- 1Institute of Plant Nutrition, Resources and Environment, Beijing Academy of Agricultural and Forestry Sciences, Beijing, China
- 2College of Mechanical and Electronical Engineering, Northwest A&F University, Yangling, Shaanxi, China
- 3College of Resources and Environmental Sciences, Hebei Agriculture University, Baoding, China
Long-term application of nitrogen (N) fertilizer adversely degrades soil and decreases crop yield. Biochar amendment with N fertilizer not only can increase yield but also can improve the soil. A 3-year field experiment was conducted to determine the effect of biochar doses with N fertilizer on maize yield and soil N and water dynamics under border irrigation (BI) and drip irrigation (DI) methods. Treatments were 260 kg N ha−1 without biochar addition and combined with low, medium, and high doses of biochar, namely, 15.5 t ha−1, 30.7 t ha−1, and 45.3 t ha−1 (NB0, NB1, NB2, and NB3), respectively. The biochar doses and irrigation methods significantly (p < 0.05) increased maize growth and yield characteristics, irrigation water use efficiency (IWUE), and fertilizer N use efficiency (FNUE) and enhanced the soil properties. In the BI and DI method, the NB1, NB2, and NB3 treatments increased yield by 4.96%–6.10%, 8.36%–9.85%, and 9.65%–11.41%, respectively, compared to NB0. In terms of IWUE and FNUE, the non-biochar treatment had lower IWUE and FNUE compared to biochar combined with N fertilizer treatments under both BI and DI methods. In the BI method, the IWUE in NB2 and NB3 ranged from 3.36 to 3.43 kg kg−1, and in DI, it was maximum, ranging from 5.70 to 5.94 kg kg−1. Similarly, these medium and high doses of biochar increased the FNUE of maize. The FNUEs in NB2 and NB3 under BI ranged from 38.72 to 38.95 kg kg−1 and from 38.89 to 39.58 kg kg−1, while FNUEs of these same treatments under DI ranged from 48.26 to 49.58 kg kg−1 and from 48.92 to 50.28 kg kg−1. The effect of biochar was more obvious in DI as compared to the BI method because soil water content (SWC) and soil N concentrations (SNCs) were higher at rhizosphere soil layers under DI. Biochar improved SWC and SNC at 0–20 cm and 20–40 cm soil layers and decreased below 60-cm soil layers. In contrast, despite biochar-controlled SWC and SNCs, still, values of these parameters were higher in deeper soil layers. In the BI method, the SNCs were higher at 60–80 cm and 80–100 cm compared to the top and middle soil layers. Depth-wise results of SNC demonstrated that the biochar’s ability to store SNC was further enhanced in the DI method. Moreover, biochar increased soil organic matter (OM) and soil aggregate stability and maintained pH. The NB0 treatment increased soil OM by 11.11%–14.60%, NB2 by 14.29%–19.42%, and NB3 by 21.98%–23.78% in both irrigation methods. This increased OM resulted in improved average soil aggregates stability by 2.45%–11.71% and 4.52%–14.66% in the BI and DI method, respectively. The results of our study revealed that combined application of N fertilizer with a medium dose of biochar under the DI method would be the best management practice, which will significantly increase crop yield, improve SWC, enrich SNC and OM, improve soil structure, and maintain pH.
1 Introduction
Maize is an important cereal crop that provides food to the world’s rising population (Haider et al., 2023). Maize contributes 35% of grain production in China (NBS, 2009) and consumes approximately 11.57b bushels (Taylor and Koo, 2013). Several factors can impact maize production, including soil fertility, water availability, and nutrient management practices (Kugedera et al., 2022). To increase grain yield, farmers apply excessive N fertilizer (Huang et al., 2021). However, the rate of N recovery from soil–plant systems is very low, hardly exceeding 50% of the applied fertilizer (Yang et al., 2020) due management practices (Geng et al., 2019). Soil condition has the greatest impact on N loss in agricultural systems (Bowles et al., 2018).
Nitrogen is a key component of chlorophyll, proteins, and nucleic acids, which are involved in various physiological processes, such as photosynthesis, respiration, and enzyme activity (Hassan et al., 2022). An adequate application of N fertilizer boosts vegetative growth, improves photosynthetic efficiency, and increases the grain yield of maize (Yue et al., 2021), while long-term N fertilizer use results in environmental pollution, particularly groundwater (Ju et al., 2006), surface water deterioration (Zhang et al., 2010), soil acidification (Schroder et al., 2011), and air pollution (Liu et al., 2011). In recent years, N fertilizer application increased (Du et al., 2018), with China’s agriculture system being the largest N fertilizer consumer (Tahir et al., 2021). The chemical fertilizer application in China has increased from 8.8 million tons in 1978 to 56.5 million tons in 2018, with chemical fertilizers used in China accounting for approximately 49% of total chemical fertilizer utilization worldwide (Chen, 2020). Usually, excessive N fertilizer is used to maximize crop yield in China, which surpasses crop N demand (Cui et al., 2010). Consequently, great amounts of N fertilizer losses via leaching and emissions (Khan et al., 2018). The nitrate form is highly water-soluble; therefore, it is easily leach down from the topsoil layer to bottom (Letey and Vaughan, 2013). Nitrate leaching accounts for approximately 18% to 20% (Ju and Zhang, 2017). Nitrate leaching adversely affects fertilizer N use efficiency (FNUE) of crop. FNUE commonly ranges between 30% and 35%. It is crucially important to improve FNUE by optimizing management practices, such as combining biochar with N fertilizer application.
Biochar, a multifunctional porous substance with a tiny particle size, a large surface area, a low bulk density, a high adsorption capacity, and an abundant carbon content, has received a great deal of attention because it provides numerous advantageous functions for crop production and soil science (Li et al., 2023). Biochar enhances soil fertility and nutrient availability, increases the efficiency of crop nutrient uptake (Gu et al., 2021), improves water retention, and reduces environmental risk (Yang et al., 2022). There are approximately 500 billion tons of biochar reserves in the world. It has been demonstrated to minimize ammonia emissions from animal dung composts while also improving compost quality (Li et al., 2020). Previous studies have shown that biochar decreased N leaching and emissions (Borchard et al., 2019) and improved N effectiveness for plant growth and development.
The combined application of N fertilizer and biochar has received a lot of interest because of its particular advantages in boosting soil N content (SNC) and improving FNUE (Xia et al., 2020). Mixed application of biochar with N fertilizer provided many benefits: (i) it helps in N nutrient release (Jeffery et al., 2017); (ii) its high cation exchange capacity, abundant pores, vast surface areas, and negatively charged surface improve electrostatic adsorption and retention of NH4 + (Mehmood et al., 2020); (iii) it has higher soil water-holding capacity (Farahani et al., 2020); (iv) it improves soil moisture at the topsoil layer, which helps in preventing N volatilization (Borchard et al., 2019); and (v) it improves soil microbe growth and N mineralization (Phares et al., 2022). The benefits of biochar for N adsorption and retention limit N losses due to volatilization and leaching, resulting in a progressive release of N for plant uptake and usage (Liu et al., 2021) and high FNUE and crop yield (Phares et al., 2020). Also, biochar binds to micronutrients. Combined trace elements and minerals are more easily absorbed by plants. Biochar loosens compacted soil, thereby resulting in soil aeration and plant root growth. A meta-analysis study reported that biochar application with N fertilizer increases FNUE and crop yield by 10%–12.0% (Liu et al., 2022). However, the increase in FNUE and crop yield is also influenced by biochar doses, N fertilizer rates, and irrigation methods.
Border irrigation (BI) and drip irrigation (DI) are two commonly used irrigation methods in maize production systems. BI comprises flooding the field with water through border ridges, allowing the water to seep into the soil (Zhao et al., 2020). This method of irrigation is not controllable and does not provide uniform water distribution, resulting in maximum water loss and eroding N from the topsoil layer, but it is an easy and low-cost irrigation method compared to the DI method. On the other hand, the DI method is a precise and efficient method that delivers water directly and slowly to the root zone of plants through a network of drip lines (Fang and Su, 2019). The DI method is controllable, ensures optimal SWC, and decreases N loss from topsoil layers and nutrient uptake while ensuring focused water delivery, minimizing water loss from evaporation and runoff (Hallett et al., 2017).
The combined application of N fertilizer rates with different biochar dosages under BI and DI methods could have interactive effects on maize growth, nutrient uptake, and yield. The interaction between N and biochar may influence nutrient availability and water retention at different depths of the soil, thereby affecting plant growth and productivity. Yang et al. (2022) studied the effect of different doses of biochar on maize growth, yield and soil nutrient variation under the DI method and reported that increasing biochar amendment significantly improved the soil NPK availability, organic matter (OM) content, and maize growth and yield attributes. Previous studies have greatly focused on biochar and N fertilizer coupling effect on maize yield and soil indicators. Still, much work is required to understand the interaction between biochar doses and N fertilizer in the soil–plant system. We hypothesized that the combination of increased biochar doses with N fertilizer will improve soil and increase maize yield compared to a small dose of biochar. Therefore, the aim of this study was to investigate and compare the combined effects of biochar doses and N fertilizer rate on maize growth and yield, and SNC and SWC dynamics under BI and DI methods.
2 Materials and methods
2.1 Experiment site
The experiment site is located in Qiqihar (47°21′15.65″ N latitude and 123°55′5.47″ E longitude), in the west-central part of Heilongjiang Province, northeastern China. The region has a temperate continental monsoon climate at a high altitude, spring is dry and windy, and summer is hot and rainy. Autumn is shorter, and winter is chilly and long, where the 24-h average summer temperature is 21.5°C, and the average annual rainfall is 410–540 mm; approximately 70% of rainfall occurs in the summer season. In the case of weather conditions during the present study, the rainfall was low in 2017 compared to 2018 and 2019. Rainfall mainly occurred in June and July as well as in August. The temperature and rainfall during the maize growing season are shown in Figure 1, and the basic soil properties are presented in Table 1.
2.2 Experimental design
The field experiments were conducted from 2017 to 2019. Treatments were 260 kg N ha−1 alone and combined with low, medium, and high doses of biochar (B), namely, 15.5 t ha−1, 30.7 t ha−1, and 45.3 t ha−1 (NB0, NB1, NB2, and NB3), respectively, under BI and DI methods. There were three replications. The maize variety was Nendan 19, and sowing dates were 7–9 May of each year. To achieve the recommended plant population of 67,000 ha−1, 28–30 kg·ha−1 seed was used. Seeds were sown in rows approximately 75 cm apart, and plant-to-plant spacing was 30 cm. The biochar used in this study was provided by Liaoning Biochar Engineering Technology Center and made from corn straw pyrolysis. It contained NPK 9.85 N, 1.63 P, 19 K, 55 Si, 2.9 Mg, 3.7 Ca, 163 O, and 595 OC g·kg−1. The basic pH of biochar was 7.5, and particle composition % was as follows: 14 (<0.1 mm), 61.3 (0.1–2 mm), and 25.1 (>2 mm). In the DI method, the irrigation amount was 220 mm and applied in small doses, with each application being 31.42 mm, while in the BI method, the irrigation amount was 300 mm and applied five times, with each application being 60 mm. In the DI method, the sub-main pipeline system supplied water to the laterals, while the lateral pipes on the plot carried water directly to the root zones of the crops. The inner diameter of the lateral pipes was 1.5 cm at a flow rate of 1.3 L h−1. The treatment plots were separated from adjoining plots by 1-m-wide isolation strips in order to account for the marginal effects of various irrigation techniques, and each plot (144 m2) was 18 m long and 8 m wide. In the experimental plot, the planting density was 7–8 plants/m2. The spacing between plants within a row was 14.4 cm, and the drip tapes were spaced apart by 110 cm. The plants were sowed in alternately wide and narrow rows, measuring 0.8 m and 0.3 m. A water reading meter was used to measure the irrigation water amount. To promote optimal seed germination and vigorous seedling establishment, the total dose of phosphorus (50 kg ha−1) and potassium fertilizers (250 kg ha−1) was incorporated into the soil during seedbed preparation. The source of phosphorus was monoammonium phosphate with an NPK content of 12:61:0, produced by Guizhou Kai Phosphorus Group Co., Ltd. (Guiyang, China). Potassium sulfate was used as the source of potassium, and its NPK ratio was 12:0:50, which is produced by Luobupo Potassium Salt Co., Ltd. (Xinjiang, China). To control the weed infestation in the experimental field, mechanical plough operation was performed between space of rows at the 3–5 leaf stage, and manual hand hoeing was performed at the 6–7 leaf stage. The cropping scheme is shown in Figure 2.
