- 1School of Agriculture, Ludong University, Yantai, China
- 2School of Life Sciences, Liaoning University, Shenyang, China
- 3CAS Key Laboratory of Coastal Environmental Processes and Ecological Remediation, Yantai Institute of Coastal Zone Research, Chinese Academy of Sciences (CAS), Yantai, China
A two-year field trial was conducted to investigate the effects of partial substitution of chemical fertilizer (CF) by Trichoderma biofertilizer (TF) on nitrogen (N) use efficiency and associated mechanisms in wolfberry (Lycium chinense) in coastal saline land. As with plant biomass and fruit yield, apparent N use efficiency and plant N accumulation were also higher with TF plus 75% CF than 100% CF, indicating that TF substitution promoted plant growth and N uptake. As a reason, TF substitution stabilized soil N supply by mitigating steep deceases in soil NH4+-N and NO3–N concentrations in the second half of growing seasons. TF substitution also increased carbon (C) fixation according to higher photosynthetic rate (Pn) and stable 13C abundance with TF plus 75% CF than 100% CF. Importantly, leaf N accumulation significantly and positively related with Pn, biomass, and fruit yield, and structural equation modeling also confirmed the importance of the causal relation of N accumulation coupled with C fixation for biomass and yield formation. Consequently, physiological and agronomical N use efficiencies were significantly higher with TF plus 75% CF than 100% CF. Overall, partial substitution of CF by TF improved N use efficiency in wolfberry in coastal saline land by stabilizing soil N supply and coupling N accumulation with C fixation.
1 Introduction
Saline land is a worldwide resource that must be used rationally to satisfy increasing food demand (Rozema and Schat, 2013; Qin et al., 2015; Nikalje et al., 2017; Zhang et al., 2021). In China, the Yellow River Delta has a large area of coastal saline land with poor agricultural productivity. An important barrier to increase productivity is that nitrogen (N) fertilizer is not used efficiently in saline cropland, because salinity stress inhibits plant N uptake and assimilation (Ashraf et al., 2018). Nitrogen is also easily lost in saline soil, further restricting plant N uptake (Duan et al., 2018; Zhu et al., 2020; Li et al., 2020b). In the Yellow River Delta, hydrological and climatic characteristics such as shallow groundwater table, concentrated rainfall, and high evaporation to precipitation ratio aggravate soil N loss in nitrate leaching and NH3 volatilization (Zhu et al., 2021).
Nitrogen is an essential macronutrient for plants, and crop N deficiency under saline stress is typically expressed in poor growth and yield (Abouelsaad et al., 2016; Yu et al., 2016; Cui et al., 2019). Plant N use efficiency is a comprehensive proxy of N uptake and use and is closely associated with photosynthetic carbon (C) fixation (Wu et al., 2019). Photosynthesis provides the C skeleton and energy for N uptake and assimilation, whereas N uptake and assimilation contribute to the synthesis of photosynthetic pigments and C assimilation enzymes. Thus, coordination between C and N processes is responsible for efficient N use and healthy plant growth. In addition to the decrease in N use, saline stress also depresses photosynthesis by inducing leaf stomatal closure and photosystem photoinhibition (Yan et al., 2013; Yan et al., 2020; Zhao et al., 2020). However, whereas many studies focus on the response of either photosynthesis or N accumulation to salt stress, only a few simultaneously examine salt-induced variations in both processes by using pot experiments (Nazar et al., 2011; Liu et al., 2013). Thus, to determine plant N use in saline land, the synergism between photosynthesis and N accumulation needs to be explored further by field trials.
Chemical fertilizer is habitually overused to increase crop yields in China, but the yield-increasing effect has reached a bottleneck, and much chemical N is wasted to exacerbate the environmental burden (Liang et al., 2019; Li et al., 2021). Partial organic substitution of chemical fertilizer is a sustainable measure that has been used to solve the problem in various agricultural ecosystems, including tea plantations, vegetables, and field crops, because it can guarantee yields while simultaneously reduce chemical N input and loss (Tang et al., 2019; Zhou et al., 2019; Ji et al., 2020; Li et al., 2021; Hou et al., 2023). Soil organic matter is generally low in saline land, which can decrease microbial N immobilization and result in large N loss, and accordingly, organic amendment is a feasible approach to mitigate N loss and improve crop yield by increasing N retention in saline soil (Xiao et al., 2020; Zhu et al., 2020). Plant growth-promoting microorganisms have recently gained increasing attention, because they can be used in a green approach to abate saline barriers, in contrast to other remediation protocols with high investment costs (Arora et al., 2020; Kaushal, 2020). Biofertilizer is a type of organic fertilizer rich in plant growth-promoting microorganisms, and compared with organic fertilizer, biofertilizer application combined with chemical N more effectively reduces N loss in saline soil and increases crop yield (Wang et al., 2018; Xue et al., 2021). Trichoderma is a growth-promoting fungus and also can strengthen plant resistance to salt stress by regulating hormone synthesis, increasing osmotic and antioxidant defenses, and maintaining ion balance and photosynthetic performance (Chen et al., 2016; Zhang et al., 2016; Zhang et al., 2019a; Zhang et al., 2019b; Oljira et al., 2020). Trichoderma inoculation was evidenced to aid in elevating yield and quality of onion and leafy vegetables iceberg lettuce and rocket and reducing fertilizer investment (Ortega-Garcia et al., 2015; Fiorentino et al., 2018). In addition, it has been well documented that partial substitution of chemical fertilizer by Trichoderma biofertilizer (TF) was superior to substitution by organic fertilizer, and could increase soil fertility and nutrient availability, regulate soil microflora composition and enhance crop yield (Cai et al., 2015; Pang et al., 2017; Zhang et al., 2018; Qiao et al., 2019; Liu et al., 2020; Ye et al., 2020; Liu et al., 2021). However, the knowledge about TF application for crop cultivation in saline soil remains very limited and mainly originates from pot experiments. Wang et al. (2018) reported that combined application of TF with urea reduced NH3 loss from saline soil and promoted potted sweet sorghum growth, and likewise, TF effectively altered saline soil properties and microbial composition, leading to the increase in potted Medicago sativa biomass (Zhang et al., 2020). However, the application of Trichoderma spore powder did not improve potted tomato yield under saline irrigation (Daliakopoulosa et al., 2019). Whether TF application can promote crop growth by improving N utilization in coastal saline land still needs to be examined by field trials. In particular, it is largely unknown whether TF substitution can increase N retention in saline soil and stabilize soil N supply.
Wolfberry (Lycium chinense) is a deciduous shrub in the Solanaceae, and its fruit has long been used as traditional medicine and health food in China. Wolfberry may be an ideal economic crop to plant in coastal saline land, because it is a halophyte naturally distributed in coastal zones. However, despite high adaptability to salt, saline stress also inhibits wolfberry photosynthesis and growth, and cultivation methods need to be developed to achieve high fruit yields in saline land (Feng et al., 2017; Dimitrova et al., 2019). Chemical fertilizer was commonly used to improve growth and yield of wolfberry, and the importance of application with organic fertilizer has gradually been recognized, however, it remains unknown whether partial substitution of chemical fertilizer by Trichoderma biofertilizer is more superior for wolfberry growth in coastal saline land. Recently, a Trichoderma asperellum strain was isolated and then successfully prepared as a TF biofertilizer. In this study, the effect of partial substitution of chemical fertilizer (CF) with the TF on N use efficiency in wolfberry was investigated by a two-year field trial in coastal saline land. In particular, mechanisms were examined that affected soil N supply, C and N coupling, and root N absorption. This study may deeply disclose the mechanisms by which TF substitution improve crop growth under salt stress, and also can provide technical guidance for the cultivation of crops in saline land.
2 Materials and methods
2.1 Trichoderma biofertilizer preparation
Trichoderma asperellum strain used in the study was deposited in the China general microbiological culture collection center (No. 13187) in Beijing. Apple residue is a typical organic waste in Yantai city, China and has been utilized as the material to prepare TF by us. A Trichoderma potato glucose agar plate was punched to obtain three 1 cm diameter blocks, which were shaken in potato glucose liquid medium at 150 rpm and 28°C for 5 d to prepare fermentation broth. Similar with the method in previous studies (Chen et al., 2016; Daneshvara et al., 2023), the fermentation broth was added to apple residue compost and mixed thoroughly for fermentation. After 10 days of fermentation, the product was dried to 30% water content to prepare TF. Trichoderma viable colony count was assessed by a dilution method with a selective potato glucose agar plate, which reached 2 × 108 cfu g-1 dry weight in the TF. The selective plate contained nystatin, sodium propionate, and streptomycin and was optimized based on Papavizas and Lumsden (1982). In TF, the content of organic matter, total nitrogen, phosphorus pentoxide and potassium oxide was 641.08 mg g-1, 26.45 mg g-1, 3.37 mg g-1 and 27.77 mg g-1, respectively.
