- 1Univ Rouen Normandie, Laboratoire Glyco-MEV UR 4358, Rouen, France
- 2Dipartimento di Biologia e Biotecnologie “Charles Darwin”, Sapienza Università di Roma, Rome, Italy
- 3Centro di Ricerca per le Scienze applicate alla Protezione dell’Ambiente e dei Beni Culturali (CIABC), Sapienza Università di Roma, Rome, Italy
Editorial on the Research Topic
Plant cell wall in pathogenesis, parasitism and symbiosis, Volume II
A wide range of organisms that interact with plants must interface with the plant cell wall (CW) (Lionetti and Metraux, 2014). The view of the CW as only a static cellular barrier in these interactions is outdated. Cell wall polysaccharides, phenolic compounds, and proteins, in addition to regulating important growth and development processes, are also sources of elicitors that activate cell signaling pathways (Nguema-Ona et al., 2013). Surveillance mechanisms detect CW contacts with other organisms, and specific signaling pathways and responses are activated (Swaminathan et al., 2022) (Figure 1). During biotic interactions and abiotic stresses, the structure and composition of plant CW can be regulated at the biosynthetic level and through precise, continuous post-synthetic remodeling. As a consequence, the CW must be understood as a strategic space between organisms where intelligent and dynamic molecular strategies are implemented to overwhelm a fight or cooperate for specific physiological processes (Bacete et al., 2018; Castilleux et al., 2018; De Lorenzo et al., 2019).
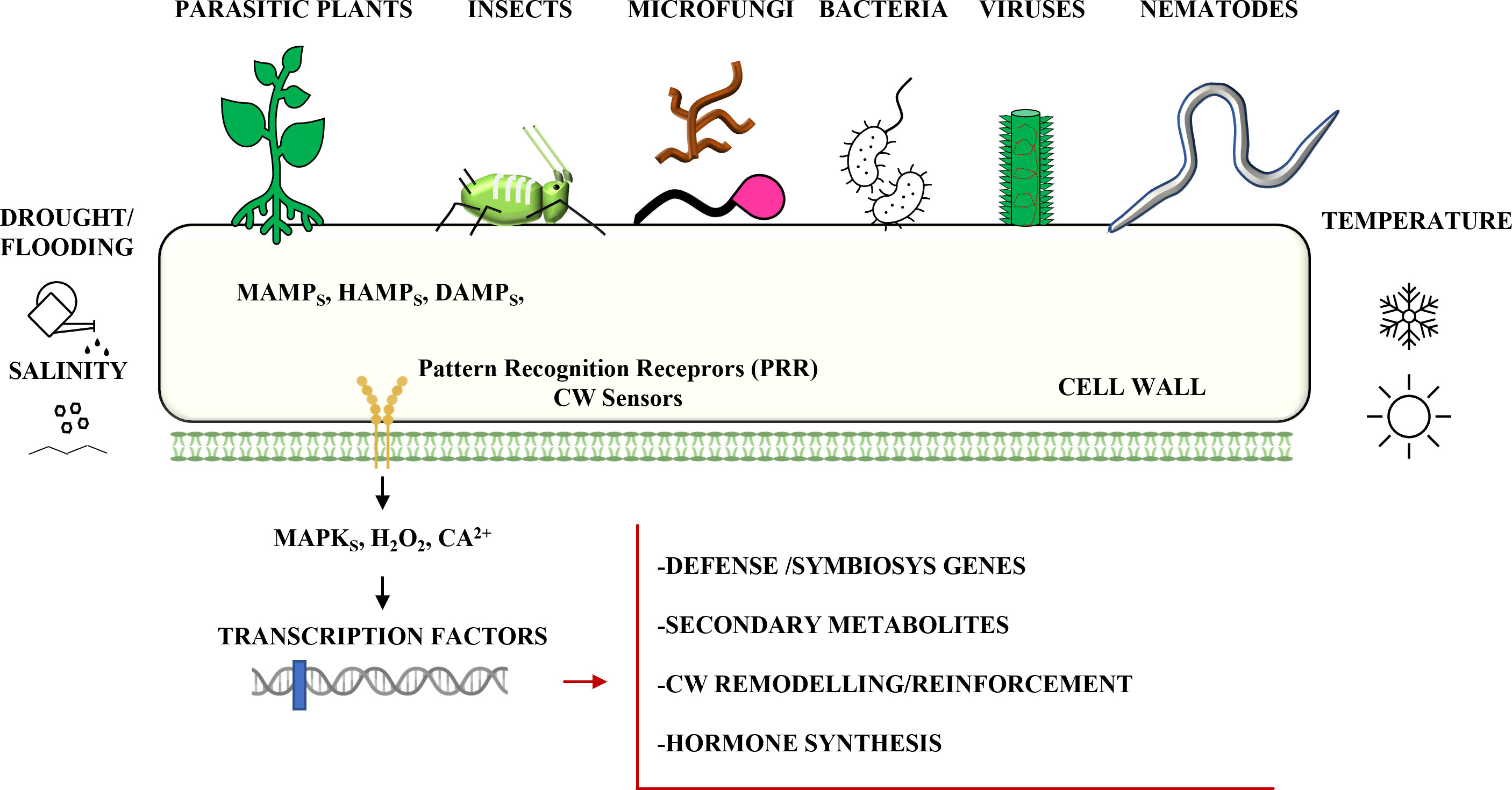
Figure 1 Schematic representation of the main systems of perception and responses related to the plant cell wall (CW) in the different biotic interactions. CW can play a central role in combined biotic and abiotic stress. Symbiont-induced CW modifications can improve plant development, nutrition, and tolerance to abiotic stresses. Abiotic stresses can alter CW composition, affecting efficient parasitism. M/H/DAMPS=Microbe/Herbivore/Damage Associated Molecular Patterns.
