- 1College of Biology and Food Engineering, Chongqing Three Gorges University, Wanzhou, China
- 2Chongqing Three Gorges Vocational College, Wanzhou, China
- 3College of Plant Protection, Shenyang Agricultural University, Shenyang, China
As the primary energy source for a plant host and microbe to sustain life, sugar is generally exported by Sugars Will Eventually be Exported Transporters (SWEETs) to the host extracellular spaces or the apoplast. There, the host and microbes compete for hexose, sucrose, and other important nutrients. The host and microbial monosaccharide transporters (MSTs) and sucrose transporters (SUTs) play a key role in the “evolutionary arms race”. The result of this competition hinges on the proportion of sugar distribution between the host and microbes. In some plants (such as Arabidopsis, corn, and rice) and their interacting pathogens, the key transporters responsible for sugar competition have been identified. However, the regulatory mechanisms of sugar transporters, especially in the microbes require further investigation. Here, the key transporters that are responsible for the sugar competition in the host and pathogen have been identified and the regulatory mechanisms of the sugar transport have been briefly analyzed. These data are of great significance to the increase of the sugar distribution in plants for improvement in the yield.
Introduction
The contradiction between the growing population’s demand for food and the safety of food production is becoming increasingly severe. Furthermore, plant diseases continue to seriously threaten the safety of food production worldwide (Strange and Scott, 2009). The global yield loss due to plant diseases and insect pests in different crops including potato, soybean, wheat, maize, and rice can reach 17.2%-30.3% annually (Ristaino et al., 2021). To facilitate the reduction of food loss caused by plant diseases and maintain food security, future studies should focus on identifying the QTLs of partial and persistent resistance of plants and the relatively conservative pathogenic factors, including pathogen-associated molecular patterns (PAMPs) and effectors, in the pathogenic bacteria from the level of host-pathogen interaction, the disease-resistant gene as one of the effective tools of disease management will be exchanged and managed in a wide range (Boyd et al., 2013; Garrett et al., 2017). Therefore, to identify effective and stable resistance genes, the key factors involved in the competition between pathogens and hosts are of significant interest.
Photosynthetic sugar is the key nutrient for plants and microbes
The primary competitive nutrients between plants and pathogens, such as sugars, may become significant factors in disease control. Sugar, as the main source of energy, is vital for plants and pathogens and involved in regulating different physiological functions and metabolic pathways, including growth regulation, signal transduction, structural component synthesis (i.e., carbon skeleton), and osmotic pressure (Rolland et al., 2006; Saddhe et al., 2021). Although fatty acids, as organic carbon sources for fungi, play a significant role in fungal symbiosis, sugars also function as precursors or nutrients in both plants and pathogens(Luginbuehl et al., 2017; Bouwmeester, 2021). As a producer, plants synthesize carbohydrates that are transported from ‘source’ to ‘sink’ by sugar transporters in the form of sucrose via photosynthesis and assimilated in the mesophyll (Julius et al., 2017). During the invasion process, pathogenic bacteria must secure sugar from the plant host to supply their own growth. To capture more sugar, the pathogen must force the host plant to transport the sugar (e.g., glucose, fructose, or sucrose) from within the cell to the apoplast, a frontline where the host and the pathogen can interact with each other. This process requires the participation of the plasma membrane-localized sugar transporters (Julius et al., 2017) (Naseem et al., 2017).
The host sugar transporter is hijacked by the microbes to export sugar from the cell
Sugars Will Eventually be Exported Transporters (SWEETs) are one of the three known sugar transporter families, including monosaccharide transporters (MSTs) and sucrose transporters (SUTs)(Misra et al., 2019). They are involved in the intracellular and extracellular transport of sugar by pH-independent uniporters and undertake the central role in phloem loading, nectar secretion, seed filling, pollen nutrition, and plant senescence (Chen et al., 2012; Eom et al., 2015). The SWEET protein is composed of α-helix transmembrane domains (TMs) and functions as a sugar efflux carrier by forming oligomers (Xuan et al., 2013). In addition, the SWEET proteins play an important regulatory role in the plant-pathogen interaction (Breia et al., 2021). Within the pathosystem, SWEETs function as susceptible (S) genes and are induced during pathogen infection, resulting in increased pathogen virulence (Gupta, 2020; Mondal et al., 2021). The transcriptome data of different rice lines (resistant Teqing (T) and susceptible Lemont (L) were analyzed. The results demonstrated that eight SWEET genes (OsSWEET2a, 6b, 7d, 11, 13, 14, 15, and 16) were up-regulated following Rhizoctonia solani AG1-IA strain inoculation (Wang et al., 2021) (Figure 1); two SWEETs (OsSWEET11 and 14), of the susceptible Pujiang6 rice line were up-regulated (3.4 and 5.8-fold, respectively) after nine days following the inoculation with Ustilaginoidea virens P4 strain (Fan et al., 2020) (Figure 1A). Ten SWEET genes (OsSWEET2a, 2b, 3a, 3b, 6a, 6b, 7d, 11, 14, and 16) of the resistant Hui1586 and susceptible Nipponbare (NIP) lines responded to Magnaporthe oryzae Guy11 strain induction (Yang et al., 2021) (Figure 1B). In the susceptible CT 9737-6-1-3P-M rice line, six SWEET genes (OsSWEET1b, 2a, 2b, 11, 13, and 16) were up-regulated by Xanthomonas oryzae pv. oryzae P3 and P6 strains following inoculation. However, in the resistant NSIC RC154 line, seven SWEET genes (OsSWEET1b, 2a, 2b, 3b, 13, 14, and 16) were up-regulated following inoculation while two were not (OsSWEET4 and 7d) (Shu et al., 2021)(Figure 1C). Transcriptomic analysis, as well as the analysis of gene expression and sugar contents, of the inoculated samples has shown that SWEETs are up-regulated in response to competition with invading pathogens for sugar. Twenty-one SWEETs have been identified in rice, among which Xa13/OsSWEET11 (targeted by PthXO1) and Os11N3/OsSWEET14 (targeted by TALC) are induced by various transcription activator-like (TAL) effectors secreted via type III secretion system of Xoo, which cause bacterial leaf blight. Xa25/OsSWEET13 has been shown to be induced by Xoo PXO339 strain as a susceptible allele (Yang et al., 2006; Antony et al., 2010; Liu et al., 2011). SWEET-mediated susceptibility dependence on TAL effector also occurs in other plants, where GhSWEET10 in cotton is specifically activated by an Avrb6-mediated effector (Cox et al., 2017) and CsSWEET1 in citrus is activated by effector pthAw or pthA4 from the X. citri strain (Hu et al., 2014). Streubel et al. identified that OsSWEET12 and 15 are induced by artificial TALs to promote the distribution of sugar flow (primarily sucrose) in pathogens (Streubel et al., 2013). However, SWEET-targeting has been reported in other bacteria and fungi. OsDOF11 (DNA BINDING WITH ONE FINGER 11) regulates the expression of OsSWEET11, OsSWEET14, and OsSUT1 by directly binding to the gene promoter. Furthermore, the overexpression of DOF activates SWEET14 gene expression, therefore improving rice resistance to sheath blight caused by the invasion of R. solani AG1-IA (Wu et al., 2018; Kim et al., 2020). Moreover, Gao et al. determined that R. solani promotes sugar efflux and increases pathogenicity by activating SWEET11 (Gao et al., 2018). Recent research has demonstrated that the infection by the rice blast fungus may activate the BR signal via WRKY53 and induce SWEET2a expression via negative regulation of rice ShB resistance (Gao et al., 2021). Further research identified that the effector AOS2 secreted by R. solani interacts with WRKY53 and GT1 to form a transcription factor complex, activating the SWEET genes (including SWEET2a and 3a) and increasing the virulence of fungi through nutritional competition (Yang et al., 2022). During the symbiosis of Medicago truncatula and arbuscular mycorrhizal (AM) fungi, MtSWEET1b is also induced to promote a glucose efflux carrier (An et al., 2019). Phloem loading is a central link to the long-distance transport of sugars in plants, where SWEET-mediated sugar efflux carriers transport sugars from phloem parenchyma cells to the apoplast. Then, the sugars are transferred into sieve tubes and companion cells via SUC, a sucrose carrier (Ruan, 2014). Plant SWEET genes are systematically divided into four subclasses. Clades I, II, and IV primarily contain SWEET genes that are monosaccharide efflux transporters that transport glucose, galactose, and/or fructose. However, Clade III members preferentially transport sucrose (Chandran, 2015). The discovery that pathogens with different types and sugar requirements alter the expression of plant SWEET transporters demonstrates that pathogens use a common strategy to extract sugar from the host (Schüßler et al., 2006; Wahl et al., 2010). The strategies used by pathogens to target plant SWEETs differ according to their sugar preferences, and multiple SWEETs in different clades of the SWEET family have been found to be targeted. Therefore, SWEET genes play a primary role in sugar efflux and have a positive effect on microbial pathogenesis (Figure 2).