2.2 Field trial
The field trial was conducted in moderate saline land in the Yellow River Delta, Dongying, China (37°17′N, 118°38′E). The site has a warm temperate continental monsoon climate, and annual average temperature and precipitation are approximately 13.5°C and 700 mm, respectively. Soil texture was silty loam, and soil chemical properties were the following: electrical conductivity, 433.7 µs cm-1; pH, 8.3; organic matter, 15.3 g kg-1; total N, 1.0 g kg-1; NH4+-N, 2.2 mg kg-1; NO3–N, 15.3 mg kg-1; Olsen-P, 7.3 mg kg-1; and available K, 267.2 mg kg-1. The field trial had the following four treatments: (1) NF (no N application); (2) 50% CF plus TF (combined application of 50% chemical N fertilizer and TF); (3) 75% CF plus TF (combined application of 75% CF and TF); (4) 100% CF. In each treatment, four replicate plots (5.5 m × 4 m) were randomly distributed in the field. Plots were separated by 0.3 m wide and 0.3 m high ridges to avoid border effects, and 2 m remained around the plots as an isolation belt. The NF plots were designed as the control without N application. The N application rate was 400 kg ha-1 in 100% CF plots, whereas the TF application rate was 5,000 kg ha-1 with reduced chemical nitrogen application in 75% CF or 50% CF plus TF treatments. The amount of TF applied was based on a conventional organic fertilizer dose, and the total N application rate in plots with 75% CF plus TF was the same as that in 100% CF plots. Phosphorus application rate was 50 kg ha-1 in all plots. Urea and calcium superphosphate were applied as chemical N and P fertilizers, respectively. In April, 2020, twelve two-year bare-rooted wolfberry plants were planted in each plot, and plant and row spacing were 1.5 m. In April, 2020 and 2021, fertilizer was applied to circular furrows, which were 20 cm around plants and 20 cm deep. During the growing seasons in 2020 and 2021, three plants were randomly selected in each plot for measuring gas exchange and modulated and prompt chlorophyll fluorescence parameters. The topsoil (0–20 cm) was also collected in each plot by a five-point sampling method with a soil auger (diameter, 38 mm) in the growing seasons. At the end of October in 2020 and 2021, one plant was selected in each plot and excavated to measure biomass, N and C contents and leaf stable N and C compositions.
2.3 Assay of soil nitrogen concentration
Soil samples were sieved through a 2 mm screen and homogenized. One subsample was used to measure soil NH4+-N and NO3–N concentrations and moisture. Soil NH4+-N and NO3–N were extracted with 2 M KCl for 1 h, and filtrates were analyzed for NH4+-N and NO3–N concentrations by a continuous flow analyzer (AutoAnalyzer III, Seal, Germany). Another soil subsample was air-dried, ground, and sieved through a 150 μm screen, and soil total N was determined by an elemental analyzer (VarioMACRO cube, Elementar Analysensysteme, Germany). Soil available K was extracted by using ammonium acetate to measure its content through flame photometry (Lu et al., 2017).
2.4 Assay of plant nitrogen content
Plants from plots were separated to leaves, roots, stems, and fruit, which were oven-dried at 60°C to constant weight, ground, and sieved through a 250 μm screen. Powders were digested by H2SO4–H2O2, and total N in digests was determined by indophenol blue colorimetry (Ivancic and Degobbis, 1984). In treatments, FDWt, BDWt, and Nt indicate fruit dry weight, plant total biomass, and N accumulation, respectively, whereas in the control, FDWc, BDWc, and Nc respectively indicate those variables. The variable Nf is the amount of N applied. Agronomic, apparent, and physiological N use efficiencies were calculated as (FDWt-FDWc)/Nf, (Nt-Nc)/Nf, and (BDWt-BDWc)/(Nt-Nc), respectively (Chen et al., 2020).
2.5 Measurements of leaf stable carbon and nitrogen isotopic compositions and nitrogen and carbon concentrations
Fully expanded leaves in plots were selected, oven-dried at 60°C to constant weight, ground, and sieved through a 250 μm screen. Leaf powder (0.06 mg) was packed in tin capsules, which were sealed and measured using an Elemental Analyzer (FlashEATM 1112, ThermoScientific, Germany) coupled with an isotope ratio mass spectrometer (Finnigan Delta Plus XPTM, ThermoScientific). Stable C and N isotope compositions were expressed using standard delta notation: (δ, ‰) = Rsample/Rstandard - 1, where Rsample and Rstandard were isotopic ratios in samples and standards, respectively. Total N and C in leaf powders were also measured using an elemental analyzer (VarioMACRO cube).
2.6 Gas exchange and modulated and prompt chlorophyll fluorescence measurements
In fully expanded leaves in plots, gas exchange and modulated chlorophyll fluorescence parameters were simultaneously detected by using an open photosynthetic system (LI-6400XTR, Li-Cor, USA) equipped with a fluorescence leaf chamber (6400-40 LCF, Li-Cor). Temperature and CO2 concentration in the leaf cuvette were set at 25°C and 400 μmol mol-1, respectively. Actinic light intensity was set at 1200 µmol m-2 s-1 in the field trial. Photosynthetic rate (Pn) and stomatal conductance (Gs) were simultaneously recorded, and modulated chlorophyll fluorescence was also recorded to calculate photosynthetic electron transport rate (ETR) according to a previous study (Yan et al., 2020).
A multifunctional plant efficiency analyzer (MPEA, Hansatech, UK) was used to record prompt chlorophyll fluorescence in the first 1 s of illumination with red light, and the PSII performance index (PIabs) was calculated according to Strasser et al. (2010).
2.7 Statistical analyses
Data are presented as the mean of samples from four replicate plots in the field trial. Means were tested for significant differences using an LSD test following one-way ANOVA. Statistical analyses were conducted in SPSS 22.0 (SPSS Inc., Chicago, IL, USA), and differences were considered significant at P < 0.05. Regression analysis was also performed using SPSS 22.0.
Structural equation modeling (SEM) was used to evaluate direct and indirect relations among N accumulation, C fixation, and yield in different fertilization treatments and was performed using AMOS Graphics 23.0 (IBM Corp., Armonk, NY, USA). The fit of the resulting model was evaluated using P-values, χ2 values, a goodness-of-fit index (GFI), and the root mean square error of approximation (RMSEA).
3 Results
3.1 Fruit yield, biomass, and nitrogen content and use efficiency
Fruit yield, plant biomass, and N content increased significantly with application of N fertilizer, and the increase was significantly higher in plots with TF plus 75% CF than in plots with 100% CF and TF plus 50% CF (Figures 1A, C, E). Compared with 100% CF plots, agronomic, apparent, and physiological N efficiencies increased significantly in plots with TF plus 75% CF by 78.23%, 80.76%, and 35.32% in 2020 and by 82.70%, 31.67%, and 46.60% in 2021, respectively. However, compared with 100% CF, only physiological N efficiency increased significantly in plots with TF plus 50% CF (Figures 1B, D, F).
