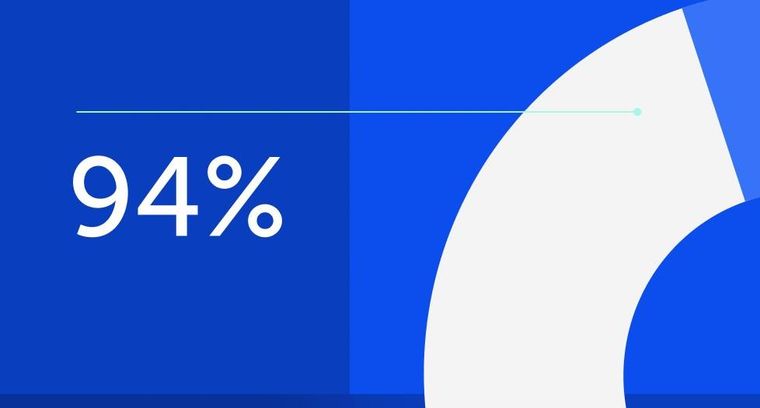
94% of researchers rate our articles as excellent or good
Learn more about the work of our research integrity team to safeguard the quality of each article we publish.
Find out more
ORIGINAL RESEARCH article
Front. Plant Sci., 06 September 2023
Sec. Plant Physiology
Volume 14 - 2023 | https://doi.org/10.3389/fpls.2023.1227492
This article is part of the Research TopicPhotosynthetic Regulation and Carbon/Nitrogen Metabolism in Genetically Engineered AlgaeView all 5 articles
All known photosynthetic cyanobacteria carry a cytochrome c6 protein that acts transferring electrons from cytochrome b6f complex to photosystem I, in photosynthesis, or cytochrome c oxidase, in respiration. In most of the cyanobacteria, at least one homologue to cytochrome c6 is found, the so-called cytochrome c6B or cytochrome c6C. However, the function of these cytochrome c6-like proteins is still unknown. Recently, it has been proposed a common origin of these proteins as well as the reclassification of the cytochrome c6C group as c6B, renaming the new joint group as cytochrome c6BC. Another homologue to cytochrome c6 has not been classified yet, the formerly called cytochrome c6-3, which is present in the heterocyst-forming filamentous cyanobacteria Nostoc sp. PCC 7119. In this work, we propose the inclusion of this group as an independent group in the genealogy of cytochrome c6-like proteins with significant differences from cytochrome c6 and cytochrome c6BC, with the proposed name cytochrome c6D. To support this proposal, new data about phylogeny, genome localisation and functional properties of cytochrome c6-like proteins is provided. Also, we have analysed the interaction of cytochrome c6-like proteins with cytochrome f by isothermal titration calorimetry and by molecular docking, concluding that c6-like proteins could interact with cytochrome b6f complex in a similar fashion as cytochrome c6. Finally, we have analysed the reactivity of cytochrome c6-like proteins with membranes enriched in terminal oxidases of cyanobacteria by oxygen uptake experiments, concluding that cytochrome c6D is able to react with the specific copper-oxidase of the heterocysts, the cytochrome c oxidase 2.
Oxygenic photosynthesis is the major biochemical reaction that facilitates life on earth (Falkowski and Isozaki, 2008). Cyanobacteria constitute a broad group of Gram-negative prokaryotes with the ability to perform oxygenic photosynthesis in a similar fashion as higher plants (Stanier and Cohen-Bazire, 1977; Woese, 1987). They are considered the main organisms responsible for the ‘Great Oxidation Event’, transforming the atmosphere from a primordial reduced state (with no O2 available) to the oxidative atmosphere (with free O2) that we have in the present day (Lyons et al., 2014). These photosynthetic organisms live in a wide variety of environments, such as freshwater or marine ecosystems, and play a crucial role on primary biomass production, carbon, and nitrogen cycle (Fuchsman et al., 2019).
In cyanobacteria, both photosynthetic and respiratory electron transport chains are present in the same membrane systems (Figure 1), sharing some key elements, such as cytochrome (Cyt) b6f complex and soluble electron carriers plastocyanin (Pc) and Cyt c6 (Mullineaux, 2014). Both soluble electron carriers are present in a great number of cyanobacteria and green algae and can perform the same function, oxidising Cyt f and donating electrons to photosystem (PS) I, in photosynthesis, or to Cyt c oxidase (COX), in respiration (Hervás et al., 2003). However, throughout evolution Pc has replaced Cyt c6, and therefore plants only produce Pc, and, in some cases, a cryptic version of Cyt c6, Cyt c6A, the function of which is still unknown (Molina-Heredia et al., 2003; Worrall et al., 2008). Cyt c6 is present in all sequenced cyanobacteria (Ki, 2005). Besides, most of the cyanobacteria also contain other homologues to Cyt c6 (Ki, 2005; Bialek et al., 2008). These Cyt c6-like proteins have been classified as Cyt c6B or Cyt c6C, according to their resemblance to Cyt c6A from plants or Cyt c6 from cyanobacteria, respectively (Bialek et al., 2008). In a recent study, Slater and collaborators (2021) proposed the common origin of both Cyt c6B and Cyt c6C, being Cyt c6B a paralogous of Cyt c6 and Cyt c6C an orthologue of Cyt c6B, with the suggested name of Cyt c6BC. However, this study does not cover the presence of a third Cyt c6-like protein that is homologous to Cyt c6, the so-called Cyt c6-3 (Torrado et al., 2017). In that study, the newly discovered Cyt c6-like protein was characterised, and it was found that its redox potential was closer to Cyt c6 (+300 mV). Members of the Cyt c6BC branch have shown to have a less positive redox potential (around +150-200 mV), which will make them unable to physiologically oxidise Cyt f, the main electron donor to Cyt c6 (Molina-Heredia et al., 2003; Bialek et al., 2008; Reyes-Sosa et al., 2011; Bialek et al., 2014). Furthermore, the specific residue in the position 61, which is a tyrosine conserved in Cyt c6BC branch, was not conserved neither in Cyt c6 nor in Cyt c6-3, which discarded Cyt c6-3 as a possible member of Cyt c6BC group. The present study expands the current understanding of the Cyt c6-like proteins by reconciling the recently discovered Cyt c6-3, with the proposed name of Cyt c6D. We support our statement with novel physiological data of these Cyt c6-like proteins in photosynthesis and respiration and shed light on the possible function of these proteins homologous to Cyt c6.