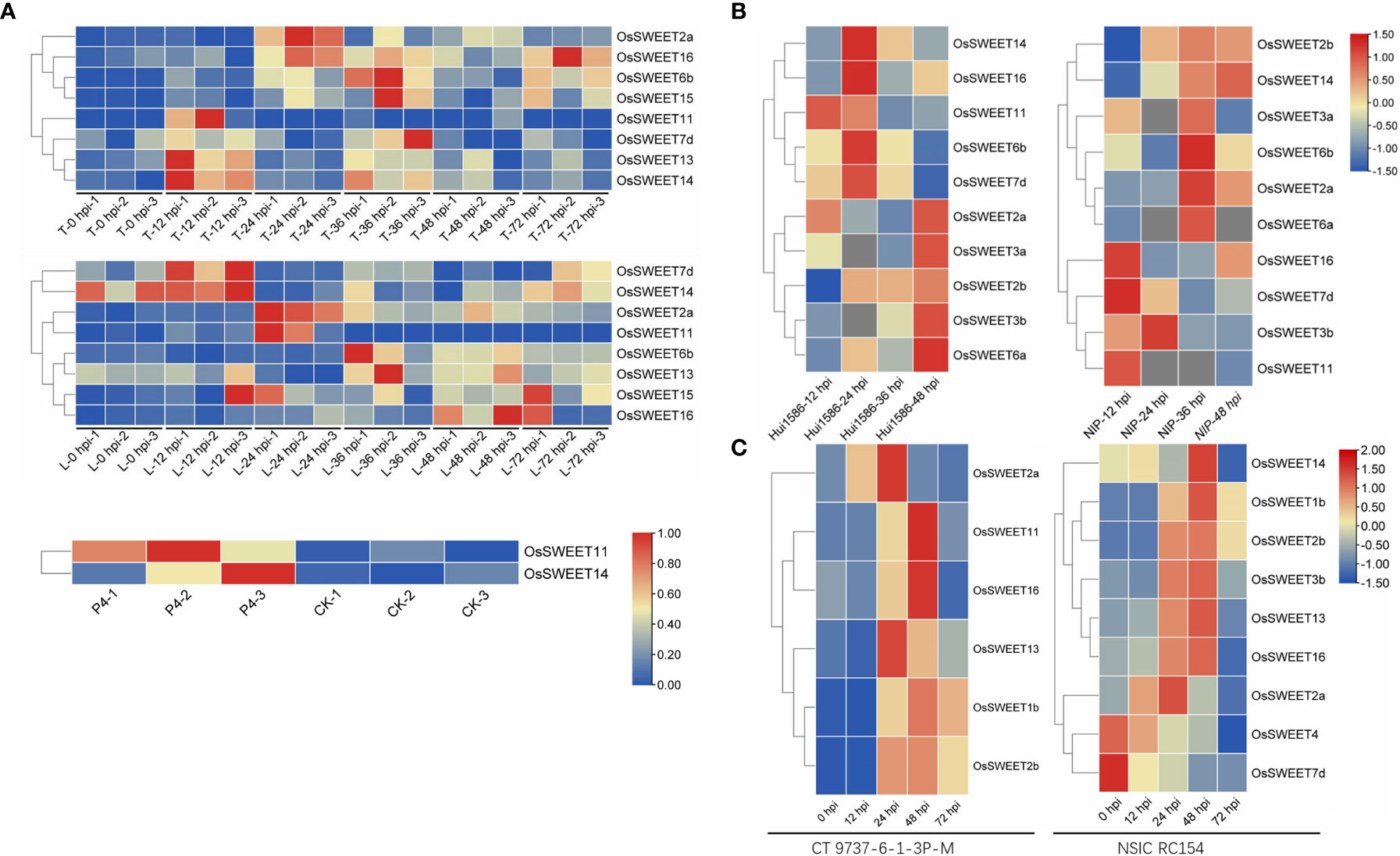
Figure 1 Heat map of the expression pattern of the differentially expressed SWEET genes in the rice transcriptome induced by four pathogens. (A) Gene expression at 0, 12, 24, 48, and 72 h post-inoculation with R. solani AG1-IA in the resistant Teqing (T) or susceptible Lemont (L) rice lines and the FPKM at 9 days post-inoculation with Ustilaginoidea virens P4 strain in susceptible Pujiang6 rice variety. Every three columns marketed by 1, 2, or 3 correspond to a time or a treatment result (Fan et al., 2020; Wang et al., 2021). (B) log2 fold gene expression at 12, 24, 36, and 48 h post-inoculation with Magnaporthe oryzae Guy11 strain in the resistant Hui1586 or susceptible Nipponbare (NIP) rice lines (Yang et al., 2021). (C) Gene expression at 0, 12, 24, 48, and 72 h post-inoculation with Xanthomonas oryzae pv. oryzae P3 and P6 strains in resistant NSIC RC154 or susceptible CT 9737-6-1-3P-M lines (Shu et al., 2021).
