- 1Citrus Research Institute, Southwest University/National Citrus Engineering Research Center, Chongqing, China
- 2State Key Laboratory for Biology of Plant Diseases and Insect Pests, Chinese Academy of Agriculture Sciences, Institute of Plant Protection, Beijing, China
- 3Guangxi Citrus Breeding and Cultivation Engineering Technology Center Academy of Specialty Crops, Guangxi, Guilin, China
Citrus Huanglongbing (HLB) is the most destructive citrus disease worldwide, mainly caused by ‘Candidatus Liberibacter asiaticus’ (CLas). It encodes a large number of Sec-dependent effectors that contribute to HLB progression. In this study, an elicitor triggering ROS burst and cell death in Nicotiana benthamiana, CLIBASIA_04425 (CLas4425), was identified. Of particular interest, its cell death-inducing activity is associated with its subcellular localization and the cytoplasmic receptor Botrytis-induced kinase 1 (BIK1). Compared with CLas infected psyllids, CLas4425 showed higher expression level in planta. The transient expression of CLas4425 in N. benthamiana and its overexpression in Citrus sinensis enhanced plant susceptibility to Pseudomonas syringae pv. tomato DC3000 ΔhopQ1-1 and CLas, respectively. Furthermore, the salicylic acid (SA) level along with the expression of genes NPR1/EDS1/NDR1/PRs in SA signal transduction was repressed in CLas4425 transgenic citrus plants. Taken together, CLas4425 is a virulence factor that promotes CLas proliferation, likely by interfering with SA-mediated plant immunity. The results obtained facilitate our understanding of CLas pathogenesis.
Introduction
Citrus Huanglongbing (HLB), the most devastating disease of citrus, has been known in East Asia for over a century (Reinking, 1919; Lin, 1956). Diseased trees have leaves with a blotchy mottle, and the branches gradually die as the disease progresses (Martinelli and Dandekar, 2017). It has occurred in ca. 50 countries throughout the world and over 300 counties of 10 provinces in the mainland of China (Zhou, 2020). Even though the fight against HLB has never stopped in China, the disease still causes tremendous losses to the citrus industry. Since HLB arrived in 2005, citrus production in Florida has decreased by 74% (Singerman and Rogers, 2020). The causal agents of HLB are three Candidatus Liberibacter species bacteria, named for their geographic distributions: ‘Ca. Liberibacter asiaticus’ (CLas), ‘Ca. Liberibacter africanus’ (CLaf), and ‘Ca. Liberibacter americanus’ (CLam) (Bové, 2006). Realizing the disease severity, researchers have made tremendous efforts to uncover the pathosystem of the HLB causal agents. However, their obligate and phloem-limited characteristics hamper mechanistic studies of the pathogenicity (Perilla-Henao and Casteel, 2016). Among three Liberibacter species, CLas is the most prevalent strain and thus is commonly studied. Exploring the battle strategies between CLas and citrus plants are critical for the establishment of control remedies.
A ‘zig-zag’ model has been used to clarifiy the plant-pathogen interaction (Jones and Dangl, 2006). In this theory, pathogens generally utilize pathogen-associated molecular patterns (PAMPs) and effectors to attack the host. CLas has been observed to stimulate non-self-recognition immune responses in planta, including phloem blockage, increased levels of salicylic acid, and production of reactive oxygen species (ROS) (Wang and Trivedi, 2013). Nevertheless, a molecular understanding of the process remains undiscovered. Suppression or avoidance of plant immunity is necessary for successful pathogen colonization. Transmitted by Diaphorina citri Kuwayama (ACP) into phloem elements, CLas evades recognition by plant outer membrane receptors. Effectors serve as an important component of pathogens, playing critical roles in pathogenesis. Lacking common type III and IV secretion systems (T3SS and T4SS), CLas delivers effectors via general secretion pathway (Sec), T1SS, and noncanonical secretion pathway (Andrade et al., 2020). Among them, Sec-dependent effectors are mostly investigated (Prasad et al., 2016). Transient expression of CLas effectors in Nicotiana benthamiana is used for large-scale screening of virulence factors, and many are identified to affect plant innate immunity (Pitino et al., 2016; Du P. et al., 2021).
Hypersensitive response (HR)-based cell death is a common consequence of pathogen recognition by the plant immune system. To date, CLIBASIA_05315 (SDE1), CLIBASIA_05150, and AGH17470 have been shown to stimulate HR, including ROS burst and programmed cell death (Pitino et al., 2016; Ying et al., 2019; Du et al., 2022). Interacting with the papain-like cysteine proteases (PLCPs), SDE1 contributes to HLB progression via attenuating PLCPs activity (Clark et al., 2018; Clark et al., 2020). By contrast, many effectors are determined to block HR induced by two common elicitors, pro-apoptotic mouse protein BCL2-associated X protein (BAX) and Phytophthora infestans elicitin inverted formin 1 (INF1) (Zhang et al., 2019; Pang et al., 2020). SDE15 suppression of plant immunity is dependent on the citrus protein accelerated cell death 2 (ACD2). Moreover, CLIBASIA_05330 can even suppress SDE1-triggered HR (Shen et al., 2022). Intriguingly, the virulence of CLIBASIA_00460 relies on temperature, which affects its pathogenicity via interfering with subcellular localization (Liu et al., 2019).
Here, we investigate a CLas Sec-dependent effector CLIBASIA_04425 (CLas4425) that can also trigger cell death by transient expression in N. benthamiana. Additionally, subcellular localization and the cytoplasmic receptor Botrytis-induced kinase 1 (BIK1) are indispensable for its cell death-inducing activity. CLas4425-expressing N. benthamiana and citrus plants are more vulnerable to pathogen infection. Molecular evidence showed CLas4425 impairs the salicylic acid (SA) signaling pathway. Accordingly, we conclude that CLas4425 is a virulence effector of CLas and can be perceived by the plant immune system.
Materials and methods
Plants, bacteria, and growth conditions
The seedlings of N. benthamiana were grown for 5-6 weeks at 25°C in a greenhouse with 18 h light/6 h darkness. The citrus plants were grown at 28°C. CLas-infected sugar orange plants (Citrus reticulata) were used for DNA extraction, as well as a source for graft-inoculation. The epicotyl segments of Wanjincheng (C. sinesis) were used for gene transformations. Pseudomonas syringae pv. tomato DC3000 (DC3000) ΔhopQ1-1 was grown on King’s B (KB) medium.
Nucleotide extraction and detection analysis
For CLas detection, total DNA was extracted from midrib sections of sugar orange plants using Biospin Omini Plant Genomic DNA Extraction Kit (BioFlux, Hangzhou, China). The CLas detection was conducted by both PCR and quantitative PCR (qPCR). The PCR was performed using 16S rDNA primer OI1/OI2c with 2X Taq Master Mix (Vazyme, Nanjing, China) in a 20 μL reaction system. The bacterial populations (CLas cells μg-1 of citrus DNA) were quantified with qPCR assay that was described by Du M. et al. (2021). In the qPCR assay, CLasgyrA of CLas was detected, and 18S rRNA of citrus was used as the internal reference. BlastTaq™ 2X qPCR MasterMix (abm, Canada) was used for qPCR amplification. Primer pairs are listed in Table S1.
Total RNA was extracted from plant tissues and psyllids using RNAiso Plus (Takara, Japan). In a 20-μL volume, 1μg of total RNA was reverse transcribed with All-In-One 5X RT MasterMix (abm) following the manufacturer’s instructions. Quantitative RT-PCR (qRT-PCR) assays were conducted to analyze gene transcript level. Primer pairs of selected genes are listed in Table S1. The genes NbACTIN and CsGAPDH were used as endogenous controls. The qRT-PCR analyses were performed in a 10 μL reaction system. The 2-ΔΔCt method was used for the determination of relative gene transcription (Livak and Schmittgen, 2001).
Plasmid constructs and preparation
Primer pairs and vectors used are listed in Table S1. Fragments were amplified by PCR by using Q5 high-fidelity DNA polymerase and digested with appropriated restriction endonucleases (NEB, Peking, China), followed by ligation into vectors using ClonExpress II One Step Cloning Kit (Vazyme). For phoA assay, the fragment of CLas4425 signal peptide (4425SP) was inserted into pET-mphoA (T7 promoter). For the transient expression assay, the coding sequences were cloned into the binary Potato virus X (PVX) vector pGR107. To construct the overexpression vector, CLas4425 was ligated into pLGN (35S promoter). For the VIGS assay, fragments amplified from N. benthamiana cDNA were cloned into the binary vector pTRV2. All constructs were sequenced by Tsingke (Chongqing, China).
