- 1Irrigated Agriculture Research and Extension Center, Washington State University, Prosser, WA, United States
- 2Global Pathology Support Platform, HM Clause Seed Company, Davis, CA, United States
- 3Temperate Tree Fruit and Vegetable Research Unit, United States Department of Agriculture Agricultural Research Service (USDA-ARS), Prosser, WA, United States
- 4Tropical Agriculture Research Station, United States Department of Agriculture Agricultural Research Service (USDA-ARS), Mayagüez, Puerto Rico
- 5Soybean Genomics and Improvement Laboratory, United States Department of Agriculture Agricultural Research Service (USDA-ARS), Beltsville, MD, United States
- 6Northwest Irrigation and Soils Research Laboratory, United States Department of Agriculture Agricultural Research Service (USDA-ARS), Kimberly, ID, United States
- 7Grain Legume Genetics and Physiology Research Unit, United States Department of Agriculture Agricultural Research Service (USDA-ARS), Prosser, WA, United States
Beet curly top virus (BCTV), which is synonymous with curly top virus (CTV), causes significant yield loss in common bean (snap and dry beans) cultivars and several other important crops. Common bean cultivars have been found to be resistant to CTV, but screening for resistance is challenging due to the cyclical nature of epidemics and spotty feeding by the leafhopper that vectors the virus. We used an SNP dataset for the Snap Bean Association Panel (SnAP) agro-inoculated with CTV-Logan (CA/Logan) strain to locate the Bct gene region to a 1.7-Mb interval on chromosome Pv07 using genome-wide association study (GWAS) analysis. Recombinant lines from the SnAP were used to further narrow the Bct region to a 58.0-kb interval. A missense SNP (S07_2970381) in candidate gene Phvul.007G036300 Exonuclease V (EXO5) was identified as the most likely causal mutation, and it was the most significant SNP detected by GWAS in a dry bean population (DBP) naturally infected by the CTV-Worland (Wor) strain. Tm-shift assay markers developed for SNP S07_2970381 and two linked SNPs, S07_2970276 and S07_2966197, were useful for tracking different origins of the Bct EXO5 candidate gene resistance to CTV in common bean. The three SNPs identified four haplotypes, with haplotype 3-1 (Haplo3-1) of Middle American origin associated with the highest levels of CTV resistance. This SNP-haplotype assay will enable breeders to track resistance sources and to develop cultivars with better CTV resistance.
Introduction
Beet curly top virus (BCTV) can cause severe yield loss in susceptible cultivars of snap and dry beans (collectively known as common bean, Phaseolus vulgaris L.) and affects many other crops, including sugar beet (Beta vulgaris L.), pepper (Capsicum annuum L.), and tomato (Solanum lycopersicum L.) (Creamer, 2020). BCTV is endemic to the semi-arid regions of the western US, which are of paramount importance for producing high-quality and disease-free snap and dry bean seeds. BCTV is a single-stranded DNA virus of the genus Curtovirus within the family Geminiviridae and is transmitted in a persistent manner by the beet leafhopper (Circulifer tenellus B.). Among the 11 strains of BCTV described in the literature, Colorado (CO) and Worland (Wor) have been two of the more commonly isolated strains from plants and beet leafhoppers in recent studies in the western US. In contrast, the California/Logan (CA/Logan) strain has recently only been found in Idaho (Rondon et al., 2016; Strausbaugh et al., 2017; Giladi et al., 2020; Chiginsky et al., 2021; Hu et al., 2021; Swisher Grimm et al., 2021; Melgarejo et al., 2022; Rivedal et al., 2022) and has been distinct. The CA/Logan strain was the first to be collected and sequenced, while the CO and Wor strains have been described and established more recently (Stenger et al., 1990; Hormuzdi and Bisaro, 1993; Varsani et al., 2014; Strausbaugh et al., 2017). Endemics are cyclical in nature, with severity dependent on climatic events and their timing, which favor spring hatches of the beet leafhopper vector from weed host plants infected by BCTV, such as wild mustard (Sinapis arvensis L.), Russian thistle (Salsola tragus L.), and kochia (Kochia scoparia L. Schrad.) (Bennett, 1971).
BCTV [synonymous with curly top virus (CTV)] causes symptoms in common bean, including leaf curling, chlorosis, and severe plant stunting (Silbernagel and Morales, 2005). Early seedling infection of susceptible plants causes significant crop loss as seedlings typically wither and die. The reduced plant stands from early CTV infection mimic root rotting problems. Symptoms are generally less severe in susceptible bean plants infected at later growth stages and in plants with partial resistance, although seed yields may be significantly reduced. Seed treatments containing systemic insecticides provide good protection during the early seedling growth stages, but the most effective control is obtained by deploying natural genetic resistance in the host (Singh and Schwartz, 2010). The most effective and widely deployed resistance in common bean is conditioned by the major dominant Bct allele identified by Larsen and Miklas (2004) on chromosome Pv07 in a snap bean RIL population. Furthermore, the Bct locus, derived from the landrace ‘Jatu Rong’ (G122), was associated with a minor-effect quantitative trait locus (QTL) conferring resistance against CTV in the G122/’Taylor Horticulture’ dry bean RIL population (Larsen et al., 2010). The same study identified a major-effect QTL on Pv06 for CTV resistance. The Bct region was also associated with a major quantitative resistance effect against bean dwarf mosaic virus (BDMV) (Miklas et al., 2009).
Strategic implementation of host resistance in common bean has become a common practice for mitigating CTV risk to commercial seed production west of the US Continental Divide. Unfortunately, the selection for resistance, including that conditioned by the Bct allele, is difficult because field-based screening is unreliable due to the cyclical nature of epidemics. Additionally, there is a lack of uniform disease pressure during an epidemic because of spotty feeding by the leafhopper, as common bean is a non-preferred food source (Munyaneza and Upton, 2005), and BCTV strains can vary over time (Strausbaugh et al., 2017). Moreover, the virus is not mechanically transmissible, greatly complicating high throughput greenhouse testing. These constraints to screening for resistance to CTV clearly favor marker-assisted selection (MAS) as the most effective and efficient selection method.
Larsen and Miklas (2004) identified a sequence-characterized amplified region (SCAR) marker linked with Bct gene. This marker, known as ‘SAS8.1550’, has led to greater accessibility and increased deployment of Bct. However, the SCAR ‘SAS8.1550’, based on a polymorphism tightly linked to Bct, is subject to potential recombination events that can lead to false positives (selected plants carrying the marker allele for resistance but expressing a susceptibility phenotype) or false negatives (discarding plants that carry the marker allele for susceptibility but express a resistant phenotype). In addition, it was demonstrated that ‘SAS8.1550’ had reduced diagnostic value in snap bean genotypes derived primarily from the Middle American gene pool (Larsen and Miklas, 2004), which includes a large complement of economically important germplasm.
Our research objectives aimed to i) acquire a comprehensive understanding of the genetic basis of the Bct allele and ii) enhance the efficiency and reliability of MAS for Bct in snap and dry bean germplasm.
Materials and methods
Plant materials
The Snap Bean Association Panel (SnAP) is comprised of 378 historic-to-modern cultivars that span both fresh market and processing types, including large sieve, small sieve, wax, bush blue lake, Kentucky, Romano, pole, and others. The SnAP includes public and private industry-released snap bean cultivars predominantly from the United States, some landrace cultivars, and a few breeding lines. The SnAP has recently been used for Genome-Wide Association Studies (GWAS) of herbicide tolerance (Saballos et al., 2022) and resistance to white mold (Arkwazee et al., 2022). The SnAP expands on the 149 snap bean accessions for the Snap Bean Diversity Panel (SBDP) that composed part of the BeanCAP (Common Bean Coordinated Agricultural Project) panel, which was comprised of both dry and snap beans (http://arsftfbean.uprm.edu/beancap/). The SBDP was used in a diversity analysis of snap bean (Wallace et al., 2018) and for GWAS of total phenolic content and root rot resistance (Myers et al., 2019; Huster et al., 2021). In addition to the SnAP, a dry bean population (DBP) comprised of 88 advanced inbred breeding lines and cultivars representing different market classes was included from the USDA-ARS bean breeding program in Prosser, WA.
Phenotypic evaluations
Disease resistance or susceptibility reactions to CTV were obtained for 83 of the SnAP accessions from published information, including from the Agricultural Marketing Service-USDA scanned Plant Variety Protection (PVP) database, cultivar registration articles, and the Germplasm Resources Information Network (https://npgsweb.ars-grin.gov/gringlobal/search). An additional 289 SnAP accessions were phenotyped for CTV disease reaction using an infectious CA/Logan clone of CTV and an agro-inoculation screening procedure in a controlled growth room environment at Harris Moran Seed Company in Sun Prairie, Wisconsin. The CTV CA/Logan strain was cloned into the binary vector pBIN19 in Agrobacterium tumefaciens strain GV3111 (Ti plasmid pTiB6S3SE) obtained originally from Dr. Drake Stenger. This unpublished infectious clone had increased infectivity for agro-inoculation of common bean compared to earlier-developed clones (Stenger et al., 1990; Stenger et al., 1992).
For each of the 289 accessions, two pots with a diameter of 15 cm were filled with MetroMix-510 growing medium (Premier Horticulture, Inc., Red Hill, PA), and each pot contained two plants. The plants were covered and placed in a dark growth chamber for 24 h after inoculation. After 24 h, they were maintained in a growth chamber with a 14-/10-h light cycle and a temperature range of 18°C–27°C. The seedlings were transplanted into 1-gallon pots (two plants/pot) 2–6 days post-inoculation. The accessions were tested in subsets of approximately 30 accessions, in 4-day intervals, in a growth room. Each subset included two pots with two plants, each of the susceptible ‘Caprice’ and resistant ‘Hystyle’ cultivars as controls. Six accessions did not germinate, including ‘Angers’, ‘Processor’, ‘RH13’, ‘Serin’, ‘Golden Rod’, and ‘Sundial’. Disease reactions were recorded 17–24 days post-inoculation (dpi) as resistant (no visible symptoms), susceptible (with assorted symptoms: leaf rugosity, leaf cupping, leaf curling, plant stunting), or intermediate resistant (with fewer and less severe symptoms) (Larsen et al., 2010).
The DBP accessions were sown during the summer of 2022 in two replications at the Washington State University (WSU) Research Station—Roza Unit (46°17′43.6ʺN 119°43′48.0ʺW) in Prosser, WA, USA, where CTV is endemic. The experimental design was a randomized complete block, and each plot consisted of four rows of 3-m row length, with a spacing of 0.56 m between rows. To determine the CTV strain(s) causing infection in the trial, leaf tissue was collected from 1 asymptomatic plant and 16 symptomatic plants during the early pod-fill R3 growth stage. The tissue was extracted using a modified Dellaporta protocol (Crosslin et al., 2006) and analyzed by conventional PCR using the strain-specific primers previously described in Strausbaugh et al. (2008) to detect three strains of CTV, namely, the CFH strain [also referred to as beet severe curly top virus (BSCTV)], Wor strain [beet mild curly top virus (BMCTV)], and CA/Logan strain. All PCR reactions consisted of 5 µl of 5× GoTaq Green PCR buffer (Promega Corp, USA), 0.25 µl 10 mM dNTP (each), 0.5 µl of 20 µM forward and reverse primers, 17.65 µl H2O, 1 µl of total nucleic acid extract, and 0.1 µl GoTaq DNA polymerase (Promega Corp., USA). Thermal cycler conditions consisted of 95°C for 2 min, followed by 40 cycles of 95°C for 1 min, 54°C for 1 min, and 72°C for 1 min, and a final extension step of 72°C for 5 min. Amplicons were visualized on a 1.5% agarose gel with ethidium bromide staining.
Disease reaction was rated 60 days after planting on a scale from 1 to 9, where 1 = no symptoms and 0% of plants infected, 5 = moderate disease symptoms and up to 30% of plants infected, and 9 = severe disease symptoms with ≥80% of the plants dead or dying (Larsen and Miklas, 2004). Trait data were spatially corrected, and best linear unbiased predictors (BLUPs) were calculated using a two-dimensional P-spline mixed model with the Mr.Bean web application (Aparicio Arce, 2018) and the R package SpATS v1.0-11 (Rodríguez-Álvarez et al., 2018).
