- 1Hubei Key Laboratory of Germplasm Innovation and Utilization of Fruit Trees, Institute of Fruit and Tea, Hubei Academy of Agricultural Science, Wuhan, China
- 2Hubei Hongshan Laboratory, Wuhan, China
- 3College of Horticulture and Forestry Sciences, Huazhong Agricultural University, Wuhan, China
‘Lane Late’, a late-maturing navel orange cultivar, is mainly distributed in the Three Gorges Reservoir area, which matures in the late March of the next year and needs overwintering cultivation. Citrus fruit granulation is a physiological disorder, which is characterized by lignification and dehydration of juice sac cells, seriously affecting the commercial value of citrus fruits. The pre-harvest granulation of late-maturing navel orange is main caused by low temperature in the winter, but its mechanism and regulation pattern remain unclear. In this study, a SG2-type R2R3-MYB transcription factor, CsMYB15, was identified from Citrus sinensis, which was significantly induced by both juice sac granulation and low temperature treatment. Subcellular localization analysis and transcriptional activation assay revealed that CsMYB15 protein was localized to the nucleus, and it exhibited transcriptional activation activity in yeast. Over-expression of CsMYB15 by stable transformation in navel orange calli and transient transformation in kumquat fruits and navel orange juice sacs significantly increased lignin content in the transgenic lines. Further, Yeast one hybrid, EMSA, and LUC assays demonstrated that CsMYB15 directly bound to the Cs4CL2 promoter and activated its expression, thereby causing a high accumulation of lignin in citrus. Taken together, these results elucidated the biological function of CsMYB15 in regulating Cs4CL2-mediated lignin biosynthesis, and provided novel insight into the transcriptional regulation mechanism underlying the juice sac granulation of late-maturing navel orange.
1 Introduction
Citrus is the largest fruit crop in the world, and it is valued due to its abundant functional components beneficial to human health. However, granulation (also known as crystallization) has been a serious problem in the fruit juice sacs of many citrus varieties, including sweet orange (Citrus sinensis) (Jia et al., 2018; Jia et al., 2019), mandarin (Citrus reticulata) (Yao et al., 2018), grapefruit (Citrus paradisi) (Burns and Albrigo, 1998), and pummelo (Citrus grandis) (Wu et al., 2014; Shi et al., 2020; Chen et al., 2021). Granulation is a physiological disorder, which causes a reduction in juice, sugar, acid, and flavor substances of citrus fruits, leaving behind the dry, tough, and colorless granulated juice sacs (Theanjumpol et al., 2019; Chen et al., 2021). Granulation usually occurs in either post-harvest storage stage of most citrus varieties or on-tree stage of late-ripening citrus varieties (Burns and Albrigo, 1998; Wu et al., 2014; Chen et al., 2021). Due to the serious damage of granulation to citrus industry, numerous studies have been undertaken to investigate the physiological and molecular mechanisms of citrus granulation, as well as its prevention and control measures (Burns and Albrigo, 1998; Wang et al., 2014; Wu et al., 2014; Yao et al., 2018; Theanjumpol et al., 2019; Shi et al., 2020; Chen et al., 2021). However, most studies are focused on the granulation during post-harvest storage stage, the studies about the juice sac granulation during pre-harvest on-tree ripening stage are very limited (Wu et al., 2020).
Previous studies have revealed that citrus fruit granulation is correlated with lignin deposition, and lignin contents are observed to increase in the granulated juice sacs in many citrus varieties such as mandarin (Sharma et al., 2015; Yao et al., 2018), sweet orange (Jia et al., 2018; Wu et al., 2020; Zhang et al., 2021), and pummelo (Wu et al., 2014; Shi et al., 2020). Lignin, an important component of secondary cell wall, has been reported to play a critical role in citrus granulation process (Jia et al., 2018; Shi et al., 2020). In plants, the lignin biosynthetic pathway involves a series of sequential enzymes, including phenylalanine ammonia lyase (PAL), cinnamate 4-hydroxylase (C4H), 4-coumarate CoA ligase (4CL), shikimate/quinate hydroxy cinnamoyl transferase (HCT), p-coumarate 3-hydroxylase (C3H), caffeic acid O-methyltransferase (COMT), cinnamoyl CoA reductase (CCR), cinnamyl alcohol dehydrogenase (CAD), peroxidase (POD), laccase (LAC), and ferulate 5-hydroxylase (F5H) (Shi et al., 2020; Xie et al., 2020).
The NAC (NAM-ATAF-CUC)-MYB (myeloblastosis)-mediated gene regulatory network responsible for lignin biosynthesis has been well studied in plants (Liu et al., 2015; Ohtani and Demura, 2019). In this regulatory hierarchy, AtNST1 (NAC secondary wall thickening promoting factor 1), AtSND1 (NAC domain protein 1), and AtVND6/7 (vascular-related NAC domain 6/7) have been identified as upstream key regulators for lignin biosynthesis and secondary cell wall formation (Kubo et al., 2005; Mitsuda et al., 2007; Geng et al., 2019). The R2R3-MYB domain MYB transcription factors (TFs) AtMYB46 (Zhong et al., 2007) and AtMYB83 (McCarthy et al., 2009) are direct target genes of AtSND1, and the induction of these two TFs in turn stimulates the expression of downstream lignin biosynthesis genes, including PAL1, C4H, 4CL1, CCoAOMT, HCT, CCR1, and F5H1 (Ko et al., 2009; McCarthy et al., 2009; Zhong and Ye, 2011; Kim et al., 2012; Kim et al., 2014). In addition, several MYB genes are also involved in the regulation of lignin biosynthesis, including AtMYB20, AtMYB42, AtMYB43, AtMYB58, AtMYB63, and AtMYB85 (Zhou et al., 2009; Geng et al., 2019).
In Citrus sinensis, the CsMYB330 and CsMYB308 act as transcriptional activator and repressor of fruit juice sac lignification by directly interacting with Cs4CL1 (Jia et al., 2018). Transcription factor CsMYB85 has been reported to interact with CsMYB308 and bind to the promoter of CsMYB330, thus regulating the expression of Cs4CL1 and fruit juice sacs lignification (Jia et al., 2019). In Citrus grandis, CgMYB58 (homolog of CsMYB85) has been reported to regulate the expression of CgPAL1, CgPAL2, Cg4CL1, and CgC3H during fruit juice sac granulation through the direct interaction with the AC elements in their promoters (Shi et al., 2020). However, all these transcription factors have been identified in the post-harvest induced granulation, the transcription factors involved in the cold-induced granulation remain very limited.
In this study, a typical SG2-type R2R3 MYB transcription factor, CsMYB15, was identified and characterized in Citrus sinensis. The expression of CsMYB15 was up-regulated under different granulation degrees and induced by low temperature treatment in navel orange fruits. Subcellular localization analysis showed that CsMYB15 protein was localized in the nucleus and had transcriptional activation activity in yeast. Over-expression of CsMYB15 increased lignin content in juice sacs, kumquat fruits, and navel orange calli. CsMYB15 bound to the Cs4CL2 promoter to regulate its expression, thus mediating lignin biosynthesis, which indicated that CsMYB15 was involved in the low temperature-induced juice sac granulation progress. This study not only provides a comprehensive analysis of the biological function and regulating mechanism of CsMYB15, but also sheds novel insight into the transcriptional regulation network of lignin biosynthesis in low temperature-induced fruit juice sac granulation process in ‘Lane late’ navel orange.
2 Materials and methods
2.1 Plant materials
A total of 9 mature ‘Lane late’ navel orange (Citrus sinensis Osbeck) trees (10-year-old) with red tangerine (Citrus tangerina Hort) as rootstock from an orchard located in the Three Gorges Reservoir area (E 110°41’, N 30°54’) in Zigui County were selected for sampling in this study, and fruits were collected at 350 days after flowering according to our previous study (Wu et al., 2020). The experiments were conducted with three biological replicates, and 15 fruits collected from 3 trees in different directions were mixed together as one biological replicate. The juice sacs were isolated from the fruits, immediately frozen with liquid nitrogen, and stored at -80 °C for RNA extraction. For low temperature treatment, the juice sacs isolated from normal ‘Lane late’ navel orange fruits were placed on a plate and covered with sterilized gauze to keep moist. Then, the plates with juice sacs were transported into an incubator at 4 °C for low temperature treatment. The samples were collected at 0 h, 3 h, 9 h, 12 h, and 24 h, and 30 individual juice sacs were collected as one biological replicate. Each sample had three biological replicates, and all the juice sacs were immediately frozen with liquid nitrogen and stored in -80 °C for RNA extraction.
2.2 Phylogenetic analysis
To explore the phylogenetic relationship of CsMYB15 and other MYB family transcription factors, a multiple sequence alignment of the amino acid sequences of CsMYB15 and other 32 MYB proteins was performed using MUSCLE (www.ebi.ac.uk/Tools/msa/muscle/ ) with default parameters (Edgar, 2004). The phylogenetic tree was constructed using the maximum likelihood method of MEGA X software with 1000 bootstraps (Kumar et al., 2018). The final phylogenetic tree was visualized and polished with the Interactive Tree of Life software (iTOL, version 5, https://itol.embl.de/) (Letunic and Bork, 2016).
2.3 RNA extraction, cDNA synthesis and qRT-PCR
Total RNA was extracted from the juice sacs using TRIzol™ reagent according to the manufacturer’s instructions (Thermo Scientific). RNA quality was assessed by agarose gel electrophoresis, and 5X All-In-One RT MasterMix with AccuRT (Applied Biological Materials) kit was used for ss/dsDNA digestion and cDNA synthesis. Quantitative RT-PCR was performed on a QuantStudio 7 Flex system (Thermo Scientific, USA) according to the manufacturer’s instruction with EvaGreen 2X qPCR MasterMix kit (Applied Biological Materials, Canada). Elongation factor 1 (Ef1, Cs8g16990) gene was utilized as an internal reference gene (Li et al., 2022), and the relative expression was calculated with 2-ΔΔCt method. Student’s t-test was performed to determine statistical significance, and ** indicated significant differences at the level of P < 0.01. The primers used for qRT-PCR were listed in Supplementary Table S1.
