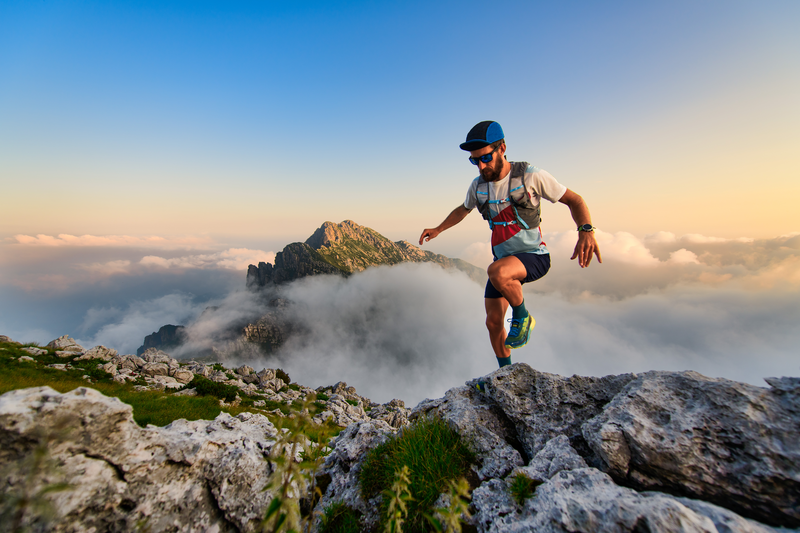
94% of researchers rate our articles as excellent or good
Learn more about the work of our research integrity team to safeguard the quality of each article we publish.
Find out more
ORIGINAL RESEARCH article
Front. Plant Sci. , 10 August 2023
Sec. Plant Biotechnology
Volume 14 - 2023 | https://doi.org/10.3389/fpls.2023.1236838
Passion fruit (Passiflora edulis) is a perennial evergreen vine that grows mainly in tropical and subtropical regions due to its nutritional, medicinal and ornamental values. However, the molecular biology study of passion fruit is extremely hindered by the lack of an easy and efficient method for transformation. The protoplast transformation system plays a vital role in plant regeneration, gene function analysis and genome editing. Here, we present a new method (‘Cotyledon Peeling Method’) for simple and efficient passion fruit protoplast isolation using cotyledon as the source tissue. A high yield (2.3 × 107 protoplasts per gram of fresh tissues) and viability (76%) of protoplasts were obtained upon incubation in the enzyme solution [1% (w/v) cellulase R10, 0.25% (w/v) macerozyme R10, 0.4 M mannitol, 10 mM CaCl2, 20 mM KCl, 20 mM MES and 0.1% (w/v) BSA, pH 5.7] for 2 hours. In addition, we achieved high transfection efficiency of 83% via the polyethylene glycol (PEG)-mediated transformation with a green fluorescent protein (GFP)-tagged plasmid upon optimization. The crucial factors affecting transformation efficiency were optimized as follows: 3 μg of plasmid DNA, 5 min transfection time, PEG concentration at 40% and protoplast density of 100 × 104 cells/ml. Furthermore, the established protoplast system was successfully applied for subcellular localization analysis of multiple fluorescent organelle markers and protein-protein interaction study. Taken together, we report a simple and efficient passion fruit protoplast isolation and transformation system, and demonstrate its usage in transient gene expression for the first time in passion fruit. The protoplast system would provide essential support for various passion fruit biology studies, including genome editing, gene function analysis and whole plant regeneration.
Passion fruit (Passiflora edulis), also known as passiflora, is a perennial evergreen vine that grows mainly in tropical and subtropical regions due to its nutritional, medicinal and ornamental values. It belongs to the genus Passiflora, the largest genus in the Passifloraceae family, and originated in South America (Fischer and Rezende, 2008; Xia et al., 2021; Yu et al., 2021; Phong et al., 2022). Passion fruit has been gaining popularity as a flavor in both drinks and foods as it’s rich in vitamins, antioxidants, and plant compounds that could benefit human health. In China, it is widely distributed in several provinces with warm and humid climates, including Hainan, Taiwan, Fujian, Yunnan, Guangxi and Guizhou. In the past decades, passion fruit plantations soared in China as an economic investment (Yu et al., 2021). However, the molecular biology study of passion fruit falls far behind. It was not until 2021 that a chromosome-scale genome assembly of passion fruit (Passiflora edulis Sims) was just reported (Xia et al., 2021). This work enables several documentations for the identification of passion fruit genes, including eceriferm (CER), aquaporin (AQP), β-Ketoacyl-CoA synthase (KCS), peroxidase (POD), lateral organ boundary domain (LBD), and lipoxygenase (LOX) gene families (Huang et al., 2022; Liang et al, 2022; Liang et al, 2022; Rizwan et al., 2022a; Rizwan et al., 2022b; Song et al., 2022). However, one of the major barriers in gene functional studies is the lack of a simple and efficient transformation system in passion fruit.
Plant transformation is a fundamental and powerful tool for molecular plant biology studies. There are two types of plant transformation: stable transformation and transient transformation. The Agrobacterium-mediated transformation has been extensively used to generate transgenic plants. For passion fruit, G. Manders and his colleagues generated the first transgenic passion fruit plants by Agrobacterium tumefaciens-mediated transformation using leaf as explant in 1994 (Manders et al., 1994). After that, many groups worldwide have reported the successful in vitro regeneration and Agrobacterium-mediated transformation of passion fruit (Trevisan et al., 2006; Correa et al., 2015; Rizwan et al., 2021) The Agrobacterium-mediated transformation method represents a powerful platform for transgenic passion fruit lines, but exhibits the disadvantages of time-consuming, relatively low transformation efficiency and inconvenience for large-scale gene functional analyses. Alternatively, transient gene expression represents a rapid and high-throughput method for gene functional studies (Zhao et al., 2016). The most common transformation methods for transient gene expression include protoplast transformation, biolistic bombardment and Agrobacterium tumefaciens-mediated transformation (Pitzschke and Persak, 2012). Although protoplast transformation has been widely used for transient gene expression analysis in a large number of plants, including Arabidopsis, maize and rice, there is currently no report for transient gene expression in passion fruit.
