- 1State Key Laboratory of Rice Biology and Breeding, Ministry of Agriculture Key Laboratory of Molecular Biology of Crop Pathogens and Insects, Key Laboratory of Biology of Crop Pathogens and Insects of Zhejiang Province, Institute of Biotechnology, Zhejiang University, Hangzhou, China
- 2Department of Plant Pathology, Faculty of Agriculture, Minia University, ElMinya, Egypt
- 3Department of Agricultural Botany, Faculty of Agriculture, Tanta University, Tanta, Egypt
- 4Department of Biology, College of Science, Princess Nourah bint Abdulrahman University, Riyadh, Saudi Arabia
- 5Botany and Microbiology Department, Faculty of Science, Beni-Suef University, Beni-Suef, Egypt
- 6Institute of Eco-Environmental Protection, Shanghai Academy of Agricultural Sciences, Shanghai, China
- 7Institute of Biotechnology, Ningbo Academy of Agricultural Sciences, Ningbo, China
- 8State Key Laboratory for Managing Biotic and Chemical Threats to the Quality and Safety of Agro-products, Key Laboratory of Biotechnology in Plant Protection of Ministry of Agriculture and Zhejiang Province, Institute of Plant Virology, Ningbo University, Ningbo, China
Introduction: Bacterial leaf blight (BLB) caused by Xanthomonas oryzae pv. oryzae (Xoo) is one of the most devastative diseases that threatens rice plants worldwide. Biosynthesized nanoparticle (NP) composite compounds have attracted attention as environmentally safe materials that possess antibacterial activity that could be used in managing plant diseases.
Methods: During this study, a nanocomposite of two important elements, nickel and silicon, was biosynthesized using extraction of saffron stigmas (Crocus sativus L.). Characterization of obtained nickel-silicon dioxide (Ni-SiO2) nanocomposite was investigated using Fourier transform infrared spectroscopy (FTIR), X-ray diffraction (XRD), Transmission/Scanning electron microscopy (TEM/SEM), and energy-dispersive spectrum (EDS). Antibacterial activities of the biosynthesized Ni-SiO2 nanocomposite against Xoo were tested by measuring bacterial growth, biofilm formation, and dead Xoo cells.
Results and discussions: The bacterial growth (OD600) and biofilm formation (OD570) of Xoo treated with distilled water (control) was found to be 1.21 and 1.11, respectively. Treatment with Ni-SiO2 NPs composite, respectively, reduced the growth and biofilm formation by 89.07% and 80.40% at 200 μg/ml. The impact of obtained Ni-SiO2 nanocomposite at a concentration of 200 μg/ml was assayed on infected rice plants. Treatment of rice seedlings with Ni-SiO2 NPs composite only had a plant height of 64.8 cm while seedlings treated with distilled water reached a height of 45.20 cm. Notably, Xoo-infected seedlings treated with Ni-SiO2 NPs composite had a plant height of 57.10 cm. Furthermore, Ni-SiO2 NPs composite sprayed on inoculated seedlings had a decrease in disease leaf area from 43.83% in non-treated infected seedlings to 13.06% in treated seedlings. The FTIR spectra of biosynthesized Ni-SiO2 nanocomposite using saffron stigma extract showed different bands at 3,406, 1,643, 1,103, 600, and 470 cm−1. No impurities were found in the synthesized composite. Spherically shaped NPs were observed by using TEM and SEM. EDS revealed that Ni-SiO2 nanoparticles (NPs) have 13.26% Ni, 29.62% Si, and 57.11% O. Xoo treated with 200 µg/ml of Ni-SiO2 NPs composite drastically increased the apoptosis of bacterial cells to 99.61% in comparison with 2.23% recorded for the control.
Conclusions: The application of Ni-SiO2 NPs significantly improved the vitality of rice plants and reduced the severity of BLB.
1 Introduction
Rice (Oryza sativa L.) is the most consumed cereal crop worldwide. Food and Agriculture Organization considers rice as an important crop for food security in the world (Wang et al., 2023). One of the most serious diseases infecting rice plants is bacterial leaf blight (BLB) caused by Xanthomonas oryzae pv. oryzae (Xoo). BLB is a dominant diseases among various rice varieties (Singh et al., 2015). Infection by Xoo reduces the efficiency of photosynthesis and metabolism of rice plants, which subsequently leads to yield loss of up to 80% (Yasmin et al., 2017; Ma et al., 2023).
Diverse management strategies have been applied to control plant diseases. Use of chemical bactericides could be effective in controlling BLB. However, because of the extensive application of traditional chemical bactericides and antibiotics, it may catalyze mutations and lead to durable resistant races of pathogenic bacteria (Russo et al., 2008; Xu et al., 2010).
Marques et al. (2009) reported that the widespread occurrence of copper and streptomycin resistance in field isolates and its adaptation to bactericides have a negative impact on the chemical management of Xanthomonas campestris pv. viticola. In China, different studies have reported streptomycin resistance in various phytopathogens. Choi et al. (2015) concluded that more than 50% of tested field strains of Pseudomonas syringae pv. tabaci showed medium- to high-level resistance to streptomycin. The evolution of Xoo strains in overcoming single-gene–based resistance has been reported. For instance, Xa4, a single-based breeding gene for BLB management has been defeated by Xoo sub-population evolution (Shanti et al., 2010).
Use of nanoparticles (NPs) to combat plant diseases is one of the best tools to enhance pathogen suppression while maintaining an eco-friendly and safe method as it results in the bioreduction of metals to stable metallic NPs through a green route (Melo et al., 2018). While many NPs have existed, currently, they have not been widely applied in plant pathology. However, the recent use of nano-medicine against human pathogens has re-evolution plant disease management approach (Elmer et al., 2018). Recently, NPs of metallic oxides (single and composites) have gained momentum in phytopathology. The antibacterial action of NPs against phytopathogens is confirmed by many studies (Abdallah et al., 2020). For instance, ZnO NPs are found to be efficient against different pathogenic bacteria including Xoo and fungi (Ogunyemi et al., 2019). In addition, Cai et al. (2018) reported the antibacterial action of magnesium oxide NPs against Ralstonia solanacearum. The physicochemical characteristics of NPs increase their interaction with bacteria and improves their anti-microbial activities (Aziz et al., 2016; Rudramurthy et al., 2016; Ihtisham et al., 2021). NPs bind to the pathogen’s cell wall causing deformation of cell membranes due to high-energy transfer and, subsequently, lead to the death of the pathogen (Pereira et al., 2022). In bacteria, metal NPs (MNPs) increase cell membrane permeability and cell destruction. Among known NPs, nickel has gained wide interest as an antifungal and antibacterial element. Nickel-based NPs have been used for controlling several plant pathogenic fungi (Ahmed et al., 2016; Markowicz, 2023). Jeyaraj Pandian et al. (2016) and Mirhosseini et al. (2018) reported a high growth inhibition against Gram-negative bacteria and Candida species (C. albicans and C. tropicalis) by using nickel oxide (NiO) NPs.
To improve NP properties and increase their efficacy, the synthesis of nanocomposites was recently tested for that purpose (Prakasham et al., 2010; Baig et al., 2021). Omanović-Mikličanin et al. (2020) explained that the synthesis of nanocomposites consists of an assemblage of two different natural materials, which introduces material with greater performance characteristics than that of the original components separately. Lattuada and Hatton (2011) and Tao et al. (2008) reported that nanocomposites include the interaction between their different materials, and these interactive nanocomposites usually possess distinct properties that are not expressed in their individual elemental components. One of the most popular inert support materials is silica (Liou, 2004; Adam and Andas, 2007). The potential changes in the characterization of NiO NPs and their efficacy as a bactericide, when combined with supported component such as silica, and the role it could play in management of BLB are not known. Therefore, this study will investigate the potential use of such a nanocomposite in suppressing Xoo resulting in decreased disease severity.
MNPs are synthesized by many physiochemical methods such as co-precipitation, sol-gel, microemulsion, hydrothermal reaction, electrospray synthesis, and laser ablation. Biogenic methods such as using plant extracts can also be used for the synthesis of MNPs. Biosynthesis of NPs via plant extracts is economical, eco-friendly, and non-hazardous (Dubey et al., 2010; Alharbi et al., 2022). The ability of various plants (pomegranate, rose, banana, hibiscus, geranium leaves, cinnamomum, aloe, and basil) for the synthesis of NPs has been studied (Sathishkumar et al., 2009; Ahmad et al., 2010; Philip, 2010). Saffron (Crocus sativus), a bulbous perennial belonging to the iris family (Iridaceae) (Siddiqui et al., 2018), has been successfully used in the biosynthesis of several MNPs (Abootorabi et al., 2016; Bagherzade et al., 2017). The aqueous extract of saffron stigmas has OH groups from variety of phenolic compounds. These OH groups improve saffron’s capability for the biosynthesis of NPs, as it reacts with metal ions and plays a role for the reduction of metal raw materials to MNPs (Khan and Rizvi, 2014). Therefore, this study aims to biosynthesize nickel-silicon NP composite using extract of saffron stigmas, to characterize the obtained composite, and to investigate the antibacterial action of obtained biosynthesized nickel and silica NPs against Xoo and its impact on rice plants challenged with Xoo.
2 Materials and methods
2.1 Extraction of aqueous saffron
Aqueous saffron extraction was carried out in accordance to the method of Amin et al. (2017). A gram of dried saffron stigmas was added to 100 ml of deionized water in a beaker and then placed in a water bath for 4 h at 60°C. The extract was filtered twice using filter paper Whatman no.1 that was used directly for the synthesis of Ni-SiO2 NP composite.