Genotyping
DNA was extracted from each SnAP accession using the Qiagen Plant DNeasy Mini Kit (Hilden, Germany) and diluted to 10 ng/μl with nuclease-free water. Multiplexed genotyping-by-sequencing (GBS) libraries were generated through digestion with ApeKI following the protocol and with barcodes detailed in Elshire et al. (2011). Single-end sequencing of multiplex GBS libraries was performed using the Illumina HiSeq2000 (Illumina, San Diego, CA, USA). The barcodes of raw Illumina sequence were trimmed, and reads were aligned to the reference Andean G19833 Phaseolus vulgaris v2.1 genome sequence (https://phytozome-next.jgi.doe.gov/info/Pvulgaris_v2_1) using Bowtie2 v2.4.1 (Langmead and Salzberg, 2012). The alignments in BAM format were sorted by SAMtools v1.9 (Li et al., 2009).
SNP discovery and genotype calling were conducted using NGSEP v3.3.0 (Next Generation Sequencing Experience Platform) pipeline (Perea et al., 2016) with G19833 Phaseolus vulgaris v2.1 as reference genome (Schmutz et al., 2014). Maximum base quality score was set to 30, and the quality minimum was set to 40 for reporting a variant. All SNP markers detected with <40% missing values, and a minor allele frequency (MAF) of 0.01 were retained to perform imputation with the ImputeVCF module in NGSEP, which is a reimplementation of the Hidden Markov Model (HMM) implemented in the package fastPHASE (Scheet et al., 2006). Annotation of variants was performed using the command Annotate by NGSEP.
For the DBP accessions, the leaf tissue was collected from individual plants grown in the USDA-ARS greenhouses at Prosser, WA. Genomic DNA was isolated from 20 mg of the leaf tissue using a Qiagen DNeasy 96 Plant Kit (Hilden, Germany) and sent to the US Department of Agriculture Agricultural Research Service (USDA-ARS), Soybean Genetics and Improvement Laboratory, Beltsville, MD, for the 11,292 SNPs BARC Illumina chip assay (Miklas et al., 2020). The SNP genotyping was conducted on the Illumina platform following the Infinium HD Assay Ultra protocol (Illumina Inc., San Diego, CA) (Song et al., 2015). The SNP positions were updated by alignment to the v2.1 reference genome assembly of G19833 and were filtered based on a minimum allele frequency (MAF) of 0.01 and a missing data rate of <20%.
Population genetic analysis
The SNP data sets used for population structure analysis in SnAP and DBP were adjusted by linkage disequilibrium (LD) pruning using a 0.5 r2 threshold and the –indep-pairwise function in Plink v2.0 (https://www.cog-genomics.org/plink/2.0/), with a sliding window of 50 kb shifting by five bases. In addition, two dry bean lines, ‘BAT 93’ (representing the Middle American gene pool) and ‘Jalo EPP 558’ (representing the Andean gene pool), were added to assess the structure and diversity in SnAP. The sequence data for ‘BAT 93’ and ‘Jalo EPP 558’ was obtained by GBS, as described above.
The pruned set of SNPs with <20% missing values and a MAF > 0.01 were retained. Population structure was estimated using a Bayesian Markov chain Monte Carlo model (MCMC) implemented in STRUCTURE 2.3 software (Pritchard et al., 2000). Ten runs (k) were performed for each number of populations set from 1 to 10. Burn-in time and MCMC replication number were set to 100,000 and 200,000, respectively. The most likely number of populations (K) in the SnAP was calculated with the Evanno method (Evanno et al., 2005) using Pophelper v2.3.1 (Francis, 2017). Finally, the merge Q-matrix was obtained using the CLUMPP v1.1 software (Jakobsson and Rosenberg, 2007) to determine the robustness of the population assignments at each K.
GWAS
GWAS analysis was conducted by mixed linear model (MLM) using the genome association and prediction integrated tool (GAPIT) (Lipka et al., 2012). An identity-by-state kinship matrix was created using the Efficient Mixed Model Association (EMMA) algorithm implemented in GAPIT R package with corrections for kinship and population structure. Five principal components (PCs) generated from GAPIT were included as covariates, and the Bonferroni procedure was applied to control the experiment-wise type I error rate at 0.05. The GWAS results were visualized using CMPlot v3.62 (Yin, 2016) and IntAssoPlot v3 (He et al., 2020) for a graphical representation of regional-based marker–trait associations.
Candidate gene identification
Candidate genes annotated within the Bct region were identified using the gene models from G19833 v2.1 common bean reference genome. Selected candidate genes were amplified and sequenced in a subset of 14 snap and 14 dry bean genotypes from Andean and Middle American gene pools.
A set of primers were designed to obtain full-length candidate genes using Primer3 software (Koressaar and Remm, 2007) (http://bioinfo.ut.ee/primer3-0.4.0/). The PCR volume was 25 µl, containing 1× Taq buffer, 1.8 mM of MgCl2, 0.4 mM of dNTPs mix (Promega®, Madison, WI), 0.25 µM of each primer (forward and reverse), 1 U of Taq polymerase (Promega®, Madison, WI), and 25 ng of genomic DNA. The amplification conditions were 2 min at 95°C, followed by 38 cycles at 94°C for 20 s, 57°C for 30 s, and 72°C for 90 s, and a final extension at 72°C for 5 min, performed in a PCR Eppendorf Mastercycler (Eppendorf AG, Hamburg, Germany). PCR products were visualized by gel electrophoresis on 2% (w/v) agarose. Sanger sequencing from PCR products obtained was performed using the services of Eurofins MWG Operon (Louisville, KY, USA). Sequencing data were analyzed using Geneious 9.1.8 (Kearse et al., 2012), which was used for sequence trimming, alignment, and variant detection.
Development of single nucleotide polymorphism markers in the Bct region
A set of five SNPs and one InDel marker were developed from a variants catalog obtained from Lobaton et al. (2018) and used to refine the Bct interval identified by GWAS in SnAP (Supplementary Table S1). From the refined interval, three nonsense variants (S07_2966197, S07_ 2970276, and S07_ 2970381) were selected in candidate gene sequences based on polymorphism among 14 snap and 14 dry beans genotypes and converted to markers using the allele-specific method (Wang et al., 2005). These three variants (S07_2966197, S07_ 2970276, and S07_ 2970381), and the original ‘SAS8.1550’ SCAR marker, using the protocol described by Larsen and Miklas (2004), were assayed in the SnAP and DBP. Lastly, three additional SNP markers (Pv07_3044767, Pv07_331344, and Pv07_3644749), identified by Ariza-Suarez et al. (2023) to be linked with resistance to bean leaf crumple virus (BLCrV) in proximity to the Bct region, were converted to Tm-shift assays for genotyping the DBP (Table S1). BLCrV is a single-stranded DNA Begomovirus in family Geminiviridae.
Tm-shift SNP markers were amplified by PCR on an Eppendorf Mastercycler (Eppendorf AG, Hamburg, Germany). The PCR volume was 20 μl containing 20 ng of genomic DNA, 1× Taq buffer, 1.5 mM of MgCl2, 0.2 mM of dNTPs mix (Promega), 0.15 μM of each primer (two allele-specific forward primers and the common reverse primer), 1× EvaGreen® dye, and 0.1 μl of Taq polymerase (Promega) under the following thermal profile: 94°C for 2 min, then 38 cycles of denaturation at 92°C for 20 s, annealing for 20 s (the temperature was specific to each primer trio), and extension at 72°C for 20 s, and final extension at 72°C for 5 min. Melting point analysis for allele determination of the PCR products was performed with a fluorescence-detecting thermocycler (LightCycler® 4890 Instrument II, Roche, Basal Switzerland). EvaGreen Fluorescent detection was performed for 1 min at 95°C, and the melting curve step ramped from 65°C to 95°C in increments of 1°C every 20 s.
Identification of haplotypes in Bct candidate gene in DBP accessions
The S07_2966197, S07_ 2970276, and S07_ 2970381 markers were used to identify haplotypes correlated with quantitative field reaction to CTV in the DBP. An analysis of variance between haplotypes was carried out by Ftrimmed-means one-way ANOVA using the ggbetweenstats function in the R-Package ggstatsplot. Yuen’s trimmed means test was implemented using Robust p-value fitting method using the ggbetweenstats function in the R-Package ggstatsplot (Patil, 2021). Overall, the Ftrimmed-means method provides a robust approach for analyzing data that may contain outliers and can help identify statistically significant differences between groups.
Results
Curly top virus screening
Out of the 289 SnAP accessions screened by agro-inoculation with the CTV CA/Logan strain, 166 accessions were resistant, 66 were susceptible, 4 showed intermediate resistance, and 53 were segregating with plants exhibiting either resistant or susceptible (20 accessions); resistant or intermediate resistance (18 accessions); or resistant, intermediate resistant, or susceptible responses (15 accessions). The disease reactions for 83 SnAP accessions, compiled from various public information sources, were categorized as 42 resistant, 32 susceptible, and 9 intermediate resistant to CTV. For upstream analysis, the qualitative reactions of each accession were transformed into a quantitative score, with resistant being coded as 1, susceptible as 0, and intermediate resistant as 2 (Supplementary Table S2).
In addition, 88 DBP accessions were evaluated for CTV reaction (scored from 1 to 9) under natural field infection. Based on the BLUP values for CTV reaction, 43 accessions were resistant (disease score between 1 to 4), 33 were tolerant (between 4.1 to 6.9), and 14 were susceptible (between 7 to 9) to the Wor strain 60 days after planting (Supplementary Table S3). PCR analysis with strain-specific primers detected the Wor strain in leaf tissue collected from this naturally infected field. Specifically, one asymptomatic plant and 11 of 16 symptomatic plants amplified a product of the expected size (397 bp) with the Wor strain primers.
Population structure
According to STRUCTURE analysis of the SnAP (378 accessions), using a pruned set of 1,833 SNPs, a likelihood of K=2 was estimated, indicating the presence of two sub-populations belonging to the Andean and Middle American gene pools, as confirmed by gene-pool checks ‘Jalo EEP 558’ and ‘BAT 93’ (Supplementary Figure S1). Accessions were further categorized based on a membership coefficient of Q ≥ 0.85. The Andean population comprised 166 accessions (43.9%), and the Middle American, 27 accessions (7.1%). The remaining accessions were grouped as admixtures according to gene-pool predominancy, using a membership coefficient Q ≥ 0.5, with 108 accessions as Andean admixtures (28.6%) and 77 as Middle American admixtures (20.4%) (Supplementary Table S4A).
A similar STRUCTURE analysis was performed in the DBP (88 accessions), using an LD-pruned set of 974 SNP markers, and K=2 was estimated (Supplementary Figure S2), with 59 Andean (67.0%) and 29 Middle American (33.0%) accessions (Supplementary Table S4B). Gene pool classification was based on previous diversity studies (Cichy et al., 2015; Moghaddam et al., 2016).
GWAS in SnAP and DBP
A filtered set of 25,472 biallelic SNPs from 378 SnAP accessions were obtained by GBS. Multiple GWAS analyses were conducted to identify the genomic regions associated with resistance to CTV in the SnAP. The first GWAS compared 208 resistant, 13 intermediate-resistant, and 98 susceptible SnAP accessions, and 53 accessions segregating for CTV symptoms were excluded. A significant interval from 2.79 to 4.32 Mb on chromosome Pv07 was identified, exceeding the Bonferroni-corrected significance threshold of α = 0.05 (p = 1.7E−36), which was associated with resistance and intermediate resistance to CTV (Supplementary Figure S3A). This interval corresponds with the location of the Bct gene that was identified by Larsen and Miklas (2004) in a snap bean RIL population and a QTL (BCT7.1GT) that conditions resistance to CTV found in the dry bean landrace G122 named ‘Jatu Rong’ (PI 163120) from India (Larsen et al., 2010).