Plant protoplasts are the plant single cells excluding the rigid cell wall, and serve as a versatile single-cell-based system for transient gene expression (Davey et al., 2005; Dai and Wang, 2022; Wang et al., 2023). Foreign plasmid DNA of interest can be easily delivered into protoplasts typically by polyethylene glycol (PEG)-mediated transformation, electroporation and microinjection (Yoo et al., 2007; Priyadarshani et al., 2018; Dai and Wang, 2022). Since the first report of successful plant protoplasts isolation from tomato seedlings in 1960 by Cocking (Cocking, 1960), the protoplast transformation system has been extensively applied in transient gene expression such as intracellular localization, protein-protein interaction and genome editing studies (Davey et al., 2005; Yu et al., 2017; Priyadarshani et al., 2018). This system relies on efficient protoplast isolation from plant tissue and successful downstream transformation. Multiple parameters can influence the transformation efficiency of PEG-mediated transformation, including incubation time, PEG concentration, protoplast density and plasmid amount.
The aim of this study was to establish a platform for passion fruit transient gene expression. Owing to the wealth of knowledge of plant protoplast for transient gene expression, the protoplast system would likely provide a feasible way for transformation in passion fruit. Toward this goal, we report here a simple method of using peeled seedling cotyledon for passion fruit protoplast isolation and achieved high transfection efficiency of 83% upon optimization. The simple and efficient protoplast transformation system can be broadly applied in transient gene expression, illustrated by intracellular localization analysis of multiple fluorescent markers as well as protein-protein interaction study. This is the first study to describe protoplast transformation system for transient gene expression in passion fruit. The significance of these results for promoting the molecular biology study of passion fruit is discussed.
Passion fruit (Passiflora edulis) seeds were placed in the soil at a depth of 1-2 cm and germinated after 7-10 days with 16 hours light/8 hours dark regime at 28±2°C. The cotyledons were fully expanded after approximately 7 days upon germination and could be readily used for protoplast isolation.
The green fluorescent protein (GFP)-expressing plasmid pGreen0029-GFP was a gift from Dr. Ji Li of Nanjing Agriculture University, China. H2B-RFP and ER-mCherry-HDEL were obtained from Dr. Guanwei Wu of Ningbo University, China.
YN-CP and YC-CP are constructed by the following methods: The full length of CP gene (GenBank: MG944249.2) was amplified from the infectious cDNA clone of telosma mosaic virus (TelMV) using PCR with gene-specific primers (CP-F: 5’ GGGGACAAGTTTGTACAAAAAAGCAGGCTT CATGTCTGGAAAGGTTGATGATG3’; CP-R: 5’GGGGACCACTTTGTACAAGAAAGCTGGG TCCTGCACAGAACCTACTCC3’) and subcloned into the Gateway entry vector pDONR 221 (Invitrogen) by BP reaction and finally recombined into the destination vector pEarleyGate 201-nYFP and pEarleyGate 202-cYFP vector (Dai et al., 2020) by LR reaction, respectively.
All the constructed plasmids are validated by double digestion and sequencing. Plasmids were extracted using the Maxi Plasmid Kit Endotoxin Free (Geneaid) according to the manufacturer’s instructions.
The fully expanded cotyledons were attached to the 3M Masking tape with the upper side facing down and peeled away the lower epidermal surface by directly pulling the cotyledons. The peeled cotyledons were further immersed in the enzyme solution [1% (w/v) cellulase R10 (Yakult Pharmaceutical Ind. Co., Ltd., Japan), 0.25% (w/v) macerozyme R10 (Yakult), 0.4 M mannitol, 10 mM CaCl2, 20 mM KCl, 20 mM MES and 0.1% (w/v) BSA, pH 5.7] in a Petri dish (Wu et al., 2009; Dai and Wang, 2022). The peeled cotyledons were incubated for 2-4 hours with gently shake (40 rpm on a platform shaker) in the dark at room temperature (25 °C) for releasing protoplasts and were followed by rinsing using the iced W5 (154 mM NaCl, 125 mM CaCl2, 5 mM KCl, 5 mM Glucose, 2 mM MES, pH 5.7).
Passion fruit protoplasts were then transferred to round-bottom centrifuge tubes by passing through a 100 μm nylon mesh filter and pelleted for 3 min at 100 ×g. The pelleted protoplasts were washed with ice-cold W5 once. Re-suspended the protoplasts in 300 μl W5 and rest on ice for 30 min. Finally, resuspended the protoplasts in MMg solution (0.4 M mannitol, 15 mM MgCl2, 4 mM MES, pH 5.7) at a density of 100 × 104 cells /ml at room temperature.
We adapted the classic PEG-mediated protoplast transformation method for passion fruit protoplast transformation (Dai and Wang, 2022). Specifically, 30 μl of freshly isolated protoplasts and 3 μg of plasmid were mixed in a 2 ml round-bottom centrifuge tube. Then, an equal volume of freshly made PEG transfection solution (40% (w/v) PEG 4000, 0.1 M CaCl2, 0.2 M mannitol) were added.Samples were mixed well by gentle swirling and incubated for a certain time at room temperature. W5 was added to stop the transformation and subjected to centrifugation for 2 min at 100 g. Protoplasts were washed again using W5 and finally stored in 50 μl of W5 at room temperature in the dark.