Alkaline phosphatase assay
The resulting vector pET-4425SP-mphoA, pET-phoA (positive control), and pET-mphoA (negative control) were transformed into Escherichia coli Rosetta-gami2 (DE3), then the E. coli cells were incubated at 37°C overnight in indicator LB solid medium [90 ng/mL 5-bromo-4-chloro-3-indolyl phosphate (BCIP), 0.1 mM isopropyl-β-D-thiogalactopyranoside (IPTG), 75 μM Na2HPO4, and 50 μg/mL Kanamycin]. The blue transformants indicated that fusion proteins were secreted outside the cells, while white colonies demonstrated a lack of phoA activity.
Agrobacterium tumefaciens-mediated transient expression
A. tumefaciens GV3101 (pJIC SA_Rep) strains harboring the respective constructs were cultured in LB medium supplemented with the appropriate antibiotics at 28°C, and then harvested and suspended in infiltration buffer [10 mM 2-(N-morpholine)-ethane sulfonic acid (MES), 10 mM MgCl2, 0.2 mM acetosyringone to pH 5.6] to appropriate concentrations (OD600 of 0.2 for INF1, BAX, otherwise OD600 of 0.6 was used). After dark incubation for 2 h at 28°C, the A. tumefaciens suspensions were infiltrated into the upper leaves of 6-leaf-old N. benthamiana plants with needless syringes. Cell death was observed at 5-8 dpi. For the gene expression analysis, 0.1 mg of samples was detached at 2, 4, 6, and 8 dpi, respectively. The collected samples were quickly frozen in liquid nitrogen and stored in -80°C.
3, 3’-diaminobenzidine and trypan blue staining
H2O2 accumulation was detected using DAB staining. The N. benthamiana leaves were detached at 3 dpi and incubated in DAB solution (1 mg/mL DAB, 1:2000 Tween-20, and 10 mM Na2HPO4), then vacuumed until the leaves were infiltrated with DAB solution (Du et al., 2022). The sample leaves were placed on a shaker at 60 rpm overnight, then distained in 95% ethanol and photographed.
For programmed cell death (PCD) observation, infiltrated leaves were incubated in trypan blue staining solution (0.067% w/v trypam blue, 11.11% w/v phenol, 1 volume of glycerol, lactic acid and distilled water, respectively, and 6 volume of 95% ethanol), boiled for 2 min, then transferred into chloral hydrate (1.25 g/mL). The sample leaves were places on a shaker at 60 rpm until they were fully destained (Shen et al., 2022).
Subcellular localization of CLas4425 in plant cells
The mature form of CLas4425 fused with red fluorescent protein (RFP) was cloned into PVX. For its entrance into the nuclei, the nuclear localization signal (NLS) was added at N-terminus of CLas4425:RFP. Leaves of N. benthamiana were collected at 3 dpi. Subcellular localization of CLas4425 was determined using an FV3000 confocal equipped with a UV light source (Olympus, Tokyo, Japan). H2B:green fluorescent protein (GFP) and Pm : GFP were used as markers for the nucleus and cytoplasmic membrane, respectively.
Virus-induced gene silencing assay in N. benthamiana
The TRV-based gene silencing system was conducted to silence BAK1, SOBIR1, and BIK1 in N. benthamiana. A. tumefaciens GV3101 strains carrying respective pTRV2 constructs were mixed with pTRV1 in equal ratios to a final OD600 of 0.2. pTRV2:GUS served as a control, and pTRV2:PDS was used to visualize the silencing process (Liu et al., 2002). A. tumefaciens suspensions were infiltrated into cotyledons of 4-leaf-old seedlings. The silencing efficiency was validated with qRT-PCR of RNA from the leaves in the locations corresponding to the albino leaves.
Immunoblotting
Protein was extracted from infiltrated leaves using the Plant Protein Extraction Kit (Solarbio, Peking, China). Total protein was separated by 12% SDS-PAGE, and the protein samples were transferred to a polyvinlidene difluoride (PVDF) membrane. Anti-HA-tag served as primary monoclonal antibody at a dilution of 1:2000. HRP-conjugated goat anti-mouse IgG was used as secondary antibody at a dilution of 1:5000.
Citrus transformation
Epicotyl segments of Wanjincheng seedlings were used for A. tumefaciens-mediated transformation. The details of performance were as previously described (Peng et al., 2015). Transgenic shoots were determined by GUS staining before being micrografted onto Wanjingcheng seedlings in vitro. The resulting plantlets were further grafted onto C. limon in a greenhouse. The expression levels of CLas4425 in transgenic plants were validated with qRT-PCR.
Pathogen inoculation
To analyze the effect of CLas4425 on N. benthamiana immunity, N. benthamiana plants were infiltrated with CLas4425, NLS : CLas4425, and GFP, respectively one day before DC3000 ΔhopQ1-1 inoculation. DC3000 was prepared with OD600 = 0.0001 (104 cfu/mL) and injected into six N. benthamiana plants for each treatment. Leaves were detached at 3 dpi and used for symptom observation and bacterial growth analysis.
To determine the role of CLas4425in HLB pathogenicity, five independent CLas4425-overexpressed lines were used via a grafting method. WT Wanjingcheng plants of the same age were used as controls. Branches from each of these plants were inoculated by stems of CLas-infected sugar orange plants, with the presence of CLas confirmed by PCR. Leaf samples were collected monthly, and midribs from three mature leaves per plant were used for DNA extraction and the determination of CLas populations.
H2O2 measurement
Quantification of H2O2 production was performed using Hydrogen Peroxide (H2O2) Content Assay Kit (Molfarming, Nanjing, China), and the OD was measured using Varioskan Flash Microplate Reader (Thermo Scientific, China). Fresh tissues were collected from fully mature leaves and finely ground with liquid nitrogen, and H2O2 accumulation was assayed following the manufacturer’s instructions. The experiment comprised three biological replicates and was repeated three times.
Results
CLas4425 is a Sec-dependent effector and expressed mainly in planta
An in silico analysis suggested CLas4425 contained a 19 amino signal peptide in the N-terminal (Figure 1A). Accordingly, an alkaline phosphatase (phoA) fusion assay was employed to validate the export of CLas4425 via the Sec translocon (Liu et al., 2019). After overnight incubation, E. coli cells harboring pET-4425SP-mphoA turned blue on indicator LB medium as the positive control, whereas the negative control remained white (Figure 1B). Based on these data, CLas4425 was identified as a typical Sec-delivered effector.
