- 1State Key Laboratory of Subtropical Silviculture, Zhejiang A & F University, Lin’An, Zhejiang, China
- 2Forest and Bamboo Resources Conservation and Cultivation Institute, Yibin Forestry and Bamboo Industry Research Institute, Yibin, Sichuan, China
- 3Sichuan Changning Century Bamboo Garden, Yibin, Sichuan, China
Bamboo is one of the fastest-growing plants commonly used in food, fibre, paper, biofuel, ornamental and medicinal industries. Natural hybridization in bamboo is rare due to its long vegetative period followed by gregarious flowering and death of the entire population. In the current study, a new bamboo species, Bambusa changningensis, shows intermediate characteristics of Dendrocalamus farinosus and B. rigida morphologically, but it is unknown whether B. changningensis is a natural hybrid. Moreover, B. changningensis has been identified as a superior variety of Sichuan Province with high pulping yield, fibre length and width. Therefore, we analyzed the morphological characteristics, DNA markers, DNA barcoding and chloroplast genomes to identify the hybrid origin of B. changningensis and possible maternal parent. We have developed the transcriptomic data for B. changningensis and mined the SSR loci. The putative parental lines and hybrid were screened for 64 SSR makers and identified that SSR14, SSR28, SSR31 and SSR34 markers showed both alleles of the parental species in B. changningensis, proving heterozygosity. Sequencing nuclear gene GBSSI partial regions and phylogenetic analysis also confirm the hybrid nature of B. changningensis. Further, we have generated the complete chloroplast genome sequence (139505 bp) of B. changningensis. By analyzing the cp genomes of both parents and B. changningensis, we identified that B. rigida might be the female parent. In conclusion, our study identified that B. changningensis is a natural hybrid, providing evidence for bamboo’s natural hybridization. This is the first report on confirming a natural bamboo hybrid and its parents through SSR and chloroplast genome sequence.
1 Introduction
Bamboos are the only grass and non-timber woody plant species widely distributed across the Americas, Africa, and Asia Pacific (Ramakrishnan et al., 2020). Bamboo plants are vital economic and ecological forestry resources commonly used in papermaking, fuel, food processing, construction and the medicinal industry (Meena et al., 2019; Manandhar et al., 2019). The tribe Bambuseae contains 91 genera and ~1500 bamboo species. According to the number of basic sets of bamboo chromosomes, all bamboo species were divided into four monophyletic lineages with different ploidy levels. The herbaceous bamboos (2n = 20–24) are diploids, neotropical woody bamboos (2n = 40–48), and temperate woody bamboos (2n = 46–48) are tetraploids, whereas the palaeotropical woody bamboos (2n = 70–72) are hexaploids (Zhou et al., 2017a; Guo et al., 2019). Further phylogenomic analyses revealed that woody bamboos originated from complex reticulate evolution, including four extinct diploid lineages over three independent allopolyploid events after the divergence of the herbaceous bamboo lineage (Guo et al., 2019).
Natural hybridization is an important mechanism in plant evolution that periodically stimulates plant speciation (Hegarty and Hiscock, 2005). Combining different genomes in hybrid lineages has broad evolutionary and ecological implications and may promote evolutionary innovation and adaptive expansion (Paun et al., 2009). Bamboo species are monocarpic plants with a prolonged vegetative stage and uncertain flowering cycle (Zheng X. et al., 2020). The flowering cycles of different bamboo species, even those belonging to the same genus, fluctuate considerably. For example, the flowering cycle of Bambusa varies from 30 to 150+ years, Phyllostachys from 13 to 120 years and Dendrocalamus from 8 to 117 years (Zheng X et al., 2020; Hou et al., 2021). Mizuki et al. (2014) estimated the self-pollination rate of three temperate bamboo species at five sites is 96.3%. Therefore, the simultaneous flowering of different bamboo species at the same place and time and the development of hybrid bamboo plants, especially in the natural environment, is rare. Thus, identifying natural bamboo hybrids helps in studying bamboo taxonomy and the origin of bamboo evolution (Goh et al., 2013).
The traditional identification of bamboo species or hybrid plants is mainly based on morphological characteristics such as flowers and bamboo shoots (Lichtenthaler, 1987). Due to the unique flowering characteristics of most bamboo species, it is difficult to rely solely on morphological features, which are unreliable because they are easily influenced by ecological factors (Das et al., 2007). The development of molecular markers and DNA sequencing has brought a new approach to hybrid identification, taxonomy and phylogenetic analysis (Jiang and Zhou, 2014). The DNA marker technology provided a theoretical and practical basis for bamboo breeding and classification and eliminated the errors of traditional classification methods (Zhang and Tang, 2007). Among various kinds of DNA markers, Simple Sequence Repeat (SSR) is the most widely used marker technology in many aspects, such as DNA fingerprinting, genetic map construction, genetic diversity studies, hybrid identification, and genetic resource conservation (Lin et al., 2010; Bhandawat et al., 2016; Cai et al., 2019; Wu et al., 2023). Whereas molecular phylogenetic analysis based on DNA sequencing also provides a powerful method for studying the process and mechanism of hybridization (Horiike, 2016). The nuclear GBSSI gene, which encodes granule-bound starch synthase, proved to be more suitable for molecular phylogenetic analysis among bamboo species compared with other nuclear gene fragments (Guo and Li, 2004).
The nuclear genome shows biparental inheritance, whereas the cp genome shows maternal inheritance in angiosperms and patrilineal inheritance in gymnosperms (Wolfe et al., 1987; Pharmawati et al., 2004; Zheng Y et al., 2020). Therefore, the phylogenetic tree of cp DNA often represents a parthenogenetic spectrum. Thus, selecting suitable cytoplasmic molecular markers between the parents and hybrid can determine the maternal and paternal origin in angiosperms and gymnosperms, respectively (Tian and Li, 2002; Zheng et al., 2009). Hence, studying the combination of morphological characters, nuclear and cytoplasmic DNA, has become the criteria for identifying hybrids and their putative parents (Sang and Zhong, 2000).
In the current study, we used three sympodial bamboo species, Bambusa changningensis, B. rigida and D. farinosus, from China’s Sichuan province. B. rigida is one of the native species in Sichuan, considered to have economic and ornamental value with high utilization (Hu et al., 2009). D. farinosus is an essential economic sympodial bamboo species in Southwest China. It has essential characteristics such as cold resistance, barren tolerance, and high cellulose content, making it an excellent raw material for pulping and paper making (Jiang et al., 2008). The B. changningensis is a newly identified bamboo species that occupies an important position in the bamboo industry in Sichuan province, China with the characteristics of long bamboo shoot period, high bamboo shoot yield, wide adaptability, tolerance to fertilizer and humidity, and also resistance to both abiotic and biotic stress. The phenotypic characteristics of B. changningensis are similar or intermediate between the bamboo species B. rigida and the D. farinosus, considered a potential natural hybrid (Wang et al., 2016). Further, B. rigida and the D. farinosus were hexaploid bamboo plants containing the same chromosomal number 2n=70 ± 2 (Chen, 2003). Therefore, these plants might be easily crossable, provided they flower at the same time. Moreover, recently, it has been selected for increasing cultivation in Sichuan province because it has superior characteristics like high yield and better pulp quality than other local bamboos (Wang et al., 2020; Zhou et al., 2020).
In 1968, Mr. Daigui Wang, a farmer, identified that B. rigida and D. farinosus flowered simultaneously at Xinjia, Zhuhai, Changning, Sichuan province, China. He collected the seedlings from the same area and transplanted them near his home the following year. In 2012, Prof. Tongpei identified that along with B. rigida and D. farinosus, a new bamboo species is also present in those plants raised from seedlings and named it B. changningensis, which means similar to B. rigida in Chinese (Yi and Li, 2012). Further, he also says that B. changningensis might be the hybrid between B. rigida and D. farinosus, but no evidence exists. Therefore, in the current study, we analyzed the phenotypical characteristics of the B. changningensis, B. rigida and D. farinosus, combined SSR molecular markers, nuclear gene GBSSI partial regions and complete chloroplast genomes together to prove the hybrid authenticity of B. changningensis.
2 Materials and methods
2.1 Plant materials and morphological analysis
In this study, we used the young and healthy leaves of D. farinosus, B. changningensis (R-WTS-BC-005-2015) and B. rigida collected from Changning, Sichuan Province, China (28°29’N, 104°58’E). For molecular studies 21 B. changningensis individual plant samples collected from three sites were used, whereas three individual plants each for B. rigida and D. farinosus were used. Further, 15 individuals per species were used for analysing fibre length, fibre width, fibre wall cavity ratio, pulping yield, cellulose content, stem height and stem diameter.
2.2 DNA extraction and nuclear sequence amplification
Total genomic DNA was extracted using the CTAB protocol (Doyle, 1991). The total genomic DNA was used as a template for PCR amplification, and the GBSSI gene was amplified and sequenced. The primer sequences of GBSSI partial regions mentioned by Ye (2010) for PCR amplification (Table S1). The PCR amplification was carried out in a 50 μl reaction volume containing 25 μl Taq master mix (2x Specific Taq Master Mix, 250 units, novoprotein, Suzhou, China), 22 μl ddH2O, 100 ng total DNA and 2 umol/L of each primer. The PCR program followed was initial denaturation of 95 °C for 5 min, followed by 35 cycles of 95 °C for 30 s (denaturation), 65 °C for 30 s (annealing) and 72 °C for 40 s (extension) and a final extension at 72 °C for 10 min. The PCR products were separated in the 1% agarose gels, and the PCR products were extracted from the gel using SanPrep Column DNA Gel Extraction Kit (Simgen, Hangzhou, China) and sequenced by Sanger sequencing.