Figure 1 Schematic diagram of the photosynthetic and respiratory electron transport chain of cyanobacteria. Cytochrome b6f complex (Cyt b6f) and the redox transporters plastoquinone (PQ), plastocyanin (Pc) and Cytochrome c6 (Cyt c6) are shared by both photosynthesis and respiration. NADH dehydrogenase (NDH); Photosystem II (PSII); Photosystem I (PSI); Cytochrome c oxidase (COX); Cytochrome c6B (Cyt c6BC).
Nostoc sp. PCC 7119 (ATCC 29151) (Adolph and Haselkorn, 1971) was grown in BG11 (Rippka et al., 1979) (containing NaNO3 as N source) or BG110 (lacking any source of combined nitrogen) medium at 30 °C under standard light conditions (25 μmol photons m-2·s-1 from led white lamps). Cultures were maintained in an orbital shaker (100 rpm) during liquid experiments or in solidified medium (1% w/v Difco Agar). For selection of mutant strains, Streptomycin and Spectinomycin antibiotics were added to the media at a final concentration of 5 μg·mL-1 each as described previously (Torrado et al., 2019). Heterocyst formation was induced by transferring cultures grown in BG11 to BG110, after centrifugation at 3000 ×g and washing of the cultures with BG110.
Cyt c6, c6BC, c6D and f were expressed and purified as described previously (Molina-Heredia et al., 1998; Molina-Heredia et al., 1999; Molina-Heredia et al., 2001; Albarrán et al., 2005; Reyes-Sosa et al., 2011; Torrado et al., 2017). In short, Escherichia coli strains were co-transformed with the plasmids bearing each Cyt and the support plasmid pEC86, which encodes the E. coli genes required for Cyt c maturation (Arslan et al., 1998), in LB medium supplemented with the corresponding antibiotic at 37 °C during 24 h with continuous shaking (300 rpm). After the incubation, proteins were extracted from the periplasmic fraction after three freeze-thawing cycles and subsequent purification in an ion exchange chromatography column. The fractions containing Cyt were followed and analysed spectrophotometrically and in case that the purity was inadequate, another step of chromatography was applied. The A280/A55X absorbance ratio was used to estimate the purity of the sample, whereas Cyt c6, c6BC, c6D and f had a maximum absorption peak of the reduced α-band of 553, 553, 552 and 556, respectively. Isolated heterocysts were purified as described in Torrado et al., 2019. Vegetative and heterocyst membranes enriched in terminal oxidases were purified as described previously (Schmetterer et al., 2001; Torrado et al., 2019). In short, isolated membranes from Nostoc sp. PCC 7119 were prepared from 800 mL of cultures at a cellular density of 3-6 µg of Chl a·mL-1. Cells were centrifuged at 12,000 ×g for 5 minutes, resuspended in 10 ml HEPES buffer (10 mM HEPES pH 7.4 and 6 mM NaCl) with supplementation of 20% sucrose (w/v) and 10 mg of lysozyme. The suspension was incubated 30 minutes at 37°C and centrifuged at 12,000 ×g for 5 minutes. The pellet was resuspended in 10 mL of ice-cold HEPES buffer and incubated on ice for 1 hour. The suspension was supplemented with 1 mM phenylmethylsulfonyl fluoride and 0.005% (w/v) DNase I and passed through a French press three times at 11,000 psi. After this, the suspension was centrifuged at 4°C at 12,000 ×g for 10 minutes, resuspended in 5 mL of ice-cold HEPES buffer and homogenizer in a Potter, to a final concentration of 3-5 mg·mL-1 of total protein.
Cyt samples for isothermal titration calorimetry (ITC) were oxidised (Cyt c6, c6BC and c6D) or reduced (Cyt f) with 10 µM potassium ferrocyanide or 5 mM sodium ascorbate, respectively. Subsequently, samples were extensively dialysed with 5 mM of phosphate buffer (pH 7.0) to remove the oxidising/reducing agents. ITC experiments were carried out in 5 mM phosphate buffer, pH 7.5, supplemented with 0.02% (w/v) Triton X-100, using an Auto-iTC200 instrument (MicroCal, Malvern-Panalytical) at 25 °C and a stirring speed of 1000 rpm. The reference cell was filled with distilled water. The experiments were carried on through successive additions (2 μL injections) of concentrated Cyts c6, c6BC and c6D proteins (400 µM) to the sample cell containing the binding partner Cyt f (40 µM). All solutions were degassed before titrations. Titrant was injected at appropriate time intervals to ensure that the thermal power signal returned to the baseline prior to the next injection. Control experiments of the dilution of individual injected proteins were conducted and these reference heat values were subtracted from measuring values of test titrations when appropriate. The binding isotherm (ligand-injected normalized heat per injection as a function of the molar ratio) was analysed with Origin 7 (OriginLab). In all cases the heat evolved during titrations could be well fitted to a 1:1 binding stoichiometry, and the association constant, KA (and the dissociation constant, KD), and the binding enthalpy (ΔH) and entropy (ΔS) values for the interaction process were estimated (Velázquez-Campoy et al., 2004). Estimated errors in the determined values were 15% for the equilibrium constants, 5% for the binding enthalpy and entropy, and 2% for the binding Gibbs energy.
The ClusPro online server (Comeau et al., 2004, https://cluspro.bu.edu/queue.php) was used to perform the protein-protein docking. Model zero was selected out of the top ten models in the balanced order. The structural model was created and visualised using Chimera 1.11 (Pettersen et al., 2004) with colours applied to label the two proteins and their heme groups to facilitate visualization and interpretation.