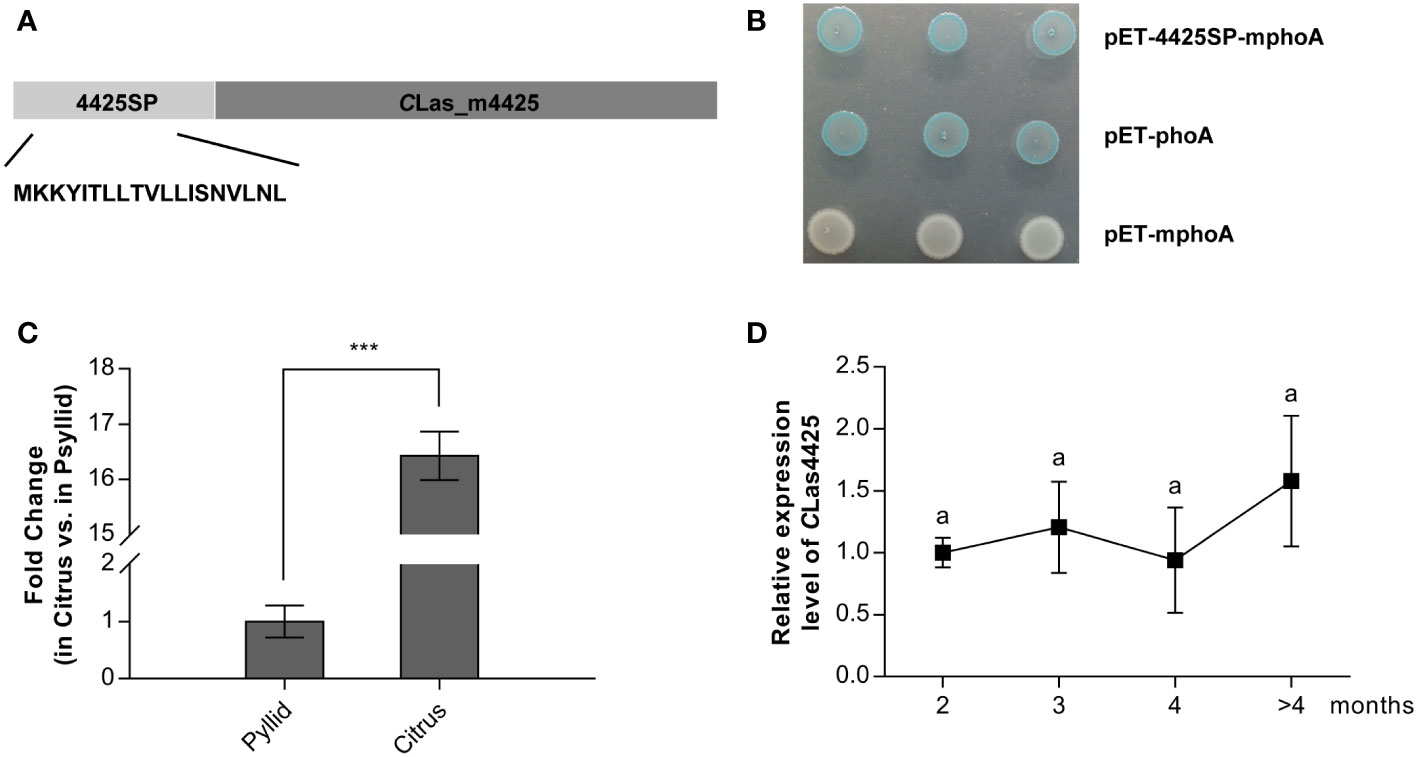
Figure 1 The secretion and expression analysis of CLas4425. (A), Schematic of the CLas4425 protein. In silico analysis revealed a signal peptide at the N-terminus of CLas4425, 19 amino acids in length. 4425SP, the signal peptide of CLas4425; CLas_m4425, the mature form of CLas4425. (B) Alkaline phosphatase fusion assay confirmed the secretion of CLas4425. The Escherichia coli cells harboring the recombinant plasmids were incubated on Luria-Bertani (LB) plates amended with 5-bromo-4-chloro-3-indolyphosphate (BCIP) and sodium phosphate. As the negative control, pET-mphoA did not cause color change, while pET-phoA (positive control) and the recombinant turned to blue. (C), Transcript levels of CLas4425 in psyllid and citrus. Transcript levels analyzed with qRT-PCR were normalized to levels in psyllid using CLasgyrA (GenBank no. CP001677.5) as the internal reference. Means and standard errors from three biological replicates are shown. Asterisks reveal significant differences based on Student’s t-test (***p<0.001, n=3). (D), Monitoring CLas4425 transcription in CLas-graft inoculated Wanjincheng (C. sinesis), measured using qRT-PCR and normalized to the level of CLas4425 at 2 months after CLas successful colonization using CLasgyrA as the internal reference. Means and standard errors from three biological replicates are shown. Letters exhibit the differences as measured using Fisher’s LSD test (p=0.05, n=3).
To explore the importance of CLas4425 to CLas pathogenicity, we investigated its expression profiles in CLas-infected citrus and psyllids, as well as during the disease process. As a result, CLas4425 was highly expressed (ca. 16-fold) in infected citrus plants compared to that in psyllids (Figure 1C). In addition, CLas4425 could be detected at 2 months after CLas colonization, and its expression level stayed the same during disease development (Figure 1D). Our findings imply that CLas4425 plays a critical role in planta at early infection stage.
CLas4425-triggered cell death in N. benthamiana is dependent on subcellular localization and the cytoplasmic receptor BIK1
A transient expression system was applied to assess the potential role of CLas4425 in CLas virulence. The coding sequence of CLas4425, without the secretion signal, was cloned into the binary PVX vector pGR107. PVX-GFP and PVX-BAX served as negative and positive control, respectively. Compared to BAX, CLas4425 induced mild cell death symptom (Figure 2A). The progression, in which CLas4425-triggered cell death happened, was recorded (Figures S1A, B). To avoid the influence of PVX, we sequentially constructed pLGN-CLas4425. Cell death was also observed in CLas4425-infiltrated leaves (Figure S1C).
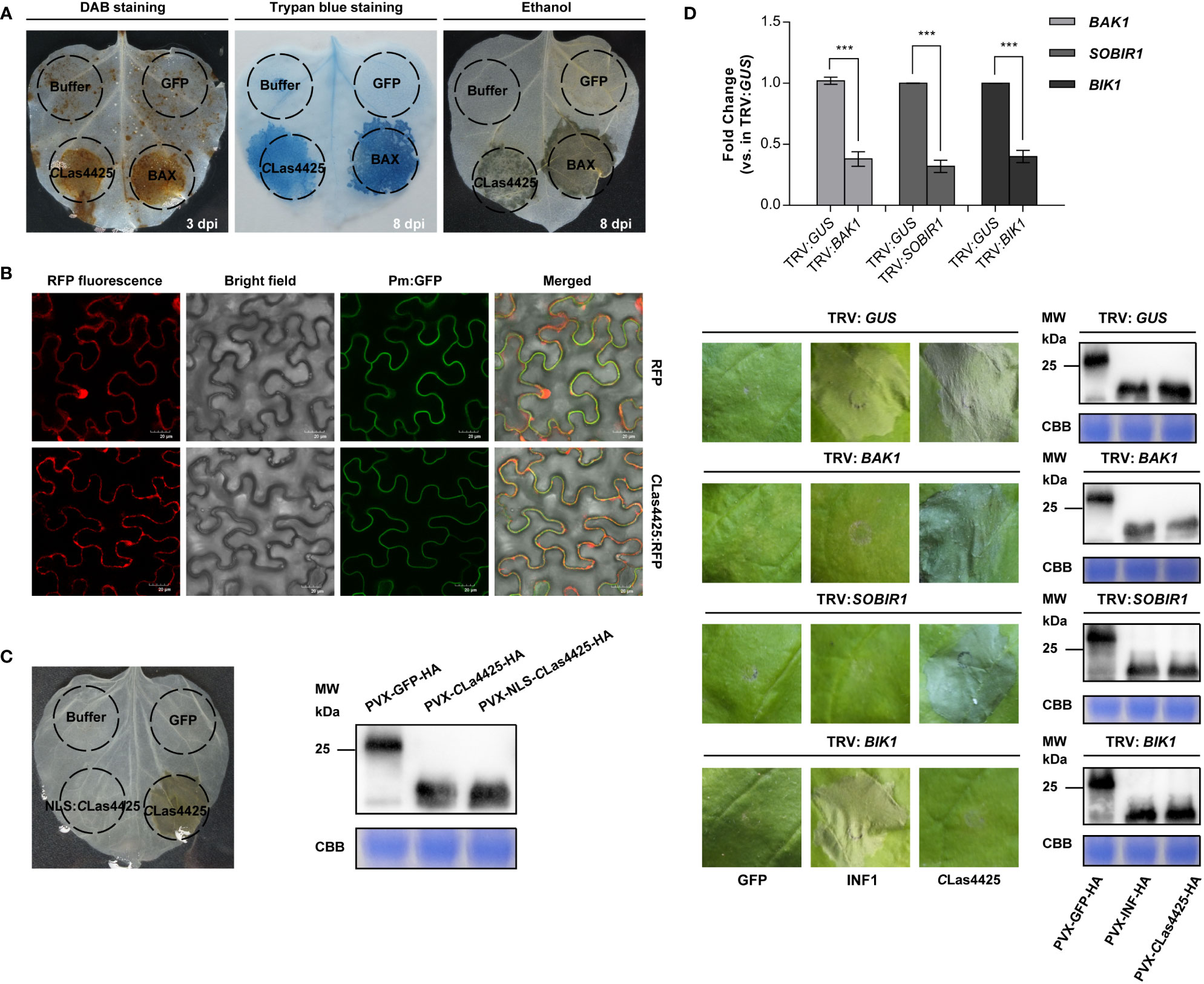
Figure 2 Analysis of impact factors in determination of CLas4425-triggered hypersensitive response (HR) in leaves of Nicotiana benthamiana. (A), Programmed cell death and ROS burst induced by CLas4425. Leaves were infiltrated with inoculation buffer, PVX-GFP, PVX-BAX, and PVX-CLas4425. To visualize ROS burst, leaves were detached at 3 dpi for 3, 3’-diaminobenzidine (DAB) staining. The leaves for cell death observation were photographed at 8 days’ post inoculation (dpi) after trypan blue staining and ethanol decolorization. The experiment had three independent biological replicates and was repeated three times. (B), Subcellular localization analysis. CLas4425 fused with RFP, the signals were detected at 3 dpi using a confocal laser scanning microscope. RFP was used as the location control while Pm : GFP was used as plasma membrane location marker. Scale bar: 20 μm. The experiment was repeated three times. (C), Subcellular localization is required for CLas4425-triggered cell death. Corresponding proteins were expressed in N. benthamiana to assay triggering of cell death. Symptoms were photographed at 8 dpi after ethanol decolorization. Immunoblot of proteins from infiltrated leaves transiently expressing the indicated proteins from a PVX-3*HA vector. NLS, nuclear localization signal. (D), CLas4425-induced cell death relies on a cytoplasmic receptor, BIK1. N. benthamiana plants were subjected to virus-induced gene silencing (VIGS) by inoculation with TRV : GUS, TRV : BAK1, TRV : SOBIR1, and TRV : BIK1, respectively. The expression levels of silenced genes after VIGS treatment were determined by qRT-PCR analysis using the NbACTIN endogenous control (Student’s t-test, ***p<0.001, n=6). Three weeks after inoculation, GFP, INF1, and CLas4425 were transiently expressed in the gene-silenced N. benthamiana, and then leaves were photographed at 8 dpi. Immunoplot analysis of GFP, INF1, and CLas4425 protein fused with 3*HA tags transiently expressed in VIGS-silenced N. benthamiana plants. The experiment was performed three times with six plants for each TRV construct.
