- French Associates Institute for Agriculture and Biotechnology of Drylands, Blaustein Institutes for Desert Research, Ben-Gurion University of the Negev, Beer Sheva, Israel
The fitness of self-progeny individuals is inferior to that of their outcrossed counterparts, resulting in a reduction in a plant population’s ability to survive and reproduce. To prevent self‐fertilization, angiosperms with hermaphrodite flowers may exploit a variety of mechanisms, including synchronous dichogamy and self-incompatibility. Synchronous dichogamy involves two flowering morphs, with strict within-morph synchronization, thereby preventing not only autogamy and geitonogamy but also intra-morph mating. Self-fertilization is also prevented by self-incompatibility, a genetic mechanism that allows the identification and rejection of “self” pollen, thereby preventing both autogamy and geitonogamy. Here, I seek to provide a perspective of flowering in Ziziphus species exhibiting both synchronous (i.e., “Early” morph flowers open in the morning and “Late” morph flowers open in the afternoon) protandrous dichogamy (i.e., pollen dispersal before the stigma becomes receptive) and self-incompatibility.
Introduction
Most angiosperm species produce hermaphrodite flowers, with both male and female organs in the same flower (Ainsworth, 2000; Renner, 2014). Hermaphroditism appears to increase pollination efficiency and fruit and seed set via self-pollination, i.e., transfer of pollen grains from the anther of one flower to the stigma of the same flower (autogamy) or to a genetically similar flower (geitonogamy), thus ensuring seed production. However, self-progeny plants exhibit reduced fitness, i.e., lower levels of heterozygosity, than their outcrossed counterparts, giving rise to a phenomenon known as inbreeding depression or a reduction in a population’s ability to survive and reproduce (Barrett, 1998; Kelly, 2005). To prevent self-pollination and hence self‐fertilization, plant species with hermaphrodite flowers often develop morphological, molecular and/or phenological adaptations (Lloyd and Webb, 1986; Bertin, 1993; Barrett, 1998; Opedal, 2018) that are exploited in pre- or post-pollination mechanisms [see Narbona et al., 2011 and references within).
The pre-pollination mechanisms are herkogamy and dichogamy. These two mechanisms are similar in that they both facilitate a separation of the presentation of mature anthers and stigmas and hence prevent self-fertilization (Lloyd and Webb, 1986; Wang H, et al., 2021). In herkogamy, morphological barriers provide a spatial separation of sexual functions, which reduces the possibility of intra-flower self-pollination; for example, hermaphrodite flowers may have long stamens and short styles or short stamens and long styles (Webb and Lloyd, 1986; Opedal, 2018). In contrast, in dichogamy, the maturation sequence of the sex organs in hermaphrodite flowers (Stout, 1928 and references within) results in a temporal separation of male and female functions. As such, there is no overlap between staminate and pistillate maturity in any particular flower, and that flower will thus be functionally male or female at a specific developmental time. Dichogamy, being a phenological adaptation that facilitates a temporal separation of male and female reproductive functions within each flower, is thus also referred to as “temporal dioecism” (Cruden, 1988; Molano-Flores, 2001). The term “protandrous dichogamy” is used when the male organs mature first, and “protogynous dichogamy,” when the first phase is female. Protandry is considered less effective in preventing self-fertilization, because pollen may often remain in the anthers and allow self-fertilization when stigmas become receptive (Bertin, 1993).
Dichogamy can be expressed at the whole plant level. As such, flower maturation and anthesis are synchronized at the whole plant level (synchronous dichogamy), meaning that pollen grains are released or stigmas mature within a small window of time (a few hours) in all the flowers on the plant (Narbona et al., 2011; Endress, 2020), producing two morphs with a reciprocal timing of male and female sexual functions. In other words, this developmental synchronization results in a “female” or “male” plant phase at a specific time of the day.