Protoplast yield calculation and viability assessment were performed as described in our previous paper (Wang et al., 2023)
The protoplasts were observed under a fluorescent microscope (OLYMPUS DP80, Japan). For detailed visualization of GFP, organelle markers, BiFC and chloroplast auto-fluorescence, the transformed protoplasts were observed under a confocal microscope (OLYMPUS FV1000, Japan). Excitation wavelengths and emission filters were 488 nm/bandpass 500-520 nm for GFP, 514 nm/band-pass 510-546 nm for YFP, 543 or 563 nm/bandpass 580-620 nm for RFP, and 488 nm/band-pass 680-720 nm for chloroplast auto-fluorescence.
Statistical differences between samples were determined by the unpaired two-tailed Student’s t-test and considered significant at P < 0.05. Data are presented as means ± SD of the mean from at least three experiments.
To establish a simple and efficient protoplast isolation protocol from fresh tissue of passion fruit, the fully expanded cotyledons of a 15-day-old passion fruit seedling were selected for the source material (Figures 1A, B). Upon peeling off the lower epidermis (Figure 1C), the cotyledons were incubated with the enzyme solution for 2-4 hours (Figures 1D, E) and the solution appeared green upon rinsing (Figure 1F), indicating protoplasts were released. The passion fruit protoplasts were harvested essentially following the protocol developed for Arabidopsis protoplast isolation with a few modifications (Yoo et al., 2007) (Figure 1G). With the current protocol, a high yield of protoplasts (2.3 × 107 protoplasts per gram of fresh seedling cotyledons) was achieved. The isolated passion fruit protoplast remains round-shape and intact under the light microscope (Figure 1H). Furthermore, the protoplast viability test revealed that the purified protoplasts remain viable upon fluorescein diacetate (FDA) staining (Figure 1I). We were able to achieve an average viability of passion fruit protoplast of 76%. These data demonstrated that the seedling cotyledon served as an excellent protoplast source and we have established a simple and efficient protoplast isolation system for passion fruit.
Figure 1 Schematic illustration of passion fruit protoplast isolation from seedling cotyledons. (A) A healthy 15-day-old passion fruit seeding plant for cotyledon protoplasts isolation. Arrows indicate the cotyledons to be detached for protoplast isolation. Scale bar, 2 cm; (B) Detached cotyledon attached to masking tape with lower side facing up; (C) Cotyledon with the lower epidermal surface been pulled away; (D) Peeled cotyledon sections incubated with the enzyme solution in a petri dish; (E) Cotyledon after digested with enzyme solution; (F) Released protoplasts in the enzyme solution after digestion; (G) Protoplasts pellet upon low-speed centrifugation; (H) Intact protoplasts in MMg solution under a light microscope after protoplasts purification. Scale bar, 100 µm; (I) Fluorescence micrograph of protoplasts emitting green fluorescence upon FDA staining. Scale bar, 100 µm.
Next, we adapted the classic polyethylene-glycol (PEG)-mediated technique (Dai and Wang, 2022) for passion fruit protoplast transformation. A green fluorescent protein (GFP)-tagged plasmid driven by the constitutive CaMV35S promoter (pGreen0029-GFP, 6.0 kb) was used to deliver into passion fruit protoplast to determine the transformation efficiency using a fluorescent microscope. To obtain relatively higher transformation efficiency, various transformation parameters, including plasmid DNA amount, protoplast density, PEG 4000 concentration and incubation time were optimized accordingly.
The first variable optimized was the plasmid DNA amount of pGreen0029-GFP. A series of plasmid amounts (0.5, 1, 3, and 6 μg, respectively) was applied to identify the optimal amount of DNA required for PEG-mediated transformation. When using 0.5 μg plasmid for transformation, very few protoplasts exhibited green fluorescence under a fluorescent microscope at 24 hours-post transfection (hpt) (Figure 2A) and the transfection efficiency was only 12% on average (Figure 2B). By contrast, the transfection efficiency increased to 30% and 74% as the plasmid amount increases to 1 and 3 μg, respectively (Figure 2B). When using higher plasmid amount of 6 μg, the transfection efficiency remains high (71%), but no significant difference compared with that of 3 μg (p= 0.45) (Figure 2B). Thus, we conclude that 3 μg of plasmid is the optimal amount per 30 μl protoplasts transformation reaction.
Figure 2 Effect of plasmid amount and PEG 4000 concentration on passion fruit protoplast transfection. (A) Microscopic images of passion fruit protoplasts transfected with different amounts of GFP-expressing plasmid (0.5, 1, 3, and 6 μg, respectively). Scale bar, 50 μm. (B) The transformation efficiency of passion fruit protoplasts using different amounts of plasmids. Bars represent standard errors. Different letters indicate a statistically significant difference at P < 0.05 among samples according to Duncan’s multiple range tests. (C) Microscopic images of passion fruit protoplasts transfected with a GFP-expressing plasmid using varying concentrations of PEG 4000. Scale bar, 50 μm. (D) The transformation efficiency of passion fruit protoplasts at various concentrations of PEG4000. Different letters indicate a statistically significant difference at P < 0.05 among samples according to Duncan’s multiple range tests. Bars represent standard errors.
The second variable optimized was the protoplast density (50, 100, 150 and 200 × 104 cells/ml, respectively). We found that low protoplast density at 50 cells/ml produced a transformation efficiency of about only 42% (Figures 2C, D). By contrast, the highest transformation efficiency (70 ± 1 %) was achieved with the protoplast density at 100 × 104 cells/ml (Figures 2C, D). Further increasing the protoplast density to 150 × 104 cells/ml did not increase the transformation efficiency (p= 0.06) (Figure 2D). In addition, a higher protoplast density of 200 × 104 cells/ml resulted in a significant reduction (p= 0.0001) than that of 100 × 104 cells/ml. Hence, we conclude that protoplast density at 100 × 104 cells/ml is optimal in the current protocol.