2.3 Transcriptome sequencing
The total RNA was isolated by Polysaccharides & Polyphenolics-rich Plant Total RNA Kit (Simgen, Hangzhou, China) from the bulk tissues of B. changningensis leaves, stem, root and apical meristem as per the manufacturer’s instructions. The quality and quantity of RNA were detected using the NanoPhotometer spectrophotometer (Implen, CA, USA) and electrophoresis (1% agarose gel). The libraries were synthesized utilizing the NEB Next® UltraTM RNA Library Prep Kit for Illumina® (NEB, USA). These constructed libraries were sequenced using the Illumina HiSeq forum to obtain paired-end reads (150 bp). After quality control (QC), transcriptome data were further handled and assembled following the procedure described in (Grabherr et al., 2011) using Trinity v2.8.4. The Assembled results by Trinity were processed using Corset as described in (Davidson and Oshlack, 2014).
2.4 Microsatellite analysis
The SSR motifs from the transcriptomic data were mined using the online software MISA (Beier et al., 2017) with 10, 6, 5, 4, 3, and 3 values for mono-, di-, tri-, tetra-, penta- and hexa- SSR motifs, respectively (Table S2). The SSR primers were designed by Oligo7 (https://www.oligo.net/downloads.html) (Rychlik, 2007) and synthesized by the Tsingke company (Beijing, China). PCR amplification was performed as described above. The PCR products were separated in the 5% agarose gels, and the PCR products were extracted from the gel using SanPrep Column DNA Gel Extraction Kit (Simgen, Hangzhou, China) and sequenced by Sanger sequencing.
2.5 Complete chloroplast genome sequencing and analysis
The total DNA of B. changningensis was used to develop the whole genome reads through the Illumina NovaSeq PE150 platform (Novogene Bioinformatics Technology Co. Ltd, Beijing, China). The complete chloroplast genome was assembled by mapping the whole genome reads to reference genome B. emeiensis (HQ337797) using MITObim v1.8 (Hahn et al., 2013) and annotated with the PGA (Qu et al., 2019).
2.6 Construction of phylogenetic tree
The phylogenetic tree for GBSSI partial regions were constructed using PAUP 4.0a software (Swofford, 2008). Twenty-five GBSSI sequences from different bamboo species were downloaded from the NCBI database and used in this study (Table S3a). The DNA sequences were aligned using MEGAX (Kumar et al., 2018), and highly aligned sequences were used to generate the phylogenetic tree using the maximal parsification method in PAUP 4.0a software as per the parameters described in (Winkworth and Donoghue, 2004).
The 24 cp genomes of different bamboo species were downloaded from the NCBI database (Table S3b) and all the genes were extracted and concatenated to get the final sequences matrix to construct a phylogenetic tree. The sequences were aligned by MAFFT (Katoh and Standley, 2013) and the phylogenetic tree was constructed by MEGA X software using the Neighbor-Joining method (Kumar et al., 2018). A bootstrap value of 1000 replicates was used to assess the statistical significance.
3 Results
3.1 Morphological analysis of putative parents and hybrid plants
In this study, we analyzed morphological characteristics such as fibre length, fibre width, fibre wall cavity ratio, pulping yield, cellulose content, stem height, stem diameter, sheath and leaves for B. changningensis, D. farinosus and B. rigida (Table 1 and Figure 1). As expected, B. changningensis shows the intermediate characteristics of D. farinosus and B. rigida in biological traits such as the sheath size, leaf width, and cellulose content. The stem height and diameter were more similar to the putative parent D. farinosus. Interestingly, B. changningensis also contains superior morphological characteristics like longer fibre length, fibre width, lower fibre wall cavity ratio and higher pulping yield than putative parents D. farinosus and B. rigida.
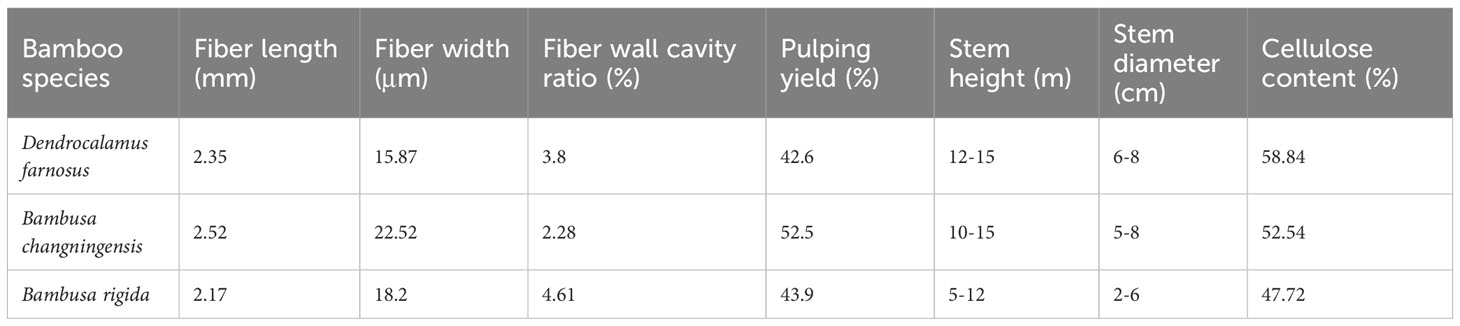
Table 1 The biological characteristics comparison of Dendrocalamus farnosus, Bambusa changningensis and Bambusa rigida.
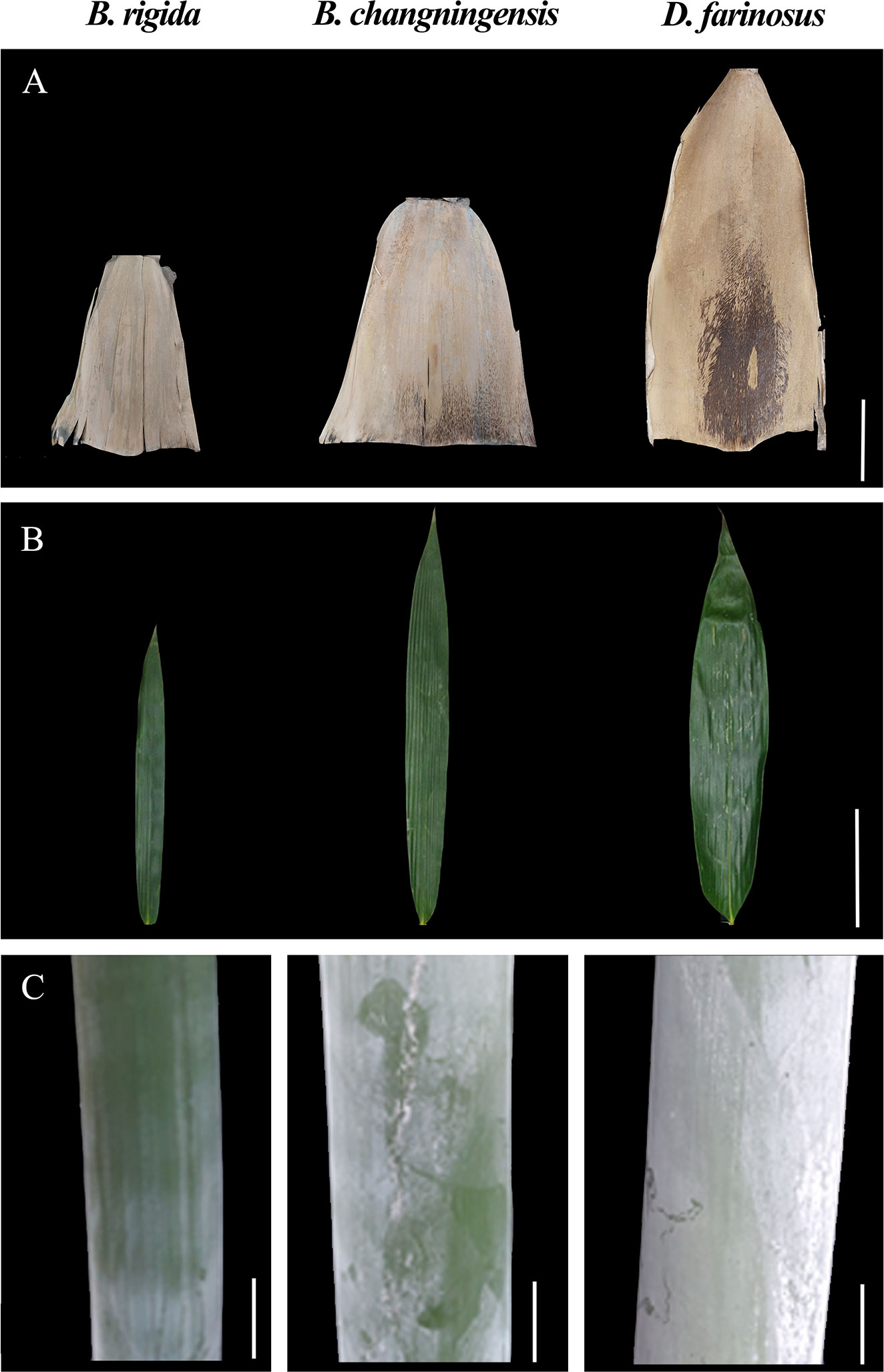
Figure 1 The morphological characteristics comparison of B. rigida, B. changningensis, and D. farinosus. (A) bamboo sheath, (B) Leaf blade, and (C) Stem. Bar size A 50 cm, B 10 cm and C 50 cm.
