucleic Acids Res. 35, W182–W185. doi: 10.1093/nar/gkm321
Munaiz, E. D., Groves, R. L., Havey, M. J. (2020). Amounts and types of epicuticular leaf waxes among onion accessions selected for reduced damage by onion thrips. J. Am. Soc Hortic. Sci. 145 (1), 30–35. doi: 10.21273/JASHS04773-19
Munaiz, E. D., Havey, M. J. (2020). Genetic analyses of epicuticular waxes associated with the glossy foliage of ‘White Persian’onion. J. Am. Soc. Hortic. Sci. 145 (1), 67–72. doi: 10.21273/JASHS04840-19
Pascal, S., Bernard, A., Sorel, M., Pervent, M., Vile, D., Haslam, R. P., et al. (2013). The Arabidopsis cer26 mutant, like the cer2 mutant, is specifically affected in the very long chain fatty acid elongation process. Plant J. 73 (5), 733–746. doi: 10.1111/tpj.12060
Patro, R., Duggal, G., Love, M. I., Irizarry, R. A., Kingsford, C. (2017). Salmon provides fast and bias-aware quantification of transcript expression. Nat. Methods 14 (4), 417–419. doi: 10.1038/nmeth.4197
Pu, Y., Gao, J., Guo, Y., Liu, T., Zhu, L., Xu, P., et al. (2013). A novel dominant glossy mutation causes suppression of wax biosynthesis pathway and deficiency of cuticular wax in Brassica napus. BMC Plant Biol. 13 (1), 1–14. doi: 10.1186/1471-2229-13-215
Qin, B. X., Tang, D., Huang, J., Li, M., Wu, X. R., Lu, L. L., et al. (2011). Rice OsGL1-1 is involved in leaf cuticular wax and cuticle membrane. Mol. Plant 4 (6), 985–995. doi: 10.1093/mp/ssr028
Rhee, Y., Hlousek-Radojcic, A., Ponsamuel, J., Liu, D., Post-Beittenmiller, D. (1998). Epicuticular wax accumulation and fatty acid elongation activities are induced during leaf development of leeks. Plant Physiol. 116 (3), 901–911. doi: 10.1104/pp.116.3.901
Sas Institute (2009). SAS Scoring Accelerator 1.5 for Teradata: User's Guide (Cary, NC, USA: SAS institute).
Serrano, M., Coluccia, F., Torres, M., L’Haridon, F., Métraux, J. P. (2014). The cuticle and plant defense to pathogens. Front. Plant Sci. 5. doi: 10.3389/fpls.2014.00274
Singh, S., Das, S., Geeta, R. (2018). “Role of cuticular wax in adaptation to abiotic stress: a molecular perspective,” in Abiotic Stress-Mediated Sensing and Signaling in Plants: An Omics Perspective. Eds. Zargar, S., Zargar, M. (Singapore: Springer), 155–182. doi: 10.1007/978-981-10-7479-0_5
Starks, K. J., Weibel, D. E. (1981). Resistance in bloomless and sparse-bloom sorghum to greenbugs. Environ. Entomol. 10 (6), 963–965. doi: 10.1093/ee/10.6.963
Voorrips, R. E., Steenhuis-Broers, G., Tiemens-Hulscher, M., Van Bueren, E. T. L. (2008). Plant traits associated with resistance to Thrips tabaci in cabbage (Brassica oleracea var capitata). Euphytica 163, 409–415. doi: 10.1007/s10681-008-9704-7
Xue, D., Zhang, X., Lu, X., Chen, G., Chen, Z. H. (2017). Molecular and evolutionary mechanisms of cuticular wax for plant drought tolerance. Front. Plant Sci. 8. doi: 10.3389/fpls.2017.00621
Yang, L., Liu, Q., Wang, Y., Liu, L. (2017). Identification and characterization of a glossy mutant in Welsh onion (Allium fistulosum L.). Sci. Hortic. 225, 122–127. doi: 10.1016/j.scienta.2017.05.014
Yeats, T. H., Rose, J. K. (2013). The formation and function of plant cuticles. Plant Physiol. 163 (1), 5–20. doi: 10.1104/pp.113.222737
Zeng, A., Li, J., Wang, J., Song, L., Shi, L., Yan, J. (2017). Studies of genetic characteristics of two heart-shaped glossy cabbage mutants. Sci. Hortic. 226, 91–96. doi: 10.1016/j.scienta.2017.08.033
Keywords: cuticular wax, glossy, mutant, wax biosynthesis, RNA-seq
Citation: Manape TK, Soumia PS, Khade YP, Satheesh V and Anandhan S (2023) A glossy mutant in onion (Allium cepa L.) shows decreased expression of wax biosynthesis genes. Front. Plant Sci. 14:1245308. doi: 10.3389/fpls.2023.1245308
Received: 23 June 2023; Accepted: 07 August 2023;
Published: 23 August 2023.
Edited by:
Poonam Yadav, Banaras Hindu University, IndiaReviewed by:
Debojyoti Moulick, Independent researcher, Kolkata, IndiaRajkumar U. Zunjare, Indian Agricultural Research Institute (ICAR), India
Copyright © 2023 Manape, Soumia, Khade, Satheesh and Anandhan. This is an open-access article distributed under the terms of the Creative Commons Attribution License (CC BY). The use, distribution or reproduction in other forums is permitted, provided the original author(s) and the copyright owner(s) are credited and that the original publication in this journal is cited, in accordance with accepted academic practice. No use, distribution or reproduction is permitted which does not comply with these terms.
*Correspondence: Sivalingam Anandhan, YW5hbmRoYW5zQGdtYWlsLmNvbQ==
†These authors have contributed equally to this work
‡ORCID: Sivalingam Anandhan, orcid.org/0000-0002-1310-0807