We next examined the effect of PEG concentration on the transformation efficiency of passion fruit protoplasts. A series of PEG 4000 concentrations (20, 30, 40, 50 and 60%, respectively) was set to test the protoplast transformation efficiency. Based on the results shown in Figures 3A, B, transfection efficiency increases significantly as the PEG concentration raise from 20% to 30% and 40%, and reaches a peak of approximately 83% transfection rate at 40% PEG. However, further increasing the PEG concentration from 40% to 50% and 60% gradually reduced the transfection rate. These data demonstrated that the optimal PEG 4000 concentration is 40% for the PEG-based transformation of passion fruit protoplast.
Figure 3 Effect of protoplast density and incubation time on passion fruit protoplast transfection. (A) Microscopic images of passion fruit protoplasts transfected with a GFP-expressing plasmid at different densities of protoplast. Scale bar, 50 μm. (B) Effect of protoplast density on the transformation efficiency of passion fruit protoplasts. Different letters indicate a statistically significant difference at P < 0.05 among samples according to Duncan’s multiple range tests. Bars represent standard errors. (C) Microscopic images of passion fruit protoplasts transfected with a GFP-expressing plasmid at varying incubation time. Scale bar, 50 μm. (D) Effect of incubation time on the transformation efficiency of passion fruit protoplasts. Different letters indicate a statistically significant difference at P < 0.05 among samples according to Duncan’s multiple range tests. Bars represent standard errors.
The last factor to be optimized is the incubation time (2, 5, 10, 15, and 20 min, respectively). As shown in Figures 3C, D, the highest transfection efficiency (75 ± 2 %) was achieved upon incubation with PEG-calcium transfection solution for 5 min, although there is no significant difference between 5 min and 10 min (P=0.83). Further prolongation of incubation time to 15 min and 20 min significantly decreased the transfection rate to only 59% and 46%, respectively. Therefore, 5 min was taken as the optimal transfection time for PEG-mediated transformation in passion fruit.
Upon all these optimizations, we have successfully established the highly-efficient passion fruit protoplast transformation illustrated by the following points: 1) GFP expression was visualized at 24 hpt under a fluorescence microscope (Figure 4A). Moreover, confocal laser scanning microscopy (CLSM) revealed that GFP localized both in the nucleus and cytoplasm (Figure 4B), suggesting GFP was successfully expressed in the passion fruit protoplasts; 2) the optimal method was found to be inoculation of 3 μg plasmid DNA for 5 min with PEG concentration of 40% at the protoplast density of 100 × 104 cells/ml (Figures 2, 3); 3) Using this method, a high transformation efficiency of 83% was achieved in passion fruit cotyledon protoplasts (Figure 3B).
Figure 4 Highly efficient PEG-mediated transformation of passion fruit protoplasts. (A) Highly efficient PEG-mediated transformation of passion fruit protoplast with a transformation efficiency of 71.7%, transformed with a green fluorescent protein (GFP)-expressing plasmid (pGreen0029-GFP, 6.0 kb) at 24 h post-transfection (hpt). Scale bar, 100 μm; (B) Closed view of GFP expression in passion fruit protoplast under a confocal microscope at 24 hpt. Scale bar, 20 μm.
To assess the suitability of passion fruit cotyledon protoplasts for intracellular localization studies, we used two fluorescent organelle markers for protoplast transformation, including a nucleus marker (H2B-RFP) and an endoplasmic reticulum marker (ER-mCherry-HDEL). At 48 hpt, confocal laser scanning microscopy (CLSM) measurements revealed the correct observation of predicted fluorescence in the corresponding organelles (Figure 5), suggesting that the cotyledon protoplast serves as an excellent platform for the subcellular localization studies in passion fruit.
Figure 5 Subcellular localization of various organelle markers. Upper panel: confocal microscopy images of endoplasmic reticulum (ER) marker tagged with mCherry-HDEL at 48 hpt. Scale bar, 20 μm; Lower panel: confocal microscopy images of nucleus marker (H2B protein) tagged with red fluorescent protein (RFP) at 48 hpt. Scale bar, 20 μm.
Further, the passion fruit protoplast system was applied to investigate protein-protein interactions using the bimolecular fluorescence complementation (BiFC) assay. The viral coat proteins (CPs) have been well known as capable of self-interactions. In this study, the cp gene of telosma mosaic virus (TelMV) was cloned into Gateway-based BiFC vectors (YN and YC), respectively (Figure 6A) and subsequently delivered into passion fruit protoplasts. As shown in Figure 6B, co-expression of YN-CP and YC-CP resulted in strong yellow fluorescence throughout the cytoplasm under a confocal microscope, consistent with the CP localization of other potyviruses in intact plant cells (Dai et al., 2020). By contrast, we did not observe any yellow fluorescence in the passion fruit protoplasts transformed with the negative controls YN-CP/YC or YN/-YC-CP. These results demonstrated that the cotyledon protoplast was suitable for protein-protein interaction studies in passion fruit.
Figure 6 Bimolecular fluorescence complementation (BiFC) analysis of coat protein (CP) of telosma mosaic virus in passion fruit protoplast. (A) The schematic illustration of BiFC constructs for viral coat protein expression in passion fruit protoplast; (B) BiFC analysis of CP-CP interactions in passion fruit protoplasts. Construct pair of YN-CP/YC-CP was transiently co-expressed in passion fruit protoplasts. Combinations of YN-CP/YC and YC-CP/YN serve as the negative controls. Experiments were repeated three times with similar results. Scale bar, 20 μm.