3.2 Transcriptome analysis and SSR primers screening
We have developed pair-end transcriptomic data of B. changningensis using the Illumina HiSeq platform to obtain genomic information to develop the DNA markers. A total of 23,270,949 reads containing 6,961,574,574 bp were retained after quality trimming. After de novo assembly, a total of 154,983 contigs and 100,364 unigenes were obtained (Tables S4, S5). The contigs were subjected to SSR mining through MISA software and identified a total of 3,732 SSR loci, including di-, tri-, tetra-, penta- and hexa-nucleotide motifs. The di- and tetra-nucleotide repeats were the most abundant SSR loci detected, accounting for 34.5% and 35.2%, respectively (Table S2). Sixty-four sequences containing SSR loci were selected randomly for PCR amplification to identify polymorphism between the B. changningensis and its putative parents, D. farinosus and B. rigida using bulk DNA (Table S1). Out of 64 primer pairs, four primer pairs, SSR14, SSR28, SSR31 and SSR34 produced polymorphic PCR amplification (Figure 2). The SSR14 primers had an amplification of 139 bp in D. farinosus, whereas 144 and ~135 bp bands in B. rigida. SSR28 had an amplification size of 168 bp in D. farinosus, while B. rigida contains 159 and ~180 bp bands. The SSR31 primers had an amplification of 255 bp in D. farinosus, whereas 200 bp bands in B. rigida. Similarly, The SSR34 primers had an amplification of 160 in D. farinosus, whereas 167 bp bands in B. rigida. These results confirm that the B. changningensis had both putative parental complementary bands, except the ~135 bp bands of B. rigida.
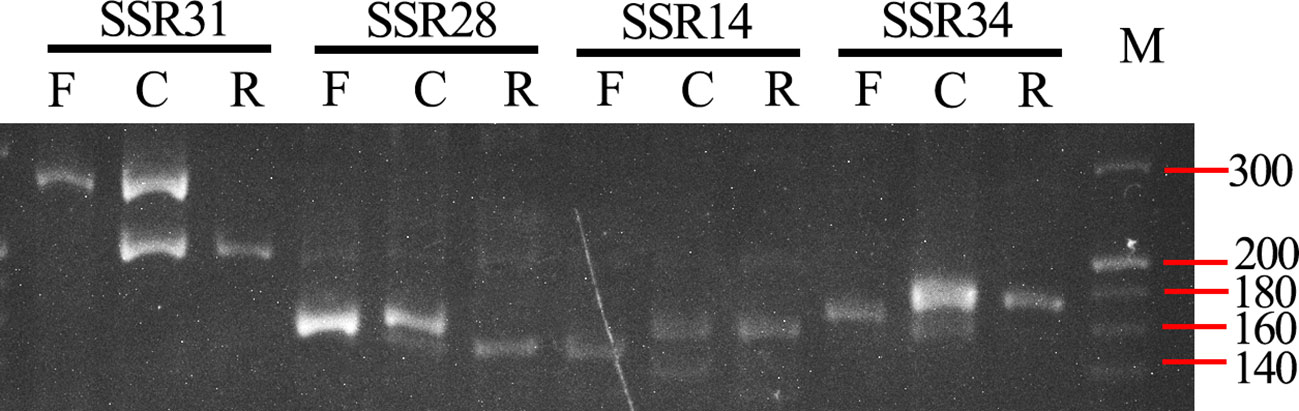
Figure 2 SSR polymorphism between B. rigida, B. changningensis, and D. farinosus. PCR was performed with SSR14, SSR28, SSR31 and SSR34. M. 20 bp ladder.
Moreover, we analysed these four primers in 21 induvial plants of B. changningensis, the presence of putative parental bands in all individual plants confirms the heterozygous nature (Figure 3). Further, we sequenced the 139, 168, 255, and 160 bp bands in D. farinosus and B. changningensis, 144, 159, 200, and 167 bp bands in B. changningensis, and B. rigida to identify the difference in the repeats of the SSR motif. The repeat units of SSR14 were CTCTC and contained a single repeat unit difference between the D. farinosus and B. rigida (Figure S1A). At the same time, SSR28 had three repeat unit differences in the CTC motif (Figure S1B). B. changningensis contains both putative parental SSR motifs. The repeat unit of SSR31 was AGG and contained a single repeat unit difference between the D. farinosus and B. rigida (Figure S1C). Interestingly, this SSR also has 56 bp single insertion and 1 bp deletion in D. farinosus. The repeat units of SSR34 were GCCTC and contained a single repeat unit difference between the D. farinosus and B. rigida (Figure S1D). These results suggest that B. changningensis might be the hybrid plant of D. farinosus and B. rigida.
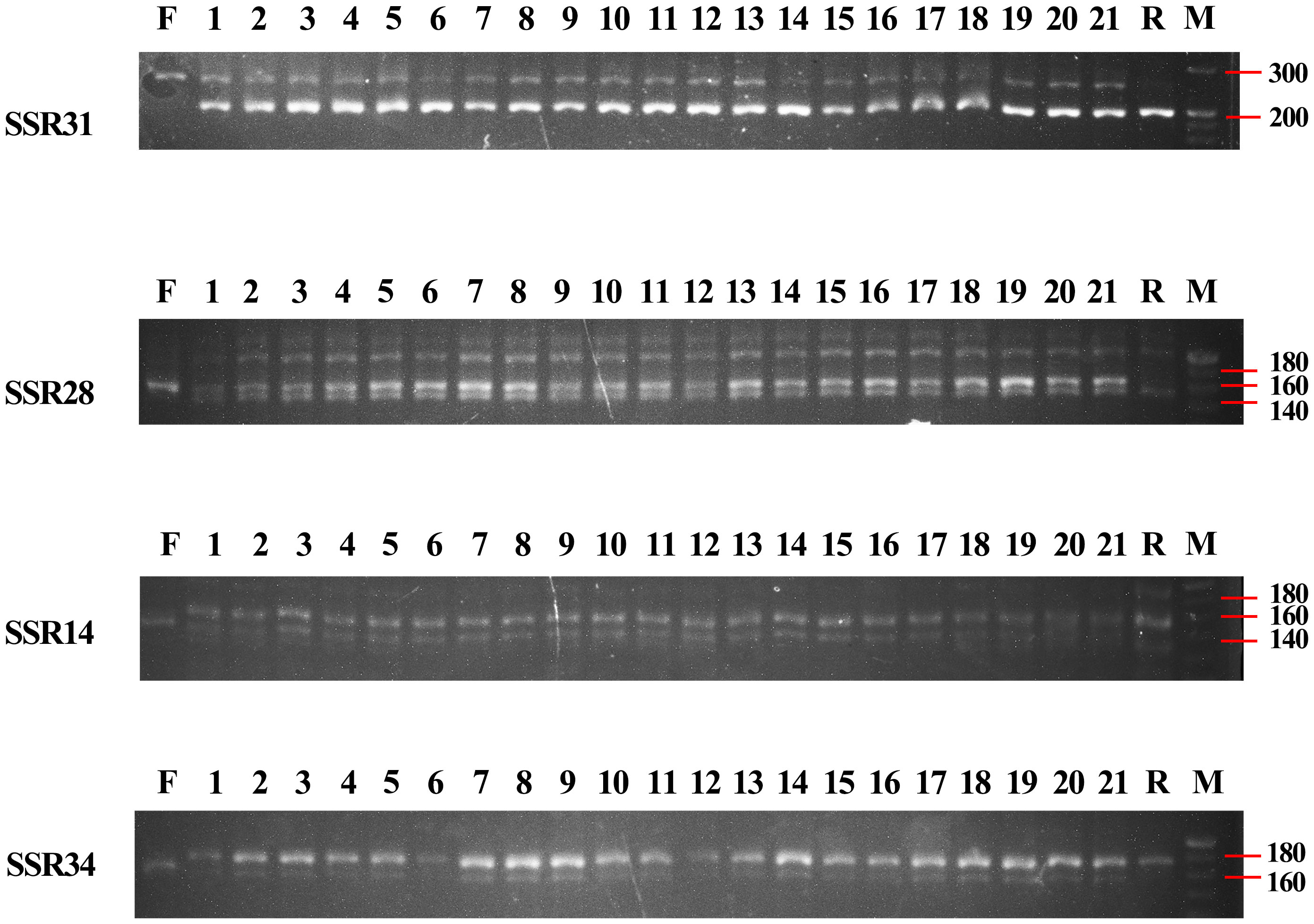
Figure 3 PCR amplification of SSR14, SSR28, SSR31 and SSR34 makers for individuals of B. changningensis. F. D. farinosus; 1-21. B. changningensis; R. B. rigida. M. 20 bp DNA ladder.
3.3 GBSSI gene fragment cloning and evolutionary tree construction
The GBSSI gene is about 3 kb in length, and due to the difficulty in PCR amplification, a portion of the sequence (~820 bp) was used for analysis. Using the genomic DNA of B. changningensis, B. rigida and D. farinosus as a template for amplification, the GBSSI gene was amplified. The PCR product was cloned into TA cloning vector PMD18, and 30 clones for each species were sequenced. Sequencing results identified that a total of three different kinds of clones, BchGBSSI-1, BchGBSSI-2 and BchGBSSI-3, were present in B. changningensis. At the same time, B. rigida contains two clones, and D. farinosus has only one type of clone, BriGBSSI-1, BriGBSSI-2 and DfaGBSSI-1, respectively. Further, we aligned these sequences and identified BchGBSSI-1 and BriGBSSI-1, BchGBSSI-2 and BriGBSSI-2, BchGBSSI-3 and DfaGBSSI-1, which have similar kinds of SNPs. Moreover, a Maximum Parsimonious evolutionary tree was constructed using 23 GBSSI sequences downloaded from the NCBI database (Figure 4). Similar to the above results, B. changningensis two GBSSI genes, BchGBSSI-1 and BchGBSSI-2, clustered with BriGBSSI-1 and BriGBSSI-2, respectively, and the 3rd GBSSI gene, BchGBSSI-3 was clustered together with DfaGBSSI-1. These results confirm that B. changningensis is the hybrid between the parental bamboo species B. rigida and D. farinosus.