Multiple sequence alignment was built using ClustalW using 152, 149 and 86 sequences identified as Cyt c6, Cyt c6BC or Cyt c6D, respectively (Supplementary Table 1). Sequence identification was performed using the CyanoOmicsDB database (Zhou et al., 2021). Genome comparison analysis performed using the bioinformatics tools available on the online platform Biocyc (Karp et al., 2019; Karp et al., 2020). Phylogenetic tree was constructed by maximum likelihood method with Geneious version 2023.0 created by Biomatters (https://www.geneious.com), inferred using a neighbour-joining algorithm and Jukes-Cantor as the genetic distance model, using the Bootstrap (n = 100) method (Felsenstein, 1985).
Rates of respiration were assessed by measuring the consumption of O2 over time in an Oxygraph O2 electrode (Hansatech, Cambridge, UK) in a double-jacket thermoregulated glass vessel as described previously (Torrado et al., 2019). The reaction mixture contained, in a final volume of 1 mL, 5 mM of HEPES buffer (pH 7.5), 2.5 mM of NaCl, 2 mM of sodium ascorbate, COX-enriched membranes equivalent to 3-5 mg·mL-1 of total protein and 20 µM of Cyt c6, Cyt c6B or Cyt c6D.
The phylogeny of Cyt c6-like proteins has been the subject of study for the past 15 years (Bialek et al., 2008; Reyes-Sosa et al., 2011; Bialek et al., 2014; Howe et al., 2016; Torrado et al., 2016; Torrado et al., 2017; Slater et al., 2021). Bialek et al. (2008) performed a comparative study of the sequence of Cyt c6 and Cyt c6-like proteins, concluding that Cyt c6-like proteins constitutes a new branch separated from Cyt c6. In addition, they divided them into two groups which they called Cyt c6B and Cyt c6C based on a phylogenetic analysis. A new analysis performed by Slater et al. (2021) including a higher number of sequences showed that the distinction between Cyt c6B and Cyt c6C can occur by taxon sampling rather than by differences in function. The similarity of crystal structures, surface electrostatic potential distribution, and midpoint redox potentials of proteins from both B and C subgroups, points to the fact that Cyt c6B and Cyt c6C could perform a similar function and are, probably, orthologs (Zatwarnicki et al., 2014; Slater et al., 2021). However, a group of Cyt c6 homologous that was first found in heterocyst-forming filamentous cyanobacteria, named as Cyt c6-3 (Torrado et al., 2017), has not been integrated in the current phylogeny. We are aware that the nomenclature of these Cyt c6-like proteins can result confusing, but because the function of these Cyt c6-like proteins is not elucidated, a clear nomenclature cannot be established. For now, we propose to keep the former nomenclature, whereas the first Cyt c6-like protein found in cyanobacteria will be Cyt c6BC and the second one, the formerly called Cyt c6-3 which only appears in filamentous cyanobacteria, Cyt c6D.
To investigate the distribution of Cyt c6 of cyanobacteria and to include the presence of this new group, we mapped a phylogenetic tree of cyanobacterial sequences of Cyt c6-like proteins including the new group of Cyt c6D (Figure 2A). The phylogenetic analysis was based on 387 sequences of Cyt c6, Cyt c6BC and Cyt c6D found in cyanobacteria from public databases. The sequences were aligned using ClustalW method and a phylogenetic tree was inferred using maximum-likelihood method (see Material and Methods). The results (Figure 2A) showed that the newly classified Cyt c6D forms an independent group clearly separated from Cyt c6 or Cyt c6BC. In the previous publication in which Cyt c6D was described (Torrado et al., 2017), where the genomes and tools were more limited, Cyt c6D was found only in heterocyst-forming filamentous cyanobacteria. A more comprehensive study involving a higher number of Cyt c6 protein sequences has revealed that Cyt c6D is present in all type of filamentous cyanobacteria, independently of the heterocyst formation, but it is not present in unicellular cyanobacteria. Besides, this sequence analysis has revealed a conserved sequence pattern that is present in all Cyt c6D (Figure 2B). The conserved pattern has been identified as L-X-X-Y and starts at position 40 of the consensus sequence (most common amino acid at that position; Liljas, 2013) of Cyt c6D. Also, in Cyt c6 and in Pc appears a single arginine residue at position 64 in Cyt c6 (Figure 2B) that is strictly conserved in both proteins and results crucial for their interaction with PS I (Molina-Heredia et al., 2001). This residue is also strictly conserved in the position 64 in Cyt c6BC, but it has been replaced by lysine (position 68, black triangle in Figure 2B) in Cyt c6D. In Nostoc, we have previously reported that Cyt c6BC can react with PS I, but in a less efficient manner than Cyt c6. (Reyes-Sosa et al., 2011). However, Cyt c6D does not react with PS I (Torrado et al., 2017).
Figure 2 Sequence analysis of 387 sequences identified as Cyt c6, Cyt c6BC or Cyt c6D. (A) Phylogenetic tree of soluble cytochromes described in cyanobacteria using Neighbour-Joining clustering method. In boxes, clustered sequences from Cyt c6 (Green), Cyt c6BC (Blue) and Cyt c6D group (Red). Dashed lines inside Cyt c6BC group highlight the formerly classified Cyt c6B proteins subgroup, while the rest of the sequences of that group were classified as Cyt c6C proteins subgroup. (B) Consensus sequence of Cyt c6, Cyt c6BC and Cyt c6D, using 152, 149 and 86 sequences of each protein, respectively, using ClustalW alignment for each group of sequences. Black dots point to the two key residues conserved only in Cyt c6D group. Black triangle points to the conserved arginine crucial to the interaction with PS I in Cyt c6 and Cyt c6BC. Sequences are numbered according to the longest consensus sequence which is Cyt c6D. The bar represents 0.2 substitutions per site.