Cell wall enzymes and their inhibitors play key roles in apoplastic metabolism (Rui and Dinneny, 2020). Invertases (INVs) and pectin methylesterases (PMEs) play essential roles in carbohydrate metabolism, stress responses, and sugar signaling (Bellincampi et al., 2014; Tauzin and Giardina, 2014; Del Corpo et al., 2020). In this collection, Coculo and Lionetti reviewed the roles of invertase inhibitors (INVI) and pectin methylesterase inhibitors (PMEI) belonging to the “Plant Invertase/Pectin Methylesterase Inhibitor Superfamily” (Lionetti et al., 2017). An updated overview of the specific activity of the characterized isoforms, their specific functions in plant physiology, and their applications in biotechnology is provided. After the pioneering work in 2007 (Lionetti et al., 2007), several piece of evidence supported the role of PMEIs in plant resistance to stresses (An et al., 2008; Liu et al., 2018). With a genome-wide analysis and transcriptomics of the PMEI genes in Brassica napus, Wang et al. identified several BnPMEIs as resistance gene candidates in response to Sclerotinia sclerotiorum, suggesting them as possible tools to breed new and improved genotypes more resistant to Sclerotinia stem rot.
Specific CW changes can occur in the vicinity of plasmodesmata during viral infection (Lionetti et al., 2015; Stavolone and Lionetti, 2017). Viruses can modify pectin, callose, and structural proteins near plasmodesmata by increasing their size exclusion limit (Kozieł et al., 2021). López-González et al. addressed the little-explored impact of virus infections on secondary CW and plant development. The authors found a correlation between the developmental alterations induced in Arabidopsis by specific strains of turnip mosaic virus and specific changes in xylan and lignin biosynthesis. Although callose deposition is a response to both elicitors and pathogens, the mechanisms involved in its biosynthesis and degradation remain to be unraveled (German et al., 2023). Li et al. summarized the research progress on plant callose and its synthesizing enzymes in plant physiology.