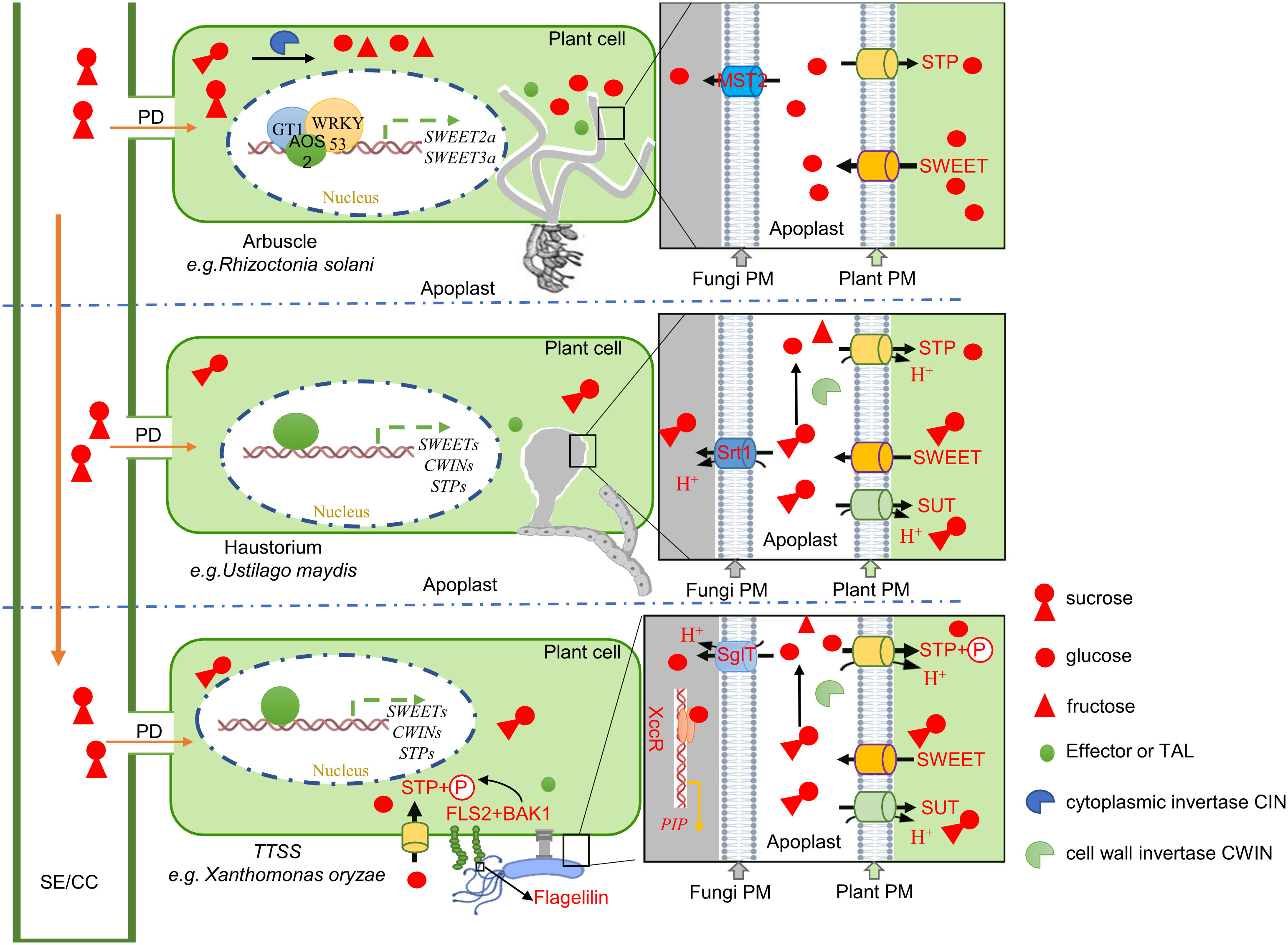
Figure 2 An illustration demonstrating the putative mechanism of sugar transporter regulated by a signal or regulator molecule from different microbes during host-pathogen interaction. To meet the demand for sugar, microbes directly or coupled with transcription factors (TF) such as WRKY53 or GT1, activate the SWEET genes in the plant that mediate glucose or sucrose efflux via effector protein (AOS2) or transcription activator-like (TAL) (e.g., PthXO1, TALC, and pthAw) effector secretion into the host (Yang et al., 2006; Antony et al., 2010; Liu et al., 2011; Hu et al., 2014; Yang et al., 2022). However, CWIN in plants is also activated to hydrolyze the sucrose in the apoplast increasing the content of extracellular hexose and causing the host and pathogen to compete for hexose via hexose transporter (STP/H+ in plants and MST/H+ or SglT/H+ in microbes) (Voegele et al., 2001; Helber et al., 2011; Proels and Hückelhoven, 2014; Zhang et al., 2023). Following microbial absorption, hexose (especially glucose) is perceived by the LuxR receptor as a signal molecule, leading to the activation of an important pathogenic protein (PIP) (Zhang et al., 2023). Similarly, glucose also mediates the activation of the host immune response when sugar leakage occurs in the host-microbe interaction interface (Schuler et al., 2015). In addition, the host FLS2 responds to bacterial flagellin and interacts with BAK1 to phosphorylate STP to accelerate hexose absorption by the host(Yamada et al., 2016). This restriction is relieved by the nutrition strategy of microbes absorbed by sucrose. Pathogenic bacteria have a greater advantage in competition with low-affinity sucrose transporters (SUTs) in plants via high-affinity sucrose transporters (SRT) (Wahl et al., 2010).
Host immunity and sugar recovery are activated following response to sugar leakage
With the pathogen hijacking SWEET in the host to expel sugar to the apoplast for its growth, the plant also made a counterattack. In plants and pathogenic bacteria, invertases (INV) are divided into the following categories according to subcellular localization and cleavage of sucrose into fructose and glucose: cell wall invertase (CWIN), cytoplasmic invertase (CIN), and vacuolar invertase (VIN). CWIN plays a key role in the production of crops, particularly in the development of seeds and fruits (Kocal et al., 2008; Ruan, 2014). GIF1 (GRAIN INCOMPLETE FILLING 1) encoding a CWIN is a putative domestication gene, which is required for carbon distribution at the early stage of grain filling in rice (Wang et al., 2008). Furthermore, the activity and expression of CWIN are induced during a pathogen invasion in a plant. Therefore, CWIN is key invertase regulating sugar, specifically sucrose metabolism during plant defense response and is responsible for the hydrolysis of sucrose into fructose and glucose in the apoplast. These hexoses are further recovered by sugar transport proteins in the host and function as signal molecules in the sugar signal pathway (Proels and Hückelhoven, 2014; Liao et al., 2020). Following the infection with Xanthomonas campestris pv. vesicatoria in tomato, the increased CWIN activity induced by pathogenic bacteria causes the increase of hexose formation, which may further trigger increased negative regulation of photosynthesis and photosynthetic gene expression and positive regulation of sugar-induced pathogenesis-related genes (Kocal et al., 2008). Reduced photosynthesis and improved basic defense response levels are accompanied by reduced sugar outflow and high resistance of the host.
To respond to the microbe-induced sugar leakage, plants can also “starve the pathogens to death” by retrieving sugars (mainly sucrose and glucose) from apoplasts via sugar transporters. In plants, along with SWEET genes, monosaccharide transporters (MST) (generally including the sugar transporters protein (STP)) also play an important role in sugar transport, specifically in the process of phloem loading and unloading, pathogen defense, and yield formation (Doidy et al., 2012; Li et al., 2018; Zhang et al., 2019) The MST family is subdivided according to sequence differences and substrate specificities into the STP, PLT (polyol/monosaccharide transporter), VGT (vacuolar glucose transporter), INT (inositol transporter), TMT (tonoplast monosaccharide transporter), pGlcT (plastidic glucose translocator), and ERD (early response to dehydration 6-like) subgroups (Büttner, 2007; Deng et al., 2019).
SUT is a sucrose-H+ symporter and is characterized into five groups: SUT1 to SUT5. SUT1 is present in a specific branch of dicotyledonous plants and members of SUT2 and SUT4 branches are found in both monocotyledonous and dicotyledonous plants (Figure 3). SUT is expressed in the source leaves and sink cells, and participates in the process of phloem loading and unloading, and responds to dehydration, photosynthesis, circadian rhythm, and the development of nodules (Misra et al., 2019). In the phloem loading process, sucrose is discharged into the apoplast via the SWEET family sugar transporters in the sink cells and then absorbed or loaded into the phloem by SUT or SUC sugar transporters. Sucrose transported via the companion cells-sieve elements (CC-SE) complexes will eventually be exported or unloaded into the phloem apoplasmically by SWEETs and then absorbed into the sink cells by the action of sucrose/H+ cotransporter. Alternatively, this sucrose can be hydrolyzed by the CWIN to produce hexose and then enter the surrounding source cells via the hexose transport protein (Braun, 2022). OsSUT1 plays a role in seed filling, germination, and early seedling growth, but is also responsible for sucrose recovery from plastids along the transport path during phloem loading in rice (Scofield et al., 2007). TaSUT1 also functions to recover leaked sucrose from phloem apoplast (Aoki et al., 2014). The source-sink distribution of sucrose plays a key role in regulating plant yield development (Chen and Ham, 2022). Therefore, SWEET-SUT regulates the long-distance transportation of sugars to regulate yield rather than immunity.