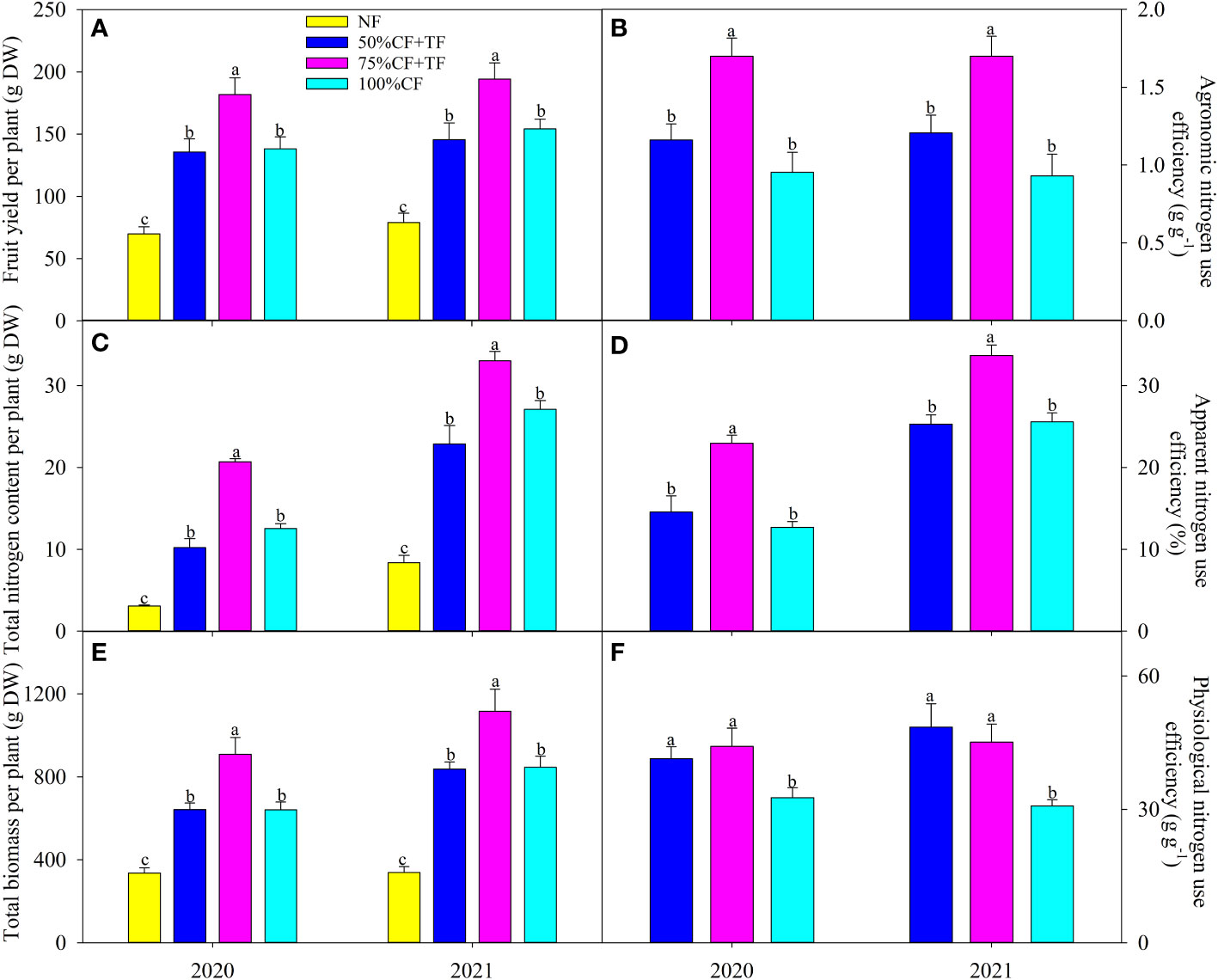
Figure 1 Wolfberry (A) fruit yield, (C) total nitrogen, and (E) biomass and (B) apparent, (D) physiological, and (F) agronomical nitrogen use efficiency in different fertilizer treatments in coastal saline land. Values are the mean of four replicate plots ( ± SD), and different letters indicate significant differences among treatments at P < 0.05. NF, no nitrogen fertilizer; 100% CF, 100% chemical nitrogen fertilizer; 50% CF+TF, 50% CF plus Trichoderma biofertilizer; 75% CF+TF, 75% CF plus Trichoderma biofertilizer.
3.2 Leaf δ13C, δ15N, carbon, and nitrogen contents
Applying N fertilizer did not significantly affect leaf δ13C, except for a significant increase in TF plus 75% CF plots in 2020 and 2021 (Figure 2A). Leaf δ15N increased significantly with N fertilizer application, but there was no significant difference in leaf δ15N between plots with TF plus 75% CF and those with 100% CF (Figure 2B). Carbon and N contents per leaf increased significantly with application of N fertilizer, but the increases in TF plus 75% CF plots were significantly greater than those in 100% CF and TF plus 50% CF plots (Figures 2C, D).
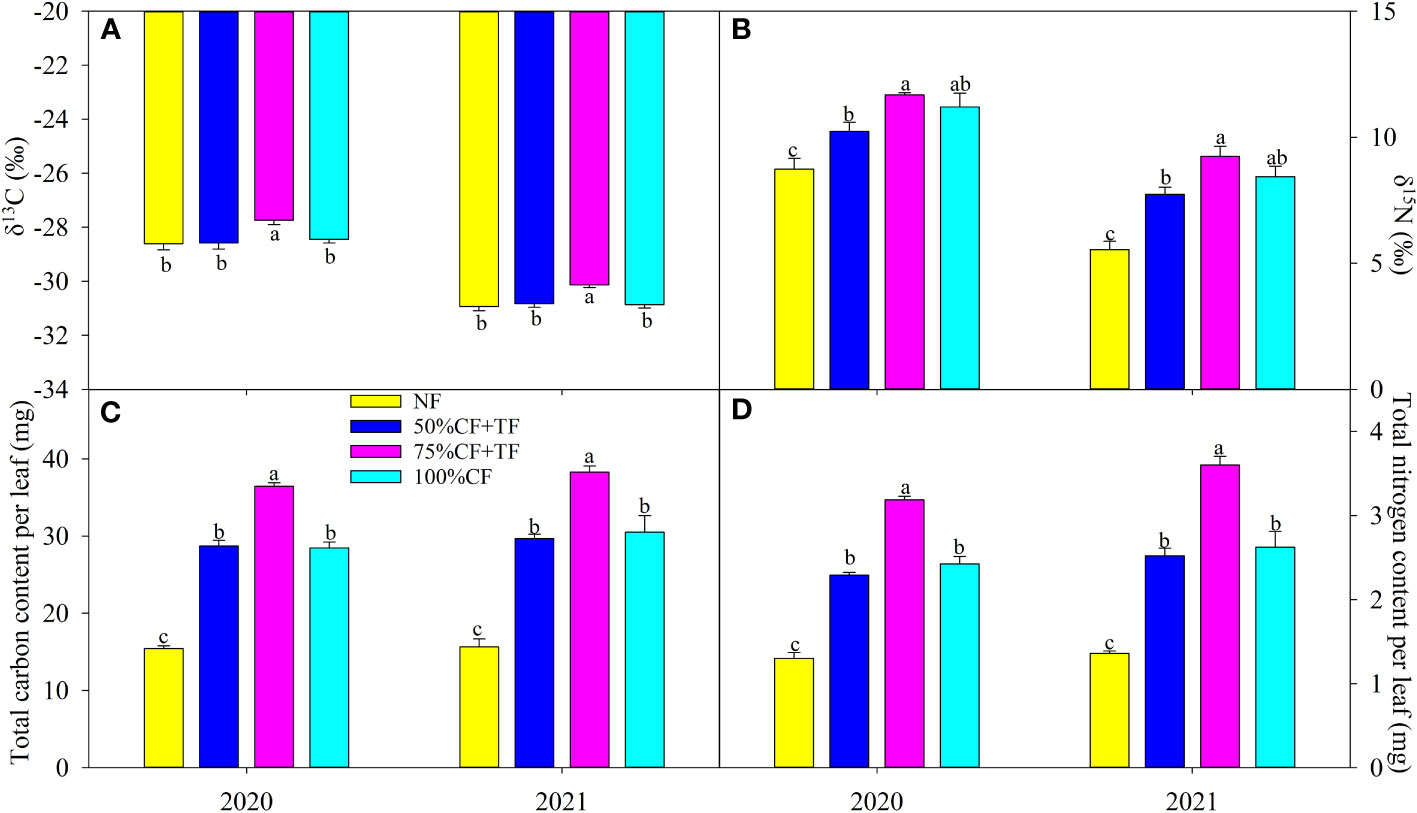
Figure 2 Leaf (A) carbon (δ13C) and (B) nitrogen (δ15N) isotope composition and (C) carbon, and (D) nitrogen accumulation in wolfberry in different fertilizer treatments in coastal saline land. Values are the mean of four replicate plots ( ± SD), and different letters indicate significant differences among treatments at P < 0.05. NF, no nitrogen fertilizer; 100% CF, 100% chemical nitrogen fertilizer; 50% CF+TF, 50% CF plus Trichoderma biofertilizer; 75% CF+TF, 75% CF plus Trichoderma biofertilizer.
3.3 Photosynthetic parameters in the field trial
In 2020 and 2021 growing seasons, Pn, Gs, ETR, and PIabs generally increased with N fertilizer application. Changes in those parameters were relatively greater in TF plus 75% CF plots than in 100% CF and TF plus 50% CF plots, although differences were not always significant in the monthly samplings (Figure 3).
