- 1Research Center for Genetic Engineering, National Research and Innovation Agency, Bogor, West Java, Indonesia
- 2Research Center for Biotechnology, Universitas Gadjah Mada, Yogyakarta, Indonesia
- 3Department of Agronomy, Faculty of Agriculture, Universitas Gadjah Mada, Yogyakarta, Indonesia
- 4Department of Tropical Biology, Faculty of Biology, Universitas Gadjah Mada, Yogyakarta, Indonesia
The effects of exogenously supplied osmoprotectants in crops have not yet been extensively studied. In this study, an osmoprotectant containing a high concentration of proline (2.5 g mol−1 FW) was obtained from a Casuarina equisetifolia leaf extract. The effect of the extract was evaluated in local Indonesian rice cultivars Boawae Seratus Malam (BSM), Gogo Jak (GJ), Situ Bagendit (SB) (drought-tolerant), Kisol Manggarai (KM) and Ciherang (drought-susceptible) cultivars under drought at the morphological, physiological, and genetic levels. Under drought, the KM showed an increased level of OsWRKY, OsNAC, OsDREB1A, and OsDREB2A expression after application of the osmoprotectant, leading to the activation of proline synthesis genes including OsP5CS1, OsP5CR, and OsProDH, while the tolerant cultivars (BSM, GJ, and SB) showed no difference. The content of chlorophyll, carotenoids, anthocyanins, ascorbate peroxidase, catalase, and superoxide dismutase activities also increased in GJ and KM, during drought stress and applied osmoprotectants, but remained low in the BSM. We conclude that the foliar application of osmoprotectants derived from C.equisetifolia caused an accumulation of proline in susceptible plants. The existence of these extracts stabilizes leaf cells and supports photosynthetic compartments and carbon assimilation in plants, leading to growth.
Introduction
Rice (Oryza sativa L.) is the world’s largest food crop, mostly cultivated in Asia (Aslam et al., 2022). Increasing the global production of rice now and in the future has many challenges, including limited land and water availability due to drought (Dawe, 2013; Shahzad et al., 2020). There are several strategies to mitigate the threat of drought stress, such as screening drought-tolerant cultivars, designing water-efficient planting, and applying protectant compounds and biostimulants that can induce plant tolerance to drought (Bertolino et al., 2019; Upadhyay, 2019; Aslam et al., 2022).
Different ways of using plant extracts to prevent the effects of drought have been studied. Research by Pandey et al. (2016) showed that the application of an Ocimum extract increased the expression of the Dehydrin gene, while the expression of the Aquaporin gene was decreased in drought-stressed rice plants. Application of other plant extracts including kirinyuh leaves (Chromolaena odorata) has no significant effect on shoot length, fresh weight, or dry weight of rice seedlings, but affects the relative water content of rice seedlings (Wahyuni et al., 2018). Another study (Lalarukh et al., 2022) showed that organic extracts from Moringa leaves can induce immunity in plants under nutritional and drought stress to increase their survival. Providing plant extracts is one way to increase crop productivity in arid areas through organic methods, but the regulatory impact of these extracts as osmoprotectants on crop plants under drought has not been sufficiently elucidated.
Drought stress threatens plants in an extremely harmful way by causing abnormal growth, reducing water use efficiency, and disrupting physiological processes through restricted water transport (Kulkarni et al., 2017). Drought stress decreases turgescence and damages the osmotic balance of plants (Hassanein et al., 2021). To survive, plants have evolved various responses as adaptations to drought stress conditions. Adaptation strategies include the development of an extended root system (Kim and Lee, 2020), development of smaller and thicker leaves (Bertolino et al., 2019; Salsinha et al., 2021), increased diffusion resistance (Darmadi et al., 2021), reduced water loss through leaf wilting (Hassanein et al., 2021), and reduction in transpiration rate (Farooq et al., 2009; Aslam et al., 2022).
Drought tolerance is a complex property of plants that result from several genetics, morphological, biochemical, physical, and physiological adaptive traits (Aslam et al., 2022). At the transcriptional level, the regulatory response to drought stress involves members of several transcription factor (TF) families, such as WRKY (a 60-amino-acid region that is defined by the conserved amino acid sequence WRKYGQK at its N-terminal end), NAC (NAM, ATAF1/2, and CUC1/2), and AP2 (APETALA2)/ERF (ethylene-responsive factor) (Kulkarni et al., 2017; Wu et al., 2022). One AP2 subfamily is the DREBs (dehydration-responsive element-binding proteins) (Baillo et al., 2019; Herawati et al., 2021).