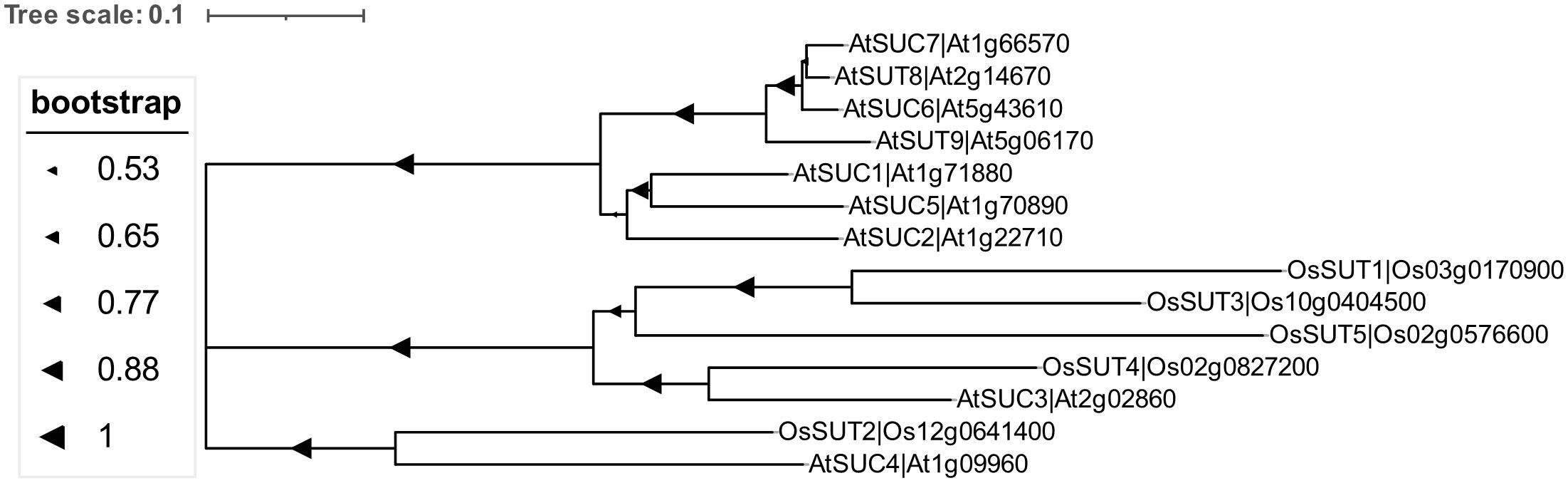
Figure 3 Phylogenetic tree of the sucrose transporters (SUTs) in monocotyledonous plants (Oryza sativa) and dicotyledonous plants (Arabidopsis thaliana). Multiple sequence alignment analysis of sucrose transporters was conducted by Cluster W (version 2) and the phylogenetic evolution tree was constructed using the Neighbor-Joining (NJ) method using MEGA7.0 software. The phylogenetic analysis was performed using 1,000 bootstrap reiterations with the Jones-Taylor-Thornton (JTT) model.
For monosaccharide-dependent pathogenic bacteria, plants can recover hexose from the extracellular spaces via highly efficient and plasma membrane-localized monosaccharide transport proteins to inhibit the loss of sugar. Similar to SUT, as a member of the major facilitator superfamily, MSTs contain 12 transmembrane domains linked by hydrophilic loops and have the ability of H+-sugar cotransporters. STP, as a member of the monosaccharide transporters family, absorbs broad-spectrum monosaccharide substrates with high affinity and retrieves hexose from the plant extracellular spaces, especially when challenged by microbe (Büttner, 2010; Deng et al., 2019; Sosso et al., 2019). In Arabidopsis, the widespread biotrophic pathogen Erysiphe cichoracerum significantly induces AtSTP4 gene expression following inoculation. The pathogenic fungus Botrytis cinerea causing grey mold disease induces AtSTP13 gene expression to enhance the level of glucose absorption of plants and improves plant resistance to Botrytis cinerea (Fotopoulos et al., 2003; Lemonnier et al., 2014). Furthermore, AtSTP13 is phosphorylation-dependent and functions as a positive regulator of disease resistance in Arabidopsis. In the presence of pathogenic bacteria, flagellin sensitive 2 (FLS2), the pattern recognition receptors (PRRs) expressed on the cell surface, and BRASSINOSTEROID INSENSITIVE 1–associated receptor kinase1 (BAK1) interacts with STP13 by phosphorylating it at the threonine 485 site, resulting in improved hexose absorption capacity by the plant. The pathogenicity of bacteria is therefore weakened by limiting the nutrient absorption from the host (Yamada et al., 2016). Therefore, SUT and STP located on the plant plasma membrane absorb sucrose into the sink tissue and retrieve monosaccharides from the apoplast to reduce sugar loss (Figure 2). These sugar transporter functions are instrumental to the development of plant yield and the improvement of resistance.
Microbes scramble for sugar to survive at the conflict border
The ability to utilize sugars in plant tissues promotes the intensity of the synthesis of photosynthesis products to a certain extent (Braun et al., 2014). Following the invasion of a pathogen, the increased sugar utilization in plant tissues also causes increased sugar flow to the pathogen, resulting in the loss of yield (Figure 2) (Pommerrenig et al., 2020). The glomeromycotan fungus (Geosiphon pyriformis) monosaccharide transporter GpMST1 is a proton cotransporter and contains a very low GC content and has the highest affinity for monosaccharides, including glucose, mannose, galactose, and fructose (Schüßler et al., 2006). Due to the hydrolysis of sucrose by plant CWIN at the host-microbe interaction interface numerous glucose and fructose molecules are present in the apoplast. In order to avoid the immune defense reaction caused by the activation of plant CWIN, the microbes also encode their own CWIN (Doidy et al., 2012). The invertase UfINV1 of Uromyces fabae rust fungus has been identified and has been found to disturb sugar partitioning during host-pathogen interaction (Voegele et al., 2007). Pathogens can directly absorb these hexoses to maintain growth and toxicity. In B. cinerea, a gray mold pathogen, a high-affinity proton symporter FRT1 transports fructose specifically and plays a key role in the process of vegetative growth and pathogenesis (Doehlemann et al., 2005). Another high-affinity monosaccharide transporter MST2 expressed on symbiotic hyphae of Rhizophagus irregularis mediates sugar absorption from the interface of Glomus-Medicago truncatula interaction (Helber et al., 2011). U. fabae obtains sugar from Vicia faba via haustoria, where concentrated gene expression encoding hexose transport proteins, including HXT1, specifically combines the substrate D-glucose and D-fructose (Voegele et al., 2001). HXT1 has a high substrate affinity for glucose, fructose, and mannose. The hexose absorption capacity and toxicity of the microbe are therefore significantly weakened with an HXT1 mutation. The heterologous expression of CgHXT3 and AtSTP1, which have high-affinity hexose transporter comparable to HXT1, fail to repair the pathogenicity defect, suggesting that HXT has dual functions of a hexose receptor and transporter during the plant invasion process (Schuler et al., 2015). In line with this, pathogen invasion as an exogenous stress activates CWIN (AtCWINV1) and SWEET (AtSWEET2 and 15) genes in plants, resulting in glucose accumulation in the infected plastids. The glucose absorbed by the sugar transporter SglT of Xanthomonas campestris pv. campestris (Xcc) combines with the orphan governor XccR, the homologous protein of cognate receptors LuxR, to activate the proline aminopeptidase-encoded gene PIP and enhance virulence (Zhang et al., 2023). Directly-absorbed and abundant hexose, represented by glucose, is the necessary nutrient and signal molecule for the pathogen. Hexose-dependent nutrition strategy is limited by what plants have evolved as a defense mechanism to reprogram immune-related genes in response to the changes in glucose content at the infection sites (Ehness et al., 1997).
However, the strategy of reducing energy to limit microbial virulence is overcome by pathogens with sucrose as the primary carbon source (Talbot, 2010). Sucrose, as the major product of photosynthesis and the main form of long-distance transport, is very abundant in plants. This kind of pathogen avoids utilizing the invertase to cleave sucrose to hexose in the extracellular spaces and avoids the recognition and activation of plant defense reactions, including active oxygen burst and cell death. The sucrose transporter (Srt1) is localized on the plasma membrane of Ustilago maydis, which causes corn smut. Srt1 has extremely high substrate affinity and specificity for sucrose, outperforming plant sucrose transporters (such as SUT) with low sucrose affinity and energy dependence to absorb sucrose from the apoplast of the host directly and avoids the plant immune response triggered by monosaccharide leakage (Talbot, 2010; Wahl et al., 2010). This strategy of pathogenic bacteria increases the sugars in the apoplast flow in the form of sucrose to support its own virulence and growth requirements.