Microfungi are also important etiological agents for plants (Doehlemann et al., 2017). The fungal pathogen Sphaerulina musiva causes stem canker with the consequent mortality of Populus trees. Populus deltoides can induce a lignified periderm to contain the pathogen, but the precise characterization of lignin changes in response to S. musiva infection is unknown. Bryant et al.identified a higher syringyl:guaiacil ratio, a higher Klason lignin content and lower p-hydroxybenzoate content in Septoria-infected P. deltoides trees compared to the healthy plant. This knowledge can favor biotechnological approaches aimed at improving the resilience and increasing the biomass yield of Populus for biofuel production.
Interesting contributions in this collection concern plant-parasite interactions. A fine-tuned re-arrangement of host CW is induced in response to infection by both plant-parasitic cyst nematodes and root-knot nematodes (Zhang et al., 2017; Bozbuga et al., 2018; Meidani et al., 2019). Veronico et al. found that drought stress affects CW metabolism in tomato roots, limiting feeding site development and reproduction of the nematode Meloidogyne incognita. Parasitic plants, such as Cuscuta species, severely damage economically important crops (Jhu and Sinha, 2022). These green parasites absorb resources through an invasive organ called the haustorium, which differentiates into vascular hyphae that establish a connection with the host plant’s vasculature. The degradation and modification of host CWs allow haustorium to effectively invade host tissues. Yokoyama et al. propose that Cuscuta campestris APETALA2/ETHYLENE RESPONSE FACTORS (ERFs) can activate the transcription of the CW enzymatic genes in haustorium to favor its invasion of tobacco and Arabidopsis plants.
Plant CW is a field of molecular dialogues and agreements with symbiotic microbes to establish intimate interfaces for developmental coordination and nutrient exchange (Balestrini and Bonfante, 2014). Plants can establish mutualistic symbiosis with arbuscular mycorrhizal fungi, and phosphorus transfer across the CW specialized interfacial compartment is an important process in the mycorrhizal pathway (Begum et al., 2019). Exploiting the Rhizophagus irregularis-Lotus japonicus interaction, Nguyen and Saito showed that polyphosphate in fungal CWs and apoplastic phosphatases play an important role in phosphorus transfer at the symbiotic interface in arbuscules. Mycorrhizal fungi can be involved in mutualistic interactions during orchid seed germination (Põlme et al., 2018). Chen et al. identified several genes codifying CW structural proteins such as epidermis-specific secreted glycoprotein, proline-rich receptor-like protein, and leucine-rich repeat (LRR) extensin-like protein, which are particularly involved in the symbiosis of Tulasnella and Serendipita fungi with Dendrobium officinale.
Bioinoculants represent an environmentally-friendly agricultural practice to alleviate drought stress in crops (Kour et al., 2022). The work presented by Wilmowitz et al. indicates that the inoculation of maize seeds with Glomus sp. and Bacillus sp. can help to cope with drought stress, preventing inhibition of photosynthesis and disruption of redox balance. Glomus sp. and Bacillus sp. can modify pectin methylesterification and hemicellulose content of maize leaves, possibly leading to alleviation of the negative effects of drought.
These important contributions advance our understanding of the relationships between plants and the environment at the CW interface, which will be helpful to engineer biotechnological strategies for agriculture and bioenergy fields. As a closing remark, we are grateful to the authors and reviewers for their invaluable contributions to this Research Topic.
Author contributions
VL draft the editorial text. MV revised and approved the final version of the editorial. All authors approved the submitted version.
Funding
The research was supported by Sapienza University of Rome, Grants RM120172B78CFDF2, RM11916B7A142CF1, RG12117A898EABE0, RM122181424F1F42 and - Rome Technopole” - European Union Next-GenerationEU PNRR -RT12218451D2C509 to VL.
Conflict of interest
The authors declare that the research was conducted in the absence of any commercial or financial relationships that could be construed as a potential conflict of interest.
Publisher’s note
All claims expressed in this article are solely those of the authors and do not necessarily represent those of their affiliated organizations, or those of the publisher, the editors and the reviewers. Any product that may be evaluated in this article, or claim that may be made by its manufacturer, is not guaranteed or endorsed by the publisher.