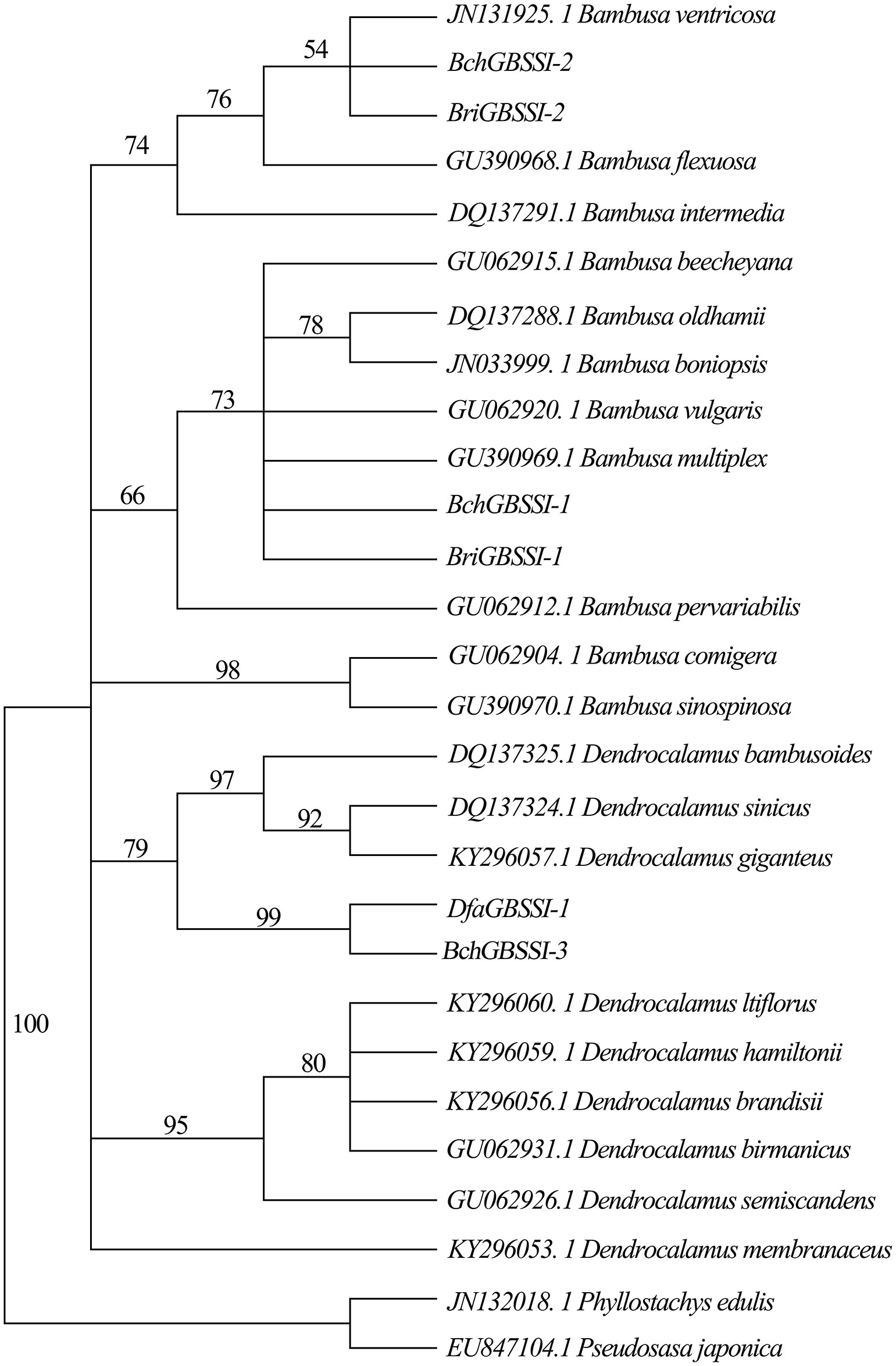
Figure 4 The phylogenetic relationship among GBSSI genes of Bambusoideae. The partial DNA sequences of GBSSI genes were used to construct the phylogenetic tree. The bootstrap support values are shown on each node. Dfa,. D. farinosus; Bch,. B. changningensis; Bri, B. rigida.
3.4 B. changningensis complete chloroplast genome sequencing and annotation
The complete chloroplast genome of B. changningensis was sequenced and analyzed in this study. In the current study, a total of 99,346,872 raw data was developed from the total DNA of B. changningensis. After quality trimming, the raw data was reduced to 83416080, and the GC content was 44.98%. Further, the average length of the reads was 150 bp. We mapped the quality reads to the reference cp genome B. emeiensis (HQ337797), and 14.69% of the reads were mapped. The complete chloroplast genome sequence of B. changningensis (GenBank accession: OM065947) generated was 139505 bp (Figure 5). After annotation, a total of 132 genes were found in the cp genome of B. changningensis. These include 84 genes coding for essential chloroplast function, 40 tRNA genes, and eight rRNA genes. Further, the cp genome consists of a pair of identical inverted repeat regions of size 21,794 bp separated by a large single-copy (LSC) region of 83,041 bp in size and a small single-copy (SSC) region of 12,876 bp.
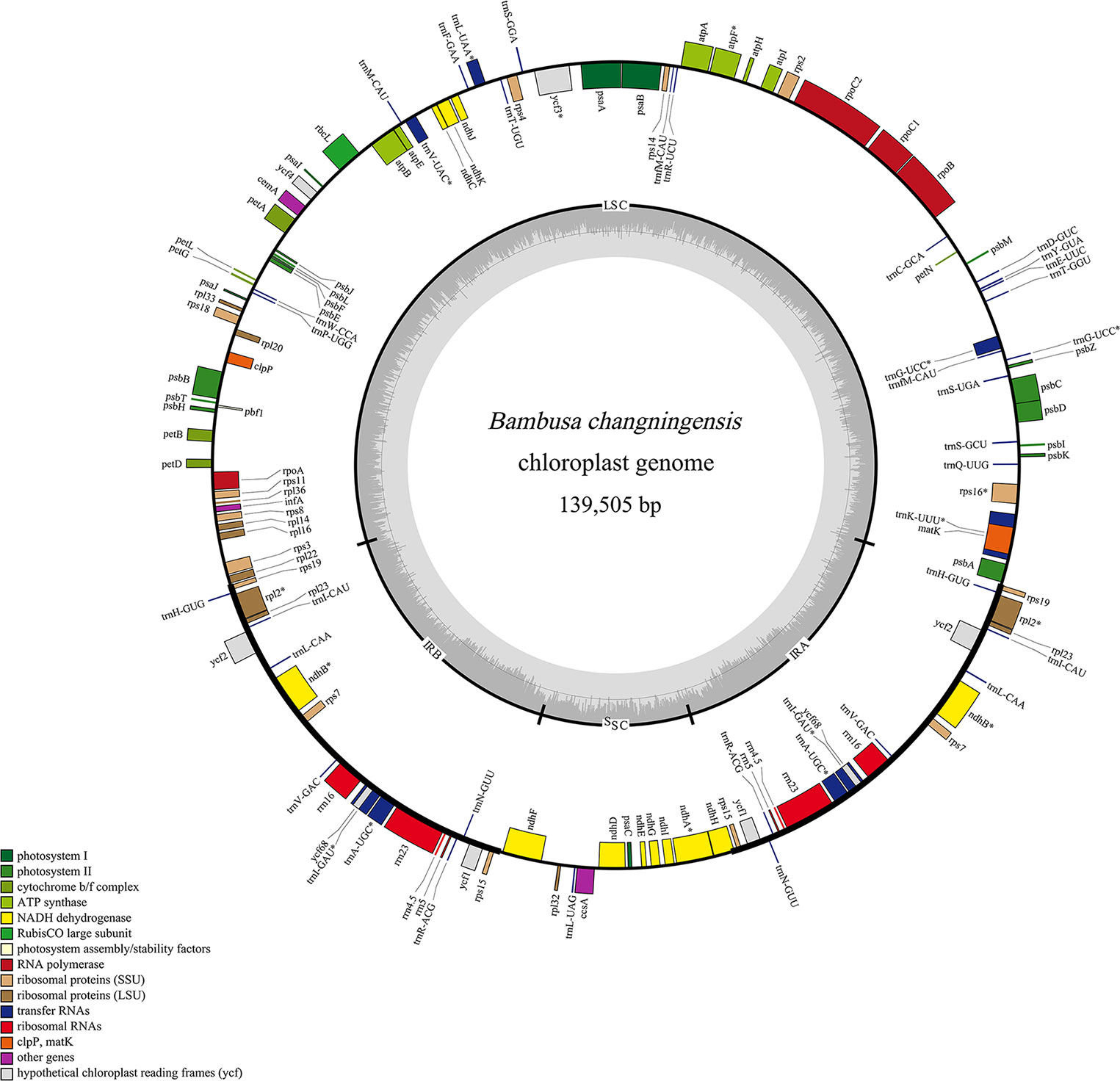
Figure 5 The chloroplast genome of B. changningensis. Genes are represented on the inner or outer side of the outer circle to indicate the coding strands.
