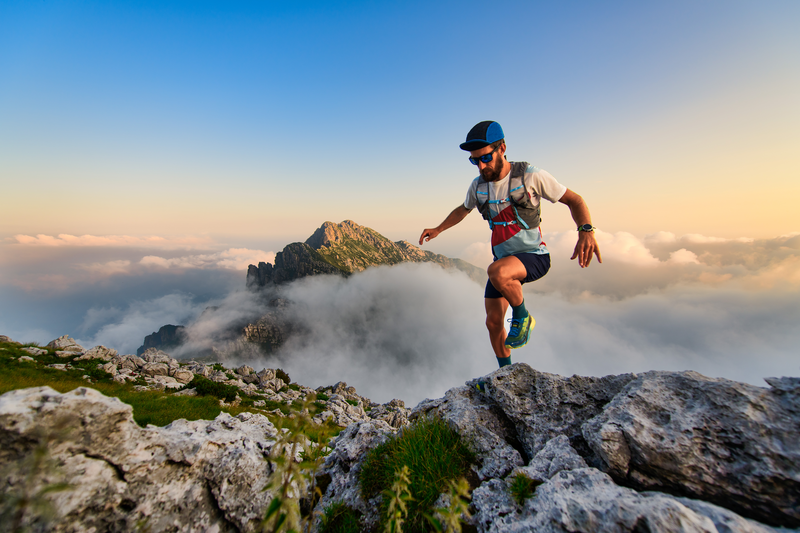
95% of researchers rate our articles as excellent or good
Learn more about the work of our research integrity team to safeguard the quality of each article we publish.
Find out more
ORIGINAL RESEARCH article
Front. Plant Sci. , 04 September 2023
Sec. Crop and Product Physiology
Volume 14 - 2023 | https://doi.org/10.3389/fpls.2023.1222755
The duration of soybean growth and development is regulated by E1-E4 allele genes, which form the basis for ecological adaptation related to biomass accumulation, flowering and pod formation, maturation, and yield. To elucidate the effects of different combinations of E1-E4 allele genes on soybean ecological adaptation, this study conducted competitive allele-specific PCR (KASP) analysis and photoperiod gene typing on 101 main soybean cultivars from different latitudes in China. The ecological adaptation of these cultivars in Sichuan was also investigated. The results showed that within a certain range (60-95 days), soybean varieties with a genotype combination of E1/e2-ns/E3/E4 exhibited a longer growth period and demonstrated higher biomass and yield, displaying overall better performance. These varieties showed strong ecological adaptation in the Chengdu Plain region and are suitable for introduction in similar low to mid-latitude areas like the Chengdu Plain (30°N~32°N). Conversely, soybean varieties carrying a higher number of recessive alleles of E1-E4 are not suitable for introduction in this region.
Soybean (Glycine max (Linn.) Merr), originally from China, is currently cultivated worldwide and is an important grain and oil crop. In 2022, China’s soybean production reached 20.285 million tons, showing a 23.7% increase compared to the previous year. The Food and Agriculture Organization of the United Nations (FAO) predicts that the global population will reach 10 billion by 2050, necessitating a substantial increase in grain and oil production to meet future food demands. Soybean is a short-day plant that exhibits high sensitivity to photoperiod (Hadley et al., 1984). Photoperiodic response is one of the important factors influencing soybean’s regional adaptation and yield. Flowering marks the transition of soybean from the vegetative growth phase to the reproductive growth phase. The duration of growth and development is a crucial ecological trait for crops (Wang, 1981). Soybean varieties originating from different latitudes and environmental conditions exhibit varying photoperiod sensitivities. With the advancement of molecular biology techniques, molecular markers have become important tools for selecting target traits in crop varieties. Competitive allele-specific PCR (KASP) genotyping technology enables high-throughput screening of large populations and plays a significant role in gene mapping and germplasm diversity analysis (Grogan et al., 2016; Wu et al., 2018). Fang et al. applied the KASP genotyping technology to soybean photoperiod genes and used it to predict the adaptation regions of high-yielding soybean lines (Fang et al., 2021).
The growth period of soybean is mainly determined by factors that control its flowering and maturity (Bernard, 1971). The length of the growth period is the most fundamental characteristic for the formation of regional and seasonal types of soybean varieties (Hao et al., 1992). Studies by Han Tianfu et al. have shown that different soybean varieties exhibit significant differences in their growth period structure, but the proportion of flowering-podding stages (R1-R5) and filling stage (R6) in soybean varieties with the same growth period and growth period structure ratio also varies (Han et al., 1998; Wang et al., 2015). The entire growth period, vegetative growth period (V), reproductive growth period (R), and growth period structure (R/V) constitute the growth period traits of soybean, and selective breeding of growth period structure can improve the selection efficiency for soybean yield (Fehr, 1977; Sun et al., 1990). However, genetic variation in the main effect genes and quantitative trait loci (QTLs) that control delayed flowering and maturity enables soybean to have broad ecological adaptability (Garner and Allard, 1920). Based on relevant studies, it is known that the various allelic genes of E1-E4 are the foundation for soybean flowering, maturity, and ecological adaptability. The soybean growth period gene E1 is the first classic soybean maturity locus discovered, with the greatest impact on flowering and maturity periods, and all low-latitude varieties have E1. The E2 gene has a small response to photoperiod, but a large contribution to growth period and stronger geographical adaptability, while E3 and E4 have significant correlations with photoperiod sensitivity. Under long day conditions, E3 and E4 have different sensitivities to R:FR, inducing the expression of E1 and E1L (Watanabe et al., 2009; Jiang et al., 2014; Zhai et al., 2014; Kurasch et al., 2017). In natural environments, E3 has a significant impact on flowering over a wide range of latitudes, while the impact of E4 is limited to high latitude areas (Lu et al., 2015).