A separate GWAS comparing 208 resistant with 98 susceptible accessions and 13 intermediate-resistant with 98 susceptible accessions revealed similar significant peak intervals of 2.66–4.32 Mb (p = 7.81E−44) and 2.79–3.60 Mb (p = 1.89.17) on Pv07, respectively (Supplementary Figures S3B, C). No significant SNPs were detected by the GWAS comparing 208 resistant and 13 intermediate-resistant SnAP accessions. Therefore, a GWAS was conducted comparing 239 resistant and intermediate-resistant accessions coded as 1 with 98 susceptible accessions coded as 0. This analysis showed a genomic interval (containing 129 significant SNPs) from 2.66 to 4.32 Mb on chromosome Pv07 with significantly increased significance (p =1.12E−45), as expected for a single major allele (Figure 1A). One LD block within the 2.66–4.32 interval, from 2,794,761 to 3,199,136 bases (Supplementary Table S5), consisting of 50 significant SNPs with the highest p-values ranging from p = 1.08E−34 to 1.12E−45, became the target region for the Bct allele for CTV resistance on Pv07 (Figure 1B). Other LD blocks with CTV resistance associations less than p = 2.43E−28 were excluded as targets within the 2.66 – 4.32 Mb interval.
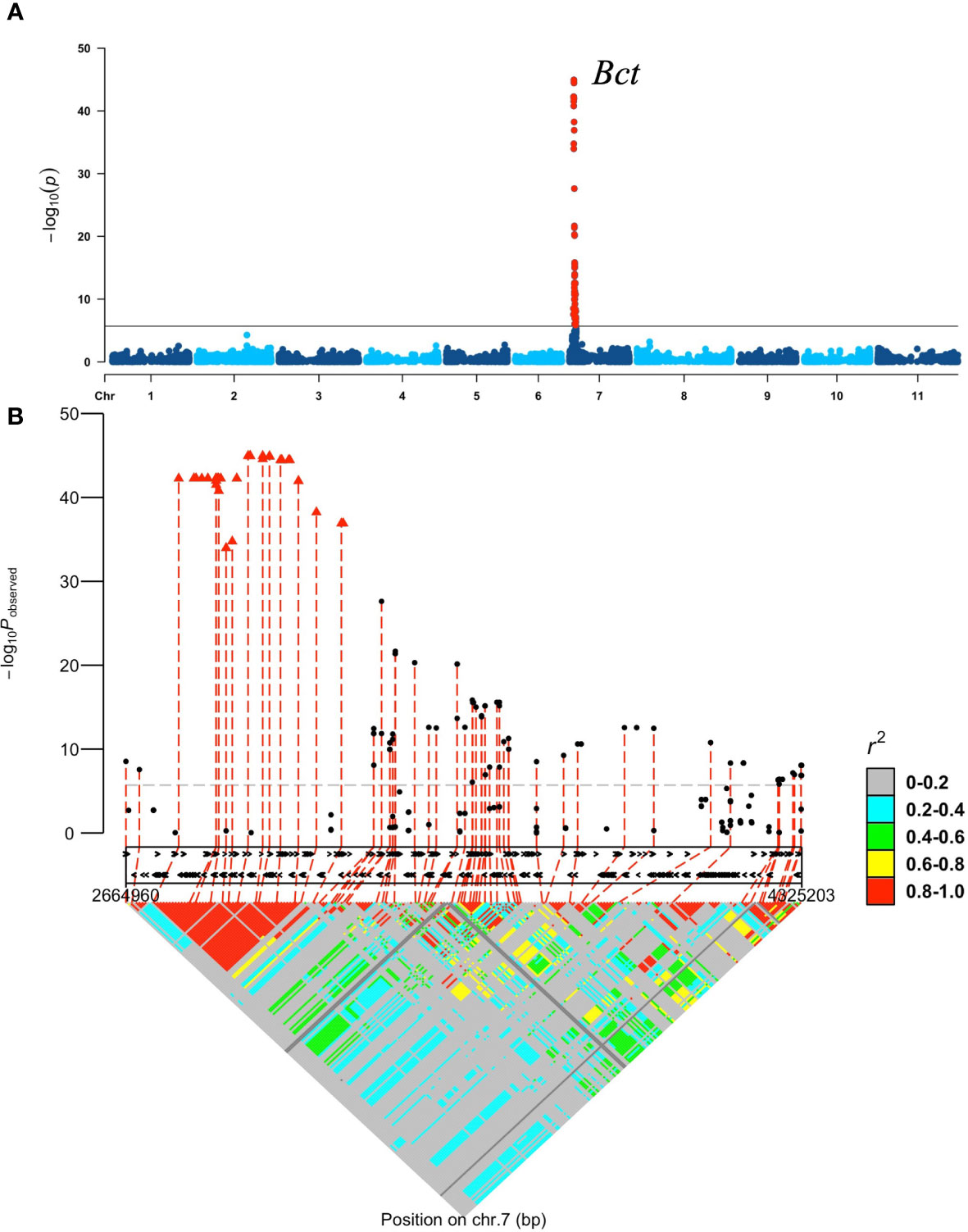
Figure 1 GWAS for CTV reaction by agro-filtration (Logan strain) in the SnAP, accessions with resistant and intermediate resistant phenotypes coded 1 versus susceptible lines coded 0: (A) Manhattan plot produced by GWAS analysis with GAPIT. Each dot represents each SNP obtained by GBS after filtering. The horizontal line represents a significance level at p-value < 1.96E−06. The peak region corresponds with the previous location for the Bct gene and BCT7.1 QTL (Larsen and Miklas, 2004; Larsen et al., 2010); (B) 128 significant SNPs from GWAS, which define the Bct gene region on Pv07. The x-axis depicts candidate genes (black arrows) within the 1.6-Mb region. Pairwise linkage disequilibrium using the significant SNPs for the CTV resistance region identifies one LD block with 50 significant SNPs (red triangles) with the highest p-value ranging from 1.08E−34 to 1.12E−45 [−log10(p-value) = 33.97 to 44.95] in an interval from 2,794,761 to 3,199,136 bases on Pv07.
GWAS with the 88 DBP accessions, using 7,983 SNP markers after filtering, did not detect any significant peaks for resistance to CTV. To improve the analysis, we incorporated six additional SNP markers, including three identified from sequencing candidate genes in the Bct region described below and three associated with resistance to bean leaf crumple virus (BLCrV) from Ariza-Suarez et al. (2023). Using these additional SNPs, the GWAS analysis identified a missense SNP, S07_2970381 (G19833v2.1 reference genome), within a candidate gene in Bct region with a high p-value (p =1.84E−07) (Figure 2A). The three SNP markers linked to BLCrV resistance (Figure 2B) had low LD with the sBct region and did not associate with CTV resistance.
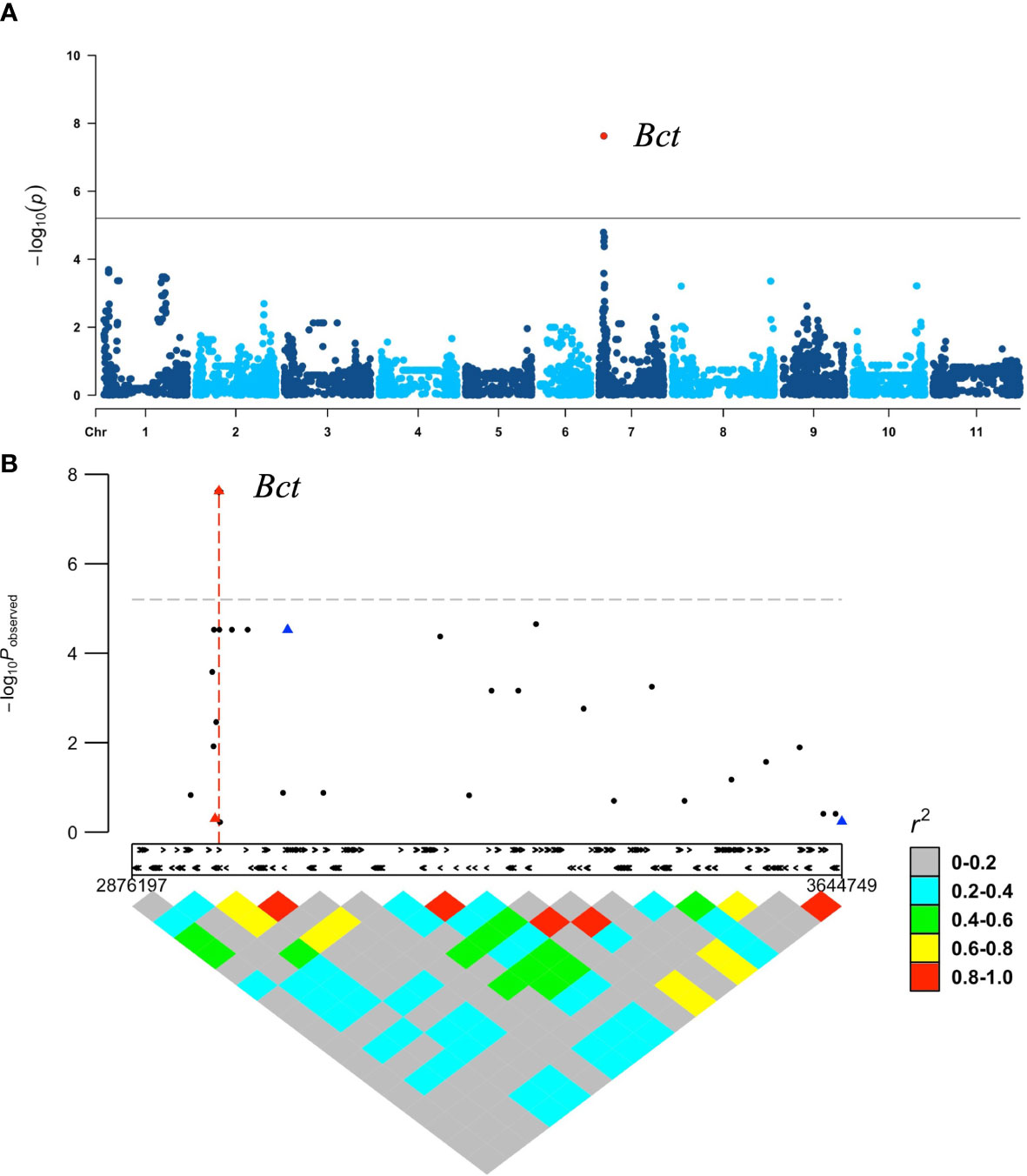
Figure 2 GWAS in DBP evaluated for CTV reaction (Worland strain) under field conditions using 7,983 SNP markers obtained from 12_K BARC Illumina chip assay with six additional select Tm-shift assay SNP markers: (A) Manhattan plot produced by GWAS analysis with GAPIT. Only one significant SNP S07_2970381 (red dot) for Bct region was identified; (B) low LD, and no associations were observed between Bct region (red triangle) and SNP markers (blue triangles) linked to BLCrV resistance.
Candidate gene identification in Bct region
Six resistant SnAP accessions displayed recombination within the targeted LD block (2,794,761–3,199,136 bp) for the Bct region on Pv07 (Supplementary Table S6). These accessions were ‘Goldcrop’ (SnAP152) and ‘Keygold’ (SnAP191) from the Andean gene pool; ‘Blue Mountain’ (SnAP037) from the Middle American gene pool; and Andean admixtures ‘Early Bird’ (SnAP107), ‘FR 266’ (SnAP136), and ‘Labrador’ (SnAP194). Haplotypes for the six developed markers spanning the LD block in the recombinant SnAP accessions narrowed the interval for the Bct region to 58.0 kb from 2,943,470 to 3,001,466 bases (Figure 3; Supplementary Table S6).