Conclusions and future prospects
Sugar is a key nutrient for which both plants and pathogens compete (Saddhe et al., 2021). Pathogens, irrespective of whether they are necrotrophic, biotrophic, or hemibiotrophic, compete for sugar with the host at the extracellular boundary formed between plants and pathogens (apoplast) during the establishment of the parasitic relationship (Naseem et al., 2017). The absorption of sugar by fungi and bacteria is mediated by sugar transporters, which differ according to the type of sugar absorbed, the way the sugar is ultimately utilized, and interactions with plant immunity, all of which are dependent on the varying sugar requirements of different pathogens. The competitive absorption of sugar generally includes two kinds of sugars: sucrose and hexose. The outcome of the competition is determined by characteristics and substrate specificities of sucrose and monosaccharide transporters in both host and pathogen. Therefore, investigation of the functions of proteins responsible for sugar transport in pathogens with different sugar preferences assists in the identification of immune-related regulatory pathways associated with pathogens with the same infection strategy and the overall spectrum of disease-resistance strategies. (Figure 4).
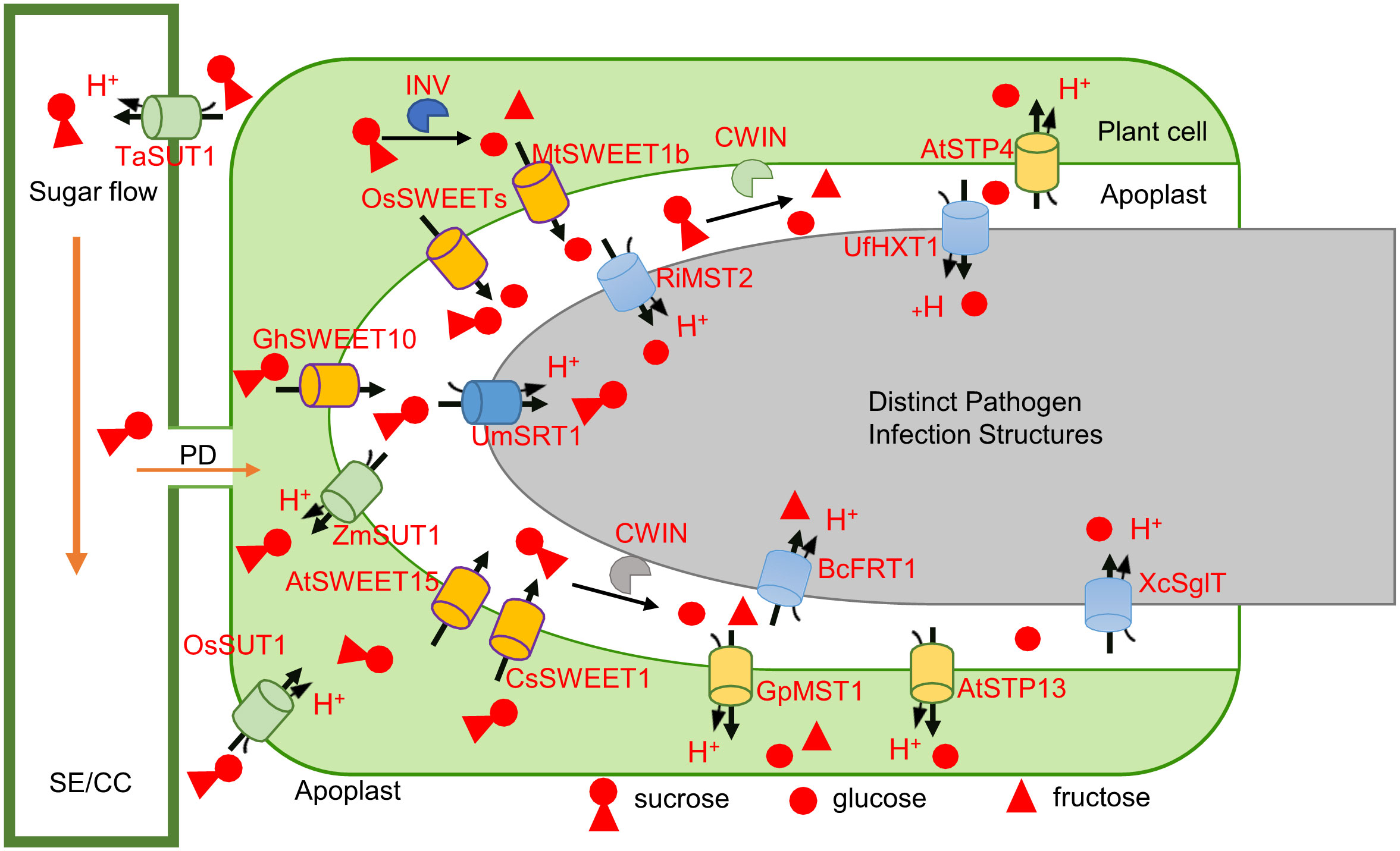
Figure 4 Sugar competition in plant-microbe interactions involves the participation of SWEETs, STPs, SUTs, and INVs of plants and pathogens. Once the pathogen successfully invades the host, the SWEET genes [OsSWEET2a (Gao et al., 2021), OsSWEET3a (Scofield et al., 2007), MtSWEET1b (An et al., 2019), OsSWEET11 (Gao et al., 2018), AtSWEET15 (Zhang et al., 2023), CsSWEET1 (Hu et al., 2014), and GhSWEET10 (Cox et al., 2017)] in the host are activated by the microbe target, and the INVs (CWINs) of the plant or microbe is induced, causing the sucrose (and glucose) in the host to flow out to the apoplast and partially hydrolyze into glucose and fructose (Proels and Hückelhoven, 2014; Liao et al., 2020). The extracellular sugar concentration therefore increases. Hexose is competitively absorbed by plant [AtSTP4 (Fotopoulos et al., 2003), AtSTP13 (Misra et al., 2019), and GpMST1 (Schüßler et al., 2006)] and pathogen [XcSglT (Zhang et al., 2023), BcFRT1 (Lemonnier et al., 2014), RiMST2 (Helber et al., 2011), and UfHXT1(Schuler et al., 2015)] MSTs, while sucrose is competing for the absorption by the plant [ZmSUT1 (Sosso et al., 2019), OsSUT1 (Scofield et al., 2007), and TaSUT1 (Aoki et al., 2014)] and microbe [UmSRT1 (Wahl et al., 2010)] sucrose transporters. Plants respond to the changes in the extracellular glucose concentration caused by microbial colonization to activate the immune response. The CWIN secreted by the microbe and the pathogenic sucrose absorption strategies removes the restriction imposed by the sugar leakage in plants.
In the “sugar attack and defense” between plants and pathogens, plant sugar metabolism, signal transduction, and sugar transport proteins have received significant attention while the research on the sugar transport and metabolism of pathogens requires further investigation (Liu et al., 2022). More proteins related to sugar transport and metabolism of pathogenic bacteria require identification to improve the known regulatory networks of plant-pathogenic bacteria interaction, providing more direction for disease prevention and control. At present, the research on host sugar transporters reveals that pathogenic bacteria obtain sugar in plants via regulation of the host sugar transporters (such as SWEET) (Ji et al., 2022) and that some pathogenic bacteria avoid the host innate immune defense response caused by monosaccharide loss via sucrose absorption (Wahl et al., 2010). Therefore, inhibiting SWEET gene expression induced by pathogenic bacteria or increasing the expression level of plant transporters that mediate sugar recovery can be used as an effective strategy to improve plant disease resistance (Breia et al., 2021). AtSWEET2, located in vacuoles, reduces the availability of sugar released by vacuoles in the root after Pythium invasion, thereby increasing resistance (Chen et al., 2015). Therefore, the transport protein SWEET with its bidirectional transport function is crucial in the competition with pathogens for nutrients. In addition, SWEET genes play an important role in the process of phloem loading and unloading, specifically in the regulation of sugar distribution between source and sink, which is crucial for crop yield development (Braun, 2012). Consequently, the increase of plant resistance mediated by the SWEET mutation is accompanied by the reduction of crop yield. For example, the mutation of seed-filling related genes OsSWEET4, 11, 15, and ZmSWEET4c is accompanied by the reduction of grain weight of rice and maize (Gao et al., 2018; Li et al., 2021). There is a trade-off between yield and immunity mediated by SWEET proteins, which may be more fully understood as more SWEET gene functions are discovered.