2.2 Biosynthesis of Ni-SiO2 NP composite
To synthesize Ni-SiO2 composite, a 100-ml solution of each element (1 mM bulk NiO and 1 mM bulk SiO2) was prepared separately by adding previously prepared 100 ml of aqueous saffron extract to each one and then stirred at 180 Revolution Per Minute (rpm) for 4 h at 60°C. Then, Ni-SiO2 composite was prepared by mixing the previously prepared solutions of NiO and SiO2 using a ratio of 1:1 (v/v). The new mixture was swirled for 4 h at 60°C. The final solution was divided into 50-ml tubes and centrifuged (10,000 rpm/20 min). The pellets were retrievd and washed gently using ddH2O. Obtained pellets were lyophilized for 8 h.
2.3 Characterization of Ni-SiO2 composite
To evaluate the formation of Ni-SiO2 NPs in the obtained powder, Fourier transform infrared spectroscopy (FTIR) analysis was done by employing spectrometer (Vector 22, Bruker, Germany) at the range of 500–4,000 cm−1 region at a resolution of 4 cm−1. X-ray diffraction (XRD) was adopted to test the purity of obtained particles, and the mean crystallite size from XRD was calculated adopting the Scherrer equation (Jeffery, 1957). Transmission electron microscopy (TEM) was employed to observe morphology of NPs using (JEM-1230, JEOL, Akishima, Japan). Obtained NP powder was scanned by scanning electron microscopy (SEM) using (TM-1000, Hitachi, Japan). The SEM microscope was connected to energy-dispersive spectrum (EDS) to be assured of the presence of the elements.
2.4 In vitro inhibitory effect of Ni-SiO2 NP composite and determination of minimum inhibition of concentration
Xoo strain GZ 0005 used for this investigation was collected from the Institute of Biotechnology, College of Agriculture and Biotechnology, Zhejiang University, China. The virulence of Xoo was tested and confirmed before the study. The antibacterial activity of Ni-SiO2 NP composite against Xoo was evaluated by using the agar well diffusion assay as explained by Monteiro et al. (2013). An overnight 100 µl of Xoo culture (approximately 1 × 108 Colony forming unit (CFU)/ml) was added to 5 ml of Nutrient Agar (NA) medium, and, then, 50 µl each of previously prepared concentration (Ni-SiO2 NP composite at 50, 100, and 200 μg/ml) was poured into 6-mm-diameter agar wells. Five replications were done for this assay; each replication was typified by a plate consisting of a well for each of the three concentrations. The plates were incubated at 30°C for 48 h. The clearance zone around the well was scaled after 48 h. The experiment was repeated following the same condition.
The minimum inhibition of concentration (MIC) of Ni-SiO2 NP composite against Xoo was investigated as explained by Wiegand et al. (2008). In detail, 100 µl of an overnight culture of Xoo (approximately 1 × 108 CFU/ml) was poured into sterile tubes containing 5 ml of nutrient broth. Ni-SiO2 NP composite was added to each tube, and the concentrations were adjusted to 50, 100, and 200 µg/ml each in respective tube. The tubes were kept in 30°C with shaking at approximately 180 rpm. After 48 h of incubation, MIC was measured using a UV spectrophotometer by the optical density at 600 nm (OD600). The investigation was repeated twice.
2.5 Effect of Ni-SiO2 NP composite on biofilm formation of Xoo
The ability of Ni-SiO2 NP composite to inhibit Xoo biofilm was measured as described by Merritt et al. (2005). A 100 μl of overnight Xoo culture (1 × 108 CFU/ml) was added to Nutrient Broth (NB) medium containing Ni-SiO2 NP composite to get a final concentration of 50, 100, and 200 μg/ml. The mixture was kept static in a 30°C incubator for 48 h to develop a biofilm in a 96-well plate. To stain the attached biofilm, crystal violet (CV) was added to the wells after discarding the supernatant. CH3COOH (33%) was used in solubilizing the CV attached to the biofilm and measured at OD570.
2.6 Live/dead assays to infer the cell membrane integrity
Fluorescence emitted from propidium iodide (PI) of dead bacterial cells after incubation with the NPs was measured by flow cytometer (Kumar et al., 2011). Xoo culture (1 × 108 CFU/ml) was centrifuged (5,000 rpm/5 min), and Ni-SiO2 NP (200 µg/ml) composite was added to the obtained pellets for 4 h. PI was added in the dark for 30 min to stain the chromatin of bacterial cells. Subsequently, the dead cell ratio of Xoo cells was measured by flow cytometry (FC) (Gallios Beckman Coulter, Germany).
2.7 Effect of Ni-SiO2 composite on rice seedlings infected with Xoo
The experiment was conducted as complete randomized blocks. Five replications were used per treatment. Three rice seedlings (cv. II You 023 Oryza sativa L.) in each replicate were sown in small pots filled with sterile soil and kept in the growth chamber under 28 ± 2°C, 80% relative humidity with a photoperiod of 16-h light and 8-h dark. This experiment consisted of four treatments that include the following:
1. In the first treatment, 3-week-old rice seedlings were sprayed with a suspension of Ni-SiO2 NP composite (200 µg/ml); after 48 h, the rice seedlings were inoculated with Xoo strain GZ 0005 culture (1 × 108 CFU/ml) via leaf clipping.
2. In the second test treatment, 3-week-old rice seedlings were sprayed with distilled water; after 48 h, the rice seedlings were inoculated with Xoo strain GZ 0005 culture (1 × 108 CFU/ml) via leaf clipping.
3. In the third test treatment, 3-week-old rice seedlings were sprayed with a suspension of Ni-SiO2 NP composite (200 µg/ml), and no Xoo inoculation was applied.
4. The fourth treatment, 3-week-old rice seedlings were sprayed with distilled water, and no Xoo inoculation was applied.
The experiment was carried out at 11:00 a.m. to ensure that the stomata had opened. Diseased leaf area, plant height, and fresh and dry biomass weight were recorded 1 month after application of Ni-SiO2 NP composite on rice plants. The percentage of diseased leaf area (DLA%) was calculated as follows:
2.8 Statistical analysis
Data were subjected to analysis of variance using SAS, 2003 software (SAS Institute, Cary, NC, USA). The general linear model procedure was used to check the significant differences among the main treatments. Individual comparisons between mean values were performed using Duncan’s method (P ≤ 0.05). Simple linear regression (SLR) analysis was performed to better understand the relationship between concentrations of Ni-SiO2 NP composite and inhibition zone, bacterial growth inhibition, and biofilm formation inhibition. The fitted regression model was stated as a regression equation, coefficient of determination (R2), , and p-value as determined by the F-test (P ≤ 0.05).
3 Results
3.1 Characterization of Ni-Si O2 NP composite
The FTIR spectra of biosynthesized Ni-SiO2 NP composite revealed various bands at 3,406, 1,643, 1,103, 800, and 470 cm−1 (Figure 1A). The band at 3,406 cm−1 was assigned to hydroxl stretch hydrogen bonds, the band at 1,643 cm−1 was related to C=C stretch, and the band at 1,103 cm−1 indicates C–O stretches. The peaks at 800 and 470 cm−1 were attributed to the symmetric vibration of Si atoms. XRD pattern showed no impurities in tested samples. The sharpest diffraction peaks were recorded at 2θ around 43°, which can be indexed as (202) for nickel, and at 2θ around 20°, representing (101) for silica (Figure 1B). Spherically shaped NPs were observed by using TEM (Figure 1C) and SEM (Figure 1D). Data from the EDS of Ni (Figure 2A), Si (Figure 2B), O (Figure 2C), and Ni-SiO2 NPs (Figure 2D) revealed that Ni-SiO2 NP composite has 13.26% Ni, 29.62% Si, and 57.11% O (Figure 2E).
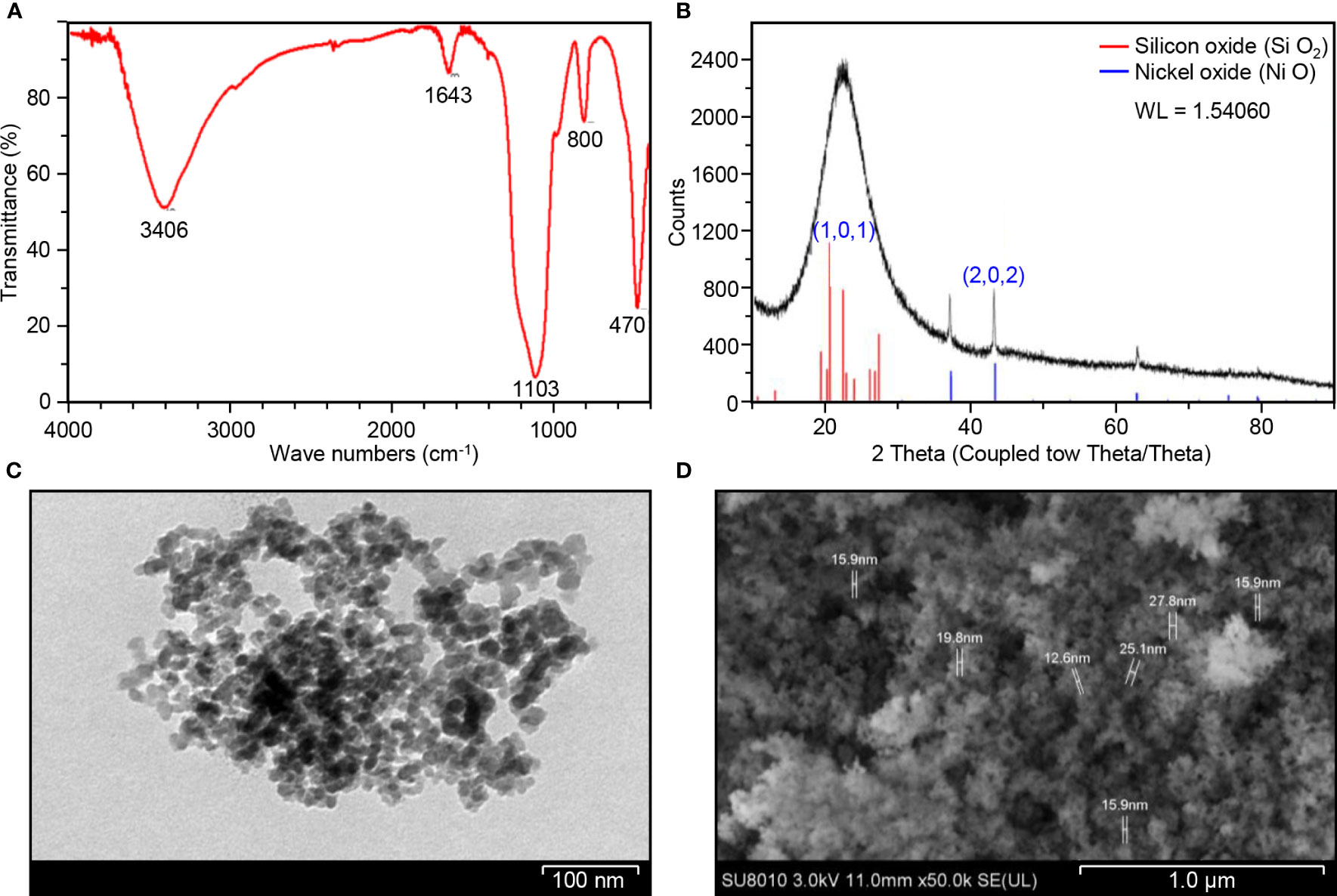
Figure 1 Structural and compositional characterization of Ni-SiO2 NP composite. (A) FTIR spectrum of Ni-SiO2 NPs. (B) X-ray diffraction patterns of Ni-SiO2 NP composite. (C) Bright-field TEM image of Ni-SiO2 NP composite. (D) SEM image of Ni-SiO2 NP composite.