The flowering time determines the adaptability of soybean to different ecological regions. As a short-day photoperiod-sensitive crop, soybean is highly sensitive to the duration of sunlight, and the critical photoperiod of varieties decreases from high latitudes to low latitudes, resulting in a narrow ecological adaptability of each soybean variety (Fehr, 1987; Câmara et al., 1997; Agarwal et al., 2013). Under short-day conditions, photoperiod-insensitive varieties have early flowering and maturation, resulting in reduced yield (Spehar, 1995), while being adapted to high-latitude regions (Tsubokura et al., 2013). For example, soybean in high-latitude regions must flower under long daylight conditions in early summer and mature before autumn frost. However, in high-latitude regions, soybean flowering and maturation are often delayed. The growth period of soybean can be shortened by reducing sensitivity to long daylight. In low-latitude tropical and subtropical regions, high temperatures and short daylight promote rapid flowering and early maturity in photoperiod-insensitive soybean varieties, resulting in a short vegetative growth period and low yield. Therefore, delaying flowering is necessary in low-latitude regions to maintain a longer vegetative growth period and achieve high yield (Liu et al., 2008). Photoperiod genes play a crucial role in expanding the adaptability of soybean varieties to different latitude regions.
In this study, a total of 101 soybean (Glycine max (Linn.) Merr.) varieties from 21 provinces in 7 regions of China were collected for genotyping (Table S1).
The 101 soybean varieties collected from 21 provinces in 7 regions of China were field-tested in 2021-2022 at Chongzhou (Sichuan Province, China) (30°33′N, 103°39′E), for phenotypic identification. The daily average sunlight duration during the growth period of the test site was about 13.76 h. These varieties were planted according to a randomized complete block design, with a row spacing of 0.5 m, a plant spacing of 0.1 m, and a distance of 0.5 m between plots with three replicates. The average temperature, sunlight duration, and precipitation in Chongzhou varied as shown in Table 1. From April to August, the average temperature during the entire soybean growth period was 1.3°C higher in 2022 than in 2021, especially in the later growth period of soybean from early July to late August, where the temperature was 2.3°C higher in 2022 than in 2021. The longest sunlight duration in mid-late June was 14.12 h, and decreased to 12.97 h in late August (Table 1).
The whole growth period of soybean was recorded based on the identification method proposed by Fehr and Carviness (Fehr, 1977). The flowering period is from emergence (VE) to beginning of flowering (R1), and the maturation period is from emergence (VE) to initial maturity (R7). Three plants were selected for individual plant determination in each plot, and dynamic changes in plant height were measured weekly. For the full pod stage (R6), five plants were selected in each plot for measurement of plant height and aboveground biomass. At full maturity (R8), five plants were selected in each plot for measurement of yield traits such as single plant grain weight and effective pod number.
2.4 DNA extraction
Soybean DNA was extracted using a plant genomic DNA extraction kit (Solarbio, Beijing, https://www.solarbio.com). The OD260/280 and DNA concentration were measured using a micro-volume spectrophotometer (GE GeneQuant 100, General Electric Company, Boston, https://www.ge.com). DNA samples with OD260/280 ratios of 1.7-2.0 were selected and diluted to a concentration of 50-100 ng/μL with ddH2O.
The photoperiodic SNP markers and KASP genotyping primers (Table S2) were designed based on the study by Liu et al (Liu et al., 2020). The designed primers need to add a different linker sequence (FAM: 5’ GAAGGTGACCAGTTCATGCATGCT 3’ and HEX: 5’ GAAGGTCGGAGTCAACGGATT 3’) to enable identification by fluorescent probes labeled with FAM and HEX, respectively, and to produce fluorescent signals during PCR amplification. We performed using the Zhonghuang 13 (E1/e2- ns/E3/E4) and Zhonghuang 30 (e1-as/E2/E3/E4) (Liu et al., 2020; Lu et al., 2020) were used as positive controls during the KASP amplification process.