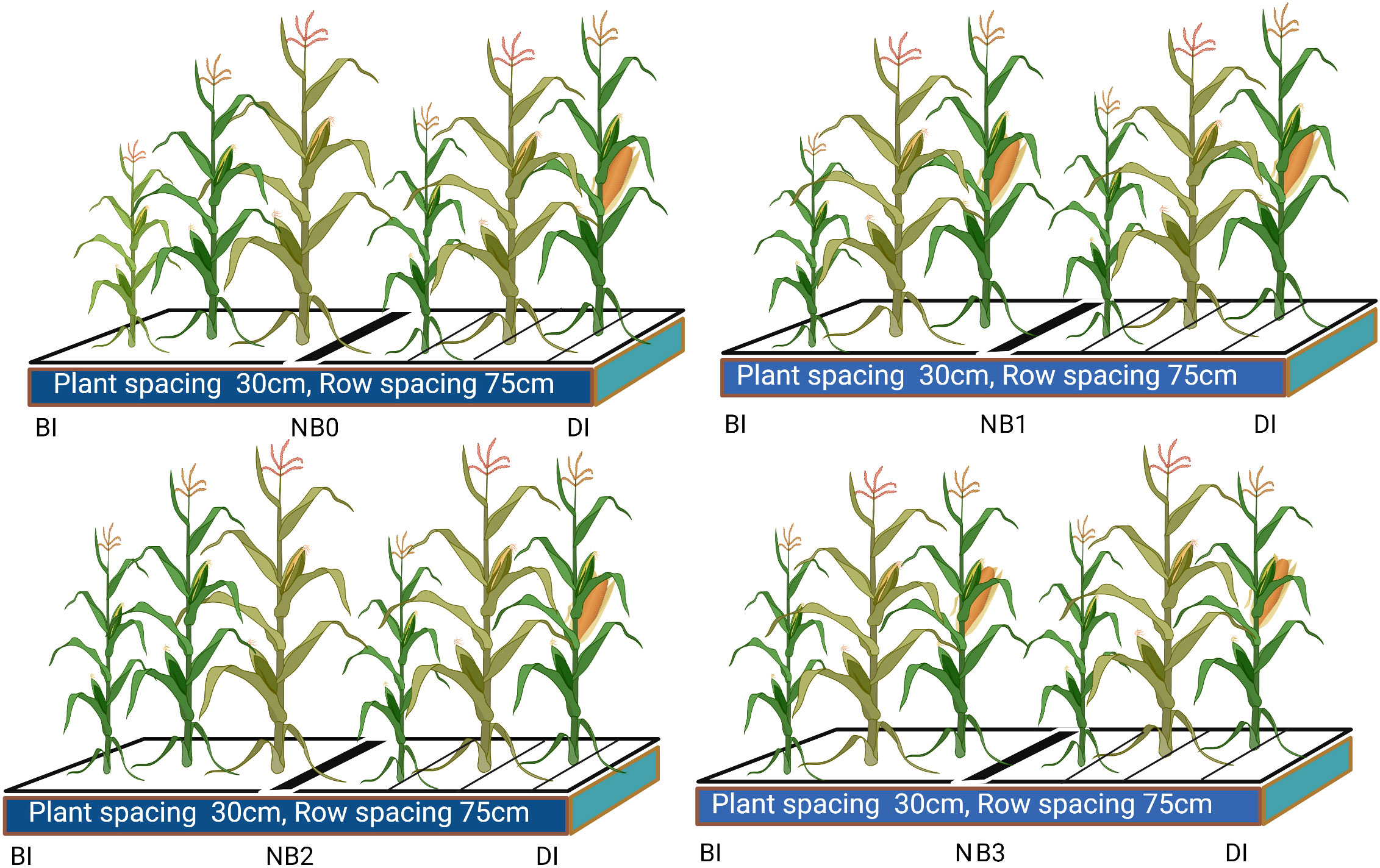
Figure 2 Cropping scheme. BI is the border irrigation method; DI is the drip irrigation method. NB0 is 260 kg N ha−1 without biochar amendment; NB1, NB2, and NB3 are combinations of N fertilizer with low, medium, and high doses of biochar, namely, 15.5 t ha−1, 30.7 t ha−1, and 45.3 t ha−1, respectively.
2.3 Field observations and measurement methods
The soil particle fraction was determined by using a hydrometer (Beretta et al., 2014). The soil water content was measured from soil depths of 0–120 cm at 20-cm intervals in each treatment plot of DI and BI using tubular FDR sensors (Zhang et al., 2020). Fresh soil samples were extracted with 1 mol L−1 KCl (1:10, soil/water) to estimate the soil mineral N concentration (SNC), which was then measured using a continuous flow analyzer (AA3, Bran and Luebbe, Germany). The leaf area index (LAI) was measured on different growth stages using a portable leaf area meter. When maize reached its full physiological maturity and grain moisture dropped below 13%, then it was harvested, and grain yields and dry matter (DM) were obtained. The irrigation water use efficiency (IWUE), fertilizer nitrogen use efficiency (FNUE), and yield changes were calculated as follows:(Si et al., 2020); (Qin et al., 2015); (Leghari et al., 2021)
where I is irrigation (mm), P is precipitation (mm), ET is evapotranspiration (mm), WUE is water use efficiency, IWUE is irrigation use efficiency, and FNUE is fertilizer N use efficiency. NB0 is 260 kg N ha−1 without biochar amendment; NB1, NB2, and NB3 are combinations of N fertilizer with low, medium, and high doses of biochar, namely, 15.5 t ha−1, 30.7 t ha−1, and 45.3 t ha−1, respectively.
2.4 Statistical analysis
The data were statistically analyzed using IBM SPSS Statistics 26. Analysis of variance (ANOVA) was used to determine differences between the control treatment, chemical fertilizer alone, and the interaction of biochar with chemical fertilizer on maize yield. The significant differences were detected at p < 0.05 using Duncan’s multiple ranges. Simple data processing was done in Excel and graphically visualized using Origin2021 software.
3 Results
3.1 Soil water content under the effect of biochar amendments with different irrigation methods
Figure 3 shows the temporal and spatial distribution of SWC from the 0- to 120-cm soil profile under the effect of biochar with BI and DI methods. We measured soil water dynamics in the first and last seasons. In both seasons, it can be seen in Figure 3 that SWC varied with biochar application levels, changing soil depths, and changing irrigation methods. A pattern of SWC distribution was totally different in the DI method as compared to the BI method. In the first season of the BI method, the SWC was increased below 60 cm depth. At the same time, it was decreased with the DI method. The DI method showed higher SWC in the topsoil layer and decreased in deeper soil layers. In non-biochar treatment NB0, the SWC was high at 60–80 cm and 80–100 cm soil depths under the BI method, and it was also higher in the DI method as well. All biochar-added treatments decreased ineffective SWC and maintained SWC at the optimum level. The NB1, NB2, and NB3 treatments under the BI method decreased SWC content at the 80[cm soil depth compared to NB0. The same treatments, NB1, NB2, and NB3, under the DI method, decreased SWC at 60-cm to 100-cm soil depths. The biochar doses were high in NB2 and NB3 treatments; therefore, these treatments mainly enhanced SWC at 20-cm to 40-cm soil depths. Despite the high dose of biochar in NB2 and NB3 treatments restricting water from infiltrating in the deeper soil layers, water still moved into deeper soils under the BI method. However, this treatment showed a clear effect in the DI method. Considering the last season of the experiment, the SWC showed a different pattern of water distribution in the soil profile as compared to the first season. It could be because biochar does not decompose well in the early stage, so biochar particles absorb and store water. In the last season, still, the effect of biochar on different soil depths was positive under the DI method as well as the BI method. Biochar application also decreased ineffective SWC in the soil profile during the last season of the experiment, particularly in the deep soil layer. Maize is a shallow-rooted crop; therefore, it is critical that SWC content must be higher in the topsoil layer. Overall, experimental results related to SWC indicate that biochar application would be effective in BI and DI methods; however, more benefits of biochar can be achieved under the DI method as compared to the BI method.