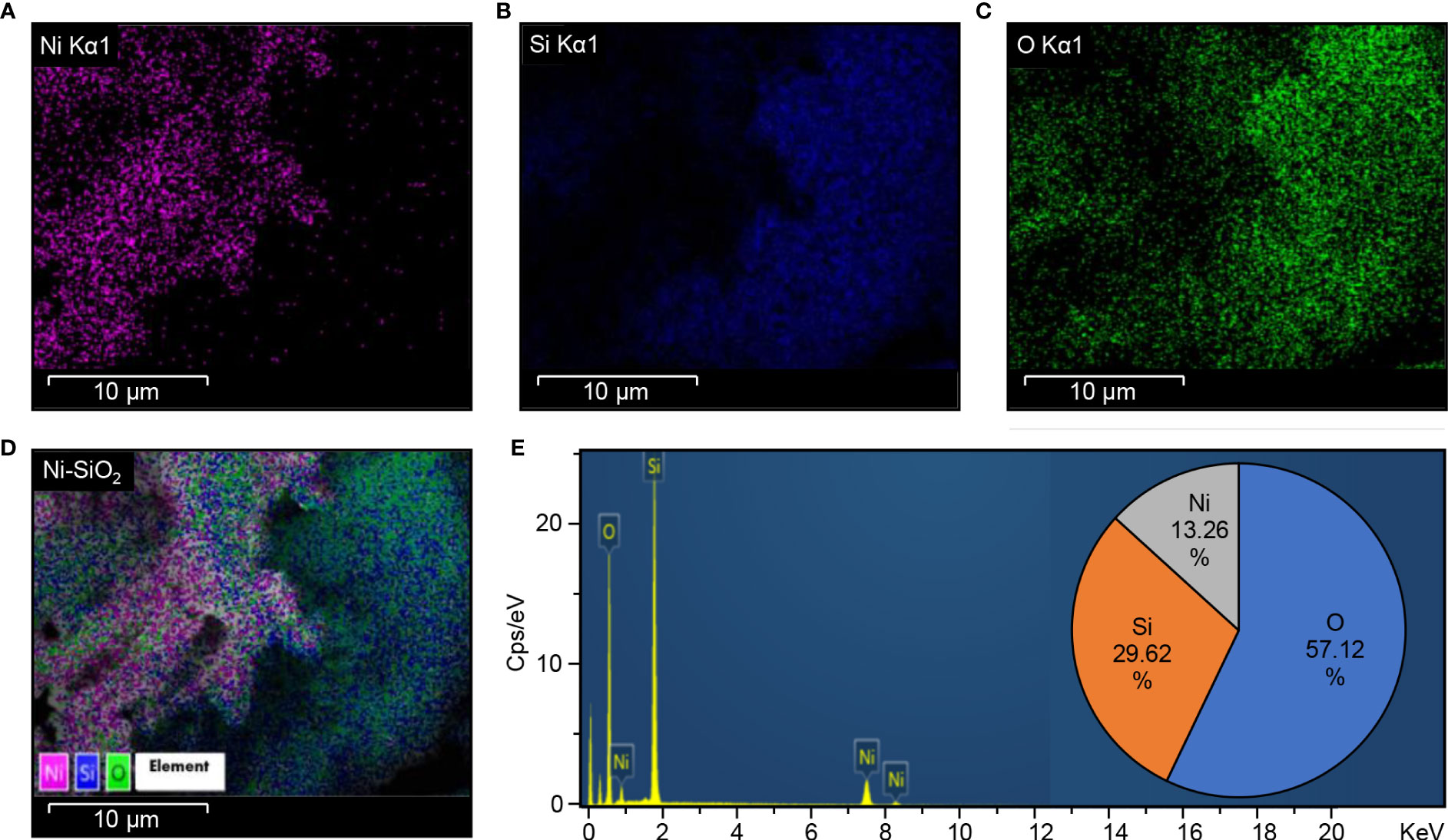
Figure 2 Energy dispersion spectrum (EDS) Ni-SiO2 NP composite. (A) Ni Kα1, (B) Si Kα1, (C) O Kα1, (D) Ni-SiO2, and (E) composite. Energy-dispersive spectrum showing the predominance of Ni, Si, and O elements and the percentage of each element in the Ni-SiO2 NP composite.
3.2 Composition of biosynthesized Ni and SiO2 NPs enhances their antibacterial activity against Xoo
The ability of Ni, Si, and Ni-SiO2 NPs to inhibit Xoo bacteria was investigated by using plate assay technique (Figures 3A–C, respectively). Three concentrations (50, 100, and 200 µg/ml) of each NP were tested. In general, all the tested NPs had a dose-dependent antibacterial action against Xoo that significantly inhibited its growth in vitro (Figure 3D). It is worth mentioning that the Ni-SiO2 NP composite was the most efficient NPs suppressing Xoo growth (Figures 3C, D). The three tested concentrations of Ni-SiO2 NP composite (50, 100, and 200 µg/ml) produced inhibition zones of 2.1, 2.4, and 2.9 cm, respectively, compared with 0.9, 1.3, and 1.5 cm for SiO2 and 0.8, 1.1, and 1.2 cm for NiO NPs (Figure 3D). The MIC of the Ni-SiO2 NPs was 200 μg/ml, in which Xoo growth was inhibited by 89.07%, whereas using 50 and 100 μg/ml resulted in 21.50% and 54.37% inhibition, respectively.
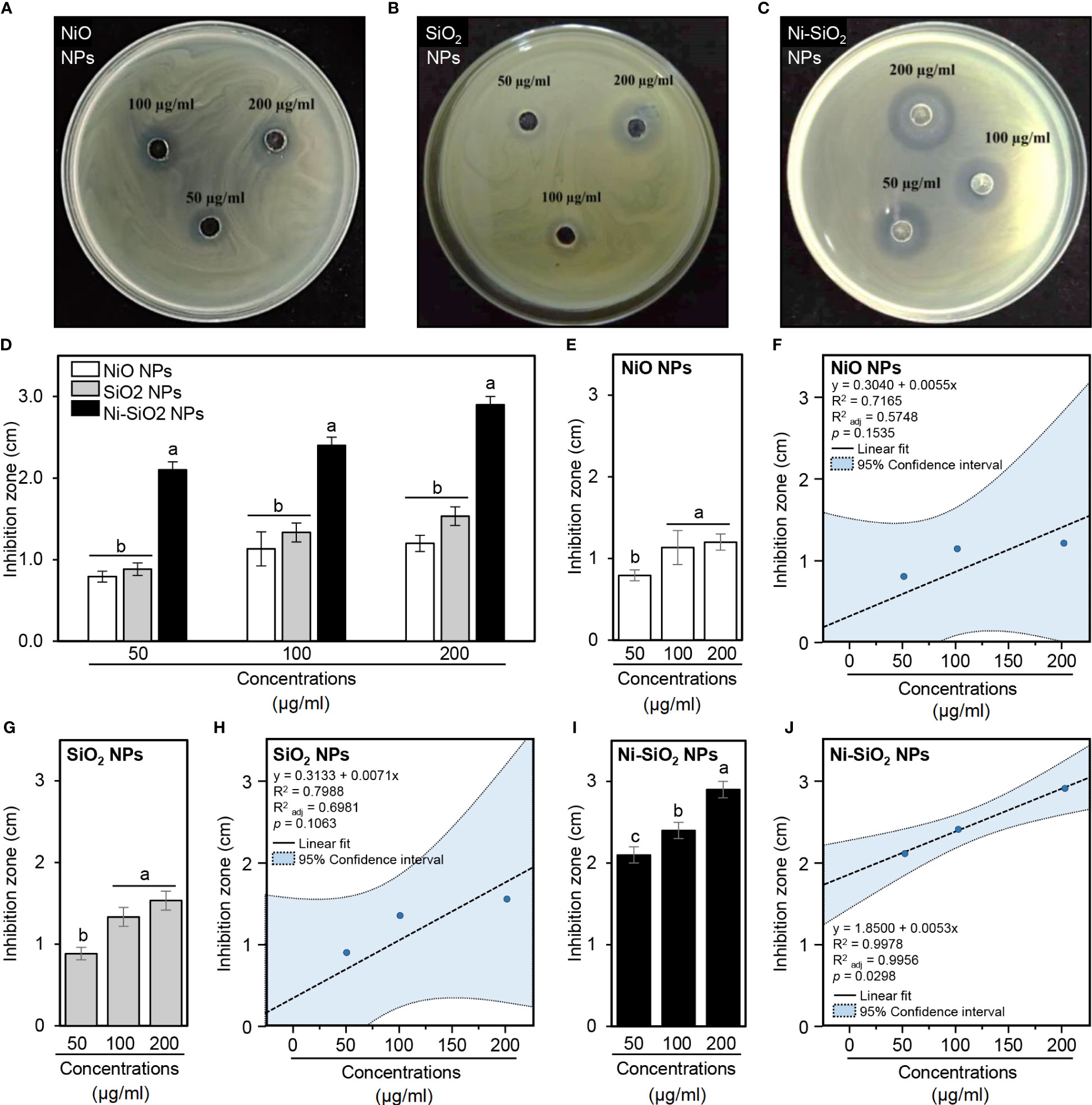
Figure 3 In vitro antibacterial action of NiO, SiO2, and Ni-SiO2 NP composite against Xoo. (A–C) Antibacterial activity of different concentrations (50, 100, and 200 µg/ml) NiO, SiO2, and Ni-SiO2 NP composite, respectively, against Xoo. (D) Diameters of the inhibition zones of Xoo after the treatment of NPs (50, 100, and 200 µg/ml). (E, G, I) Diameters of the inhibition zones of Xoo after the treatment with different concentrations of NiO, SiO2, and Ni-SiO2 NP composite, respectively. Vertical bars represent the means ± standard deviation (means ± SD) of three biological replicates (n = 3). Different letters indicate statistically significant differences among treatments, whereas bars followed by the same letter(s) are not significantly different (P ≤ 0.05). (F, H, J) Simple linear regression between concentrations (µg/ml) NiO, SiO2, and Ni-SiO2 NP composite, respectively, and the inhibition zones (cm). The linear fit regression line is presented as a dashed line, whereas the 95% confidence intervals are light blue–shaded and edged by dotted lines. Regression equations, R2, R2adj, and p-value based on the F-test (P< 0.05) were also obtained and presented within the graph.