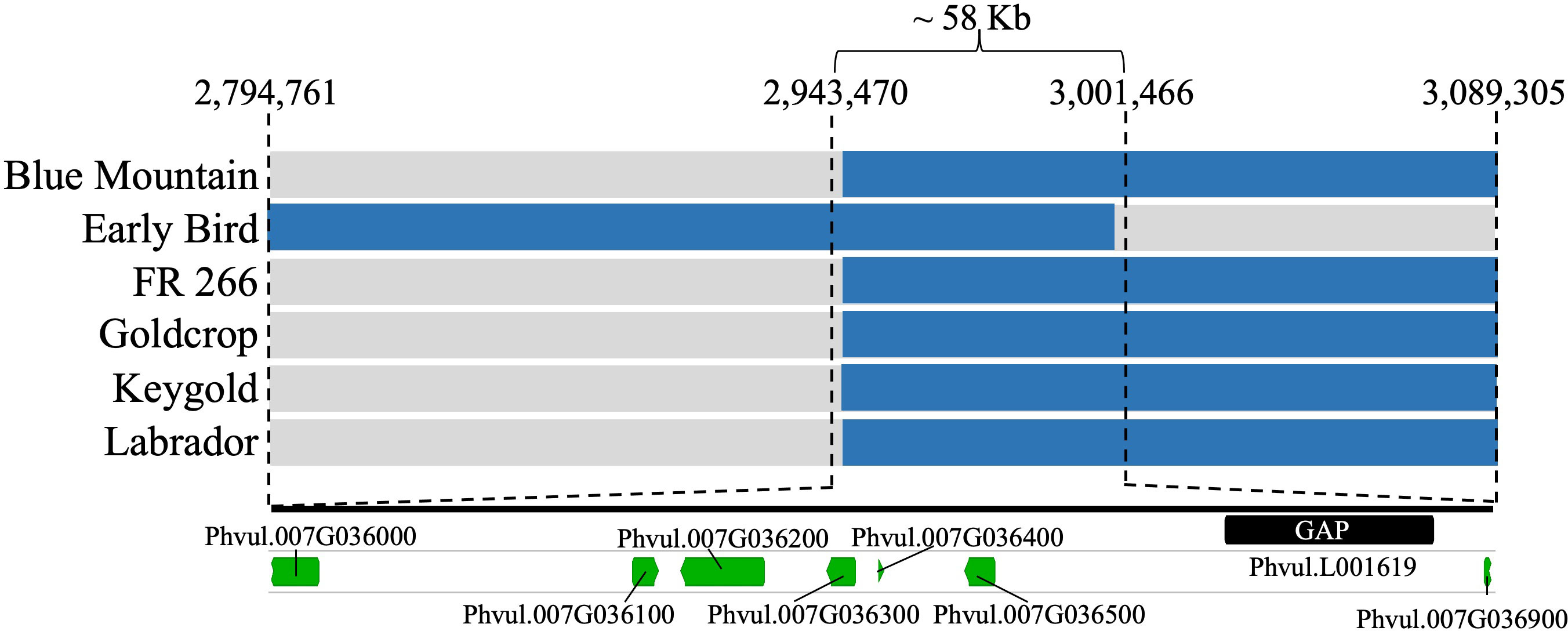
Figure 3 SnAP accessions with recombinant haplotype in Bct region, located seven gene models (green bars) distributed in 58 kb, and one additional gene model in Scaffold 1137 located in a gap (black bar) region in G19833 v2.1 reference genome. Due to presence of the susceptible allele from the S07_2943470 and S07_3001466 SNP markers in the six recombinant lines, the Phvul.007G036000 and Phvul.007G036900 gene models were excluded. More details can be found in Supplementary Table S6.
Six candidate genes were annotated in the refined 58.0 kb Bct region based on the Andean G19833 common bean v2.1 gene models (Table 1). However, one of these gene models, Phvul.L001619, was missing from a gap segment (Pv07: 2,988,789–2,998,791 bases) in the Bct region of G19833 v2.1. A BLASTn search in the previous version of the G19833 v1.0 reference genome revealed that this gene model had 100% similarity with the former Phvul.007G036700 in G19833 v1.0.
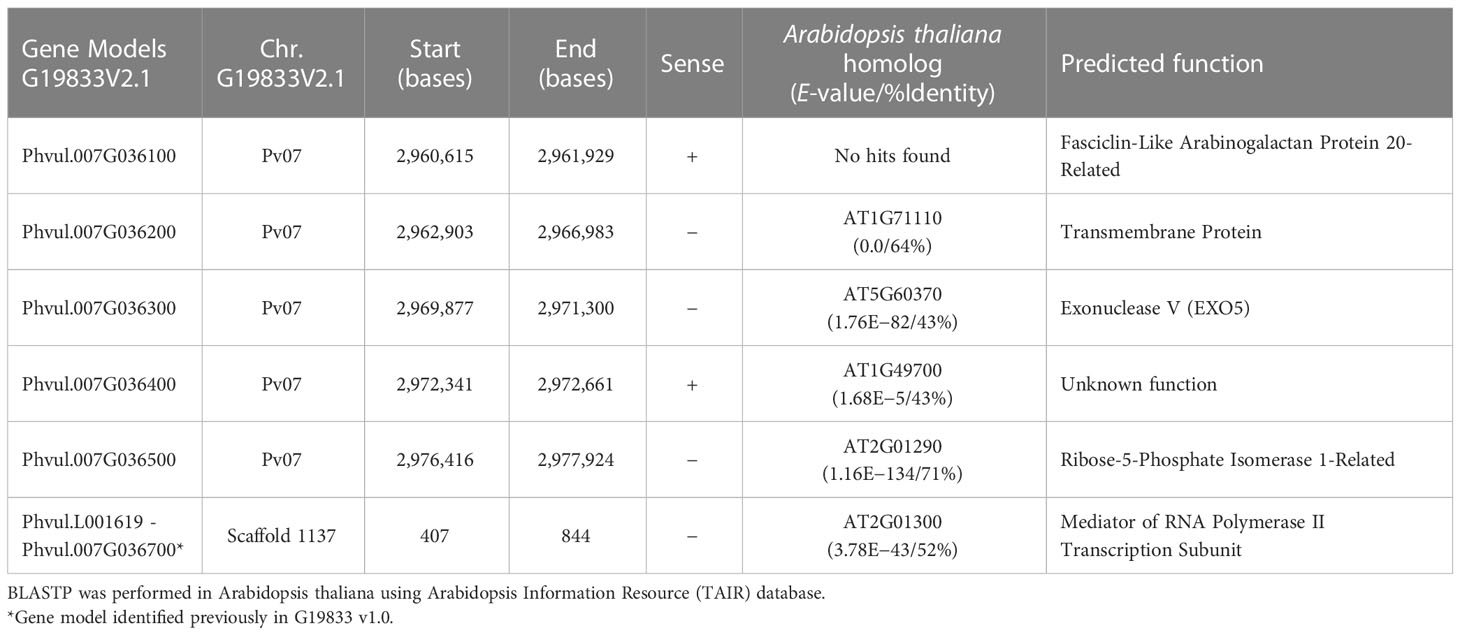
Table 1 Candidate gene models in G19833 v2.1 reference genome for the narrowed 58-kb interval for Bct gene region in the SnAP.
Interestingly, two candidate genes in the Bct region, Phvul.007G036300 encoding a 5′ deoxyribonuclease domain-containing protein (Exonuclease V) and Phvul.L001619, which functions as a mediator of RNA polymerase II transcription subunit, showed homology with gene models from other plant species involved in the replication of geminiviruses (Hanley-Bowdoin et al., 2013). Another candidate gene, Phvul.007G036200 (transmembrane protein), may play a role in defense response to other organisms based on its homology to the Arabidopsis thaliana gene AT1G71110. Target open reading frame (ORF) sequencing of the three candidate genes revealed 29 variants, 28 SNPs, and 1 InDel among a subset of 28 snap and dry bean genotypes from diverse backgrounds (Supplementary Table S7). Additionally, Phvul.007G036400 and Phvul.007G036500 gene models within the Bct region were sequenced, with two nonsense InDel variants and two synonymous SNP variants identified, respectively. Overall, for the 33 variants in the five candidate genes assayed across the 28 genotypes, only one variant, a missense SNP on Pv07: 2,970,381 bases in candidate gene Phvul.007G036300, was completely associated with BCTV resistance.
The in silico analysis of the Exonuclease V (EXO5) protein of the sequenced ORF for gene Phvul.007G036300 did not uncover any disruptions in the EXO5 domain. Nevertheless, the missense SNP variant located in Pv07: 2,970,381 bases involves a transition of Adenine (A) to Thymine (T) base, resulting in radical replacement of a polar, uncharged amino acid, glutamine (Q), with a non-polar, hydrophobic amino acid, leucine (L), at position 279 in the EXO5 domain (i.e., p.Q279L) (Table 2).
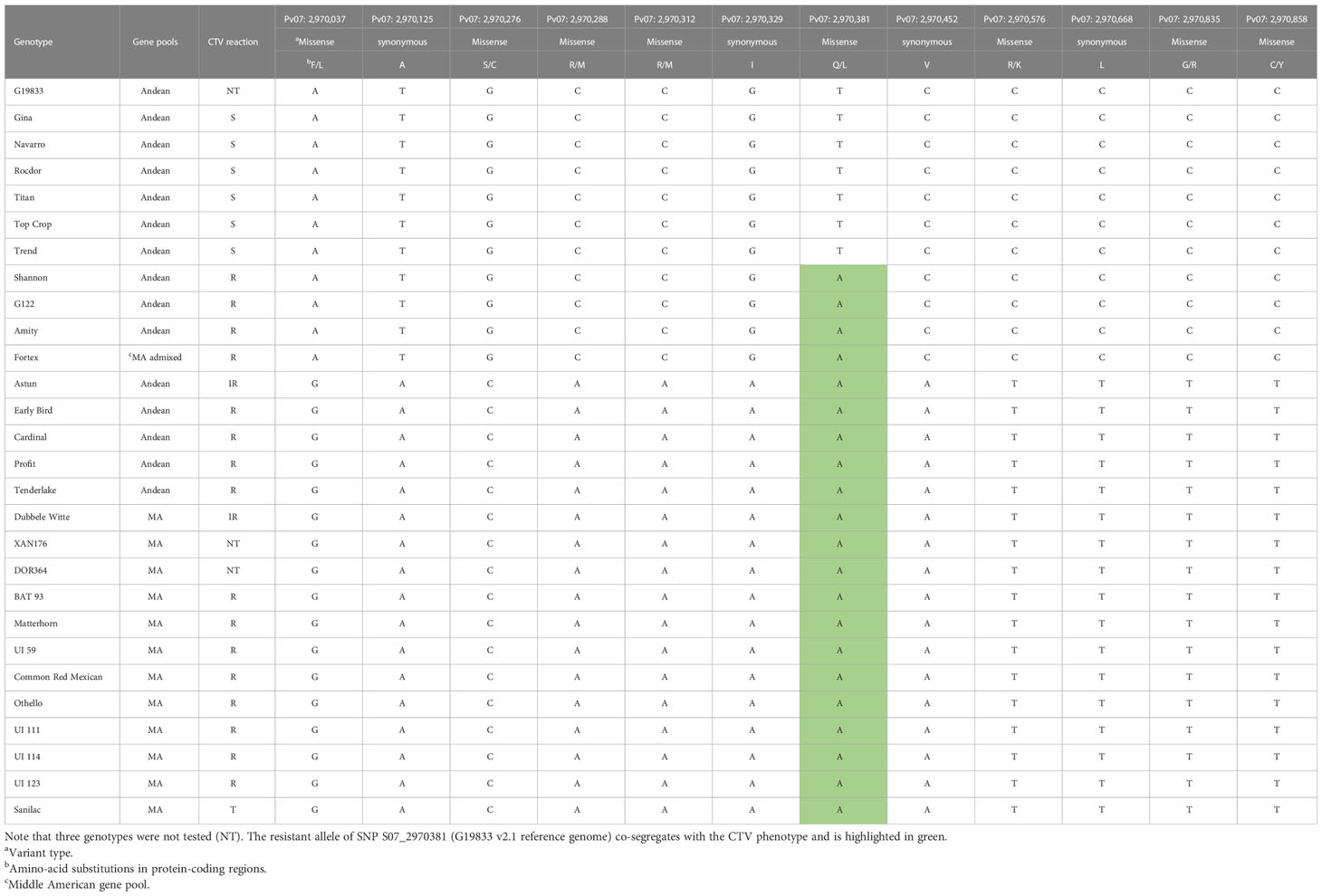
Table 2 Polymorphisms detected within the ORF for Bct candidate gene Phvul.07G36300 among 28 genotypes—6 susceptible (S), 16 resistant (R), 2 intermediate resistance (IR), and 1 tolerant (T) to CTV conditioned by Bct.