KASP amplification was performed using a real-time PCR instrument QuantStudio 6 Flex (Thermo Fisher Scientific, Massachusetts, https://corporate.thermofisher.com). For the KASP analysis, the mixed primers included 12 μL (100 μM) of each allele-specific forward primer, 30 μL (100 μM) of a common reverse primer, and 46 μL of ddH2O. Each 10 μL KASP reaction contained 1 μL of genomic DNA (50-100 ng/μL), 0.14 μL of the mixed primers, 5 μL of HiGeno 2x Probe Mix (Jasongen Biotech, Beijing, https://www.jasongen.com), and 4 μL of ddH2O. The amplification was carried out using the AQP™ genotyping system in a PCR cycling program: pre-denaturation at 95°C for 10 min, followed by 10 cycles of denaturation/annealing (95°C for 20 s, annealing temperature decreased from 65°C to 55°C by 0.6°C per cycle for 40 s), and then 34 additional cycles (95°C for 20 s, 55°C for 40 s). The fluorescent endpoint reading was performed at 35°C for 30 s.
OriginPro 2022b (https://www.originlab.com) and IBM SPSS Statistics 26.0 (https://ibm.com/products/spss-statistics) were used to perform analysis of variance (ANOVA) on different allele combinations of E1-E4 and agronomic traits. Based on the genotype results, conduct a comprehensive evaluation of different photoperiod gene types, soybean plant type traits, yield, and reproductive period structure using principal component analysis, cluster analysis, and other methods.
In the formula, α1 and α2 are the initial eigenvalues of the first and second components in principal component analysis, x1i and x2i represent the component matrix values of each trait indicator for components 1 and 2, zi is the standardized value of each sample trait indicator, b1 and b2 are the variances (%) of the initial eigenvalues of components 1 and 2, and y1 and y2 are the scores of each sample for components 1 and 2, respectively. y represents the comprehensive score of each sample’s principal components.
All 101 soybean varieties were genotyped for E1-E4 using KASP, and the genotyping results are shown in Figure 1. The photoperiodic genotypes of soybean varieties Zhonghuang 13 and Zhonghuang 30 used as positive controls were previously identified as E1/e2-ns/E3/E4 and e1-as/E2/E3/E4, respectively.
After KASP genotyping, the photoperiodic genotyping results of 101 soybean varieties from different regions of China were obtained (Table S1). According to Table S1, 74 soybean varieties had a photoperiodic genotype of E1/e2-ns/E3/E4, 7 varieties had a genotype of e1-as/E2/E3/E4, 11 varieties had a genotype of e1-as/e2-ns/E3/E4, 1 variety had a genotype of e1-as/e2-ns/e3-tr/E4, 2 varieties had a genotype of E1/e2-ns/e3-fs/E4, and 6 varieties had a genotype of E1/E2/E3/E4.
Soybean varieties from seven different latitude regions, including Northeast, North China, East China, Central China, Northwest, Southwest, and South China, were introduced to Sichuan for spring planting. The average latitude of the seven regions was ranked as follows: Northeast > North China > Northwest > East China > Central China > Southwest > South China. The reproductive growth period of these soybean varieties was longer than their vegetative growth period. Among them, soybean varieties from Northeast region, which had the highest latitude when introduced to Sichuan, had both shorter vegetative and reproductive growth periods compared to varieties from other regions. Southern soybean and southwestern soybean have longer vegetative growth periods, while northwestern soybean has a shorter vegetative growth period but a longer reproductive growth process. The overall growth period of northwestern soybean (from emergence to physiological maturity) is the longest (Figure 2). During the vegetative growth phase, the duration increases as the latitude of the soybean origin decreases. In the overall growth process of soybean, most of the durations decrease as the latitude of the soybean origin decreases, showing a consistent trend with the reproductive growth process.
Figure 2 Comparison of soybean growth period in different regions under monocropping. NE-S, Northeast Soybean; NC-S, North China Soybean; NW-S, Northwest Soybean; EC-S, East China Soybean; CC-S, Central China Soybean; SW-S, Southwest soybean; SC-S, South China soybean.
The E gene is the main genetic factor that determines the growth period of soybeans (Li et al., 2017). We assessed the phenotypic and growth period characteristics of 101 cultivated soybean varieties in Chongzhou based on their combinations of E1-E4 alleles (Figure 3; Table 2). During the years 2021-2022, varieties carrying the e1-as/e2-ns/e3-tr/E4 and e1-as/e2-ns/E3/E4 allele combinations exhibited the shortest flowering and maturation times, while those carrying the E1/e2-ns/E3/E4 and E1/E2/E3/E4 allele combination had the latest flowering time. Varieties with the e1-as/E2/E3/E4 allele combination had the longest podding and maturation times. The E1/e2-ns/E3/E4 allele combination had the smallest growth period structure ratio, while the e1-as/E2/E3/E4 and e1-as/e2-ns/E3/E4 allele combination had the largest growth period structure ratio.
Figure 3 Growth period structure (vegetative growth period: days from VE to R1; reproductive growth period: days from R1 to R7) and maturity time (days from VE to R7) of different photoperiod genotypes.
Based on the combined results of the two years of field experiments, varieties carrying a higher number of recessive E1-E4 allele combinations exhibited shorter flowering and maturation times. However, no consistent pattern was observed between the growth period structure ratio and the photoperiod genotype.