The post-pollination mechanism for preventing self-fertilization is the molecular mechanism of self-incompatibility, which is a genetic mechanism that is controlled by one or more multi-allelic loci and that relies on a series of cellular interactions to prevent self-fertilization (Allen and Hiscock, 2008; Fujii and Kubo, 2016). The self-incompatibility mechanism involves a process of self- and non-self-recognition between the pollen grain and the pistil, which will lead to inhibition of the fertilization of the self-pollen grain (Takayama and Isogai, 2005; Fujii and Kubo, 2016). Self-incompatibility enforces outcrossing, playing a vital role in species diversity in flowering plants (Goldberg et al., 2010).
While each one of the above-described adaptations is in itself thought to effectively prevent self-fertilization, some species have more than one mechanism, as is manifested in the model for this perspective—the hermaphroditic self-incompatible protandrous dichogamous flowers of Ziziphus. Since in protandrous flowers the possibility of self-fertilization by geitonogamy is higher than in protogynous, self-incompatibility may have evolved in response to geitonogamy (Porcher and Lande, 2005).
Here, I review the current literature addressing the role of synchronous dichogamy and self-incompatibility in preventing self-fertilization in Ziziphus species.
Preventing selfing—Ziziphus as a case study
The diversity of mechanisms allowing/preventing sexual reproduction (reproductive strategies) in the short-lived (two-day) flowers of species of Ziziphus (Rhamnaceae) (Figure 1) is well illustrated in studies of the morphology, physiology, genetics and reproduction of Ziziphus (Galil and Zeroni, 1967; Lyrene, 1983; Weekley et al., 2002; Tel-Zur and Schneider, 2009; Asatryan and Tel-Zur, 2014; Cerino et al., 2015). Protandrous synchronous dichogamy was first reported in the evergreen species, Z. spina-christi (L.) Willd., commonly known as Christ’s thorn (Galil and Zeroni, 1967). Later, it was also reported in Z. jujuba, Z. mucronata and Z. mauritiana, all Old World Ziziphus species (Islam and Simmons, 2006). In these species, protandrous dichogamy is synchronized at the tree level, i.e., the flowers of each individual plant mature in synchronization, with little or no overlap between the sexual stages (Galil and Zeroni, 1967; Lyrene, 1983; Zietsman and Botha, 1992; Tel-Zur and Schneider, 2009; Wajnberg et al., 2019; Tel-Zur and Keasar, 2020). This rigid synchronization generates two genotype-specific morphs (Table 1)—one in which the male phase occurs in the morning (type A or “Early morph”) and the other in which the male phase occurs in the afternoon (type B or “Late morph”)—in a ratio of 1:1 in wild populations (Galil and Zeroni, 1967; Renner, 2001). The offspring of a hand pollination trial between the genotypes A and B segregated according to a ratio of 1:1 (“Early morph”: “Late morph”) (Supplementary Data), supporting the assumption that a single pair of alleles controls this trait (Renner, 2001 and references within).