The dendrogram based on the genetic distance of the translated protein for the Bct candidate gene Phvul.007G036300 in the 28 sequenced genotypes showed one cluster for susceptibility and two distinct clusters for resistance/intermediate resistance to CTV (Figure 4). The first cluster consisted of susceptible snap bean genotypes from the Andean gene pool. The second cluster included the resistant Andean snap bean genotypes ‘Shannon’, ‘Amity’, ‘Fortex’, and the Andean dry bean ‘G122’. The third cluster included the resistant Andean snap bean genotypes ‘Early Bird’, ‘Astun’, ‘Profit’, ‘Tenderlake’, the Middle American snap bean ‘Dubbele Witte’, and all the sequenced dry beans from the Middle American gene pool. The Bct EXO5 candidate gene protein sequence exhibits 99.75% identity between Andean-resistant and Andean-susceptible genotypes, with only one amino acid change observed for the codon containing SNP S07_2970381. In contrast, resistant and tolerant Middle American genotypes display 100% identity for the EXO5 protein.
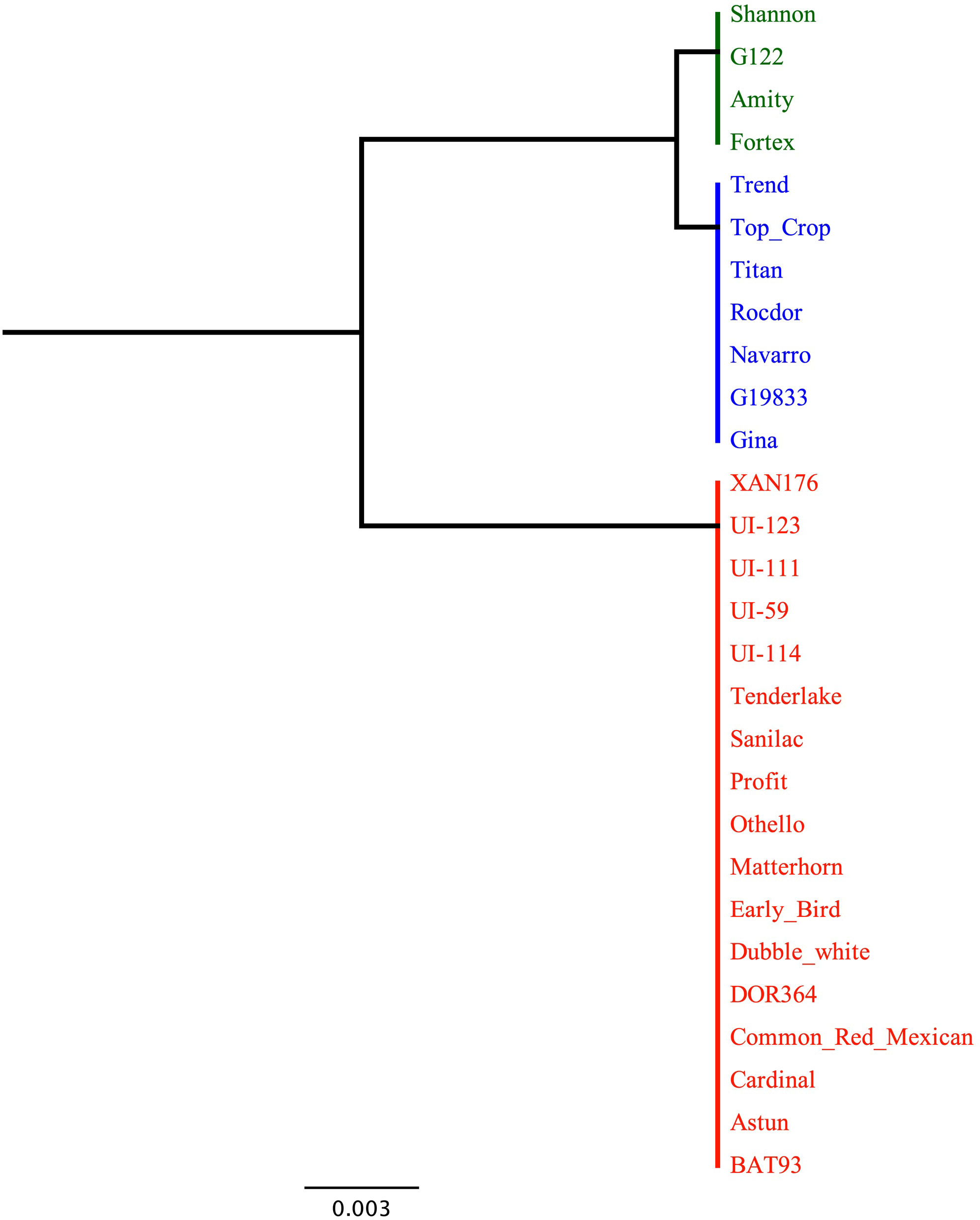
Figure 4 Dendrogram based on genetic distance for sequence diversity for candidate gene Phvul.007G036300 among 28 select SnAP and dry bean genotypes; 19 with and 6 without CTV resistance conditioned by Bct. Green cluster represents Andean susceptible genotypes, blue cluster Andean resistant (except for Middle American ‘Dubbele Witte’) and red cluster Middle American resistant to CTV. Blosum62 for alignment. Genetic distance Jukes-cantor. Tree build method UPGMA. Note three genotypes untested for CTV reaction were included: reference genome ‘G19833’ and ‘DOR 364’ and ‘XAN 176’ parents of the DX RIL population used by Soler-Garzón et al. (2021) to map BGY7.1 QTL for resistance to BGYMV.
Tm-shift assays for diagnostic SNPs in Bct region
A Tm-shift assay named S07_2970381 was specifically designed to detect the missense SNP Pv07: 2,970,381 in the Bct candidate gene Phvul.007G036300 (EXO5), which exhibited polymorphism between resistant and susceptible genotypes to CTV. Additionally, two Tm-shift assays were developed to distinguish introgression haplotypes of the Bct region from Andean and Middle American gene pools. The first assay, named S07_2970276, targeted the missense SNP variant Pv07: 2,970,276 bases within Phvul.007G036300 gene and differentiated genotypes from Andean and Middle American gene pools. The second assay, named S07_2966197, focuses on another missense SNP variant, Pv07: 2,966,197 bases, found in the Phvul.007G36200 gene model by Soler-Garzón et al. (2021) in a race Durango dry bean diversity panel. This variant differentiated genotypes within the Middle American genepool. The primer sequences and Tm-shift melting curves for each SNP marker can be found in Table 3 and Supplementry Figure S4, respectively.
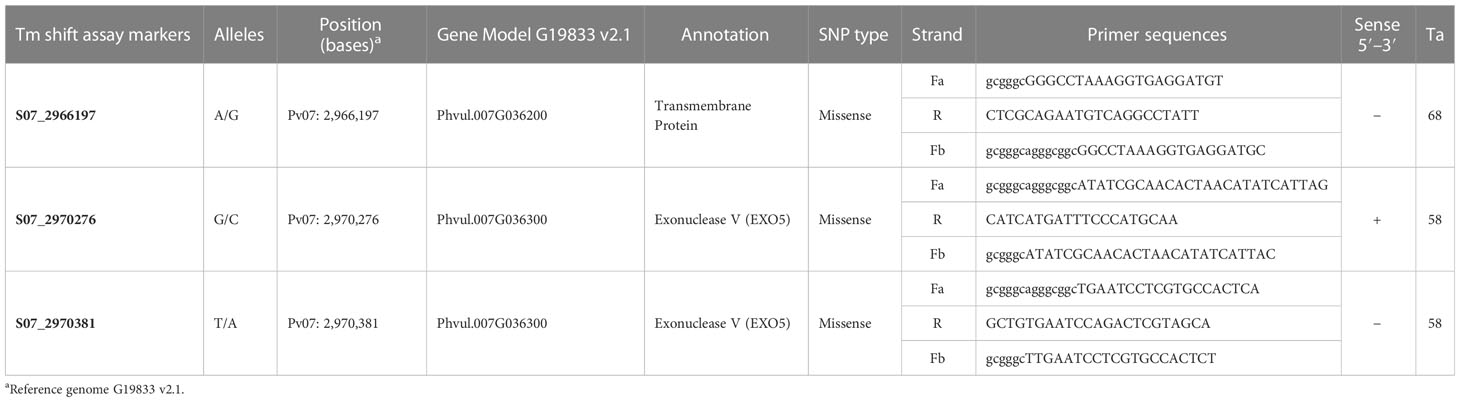
Table 3 Primers used to assay select SNP markers for haplotyping the Bct EXO5 candidate gene region.
Bct-linked markers assays
The S07_2970381 marker exhibited a 100% correlation with the CTV phenotype observed in SnAP. For the 378 SnAP accessions, 238 (63.0%) showed resistance or intermediate resistance to the CTV CA/Logan strain and possessed the resistance allele of the S07_2970381 marker, and 100 (26.5%) were susceptible and possessed the susceptible allele of the S07_2970381 marker, while 33 (8.7%) were segregating for CTV reaction, and 7 (1.8%) had missing data (Supplementary Table S8).
For the DBP (88 accessions) infected with the Wor strain, a correlation of 99% was observed between the S07_2970381 SNP marker and the disease score. There were 42 accessions with resistant disease scores ≤ 4.0, which possessed the resistant allele of the S07_2970381 SNP marker, and 13 with susceptible disease scores from 7.0 to 9.0, which possessed the susceptible allele (Supplementary Table S9). The 31 tolerant accessions, with disease scores from 4.1 to 6.9, possessed the resistant marker allele with two exceptions: ‘SVS-0683’ (DBP42) with a disease score of 6.3 and ‘Beluga’ (DBP45) with a disease score of 6.2, possessed the susceptible marker allele. Note that the ‘Beluga’ genotype exhibited a susceptible reaction when agro-infiltrated with Logan strain, while SVS-0863 was untested.
A comparison of genotypes for the S07_2970381 SNP marker and the ‘SAS8.1500’ SCAR marker developed by Larsen and Miklas (2004) showed a 97.1% similarity in the SnAP and 47.4% in the DBP. The ‘SAS8.1550’ SCAR marker, Pv07: 2,975,432–2,977,000 bases, is 4.1 kb downstream of the EXO5 candidate gene Phvul.007G036300 and in high linkage disequilibrium (LD) with the S07_2970381 and S07_2970276 SNP markers in the SnAP and DBP. In fact, ‘SAS8.1550’ and S07_2970276 markers were 100% identical in both bean panels.
Bct region haplotypes
The SnAP and DBP were assayed for the S07_2970381, S07_2970276, and S07_2966197 markers to identify haplotypes for the Bct region. Four haplotypes were identified: Haplo1 with AGT sequence for the S07_2966197 (A/G), S07_2970276 (G/C), and S07_2970381 (T/A) SNPs, respectively; Haplo2 with AGA sequence; and Haplo3 divided into two sub-haplotypes, Haplo3-1 with ACA sequence and Haplo3-2 with GCA sequence, based on polymorphism for S07_2966197 in Middle American accessions.
In the SnAP (Table 4), Haplo1 was found in all 98 susceptible accessions: 97% Andean and Andean admixtures and 3% Middle American and Middle American admixtures. Haplo2 was a rare haplotype found in only five resistant accessions, four Andean/Andean admixtures, and one Middle American admixture. Haplo3-1 was observed in 162 resistant/intermediate resistant accessions: 76% Andean and Andean admixtures and 24% Middle American and Middle American admixtures. Haplo3-2 was found in 71 resistant/intermediate resistance accessions: 68%) Middle American and Middle American admixtures and 32% Andean and Andean admixtures.