Protein functions are correlated with their subcellular localization. To visualize the distribution of CLas4425 in plant cells, we transiently expressed RFP and CLas4425:RFP in N. benthamiana leaves, and observed their localization pattern with a confocal microscope. Free RFP was found to be distributed in multiple subcellular compartments (Figures 2B; S2), while CLas4425:RFP was localized in the cytoplasm and cytoplasmic membrane (Figure 2B). To reveal the role of subcellular localization involved in CLas4425-triggered cell death, the nuclear localization sequence (NLS) (Liu et al., 2019) was fused in frame to CLas4425 in PVX (Figure S2). Transient expression illustrated that NLS : CLas4425 could not induce cell death, and the protein expression was validated (Figure 2C). Therefore, localization in cytoplasm and cytoplasmic membrane is required for CLas4425-activated cell death.
Brassinosteroid insensitive 1-associated kinase 1 (BAK1), a suppressor of BIR1-1 (SOBIR1), and BIK1 participate in several types of PTI signaling pathways (Liebrand et al., 2014; Kadota et al., 2015). Moreover, BIK1 is necessary for bacterial resistance during ETI (Yuan et al., 2021). As a PAMP, INF1-induced cell death relies on BAK1 and SOBIR1 (Heese et al., 2007; Franco-Orozco et al., 2017). Here, we expressed CLas4425 in N. benthamiana silenced for BAK1, SOBIR1, and BIK1. The silencing of these genes was verified using qRT-PCR (p<0.001) (Figure 2D). CLas4425-triggered cell death developed in the TRV : BAK1, TRV : SOBIR1, and TRV : GUS treated plants. On the contrary, cell death was abolished in BIK1-silenced N. benthamiana plants. However, INF1-activated cell death was not altered in TRV : BIK1-treated plants. Immunoblot verified that GFP, INF1, and CLas4425 were correctly expressed in N. benthamiana. Conclusively, CLas4425-elicited cell death is dependent on BIK1.
CLas4425 hinders plant defense responses in N. benthamiana
DC3000 ΔhopQ1-1 was used to determine the virulence of CLas4425. We inoculated N. benthamiana leaves with DC3000 following the infiltration with either CLas4425 or NLS : CLas4425. qRT-PCR analysis revealed that the PVX accumulation level of PVX-CLas4425 made no significant difference to PVX-GFP in N. benthamiana (Figure S3A). N. benthamiana expressing CLas4425 exhibited more severe leaf blight than those infiltrated with NLS : CLas4425 and GFP (Figure 3A). Analysis of bacterial growth revealed that CLas4425 promoted DC3000 proliferation in N. benthamiana (Figure 3B). Since CLas4425 had a negative effect on N. benthamiana immunity reacting to DC3000 infection, we probed the molecular evidence for its interference with plant innate immunity. The expression of a PTI marker gene, NbWRKY29, and genes representing phytohormones were detected (Pang et al., 2020; Situ et al., 2020). The transcript of NbPR2 declined significantly from 4 dpi (Figure 3C), whereas the expression level of other detected genes did not vary dramatically (Figure S3B). Considering NbPR2 marked the SA pathway, we examined the expression manners of SA upstreaming genes, namely NbEDS1 and NbNPR1. As expected, their expressions were decreased in plants expressing CLas4425 (Figure 3D). Hence, CLas4425 probably impairs N. benthamiana defense via targeting the SA signaling pathway.
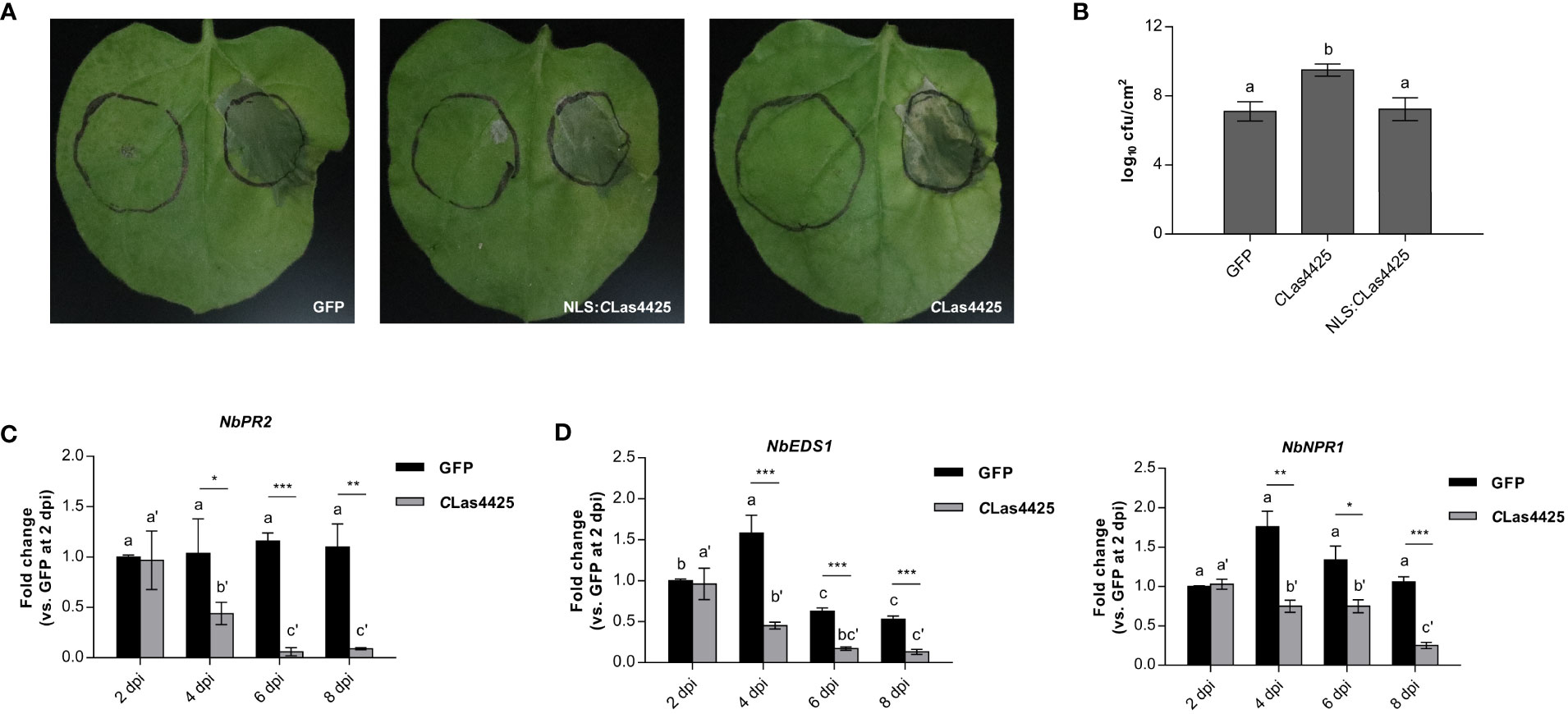
Figure 3 CLas4425 promotes the infection of Pseudomonas syringae pv. tomato (DC3000) ΔhopQ1-1 in N. benthamiana. (A), The symptoms of N. benthamiana leaves inoculated with DC3000 ΔhopQ1-1. Leaves were infiltrated with GFP, CLas4425, and NLS: CLas4425 one day before inoculation with DC3000 ΔhopQ1-1. The photographs were taken at 3 dpi. The experiment was repeated three times, and at each time six independent biological replicates were taken for agro-infiltration with corresponding plasmids. (B), Colony-forming units per square centimeter (cfu/cm2) were counted to assess the bacterial population. Significant difference was determined with Fisher’s LSD test (p=0.05, n=3). (C), qRT-PCR analysis of NbPR2 after PVX-CLas4425 infiltration compared with PVX-GFP. (D), The expression of SA upstreaming genes NbEDS1 and NbNPR1. In (C, D) the leaves were collected for RNA extraction of CLas4425 or GFP control at 2, 4, 6, and 8 dpi. The transcript levels measured with qRT-PCR were normalized to levels in GFP at 2 dpi using the NbACTIN endogenous control. The differences between CLas4425- and GFP-expressing samples were analyzed using Student’s t-test (*p<0.05, ** p<0.01, ***p<0.001, n=3). Fisher’s LSD test was used to compare gene expression within the timelines (p=0.05, n=3).