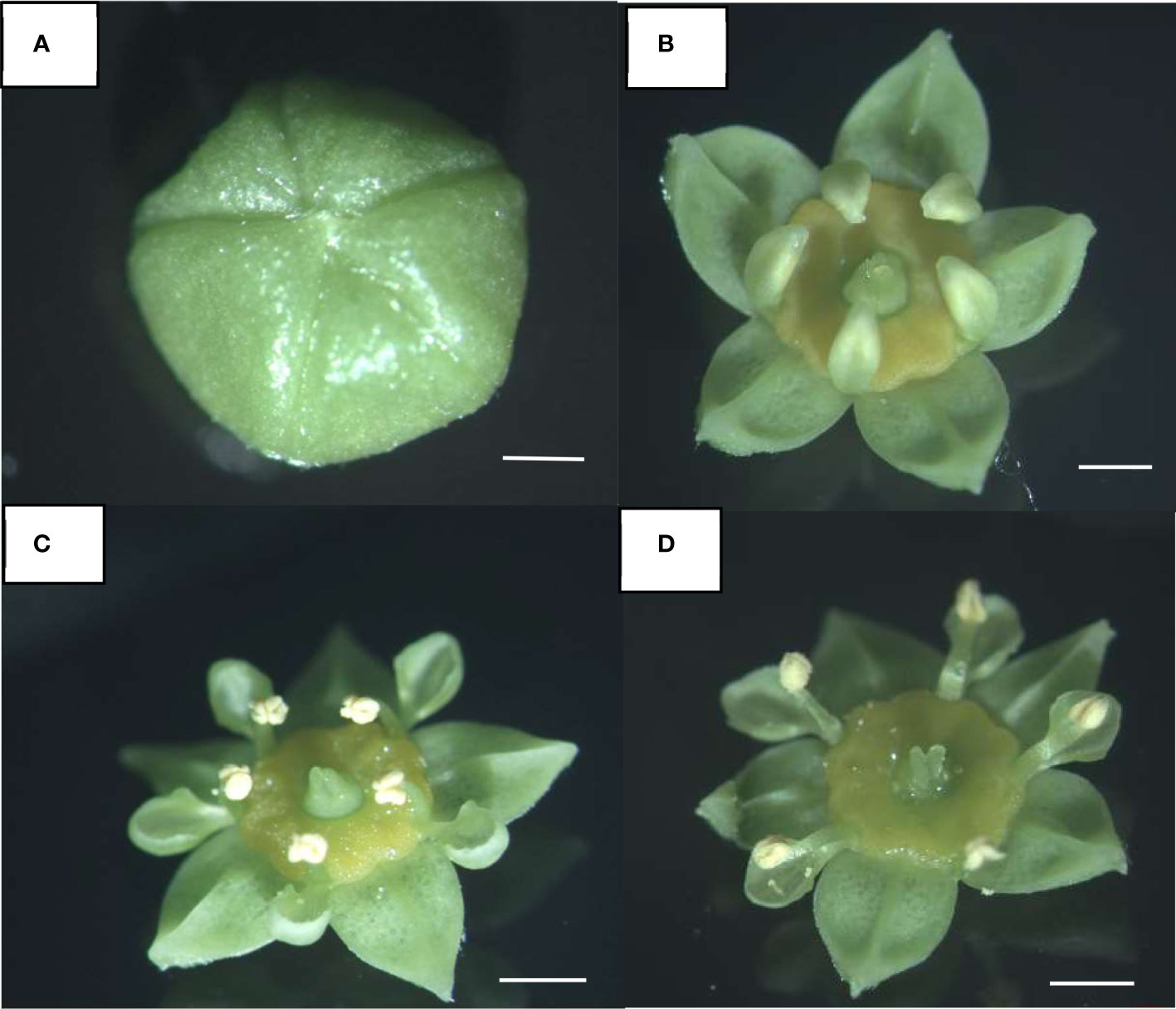
Figure 1 Floral developmental stages in Ziziphus jujuba. (A) Flower bud before opening. (B) Flower opening. (C) Male phase: erect stamens, open anthers, and short style. (D) Female phase: elongated pistil and developed stigma. Stamens recurve between the sepals. The developmental stages, i.e., anthesis and flower maturation, are synchronized at the whole plant level. Scale bar: 1 mm.
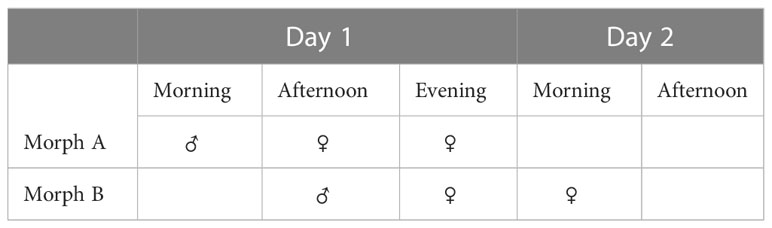
Table 1 Synchronous dichogamy morphs. “Early” morph and “Late” morph in Z. spina-christi [in a ratio of 1:1 in wild populations, see Galil and Zeroni (1967)].