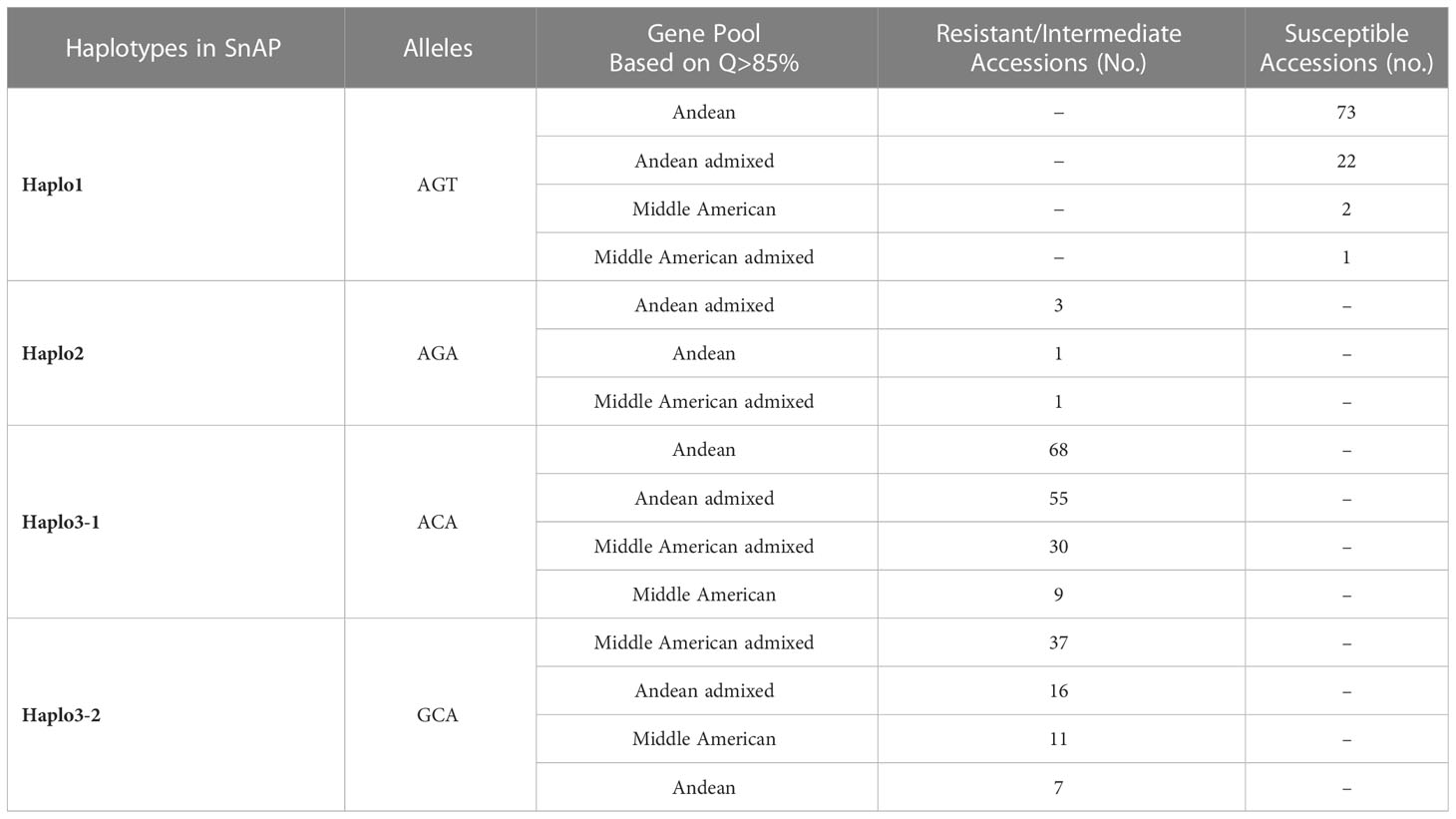
Table 4 Haplotypes identified in SnAP (336 Accessions) phenotyped by agro-inoculation with Logan strain and genotyped with the S07_2966197 (A/G), S07_ 2970276 (G/C), and S07_ 2970381 (T/A) markers.
For the DBP (Table 5), Haplo1 was found in 15 Andean accessions with a mean disease score of 8.0, 13 with susceptible reactions and 2 tolerant accessions, ‘SVS-0683’ and ‘Beluga’ with scores of 6.3 and 6.2, respectively. Haplo2 was observed in 27 resistant/tolerant Andean accessions (3.8 mean score). There were 30 resistant/tolerant accessions (3.5 mean score) with Haplo3-1, 63% Middle American and 37% Andean. For Haplo3-2, 16 resistant/tolerant accessions (4.4 mean score), 62% Middle American and 38% Andean, were observed. CTV mean score comparisons among haplotypes in the DBP, using pHolm-adjusted p-values, showed that the susceptible Haplo1 was significantly different from the three resistant haplotypes (p < 0.0001), while the only significant difference among resistant haplotypes mean scores was between Haplo3-1, which was more resistant than Haplo3-2 (p = 0.05) (Figure 5).
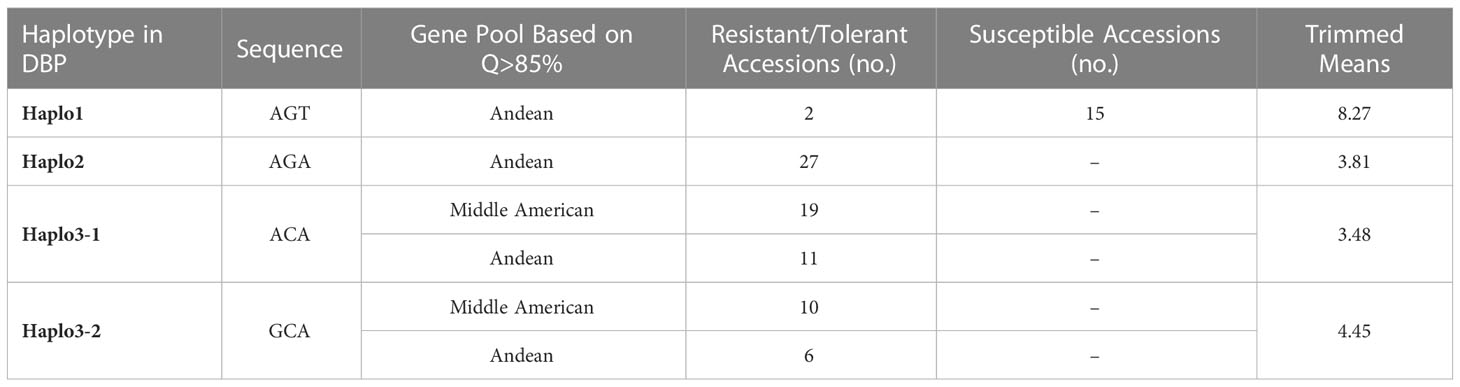
Table 5 Haplotypes identified in DBP (88 accessions) phenotyped in the field with Worland strain and genotyped with the S07_2966197 (A/G), S07_ 2970276 (G/C), and S07_ 2970381 (T/A) markers.
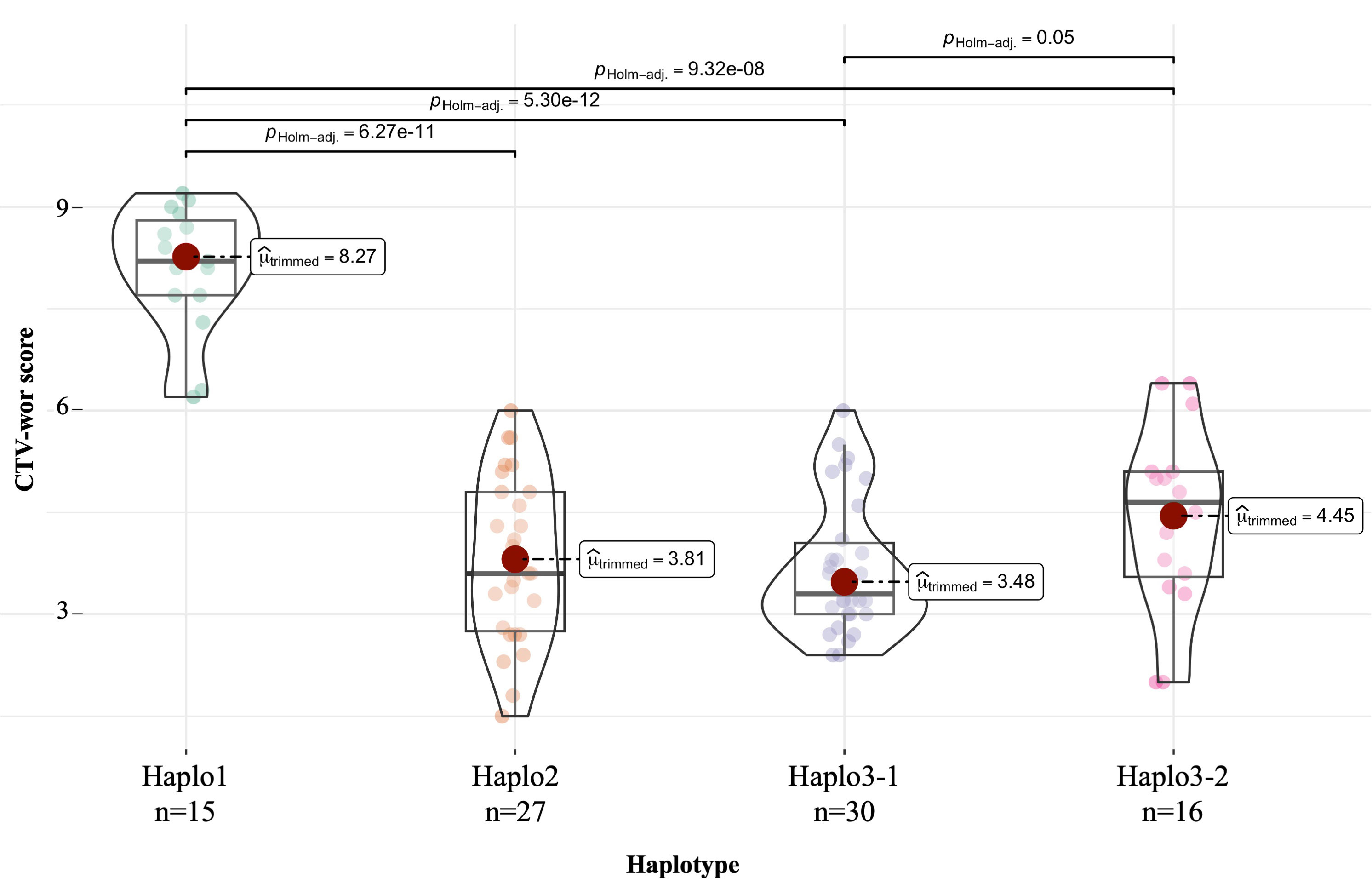
Figure 5 One-way ANOVA for quantitative CTV-Wor reaction (1 to 9 scale) in DBP accessions among four haplotypes compared by Ftrimmed-means using the ggbetweenstats function in the R-Package ggstatsplot. Haplotypes based in polymorphism combination of S07_2966197, S07_2970276, and S07_297038 SNPs markers.
The haplotype survey using the same three markers was expanded to 57 dry bean genotypes, 30 Andean and 27 Middle American, which were agro-inoculated with the CA/Logan strain in Sun Prairie, WI (Supplementary Table S10). Both Haplo1 and Haplo2 were composed of only Andean genotypes, 7 with susceptible and 12 with resistant reactions to CTV, respectively. All Middle American genotypes were resistant to the CA/Logan strain and exhibited either Haplo3-1 (19 genotypes) or Haplo3-2 (8 genotypes). In addition, there were seven resistant Andean accessions with Haplo3-1 and 4 with Haplo3-2. Qualitative reactions to agro-inoculation with the CA/Logan strain matched the quantitative field reactions to Wor strain, for the 12 genotypes included in both the DBP and this additional survey, except for Beluga, which was susceptible to agro-inoculation but tolerant in the field.
Discussion
The Bct gene region was first located to a 1.7-Mb interval on the proximal arm of chromosome Pv07 by GWAS using the GBS-generated SNP dataset for the SnAP panel agro-inoculated with the CA/Logan strain. A high LD block of the 50 most significant SNPs narrowed the Bct region to a 0.4-Mb interval. Six recombinant SnAP accessions assayed with designed SNPs further narrowed the interval to a 58-kb region. The ORF for five of the six gene models that spanned the 58-kb interval were sequenced across a select set of 28 snap and dry bean accessions. Of 33 SNP and InDel variants identified across the five genes, only one missense SNP (S07_2970381) in candidate gene Phvul.007G036300 (Exonuclease V) was identified as the most likely causal mutation conditioning Bct resistance. Moreover, GWAS of the DBP identified the S07_2970381 SNP variant as the only significant SNP for BCTV reaction in the field.
