- 1Institute of Plant Protection, Fujian Academy of Agricultural Sciences/Fujian Key Laboratory for Monitoring and Integrated Management of Crop Pests/ Fujian Engineering Research Center for Green Pest Management, Fuzhou, China
- 2College of Food Engineering, Zhangzhou Institute of Technology, Zhangzhou Institute of Technology, Zhangzhou, China
- 3Institute of Plant Protection, Chinese Academy of Agricultural Sciences, Beijing, China
Empoasca onukii is a major pest that attacks tea plants. To seek effective and sustainable methods to control the pest, it is necessary to assess its host preference among different species of tea and understand the critical factors behind this behavior. In this study, the behavioral preference of E. onukii for volatile organic compounds (VOCs) of three potted tea species was evaluated. The VOCs released by the three tea species were analyzed using gas chromatography-mass spectrometry, and the major components were used to test the pest’s preference. Transcriptome analysis was used to infer the key genes that affect the biosyntheses of the VOCs. The results showed that the tendency of E. onukii toward the VOCs of the three tea species was the strongest in green tea, followed by white tea, and the weakest in red tea. This behavioral preference was significantly and positively correlated with the relative levels of hexanol, linalool, and geraniol in tea volatiles. Relative hexanol was significantly and positively correlated with the expression of genes TEA009423 (LOX2.1), TEA009596 (LOX1.5), TEA008699 (HPL), TEA018669 (CYPADH), and TEA015686 (ADHIII). Relative linalool was significantly and positively correlated with the expression of genes TEA001435 (CAD) and Camellia_sinensis_newGene_22126 (TPS). Relative geraniol was significantly and positively correlated with the expression of genes TEA001435 (CAD), TEA002658 (CYP76B6), TEA025455 (CYP76T24), and Camellia_sinensis_newGene_22126 (TPS). The above findings suggested that three volatiles (hexanol, linalool, and geraniol) determined the behavioral preference of E. onukii toward tea plants, and their biosynthesis was mainly affected by nine genes (TEA009423, TEA009596, TEA008699, TEA018669, TEA015686, TEA001435, TEA002658, TEA025455, and Camellia_sinensis_newGene_22126).
1 Introduction
Tea is one of the most popular nonalcoholic beverages worldwide, with an attractive aroma and pleasant taste. China is the birthplace of Camellia sinensis and is the largest producer and consumer of tea in the world. In 2022, the global tea cultivation area, production, and export volume were 4.37 million hectares, 6.397 million tons, and 1.827 million tons, respectively, whereas in China they were 2.65 million hectares, 3.35 million tons, and 0.375 million tons, respectively, which accounts for 60.6%, 52.4% and 20.5% of the global tea cultivation area, production and export volume, respectively (Hua, 2023; National Bureau of Statistics, 2023). Fujian is the largest tea-producing and exporting province in China, and among the main tea varieties grown are green tea (GT), red tea (RT), and white tea (WT). In 2022, the tea production and export volume of this province accounted for 15.54% and 25.52% of the national tea production, respectively, and the output value of the whole tea industry chain exceeded 150 billion yuan (Fujian Provincial Bureau of Statistics, 2023; Hua, 2023). In recent years, the expansion of tea planting has increased the occurrence of Empoasca onukii, which has become one of the main pests of tea. It can reduce the yield of tea by 20–30% in general and by at least 50% in severe cases, and may even cause extinctions; this seriously affects the yield and quality of tea and needs urgent prevention and control (Zhang et al., 2022). At present, E. onukii is controlled mainly by using chemical pesticide sprays and accounts for 40–60% of the total pesticide use in tea plantations (Zhang et al., 2020a). However, the frequent use of chemical pesticides leads to residues, resistance, and re-emergence. The “push-pull” pest management strategy is a novel and efficient integrated pest management tool that can use color (visual), chemical pheromones (olfactory), and sound (acoustic) as “push” or “pull” components (Cook et al., 2007). Based on the selection behavior of pests for plant volatiles, repellents that protect target plants or lures to attract target pests have been developed in the integrated pest management of Leptinotarsa decemlineata (Martel et al., 2005), E. vitis (Mu et al., 2012; Zhang et al., 2014), E. flavescens (Niu et al., 2022), and Frankliniella occidentalis (Kim et al., 2023). However, the application of the “push-pull” strategy in the control of E. onukii has not been reported so far. This is mainly because the selection behavior of E. onukii on host plants such as tea leaves is unclear, which limits the development and application of lures or repellents for this insect. Therefore, there is an urgent need to conduct relevant research to support the subsequent green and sustainable control of this insect.
Many factors are known to determine the host selection of phytophagous insects, including secondary metabolites produced by plants (Kong and Lou, 2010). Volatile organic compounds (VOCs) released by plants are small compounds with low boiling points that are produced through secondary metabolic pathways (Dudareva et al., 2013; Goh et al., 2016). The volatiles released by different plants vary greatly and play a crucial role in plant–insect interactions (Mody et al., 2015; Sun et al., 2019; Sharma et al., 2021). Evidence indicates that VOCs are chemical cues for phytophagous insects to search for host plants, as well as self-defense weapons for plants to defend themselves against phytophagous insects (Bruce et al., 2005; D’Alessandro and Turlings, 2005 and Dudareva and Negre, 2005; D’Alessandro and Turlings, 2006; Tholl, 2006; Hare, 2011; Poelman et al., 2012; Sun et al., 2012; Anderson and Anton, 2014). Therefore, the search for plant volatiles with lure or repellent functions for phytophagous insects and the identification of genes related to the synthesis of these specific volatiles are of great significance in the development of specific lures or repellents for pests and the cultivation of insect-resistant varieties using genetic engineering technology for the development of green pest control.
How E. onukii selects volatiles from different tea varieties, the specific components of the volatiles involved in these behavioral preferences or the genes involved in the synthesis of these components is unclear. Resolving these problems can provide a basis for the development of specific lures or repellents for E. onukii, as well as the breeding of tea varieties resistant to E. onukii through genetic engineering technology. This could not only effectively control the damage by the insect and improve the quality and yield of tea but also promote the application of green pest control measures, reduce the use of chemical pesticides, and promote the green development of the tea industry, with significant economic, social and ecological benefits. In this study, we selected potted green, red, and white tea grown in Fujian as the test plants to observe the behavioral preference of E. onukii to their volatiles. The components and contents of the three tea volatiles were measured, and the correlation between the behavioral preference of the insect and the tea volatiles was analyzed to detect the specific volatile components that determine the selection behavior of the insect. Then, the behavioral preference of E. onukii to the standards of these specific volatile components was observed to verify the specificity of each volatile component. Finally, we analyzed the transcriptome changes of the three tea species to find the genes related to the synthesis of specific volatiles. This study could provide key components for the subsequent development of specific attractants or repellents of E. onukii and candidate target genes for the breeding of resistant tea varieties using genetic engineering technology to promote the development of green pest control.
2 Materials and methods
2.1 Materials
2.1.1 Test plants
GT ‘Jiulongcuiya’, RT ‘Jinmudan’, and WT ‘Jiulongdabai’ were selected from the tea planting base in Nanping City, Fujian Province (27.48° N, 118.82° E) with potted tea seedlings plants 20–22 cm in height, then the seedlings were transferred to 12 cm (diameter) × 10 cm (height) × 8 cm (bottom diameter) pots with peat soil and restored for 10 d (Figure S1).
2.1.2 Test insects
E. onukii was collected from the same tea planting site as above and grown at room temperature 24–27°C, relative humidity 55%–65%, and photoperiod 16L:8D. The adults in the next generation fledged for 2 d of the second generation were starved for 12 h and kept in cages.
2.2 Methods
2.2.1 Determination of the behavioral preference of E. onukii to tea VOCs
The behavioral preference of E. onukii to volatiles from GT, RT, and WT was analyzed in a two-way selection test using a Y-type olfactometer at a room temperature of 24–27°C and in darkness and confinement, following the methods of Lin et al. (2016) and Niu et al. (2022). Six groups were set in the experiment: (1) GT vs. fresh air (FA), (2) RT vs. FA, (3) WT vs. FA, (4) GT vs. RT, (5) GT vs. WT, and (6) RT vs. WT; each treatment was replicated three times.
The three different potted tea seedlings were sealed in seedling pots and peat soil under polyethylene cling film and placed in a 35 cm (height) × 25 cm (diameter) glass air collector for 24 h. Then fresh air was blown by an air pump at a flow rate of 200 ± 10 mL/min and filtered by a molecular sieve, color-changing silica gel, and activated carbon, through the air inlet at the top of the air collector and blown out through the air outlet along the top of the air collector, then into the Y-type olfactometer. A small amount of cotton was inserted at the interface between the two arms of the olfactometer and the silicone tube to prevent E. onukii from crawling into the collection tank along the hose during the test (Figure 1). An adult E. onukii was placed in the entrance of the main arm of the Y-type olfactometer, and then the entrance was blocked with cotton to prevent the insect from escaping. The selection was considered valid if E. onukii crawled into more than 1/3 of both arms of the Y-type olfactometer within 5 min and continued for more than 30 s. If E. onukii did not select after 5 min, the selection was considered abandoned. Each E. onukii was used only once in the experiment, and the Y-type olfactometer was changed every 5 insects. The number of effective selections of E. onukii in each treatment was counted at the end of each group, and the Y-type olfactometer, silicone tubes, and glass collection cylinders were washed with ethanol and hot water at 60–70°C. Student’s t-test in GraphPad Prism 7 (GraphPad Software Inc., San Diego, CA, USA) was then used to compare the differences in olfactory responses of E. onukii in each treatment.