2.4 Cloning of CsMYB15 gene and promoter
The cDNA synthesized from the total RNA extracted from juice sacs of ‘Lane late’ navel orange (Citrus sinensis Osbeck) was used to amplify the full-length coding sequence (CDS) of CsMYB15 using the following primers: Forward 5’ -ATGATGGGGAGGGCTCC- 3’ and Reverse 5’ -GGAAATGGTAATGTTAATGAGTCTGCC-3’. PCR amplification was performed using the Phanta Max Super-Fidelity DNA Polymerase (Vazyme, Nanjing, China) following the manufacturer’s instructions. The PCR product was purified and cloned into pTOPO vector for sequencing. The coding sequence of CsMYB15 was deposited in Genebank with the accession number of OQ985393. The 2000 bp region upstream the start codon ATG of CsMYB15 was cloned as the promoter sequence from the total DNA extracted from the leaf of ‘Lane late’ navel orange. The promoter of CsMYB15 was further analyzed using the online software of plantPan 3.0 (http://plantpan.itps.ncku.edu.tw/) and plantCARE (http://bioinformatics.psb.ugent.be/webtools/plantcare/html/). The promoter sequence of CsMYB15 was provided in Supplementary Table S2.
2.5 Subcellular localization analysis of CsMYB15
The CDS of CsMYB15 without stop codon was ligated with GFP (green fluorescent protein), and then cloned into pICH86988 vector containing cauliflower mosaic virus 35S promoter to construct 35S:CsMYB15:GFP vector by Golden Gate method (Engler et al., 2014). Subsequently, the recombinant 35S:CsMYB15:GFP vector was transformed into Agrobacterium tumefaciens (strain GV3101 with pSoup-p19), and co-expressed with a nucleolus marker 35S:FIB2:mCherry in tobacco leaves (Chang et al., 2016) according to a previous study (Sparkes et al., 2006). The subcellular localization of CsMYB15 was observed via a confocal laser-scanning microscope (TCS SP6, Leica, Germany).
2.6 Validation of CsMYB15 transcriptional activity
A yeast system was utilized to examine the transcriptional activity of CsMYB15 according to a previously described method (Ma et al., 2009). The coding sequences of CsMYB15 were cloned into the bait vector pGBKT7 by homologous recombination method using HiFi DNA Assembly Master Mix (NEB, Ipswich, USA) according to the manufacturer’s protocol. The CsMYB15-pGBKT7 + pGADT7-AD, pGBKT7-lam + pGADT7-T (negative control), and pGBKT7-53 + pGADT7-T (positive control) were transformed into Y2H strain yeast independently. After being cultured on SD/-Trp medium at 30 °C for 3 days, single colonies were selected and further amplified. Subsequently, the positive transformants were grown on SD/-Leu/-Trp and SD/-Leu/-Trp/-His/-Ade media, and the growth of all the transformants were observed after 3 days. Additionally, the galactosidase assay was performed by adding X-α-Gal to the medium according to a previously described method (Ma et al., 2009).
2.7 Transformation of CsMYB15 in juice sacs, kumquat fruits, and citrus calli
The CDS of CsMYB15 was cloned into pAML4 vector (containing cauliflower mosaic virus 35S promoter-driven GFP reporter module) together with CaMV35S promoter and nos terminator through Golden Gate method (Engler et al., 2014). The recombinant plasmid was transformed into Agrobacterium tumefaciens (strain GV3101) for further transformation. For juice sac transient expression, juice sacs were separated from ‘Lane late’ navel orange (Citrus sinensis Osbeck) fruits, and CsMYB15 was transiently over-expressed in juice sacs, as described in previous study (Shi et al., 2019). The transformed juice sacs were carefully collected at 6 days post infection. For kumquat (F. crassifolia Swingle) fruit transient expression, kumquat fruits at the green ripening stage (120-180 days after flowering) were selected for transformation. These fruits were infiltrated with A. tumefaciens carrying the CsMYB15 expression vector by using a sterile 1 ml hypodermic syringe according to the method described previously (Gong et al., 2021a). The infiltrated fruit sections were screened with GFP fluorescence and sampled at 5 days post infection by using a fluorescence microscope (Olympus, Japan). For citrus calli stable transformation, the healthy and plump calli of navel orange (Citrus sinensis Osbeck) were selected, and the transformation was performed according to a previously described method (Shi et al., 2020). The positive calli were selected by GFP fluorescence using a fluorescence microscope (Olympus, Japan), and then validated by PCR amplification.
Half of the juice sac, kumquat fruit, and citrus callus samples were immediately frozen with liquid nitrogen and stored at −80°C for RNA extraction and qRT-PCR analysis. The remaining samples were stoved in a bake oven for lignin content determination.
2.8 Determination of lignin contents
The lignin contents of the juice sacs, kumquat fruits, and citrus calli were measured by using a lignin assay kit (Solarbio, China) according to the manufacturer’s instruction (Yang et al., 2022), and each sample has three biological replicates.
2.9 Yeast one-hybrid assay (Y1H)
Yeast one-hybrid assay was conducted as described previously (Gong et al., 2021b). The full-length CDS of CsMYB15 and promoter sequences of Cs4CL1, Cs4CL2, CsC3H, CsCCR2, CsPOD2, and CsPOD3 were cloned into pGADT7 and pAbAi vectors to produce prey construct of pGADT7:CsMYB15 and bait constructs of pAbAi:promoters, respectively. Then, the bait constructs were transformed into the yeast strain Y1H Gold to produce reporter strains, and their autoactivation was determined on SD/-Ura+ aureobasidin A (AbA) plates. The prey construct was introduced into the reporter strains, and pGADT7 empty vector was utilized as a negative control. The transformed yeast cells were cultured on SD/-Ura/-Leu/AbA medium for Y1H assay to examine the interaction between CsMYB15 and the promoters of Cs4CL1, Cs4CL2, CsC3H, CsCCR2, CsPOD2 and CsPOD3. The primers used for constructing Y1H vectors were listed in Supplementary Table S1. The promoter sequences of Cs4CL1, Cs4CL2, CsC3H, CsCCR2, CsPOD2 and CsPOD3 were deposited in Genebank with the accession number of OQ985394 to OQ985399.
2.10 Electrophoretic mobility shift assay (EMSA)
The electrophoretic mobility shift assay (EMSA) was conducted as described previously with minor modifications (Shi et al., 2020). Briefly, the MBP-CsMYB15 protein was expressed and purified, as described previously (Lu et al., 2018). The 5’ FAM-labeled oligonucleotide probes were directly synthesized and labeled by the Tianyi Huiyuan Company (Wuhan, China). The same oligonucleotides without labels were utilized as competitors. Probe information was provided in Supplementary Table S1.
2.11 Dual luciferase transcriptional activity assay (LUC)
Dual luciferase transcriptional activity assay was performed using Nicotiana benthamiana leaves according to a previous report (Lu et al., 2018). Specifically, the the promoter sequence of Cs4CL2 was inserted into the upstream of LUC coding sequence in pGreen0800-LUC vector to produce reporter construct. The full-length CDS of CsMYB15 was cloned into pAML4 over-expression vector to produce 35S::CsMYB15 effector construct. The effector construct and reporter construct were respectively transformed into A. tumefaciens (strain GV3101 with pSoup-p19), and co-expressed in tobacco leaves according to a previously reported method (Hellens et al., 2005). The LUC activity was measured according to the method reported by Lu et al. (2018). All the primers were listed in Supplementary Table S1.
3 Results
3.1 Cloning and characterization of CsMYB15 in Citrus sinensis
The coding sequence of CsMYB15 was identified and cloned from ‘Lane late’ navel orange (Citrus sinensis Osbeck) to investigate its biological function. The coding sequence of CsMYB15 was 804 bp in length, and this coding sequence encoded a 268-amino acid protein. As shown in Figure 1A, the open reading frame of CsMYB15 was constructed with three exons. Then, the coding sequence of CsMYB15 was aligned with that of 8 other MYB genes with high homology. CsMYB15 protein was found to contain four conserved domains, including one R2-domain, one R3-domain, and two SG2 domains, indicating that CsMYB15 was an typical SG2-Type R2R3-MYB protein (Figure 1B).
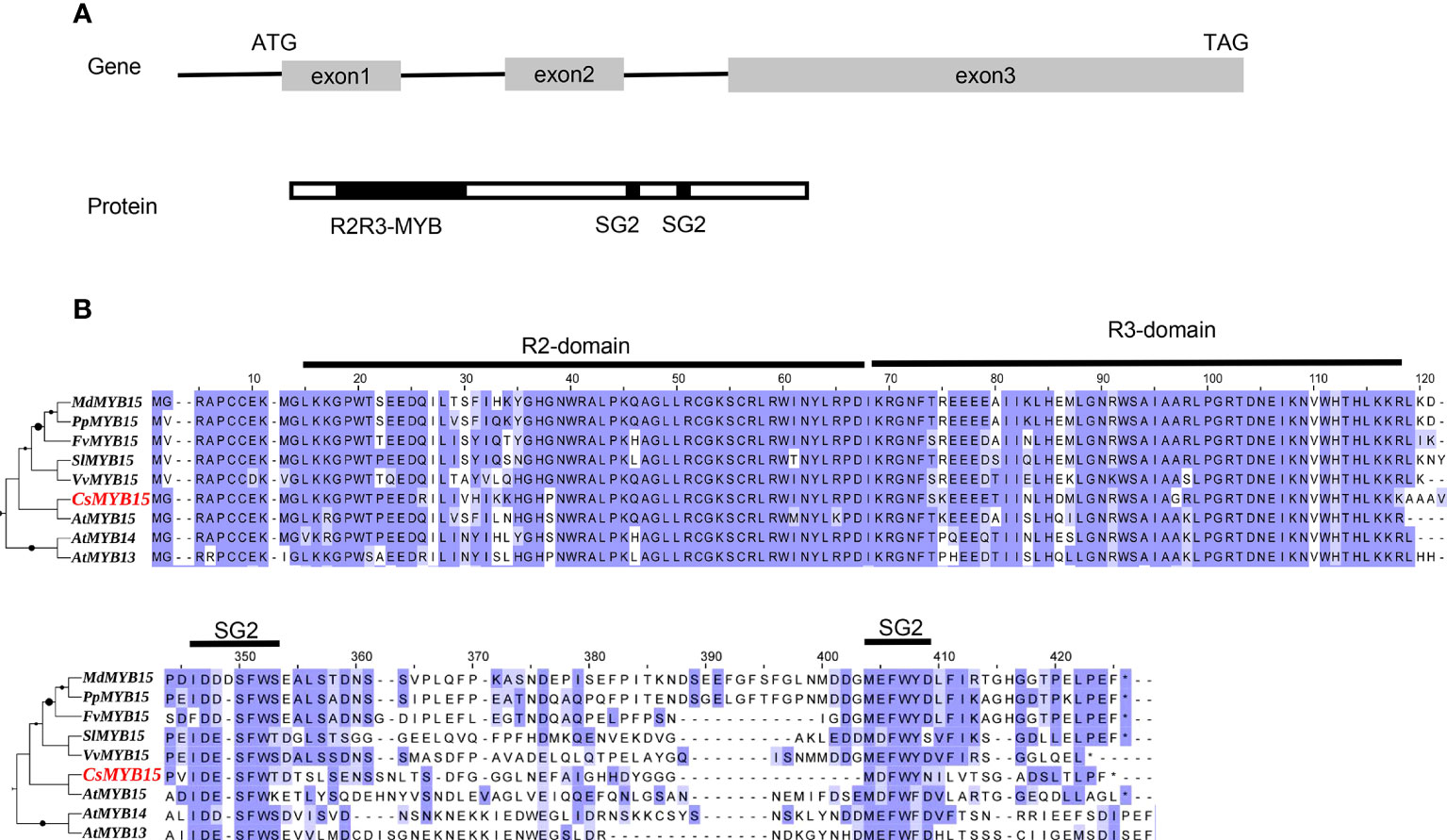
Figure 1 Schematic diagram and amino acid sequence alignment of CsMYB15. (A). Schematic diagram of the CsMYB15 gene (top) and protein (bottom). (B). Multiple sequence alignment of CsMYB15 amino acid sequence with homologous MYBs in other species. R2, R3, and SG2 domains were marked with dark lines.