Herein, we successfully isolated the cotyledon protoplasts from healthy passion fruit seedlings with high yield (2.3 × 107 protoplasts per gram of fresh tissues) and viability (76%) (Figure 1). With optimization of various transformation parameters, including plasmid amount, protoplast density, PEG concentration and inoculation time, we were able to achieve high transformation efficiency of 83%, delivering a GFP-expressing plasmid DNA into the protoplast through PEG-mediated transformation (Figure 2-4). Furthermore, the passion fruit protoplast system was successfully applied in subcellular localization and protein-protein interaction studies (Figures 5, 6).
Traditionally, the leaf serves as the most common source tissue for protoplast isolation of a large number of species, including the model plants Arabidopsis, tobacco, maize, and rice (Ren et al., 2021). However, cotyledon has also been applied in successful protoplast isolation for several species, such as cabbage, lettuce, Malus Pumila Mill and Ricinus communis L (Reed and Bargmann, 2021; Ren et al., 2021; Xu et al., 2021). In the present paper, cotyledon was demonstrated to serve as an excellent source tissue for passion fruit isolation. The protoplast yield was 2.3 × 107 g−1FW and is relatively higher than that of Malus Pumila Mill (3.72 × 106 g−1FW) and Ricinus communis L (6.1 × 106 g−1FW). The protoplast viability is also comparable to that of the later two species (76% VS 80% and 85%, respectively) (Xu et al., 2021).
In the classic method of protoplast isolation from the model plants Arabidopsis and tobacco, selected leaves were sliced into 0.5-1mm-strips followed by vacuumizing processes (Yoo et al., 2007; Wu et al., 2009). This is also the case for passion fruit, where two independent groups sliced the source tissues into strips for enzyme digestion and protoplast isolation in 1993 (d'Utra Vaz et al., 1993; Dornelas and Vieira, 1993). By contrast, our so-called ‘Cotyledon peeling method’ eliminates the slicing and vacuumizing processes by attaching the cotyledon to the 3M Masking tape with the upper side facing down, and directly peeling away the lower epidermis (Figure 1). It requires less equipment, time and experimental skills. Furthermore, upon the lower epidermis directly peeling away, it allows the enzyme solution to get much easier access to the mesophyll cells and the intercellular spaces. This method offers great potential for the protoplast isolation of other species in the Passifloraceae family.
Previously, plasmid amount has been revealed to affect protoplast transformation efficiency in various species, including grapevine and camellia (Zhao et al., 2016; Li et al., 2022). The trend of passion fruit transformation efficiency upon adding different amounts of plasmid is raising first and then declining (Figure 2A, B), which is consistent with that of grape and camellia protoplast. We questioned if this may be partly due to the impurities of the transformation mixture as increasing the plasmid brings in water. However, the plasmid DNA required for optimal passion fruit protoplast transfection was about twofold lower (Wang et al., 2015; Zhao et al., 2016; Li et al., 2022). In this study, we found that a passion fruit protoplast density of 1.0 × 106 cells/ml resulted in the best transformation efficiency (Figures 2C, D), which is similar to maize and areca palm (Gentzel et al., 2020; Wang et al., 2023). When the protoplast density exceeds 1.0 × 106 cells/ml, transfection efficiency decreased, this might be explained by the increased densities resulted in cell debris, as shown in Figure 2C. Different from this, the protoplast density is adjusted to 2-5 × 105 cells/ml in the classic protoplast system of Arabidopsis and tobacco (Yoo et al., 2007; Wu et al., 2009). PEG concentration has been reported to play a major role in protoplast transfection. In our experiment, we found that 40% PEG concentration was optimal for the transformation of passion fruit protoplast (Figures 3A, B). We have to mention that 40% PEG is the most often PEG concentration used for model plants, including Arabidopsis and tobacco (Yoo et al., 2007; Wu et al., 2009). Our result is also consistent with the detailed optimization of protoplast transformation for multiple species, including Camellia oleifera (Li et al., 2022) and Chinese cabbage (Sivanandhan et al., 2021). Different from this, in Magnolia and Freesia protoplast transformation, 20% was the optimal PEG concentration (Shen et al., 2017; Shan et al., 2019). The difference could be due to different species used for protoplast isolation and/or the physiological status of the plants used. The optimal incubation time for protoplast transfection typically ranges from 2 to 40 mins, depending on the plant species. For example, the optimal incubation time for grapevine, Magnolia and cassava protoplast was 2 min, 5 min and 10 min respectively. (Zhao et al., 2016; Shen et al., 2017; Wu et al., 2017). In our system, the most effective transfection time for passion fruit protoplast is 5 min (Figures 3C, D), which is consistent with the optimization results for Magnolia (Shen et al., 2017), but different from Camellia oleifera, Chinese cabbage and cassava (Wu et al., 2017; Sivanandhan et al., 2021; Li et al., 2022). Although PEG exhibits a very low degree of toxicity to cells, it could be toxic at high concentrations and prolonged incubation time (Boni and Hui, 1987; Wang et al., 2021). This could possibly explain why protoplast transfection efficiency was hindered at PEG concentration at 50% and 60%, and incubation time exceeding 10 min in the present study.