Furthermore, we conducted analysis of variance to investigate the effects of E1-E4 genes on flowering and maturation times in soybean. Due to the presence of only dominant alleles for E4 in all cultivated varieties, variance analysis was not performed for the E4 gene. Based on the combined field data from two years, we observed that both the E1 and E2 genes collectively influenced the soybean growth period. Specifically, E1 and E3 significantly influenced soybean flowering time, with E1 exerting a greater effect than E3. Moreover, both E1 and E3 had highly significant impacts on soybean maturation time. However, the effect of E2 on soybean maturation time was greater than that of E1 (Table 3).
We analyzed the geographic distribution of cultivated soybean varieties from 15 provinces (autonomous regions) in China (Figure 4). The vast majority of soybean varieties in most provinces (autonomous regions) carry the E1/e2-ns/E3/E4 allele combination (Figure 4A). In the Northeast region, there are E1/e2-ns/E3/E4, e1-as/e2-ns/E3/E4, and e1-as/e2-ns/e3-tr/E4, while e1-as/e2-ns/E3/E4 and e1-as/e2-ns/e3-tr/E4 are mainly distributed in this region (Figure 4B), with Heilongjiang province having the largest proportion (Figure 4A). In the North China region, the E1-E4 allele combinations are the most diverse, with Beijing having the widest distribution. In the East China region, E1/e2-ns/E3/E4, e1-as/E2/E3/E4, and E1/E2/E3/E4 are distributed in this area, with the vast majority of E1/E2/E3/E4 distributed in this region (Figure 4B). In the Central and Southern China regions, the main allele combination is E1/e2-ns/E3/E4, while in the Northwest region, it is mainly e1-as/E2/E3/E4, and in the Southwest region, it is mainly E1/e2-ns/E3/E4 and e1-as/E2/E3/E4.
Figure 4 Geographical distribution of E1-E4 allele combinations in China. (A) Each pie chart shows the proportional distribution of the E1-E4 allele combination in its province (autonomous region). The size of the pie chart represents the number of varieties originating in each province (autonomous region). (B) Each pie chart shows the proportional distribution of the region of origin where the E1-E4 alleles are located.
Based on the comprehensive analysis of two years of field experiments, we observed that the height distribution of the 101 cultivated soybean varieties fell within the range of 21 to 80 cm (Figure 5), respectively. Among the investigated soybean varieties, those with the genotype E1/e2-ns/E3/E4 exhibited the widest height distribution, with the highest density of plants observed in the 30-60 cm range (Figure 5). In contrast, plants with the genotype E1/E2/E3/E4 showed a more dispersed height distribution, ranging from 28 cm to 76 cm. Plants with the genotype E1/e2-ns/e3-fs/E4 had a height of approximately 30-40 cm, while those with the genotype e1-as/E2/E3/E4 exhibited a height distribution ranging from 38 cm to 67 cm. The e1-as/e2-ns/E3/E4 genotype displayed a height distribution between 21 to 55 cm, with a primary concentration between 32 to 41 cm. Finally, plants with the genotype e1-as/e2-ns/e3-tr/E4 exhibited heights of 34.5 cm and 23.3 cm in the two respective years, respectively. Overall, soybean varieties carrying more recessive alleles of E1-E4 exhibited lower plant heights, while those carrying more dominant alleles of E1-E4 exhibited higher plant heights.
The genotype of photoperiodic genes is closely related to soybean pod biomass and yield per plant. Soybean varieties with a higher frequency of dominant alleles E1-E4 have more effective pods and higher yield per plant. Soybean varieties with mutated alleles of E1-E4 have fewer pods, lower pod biomass, lower final yield per plant, and lower total dry matter (Figure 6). Among the genotypes, E1/E2/E3/E4 exhibited the highest pod biomass (Figure 6A), while E1/e2-ns/E3/E4 and e1-as/E2/E3/E4 showed higher stem, leaf, and branch biomass (Figure 6B). In contrast, E1/e2-ns/e3-fs/E4 had the lowest pod biomass, and e1-as/e2-ns/e3-tr/E4 displayed the lowest stem, leaf, and branch biomass. Regarding yield components, E1/E2/E3/E4 displayed the highest individual seed weight, whereas e1-as/e2-ns/e3-tr/E4 had the lowest individual seed weight (Figure 6C). Furthermore, e1-as/E2/E3/E4 exhibited the highest number of effective pods per plant, while E1/e2-ns/e3-fs/E4 displayed the lowest (Figure 6D). Consequently, soybean varieties carrying a higher number of recessive E1-E4 gene combinations exhibited lower yield and biomass.
Figure 6 Biomass and yield of soybean with different photoperiod genotypes. (A) pod dry weight in R6 period; (B) plant dry weight (except pod) in R6 period; (C) effective pod number per plant in R8 period; (D) grain weight per plant in R8 period.