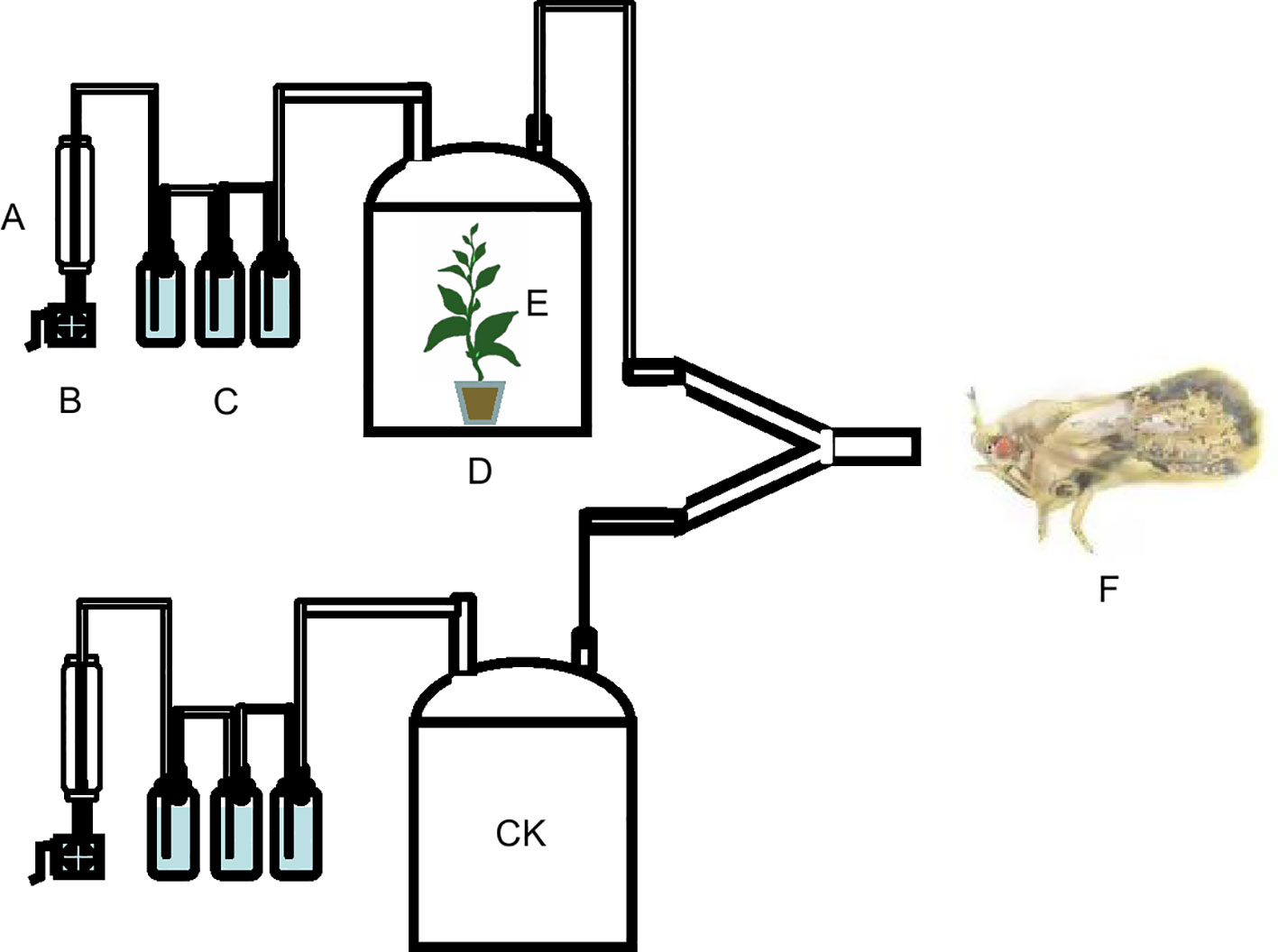
Figure 1 Schematic diagram of the olfactory experiment of Empoasca onukii to natural volatile organic compounds in three potted tea plants. (A) Flow meter, (B) pump, (C) air-purifying cups, (D) glass jar, (E) tea plants, and (F) Empoasca onukii adult, (CK) Fresh air/Tea plants.
2.2.2 Determination of VOCs in tea leaves and its correlation with the behavioral preference of E. onukii
The volatiles of the three tea species were extracted by solid-phase microextraction (SPME) and detected by gas chromatography-mass spectrometry (GC-MS) (7890B-5977A; Agilent, Palo Alto, CA, USA), referring to Mei et al. (2017); Vallarino et al. (2018) and Niu et al. (2022). Three seedlings of the three different tea plants were taken at room temperature of 24–27 °C and the leaves of each plant were removed and immediately fixed in liquid nitrogen for 15 min to prevent the volatiles from changing due to trauma. Then, 5 g of each tea plant was randomly taken and placed into a 100 mL headspace vial. A 57328-U solid-phase microextraction needle (Sigma-Aldrich, St. Louis, MO, USA) was inserted into the headspace vial. The extraction was performed for 60 min and volatiles were detected on the machine.
Chromatographic column: An HP-5MS capillary column (30 mm × 0.25 mm, i.d., 1.0 µm film thickness, Agilent); inlet temperature 250 °C, interface temperature 280 °C; programmed temperature rise: initial temperature 50 °C for 5 min, then 3.0 °C/min to 190 °C for 5 min, then 8.0 °C/min to 200 °C for 5 min, then 15.0 °C/min to 280 °C for 15 min. Mass spectrometry conditions were an EI ionization electron energy of 70 eV. Mass spectra were acquired by scanning from 25–500 m/z. Based on the retention time of each volatile substance in the chromatogram during the detection of tea samples, the NIST library of GC-MS was searched, and the search results were checked with references to the standard spectra. The components of the volatiles from the three tea varieties were identified and analyzed, and the relative content of each component was calculated by the normalization method of peak area. The Tukey’s test in GraphPad Prism 7 software was used to compare the differences in the relative contents of the volatile components of the three tea leaves. The effective selection number of E. onukii on the three tea leaves and the relative contents of each volatile component among the three tea leaves were used as variables. The correlation between two variables was analyzed by Pearson correlation and a significance test p < 0.05 was used as the criterion for screening the specific components that determine the host selection behavior of E. onukii.
2.2.3 Determination of the behavioral preference of E. onukii to volatile component standards
Referring to the methods of Lin et al. (2016) and Niu et al. (2022), hexanol, linalool, and geraniol, were selected from tea volatiles that showed a significant correlation with the behavioral preference of E. onukii. The three components (97–99% purity) were purchased from Shanghai Macklin Biochemical Co., Ltd. Each component was dissolved in triethyl citrate (98% purity), a slow-release solvent, and diluted to different concentrations before the test.
In the single component treatment, the concentration of each component standard was based on its relative content in the three tea volatiles, including 2, 4, and 6 µg/100 µL for hexanol, 4, 8, and 12 µg/100 µL for linalool, and 3, 6 and 9 µg/100 µL for geraniol. Air was used as blank control for each treatment. In the mixed component treatment, the concentration ratio of each component standard was set to simulate the relative content of the corresponding volatiles in the three tea species. The GT blend contained 3.97 µg/100 µL of hexanol, 8.56 µg/100 µL of linalool, and 5.65 µg/100 µL of geraniol, the RT blend contained 0.91 µg/100 µL of hexanol, 2.18 µg/100 µL of linalool and 1.37 µg/100 µL of geraniol, and the WT blend contained 2.10 µg/100 µL of hexanol, 4.64 µg/100 µL of linalool and 3.51 µg/100 µL of geraniol. Three sets of comparison treatments were set up for the three mixed components: (1) blend GT vs. blend RT, (2) blend GT vs. blend WT, and (3) blend RT vs. blend WT. After that, 1 mL of the suspension solution of each single ingredient or blend of ingredients for each treatment was pipetted onto a small piece of cotton; then the small piece of cotton was placed in an open glass Petri dish, and then the Petri dish was placed in an air collection jar, after which the effective selection number of E. onukii in each treatment was observed and counted according to the previous method. Thirty E. onukii were observed in each treatment, and this was repeated three times. The t-test in GraphPad Prism 7 software was used to compare the differences in the behavioral preference of E. onukii in each treatment of the three compound standards to verify the specificity of the three volatile components.
2.2.4 Expression analysis of genes related to the synthesis of VOCs in tea leaves
Transcriptome analysis: Leaves from three seedlings of the three different tea plants were cut quickly and immediately fixed in liquid nitrogen for 15 min to prevent transcriptional changes caused by trauma. Then, 5 g of leaves from each potted tea plant were randomly taken into 50 mL lyophilization tubes, immediately buried in dry ice, and sent for total RNA extraction, library construction, and sequencing. Total RNA was analyzed by Nanodrop 1000 ultra-micro spectrophotometer (Thermo Fisher, Waltham. MA, USA), Qubit 2.0 fluorescence quantification instrument, and Agilent 2100 Bioanalyzer. After library construction, high-throughput sequencing was performed using an Illumina HiSeq 2000 (Illumina, SanDiego, CA, USA). After the sequencing and datas quality control were completed (Tables S1-S3), the Camellia sinensis genome (http://tpdb.shengxin.ren/) was used as the reference, and the HISAT2 system and StringTie software were applied to compare and assemble the valid data volume (clean reads) obtained from the sequencing, and the Unigene sequences obtained were compared with BLASTx on NCBI NR, Swiss-Prot, GO, COG, KOG, EggNOG, Pfam, and KEGG databases. Those with similarity > 30% and e < 1e-5 were used for Unigene annotation and adjusted with the Benjamini-Hochberg method. Using the Benjamini-Hochberg method, some genes related to the synthesis of three specific volatiles were initially selected based on the false discovery rate (FDR) < 0.01 and fold change |(FC)| > 2, and the functional annotation of the genes. Then the expression of these related genes was normalized (Benjamini and Hochberg, 1995; Benjamini and Yekutieli, 2001), and the correlation between the three specific volatile components and the expression of the related genes was analyzed by Pearson’s correlation in GraphPad Prism 7 software, followed by a significance test of p< 0.05 was used as the criterion to screen the key genes.
Real-time quantitative fluorescence PCR (RT-qPCR) validation: Referring to the method of Fang et al. (2022), we designed the specific primers (Table S4) for genes related to the synthesis of three specific volatile components using Primer Premier 5.0 software, using β-actin as the internal reference gene, and the cDNA was synthesized using the TRUEscript 1st Strand cDNA Synthesis Kit Reverse Transcription Kit (Beijing Aidlab Biotechnologies Co. Ltd., Beijing, China). The amplified products were detected on a qTOWER2.2 real-time fluorescence PCR system (Analytikjena, Jena, Germany) using 2 × SYBR Green Premix (Chengdu Danfeng Technology Co. Ltd., Chengdu, China)), and repeated three times for each sample. The relative expression of the genes related to the synthesis of the three specific volatile components was calculated using the 2−ΔΔCT method (Livak and Schmittgen, 2001).
3 Results
3.1 Behavioral preference of E. onukii to tea VOCs
The effective selection numbers of E. onukii for the three tea tree volatiles were significantly different. The highest effective selection numbers were for GT, GT vs. FA, GT vs. RT, and GT vs. WT were 23. 67, 18.67, and 16.33, respectively. The effective selection numbers for GT were significantly more than for air (t=16.8375, p=0.0001, df=4), RT (t=7.5378, p=0.0017, df=4), and WT (t=3.2071, p=0.0327, df=4). The effective number of selection on WT was the next highest, WT vs. FA, RT vs. WT, and GT vs. WT at 19.67, 14.67, and 12.33, respectively, and significantly more than for air (t=13.9140, p=0.0002, df=4) and RT (t=3.2118, p=0.0325, df=4). The least effective number of selections was on RT, which was only 16.67, 10.33, and 10.00 in RT vs. FA, GR vs. RT, and RT vs. WT, respectively, but significantly higher than air (t=6.7082, p=0.0026, df=4) (Figure 2).