Exonuclease V has not been extensively studied in plants, and its function in viral resistance is yet to be elucidated. However, the Arabidopsis homolog AT5G60370, which encodes an Exonuclease V-Like (EXOVL) protein, exhibited 43% sequence identity (E-value = 1.76E−82) with the Phvul.007G036300 Bct candidate gene. EXOVL has been reported to play critical roles in biological processes underlying morphological traits in A. thaliana (Huang et al., 2022). Initially, Exonuclease V was identified and purified from Saccharomyces cerevisiae, encoded by the YBR163w gene (subsequently renamed EXO5). This protein is capable of degrading single-stranded DNA (ssDNA) from the 5′-end and plays a critical role in mitochondrial maintenance (Burgers et al., 2010). Furthermore, EXO5 is distantly related to the nuclease domain of RecB, a subunit of the bacterial RecBCD recombinase, which actively degrades linear double-stranded DNA (dsDNA), such as phage DNA, and thereby serves an antiviral function for the bacterium (Dillingham and Kowalczykowski, 2008).
The Bct EXO5 candidate gene is located 340.8 kb upstream from the BLC7.1 locus, which confers resistance to BLCrV, a Begomovirus (Ariza-Suarez et al., 2023). BCL7.1 is a minor-effect QTL that was associated with a Leucine-rich repeat receptor-like kinase (LRR-RLK) encoded by the gene model Phvul.007G040400 (Pv07: 3,312,090–3,315,269 bases). Ariza-Suarez et al. (2023) suggested previous breeding efforts for resistance to geminiviruses [e.g., CTV, bean golden yellow mosaic virus (BGYMV)] contributed to the accumulation of positive alleles against emerging viruses like BCL7.1. Although both Bct and BCL7.1 regions provide resistance to geminiviruses, a Curtovirus and Begomovirus, respectively, they do not physically overlap and exhibit low linkage disequilibrium. Perhaps, breeding for quantitative resistance to BGYMV, also a Begomovirus, conditioned by the BGY7.1 QTL spanning 2,724,611–3,525,083 bases on Pv07 (Soler-Garzón et al., 2021), contributed to the partial quantitative resistance observed against BLCrV. The broad genomic interval for BGY7.1 notably overlaps the Bct and BLC7.1 regions. Miklas et al. (2009) postulated that race Durango cultivars within the Middle American gene pool, such as ‘UI 35’ small red, ‘UI 114’ pinto, and others, which possess combined levels of resistance to CTV, BGYMV, and BDMV, could result from a common resistance gene or linked resistance genes located in the Bct region on Pv07. Continued research is warranted to further characterize and identify candidate genes within this “hot-spot” region, and mutations therein, that confer resistance to different geminiviruses.
The Tm-shift assay marker developed for SNP S07_2970381 will be useful for tracking CTV resistance conferred by the Bct EXO5 candidate gene in both snap and dry bean. The CTV reaction for 471 snap and dry bean accessions (excluding those segregating or missing data) from the SnAP, DBP, and additional survey of 57 dry bean genotypes clearly co-segregated with alleles of the S07_2970381 SNP marker. SVS-0863 dry bean with a tolerant disease score in the field but lacking the resistant marker allele is the only minor exception. The ‘Beluga’ dry bean with similar field tolerance but lacking the marker allele for resistance was later found to be susceptible to CTV via agro-inoculation.
The Bct EXO5 candidate gene is nearly ubiquitous in the Middle American gene pool as 98% (140/143) Middle American snap (including admixtures) and dry bean genotypes exhibited CTV resistance (including intermediate resistance and tolerance) and possessed the resistance allele for the EXO5 candidate gene mutation (S07_2970381 SNP). Conversely, only 65% (214/327) of Andean (including admixtures) snap and dry bean genotypes tested were resistant to CTV and possessed the resistance allele for the marker. In addition, all 113 Andean snap (including admixtures) and dry bean genotypes susceptible to CTV lacked the resistance allele for the Bct EXO5 candidate gene marker. Finally, only three Middle American snap bean accessions were susceptible to CTV, and they lacked the resistance allele for the marker.
The differences in percentage correlation between S07_2970381 and ‘SAS8.1550’, and S07_2970276 and ‘SAS8.1550’ markers, together with distinct haplotypes for combinations of the S07_2966197, S07_2970276, and S07_2970381 SNP markers in the SnAP and DBP, suggest different origins of the Bct candidate allele. The susceptible Andean beans all possess the same haplotype (Haplo1) for the three SNP markers S07_2966197, S07_2970276, and S07_2970381 in the Bct region. Haplo2 represents a small set of Andean genotypes with resistance to CTV. Among these genotypes, there are a few Andean dry bean landrace cultivars, including IJR (PI 163122, G8088) and G122 from India, Canadian Wonder (ADP_010) and Kijivu (ADP_033) from East Africa, Pompadour B from the Caribbean, and California LRK, with Haplo2, which suggests that this haplotype and associated Bct resistance is of Andean origin. This ‘Haplo2’ Bct resistance source was unlikely to have been purposely used in breeding for CTV resistance. All the dry bean genotypes in the DBP with Haplo2 represent mostly breeding lines that had never been screened for CTV reaction prior to this study, and their pedigrees reveal the use of Canadian Wonder, IJR, and Kijivu landrace cultivars as parents. Moreover, only 5 of 177 CTV-resistant snap bean accessions possessed Haplo2.Resistance to CTV in snap bean was purposely initiated from crosses with Middle American dry bean genotypes like ‘Burtner’ navy bean in 1936 and small red (~ ‘Common Red Mexican’) beans during the 1950s (Thomas and Zaumeyer, 1953). Haplo3-1 represents the source of Bct resistance from ‘Common Red’ landrace, and it is the most widely represented haplotype among resistant snap (70%) and dry bean (45%) genotypes in this study. Many of the dry bean genotypes with Haplo3-1 come from breeding programs that were actively selected for CTV resistance.
Haplo3-2, on the other hand, is represented by older great northern bean cultivars (i.e., UI-59, Harris, Beryl) and more modern race Durango cultivars (i.e., Matterhorn, Merlot, Kodiak) with upright architecture obtained from race Mesoamerica introgressions. Few, if any, of the dry bean genotypes with Haplo3-2 were developed by breeding programs with active or passive selection for Bct resistance. The mean disease score (3.5) for the Haplo3-1 dry bean genotypes indicates a significantly (p = 0.05) higher level of resistance than the mean score (4.4) for Haplo3-2 genotypes. Resistance levels for snap beans with Haplo2, Haplo3-1, or Haplo3-2 were undiscernible because only qualitative data existed for CTV reaction.
In light of these findings, breeding programs using S07_2970381 for MAS for CTV resistance conferred by the Bct EXO5 candidate gene should also include SNP markers S07_2966197 and S07_2970276 to monitor the different haplotypes and corresponding associations that they may have with quantitative levels of resistance observed by phenotyping. Meanwhile, based on information from this study, our recommendation is to use Haplo3-1 for MAS of CTV resistance.
Data availability statement
The original contributions presented in the study are included in the article/Supplementary Material. Further inquiries can be directed to the corresponding author.
Author contributions
AS-G, JH, and PM conceived and designed the experiments. AS-G and JH implemented statistic and bioinformatic analyses. DG, AT, and CS performed and conducted phenotyping strategies. QS performed SNP chip assay genotyping. KG conducted virus detection in the field. All authors contributed to the article and approved the submitted version.
Conflict of interest
Authors DG and AT were employed by HM Clause Seed Company.
The remaining authors declare that the research was conducted in the absence of any commercial or financial relationships that could be construed as a potential conflict of interest.
Publisher’s note
All claims expressed in this article are solely those of the authors and do not necessarily represent those of their affiliated organizations, or those of the publisher, the editors and the reviewers. Any product that may be evaluated in this article, or claim that may be made by its manufacturer, is not guaranteed or endorsed by the publisher.
Supplementary material
The Supplementary Material for this article can be found online at: https://www.frontiersin.org/articles/10.3389/fpls.2023.1215950/full#supplementary-material
References
Aparicio Arce, J. S. (2018) Mr. bean. Available at: https://apariciojohan.shinyapps.io/Mrbean/_w_d65167a5/ (Accessed February 03, 2023).
Ariza-Suarez, D., Keller, B., Spescha, A., Aparicio, J. S., Mayor, V., Portilla-Benavides, A. E., et al. (2023). Genetic analysis of resistance to bean leaf crumple virus identifies a candidate LRR-RLK gene. Plant J. 114, 23–38. doi: 10.1111/tpj.15810
Arkwazee, H. A., Wallace, L. T., Hart, J. P., Griffiths, P. D., Myers, J. R. (2022). Genome-wide association study (GWAS) of white mold resistance in snap bean. Genes (Basel) 13, 2297. doi: 10.3390/genes13122297
Bennett, C. W. (1971). The curly top disease of sugarbeet and other plants, monograph no. 7. (St. Paul, MN: The American Phytopathological Society).
Burgers, P. M., Stith, C. M., Yoder, B. L., Sparks, J. L. (2010). Yeast exonuclease 5 is essential for mitochondrial genome maintenance. Mol. Cell. Biol. 30, 1457–1466. doi: 10.1128/mcb.01321-09
Chiginsky, J., Langemeier, K., MacWilliams, J., Albrecht, T., Cranshaw, W., Fulladolsa, A. C., et al. (2021). First insights into the virus and viroid communities in hemp (Cannabis sativa). Front. Agron. 3. doi: 10.3389/FAGRO.2021.778433/BIBTEX
Cichy, K. A., Porch, T. G., Beaver, J. S., Cregan, P., Fourie, D., Glahn, R. P., et al. (2015). A Phaseolus vulgaris diversity panel for Andean bean improvement. Crop Sci. 55, 2149–2160. doi: 10.2135/cropsci2014.09.0653
Creamer, R. (2020). Beet curly top virus transmission, epidemiology, and management. In: Applied Plant Virology: Advances, Detection, and Antiviral Strategies. ed. Awasthi, L. P. (San Diego, CA: Elsevier) 521–527.