In controlled hand self-pollination studies, a mechanism of self-incompatibility was identified in three Ziziphus species; in these trials, Z. mauritiana and Z. spina-christi produced fruits that dropped off soon after pollination or before maturation, while in Z. jujuba some flowers set small fruits lacking viable seeds (Galil and Zeroni, 1967; Lyrene, 1983; Asatryan and Tel-Zur, 2013; Wang F, et al., 2021). The presence of binucleate pollen grains and the cessation of pollen tube growth in the style observed in these three Ziziphus species support the assumption of a gametophytic self-incompatibility system (Asatryan and Tel-Zur, 2013). Self-incompatibility was also reported in Z. mucronate and Z. celata (Zietsman and Botha, 1992; Weekley and Race, 2001; Weekley et al., 2002; Cerino et al., 2015).
Two other Ziziphus species, both native to the New World, Z. mistol (Islam and Simmons, 2006) and Z. celata, also exhibit protandrous dichogamy, but the sexual phases overlap, i.e., there is a lack of synchronization at the plant level, thus potentially allowing geitonogamy and fertilization within a morph (Weekley et al., 2002; Cerino et al., 2015). In Z. celata, geitonogamy can occur, but self-fertilization is prevented by self-incompatibility (Weekley et al., 2002). In contrast, Z. mistol sets fruits and viable seeds after self-pollination, showing that this species is self-compatible, but a higher fruit set in obtained after cross-pollination (Cerino et al., 2015). Porcher and Lande (2005) developed a theoretical model to explain the breakdown of the gametophytic self-incompatibility system, showing that the spread of the self-compatible genotypes is favored by low or high selfing rates, a low number of S-alleles and pollen limitation. The fact that cross-pollination results in a higher fruit set in Z. mistol suggest a partial self-fertility, as reported in Acca sellowiana (Ramírez and Kallarackal, 2017). In Leavenworthia alabamica self-compatible plants were backcrossed into a self-incompatible population showing that self-compatible plants produced more seeds but those are less viable than outcrossed seeds, evoking that seed discounting and inbreeding depression may explain the fact that self-incompatibility are wide maintained also after selfing mutations in a population (Layman et al., 2017).
Synchronous dichogamy is a very effective mechanism to prevent self-fertilization, geitonogamy, and fertilization within a morph (Lyrene, 1983; Asatryan and Tel-Zur, 2013). However, a breakdown of the synchronization has been observed in natural populations of Z. spina-christi at the end of the flowering season (Galil and Zeroni, 1967), potentially allowing fertilization within a morph (but not via geitonogamy due to the self-incompatibility system) and thereby temporarily changing the species’ reproductive strategy to create a “window” for producing more seeds. To test this premise, Tel-Zur and Keasar (2020) investigated the outcome of hand cross pollination within trees of the same morph in a natural Z. spina-christi population. Fruit set was obtained, but at a significantly lower rate than open pollination. Important to note that the breakdown of the synchronization at the tree level was later reported in “Early morph” trees at the start of the flowering season, but it was particularly marked in both morphs at the end of the flowering season (Tel-Zur and Keasar, 2020), as was also reported by Galil and Zeroni (1967). A similar behavior was observed in the self-incompatible protandrous Aconitum grossedentatum (Ida and Minato, 2020). The collapse of the flower synchronization at the end of the flowering season suggests that synchronous protandry reduces only pre-pollination (selfing) events while post-pollination is blocked by the self-incompatibility system; showing that the benefit of the synchronous protandry decreases over the flowering season (Ida and Minato, 2020). Along with the breakdown of the flower synchronization in Ziziphus species, many of the flowers did not make the transition to the female phase due to the failure of the style to elongate and non-maturation of the stigma (Galil and Zeroni, 1967; Tel-Zur and Schneider, 2009; Tel-Zur and Keasar, 2020), resulting in a higher proportion of male-phase flowers. This phenomenon together with the breakdown of synchronization described above led to a marked alteration in the ovule:pollen grain ratio; which can contribute to the evolution of dioecy.