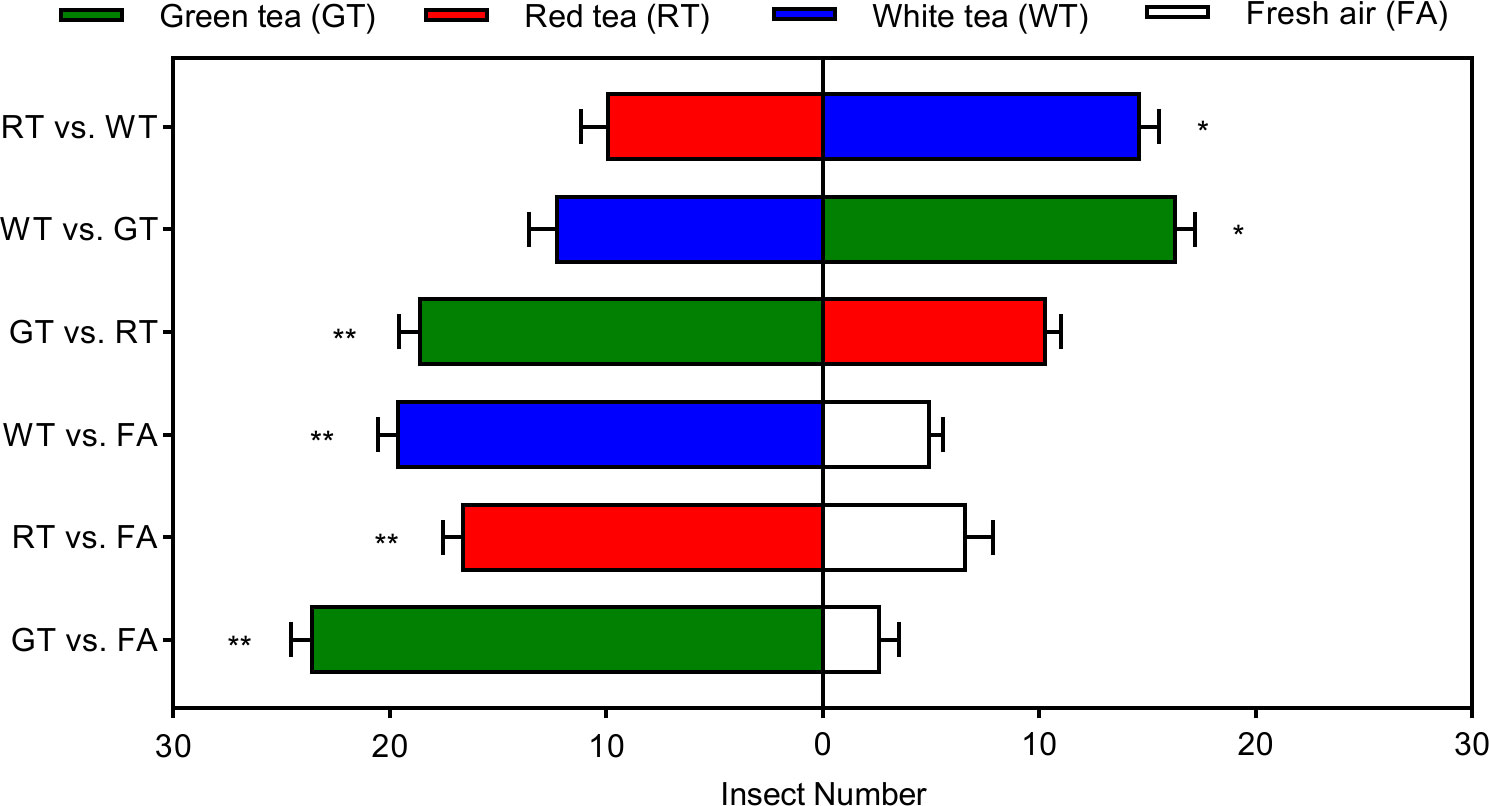
Figure 2 Behavioral preference of Empoasca onukii to natural volatile organic compounds in three potted tea plants. Bars indicate mean±SE. The * and ** indicate significant difference (P<0.05) and highly significant difference (P<0.01), respectively.
3.2 Correlation between VOCs of tea leaves and behavioral preference of E. onukii
A total of 44 volatile components were identified from the three tea species, and the number of volatiles was not significantly different among the three species, including 30 for GT, 29 for RT, and 39 for WT. The compositions were also similar, with 24 volatiles identical between GT and RT, 26 volatiles identical between GT and WT, 27 volatiles identical between RT and WT, and 23 volatile components identical among the three species (Figure 3). However, the relative contents of each component in the three tea volatiles were different, among which the relative contents of hexanol, linalool, and geraniol were not high, ranging from 0.91% to 3.97%, 2.18% to 8.56%, and 1.37% to 5.65%, respectively, but the differences were significant among the varieties (F=12.0810–137.1140, p=0.0001–0.0079, df=8), and all showed significant positive correlations (r≥0.9976, p<0.05) with the behavioral preference of E. onukii (Figure 3, S2; Tables 1, S5).
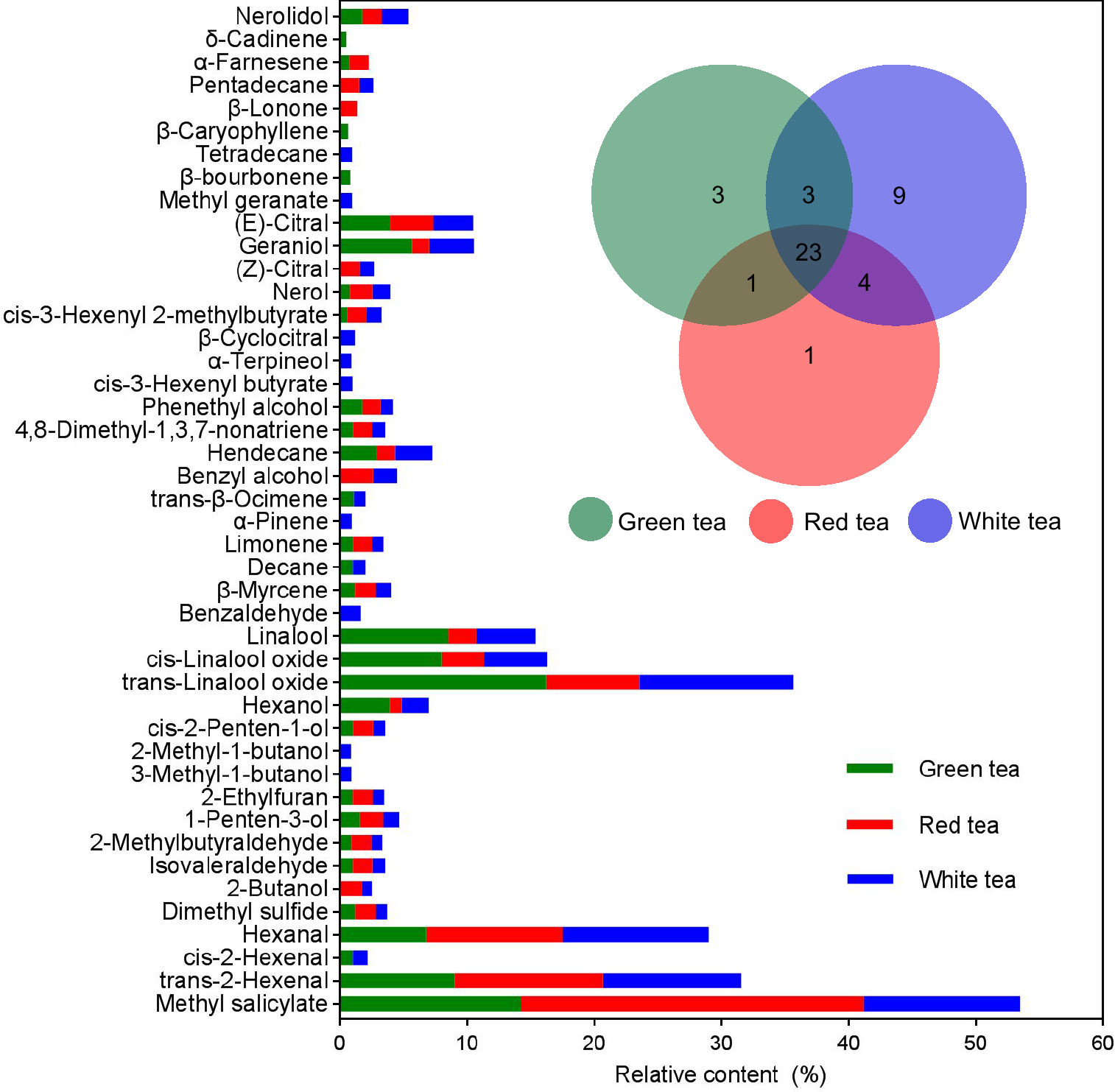
Figure 3 Relative content and Venn set of volatile organic compounds (VOCs) in three potted tea plants. Different color columns and circles indicate relative content and the number of the digits in different color circles indicates unique (nonoverlapping color parts) and shared (overlapping color parts) VOC number among three potted tea plants. The digits in different color circles indicate unique (nonoverlapping color parts) and shared (overlapping color parts) VOC number among three potted tea plants.