An interesting feature found in this analysis was the position and distribution of Cyt c6D within cyanobacteria. We analysed the genomic context of the gene encoding Cyt c6D in filamentous cyanobacteria, finding that the position and distribution of the gene in the genome seems to follow a specific pattern in most of the studied organisms (Figure 3, Supplementary Table 1). Gene encoding Cyt c6D is located in a conserved region, whereas the genes psbV (Cyt c550), petE (Pc) and petJ-3 (Cyt c6D) are located in the same order and direction of the expression (Figure 3), in contraposition to the positions of petJ (Cyt c6) or petJ-2 (Cyt c6B) which are unevenly distributed (Supplementary Figure 1). Cyt c550 is a component of the PS II complex in cyanobacteria and some eukaryotic algae, such as red and brown algae (Roncel et al., 2012), and Pc acts as soluble electron carrier between Cyt b6f complex and PS I. The fact that the gene that codes for Cyt c6D is in the same cluster as the genes that code for Cyt c550 and Pc may lead us to think that Cyt c6D function is related to the photosynthetic electron transport chain. However, this function should be different from Cyt c6 and Pc, because as we mentioned before, Cyt c6D is not able to reduce PS I (Torrado et al., 2017). Another gene is frequently found downstream of Cyt c6D, a hypothetical protein with partial similarity to TonB (BlastP), but no further information is provided in this study (Figure 3).
Figure 3 Genome localisation of Cyt c6D in filamentous cyanobacteria. In red, petJ-3 (Cyt c6D); in green, petE (Plastocyanin); in pale blue, psbV (Cyt c550); in dark blue, hypothetical protein conserved upstream petJ-3.
We investigated whether Cyt c6BC and Cyt c6D can interact with the subunit Cyt f of Cyt b6f complex. One of the key factors for this interaction is the redox potential of the Cyts. As we discussed before, the redox potential of Cyt c6BC (+199 mV) and Cyt c6D (+300 mV) is lower than that of Cyt c6 (+335 mV), which at first glance indicates that this interaction is unlikely to happen (Molina-Heredia et al., 1998; Reyes-Sosa et al., 2011; Torrado et al., 2017). However, in our previous study (Torrado et al., 2017), we analysed the redox potential under the physiological condition of thylakoidal lumen of pH 4, instead of the standard pH 7. We found that the redox potential of Cyt c6D (+343 mV) became isopotential with Cyt c6 (+340 mV) under that condition. Still, Cyt c6BC (+230 mV) was found to be far from the redox potential of the other Cyts. However, we could not measure the empirical redox potential of soluble Cyt f in vitro at pH 4, as this protein was denatured and degraded at that pH. We explain this phenomenon because physiologically, Cyt f should be embedded within Cyt b6f membrane complex, but in the soluble form heterologously expressed, the integrity of this protein cannot withstand the acidic pH. In summary, with the redox potential found under those conditions, Cyt c6D would be able to oxidise Cyt f while Cyt c6BC will be unable to do it. However, the redox potential is not a sufficient condition to demonstrate that the interaction can happen between the proteins, since it only establishes the direction of the electron transfer in case they would interact. To evaluate if the proteins can interact in vitro, we performed Isothermal Titration Calorimetry (ITC) experiments and protein-protein docking analysis.
For ITC experiments we prepared purified samples of each soluble Cyts in an oxidised state, and samples with Cyt f completely reduced to facilitate the interaction, at 25 °C. In all the cases, we observed interaction between the proteins, with a 1:1 binding stoichiometry (Figure 4A). The analysis of the binding isotherms, according to a ligand-binding model with a single binding site, allowed us to determine the thermodynamic interaction parameters (Figure 4B). Both, KA and KD for the interaction of Cyt c6BC with Cyt f are similar to those obtained with Cyt c6. However, KA and KD for the interaction of Cyt c6D with Cyt f are 6-fold higher and lower, respectively, than those obtained with both Cyt c6 and Cyt c6BC. These results show that Cyt c6D has a higher affinity for Cyt f than Cyt c6. Looking at the thermodynamic parameters, although the ΔG values of the interaction of the three Cyts are somewhat similar, the values of ΔH and –TΔS indicate that the three interactions are quite different. In the case of Cyt c6, whose isoelectric point (pI) is 9.0 (Molina-Heredia et al., 1998), both ΔH and –TΔS are negative, indicating that the interaction with Cyt f might be driven by attractive electrostatic interactions and by hydrophobic interactions. This agrees with the fact that Cyt c6 presents electrostatic and hydrophobic regions interacting with both PS I and Cyt f (Molina-Heredia et al., 1999; Crowley et al., 2002; Albarrán et al., 2005). In the case of Cyt c6BC, ΔH and –TΔS are large and of opposite sign, cancelling partially each other. These values indicate that, although an electrostatic repulsion may occur, the interaction is exergonic and might be driven primarily by hydrophobic interactions. This is in agreement with the data previously reported (Reyes-Sosa et al., 2011) that described the conserved hydrophobic interaction surface with Cyt f in Cyt c6BC; but not the surface of electrostatic interaction, being positive in Cyt c6, but negative in Cyt c6BC, even though it has a pI of 8.0, very close to that of Cyt c6. Likewise, in the case of Cyt c6D, whose pI is 5.2 (Torrado et al., 2017), the interaction with Cyt f is driven by hydrophobic forces, not presenting attractive electrostatic interactions. As in the case of Cyt c6BC, Cyt c6D preserves the hydrophobic surface for the interaction with Cyt f; however, the region for the electrostatic interaction that is present in Cyt c6 results practically neutral in Cyt c6D (Torrado et al., 2017).
Figure 4 Calorimetric titrations of Cyt c6, Cyt c6BC and Cyt c6D corresponding to the formation of the complex with Cyt f. (A) Thermograms (thermal power as a function of time, upper plots) and binding isotherms (ligand-normalised heat effects per injection as a function of the molar ratio, lower plots). The continuous thin lines correspond to the fits according to the single binding site model. (B) Thermodynamic binding parameters for the complexes. KA: association constant; ΔH: binding enthalpy; ΔG: binding Gibbs energy; –TΔS: binding entropic contribution; n: binding stoichiometry; KD: dissociation constant. Each experiment was performed with three independent replicates.