Discussion
Since dichogamy has been interpreted as a mechanism for preventing inbreeding depression, the combination of dichogamy and self-incompatibility elicits questions about the functional significance of synchronous dichogamy. According to Bertin (1993), a study of angiosperm families revealed that the prevalence of dichogamy was similar in self-incompatible and self-compatible species, i.e., it was found in 73% of 239 self-incompatible species (from 59 families) and in 75% of 673 self-compatible species (from 89 families). Thus, Bertin postulated that – even if it can prevent self-fertilization – the main role of synchronous dichogamy does not lie in preventing self-fertilization but rather in other evolutionary mechanisms, such as preventing pollen-pistil interference or reducing pollen waste. It would thus appear that synchronous dichogamy provides only partial protection from self-fertilization (as evidenced by the breakdown of synchronization described above), whereas self-incompatibility provides complete protection. With this notion in mind, Routley et al. (2004) used published data to construct a phylogenetic framework. They did indeed show positive correlations between protandry (male function first) and self-incompatibility (as in Ziziphus species) and also between protogyny (female function first) and self-compatibility, leading to the conclusion that protandry probably evolved to reduce pollen-pistil interference, and protogyny, to reduce inbreeding. Therefore, it may be suggested that the principal function of synchronous dichogamy is to reduce anther-stigma interference, thus promoting efficient pollen dispersal (Lloyd and Webb, 1986; Harder et al., 2000). Notwithstanding this notion regarding the improved pollination efficiency in dichogamous species (which remains to be confirmed in future studies), it is clear that the temporal separation of floral sex morphs per se prevents, or strongly reduces, selfing. Indeed, the simultaneous occurrence of three mechanisms that prevent inbreeding, namely, dichogamy, synchronization of anthesis at the whole tree level, and self-incompatibility, seems to indicate complementary and redundant mechanisms to prevent self-pollination. For example, in a study of two Euphorbia species, geitonogamy was prevented in the self-compatible species E. nicaeensis due synchronized protogyny, but in E. boetica selfing was prevented due to partial self-incompatibility (Narbona et al., 2011). The authors of that study concluded that synchronous dichogamy and self-incompatibility cannot occur in the same species, since a single mechanism is sufficient to prevent (or strongly reduce) self-fertilization. However, the data collected in Ziziphus species stands in contradiction to this conclusion: in Ziziphus the functions of self-incompatibility and synchronous protandrous dichogamy do overlap (at least in part), although these redundant mechanisms relax at the breakdown of synchrony (Galil and Zeroni, 1967; Tel-Zur and Keasar, 2020), thus increasing the pool of potential reproductive partners for within-morph crossing. In keeping with this idea, trials using marker genes and floral manipulations have supported the assumption that herkogamy and dichogamy reduce self-pollination and promote pollen dispersal (Barrett et al., 2003).
The literature does not offer any clues to the evolutionary origin of the mechanisms that serve to prevent self-fertilization. However, the fact that some Ziziphus species have ‘abandoned’ synchronization and are self-compatible may be connected to the notion that synchronous dichogamy will probably lead to dioecy, i.e., to facilitating the evolution of separate sexes (Renner, 2001 and references within). In this regard, a pioneering study in Z. spina-christi used an evolutionary probabilistic model to test the possible role of insect pollinators in driving such an evolutionary process (Wajnberg et al., 2019). In that study, flower development patterns, floral food rewards, pollinator visits and fruit production were compared between “Early” and “Late” morphs. The data showed that the “Early” morph functions mainly as the pollen donor, while the “Late” morph sets more fruit (Wajnberg et al., 2019), suggesting that “Early” and “Late” morphs will specialize into male and female plants, respectively.
