- 1College of Chemistry and Environmental Engineering, Ping Dingshan University, Pingdingshan, Henan, China
- 2Henan Province Key Laboratory of Germplasm Innovation and Utilization of Eco-economic Woody Plant, Pingdingshan, Henan, China
- 3People’s Park Management Office of Nanyang City Garden and Greening Center, Garden and Greening Center of Nanyang City, Nanyang, Henan, China
Abiotic stresses pose significant threat to horticultural crop production worldwide. These stresses adversely affect plant growth, development, and ultimately declined crop growth, yield and quality. In recent years, plant scientists have been actively investigating innovative strategies to enhance abiotic stress resilience in crops, and one promising avenue of research focuses on the use of brassinosteroids (BRs). BRs are a class of plant hormones that play crucial roles in various physiological processes, including cell elongation, differentiation, and stress responses. They have emerged as potent regulators of plant growth and development, and their role in improving abiotic stress tolerance is gaining considerable attention. BRs have been shown to mitigate the negative effects of abiotic stresses by modulating key physiological and biochemical processes, including stomatal regulation, antioxidant defense, osmotic adjustment, and nutrient uptake. Abiotic stresses disrupt numerous physiological functions and lead to undesirable phenotypic traits in plants. The use of BRs as a tool to improve crop resilience offers significant promise for sustainable agriculture in the face of increasing abiotic stresses caused by climate change. By unraveling the phenomenon of BRs, this review emphasizes the potential of BRs as an innovative approach for boosting abiotic stress tolerance and improving the overall productivity and quality of horticultural crops. Further research and field trials are necessary to fully harness the benefits of BRs and translate these findings into practical applications for crop production systems.
Introduction
Plants possess intrinsic mechanisms to enhance their tolerance to abiotic stresses (Kanwar et al., 2012). Different physiological and biochemical adjustments are necessary under abiotic stress in horticultural crops (Figure 1). These interactions comprise variations in gene regulation, the production of particular proteins and many metabolites, changes in hormonal signaling, and antioxidant capacity (Shahzad et al., 2022). A collection of organic, naturally occurring molecules is known as plant hormones (Rao et al., 2019). Low concentrations of these hormones could influence important plant life cycle activities (Rhaman et al., 2020). Phytohormones are involved in physiological changes and expression of genes under abiotic stresses. Brassinosteroids (BRs), Auxins, gibberellins, cytokinin, abscisic acid, jasmonates, salicylic acid, ascorbic acid, melatonin, and ethylene are only a few examples of the class of naturally occurring compounds known as phytohormones (Sardar et al., 2021). Since the 1940s, they have been utilized in horticultural crops. A few examples of well-studied expressions are ethylene’s encouragement of fruit ripening, auxin and cytokinin control of the cell cycle, gibberellins initiation, seed germination and stem length, and ABA’s maintenance of seed dormancy (Tang et al., 2020). Hormonal processes determine the growth and development of plants (Rabnawaz et al., 2020). Phytohormones involved in signal transduction networks under abiotic stresses resulting in improved growth and yield of horticultural crops (Muhammad et al., 2022).
The BRs are a class of naturally occurring plant steroids that play a key role in a wide range of biological and cellular mechanisms, which include stem elongation, pollen tube progression, leaf twisting and subscale, root suppression, fruit ripening, ethylene synthesis, proton pump occurrence, xylem segmentation, chlorophyll content, and expression levels (Bajguz, 2011; Ahmad et al., 2023). Since the 1980s, research has been carried out to look into the potential economic advantages of BRs in horticultural crops. The chemical synthesis of BRs analogs then provides a way for the commercial manufacture of active BRs for greenhouse and field studies, confirming structure-activity connections (Altmann, 1999; Rhaman et al., 2020). 24-epibrassinolide (EBR) and 28-homobrassinolide (HBR) have been recognized as plant growth regulators (Figure 2). An extensive study on EBR and HBR studied that exogenously applied BRs can significantly boost yield and quality in several plant species (Tarkowská and Strnad, 2017). However, the outcomes can vary depending on the useful way, development stage, and external factors (Figure 3). Plants had potential to withstand environmental challenges such as water deficit conditions, salinity stress, and low and high temperatures due to BRs applications (Krishna, 2003). The extra benefit of using BRs in agriculture to increase agricultural output is their capacity to endow plants with resilience to abiotic stressors (Baghel et al., 2019). The role of BRs in protecting plants against environmental stresses is more vital for sustainable production (Ahmad et al., 2023). Future farming will benefit much economically from the merging of two qualities (growth stimulation and stress resistance) as transmitted by BRs for increased crop yield (Altmann, 1998; Sultan and Raza, 2015).
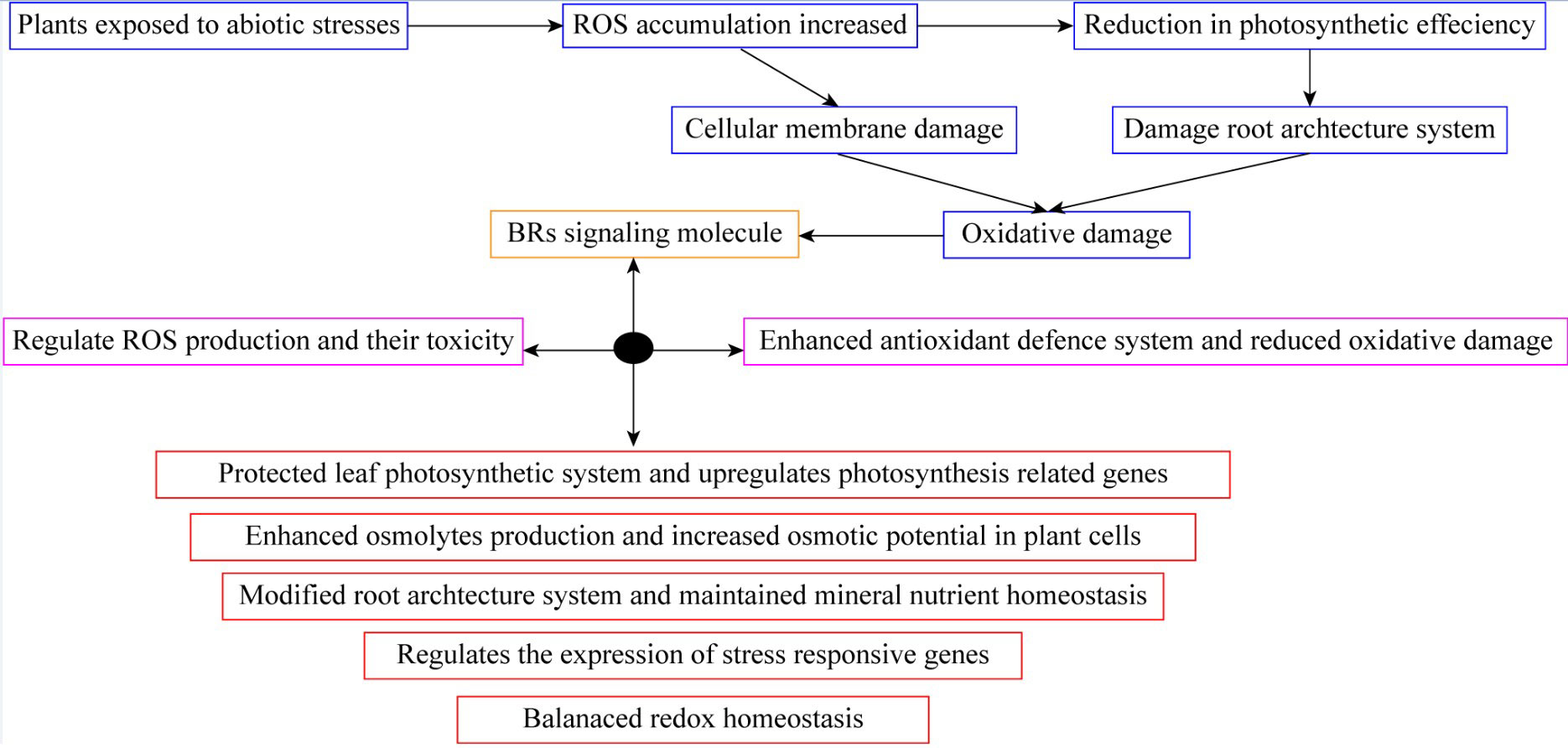
Figure 3 Brassinosteroid application protected leaf photosynthetic system and reduced oxidative damage in plants.
The variations in plant form, size, color, flavor, and aroma as well as flower or fruit color also contribute to the diversity and value of these plants (Ahmad and Anjum, 2023). The sectors of horticulture and the related green industries are professionally expanding quickly and are becoming more and more significant to society (Bajguz, 2012). It is also well-recognized that horticultural plant cultivation is characterized by rigorous and highest management costs, climate control, extensive technological utilization, and serious risk (Pereira-Netto et al., 2006). Therefore, the main objective of this review is to describe the function of BRs, a form of harmless and ecologically friendly hormone, on horticultural crops to satisfy the rise of the industrial sectors. The practical implementation of BRs in horticultural crops for improving yield, quality, and stress tolerance may have a promising future with the advancement of chemical synthesis capabilities (Tang et al., 2016; Chaudhary et al., 2023; Sardar et al., 2023).
The adoption of management strategies to increase plant yield while cultivating under abiotic stressors was advised by plant researchers. Exogenous phytohormone spray is a more attractive method to counteract the detrimental effects of abiotic stresses on long-term plant yield (Souza et al., 2017). Plant researchers are paying close attention to hormones because of their multifaceted behavior against various adverse environmental factors. For increased yields, their usage in horticultural crops growing under abiotic stressors is beneficial. Due to the multiple environmental challenges that horticulture crops face, the current study explores how phytohormones are utilized in these crops. There were also in-depth analyses of the physicochemical and molecular programming that gives plants the ability to fight against the harmful impacts of climatic extremes.