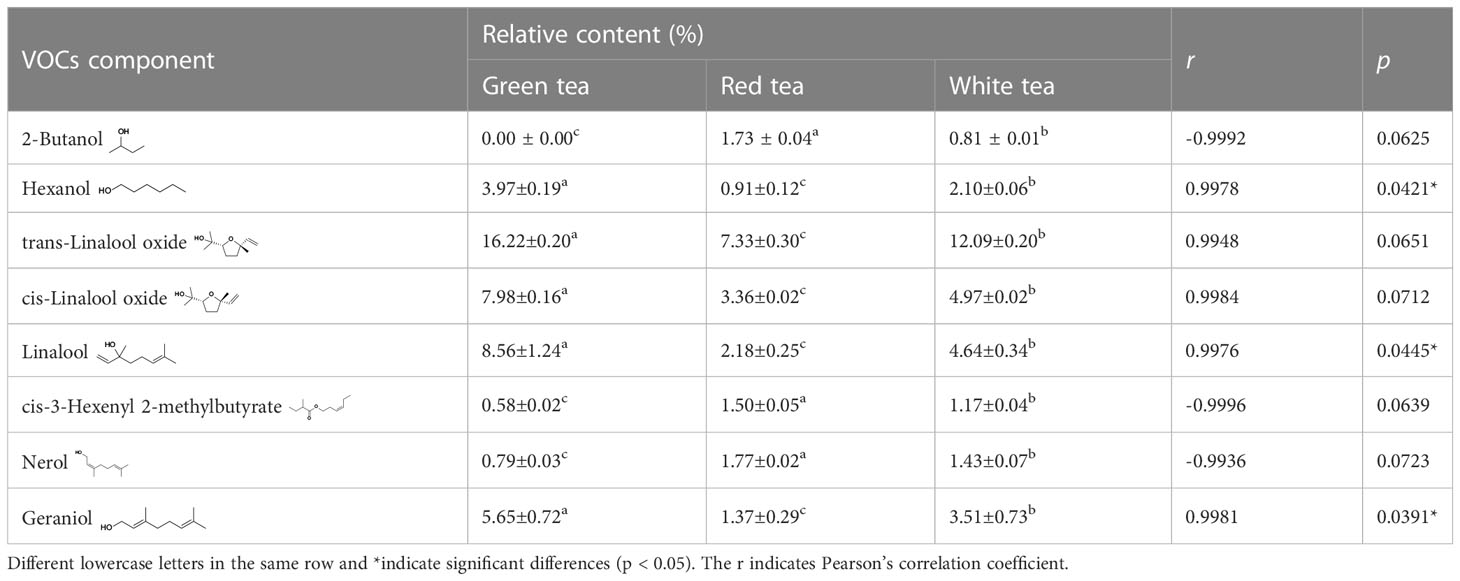
Table 1 Correlations between relative content of volatile organic compounds of tea plants and behavioral preference of Empoasca onukii (mean ± SE, p < 0.1).
3.3 Behavioral preference of E. onukii to volatile component standards
The behavioral preference of E. onukii to the three volatile constituent standards was strongly influenced by the concentrations. The effective selection number of E. onukii increased with increasing volatile concentration in the experimental design concentration range of a single constituent. In hexanol, the effective selection number was significantly higher for 2 µg/100 µL (t=4.1110, p=0.0283, df=4) and 4 µg/100 µL (t=3.3588, p=0.0147, df=4) than for air, and the effective selection number was significantly higher for 6 µg/100 µL (t=4.7246, p=0.0091, df=4) than air. In linalool, the effective selection number of E. onukii was significantly higher for 4 µg/100 µL (t=3.5907, p=0.0229, df=4) than air, and the effective selection number of E. onukii for 8 µg/100 µL (t=8.2851, p=0.0012, df=4) and 12 µg/100 µL (t=6.3640, p= 0.0031, df=4) was significantly higher than air. In geraniol, 3 µg/100 µL (t=10.7517, p=0.0004, df=4), 6 µg/100 µL (t=7.8889, p=0.0014, df=4), and 9 µg/100 µL (t=9.4705, p=0.0007, df=4) were significantly higher than the control (Figure 4).
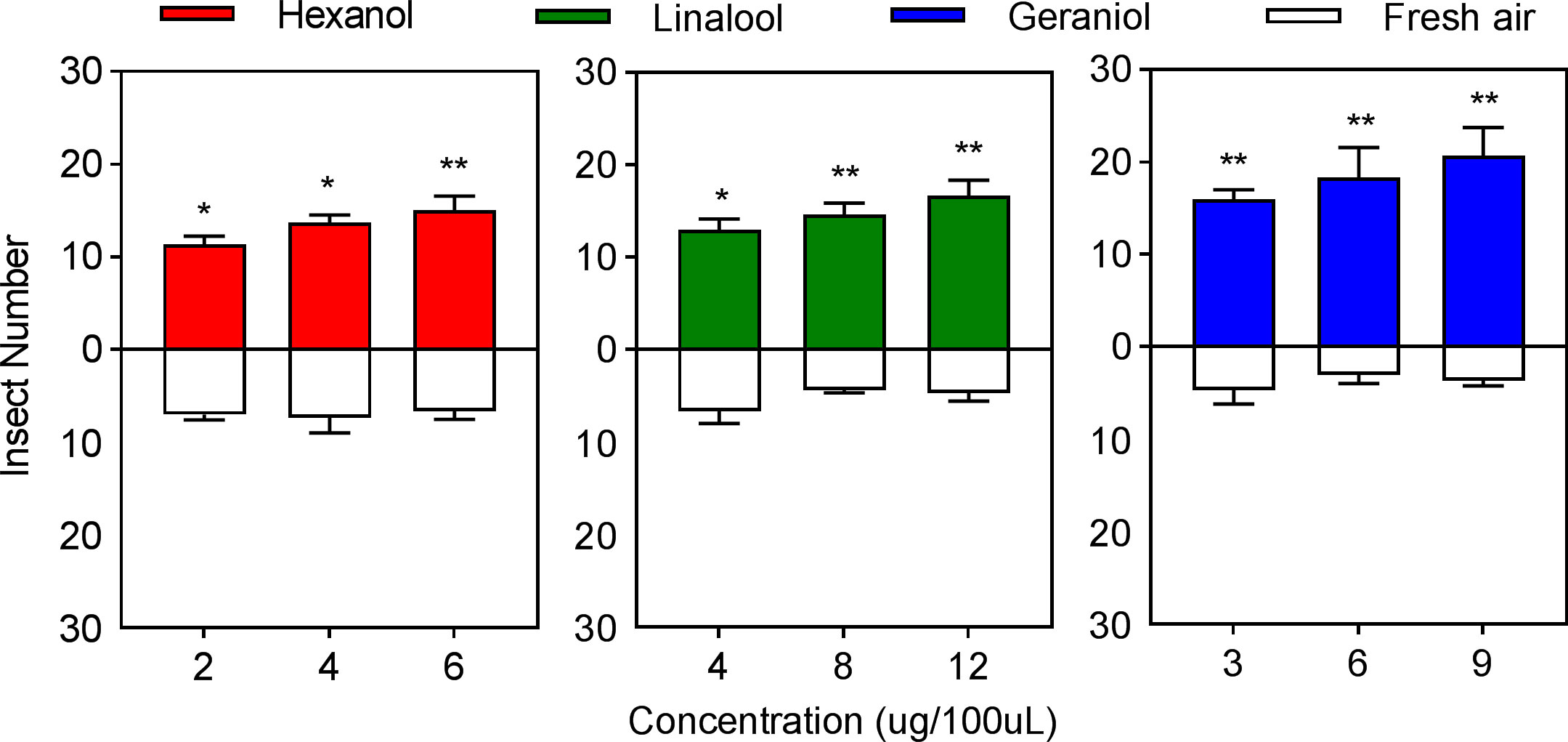
Figure 4 Behavioral preference of Empoasca onukii to three volatile organic compounds. Bars indicate mean±SE. The * and ** indicate significantly different parameters (P<0.05) and bars indicate the mean±SE; the * and ** indicate significantly different (P<0.05) and highly significantly different parameters (P<0.01), respectively.
There was also a significant difference in the number of effective selections of E. onukii among the experimental design treatments for the volatile blend components. E. onukii had the highest number of effective selections in the GT blend, which was significantly higher than in the RT blend (t= 4.1576, p=0.0142, df=4) and WT blend (t= 3.4641, p=0.0257. df=4). E. onukii had the next highest number of effective selections in the WT blend, significantly more than in the RT blend (t=3.0237, p=0.0390, df=4). The lowest number of effective E. onukii selections occurred in the RT blend (Figure 5). It can be seen that the behavioral preference of E. onukii for synthetic and natural volatiles was similar.
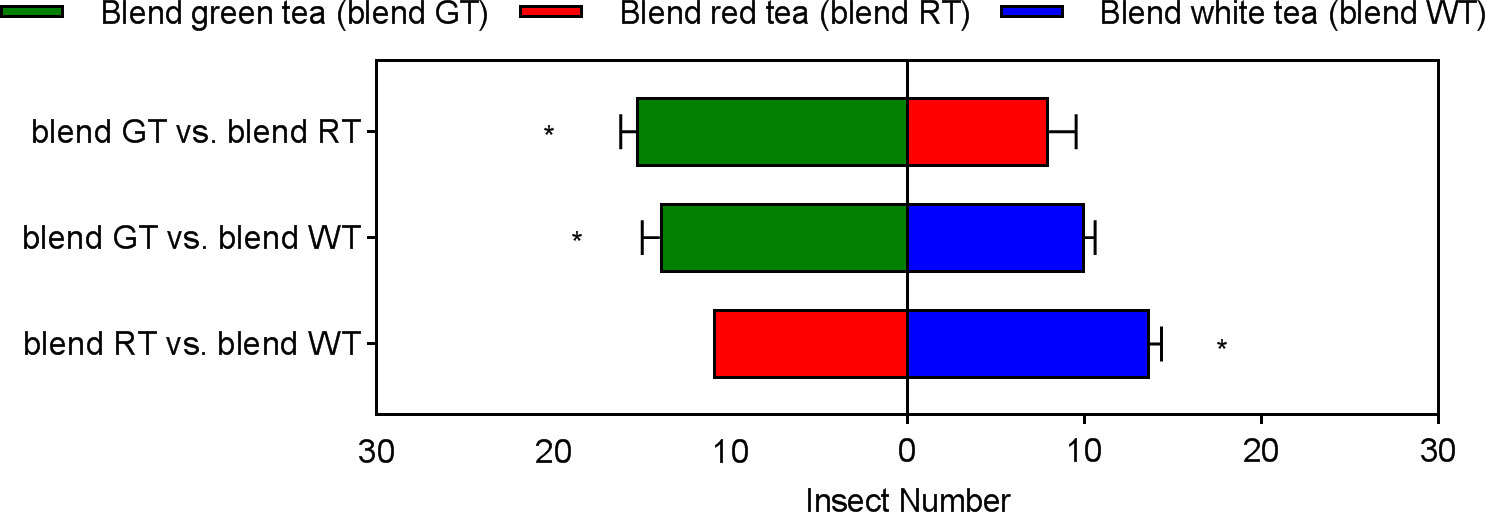
Figure 5 Behavioral preference of Empoasca onukii to three volatile blends. Bars indicate the mean±SE. The * indicates a significant difference (P<0.05).
3.4 Expression of genes related to the synthesis of VOCs in tea leaves
Transcriptome analysis of the leaves of GT, RT, and WT revealed that there were 53 differentially expressed genes directly involved in hexanol synthesis (lipoxygenase pathway) and 82 differentially expressed genes directly involved in linalool and geraniol synthesis (monoterpenoid biosynthesis pathway) in the three tea species (Figure 6).