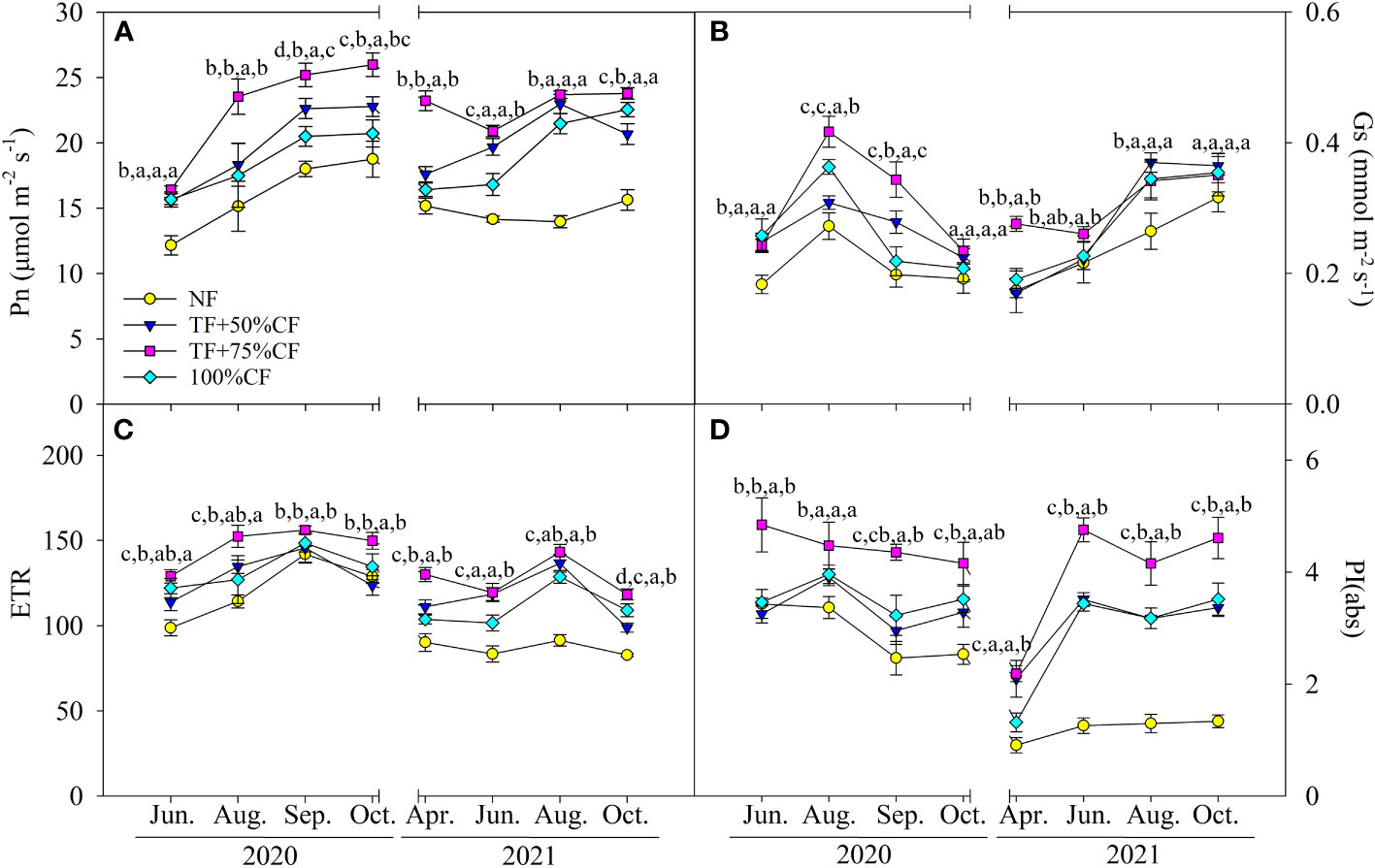
Figure 3 Wolfberry (A) photosynthetic rate (Pn), (B) stomatal conductance (Gs), (C) photosynthetic electron transport rate (ETR), and (D) performance index (PIabs) in different fertilizer treatments in coastal saline land. Values are the mean of four replicate plots ( ± SD), and different letters indicate significant differences among treatments at P < 0.05. NF, no nitrogen fertilizer; 100% CF, 100% chemical nitrogen fertilizer; 50% CF+TF, 50% CF plus Trichoderma biofertilizer; 75% CF+TF, 75% CF plus Trichoderma biofertilizer.
3.4 Structural equation model analysis and relations between leaf nitrogen accumulation and photosynthetic rate, plant biomass, and fruit yield
In regression analysis, leaf N accumulation was significantly positively related to Pn, plant biomass, and fruit yield (Figures 4A-C). An SEM analysis was conducted to identify direct and indirect relations among N accumulation, C fixation, plant biomass, and fruit yield (Figure 4D). In the SEM, leaf N accumulation directly positively regulated leaf C accumulation and Pn and also significantly indirectly affected fruit yield and plant biomass by affecting C fixation (Figure 4D). Thus, the coupling of accumulated N with C fixation was responsible for increases in plant growth and yield with TF substitution fertilization.
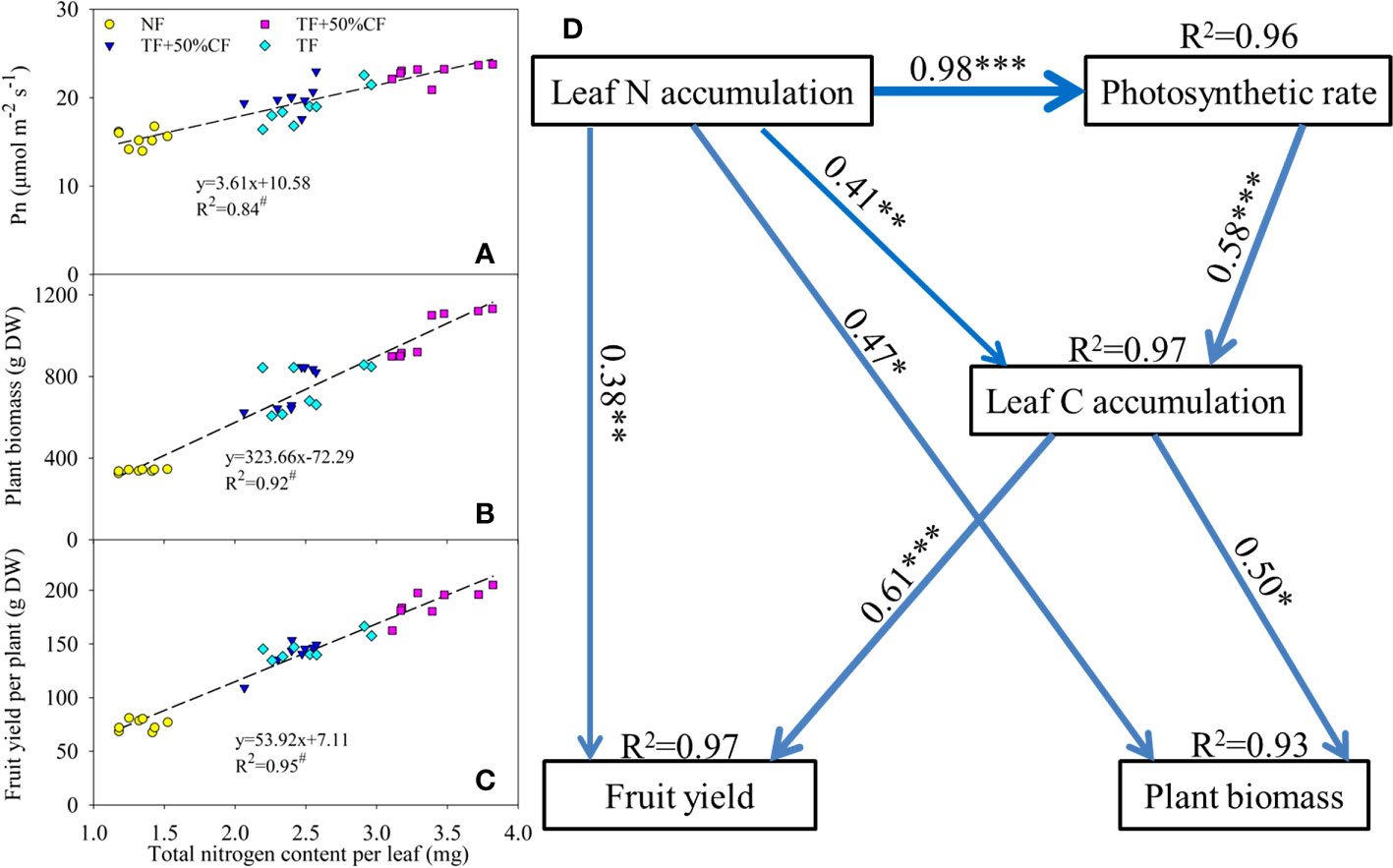
Figure 4 Regression analysis of leaf nitrogen accumulation with (A) photosynthetic rate (Pn), (B) biomass, and (C) fruit yield in wolfberry in coastal saline land. Significant relation at P < 0.01 is indicated by #. NF, no nitrogen fertilizer; 100% CF, 100% chemical nitrogen fertilizer; 50% CF+TF, 50% CF plus Trichoderma biofertilizer; 75% CF+TF, 75% CF plus Trichoderma biofertilizer. (D) Structural equation model of hypothesized causal relations among leaf nitrogen and carbon accumulation, Pn, biomass, and yield formation in wolfberry in a two-year field trail. Model fit: χ2 = 0.415, P = 0.937, GFI = 0.995, RMSEA < 0.001. Blue solid lines indicate significant positive effects (P < 0.05). Standardized path coefficients are listed beside each path (line width indicates the proportion of factorial contribution). The R2 values indicate the strength of explanation by independent variables. Significant effects: *P < 0.05; **P < 0.01; ***P < 0.001.