Impact of stressors on horticultural crops
A group of polyhydroxylated steroidal plant hormones is known as BRs, involved in a wide range of physiological, biochemical, and molecular reactions in plants, including germination of seeds, cell growth and elongation, capillary distinctions, photomorphogenesis, chlorophyll content, enzyme activation, metabolism, and inhibition of cells are widely distributed all over the kingdom Plantae (Vives-Peris et al., 2017). Furthermore, it has been revealed that they can shield plants against several biotic and abiotic stresses, including pathogens, toxic substances, water, heat, and salt. Similarly, BRs increase the productivity of a majority of horticultural crops. They also improve fruit quality and yield in certain plants with significant horticultural value. Despite the fact that there are not as many of these reports, a thorough review of them has been done and is reviewed here (Khan et al., 2020). BRs control the regulation of photosynthesis, root extension, stomatal growth, leaf senescence, chlorophyll breakdown, and nutritional balance (Daszkowska-Golec, 2011; Kumar et al., 2016). It is well known that BRs play a critical role in how effectively plants can withstand stress and acclimatize to it. It’s interesting to note that BRs boost plants’ tolerance to environmental stressors. Through ROS-mediated oxidative damage control and an improved antioxidative defense strategy, exogenous BRs promoted cold stress resistance in peach (Wang X. et al., 2019). The use of BRs improved the antioxidant status of grape seedlings under high temperature conditions (Khan et al., 2020). In bitter melon under salt stress, BRs successfully enhanced growth traits, proline, and metabolite levels, and decreased oxidative injury (Souza et al., 2017). Previous research showed that BRs positively influenced strawberry pigments content, strawberry root development, cauliflower antioxidant defense system, tomato osmolytes activities, apple ROS generation, and many other horticultural crops under an abiotic stress condition (Pereira-Netto et al., 2006; Tang et al., 2016). It also enhanced leaf gas exchange parameters, lesser ROS production, and decreased heavy metal concentrations. The most prevalent, well-known, and best-characterized substance is BRs. Once horticultural crops are subjected to abiotic challenges such as drought, salinity, cold, alkalinity, heat, and metal stress, BRs effectively regulate the defensive mechanisms of the plants (Ahmad et al., 2023). The BRs can therefore lessen a range of environmental stressful threats. Additionally, exogenous BRs assisted in raising endogenous BRs concentrations in pea plants. Due to the cooling damage index, BRs treatment lowered phenolic concentration while increasing antioxidant enzyme activity (Sardar et al., 2021). Proline content and antioxidant activity were considerably increased in citrus after BRs treatment, and ROS-induced oxidative injury was reduced (Ahmad et al., 2023). Through foliar applications of BRs under waterlogging conditions in pepper plants, the metabolic activity, root aeration, antioxidative enzyme efficiency, and osmolytes content were greatly increased, while the formation of hydroxyl free radicals, MDA, and electrolyte leakage (EL) decreased (Bajguz, 2011). As a result, BRs play a favorable role in controlling how well horticultural crops respond to abiotic stress situations (Mandava et al., 2022). In numerous studies, tomatoes have undergone BRs treatment at various periods when seeds were pre-sown, root dipping, and foliar spraying (Tarkowská and Strnad, 2017). Tomato plants grown in greenhouses produced more when pre-sowing seeds with BRs for 4 hours in a 1 ppm solution. Applying 22, 23, 24-triepibrassinolide, and 28-homobrassinolide improved tomato fruit set by 43–111%, 118–129%, and 43–111%, respectively (Sridhara et al., 2021).
Impact of BRs against abiotic stresses
There has been some exploration into how phytohormones concentrations and signaling status alter concerning abiotic stress for many years. (Ali et al., 2006). These variations show that phytohormones do not act as early stress signal transducers but rather as mediators of numerous upstream signals. The goal of this review is to describe the function of BRs, a type of harmless and ecologically friendly hormones, on horticultural crops in order to satisfy the evolution of the horticulture sector. Additionally, the development of biological synthesis knowledge and the actual use of BRs in horticulture crops to improve yield, quality, and stress resistance have excellent future potential (Figure 4). Therefore, before actually responding with BRs directly, it is imperative to look at these underlying signals (Tables 1–3).
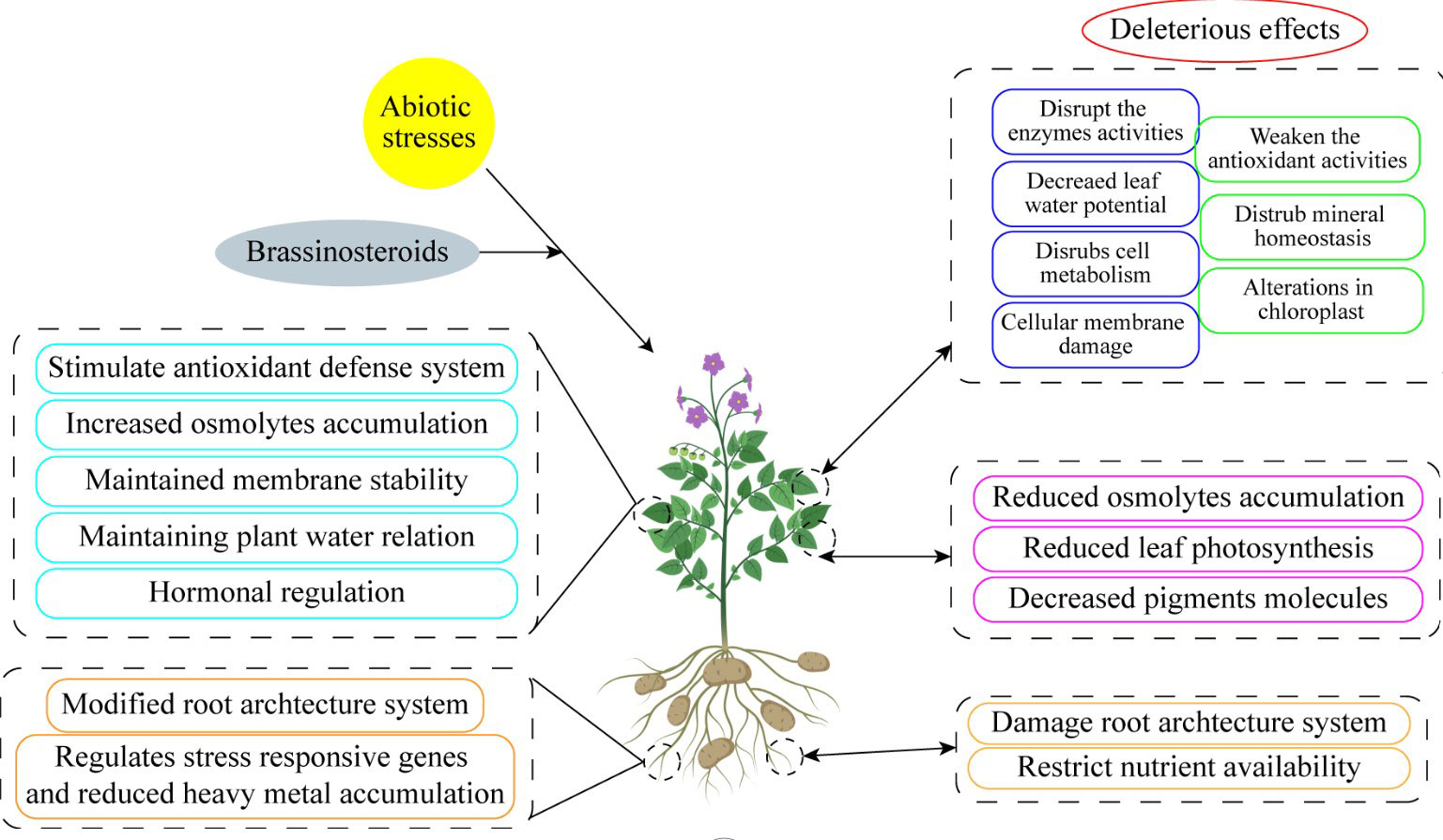
Figure 4 Exogenous brassinosteroid supplementation enhanced abiotic stress tolerance by increasing physiological and morphological traits.
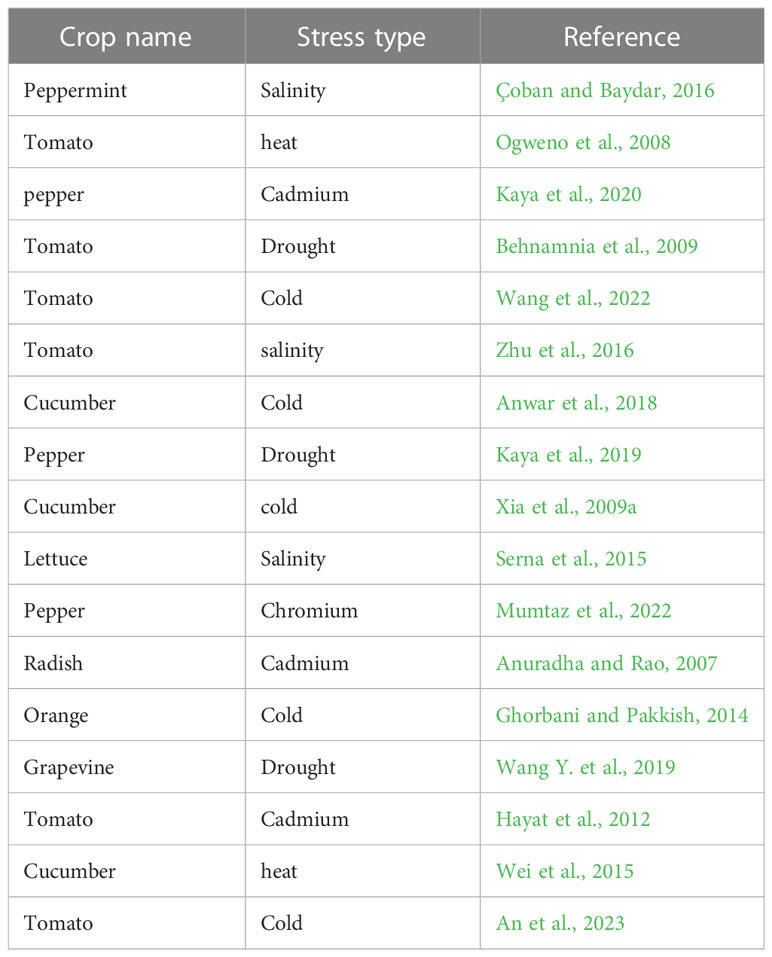
Table 2 Exogenous brassinosteroid application enhanced abiotic stress tolerance in horticultural plants.