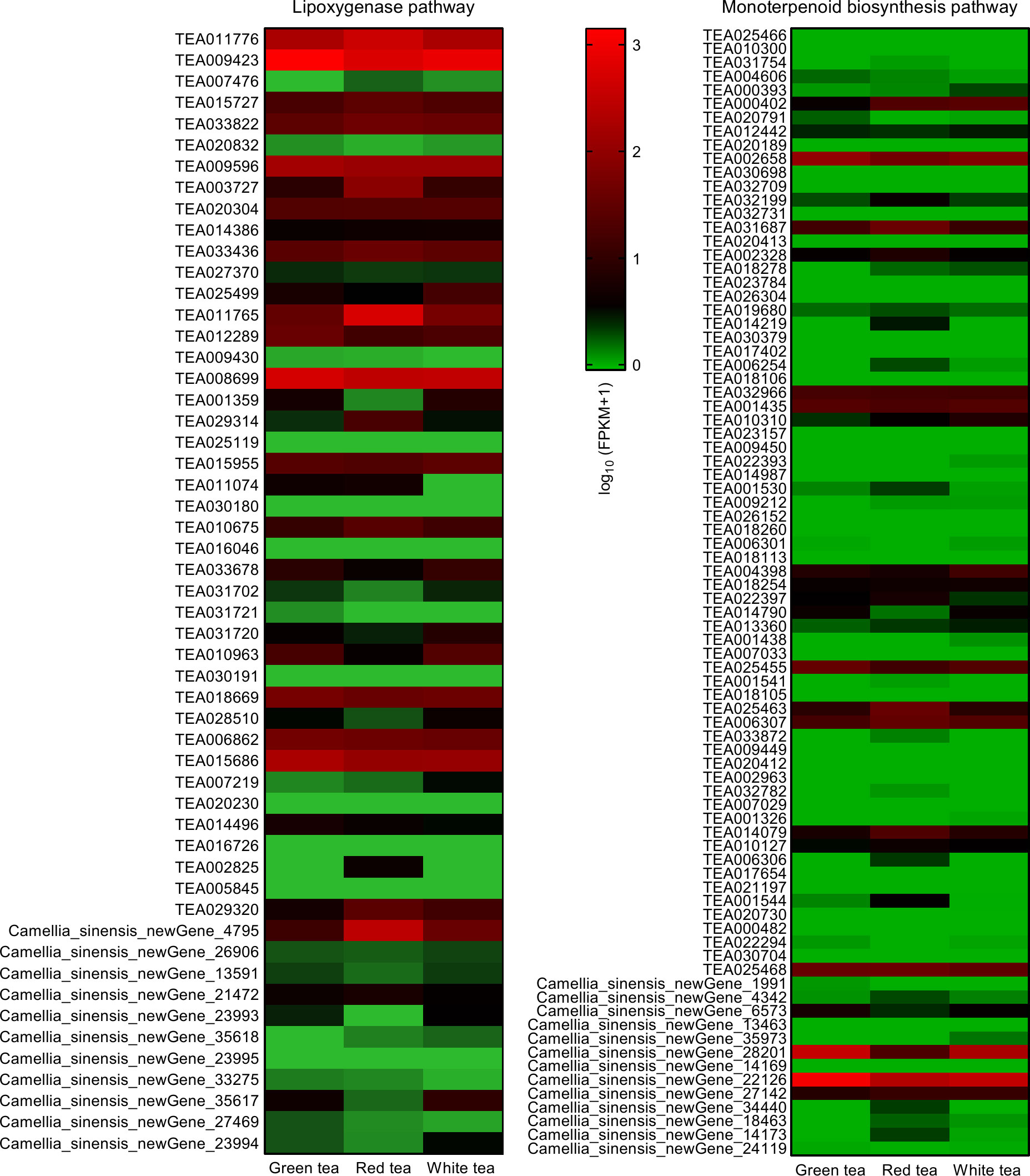
Figure 6 Expression heat map of lipoxygenase genes and monoterpene synthase genes of leaves in three potted tea plants. FPKM, fragments per kilobase of exon per million mapped fragments.
Combined with Pearson’s correlation analysis, the relative hexanol content in the three teas was found to be correlated with the genes in the lipoxygenase pathway TEA009423 (LOX2.1) (r=0.9981, p=0.0390, df=4), TEA009596 (LOX1.5) (r=0.9976, p=0.0443, df=4), TEA008699 (HPL) (r=0.9978, p=0.0423, df=4), TEA018669 (CYPADH) (r=0.9982, p=0.0379, df=4), and TEA015686 (ADHIII) (r=0.9992, p=0.0250. df=4) expression were consistent with each other and showed a significant positive correlation. The relative content of linalool was significantly correlated with the expression of the genes TEA001435 (CAD) (r=0.9978, p=0.0426, df=4) and Camellia_sinensis_newGene_22126 (TPS) (r=0.9978, p=0.0419, df=4) in the monoterpenoid biosynthesis pathway showed the same trend and a significant positive correlation. The relative content of geraniol was significantly correlated with the expression of genes TEA001435 (CAD) (r=0.9979, p=0.0410, df=4), TEA002658 (CYP76B6) (r=0.9999, p=0.0090, df=4), TEA025455 (CYP76T24) (r=0.9997, p= 0.0143, df=4), and Camellia_sinensis_newGene_22126 (TPS) (r=0.9979, p=0.0417, df=4) showed consistent and significant positive correlation in expression (Tables 2, S6). A q-PCR analysis also confirmed that the trends of relative expression of these nine volatile compound synthesis-related genes in the three tea species were consistent with the results of digital gene expression profiling (Figure 7).
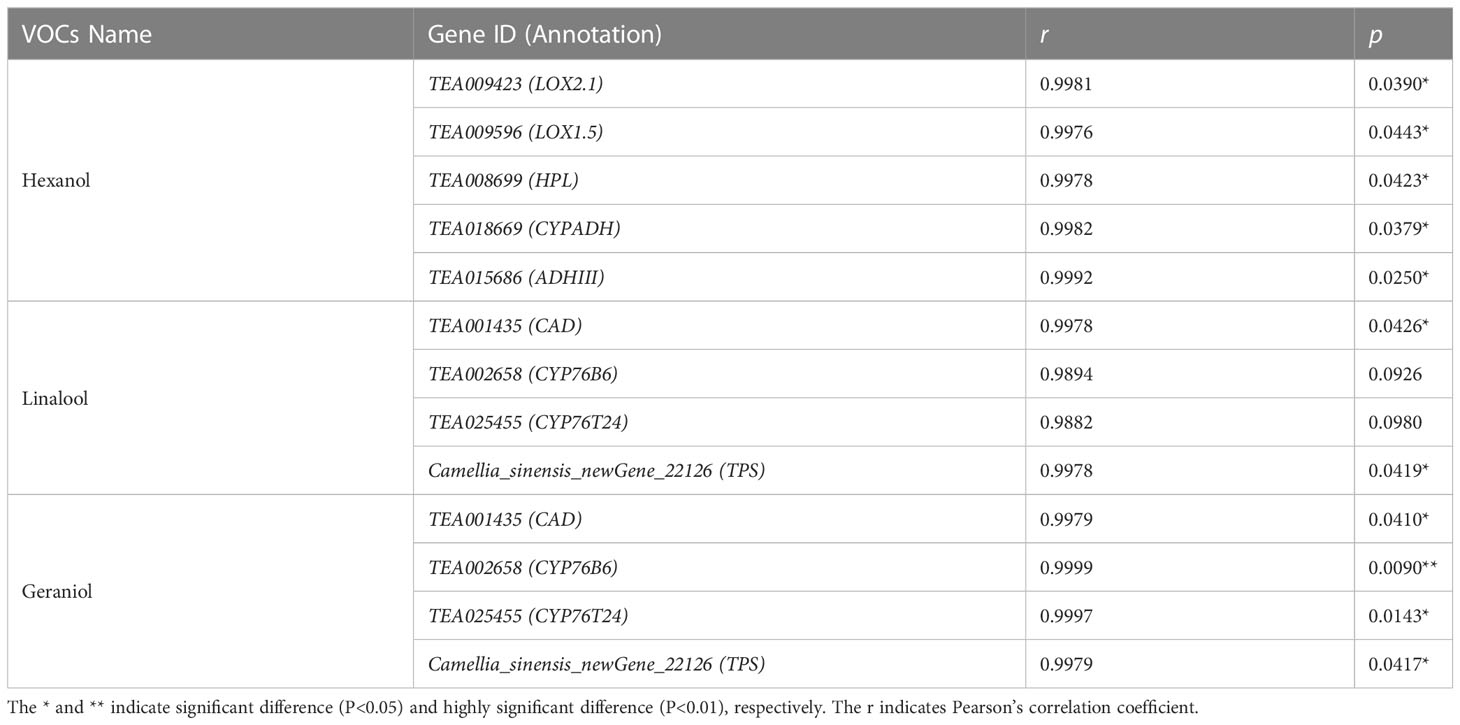
Table 2 Correlations between relative content of three volatiles and expression of nine genes in three potted tea plants (P<0.1).