References
An, S. H., Sohn, K. H., Choi, H. W., Hwang, I. S., Lee, S. C., Hwang, B. K. (2008). Pepper pectin methylesterase inhibitor protein CaPMEI1 is required for antifungal activity, basal disease resistance and abiotic stress tolerance. Planta 228, 61–78. doi: 10.1007/s00425-008-0719-z
Bacete, L., Mélida, H., Miedes, E., Molina, A. (2018). Plant cell wall-mediated immunity: cell wall changes trigger disease resistance responses. Plant J. 93, 614–636. doi: 10.1111/tpj.13807
Balestrini, R., Bonfante, P. (2014). Cell wall remodeling in mycorrhizal symbiosis: a way towards biotrophism. Front.Plant Sci. 5. doi: 10.3389/fpls.2014.00237
Begum, N., Qin, C., Ahanger, M. A., Raza, S., Khan, M. I., Ashraf, M., et al. (2019). Role of arbuscular mycorrhizal fungi in plant growth regulation: implications in abiotic stress tolerance. Front. Plant Sci. 10. doi: 10.3389/fpls.2019.01068
Bellincampi, D., Cervone, F., Lionetti, V. (2014). Plant cell wall dynamics and wall-related susceptibility in plant-pathogen interactions. Front.Plant Sci. 5, 228. doi: 10.3389/fpls.2014.00228
Bozbuga, R., Lilley, C. J., Knox, J. P., Urwin, P. E. (2018). Host-specific signatures of the cell wall changes induced by the plant parasitic nematode, meloidogyne incognita. Sci. Rep. 8, 17302. doi: 10.1038/s41598-018-35529-7
Castilleux, R., Plancot, B., Ropitaux, M., Carreras, A., Leprince, J., Boulogne, I., et al. (2018). Cell wall extensins in root–microbe interactions and root secretions. J. Exp. Bot. 69, 4235–4247. doi: 10.1093/jxb/ery238
Del Corpo, D., Fullone, M. R., Miele, R., Lafond, M., Pontiggia, D., Grisel, S., et al. (2020). AtPME17 is a functional arabidopsis thaliana pectin methylesterase regulated by its PRO region that triggers PME activity in the resistance to botrytis cinerea. Mol. Plant Pathol. 21, 1620–1633. doi: 10.1111/mpp.13002
De Lorenzo, G., Ferrari, S., Giovannoni, M., Mattei, B., Cervone, F. (2019). Cell wall traits that influence plant development, immunity, and bioconversion. Plant J. 97, 134–147. doi: 10.1111/tpj.14196
Doehlemann, G., Ökmen, B., Zhu, W., Sharon, A. (2017). Plant pathogenic fungi. Microbiol. Spectr. 5, 5.1.14. doi: 10.1128/microbiolspec.FUNK-0023-2016
German, L., Yeshvekar, R., Benitez-Alfonso, Y. (2023). Callose metabolism and the regulation of cell walls and plasmodesmata during plant mutualistic and pathogenic interactions. Plant Cell Environ. 46, 391–404. doi: 10.1111/pce.14510
Jhu, M.-Y., Sinha, N. R. (2022). Parasitic plants: an overview of mechanisms by which plants perceive and respond to parasites. Annu. Rev. Plant Biol. 73, 433–455. doi: 10.1146/annurev-arplant-102820-100635
Kour, D., Khan, S. S., Kaur, T., Kour, H., Singh, G., Yadav, A., et al. (2022). Drought adaptive microbes as bioinoculants for the horticultural crops. Heliyon 8, e09493. doi: 10.1016/j.heliyon.2022.e09493
Kozieł, E., Otulak-Kozieł, K., Bujarski, J. J. (2021). Plant cell wall as a key player during resistant and susceptible plant-virus interactions. Front. Microbiol. 12. doi: 10.3389/fmicb.2021.656809
Lionetti, V., Fabri, E., De Caroli, M., Hansen, A. R., Willats, W. G. T., Piro, G., et al. (2017). Three pectin methylesterase inhibitors protect cell wall integrity for arabidopsis immunity to botrytis. Plant Physiol. 173, 1844–1863. doi: 10.1104/pp.16.01185