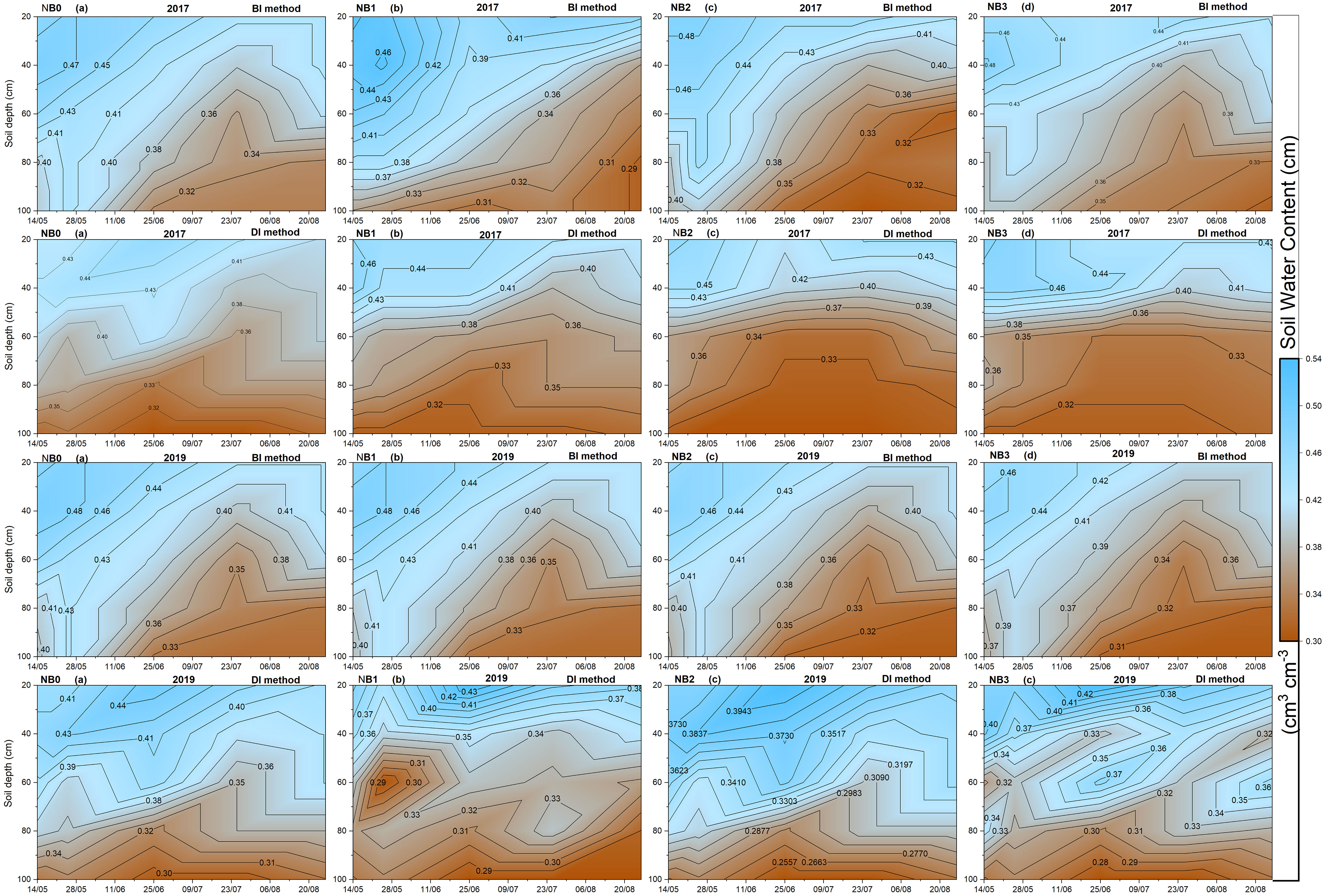
Figure 3 Temporal and spatial distribution of SWC in different depths under the effect of biochar. BI is the border irrigation method; DI is the drip irrigation method. NB0 is 260 kg N ha−1 without biochar amendment; NB1, NB2, and NB3 are combinations of N fertilizer with low, medium, and high doses of biochar, namely, 15.5 t ha−1, 30.7 t ha−1, and 45.3 t ha−1, respectively.
3.2 Soil nitrogen concentration under the effect of biochar amendments with different irrigation methods
Figure 4 shows the effect of biochar on SNC under BI and DI methods. As can be seen in Figure 4, the SNC varied with changing biochar doses, soil depths, and irrigation methods.
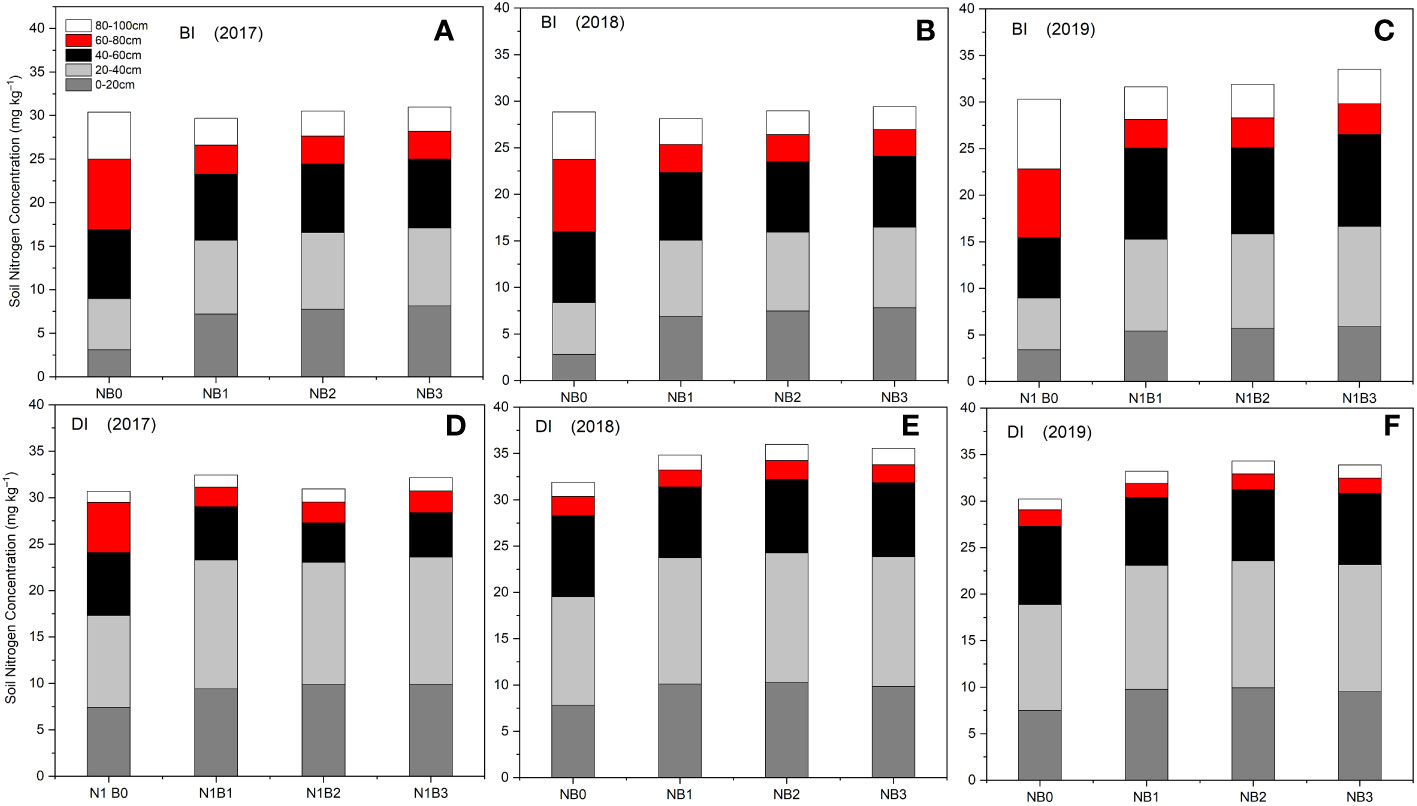
Figure 4 Distribution of SNC in different depths under the effect of biochar in 2017 (A, D), 2018 (B, E) and 2019 (C, F). BI is the border irrigation method; DI is drip irrigation method. NB0 is 260 kg N ha−1 without biochar amendment, NB1, NB2, and NB3 are combinations of N fertilizer with low, medium, and high doses of biochar, namely, 15.5 t ha−1, 30.7 t ha−1, and 45.3 t ha−1, respectively.
3.2.1 BI method
In this irrigation method, the SNC at 0–20 cm, 20–40 cm, and 40–60 cm depths were significantly lower in non-biochar treatment NB0 and higher in 60–80 cm and 80–100 cm depths. This could be due to the maximum transportation of N along with water from the topsoil layers and accumulation in deeper soil layers. Despite the irrigation amount being high in the BI method, biochar holds N in upper soil layers in all treatments containing biochar sources. Biochar combined treatments, including NB1, NB2, and NB3, resulted in maximum SNC at 0–20 cm, 20–40 cm and 40–60 cm depths and decreased SNC in 60–80 cm and 80–100 cm soil depths. This trend of N accumulation in soil was similar in the 2017, 2018, and 2019 seasons. However, SNCs were relatively higher in the last year of the experimental field. In the BI method, SNC level of NB0 ranged from 2.81 to 3.41 mg·kg−1 at 0–20 cm soil depth, from 5.86 to 5.55 mg·kg−1 at 20–40 cm depth, from 6.45 to 7.91 mg·kg−1 at 40–60 cm depth, from 7.41 to 8.12 mg·kg−1 at 60–80 cm depth, and from 5.06 to 7.50 mg·kg−1 at 80–100 cm soil depth. In NB1, NB2, and NB3 treatments, the SNC ranged from 5.41 to 8.13 mg·kg−1 at 0–20 cm soil depth, from 9.86 to 10.76 mg·kg−1 at 20–40 cm depth, from 7.27 to 9.86 mg·kg−1 at 40–60 cm depth, from 2.88 to 3.32 mg·kg−1 at 60–80 cm depth, and from 2.46 to 3.72 mg·kg−1 at 80–100 cm soil depth.
3.2.2 DI method
In this irrigation method, compared to the BI method, the SNCs at 0–20 cm and 20–40 cm were significantly higher in all treatments, including non-biochar treatment, mainly SNCs in NB1, NB2, and NB3 treatments. Unlike the BI method, the DI method decreased SNC at 60–80 cm and 80–100 cm deeper soil layer depths. This could be because N did not transport along with water application from the topsoil layers and accumulated in topsoil layers because the amount of water in the DI method was significantly lower than in the BI method. Overall, results indicated that biochar holds maximum N in upper soil layers under the DI method. In all treatments, the SNC at 60–80 cm and 80–100 cm were substitutionally lower than in the BI method. The biochar’s ability to store SNC was further enhanced in the DI method. In the DI method, SNC in NB0 ranged from 7.41 to 7.84 mg·kg−1 at 0–20 cm soil depth, from 9.90 to 11.72 mg·kg−1 at 20–40 cm depth, from 6.79 to 8.72 mg·kg−1 at 40–60 cm depth, from 1.77 to 5.41 mg·kg−1 at 60–80 cm depth, and from 1.17 to 1.50 mg·kg−1 at 80–100 cm soil depth. In NB1, NB2, and NB3 treatments, SNC ranged from 9.41 to 10.27 mg·kg−1 at 0–20 cm soil depth, from 13.16 to 14.01 mg·kg−1 at 20–40 cm depth, from 4.26 to 7.99 mg·kg−1 at 40–60 cm depth, from 1.50 to 2.33 mg·kg−1 at 60–80 cm depth, and from 1.30 to 1.76 mg·kg−1 at 80–100 cm soil depth. Thus, experimental results related to SNC indicate that biochar application would be effective in both BI and DI methods; however, soil N holding efficiency of biochar under the DI method can be high compared to the BI method.
3.3 Soil organic matter, pH, and aggregate composition changes under the effect of biochar amendments with different irrigation methods
Figure 5 shows the effect of biochar on soil OM and pH and Aggregate composition under BI and DI methods. The soil OM content varied with biochar application doses in both BI and DI methods. The soil OM content significantly increased with biochar amendment as compared to non-biochar treatment. The OM contents in NB1, NB2, and NB3 were significantly higher than in NB0. OM content gradually increased from the first season to the third season of the experiment. The OM contents were 13.3–14.3 g·kg−1, 14.8–16.1 g·kg−1, 15.2–17.0 g·kg−1, and 15.5–17.7 g·kg−1 in NB0, NB1, NB2, and NB3 treatments, respectively under BI and DI methods. Compared to non-biochar treatment under the BI method, NB1 increased OM by 11.28%–14.60%, NB2 by 14.29%–18.98%, and NB3 by 21.98%–23.36% from the first to the last season of the experiment. In the DI method, NB1 increased OM by 11.11%–14.39%, NB2 by 14.81%–19.42%, and NB3 by 16.30%–23.78%, compared to the NB0 treatment. This indicated that soil OM highly changes due to biochar application rates, but the irrigation method has some effect. The medium and high doses of biochar contributed largely to soil OM increments. The higher the biochar application rate, the higher the soil OM content, because biochar is a charred organic substance itself. Furthermore, the increase in OM content increased soil aggregate stability. Results showed that compared to non-biochar treatment, the NB1, N1B2, and N1B3 treatments improved the weight diameter of soil aggregates, and it is varied with irrigation methods as well. In the BI method, NB1, NB2, and NB3 increased the average soil aggregates stability by 2.45%, 4.01%, and 11.71%, and in the DI method, it increased by 4.52%, 8.19%, and 14.66%, respectively. In terms of soil pH, results revealed that biochar combination with N fertilizer could regulate soil pH under BI and DI methods. The NB1, NB2, and NB3 treatments significantly decreased soil pH compared to N fertilizer alone. In 3 years, NB1 decreased soil pH on average by 1.93%–4.99% and NB2 and NB2 by 2.44%–6.36%, compared to NB0 treatment under BI and DI methods. The pH, OM, and soil aggregate stability indicators indicated that biochar application would be crucial to improve soil properties.