In addition, we also conducted a variance analysis of the effects of E1-E4 on soybean agronomic traits (Table 4). The results show that plant height of various cultivated soybean varieties is influenced by the E2 and E3 gene, with E2 having a greater effect on plant height than E3; The accumulation of biomass in soybean was jointly affected by E1 and E3, where stem, leaf, and branch biomass, as well as pod biomass, were primarily influenced by the E1 gene. Notably, the influence of E1 on pod biomass and total biomass accumulation was greater than that of E3. Additionally, E1-E4 genes significantly influenced soybean yield components. Specifically, the E3 gene significantly affected the formation of effective pods in soybean, while the E1 gene had a significant impact on grain weight per plant.
The data on the developmental structure (VE-R1, VE-R7), plant morphology (plant height, stem diameter), and yield traits (single seed weight, number of effective pods, pod dry weight, stem and leaf dry weight) of 101 soybean varieties were standardized using the Z-score method. The suitability of factor analysis was tested using the KOM and Bartlett’s sphericity tests (Table 5). The KOM value was 0.778, and the Bartlett’s sphericity test result was 400.718 with a Sig value of 0.000. Both tests indicated that there was a certain correlation between the various indicators of the developmental structure, plant morphology, and yield traits, and therefore, all indicators were suitable for factor analysis.
Table 5 showed that the first two principal components explained 67.136% of the total variance, indicating that the two principal components extracted could represent 67.136% of the original eight indicators, including developmental structure, plant morphology, and yield traits. According to the coefficients of the two principal components in Table 6, it was found that the coefficients of stem and leaf dry weight, maturity time (VE-R7), flowering time (VE-R1), plant height, pod dry weight, and number of effective pods in principal component Y1 were larger than those of other variables, indicating that principal component Y1 was a comprehensive reflection of these six trait indicators. In principal component Y2, the coefficients of stem diameter and single seed weight were larger than those of other variables, indicating that principal component Y2 was mainly reflected by these two trait indicators.
Based on the principal component analysis, a comprehensive score was given to the eight trait indicators. Soybean varieties with higher scores were mainly from the eastern and northern regions of China, mainly in the Yellow-Huaihe region, with the E1/e2-ns/E3/E4 gene combination. These varieties had higher biomass, yield, and longer developmental periods. The comprehensive scores of soybean varieties with more E1 and E2 recessive alleles were generally lower. Most soybean varieties from the northeastern region had lower comprehensive scores. This method can be used to screen for ideal soybean varieties suitable for different geographical cultivation patterns.
Soybeans carrying different allele combinations of E1-E4 genes exhibit variations in flowering and maturation time. Chongzhou (30°33′N, 103°39′E) is located in the southwestern region of China, and the majority of the cultivated soybean varieties used in this experiment originate from areas north of Chongzhou, where daylight duration exceeds that of the experimental site. Previous studies have demonstrated significant interactions between major genes controlling the growth period of soybeans, as well as interactions between genes and photoperiod, which directly influence soybean’s growth and development. Soybeans regulate their flowering in response to changes in day length by perceiving the duration of the photoperiod (Garner and Allard, 1920). E1 expression is suppressed under short-day conditions, leading to prolonged flowering time under long-day induction. Similarly, E2 affects soybean maturation time under short-day conditions (Wang et al., 2008; Thakare et al., 2010; Xia et al., 2012; Thakare et al., 2011). In this study, we confirmed that E1 has a greater effect on the flowering and maturity of soybean, and its fertility period is generally shorter than that of the source, but E3 also has a greater effect on the structure of the fertility period of soybean, and the effect is greater than that of E2 (Table 3); and the previous study found that the E1-E3 genes have a greater effect on the flowering time and maturity time of soybeans, but the effect of E3 is smaller than that of E1 and E2 (Liu et al., 2020). E1 has a highly significant effect on the length of flowering time of soybean, more than 80% of cultivated soybean varieties originating from latitudes higher than the Chengdu Plain, the length of sunshine is relatively shortened, so the flowering time of soybean is shortened under short sunshine conditions. E2 had a large effect on the different stages of soybean growth and development (Table 2). Soybean varieties containing the e2-ns allele exhibit smaller R/V values (1.54-2.14), significantly lower than those containing the E2 allele (R/V values of 1.95-2.12). Under spring planting conditions, recessive E1 and E3 genes promote soybean flowering, while recessive E1-E3 genes shorten the reproductive growth period (R1-R7) and reduce the R/V value. Moreover, a higher number of recessive genes in the genetic combination leads to a certain degree of shortening in various growth stages. In the background of e2-ns/e3-tr/E4 or e2-ns/e3-fs/E4 gene combinations, varieties carrying recessive E1 or E1 genes exhibit early flowering and maturation. However, in the background of e2-ns/E3/E4, soybean varieties containing the E1 gene exhibit delayed flowering and maturation compared to e1-as. This conclusion is consistent with previous studies (Liu et al., 2020). Under the E2/E3/E4 background, soybean varieties have longer reproductive growth periods and maturation times. Compared to e1-as, soybean varieties containing the E1 gene have shorter reproductive growth periods, but the difference in maturation time is not significant.