CLas4425 enhances citrus susceptibility to CLas infection
To assess the significance of CLas4425 in CLas pathogenicity, we generated CLas4425-transgenic Wanjincheng (4425-OE). The obtained transgenic plants were clarified using PCR, qRT-PCR, and GUS assay (Figure S4A), and there was no difference between 4425-OEs and wild-type group (WT) in growth and phenotype (Figure S4B). Among seven independent transgenic lines, we selected five of them with relatively higher expression of CLas4425 for further studies. The five 4425-OEs (one-year old) were graft-inoculated using CLas-infected stems. Five WTs of the same age served as a control group and were grafted in the same way. qPCR was applied monthly to monitor bacterial growth. Comparison of bacterial titers demonstrated that CLas growth was faster in 4425-OEs than in WTs at 2 (p<0.001) and 3 months post inoculation (MPI) (p<0.01), whereas no difference was observed after 4 MPI (p>0.05) (Figure 4A). Leaves from transgenic plants exhibited a blotchy mottle at 6 MPI, while WTs remained asymptomatic (Figure 4B). Previous reports documented the accumulation of starch granules and phloem blockage in infected citrus plants (Wang and Trivedi, 2013). We therefore detected the transcription of genes associated with phloem protein (CsPP2), sugar metabolism (CsAPS1), and starch biosynthesis (CsGBSS1 and CsSS1) (Kim et al., 2009). Consequently, CLas promoted the expression of all the detected genes in both WTs and 4425-OEs while CLas4425 enhanced the expression of CsPP2, CsAPS1, and CsGBSS1 in uninfected citrus plants. Compared to those in WTs infected with CLas, the expression of CsPP2 and CsAPS1 was upregulated in CLas-infected 4425-OEs (Figure 4C). To sum up, CLas4425 promotes CLas multiplication, and enhances HLB progression.
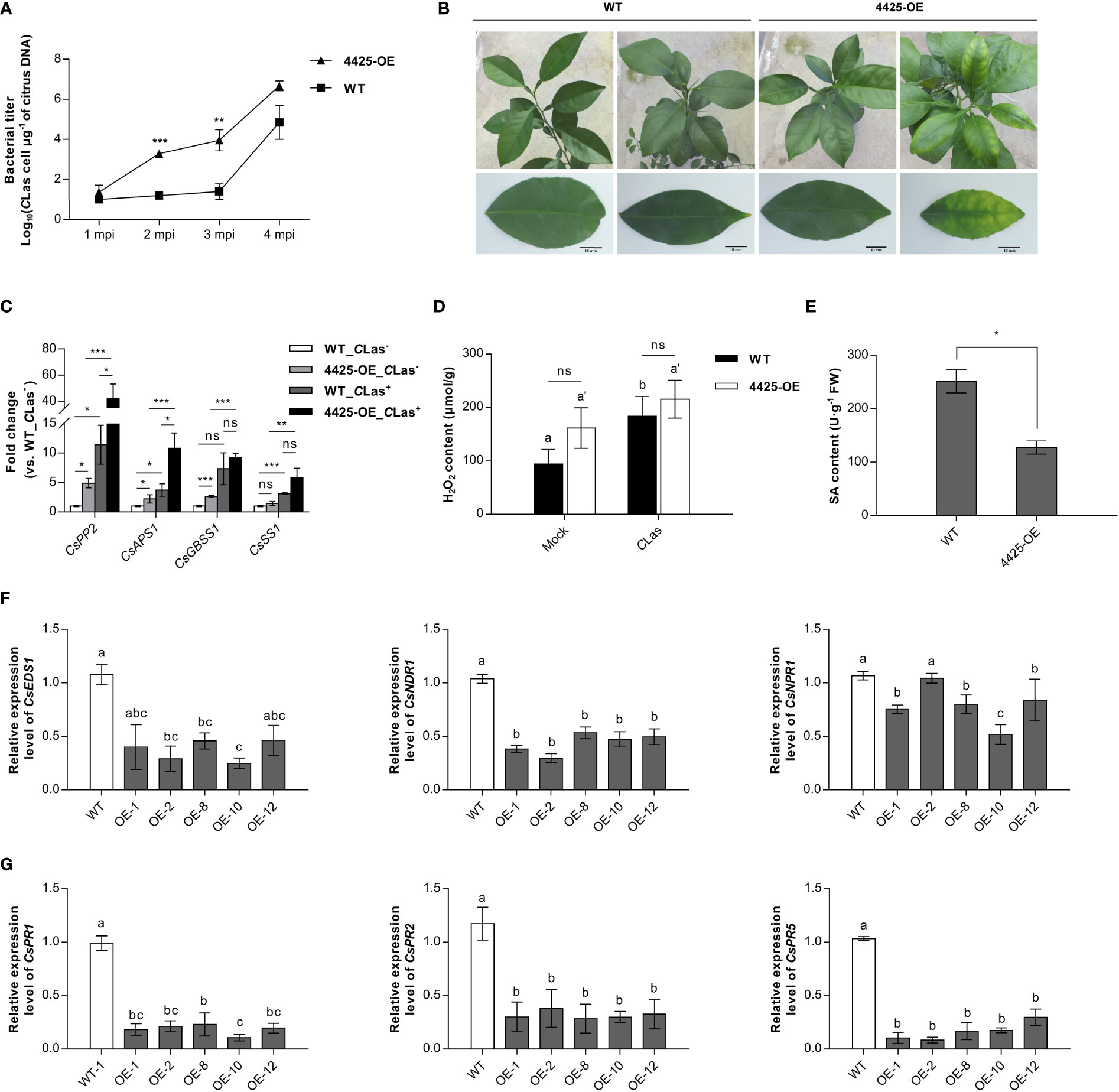
Figure 4 CLas4425 accelerates ‘Candidatus Liberibacter asiaticus’ (CLas) colonization and symptom development in the transgenic citrus plants. (A), Quantitative analysis of CLas growth during a 4-month period after graft inoculation in CLas4425 transgenic citrus lines (4425-OE) and wild-type plants (WT). Wanjincheng (C. sinesis) were used for generation of CLas4425 transgenic citrus. Wild-type Wanjincheng of the same age were used as controls. MPI=months post-inoculation. Transgenic and WT plants contained five independent biological replicates each. The bacterial populations (CLas cells μg-1 of citrus DNA) were determined using qPCR. Asterisks represent significant differences based on Student’s t-test (**p<0.01, ***p<0.001, n=5). (B), Leaves of 4425-OE lines exhibited a blotchy mottle at 6 MPI. Scale bar: 10 mm. (C), Transcript levels of genes associated with phloem blockage (CsPP2), sugar metabolism (CsAPS1), and starch granule accumulation (CsGBSS1 and CsSS1) in CLas-infected plants. Transcript levels measured with qRT-PCR were normalized to levels in CLas-infected WTs using the CsGAPDH endogenous control. The differences were analyzed using Student’s t-test (***p<0.001, n=3). (D), H2O2 accumulation in transgenic and WT plants at 6 MPI. Significant differences are based on Student’s t test (n=3). The asterisks indicate the differences between the transgenic and WT plants (ns, no significance), and the letters indicate the significant differences between the mock and CLas-infected samples (p<0.05). (E), SA accumulation in transgenic and WT plants at 6 MPI. The differences between the transgenic and WT plants were analyzed using Student’s t-test (*p<0.05, n=3). (F), Transcript levels of SA-mediated signal transduction-related genes. (G), Transcript levels of pathogenic-related (PR) genes. In (F, G), transcript levels measured with qRT-PCR were normalized to levels in WT plants using the CsGAPDH endogenous control. Letters exhibit the differences as measured using Fisher’s LSD test (p=0.05, n=3).
To understand how CLas4425 promotes CLas colonization, the H2O2 and SA levels in citrus were further investigated. The H2O2 content was not significantly different between 4425-OEs and WTs. Of interest, CLas stimulated H2O2 accumulation in WTs but not in 4425-OEs (Figure 4D). Herein, CLas4425 stimulated a slight increase of H2O2 in citrus plants, which is consistent with mild HR induced by CLas4425 in N. benthamiana. Moreover, CLas4425 hindered SA accumulation (p<0.05) (Figure 4E). We then detected the expression of SA signaling pathway genes. The expression of CsEDS1, CsNDR1, CsNPR1, CsPR1, CsPR2, and CsPR5 was depressed in 4425-OE lines (Figures 4F, G). Therefore, CLas4425 suppresses the plant immune system through interfering with the SA signaling pathway.