3.5 Comparison of chloroplast genomes of B. changningensis, D. farinosus and B. rigida
To identify the female parent of the B. changningensis, we aligned the cp genome sequences of B. changningensis, B. rigida (GenBank accession: MT648824), and D. farinosus (GenBank accession: OM177223). The aligned sequences were examined for the presence/absence of Indels/SNPs. Of the 132 cp genes identified in the B. changningensis, 33 contain the Indels/SNPs. Among them, 32 genes of the D. farinosus displayed Indels/SNPs, whereas the rpoc2 gene has 8 SNPs and 1 Indel in D. farinosus, 4 SNPs and 4 Indels in B. rigida and 3 SNPs in B. changningensis (Table 2). Further, the D. farinosus noncoding region displayed 176 SNPs, 47 insertions, and 34 deletions. The largest insertion and deletions are 50 and 31 bp, respectively (Table S6). Further, a single bp deletion was also found in B. rigida. These results indicate that the cp genomes of B. changningensis and B. rigida were almost similar, and D. farinosus contained SNPs and Indels. Thus B. rigida might be the female parent of the hybrid B. changningensis. Moreover, we also constructed a phylogenetic tree based on the extracted gene sequences from 24 complete chloroplast genomes using the Neighbor-Joining method with 1,000 bootstrap replicates (Figure 6). The hybrid B. changningensis was clustered with B. rigida with high support values, confirming that B. rigida is the female parent of B. changningensis and D. farinosus is the male parent.
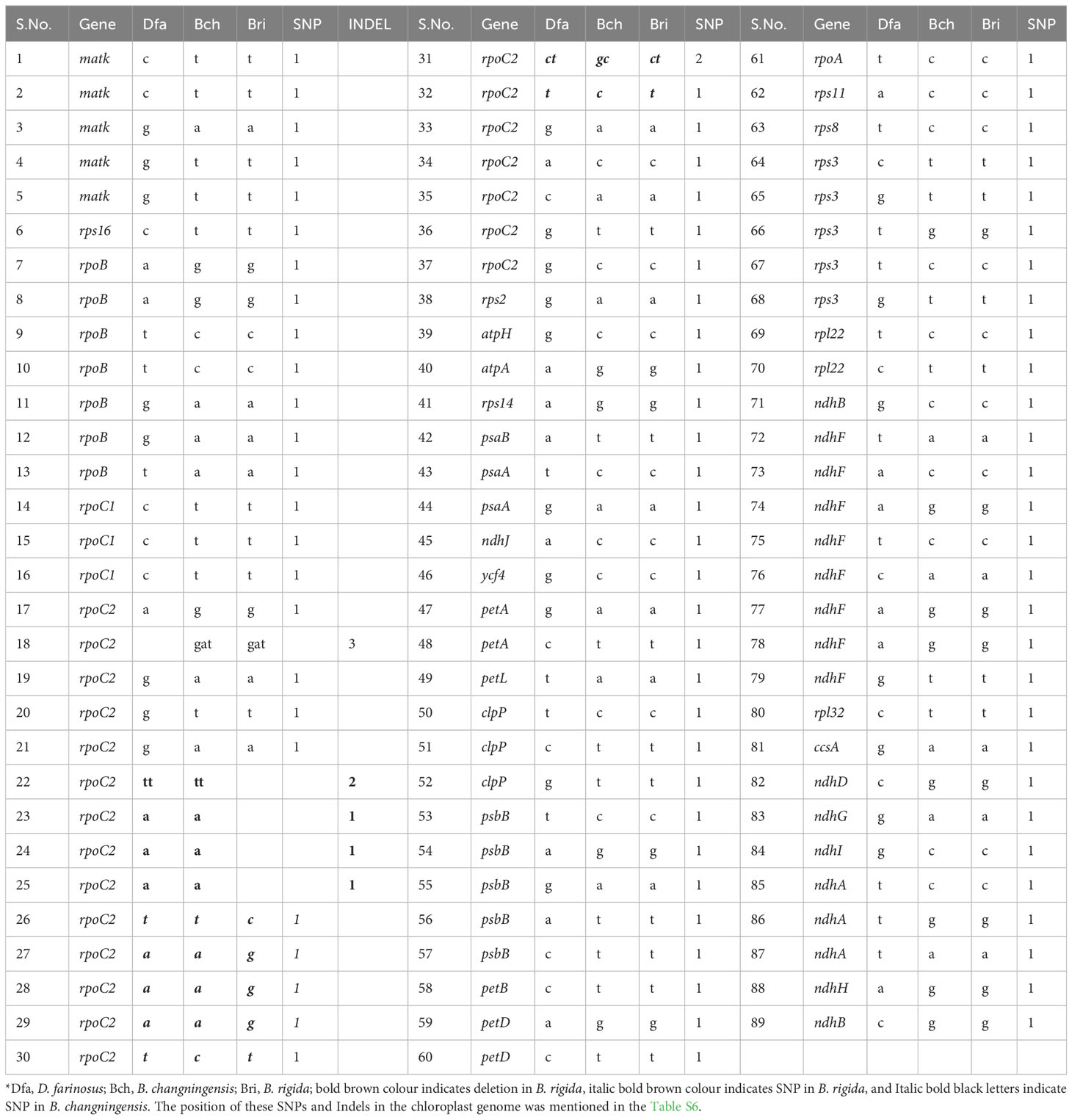
Table 2 Details of SNPs and INDELs in the D. farinosus, B. changningensis and B. rigida chloroplast genes.
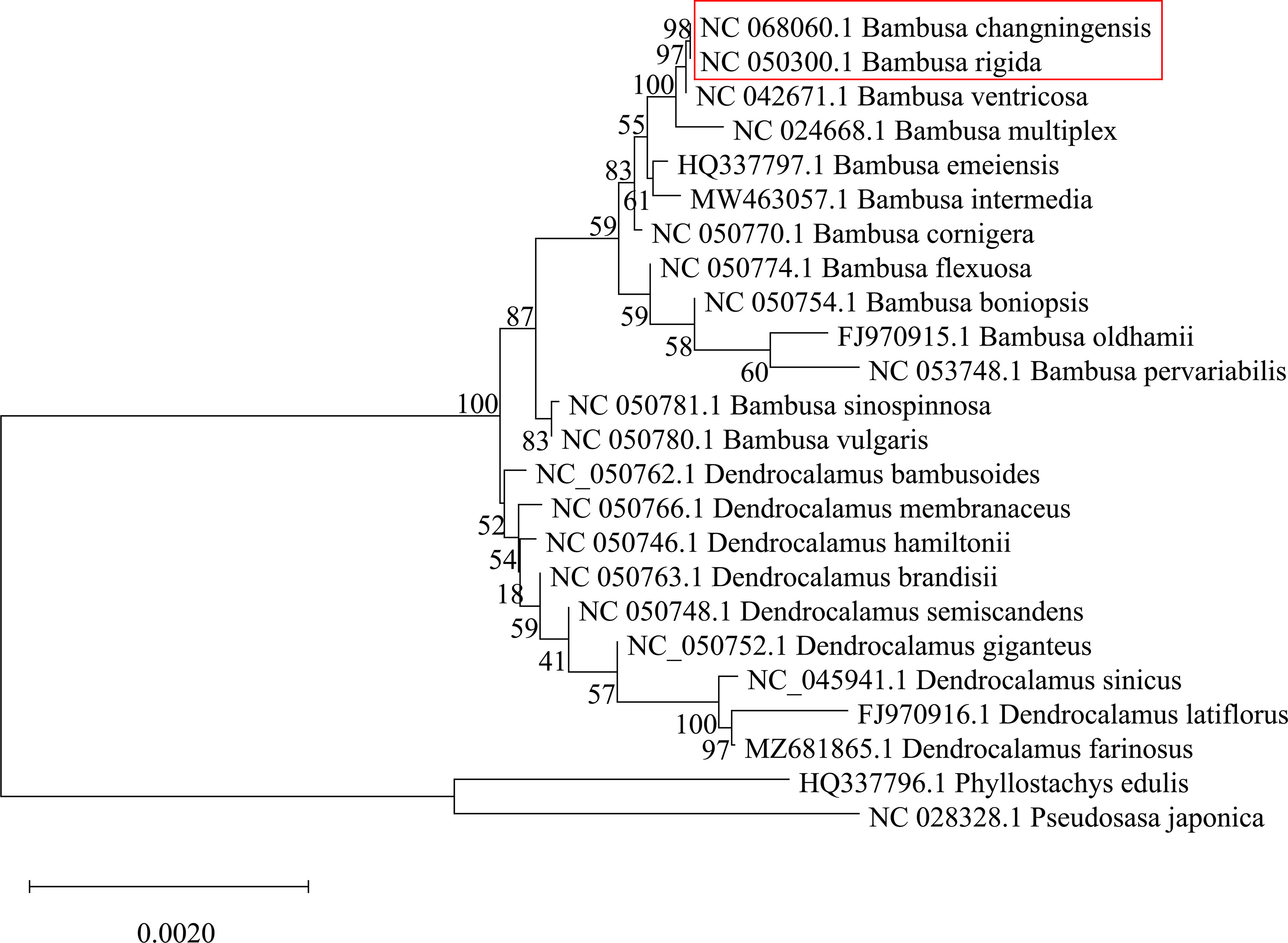
Figure 6 The phylogenetic relationship among extracted gene sequences from complete chloroplast genomes of Bambusoideae. The bootstrap support values are shown on each node.
4 Discussion
A natural plant hybrid is more common in the natural environment than animals, which is considered a necessary evolutionary process (Rieseberg, 1995; Hegarty and Hiscock, 2005; Zheng et al., 2021). The hybridization is widespread in grass plants (Stebbins, 1956; Singh et al., 2010) but rarely recorded in woody bamboo (Triplett et al., 2010). Because most woody bamboos take a long time to flower, some even as long as 120 years (Janzen, 1976). Therefore, natural bamboo hybridization is considered rare. However, a couple of natural hybridization events are reported in bamboo species based on morphological characteristics suggesting that hybridization events are possible in bamboo, but there is not enough evidence to support this (Maruyama et al., 1979; Muramatsu, 1981). In the current study, we identified a new natural intergeneric bamboo hybrid species B. changningensis. Further, we also identified that B. rigida is the maternal parent of B. changningensis.