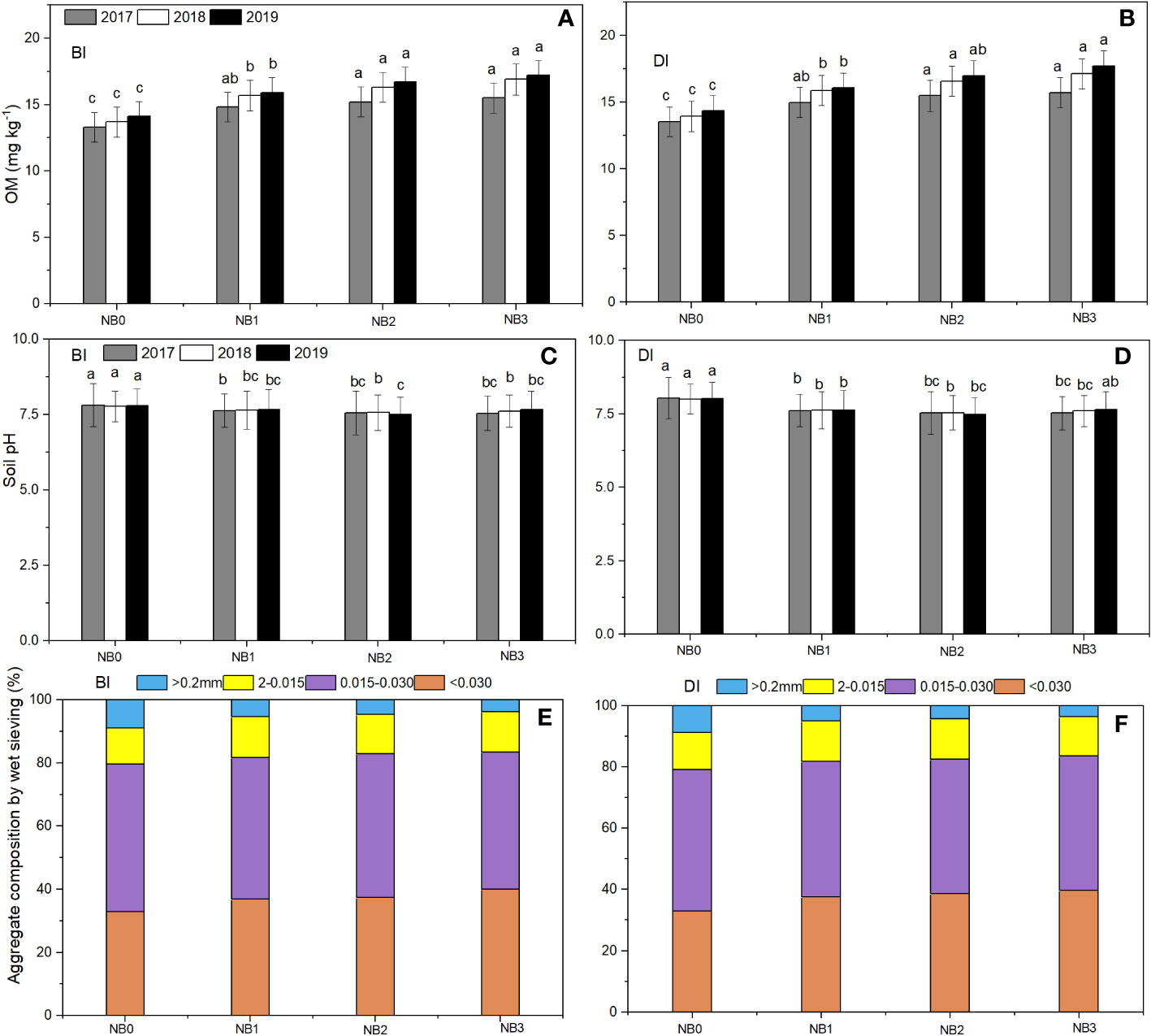
Figure 5 Soil OM, pH, and aggregate composition changes under the effect of biochar amendments. BI is the border irrigation method (A, C, E); DI is the drip irrigation method (B, D, F). NB0 is 260 kg N ha−1 without biochar amendment; NB1, NB2, and NB3 are combinations of N fertilizer with low, medium, and high doses of biochar, namely, 15.5 t ha−1, 30.7 t ha−1, and 45.3 t ha−1, respectively.
3.4 Maize leaf area index under biochar amendments with different irrigation methods
Figure 6 shows the effect of biochar on the LAI of maize crops under BI and DI methods. It can be seen in Figure 6 that irrigation methods have a significant (p < 0.05) effect on maize LAI development, and LAI development is also influenced by biochar doses, but the final LAI was not significantly different (p > 0.05). This could be due to the effect of rainfall or the maize plant recovered vegetative growth rate at the maturity stage, but it did not contribute to yield. The LAI was relatively larger during the second and third seasons as compared to the first season. It could be due to biochar increased nutrient availability in soil with time. The final LAI of maize in NB0 under the BI method was 3.80 m2 m−2, 3.90 m2 m−2, and 3.97 m2 m−2, and under the DI method, it was 4.20 m2 m−2, 4.40 m2 m−2, and 4.36 m2 m−2 in 2017, 2018, and 2019, respectively. The LAI of maize in NB1 under the DI method was 3.90 m2 m−2, 4.00 m2 m−2, and 4.07 m2 m−2, and under the DI method, it was 4.30 m2 m−2, 4.40 m2 m−2, and 4.46 m2 m−2 in 2017, 2018, and 2019, respectively. The maximum LAI of maize was in NB2 and NB3 under both BI and DI methods. The LAI of maize in NB2 and NB3 reached up to 4.56 m2 m−2. Compared to a maximum value of LAI in NB0 treatment under the BI method, the LAI of maize in NB2 and NB3 increased by 14%–15.11% under the DI method. Results demonstrated that the application of biochar could promote maize vegetative growth, and it could be clearly measured at different growth stages. In this regard, further research work is required.
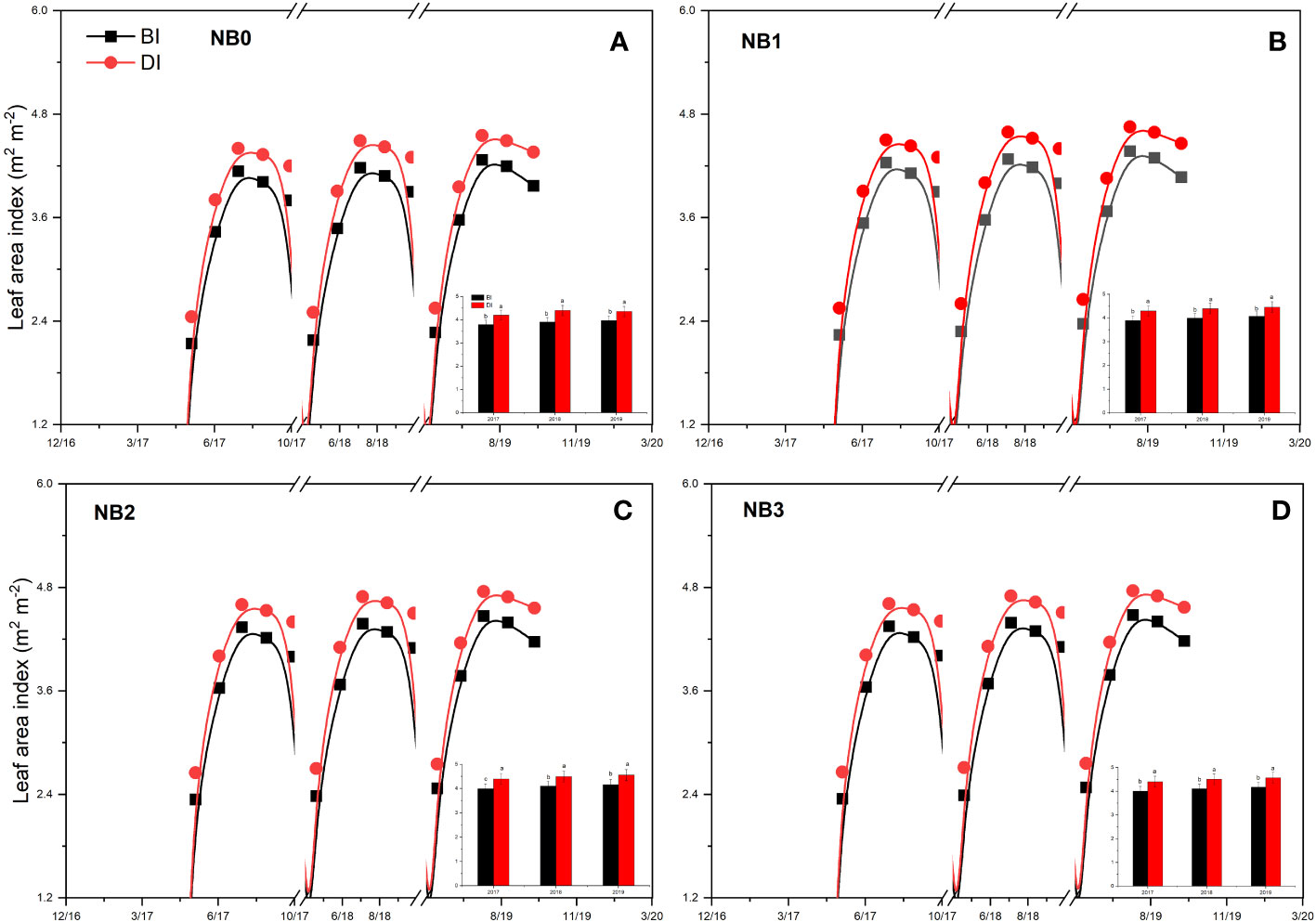
Figure 6 Maize LAI under the effect of biochar amendments. BI is the border irrigation method; DI is the drip irrigation method. NB0 is 260 kg N ha−1 without biochar amendment (A); NB1, NB2, and NB3 are combinations of N fertilizer with low (B), medium (C), and high (D) doses of biochar, namely, 15.5 t ha−1, 30.7 t ha−1, and 45.3 t ha−1, respectively.