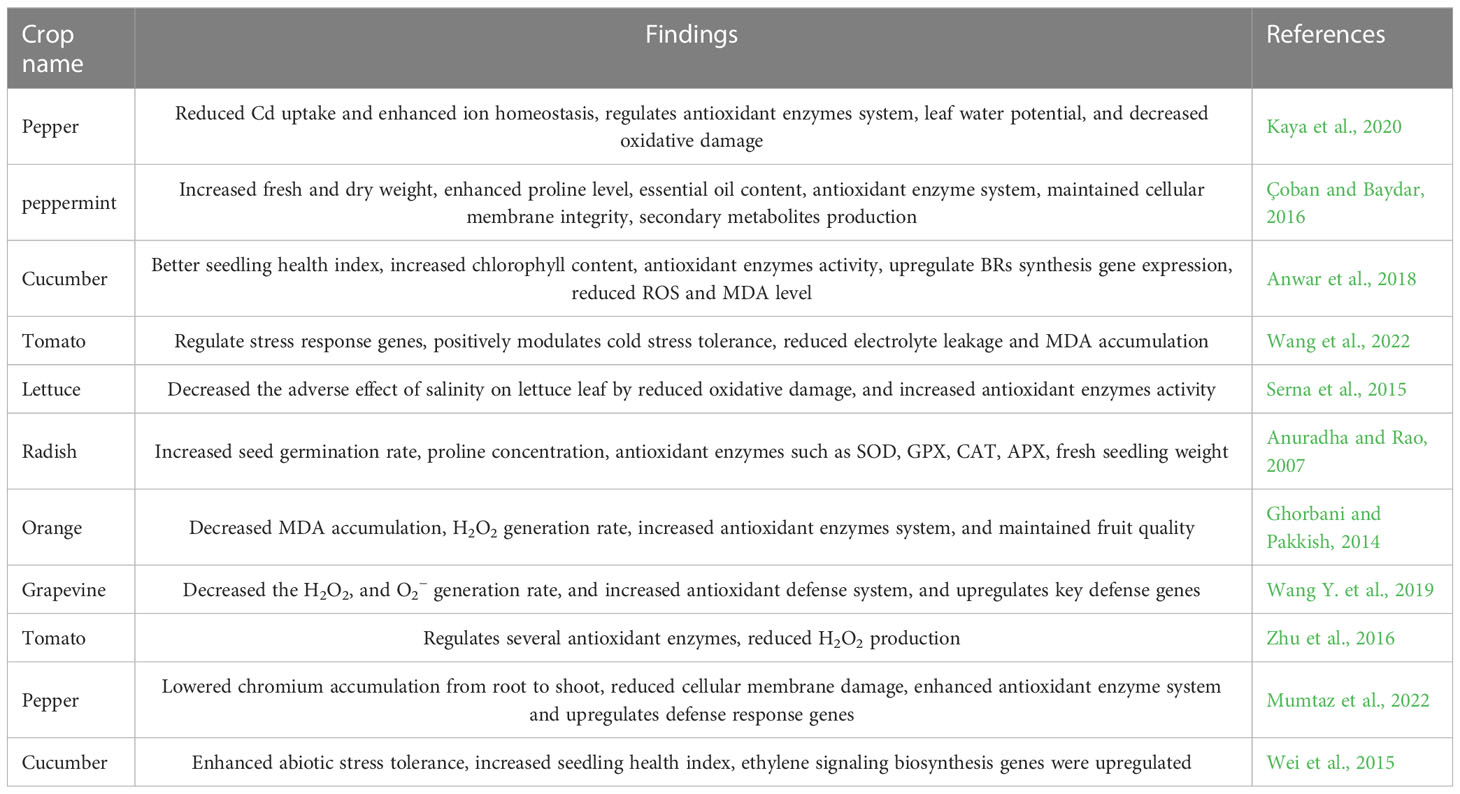
Table 3 Brassinosteroid enhanced physiological, morphological and metabolic processes of horticultural plants.
BRs and drought
Drought is caused by a lack of water or precipitation, which significantly lowers crop yield. In locations with limited or inconsistent rainfall, the issue is worse (Ali et al., 2022). Osmotic stress, which is eventually brought on by drought, interferes with normal physiological functions by upsetting redox balance and ion allocation in the cells through absorption, extrusion, and retention (Kaya et al., 2019). The abscisic acid (ABA) buildup is intimately related to drought resistance. Exogenous BRs treatment increased ABA levels and lessen the negative impacts of drought on plants. EBR dosing in tomatoes increases drought resistance as seen by enhanced photosynthetic apparatus, leaf hydration status, and antioxidant defense in stressful conditions (Kaya et al., 2020). Exogenous BRs spray (0.02 M) in pepper seedlings can improve light use and stimulation energy absorption in the PSII antennae during dry conditions (Khamsuk et al., 2018). Exogenous BRs spray (0.1 M EBR) can improve Chorispora’s resilience to polyethylene glycol (PEG) treatment-induced dehydration (Devi et al., 2022). The transcript of genes that code for both structural and regulatory proteins is changed by BRs treatment. BRs-induced higher drought resistance in canola plants is partially attributed to EBR-induced elevated transcript levels of BnCBF5 and BnDREB (two important drought-sensitive genes) (Li J. et al., 2015). BRs interventions can lessen the long-term effects of drought on plants (Nawaz et al., 2017). Despite 60 days following a week-long water deficit, Indian mustard seedlings still showed low growth and photosynthesis (Naz et al., 2022). Nevertheless, after 30 days of sowing, treatment with 28-homobrassinolide (0.01 µM) might significantly enhance both growth and photosynthesis. While drought stress causes an excessive production of ROS, BRs intervention can significantly lower ROS, MDA, and lipid peroxidation levels when drought stress is present (Sirhindi et al., 2017). When BRs are applied exogenously, tolerance to various abiotic stimuli, such as drought, is increased. Both BR-deficient and BR-insensitive mutants exhibit increased stress tolerance (Samancıoğlu et al., 2014). Moreover, a study on tomatoes revealed that drought tolerance is improved by an increase in endogenous BRs level but not BRs signaling potency. The study also found that tomato drought tolerance was negatively impacted by BRI1 overexpression, indicating that abnormalities in the BRs network may either enhance or reduce stress tolerance, illustrating the complexity of the connections between stressors and BRs (Lv et al., 2020).
BRs and salinity
Salinity is a significant contributor to osmotic stress, also known as physiological drought (Yin et al., 2010). Crop growth, yield, and development are all adversely affected (Zhang et al., 2016). With regards to a broad range of plants, including Arabidopsis, pepper, cucumber, common bean, and black locust, BRs have been established to lessen the harmful impacts of salinity (Kumari et al., 2021). The elevated antioxidant enzyme activities, reduced Na+ and Cl-, and improved K+ and Ca2+ levels in eggplants are signs of the treatment’s ability to boost their tolerance to saline conditions (Sardar et al., 2023). EBR treatment can lower NO3 and NH4 concentrations in cucumber plants that are under saline conditions (Naz et al., 2022). However, at 30 and 45 days after sowing, foliar treatment of HBL to rapeseed may successfully mitigate the negative effects of salinity stress (Zhang et al., 2022). Higher photosynthesis, nitrogen efficiencies, and total polyamines are all linked to cucumber plants improved BR-induced resistance to the saline condition (Wei et al., 2015). Exogenous EBR exposure enhanced the net rate of photosynthesis, stomatal regulation, evapotranspiration, net photosynthetic, and maximal quantum yield of PSII under the saline condition by lowering leaf Na+ levels and membrane permeability (Chaudhary et al., 2023; Sardar et al., 2023). BRs is also efficient in minimizing the effects of several stresses on plants. EBR (1 µM) can reduce simultaneous stress brought on by NaCl in Brassica species, while HBL (0.01 µM) can reduce combined stress brought on by salt and elevated heat in mung bean (Fu et al., 2008). This wide range in BRs concentrations significantly emphasized the dose-effect relationship between BRs and plant species (Cui et al., 2011). It has been demonstrated that ubiquitin-conjugating enzymes (UBC 32) have a function in BR-induced salt stress resistance. UBC32 affects the BRI1 receptor’s accumulation in cells as a functional member of the endoplasmic reticulum-associated protein degradation (ERAD) pathway, and also guides the ERAD pathway towards BR-enhanced osmotic adjustment in Arabidopsis (Chi et al., 2016). Additionally, BRs has been regulating DNA methylation, which is crucial for salt tolerance. A role for BRs in epigenetic alteration under salinity stress is suggested by the fact that seed priming with EBR raises total methylation and enhances salt tolerance (Wang et al., 2011).