At the metabolic level, enzymatic and non-enzymatic oxidative reactions are mandatory for the protection against drought stress. Another protective approach for the osmotic adjustment of plants is the application of osmoprotectant (Chen et al., 2020). Organic compounds used as osmoprotectants are proline (Pro), glycine betaine (GB), and dissolved sugars (Cha-um et al., 2013). Their accumulation lowers the intracellular water potential. In addition, Pro also acts as a metabolic marker against drought stress (Islam et al., 2022), thereby stabilizing the intracellular and macromolecular structures of soluble and membrane-bound proteins in complexes during abiotic stress (Nounjan et al., 2012; Herawati et al., 2021; Shemi et al., 2021).
This protective effect Pro and GB in rice are also achieved by their exogenous supply to plants under drought stress (Hasanuzzaman et al., 2014; Shemi et al., 2021). The application of foliar osmoprotectants, such as polyamine, Pro, ABA, GB, GA3, sugar compounds, and salicylic acid, can increase turgor pressure and induce antioxidant activity against ROS (reactive oxygen species) (Chen et al., 2020; Aslam et al., 2022; Atteya et al., 2022).
Exogenous GABA application (Yong et al., 2017) activates 1-pyrroline-5-carboxylate synthetase (P5CS) activity, which leads to Pro accumulation and elevated proline dehydrogenase (PRODH) activity in periods of prolonged drought. Based on their increased antioxidant defense, plants under high-salinity stress revealed a better protective function for Pro than for GB (Hasanuzzaman et al., 2014). Regarding productivity, Farooq et al. (2008) showed that supplemented GB to plants potentially maintains optimal growth and even increases the productivity of rice plants. Foliar application of 100 mM GB also stabilizes plant metabolic processes and increases productivity (Jalal-ud-Din et al., 2015). The application of Pro and sucrose on rice grains also improved the flavor quality and nutrient content of rice (Arsa et al., 2016).
The molecular action of Pro as an osmoprotectant has not extensively been studied, particularly with respect to its roles as a free radical scavenger, growth promoter, enzyme activator, osmoprotectant in abiotic stress, and as a regulatory factor in other physiological processes (Pandey and Shukla, 2015; Hosseinifard et al., 2022). The objective of the present study was to analyze the specific impact of an exogenously applied Pro-rich leaf extract as a source of osmoprotectants for local rice cultivars in Indonesia. A crude leaf extract was derived from the halophyte plant Casuarina equisetifolia. We hypothesized that this plant extract with a higher Pro content would be an adequate source of osmoprotectants for the improved adaptation of local rice plants with increased growth rate and elevated resistance in drought. The effect of applied exogenous osmoprotectant could be observed at the expression level of genes related to the proline synthesis pathway as well as to other physiological processes downstream from the Pro accumulation.
Materials and methods
Materials used and research design
Five rice cultivars (Table 1) were used. Cultivation and treatment were carried out in a greenhouse of the Sawitsari Research Station (7°45’22” latitude, 110°23’18” longitude), Faculty of Biology, Universitas Gadjah Mada Yogyakarta, Indonesia with the air temperature ranging between 29–34°C (day) and 21–25°C (night) during January–June, photosynthetic photon flux density about 500 µmol m−2 s−1, air humidity >80%, and average rainfall 300–400 mm.