4.1 Simple sequence repeats results suggest the possibility that B. changningensis might be the hybrid of D. farinosus and B. rigida
SSRs have been widely used in identifying hybrids as a common DNA molecular marker due to the advantages of codominance, simple operation and immunity from environmental effects (Momotaz et al., 2004; Sundaram et al., 2008). After the NGS platform’s discovery, SSR makers’ development became much easier than earlier (Subramanian et al., 2003). In the current study, we have developed the transcriptome data of B. changningensis, which can be used for phylogenetic analyses, variety or species identification and molecular plant breeding belonging to the Bambusa genus besides the hybrid identification studies. Earlier, using 15 EST-SSR markers, (Wu et al., 2009) identified four artificial bamboo hybrid species. Further, (Lu et al., 2009) also identified three artificial hybrid bamboos using SSR markers. Similarly, Yuan identified the artificial hybrids derived from Bambusa multiplex×B. chungii and B. multiplex × Dendrocalamus latiflorus using SSR markers (Yuan et al., 2021). Likewise, our study also determined that B. changningensis is the natural hybrid of D. farinosus and B. rigida.
4.2 Evolutionary tree based on the nuclear gene (GBSSI partial regions) provides further evidence for the natural hybrid Bambusa changningensis
Molecular phylogenetic studies have shown the surprising ability of gene trees to detect hybrids based on their branching behaviour (Sang and Zhong, 2000; Barraclough and Nee, 2001). ITS gene is one of the most widely used genes for phylogenetic analysis at the generic and infrageneric levels in plants (Álvarez and Wendel, 2003). However, some studies have shown that the variation of the ITS gene fragment is negligible in woody bamboo, and the taxa cannot be well distinguished (Guo et al., 2001). By contrast, GBSSI fragments have more variation than other DNA sequences previously used in woody bamboo (Guo and Li, 2004). Moreover, the GBSSI gene exists as a single copy and evolves more quickly in most Gramineae family genomes like rice, wheat, Leymus, Agropyron, and Arundinarieae (Soltis et al., 1998; Agafonov et al., 2021). As a single-copy nuclear gene, the GBSSI gene has been proven to exhibit higher genetic differentiation in introns of this gene than in ITS regions in closely related species (Mason-Gamer et al., 1998; Peralta and Spooner, 2001). Therefore, we chose GBSSI partial regions for hybrid identification and phylogenetic studies.
Our study identified three copies of GBSSI genes in B. changningensis. Among them, two B. changningensis genes clustered with two copies of B. rigida GBSSI genes, whereas another copy of B. changningensis GBSSI gene clustered with D. farinosus with strong support. These observations might be due to the hybridization of B. changningensis between the parents B. rigida and D. farinosus. Similar to our results, using the GBSSI gene, Goh et al. (2011) also identified a natural bamboo hybrid between D. pendulus and Gigantochloa scortechinii in Peninsular Malaysia. Further, Spooner identified allopolyploidy in wild potatoes using the GBSSI gene (Spooner et al., 2008).
4.3 Complete cp genome sequencing and an evolutionary tree based on cp genomes prove that B. rigida might be the female parent of B. changningensis
Chloroplast DNA is unaffected by genetic recombination, which is of great significance in elucidating the complex phylogenetic relationship of plants (Huang et al., 1994). Further, cp genomes have a maternal inheritance for most angiosperms, and the molecular system reconstruction based on chloroplast genome data is very effective (Gielly and Taberlet, 1994). Therefore, most researchers used cp genes or cp whole genome sequences to identify maternal parents and analyze the genetic relationship. For example, (Nandhini et al., 2013) identified interspecific hybrid Coffea congensis × Coffea canephora and its parents based on the SNPs present in the rbcL and matK. Similarly, (Khew and Chia, 2011) identified the female parent of orchid hybrid Vanda Miss Joaquim using the matK. Further, (Uchoi et al., 2016) analyzed the phylogenetic relationship of 23 Citrus genotypes using rbcL and matK genes. Chen et al. (2019) used psbA-trnH, matK and trnL sequences as DNA barcoding to establish a rapid identification of Chrysanthemum indicum and Ch. Morifolium. Yu et al. (2022) identified Pinus sylvestris as the paternal parent for P. funebris and P. takahasii through the complete cp genome (Pinaceae species cp genome inherits paternally). Park et al. (2021) identified the maternal and paternal parents in cumber two inbred lines and their F1 hybrid based on the SNPs and indels present on the cp whole-genome sequences. In our study, to avoid interference between B. changnengesis and B. ventricosa, we extracted all the genes from 24 cp genomes and concatenate them to get the final sequences matrix to establish the phylogenetic relationship. Our study also identified that B. rigida is the maternal parent of the B. changningensis based on the SNPs and indels present on the cp whole genome sequence. Interestingly, the rpoC2 gene contains SNPs or indels in the B. changningensis and also in both parents. These results might be because we sequenced the wild species of B. changningensis and don’t know the exact individual parents. These minor variations in the SNPs and indels in the cp genome of wild species might be possible (Dally and Second, 2009). The rpoC2 gene can be considered as the fast-evolving region in these bamboo species that could be used for further evolutionary studies. In conclusion, our results identified that B. changningensis is the natural hybrid between the parents B. rigida × D. farinosus. These findings pave a path toward bamboo speciation and evolutionary studies. The SSR markers developed in this study could be practically used for genotyping of Bambusa and Dendrocalamus genus plants.
Data availability statement
The data presented in the study are deposited in the NCBI repository, accession number PRJNA824949 (https://www.ncbi.nlm.nih.gov/genbank/), OM065947 (https://www.ncbi.nlm.nih.gov/genbank/).
Author contributions
XL, JZ and YZ, DH designed the experiments; JZ performed the experiments; JZ and NV analyzed the data and wrote the manuscript; YW, GZ, HB and BL provided the experimental materials; XL supervised and administrated the project. All the authors have read and approved the final manuscript.
Funding
This work was supported by grants from Study on tissue culture System construction and molecular biology of bamboo for fine fiber (2022NY027); the Research Fund for International Young Scientist by National Natural Science Foundation of China (32150410354).
Conflict of interest
The authors declare that the research was conducted in the absence of any commercial or financial relationships that could be construed as a potential conflict of interest.
Publisher’s note
All claims expressed in this article are solely those of the authors and do not necessarily represent those of their affiliated organizations, or those of the publisher, the editors and the reviewers. Any product that may be evaluated in this article, or claim that may be made by its manufacturer, is not guaranteed or endorsed by the publisher.
Supplementary material
The Supplementary Material for this article can be found online at: https://www.frontiersin.org/articles/10.3389/fpls.2023.1231940/full#supplementary-material
Supplementary Figure 1 | Alignment of B. rigida, B. changningensis, and D. farinosus allele sequences. (A) SSR14; (B) SSR28. (C) SSR31; (D) SSR 34. * Indicates the mutation sites. -Indicates the deletion sites.
References
Agafonov, A. V., Emtseva, M. V., Shabanova, E. V. (2021). Microevolutionary relationships between biotypes of Elymus confusus, E. peschkovae, and E. sibiricus (Poaceae) according to hybridization and sequencing of the nuclear gene GBSS1 (waxy). Bio Web Conf. 38, 6. doi: 10.1051/bioconf/20213800002
Álvarez, I., Wendel, J. F. (2003). Ribosomal ITS sequences and plant phylogenetic inference. Mol. Phylogenet. Evol. 29 (3), 417–434. doi: 10.1016/S1055-7903(03)00208-2
Barraclough, T. G., Nee, S. (2001). Phylogenetics and speciation. Trends Ecol. Evol. 16 (7), 391–399. doi: 10.1016/S0169-5347(01)02161-9
Beier, S., Thiel, T., Münch, T., Scholz, U., Mascher, M. (2017). MISA-web: a web server for microsatellite prediction. Bioinformatics. 33 (16), 2583–2585. doi: 10.1093/bioinformatics/btx198
Bhandawat, A., Singh, G., Raina, A. S., Kaur, J., Sharma, R. K. (2016). Development of genic SSR marker resource from RNA-Seq data in Dendrocalamus latiflorus. J. Plant Biochem. Biotechnol. 25 (2), 179–190. doi: 10.1007/s13562-015-0323-9
Cai, K., Zhu, L. F., Zhang, K. K., Li, L., Zhao, Z. Y., Zeng, W., et al. (2019). Development and characterization of EST-SSR markers from RNA-Seq data in Phyllostachys violascens. Front. Plant Sci. 10, 50. doi: 10.3389/fpls.2019.00050
Chen, R. Y. (2003). Chromosome atlas of Chinese principal economic plants IV (Beijing, China: Science Press).
Chen, F. R., Wang, T., Guo, Q. S., Zhu, Z. B., Yang, F., Zou, Q. J., et al. (2019). Identification of Chrysanthemum indicum in different geographical populations and Ch. morifolium based on DNA barcodes of psbA-trnH, matK and trnL. China J. Chin. Materia. Medica. 44 (4), 660–665. doi: 10.19540/j.cnki.cjcmm.2019.0015
Dally, A. M., Second, G. (2009). Chloroplast DNA diversity in wild and cultivated species of rice (Genus Oryza, section Oryza). Cladistic-mutation and genetic-distance analysis. Theor. Appl. Genet. 80 (2), 209–222. doi: 10.1007/BF00224389
Das, M., Bhattacharya, S., Basak, J., Pal, A. (2007). Phylogenetic relationships among the bamboo species as revealed by morphological characters and polymorphism analyses. Biol. Plantarum. 51 (4), 667–672. doi: 10.1007/s10535-007-0140-7
Davidson, N. M., Oshlack, A. (2014). Corset: enabling differential gene expression analysis for de novo assembled transcriptomes. Genome Biol. 15 (7), 1–14. doi: 10.1186/s13059-014-0410-6
Doyle, J. (1991). DNA protocols for plants. Mol. Biol. Evol. 57, 283–293. doi: 10.1007/978-3-642-83962-7_18
Gielly, L., Taberlet, P. (1994). The use of chloroplast DNA to resolve plant phylogenies: noncoding versus rbcL sequences. Mol. Biol. Evol. 11 (5), 769–777. doi: 10.1016/0303-7207(94)90126-0
Goh, W. L., Chandran, S., Franklin, D. C., Isagi, Y., Koshy, K. C., Sungkaew, S., et al. (2013). Multi-gene region phylogenetic analyses suggest reticulate evolution and a clade of Australian origin among paleotropical woody bamboos (Poaceae: Bambusoideae: Bambuseae). Plant Syst. Evol. 299, 239–257. doi: 10.1007/s00606-012-0718-1
Goh, W. L., Chandran, S., Kamiya, K., Wong, K. M. (2011). A natural hybrid between Dendrocalamus pendulus and Gigantochloa scortechinii (Poaceae: Bambusoideae: Bambuseae) in Peninsular Malaysia. Gard Bull. Singapore. 62 (2), 223–238. Available at: http://biostor.org/reference/140254.