The molecular and genetic regulation of synchronous dichogamy are not yet understood. Further research is needed to uncover the specific genetic and molecular components that control synchronous dichogamy by studying the expression patterns of candidate genes, analyzing genetic mutants, and exploring the influence of environmental cues on reproductive success. The specific genes and molecular pathways involved in the timing and coordination of reproductive organ development remain to be elucidated. Further phylogenetic research aiming to understand the evolution and diversification of synchronous dichogamy would trace the evolutionary relationships among different plant species and identify patterns of trait evolution, including the presence or absence of self-incompatibility. In conclusion, synchronous protandrous dichogamy flowering may thus play a more complex role than previously appreciated in regulating the reproductive system in Ziziphus and other species.
Data availability statement
The original contributions presented in the study are included in the article/Supplementary Files, further inquiries can be directed to the corresponding author.
Author contributions
NT: Conceptualization, Writing.
Acknowledgments
I thank Dr. Udi Zurgil for his work in the lab and field, Ms. Lina Zhao for the pictures and Inez Mureinik for editing the manuscript.
Conflict of interest
The author declares that the research was conducted in the absence of any commercial or financial relationships that could be construed as a potential conflict of interest.
Publisher’s note
All claims expressed in this article are solely those of the authors and do not necessarily represent those of their affiliated organizations, or those of the publisher, the editors and the reviewers. Any product that may be evaluated in this article, or claim that may be made by its manufacturer, is not guaranteed or endorsed by the publisher.
Supplementary material
The Supplementary Material for this article can be found online at: https://www.frontiersin.org/articles/10.3389/fpls.2023.1226502/full#supplementary-material
References
Ainsworth, C. (2000). Boys and girls come out to play: The molecular biology of dioecious plants. Ann. Bot. 86, 211–221. doi: 10.1006/anbo.2000.1201
Allen, A. M., Hiscock, S. J. (2008). Evolution and phylogeny of self-incompatibility systems in angiosperms Self-incompatibility in flowering plants. 73–101.
Asatryan, A., Tel-Zur, N. (2013). Pollen tube growth and self-incompatibility in three Ziziphus species (Rhamnaceae). Flora 208, 390–399. doi: 10.1016/j.flora.2013.04.010
Asatryan, A., Tel-Zur, N. (2014). Intraspecific and interspecific crossability in three Ziziphus species (Rhamnaceae). Genet. Resour. Crop Evol. 61, 215–233. doi: 10.1007/s10722-013-0027-8
Barrett, S. C. H. (1998). The evolution of mating strategies in flowering plants. Trends Plant Sci. 3, 335–341. doi: 10.1016/S1360-1385(98)01299-0
Barrett, S. C. H., Richards, A. J., Bayliss, M. W., Charlesworth, D., Abbott, R. J. (2003). Mating strategies in flowering plants: The outcrossing-selfing paradigm and beyond. In: Philosophical Transactions of the Royal Society B: Biological Sciences. R. Soc., 991–1004. doi: 10.1098/rstb.2003.1301
Bertin, R. I. (1993). Incidence of monoecy and dichogamy in relation to self-fertilization in angiosperms. Am. J. Bot. 80, 557–560. doi: 10.1002/j.1537-2197.1993.tb13840.x
Cerino, M. C., Richard, G. A., Torretta, J. P., Gutiérrez, H. F., Pensiero, J. F. (2015). Reproductive biology of Ziziphus mistol Griseb. (Rhamnaceae), a wild fruit tree of saline environments. Flora 211, 18–25. doi: 10.1016/j.flora.2014.12.002
Cruden, R. W. (1988). Temporal dioecism: systematic breadth, associated traits, and temporal patterns. Botanical Gazette 149 (1), 1–15.