BRs and waterlogging
Plants faced serious injuries at the post-waterlogging stage due to a rapid flow in oxygen as reported in the peas (Jackson, 1979). Reoxygenation also referred to as post-waterlogging injuries, is the basic way of this harm. When suddenly exposed again to ambient oxygen, plants overproduce ROS as a result of reoxygenation. Photo-inhibition, or high light after water stress, harms the photosynthetic machinery (Najeeb et al., 2015). The formation of ROS in photosystem I and photosystem II, which are located in the thylakoids of the plastid, is significantly correlated with the deactivation of the photosynthesis. High light can also obliterate photosystem II directly, increasing H2O2 production. ROS also limits the production of new proteins necessary for rebuilding photo-damaged PSII (Bhusal et al., 2020). The four primary organelles for ROS generation in plants are the plastid, cellular organelles, endosomes, and endoplasmic reticulum (ER). In addition to these, enzymes for the production of ROS are also present in the apoplast, cell wall, and cell membrane. Similarly, ROS are usually created in plant metabolic activities. Singlet oxygen (1O2), superoxide anion (O2), hydrogen peroxide (H2O2), hydroxyl radical (HO), hydroperoxyl radical (HO2), and ozone are some of the radical and non-radical ROS found in plants (Geldhof et al., 2022). Cellular proteins, lipids, and nucleic acids can all be harmed by ROS. Although a multiplicity of plant hormones is in charge of controlling reoxygenation or dehydration damage, little is known about how these hormones interact when plants are under post-waterlogging stress (Jiao et al., 2023). But the key point is that all of these hormones work to overcome reoxygenation or dehydration stress in plants under post-waterlogging conditions, either by opening or closing stomata or by detoxifying ROS through various physiological and signaling mechanisms (Zhu et al., 2018).
BRs and temperature
In many parts of the world, chilling or freezing damage caused by low temperatures is a serious hindrance to agricultural production, especially for thermophilic plants (Dong et al., 2021). Adjustments to membrane fluidity, changes in macromolecule interactions, a reduction in cell osmotic pressure, as well as mechanical restrictions, are all consequences of cold stress in plants. The effects of cold exposure on plant photosynthetic activities include a decrease in the pace of CO2 assimilation, photo-inhibition at PSI and PSII, and a decline in enzyme activity (Ma et al., 2021). The increased cold tolerance caused by BRs does not just affect whole plants but also harvested plant products like fruits. According to studies, BRs can increase the shelf life under low-temperature stress, enhancing the post-harvest integrity of horticultural crops (Zhao et al., 2019). However, compared to the amounts that are utilized to impart stress resistance in the complete plant, relatively large quantities of EBR are applied for post-harvest regulation. Fruit integrity of tomatoes is severely degraded by cold stress. However, 6 µM EBR treatment decreased the chilling-induced damage on tomato fruits (Chang and Reed, 2000; Lopez et al., 2011). Mango fruits are protected from cold-related damage by 10 mM EBR treatment by having higher amounts of a group of proteins (remorin, type II SK2 dehydrin, and temperature-induced lipocalin) (Li et al., 2012). In contrast, BRs elevated the unsaturated fatty acids in the plasma membrane phospholipids of mango fruits, lowering the phase transition temperatures and increasing fluidity under coldness (Nham et al., 2017; Shi et al., 2019). The impact of various EBR levels on the freshness of peppers at low temperatures (3°C) has been explored. Moreover, 15 µM EBR was sufficient to reduce adverse effects of chilling on the green sweet peppers (Raza et al., 2023). BRs supplementation on peppers improved antioxidant activity and photosynthetic pigments and L-ascorbic acid and expected to reduce oxidative damage and EL under cold stress (Gisbert-Mullor et al., 2021). The metabolic process that is most vulnerable to heat stress is photosynthetic machinery. High temperatures hinder photosystem II related to photochemical activities in addition to reducing net photosynthetic rate (Li T. et al., 2015). By enhancing the antioxidant enzyme functions that reduce oxidative damage under distress, EBR (0.2 µM) pretreatment in tomato can reduce losses in photosynthesis brought on by high temperatures. It is fascinating to note that BRs can regulates thermo-tolerance in plant cultivars that are both heat-tolerant and heat-sensitive (Zhang et al., 2013). In the case of both heat-tolerant and heat-sensitive ecotypes of melon, EBR pretreatment significantly increases the photosynthetic pigment levels, net CO2 absorption rate, stomatal closure, photo-degradation activity of PSI, and water-use efficiency under heat exposure (Raza et al., 2023). By boosting antioxidant capability, EBR treatment (0.05 - 0.2 mM) reduces heat stress in eggplant and, reduces the amount of ROS that accumulates when temperatures are high (Mohapatra et al., 2022). According to these results, BRs have specific modulatory impacts on plant photosynthesis and antioxidant properties, which significantly help to mitigate the harmful consequences of heat stress.
BRs and heavy metals
Metal-induced stress is a problem for plants cultivated in contaminated soil. Stress brought on by heavy metals has certain distinctive impacts. First off, the quantity and quality of crops grown in heavy metal-contaminated soils are harmed. Second, due to possible food chain contaminants, there are severe dangers connected to consuming heavy metal-contaminated natural substances (Bücker-Neto et al., 2017). High levels of harmful metals are frequently found in crops cultivated in such metal-affected soils, and eating these contaminated foods carries additional dangers. Numerous studies using different methodologies were carried out to address these problems (Sytar et al., 2019). Heavy metal stress can be reduced through the application of plant growth regulators, bioactive substances, and modification of endogenous hormones and signaling pathways. Similarly, BRs can reduce the effects of heavy metal stress on a variety of plant species (Singh et al., 2016) (Figure 5). The ability of plants to absorb CO2 and their ability to photosynthesize are significantly impacted by heavy metals. Increasing data indicate that heavy metals like Cd reduce photosynthetic activity by restricting the Calvin cycle’s use of ATP and NADPH (Rady and Osman, 2012.). The net photosynthetic activity, stomatal functioning, maximum quantum yield of PSII, the quantum efficiency of PSII, and photochemical quenching coefficient were all considerably reduced in tomato under cadmium (Cd) stress for 40 days. BRs regulates net photosynthetic rate, restrictions of Cd accumulation in leaves, and improves CO2 absorption ability (Ahammed et al., 2013). As a result, Cd stress severely inhibits the formation of biomass in plants. Nevertheless, foliar EBR (0.1 M) exposure considerably boosts CO2 absorption capability, Fv/Fm, and total chlorophyll content during Cd stress (Vardhini, 2016). A foliar spray of Exogenous BRs also reduces Cd absorption in the roots and its transfer toward leaves. Under chromium (Cr) exposure, tobacco leaf mesophyll cells’ transmission electron micrographs revealed a deformed cellular structure and cytoplasmic membrane as well as dilated thylakoid (Jan et al., 2020). However, EBR treatment aided to maintain the organization of grana and thylakoids under Cr stress and safeguarded the chloroplast from Cr-induced injury. EBR exhibits a stress-protective function in reducing the stress caused by heavy metals. Treatment with HBL could reduce the effects of Cd on tomato seedlings’ development, photosynthetic capacity, and PSII redox reactions (Basit et al., 2022). Heavy metal exposure causes plants to produce ROS at the microscopic level, which severely affects metabolic reactions and results in oxidative damage to proteins, lipids, and biomolecules. It’s interesting to observe that plants produce more distinct BRs when exposed to heavy metals like nickel (Ni). It has been demonstrated that BRs protect plants from stress brought on by heavy metals (Fariduddin et al., 2011). Moreover, EBR treatment (0.1 M) in tomato plants can increase adaptability to Cd stress. Moreover, in soils containing less than 12 mg kg-1 Cd, foliar treatment of BRs (0.01 M EBR) can increase tomato fruit yields and quality. Within a short period of exposure, BRs have a potent protective effect against Cd stress (Yang et al., 2019). For contrast, under a 60-day Cd load, a single foliar dose of EBR (0.01 mM) applied 24 hours before the assessment can significantly increase the photosynthetic processes in tomato leaves. Leguminous crops treated with BRs have better nodule production when exposed to heavy metals. Vigna radiata plant development especially nodulation was disturbed under Ni stress (Posmyk and Janas, 2007). Similarly, HBL treatment reduced Cd phytotoxicity by increasing Cicer arietinum concentrations of both enzymatic and non-enzymatic antioxidants (Xia et al., 2009b). By increased levels of antioxidant enzymes and redox balance, the addition of EBR (5 nM) in the half-strength MS medium increases the resilience of tomato seedlings to zinc-oxide nanoparticle-induced stress (Ahmed et al., 2021). Likewise, increased endurance to heavy metals caused by exogenous BRs is considered to be due to significant improvements in photosynthetic pigments, antioxidative defense, ROS scavenging capabilities, glutathione content, phytochelatin content, and cell cycle under stress conditions (Bajguz and Hayat, 2009). So, it has been assumed that BRs had protecting role against heavy metals stress. BRs have shown great potential in reducing the detrimental effects of abiotic stress, including vanadium (V) and chromium (Cr) stress, on plants. V and Cr are heavy metals that can accumulate in plants, leading to oxidative stress and disruption of cellular processes. Studies have demonstrated that BRs can alleviate V and Cr stress by enhancing antioxidant defense systems, regulating ROS scavenging, and promoting the synthesis of stress-related proteins (Fariduddin et al., 2011). BRs also play a crucial role in maintaining cellular ion homeostasis and restricting metal accumulation in plants. The application of BRs offers a promising strategy to mitigate the toxic effects of V and Cr stress on plant growth and development, contributing to improved crop productivity and environmental sustainability (Ahammed et al., 2013).