Tobacco leaves and onion epidermal cells are the commonly used plant materials for the subcellular localization studies of heterogenous proteins (Zhao et al., 2016; Priyadarshani et al., 2018). Alternatively, the protoplasts system provides insights into subcellular localization analysis of homogenous proteins at the single-cell level. In the current work, we demonstrated that the passion fruit protoplast could be exploited for protein subcellular localization studies. Upon the successful uptake of plasmid DNAs into protoplasts by the PEG-mediated transformation, the T-DNA binary vectors (H2B-RFP and ER-mCherry-HDEL) could be transferred into the nucleus for transcription and subsequently encode fluorescent proteins in the cytoplasm and finally be sorted and delivered into the corresponding subcellular sites (the nucleus and endoplasmic reticulum, respectively) in passion fruit protoplasts (Figure 5). Future experiments include the investigation of the roles of various passion fruit proteins by subcellular localization and colocalization studies using the established protoplast-based transient gene expression system in passion fruit.
Protein-Protein interaction analysis using protoplasts have been broadly used in Arabidopsis and tobacco (Wu et al., 2009; Schweiger and Schwenkert, 2014). Bimolecular fluorescence complementation (BiFC) is an in vivo method for studying protein-protein interactions in plant cells. The BiFC technique is based on the restoration of fluorescent signals by two non-fluorescent fragments of the yellow fluorescent protein (YFP) brought together by the association of interacting proteins fused to these fragments (Schutze et al., 2009; Schweiger and Schwenkert, 2014). Here, we also show our protoplast transient gene expression system could be used for protein-protein interaction studies in passion fruit, illustrated by the BiFC experiments to study two pairs of fusion proteins: YN-CP and YC-CP. A clear YFP signal was observed throughout the cytoplasm upon co-expressing YN-CP and YC-CP (Figure 6). This result is consistent with its interaction status in the epidermal cells using tobacco leaf (data not shown). Our system provides a vital tool for protein-protein studies in vivo when dealing with passion fruit proteins.
In summary, our study presents the first report of a simple and efficient passion fruit protoplast isolation protocol by the direct cotyledon-peeling method, and establishment of the highly efficient PEG-mediated transformation system upon optimizations. Furthermore, we demonstrate its detailed usage in transient gene expression studies for the first time in passion fruit, including subcellular localization and protein-protein interaction studies. The established protoplast system would provide an essential platform for various passion fruit biology studies, including whole plant regeneration, transgenic studies, gene function analysis and genome editing.
The datasets presented in this study can be found in online repositories. The names of the repository/repositories and accession number(s) can be found in the article.
ZD, HC, and LW designed the experiment and wrote the manuscript. LW and ZD performed the experiments. All authors contributed to the article and approved the submitted version.
This work was supported by grants from the Hainan Provincial Natural Science Foundation (grant nos. 321QN181 and 322RC564), the National Natural Science Foundation of China (grant no. 32102157), the Scientific Research Foundation for Advanced Talents [grant no. KYQD(ZR)-21040], and Collaborative Innovation Center of Nanfan and High-Efficiency Tropical Agriculture (grant no. XTCX2022NYB11), Hainan University.
We thank Dr. Ji Li (Nanjing Agriculture University, China) for providing pGreen0029-GFP plasmid.
The authors declare that the research was conducted in the absence of any commercial or financial relationships that could be construed as a potential conflict of interest.
All claims expressed in this article are solely those of the authors and do not necessarily represent those of their affiliated organizations, or those of the publisher, the editors and the reviewers. Any product that may be evaluated in this article, or claim that may be made by its manufacturer, is not guaranteed or endorsed by the publisher.
Boni, L. T., Hui, S. W. (1987). The mechanism of polyethylene glycol-induced fusion in model membranes. Cell Fusion. 301-330. doi: 10.1007/978-1-4757-9598-1_14
Cocking, E. C. (1960). A method for the isolation of plant protoplasts and vacuoles. Nature. 187, 962–963. doi: 10.1038/187962a0
Correa, M. F., Pinto, A. P. C., Rezende, J. A. M., Harakava, R., Mendes, B. M. J. (2015). Genetic transformation of sweet passion fruit (Passiflora alata) and reactions of the transgenic plants to Cowpea aphid borne mosaic virus. Eur. J. Plant Pathol. 143, 813–821. doi: 10.1007/s10658-015-0733-5
d'Utra Vaz, F. B., Santos, A. V., Manders, G., Cocking, E. C., Davey, M. R., Power, J. B. (1993). Plant regeneration from leaf mesophyll protoplasts of the tropical woody plant, passionfruit (Passiflora edulis fv flavicarpa Degener.): the importance of the antibiotic cefotaxime in the culture medium. Plant Cell Rep. 12, 220–225. doi: 10.1007/BF00237058
Dai, Z., He, R., Bernards, M. A., Wang, A. (2020). The cis-expression of the coat protein of turnip mosaic virus is essential for viral intercellular movement in plants. Mol. Plant Pathol. 21, 1194–1211. doi: 10.1111/mpp.12973
Dai, Z., Wang, A. (2022). Isolation and transfection of plant mesophyll protoplasts for virology research. Methods Mol. Biol. 2400, 43–53. doi: 10.1007/978-1-0716-1835-6_5
Davey, M. R., Anthony, P., Power, J. B. (2005). and lowe, KPlant protoplasts: status and biotechnological perspectives. C.Biotechnol. Adv. 23, 131–171. doi: 10.1016/j.biotechadv.2004.09.008
Dornelas, M. C., Vieira, M. L. (1993). Plant regeneration from protoplast cultures of Passiflora edulis var. flavicarpa Deg., P. amethystina Mikan. and P. cincinnata Mast. Plant Cell Rep. 13, 103–106. doi: 10.1007/BF00235300
Fischer, I. H., Rezende, J. A. (2008). Diseases of passion flower (Passiflora spp.). Pest technology. 2, 1–19.