In general, in vitro experiments showed that NiO NPs efficiently suppressed the bacterial growth of Xoo in a concentration-dependent fashion with no significant differences between the two highest concentrations (100 and 200 µg/ml) (Figure 3E). However, SLR between NiO NP concentrations (µg/ml) and inhibition zone (cm) showed a positive correlation between them (y = 0.3040 + 0.0055x, R2 = 0.7165, R2adj = 0.5748, and P = 0.1535; Figure 3F). Likewise, the antibacterial activity of SiO2 NPs was identical to that of NiO NPs (Figure 3G) even without significant differences between them at all studied concentrations (Figure 3D). Moreover, SLR showed a positive correlation between the concentrations of SiO2 NPs and clearance zone (y = 0.3133 + 0.0071x, R2 = 0.7988, R2adj = 0.6981, and P = 0.1063; Figure 3H). Furthermore, the most effective NP, Ni-SiO2 composite, exhibited a clear progressive increase in inhibition zones (Figure 3I), which was strongly correlated with its concentrations (y = 1.8500 + 0.0053x, R2 = 0.9978, R2adj = 0.9956, and P = 0.0298; Figure 3J).
3.3 Ni-SiO2 NP composite inhibits bacterial growth of Xoo in nutrient broth
Furthermore, because of the superiority of Ni-SiO2 NP composite over NiO and SiO2 NPs, the focus was placed on it throughout the rest of this study. Briefly, in nutrient broth, Ni-SiO2 NP composite significantly inhibited the growth of Xoo in a dose-dependent manner as revealed by OD600 (Figure 4A). In other words, the inhibition extents increased from 50< 100< 200 µg/ml. In agreement with these findings, SLR showed strong negative correlation (y = 1.1876 − 0.0055x, R2 = 0.9833, = 0.9750, and P = 0.0084) between Xoo bacterial growth (OD600) and Ni-SiO2 NP concentrations (µg/ml) (Figure 4B).
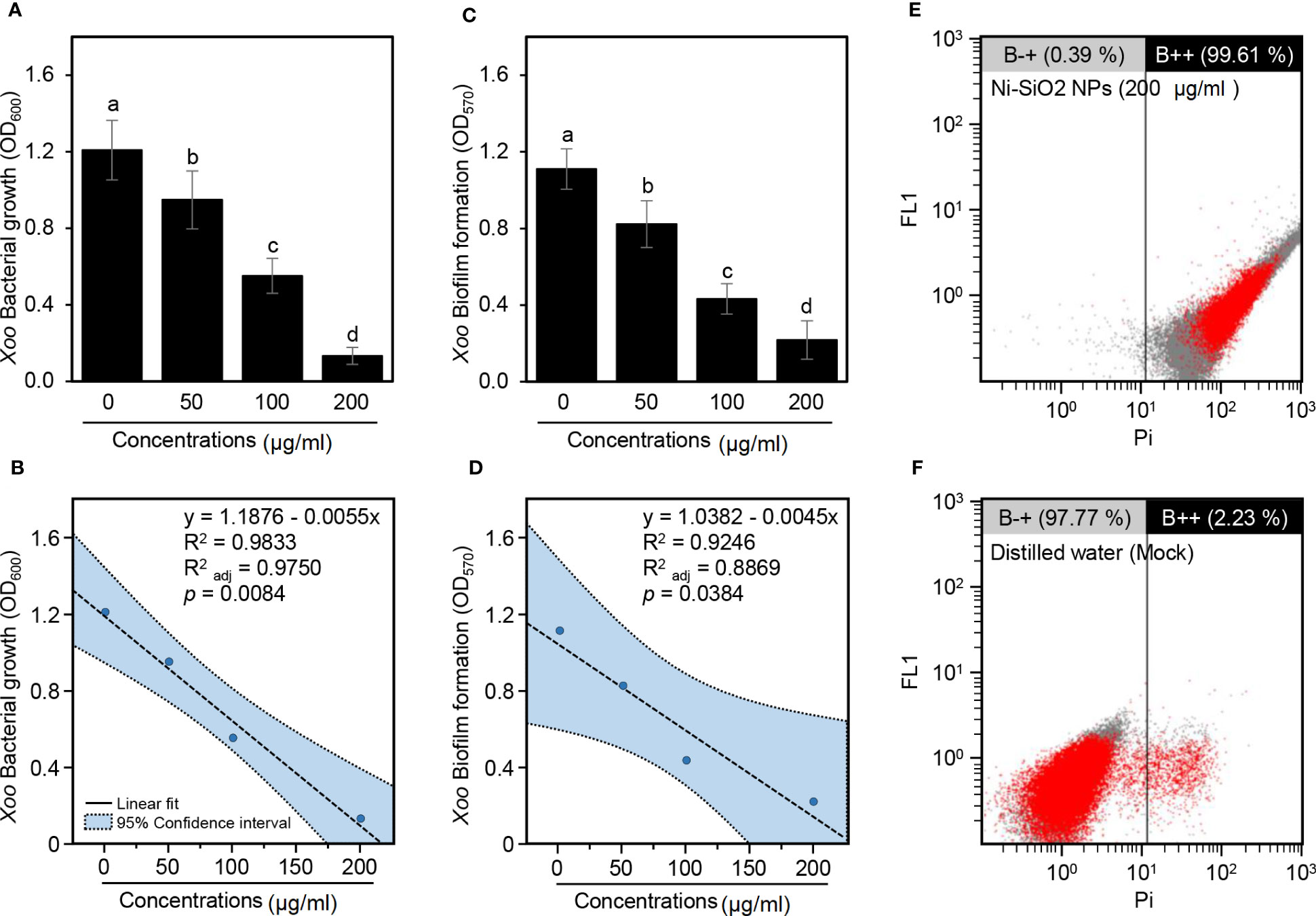
Figure 4 Antibacterial activity of Ni-SiO2 NP composite against Xoo. (A) Bacterial growth of Xoo in nutrient broth containing different concentrations of Ni-SiO2 NP composite (0, 50, 100, or 200 µg/ml) as indicated by optical density at 600 nm (OD600). (B) Simple linear regression between concentrations of Ni-SiO2 NP composite (µg/ml) and Xoo Bacterial growth (OD600). (C) Biofilm formation of Xoo after the treatment with different concentrations of Ni-SiO2 NP composite (0, 50, 100, or 200 µg ml−1) as indicated by optical density at 570 nm (OD570). (D) Simple linear regression between concentrations of Ni-SiO2 NP composite (µg/ml) and Xoo biofilm formation (OD570). (E, F) Flow cytometry observations of Xoo cells after incubation with Ni-SiO2 NP composite (200 μg/ml) or distilled water, respectively. In panels (A) and (C), bars represent the means ± standard deviation (means ± SD) of three biological replicates (n = 3). Different letters indicate statistically significant differences among treatments (P ≤ 0.05). In panels (B) and (D), the linear fit regression line is presented as a dashed line, whereas the 95% confidence intervals are light blue–shaded and edged by dotted lines). Regression equations, R2, R2adj, and P-value based on the F-test (P< 0.05) were also obtained and presented within the graph.
3.4 Ni-SiO2 NP composite inhibits biofilm formation of Xoo
Likewise, Ni-SiO2 NP composite significantly hindered biofilm development of Xoo cells in a dose-dependent manner because the higher concentrations showed lower biofilm formation, and vice versa, as indicated by optical density at 570 nm (OD570) (Figure 4C). Antibiofilm activity of 25.91%, 61.06%, and 80.40% were detected as a result of using Ni-SiO2 NPs of 50, 100, and 200 µg/ml, respectively. In addition, SLR showed a strong negative correlation (y = 1.0382 − 0.0045x, R2 = 0.9246, = 0.8869, and P = 0.0384) between biofilm formation (OD570) and Ni-SiO2 concentrations (µg/ml) (Figure 4D).