Endress, P. K. (2020). Structural and temporal modes of heterodichogamy and similar patterns across angiosperms. Bot. J. Linn. 193, 5–18. doi: 10.1093/botlinnean/boaa001
Fujii, S., Kubo, K. T. S. (2016). Non-self- and self-recognition models in plant self-incompatibility. Nat. Plants 2, 16130. doi: 10.1038/nplants.2016.130
Galil, J., Zeroni, M. (1967). On the pollination of Zizyphus spina-christi (L.) Willd. in Israel. Isr. J. Bot. 16, 71–77.
Goldberg, E. E., Kohn, J. R., Lande, R., Robertson, K. A., Smith, S. A., Igic, B. (2010). Species selection maintains self-incompatibility. Science 330, 493–495. doi: 10.1126/science.1194513
Harder, L. D., Barrett, S. C. H., Cole, W. W. (2000). The mating consequences of sexual segregation within inflorescences of flowering plants. Proc. R. Soc Lond. B. 267, 315–320. doi: 10.1098/rspb.2000.1002
Ida, T. Y., Minato, E. (2020). Multi-cycle synchronous protandry in raceme-like inflorescences of a bumblebee-pollinated herb Aconitum grossedentatum. Plant Ecol. 221, 965–978. doi: 10.1007/s11258-020-01054-9
Islam, M. B., Simmons, M. P. (2006). A thorny dilemma: Testing alternative intrageneric classifications within Ziziphus (Rhamnaceae). Syst. Bot. 31, 826–842. doi: 10.1600/036364406779695997
Kelly, J. K. (2005). Family level inbreeding depression and the evolution of plant mating systems. New Phytol. 165, 55–62. doi: 10.1111/j.1469-8137.2004.01184.x
Layman, N. C., Fernando, M. T. R., Herlihy, C. R., Busch, J. W. (2017). Cost of selfing prevent the spread of a self-compatibility mutation that causes reproductive assurance. Evolution 71-4, 884–897. doi: 10.1111/evo.13167
Lloyd, D. G., Webb, C. J. (1986). The avoidance of interference between the presentation of pollen and stigmas in angiosperms I. Dichogamy. New Zeal. J. Bot. 24, 135–162. doi: 10.1080/0028825X.1986.10409725
Lyrene, P. M. (1983). Flowering and fruiting of chinese jujubes in Florida. HortScience 18, 208–209. doi: 10.21273/HORTSCI.18.2.208
Molano-Flores, B. (2001). Reproductive biology of Eryngium yuccifolium (Apiaceae ), a prairie species. J. Torrey Bot. Soc, 1–6. doi: 10.2307/3088654
Narbona, E., Ortiz, P. L., Arista, M. (2011). Linking self-incompatibility, dichogamy, and flowering synchrony in two Euphorbia species: Alternative mechanisms for avoiding self-fertilization? PloS One 6 (6). doi: 10.1371/journal.pone.0020668
Opedal, Ø.H. (2018). Herkogamy, a principal functional trait of plant reproductive biology. Int. J. Plant Sci. 179, 677–687. doi: 10.1086/700314
Porcher, E., Lande, R. (2005). Loss of gametophytic self-incompatibility with evolution of inbreeding depression. Evolution 59, 46. doi: 10.1111/j.1420-9101.2005.00905.x
Ramírez, F., Kallarackal, J. (2017). Feijoa [Acca sellowiana (O. Berg) Burret] pollination: A review. Sci. Hortic. 226, 333–341. doi: 10.1016/j.scienta.2017.08.054
Renner, S. S. (2001). How common is heterodichogamy? Trends Ecol. Evol. 16, 595–597. doi: 10.1016/S0169-5347(01)02280-7
Renner, S. S. (2014). The relative and absolute frequencies of angiosperm sexual systems: Dioecy, monoecy, gynodioecy, and an updated online database. Am. J. Bot. 101, 1588–1596. doi: 10.3732/ajb.1400196