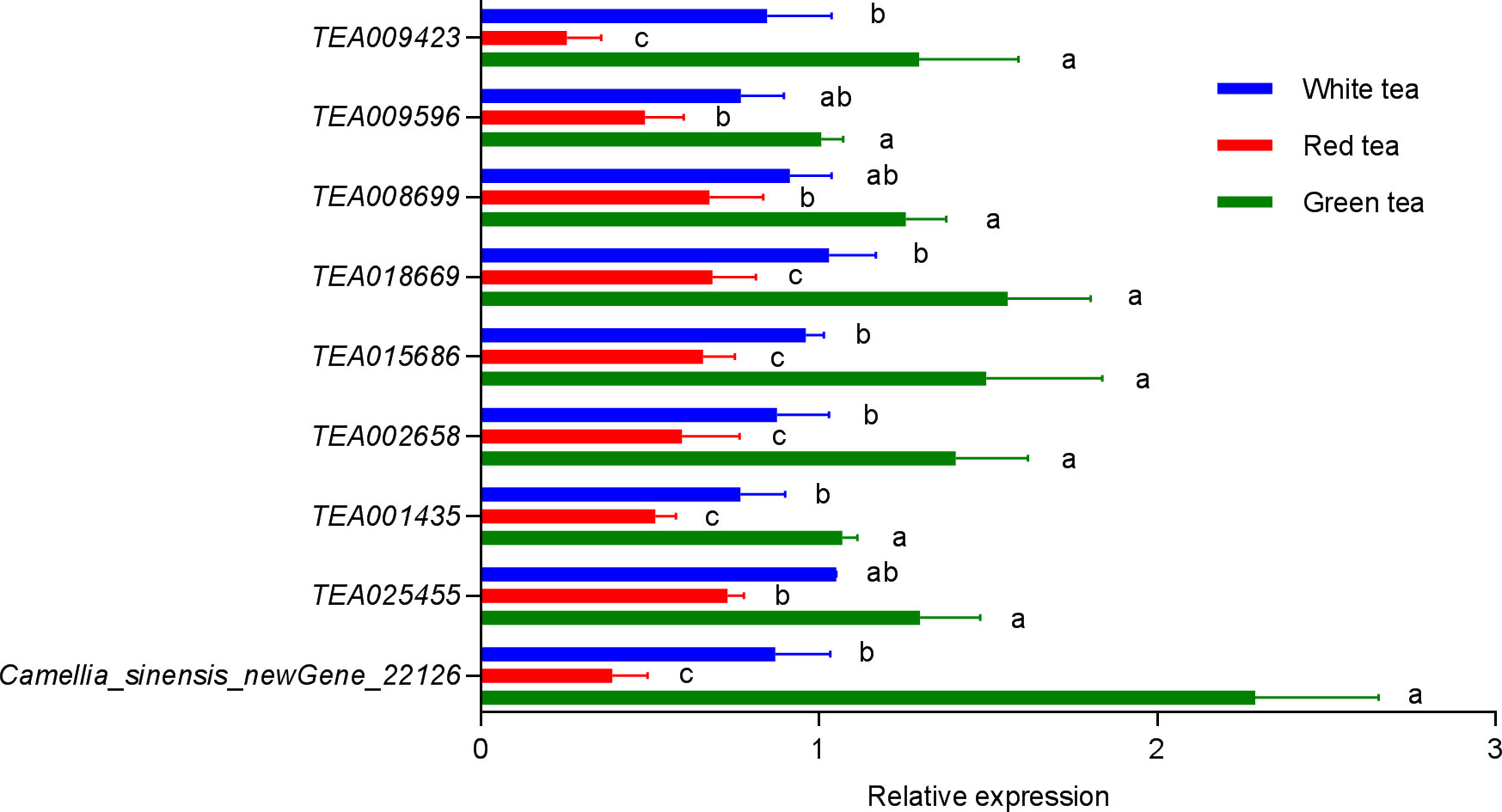
Figure 7 Relative expression of nine genes related to volatile synthesis in three potted tea plants. Bars indicate the mean±SE. Different lowercase letters indicated a significant difference (P<0.05).
4 Discussion
Insects and plants have evolved together over a long period of time to form a variety of interactions, in which phytophagous insects express their predation in a series of behavioral responses, including insect orientation and recognition of host plants (Qin and Wang, 2001). It has been shown that volatiles released from plants can induce directional behavior of insects and are important stimulus signals for phytophagous insects to search for host plants. For example, Tingle et al. (1990) studied the selection of Heliothis virescens and H. subflexa female moths for insect-susceptible and insect-resistant host plants and found that H. virescens showed a tendency to volatiles from insect-susceptible Nicotiana tabacum, Desmodium tortuosum, Gossypium and Physais plants, while H. subflexa showed a significant tendency to volatiles from insect-susceptible Physais host plants, but both nocturnal moths showed no tendency to volatiles from insect-resistant tobacco species. In the present study, we found that the tendency of E. onukii to the volatiles of the three tea species was strongest in GT, followed by WT, and weakest in RT, which may be related to the difference in the resistance of tea species to E. onukii.
Plant volatiles are usually a mixture of secondary metabolites, consisting of fatty acid derivatives, terpenoids and their derivatives, sulfides, aromatic compounds, etc. (Bernays and Chapman, 1994; Belostotsky, 2009). The volatiles contained in different plants can vary greatly in composition or content, which is the fundamental reason for the difference in the selection behavior of phytophagous pests toward different plants (Kong and Lou, 2010). Similarly, phytophagous insects do not sense all volatiles. For example, Smicronyx fulvus only has a preference for a specific-proportion of five monoterpenes in the odor released by Helianthus annuus (Roseland et al., 1992). Plutella xylostella is strongly influenced by the mustard oil (Isothiocyanates) emitted by plants of this family when making selections for Brassicaceae plants (Verkerk and Wright, 1994). Farnesene released by Zea mays has a strong attractive effect on Ostrinia nubilalis (Binder et al., 1995). The odor of Datura wrightii flowers consists of about 60 compounds, but Manduca sexta only responds behaviorally to nine of them (Riffell et al., 2009). During pollination, Ceratosolen solmsi can sense three sesquiterpenes and benzyl benzoate in Ficus hispida flowers, whereas C. gravelyi can sense 4-methylanisole in F. semicordata flowers (Zhang et al., 2020b). The decanal released by F. pumila during the pollination stage was significantly attractive to Wiebesia pumilae (Wang et al., 2021). In this study, we found that the amount and composition of the volatiles in the three tea species were not significantly correlated, but the relative contents of each component were different. The relative contents of hexanol, linalool, and geraniol, although not high, were significantly and positively correlated with the behavioral preference of E. onukii. This correlation was confirmed in single and mixed tests of these three components. These three volatile components could be the specific components that determine the host selection behavior of E. onukii, and the results of this study can provide key components for the subsequent development of specific lures for this insect.
Plant volatiles are important components for insects to find hosts and have effects on insects such as luring, repelling, and transmitting information (Schoonhoven et al., 2005). There are about 100 kinds of volatiles in fresh tea leaves, including terpenoids, alcohols, hydrocarbons, aldehydes, ketones, esters, benzenes, phenols, acids, lactones and nitrogenous compounds, which are mainly formed by the pathways such as terpenoid biosynthesis, benzene ring compound synthesis, carotene oxidative degradation, and fatty acid derivative synthesis (Lian et al., 2014). Hexanol is a fatty alcohol compound, which has been reported to have a luring effect on Melanoplus sanguinipes (Kang and Hopkins, 2004). In the present study, this compound was also found to have a significant luring effect on E. onukii at the tested concentration range, reaching a maximum at 6 µg/100 µL. Linalool and geraniol are monoterpene compounds, of which linalool has been reported to have a significant effect on E. vitis (Zhao et al., 2002; Wang et al., 2016), M. sexta (He et al., 2019), and Thrips hawaiiensis (Li et al., 2022a), whereas geraniol has also been reported to have eliciting effects on E. vitis (Chen et al., 2003), Chrysopa septempunctata (Han and Zhou, 2004), and Frankliniella occidentalis (Li et al., 2022b), both of which were also found to have significant elicitation effects on E. onukii in the tested concentration range, reaching a maximum at 12 µg/100 µL and 9 µg/100 µL, respectively. These results not only validated the selection of the three tea volatiles by E. onukii but also provided a basis for the concentration configuration of the key components in the subsequent development of this insect-specific lure, as well as a reference for the study of the effect of these components on the behavioral preference of other insects.
The type and content of volatiles produced by plants are genetically controlled (Schoonhoven et al., 2005; Kong and Lou, 2010). Fatty alcohol compounds are synthesized mainly through the lipoxygenase pathway catalyzed by related genes controlling lipoxygenase (LOX), hydroperoxide lyase (HPL), and alcohol dehydrogenase (ADH) (Contreras and Beaudry, 2013; Zhou et al., 2019; Niu et al., 2022; Wang et al., 2022). Monoterpenes are mainly synthesized through the monoterpenoid biosynthesis pathway catalyzed by terpene synthase (TPS) controlled by related genes (Schilmiller et al., 2009; Yu and Utsumi, 2009; Zhou et al., 2019). Among the three volatiles involved in this study, hexanol was reported to be highly significantly correlated with the expression levels of the LOX1 and ADH genes in C. sinensis (Zhou et al., 2019) . The hexanol content in Malus pumila × M. asiatica (Wang et al., 2022) was significantly and positively correlated with the expression of LOX2a, and in Vitis vinifera it was correlated with the expression of LOXA, HPL1, and ADH3 (Li et al., 2022b). The present study also found a significant positive correlation between the relative content of this volatile substance and the expression of TEA009423 (LOX2.1), TEA009596 (LOX1.5), TEA008699 (HPL), TEA018669 (CYPADH), and TEA015686 (ADHIII). Linalool has been previously reported to be significantly correlated with the expression of linalool in the strawberry plants Fragaria spp. (Aharoni et al., 2004), Antirrhinum majus (Nagegowda et al., 2008), Gossypium hirsutum (Huang et al., 2018), Nicotiana attenuata (He et al., 2019) and C. sinensis (Mei et al., 2017) are regulated by genes FaNES1, AmNES/LIS-2, GhTPS12, NaLIS and CsLISs, respectively. Geraniol has been previously reported in Ocimum basilicum (Iijima et al., 2004), Madagascar periwinkle (Simkin et al., 2012), Rosa hybrida (Magnard et al., 2015), and Camptotheca acuminata (Chen et al., 2016), which are regulated by the genes ObGES, CrGES, RhNUDX1, and CaGES, respectively. The present study found that linalool was significantly and positively correlated with the expression of the genes TEA001435 (CAD) and Camellia_sinensis_newGene_22126 (TPS). Although the sequences of these two genes are only 42.75% similar to the identified linalool synthase genes CsLIS1 and CsLIS2 in C. sinensis by Mei et al. (2017), they all belong to the terpene synthase gene and are probably involved in linalool synthesis in C. sinensis. The relative content of geraniol was significantly correlated with the expression of the genes TEA001435 (CAD), TEA002658 (CYP76B6), TEA025455 (CYP76T24), and Camellia_sinensis_newGene_22126 (TPS). It can be seen that the nine genes identified in this study may be key genes for the synthesis of three specific volatiles in tea, and may provide candidate target genes for the subsequent breeding of tea varieties resistant to E. onukii.