Besides the fact that the interaction can happen between Cyt c6 paralogous proteins and Cyt f, one of the key factors for a redox interaction involving cytochromes lays in the orientation of their heme groups (Cruz-Gallardo et al., 2012). The interaction may be happening but the heme groups might be so distant that the electron transfer would not be possible. To assess such orientation along the interaction, we performed a structural modelling by molecular docking of the soluble Cyts with Cyt f (Figure 5). As a control, we used Cyt c6, considering that its interaction and orientation with Cyt f has been previously reported (Díaz-Moreno et al., 2005). The molecular docking was conducted using the Cluspro V.2.0 server, selecting the equilibrated models with minimum energy. According to these models, Cyt c6BC and Cyt c6D (Figures 5B, C) will interact with Cyt f through the same hydrophobic area as Cyt c6 (Figure 5A). Further, their heme groups will be oriented opposed to the Cyt f heme group, as it happens as well with Cyt c6. In conclusion, Cyt c6BC and Cyt c6D could interact with Cyt f in the same areas, with the same orientation as Cyt c6, and with a distance between the redox centres similar to that of Cyt c6, which would allow an electron transfer between them.
Figure 5 Molecular docking of the interaction between of the soluble Cyt c6-like proteins and Cyt f. The molecular interaction of Cyt f with (A) Cyt c6, (B) Cyt c6BC and (C) Cyt c6D is represented, with the heme groups aligned. Cyt f from Cyt b6f complex is shown in blue; soluble Cyts are shown in red; heme groups are shown in yellow, with the characteristic iron in orange. The equilibrated models of minimum energy were selected for each case, using the online server Cluspro V.2.0. The PDB data with the structures used can be found in the supplementary data.
One of the key questions yet to be addressed is the role of these Cyt c6-like proteins in respiration. As we described before, Cyt c6 and Pc are the main interaction partners with terminal oxidases in respiration. However, little information has been provided about the interaction of Cyt c6-like proteins in respiration (Torrado et al., 2016). Nostoc sp. PCC 7119 is an heterocyst forming filamentous cyanobacterium that can express two different set of aa3-type copper oxidases. One of these oxidases is expressed in vegetative cells and is the main respiratory oxidase, also called COX1 (Valladares et al., 2014). In heterocyst, which are differentiated cells under nitrogen-deficient conditions, COX2, the alternative aa3-type oxidase, is expressed. Thus, under nitrogen-deficient conditions where vegetative cells and heterocysts cohabit in the filament, COX1 is expressed only in vegetative cells and COX2 is expressed only in heterocysts. As we described before, Cyt c6D gene is only found in filamentous cyanobacteria, including heterocyst-forming cyanobacteria. Hence, our approach comprised the study of both terminal oxidases, with particular interest on the heterocyst-specific COX2 (Valladares et al., 2014). To address this question, we performed O2 uptake experiments with membranes enriched in terminal oxidases in the dark (Figure 6), using an oxygen electrode (Oxygraph, Hansatech). First, we evaluated the reactivity with the main oxidase that is expressed only in vegetative cells, COX1. In this condition, membranes enriched in COX1 were isolated and assessed against the three Cyts. As expected, Cyt c6 reacts with these membranes producing a high rate of oxygen consumption. However, neither Cyt c6BC or Cyt c6D produced any significant increase in oxygen consumption, indicating no reaction with COX1. Next, we purified heterocysts, and from these preparations, we isolated membranes enriched in COX2. In this condition, we found that Cyt c6 behaved as described previously, reacting with COX2 and producing an elevated oxygen consumption (Torrado et al., 2019). However, Cyt c6BC was not able to react with these membranes enriched in COX2, observing only an unspecific oxygen consumption, at the level of a non-native Cyt c. Surprisingly, Cyt c6D was able to react with membranes enriched in COX2 with a similar rate of oxygen consumption as Cyt c6. Thus, contrary to Cyt c6, Cyt c6D reacts specifically with COX2.
Figure 6 Oxygen uptake rate of Cyt c6, Cyt c6BC and Cyt c6D with isolated membranes enriched in terminal oxidases of Nostoc sp. PCC 7119. Rates of oxygen consumption were measured in the presence of Cyt c6 (black), Cyt c6BC (grey), Cyt c6D (white) and Cyt c from horse (striped) in either vegetative or heterocyst cell membranes. Oxygen consumption rate was calculated per mg of membrane protein of the sample. Cyt c was used as a control for unspecific interaction of a Cyt c-type protein. Vegetative membranes: membranes obtained from vegetative cells, cultured in continuous light and with combined nitrogen. Heterocyst membranes: membranes obtained from isolated heterocyst, cultured in continuous light and without combined nitrogen. Plotted bars are the average from three independent replicates; error bars represent standard deviations from the mean.
These results could lead us to think that Cyt c6D may transport electrons from Cyt b6f complex to the COX2, specific to heterocysts. However, previous in vivo GFP report of the Cyt c6D promoter activity experiments showed that in the presence of combined nitrogen, Cyt c6D is homogeneously expressed in all the cells of the filament, and that under nitrogen starvation, the expression of Cyt c6D was partially repressed in vegetative cells, but such repression was practically complete in heterocysts (Torrado et al., 2017).