Gentzel, I. N., Park, C. H., Bellizzi, M., Xiao, G., Gadhave, K. R., Murphree, C., et al. (2020). A CRISPR/dCas9 toolkit for functional analysis of maize genes. Plant Methods 16, 133. doi: 10.1186/s13007-020-00675-5
Huang, D., Ma, F., Wu, B., Lv, W., Xu, Y., Xing, W., et al. (2022). Genome-wide association and expression analysis of the lipoxygenase gene family in passiflora edulis revealing peLOX4 might be involved in fruit ripeness and ester formation. Int. J. Mol. Sci. 23, 12496. doi: 10.3390/ijms232012496
Li, S., Zhao, R., Ye, T., Guan, R., Xu, L., Ma, X., et al. (2022). Isolation, purification and PEG-mediated transient expression of mesophyll protoplasts in Camellia oleifera. Plant Methods 18, 141. doi: 10.1186/s13007-022-00972-1
Liang, D., Ali, M. M., ALAM, S., Huang, X., YOUSEF, A. F., Mosa, W. F., et al. (2022). Genome-wide analysis of peroxidase genes in passion fruit (Passiflora edulis sims.) and their expression patterns induced by root colonization of Piriformospora indica under cold stress. Turk J. Agric. For. 46, 496–508. doi: 10.55730/1300-011X.3021
Liang, J., Hou, Z., Liao, J., Qin, Y., Wang, L., Wang, X., et al. (2022). Genome-wide identification and expression analysis of LBD transcription factor genes in passion fruit (Passiflora edulis). Int. J. Mol. Sci. 23, 4700. doi: 10.3390/ijms23094700
Manders, G., Otoni, W. C., d'Utra Vaz, F. B., Blackball, N. W., Power, J. B., Davey, M. R. (1994). Transformation of passionfruit (Passiflora edulis fv flavicarpa Degener.) using Agrobacterium tumefaciens. Plant Cell Rep. 13, 697–702. doi: 10.1007/BF00231627
Phong, T. H., Hieu, T., Tung, H. T., Mai, N. T. N., Khai, H. D., Cuong, D. M., et al. (2022). Silver nanoparticles: a positive factor for in vitro flowering and fruiting of purple passion fruit (Passiflora edulis Sim f. edulis). Plant Cell Tiss Organ Cult. 151, 401–412. doi: 10.1007/s11240-022-02361-x
Pitzschke, A., Persak, H. (2012). Poinsettia protoplasts - a simple, robust and efficient system for transient gene expression studies. Plant Methods 8, 14. doi: 10.1186/1746-4811-8-14
Priyadarshani, S. V. G. N., Hu, B., Li, W., Ali, H., Jia, H., Zhao, L., et al. (2018). Simple protoplast isolation system for gene expression and protein interaction studies in pineapple (Ananas comosus L.). Plant Methods 14, 95. doi: 10.1186/s13007-018-0365-9
Reed, K. M., Bargmann, B. O. R. (2021). Protoplast regeneration and its use in new plant breeding technologies. Front. Genome Ed. 3, 734951. doi: 10.3389/fgeed.2021.734951
Ren, R., Gao, J., Yin, D., Li, K., Lu, C., Ahmad, S., et al. (2021). Highly efficient leaf base protoplast isolation and transient expression systems for orchids and other important monocot crops. Front. Plant Sci. 12, 626015. doi: 10.3389/fpls.2021.626015
Rizwan, H. M., Shaozhong, F., Li, X., Bilal Arshad, M., Yousef, A. F., Chenglong, Y., et al. (2022a). Genome-Wide Identification and Expression Profiling of KCS Gene Family in Passion Fruit (Passiflora edulis) Under Fusarium kyushuense and Drought Stress Conditions. Front. Plant Sci. 13, 872263. doi: 10.3389/fpls.2022.872263
Rizwan, H. M., Waheed, A., Ma, S., Li, J., Arshad, M. B., Irshad, M., et al. (2022b). Comprehensive Genome-Wide Identification and Expression Profiling of Eceriferum (CER) Gene Family in Passion Fruit (Passiflora edulis) Under Fusarium kyushuense and Drought Stress Conditions. Front. Plant Sci. 13, 898307. doi: 10.3389/fpls.2022.898307
Rizwan, H. M., Yang, Q., Yousef, A. F., Zhang, X., Sharif, Y., Kaijie, J., et al. (2021). Establishment of a novel and efficient agrobacterium-mediated in planta transformation system for passion fruit (Passiflora edulis). Plants (Basel). 10, 2459. doi: 10.3390/plants10112459
Schutze, K., Harter, K., Chaban, C. (2009). Bimolecular fluorescence complementation (BiFC) to study protein-protein interactions in living plant cells. Methods Mol. Biol. 479, 189–202. doi: 10.1007/978-1-59745-289-2_12
Schweiger, R., Schwenkert, S. (2014). Protein-protein interactions visualized by bimolecular fluorescence complementation in tobacco protoplasts and leaves. J. Vis. Exp. 85, 51327. doi: 10.3791/51327
Shan, X., Li, Y., Zhou, L., Tong, L., Wei, C., Qiu, L., et al. (2019). Efficient isolation of protoplasts from freesia callus and its application in transient expression assays. Plant Cell Tiss Organ Cult. 138, 529–541. doi: 10.1007/s11240-019-01649-9
Shen, Y., Meng, D., McGrouther, K., Zhang, J., Cheng, L. (2017). Efficient isolation of Magnolia protoplasts and the application to subcellular localization of MdeHSF1. Plant Methods 13, 44. doi: 10.1186/s13007-017-0193-3
Sivanandhan, G., Bae, S., Sung, C., Choi, S. R., Lee, G. J., Lim, Y. P. (2021). Optimization of Protoplast Isolation from Leaf Mesophylls of Chinese Cabbage (Brassica rapa ssp. pekinensis) and Subsequent Transfection with a Binary Vector. Plants (Basel). 10, 2636. doi: 10.3390/plants101226369