3.5 Soil total and mineral nitrogen contents
Compared with NF and 100% CF plots, soil total N content increased significantly in TF plus 75% CF plots at the end of the growth seasons in October 2020 and 2021 (Figures 5A, C). Soil NH4+-N and NO3–N contents increased significantly in June in plots with N fertilizer application and then gradually declined during the growing seasons (Figures 5B, D). The decreases in soil NH4+-N and NO3–N contents after June were not as great in TF plus 75% CF plots as in 100% CF plots, and soil NH4+-N and NO3–N contents remained generally higher in TF plus 75% CF plots in the second half of the seasons (Figures 5B, D).
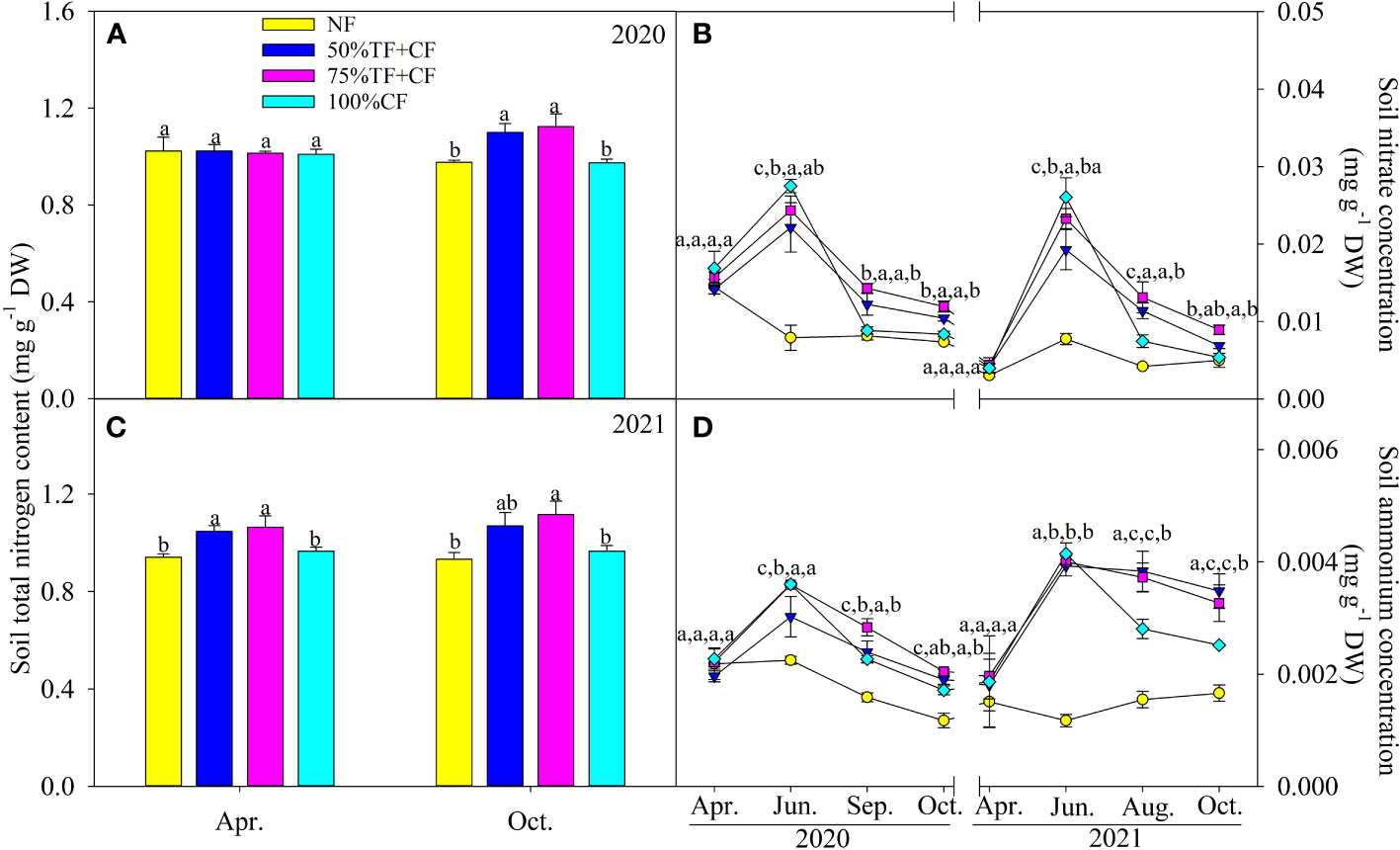
Figure 5 Topsoil total nitrogen content in (A) 2020 and (C) 2021 and (B) nitrate and (D) ammonium content in different fertilizer treatments in coastal saline land. Values are the mean of four replicate plots ( ± SD), and different letters indicate significant differences among treatments at P < 0.05. NF, no nitrogen fertilizer; 100% CF, 100% chemical nitrogen fertilizer; 50% CF+TF, 50% CF plus Trichoderma biofertilizer; 75% CF+TF, 75% CF plus Trichoderma biofertilizer.
4 Discussion
High N application is commonly used for planting wolfberry by farmers because N in saline soil is liable to loss, and maybe, a lower chemical N application rate is appropriate considering that excessive N availability can lead to a systemic repression of root growth with yield reduction (Giehl and von Wiren, 2014; Liu et al., 2022). For example, Liu et al. (2018) reported that high N application rate at 300 kg ha-1 reduced wheat yield and root weight density in contrast to an optimal application rate at 240 kg ha-1. However, this study focused on the approach of TF substitution to reduce chemical N application. TF substitution could reduce 50% CF investment without influencing wolfberry growth and yield in contrast to 100% CF application, and notably, TF substitution with 25% reduction of chemical fertilizer improved wolfberry growth and yield (Figures 1A, E). Thus, TF proved to be functional and beneficial for wolfberry cultivation in coastal saline land. The growth-promoting effect of TF probably related to N uptake and use, considering greater plant nitrogen accumulation in plots with TF plus 75% CF than 100% CF (Figure 1C). Consistently, higher apparent, physiological, and agronomic N use efficiencies in plants with TF plus 75% CF further verified that growth-promoting function of TF involved plant N uptake and use (Figures 1B, D, F). As a proxy of N uptake and use in plants, δ15N has a positive relation with crop growth and yield (Yousfi et al., 2009; Yousfi et al., 2012). In this study, δ15N increased in plants with N fertilization. However, the relatively minor difference in leaf δ15N did not match the greater yield and biomass in 75% CF plus TF plots than in 100% CF plots (Figures 1A, C; 2B). Nitrogen isotope fractionation mainly occurs during enzymatic assimilation of nitrate or ammonium into organic forms. High N assimilation capacity can directly increase δ15N by decreasing discrimination against 15N, whereas high N uptake indirectly increases discrimination against 15N by increasing available N (Evans, 2001; Tcherkez, 2011). Thus, greater N uptake in plants with TF plus 75% CF than with 100% CF might attenuate leaf 15N discrimination. Yousfi et al. (2012) also found increases in biomass and N accumulation with insignificant variation in shoot δ15N in salt-tolerant cultivar RIL47 of durum wheat in contrast to salt-sensitive cultivar RIL 24 under salt stress. Overall, TF substitution increased wolfberry N uptake and use in coastal saline land.