The processes by which hosts and pathogens recognize each other are as important as the processes involved in competition. Previous studies have demonstrated changes in the expression of plant CWIN genes in response to signals of pathogen invasion, accompanied by local hydrolysis of sugars and promoting immune responses (Kocal et al., 2008). Glucose is not only a nutrient but also a signaling molecule that is recognized by sugar signaling receptors, such as HXT1 (Ehness et al., 1997; Patrick et al., 2013; Schuler et al., 2015). Therefore, the extracellular accumulation of hexose mediated by CWIN is the key factor in the induction of sugar-signaling immunity. The sugar-associated immune pathway involving the combination of monosaccharide transporter proteins for sugar recovery and receptor molecules for the recognition of hexose accumulation thus expands the immune regulatory network of the plant.
However, based on the recent research and according to the different modes of inducing plant susceptibility genes by different pathogens, the disease resistance of plants such as those with quantitative trait resistance (i.e., Oryza sativa) (Costanzo et al., 2011), can be enhanced to some extent by simultaneously combining multiple genes and providing more effective gene resources to direct broad-spectrum disease resistance breeding.
Author contributions
YX, SY and GX designed this work, JC, YX, MS, RS and CZ wrote the manuscript. All authors contributed to the article and approved the submitted version.
Funding
The work was supported by the Supported by the Science and Technology Research Program of Chongqing Municipal Education Commission (Grant No. KJQN 202101205), the Project of Chongqing Science and Technology Commission (CSTB2022NSCQ-MSX1436), the Project of Wanzhou Science and Technology Bureau (WZSTC-20220123).
Conflict of interest
The authors declare that the research was conducted in the absence of any commercial or financial relationships that could be construed as a potential conflict of interest.
Publisher’s note
All claims expressed in this article are solely those of the authors and do not necessarily represent those of their affiliated organizations, or those of the publisher, the editors and the reviewers. Any product that may be evaluated in this article, or claim that may be made by its manufacturer, is not guaranteed or endorsed by the publisher.
References
An, J., Zeng, T., Ji, C., de Graaf, S., Zheng, Z., Xiao, T. T., et al. (2019). A Medicago truncatula SWEET transporter implicated in arbuscule maintenance during arbuscular mycorrhizal symbiosis. New Phytol. 224, 396–408. doi: 10.1111/nph.15975
Antony, G., Zhou, J., Huang, S., Li, T., Liu, B., White, b F., et al. (2010). Rice xa13 recessive resistance to bacterial blight is defeated by induction of the disease susceptibility gene os-11N3. J. Plant Cell 22 (11), 3864–3876. doi: 10.1105/tpc.110.078964
Aoki, N., Scofield, G. N., Wang, X.-D., Patrick, J. W., Offler, C. E., Furbank, R. T. (2014). Expression and localisation analysis of the wheat sucrose transporter TaSUT1 in vegetative tissues. Planta 219, 176–184. doi: 10.1007/s00425-004-1232-7
Bouwmeester, H. J. (2021). Plant lipids enticed fungi to mutualism. Science 372 (6544), 789–790. doi: 10.1126/science.abi8016
Boyd, L. A., Ridout, C., O'Sullivan, D. M., Leach, J. E., Leung, H. (2013). Plant-pathogen interactions: disease resistance in modern agriculture. Trends Genet. 29 (4), 233–240. doi: 10.1016/j.tig.2012.10.011
Braun, D. M. (2012). SWEET! The pathway is complete. Science 335 (6065), 173–174. doi: 10.1126/science.1216828
Braun, D. M. (2022). Phloem loading and unloading of sucrose: what a long, strange trip from source to sink. Annu. Rev. Plant Biol. 73 (1), 553–584. doi: 10.1146/annurev-arplant-070721-083240
Braun, D. M., Wang, L., Ruan, Y.-L. (2014). Understanding and manipulating sucrose phloem loading, unloading, metabolism, and signalling to enhance crop yield and food security. J. Exp. Bot. 65 (7), 1713–1735. doi: 10.1093/jxb/ert416
Breia, R., Conde, A., Badim, H., Fortes, A. M., Gerós, H., Granell, A. (2021). Plant SWEETs: from sugar transport to plant-pathogen interaction and more unexpected physiological roles. Plant Physiol. 186 (2):836–852. doi: 10.1093/plphys/kiab127
Büttner, M. (2007). The monosaccharide transporter(-like) gene family in Arabidopsis. FEBS Lett. 581, 2318–2324. doi: 10.1016/j.febslet.2007.03.016
Büttner, M. (2010). TheArabidopsissugar transporter (AtSTP) family: an update. Plant Biol. 12 (s1), 35–41. doi: 10.1111/j.1438-8677.2010.00383.x
Chandran, D. (2015). Co-option of developmentally regulated plant SWEET transporters for pathogen nutrition and abiotic stress tolerance. IUBMB Life. 67 (7), 461–471. doi: 10.1002/iub.1394
Chen, J., Ham, B.-K. (2022). Systemic signaling: A role in propelling crop yield. Plants 11 (11), 1400. doi: 10.3390/plants11111400
Chen, H. Y., Hu, J. H., Yu, Y. C., Ho, L. H., Chen, L. Q., Tholl, D., et al. (2015). The Arabidopsis vacuolar sugar transporter SWEET2 limits carbon sequestration from roots and restricts Pythium infection. Plant J. 83, 1046–1058. doi: 10.1111/tpj.12948
Chen, L.-Q., Qu, X.-Q., Hou, B.-H., Sosso, D., Osorio, S., Fernie, A. R., et al. (2012). Sucrose efflux mediated by SWEET proteins as a key step for phloem transport. Science 335 (6065), 207–211. doi: 10.1126/science.1213351
Costanzo, S., Jackson, A. K., Brooks, S. A. (2011). High-resolution mapping of Rsn1, a locus controlling sensitivity of rice to a necrosis-inducing phytotoxin from Rhizoctonia solani AG1-IA. Theor. Appl. Genet. 123 (1), 33–41. doi: 10.1007/s00122-011-1564-1
Cox, K. L., Meng, F., Wilkins, K. E., Li, F., Wang, P., Booher, N. J., et al. (2017). TAL effector driven induction of a SWEET gene confers susceptibility to bacterial blight of cotton. Nat. Commun. 8, 15588. doi: 10.1038/ncomms15588
Deng, X., An, B., Zhong, H., Yang, J., Kong, W., Li, Y. (2019). A novel insight into functional divergence of the MST gene family in rice based on comprehensive expression patterns. genes 10), 239. doi: 10.3390/genes10030239
Doehlemann, G., Molitor, F., Hahn, M. (2005). Molecular and functional characterization of a fructose specific transporter from the gray mold fungus Botrytis cinerea. Fungal Genet. Biol. 42 (7), 601–610. doi: 10.1016/j.fgb.2005.03.001
Doidy, J., Grace, E., Kühn, C., Simon-Plas, F., Casieri, L., Wipf, D. (2012). Sugar transporters in plants and in their interactions with fungi. Trends Plant Sci. 17 (7), 413–422. doi: 10.1016/j.tplants.2012.03.009
Ehness, R., Ecker, M., Godt, D. E., Roitsch, T. (1997). Glucose and stress independently regulate source and sink metabolism and defense mechanisms via signal transduction pathways involving protein phosphorylation. Plant Cell. 9 (10), 1825–1841. doi: 10.1105/tpc.9.10.1825