Relevant studies have shown that the E3 and E4 genotypes not only regulate the flowering period of soybeans but also determine plant architectural traits such as the number of main stems, pod development, and stem growth after flowering, all of which are closely associated with yield (Xu et al., 2013). Additionally, the progression of the soybean growth period directly impacts plant architectural traits. The diversity of E1-E4 allele combinations leads to variations in plant architectural traits and agronomic characteristics, including yield-related factors. In this study, we found that E1 had some effect on soybean yield composition both on soybean pod formation was significant, and the effect of E2 and E3 genes on plant height of introduced soybeans was significant, and the effect of E2 was greater than that of E3 (Table 4). Previous research has revealed that varieties carrying the E2/E3 combination can produce a greater number of main stems and flowers while maintaining a consistent pod set ratio, thereby resulting in increased pod production (Kawasaki et al., 2018). We also found that E1 and E3 significantly affected the composition of soybean plant biomass, with E1 being the genetic locus that mainly affected the biomass of each component of soybean. Varieties carrying a higher number of recessive E1-E4 alleles exhibited lower plant height and biomass in the Chongzhou region, while those with a greater number of dominant E1-E4 alleles displayed higher levels of these agronomic traits. This can be attributed to the effects of E1-E3 on soybean flowering and maturation time, with E1 specifically prolonging the flowering (vegetative growth) period. The extension of the vegetative growth phase in soybeans is advantageous for increased flower and leaf production, thereby promoting reproductive growth (Cober et al., 2005; Zhang et al., 2007).
Among the different genotypes, the agronomic traits (plant height, biomass, and yield) of the e1-as/e2-ns/e3-tr/E4 combination exhibited the lowest levels. Therefore, based on a comprehensive evaluation of genotypes and agronomic traits, the soybean variety VE-R7, characterized by a longer growth period within the range of 60-95 days and the genomic combination of E1/e2-ns/E3/E4, displayed higher biomass and yield. It exhibited strong ecological adaptability in regions with moderate latitudes, making it suitable for cultivation in areas similar to the Chengdu Plain, characterized by relatively lower latitudes. Within the same growth period range of 60-95 days, soybean varieties carrying a higher number of recessive E1-E4 alleles primarily originated from high latitude regions, and their agronomic traits exhibited the lowest levels, rendering them unsuitable for cultivation in regions with relatively lower latitudes.
The various alleles of E1-E4 genes serve as the foundation for soybean flowering, maturation, and ecological adaptation, with mutations in the E1-E4 loci leading to early flowering and maturation. The growth period, yield traits, stress resistance, and ecological adaptability are relevant factors to consider in germplasm introduction, and the degree of ecological differences between the introduction region and the source region is crucial for successful introduction. Within a certain range (60-95 days), soybean varieties with the genotype E1/e2-ns/E3/E4 and longer growth periods, known as VE-R7, exhibit higher biomass and yield, demonstrating overall better performance. They also demonstrate strong ecological adaptability in the Chengdu Plain region, making them suitable for introduction in similar low- to mid-latitude areas (30°N~32°N), such as the Chengdu Plain. However, caution should be exercised when introducing soybean varieties carrying multiple recessive alleles of E1-E4 in this region to avoid potential issues of early flowering and maturation, which could result in reduced yields.
The original contributions presented in the study are included in the article/Supplementary Material. Further inquiries can be directed to the corresponding author.
Conceptualization; NZ. Methodology; NZ, MX and WW. Writing original draft preparation; NZ. Software; NZ and YKG. Data curation; NZ and YKGu. Investigation; NZ, YKG, SW and HZ. Experiment; NZ, YKG, LW and YG. Supervision; WL and WY. All authors have read and agreed to the published version of the manuscript.
The work was funded by the Major Project of Agricultural Biotechnology Breeding of China (2022ZD0400705) and Sichuan Innovative Team Project for Legume and Coarse Cereal of National Modern Agricultural Industry Technology System, Expert Position for Collection and Evaluation of Soybean Germplasm Resources (SC-CXTD-2020-20).
The authors declare that the research was conducted in the absence of any commercial or financial relationships that could be construed as a potential conflict of interest.
All claims expressed in this article are solely those of the authors and do not necessarily represent those of their affiliated organizations, or those of the publisher, the editors and the reviewers. Any product that may be evaluated in this article, or claim that may be made by its manufacturer, is not guaranteed or endorsed by the publisher.
The Supplementary Material for this article can be found online at: https://www.frontiersin.org/articles/10.3389/fpls.2023.1222755/full#supplementary-material
Agarwal, D. K., Billore, S. D., Sharma, A. N., Dupare, B. U., Srivastava, S. K. (2013). Soybean: introduction, improvement, and utilization in India—Problems and prospects. Agric. Res. 2, 293–300. doi: 10.1007/s40003-013-0088-0
Bernard, R. (1971). Two major genes for time of flowering and maturity in soybeans. Crop Sci. 11, 242–244. doi: 10.2135/cropsci1971.0011183X001100020022x
Câmara, G., Sediyama, T., Dourado-Neto, D., Bernardes, M. (1997). Influence of photoperiod and air temperature on the growth, flowering and maturation of soybean (Glycine max (L.) Merrill). Scientia Agricola 54, 149–154. doi: 10.1590/S0103-90161997000300017
Cober, E. R., Morrison, M. J., Ma, B., Butler, G. (2005). Genetic improvement rates of short-season soybean increase with plant population. Crop Sci. 45, 1029–1034. doi: 10.2135/cropsci2004.0232
Fang, Y., Wang, L., Sapey, E., Fu, S., Wu, T., Zeng, H., et al. (2021). Speed-breeding system in soybean: integrating off-site generation advancement, fresh seeding, and marker-assisted selection. Front. Plant Sci. 12. doi: 10.3389/fpls.2021.717077
Fehr, W. (1977). Stages of soybean development. Iowa State Univ. Special Rep. 80, 1–12. Available at: https://dr.lib.iastate.edu/handle/20.500.12876/90239.