Song, S., Zhang, D., Ma, F., Xing, W., Huang, D., Wu, B., et al. (2022). Genome-wide identification and expression analyses of the aquaporin gene family in passion fruit (Passiflora edulis), revealing peTIP3-2 to be involved in drought stress. Int. J. Mol. Sci. 23, 5720. doi: 10.3390/ijms23105720
Trevisan, F., Mendes, B. M. J., Maciel, S. C., Vieira, M. L. C., Meletti, L. M. M., Rezende, J. A. M. (2006). Resistance to Passion fruit woodiness virus in Transgenic Passionflower Expressing the Virus Coat Protein Gene. Plant Dis. 90, 1026–1030. doi: 10.1094/PD-90-1026
Wang, Y., Wang, L., Liu, H., Gou, B., Hu, W., Qin, L., et al. (2023). Direct leaf-peeling method for areca protoplasts: a simple and efficient system for protoplast isolation and transformation in areca palm (Areca catechu). BMC Plant Biol. 23, 56. doi: 10.1186/s12870-023-04048-7
Wang, H., Wang, W., Zhan, J., Huang, W., Xu, H. (2015). An efficient PEG-mediated transient gene expression system in grape protoplasts and its application in subcellular localization studies of flavonoids biosynthesis enzymes. Sci. Hortic-Amsterdam. 191, 82–89. doi: 10.1016/j.scienta.2015.04.039
Wang, Q., Yu, G., Chen, Z., Han, J., Hu, Y., Wang, K. (2021). Optimization of protoplast isolation, transformation and its application in sugarcane (Saccharum spontaneum L). Crop J. 9, 133–142. doi: 10.1016/j.cj.2020.05.006
Wu, J. Z., Liu, Q., Geng, X. S., Li, K. M., Luo, L. J., Liu, J. P. (2017). Highly efficient mesophyll protoplast isolation and PEG-mediated transient gene expression for rapid and large-scale gene characterization in cassava (Manihot esculenta Crantz). BMC Biotechnol. 17, 29. doi: 10.1186/s12896-017-0349-2
Wu, F. H., Shen, S. C., Lee, L. Y., Lee, S. H., Chan, M. T., Lin, C. S. (2009). Tape-Arabidopsis Sandwich - a simpler Arabidopsis protoplast isolation method. Plant Methods 5, 16. doi: 10.1186/1746-4811-5-16
Xia, Z., Huang, D., Zhang, S., Wang, W., Ma, F., Wu, B., et al. (2021). Chromosome-scale genome assembly provides insights into the evolution and flavor synthesis of passion fruit (Passiflora edulis Sims). Hortic. Res. 8, 14. doi: 10.1038/s41438-020-00455-1
Xu, X. F., Zhu, H. Y., Ren, Y. F., Feng, C., Ye, Z. H., Cai, H. M., et al. (2021). Efficient isolation and purification of tissue-specific protoplasts from tea plants (Camellia sinensis (L.) O. Kuntze). Plant Methods 17, 84. doi: 10.1186/s13007-021-00783-w
Yoo, S. D., Cho, Y. H., Sheen, J. (2007). Arabidopsis mesophyll protoplasts: a versatile cell system for transient gene expression analysis. Nat. Protoc. 2, 1565–1572. doi: 10.1038/nprot.2007.199
Yu, G., Cheng, Q., Xie, Z., Xu, B., Huang, B., Zhao, B. (2017). An efficient protocol for perennial ryegrass mesophyll protoplast isolation and transformation, and its application on interaction study between LpNOL and LpNYC1. Plant Methods 13, 46. doi: 10.1186/s13007-017-0196-0
Yu, C., Lian, Q., Lin, H., Chen, L., Lu, Y., Zhai, Y., et al. (2021). A clade of telosma mosaic virus from Thailand is undergoing geographical expansion and genetic differentiation in passionfruit of Vietnam and China. Phytopathol. Res. 3, 1–10. doi: 10.1186/s42483-021-00101-1
Keywords: passion fruit, Passiflora edulis, passiflora, protoplast, PEG-mediated transformation, transient gene expression
Citation: Wang L, Liu H, Liu P, Wu G, Shen W, Cui H and Dai Z (2023) Cotyledon peeling method for passion fruit protoplasts: a versatile cell system for transient gene expression in passion fruit (Passiflora edulis). Front. Plant Sci. 14:1236838. doi: 10.3389/fpls.2023.1236838
Received: 08 June 2023; Accepted: 24 July 2023;
Published: 10 August 2023.
Edited by:
Bin Xu, Nanjing Agricultural University, ChinaReviewed by:
Mat Yunus Abdul Masani, Malaysian Palm Oil Board, MalaysiaCopyright © 2023 Wang, Liu, Liu, Wu, Shen, Cui and Dai. This is an open-access article distributed under the terms of the Creative Commons Attribution License (CC BY). The use, distribution or reproduction in other forums is permitted, provided the original author(s) and the copyright owner(s) are credited and that the original publication in this journal is cited, in accordance with accepted academic practice. No use, distribution or reproduction is permitted which does not comply with these terms.
*Correspondence: Hongguang Cui, aG9uZ2d1YW5nLmN1aUBoYWluYW51LmVkdS5jbg==; Zhaoji Dai, emhhb2ppLmRhaUBoYWluYW51LmVkdS5jbg==
Disclaimer: All claims expressed in this article are solely those of the authors and do not necessarily represent those of their affiliated organizations, or those of the publisher, the editors and the reviewers. Any product that may be evaluated in this article or claim that may be made by its manufacturer is not guaranteed or endorsed by the publisher.
Research integrity at Frontiers
Learn more about the work of our research integrity team to safeguard the quality of each article we publish.