3.5 Maize grain yields under biochar amendments with irrigation methods
Figure 7 and Table 2 show the effect of biochar on maize grain yields and its characteristics under BI and DI methods. The irrigation methods and biochar doses have a significant effect on maize yield, IWUE, and FNUE at p < 0.05 and p < 0.01.
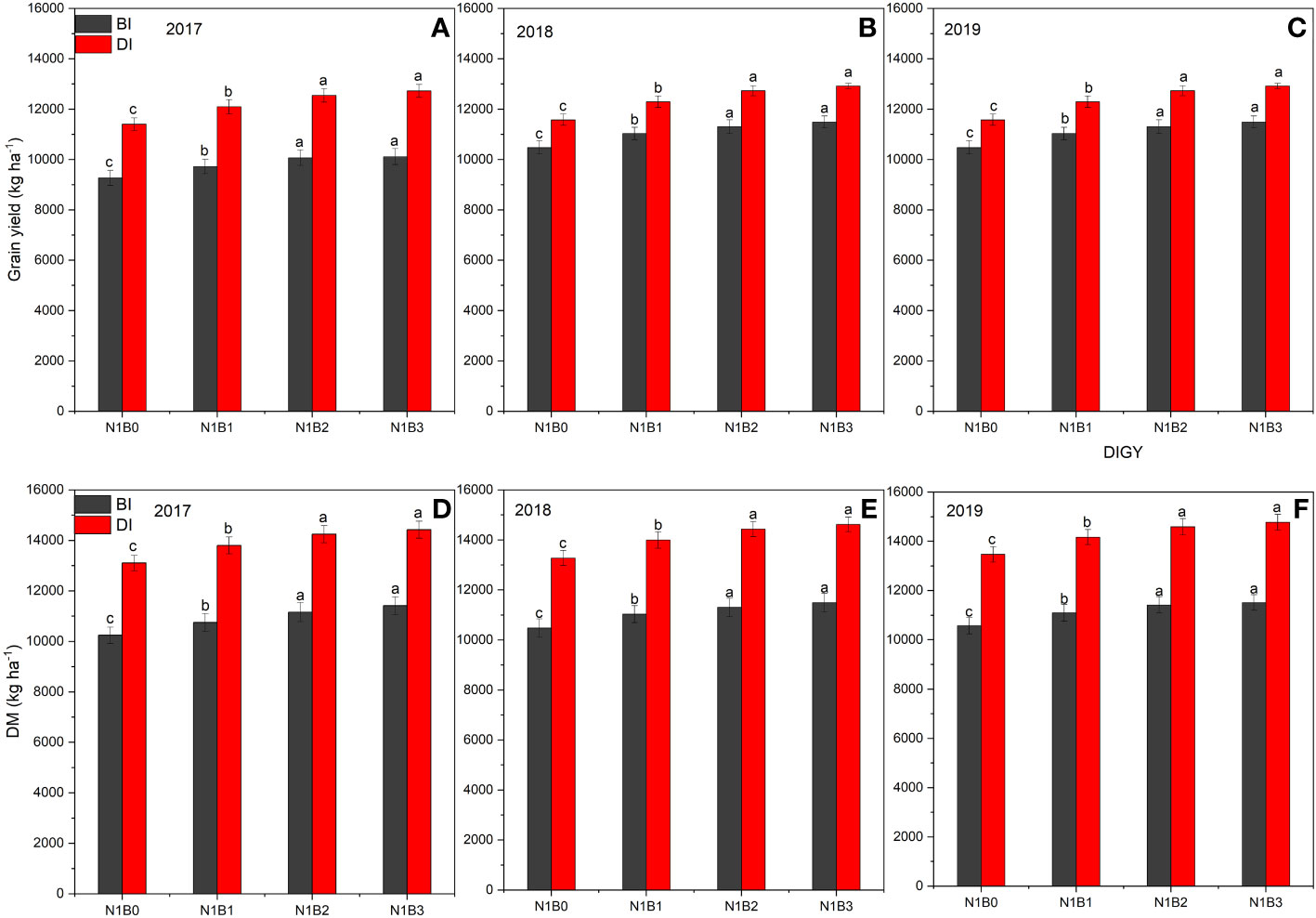
Figure 7 Maize grain yields under the effect of biochar amendmentsin 2017 (A, D), 2018, (B, E), 2019 (C, F). BI is the border irrigation method; DI is the drip irrigation method. NB0 is 260 kg N ha−1 without biochar amendment, NB1, NB2, and NB3 are combinations of N fertilizer with low, medium, and high doses of biochar, namely, 15.5 t ha−1, 30.7 t ha−1, and 45.3 t ha−1, respectively. Different lowercase letters indicate significant difference at p < 0.05.
3.5.1 BI method
In this irrigation method, the total maize productions of NB0, NB1, and NB2 treatments were 27,956 kg, 29,342 kg, and 30,294 kg, respectively, from 2017 to 2019. In t he NB0 non-biochar treatment, the maize yield was 9,270 kg ha–1 in 2017, 9,337 kg ha–1 in 2018, and 9,823 kg ha–1 in 2019. In NB1, the maize yield was 9,719 kg ha–1 in 2017, 9,800 kg ha–1 in 2018, and 12,470 kg ha–1 in 2019. In NB2, the maize yield was 10,067 kg ha–1 in 2017, 10,100 kg ha–1 in 2018, and 10,127 kg ha–1 in 2019. In NB3, the maize yield was 10,112 kg ha–1 in 2017, 10,251 kg ha–1 in 2018, and 10,291 kg ha–1 in 2019. Thus, on an annual average basis, NB0 had 9,319 kg ha–1, NB1 had 9,781 kg ha–1, NB2 had 10,098 kg ha–1, and NB3 had 10,218 kg ha–1. Compared to NB0, the NB1, NB2 and NB3 treatments increased maize yield by 4.96%, 8.36%, and 9.65%, respectively. Regarding IWUE, the non-biochar treatment NB0 had a lower IWUE compared to biochar doses combined with fertilizer. Maize IWUE in NB0 was 3.09 kg kg−1, 3.11 kg kg−1, and 3.12 kg kg−1 in 2017, 2018, and 2019, respectively, whereas maize IWUE in NB1 ranged from 3.24 to 3.27 kg kg−1. The NB2 and NB2 showed maximum IWUE, ranging from 3.36 to 3.43 kg kg−1. Considering the annual average IWUE of maize under different biochar treatments, the IWUE of NB1, NB2, and NB3 treatments increased by 5%–10% compared to non-biochar treatment. Similarly, biochar amendments also improved the FNUEs of maize crops; the maize had higher FNUE in NB1, NB2, and NB3 treatments. FNUE of the NB0 treatment ranged from 35.65 to 35.96 kg kg−1., whereas FNUE of the NB1 treatment ranged from 37.38 to 37.78 kg kg−1, FNUE of the NB2 treatment ranged from 38.72 to 38.95 kg kg−1, and FNUE of NB3 ranged from 38.89 to 39.58 kg kg−1. The order of yield, IWUE, and FNUE was NB3 ≥ NB2 > N1B1 > N1B0.
3.5.2 DI method
In this irrigation method, the total maize production of NB0, NB1, NB2, and NB3 treatments were 36,863 kg, 36,863 kg, and 38,169 kg, respectively, from 2017 to 2019. In the NB0 non-biochar treatment, the maize yield was 11,400 kg ha–1 in 2017, 11,576 kg ha–1 in 2018, and 11,770 kg ha–1 in 2019. In NB1, the maize yield was 12,100 kg ha–1 in 2017, 12,293 kg ha–1 in 2018, and 12,470 kg ha–1 in 2019. In NB2, the maize yield was 12,547 kg ha–1 in 2017, 12,732 kg ha–1 in 2018, and 12,891 kg ha–1 in 2019. In NB3, the maize yield was 12,720 kg ha–1 in 2017, 12,916 kg ha–1 in 2018, and 13,072 kg ha–1 in 2019. Thus, on an annual average basis, NB0 had 11,582 kg ha–1, NB1 had 12,288 kg ha–1, NB2 had 12,723 kg ha–1, and NB3 had 12,903 kg ha–1. Compared to NB0, the NB1, NB2, and NB3 treatments increased yield by 6.10%, 9.85%, and 11.41%, respectively. In terms of IWUE, the non-biochar treatment NB0 had a lower IWUE compared to biochar combined with fertilizer treatments. Maize IWUE in NB0 was 5.18 kg kg−1, 5.26 kg kg−1, and 5.35 kg kg−1 in 2017, 2018, and 2019, respectively, whereas maize IWUE in NB1 ranged from 5.50 kg kg−1 to 5.67 kg kg−1. The NB2 and NB2 showed higher IWUE, ranging from 5.70 kg kg−1 to 5.94 kg kg−1. Considering the annual average IWUE of maize under different biochar treatments, the IWUE of NB1, NB2, and NB3 treatments increased by 6%–11% compared to non-biochar treatment. Similarly, biochar amendments also improved the FNUEs of maize crops; the maize had higher FNUE in NB1, NB2, and NB3 treatments. FNUE of NB0 treatment ranged from 43.85 to 45.27 kg kg−1, whereas FNUE of the N1B1 treatment ranged from 46.54 to 47.96kg kg−1, FNUE of the NB2 treatment ranged from 48.26 to 49.58 kg kg−1, and FNUE of NB3 ranged from 48.92 to 50.28 kg kg−1. The order of FNUE of maize under different treatments was the same as the BI method. However, in the DI method, the FNUE of maize was much higher than in the BI method. The order of yield, IWUE, and FNUE was NB3 ≥ NB2 > N1B1 > N1B0 in the DI method, whereas considering the irrigation method, the order of yield, IWUE, and FNUE was DI > FI.
4 Discussion
4.1 Effect of biochar on maize grain yields, IWUE, and FNUE under BI and DI methods
The fertilization and irrigation methods significantly affect maize yield, IWUE, and FNUE. Chemical N fertilizer alone was not efficient enough to increase yield. Biochar is a carbon-rich substance made from the pyrolysis of biomass. Its combined application with N fertilizer stimulates water retention capacity, water, and N uptake (Yang et al., 2022). Thus, it increases water and N productivity (Wu et al., 2021). The findings of this study revealed that biochar amounts improved maize yields, IWUE, and FNUE, and there were significant differences between the BI and DI methods at p < 0.05. The addition of low, medium, and high biochar doses 15.5 t ha−1, 30.7 t ha−1, and 45.3 t ha−1 with 260 kg N ha−1 increased maize yield by 4.96%, 8.36%, and 9.65% in the BI method, respectively. The benefit of biochar amendment was further enhanced in the DI method. The same combination of chemical fertilizer with biochar in the DI method increased maize yield by 6.10%, 9.85%, and 11.41%, respectively. These results are consistent with previous research that has shown the obvious impact of biochar with N fertilizer on crop yield (Jyrinki, 2022). Our results are also aligned with He et al. (2021), and Pathy et al. (2020) reported a significant increase in grain yields with the use of biochar. The positive effect of biochar on maize yield could be attributed to the following: (i) biochar enhances the soil texture and structure and microbial activity (Phares et al., 2022); (ii) it supplies minerals and soil organic carbon for plant growth (Liu et al., 2016); and (iii) it enhances soil nutrient storage, transformation, and absorption (Uzoma et al., 2011).