Grabherr, M. G., Haas, B. J., Yassour, M., Levin, J. Z., Thompson, D. A., Amit, I., et al. (2011). Full-length transcriptome assembly from RNA-Seq data without a reference genome. Nat. Biotechnol. 29, 644–652. doi: 10.1038/nbt.1883
Guo, Z. H., Chen, Y. Y., Li, D. Z., Yang, J. B. (2001). Genetic variation and evolution of the alpine bamboos (Poaceae: bambusoideae) using DNA sequence data. J. Plant Res. 114 (3), 315–322. doi: 10.1007/PL00013993
Guo, Z. H., Li, D. Z. (2004). Phylogenetics of the Thamnocalamus group and its allies (Gramineae: Bambusoideae): inference from the sequences of GBSSI gene and ITS spacer. Mol. Phylogenet. Evol. 30 (1), 1–12. doi: 10.1016/S1055-7903(03)00161-1
Guo, Z. H., Ma, P. F., Yang, G. Q. (2019). Genome sequences provide insights into the reticulate origin and unique traits of woody bamboos. Mol. Plant 12 (10), 1353–1365. doi: 10.1016/j.molp.2019.05.009
Hahn, C., Bachmann, L., Chevreux, B. (2013). Reconstructing mitochondrial genomes directly from genomic next-generation sequencing reads-a baiting and iterative mapping approach. Nucleic Acids Res. 41 (13), e129. doi: 10.1093/nar/gkt371
Hegarty, M. J., Hiscock, S. J. (2005). Hybrid speciation in plants: new insights from molecular studies. New Phytol. 165 (2), 411–423. doi: 10.1111/j.1469-8137.2004.01253.x
Horiike, T. (2016). An introduction to molecular phylogenetic analysis. Rev. Agri Sci. 4, 36–45. doi: 10.7831/ras.4.0_36
Hou, D., Li, L., Ma, T. F., Pei, J. L., Zhao, Z. Y., Lu, M., et al. (2021). The SOC1-like gene BoMADS50 is associated with the flowering of Bambusa oldhamii. Hortic. Res. 8, 133. doi: 10.1038/s41438-021-00557-4
Hu, S. L., Jiang, Y., Chen, Q. B., Lu, X. Q., Sun, X., Cao, Y., et al. (2009). Rapd and issr analysis of bambusa rigida from the seven different regions in sichuan province. Hubei Agricultural Sciences. doi: 10.14088/j.cnki.issn0439-8114.2009.01.055
Huang, Y., Li, C. L., Ma, C., Wu, N. H. (1994). Chloroplast DNA and its application to plant systematic studies. Chin. Bull. Bot. 11 (2), 11–25. doi: 10.1007/bf02344252
Janzen, D. H. (1976). Why bamboos wait so long to flower. Annu. Rev. Ecol. Syst. 7, 347–391. doi: 10.1146/annurev.es.07.110176.002023
Jiang, Y., Hu, S. L., Cheng, Q. B., Lu, X. Q., Li, Y., Cao, Y., et al. (2008). Studies on genetic diversity of Dendrocalamus farinosus from the different regions in Sichuan Province by RAPD and ISSR markers. J. Fujian Coll. Forestry. 28 (3), 276–280. doi: 10.1016/S1872-2040(08)60061-4
Jiang, K. Y., Zhou, M. B. (2014). Recent advances in bamboo molecular biology. J. Trop. Subtrop. Bot. 22 (6), 632–642. doi: 10.11926/j.issn.1005–3395.2014.06.012
Katoh, K., Standley, D. M. (2013). MAFFT multiple sequence alignment software version 7: improvements in performance and usability. Mol. Biol. Evol. 30 (4), 772–780. doi: 10.1093/molbev/mst010
Khew, G. S. W., Chia, T. F. (2011). Parentage determination of Vanda Miss Joaquim (Orchidaceae) through two chloroplast genes rbcL and matK. AoB Plants, plr018. doi: 10.1093/aobpla/plr018
Kumar, S., Stecher, G., Li, M., Knyaz, C., Tamura, K. (2018). MEGA X: molecular evolutionary genetics analysis across computing platforms. Mol. Biol. Evol. 35 (6), 1547–1549. doi: 10.1093/molbev/msy096
Lichtenthaler, H. K. (1987). Chlorophylls and carotenoids: Pigments of photosynthetic biomembranes. Meth Enzymol. 148, 350–382. doi: 10.1016/0076-6879(87)48036-1
Lin, X. C., Lou, Y. F., Liu, J., Peng, J. S., Liao, G. L., Fang, W. (2010). Crossbreeding of Phyllostachys species (Poaceae) and identification of their hybrids using ISSR markers. GMR. 9 (3), 1398–1404. doi: 10.4238/vol9-3gmr855
Lu, J. J., Katsuhiko, Y., Fang, W., Tang, D. Q. (2009). Identification of the hybrid bamboo F1 by SSR markers. Scientia Silvae Sinicae. 45 (3), 29–34. doi: 10.1007/978-1-4020-9623-5_5
Manandhar, R., Kim, J. H., Kim, J. T. (2019). Environmental, social and economic sustainability of bamboo and bamboo-based construction materials in buildings. J. Asian Archit. Build. 18 (2), 49–59. doi: 10.1080/13467581.2019.1595629
Maruyama, I., Okamura, H., Murata, G. (1979). On a new hybrid genus Hibanobambusa. Acta Phytotax. Geobot. 30, 148–152. doi: 10.18942/bunruichiri.KJ00001078327
Mason-Gamer, R. J., Weil, C. F., Kellogg, E. A. (1998). Granule-bound starch synthase: structure, function, and phylogenetic utility. Mol. Biol. Evol. 15 (12), 1658–1673. doi: 10.1093/oxfordjournals.molbev.a025893
Meena, R. K., Bhandhari, M. S., Barhwal, S., Ginwal, H. S. (2019). Genetic diversity and structure of Dendrocalamus hamiltonii natural metapopulation: a commercially important bamboo species of northeast Himalayas. 3 Biotech. 9, 60. doi: 10.1007/s13205-019-1591-1
Mizuki, I., Sato, A., Matsuo, A., Suyama, Y., Suzuki, J. I., Makita, A. (2014). Clonal structure, seed set, and self-pollination rate in mass-flowering bamboo species during off-year flowering events. PloS One 9 (8), e105051. doi: 10.1371/journal.pone.0105051
Momotaz, A., Forster, J. W., Yamada, T. (2004). Identification of cultivars and accessions of Lolium, Festuca and Festulolium hybrids through the detection of simple sequence repeat polymorphism. Plant Breeding. 123 (4), 370–376. doi: 10.1111/j.1439-0523.2004.00962.x
Muramatsu, M. (1981). Hybridization among Bambusaceae species. In Bamboo production and utilization, 65–69. Proceedings of the Congress Group 5.3A, Production and utilization of bamboo and related species, XVII IUFRO [International Union of Forestry Research Organization] World Congress, Kyoto, Japan. IUFRO, Vienna, Austria.