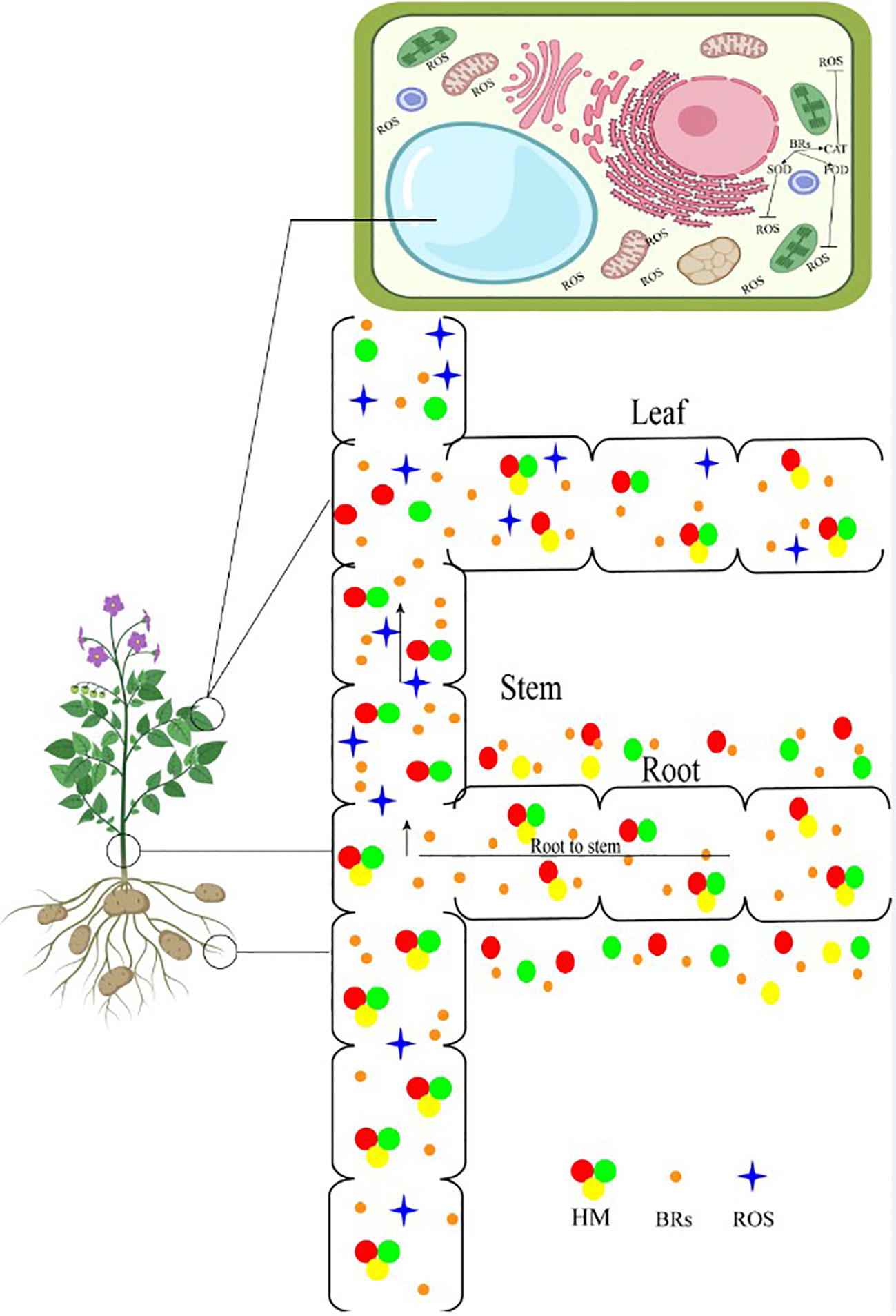
Figure 5 Brassinosteroid application reduced heavy metals accumulation from root to shoot. HM, heavy metals; ROS, reactive oxygen species; BRs, brassinosteroids.
Future scenarios
Enhancing plants’ capacity to survive abiotic stress is crucial, and there is still a lot to be done to protect subsequent generations from the impending problem. It is possible in many ways.
◼ For pollution lessening, it is necessary to stop global warming. In this regard, conferences should be held on a national and international scales, the public should be made aware of the issue, and industries should be built according to a plan.
◼ The mechanism of stress tolerance should be explored to identify the crucial components contributing to drought response, such as the function of phytohormones and the genes expression.
◼ Transgenic developments should be used for the development of climate-resilient cultivars.
◼ It is important to test stress-tolerant cultivars in the field rather than just in the lab and greenhouses. Together, these laws and innovations will assist plants in better withstanding climate change and combating abiotic stress.
Conclusion
BRs are a class of steroidal plant hormones that are produced naturally by all members of the plant family. There are several BRs analogues, but epibrassinolide, homobrassinolide, and brassinolide are the most stable ones. However, there are also a few synthetic and commercial substitutes of BRs in the market. BRs are allegedly non-toxic and environmentally safe. They are widely used to increase the yield of several crops. However, there has been very little research conducted on this broad topic, which has mostly gone unexplored. Horticultural crops can benefit from the huge potential of BRs by increasing yield. Since BRs are also well known for their function in protecting plants against biotic stress scenarios like the attack of various pathogens, which includes a variety of environmental stresses. As a result, they can quickly and effectively replace various pesticides and fungicides that pose a risk to human health and harm the environment.
Author contributions
ZZ and ZC: Conceptualization, Literature survey, Writing major original draft, Review structure. HS: Writing- review and editing, Figure designing. SC: Reviewing and editing, References collection. All authors contributed to the article and approved the submitted version.
Funding
This work was funded by Key Research Project of Henan Provincial Higher Education Institutions (22B180011), Henan Provincial Science and Technology Research Project (222102110480), Henan Provincial Science and Technology Research Project (212102310840, Key Research Project of Henan Provincial Higher Education Institutions (21B210007) and Pingdingshan University High Level Talent Research Launch Fund Project (PXY-BSQD-202109).
Conflict of interest
The authors declare that the research was conducted in the absence of any commercial or financial relationships that could be construed as a potential conflict of interest.
Publisher’s note
All claims expressed in this article are solely those of the authors and do not necessarily represent those of their affiliated organizations, or those of the publisher, the editors and the reviewers. Any product that may be evaluated in this article, or claim that may be made by its manufacturer, is not guaranteed or endorsed by the publisher.
References
Ahammed, G. J., Choudhary, S. P., Chen, S., Xia, X., Shi, K., Zhou, Y., et al. (2013). Role of brassinosteroids in alleviation of phenanthrene–cadmium co-contamination-induced photosynthetic inhibition and oxidative stress in tomato. J. Exp. Bot. 64, 199–213. doi: 10.1093/jxb/ers323
Ahmad, R., Anjum, M. A. (2023). Mineral nutrition management in fruit trees under salt stress: a review. Erwerbs-Obstbau, 1–9. doi: 10.1007/s10341-023-00830-x
Ahmad, R., Manzoor, M., Muhammad, H. M. D., Altaf, M. A., Shakoor, A. (2023). Exogenous melatonin spray enhances salinity tolerance in Zizyphus germplasm: a brief theory. Life 13, 493. doi: 10.3390/life13020493
Ahmed, R., Yusoff Abd Samad, M., Uddin, M. K., Quddus, M. A., Hossain, M. M. (2021). Recent trends in the foliar spraying of zinc nutrient and zinc oxide nanoparticles in tomato production. Agronomy 11, 2074. doi: 10.3390/agronomy11102074
Ali, M. M., Anwar, R., Malik, A. U., Khan, A. S., Ahmad, S., Hussain, Z., et al. (2022). Plant growth and fruit quality response of strawberry is improved after exogenous application of 24-epibrassinolide. J. Plant Growth Regul. 41, 1786–1799. doi: 10.1007/s00344-021-10422-2
Ali, B., Hayat, S., Hasan, S. A., Ahmad, A. (2006). Effect of root applied 28-homobrassinolide on the performance of Lycopersicon esculentum. Sci. Hortic. 110, 267–273. doi: 10.1016/j.scienta.2006.07.015
Altmann, T. (1998). Recent advances in brassinosteroid molecular genetics. Curr. Opin. Plant Biol. 1, 378–383. doi: 10.1016/S1369-5266(98)80259-8
Altmann, T. (1999). Molecular physiology of brassinosteroids revealed by the analysis of mutants. Plant. 208, 1–11.