Regarding IWUE, results demonstrated that the N fertilizer application with biochar doses has a greater impact on maize IWUE than the non-biochar dose. Incorporating biochar into the soil can increase the IWUE of maize production systems. In the BI method, the IWUE varied from 3.09 to 3.12 kg kg–1 in NB0, while the N fertilizer with biochar doses (NB1, NB2, and NB3) showed higher IWUE, ranging from 3.24 to 3.43 kg kg–1. These results are consistent with Wu et al. (2022) and Ullah et al. (2020) studies; they reported an increased IWUE with the use of biochar and found that biochar maintained soil moisture by decreasing water loss via evapotranspiration, enhanced photosynthetic efficiency, and promoted chlorophyll synthesis and biomass accumulation. Similar to this, under the DI method, the IWUE varied from 5.18 to 5.35 kg kg–1 in NB0, while the N fertilizer with biochar doses exhibited higher IWUE, ranging from 5.50 to 5.94 kg kg–1. These findings are in line with previous studies that have highlighted the water-saving potential of biochar in DI systems. Qian et al. (2023) and Zheng et al. (2022) found an improved IWUE with the use of biochar in both BI and DI methods. The improved water-holding capacity of biochar-amended soils can also lead to reduced irrigation requirements, thereby improving IWUE. However, the specific effects may differ depending on factors such as biochar characteristics, soil properties, and environmental conditions.
Furthermore, the results exhibited that biochar treatments have higher FNUE compared to the non-biochar treatment under BI and DI methods. This indicates that the addition of biochar can increase N utilization in maize. In the BI method, the FNUE varied from 35.65 to 39.58 kg ha–1 in NB0, while the N fertilizer with biochar doses showed higher FNUE, ranging from 37.38 to 39.58 kg ha–1. These results related to FNUE are in line with previous research that has reported improved FNUE with biochar amendments (Guo et al., 2021; Xu et al., 2023). Findings indicated that biochar application with N fertilizer increases plant N absorption and may be used as an N-releaser to efficiently supply sufficient substrates for plant growth and development (Ullah et al., 2021). The increase in plant N content is mainly linked to the use of biochar, which ensures more N is available to plants (Case et al., 2014) and enhances soil physicochemical qualities, such as water-holding capacity and bulk density, to provide ideal conditions for root development (Reibe et al., 2015). Under the DI method, the FNUE varied from 43.85 to 45.27 kg ha–1 in NB0, while the N fertilizer with biochar doses exhibited higher FNUE, ranging from 46.54 to 50.28 kg ha–1. These findings are consistent with Cao et al. (2019), and Tian et al. (2022) reported a significantly higher FNUE with the use of biochar, which improved soil nutrient cycling and reduced N losses. The following processes might explain boosting biochar’s effect on FNUE: (i) it binds N to create an agglomerated particle, preventing N2O emission and release of N (Shi et al., 2022), and (ii) it provides enough N by raising cation exchange capacity (Kumar et al., 2018). Overall results show that adding biochar into maize cropping systems, whether utilizing the BI or DI method, can result in higher maize yield, and increased IWUE and FNUE.
4.2 Effect of biochar on soil water and N dynamics under BI and DI methods
The SNC and SWC are crucially important in upper soil layers. The combined application of N fertilizer and biochar can boost upper soil fertility by decreasing nutrient leaching (Li et al., 2023). Biochar-amended soil water retention ability can enhance SWC by lowering water loss through evaporation and deep percolation under BI and DI methods (Zhao et al., 2020). Our findings suggest that biochar application can favorably alter soil water dynamics under various irrigation systems. The effect of biochar on SWC was observed to be significant, especially in the upper soil layers, and the pattern of SWC distribution differed between BI and DI methods. Results are consistent with earlier research studies that determined the impact of biochar on the water dynamics of soil. Liu et al. (2022) showed that biochar could improve soil water storage and infiltration, resulting in enhanced plant growth; Graber et al. (2010) reported that biochar could increase water retention capacity and decrease water loss due to evaporation, and Jeffery et al. (2011) found that biochar application can improve soil water-holding capacity, particularly in sandy soils. However, some studies have shown mixed results on the effect of biochar on soil water dynamics. For instance, Li et al. (2018) reported that the use of biochar did not significantly affect soil water retention capacity, although it did increase soil physical properties, and Major et al. (2010) found that the effect of biochar on SWC was highly dependent on biochar properties and soil type. The current study verified that adding biochar has a critical impact on soil water dynamics, especially in the DI method. The SWC varied with biochar doses and soil depths. Biochar doses under DI showed a higher SWC in the topsoil layer and decreased in deeper soil layers, and the effect of medium and high doses of biochar was obvious, which is consistent with the Dong et al. (2019) study. The results of the present study indicate that under BI, the SNC was lower in the non-biochar treatment in the upper depths and higher in the lower depths. This could be due to N fertilizer drained deep through the application of water, while the DI method decreased SNC at deeper soil layer depths. This suggests that N did not drain with water because of the low amount of irrigation application and biochar. These results agree with the finding of Gwenzi et al. (2016) who investigated the effects of different irrigation methods on SNC with the use of biochar and found that BI resulted in lower SNC at shallow depths but higher concentration at deeper depths, and Clough et al. (2013) studied the impact of biochar on N dynamics under different irrigation methods and found that the use of biochar decreased SNC in the upper soil layers for all irrigation methods. Overall, these results show that biochar stores the most N in the DI method, and this irrigation strategy also improves biochar’s capacity to store SNC. These results are related to the finding of Mehmood et al. (2020); the study found that biochar application increased SNC, reduced N leaching, and enhanced its use efficiency in maize crops. This can be attributed to the high cation exchange capacity and surface area of biochar, which promote N retention, and Lehmann et al. (2011) reported that biochar amendment enhanced accessibility in soil, resulting in improved N utilization of maize. Biochar’s porous nature makes it easier for water and nutrients to flow around, which improves water use effectiveness and decreases nutrient losses. The beneficial effects of biochar on water and N dynamics under various irrigation techniques are also demonstrated by these researchers. It is crucial to keep in mind that the specific impacts of biochar might change based on factors like the biochar feedstock, pyrolysis settings, soil characteristics, and crop varieties. In addition, various agricultural systems may require a varied biochar treatment rate and timing. Our findings support the concept that biochar can be a valuable soil amendment for improving soil moisture and nutrient status in topsoil layers.
4.3 Effect of biochar on soil OM, pH, and aggregate composition under BI and DI methods
Biochar has the ability to boost soil OM content by functioning as a long-term carbon sink in the soil as a stable form of organic carbon (Jeffery et al., 2011). Biochar can modify soil pH because of its alkaline nature, which can buffer acidic soils and potentially evaluate the pH level (Lehmann et al., 2011). However, depending on several factors, including the biochar composition, soil type, and irrigation methods, the specific impact of biochar on soil pH may change. The results indicate that the use of biochar had a major impact on the soil OM content when compared to the non-biochar treatment. The OM content of the soil increased with increasing biochar doses from low to high. Furthermore, the results also exhibited that the soil OM content gradually raised year after year. This shows that the benefits of biochar on soil OM may increase over time, resulting in even greater soil quality improvements, which agrees with Mehmood et al. (2020) findings. In all three seasons of the experiments, we saw a gradual rise in the soil OM content. The treatments with medium and high biochar application rates (30.7–45.3 t ha−1) highly increased OM content; the increase in soil OM content ranged from 11.11% to 23.78% in both BI and DI methods compared to the non-biochar treatment. These findings are consistent with previous works demonstrating that biochar amendments might increase soil OM accumulation. Yang et al. (2022) reported that the biochar additions increased soil OM content at the top 15 cm of soil by 14%–52% under different amounts of irrigation; Liu et al. (2021) reported that the biochar application rate of 20–60 t ha−1 increased soil OM by 2%–59%; and Dong et al. (2019) found an increase of soil OM by 18%–62%. The higher the biochar dose, the higher the OM content. The increased soil OM resulted in improved soil aggregate stability. Low, medium, and high doses of biochar increased soil aggregate stability to varying degrees. The NB2 and NB3 treatments increased the average soil aggregate stability by 4.01%–14.66% in both irrigation methods. Because soil structure is strangely related to OM. About a 3%–26% increase in soil aggregate stability and consistency is also reported by Blanco-Canqui (2017). In terms of soil pH, the findings showed that combining biochar with N fertilizer had a regulating impact on soil pH under both BI and DI methods. The NB1, NB2, and NB3 treatments resulted in a significant drop in soil pH compared to the NB0 treatment each year. The reduction in soil pH ranged from 1.93 to 6.36% in BI and DI methods. This finding suggests that biochar application with N fertilizer can help in pH adjustment, which is also confirmed by Phares et al. (2022), whereas N fertilizer application alone resulted in an increase in soil pH, which is consistent with the findings of Dong et al. (2022). Owing to its alkaline nature and significant potential to buffer soil pH, biochar seems to be a liming material in neutralizing the released protons in the event of N fertilizer application (Bolan et al., 2022). The findings of this study suggest that biochar application enhanced soil OM content substantially and medium and high biochar dosages have the largest impact on soil OM content. The data also demonstrate biochar’s regulating effect on soil pH when combined with N fertilizer.
5 Conclusion
In this study, the effect of different biochar doses on maize grain yields, N, and water dynamics was compared between conventional BI and DI methods. It was concluded that the application of medium (30.7 t ha−1) and high (45.3 t ha−1) doses of biochar could increase maize yield by 8.36%–9.65% and 9.85%–11.41% under BI and DI methods, respectively, compared to N fertilizer alone. Moreover, biochar increased FNUE and IWUE in both irrigation methods. Biochar application improved the SWC in topsoil layers. Results showed that in the BI method, the NB0 treatment decreased SNC at topsoil layers and increased at deep soil layers because N was transported along with a heavy application of water. When the irrigation method changed from BI to DI, the same treatment increased the SNC at the top layers and decreased it at deeper soil depths. The application of biochar doses further influenced the change in SNC. All combinations of N fertilizer with low, medium, and high biochar doses, namely, 15.5 t ha−1, 30.7 t ha−1, and 45.3 t ha−1, respectively, decreased N deposition in deeper soil layers. The SNC deposition in deeper soil layers under the DI method was much lower than in the BI method, indicating that biochar N holding efficiency in the topsoil layer was further enhanced in the DI method. Considering maize yield, FNUE, IWUE, and SNC dynamics, biochar application would be effective in the DI method, and a medium dose of biochar would be enough. Moreover, the effect of biochar was obvious on soil OM, soil aggerate stability, and regulation of pH. The soil OM increased with the application dose of biochar. Thus, it improved soil aggregate stability. The biochar application would be crucial to increase soil OM, improve soil structure, and maintain pH. In future studies, the long-term effect of biochar on soil properties should be studied with different N fertilizer application rates, and the optimal ratio of chemical fertilizer and biochar should be determined.
Data availability statement
The original contributions presented in the study are included in the article/supplementary material. Further inquiries can be directed to the corresponding authors.
Author contributions
LJ: funding acquisition, conceptualization, and supervision. JW: resources and project administration. LW and SL: investigation, writing—original draft, and methodology. LW, SL, NW, and MP: data collection. LW, SL, and JW: formal analysis. LJ, NW, and MP: writing—review and editing. All authors read and approved the final manuscript.