Discussion
Since the release of the first CLas genome in 2009, the effector and host interactions have become a hotspot for studying CLas pathogenesis. Vectored by ACP, CLas utilizes the effector repertoire to adjust to distinct insects and plants, in addition to different disease stages. Analysis of global gene expression changes in CLas during transmission between citrus and psyllid suggests that the genes up-regulated in planta are mainly involved in the virulence and/or survival of the pathogen (Yan et al., 2013). Consistent with this suggestion, the expressions of effectors, including CLas4425 and previously reported virulence factors, remarkably increased in planta compared to those in psyllids (Liu et al., 2019; Du et al., 2022; Shen et al., 2022). Additionally, temporal detection of effector transcripts is an important aspect of the study on pathogen effectors during the initial microbe-plant interactions (Shi et al., 2019). In this study, the expression level of CLas4425 was detected after two months of CLas inoculation and maintained the same level during the infection processes. The consistent expression of CLas4425 could act as a virulence factor to create a favorable phloem environment for CLas proliferation and enhancement of HLB symptom.
Transient expression of CLas4425 elicited cell death in N. benthamiana. Instead of inducing obvious symptoms in citrus plants, CLas4425 stimulated the expression of CsPP2, which plays a critical role in plant defense responses to biotic stresses (Zhang et al., 2011; Will et al., 2013). Previous reports demonstrated that SDE1 and AGH17470 are also HR elicitors, while most CLas effectors were identified to suppress defense responses (Pitino et al., 2016; Zhang et al., 2020; Du et al., 2022; Oh et al., 2022). LasBCP, SC2_gp095, and SECP8 were reported as ROS scavengers, and AGH17488 targets APX6 to eliminate ROS (Jain et al., 2015; Jain et al., 2019; Shen et al., 2022; Du et al., 2023). The phenomenon indicated effector-effector interactions, which may be due to a co-pathway targeted by the ETI-eliciting and ETI-suppressing effectors (Zhang et al., 2015; Martel et al., 2018). The interactions could result in the long incubation period before visible HLB symptoms. However, the interaction mechanism remains to be elucidated.
Effector-triggered cell death is tightly related to subcellular localization (Yu et al., 2012; Asai et al., 2018). CLas4425 exclusively localized to cytoplasm and cytoplasmic membrane, and the enforcement of its nuclear accumulation impaired its ability to initiate cell death. Intriguingly, silencing of the cytoplasmic receptor NbBIK1 rather than the membrane receptors NbBAK1 and NbSOBIR1 also perturbed CLas4425 cell death-inducing activity. In Arabidopsis, BIK1 is required for phosphorylation of respiratory burst oxidative homolog D (RBOHD) in PTI and ETI-mediated ROS generation (Yuan et al., 2021). Hence, CLas4425 is speculated to indirectly activate BIK1 to phosphorylate RBOHB, leading to ROS burst and then cell death via ETI regulation.
ETI leads to a complex array of global as well as specific defense responses, which require the participation of phytohormones (Zhang and Li, 2019). Among these, SA serves as a key plant defense hormone, and is required for the establishment of systemic acquired resistance (SAR) (Dempsey et al., 2011; Tanaka et al., 2015). The SA-associated pathway is sophisticatedly manipulated. For instance, EDS1 and NDP1 have been identified as upstream regulators of SA biosynthesis during ETI (McDowell et al., 2000; Chang et al., 2022). Increasing levels of SA accelerate NPR1 movement to the nucleus, which results in activating the expression of SA-dependent pathogenesis-related genes, PR1, PR2, and PR5 (Ali et al., 2018; Chen et al., 2021). Evidence showed that SA accumulation increases in the late stage of CLas infection, and artificially increasing SA levels can elevate HLB-tolerance (Li et al., 2021; Peng et al., 2021). Correspondingly, CLas have evolved efficient tactics to downregulate the SA-signaling pathway. For instance, CLas-encoded SahA serves as an SA hydroxylase, and SDE1 targeted citrus PLCPs proteases that were related to SA-induced defense (Li et al., 2017; Clark et al., 2018). The repressed SA level and related genes expression in both N. benthamiana and citrus plants revealed that CLas4425 may confer broad-spectrum virulence through regulating the SA-signaling pathway. Nevertheless, the insight into the role of CLas4425 in pathogenicity remains to be unveiled.
Conclusion
CLas pathogenesis is of great importance but challenging to study because of its phloem-limited nature, intracellular lifestyle, and inability to be cultured. In the study, CLas4425 was identified to be expressed at the early stage of CLas infection, and could play a critical role in CLas multiplication. CLas4425 expression results in HR in N. benthamiana, which relies on its subcellular localization and the presence of BIK1. However, CLas4425 interferes with the SA-mediated signaling pathway and promotes CLas infection and disease development.
Data availability statement
The original contributions presented in the study are included in the article/Supplementary Material. Further inquiries can be directed to the corresponding authors.
Author contributions
SSZ, TZ, Shimin Fu, and CZ designed the experiments. SSZ performed the experiments, analyzed the data, as well as wrote the draft manuscript. JH, XW, SZ, SF, and CZ revised and polished the manuscript. All authors contributed to the article and approved the submitted version.
Funding
This research was partially supported by the National Key R&D Program of China (2021YFD1400800), the National Natural Sciences Foundation of China (31871925), and the Natural Sciences Foundation of Chongqing (cstc2020jcyj-msxmX1036).
Acknowledgments
We thank Prof. D. L. Dou for providing PVX : BAX (College of Plant Protection, Nanjing Agriculture University) and Prof. X. P. Zou for providing vector pLGN (Citrus Research Institute, SWU/CAAS). Thanks are also given to Y. H. Zhang for HPLC analysis (Citrus Research Institute, SWU/CAAS).
Conflict of interest
The authors declare that the research was conducted in the absence of any commercial or financial relationships that could be construed as a potential conflict of interest.
Publisher’s note
All claims expressed in this article are solely those of the authors and do not necessarily represent those of their affiliated organizations, or those of the publisher, the editors and the reviewers. Any product that may be evaluated in this article, or claim that may be made by its manufacturer, is not guaranteed or endorsed by the publisher.
Supplementary material
The Supplementary Material for this article can be found online at: https://www.frontiersin.org/articles/10.3389/fpls.2023.1224736/full#supplementary-material
Supplementary Table 1 | Primer pairs used in this study.
Supplementary Figure 1 | The progression of CLas4425-triggered cell death. (A), The records of CLas4425-triggered cell death at 3-8 dpi with cell staining and ethanol decolorization. The diagram on the left side represents the sites infiltrated with inoculation buffer, PVX-GFP, PVX-BAX, and PVX-CLas4425, respectively. The experiment had three independent biological replicates and was repeated twice with similar results. (B), H2O2 contents in N. benthamiana expressing GFP and CLas4425. Leaves were collected at the indicated time points. The differences between CLas4425- and GFP-expressing samples were analyzed using Student’s t-test (*p<0.05, ** p<0.01, n=3). Dunnett’s T3 test was used to compare H2O2 accumulation within the timelines (p=0.05, n=3). (C), CLas4425-inducing cell death activity clarified using pLGN vector. Leaves were infiltrated with Agrobacterium tumefaciens carrying pLGN-GFP, pLGN-CLas4425, and PVX-BAX, and then photographed at 8 dpi.
Supplementary Figure 2 | NLS : CLas4425:RFP verified using the confocal laser scanning microscope. RFP was used as the location control while H2B:GFP was used as the nucleus marker. Scale bar: 20 μm.
Supplementary Figure 3 | Transcript levels of PVX and genes associated with plant innate immunity. (A), Accumulation of PVX in N. benthamiana. (B), Transcript levels of a PAMP-triggered immunity gene (NbWRKY29) and the genes corresponding to jasmonic acid and ethylene signaling pathways, namely NbLOX and, NbERF1. In (A, B), qRT-PCR was used to analyze gene expression in response to CLas4425 infiltration. The transcript levels were normalized to levels in GFP at 2 dpi using the NbACTIN endogenous control. The differences between CLas4425- and GFP-expressing samples were analyzed using Student’s t-test (*p<0.05, **p<0.01, ***p<0.001, n=3). Fisher’s LSD test was used to compare gene expression within the timelines (p=0.05, n=3).