In summary, the present work expands the current knowledge of Cyt c6-like proteins by the inclusion in the phylogeny of the recently discovered group Cyt c6-3, renamed in this work as Cyt c6D. We have provided information about the differences between Cyt c6BC and Cyt c6D, being two clearly independent groups of Cyt c6-like proteins (Figure 2A). Also, Cyt c6D seems to follow a distribution pattern along the genome of filamentous cyanobacteria that is well-conserved and should be further investigated (Figure 3). The differences between these Cyts are not only at sequence level, but also at their physico-chemical properties. The redox potential of both proteins has proved to be significantly different, being Cyt c6D isopotential with Cyt c6 at physiological pH conditions, while Cyt c6BC is a less positive redox potential protein in comparison (Torrado et al., 2017). A closer look at their interaction with the Cyt c6 partner Cyt f, both were found to be able to interact with it at protein-protein level (Figure 4). Further, the molecular docking revealed that the binding location and orientation of both proteins were adequate for the electron transfer (Figure 5). However, as we have stated before, the low redox potential of Cyt c6BC will make the electron transfer from Cyt f unlikely, from a thermodynamic point of view, (Reyes-Sosa et al., 2011). As the PS I interaction with both proteins has been already tested (Reyes-Sosa et al., 2011; Torrado et al., 2017), we decided to investigate the interaction of these proteins in the respiratory electron transport chain (Figure 6). In these experiments, Cyt c6BC was proven to not react specifically with either both terminal oxidases of the cyanobacterium. However, Cyt c6D could react specifically, with a similar kinetics as Cyt c6, with the oxidase specific from heterocyst, COX2. These findings open the door to a functional differentiation of Cyt c6-like proteins (Figure 7). A possible function of Cyt c6BC could be to connect another redox process with photosynthetic and respiratory electron transport chains, introducing the electrons at the level of Cyt f and PS I. On the other hand, Cyt c6D appears only in filamentous cyanobacteria. Interestingly, when it is present in the genome, the gene that codes for it is always found in the same cluster as the one that codes for Pc and for Cyt c550, both of which participate in photosynthesis. However, Cyt c6D is not able to react with either PS I or COX1, the specific oxidase of vegetative cells. Although it does efficiently reduce COX2, which is the heterocyst-specific oxidase, its expression is repressed in heterocysts in the standard culture conditions tested (Torrado et al., 2017). However, we cannot rule out that Cyt c6D expression could induced under stress conditions or under other culture conditions. Besides, Cyt c6D could be capable of interacting with Cyt f, showing a higher affinity than Cyt c6. These results make us think that Cyt c6D could be involved in the fine-tuning regulation of electron transport in the vegetative cells of filamentous cyanobacteria, at the level of Cyt f.
Figure 7 Proposed model of the Cyt c6-like proteins in photosynthetic and respiratory electron transport chain of heterocyst-forming cyanobacteria. Dashed lines represent the new findings of this study. Cytochrome b6f complex (Cyt b6f) and the redox transporters plastoquinone (PQ), plastocyanin (Pc) and Cytochrome c6 (Cyt c6) are shared by both photosynthesis and respiration. NADH dehydrogenase (NDH); Photosystem II (PSII); Photosystem I (PSI); Cytochrome c oxidase 1 specific of vegetative cells (COX1); Cytochrome c oxidase 2 specific of heterocysts (COX2); Cytochrome c6B (Cyt c6BC); Cytochrome c6D (Cyt c6D).
The original contributions presented in the study are included in the article/Supplementary Files. Further inquiries can be directed to the corresponding authors.
AT and FM-H conceived the project. AT, MI-P, AV-C, and FM-H carried out the experiments. AT, VM, CA, and FM-H interpreted the data and discussed the results. AT, VM, and FM-H wrote the manuscript, which was corrected, revised, and approved by all authors.
This work has been supported by the Fundación de Investigación de la Universidad de Sevilla (FIUS, Spain) (grant FIUS05710000), by NextGenerationEU ‘María Zambrano’ grant of the Universidad de Sevilla (MZAMBRANO-2021-20002), by the Andalusian Government (PAIDI AGR-288) and by Consejo Superior de Investigaciones Científicas (CSIC, Spain) (grant 20225278). MI-P is a recipient of a predoctoral contract from the University of Seville (VI PPIT-US).
The authors declare that the research was conducted in the absence of any commercial or financial relationships that could be construed as a potential conflict of interest.
All claims expressed in this article are solely those of the authors and do not necessarily represent those of their affiliated organizations, or those of the publisher, the editors and the reviewers. Any product that may be evaluated in this article, or claim that may be made by its manufacturer, is not guaranteed or endorsed by the publisher.
The Supplementary Material for this article can be found online at: https://www.frontiersin.org/articles/10.3389/fpls.2023.1227492/full#supplementary-material
Supplementary Table 1 | Sequences used in this study for the analysis and comparison of Cyt c6-like proteins in cyanobacteria.
Supplementary Figure 1 | Schematic of the petJ gene localisation in the genome of single cell and filamentous cyanobacteria. Line represents the position in the genome and the direction of the arrow, the direction of the gene expression. The double dash represents different positions within the genome of the same organism. petE: Plastocyanin; petJ: Cytochrome c6; psbV: Cytochrome c550; petJ-3: Cytochrome c6D.