Fehr, W. R. (1987). Principles of cultivar development. Theory technique 1, 219–246. doi: 10.1097/00010694-198805000-00012
Garner, W. W., Allard, H. A. (1920). Effect of the relative length of day and night and other factors of the environment on growth and reproduction in plants. Monthly Weather Rev. 48, 415. doi: 10.1175/1520-0493(1920)48<415b:EOTRLO>2.0.CO;2
Grogan, S. M., Brown-Guedira, G., Haley, S. D., Mcmaster, G. S., Reid, S. D., Smith, J., et al. (2016). Allelic variation in developmental genes and effects on winter wheat heading date in the US Great Plains. PloS One 11, e0152852. doi: 10.1371/journal.pone.0152852
Hadley, P., Roberts, E., Summerfield, R., Minchin, F. (1984). Effects of temperature and photoperiod on flowering in soya bean [Glycine max (L.) Merrill]: a quantitative model. Ann. Bot. 53, 669–681. doi: 10.1093/oxfordjournals.aob.a086732
Han, T., Gai, J., Chen, F., Qiu, J. (1998). Photoperiod response and agronomic characters of soybean varieties with different growth period structures. Acta Agronomica Sin. 24, 550–557. doi: 10.3321/j.issn:0496-3490.1998.05.006
Hao, G., Chen, X., Bu, M. (1992). Classification of the Chinese soybean cultivars into maturity groups. Acta Agronomica Sin. 4, 275–281. doi: CNKI:SUN:XBZW.0.1992-04-004
Jiang, B., Nan, H., Gao, Y., Tang, L., Yue, Y., Lu, S., et al. (2014). Allelic combinations of soybean maturity Loci E1, E2, E3 and E4 result in diversity of maturity and adaptation to different latitudes. PloS One 9, e106042. doi: 10.1080/1343943X.2018.1530063
Kawasaki, Y., Yamazaki, R., Katayama, K., Yamada, T., Funatsuki, H. (2018). Effects of maturity genes E2 and E3 on yield formation in soybean cultivar Enrei in warm region, Fukuyama in Japan. Plant Production Sci. 21, 387–397. doi: 10.1080/1343943X.2018.1530063
Kurasch, A. K., Hahn, V., Leiser, W. L., Vollmann, J., Schori, A., Betrix, C. A., et al. (2017). Identification of mega-environments in Europe and effect of allelic variation at maturity E loci on adaptation of European soybean. Plant Cell Environ. 40, 765–778. doi: 10.1111/pce.12896
Li, J., Wang, X., Song, W., Huang, X., Zhou, J., Zeng, H., et al. (2017). Genetic variation of maturity groups and four E genes in the Chinese soybean mini core collection. PloS One 12, e0172106. doi: 10.1371/journal.pone.0172106
Liu, B., Kanazawa, A., Matsumura, H., Takahashi, R., Harada, K., Abe, J. (2008). Genetic redundancy in soybean photoresponses associated with duplication of the phytochrome A gene. Genetics 180, 995–1007. doi: 10.1534/genetics.108.092742
Liu, L., Song, W., Wang, L., Sun, X., Qi, Y., Wu, T., et al. (2020). Allele combinations of maturity genes E1-E4 affect adaptation of soybean to diverse geographic regions and farming systems in China. PloS One 15, e0235397. doi: 10.1371/journal.pone.0235397
Lu, S., Dong, L., Fang, C., Liu, S., Kong, L., Cheng, Q., et al. (2020). Stepwise selection on homeologous PRR genes controlling flowering and maturity during soybean domestication. Nat. Genet. 52, 428–436. doi: 10.1038/s41588-020-0604-7
Lu, S., Li, Y., Wang, J., Srinives, P., Nan, H., Cao, D., et al. (2015). QTL mapping for flowering time in different latitude in soybean. Euphytica 206, 725–736. doi: 10.1007/s10681-015-1501-5
Spehar, C. R. (1995). Impact of strategic genes in soybean on agricultural development in the Brazilian tropical savannahs. Field Crops Res. 41, 141–146. doi: 10.1016/0378-4290(95)00007-D
Sun, Z., Tian, Z., Wang, J. (1990). Preliminary analysis of growth period structure of soybean varieties in northeast China. Soybean Sci. 9 (3), 205–284. doi: CNKI:SUN:DDKX.0.1990-03-002
Thakare, D., Kumudini, S., Dinkins, R. D. (2010). Expression of flowering-time genes in soybean E1 near-isogenic lines under short and long day conditions. Planta 231, 951–963. doi: 10.1007/s00425-010-1100-6
Thakare, D., Kumudini, S., Dinkins, R. D. (2011). The alleles at the E1 locus impact the expression pattern of two soybean FT-like genes shown to induce flowering in Arabidopsis. Planta: Int. J. Plant Biol. 234, 933–943. doi: 10.1007/s00425-011-1450-8