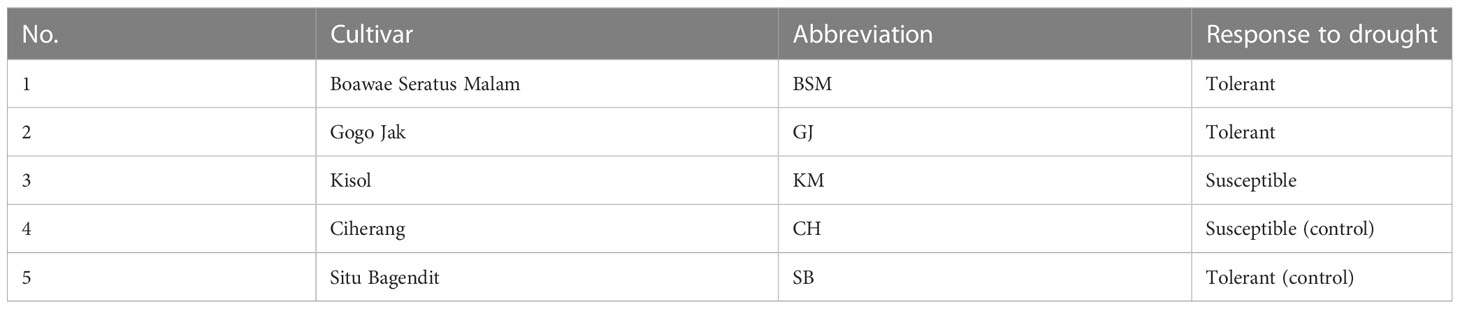
Table 1 Five rice (Oryza sativa, indica) cultivars (Salsinha et al., 2021).
The research design was a randomized complete block design with three variables: cultivar (five cultivars), drought stress treatment (two levels), and exogenous osmoprotectant treatment (three concentrations) with three replications, consisting of one individual plant each. Each block of 60 cm × 100 cm contained 15 individual plants of different cultivars at random positions. The sowing period lasted until the plants were 21 days old, at which point they were planted and drought stress treatment was applied until the plants reached the final vegetative stage at 49 DAPs (days after planting); the foliar osmoprotectant treatment was carried out at 21 DAP.
Drought stress treatment
The drought stress treatment followed the IRRI protocol (Serraj et al., 2008) by applying the FTSW (fraction of transpirable soil water). Before the FTSW treatment, the total transpiration of soil water (TTSW) of each different cultivar was analyzed. From the TTSW value, the exact weight of pot and plant at each FTSW was calculated. Two levels of FTSW were used: FTSW 1 for control and FTSW 0.2 for drought stress. The TTSW value was separately determined by calculating the difference of pot and plant weight at 100% field capacity (P0) with the weight of the pot and plant when the plant shows a permanent wilting point (Pi) character with Eq. 1 (Salsinha et al., 2022):
During the treatment, the amount of soil water (Wt) kept stable was calculated for each FTSW stage for each cultivar using Eq. 2. The weight of soil and pots (Pt) during the treatment period was kept stable using Eq. 3.
Exogenous osmoprotectant treatment
Exogenous osmoprotectant was obtained from a crude leaf extract of C. equisetifolia. GB and Pro are highly concentrated compounds in C. equisetifolia leaf extract that can enter and accumulate in rice tissue and can act as an osmoprotectant (Basuki and Prajitno, 2014). The leaves were sampled from the coastal area of Gunung Kidul, D.I. Yogyakarta, Indonesia. Physicochemical parameters during sampling included a relative humidity of 77% and an atmospheric pressure of 987–988 hPa.
C. equisetifolia leaves (5 kg) were crushed, ground, and homogenized with 5 L of ddH2O to obtain 2.5 L of leaf crude extract. The extract was filtered until the solution was clear yellow. Pro content was then measured and adjusted to 2.5 g mol−1 FW Pro as a stock crude extract (Bates et al., 1973). The stock was then diluted with ddH2O to three different Pro concentration levels: 50% (1.25 g mol−1 FW), 100% (2.5 g mol−1 FW), and a control without extract (0%). The leaf extract was stored overnight at 4°C to precipitate insoluble material in the filtrate and obtain a clear supernatant. The solution was mixed with a solution-grading [containing the active ingredients of polyacrylamide 75 g L−1 and polyvinyl acetate (PVAc) 75 g L−1] in a ratio of 1:1,000 (1 ml of solution-grading in 1 L of leaf extract). The extract was then applied to the abaxial surface of the rice leaf using a sprayer, with a total volume of 15 ml applied per block. Exogenous osmoprotectant was applied once when the plants had been treated with FTSW drought stress for 21 days (vegetative period).