Adolph, K. W., Haselkorn, R. (1971). Isolation and characterization of a virus infecting the blue-green alga Nostoc muscorum. Virology 46, 200–208. doi: 10.1016/0042-6822(71)90023-7
Albarrán, C., Navarro, J. A., Molina-Heredia, F. P., Murdoch, P. S., de la Rosa, M. A., Hervás, M. (2005). Laser flash-induced kinetic analysis of cytochrome f oxidation by wild-type and mutant plastocyanin from the cyanobacterium Nostoc sp. PCC 7119. Biochemistry 44, 11601–11607. doi: 10.1021/bi050917g
Arslan, E., Schulz, H., Zufferey, R., Künzler, P., Thöny-Meyer, L. (1998). Overproduction of the Bradyrhizobium japonicum c-type cytochrome subunits of the cbb3 oxidase in Escherichia coli. Biochem. Biophys. Res. Commun. 251, 744–747. doi: 10.1006/bbrc.1998.9549
Bialek, W., Krzywda, S., Zatwarnicki, P., Jaskolski, M., Kolesinski, P., Szczepaniak, A. (2014). Insights into the relationship between the haem-binding pocket and the redox potential of c6 cytochromes: Four atomic resolution structures of c6 and c6-like proteins from Synechococcus sp. PCC 7002. Acta Crystallographica Section D Biol. Crystallogr. 70, 2823–2832. doi: 10.1107/S1399004714013108
Bialek, W., Nelson, M., Tamiola, K., Kallas, T., Szczepaniak, A. (2008). Deeply branching c6-like cytochromes of cyanobacteria. Biochemistry 47, 5515–5522. doi: 10.1021/bi701973g
Comeau, S. R., Gatchell, D. W., Vajda, S., Camacho, C. J. (2004). ClusPro: a fully automated algorithm for protein-protein docking. Nucleic Acids Res. 32, W96–W99. doi: 10.1093/nar/gkh354
Crowley, P. B., Díaz-Quintana, A., Molina-Heredia, F. P., Nieto, P. M., Sutter, M., Haehnel, W., et al. (2022). The interactions of cyanobacterial cytochrome c6 and cytochrome f, characterized by NMR. J. Biol. Chem. 277, 48685–48689. doi: 10.1074/jbc.M203983200
Cruz-Gallardo, I., Díaz-Moreno, I., Díaz-Quintana, A., De la Rosa, M. A. (2012). The cytochrome f-plastocyanin complex as a model to study transient interactions between redox proteins. FEBS Lett. 586, 646–652. doi: 10.1016/j.febslet.2011.08.035
Díaz-Moreno, I., Díaz-Quintana, A., Ubbink, M., De la Rosa, M. A. (2005). An NMR-based docking model for the physiological transient complex between cytochrome f and cytochrome c6. FEBS letter 579, 2891–2896. doi: 10.1016/j.febslet.2005.04.031
Falkowski, P. G., Isozaki, Y. (2008). The story of O2. Science 322, 540–542. doi: 10.1126/science.1162641
Felsenstein, J. (1985). Confidence limits on phylogenies: an approach using the bootstrap. Evolution 39, 783–791. doi: 10.2307/2408678
Fuchsman, C. A., Palevsky, H. I., Widner, B., Duffy, M., Carlson, M. C. G., Neibauer, J. A., et al. (2019). Cyanobacteria and cyanophage contributions to carbon and nitrogen cycling in an oligotrophic oxygen-deficient zone. J. Int. Soc. microbial Ecol. 13, 2714–2726. doi: 10.1038/s41396-019-0452-6
Hervás, M., Navarro, J. A., De la Rosa, M. A. (2003). Electron transfer between membrane complexes and soluble proteins in photosynthesis. Accounts Chem. Res. 36, 798–805. doi: 10.1021/ar020084b
Howe, C. J., Nimmo, R. H., Barbrook, A. C., Bendall, D. S. (2016). “Cytochrome c6A of chloroplasts,” in Cytochrome complexes: evolution, structures, energy transduction and signalling. Eds. Cramer, W. A., Kallas, T. (Dordrecht: Springer), 701–712. doi: 10.1007/978-94-017-7481-9
Karp, P. D., Billington, R., Caspi, R., Fulcher, C. A., Latendresse, M., Kothari, A., et al. (2019). The BioCyc collection of microbial genomes and metabolic pathways. Briefings Bioinf. 20, 1085–1093. doi: 10.1093/bib/bbx085
Karp, P. D., Midford, P. E., Paley, S. M., Krummenacker, M., Billington, R., Kothari, A., et al. (2020). Pathway tools version 23.0: integrated software for pathway/genome informatics and systems biology. arXiv, 1–111. doi: 10.48550/arXiv.1510.03964
Ki, H. K. (2005). “Cytochrome c6 genes in cyanobacteria and higher plants,” in Handbook of photosynthesis, 2nd ed. Ed. Pessarakli, M. (Boca Ratón: CRC Press), 286–297. doi: 10.1093/aob/mcj017
Liljas, L. (2013). “Consensus sequences,” in Brenner’s encyclopedia of genetics, 2nd ed. Eds. Maloy, S., Hughes, K. (San Diego: Academic Press), 163–164. doi: 10.1016/B978-0-12-374984-0.00325-9
Lyons, T. W., Reinhard, C. T., Planavsky, N. J. (2014). The rise of oxygen in Earth's early ocean and atmosphere. Nature 506, 307–315. doi: 10.1038/nature13068
Molina-Heredia, F. P., Díaz-Quintana, A., Hervás, M., Navarro, J. A., De la Rosa, M. A. (1999). Site-directed mutagenesis of cytochrome c6 from Anabaena species PCC 7119. Identification of surface residues of the hemeprotein involved in photosystem I reduction. J. Biol. Chem. 274, 33565–33570. doi: 10.1074/jbc.274.47.33565
Molina-Heredia, F. P., Hervas, M., Navarro, J. A., De la Rosa, M. A. (1998). Cloning and correct expression in Escherichia coli of the petE and petJ genes respectively encoding plastocyanin and cytochrome c6 from the cyanobacterium Anabaena sp. PCC 7119. Biochem. Biophys. Res. Commun. 243, 302–306. doi: 10.1006/bbrc.1997.7953
Molina-Heredia, F. P., Hervás, M., Navarro, J. A., De la Rosa, M. A. (2001). A single arginyl residue in plastocyanin and in cytochrome c6 from the cyanobacterium Anabaena sp. PCC 7119 is required for efficient reduction of photosystem I. J. Biol. Chem. 276, 601–605. doi: 10.1074/jbc.M007081200
Molina-Heredia, F. P., Wastl, J., Navarro, J. A., Bendall, D. S., Hervás, M., Howe, C. J. (2003). Photosynthesis: a new function for an old cytochrome? Nature 424, 33–34. doi: 10.1038/424033b