Eom, J. S., Chen, L. Q., Sosso, D., Julius, B. T., Lin, I. W., Qu, X. Q., et al. (2015). SWEETs, transporters for intracellular and intercellular sugar translocation. Curr. Opin. Plant Biol. 25, 53–62. doi: 10.1016/j.pbi.2015.04.005
Fan, J., Liu, J., Gong, Z. Y., Xu, P. Z., Hu, X. H., Wu, J. L., et al. (2020). The false smut pathogen Ustilaginoidea virens requires rice stamens for false smut ball formation. Environ. Microbiol. 22 (2), 646–659. doi: 10.1111/1462-2920.14881
Fotopoulos, V., Gilbert, M. J., Pittman, J. K., Marvier, A. C., Buchanan, A. J., Sauer, N., et al. (2003). The monosaccharide transporter gene, AtSTP4, and the cell-wall invertase, Atbetafruct1, are induced in Arabidopsis during infection with the fungal biotroph Erysiphe cichoracearum. Plant Physiol. 132 (2), 821–829. doi: 10.1104/pp.103.021428
Gao, Y., Xue, C. Y., Liu, J. M., He, Y., Mei, Q., Wei, S., et al. (2021). Sheath blight resistance in rice is negatively regulated by WRKY53 via SWEET2a activation. Biochem. Biophys. Res. Commun. 585, 117–123. doi: 10.1016/j.bbrc.2021.11.042
Gao, Y., Zhang, C., Han, X., Wang, Z. Y., Ma, L., Yuan, D. P., et al. (2018). Inhibition of OsSWEET11 function in mesophyll cells improves resistance of rice to sheath blight disease. Mol. Plant Pathol. 19 (9), 2149–2161. doi: 10.1111/mpp.12689
Garrett, K. A., Andersen, K. F., Asche, F., Bowden, R. L., Forbes, G. A., Kulakow, P. A., et al. (2017). Resistance genes in global crop breeding networks. Phytopathology 107, 1268–1278. doi: 10.1094/PHYTO-03-17-0082-FI
Gupta, P. K. (2020). SWEET genes for disease resistance in plants. Trends Genet. 36 (12), 901–904. doi: 10.1016/j.tig.2020.08.007
Helber, N., Wippel, K., Sauer, N., Schaarschmidt, S., Hause, B., Requena, N. (2011). A versatile monosaccharide transporter that operates in the arbuscular mycorrhizal fungus glomus sp is crucial for the symbiotic relationship with plants. Plant Cell 23 (10), 3812–3823. doi: 10.1105/tpc.111.089813
Hu, Y., Zhang, J., Jia, H., Sosso, D., Li, T., Frommer, W. B., et al. (2014). Lateral organ boundaries 1 is a disease susceptibility gene for citrus bacterial canker disease. Proc. Natl. Acad. Sci. U S A. 111 (4), E521–E529. doi: 10.1073/pnas.1313271111
Ji, J., Yang, L., Fang, Z., Zhang, Y., Zhuang, M., Lv, H., et al. (2022). Plant SWEET family of sugar transporters: structure, evolution and biological functions. biomolecules 12 (2), 205. doi: 10.3390/biom12020205
Julius, B. T., Leach, K. A., Tran, T. M., Mertz, R. A., Braun, D. M. (2017). Sugar transporters in plants: new insights and discoveries. Plant Cell Physiol. 58 (9), 1442–1460. doi: 10.1093/pcp/pcx090
Kim, P., Cai Yun, X., Hyon Dok, S., Yue, G., Lu, Li, F., Xuan, Y., et al. (2020). Tissue-specific activation of DOF11 promotes rice resistance to sheath blight disease and increases grain weight via activation of SWEET14. Plant Biotechnol. J. 19 (3), 409–411. doi: 10.1111/pbi.13489
Kocal, N., Sonnewald, U., Sonnewald, S. (2008). Cell Wall-Bound Invertase Limits Sucrose Export and Is Involved in Symptom Development and Inhibition of Photosynthesis during Compatible Interaction between Tomato and Xanthomonas campestris pv vesicatoria. Plant Physiol. 148 (3), 1523–1536. doi: 10.1104/pp.108.127977
Lemonnier, P., Gaillard, C., Veillet, F., Verbeke, J., Lemoine, R., Coutos-Thévenot, P., et al. (2014). Expression of Arabidopsis sugar transport protein STP13 differentially affects glucose transport activity and basal resistance to Botrytis cinerea. Plant Mol. Biol. 85, 473–484. doi: 10.1007/s11103-014-0198-5
Li, H., Li, X., Xuan, Y., Jiang, J., Wei, Y. (2018). Genome Wide Identification and Expression Profiling of SWEET Genes Family Reveals Its Role During Plasmodiophora brassicae-Induced Formation of Clubroot in Brassica rapa. Front. Plant Sci. 9. doi: 10.3389/fpls.2018.00207
Li, P., Wang, L. h., Liu, H. b., Yuan, M. (2021). Impaired SWEET-mediated sugar transportation impacts starch metabolism in developing rice seeds. Crop J. 10 (1), 98–108. doi: 10.1016/j.cj.2021.04.012
Liao, S. j., Wang, L., Li, J., Ruan, Y. L. (2020). Cell wall invertase is essential for ovule development through sugar signaling rather than provision of carbon nutrients. Plant Physiol. 183 (3), 1126–1144. doi: 10.1104/pp.20.00400
Liu, Y.-H., Song, Y.-H., Ruan, Y.-L. (2022). Sugar conundrum in plant-pathogen interactions: roles of invertase and sugar transporters depend on pathosystems. J. Exp. Bot. 73 (7), 1910–1925. doi: 10.1093/jxb/erab562
Liu, Q. s., Yuan, M., Zhou, Y., hua Li, X., hua Xiao, J., Wang, S. p. (2011). A paralog of the MtN3/saliva family recessively confers race-specific resistance to Xanthomonas oryzae in rice. Plant Cell Environ. 34 (1), 1958–1969. doi: 10.1111/j.1365-3040.2011.02391.x
Luginbuehl, L. H., Menard, G. N., Kurup, S., Erp, H. V., Radhakrishnan, G. V., Breakspear, A., et al. (2017). Fatty acids in arbuscular mycorrhizal fungi are synthesized by the host plant. SCIENCE 356 (6343), 1175–1178. doi: 10.1126/science.aan0081
Misra, V. A., Wafula, E. K., Wang, Y., dePamphilis, C. W., Timko, M. P. (2019). Genome-wide identification of MST, SUT and SWEET family sugar transporters in root parasitic angiosperms and analysis of their expression during host parasitism. BMC Plant Biol. 19, 196. doi: 10.1186/s12870-019-1786-y
Mondal, K. K., Rashmi, E. R., Ghoshal, T. (2021). Rice SWEET proteins: the key targets by the bacterial blight pathogen, Xanthomonas oryzae pv. oryzae to ensure nutrition in the rice apoplast during infection. Indian Phytopathol. 74, 323–331. doi: 10.1007/s42360-021-00369-5
Naseem, M., Kunz, M., Dandekar, T. (2017). Plant–pathogen maneuvering over apoplastic sugars. Trends Plant Sci. 22 (9), 740–743. doi: 10.1016/j.tplants.2017.07.006
Patrick, J. W., Botha, F. C., Birch, R. G. (2013). Metabolic engineering of sugars and simple sugar derivatives in plants. Plant Biotechnol. J. 11, 142–156. doi: 10.1111/pbi.12002
Pommerrenig, B., Müdsam, C., Kischka, D., Neuhaus, H.E. (2020). Treat and trick: common regulation and manipulation of sugar transporters during sink establishment by the plant and the pathogen. J. Exp. Bot. 71 (14), 3930–3940. doi: 10.1093/jxb/eraa168
Proels, R. K., Hückelhoven, R. (2014). Cell-wall invertases, key enzymes in the modulation of plant metabolism during defence responses. Mol. Plant Pathol. 15 (8), 858–864. doi: 10.1111/mpp.12139
Ristaino, J. B., Anderson, P. K., Bebber, D. P., Brauman, K. A., Cunniffe, N. J., Fedoroff, N. V., et al. (2021). The persistent threat of emerging plant disease pandemics to global food security. Proc. Natl. Acad. Sci. U S A. 118 (23), e2022239118. doi: 10.1073/pnas.2022239118
Rolland, F., Baena-Gonzalez, E., Sheen, J. (2006). Sugar sensing and signaling in plants: conserved and novel mechanisms. Annu. Rev. Plant Biol. 57, 675–609. doi: 10.1146/annurev.arplant.57.032905.105441