3.5 Ni-SiO2 NP composite causes cell injury or death to Xoo
Moreover, cell damage/apoptosis of Xoo cells was assessed using FC and PI-based method. Briefly, incubation of Xoo with Ni-SiO2 NP composite (200 µg/ml) drastically increased the apoptosis of the bacterial cells to 99.61% (Figure 4E) compared with 2.23% for the mock control (distilled water; Figure 4F). Together, in addition to the inhibition of bacterial growth, our findings proved that Ni-SiO2 NP composite might cause cell puncture or death to Xoo when it was amended with a concentration of 200 µg/ml.
3.6 Application of Ni-SiO2 NP composite improves plant growth and reduces disease severity of BLB in rice
A notable improvement in rice growth was observed when Ni-SiO2 NP composite was applied at a concentration of 200 µg/ml as a foliar application on healthy and Xoo-infected rice plants under greenhouse conditions (Figure 5A). Interestingly, Ni-SiO2 NP composite application notably increased the leaf length of treated rice plants (Figures 5B, C); however, it reduced the total diseased leaf area in Xoo-infected rice plants (Figure 5D). Accordingly, the disease leaf area decreased from 43.83% in non-treated control plants to 13.06% when Ni-SiO2 NP composite was applied to infected plants (Figure 5D). Moreover, the amendment with Ni-SiO2 NPs significantly increased the height of non-infected rice plants to 64.8 cm in comparison with 45.2 cm of plants amended with only water (Figure 5E). Likewise, treating Xoo-infected rice plants with Ni-SiO2 NP composite significantly increased plant height to 57.1 cm compared with non-treated infected rice plants, which appeared short with an average plant height just below 20 cm. Similarly, the application of Ni-SiO2 NPs produced almost the same pattern in terms of root length (Figure 5F). In addition to improving rice growth, using Ni-SiO2 NP composite showed a positive effect on biomass. Briefly, application of Ni-SiO2 NPs significantly increased both fresh (Figure 5G) and dry (Figure 5H) weight of treated healthy and Xoo-infected rice plants compared with non-treated ones.
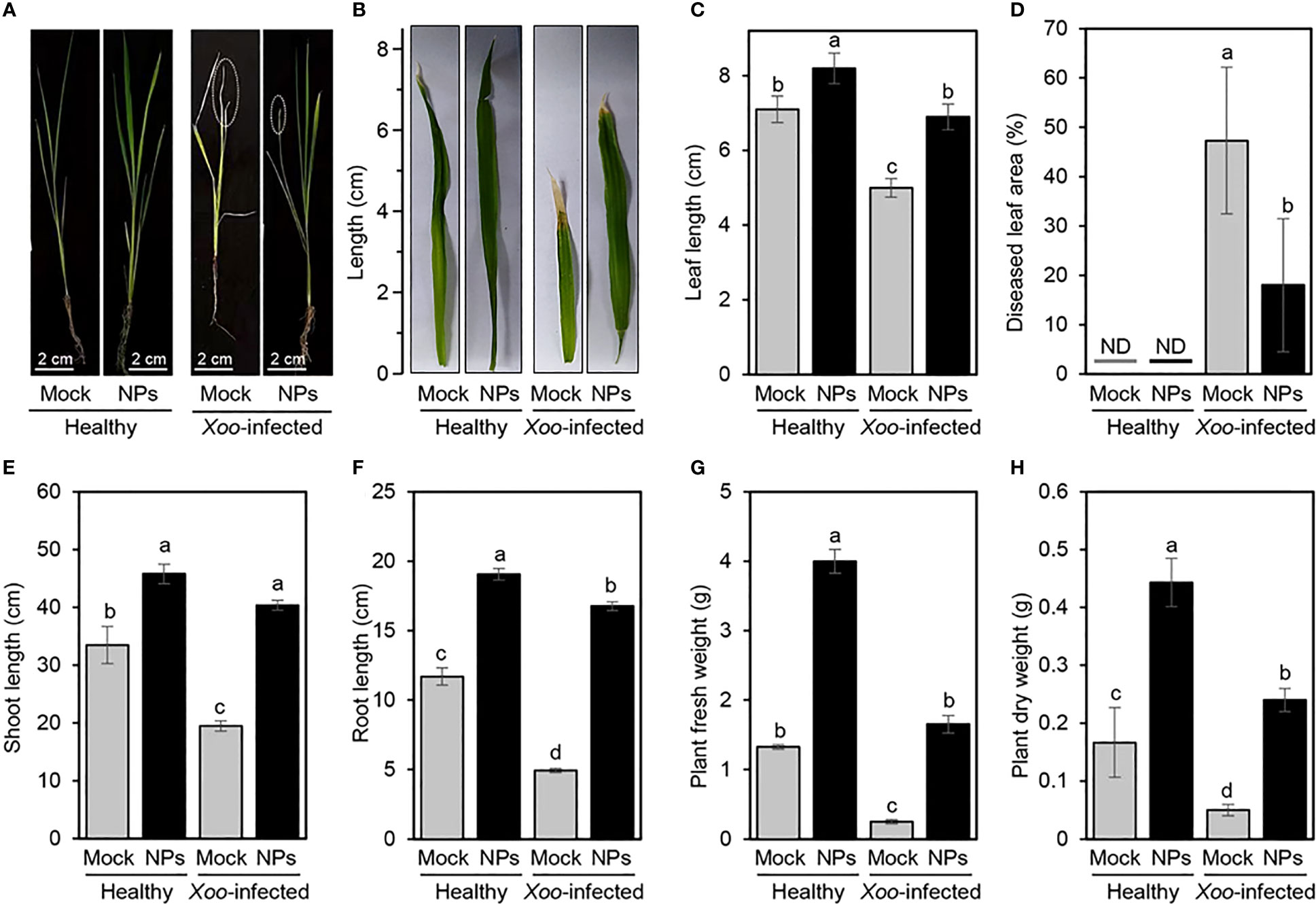
Figure 5 Effect of application of Ni-SiO2 NP composite on rice growth and disease severity of bacterial blight disease of rice caused by Xanthomonas oryzae pv. oryzae (A, B) Ni-SiO2-treated vs. non-treated healthy and Xoo-infected rice plants and leaves, respectively. (C) Leaf length (cm), (D) diseased leaf area (%), (E) shoot length (cm), (F) root length (cm), (G) plant fresh weight (g), and (H) plant dry weight (g) of Ni-SiO2treated vs. non-treated healthy and Xoo-infected rice plants. Bars represent the means ± standard deviation (means ± SD) of three biological replicates (n = 3). Different letters indicate statistically significant differences among treatments (P ≤ 0.05). Mock = Control.
4 Discussion
NPs have been applied in the field of agriculture as highly effective bactericides, fungicides, and nano fertilizers due to their small size, large surface area, and high reaction (Elmer and White, 2018; Hossain et al., 2019; Ogunyemi et al., 2019). The synthesis of NPs produced a variety of morphology, sizes, and compositions that were determined by numerous physical, chemical, and biological techniques (Pagar et al., 2023). Our study aimed to biosynthesize Ni-SiO2 NP composite with new properties that could contribute to the management of BLB by using extraction of saffron stigmas (Crocus sativus L.).
Studies of the infrared spectrum were conducted to explore the potential mechanism behind the formation of Ni-SiO2 NP composite and information about the functional groups (Irshad et al., 2018; Petousis et al., 2020). The FTIR spectra of biosynthesized Ni-SiO2 NP composite revealed various peaks that confirmed the presence of important bonds such as hydroxyl stretch, C=C stretch, C–H, and Si–O–Si bond (Majewski et al., 2013; Adel et al., 2022). Upon reviewing infrared spectrum results, plant extract of saffron stigmas could be responsible for the bio-reduction of Ni-SiO2 NP composite. Moreover, silica bonds contributed to the stability of NiO NPs. Phytochemicals easily show the ability to synthesize nickel NPs (Singh et al., 2016; Shwetha et al., 2021). The obtained results show that there are no impurities revealed by the XRD pattern in biosynthesized Ni-SiO2 composite.
Silica was able to improve the morphological characteristics of nickel NPs including particles size. Spherically shaped NPs were observed by using TEM and SEM. The size of obtained nickel-silica composite averaged between 12.6 and 27.8 nm. Our finding matches a study by Saha et al. (2015), which was able to synthesize Ni NPs of a size range of 10–30 nm in Ni-SiO2 composite prepared by sol-gel route. The size of the produced Ni NPs was smaller in comparison with that of other synthesis protocol (Lajevardi et al., 2013).
Data collected from EDS revealed that Ni-Si-O NP composite has 13.26% Ni, 29.62% Si, and 57.11% O. As reported by Saha et al. (2015), one Si atom reacts with two O2 atoms to form SiO2. Thus, 29.62% Si present in the composite combines with 57.11% O2 to produce SiO2. The crystalline nature of synthesized Ni-SiO2 NP composite was investigated by XRD technique. The wide spectrum range of 20° and 30° is attributed to the presence of an amorphous Si matrix. The formation of NiO is exempted from the phase analysis by XRD.
Ni-SiO2 NP composite was able to inhibit Xoo growth and significantly increase the ratio of Xoo dead cells to 99.61% compared with 2.23% for control. Therefore, according to the obtained results, Ni-SiO2 NP composite can be used as bactericides that have antimicrobial activity as documented by Ahmed et al. (2016) and Jeyaraj Pandian et al. (2016). As NPs have positive or low negative charges, they are electrostatically attracted and adhered to the negatively charged cell membrane of bacteria (Zein El-Abdeen and Farroh, 2019). Subsequently, it caused irregular pit formations on the cell wall of the pathogenic bacteria that facilitate the entry of NPs into periplasmic space and inside bacterial cells (Ninganagouda et al., 2014; Wang et al., 2023). The high efficacy of Ni-SiO2 NP composite against Xoo could be due to the size of the nickel NPs that have been reduced by silicon to range approximately from 10 to 30 nm, which allows nickel NPs to intensively enter the bacterial cell, resulting in ion accumulation that contributes to membrane porosity damaging the cytoplasm and cell structures. This destruction of cell structure caused the escape of the embedded cell contents, leading to bacterial cell death (Jeyaraj Pandian et al., 2016; Zhu et al., 2022).