An, S., Liu, Y., Sang, K., Wang, T., Yu, J., Zhou, Y., et al. (2023). Brassinosteroid signaling positively regulates abscisic acid biosynthesis in response to chilling stress in tomato. J. Integr. Plant Bio. 65, 10–24. doi: 10.1111/jipb.13356
Anuradha, S., Rao, S. S. R. (2007). The effect of brassinosteroids on radish (Raphanus sativus l.) seedlings growing under cadmium stress. Plant Soil Environ. 53, 465. doi: 10.17221/2307-pse
Anwar, A., Bai, L., Miao, L., Liu, Y., Li, S., Yu, X., et al. (2018). 24-epibrassinolide ameliorates endogenous hormone levels to enhance low-temperature stress tolerance in cucumber seedlings. Int. J. Mol. Sci. 19, 2497. doi: 10.3390/ijms19092497
Baghel, M., Nagaraja, A., Srivastav, M., Meena, N. K., Kumar, S. M., Kumar, A., et al. (2019). Pleiotropic influences of brassinosteroids on fruit crops: a review. Plant Growth Regul. 87, 375–388. doi: 10.1007/s10725-018-0471-8
Bajguz, A. (2011). “Brassinosteroids - occurence and chemical structures in plants,” in Brassinosteroids: a class of plant hormone. Eds. Hayat, S., Ahmad, A. (Dordrecht: Springer), 1–27. doi: 10.1007/978-94-007-0189-2_1
Bajguz, A. (2012). “Origin of brassinosteroids and their role in oxidative stress in plants,” in Phytohormones and abiotic stress tolerance in plants. Eds. Khan, N., Nazar, R., Iqbal, N., Anjum, N. (Berlin, Heidelberg: Springer). doi: 10.1007/978-3-642-25829-9_8
Bajguz, A., Hayat, S. (2009). Effects of brassinosteroids on the plant responses to environmental stresses. Plant Physiol. Biochem. 47, 1–8. doi: 10.1016/j.plaphy.2008.10.002
Basit, F., Bhat, J. A., Dong, Z., Mou, Q., Zhu, X., Wang, Y., et al. (2022). Chromium toxicity induced oxidative damage in two rice cultivars and its mitigation through external supplementation of brassinosteroids and spermine. Chem. 302, 134423. doi: 10.1016/j.chemosphere.2022.134423
Behnamnia, M., Kalantari, K., Ziaie, J. (2009). The effects of brassinosteroid on the induction of biochemical changes in Lycopersicon esculentum under drought stress. Turkish J. Bot. 33, 417–428. doi: 10.3906/bot-0806-12
Bhusal, N., Kim, H. S., Han, S. G., Yoon, T. M. (2020). Photosynthetic traits and plant–water relations of two apple cultivars grown as bi-leader trees under long-term waterlogging conditions. Environ. Exp. Bot. 176, 104111. doi: 10.1016/j.envexpbot.2020.104111
Bücker-Neto, L., Paiva, A. L. S., Machado, R. D., Arenhart, R. A., Margis-Pinheiro, M. (2017). Interactions between plant hormones and heavy metals responses. Genet. Mol. Biol. 40, 373–386. doi: 10.1590/1678-4685-GMB-2016-0087
Chang, Y., Reed, B. M. (2000). Extended alternating-temperature cold acclimation and culture duration improve pear shoot cryopreservation. Cryobiol. 40, 311–322. doi: 10.1006/cryo.2000.2251
Chaudhary, P., Xu, M., Ahamad, L., Chaudhary, A., Kumar, G., Adeleke, B. S., et al. (2023). Application of synthetic consortia for improvement of soil fertility, pollution remediation, and agricultural productivity: a review. Agron. 13, 643. doi: 10.3390/agronomy13030643
Chi, C., Shen, Y., Yin, L., Ke, X., Han, D., Zuo, Y. (2016). Selection and validation of reference genes for gene expression analysis in vigna angularis using quantitative real-time RT-PCR. PloS One 11, e0168479. doi: 10.1371/journal.pone.0168479
Çoban, Ö., Baydar, N. G. (2016). Brassinosteroid effects on some physical and biochemical properties and secondary metabolite accumulation in peppermint (Mentha piperita l.) under salt stress. Indus. Crops Prod. 86, 251–258. doi: 10.1016/j.indcrop.2016.03.049
Cui, J. X., Zhou, Y. H., Ding, J. G., Xia, X. J., Shi, K. A. I., Chen, S. C., et al. (2011). Role of nitric oxide in hydrogen peroxide-dependent induction of abiotic stress tolerance by brassinosteroids in cucumber. Plant Cell Environ. 34, 347–358. doi: 10.1111/j.1365-3040.2010.02248.x
Daszkowska-Golec, A. (2011). Arabidopsis seed germination under abiotic stress as a concert of action of phytohormones. OMICS: J. Integr. Biol. 15, 763–774. doi: 10.1089/omi.2011.0082
Devi, L. L., Pandey, A., Gupta, S., Singh, A. P. (2022). The interplay of auxin and brassinosteroid signaling tunes root growth under low and different nitrogen forms. Plant Physiol. 189, 1757–1773. doi: 10.1093/plphys/kiac157
Dong, H., Chen, Q., Dai, Y., Hu, W., Zhang, S., Huang, X. (2021). Genome-wide identification of PbrbHLH family genes, and expression analysis in response to drought and cold stresses in pear (Pyrus bretschneideri). BMC Plant Biol. 21, 1–20. doi: 10.1186/s12870-021-02862-5
Fariduddin, Q., Yusuf, M., Chalkoo, S., Hayat, S., Ahmad, A. (2011). 28-homobrassinolide improves growth and photosynthesis in Cucumis sativus l. through an enhanced antioxidant system in the presence of chilling stress. Photosynthetic. 49, 55–64. doi: 10.1007/s11099-011-0022-2
Fu, F. Q., Mao, W. H., Shi, K., Zhou, Y. H., Asami, T., Yu, J. Q. (2008). A role of brassinosteroids in early fruit development in cucumber. J. Exp. Bot. 59, 2299–2308. doi: 10.1093/jxb/ern093
Geldhof, B., Novák, O., Van de Poel, B. (2022). Waterlogging shifts ontogenic hormone dynamics in tomato leaves and petioles. BioRxiv., 2022–2012. doi: 10.1101/2022.12.02.518842
Ghorbani, B., Pakkish, Z. (2014). Brassinosteroid enhances cold stress tolerance of Washington navel orange (Citrus sinensis l.) fruit by regulating antioxidant enzymes during storage. Agricul. Conspectus Scientific. 79, 109–114.
Gisbert-Mullor, R., Padilla, Y. G., Martínez-Cuenca, M. R., López-Galarza, S., Calatayud, Á. (2021). Suitable rootstocks can alleviate the effects of heat stress on pepper plants. Sci. Hortic. 290, 110529. doi: 10.1016/j.scienta.2021.110529
Hayat, S., Alyemeni, M. N., Hasan, S. A. (2012). Foliar spray of brassinosteroid enhances yield and quality of Solanum lycopersicum under cadmium stress. Saudi J. Bio. Sci. 19, 325–335. doi: 10.1016/j.sjbs.2012.03.005
Jackson, M. B. (1979). Rapid injury to peas by soil waterlogging. J. Sci. Food Agric. 30, 143–152. doi: 10.1002/jsfa.2740300208
Jan, S., Noman, A., Kaya, C., Ashraf, M., Alyemeni, M. N., Ahmad, P. (2020). 24-epibrassinolide alleviates the injurious effects of cr (VI) toxicity in tomato plants: insights into growth, physio-biochemical attributes, antioxidant activity and regulation of ascorbate–glutathione and glyoxalase cycles. J. Plant Growth Regul. 39, 1587–1604. doi: 10.1007/s00344-020-10169-2
Jiao, Y., Sha, C., Xie, R., Shu, Q. (2023). Comparative analysis of the potential physiological and molecular mechanisms involved in the response to root zone hypoxia in two rootstock seedlings of the Chinese bayberry via transcriptomic analysis. Funct. Integrat. Genom. 23, 11. doi: 10.1007/s10142-022-00944-7
Kanwar, M. K., Bhardwaj, R., Arora, P., Chowdhary, S. P., Sharma, P., Kumar, S. (2012). Plant steroid hormones produced under Ni stress are involved in the regulation of metal uptake and oxidative stress in Brassica juncea l. Chem. 86, 41–49. doi: 10.1016/j.chemosphere.2011.08.048
Kaya, C., Ashraf, M., Alyemeni, M. N., Ahmad, P. (2020). The role of nitrate reductase in brassinosteroid-induced endogenous nitric oxide generation to improve cadmium stress tolerance of pepper plants by upregulating the ascorbate-glutathione cycle. Ecotoxicol. Environ. Saf. 196, 110483. doi: 10.1016/j.ecoenv.2020.110483
Kaya, C., Ashraf, M., Wijaya, L., Ahmad, P. (2019). The putative role of endogenous nitric oxide in brassinosteroid-induced antioxidant defence system in pepper (Capsicum annuum l.) plants under water stress. Plant Physiol. Biochem. 143, 119–128. doi: 10.1016/j.plaphy.2019.08.024
Khamsuk, O., Sonjaroon, W., Suwanwong, S., Jutamanee, K., Suksamrarn, A. (2018). Effects of 24-epibrassinolide and the synthetic brassinosteroid mimic on chili pepper under drought. Act. Physiol. Plant 40, 1–12. doi: 10.1007/s11738-018-2682-z
Khan, N., Bano, A., Ali, S., Babar, M. A. (2020). Crosstalk amongst phytohormones from planta and PGPR under biotic and abiotic stresses. Plant Growth Regul. 90, 189–203. doi: 10.1007/s10725-020-00571-x
Krishna, P. (2003). Brassinosteroid-mediated stress responses. J. Plant Grow. Regul. 22, 289–297. doi: 10.1007/s00344-003-0058-z
Kumar, V., Sah, S. K., Khare, T., Shriram, V., Wani, S. H. (2016). “Engineering phytohormones for abiotic stress tolerance in crop plants,” in Plant hormones under challenging environmental factors. Eds. Ahammed, G., Yu, J. Q. (Berline, Dordrecht: Springer, Dordrecht). doi: 10.1007/978-94-017-7758-2_10
Kumari, S., Chhillar, H., Chopra, P., Khanna, R. R., Khan, M. I. R. (2021). Potassium: a track to develop salinity tolerant plants. Plant Physiol. Biochem. 167, 1011–1023. doi: 10.1016/j.plaphy.2021.09.031
Li, J., Li, X., Wang, L., Wang, S. (2021). The regulation of brassinolide biosynthesis and physiological effects in grape berries. Mol. Plant Breed. 12, 1–10. doi: 10.5376/mpb.2021.12.0033
Li, T., Xu, X., Li, Y., Wang, H., Li, Z., Li, Z. (2015). Comparative transcriptome analysis reveals differential transcription in heat-susceptible and heat-tolerant pepper (Capsicum annum l.) cultivars under heat stress. J. Plant Biol. 58, 411–424. doi: 10.1007/s12374-015-0423-z
Li, J., Yang, P., Gan, Y., Yu, J., Xie, J. (2015). Brassinosteroid alleviates chilling-induced oxidative stress in pepper by enhancing antioxidation systems and maintenance of photosystem II. Acta Physiol. Plant 37, 1–11. doi: 10.1007/s11738-015-1966-9
Li, B., Zhang, C., Cao, B., Qin, G., Wang, W., Tian, S. (2012). Brassinolide enhances cold stress tolerance of fruit by regulating plasma membrane proteins and lipids. Amino Acid. 43, 2469–2480. doi: 10.1007/s00726-012-1327-6