3.2 Phylogenetic analysis of CsMYB15
To explore the phylogenetic relationship of CsMYB15, a phylogenetic tree was established with the amino acid sequences of 32 MYB proteins using the maximum likelihood method of MEGA. As shown in Figure 2, the MYB genes were divided into 5 clades, including Clade I, Clade II, Clade III, Clade IV and Clade V, and the CsMYB15 was highly homologous to MYB15-like genes from Arabidopsis thaliana, Vitis vinifera, Solanum lycopersicum, Fragaria vesca, Prunus persica, and Malus domestica in Clade I. It was worth noting that CsMYB15 and AtMYB15 were clustered into a subclade with the closest genetic relationship and the highest homology. Further blast result revealed that CsMYB15 was the orthologous gene of cold tolerance-related AtMYB15.
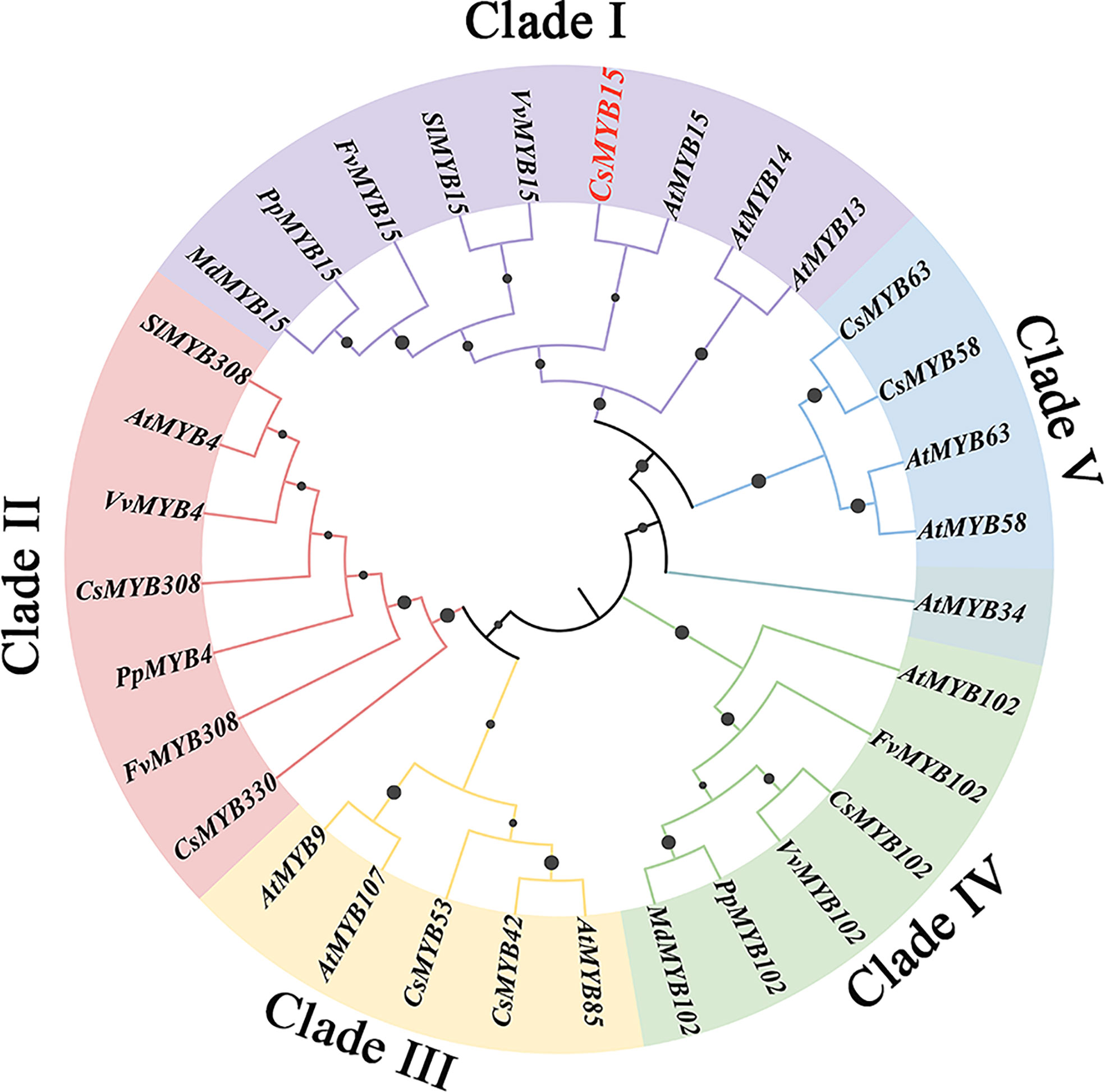
Figure 2 Phylogenetic analysis of MYB15-related proteins and lignin biosynthesis-related MYBs in different plant species. At, Arabidopsis thaliana; Vv, Vitis vinifera; Sl, Solanum lycopersicum; Fv, Fragaria vesca; Pp, Prunus persica; and Md, Malus domestica. CsMYB15 (CS00088G00320 or orange1.1g024484m), FvMYB4 (FV4G00360.1), MdMYB15 (MD00G513530), PpMYB15 (PPE_001G03850), SlMYB15 (SL09G090130), VvMYB15 (VV05G08250), AtMYB14 (AT2G31180), AtMYB13 (AT1G06180), AtMYB107 (AT3G02940), CsMYB102 (CS00010G02180), AtMYB102 (AT4G21440), PpMYB102 (PPE_004G03340), FvMYB39 (FV3G07360), MdMYB102 (MD00G267480), VvMYB102 (VV00G07100), CsMYB9 (CS00312G00030), AtMYB9 (AT5G16770), AtMYB63 (AT1G79180), AtMYB58 (AT1G16490), AtMYB34 (AT5G60890), AtMYB4 (AT4G38620), CsMYB4 (CS00023G00610), PpMYB4 (PPE_008G18180), FvMYB308 (FV2G07290), VvMYB4 (VV03G04830), SlMYB308 (SL01G111500), AtMYB85 (AT4G22680), CsMYB308 (CS00271G00230), CsMYB330 (CS00002G01660), CsMYB58 (CS00052G00740).
3.3 Expression profiles of CsMYB15
To further investigate the role of CsMYB15 in low temperature-induced juice sac granulation, the expression of CsMYB15 under different degrees of low temperature-induced juice sac granulation was assessed by qRT-PCR. As shown in Figure 3A, the expression of CsMYB15 was significantly induced by juice sac granulation, and the induction of CsMYB15 was gradually increased with the increasing granulation degrees, indicating that CsMYB15 might be involved in the regulation network during juice sac granulation process. Interestingly, we also found that the expression of CsMYB15 was significantly induced under low temperature treatment (Figure 3B). Although the expression of CsMYB15 was increased first and then decreased under low temperature treatment, it was relatively higher at all treatment time points than at 0 h, suggesting a significant induction of CsMYB15 under low temperature stress. Further, we amplified the promoter sequence of CsMYB15 from ‘lane late’ navel orange, and analyzed the cis-elements involved in the promoter sequence. As shown in Figure 3C, the potential regulatory cis-elements were predicted, including low temperature responsive element, MYC recognition site, which have been predicted to be involved in low temperature responsiveness in many cases. We also predicted the light responsiveness elements, Box 4, GT-1, and G Box, and hormone responsiveness elements from the promoter of CsMYB15, indicating that the expression level of CsMYB15 might also been regulated by light and plant hormones. Additionally, qRT-PCR revealed that the expression level of 6 lignin biosynthesis genes were up-regulated under low temperature treatment (Figure 3D). Expect for the expression level of CsPAL2 was only induced at 3h, the expression levels of Cs4CL1, Cs4CL2, CsCCR1, CsCCR2, and CsPOD2 were increased first and then decreased, and the expression levels were relatively higher at all treatment time points than at 0 h, which was in line with the expression level of CsMYB15. In a word, these results indicating that CsMYB15 was induced by both juice sac granulation and low temperature treatment.