Lionetti, V., Metraux, J. P. (2014). Plant cell wall in pathogenesis, parasitism and symbiosis. Front.Plant Sci. 5. doi: 10.3389/fpls.2014.00612
Lionetti, V., Raiola, A., Camardella, L., Giovane, A., Obel, N., Pauly, M., et al. (2007). Overexpression of pectin methylesterase inhibitors in arabidopsis restricts fungal infection by botrytis cinerea. Plant Physiol. 143, 1871–1880. doi: 10.1104/pp.106.090803
Lionetti, V., Raiola, A., Cervone, F., Bellincampi, D. (2015). How do pectin methylesterases and their inhibitors affect the spreading of tobamovirus? Plant Signal.Behav. 9, e972863. doi: 10.4161/15592316.2014.972863
Liu, N., Sun, Y., Pei, Y., Zhang, X., Wang, P., Li, X., et al. (2018). A pectin methylesterase inhibitor enhances resistance to verticillium Wilt1[OPEN]. Plant Physiol. 176, 2202–2220. doi: 10.1104/pp.17.01399
Meidani, C., Ntalli, N. G., Giannoutsou, E., Adamakis, I.-D. S. (2019). Cell wall modifications in giant cells induced by the plant parasitic nematode meloidogyne incognita in wild-type (Col-0) and the fra2 arabidopsis thaliana katanin mutant. Int. J. Mol. Sci. 20, 5465. doi: 10.3390/ijms20215465
Nguema-Ona, E., Vicré-Gibouin, M., Cannesan, M.-A., Driouich, A. (2013). Arabinogalactan proteins in root-microbe interactions. Trends Plant Sci. 18, 440–449. doi: 10.1016/j.tplants.2013.03.006
Põlme, S., Bahram, M., Jacquemyn, H., Kennedy, P., Kohout, P., Moora, M., et al. (2018). Host preference and network properties in biotrophic plant-fungal associations. New Phytol. 217, 1230–1239. doi: 10.1111/nph.14895
Rui, Y., Dinneny, J. R. (2020). A wall with integrity: surveillance and maintenance of the plant cell wall under stress. New Phytol. 225, 1428–1439. doi: 10.1111/nph.16166
Stavolone, L., Lionetti, V. (2017). Extracellular matrix in plants and animals: hooks and locks for viruses. Front. Microbiol. 8. doi: 10.3389/fmicb.2017.01760
Swaminathan, S., Lionetti, V., Zabotina, O. A. (2022). Plant cell wall integrity perturbations and priming for defense. Plants 11, 3539. doi: 10.3390/plants11243539
Tauzin, A. S., Giardina, T. (2014). Sucrose and invertases, a part of the plant defense response to the biotic stresses. Front.Plant Sci. 5. doi: 10.3389/fpls.2014.00293
Keywords: cell wall remodeling, symbiosis, cell wall integrity, plant immunity, plant parasitism, plant cell wall
Citation: Vicré M and Lionetti V (2023) Editorial: Plant cell wall in pathogenesis, parasitism and symbiosis, Volume II. Front. Plant Sci. 14:1230438. doi: 10.3389/fpls.2023.1230438
Received: 28 May 2023; Accepted: 06 June 2023;
Published: 20 June 2023.
Edited and Reviewed by:
Prem Lal Kashyap, Indian Institute of Wheat and Barley Research (ICAR), IndiaCopyright © 2023 Vicré and Lionetti. This is an open-access article distributed under the terms of the Creative Commons Attribution License (CC BY). The use, distribution or reproduction in other forums is permitted, provided the original author(s) and the copyright owner(s) are credited and that the original publication in this journal is cited, in accordance with accepted academic practice. No use, distribution or reproduction is permitted which does not comply with these terms.
*Correspondence: Vincenzo Lionetti, dmluY2Vuem8ubGlvbmV0dGlAdW5pcm9tYTEuaXQ=