Funding
This research received funding from the Science and Technology Capacity Improvement Project of Beijing Academy of Agricultural and Forestry Sciences (ZHS202304), Technological Innovation for the Protection and Utilization of Black Land (XDA28130200), the National Key R&D Plan (2022YFD1500204), the Beijing Academy of Agricultural and Forestry Sciences Youth Fund (QNJJ202214), the general program of NSFC (41977095), and the Beijing Academic Program.
Conflict of interest
The authors declare that the research was conducted in the absence of any commercial or financial relationships that could be construed as a potential conflict of interest.
Publisher's note
All claims expressed in this article are solely those of the authors and do not necessarily represent those of their affiliated organizations, or those of the publisher, the editors and the reviewers. Any product that may be evaluated in this article, or claim that may be made by its manufacturer, is not guaranteed or endorsed by the publisher.
References
Beretta, A. N., Silbermann, A. V., Paladino, L., Torres, D., Bassahun, D., Musselli, R., et al. (2014). Análisis de textura del suelo con hidrómetro: Modificaciones al método de Bouyoucus. Ciencia e Investigacion Agraria 41 (2), 263–271. doi: 10.4067/S0718-16202014000200013
Blanco-Canqui, H. (2017). Biochar and soil physical properties. Soil Sci. Soc. America J. 81, 687–711. doi: 10.2136/sssaj2017.01.0017
Bolan, N., Sarmah, A., Bordoloi, S., Bolan, S., Padhye, L., Van Zwieten, L., et al. (2022). Soil acidification and the liming potential of biochar. Environmental Pollution 317, 120632. doi: 10.1016/j.envpol.2022.120632
Borchard, N., Schirrmann, M., Cayuela, M., Kammann, C., Wrage-Mönnig, N., Estavillo, J. M., et al (2019). Biochar, soil and land-use interactions that reduce nitrate leaching and N2O emissions: a meta-analysis. Science of the Total Environment 651, 2354–2364. doi: 10.1016/j.scitotenv.2018.10.060
Bowles, T., Atallah, S., Campbell, E. E., Gaudin, A. C., Wieder, W. R., Grandy, A. S. (2018). Addressing agricultural nitrogen losses in a changing climate. Nat. Sustain. 1(8), 399–408. doi: 10.1038/s41893-018-0106-0
Cao, H., Ning, L., Xun, M., Feng, F., Li, P., Yue, S., et al. (2019). Biochar can increase nitrogen use efficiency of Malus hupehensis by modulating nitrate reduction of soil and root. Appl. Soil Ecol. 135, 25–32. doi: 10.1016/j.apsoil.2018.11.002
Case, S. D. C., Mcnamara, N. P., Reay, D. S., Stott, A. W., Grant, H.K., Whitaker, J. (2014). Biochar suppresses N2O emissions while maintaining N availability in a sandy loam soil. Soil Biol Biochem 81, 178–185. doi: 10.1016/j.soilbio.2014.11.012
Chen, J. (2020) Food Security Drivers: the case of China. Available at: http://dspace.unive.it/handle/10579/16756.
Clough, T., Condron, L., Kammann, C., Müller, C. (2013). A Review of Biochar and Soil Nitrogen Dynamics. doi: 10.3390/agronomy3020275
Cui, Z., Chen, X., Zhang, F. (2010). Current Nitrogen management status and measures to improve the intensive wheat-maize system in China. Ambio 39 (5), 376–384. doi: 10.1007/s13280-010-0076-6
Dong, Z., Li, H., Xiao, J., Sun, J., Liu, R., Zhang, A. (2022). Soil multifunctionality of paddy field is explained by soil pH rather than microbial diversity after 8-years of repeated applications of biochar and nitrogen fertilizer. Science of The Total Environment 853, 158620. doi: 10.1016/j.scitotenv.2022.158620
Dong, X., Singh, B. P., Li, G., Lin, Q., Zhao, X. (2019). Biochar increased field soil inorganic carbon content five years after application. Soil Tillage Res. 186, 36–41. doi: 10.1016/j.still.2018.09.013
Du, L., Xu, C. C., Wu, Y., Wang, M., Chen, F. (2018). Water degradation footprint of crop production in Hebei Province. J. Agro-Environment Sci. 37 (2), 286–293. doi: 10.11654/jaes.2017-0987
Fang, J., Su, Y. (2019). Effects of soils and irrigation volume on maize yield, irrigation water productivity, and nitrogen uptake. Sci .Rep. 9(1), 7740. doi: 10.1038/s41598-019-41447-z
Farahani, S. S., Asoodar, M. A., Moghadam, B. K. (2020). Short-term impacts of biochar, tillage practices, and irrigation systems on nitrate and phosphorus concentrations in subsurface drainage water. Environ. Sci. Pollut. Res. 27 (1), 761–771. doi: 10.1007/S11356-019-06942-W
Geng, Y., Cao, G., Wang, L., Wang, S. (2019). Effects of equal chemical fertilizer substitutions with organic manure on yield, dry matter, and nitrogen uptake of spring maize and soil nitrogen distribution. PloS One 14 (7), 1–16. doi: 10.1371/JOURNAL.PONE.0219512
Graber, E. R., Harel, Y. M., Kolton, M., Cytryn, E., Silber, A., David, D. R., et al. (2010). Biochar impact on development and productivity of pepper and tomato grown in fertigated soilless media. Plant Soil 337 (1), 481–496. doi: 10.1007/S11104-010-0544-6
Gu, L., Dong, G., Yu, H., Zhang, K., Lu, X., Wen, H., et al (2021). Preparation of porous biochar by urine assisted pyrolysis of sewage sludge and their application for Eriochrome Black T adsorption. J. Anal. Appl. Pyrolysis 153, 104975. doi: 10.1016/j.jaap.2020.104975
Guo, J., Yang, S., He, Q., Chen, Y., Zheng, F., Zhou, H., et al (2021). Improving benzo (a) pyrene biodegradation in soil with wheat straw-derived biochar amendment: performance, microbial quantity, CO2 emission, and soil properties. J. Anal. Appl. Pyrolysis 156, 105132. doi: 10.1016/j.jaap.2021.105132
Gwenzi, W., Muzava, M., Mapanda, F., Tauro, T. (2016). Comparative short-term effects of sewage sludge and its biochar on soil properties, maize growth and uptake of nutrients on a tropical clay soil in Zimbabwe. J. Integr. Agric. 15 (6), 1395–1406. doi: 10.1016/S2095-3119(15)61154-6
Haider, G., Farooq, M., Shah, T., Malghani, S. (2023) Cereal responses to nutrients and avenues for improving nutrient use effciency. Available at: https://books.google.com/books?hl=en&lr=&id=SPu6EAAAQBAJ&oi=fnd&pg=PA79&dq=5+Cereal+Responses+to+Nutrients+and+Avenues+for+Improving+Nutrient+Use+Effciency&ots=glmGgt5U3F&sig=jDfEnMMdKyzDHX5c9-Se_fSzPKY.
Hallett, S. H., Sakrabani, R., Thompson, A. J., Deeks, L. K., Knox, J. (2017). Improving soil and water management for agriculture: insights and innovation from Malta. MCAST Journal of Applied Research & Practice. 1(1). doi: 10.5604/01.3001.0014.4356
Hassan, A., Gulzar, S., Nawchoo, I. A. (2022). Role of nitrogen in photosynthesis. Adv. Plant Nitrogen Metab. (CRC Press), 86–95. doi: 10.1201/9781003248361-7
He, M., Xiong, X., Wang, L., Hou, D., Bolan, N., Ok, Y. S., et al (2021). A critical review on performance indicators for evaluating soil biota and soil health of biochar-amended soils. J. Hazard. Mater. 414, 125378. doi: 10.1016/j.jhazmat.2021.125378
Huang, S., Yang, W., Ding, W., Jia, L., Jiang, L., Liu, Y., et al. (2021). Estimation of nitrogen supply for summer maize production through a long-term field trial in China. Agronomy 11(7), 1358. doi: 10.3390/agronomy11071358
Jeffery, S., Abalos, D., Prodana, M., Bastos, A. C., Van Groenigen, J. W., Hungate, B. A., et al (2017). Biochar boosts tropical but not temperate crop yields. Environmental Research Letters 12(5), 053001. doi: 10.1088/1748-9326/aa67bd
Jeffery, S., Verheijen, F., Agriculture, M., van der, V., Bastos, A. C. (2011). A quantitative review of the effects of biochar application to soils on crop productivity using meta-analysis. Agric. Ecosyst. Environ. 144(1), 175–187. doi: 10.1016/j.agee.2011.08.015
Ju, X. T., Kou, C. L., Zhang, F. S., Christie, P. (2006). Nitrogen balance and groundwater nitrate contamination: Comparison among three intensive cropping systems on the North China Plain. Environ. Pollut. 143 (1), 117–125. doi: 10.1016/j.envpol.2005.11.005
Ju, X.-T., Zhang, C. (2017). Nitrogen cycling and environmental impacts in upland agricultural soils in North China: A review. J. Integr. Agric. 16 (12), 2848–2862. doi: 10.1016/S2095-3119(17)61743-X
Jyrinki, V. (2022) Impact of biochars on microbial biomass carbon and soil respiration in forest soils. Available at: https://helda.helsinki.fi/handle/10138/349926.