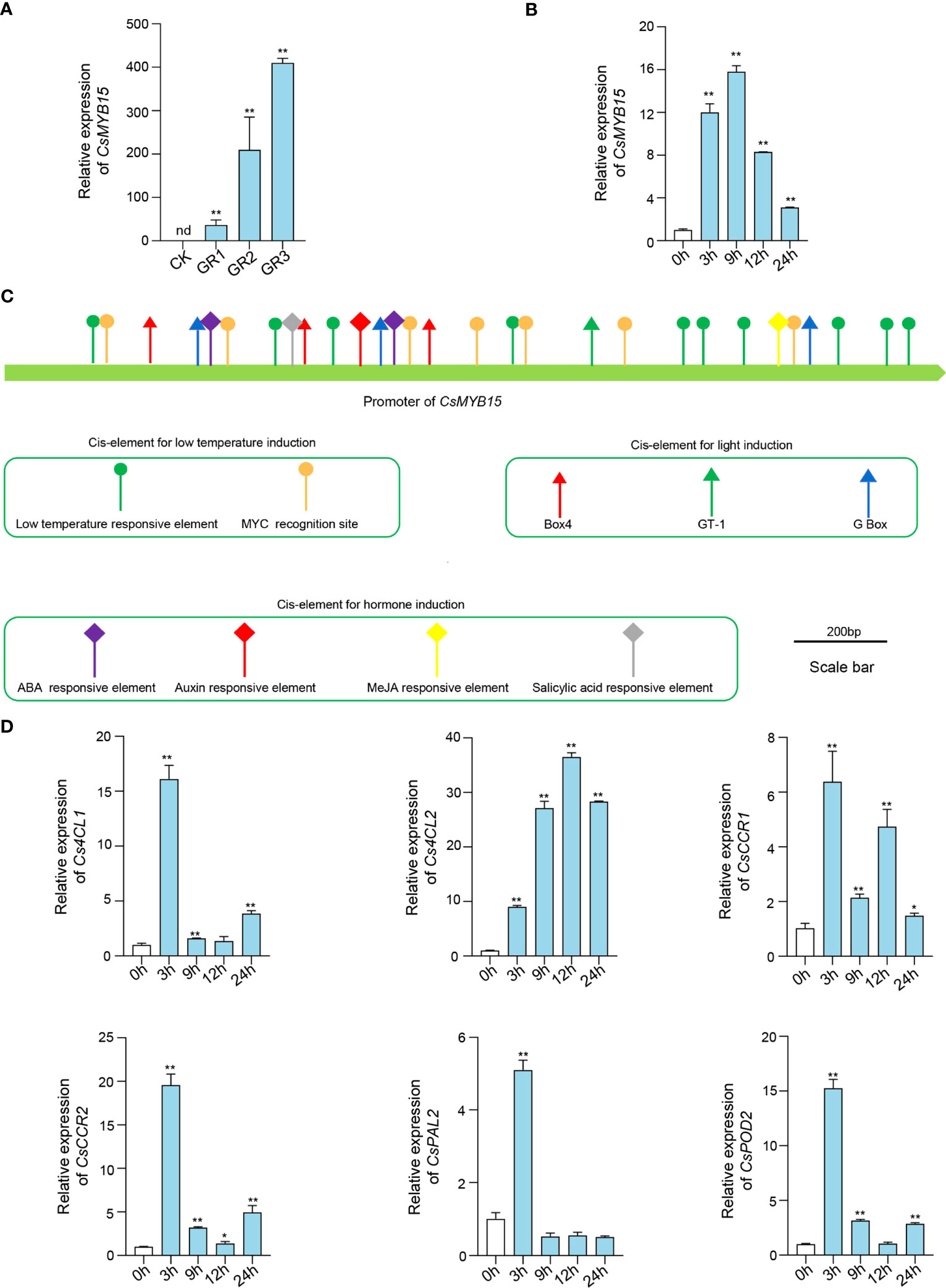
Figure 3 Expression profiles of CsMYB15 under different juice sac granulation stages and low temperature treatment. (A). qRT-PCR analysis of transcript abundance of CsMYB15 in ‘Lane late’ navel orange fruits under different juice sac granulation stages. CK, non-granulated fruit; GR1, slight granulated fruit; GR2, moderate granulated fruit; GR3, serious granulated fruit; nd, not detected. (B). qRT-PCR analysis of the relative expression of CsMYB15 in the fruits treated with low temperature. (C). Schematic diagram of the CsMYB15 promoter in ‘Lane late’ navel orange. (D). qRT-PCR analysis of the relative expression of lignin biosynthesis genes in the fruit juice sacs treated with low temperature.CsEF1 was used as an internal control. All data were expressed as mean ± SE. **, P < 0.01, *, P < 0.05 (student’s t-test).
3.4 Subcellular localization and transcriptional activity of CsMYB15
To explore the subcellular localization of CsMYB15 protein, CsMYB15-GFP fusion construct in pICH86988 vector was transiently co-expressed with a nucleolus marker (FIB2:mCherry) in tobacco leaves. As shown in Figure 4A, the green fluorescence of CsMYB15:GFP was localized to the nucleus, including both nucleolus and nucleoplasm. The GFP fluorescence of nucleolus was overlapped with the red fluorescence of FIB2:mCherry (a nucleolus marker), and the nucleolus was surrounded by the GFP fluorescence of nucleoplasm. A yeast system was adopted for assessing the transcriptional activity of CsMYB15. As shown in Figure 4B, the positive control and pGBKT7-CsMYB15 strains grew well on both SD/-Leu-Trp and SD/-Leu-Trp-His-Ade media, and these two strains turned blue on the medium containing X-α-gal, whereas the negative control strain failed to grow on SD/-Leu-Trp-His-Ade medium, indicating that CsMYB15 had transcriptional activation activity in yeast. Taken together, the above results suggested that CsMYB15 was a nuclear-localized protein with transcriptional activation activity.
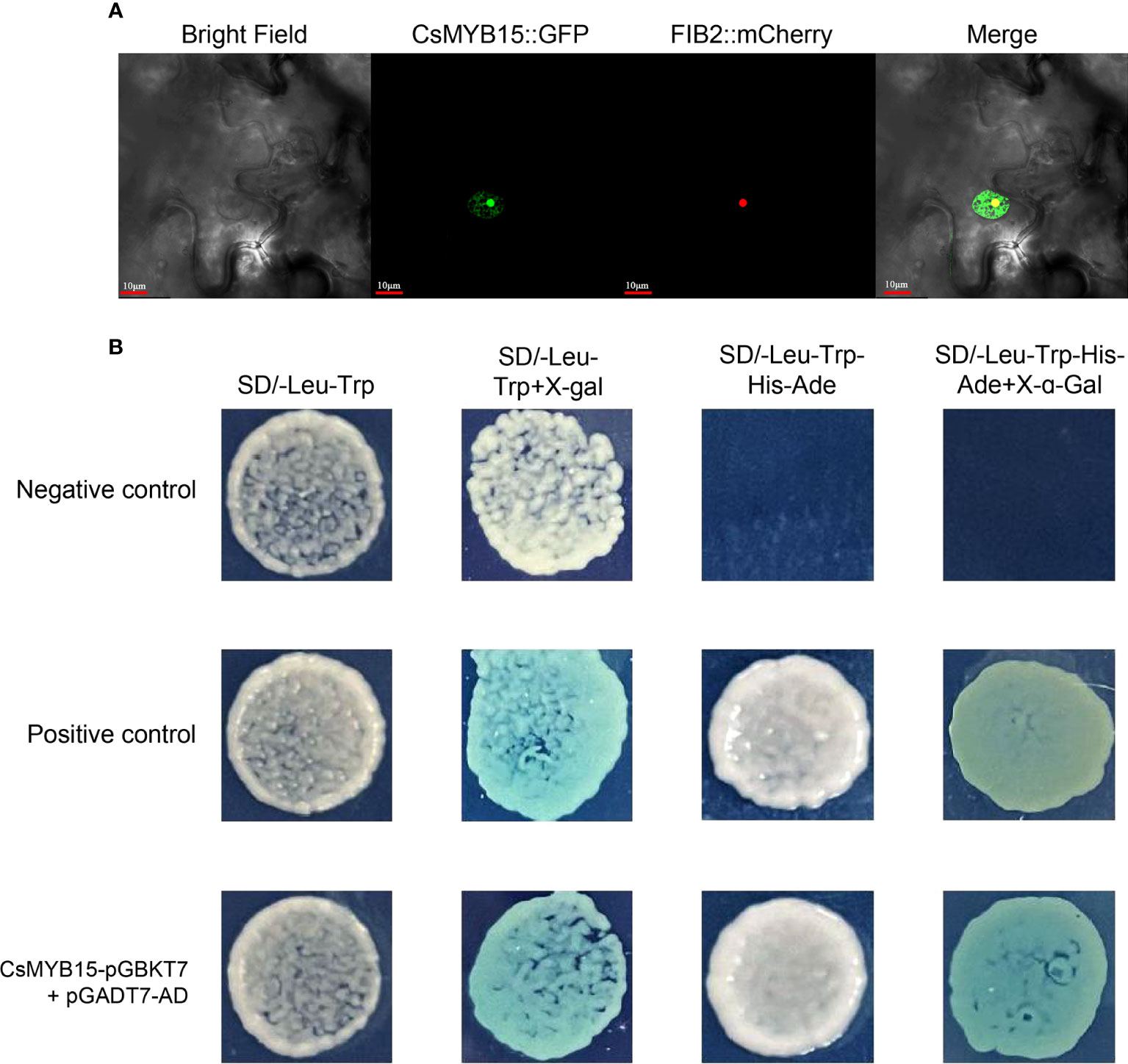
Figure 4 Subcellular localization and transcriptional activity of CsMYB15. (A). Subcellular localization of CsMYB15 in N. benthamiana leaves. Scale bars = 10 μm. (B). Transcription activity analysis of CsMYB15 protein in yeast. pGBKT7-lam + pGADT7-T were co-transformed into yeast as the negative control, and pGBKT7-53 + pGADT7-T were co-transformed into yeast as the positive control.
3.5 Over-expression of CsMYB15 increases lignin content in transgenic plants
The coding sequence of CsMYB15 was cloned from ‘Lane late’ navel orange, and individual transgenic experiments were conducted to explore the biological function of CsMYB15. Firstly, CsMYB15 was transiently expressed in the juice sacs isolated from ‘Lane late’ navel orange fruits. As shown in Figure 5B, qRT-PCR revealed that the expression level of CsMYB15 was up-regulated in the juice sac over-expressing CsMYB15. Comparing with that of wild type, the morphology of juice sacs transiently expressing CsMYB15 was slim, deformed, and granulated (Figure 5A). The lignin content in juice sacs over-expressing CsMYB15 was significantly higher than that in wide type (Figure 5C). These results were further verified by the transient expression of CsMYB15 in ‘Huapi’ kumquat (Fortunella crassifolia Swingle) fruits (Figure S1).