Similar to the accumulation of plant N, the stock of soil total N also increased in 75% CF plus TF plots compared with 100% CF plots at the end of growth seasons (Figures 5A, C), suggesting that TF increased retention of fertilizer N in saline soil and as a result, could benefit plant N uptake. This finding was supported by previous reports that organic supplements could inhibit N loss through NH3 volatilization from saline soil (Al-Busaidi et al., 2014; Xiao et al., 2020; Yao et al., 2021). Notably, in a pot experiment, N storage in saline soil increased with application of Trichoderma spore powder (Daliakopoulosa et al., 2019). In addition, in contrast to 100% CF plots, TF substitution mitigated the steep decrease in soil mineral N concentrations that occurred in the second half of growing seasons. Therefore, by slowing the decline in soil mineral N concentrations, TF increased stability of soil mineral N supply (Figures 5B, D). In agreement with our finding, Wang et al. (2018) found that in a pot experiment, TF reduced NH3 volatilization loss from saline soil by increasing soil nitrification which also increased N supply. Recent studies also show that addition of organic materials such as crop straw can optimize soil N supply by regulating N transformations (Lu et al., 2018; Li et al., 2020a; Chen et al., 2021; Yuan et al., 2021). Therefore, soil N transformations in coastal saline land under combined application of CF with TF should be investigated further. However, in the field trial in this study, TF substitution helped to improve soil N supply to plants in coastal saline land.
Plant N use for growth and yield formation is dependent on photosynthetic C fixation. The greater increase in Pn with 75% CF plus TF than with 100% CF suggested that TF substitution helped increase photosynthetic capacity in wolfberry (Figure 3A). PSII is the initiation site for driving photosynthetic electron transport, and it is also susceptible to photoinhibition under environmental stresses (Takahashi and Murata, 2008). In accordance with Pn, the increase in PI(abs) was greater with 75% CF plus TF than with 100% CF, which maintained ETR at a higher level to promote C assimilation (Figures 3C, D). In addition, the greater increase in Gs with 75% CF plus TF than with 100% CF also benefited photosynthesis by increasing CO2 diffusion into leaves (Figure 3B). Increases in CO2 supply with high Gs tend to decrease δ13C by increasing 13C fractionation, whereas strong CO2 fixation can depress discrimination against 13C and increase δ13C (Farquhar et al., 1989; Li et al., 2017). Thus, the increase in δ13C without reduction in Gs indicated greater CO2 fixation in plants with 75% CF plus TF than with 100% CF (Figures 2A; 3B). Overall, TF substitution improved photosynthetic capacity of wolfberry in coastal saline land and then increased leaf C accumulation (Figure 2C). Importantly, leaf N accumulation was significantly and positively related with Pn, biomass, and fruit yield, and the SEM also confirmed the importance of the causal relation of N accumulation coupled with C fixation for biomass and yield formation (Figure 4). Therefore, elevated N accumulation could well couple with photosynthetic C fixation to increase wolfberry biomass and yield with 75% CF plus TF, and led to higher physiological and agronomic nitrogen use efficiency (Figures 1B, F).
5 Conclusion
In summary, partial substitution of CF by TF improved N use efficiency in wolfberry in coastal saline land by stabilizing soil N supply and increasing the synergism between N accumulation and C fixation.
Data availability statement
The raw data supporting the conclusions of this article will be made available by the authors, without undue reservation.
Author contributions
KY designed the experiment, performed the experiment, and wrote the manuscript. YR and HS participated in data analysis. HM, SY, YZ, SL, and YS participated in the experiment. All authors contributed to the article and approved the submitted version.
Funding
This work was supported by Joint Funds of the National Natural Science Foundation of China (U2106214), Shandong Provincial Natural Science Foundation (ZR2022MC079; ZR2020MC153), Yantai Science and Technology Innovation Development Plan (2020MSGY065), the National Key Research & Development Project in China (2019YFD1002702) and the Scientific Instrument Developing Project of the Chinese Academy of Sciences (YJKYYQ20190035).
Conflict of interest
The authors declare that the research was conducted in the absence of any commercial or financial relationships that could be construed as a potential conflict of interest.
Publisher’s note
All claims expressed in this article are solely those of the authors and do not necessarily represent those of their affiliated organizations, or those of the publisher, the editors and the reviewers. Any product that may be evaluated in this article, or claim that may be made by its manufacturer, is not guaranteed or endorsed by the publisher.
References
Abouelsaad, I., Weihrauch, D., Renault, S. (2016). Effects of salt stress on the expression of key genes related to nitrogen assimilation and transport in the roots of the cultivated tomato and its wild salt-tolerant relative. Sci. Hortic. 211, 70–78. doi: 10.1016/j.scienta.2016.08.005
Al-Busaidi, K. T. S., Buerkert, A., Joergensen, R. G. (2014). Carbon and nitrogen mineralization at different salinity levels in Omani low organic matter soils. J. Arid. Environ. 100-101, 106–110. doi: 10.1016/j.jaridenv.2013.10.013
Arora, N. K., Fatima, T., Mishra, J., Mishra, I., Verma, S., Verma, R., et al. (2020). Halo-tolerant plant growth promoting rhizobacteria for improving productivity and remediation of saline soils. J. Adv. Res. 26, 69–82. doi: 10.1016/j.jare.2020.07.003
Ashraf, M., Shahzad, S. M., Imtiaz, M., Rizwan, M. S. (2018). Salinity effects on nitrogen metabolism in plants - focusing on the activities of nitrogen metabolizing enzymes: A review. J. Plant Nutr. 41, 1065–1081. doi: 10.1080/01904167.2018.1431670
Cai, F., Chen, W., Wei, Z., Pang, G., Li, R., Ran, W., et al. (2015). Colonization of Trichoderma harzianum strain SQR-T037 on tomato roots and its relationship to plant growth, nutrient availability and soil microflora. Plant Soil 388, 337–350. doi: 10.1007/s11104-014-2326-z
Chen, J. G., Liu, X. Q., Liu, S. H., Fan, X. R., Zhao, L. M., Song, M. Q., et al. (2020). Co-overexpression of OsNAR2.1 and OsNRT2.3a increased agronomic nitrogen use efficiency in transgenic rice plants. Front. Plant Sci. 11. doi: 10.3389/fpls.2020.01245
Chen, H., Liu, Y., Lu, L., Yuan, L., Jia, J., Chen, X., et al. (2021). Effects of no-tillage and stover mulching on the transformation and utilization of chemical fertilizer N in Northeast China. Soil Tillage Res. 213, 105131. doi: 10.1016/j.still.2021.105131
Chen, L. H., Zheng, J. H., Shao, X. H., Shen, S. S., Yu, Z. H., Mao, X. Y., et al. (2016). Effects of Trichoderma harzianum T83 on Suaeda salsa L. in coastal saline soil. Ecol. Eng. 91, 58–64. doi: 10.1016/j.ecoleng.2016.01.007
Cui, G. C., Zhang, Y., Zhang, W. J., Lang, D. Y., Zhang, X. J., Li, Z. X., et al. (2019). Response of carbon and nitrogen metabolism and secondary metabolites to drought stress and salt stress in plants. J. Plant Biol. 62, 387–399. doi: 10.1007/s12374-019-0257-1
Daliakopoulosa, I. N., Apostolakisa, A., Wagnera, K., Deligiannia, A., Koutskoudisa, D., Stamatakisb, A., et al. (2019). Effectiveness of Trichoderma harzianum in soil and yield conservation of tomato crops under saline irrigation. Catena 175, 144–153. doi: 10.1016/j.catena.2018.12.009