Tsubokura, Y., Matsumura, H., Xu, M., Liu, B., Nakashima, H., Anai, T., et al. (2013). Genetic Variation in Soybean at the Maturity Locus E4 Is Involved in Adaptation to Long Days at High Latitudes. Agronomy 3, 117–134. doi: 10.3390/agronomy3010117
Wang, J. (1981). Ecological traits and germplasm resources of soybean. Oil Crops China 1, 3–11. Available at: http://www.cnki.com.cn/Article/CJFDTotal-ZGYW198101000.htm
Wang, Y., Cheng, L., Leng, J., Wu, C., Shao, G., Hou, W., et al. (2015). Genetic analysis and quantitative trait locus identification of the reproductive to vegetative growth period ratio in soybean (Glycine max (L.) Merr.). Euphytica 201, 275–284. doi: 10.1007/s10681-014-1209-y
Wang, Y., Wu, C. X., Zhang, X. M., Wang, Y. P., Han, T. F. (2008). Effects of soybean major maturity genes under different photoperiods. ACTA, 34, 1160–1168. doi: 10.3724/SP.J.1006.2008.01160
Watanabe, S., Hideshima, R., Xia, Z., Tsubokura, Y., Sato, S., Nakamoto, Y., et al. (2009). Map-based cloning of the gene associated with the soybean maturity locus E3. Genetics 182, 1251–1262. doi: 10.1534/genetics.108.098772
Wu, J., Liu, S., Wang, Q., Zeng, Q., Mu, J., Huang, S., et al. (2018). Rapid identification of an adult plant stripe rust resistance gene in hexaploid wheat by high-throughput SNP array genotyping of pooled extremes. Theor. Appl. Genet. 131, 43–58. doi: 10.1007/s00122-017-2984-3
Xia, Z., Watanabe, S., Yamada, T., Tsubokura, Y., Nakashima, H., Zhai, H., et al. (2012). Positional cloning and characterization reveal the molecular basis for soybean maturity locus E1 that regulates photoperiodic flowering. Proc. Natl. Acad. Sci. 109, E2155–E2164. doi: 10.1073/pnas.1117982109
Xu, M., Xu, Z., Liu, B., Kong, F., Tsubokura, Y., Watanabe, S., et al. (2013). Genetic variation in four maturity genes affects photoperiod insensitivity and PHYA-regulated post-flowering responses of soybean. BMC Plant Biol. 13, 91. doi: 10.1186/1471-2229-13-91
Zhai, H., Lu, S. X., Wang, Y. Q., Chen, X., Ren, H. X., Yang, J. Y., et al. (2014). Allelic variations at four major maturity E genes and transcriptional abundance of the E1 gene are associated with flowering time and maturity of soybean cultivars. PloS One 9 (5), e97636. doi: 10.1371/journal.pone.0097636
Keywords: soybean, photoperiod genotype, E gene, agronomic traits, KASP genotyping
Citation: Zheng N, Guo Y, Wang S, Zhang H, Wang L, Gao Y, Xu M, Wang W, Liu W and Yang W (2023) Identification of E1-E4 allele combinations and ecological adaptability of soybean varieties from different geographical origins in China. Front. Plant Sci. 14:1222755. doi: 10.3389/fpls.2023.1222755
Received: 15 May 2023; Accepted: 17 August 2023;
Published: 04 September 2023.
Edited by:
V. Mohan Murali Achary, International Centre for Genetic Engineering and Biotechnology, IndiaReviewed by:
Elroy Cober, Agriculture and Agri-Food Canada (AAFC), CanadaCopyright © 2023 Zheng, Guo, Wang, Zhang, Wang, Gao, Xu, Wang, Liu and Yang. This is an open-access article distributed under the terms of the Creative Commons Attribution License (CC BY). The use, distribution or reproduction in other forums is permitted, provided the original author(s) and the copyright owner(s) are credited and that the original publication in this journal is cited, in accordance with accepted academic practice. No use, distribution or reproduction is permitted which does not comply with these terms.
*Correspondence: Weiguo Liu, bHdnc3lAMTI2LmNvbQ==
†These authors have contributed equally to this work and share first authorship
Disclaimer: All claims expressed in this article are solely those of the authors and do not necessarily represent those of their affiliated organizations, or those of the publisher, the editors and the reviewers. Any product that may be evaluated in this article or claim that may be made by its manufacturer is not guaranteed or endorsed by the publisher.
Research integrity at Frontiers
Learn more about the work of our research integrity team to safeguard the quality of each article we publish.