Plant volatiles play a crucial role in the host selection of phytophagous insects (Kong and Lou, 2010). Volatiles with luring and repellent effects on phytophagous insects can not only be used to develop specific lures or repellents but also the types and levels of volatiles in plants can be regulated by genetic breeding and genetic engineering technology. The components that lure phytophagous insects can be reduced and the components that repel phytophagous insects can be increased. In addition, the components that lure the natural enemies of phytophagous insects can be amplified. This would maximize the role of plant insect resistance and reduce the damage by phytophagous insects. In this way, we can not only reduce the damage of phytophagous insects by giving full play to plant insect resistance but also protect the ecological environment and achieve the unity of economic, social, and ecological benefits, which is in line with the goal of green and sustainable pest control (Xiang et al., 2015). In this study, Three volatile compounds in tea that have attractive effects on its main pest E. onukii were found, and revealed nine genes potentially related to the synthesis of these volatile compounds. But we still need to do lots of work to verify the function of related genes that regulate the VOCs in three tea species. For example, Bate and Riley (1998) confirmed that the gene ADH1 mutant Arabidopsis thaliana release lower amounts of hexanol compared to wild type plant by transient silencing. Mei et al. (2017) confirmed that genes CsLIS1 and CsLIS2 produced linalool from geraniol diphosphate in C. sinensis by enzyme-catalyzed reaction. In the future, we will further explore the optimal ratio of these three volatiles, and verify the functions of genes related to the synthesis of these specific volatiles by enzyme-catalyzed reaction, transgenic plants and transient expression (or silencing) (Mei et al., 2017). This will contribute to the development of E. onukii specific attractants and the cultivation of tea insect resistant varieties, promote the development of the "push pull" management strategy for this pest, and provide important basis for the green prevention and control of tea garden pests and the protection of the ecological environment (Mei et al., 2017).
Data availability statement
The datasets presented in this study can be found in online repositories. The names of the repository/repositories and accession number(s) can be found below: GenBank, OR179901-OR179909.
Author contributions
J-FH and X-GL designed the study and approved the final draft. FC, PH, and JW conducted the experiments, analyzed the data, and wrote the first draft of the manuscript. WW and Y-WL revised the manuscript and did the supervision. All authors contributed to the article and approved the submitted version.
Funding
This study was financially supported by the Special Fund for Agro-Scientific Research in the Public Interest of Fujian Province (2022R1024003). “5511” Collaborative Innovation Project of High-quality Agricultural Development and Surpassment in Fujian Province (XTCXGC2021011, XTCXGC2021017).
Acknowledgments
We thanks two reviewers for providing constructive feedback. Some knowledge provided by them will help us do better in the future.
Conflict of interest
The authors declare that the research was conducted in the absence of any commercial or financial relationship that could be construed as a potential conflict of interest.
Publisher’s note
All claims expressed in this article are solely those of the authors and do not necessarily represent those of their affiliated organizations, or those of the publisher, the editors and the reviewers. Any product that may be evaluated in this article, or claim that may be made by its manufacturer, is not guaranteed or endorsed by the publisher.
Supplementary material
The Supplementary Material for this article can be found online at: https://www.frontiersin.org/articles/10.3389/fpls.2023.1239237/full#supplementary-material
References
Aharoni, A., Giri, A. P., Verstappen, F. W. A., Bertea, C. M., Sevenier, R., Sun, Z. K., et al. (2004). Gain and loss of fruit flavor compounds produced by wild and cultivated strawberry species. Plant Cell. 16, 3110–3131. doi: 10.1105/tpc.104.023895
Anderson, P., Anton, S. (2014). Experience-based modulation of behavioural responses to plant volatiles and other sensory cues in insect herbivores. Plant Cell Environ. 37, 1826–1835. doi: 10.1111/pce.12342
Bate, N., Riley, J. (1998). Quantitative and qualitative differences in C6-volatile production from the lipoxygenase pathway in an alcohol dehydrogenase mutant of Arabidopsis thaliana. Physiol. Plant 104, 97–104. doi: 10.1034/j.1399-3054.1998.1040113.x.
Benjamini, Y., Hochberg, Y. (1995). Controlling the false discovery rate: a practical and powerful approach to multiple testing. J. R. Stat. Soc B (Methodol.). 57, 289–300. doi: 10.1111/j.2517-6161.1995.tb02031.x
Benjamini, Y., Yekutieli, D. (2001). The control of the false discovery rate in multiple testing under dependency. Ann. Stat. 29, 1165–1188. doi: 10.1214/aos/1013699998
Bernays, E. A., Chapman, R. E. (1994). Host-Plant Selection by Phytophagous Insects (New York: Chapman & Hall Press).
Binder, B. F., Robbins, J. C., Wilson, R. L. (1995). Chemically mediated ovipositional behaviors of the European corn borer, Ostrinia nubilalis (Lepidoptera: Pyralidae). J. Chem. Ecol. 21, 1315–1327. doi: 10.1007/BF02027564
Bruce, T. J. A., Wadhams, L. J., Woodcock, C. M. (2005). Insect host location: a volatile situation. Trends Plant Sci. 10, 269–274. doi: 10.1016/j.tplants.2005.04.003
Chen, F., Li, W., Jiang, L. Z., Pu, X., Yang, Y., Zhang, G. l., et al. (2016). Functional characterization of a geraniol synthase−encoding gene from Camptotheca acuminata and its application in production of geraniol in Escherichia coli. J. Ind. Microbiol. Biotechnol. 43, 1281–1292. doi: 10.1007/s10295-016-1802-2
Chen, Z. M., Xu, N., Han, B. Y., Zhao, D. X. (2003). Chemical communication between tea plant-herbivore-natural enemies. J. Tea Sci. 23, 38–45. doi: 10.3969/j.issn.1000-369X.2003.z1.007
Contreras, C. H., Beaudry, R. (2013). Lipoxygenase-associated apple volatiles and their relationship with aroma perception during ripening. Postharvest Biol. Tec. 82, 28–38. doi: 10.1016/j.postharvbio.2013.02.006
Cook, S. M., Khan, Z. R., Pickett, J. A. (2007). The use of push-pull strategies in integrated pest management. Annu. Rev. Entomol. 52, 375–400. doi: 10.1146/annurev.ento.52.110405.091407
D’Alessandro, M., Turlings, T. C. J. (2005). In situ modification of herbivore-induced plant odors: A novel approach to study the attractiveness of volatile organic compounds to parasitic wasps. Chem. Senses. 30, 739–753. doi: 10.1093/chemse/bji066
D’Alessandro, M., Turlings, T. C. J. (2006). Advances and challenges in the identification of volatiles that mediate interactions among plants and arthropods. Anal. 131, 24–32. doi: 10.1039/b507589k
Dudareva, N., Klempien, A., Muhlemann, J. K., Kaplan, I. (2013). Biosynthesis, function and metabolic engineering of plant volatile organic compounds. ew Phytol. 198, 16–32. doi: 10.1111/nph.12145
Dudareva, N., Negre, F. (2005). Practical applications of research into the regulation of plant volatile emission. Curr. Opin. Plant Biol. 8, 113–118. doi: 10.1016/j.pbi.2004.11.007
Fang, Z. T., Jin, J., Ye, Y., He, W. Z., Shu, Z. F., Shao, J., et al. (2022). Effects of different shading treatments on the biomass and transcriptome profiles of tea leaves (Camellia sinensis L.) and the regulatory effect on phytohormone biosynthesis. Front. Plant Sci. 3. doi: 10.3389/fpls.2022.909765
Fujian Provincial Bureau of Statistics (2023) Fujian statistical yearbook-2022. Available at: https://tjj.fujian.gov.cn/tongjinianjian/dz2022/index.htm (Accessed March 28, 2023).
Goh, H. H., Khairudin, K., SukIran, N. A., Normah, M. N., Baharum, S. N. (2016). Metabolite profiling reveals temperature effects on the VOCs and flavonoids of different plant populations. Plant Biol. 18, 130–139. doi: 10.1111/plb.12403
Han, B. Y., Zhou, C. S. (2004). Attraction effect of main volatile components from tea shoots and flowers on Sphaerophoria menthastri (Diptera: Syrphidae) and Chrysopa septempunctata (Neuroptera: Chrysopidae). Chin. J. Appl. Ecol. 15, 623–626.
Hare, J. D. (2011). Ecological role of volatiles produced by plants in response to damage by herbivorous insects. Annu. Rev. Entomol. 56, 161–180. doi: 10.1146/annurev-ento-120709-144753
He, J., Richard, A. F., Rayko, H., Katrin, L., Tobias, G. K., Mark, H. M., et al. (2019). An unbiased approach elucidates variation in (S)-(+)-linalool, a context-specific mediator of a tri-trophic interaction in wild tobacco. Proc. Natl. Acad. Sci. U.S.A. 116, 14651–14660. doi: 10.1073/pnas.1818585116
Hua, X. (2023) China's tea exports up 1.59 pct in 2022. Available at: https://english.news.cn/20230307/87ee1d55f2f240768c89c39bc4148ca6/c.html (Accessed March 28, 2023).
Huang, X. Z., Xiao, Y. T., Köllner, T. G., Jing, W. X., Kou, J. F., Chen, J. Y., et al. (2018). The terpene synthase gene family in Gossypium hirsutum harbors a linalool synthase GhTPS12 implicated in direct defence responses against herbivores. Plant Cell Environ. 41, 261–274. doi: 10.1111/pce.13088
Iijima, Y., Gang, D. R., Fridman, E., Lewinsohn, E., Pichersky, E. (2004). Characterization of geraniol synthase from the peltate glands of sweet basil. Plant Physiol. 134, 370–379. doi: 10.1104/pp.103.032946
Kang, L., Hopkins, T. L. (2004). Behavior and olfactory responses of plant black mites to plant odors and plant volatile compounds. Chin. Sci. Bull. 49, 81–85. doi: 10.1360/03wc0274
Kim, C. Y., Khan, F., Kim, Y. (2023). A pushpull strategy to control the western flower thrips, Frankliniella occidentalis, using alarm and aggregation pheromones. PLoS One 18, e0279646. doi: 10.1371/journal.pone.0279646
Li, X. W., Cheng, J. H., Han, H. B., Lü, Y. B. (2022a). Behavioral manipulation of the plant secondary metabolites to thrips and their application in thrips management. Acta Entomol. Sin. 65, 1222–1246. doi: 10.16380/j.kcxb.2022.09.015
Li, Y., Tian, S. F., Wang, C. X., Su, H., Li, K., Huang, L. Q. (2022b). Effects of different leaf-berry ratio on volatile aroma synthesis of 'Muscat Hamburg' grape fruits. Wine. 245, 59–65. doi: 10.13414/j.cnki.zwpp.2022.05.009
Lian, M., Lv, S. D., Wu, Y. S., He, Y. L., Zhou, J. S., Meng, Q. X. (2014). Progresses on the formation mechanism of the volatile component in tea. Life Sci. Res. 18, 550–556. doi: CNKI:SUN:SMKY.0.2014-06-016
Lin, Y. W., Lin, S., Akutse, K. S., Hussain, M., Wang, L. D. (2016). Diaphorina citri induces huanglongbing-infected citrus plant volatiles to repel and reduce the performance of Propylaea japonica. Front. Plant Sci. 7. doi: 10.3389/fpls.2016.01969
Livak, K. J., Schmittgen, T. D. (2001). Analysis of relative gene expression data using real-time quantitative PCR and the 2−ΔΔCT method. Methods. 25, 402–408. doi: 10.1006/meth.2001.1262
Magnard, J. L., Roccia, A., Caissard, J. C., Vergne, P., Sun, P., Hecquet, R., et al. (2015). Biosynthesis of monoterpene scent compounds in roses. Science. 81, 349. doi: 10.1126/science.aab0696
Martel, J. W., Alford, A. R., Dickens, J. C. (2005). Syntheitc host volatiles increase efficacy of trap cropping for management of Colorado potato beetle, Leptinotarsa decemlineata (Say). Agric. Forset Entomol. 7, 79–86. doi: 10.1111/j.1461-9555.2005.00248.x
Mei, X., Liu, X., Zhou, Y., Wang, X., Zeng, L., Fu, X., et al. (2017). Formation and emission of linalool in tea (Camellia sinensis) leaves infested by tea green leafhopper (Empoasca (Matsumurasca) onukii Matsuda). Food Chem. 237, 356–363. doi: 10.1016/j.foodchem.2017.05.124
Mody, K., Collatz, J., Dorn, S. (2015). Plant genotype and the preference and performance of herbivores: Cultivar affects apple resistance to the florivorous weevil Anthonomus pomorum. Agr. For. Entomol. 17, 337–346. doi: 10.1111/afe.12112
Mu, D., Cui, L., Ge, J., Wang, M. X., Liu, L. F., Yu, X. P. (2012). Behavioral responses for evaluating the attractiveness of specific tea shoot volatiles to the tea green leafhopper Empoasca vitis. Insect Sci. 19, 229–2238. doi: 10.1111/j.1744-7917.2011.01476.x
Nagegowda, D. A., Gutensohn, M., Wilkerson, C. G., Dudareva, N. (2008). Two nearly identical terpene synthases catalyze the formation of nerolidol and linalool in snapdragon flowers. Plant J. 55, 224–239. doi: 10.1111/j.1365-313X.2008.03496.x
National Bureau of Statistics (2023) China Statistical Yearbook-2022. Available at: https://data.stats.gov.cn/english/easyquery.htm?cn=C01 (Accessed March 28, 2023).