Daneshvara, H., Babalar, M., Diaz-Perez, J. C., Nambeesan, S., Delshad, M., Tabrizi, L. (2023). Evaluation of organic and mineral fertilizers on plant growth, minerals, and postharvest quality of celery (Apium graveolens L.). J. Plant Nutr. 46, 1712–1729. doi: 10.1080/01904167.2022.2097921
Dimitrova, V. L., Paunov, M. M., Goltsev, V., Geneva, M. P., Markovska, Y. K. (2019). Effect of soil salinity on growth, metal distribution and photosynthetic performance of two Lycium species. Photosynthetica 57, 32–39. doi: 10.32615/ps.2019.006
Duan, M., House, J., Liu, Y., Chang, S. X. (2018). Contrasting responses of gross and net nitrogen transformations to salinity in a reclaimed boreal forest soil. Biol. Fertil. Soils 54, 385–395. doi: 10.1007/s00374-018-1268-7
Evans, R. D. (2001). Physiological mechanisms influencing plant nitrogen isotope composition. Trends Plant Sci. 6, 121–126. doi: 10.1016/s1360-1385(01)01889-1
Farquhar, G. D., Ehleringer, J. R., Hubick, K. T. (1989). Carbon isotope discrimination and photosynthesis. Annu. Rev. Plant Physiol. Plant Mol. Biol. 40, 503–537. doi: 10.1146/annurev.pp.40.060189.002443
Feng, X. H., An, P., Guo, K., Li, X. G., Liu, X. J., Zhang, X. M. (2017). Growth, root compensation and ion distribution in Lycium chinense under heterogeneous salinity stress. Sci. Hortic. 226, 24–32. doi: 10.1016/j.scienta.2017.08.011
Fiorentino, N., Ventorino, V., Woo, S. L., Pepe, O., De Rosa, A., Gioia, L., et al. (2018). Trichoderma-based biostimulants modulate rhizosphere microbial populations and improve N uptake efficiency, yield, and nutritional quality of leafy vegetables. Front. Plant Sci. 9, 743. doi: 10.3389/fpls.2018.00743
Giehl, R. F. H., von Wiren, N. (2014). Root nutrient foraging. Plant Physiol. 166, 509–517. doi: 10.1104/pp.114.245225
Hou, Q., Ni, Y., Huang, S., Zuo, T., Wang, J., Ni, W. (2023). Effects of substituting chemical fertilizers with manure on rice yield and soil labile nitrogen in paddy fields of China: A meta-analysis. Pedosphere 33, 172–184. doi: 10.1016/j.pedsph.2022.09.003
Ivancic, I., Degobbis, D. (1984). An optimal manual procedure for ammonia analysis in natural waters by the indophenol blue method. Water Res. 18, 1143–1147. doi: 10.1016/0043-1354(84)90230-6
Ji, L., Ni, K., Wu, Z., Zhang, J., Yi, X., Yang, X., et al. (2020). Effect of organic substitution rates on soil quality and fungal community composition in a tea plantation with long-term fertilization. Biol. Fertil. Soils 56, 633–646. doi: 10.1007/s00374-020-01439-y
Kaushal, M. (2020). Insights into microbially induced salt tolerance and endurance mechanisms (STEM) in plants. Front. Microbiol. 11. doi: 10.3389/fmicb.2020.01518
Li, P., Li, Y. B., Xu, L. Y., Zhang, H. J., Shen, X. S., Xu, H. F., et al. (2021). Crop yield-soil quality balance in double cropping in China's upland by organic amendments: A meta-analysis. Geoderma 403, 115197. doi: 10.1016/j.geoderma.2021.115197
Li, X., Li, Z., Zhang, X., Xia, L., Zhang, W., Ma, Q., et al. (2020a). Disentangling immobilization of nitrate by fungi and bacteria in soil to plant residue amendment. Geoderma 374, 114450. doi: 10.1016/j.geoderma.2020.114450
Li, Y., Xu, J., Liu, S., Qi, Z., Wang, H., Wei, Q., et al. (2020b). Salinity-induced concomitant increases in soil ammonia volatilization and nitrous oxide emission. Geoderma 361, 114053. doi: 10.1016/j.geoderma.2019.114053
Li, R. S., Yang, Q. P., Zhang, W. D., Zheng, W. H., Chi, Y. G., Xu, M., et al. (2017). Thinning effect on photosynthesis depends on needle ages in a Chinese fir (Cunninghamia lanceolata) plantation. Sci. Total Environ. 580, 900–906. doi: 10.1016/j.scitotenv.2016.12.036
Liang, L., Ridoutt, B. G., Lal, R., Wang, D., Wu, W., Peng, P., et al. (2019). Nitrogen footprint and nitrogen use efficiency of greenhouse tomato production in North China. J. Clean Prod. 208, 285–296. doi: 10.1016/j.jclepro.2018.10.149
Liu, Z. X., Bie, Z. L., Huang, Y., Zhen, A., Niu, M. L., Lei, B. (2013). Rootstocks improve cucumber photosynthesis through nitrogen metabolism regulation under salt stress. Acta Physiol. Plant 35, 2259–2267. doi: 10.1007/s11738-013-1262-5
Liu, X., Cui, Z., Hao, T., Yuan, L., Zhang, Y., Gu, B., et al. (2022). A new approach to holistic nitrogen management in China. Front. Agr. Sci. Eng. 9 (3), 490–510. doi: 10.15302/J-FASE-2022453
Liu, H., Duan, W., Liu, C., Meng, L., Li, H., Li, R., et al. (2021). Spore production in the solid-state fermentation of stevia residue by Trichoderma guizhouense and its effects on corn growth. J. Integr. Agr. 20, 1147–1156. doi: 10.1016/S2095-3119(20)63478-5
Liu, Q., Meng, X., Li, T., Raza, W., Liu, D., Shen, Q. (2020). The growth promotion of peppers (Capsicum annuum L.) by Trichoderma guizhouense NJAU4742-based biological organic fertilizer: Possible role of increasing nutrient availabilities. Microorganisms 8, 1296. doi: 10.3390/microorganisms8091296
Liu, W., Wang, J., Wang, C., Ma, G., Wei, Q., Lu, H., et al. (2018). Root growth, water and nitrogen use efficiencies in winter wheat under different irrigation and nitrogen regimes in north China plain. Front. Plant Sci. 9. doi: 10.3389/fpls.2018.01798
Lu, D., Li, C., Sokolwski, E., Magen, H., Chen, X., Wang, H., et al. (2017). Crop yield and soil available potassium changes as affected by potassium rate in rice–wheat systems. Field Crops Res. 214, 38–44. doi: 10.1016/j.fcr.2017.08.025
Lu, C., Wang, H., Chen, H., Yuan, L., Ma, J., Shi, Y., et al. (2018). Effects of N fertilization and maize straw on the transformation and fate of labeled (15NH4)2SO4 among three continuous crop cultivations. Agric. Water Manage. 208, 275–283. doi: 10.1016/j.agwat.2018.06.031
Nazar, R., Iqbal, N., Syeed, S., Khan, N. A. (2011). Salicylic acid alleviates decreases in photosynthesis under salt stress by enhancing nitrogen and sulfur assimilation and antioxidant metabolism differentially in two mungbean cultivars. J. Plant Physiol. 168, 807–815. doi: 10.1016/j.jplph.2010.11.001
Nikalje, G. C., Srivastava, A. K., Pandey, G. K., Suprasanna, P. (2017). Halophytes in biosaline agriculture: Mechanism, utilization, and value addition. Land. Degrad. Dev. 29, 1081–1095. doi: 10.1002/ldr.2819
Oljira, A. M., Hussain, T., Waghmode, T. R., Zhao, H., Sun, H., Liu, X., et al. (2020). Trichoderma enhances net photosynthesis, water use efficiency, and growth of wheat (Triticum aestivum L.) under salt stress. Microorganisms 8, 1565. doi: 10.3390/microorganisms8101565
Ortega-García, J. G., Montes-Belmont, R., Rodríguez-Monroy, M., Ramírez-Trujillo, J. A., Suárez–Rodríguez, R., Sepúlveda -Jiménez , G. (2015). Effect of Trichoderma asperellum applications and mineral fertilizationon growth promotion and the content of phenolic compounds and flavonoids in onions. Sci. Hortic. 195, 8–16. doi: 10.1016/j.scienta.2015.08.027
Pang, G., Cai, F., Li, R., Zhao, Z., Li, R., Gu, X., et al. (2017). Trichoderma-enriched organic fertilizer can mitigate microbiome degeneration of monocropped soil to maintain better plant growth. Plant Soil 416, 181–192. doi: 10.1007/s11104-017-3178-0
Papavizas, G. C., Lumsden, R. D. (1982). Improved medium for isolation of Trichoderma spp. from soil. Plant Dis. 66, 1019–1020. doi: 10.1094/PD-66-1019
Qiao, C., Penton, C. R., Xiong, W., Liu, C., Wang, R., Liu, Z., et al. (2019). Reshaping the rhizosphere microbiome by bio-organic amendment to enhance crop yield in a maize-cabbage rotation system. Appl. Soil Ecol. 142, 136–146. doi: 10.1016/j.apsoil.2019.04.014
Qin, P., Han, R., Zhou, M., Zhang, H., Fan, L., Seliskar, D. M., et al. (2015). Ecological engineering through the biosecure introduction of Kosteletzkya virginica (seashore mallow) to saline lands in China: A review of 20 years of activity. Ecol. Eng. 74, 174–186. doi: 10.1016/j.ecoleng.2014.10.021