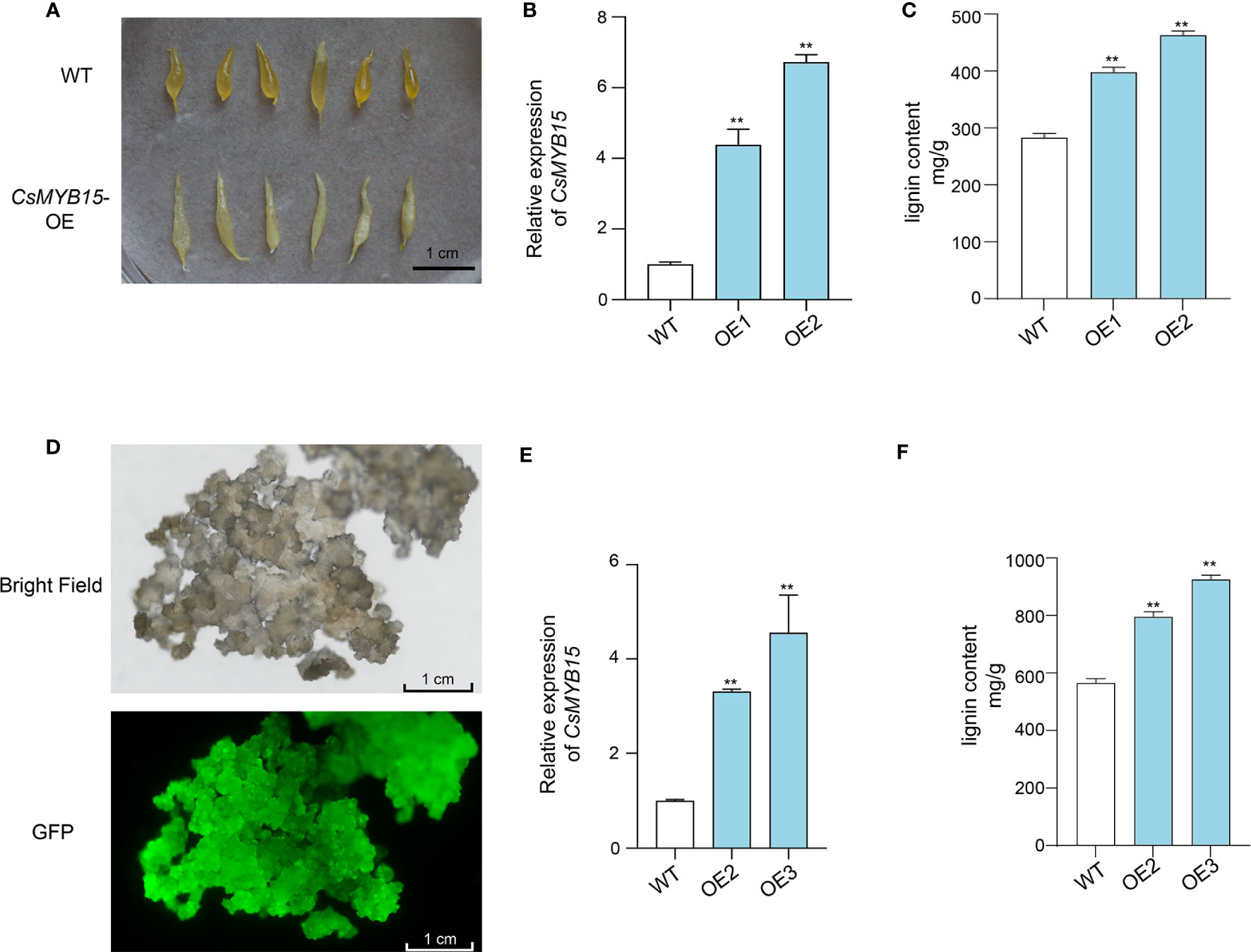
Figure 5 Transient expression of CsMYB15 in navel orange juice sacs and stable expression of CsMYB15 in navel orange calli. (A). The morphology of transgenic juice sacs over-expressing CsMYB15 was slim, deformed, and granulated. (B). Relative expression levels of CsMYB15 in WT and two transgenic juice sac lines over-expressing CsMYB15. (C). Lignin content in WT and two transgenic juice sac lines over-expressing CsMYB15. (D). Positive citrus calli over-expressing CsMYB15 under bright field and GFP fluorescence. (E). Relative expression level of CsMYB15 in WT and two transgenic citrus callus lines over-expressing CsMYB15. (F). Lignin content in WT and two transgenic citrus callus lines over-expressing CsMYB15. All data were expressed as mean ± SE. The asterisks indicate significant differences according to student’s t-test (** P < 0.01).
Then, CsMYB15 was over-expressed in citrus calli through Agrobacterium-mediated stable transformation system. Two independent transgenic lines were selected through GFP fluorescence (Figure 5D). The expression of CsMYB15 in representative lines was validated by qRT-PCR (Figure 5E). As expected, the lignin content was significantly higher in the transgenic citrus calli over-expressing CsMYB15 than in wide type calli (Figure 5F). Taken together, these results indicated that CsMYB15 positively regulated lignin biosynthesis in citrus.
3.6 CsMYB15 activates the expression of Cs4CL2 by directly binding to its promoter
We speculated that CsMYB15 could directly bind to the promoters of lignin biosynthesis genes, thus affecting their expression, eventually regulating the lignin biosynthesis. Thus, 2000 bp sequences upstream the initiation codon were cloned as the promoters of Cs4CL1, Cs4CL2, CsC3H, CsCCR2, CsPOD2 and CsPOD3, and the Yeast one-hybrid assay (Y1H) was conducted to verify the interaction between CsMYB15 and the promoters of these genes. As shown in Figure 6A, clone CsMYB15 + proCs4CL2 was grown well on the SD-leu + 75 ng/μL AbAi (aureobasidin A) medium, indicating that CsMYB15 could bind to the promoter of Cs4CL2. However, CsMYB15 did not interact with the promoters of Cs4CL1 (Figure S2), it did not interact with those of CsC3H, CsCCR2, CsPOD2 and CsPOD3, either (data not shown).
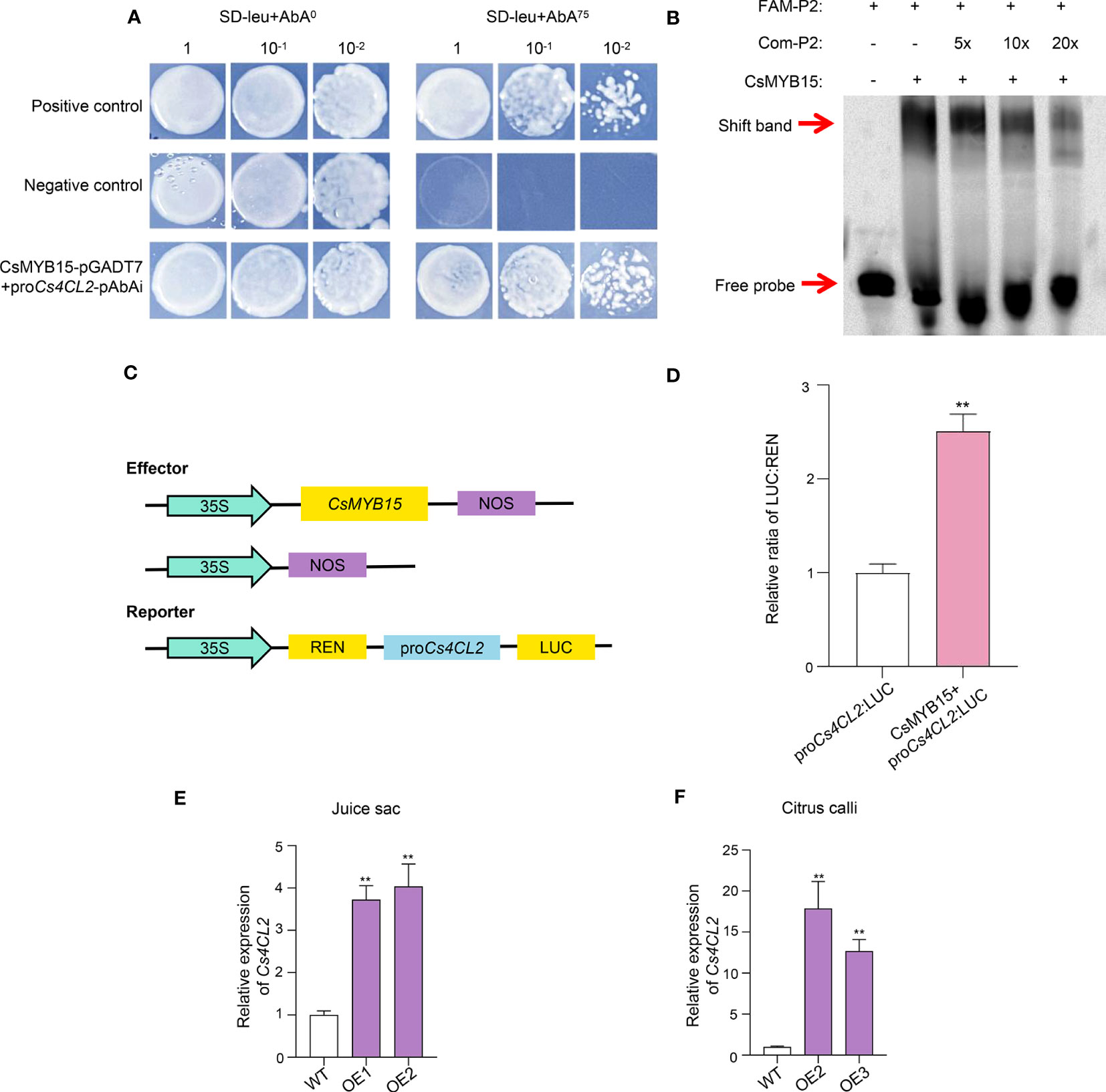
Figure 6 CsMYB15 directly binds to and transactivates the promoter of Cs4CL2. (A) CsMYB15 could interact with the promoter of Cs4CL2 in yeast one-hybrid assay. (B) CsMYB15 directly bound to the MRS elements in the promoter of Cs4CL2 according to EMSA assay. (C) Schematic diagram of effector and reporter structures used for dual luciferase assay. LUC, firefly luciferase; REN, Renilla luciferase. (D) CsMYB15 increased the activity of the Cs4CL2 promoter according to the LUC assay. (E) Relative expression levels of Cs4CL2 in WT and two transgenic juice sac lines over-expressing CsMYB15. (F) Relative expression levels of Cs4CL2 in WT and two transgenic citrus callus lines over-expressing CsMYB15. The asterisks indicate significant differences according to student’s t-test (** P < 0.01).
We predicted that CsMYB15 might bind to the MYB recognition sites (MRS, TGGTTG/A) on the Cs4CL2 promoter. To verify our prediction, the electrophoretic mobility shift assay (EMSA) was performed using purified MBP-CsMYB15 protein with a FAM-labeled Cs4CL2 promoter fragment (−51 to −89 bp region) used as a probe. As shown in Figure 6B, a shifted band was observed after the probe was incubated with MBP-MYB15, but this band became weaker under the actions of the corresponding competitors in a dosage-dependent manner. These results further demonstrated that CsMYB15 directly bound to the MRS cis-element of Cs4CL2 promoter in vitro.