Crosslin, J. M., Vandemark, G. J., Munyaneza, J. E. (2006). Development of a real-time, quantitative PCR for the detection of the Columbia basin potato purple top phytoplasma in plants and beet leafhoppers. Plant Dis. 90, 663–667. doi: 10.1094/PD-90-0663
Dillingham, M. S., Kowalczykowski, S. C. (2008). RecBCD enzyme and the repair of double-stranded DNA breaks. Microbiol. Mol. Biol. Rev. 72, 642–671. doi: 10.1128/mmbr.00020-08
Elshire, R. J., Glaubitz, J. C., Sun, Q., Poland, J. A., Kawamoto, K., Buckler, E. S., et al. (2011). A robust, simple genotyping-by-sequencing (GBS) approach for high diversity species. PloS One 6, e19379. doi: 10.1371/journal.pone.0019379
Evanno, G., Regnaut, S., Goudet, J. (2005). Detecting the number of clusters of individuals using the software STRUCTURE: a simulation study. Mol. Ecol. 14, 2611–2620. doi: 10.1111/j.1365-294X.2005.02553.x
Francis, R. M. (2017). Pophelper: an r package and web app to analyse and visualize population structure. Mol. Ecol. Resour. 17, 27–32. doi: 10.1111/1755-0998.12509
Giladi, Y., Hadad, L., Luria, N., Cranshaw, W., Lachman, O., Dumbrovsky, A. (2020). First report of Beet curly top virus infecting Cannabis sativa in western Colorado. Plant Dis. 104, 999. doi: 10.1094/PDIS-08-19-1656-PDN
Hanley-Bowdoin, L., Bejarano, E. R., Robertson, D., Mansoor, S. (2013). Geminiviruses: masters at redirecting and reprogramming plant processes. Nat. Rev. Microbiol. 11, 777–788. doi: 10.1038/nrmicro3117
He, F., Ding, S., Wang, H., Qin, F. (2020). IntAssoPlot: an r package for integrated visualization of genome-wide association study results with gene structure and linkage disequilibrium matrix. Front. Genet. 11. doi: 10.3389/fgene.2020.00260
Hormuzdi, S. G., Bisaro, D. M. (1993). Genetic analysis of beet curly top virus: evidence of three virion sense genes involved in movement and regulation of single- and double-stranded DNA levels. Virology 193, 900–909. doi: 10.1006/viro.1993.1199
Hu, J., Masson, R., Dickey, L. (2021). First report of Beet curly top virus infecting industrial hemp (Cannabis sativa) in Arizona. Plant Dis. 105, 1233. doi: 10.1094/PDIS-11-20-2330-PDN
Huang, Y., Chen, J., Dong, C., Sosa, D., Xia, S., Ouyang, Y., et al. (2022). Species-specific partial gene duplication in arabidopsis thaliana evolved novel phenotypic effects on morphological traits under strong positive selection. Plant Cell 34, 802–817. doi: 10.1093/plcell/koab291
Huster, A. R., Wallace, L. T., Myers, J. R. (2021). Associated SNPs, heritabilities, trait correlations, and genomic breeding values for resistance in snap beans (Phaseolus vulgaris l.) to root rot caused by Fusarium solani (Mart.) f. sp. phaseoli (Burkholder). Front. Plant Sci. 12. doi: 10.3389/fpls.2021.697615
Jakobsson, M., Rosenberg, N. A. (2007). CLUMPP: a cluster matching and permutation program for dealing with label switching and multimodality in analysis of population structure. Bioinformatics 23, 1801–1806. doi: 10.1093/bioinformatics/btm233
Kearse, M., Moir, R., Wilson, A., Stones-Havas, S., Cheung, M., Sturrock, S., et al. (2012). Geneious basic: an integrated and extendable desktop software platform for the organization and analysis of sequence data. Bioinformatics 28, 1647–1649. doi: 10.1093/bioinformatics/bts199
Koressaar, T., Remm, M. (2007). Enhancements and modifications of primer design program Primer3. Bioinformatics 23, 1289–1291. doi: 10.1093/bioinformatics/btm091
Langmead, B., Salzberg, S. L. (2012). Fast gapped-read alignment with bowtie 2. Nature Methods 9, 357–359. doi: 10.1038/nmeth.1923
Larsen, R. C., Kurowski, C. J., Miklas, P. N. (2010). Two independent quantitative trait loci are responsible for novel resistance to Beet curly top virus in common bean landrace G122. Phytopathology 100, 972–978. doi: 10.1094/PHYTO-02-10-0049
Larsen, R. C., Miklas, P. N. (2004). Generation and molecular mapping of a sequence characterized amplified region marker linked with the Bct gene for resistance to Beet curly top virus in common bean. Phytopathology 94, 320–325. doi: 10.1094/PHYTO.2004.94.4.320
Li, H., Handsaker, B., Wysoker, A., Fennell, T., Ruan, J., Homer, N., et al. (2009). The sequence Alignment/Map format and SAMtools. Bioinformatics 25, 2078–2079. doi: 10.1093/bioinformatics/btp352
Lipka, A. E., Tian, F., Wang, Q., Peiffer, J., Li, M., Bradbury, P. J., et al. (2012). GAPIT: genome association and prediction integrated tool. Bioinformatics 28, 2397–2399. doi: 10.1093/bioinformatics/bts444
Lobaton, J. D., Miller, T., Gil, J., Ariza, D., de la Hoz, J. F., Soler, A., et al. (2018). Resequencing of common bean identifies regions of inter-gene pool introgression and provides comprehensive resources for molecular breeding. Plant Genome 11, 170068. doi: 10.3835/plantgenome2017.08.0068
Melgarejo, T. A., Chen, L.-F., Rojas, M. R., Schilder, A., Gilbertson, R. L. (2022). Curly top disease of hemp (Cannabis sativa) in California is caused by mild-type strains of Beet curly top virus often in mixed infection. Plant Dis. 106, 3022–3026. doi: 10.1094/PDIS-04-22-0856-SC
Miklas, P., Chilagane, L., Fourie, D., Nchimbi, S., Soler-Garzón, A., Hart, J. P., et al. (2020). QTL for resistance to angular leaf spot and rust in Tanzania vs. south Africa for the Andean panel & Rojo/Cal 143 RIL population. Annu. Rep. Bean Improv. Coop. 63, 83–84.
Miklas, P. N., Seo, Y.-S., Gilbertson, R. L. (2009). Quantitative resistance to Bean dwarf mosaic virus in common bean is associated with the Bct gene for resistance to Beet curly top virus. Plant Dis. 93, 645–648. doi: 10.1094/PDIS-93-6-0645
Moghaddam, S. M., Mamidi, S., Osorno, J. M., Lee, R., Brick, M., Kelly, J., et al. (2016). Genome-wide association study identifies candidate loci underlying agronomic traits in a middle American diversity panel of common bean. Plant Genome 9. doi: 10.3835/plantgenome2016.02.0012
Munyaneza, J. E., Upton, J. E. (2005). Beet leafhopper (Hemiptera: cicadellidae) settling behavior, survival, and reproduction on selected host plants. J. Econ. Entomol. 98, 1824–1830. doi: 10.1093/jee/98.6.1824
Myers, J. R., Wallace, L. T., Moghaddam, S. M., Kleintop, A. E., Echeverria, D., Thompson, H. J., et al. (2019). Improving the health benefits of snap bean: genome-wide association studies of total phenolic content. Nutrients 11 (10), 2509. doi: 10.3390/nu11102509
Patil, I. (2021). Visualizations with statistical details: the 'ggstatsplot' approach. J. Open Source Software 6 (61), 3167. doi: 10.21105/joss.03167
Perea, C., de la Hoz, J. F., Cruz, D. F., Lobaton, J. D., Izquierdo, P., Quintero, J. C., et al. (2016). Bioinformatic analysis of genotype by sequencing (GBS) data with NGSEP. BMC Genomics 17, 498. doi: 10.1186/s12864-016-2827-7
Pritchard, J. K., Stephens, M., Donnelly, P. (2000). Inference of population structure using multilocus genotype data. Genetics 155, 945–959. doi: 10.1093/genetics/155.2.945
Rivedal, H. M., Funke, C. N., Frost, K. E. (2022). An overview of pathogens associated with biotic stresses in hemp crops in oregon 2019 To 2020. Plant Dis. 106, 1334–1340. doi: 10.1094/PDIS-11-21-2415-SR
Rodríguez-Álvarez, M. X., Boer, M. P., van Eeuwijk, F. A., Eilers, P. H. C. (2018). Correcting for spatial heterogeneity in plant breeding experiments with p-splines. Spat. Stat. 23, 52–71. doi: 10.1016/j.spasta.2017.10.003
Rondon, S. I., Roster, M. S., Hamlin, L. L., Green, K. J., Karasev, A. V., Crosslin, J. (2016). Characterization of Beet curly top virus strains circulating in beet leafhoppers (Hemiptera: cicadellidae) in northeastern Oregon. Plant Dis. 100, 1586–1590. doi: 10.1094/PDIS-10-15-1189-RE
Saballos, A., Soler-Garzón, A., Brooks, M., Hart, J. P., Lipka, A. E., Miklas, P., et al. (2022). Multiple genomic regions govern tolerance to sulfentrazone in snap bean (Phaseolus vulgaris l.). Front. Agron. 4. doi: 10.3389/fagro.2022.869770
Scheet, P., Stephens, M., Scheet, M. P. (2006) A fast and flexible statistical model for large-scale population genotype data: applications to inferring missing genotypes and haplotypic phase. Available at: www.ajhg.org (Accessed January 16, 2021).
Schmutz, J., McClean, P. E., Mamidi, S., Wu, G. A., Cannon, S. B., Grimwood, J., et al. (2014). A reference genome for common bean and genome-wide analysis of dual domestications. Nat. Genet. 46, 707–713. doi: 10.1038/ng.3008
Silbernagel, M. J., Morales, F. J. (2005). “Curly top virus,” in Compendium of bean diseases. Ed. Schwartz, H. F. (St. Paul, MN:American Phytopathological Society) Pages 77–79.
Singh, S. P., Schwartz, H. F. (2010). Breeding common bean for resistance to diseases: a review. Crop Sci. 50, 2199–2223. doi: 10.2135/cropsci2009.03.0163
Soler-Garzón, A., Oladzadabbasabadi, A., Beaver, J., Beebe, S., Lee, R. K., Lobaton, J., et al. (2021). NAC candidate gene marker for bgm-1 and interaction with QTL for resistance to bean golden yellow mosaic virus in common bean. Front. Plant Sci. 12. doi: 10.3389/FPLS.2021.628443
Song, Q., Jia, G., Hyten, D. L., Jenkins, J., Hwang, E.-Y., Schroeder, S. G., et al. (2015). SNP assay development for linkage map construction, anchoring whole genome sequence and other genetic and genomic applications in common bean. Genes Genomes Genet. 5, 2285–2290. doi: 10.1534/g3.115.020594
Stenger, D. C., Carbonaro, D., Duffus, J. E. (1990). Genomic characterization of phenotypic variants of beet curly top virus. J. Gen. Virol. 71, 2211–2215. doi: 10.1099/0022-1317-71-10-2211
Stenger, D. C., Stevenson, M. C., Hormuzdi, S. G., Bisaro, D. M. (1992). A number of subgenomic DNAs are produced following agroinoculation of plants with beet curly top virus. J. Gen. Virol. 73, 237–242. doi: 10.1099/0022-1317-73-2-237
Strausbaugh, C. A., Eujayl, I. A., Wintermantel, W. M. (2017). Beet curly top virus strains associated with sugar beet in Idaho, Oregon, and a Western U.S. collection. Plant Dis. 101, 1373–1382. doi: 10.1094/PDIS-03-17-0381-RE
Strausbaugh, C. A., Wintermantel, W. M., Gillen, A. M., Eujayl, I. A. (2008). Curly top survey in the western united states. Phytopath. 98, 1212–1217. doi: 1094/PHYTO-98-11-1212
Swisher Grimm, K. D., Crosslin, J. M., Cooper, W. R., Frost, K. E., du Toit, L. J., Wohleb, C. H. (2021). First report of curly top of Coriandrum sativum caused by Beet curly top virus in the Columbia basin of Washington state. Plant Dis. 105, 3313. doi: 10.1094/PDIS-01-21-0041-PDN
Thomas, H. R., Zaumeyer, W. J. (1953). “Developing healthier vegetables,” in 1953 yearbook of agriculture (Washington, D.C: U.S. Department of Agriculture), Pages 493–508.
Varsani, A., Martin, D. P., Navas-Castillo, J., Moriones, E., Hernández-Zepeda, C., Idris, A., et al. (2014). Revisiting the classification of curtoviruses based on genome-wide pairwise identity. Arch. Virol. 159, 1873–1882. doi: 10.1007/s00705-014-1982-x
Wallace, L., Arkwazee, H., Vining, K., Myers, J. R. (2018). Genetic diversity within snap beans and their relation to dry beans. Genes 9, 587. doi: 10.3390/genes9120587
Wang, J., Chuang, K., Ahluwalia, M., Patel, S., Umblas, N., Mirel, D., et al. (2005). High-throughput SNP genotyping by single-tube PCR with Tm-shift primers. Biotechniques 39, 885–893. doi: 10.2144/000112028
Yin, L. (2016) CMplot. Available at: https://github.com/YinLiLin/CMplot (Accessed March 17, 2021).
Keywords: geminivirus, dry bean, exonuclease V, genome-wide association study, marker-assisted selection, snap bean
Citation: Soler-Garzón A, Goldoff D, Thornton A, Swisher Grimm KD, Hart JP, Song Q, Strausbaugh CA and Miklas PN (2023) A robust SNP-haplotype assay for Bct gene region conferring resistance to beet curly top virus in common bean (Phaseolus vulgaris L.). Front. Plant Sci. 14:1215950. doi: 10.3389/fpls.2023.1215950
Received: 02 May 2023; Accepted: 12 June 2023;
Published: 14 July 2023.
Edited by:
Maeli Melotto, University of California, Davis, United StatesReviewed by:
Maria Celeste Gonçalves-Vidigal, Universidade Estadual de Maringá, BrazilYheni Dwiningsih, University of Arkansas, United States
Kelvin Kamfwa, University of Zambia, Zambia
Copyright © 2023 Soler-Garzón, Goldoff, Thornton, Swisher Grimm, Hart, Song, Strausbaugh and Miklas. This is an open-access article distributed under the terms of the Creative Commons Attribution License (CC BY). The use, distribution or reproduction in other forums is permitted, provided the original author(s) and the copyright owner(s) are credited and that the original publication in this journal is cited, in accordance with accepted academic practice. No use, distribution or reproduction is permitted which does not comply with these terms.
*Correspondence: Phillip N. Miklas, cGhpbC5taWtsYXNAdXNkYS5nb3Y=
†ORCID: Phillip N. Miklas, orcid.org/0000-0002-6636-454X