Nandhini, R. B., Rahul, R. N., Thilaga, S., Rao, N. S. P., Ganesh, D. (2013). Molecular distinction of C× R hybrid (Coffea congensis× Coffea canephora) from morphologically resembling male parent using rbcL and matK gene sequences. S Afr J. Bot. 88, 334–340. doi: 10.1016/j.sajb.2013.08.011
Park, H. S., Lee, W. K., Lee, S. C., Lee, H. O., Joh, H. J., Park, J. Y., et al. (2021). Inheritance of chloroplast and mitochondrial genomes in cucumber revealed by four reciprocal F1 hybrid combinations. Sci. Rep. 11 (1), 2506. doi: 10.1038/s41598-021-81988-w
Paun, O., Forest, F., Fay, M. F., Chase, M. K. (2009). Hybrid speciation in angiosperms: parental divergence drives ploidy. New Phytol. 182, 507–518. doi: 10.1111/j.1469-8137.2009.02767.x
Peralta, I. E., Spooner, D. M. (2001). Granule-bound starch synthase (GBSSI) gene phylogeny of wild tomatoes (Solanum L. section Lycopersicon [Mill.] Wettst. subsection Lycopersicon). Am. J. Bot. 88 (10), 1888–1902. doi: 10.2307/3558365
Pharmawati, M., Yan, G., Sedgley, R., Finnegan, P. M. (2004). Chloroplast DNA inheritance and variation in Leucadendron species (Proteaceae) as revealed by PCR-RFLP. Theor. Appl. Genet. 109, 1694–1701. doi: 10.1007/s00122-004-1800-z
Qu, X. J., Moore, M. J., Li, D. Z., Yi, T. S. (2019). PGA: a software package for rapid, accurate, and flexible batch annotation of plastomes. Plant Methods 15, 50. doi: 10.1186/s13007-019-0435-7
Ramakrishnan, M., Yrjälä, K., Vinod, K. K., Sharma, A., Cho, J., Satheesh, V., et al. (2020). Genetics and genomics of moso bamboo (Phyllostachys edulis): Current status, future challenges, and biotechnological opportunities toward a sustainable bamboo industry. Food Energy Secur. 9 (4), e229. doi: 10.1002/fes3.229
Rieseberg, L. H. (1995). The role of hybridization in evolution: old wine in new skins. Am. J. Bot. 82 (7), 944–953. doi: 10.1002/j.1537-2197.1995.tb15711.x
Rychlik, W. (2007). OLIGO 7 primer analysis software. Methods Mol. Biol. 402, 35–39. doi: 10.1007/978-1-59745-528-2_2
Sang, T., Zhong, Y. (2000). Testing hybridization hypotheses based on incongruent gene trees. Syst. Biol. 49 (3), 422–434. doi: 10.1080/10635159950127321
Singh, A. K., Singh, L., Kumar, C., Kumar, P., Dimree, S. K. (2010). Para grass hybrid (Brachiaria sp.) – A potential forage for India. Environ. Ecol. 28 (3), 1602–1606. doi: 10.1109/78.875477
Soltis, D. E., Soltis, P. S., Doyle, J. J. (1998). Molecular Systematics of Plants II: DNA Sequencing. London: Chapman and Hall Press. doi: 10.1007/978-1-4615-5419-6
Spooner, D. M., Rodríguez, F., Polgár, Z., Ballard, H. E., Jansky, S. H. (2008). Genomic origins of potato polyploids: GBSSI gene sequencing data. Crop Science. 48 (S1), S27–S36. doi: 10.2135/cropsci2007.09.0504tpg
Stebbins, G. L. (1956). Cytogenetics and evolution of the grass family. Am. J. Bot. 43 (10), 890–905. doi: 10.1002/j.1537-2197.1956.tb11182.x
Subramanian, S., Mishra, R. K., Singh, L. (2003). Genome-wide analysis of microsatellite repeats in humans: their abundance and density in specific genomic regions. Genome Biol. 4 (2), 1–10. doi: 10.1186/gb-2003-4-2-r13
Sundaram, R. M., Naveenkumar, B., Biradar, S. K., Balachandran, S. M., Mishra, B., IlyasAhmed, M., et al. (2008). Identification of informative SSR markers capable of distinguishing hybrid rice parental lines and their utilization in seed purity assessment. Euphytica. 163, 215–224. doi: 10.1007/s10681-007-9630-0
Swofford, D. L. (2008). PAUP (phylogenetic analysis using parsimony). In: Encyclopedia of Genetics, Genomics, Proteomics and Informatics. Dordrecht: Springer. doi: 10.1007/978-1-4020-6754-9_12413
Tian, X., Li, D. Z. (2002). Application of DNA sequences in plant phylogenetic study. Acta Botanica Yunnanica. 24 (2), 170–184. doi: 10.3969/j.issn.2095-0845.2002.02.004
Triplett, J. K., Oltrogge, K. A., Clark, L. G. (2010). Phylogenetic relationships and natural hybridization among the North American woody bamboos (Poaceae: Bambusoideae: Arundinaria). Am. J. Bot. 97 (3), 471–492. doi: 10.3732/ajb.0900244
Uchoi, A., Malik, S. K., Choudhary, R., Kumar, S., Rohini, M. R., Pal, D., et al. (2016). RETRACTION: Inferring Phylogenetic Relationships of Indian Citron (Citrus medica L.) based on rbcL and matK Sequences of Chloroplast DNA. Biochem. Genet. 54 (3), 249–269. doi: 10.1007/s10528-019-09939-9
Wang, Y., Lei, Y. C., Chen, P., Zhou, G. Q., Yu, Y. (2020). Analysis of Fiber Morphology and Pulping Properties of Bambusa changningensis Yi et B. X. Li. Word Bamboo And Rattan. 18 (5), 51.
Wang, Y., Zhou, G. Q., Jia, T. B., He, X. B., Hu, Y. B. (2016). Studies on biological and ecological characteristics of Bambusa Changningsis. J. Sichuan For Sci. Tech. 37 (06), 94–96. doi: 10.16779/j.cnki.1003-5508.2016.06.021
Winkworth, R. C., Donoghue, M. J. (2004). Viburnum phylogeny: evidence from the duplicated nuclear gene GBSSI. Mol. Phylogenet. Evol. 33 (1), 109–126. doi: 10.1016/j.ympev.2004.05.006
Wolfe, K. H., Li, W. H., Sharp, P. M. (1987). Rates of nucleotide substitution vary greatly among plant mitochondrial, chloroplast, and nuclear DNAs. PNAS. 84 (24), 9054–9058. doi: 10.1073/pnas.84.24.9054
Wu, M. D., Dong, W. J., Tang, D. Q. (2009). Identification of four caespitose hybrid bamboos by using SSR markers. Mol. Plant Breeding. 7 (5), 959–965. doi: 10.3969/mpb.007.000959
Wu, Y. D., Wang, Y., Fan, X. C., Zhang, Y, Jiang, J. F., Sun, L, et al. (2023). QTL mapping for berry shape based on a high-density genetic map constructed by whole-genome resequencing in grape. Hortic. Res. 9 (4), 729–742. doi: 10.1016/J.HPJ.2022.11.005
Ye, J. (2010). A preliminary study on the origin of natural hybridization of Bambusa subgen. Dendrocalamopsis. [master’s thesis] [China(Beijing)]: Chinese Academy of Sciences.
Yi, T. P., Li, B. X. (2012). Bambusa Changningensis Yi et B.X.Li——a new Species (Babusoideae, Poaceae) of Economical Bamboos in China. J. Sichuan For. Sci. Technology. 33 (3), 7–10. doi: 10.3969/j.issn.1003-5508.2012.03.002
Yu, T., Jia, Z. Y., Dayananda, B., Li, J. Q., Guo, X. L., Shi, L., et al. (2022). Analysis of the chloroplast genomes of four Pinus species in Northeast China: Insights into hybrid speciation and identification of DNA molecular markers. J. For. Res. 33, 1881–1890. doi: 10.1007/s11676-021-01432-7
Yuan, J., Ma, J. X., Zhong, Y. B., Yue, J. J. (2021). SSR-based hybrid identification, genetic analyses and fingerprint development of hybridization progenies from sympodial bamboo (Bambusoideae, Poaceae). J. Nanjing For Univ(Nat Sci. Ed). 45 (5), 10–18. doi: 10.12302/j.issn.1000-2006.202012046
Zhang, S., Tang, D. Q. (2007). A review on application of DNA marker in bamboos and its limits. J. Bamboo Res. 26 (1), 10–14. doi: 10.3969/j.issn.1000-6567.2007.01.003
Zheng, W., Gao, D. H., Wang, L. Y. (2009). Review of plant hybrid origination and its research progress. J. Anhui Agri Sci. 37 (22), 10378–10381. doi: 10.3969/j.issn.0517-6611.2009.22.022
Zheng, Y., Hou, D., Zhuo, J., Zheng, R. H., Wang, Y., Li, B. X., et al. (2020). Complete chloroplast genome sequence of Bambusa rigida (Bambuseae). Mitochondrial DNA B Resour 5 (3), 2972–2973. doi: 10.1080/23802359.2020.1793699
Zheng, X., Lin, S. Y., Fu, H. J., Wan, Y. W., Ding, Y. L. (2020). The bamboo flowering cycle sheds light on flowering diversity. Front. Plant Sci. 11, 381. doi: 10.3389/fpls.2020.00381
Zheng, W., Yan, L. J., Burgess, K. S., Luo, Y. H., Zou, J. Y., Qin, H. T., et al. (2021). Natural hybridization among three Rhododendron species (Ericaceae) revealed by morphological and genomic evidence. BMC Plant Biol. 21 (1), 1–12. doi: 10.1186/s12870-021-03312-y
Zhou, G. Q., Wang, Y., Jia, T. B. (2020). Superior Clone Selection and Breeding for Bambusa changningensis Yi et B. X. Li. Word Bamboo And Rattan. 18 (4), 30–34. doi: 10.12168/sjzttx.2020.04.006
Keywords: natural hybrid bamboo, transcriptomic data, SSR, GBSSI, chloroplast genome
Citation: Zhuo J, Vasupalli N, Wang Y, Zhou G, Gao H, Zheng Y, Li B, Hou D and Lin X (2023) Molecular identification of Bambusa changningensis is the natural bamboo hybrid of B. rigida × Dendrocalamus farinosus. Front. Plant Sci. 14:1231940. doi: 10.3389/fpls.2023.1231940
Received: 31 May 2023; Accepted: 09 August 2023;
Published: 01 September 2023.
Edited by:
Christopher John Lambrides, The University of Queensland, AustraliaReviewed by:
Eduardo Ruiz-Sanchez, University of Guadalajara, MexicoXiaoxuan Tian, Tianjin University of Traditional Chinese Medicine, China
Diaga Diouf, Cheikh Anta Diop University, Senegal
Copyright © 2023 Zhuo, Vasupalli, Wang, Zhou, Gao, Zheng, Li, Hou and Lin. This is an open-access article distributed under the terms of the Creative Commons Attribution License (CC BY). The use, distribution or reproduction in other forums is permitted, provided the original author(s) and the copyright owner(s) are credited and that the original publication in this journal is cited, in accordance with accepted academic practice. No use, distribution or reproduction is permitted which does not comply with these terms.
*Correspondence: Xinchun Lin, bGlueGN4QDE2My5jb20=
†These authors have contributed equally to this work