In addition, a dual luciferase transcriptional activity assay (LUC) was performed in N. benthamiana leaf to reveal how CsMYB15 regulated the expression of Cs4CL2. The LUC: renillia (REN) ratio of leaf co-expressing CsMYB15 and Cs4CL2 promoter was significantly higher than that of the negative control, indicating that CsMYB15 acted as transcriptional activators of Cs4CL2 by directly interacting with the MRS elements (Figures 6C, D). Further, we detected the expression patterns of Cs4CL2 in the transgenic juice sac and citrus callus lines using qRT-PCR. As shown in Figures 6E, F, the expression level of Cs4CL2 was significantly higher in the transgenic plant lines over-expressing CsMYB15 than the wide type, which was further proof of the activation of CsMYB15 transcription factor on the Cs4CL2.
4 Discussion
Juice sac granulation is a severe physiological disorder in citrus fruit, causing dramatic decline in fruit quality and commercial value (Shi et al., 2020; Wu et al., 2020). In recent years, extensive studies have been conducted to elucidate the physiological and molecular mechanisms underlying the juice sac granulation (Wu et al., 2014; Yao et al., 2018; Jia et al., 2019; Shi et al., 2020; Zhang et al., 2021). However, most of existing studies focus on post-harvest juice sac granulation, the molecular regulating network of low temperature-induced pre-harvest juice sac granulation remains largely unknown. In this study, we identified SG2-type R2R3-MYB transcription factor CsMYB15 as a positive regulator of lignin biosynthesis, and CsMYB15 could directly bind to the Cs4CL2 promoter to activate its transcription during low temperature-induced juice sac granulation in late-maturing navel orange.
Low temperature is one of the major abiotic stress limiting the plant growth, development, quality, and yield (Ma et al., 2015; Ding et al., 2020). In horticultural plants, low temperature usually causes granulation of the chilling sensitive fruits such as loquat (Cai et al., 2006; Zhang et al., 2020a), kiwifruit (Suo et al., 2018), pear (Lu et al., 2014), and zucchini (Carvajal et al., 2015). Our previous study has shown that low temperature in winter induced pre-harvest juice sac granulation in ‘Lane late’ navel orange by affecting the cell wall metabolism and increasing the accumulation of lignin (Wu et al., 2020). MYB transcription factors play critical roles in plant stress responses, especially abiotic stress responses including cold (Agarwal et al., 2006; An et al., 2018), drought (Nakabayashi et al., 2013; Zhao et al., 2018), and salt stress responses (Sun et al., 2020; Zhang et al., 2020c). Here, CsMYB15 was identified as a typical SG2-type R2R3 MYB transcription factor in Citrus sinensis. Our phylogenetic analysis revealed that CsMYB15 was the orthologous gene of AtMYB15. In agreement with previous report that plants exhibited increased accumulation of MYB15 transcript in response to cold stress in both Arabidopsis (Agarwal et al., 2006) and tomato (Zhang et al., 2020b), we also found that CsMYB15 expression was up-regulated under low temperature treatment in navel orange fruits, and CsMYB15 was highly expressed in the granulated navel orange fruits, and its expression level was increased with the increasing severity of juice sac granulation. Additionally, CsMYB15 protein was localized to the nucleus and had transcriptional activation activity in yeast. Thus, CsMYB15 might act as a low temperature-induced transcriptional activator involved in juice sac granulation.
MYB TFs play important roles in regulating lignin biosynthesis in plants (Taylor-Teeples et al., 2014; Liu et al., 2015; Chen et al., 2019; Xiao et al., 2021). In Pinus taeda, PtMYB4 can induce lignication during wood formation (Patzlaff et al., 2003). In Populus trichocarpa, over-expression of PtrMYB3 and PtrMYB20, and PtoMYB216 activates the expression of the upstream genes in the lignin biosynthetic pathway, thus resulting in the lignin deposition. In Arabidopsis thaliana, AtMYB58, AtMYB63, and AtMYB85 regulate the lignification in vascular tissues by binding to the AC-rich elements in the promoters of lignin biosynthesis genes (Zhong et al., 2008; Zhou et al., 2009). AtMYB15, a homologous gene of CsMYB15, increases the biosynthesis of G-lignin to promote defence-induced lignification and basal immunity under pathogen infection (Chezem et al., 2017; Kim et al., 2020). The present study showed that over-expression of CsMYB15 led to an increase in the lignin content in all the transgenic juice sacs, kumquat fruits, and citrus calli, indicating that CsMYB15 positively regulated lignin biosynthesis in citrus. Our Y1H, EMSA, and LUC assays further demonstrated that CsMYB15 could bind directly to the MRS element in the promoter of Cs4CL2 to activate its expression. However, CsMYB15 failed to bind to the MRS element in the promoter of Cs4CL1, indicating that CsMYB15 might not bind to all the promoters containing MRS element. Similar results have also been reported in Arabidopsis and Citrus. AtMYB58 and AtMYB63 bound to and activated the promoter of AtLAC4, but did not bind to the promoter of AtLAC17 (Zhou et al., 2009). CsMYB330 and CsMYB308 bound to AC elements in the Cs4CL1 promoter, but not to the promoters of CsCCoAOMT1, CsPAL1, and CsPAL2 although these promoters also contained AC elements (Jia et al., 2018).
Previous studies have shown that the lignin biosynthesis pathway is centrally involved in the juice sac granulation, and four R2R3-MYB transcription factors have been identified to be involved in the post-harvest juice sac granulation process in sweet orange and pummelo by regulating lignin biosynthesis, including CgMYB58, CsMYB85 (homolog of CgMYB58), CsMYB330, and CsMYB308. In this study, a novel SG2-type R2R3-MYB transcription factor, CsMYB15, was identified as a transcriptional activator of lignin biosynthetic gene Cs4CL2, and it could directly bind to its promoter, thereby resulting in the accumulation of lignin during low temperature-induced pre-harvest juice sac granulation. Our phylogenetic analysis demonstrated that CsMYB15 was clearly separated from the reported four MYB transcription factors involved in the citrus juice sac granulation, and CsMYB15 was specifically induced by low temperature, indicating that CsMYB15-mediated lignin biosynthesis regulation network as a novel one was specific to the low temperature-induced pre-harvest juice sac granulation process.
In conclusion, we proposed a working model in which CsMYB15 regulated lignin biosynthesis during low temperature-induced juice sac granulation process. CsMYB15 was activated by cold stress in the winter, and CsMYB15 transcriptionally activated the lignin biosynthetic gene Cs4CL2 by directly binding to its promoter, thus promoting the accumulation of lignin in juice sacs, ultimately leading to the lignification of juice sacs in navel orange (Figure 7). Our findings advance our understanding of MYB transcriptional factor-mediated lignin biosynthesis regulation network during low temperature-induced pre-harvest juice sac granulation in late-maturing navel orange.
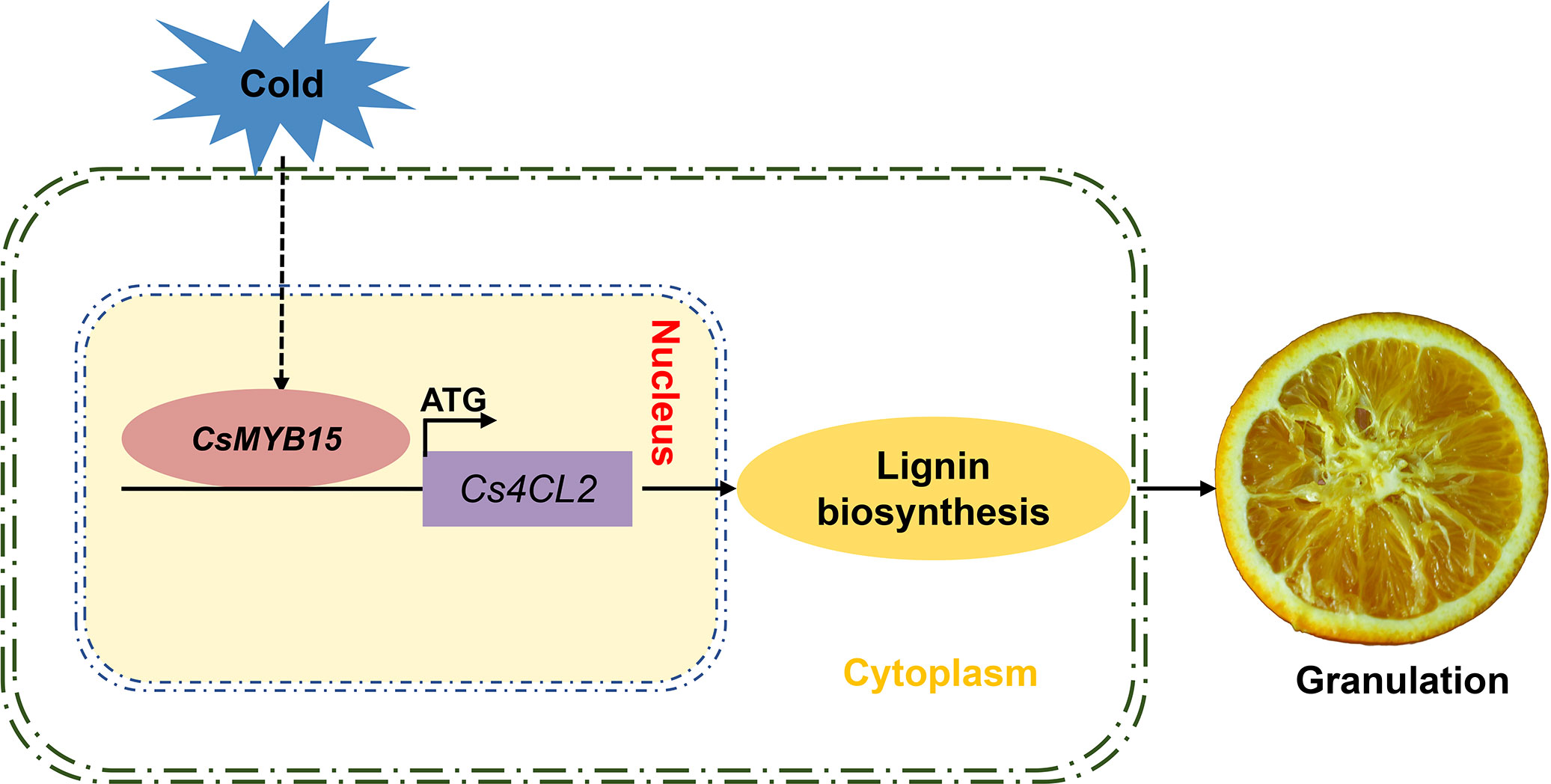
Figure 7 A proposed working model in which CsMYB15 regulates lignin biosynthesis during juice sac granulation in late-maturing navel orange. In this model, CsMYB15 is activated by cold stress in the winter, and then CsMYB15 directly binds to the promoter region of lignin biosynthesis gene Cs4CL2 and induces its expression, leading to lignin biosynthesis, ultimately causing juice sac granulation in navel orange.