The inhibition of biofilm formation, which was detected by using Ni-SiO2 NP composite, confirms and matches that of the previous studies on metal oxide NPs (Lee et al., 2014). Le Ouay and Stellacci (2015) and Pellieux et al. (2000) documented that the inhibitory effect of NPs on bacteria is linked to the formation of Reactive Oxygen Species (ROS). ROS promotes oxidative stress in cells and induces DNA, protein, lipids, and cell damage (Piao et al., 2011; Zhang et al., 2018). In addition to the bactericidal effect of Ni-SiO2 NP composite, it enhanced rice growth and significantly increased the height of the plant. It also showed a positive effect on rice seedlings’ biomass fresh and dry weight. Mirzajani et al. (2014) and Syu et al. (2014) reported that rice treated with NPs enhanced root growth, which may be due to the interaction between NPs and ROS scavenging, hormone signaling pathways, and auxin. Tarafdar et al. (2014) and Zafar et al. (2016) stated that metal oxide NPs shows enhancement on shoot length of Pennisetum americanum and Brassica nigra.
This study proved that the application of Ni-SiO2 NP composite significantly decreased the biofilm of Xoo, which subsequently decreased the virulence of the bacteria. The treatment with MgO and MnO2 NPs at the primary stages of growth caused a promotion in rice seedlings growth and increased the photosynthetic parameters while reducing BLB expression (Ogunyemi et al., 2023). On the basis of this report, it can be inferred that, because NPs had a positive impact on photosynthesis, the plant yield will invariably be positively affected. Xu et al. (2021) reported that the application of titanium dioxide NPs on two different cultivars of rice (WYJ23 and YY2640) significantly increased the agronomic data and yield. Therefore, on the basis of reports of the positive impacts of NPs application, it indicates that the treatment of rice with NPs improves both the agronomic trait and yield of rice irrespective of the cultivar or NPs used.
This present work provides helpful and useful insights for using the Ni-SiO2 NP composite as potent applications for antibacterial activities. Ni-SiO2 NP composite, which is cheap, stable, and nontoxic, indicates a promising safe result that can be used not only in the management of plant diseases but also as a medical treatment for human diseases. Ni NPs were used for their antibacterial activity in the field of medicine and were found to be effective when used for targeting cancer cells (Sudhasree et al., 2014; Ezhilarasi et al., 2016). Hence, despite numerous reports about the antibacterial activity of individual NP elements against Xoo, there are few studies of the nanocomposites against this pathogen (Namburi et al., 2021; Chauhan et al., 2023). Therefore, the report of this study is novel, which helps to bridge the gap of the management of Xoo using Ni-SiO2 NP composite.
5 Conclusion
In conclusion, the use of saffron stigma extract in biosynthesizing Ni-SiO2 NPs successfully produced a pure composite. The composite of nickel-silica particles have a small size range of 12.6–27.8 nm. The composite had the ability to inhibit Xoo growth to the point where 89.07% of Xoo cells were killed when treated with Ni-SiO2 NP composite (200 µg/ml). The obtained composite also showed that the bacterial anti-biofilm activity reached 80.40% and achieved 99.61% dead cells of Xoo. The application of Ni-SiO2 NP composite significantly promoted the growth of rice plants challenged with Xoo compared with untreated plants. Ni-SiO2 NP composite increased biomass fresh and dry weight. In general, Ni-SiO2 NP composite is a promising effective tool for suppressing Xoo infection on rice plants. On the basis of the potent antibacterial activity of the synthesize nanocomposite recorded in this study, we hereby suggest future studies to be conducted on the mechanism of nanocomposite on ROS and phytohoromones and their effect on rice plants yield using different cultivars.
Data availability statement
The original contributions presented in the study are included in the article/supplementary material. Further inquiries can be directed to the corresponding authors.
Author contributions
YA: conceptualization, investigation, formal analysis, and writing (original draft). YN, SO, MI, and TA: investigation, formal analysis, and writing (review and editing). RE, DA and WH: validation and writing (review and editing). LX, CY, JC, and BL: conceptualization, supervision, funding acquisition, and writing (review and editing). All authors contributed to the article and approved the submitted version.
Funding
This work is financially supported by the National Key Research and Development Program of Ningbo (2022Z175); National Natural Science Foundation of China (32072472 and 31872017); Key Research and Development Program of Zhejiang Province, China (2019C02006); Zhejiang Provincial Natural Science Foundation of China (LZ19C140002); Agricultural and Social Development Project of Jiangbei District, Ningbo, in 2021 (2021B01); and State Key Laboratory for Managing Biotic and Chemical Threats to the Quality and Safety of Agro-products (grant number 2010DS700124-ZZ2014;-KF202101;-KF202205); Princess Nourah bint Abdulrahman University Researchers Supporting Project number (PNURSP2023R15), Princess Nourah bint Abdulrahman University, Riyadh, Saudi Arabia.
Acknowledgments
The authors acknowledge the support from Princess Nourah bint Abdulrahman University Researchers Supporting Project number (PNURSP2023R15), Princess Nourah bint Abdulrahman University, Riyadh, Saudi Arabia.
Conflict of interest
The authors declare that the research was conducted in the absence of any commercial or financial relationships that could be construed as a potential conflict of interest.
Publisher’s note
All claims expressed in this article are solely those of the authors and do not necessarily represent those of their affiliated organizations, or those of the publisher, the editors and the reviewers. Any product that may be evaluated in this article, or claim that may be made by its manufacturer, is not guaranteed or endorsed by the publisher.
References
Abdallah, Y., Liu, M., Ogunyemi, S. O., Ahmed, T., Fouad, H., Abdelazez, A., et al. (2020). Bioinspired green synthesis of chitosan and zinc oxide nanoparticles with strong antibacterial activity against rice pathogen Xanthomonas oryzae pv. oryzae Molecules. 25, 20, 4795. doi: 10.3390/molecules25204795
Abootorabi, Z., Poorgholami, M., Hanafi-Bojd, M. Y., Hoshyar, R. (2016). Green synthesis of gold nanoparticles using barberry and saffron extracts. Mod. Care J. 134, 13:13000. doi: 10.5812/MODERNC.13000
Adam, F., Andas, J. (2007). Amino benzoic acid modified silica—An improved catalyst for the mono-substituted product in the benzylation of toluene with benzyl chloride. J. Colloid Interface Sci. 311, 135–143. doi: 10.1016/J.JCIS.2007.02.083
Adel, A. M., El-Shall, F. N., Diab, M. A., Al-Shemy, M. T. (2022). Biogenic silver-doped mesoporous silica nanoparticles for multifunctional eco-designed textile printing. Biomass Convers Biorefin. 1, 1–19. doi: 10.1007/S13399-022-03643-2
Ahmad, N., Sharma, S., Alam, M. K., Singh, V. N., Shamsi, S. F., Mehta, B. R., et al. (2010). Rapid synthesis of silver nanoparticles using dried medicinal plant of basil. Colloids Surf. B 81, 81–86. doi: 10.1016/J.COLSURFB.2010.06.029
Ahmed, A. I., Raj Yadav, D., Lee, S. Y. (2016). In vitro evaluation of nickel nanoparticles against various pathogenic Fusarium species. Int. J. ChemTech Res. 9, 174–183.
Alharbi, N. S., Alsubhi, N. S., Felimban, A. I. (2022). Green synthesis of silver nanoparticles using medicinal plants: Characterization and application. J. Radiat. Res. Appl. Sci. 15, 109–124. doi: 10.1016/J.JRRAS.2022.06.012
Amin, B., Hosseini, S., Hosseinzadeh, H. (2017). Enhancement of antinociceptive effect by co-administration of amitriptyline and Crocus Sativus in a rat model of neuropathic pain. Iran J. Pharm. Res. IJPR 16, 187.