Supplementary Figure 4 | (A), Construction of CLas4425-transgenic citrus plants. Structure of the pLGN-CLas4425 plasmid used for the overexpression assays. Identification of transgenic plants with PCR, RT-qPCR, and β-glucuronidase (GUS) histochemical staining. M, DNA marker; OE, transgenic citrus plants; WT, wildtype control. Scale bar: 9 mm. (B), Phenotypes of WT and 4425-OE. Scale bar: 10 cm.
References
Ali, S., Ganai, B. A., Kamili, A. N., Bhat, A. A., Mir, Z. A., Bhat, J. A., et al. (2018). Pathogenesis-related proteins and peptides as promising tools for engineering plants with multiple stress tolerance. Microbiol. Res. 212-213, 29–37. doi: 10.1016/j.micres.2018.04.008
Andrade, M., Li, J. Y., Wang, N. (2020). Candidatus Liberibacter asiaticus: virulence traits and control strategies. Trop. Plant Pathology. 45, 285–297. doi: 10.1007/s40858-020-00341-0
Asai, S., Furzer, O. J., Cevik, V., Kim, D. S., Ishaque, N., Goritschnig, S., et al. (2018). A downy mildew effector evades recognition by polymorphism of expression and subcellular localization. Nat. Commun. 9, 5192. doi: 10.1038/s41467-018-07469-3
Bové, J. M. (2006). Huanglongbing: a destructive, newly-emerging, century-old disease of citrus. J. Plant Pathol. 88, 7–37. doi: 10.4454/jpp.v88i1.828
Chang, M., Chen, H., Liu, F., Fu, Z. Q. (2022). PTI and ETI: convergent pathways with diverse elicitors. Trends Plant Sci. 27, 113–115. doi: 10.1016/j.tplants.2021.11.013
Chen, H., Li, M., Qi, G., Zhao, M., Liu, L., Zhang, J., et al. (2021). Two interacting transcriptional coactivators cooperatively control plant immune responses. Sci. Adv. 7, eabl7173. doi: 10.1126/sciadv.abl7173
Clark, K., Franco, J. Y., Schwizer, S., Pang, Z., Hawara, E., Liebrand, T. W. H., et al. (2018). An effector from the Huanglongbing-associated pathogen targets citrus proteases. Nat. Commun. 9, 1–11. doi: 10.1038/s41467-018-04140-9
Clark, K. J., Pang, Z., Trinh, J., Wang, N., Ma, W. (2020). Sec-delivered effector 1 (SDE1) of ‘Candidatus Liberibacter asiaticus’ promotes citrus Huanglongbing. Mol. Plant Microbe In. 33, 1394–1404. doi: 10.1094/MPMI-05-20-0123-R
Dempsey, D. A., Vlot, A. C., Wildermuth, M. C., Klessig, D. F. (2011). Salicylic Acid biosynthesis and metabolism. Arabidopsis Book. 9, e0156. doi: 10.1199/tab.0156
Du, M., Wang, S., Dong, L., Qu, R., Zheng, L., He, Y., et al. (2021). Overexpression of a "Candidatus Liberibacter Asiaticus" Effector Gene CaLasSDE115 Contributes to Early Colonization in Citrus sinensis. Front. Microbiol. 12. doi: 10.3389/fmicb.2021.797841
Du, J., Wang, Q., Shi, H., Zhou, C., He, J., Wang, X. (2023). A prophage-encoded effector from "Candidatus Liberibacter asiaticus" targets ASCORBATE PEROXIDASE6 in citrus to facilitate bacterial infection. Mol. Plant Pathol. 24, 302–316. doi: 10.1111/mpp.13296
Du, J., Wang, Q., Zeng, C., Zhou, C., Wang, X. (2022). A prophage-encoded nonclassical secretory protein of "Candidatus Liberibacter asiaticus" induces a strong immune response in Nicotiana benthamiana and citrus. Mol. Plant Pathology. 00, 1–13. doi: 10.1111/mpp.13206
Du, P., Zhang, C., Zou, X., Zhu, Z., Yan, H., Wuriyanghan, H., et al. (2021). "Candidatus Liberibacter asiaticus" Secretes Nonclassically Secreted Proteins That Suppress Host Hypersensitive Cell Death and Induce Expression of Plant Pathogenesis-Related Proteins. Appl. Environ. Microbiol. 87, e00019–21. doi: 10.1128/AEM.00019-21
Franco-Orozco, B., Berepiki, A., Ruiz, O., Gamble, L., Griffe, L. L., Wang, S., et al. (2017). A new proteinaceous pathogen-associated molecular pattern (PAMP) identified in Ascomycete fungi induces cell death in Solanaceae. New Phytologist. 214, 1657–1672. doi: 10.1111/nph.14542
Heese, A., Hann, D. R., Gimenez-Ibanez, S., Jones, A. M. E., He, K., Li, J., et al. (2007). The receptor-like kinase SERK3/BAK1 is a central regulator of innate immunity in plants. PNAS. 104, 12217–12222. doi: 10.1073/pnas.0705306104
Jain, M., Cai, L., Fleites, L. A., Munoz-Bodnar, A., Davis, M. J., Gabriel, D. W. (2019). Liberibacter crescens Is a cultured surrogate for functional genomics of uncultured pathogenic ‘Candidatus Liberibacter’ spp. and Is naturally competent for transformation. Phytopathology. 109, 1811–1819. doi: 10.1094/PHYTO-04-19-0129-R
Jain, M., Fleites, L. A., Gabriel, D. W. (2015). Prophage-encoded peroxidase in ‘Candidatus Liberibacter asiaticus’ is a secreted effector that suppresses plant defenses. Mol. Plant Microbe In. 28, 1330–1337. doi: 10.1094/MPMI-07-15-0145-R
Jones, J. D., Dangl, J. L. (2006). The plant immune system. Nature 444, 323–329. doi: 10.1038/nature05286
Kadota, Y., Shirasu, K., Zipfel, C. (2015). Regulation of the NADPH oxidase RBOHD during plant immunity. Plant Cell Physiol. 56, 1472–1480. doi: 10.1093/pcp/pcv063
Kim, J. S., Sagaram, U. S., Burns, J. K., Li, J. L., Wang, N. (2009). Response of sweet orange (Citrus sinensis) to 'Candidatus Liberibacter asiaticus' infection: microscopy and microarray analyses. Phytopathology. 99, 50–57. doi: 10.1094/PHYTO-99-1-0050
Li, J., Pang, Z., Trivedi, P., Zhou, X., Ying, X., Jia, H., et al. (2017). ‘Candidatus Liberibacter asiaticus’ encodes a functional salicylic acid (SA) hydroxylase that degrades SA to suppress plant defenses. Mol. Plant Microbe In. 30, 620–630. doi: 10.1094/MPMI-12-16-0257-R
Li, B., Zhang, Y., Qiu, D., Francis, F., Wang, S. (2021). Comparative Proteomic Analysis of Sweet Orange Petiole Provides Insights Into the Development of Huanglongbing Symptoms. Front. Plant Sci. 12. doi: 10.3389/fpls.2021.656997
Liebrand, T. W., van den Burg, H. A., Joosten, M. H. (2014). Two for all: receptor-associated kinases SOBIR1 and BAK1. Trends Plant Science. 19, 123–132. doi: 10.1016/j.tplants.2013.10.003
Lin, K. H. (1956). Observations on yellow shoot of citrus: aetiological studies of yellow shoot of citrus. Acta Phytopathologica Sinica. 2, 1–42.