Data availability statement
The original contributions presented in the study are included in the article/Supplementary Material. Further inquiries can be directed to the corresponding authors.
Author contributions
FS, and LW conceived and designed the experiment. FS and ZL conducted the experiment and data analysis. YJ, CW, ZW, LH and XM contributed to the data analysis. FS and LW wrote the manuscript. YZ, XS and J-HL revised the manuscript. All authors contributed to the article and approved the final version.
Funding
This research was funded by National Natural Science Foundation of China (32102309), Hubei Provincial Academy of Agricultural Sciences Youth Top Talent Training Program and Hubei Provincial Agricultural Science and Technology Innovation Fund (2019-620-000-001-023). Sincere gratitude goes to Professor Ping Liu from Huazhong Agriculture University, Wuhan, China for her English editing and language polishing of this manuscript.
Conflict of interest
The authors declare that the research was conducted in the absence of any commercial or financial relationships that could be construed as a potential conflict of interest.
Publisher’s note
All claims expressed in this article are solely those of the authors and do not necessarily represent those of their affiliated organizations, or those of the publisher, the editors and the reviewers. Any product that may be evaluated in this article, or claim that may be made by its manufacturer, is not guaranteed or endorsed by the publisher.
Supplementary material
The Supplementary Material for this article can be found online at: https://www.frontiersin.org/articles/10.3389/fpls.2023.1223820/full#supplementary-material
Supplementary Figure 1 | Transient expression of CsMYB15 in kumquat fruits. (A). Injection part of kumquat fruits. (B). Relative expression levels of CsMYB15 in WT and two transgenic kumquat fruit lines over-expressing CsMYB15. (C). Lignin contents were measured in WT and two transgenic kumquat fruit lines over-expressing CsMYB15. All data were expressed as mean ± SE. The asterisks indicate significant differences according to student’s t-test (** P < 0.01).
Supplementary Figure 2 | CsMYB15 does not interact with the promoter of Cs4CL1 in yeast one-hybrid assay.
References
Agarwal, M., Hao, Y., Kapoor, A., Dong, C.-H., Fujii, H., Zheng, X., et al. (2006). A R2R3 type MYB transcription factor is involved in the cold regulation of CBF genes and in acquired freezing tolerance. J. Biol. Chem. 281, 37636–37645. doi: 10.1074/jbc.m605895200
An, J.-P., Li, R., Qu, F.-J., You, C.-X., Wang, X.-F., Hao, Y.-J. (2018). R2R3-MYB transcription factor MdMYB23 is involved in the cold tolerance and proanthocyanidin accumulation in apple. Plant J. 96, 562–577. doi: 10.1111/tpj.14050
Burns, J. K., Albrigo, L. G. (1998). Time of harvest and method of storage affect granulation in grapefruit. HortSci 33, 728–730. doi: 10.21273/hortsci.33.4.728
Cai, C., Xu, C., Shan, L., Li, X., Zhou, C., Zhang, W., et al. (2006). Low temperature conditioning reduces postharvest chilling injury in loquat fruit. Postharvest Biol. Technol. 41, 252–259. doi: 10.1016/j.postharvbio.2006.04.015
Carvajal, F., Palma, F., Jamilena, M., Garrido, D. (2015). Cell wall metabolism and chilling injury during postharvest cold storage in zucchini fruit. Postharvest Biol. Technol. 108, 68–77. doi: 10.1016/j.postharvbio.2015.05.013
Chang, C.-H., Hsu, F.-C., Lee, S.-C., Lo, Y.-S., Wang, J.-D., Shaw, J., et al. (2016). The nucleolar fibrillarin protein is required for helper virus-independent long-distance trafficking of a subviral satellite RNA in plants. Plant Cell 28, 2586–2602. doi: 10.1105/tpc.16.00071
Chen, C., Nie, Z., Wan, C., Gan, Z., Chen, J. (2021). Suppression on postharvest juice sac granulation and cell wall modification by chitosan treatment in harvested pummelo (Citrus grandis l. osbeck) stored at room temperature. Food Chem. 336, 127636. doi: 10.1016/j.foodchem.2020.127636
Chen, H., Wang, J. P., Liu, H., Li, H., Lin, Y.-C. J., Shi, R., et al. (2019). Hierarchical transcription factor and chromatin binding network for wood formation in populus trichocarpa. Plant Cell 31, 602–626. doi: 10.1105/tpc.18.00620
Chezem, W. R., Memon, A., Li, F.-S., Weng, J.-K., Clay, N. K. (2017). SG2-type R2R3-MYB transcription factor MYB15 controls defense-induced lignification and basal immunity in arabidopsis. Plant Cell 29, 1907–1926. doi: 10.1105/tpc.16.00954
Ding, Y., Shi, Y., Yang, S. (2020). Molecular regulation of plant responses to environmental temperatures. Mol. Plant 13, 544–564. doi: 10.1016/j.molp.2020.02.004
Edgar, R. C. (2004). MUSCLE: multiple sequence alignment with high accuracy and high throughput. Nucleic Acids Res. 32, 1792–1797. doi: 10.1093/nar/gkh340
Engler, C., Youles, M., Gruetzner, R., Ehnert, T.-M., Werner, S., Jones, J. D. G., et al. (2014). A golden gate modular cloning toolbox for plants. ACS Synth. Biol. 3, 839–843. doi: 10.1021/sb4001504
Geng, P., Zhang, S., Liu, J., Zhao, C., Wu, J., Cao, Y., et al. (2019). MYB20, MYB42, MYB43, and MYB85 regulate phenylalanine and lignin biosynthesis during secondary cell wall formation. Plant Physiol. 182, 1272–1283. doi: 10.1104/pp.19.01070
Gong, J., Tian, Z., Qu, X., Meng, Q., Guan, Y., Liu, P., et al. (2021a). Illuminating the cells: transient transformation of citrus to study gene functions and organelle activities related to fruit quality. Hortic. Res. 8, 175. doi: 10.1038/s41438-021-00611-1
Gong, J., Zeng, Y., Meng, Q., Guan, Y., Li, C., Yang, H., et al. (2021b). Red light-induced kumquat fruit coloration is attributable to increased carotenoid metabolism regulated by FcrNAC22. EXBOTJ 72, 6274–6290. doi: 10.1093/jxb/erab283
Hellens, R., Allan, A., Friel, E., Bolitho, K., Grafton, K., Templeton, M., et al. (2005). Transient expression vectors for functional genomics, quantification of promoter activity and RNA silencing in plants. Plant Methods. vol. 13. doi: 10.1186/1746-4811-1-13
Jia, N., Liu, J., Sun, Y., Tan, P., Cao, H., Xie, Y., et al. (2018). Citrus sinensis MYB transcription factors CsMYB330 and CsMYB308 regulate fruit juice sac lignification through fine-tuning expression of the Cs4CL1 gene. Plant Sci. 277, 334–343. doi: 10.1016/j.plantsci.2018.10.006
Jia, N., Liu, J., Tan, P., Sun, Y., Lv, Y., Liu, J., et al. (2019). Citrus sinensis MYB transcription factor CsMYB85 induce fruit juice sac lignification through interaction with other CsMYB transcription factors. Front. Plant Sci. 10. doi: 10.3389/fpls.2019.00213
Kim, W.-C., Kim, J.-Y., Ko, J.-H., Kang, H., Han, K.-H. (2014). Identification of direct targets of transcription factor MYB46 provides insights into the transcriptional regulation of secondary wall biosynthesis. Plant Mol. Biol. 85, 589–599. doi: 10.1007/s11103-014-0205-x
Kim, W.-C., Ko, J.-H., Han, K.-H. (2012). Identification of a cis-acting regulatory motif recognized by MYB46, a master transcriptional regulator of secondary wall biosynthesis. Plant Mol. Biol. 78, 489–501. doi: 10.1007/s11103-012-9880-7
Kim, S. H., Lam, P. Y., Lee, M.-H., Jeon, H. S., Tobimatsu, Y., Park, O. K. (2020). The arabidopsis R2R3 MYB transcription factor MYB15 is a key regulator of lignin biosynthesis in effector-triggered immunity. Front. Plant Sci. 11. doi: 10.3389/fpls.2020.583153
Ko, J.-H., Kim, W.-C., Han, K.-H. (2009). Ectopic expression of MYB46 identifies transcriptional regulatory genes involved in secondary wall biosynthesis in arabidopsis. Plant J. 60, 649–665. doi: 10.1111/j.1365-313x.2009.03989.x
Kubo, M., Udagawa, M., Nishikubo, N., Horiguchi, G., Yamaguchi, M., Ito, J., et al. (2005). Transcription switches for protoxylem and metaxylem vessel formation. Genes Dev. 19, 1855–1860. doi: 10.1101/gad.1331305
Kumar, S., Stecher, G., Li, M., Knyaz, C., Tamura, K. (2018). MEGA X: molecular evolutionary genetics analysis across computing platforms. Mol. Biol. Evol. 35, 1547–1549. doi: 10.1093/molbev/msy096
Letunic, I., Bork, P. (2016). Interactive tree of life (iTOL) v3: an online tool for the display and annotation of phylogenetic and other trees. Nucleic Acids Res. 44, W242–W245. doi: 10.1093/nar/gkw290
Li, Z., Wang, C., Long, D., Jiang, Y., He, L., Wang, Z., et al. (2022). Genome-wide identification, bioinformatics characterization and functional analysis of pectin methylesterase inhibitors related to low temperature-induced juice sac granulation in navel orange (Citrus sinensis osbeck). Scientia Hortic. 298, 110983. doi: 10.1016/j.scienta.2022.110983
Liu, J., Osbourn, A., Ma, P. (2015). MYB transcription factors as regulators of phenylpropanoid metabolism in plants. Mol. Plant 8, 689–708. doi: 10.1016/j.molp.2015.03.012
Lu, G., Li, Z., Zhang, X., Wang, R., Yang, S. (2014). Expression analysis of lignin-associated genes in hard end pear (Pyrus pyrifolia whangkeumbae) and its response to calcium chloride treatment conditions. J. Plant Growth Regul. 34, 251–262. doi: 10.1007/s00344-014-9461-x