Khan, M. N., Mobin, M., Abbas, Z. K., Alamri, S. A. (2018). Fertilizers and Their Contaminants in Soils, Surface and Groundwater. In: DellaSala, D. A., Goldstein, M. I. (eds.) The Encyclopedia of the Anthropocene, 5, 225–240. doi: 10.1016/B978-0-12-809665-9.09888-8
Kugedera, A. T., Nyamadzawo, G., Mandumbu, R., Nyamangara, J. (2022). Potential of field edge rainwater harvesting, biomass transfer and integrated nutrient management in improving sorghum productivity in semi-arid regions: a review. Agroforestry Syst. 96 (5–6), 909–924. doi: 10.1007/S10457-022-00751-W
Kumar, A., Joseph, S., Tsechansky, L., Privat, K., Schreiter, I. J., Schüth, C., et al (2018). Biochar aging in contaminated soil promotes Zn immobilization due to changes in biochar surface structural and chemical properties. Science of the Total Environment 626, 953–961. doi: 10.1016/j.scitotenv.2018.01.157
Leghari, S. J., Hu, K., Wei, Y., Wang, T., Bhutto, T. A., Buriro, M. (2021). Modelling water consumption, N fates and maize yield under different water-saving management practices in China and Pakistan. Agric. Water Manage. 255, 107033. doi: 10.1016/j.agwat.2021.107033
Lehmann, J., Rillig, M., Thies, J., Masiello, C., Hockaday, W. C., Crowley, D. (2011). Biochar effects on soil biota–a review. Soil Biol. Biochem. 43(9), 1812–1836. doi: 10.1016/j.soilbio.2011.04.022
Letey, J., Vaughan, P. (2013). Soil type, crop and irrigation technique affect nitrogen leaching to groundwater. California Agric. 67 (4), 231–241. doi: 10.3733/ca.E.v067n04p231
Li, H., Li, Y., Xu, Y., Lu, X. (2020). Biochar phosphorus fertilizer effects on soil phosphorus availability. Chemosphere. 244, 125471. doi: 10.1016/j.chemosphere.2019.125471
Li, C., Xiong, Y., Qu, Z., Xu, X., Huang, Q., Huang, G. (2018). Impact of biochar addition on soil properties and water-fertilizer productivity of tomato in semi-arid region of Inner Mongolia, China. Geoderma. 331, 100–108. doi: 10.1016/j.geoderma.2018.06.014
Li, C., Zhao, C., Zhao, X., Wang, Y., Lv, X., Zhu, X., et al. (2023). Beneficial effects of biochar application with nitrogen fertilizer on soil nitrogen retention, absorption and utilization in maize production. Agronomy. 13(1), 113. doi: 10.3390/agronomy13010113
Liu, Y., Li, H., Hu, T., Mahmoud, A., Li, J., Zhu, R., et al. (2022). A quantitative review of the effects of biochar application on rice yield and nitrogen use efficiency in paddy fields: A meta-analysis. Sci. Total Environ. 830, 154792. doi: 10.1016/j.scitotenv.2022.154792
Liu, B., Li, H., Li, H., Zhang, A., Rengel, Z. (2021). Long-term biochar application promotes rice productivity by regulating root dynamic development and reducing nitrogen leaching. GCB Bioenergy 13 (1), 257–268. doi: 10.1111/gcbb.12766
Liu, Y., Lu, H., Yang, S., Wang, Y. (2016). Impacts of biochar addition on rice yield and soil properties in a cold waterlogged paddy for two crop seasons. Field Crops Res. 191, 161–167. doi: 10.1016/j.fcr.2016.03.003
Liu, C., Wang, K., Meng, S., Zheng, X., Zhou, Z., Han, S., et al. (2011). Effects of irrigation, fertilization and crop straw management on nitrous oxide and nitric oxide emissions from a wheat–maize rotation field in northern China. Agric. Ecosyst. Environ. 140 (1–2), 226–233. doi: 10.1016/J.AGEE.2010.12.009
Major, J., Lehmann, J., Rondon, M., Goodale, C. (2010). Fate of soil-applied black carbon: Downward migration, leaching and soil respiration. Global Change Biol. 16 (4), 1366–1379. doi: 10.1111/J.1365-2486.2009.02044.X
Mehmood, I., Qiao, L., Chen, H., Tang, Q., Woolf, D., Fan, M. (2020). Biochar addition leads to more soil organic carbon sequestration under a maize-rice cropping system than continuous flooded rice. Agric. Ecosyst. Environ. 298, 106965. doi: 10.1016/j.agee.2020.106965
Pathy, A., Ray, J., Paramasivan, B. (2020). Biochar amendments and its impact on soil biota for sustainable agriculture. Biochar 2 (3), 287–305. doi: 10.1007/S42773-020-00063-1
Phares, C. A., Amoakwah, E., Danquah, A., Akaba, S., Frimpong, K. A., Mensah, T. A. (2022). Improved soil physicochemical, biological properties and net income following the application of inorganic NPK fertilizer and biochar for maize production. Acta Ecologica Sinica 42(4), 289–295. doi: 10.1016/j.chnaes.2021.12.002
Phares, C., Atiah, K., Frimpong, K., Danquah, A. (2020). Application of biochar and inorganic phosphorus fertilizer influenced rhizosphere soil characteristics, nodule formation and phytoconstituents of cowpea. Heliyon 6 (10). doi: 10.1016/j.heliyon.2020.e05255
Qian, S., Zhou, X., Fu, Y., Song, B., Yan, H., Chen, Z., et al (2023). Biochar-compost as a new option for soil improvement: Application in various problem soils. Science of The Total Environment 870, 162024. doi: 10.1016/j.scitotenv.2023.162024
Qin, W., Wang, D., Guo, X., Yang, T., Oenema, O. (2015). Productivity and sustainability of rainfed wheat-soybean system in the North China Plain: results from a long-term experiment and crop modelling. Sci. Rep. 5 (1), 17514. doi: 10.1038/srep17514
Reibe, K., Götz, K. P., Döring, T. F., Roß, C. L., Ellmer, F. (2015). Impact of hydro-/biochars on root morphology of spring wheat. Arch. Agron. Soil Sci. 61 (8), 1041–1054. doi: 10.1080/03650340.2014.983090
Schroder, J. L., Zhang, H., Girma, K., Raun, W. R., Penn, C. J., Payton, M. E. (2011). Soil acidification from long-term use of nitrogen fertilizers on winter wheat. Soil Sci. Soc. America J. 75 (3), 957–964. doi: 10.2136/sssaj2010.0187
Shi, W., Bian, R., Li, L., Lian, W., Liu, X., Zheng, J., et al. (2022). Assessing the impacts of biochar‐blended urea on nitrogen use efficiency and soil retention in wheat production. GCB Bioenergy 14 (1), 65–83. doi: 10.1111/gcbb.12904
Si, Z., Zain, M., Mehmood, F., Wang, G., Gao, Y., Duan, A. (2020). Effects of nitrogen application rate and irrigation regime on growth, yield, and water-nitrogen use efficiency of drip-irrigated winter wheat in the North China Plain. Agric. Water Manage. 231, 106002. doi: 10.1016/j.agwat.2020.106002
Tahir, N., Li, J., Ma, Y., Ullah, A., Zhu, P., Peng, C., et al. (2021). 20 Years nitrogen dynamics study by using APSIM nitrogen model simulation for sustainable management in Jilin China. Sci. Rep. 11 (1), 1–21. doi: 10.1038/s41598-021-96386-5
Taylor, R. D., Koo, W. W. (2013). 2013 Outlook of the US and World Corn and Soybean Industries, 2012-2022 (No. 1187-2016-93647). North Dakota State University, doi: 10.22004/ag.econ.157670
Tian, X., Li, Z., Liu, Z., Wang, Y., Li, B., Zhang, K., et al. (2022). Combined effect of biochar and nitrogen fertilizer reduction on rapeseed productivity and nitrogen use efficiency. Arch. Agron. Soil Sci. 68(9), 1159–1174. doi: 10.1080/03650340.2021.1872782
Ullah, S., Liang, H., Ali, I., Zhao, Q., Iqbal, A., Wei, S., et al (2020). Biochar coupled with contrasting nitrogen sources mediated changes in carbon and nitrogen pools, microbial and enzymatic activity in paddy soil. Journal of Saudi Chemical Society 24(11), 835–849. doi: 10.1016/j.jscs.2020.08.008
Ullah, S., Zhao, Q., Wu, K., Ali, I., Liang, H., Iqbal, A., et al (2021). Biochar application to rice with 15N-labelled fertilizers, enhanced leaf nitrogen concentration and assimilation by improving morpho-physiological traits and soil quality. Saudi J. Biol. Sci. 28(6), 3399–3413. doi: 10.1016/j.sjbs.2021.03.003
Uzoma, K. C., Inoue, M., Andry, H., Fujimaki, H., Zahoor, A., Nishihara, E. (2011). Effect of cow manure biochar on maize productivity under sandy soil condition. Soil Use Manage. 27 (2), 205–212. doi: 10.1111/J.1475-2743.2011.00340.X
Wu, W., Han, J., Gu, Y., Li, T., Xu, X., Jiang, Y., et al. (2022). Impact of biochar amendment on soil hydrological properties and crop water use efficiency: A global meta-analysis and structural equation model. GCB Bioenergy 14 (6), 657–668. doi: 10.1111/GCBB.12933
Wu, Q., Lian, R., Bai, M., Bao, J., Liu, Y., Li, S., et al. (2021). Biochar co-application mitigated the stimulation of organic amendments on soil respiration by decreasing microbial activities in an infertile soil. Biol. Fertil. Soils 57 (6), 793–807. doi: 10.1007/S00374-021-01574-0
Xia, H., Riaz, M., Zhang, M., Liu, B., El-Desouki, Z., Jiang, C. (2020). Biochar increases nitrogen use efficiency of maize by relieving aluminum toxicity and improving soil quality in acidic soil. Ecotoxicol. Environ. Saf. 196, 110531. doi: 10.1016/j.ecoenv.2020.110531
Xu, P., Gao, Y., Cui, Z., Wu, B., Yan, B., Wang, Y, et al (2013). Research progress on effects of biochar on soil environment and crop nutrient absorption and utilization. Sustainability 15(6), 4861. doi: 10.3390/su15064861
Yang, W., Feng, G., Jia, Y., Yang, Y., Gao, X., Gao, L., et al (2022). Impact of single biochar application on maize growth and water-fertilizer productivity under different irrigation regimes. Front. Plant Sci. 13, 1006827. doi: 10.3389/fpls.2022.1006827
Yang, Q., Liu, P., Dong, S., Zhang, J., Zhao, B. (2020). Combined application of organic and inorganic fertilizers mitigates ammonia and nitrous oxide emissions in a maize field. Nutr. Cycl. Agroecosyst. 117 (1), 13–27. doi: 10.1007/S10705-020-10060-2
Yue, K., Li, L., Xie, J., Fudjoe, S., Zhang, R., Luo, Z., et al. (2021). Nitrogen supply affects grain yield by regulating antioxidant enzyme activity and photosynthetic capacity of maize plant in the loess plateau. Agronomy. 11(6), 1094. doi: 10.3390/agronomy11061094
Zhang, Z., Tao, F., Du, J., Shi, P., Yu, D., Meng, Y., et al. (2010). Surface water quality and its control in a river with intensive human impacts–a case study of the Xiangjiang River, China. J. Environ. Manage. 91 (12), 2483–2490. doi: 10.1016/J.JENVMAN.2010.07.002
Zhang, S., Wei, Y., Kandhro, M. N., Wu, F. (2020). Evaluation of FDR MI2X and New WiTu Technology sensors to determine soil water content in the corn and weed field. AIMS Agric. Food 5 (1), 169–180. doi: 10.3934/AGRFOOD.2020.1.169
Zhao, D., Wang, Z., Yang, F., Zhu, W., An, F., Ma, H., et al. (2020). Amendments to saline-sodic soils showed long-term effects on improving growth and yield of rice (Oryza sativa L.). PeerJ 8, e8726. doi: 10.7717/peerj.8726
Keywords: maize, biochar, water and nitrogen dynamics, border irrigation, drip irrigation
Citation: Wang L, Leghari SJ, Wu J, Wang N, Pang M and Jin L (2023) Interactive effects of biochar and chemical fertilizer on water and nitrogen dynamics, soil properties and maize yield under different irrigation methods. Front. Plant Sci. 14:1230023. doi: 10.3389/fpls.2023.1230023
Received: 27 May 2023; Accepted: 16 August 2023;
Published: 06 September 2023.
Edited by:
Haishun Yang, University of Nebraska-Lincoln, United StatesReviewed by:
Ju Min, Chinese Academy of Sciences (CAS), ChinaHaijun Sun, Nanjing Forestry University, China
Copyright © 2023 Wang, Leghari, Wu, Wang, Pang and Jin. This is an open-access article distributed under the terms of the Creative Commons Attribution License (CC BY). The use, distribution or reproduction in other forums is permitted, provided the original author(s) and the copyright owner(s) are credited and that the original publication in this journal is cited, in accordance with accepted academic practice. No use, distribution or reproduction is permitted which does not comply with these terms.
*Correspondence: Liang Jin, amlubGlhbmcxOTc2MjAwM0BhbGl5dW4uY29t
†These authors have contributed equally to this work