Niu, Y., Han, S., Wu, Z., Pan, C., Wang, M., Tang, Y., et al. (2022). A push-pull strategy for controlling the tea green leafhopper (Empoasca flavescens F.) using semiochemicals from Tagetes erecta and Flemingia macrophylla. Pest Manag. Sci. 78, 2161–2172. doi: 10.1002/ps.6840
Poelman, E. H., Bruinsma, M., Zhu, F., Weldegergis, B. T., Boursault, A. E., Jongema, Y., et al. (2012). Hyperparasitoids use herbivore-induced plant volatiles to locate their parasitoid host. PLoS Biol. 10, e1001435. doi: 10.1371/journal.pbio.1001435
Qin, J. D., Wang, C. Z. (2001). The relation of interaction between insects and plants to evolution. Acta Entomol. Sin. 44, 360–365.
Riffell, J. A., Lei, H., Christensen, T. A., Hildebrand, J. G. (2009). Characterization and coding of behaviorally significant odor mixtures. Curr. Biol. 19, 335–340. doi: 10.1016/j.cub.2009.01.041
Roseland, C. R., Bates, M. B., Carlson, R. B., Oseto, C. Y. (1992). Discrimination of sunflower volatiles by the red sunflower seed weevil. Entomol. Exp. Appl. 62, 99–106. doi: 10.1111/j.1570-7458.1992.tb00648.x
Schilmiller, A. L., Schauvinhold, I., Larson, M., Xu, R., Charbonneau, A. L., Schmidt, A., et al. (2009). Monoterpenes in the glandular trichomes of tomato are synthesized from a neryl diphosphate precursor rather than geranyl diphosphate. P. Natl. Acad. Sci. U.S.A. 106, 10865–10870. doi: 10.1073/pnas.090411310
Schoonhoven, L. M., Loon, J. J. A. V., Dicke, M. (2005). Insect-plant biology (Oxford: Oxford University Press).
Sharma, G., Malthankar, P. A., Mathur, V. (2021). Insect-plant interactions: A multilayered relationship. Ann. Entomol. Soc. Am. 114, 1–16. doi: 10.1093/aesa/saaa032
Simkin, A. J., Miettinen, K., Claudel, P., Burlat, V., Guirimand, G., Courdavault, V., et al. (2012). Characterization of the plastidial geraniol synthase from Madagascar periwinkle which initiates the monoterpenoid branch of the alkaloid pathway in internal phloem associated parenchyma. Phytochemistry 85, 36–43. doi: 10.1016/j.phytochem.2012.09.014
Sun, J. G., Huang, L. Q., Wang, C. Z. (2012). Electrophysiological and behavioral responses of Helicoverpa assulta (Lepidoptera: Noctuidae) to tobacco volatiles. Arthropod-Plant Inte. 6, 375–384. doi: 10.1007/s11829-012-9190-7
Sun, Z. X., Song, Y. Y., Zeng, R. S. (2019). Advances in studies on intraspecific and interspecific relationships mediated by plant volatiles. J. South China Agric. Univ. 40, 166–174. doi: 10.7671/j.issn.1001-411X.201905078
Tholl, D. (2006). Terpene synthases and the regulation, diversity and biological roles of terpene metabolism. Curr. Opin. Plant Biol. 9, 297–304. doi: 10.1016/j.pbi.2006.03.014
Tingle, F. C., Mitchell, E. R., Heath, R. R. (1990). Preferences of mated Heliothis virescens and H. subflexa females for host and nonhost volatiles in a flight tunnel. J. Chem. Ecol. 16, 2889–2898. doi: 10.1007/BF00979481
Vallarino, J. G., Erban, A., Fehrle, I., Fernie, A. R., Kopka, J., Osorio, S. (2018). “Acquisition of volatile compounds by gas chromatography–mass spectrometry (GC-MS),” in Plant metabolomics, vol. 1778 . Ed. António, C. (New York, NY: Humana Press). Available at: doi: 10.1007/978-1-4939-7819-9_16.
Verkerk, R. H. J., Wright, D. J. (1994). Interactions between the diamondback moth, Plutella xylostella L. and glasshouse and outdoor-grown cabbage cultivars. Ann. Appl. Biol. 125, 477–488. doi: 10.1111/j.1744-7348.1994.tb04985.x
Wang, Q. H., Gao, J. H., Wang, L., Wu, W. J., Guo, J. X., Wu, G. L., et al. (2022). Cloning and expression analysis of Binzi LOX2a. J. Fruit Sci. 39, 1977–1988. doi: 10.13925/j.cnki.gsxb.20220444
Wang, M. X., Li, H. X., Wu, W. Z., Sun, H. C., Shi, S. P., Ding, Y., et al. (2016). Behavioral responses of Empoasca vitis Göthe to volatiles from tea shoots. Chin. J. Appl. Entomol. 53, 507–515. doi: 10.7679/j.issn.2095-1353.2016.066
Wang, R., Yang, Y., Jing, Y., Segar, S. T., Zhang, Y., Wang, G., et al. (2021). Molecular mechanisms of mutualistic and antagonistic interactions in a plant-pollinator association. Nat. Ecol. Evol. 5, 974–986. doi: 10.1038/s41559-021-01469-1
Xiang, Y. Y., Liu, T. X., Zhang, S. Z. (2015). Effect and application of plant volatiles on host selecting behavior of phytophagous insects. J. Anhui Agric. Sci. 43, 92–94. doi: CNKI:SUN:YXSG.0.2015-08-002
Yu, F. N., Utsumi, R. (2009). Diversity, regulation, and genetic manipulation of plant mono and sesquiterpenoid biosynthesis. Cell. Mol. Life Sci. 66, 3043–3052. doi: 10.1007/s00018-009-0066-7
Zhang, H., Li, H. L., Wang, D. F., Wu, G. Y., Wang, Q. S. (2020a). Toxicity activity of Artemisia lavandulaefolia DC essential oil on Empoasca onukii Matsuda. Acta Tea Sin. 61, 183–186.
Zhang, H., Li, H. L., Wang, D. F., Wu, G. Y., Wang, Q. S. (2022). Effects of Artemisia argyi volatiles on the behavior of the tea green leafhopper (Empoasca onukii) in tea plantations. Chin. J. Appl. Entomol. 59, 773–784. doi: 10.7679/j.issn.2095-1353.2022.080
Zhang, Z. Q., Luo, Z. X., Gao, Y., Bian, L., Sun, X. L., Chen, Z. M. (2014). Volatiles from non-host aromatic plants repel tea green leafhopper Empoasca vitis. Entomol. Exp. Appl. 153, 156–169. doi: 10.1111/eea.12236
Zhang, X. T., Wang, G., Zhang, S. C., Chen, S., Wang, Y. B., Wen, P., et al. (2020b). Genomes of the Banyan tree and pollinator wasp provide insights into fig-wasp coevolution. Cell. 183, 875–889.e17. doi: 10.1016/j.cell.2020.09.043
Zhao, D. X., Gao, J. L., Chen, Z. M., Cheng, J. A., Xu, H. H. (2002). Orientation response of Empoasca vitis to tea shoots volatiles. J. South China Agric. Univ. 23, 27–29. doi: 10.7671/j.issn.1001-411X.2002.04.008
Keywords: Camellia sinensis, volatile organic compounds (VOCs), Empoasca onukii, behavioral preference, VOC synthesis-related genes
Citation: Chen F, Huang P, Wang J, Wu W, Lin Y-W, Hu J-F and Liu X-G (2023) Specific volatiles of tea plants determine the host preference behavior of Empoasca onukii. Front. Plant Sci. 14:1239237. doi: 10.3389/fpls.2023.1239237
Received: 14 June 2023; Accepted: 07 August 2023;
Published: 31 August 2023.
Edited by:
Islam S. Sobhy, Cardiff University, United KingdomReviewed by:
Yifan Jiang, Nanjing Agricultural University, ChinaYiwei Zhou, Guangdong Academy of Agricultural Sciences (GDAAS), China
Copyright © 2023 Chen, Huang, Wang, Wu, Lin, Hu and Liu. This is an open-access article distributed under the terms of the Creative Commons Attribution License (CC BY). The use, distribution or reproduction in other forums is permitted, provided the original author(s) and the copyright owner(s) are credited and that the original publication in this journal is cited, in accordance with accepted academic practice. No use, distribution or reproduction is permitted which does not comply with these terms.
*Correspondence: Jin-Feng Hu, aHVqaW5mZW5nMDA3QHNpbmEuY29t; Xin-Gang Liu, bGl1eGluZ2FuZ0BjYWFzLmNu
†These authors have contributed equally to this work and share first authorship