Ruan, Y. L. (2014). Sucrose metabolism: gateway to diverse carbon use and sugar signaling. Annu. Rev. Plant Biol. 65, 33–64. doi: 10.1146/annurev-arplant-050213-040251
Saddhe, A. A., Manuka, R., Suprasanna, P. (2021). Plant sugars: Homeostasis and transport under abiotic stress in plants. Physiol. Plant 171 (4), 439–355. doi: 10.1111/ppl.13283
Schüßler, A., Martin, H., Cohen, D., Fitz, M., Wipf, D. (2006). Characterization of a carbohydrate transporter from symbiotic glomeromycotan fungi. Nature 444, 933–936. doi: 10.1038/nature05364
Schuler, D., Wahl, R., Wippel, K., Vranes, M., Münsterkötter, M., Sauer, N., et al. (2015). Hxt1, a monosaccharide transporter and sensor required for virulence of the maize pathogen Ustilago maydis. New Phytol. 206 (3), 1086–1100. doi: 10.1111/nph.13314
Scofield, G. N., Hirose, T., Aoki, N., Furbank, R. T. (2007). Involvement of the sucrose transporter, OsSUT1, in the long-distance pathway for assimilate transport in rice. J. Exp. Bot. 58 (12), 3155–3169. doi: 10.1093/jxb/erm153
Shu, X., Wang, A., Jiang, B., Jiang, Y., Xiang, X., Yi, X., et al. (2021). Genome-wide association study and transcriptome analysis discover new genes for bacterial leaf blight resistance in rice (Oryza sativa L.). BMC Plant Biol. 21 (1), 255. doi: 10.1186/s12870-021-03041-2
Sosso, D., van der Linde, K., Bezrutczyk, M., Schuler, D., Schneider, K., Kämper, J., et al. (2019). Sugar Partitioning between Ustilago maydis and Its Host Zea mays L during Infection. Plant Physiol. 179 (4), 1373–1385. doi: 10.1104/pp.18.01435
Strange, R. N., Scott, P. R. (2009). Plant disease: A threat to global food security. Annu. Rev. Phytopathol. 43, 83–116. doi: 10.1146/annurev.phyto.43.113004.133839
Streubel, J., Pesce, C., Hutin, M., Koebnik, R., Boch, J., Szurek, B. (2013). Five phylogenetically close rice SWEETgenes confer TAL effector-mediated susceptibility to Xanthomonas oryzae pv.oryzae. New Phytol. 200, 808–819. doi: 10.1111/nph.12411
Talbot, N. J. (2010). Living the sweet life: how does a plant pathogenic fungus acquire sugar from plants? PLoS Biol. 8 (2), e1000308. doi: 10.1371/journal.pbio.1000308
Voegele, R. T., Struck, C., Hahn, M., Mendgen, K. (2001). The role of haustoria in sugar supply during infection of broad bean by the rust fungus Uromyces fabae. Proc. Natl. Acad. Sci. U S A. 98 (14), 8133–8138. doi: 10.1073/pnas.131186798
Voegele, R. T., Wirsel, S., Möll, U., Lechner, M., Mendgen, K. (2007). Cloning and characterization of a novel invertase from the obligate biotroph uromyces fabae and analysis of expression patterns of host and pathogen invertases in the course of infection. Mol. Plant-Microbe Interact. 19 (6), 625–634. doi: 10.1094/MPMI-19-0625
Wahl, R., Wippel, K., Goos, S., Kämper, J., Sauer, N. (2010). A novel high-affinity sucrose transporter is required for virulence of the plant pathogen ustilago maydis. PLoS Biol. 8 (2), e1000303. doi: 10.1371/journal.pbio.1000303
Wang, A., Yamamoto, N., Li, S., Liang, Y., Zou, T., Shu, X., et al. (2021). Identification of rice (Oryza sativa L.) genes involved in sheath blight resistance via a genome-wide association study.Identification of rice (Oryza sativa L.) genes involved in sheath blight resistance via a genome-wide association study. Plant Biotechnol. J. 19 (8), 1553–1566. doi: 10.1111/pbi.13569
Wang, E., Wang, J., Zhu, X., Hao, W., Wang, L., Li, Q., et al. (2008). Control of rice grain-filling and yield by a gene with a potential signature of domestication. Nat. Genet. 40, 1370–1374. doi: 10.1038/ng.220
Wu, Y., Lee, S.-K., Yoo, Y., Wei, J., Kwon, S.-Y., Lee, S.-W., et al. (2018). Rice transcription factor OsDOF11 modulates sugar transport by promoting expression of sucrose transporter and SWEET genes. Mol. Plant 11 (6), 833–845. doi: 10.1016/j.molp.2018.04.002
Xuan, Y. H., Hu, Y. B., Chen, L.-Q., Sosso, D., Ducat, D. C., Hou, B.-H., et al. (2013). Functional role of oligomerization for bacterial and plant SWEET sugar transporter family. Proc. Natl. Acad. Sci. U S A. 111 (39), E3685–E3694. doi: 10.1073/pnas.1311244110
Yamada, K., Saijo, Y., Nakagami, H., Takano, Y. (2016). Regulation of sugar transporter activity for antibacterial defense in Arabidopsis. Science 354 (6318), 1427–1430. doi: 10.1126/science.aah5692
Yang, S., Fu, Y. W., Zhang, Y., Yuan, D. P., Li, S., Kumar, V., et al. (2022). Rhizoctonia solani transcriptional activator interacts with rice WRKY53 and grassy tiller 1 to activate SWEET transporters for nutrition. J. Adv. Res. S2090-1232 (22), 00216–00218. doi: 10.1016/j.jare.2022.10.001
Yang, D. w., Li, S. P., Xiao, Y. p., Lu, L., Zheng, Z. c., Tang, D. z., et al. (2021). Transcriptome analysis of rice response to blast fungus identified core genes involved in immunity. Plant Cell Environ. 44 (9), 3103–3121. doi: 10.1111/pce.14098
Yang, B., Sugio, A., White, F. F. (2006). Os8N3 is a host disease-susceptibility gene for bacterial blight of rice. Proc. Natl. Acad. Sci. 103 (27), 10503–10508. doi: 10.1073/pnas.0604088103
Zhang, S., Kan, J., Liu, X., Wu, Y., Zhang, M., Ou, J., et al. (2023). Phytopathogenic bacteria utilize host glucose as a signal to stimulate virulence through LuxR homologues. Mol. Plant Pathol. 00, 1–15. doi: 10.1111/mpp.13302
Zhang, W., Wang, S., Yu, F., Tang, J., Yu, L., Wang, H., et al. (2019). Genome-Wide Identification and Expression Profiling of Sugar Transporter Protein (STP) Family Genes in Cabbage (Brassica oleracea var. capitata L.) Reveals their Involvement in Clubroot Disease Responses. Genes (Basel) 10 (1), 71. doi: 10.3390/genes10010071
Keywords: host-pathogen interaction, microbe, sugar transporters, sugar competition, plant immunity
Citation: Chen J, Sun M, Xiao G, Shi R, Zhao C, Zhang Q, Yang S and Xuan Y (2023) Starving the enemy: how plant and microbe compete for sugar on the border. Front. Plant Sci. 14:1230254. doi: 10.3389/fpls.2023.1230254
Received: 28 May 2023; Accepted: 20 July 2023;
Published: 02 August 2023.
Edited by:
Huan Peng, Institute of Plant Protection (CAAS), ChinaReviewed by:
Cheng Liu, University of Florida, United StatesHuiming Guo, Chinese Academy of Agricultural Sciences (CAAS), China
Yongchao Bai, Research Institute of Forestry, Chinese Academy of Forestry, China
Copyright © 2023 Chen, Sun, Xiao, Shi, Zhao, Zhang, Yang and Xuan. This is an open-access article distributed under the terms of the Creative Commons Attribution License (CC BY). The use, distribution or reproduction in other forums is permitted, provided the original author(s) and the copyright owner(s) are credited and that the original publication in this journal is cited, in accordance with accepted academic practice. No use, distribution or reproduction is permitted which does not comply with these terms.
*Correspondence: Shuo Yang, MjAyMTIwMDEzOUBzdHUuc3lhdS5lZHUuY24=; Yuanhu Xuan, eHVhbnl1YW5odTExNUBzeWF1LmVkdS5jbg==; Guosheng Xiao, eGdzMDNAMTYzLmNvbQ==