Mullineaux, C. W. (2014). Co-existence of photosynthetic and respiratory activities in cyanobacterial thylakoid membranes. Biochim. Biophys. Acta 1837, 503–511. doi: 10.1016/j.bbabio.2013.11.017
Pettersen, E. F., Goddard, T. D., Huang, C. C., Couch, G. S., Greenblatt, D. M., Meng, E. C., et al. (2004). UCSF Chimera – a visualization system for exploratory research and analysis. J. Comput. Chem. 25, 1605–1612. doi: 10.1002/jcc.20084
Reyes-Sosa, F. M., Gil-Martínez, J., Molina-Heredia, F. P. (2011). Cytochrome c6-like protein as a putative donor of electrons to photosystem I in the cyanobacterium Nostoc sp. PCC 7119. Photosynthesis Res. 110, 61–72. doi: 10.1007/s11120-011-9694-5
Rippka, R., Deruelles, J., Waterbury, J. B., Herdman, M., Stanier, R. Y. (1979). Generic assignments, strain histories and properties of pure cultures of cyanobacteria. J. Gen. Microbiol. 111, 1–61. doi: 10.1099/00221287-111-1-1
Roncel, M., Kirilovsky, D., Guerrero, F., Serrano, A., Ortega, J. M. (2012). Photosynthetic cytochrome c550. Biochim. Biophys. Acta: Bioenergetics 1817, 1152–1163. doi: 10.1016/j.bbabio.2012.01.008
Schmetterer, G., Valladares, A., Pils, D., Steinbach, S., Pacher, M., Muro-Pastor, A. M., et al. (2001). The coxBAC operon encodes a cytochrome c oxidase required for heterotrophic growth in the cyanobacterium Anabaena variabilis strain ATCC 29413. J. Bacteriology 183, 6429–6434. doi: 10.1128/JB.183.21.6429-6434.2001
Slater, B., Kosmützky, D., Nisbet, R. E. R., Howe, C. J. (2021). The evolution of the cytochrome c6 family of photosynthetic electron transfer proteins. Genome Biol. Evol. 13, evab146. doi: 10.1093/gbe/evab146
Stanier, R. Y., Cohen-Bazire, G. (1977). Phototrophic prokaryotes: the cyanobacteria. Annu. Rev. Microbiol. 31, 225–274. doi: 10.1146/annurev.mi.31.100177.001301
Torrado, A., Molina-Heredia, F. P. (2016). “Cytochrome c6-like proteins in cyanobacteria, algae, and higher plants,” in Handbook of photosynthesis, 3rd ed.. Ed. Pessarakli, M. (Boca Ratón: CRC Press), 229–240. doi: 10.1201/9781315372136
Torrado, A., Ramírez-Moncayo, C., Navarro, J. A., Mariscal, V., Molina-Heredia, F. P. (2019). Cytochrome c6 is the main respiratory and photosynthetic soluble electron donor in heterocysts of the cyanobacterium Anabaena sp. PCC 7120. Biochim. Biophys. Acta: Bioenergetics 1860, 60–68. doi: 10.1016/j.bbabio.2018.11.009
Torrado, A., Valladares, A., Puerto-Galán, L., Hervás, M., Navarro, J. A., Molina-Heredia, F. P. (2017). Cytochrome c6-3: A new isoform of photosynthetic cytochrome c6. Plant Cell Physiol. 58, 256–265. doi: 10.1093/pcp/pcw184
Valladares, A., Maldener, I., Muro-Pastor, A. M., Flores, E., Herrero, A. (2014). Heterocyst development and diazotrophic metabolism in terminal respiratory oxidase mutants of the cyanobacterium Anabaena sp. strain PCC 7120. J. Bacteriology 189, 4425–4430. doi: 10.1128/JB.00220-07
Velázquez-Campoy, A., Ohtaka, H., Nezami, A., Muzammil, S., Freire, E. (2004). Isothermal titration calorimetry. Curr. Protoc. Cell Biol. 23, 17.8.1–17.8.24. doi: 10.1002/0471143030.cb1708s23
Woese, C. R. (1987). Bacterial evolution. Microbiological Rev. 51, 221–271. doi: 10.1128/mr.51.2.221-271.1987
Worrall, J. A., Luisi, B. F., Schlarb-Ridley, B. G., Bendall, D. S., Howe, C. J. (2008). Cytochrome c6A: Discovery, structure and properties responsible for its low haem redox potential. Biochem. Soc. Trans. 36, 1175–1179. doi: 10.1042/BST0361175
Zatwarnicki, P., Barciszewski, J., Krzywda, S., Jaskolski, M., Kolesinski, P., Szczepaniak, A. (2014). Cytochrome c(6B) of Synechococcus sp. WH 8102–crystal structure and basic properties of novel c(6)-like family representative. Biochem. Biophys. Res. Commun. 443, 1131–1135. doi: 10.1016/J.BBRC.2013.10.167
Keywords: cytochrome c6, cytochrome c6-like proteins, cytochrome c oxidase, cyanobacteria, cytochrome b6f complex, photosynthesis, respiration, electron transfer
Citation: Torrado A, Iniesta-Pallarés M, Velázquez-Campoy A, Álvarez C, Mariscal V and Molina-Heredia FP (2023) Phylogenetic and functional analysis of cyanobacterial Cytochrome c6-like proteins. Front. Plant Sci. 14:1227492. doi: 10.3389/fpls.2023.1227492
Received: 25 May 2023; Accepted: 21 August 2023;
Published: 06 September 2023.
Edited by:
Chikahiro Miyake, Kobe University, JapanReviewed by:
Ginga Shimakawa, Kwansei Gakuin University, JapanCopyright © 2023 Torrado, Iniesta-Pallarés, Velázquez-Campoy, Álvarez, Mariscal and Molina-Heredia. This is an open-access article distributed under the terms of the Creative Commons Attribution License (CC BY). The use, distribution or reproduction in other forums is permitted, provided the original author(s) and the copyright owner(s) are credited and that the original publication in this journal is cited, in accordance with accepted academic practice. No use, distribution or reproduction is permitted which does not comply with these terms.
*Correspondence: Alejandro Torrado, dG9ycmFkb0BpYnZmLmNzaWMuZXM=; Fernando P. Molina-Heredia, cHVibGlvQHVzLmVz
Disclaimer: All claims expressed in this article are solely those of the authors and do not necessarily represent those of their affiliated organizations, or those of the publisher, the editors and the reviewers. Any product that may be evaluated in this article or claim that may be made by its manufacturer is not guaranteed or endorsed by the publisher.
Research integrity at Frontiers
Learn more about the work of our research integrity team to safeguard the quality of each article we publish.