Relative gene expression analysis
Total RNA was isolated from 100 mg of young leaves per treatment using a FavorPrep Plant RNA Mini Kit 001-1 (Ping Tung Agricultural Biotechnology Park, Taiwan), after homogenization of the leaves using FARB buffer containing β-mercaptoethanol. The sample was incubated at room temperature for 5 min, followed by homogenization with 70% ethanol. The filtrate was separated by centrifugation, and the pellet was washed successively with wash buffers 1 and 2 containing 96% ethanol. RNA was dissolved in 50 μl of nuclease-free water and its purity was measured using a NanoDrop spectrophotometer. Before cDNA synthesis, RNA was further purified with a DNase I treatment kit (Sigma-Aldrich, Germany). cDNA was synthesized using an Excel RT Kit II (SMOBIO Technology, Taiwan). For gene expression analysis, the concentration of cDNA was adjusted to 500 ng µl−1. Primers for the targeted and reference genes are shown in Table 2.
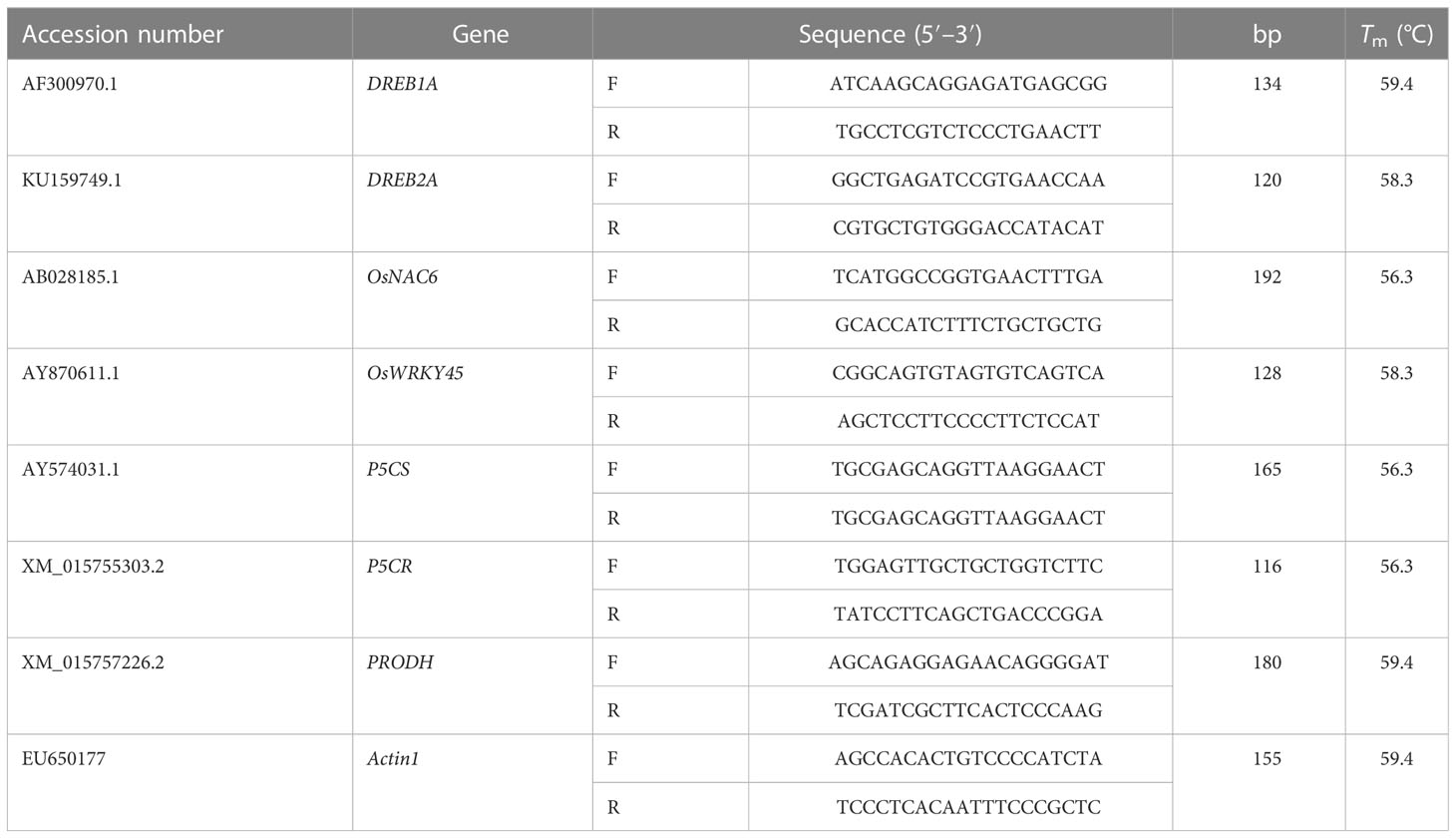
Table 2 Targeted and reference gene primer sequences (Salsinha et al., 2022).