Lopez, G., Larrigaudière, C., Girona, J., Behboudian, M. H., Marsal, J. (2011). Fruit thinning in ‘Conference’ pear grown under deficit irrigation: implications for fruit quality at harvest and after cold storage. Sci. Hortic. 129, 64–70. doi: 10.1016/j.scienta.2011.03.007
Lv, J., Zong, X. F., Ahmad, S. A., Wu, X., Wu, C., Li, Y. P., et al. (2020). Alteration in morpho-physiological attributes of Leymus chinensis (Trin.) tzvelev by exogenous application of brassinolide under varying levels of drought stress. Chil. J. Agric. Res. 80, 61–71. doi: 10.4067/S0718-58392020000100061
Ma, M., Chen, Q., Dong, H., Zhang, S., Huang, X. (2021). Genome-wide identification and expression analysis of the bZIP transcription factors, and functional analysis in response to drought and cold stresses in pear (Pyrus breschneideri). BMC Plant Biol. 21, 583. doi: 10.1186/s12870-021-03356-0
Mandava, S., Mandava, N. B., Sotomayor, C. (2022). Effects of homobrassinolide 0.1% on almond maturation, reproduction, and yield. Acta Hortic. 1344, 107–114. doi: 10.17660/ActaHortic.2022.1344.17
Martins, S., Montiel-Jorda, A., Cayrel, A., Huguet, S., Roux, C. P. L., Ljung, K., et al. (2017). Brassinosteroid signaling-dependent root responses to prolonged elevated ambient temperature. Nat. Commun. 8, 309. doi: 10.1038/s41467-017-00355-4
Mohapatra, S., Sirhindi, G., Dogra, V. (2022). Seed priming with brassinolides improves growth and reinforces antioxidative defenses under normal and heat stress conditions in seedlings of Brassica juncea. Physiol. Plant 174, e13814. doi: 10.1111/ppl.13814
Muhammad, H. M. D., Abbas, A., Ahmad, R. (2022). Fascinating role of silicon nanoparticles to mitigate adverse effects of salinity in fruit trees: a mechanistic approach. Silicon 14, 8319–8326. doi: 10.1007/s12633-021-01604-4
Mumtaz, M. A., Hao, Y., Mehmood, S., Shu, H., Zhou, Y., Jin, W., et al. (2022). Physiological and transcriptomic analysis provide molecular insight into 24-epibrassinolide mediated cr (VI)-toxicity tolerance in pepper plants. Environ. Poll. 306, 119375. doi: 10.1016/j.envpol.2022.119375
Najeeb, U., Bange, M. P., Tan, D. K., Atwell, B. J. (2015). Consequences of waterlogging in cotton and opportunities for mitigation of yield losses. AoB Plant 7, 80. doi: 10.1093/aobpla/plv080
Nawaz, F., Naeem, M., Zulfiqar, B., Akram, A., Ashraf, M. Y., Raheel, M., et al. (2017). Understanding brassinosteroid-regulated mechanisms to improve stress tolerance in plants: a critical review. Environ. Sci. pollut. Res. 24, 15959–15975. doi: 10.1007/s11356-017-9163-6
Naz, S., Mushtaq, A., Ali, S., Muhammad, H. M. D., Saddiq, B., Ahmad, R., et al. (2022). Foliar application of ascorbic acid enhances growth and yield of lettuce (Lactuca sativa) under saline conditions by improving antioxidant defence mechanism. Funct. Plant Biol. doi: 10.1071/fp22139
Nham, N. T., Macnish, A. J., Zakharov, F., Mitcham, E. J. (2017). ‘Bartlett’pear fruit (Pyrus communis l.) ripening regulation by low temperatures involves genes associated with jasmonic acid, cold response, and transcription factors. Plant Sci. 260, 8–18. doi: 10.1016/j.plantsci.2017.03.008
Ogweno, J. O., Song, X. S., Shi, K., Hu, W. H., Mao, W. H., Zhou, Y. H., et al. (2008). Brassinosteroids alleviate heat-induced inhibition of photosynthesis by increasing carboxylation efficiency and enhancing antioxidant systems in lycopersicon esculentum. J. Plant Growth Regul. 27, 49–57. doi: 10.1007/s00344-007-9030-7
Pereira-Netto, A. B., Cruz-Silva, C. T. A., Schaefer, S., Ramírez, J. A., Galagovsky, L. R. (2006). Brassinosteroid-stimulated branch elongation in the marubakaido apple rootstock. Trees 20, 286–291. doi: 10.1007/s00468-005-0041-3
Planas-Riverola, A., Gupta, A., Betegón-Putze, I., Bosch, N., Ibañes, M., Caño-Delgado, A. I. (2019). Brassinosteroid signaling in plant development and adaptation to stress. Develop. 146, 151894. doi: 10.1242/dev.151894
Posmyk, M. M., Janas, K. M. (2007). Effects of seed hydropriming in presence of exogenous proline on chilling injury limitation in Vigna radiata l. seedlings. Acta Physiol. Plant 29, 509–517. doi: 10.1007/s11738-007-0061-2
Rabnawaz, A., Ahmad, R., Anjum, M. A. (2020). Effect of seed priming on growth, flowering and cut flower quality of carnation. Ind. J. Hortic. 77, 527–531. doi: 10.5958/0974-0112.2020.00076.6
Rady, M. M., Osman, A. S. (2012). Response of growth and antioxidant system of heavy metal-contaminated tomato plants to 24-epibrassinolide. Afr. J. Agric. Res. 7, 3249–3254. doi: 10.5897/AJAR12.079
Rao, M. J., Hussain, S., Anjum, M. A., Saqib, M., Ahmad, R., Khalid, M. F., et al. (2019). Effect of seed priming on seed dormancy and vigor. priming and pretreatment of seeds and seedlings: implication in plant stress tolerance and enhancing productivity in crop plants. Springer Singapore., 135–145. doi: 10.1007/978-981-13-8625-1_6
Raza, A., Charagh, S., Najafi-Kakavand, S., Abbas, S., Shoaib, Y., Anwar, S., et al. (2023). Role of phytohormones in regulating cold stress tolerance: physiological and molecular approaches for developing cold-smart crop plants. Plant Stress 8, 100152. doi: 10.1016/j.stress.2023.100152
Rhaman, M. S., Imran, S., Rauf, F., Khatun, M., Baskin, C. C., Murata, Y., et al. (2020). Seed priming with phytohormones: an effective approach for the mitigation of abiotic stress. Plants 10, 37. doi: 10.3390/plants10010037
Samancıoğlu, A., Kocaçınar, F., Demirkıran, A. R., Korkmaz, A. (2014). Enhancing water stress tolerance in pepper at seedling stage by 24-epibrassinolid (EBL) applications. Acta Hortic. 1142, 109–416. doi: 10.17660/ActaHortic.2016.1142.62
Sardar, H., Khalid, Z., Ahsan, M., Naz, S., Nawaz, A., Ahmad, R., et al. (2023). Enhancement of salinity stress tolerance in lettuce (Lactuca sativa l.) via foliar application of nitric oxide. Plants 12, 1115. doi: 10.3390/plants12051115
Sardar, H., Nisar, A., Anjum, M. A., Naz, S., Ejaz, S., Ali, S., et al. (2021). Foliar spray of moringa leaf extract improves growth and concentration of pigment, minerals and stevioside in stevia (Stevia rebaudiana bertoni). Indus. Crop Product. 166, 113485. doi: 10.1016/j.indcrop.2021.113485
Serna, M., Coll, Y., Zapata, P. J., Botella, M.Á., Pretel, M. T., Amorós, A. (2015). A brassinosteroid analogue prevented the effect of salt stress on ethylene synthesis and polyamines in lettuce plants. Sci. Hortic. 185, 105–112. doi: 10.1016/j.scienta.2015.01.005
Shahzad, S., Ali, S., Ahmad, R., Ercisli, S., Anjum, M. A. (2022). Foliar application of silicon enhances growth, flower yield, quality and postharvest life of tuberose (Polianthes tuberosa l.) under saline conditions by improving antioxidant defense mechanism. Silicon 14, 1511–1518. doi: 10.1007/s12633-021-00974-z
Shi, F., Zhou, X., Yao, M. M., Zhou, Q., Ji, S. J., Wang, Y. (2019). Low-temperature stress-induced aroma loss by regulating fatty acid metabolism pathway in ‘Nanguo’pear. Food Chem. 297, 124927. doi: 10.1016/j.foodchem.2019.05.201
Singh, S., Parihar, P., Singh, R., Singh, V. P., Prasad, S. M. (2016). Heavy metal tolerance in plants: role of transcriptomics, proteomics, metabolomics, and ionomics. Front. Plant Sci. 6. doi: 10.3389/fpls.2015.0114
Singh, A. P., Savaldi-Goldstein, S. (2015). Growth control: brassinosteroid activity gets context. J. Exp. Bot. 66, 1123–1132. doi: 10.1093/jxb/erv026
Sirhindi, G., Kaur, H., Bhardwaj, R., Sharma, P., Mushtaq, R. (2017). 28-homobrassinolide potential for oxidative interface in Brassica juncea under temperature stress. Acta Physiol. Plant 39, 1–10. doi: 10.1007/s11738-017-2524-4
Souza, L. A., Monteiro, C. C., Carvalho, R. F., Gratão, P. L., Azevedo, R. A. (2017). Dealing with abiotic stresses: an integrative view of how phytohormones control abiotic stress-induced oxidative stress. Theor. Exp. Plant Physiol. 29, 109–127. doi: 10.1007/s40626-017-0088-8
Sridhara, S., Ramesh, N., Gopakkali, P., Paramesh, V., Tamam, N., Abdelbacki, A. M., et al. (2021). Application of homobrassinolide enhances growth, yield and quality of tomato. Saud. J. Biol. Sci. 28, 4800–4806. doi: 10.1016/j.sjbs.2021.05.008
Sultan, A., Raza, A. R. (2015). Steroids: a diverse class of secondary metabolites. Med. Chem. 5, 310–317. doi: 10.4172/2161-0444.1000279
Sytar, O., Kumari, P., Yadav, S., Brestic, M., Rastogi, A. (2019). Phytohormone priming: regulator for heavy metal stress in plants. J. Plant Grow. Regul. 38, 739–752. doi: 10.1007/s00344-018-9886-8
Tang, J., Han, Z., Chai, J. (2016). What are brassinosteroids and how do they act in plants? BMC Biol. 14, 1–5. doi: 10.1186/s12915-016-0340-8
Tang, G., Ma, J., Hause, B., Nick, P., Riemann, M. (2020). Jasmonate is required for the response to osmotic stress in rice. Environ. Exp. Bot. 175, 104047. doi: 10.1016/j.envexpbot.2020.104047
Tarkowská, D., Strnad, M. (2017). “Protocol for extraction and isolation of brassinosteroids from plant tissues,” in Brassinosteroids. methods in molecular biology, vol. 1564 . Eds. Russinova, E., Caño-Delgado, A. (New York, NY: Humana Press). doi: 10.1007/978-1-4939-6813-8_1
Vardhini, B. V. (2016). Brassinosteroids are potential ameliorators of heavy metal stresses in plants. Plant Metal Interac. 12, 209–237. doi: 10.1016/B978-0-12-803158-2.00008-4
Vardhini, B. V., Anuradha, S., Rao, S. S. R. (2006). Brassinosteroids-new class of plant hormone with potential to improve crop productivity. Ind. J. Plant Physiol. 11, 10–15.