Rozema, J., Schat, H. (2013). Salt tolerance of halophytes, research questions reviewed in the perspective of saline agriculture. Environ. Exp. Bot. 92, 83–95. doi: 10.1016/j.envexpbot.2012.08.004
Strasser, R. J., Tsimilli-Michael, M., Qiang, S., Goltsev, V. (2010). Simultaneous in vivo recording of prompt and delayed fluorescence and 820 nm reflection changes during drying and after rehydration of the resurrection plant Haberlea rhodopensis. Biochim. Biophys. Acta 1797, 122–122. doi: 10.1016/j.bbabio.2010.04.365
Takahashi, S., Murata, N. (2008). How do environmental stresses accelerate photoinhibition? Trends Plant Sci. 13, 178–182. doi: 10.1016/j.tplants.2008.01.005
Tang, Q., Ti, C., Xia, L., Xia, Y., Wei, Z., Yan, X. (2019). Ecosystem services of partial organic substitution for chemical fertilizer in a peri-urban zone in China. J. Clean Prod. 224, 779–788. doi: 10.1016/j.jclepro.2019.03.201
Tcherkez, G. (2011). Natural 15N/14N isotope composition in C3 leaves: are enzymatic isotope effects informative for predicting the 15N-abundance in key metabolites? Funct. Plant Biol. 38, 1–12. doi: 10.1071/fp10091
Wang, X., Xu, S., Wu, S., Feng, S., Bai, Z., Zhuang, G., et al. (2018). Effect of Trichoderma viride biofertilizer on ammonia volatilization from an alkaline soil in Northern China. J. Environ. Sci. (China) 66, 199–207. doi: 10.1016/j.jes.2017.05.016
Wu, Z., Luo, J., Han, Y., Hua, Y., Guan, C., Zhang, Z. (2019). Low nitrogen enhances nitrogen use efficiency by triggering NO3– uptake and its long-distance translocation. J. Agric. Food Chem. 67, 6736–6747. doi: 10.1021/acs.jafc.9b02491
Xiao, L., Yuan, G., Feng, L., Bi, D., Wei, J. (2020). Soil properties and the growth of wheat (Triticum aestivum L.) and maize (Zea mays L.) in response to reed (Phragmites communis) biochar use in a salt-affected soil in the Yellow River Delta. Agric. Ecosyst. Environ. 303, 107124. doi: 10.1016/j.agee.2020.107124
Xue, L., Sun, B., Yang, Y., Jin, B., Zhuang, G., Bai, Z., et al. (2021). Efficiency and mechanism of reducing ammonia volatilization in alkaline farmland soil using Bacillus amyloliquefaciens biofertilizer. Environ. Res. 202, 111672. doi: 10.1016/j.envres.2021.111672
Yan, K., He, W., Bian, L., Zhang, Z., Tang, X., An, M., et al. (2020). Salt adaptability in a halophytic soybean (Glycine soja) involves photosystems coordination. BMC Plant Biol. 20, 155. doi: 10.1186/s12870-020-02371-x
Yan, K., Shao, H. B., Shao, C. Y., Chen, P., Zhao, S. J., Brestic, M., et al. (2013). Physiological adaptive mechanisms of plants grown in saline soil and implications for sustainable saline agriculture in coastal zone. Acta Physiol. Plant 35, 2867–2878. doi: 10.1007/s11738-013-1325-7
Yao, R., Li, H., Yang, J., Yin, C., Wang, X., Xie, W., et al. (2021). Interactive effects of amendment materials and soil salinity on net rates of urea hydrolysis and nitrification in salt-affected soil. J. Soil Sci. Plant Nutr. 21, 3414–3427. doi: 10.1007/s42729-021-00616-8
Ye, L., Zhao, X., Bao, E., Li, J., Zou, Z., Cao, K. (2020). Bio-organic fertilizer with reduced rates of chemical fertilization improves soil fertility and enhances tomato yield and quality. Sci. Rep. 10, 177. doi: 10.1038/s41598-019-56954-2
Yousfi, S., Serret, M. D., Araus, J. L. (2009). Shoot δ15N gives a better indication than ion concentration or Δ13C of genotypic differences in the response of durum wheat to salinity. Funct. Plant Biol. 36, 144–155. doi: 10.1071/fp08135
Yousfi, S., Serret, M. D., Marquez, A. J., Voltas, J., Araus, J. L. (2012). Combined use of δ13C, δ18O and δ15N tracks nitrogen metabolism and genotypic adaptation of durum wheat to salinity and water deficit. New Phytol. 194, 230–244. doi: 10.1111/j.1469-8137.2011.04036.x
Yu, Y., Xu, T., Li, X., Tang, J., Ma, D., Li, Z., et al. (2016). NaCl-induced changes of ion homeostasis and nitrogen metabolism in two sweet potato (Ipomoea batatas L.) cultivars exhibit different salt tolerance at adventitious root stage. Environ. Exp. Bot. 129, 23–36. doi: 10.1016/j.envexpbot.2015.12.006
Yuan, L., Chen, X., Jia, J., Chen, H., Shi, Y., Ma, J., et al. (2021). Stover mulching and inhibitor application maintain crop yield and decrease fertilizer N input and losses in no-till cropping systems in Northeast China. Agric. Ecosyst. Environ. 312, 107360. doi: 10.1016/j.agee.2021.107360
Zhang, S., Gan, Y., Xu, B. (2016). Application of plant-growth-promoting fungi Trichoderma longibrachiatum T6 enhances tolerance of wheat to salt stress through improvement of antioxidative defense system and gene expression. Front. Plant Sci. 7, 1405. doi: 10.3389/fpls.2016.01405
Zhang, S., Gan, Y., Xu, B. (2019b). Mechanisms of the IAA and ACC-deaminase producing strain of Trichoderma longibrachiatum T6 in enhancing wheat seedling tolerance to NaCl stress. BMC Plant Biol. 19, 22. doi: 10.1186/s12870-018-1618-5
Zhang, F., Huo, Y., Xu, X., Hu, J., Sun, X., Xiao, Y., et al. (2018). Trichoderma improves the growth of Leymus chinensis. Biol. Fer. Soils 54, 685–696. doi: 10.1007/s00374-018-1292-7
Zhang, C., Li, X. B., Kang, Y. H., Wahba, M. A. S. (2021). Leaching efficiency and plant growth response in an integrated use of saline water for coastal saline soil reclamation. Land. Degrad. Dev. 32, 4595–4608. doi: 10.1002/ldr.4058
Zhang, F., Wang, Y., Liu, C., Chen, F., Ge, H., Tian, F., et al. (2019a). Trichoderma harzianum mitigates salt stress in cucumber via multiple responses. Ecotoxicol. Environ. Saf. 170, 436–445. doi: 10.1016/j.ecoenv.2018.11.084
Zhang, F., Xu, X., Wang, G., Wu, B., Xiao, Y. (2020). Medicago sativa and soil microbiome responses to Trichoderma as a biofertilizer in alkaline-saline soils. Appl. Soil Ecol. 153, 103573. doi: 10.1016/j.apsoil.2020.103573
Zhao, C., Zhang, H., Song, C., Zhu, J., Shabala, S. (2020). Mechanisms of plant responses and adaptation to soil salinity. Innovation 1, 100017. doi: 10.1016/j.xinn.2020.100017
Zhou, J., Li, B., Xia, L., Fan, C., Xiong, Z. (2019). Organic-substitute strategies reduced carbon and reactive nitrogen footprints and gained net ecosystem economic benefit for intensive vegetable production. J. Clean Prod. 225, 984–994. doi: 10.1016/j.jclepro.2019.03.191
Zhu, W., Yang, J., Yao, R., Wang, X., Xie, W., Li, P. (2021). Nitrate leaching and NH3 volatilization during soil reclamation in the Yellow River Delta, China. Environ. pollut. 286, 117330. doi: 10.1016/j.envpol.2021.117330
Keywords: nitrogen, photosynthesis, stable isotopic compositions, Trichoderma, wolfberry
Citation: Yan K, Mei H, Ruan Y, Yu S, Su H, Zhi Y, Li S and Sun Y (2023) Partial substitution of chemical fertilizer by Trichoderma biofertilizer improved nitrogen use efficiency in wolfberry (Lycium chinense) in coastal saline land. Front. Plant Sci. 14:1225028. doi: 10.3389/fpls.2023.1225028
Received: 18 May 2023; Accepted: 22 September 2023;
Published: 09 October 2023.
Edited by:
Silvana Scalon, Federal University of Grande Dourados, BrazilReviewed by:
Markus Weinmann, University of Hohenheim, GermanyKailou Liu, Jiangxi Institute of Red Soil, China
Copyright © 2023 Yan, Mei, Ruan, Yu, Su, Zhi, Li and Sun. This is an open-access article distributed under the terms of the Creative Commons Attribution License (CC BY). The use, distribution or reproduction in other forums is permitted, provided the original author(s) and the copyright owner(s) are credited and that the original publication in this journal is cited, in accordance with accepted academic practice. No use, distribution or reproduction is permitted which does not comply with these terms.
*Correspondence: Kun Yan, kyan@ldu.edu.cn; yankunacademic@163.com