Aziz, N., Pandey, R., Barman, I., Prasad, R. (2016). Leveraging the attributes of mucor hiemalis-derived silver nanoparticles for a synergistic broad-spectrum antimicrobial platform. Front. Microbiol. 7. doi: 10.3389/FMICB.2016.01984
Bagherzade, G., Tavakoli, M. M., Namaei, M. H. (2017). Green synthesis of silver nanoparticles using aqueous extract of saffron (Crocus sativus L.) wastages and its antibacterial activity against six bacteria. Asian Pac J. Trop. Biomed. 7, 227–233. doi: 10.1016/J.APJTB.2016.12.014
Baig, N., Kammakakam, I., Falath, W., Kammakakam, I. (2021). Nanomaterials: a review of synthesis methods, properties, recent progress, and challenges. Mater Adv. 2, 1821–1871. doi: 10.1039/D0MA00807A
Cai, L., Chen, J., Liu, Z., Wang, H., Yang, H., Ding, W. (2018). Magnesium oxide nanoparticles: Effective agricultural antibacterial agent against Ralstonia solanacearum. Front. Microbiol. 9. doi: 10.3389/FMICB.2018.00790
Chauhan, H., Patel, M., Patel, P., Tiwari, S., Jinah, H. N., Amaresan, N. (2023). Assessment of copper (Cu) nanoparticle for their biocontrol activity against Xanthomonas oryzae pv. oryzae, growth promotion, and physiology of rice (Oryza sativa L.) plants. Lett. Appl. Microbiol. 76, ovac066. doi: 10.1093/lambio/ovac066
Choi, Y. H., Lee, J. S., Yun, S., Baik, H. S. (2015). A LuxR-type transcriptional regulator, PsyR, coordinates regulation of pathogenesis-related genes in Pseudomonas syringae pv. tabaci. J. Life Sci. 25, 136–150. doi: 10.5352/JLS.2015.25.2.136
Dubey, S. P., Lahtinen, M., Sillanpää, M. (2010). Green synthesis and characterizations of silver and gold nanoparticles using leaf extract of Rosa rugosa. Colloids Surfaces A Physicochem Eng. Asp. 364, 34–41. doi: 10.1016/J.COLSURFA.2010.04.023
Elmer, W., Ma, C., White, J. (2018). Nanoparticles for plant disease management. Curr. Opin. Environ. Sci. Heal. 6, 66–70. doi: 10.1016/J.COESH.2018.08.002
Elmer, W., White, J. C. (2018). The future of nanotechnology in plant pathology. Annu. Rev. Phytopathol. 56, 111–133. doi: 10.1146/ANNUREV-PHYTO-080417-050108
Ezhilarasi, A. A., Vijaya, J. J., Kaviyarasu, K., Maaza, M., Ayeshamariam, A., Kennedy, L. J. (2016). Green synthesis of NiO nanoparticles using Moringa oleifera extract and their biomedical applications: Cytotoxicity effect of nanoparticles against HT-29 cancer cells. J. Photochem. Photobiol. B. 164, 352–360. doi: 10.1016/j.jphotobiol.2016.10.003
Hossain, A., Hong, X., Ibrahim, E., Li, B., Sun, G., Meng, Y., et al. (2019). Green synthesis of silver nanoparticles with culture supernatant of a bacterium Pseudomonas rhodesiae and their antibacterial activity against soft rot pathogen Dickeya dadantii. Molecules 24, 2303. doi: 10.3390/MOLECULES24122303
Ihtisham, M., Noori, A., Yadav, S., Sarraf, M., Kumari, P., Brestic, M., et al. (2021). Silver nanoparticle's toxicological effects and phytoremediation. Nanomater (Basel) 11 (9), 2164. doi: 10.3390/nano11092164
Irshad, S., Salamat, A., Anjum, A. A., Sana, S., Saleem, R. S., Naheed, A., et al. (2018). Green tea leaves mediated ZnO nanoparticles and its antimicrobial activity. Cogent Chem. 4, 1469207. doi: 10.1080/23312009.2018.1469207
Jeffery, G. A. (1957). Elements of x-ray diffraction (Cullity, B. D.). J. Chem. Educ. 34, A178. doi: 10.1021/ED034PA178
Jeyaraj Pandian, C., Palanivel, R., Dhanasekaran, S. (2016). Screening antimicrobial activity of nickel nanoparticles synthesized using Ocimum sanctum leaf extract. J. Nanoparticles 2016, 1–13. doi: 10.1155/2016/4694367
Khan, M. R., Rizvi, T. F. (2014). Nanotechnology: Scope and application in plant disease management. Plant Pathol. J. 13, 214–231. doi: 10.3923/PPJ.2014.214.231
Kumar, A., Pandey, A. K., Singh, S. S., Shanker, R., Dhawan, A. (2011). A flow cytometric method to assess nanoparticle uptake in bacteria. Cytometry A. 79, 707–712. doi: 10.1002/CYTO.A.21085
Lajevardi, S. A., Shahrabi, T., Szpunar, J. A. (2013). Synthesis of functionally graded nano Al2O3–Ni composite coating by pulse electrodeposition. Appl. Surf Sci. 279, 180–188. doi: 10.1016/J.APSUSC.2013.04.067
Lattuada, M., Hatton, T. A. (2011). Synthesis, properties and applications of janus nanoparticles. Nano Today 6, 286–308. doi: 10.1016/J.NANTOD.2011.04.008
Lee, J. H., Kim, Y. G., Cho, M. H., Lee, J. (2014). ZnO nanoparticles inhibit Pseudomonas aeruginosa biofilm formation and virulence factor production. Microbiol. Res. 169, 888–896. doi: 10.1016/J.MICRES.2014.05.005
Le Ouay, B., Stellacci, F. (2015). Antibacterial activity of silver nanoparticles: A surface science insight. Nano Today 10, 339–354. doi: 10.1016/J.NANTOD.2015.04.002
Liou, T. H. (2004). Preparation and characterization of nano-structured silica from rice husk. Mater Sci. Eng. A. 364, 313–323. doi: 10.1016/J.MSEA.2003.08.045
Ma, C., Han, L., Shang, H., Hao, Y., Xu, X., White, J. C., et al. (2023). Nanomaterials in agricultural soils: Ecotoxicity and application. Curr. Opin. Environ. Sci. Heal. 31, 100432. doi: 10.1016/J.COESH.2022.100432
Majewski, A. J., Wood, J., Bujalski, W. (2013). Nickel–silica core@shell catalyst for methane reforming. Int. J. Hydrogen Energy. 38, 14531–14541. doi: 10.1016/J.IJHYDENE.2013.09.017
Markowicz, A. (2023). The significance of metallic nanoparticles in the emerging, development and spread of antibiotic resistance. Sci. Total Environ. 871, 162029. doi: 10.1016/J.SCITOTENV.2023.162029
Marques, E., Uesugi, C. H., Ferreira, M. A. V. (2009). Sensitivity to copper in Xanthomonas campestris pv. viticola. Trop. Plant Pathol. 34, 406–411. doi: 10.1590/S1982-56762009000600007
Melo, N. F. C. B., de Mendonca Soares, B. L., Diniz, K. M., Leal, C. F., Canto, D., Flores, M. A. P., et al. (2018). Effects of fungal chitosan nanoparticles as eco-friendly edible coatings on the quality of postharvest table grapes. Postharvest Biol. Technol. 139, 56–66. doi: 10.1016/j.postharvbio.2018.01.014
Merritt, J. H., Kadouri, D. E., O’Toole, G. A. (2005). Growing and analyzing static biofilms. Curr. Protoc. Microbiol. Chapter 1. 00: 1B.1.1-1B.1.17. doi: 10.1002/9780471729259.MC01B01S00
Mirhosseini, M., Hafshejani, B. H., Dashtestani, F., Hakimian, F., Haghirosadat, B. F. (2018). Antibacterial activity of nickel and nickel hydroxide nanoparticles against multidrug resistance K. pneumonia and E. coli isolated urinary tract. Nanomed. J. 5, 19–26. doi: 10.22038/NMJ.2018.05.004
Mirzajani, F., Askari, H., Hamzelou, S., Schober, Y., Rompp, A., Ghassempour, A., et al. (2014). Proteomics study of silver nanoparticles toxicity on Oryza sativa L. Ecotoxicol Environ. Saf. 108, 335–339. doi: 10.1016/J.ECOENV.2014.07.013
Monteiro, D. R., Silva, S., Negri, M., Gorup, L. F., De Camargo, E. R., Oliveira, R., et al. (2013). Antifungal activity of silver nanoparticles in combination with nystatin and chlorhexidine digluconate against Candida albicans and Candida glabrata biofilms. Mycoses 56, 672–680. doi: 10.1111/MYC.12093
Namburi, K. R., Kora, A. J., Chetukuri, A., Kota., V. S. M. K. (2021). Biogenic silver nanoparticles as an antibacterial agent against bacterial leaf blight causing rice phytopathogen Xanthomonas oryzae pv. oryzae. Bioprocess Biosyst. Eng. 44, 1975–1988. doi: 10.1007/s00449-021-02579-7
Ninganagouda, S., Rathod, V., Singh, D., Hiremath, J., Singh, A. K., Mathew, J., et al. (2014). Growth kinetics and mechanistic action of reactive oxygen species released by silver nanoparticles from Aspergillus niger on Escherichia coli. BioMed. Res. Int. 753419, 1–9. doi: 10.1155/2014/753419
Ogunyemi, S. O., Abdallah, Y., Ibrahim, E., Zhang, Y., Bi, J., Wang, F., et al. (2023). Bacteriophage-mediated biosynthesis of MnO2NPs and MgONPs and their role in the protection of plants from bacterial pathogens. Front. Microbiol. 14. doi: 10.3389/fmicb.2023.1193206
Ogunyemi, S. O., Abdallah, Y., Zhang, M., Fouad, H., Hong, X., Ibrahim, E., et al. (2019). Green synthesis of zinc oxide nanoparticles using different plant extracts and their antibacterial activity against Xanthomonas oryzae pv. oryzae. Artif. cells nanomed. Biotechnol. 47, 341–352. doi: 10.1080/21691401.2018.1557671
Omanović-Mikličanin, E., Badnjević, A., Kazlagić, A., Hajlovac, M. (2020). Nanocomposites: A brief review. Health Technol. (Berl). 10, 51–59. doi: 10.1007/S12553-019-00380
Pagar, K., Chavan, K., Kasav, S., Basnet, P., Rahdar, A., Kataria, N., et al. (2023). Bio-inspired synthesis of CdO nanoparticles using Citrus limetta peel extract and their diverse biomedical applications. J. Drug Delivery Sci. Technol. 82, 104373. doi: 10.1016/J.JDDST.2023.104373