Vives-Peris, V., Gómez-Cadenas, A., Pérez-Clemente, R. M. (2017). Citrus plants exude proline and phytohormones under abiotic stress conditions. Plant Cell Rep. 36, 1971–1984. doi: 10.1007/s00299-017-2214-0
Wang, Y. T., Chen, Z. Y., Jiang, Y., Duan, B. B., Xi, Z. M. (2019). Involvement of ABA and antioxidant system in brassinosteroid-induced water stress tolerance of grapevine (Vitis vinifera l.). Sci. Hortic. 256, 108596. doi: 10.1016/j.scienta.2019.108596
Wang, X., Gao, Y., Wang, Q., Chen, M., Ye, X., Li, D., et al. (2019). 24-epibrassinolide-alleviated drought stress damage influences antioxidant enzymes and autophagy changes in peach (Prunus persicae l.) leaves. Plant Physiol. Biochem. 135, 30–40. doi: 10.1016/j.plaphy.2018.11.026
Wang, D., Yang, Z., Wu, M., Wang, W., Wang, Y., Nie, S. (2022). Enhanced brassinosteroid signaling via the overexpression of SlBRI1 positively regulates the chilling stress tolerance of tomato. Plant Sci. 320, 111281. doi: 10.1016/j.plantsci.2022.111281
Wang, B., Zhang, J., Xia, X., Zhang, W. H. (2011). Ameliorative effect of brassinosteroid and ethylene on germination of cucumber seeds in the presence of sodium chloride. Plant Grow. Regul. 65, 407–413. doi: 10.1007/s10725-011-9595-9
Wei, L. J., Deng, X. G., Zhu, T., Zheng, T., Li, P. X., Wu, J. Q., et al. (2015). Ethylene is involved in brassinosteroids induced alternative respiratory pathway in cucumber (Cucumis sativus l.) seedlings response to abiotic stress. Front. Plant Sci. 6. doi: 10.3389/fpls.2015.00982
Wu, L., Yang, H. (2016). Combined application of carboxymethyl chitosan coating and brassinolide maintains the postharvest quality and shelf life of green asparagus. J. Food Proc. Preserv. 40, 154–165. doi: 10.1111/jfpp.12592
Wu, X., Yao, X., Chen, J., Zhu, Z., Zhang, H., Zha, D. (2014). Brassinosteroids protect photosynthesis and antioxidant system of eggplant seedlings from high-temperature stress. Acta Physiol. Plant 36, 251–261. doi: 10.1007/s11738-013-1406-7
Xia, X. J., Huang, L. F., Zhou, Y. H., Mao, W. H., Shi, K., Wu, J. X., et al. (2009a). Brassinosteroids promote photosynthesis and growth by enhancing activation of rubisco and expression of photosynthetic genes in Cucumis sativus. Plant. 230, 1185–1196. doi: 10.1007/s00425-009-1016-1
Xia, X. J., Wang, Y. J., Zhou, Y. H., Tao, Y., Mao, W. H., Shi, K., et al. (2009b). Reactive oxygen species are involved in brassinosteroid-induced stress tolerance in cucumber. Plant Physiol. 150, 801–814. doi: 10.1104/pp.109.138230
Xia, X. J., Zhou, Y. H., Ding, J., Shi, K., Asami, T., Chen, Z., et al. (2011). Induction of systemic stress tolerance by brassinosteroid in Cucumis sativus. New Phytol. 191, 706–720. doi: 10.1111/j.1469-8137.2011.03745.x
Yang, P., Nawaz, A. M., Li, F., Bai, L., Li, J. (2019). Brassinosteroids regulate antioxidant system and protect chloroplast ultrastructure of autotoxicity-stressed cucumber (Cucumis sativus l.) seedlings. Agron. 9, 265. doi: 10.3390/agronomy9050265
Yin, R., Bai, T., Ma, F., Wang, X., Li, Y., Yue, Z. (2010). Physiological responses and relative tolerance by Chinese apple rootstocks to NaCl stress. Sci. Hortic. 126, 247–252. doi: 10.1016/j.scienta.2010.07.027
Zhang, X., Li, S., Tang, T., Liu, Y., Tahir, M. M., Wang, C., et al. (2022). Comparison of morphological, physiological, and related-gene expression responses to saline-alkali stress in eight apple rootstock genotypes. Sci. Hortic. 306, 111455. doi: 10.1016/j.scienta.2022.111455
Zhang, K. X., Tian, W., Jun, D., Bai, T. H., Kun, W., Li, C. Y. (2016). Comprehensive evaluation of tolerance to alkali stress by 17 genotypes of apple rootstocks. J. Int. Agric. 15, 1499–1509. doi: 10.1016/S2095-3119(15)61325-9
Zhang, Y. P., Zhu, X. H., Ding, H. D., Yang, S. J., Chen, Y. Y. (2013). Foliar application of 24-epibrassinolide alleviates high-temperature-induced inhibition of photosynthesis in seedlings of two melon cultivars. Photosynthetic. 51, 341–349. doi: 10.1007/s11099-013-0031-4
Zhao, L., Yang, T., Xing, C., Dong, H., Qi, K., Gao, J., et al. (2019). The β-amylase PbrBAM3 from pear (Pyrus betulaefolia) regulates soluble sugar accumulation and ROS homeostasis in response to cold stress. Plant Sci. 287, 110184. doi: 10.1016/j.plantsci.2019.110184
Zhu, T., Deng, X., Zhou, X., Zhu, L., Zou, L., Li, P., et al. (2016). Ethylene and hydrogen peroxide are involved in brassinosteroid-induced salt tolerance in tomato. Sci. Rep. 6, 1–15. doi: 10.1038/srep35392
Keywords: irregular stomatal conductance, oxidative injury, plant defense system, photosynthetic mechanisms, poor growth
Citation: Zhang Z, Chen Z, Song H and Cheng S (2023) From plant survival to thriving: exploring the miracle of brassinosteroids for boosting abiotic stress resilience in horticultural crops. Front. Plant Sci. 14:1218229. doi: 10.3389/fpls.2023.1218229
Received: 06 May 2023; Accepted: 26 June 2023;
Published: 21 July 2023.
Edited by:
Mohammad Shah Jahan, Sher-e-Bangla Agricultural University, BangladeshReviewed by:
Sami Abou Fayssal, University of Forestry, BulgariaSunny Ahmar, University of Silesia in Katowice, Poland
Copyright © 2023 Zhang, Chen, Song and Cheng. This is an open-access article distributed under the terms of the Creative Commons Attribution License (CC BY). The use, distribution or reproduction in other forums is permitted, provided the original author(s) and the copyright owner(s) are credited and that the original publication in this journal is cited, in accordance with accepted academic practice. No use, distribution or reproduction is permitted which does not comply with these terms.
*Correspondence: Zhilu Zhang, enpsdTExMTExQGdtYWlsLmNvbQ==; enpsdTExMTFAMTI2LmNvbQ==