Lu, S., Zhang, Y., Zhu, K., Yang, W., Ye, J., Chai, L., et al. (2018). The citrus transcription factor CsMADS6 modulates carotenoid metabolism by directly regulating carotenogenic genes. Plant Physiol. 176, 2657–2676. doi: 10.1104/pp.17.01830
Ma, Q., Dai, X., Xu, Y., Guo, J., Liu, Y., Chen, N., et al. (2009). Enhanced tolerance to chilling stress in OsMYB3R-2 transgenic rice is mediated by alteration in cell cycle and ectopic expression of stress genes. Plant Physiol. 150, 244–256. doi: 10.1104/pp.108.133454
Ma, Y., Dai, X., Xu, Y., Luo, W., Zheng, X., Zeng, D., et al. (2015). COLD1 confers chilling tolerance in rice. Cell 160, 1209–1221. doi: 10.1016/j.cell.2015.01.046
McCarthy, R. L., Zhong, R., Ye, Z.-H. (2009). MYB83 is a direct target of SND1 and acts redundantly with MYB46 in the regulation of secondary cell wall biosynthesis in arabidopsis. Plant Cell. Physiol. 50, 1950–1964. doi: 10.1093/pcp/pcp139
Mitsuda, N., Iwase, A., Yamamoto, H., Yoshida, M., Seki, M., Shinozaki, K., et al. (2007). NAC transcription factors, NST1 and NST3, are key regulators of the formation of secondary walls in woody tissues of arabidopsis. Plant Cell 19, 270–280. doi: 10.1105/tpc.106.047043
Nakabayashi, R., Yonekura-Sakakibara, K., Urano, K., Suzuki, M., Yamada, Y., Nishizawa, T., et al. (2013). Enhancement of oxidative and drought tolerance in arabidopsis by overaccumulation of antioxidant flavonoids. Plant J. 77, 367–379. doi: 10.1111/tpj.12388
Ohtani, M., Demura, T. (2019). The quest for transcriptional hubs of lignin biosynthesis: beyond the NAC-MYB-gene regulatory network model. Curr. Opin. Biotechnol. 56, 82–87. doi: 10.1016/j.copbio.2018.10.002
Patzlaff, A., McInnis, S., Courtenay, A., Surman, C., Newman, L. J., Smith, C., et al. (2003). Characterisation of a pine MYB that regulates lignification. Plant J. 36, 743–754. doi: 10.1046/j.1365-313x.2003.01916.x
Sharma, R. R., Awasthi, O. P., Kumar, K. (2015). Pattern of phenolic content, antioxidant activity and senescence-related enzymes in granulated vs non-granulated juice-sacs of ‘Kinnow’ mandarin (Citrus nobilis x c. deliciosa). J. Food Sci. Technol. 53, 1525–1530. doi: 10.1007/s13197-015-2112-9
Shi, C.-Y., Hussain, S. B., Yang, H., Bai, Y.-X., Khan, M. A., Liu, Y.-Z. (2019). CsPH8, a p-type proton pump gene, plays a key role in the diversity of citric acid accumulation in citrus fruits. Plant Sci. 289, 110288. doi: 10.1016/j.plantsci.2019.110288
Shi, M., Liu, X., Zhang, H., He, Z., Yang, H., Chen, J., et al. (2020). The IAA- and ABA-responsive transcription factor CgMYB58 upregulates lignin biosynthesis and triggers juice sac granulation in pummelo. Hortic. Res. 7, 139. doi: 10.1038/s41438-020-00360-7
Sparkes, I. A., Runions, J., Kearns, A., Hawes, C. (2006). Rapid, transient expression of fluorescent fusion proteins in tobacco plants and generation of stably transformed plants. Nat. Protoc. 1, 2019–2025. doi: 10.1038/nprot.2006.286
Sun, Y., Zhao, J., Li, X., Li, Y. (2020). E2 conjugases UBC1 and UBC2 regulate MYB42-mediated SOS pathway in response to salt stress in arabidopsis. New Phytol. 227, 455–472. doi: 10.1111/nph.16538
Suo, J., Li, H., Ban, Q., Han, Y., Meng, K., Jin, M., et al. (2018). Characteristics of chilling injury-induced lignification in kiwifruit with different sensitivities to low temperatures. Postharvest Biol. Technol. 135, 8–18. doi: 10.1016/j.postharvbio.2017.08.020
Taylor-Teeples, M., Lin, L., de Lucas, M., Turco, G., Toal, T. W., Gaudinier, A., et al. (2014). An arabidopsis gene regulatory network for secondary cell wall synthesis. Nature 517, 571–575. doi: 10.1038/nature14099
Theanjumpol, P., Wongzeewasakun, K., Muenmanee, N., Wongsaipun, S., Krongchai, C., Changrue, V., et al. (2019). Non-destructive identification and estimation of granulation in ‘Sai num pung’ tangerine fruit using near infrared spectroscopy and chemometrics. Postharvest Biol. Technol. 153, 13–20. doi: 10.1016/j.postharvbio.2019.03.009
Wang, X.-Y., Wang, P., Qi, Y.-P., Zhou, C.-P., Yang, L.-T., Liao, X.-Y., et al. (2014). Effects of granulation on organic acid metabolism and its relation to mineral elements in citrus grandis juice sacs. Food Chem. 145, 984–990. doi: 10.1016/j.foodchem.2013.09.021
Wu, J.-L., Pan, T.-F., Guo, Z.-X., Pan, D.-M. (2014). Specific lignin accumulation in granulated juice sacs of citrus maxima. J. Agric. Food Chem. 62, 12082–12089. doi: 10.1021/jf5041349
Wu, L.-M., Wang, C., He, L.-G., Wang, Z.-J., Tong, Z., Song, F., et al. (2020). Transcriptome analysis unravels metabolic and molecular pathways related to fruit sac granulation in a late-ripening navel orange (Citrus sinensis osbeck). Plants 9, 95. doi: 10.3390/plants9010095
Xiao, R., Zhang, C., Guo, X., Li, H., Lu, H. (2021). MYB transcription factors and its regulation in secondary cell wall formation and lignin biosynthesis during xylem development. IJMS 22, 3560. doi: 10.3390/ijms22073560
Xie, G., Yang, C., Fei, Y., Ma, L. (2020). Physiological and proteomic analyses of 1-MCP treatment on lignification in fresh common bean (Phaseolus vulgaris l) during storage. Postharvest Biol. Technol. 160, 111041. doi: 10.1016/j.postharvbio.2019.111041
Yang, B., Fang, X., Han, Y., Liu, R., Chen, H., Gao, H. (2022). Analysis of lignin metabolism in water bamboo shoots during storage. Postharvest Biol. Technol. 192, 111989. doi: 10.1016/j.postharvbio.2022.111989
Yao, S., Cao, Q., Xie, J., Deng, L., Zeng, K. (2018). Alteration of sugar and organic acid metabolism in postharvest granulation of ponkan fruit revealed by transcriptome profiling. Postharvest Biol. Technol. 139, 2–11. doi: 10.1016/j.postharvbio.2018.01.003
Zhang, L., Jiang, X., Liu, Q., Ahammed, G. J., Lin, R., Wang, L., et al. (2020b). The HY5 and MYB15 transcription factors positively regulate cold tolerance in tomato via the CBF pathway. Plant Cell Environ. 43, 2712–2726. doi: 10.1111/pce.13868
Zhang, Q., Wang, L., Wang, Z., Zhang, R., Liu, P., Liu, M., et al. (2021). The regulation of cell wall lignification and lignin biosynthesis during pigmentation of winter jujube. Hortic. Res. 8, 238. doi: 10.1038/s41438-021-00670-4
Zhang, P., Wang, R., Yang, X., Ju, Q., Li, W., Lü, S., et al. (2020c). The R2R3-MYB transcription factor AtMYB49 modulates salt tolerance in arabidopsis by modulating the cuticle formation and antioxidant defence. Plant Cell Environ. 43, 1925–1943. doi: 10.1111/pce.13784
Zhang, J., Yin, X., Li, H., Xu, M., Zhang, M., Li, S., et al. (2020a). ETHYLENE RESPONSE FACTOR39–MYB8 complex regulates low-temperature-induced lignification of loquat fruit. EXBOTJ 71, 3172–3184. doi: 10.1093/jxb/eraa085
Zhao, Y., Cheng, X., Liu, X., Wu, H., Bi, H., Xu, H. (2018). The wheat MYB transcription factor TaMYB31 is involved in drought stress responses in arabidopsis. Front. Plant Sci. 9. doi: 10.3389/fpls.2018.01426
Zhong, R., Lee, C., Zhou, J., McCarthy, R. L., Ye, Z.-H. (2008). A battery of transcription factors involved in the regulation of secondary cell wall biosynthesis in arabidopsis. Plant Cell 20, 2763–2782. doi: 10.1105/tpc.108.061325
Zhong, R., Richardson, E. A., Ye, Z.-H. (2007). The MYB46 transcription factor is a direct target of SND1 and regulates secondary wall biosynthesis in arabidopsis. Plant Cell 19, 2776–2792. doi: 10.1105/tpc.107.053678
Zhong, R., Ye, Z.-H. (2011). MYB46 and MYB83 bind to the SMRE sites and directly activate a suite of transcription factors and secondary wall biosynthetic genes. Plant Cell. Physiol. 53, 368–380. doi: 10.1093/pcp/pcr185
Keywords: ‘Lane late’ navel orange, granulation, transcription factor, CsMYB15, lignin biosynthesis
Citation: Song F, Li Z, Wang C, Jiang Y, Wang Z, He L, Ma X, Zhang Y, Song X, Liu J and Wu L (2023) CsMYB15 positively regulates Cs4CL2-mediated lignin biosynthesis during juice sac granulation in navel orange. Front. Plant Sci. 14:1223820. doi: 10.3389/fpls.2023.1223820
Received: 16 May 2023; Accepted: 19 June 2023;
Published: 30 June 2023.
Edited by:
Lijun Wang, Chinese Academy of Sciences (CAS), ChinaReviewed by:
Yuanyue Shen, Beijing University of Agriculture, ChinaWenjun Huang, Chinese Academy of Sciences (CAS), China
Copyright © 2023 Song, Li, Wang, Jiang, Wang, He, Ma, Zhang, Song, Liu and Wu. This is an open-access article distributed under the terms of the Creative Commons Attribution License (CC BY). The use, distribution or reproduction in other forums is permitted, provided the original author(s) and the copyright owner(s) are credited and that the original publication in this journal is cited, in accordance with accepted academic practice. No use, distribution or reproduction is permitted which does not comply with these terms.
*Correspondence: Jihong Liu, liujihong@mail.hzau.edu.cn; Liming Wu, wuliming2005@126.com
†These authors have contributed equally to this work