Liu, X., Fan, Y., Zhang, C., Dai, M., Wang, X., Li, W. (2019). Nuclear import of a secreted 'Candidatus Liberibacter asiaticus' protein is temperature dependent and contributes to pathogenicity in Nicotiana benthamiana. Front. Microbiol. 10. doi: 10.3389/fmicb.2019.01684
Liu, Y., Schiff, M., Dinesh-Kumar, S. P. (2002). Virus-induced gene silencing in tomato. Plant J. 31, 777–786. doi: 10.1046/j.1365-313x.2002.01394.x
Livak, K. J., Schmittgen, T. D. (2001). Analysis of relative gene expression data using real-time quantitative PCR and the 2-DDCT Method. Methods. 25, 402–408. doi: 10.1006/meth.2001.1262
Martel, A., Laflamme, B., Breit-McNally, C., Wang, P., Lonjon, F., Desveaux, D., et al. (2018). Metaeffector interactions modulate the type III effector-triggered immunity load of Pseudomonas syringae. PloS Pathog. 18, e1010541. doi: 10.1371/journal.ppat.1010541
Martinelli, F., Dandekar, A. M. (2017). Genetic mechanisms of the devious intruder Candidatus Liberibacter in Citrus. Front. Plant Science. 8. doi: 10.3389/fpls.2017.00904
McDowell, J. M., Cuzick, A., Can, C., Beynon, J., Dangl, J. L., Holub, E. B. (2000). Downy mildew (Peronospora parasitica) resistance genes in Arabidopsis vary in functional requirements for NDR1, EDS1, NPR1 and salicylic acid accumulation. Plant J. 22, 523–529. doi: 10.1046/j.1365-313x.2000.00771.x
Oh, J., Levy, J. G., Kan, C. C., Ibanez-Carrasco, F., Tamborindeguy, C. (2022). CLIBASIA_00460 Disrupts Hypersensitive Response and Interacts with Citrus Rad23 Proteins. Int. J. Mol. Sci. 23, 7846. doi: 10.3390/ijms23147846
Pang, Z., Zhang, L., Coaker, G., Ma, W., He, S.-Y., Wang, N. (2020). Citrus CsACD2 is a target of Candidatus Liberibacter asiaticus in Huanglongbing disease. Plant Physiol. 184, 792–805. doi: 10.1104/pp.20.00348
Peng, A., Xu, L., He, Y., Lei, T., Yao, L., Chen, S., et al. (2015). Efficient production of marker-free transgenic ‘Tarocco’ blood orange (Citrus sinensis Osbeck) with enhanced resistance to citrus canker using a Cre/loxP site-recombination system. Plant Cell Tissue Organ Culture (PCTOC). 123, 1–13. doi: 10.1007/s11240-015-0799-y
Peng, A., Zou, X., He, Y., Chen, S., Liu, X., Zhang, J., et al. (2021). Overexpressing a NPR1-like gene from Citrus paradisi enhanced Huanglongbing resistance in C. sinensis. Plant Cell Rep. 40, 529–541. doi: 10.1007/s00299-020-02648-3
Perilla-Henao, L. M., Casteel, C. L. (2016). Vector-Borne Bacterial Plant Pathogens: Interactions with Hemipteran Insects and Plants. Front. Plant Sci. 7. doi: 10.3389/fpls.2016.01163
Pitino, M., Armstrong, C. M., Cano, L. M., Duan, Y. (2016). Transient expression of Candidatus Liberibacter asiaticus effector induces cell death in Nicotiana benthamiana. Front. Plant Sci. 7, 982. doi: 10.3389/fpls.2016.00982
Prasad, S., Xu, J., Zhang, Y., Wang, N. (2016). SEC-translocon dependent extracytoplasmic proteins of Candidatus Liberibacter asiaticus. Front. Microbiol. 7. doi: 10.3389/fmicb.2016.01989
Reinking, O. A. (1919). Disease of economic plants in southern China. Phiilippines Agriculture. 8, 109–135.
Shen, P., Li, X., Fu, S., Zhou, C., Wang, X. (2022). A "Candidatus Liberibacter asiaticus"-secreted polypeptide suppresses plant immune responses in Nicotiana benthamiana and Citrus sinensis. Front. Plant Sci. 13. doi: 10.3389/fpls.2022.997825
Shi, Q., Pitino, M., Zhang, S., Krystel, J., Cano, L. M., Jr, R. G. S., et al. (2019). Temporal and spatial detection of Candidatus Liberibacter asiaticus putative effector transcripts during interaction with Huanglongbing-susceptible, –tolerant, and -resistant citrus hosts. BMC Plant Biol. 19, 1–12. R. G. S. doi: 10.1186/s12870-019-1703-4
Singerman, A., Rogers, M. E. (2020). The economic challenges of dealing with citrus greening: The case of Florida. J. Integr. Pest Manage. 11, 1–7. doi: 10.1093/jipm/pmz037
Situ, J., Jiang, L., Fan, X., Yang, W., Li, W., Xi, P., et al. (2020). An RXLR effector PlAvh142 from Peronophythora litchii triggers plant cell death and contributes to virulence. Mol. Plant Pathology. 21, 415–428. doi: 10.1111/mpp.12905
Tanaka, S., Han, X., Kahmann, R. (2015). Microbial effectors target multiple steps in the salicylic acid production and signaling pathway. Front. Plant Sci. 6. doi: 10.3389/fpls.2015.00349
Wang, N., Trivedi, P. (2013). Citrus Huanglongbing: A newly relevant disease presents unprecedented challenges. Phytopathology. 103, 652–665. doi: 10.1094/PHYTO-12-12-0331-RVW
Will, T., Furch, A. C., Zimmermann, M. R. (2013). How phloem-feeding insects face the challenge of phloem-located defenses. Front. Plant Sci. 4. doi: 10.3389/fpls.2013.00336
Yan, Q., Sreedharan, A., Wei, S., Wang, J., Pelz-Stelinski, K., Folimonova, S., et al. (2013). Global gene expression changes in Candidatus Liberibacter asiaticus during the transmission in distinct hosts between plant and insect. Mol. Plant Pathol. 14, 391–404. doi: 10.1111/mpp.12015
Ying, X., Wan, M., Hu, L., Zhang, J., Li, H., Lv, D. (2019). Identification of the Virulence Factors of Candidatus Liberibacter asiaticus via Heterologous Expression in Nicotiana benthamiana using Tobacco Mosaic Virus. Int. J. Mol. Sci. 20, 5575. doi: 10.3390/ijms20225575
Yu, X., Tang, J., Wang, Q., Ye, W., Tao, K., Duan, S., et al. (2012). The RxLR effector Avh241 from Phytophthora sojae requires plasma membrane localization to induce plant cell death. New Phytol. 196, 247–260. doi: 10.1111/j.1469-8137.2012.04241.x
Yuan, M., Jiang, Z., Bi, G., Nomura, K., Liu, M., Wang, Y., et al. (2021). Pattern-recognition receptors are required for NLR-mediated plant immunity. Nature. 592, 105–109. doi: 10.1038/s41586-021-03316-6
Zhang, C., Du, P., Yan, H., Zhu, Z., Wang, X., Li, W. (2020). A Sec-dependent secretory protein of the Huanglongbing-associated pathogen suppresses hypersensitive cell death in Nicotiana benthamiana. Front. Microbiol. 11. doi: 10.3389/fmicb.2020.594669
Zhang, Y., Li, X. (2019). Salicylic acid: biosynthesis, perception, and contributions to plant immunity. Curr. Opin. Plant Biol. 50, 29–36. doi: 10.1016/j.pbi.2019.02.004
Zhang, M. X., Li, Q., Liu, T. L., Liu, L., Shen, D. Y., Zhu, Y., et al. (2015). Two cytoplasmic effectors of Phytophthora sojae regulate plant cell death via interactions with plant catalases. Plant Physiol. 167, 164–175. doi: 10.1104/pp.114.252437
Zhang, C. L., Shi, H. J., Chen, L., WANG, X. M., Lü, B. B., Zhang, S. P., et al. (2011). Harpin-induced expression and transgenic overexpression of the phloem protein gene AtPP2-A1 in Arabidopsis repress phloem feeding of the green peach aphid Myzus persicae. BMC Plant Biol. 11, 1–19. doi: 10.1186/1471-2229-11-11
Zhang, C., Wang, X., Liu, X., Fan, Y., Zhang, Y., Zhou, X., et al. (2019). A novel 'Candidatus Liberibacter asiaticus'-encoded Sec-dependent secretory protein suppresses programmed cell death in Nicotiana benthamiana. Int. J. Mol. Sci. 20, 5802. doi: 10.3390/ijms20225802
Keywords: Citrus Huanglongbing (HLB), CLas, effector, CLas4425, cell death, virulence factor
Citation: Zhang S, Wang X, He J, Zhang S, Zhao T, Fu S and Zhou C (2023) A Sec-dependent effector, CLIBASIA_04425, contributes to virulence in ‘Candidatus Liberibater asiaticus’. Front. Plant Sci. 14:1224736. doi: 10.3389/fpls.2023.1224736
Received: 18 May 2023; Accepted: 05 July 2023;
Published: 24 July 2023.
Edited by:
Qibin Yu, University of Florida, United StatesReviewed by:
Amit Levy, University of Florida, United StatesWenxiu Ma, University of Florida, United States
Copyright © 2023 Zhang, Wang, He, Zhang, Zhao, Fu and Zhou. This is an open-access article distributed under the terms of the Creative Commons Attribution License (CC BY). The use, distribution or reproduction in other forums is permitted, provided the original author(s) and the copyright owner(s) are credited and that the original publication in this journal is cited, in accordance with accepted academic practice. No use, distribution or reproduction is permitted which does not comply with these terms.
*Correspondence: Tingchang Zhao, zhaotingchang@caas.cn; Shimin Fu, fumin@cric.cn; Changyong Zhou, zhoucy@cric.cn