Pellieux, C., Dewilde, A., Pierlot, C., Aubry, J. M. (2000). Bactericidal and virucidal activities of singlet oxygen generated by thermolysis of naphthalene endoperoxides. Methods Enzymol. 319, 197–207. doi: 10.1016/S0076-6879(00)19020-2
Pereira, D., Carreira, T. S., Alves, N., Sousa, A., Valente, J. F. A. (2022). Metallic structures: Effective agents to fight pathogenic microorganisms. Int. J. Mol. Sci. 23, 1165. doi: 10.3390/IJMS23031165
Petousis, M., Tzounis, L., Papageorgiou, D., Vidakis, N. (2020). Decoration of SiO2 and Fe3O4 nanoparticles onto the surface of MWCNT-Grafted glass fibers: A simple approach for the creation of binary nanoparticle hierarchical and multifunctional composite interphases. Nanomater 10, 2500. doi: 10.3390/NANO10122500
Philip, D. (2010). Green synthesis of gold and silver nanoparticles using Hibiscus rosa sinensis. Phys. E Low-dimens Syst. Nanostruct. 42, 1417–1424. doi: 10.1016/J.PHYSE.2009.11.081
Piao, M. J., Kang, K. A., Lee, I. K., Kim, H. S., Kim, S., Choi, J. Y., et al. (2011). Silver nanoparticles induce oxidative cell damage in human liver cells through inhibition of reduced glutathione and induction of mitochondria-involved apoptosis. Toxicol. Lett. 201, 92–100. doi: 10.1016/J.TOXLET.2010.12.010
Prakasham, R. S., Devi, G. S., Rao, C. S., Sivakumar, V. S. S., Sathish, T., Sarma, P. N. (2010). Nickel-impregnated silica nanoparticle synthesis and their evaluation for biocatalyst immobilization. Appl. Biochem. Biotechnol. 160, 1888–1895. doi: 10.1007/S12010-009-8726-5
Rudramurthy, G. R., Swamy, M. K., Sinniah, U. R., Ghasemzadeh, A. (2016). Nanoparticles: alternatives against drug-resistant pathogenic microbes. Molecules 21, 836. doi: 10.3390/MOLECULES21070836
Russo, N. L., Burr, T. J., Breth, D. I., Aldwinckle, H. S. (2008). Isolation of streptomycin-resistant isolates of Erwinia amylovora in New York. Plant Dis. 92, 714–718. doi: 10.1094/PDIS-92-5-0714
Saha, M., Mukherjee, S., Gayen, A., Mukherjee, S. (2015). Structural, optical and magnetic properties of nickel–silica nanocomposite prepared by a sol–gel route. J. Inst Eng. Ser. D. 96, 169–177. doi: 10.1007/S40033-014-0062-4/FIGURES/11
Sathishkumar, M., Sneha, K., Won, S. W., Cho, C. W., Kim, S., Yun, Y. S. (2009). Cinnamon zeylanicum bark extract and powder mediated green synthesis of nano-crystalline silver particles and its bactericidal activity. Colloids Surf B Biointerfaces. 73, 332–338. doi: 10.1016/J.COLSURFB.2009.06.005
Shanti, M. L., Shenoy, V., Devi, G. L., Kumar, V. M., Premalatha, P., Kumar, G. N., et al. (2010). Marker-assisted breeding for resistance to bacterial leaf blight in popular cultivar and parental lines of hybrid rice. J. Plant Pathol. 92(2):495–501. doi: 10.4454/JPP.V92I2.194
Shwetha, U. R., Rajith Kumar., C. R., Kiran, M. S., Betageri, V. S., MS, L., Veerapur, R., et al. (2021). Biogenic synthesis of NiO nanoparticles using Areca catechu leaf extract and their antidiabetic and cytotoxic effects. Molecules 26, 2448. doi: 10.3390/MOLECULES26092448
Siddiqui, M. J., Saleh, M. S. M., Basharuddin, S. N. B. B., Zamri, S. H. B., Najib, M. H. B. M., Ibrahim, M. Z. B. C., et al. (2018). Saffron (Crocus sativus L.): As an antidepressant. J. Pharm. Bioallied Sci. 10, 173. doi: 10.4103/JPBS.JPBS_83_18
Singh, S., Chand, S., Singh, N. K., Sharma, T. R. (2015). Genome-wide distribution, organisation and functional characterization of disease resistance and defence response genes across rice species. PloS One 10, e0125964. doi: 10.1371/JOURNAL.PONE.0125964
Singh, A., Singh, N. B., Hussain, I., Singh, H., Yadav, V., Singh, S. C. (2016). Green synthesis of nano zinc oxide and evaluation of its impact on germination and metabolic activity of Solanum lycopersicum. J. Biotechnol. 233, 84–94. doi: 10.1016/J.JBIOTEC.2016.07.010
Sudhasree, S., Shakila Banu, A., Brindha, P., Kurian, G. A. (2014). Synthesis of nickel nanoparticles by chemical and green route and their comparison in respect to biological effect and toxicity. Toxicol. Environ. Chem. 96, 743–754. doi: 10.1080/02772248.2014.923148
Syu, Y. Y., Hung, J. H., Chen, J. C., Chuang, H. W. (2014). Impacts of size and shape of silver nanoparticles on Arabidopsis plant growth and gene expression. Plant Physiol. Biochem. PPB. 83, 57–64. doi: 10.1016/J.PLAPHY.2014.07.010
Tao, F., Grass, M. E., Zhang, Y., Butcher, D. R., Renzas, J. R., Liu, Z., et al. (2008). Reaction-driven restructuring of Rh-Pd and Pt-Pd core-shell nanoparticles. Sci 322, 932–934. doi: 10.1126/SCIENCE.1164170
Tarafdar, J. C., Raliya, R., Mahawar, H., Rathore, I. (2014). Development of zinc nanofertilizer to enhance crop production in pearl millet (Pennisetum americanum). Agric. Res. 3, 257–262. doi: 10.1007/S40003-014-0113-Y/TABLES/4
Wang, Y., Zhou, Y., Liu, K., Wang, N., Wu, Y., Zhang, C., et al. (2023). Transcriptome-based comparative analysis of transcription factors in response to NaCl, NaOH, and Na2CO3 stresses in roots of autotetraploid rice (Oryza sativa L.). Agronomy 13, 959. doi: 10.3390/AGRONOMY13040959
Wiegand, I., Hilpert, K., Hancock, R. E. W. (2008). Agar and broth dilution methods to determine the minimal inhibitory concentration (MIC) of antimicrobial substances. Nat. Protoc. 3, 163–175. doi: 10.1038/nprot.2007.521
Xu, M., Mao, L., Du, W., Guo, H., Yin, Y. (2021). Divergence in response of japonica and hybrid rice to titanium dioxide nanoparticles. J. Soils Sediments 21, 1688–1697. doi: 10.1007/s11368-021-02890-6
Xu, Y., Zhu, X. F., Zhou, M. G., Kuang, J., Zhang, Y., Shang, Y., et al. (2010). Status of streptomycin resistance development in Xanthomonas oryzae pv. oryzae and Xanthomonas oryzae pv. oryzicola in China and their resistance characters. J. \Phytopathol. 158, 601–608. doi: 10.1111/J.1439-0434.2009.01657.X
Yasmin, S., Hafeez, F. Y., Mirza, M. S., Rasul, M., Arshad, H. M. I., Zubair, M., et al. (2017). Biocontrol of bacterial leaf blight of rice and profiling of secondary metabolites produced by rhizospheric Pseudomonas aeruginosa BRp3. Front. Microbiol. 8. doi: 10.3389/FMICB.2017.01895
Zafar, H., Ali, A., Ali, J. S., Haq, I. U., Zia, M. (2016). Effect of ZnO nanoparticles on Brassica nigra seedlings and stem explants: Growth dynamics and antioxidative response. Front. Plant Sci. 7. doi: 10.3389/FPLS.2016.00535/BIBTEX
Zein El-Abdeen, A., Farroh, Y. (2019). Preparation and characterization of nano organic soil conditioners and it’s effected on sandy soil properties and wheat productivity. Nat. Sci. 17, 115–128. doi: 10.7537/marsnsj170219.13
Zhang, L., Wu, L., Si, Y., Shu, K. (2018). Size-dependent cytotoxicity of silver nanoparticles to Azotobacter vinelandii: Growth inhibition, cell injury, oxidative stress and internalization. PloS One 13, e0209020. doi: 10.1371/JOURNAL.PONE.0209020
Zhu, X., Chen, S., Luo, G., Zheng, W., Tian, Y., Lei, X., et al. (2022). A novel algicidal bacterium, Microbulbifer sp. YX04, triggered oxidative damage and autophagic cell death in Phaeocystis globosa, which causes harmful algal blooms. Microbiol. Spectr. 10, e0093421. doi: 10.1128/SPECTRUM.00934-21/SUPPL_FILE/SPECTRUM00934-21_SUPP_1_SEQ1.PDF
Keywords: biosynthesis, nanoparticle composites, rice bacterial leaf blight, Xanthomonas oryzae pv. oryzae, biofilm
Citation: Abdallah Y, Nehela Y, Ogunyemi SO, Ijaz M, Ahmed T, Elashmony R, Alkhalifah DHM, Hozzein WN, Xu L, Yan C, Chen J and Li B (2023) Bio-functionalized nickel-silica nanoparticles suppress bacterial leaf blight disease in rice (Oryza sativa L.). Front. Plant Sci. 14:1216782. doi: 10.3389/fpls.2023.1216782
Received: 04 May 2023; Accepted: 12 July 2023;
Published: 02 August 2023.
Edited by:
Abdelwaheb Chatti, University of Carthage, TunisiaReviewed by:
Saurabh Yadav, Hemwati Nandan Bahuguna Garhwal University, IndiaSeungmin Son, Rural Development Administration, Republic of Korea
Wei Yan, Nanjing Agricultural University, China
Copyright © 2023 Abdallah, Nehela, Ogunyemi, Ijaz, Ahmed, Elashmony, Alkhalifah, Hozzein, Xu, Yan, Chen and Li. This is an open-access article distributed under the terms of the Creative Commons Attribution License (CC BY). The use, distribution or reproduction in other forums is permitted, provided the original author(s) and the copyright owner(s) are credited and that the original publication in this journal is cited, in accordance with accepted academic practice. No use, distribution or reproduction is permitted which does not comply with these terms.
*Correspondence: Chengqi Yan, eWFuY2hlbmdxaUAxNjMuY29t; Bin Li, bGliaW4wNTcxQHpqdS5lZHUuY24=