Routley, M. B., Bertin, R. I., Husband, B. C. (2004). Correlated evolution of dichogamy and self-incompatibility: A phylogenetic perspective. Int. J. Plant Sci. 165, 983–993. doi: 10.1086/423881
Stout, A. B. (1928). Dichogamy in flowering plants. Bull. Torrey Bot. Club 55, 141–153. doi: 10.2307/2480605
Takayama, S., Isogai, A. (2005). Self-incompatibility in plants. Annu. Rev. Plant Biol. 56, 467–489. doi: 10.1146/annurev.arplant.56.032604.144249
Tel-Zur, N., Keasar, T. (2020). Intraspecific seasonal variation of flowering synchronization in a heterodichogamous tree. Plants 9, 1509. doi: 10.3390/plants9111509
Tel-Zur, N., Schneider, B. (2009). Floral biology of Ziziphus mauritiana (Rhamnaceae). Sex Plant Reprod. 22, 73–85. doi: 10.1007/s00497-009-0093-4
Wajnberg, E., Tel-Zur, N., Shapira, I., Lebber, Y., Lev-Yadun, S., Zurgil, U., et al. (2019). Pollinator behavior drives sexual specializations in the hermaphrodite flowers of a heterodichogamous tree. Front. Plant Sci., 1315. doi: 10.3389/fpls.2019.01315
Wang, H., Barrett, S. C. H., Li, X. Y., Niu, Y., Duan, Y. W., Zhang, Z. Q., et al. (2021). Sexual conflict in protandrous flowers and the evolution of gynodioecy. Evolution 75, 278–293. doi: 10.1111/evo.14113
Wang, F., Sun, X., Dong, J., Cui, R., Liu, X., Li, X., et al. (2021). A primary study of breeding system of Ziziphus jujuba var. spinosa. Sci. Rep. 11, 1–10. doi: 10.1038/s41598-021-89696-1
Webb, C. J., Lloyd, D. G. (1986). The avoidance of interference between the presentation of pollen and stigmas in angiosperms II. Herkogamy. New Zeal. J. Bot. 24, 163–178. doi: 10.1080/0028825X.1986.10409726
Weekley, C., Kubisiak, T., Race, T. (2002). Genetic impoverishment and cross-incompatibility in remnant genotypes of Ziziphus celata (Rhamnaceae), a rare shrub endemic to the Lake Wales Ridge, Florida. Biodivers. Conserv. 11, 2027–2046. doi: 10.1023/A:1020810800820
Weekley, C. W., Race, T. (2001). The breeding system of Ziziphus celata Judd and D.W. Hall (Rhamnaceae), a rare endemic plant of the Lake Wales Ridge, Florida, USA: Implications for recovery. Biol. Conserv. 100, 207–213. doi: 10.1016/S0006-3207(01)00024-6
Keywords: dioecism, pre-or post-pollination mechanisms, self-incompatibility, synchronous dichogamy, hermaphroditism
Citation: Tel-Zur N (2023) Preventing self-fertilization: Insights from Ziziphus species. Front. Plant Sci. 14:1226502. doi: 10.3389/fpls.2023.1226502
Received: 21 May 2023; Accepted: 02 August 2023;
Published: 18 August 2023.
Edited by:
Maximilian Weigend, University of Bonn, GermanyReviewed by:
Ma Luisa Buide, Universidad Pablo de Olavide, SpainCopyright © 2023 Tel-Zur. This is an open-access article distributed under the terms of the Creative Commons Attribution License (CC BY). The use, distribution or reproduction in other forums is permitted, provided the original author(s) and the copyright owner(s) are credited and that the original publication in this journal is cited, in accordance with accepted academic practice. No use, distribution or reproduction is permitted which does not comply with these terms.
*Correspondence: Noemi Tel-Zur, dGVsenVyQGJndS5hYy5pbA==
†ORCID: Noemi Tel-Zur, orcid.org/0000-0001-7670-6518