Gene expression analysis was performed following the Q-PCR Master Mix (SYBR, no ROX) protocol (SMOBIO Technology) with a quantitative real-time PCR machine (Bio-Rad CFX96) (Salsinha et al., 2022). The sample mix contained 1 µl of cDNA template, 1 µl of forward primer, 1 µl of reverse primer, 5 µl of 2 -PCR Master Mix, and 2 µl of ddH2O. The PCR program consisted of a 2-min incubation at 95°C, followed by 39 cycles of 94°C denaturation for 30 s, annealing for 1 min at the Tm (melting temperature) of each targeted gene, and 72°C extension for 30 s, with subsequent storage at 4°C. A duplo–duplo experiment was used for the relative quantification of gene expression levels. Calculation of the relative expression level of each gene in each treatment followed the formula (Hong-zheng et al., 2017):
ΔCt unknown sample=Ct internal reference gene-Ct target gene
ΔCt calibrator=Ct reference gene in ref sample-Ct target gene in ref sample
ΔΔCt=ΔCt unknown sample-ΔCt calibrator
Relative expression=2^(-ΔΔCt)
Physiological parameter measurement
Photosynthetic pigment measurement
Total chlorophyll and carotenoids were analyzed by the method of Harborne (1984). A total leaf sample of about 30 mg was homogenized with 80% cold acetone in the dark. The filtrate’s absorbance was measured at 470, 645, and 664 nm. Anthocyanins were measured by the Lotkowska method (Lotkowska et al., 2015). A leaf sample (250 mg) was ground with 1 ml of buffer containing 1-propanol, 37% HCl, and ddH2O. The sample was incubated at 95°C for 5 min and then at room temperature for 2 h. Absorbance was measured at 535, 620, and 720 nm.
Determination of Pro content
About 0.25 g of leaf samples was used to measure Pro content (Bates et al., 1973). The ground sample was homogenized with 3% sulfosalicylic acid solution (~5 ml) and filtered with Whatman No. 1 paper. Acetic and ninhydrin acid solutions were added to the sample at a ratio of 1:1:1. Samples were then incubated for 1 h at 94°C followed by ice-cooling. Two milliliters of toluene was added to the cold samples and mixed for 10 s. The absorbance of the colored phase (upper layer) was measured at 520 nm (GENESYS 10 UV Scanning, Thermo Fisher Scientific). Pro content was determined with a standard proline curve and calculated according to:
Enzymatic antioxidant measurement
Enzymatic antioxidant activity analysis for superoxide dismutase (SOD), ascorbate peroxidase (APX), and catalase (CAT) began with enzyme extraction following published protocols and measurements (Elevarthi and Martin, 2010). Approximately 0.2 g of ground leaves were homogenized with ~2 ml of 50 mM potassium phosphate buffer (pH 7.0) containing 1 mM EDTA and 1% PVP. The supernatant was kept below 4°C during measurement.
SOD activity was measured according to a previous protocol (Marklund and Marklund, 1974). The extract was prepared in a buffer containing 0.1 mM EDTA and Tris-HCl (pH 8.2) at room temperature. First, the absorbance of pyrogallol was measured as a control, followed by the sample with pyrogallol as blank. The enzymatic reaction was initiated by the addition of ddH2O and 4.5 mM pyrogallol. The absorbance was measured at 325 nm for 3 min. SOD activity was expressed as 50% pyrogallol autooxidation inhibition during incubation. Enzyme activity was calculated according to the formula:
where Δtest = sample absorbance, Δ control = pyrogallol absorbance, tV (ml) = total assay volume (buffer + ddH2O + pyrogallol + enzyme sample), V (ml) = volume of enzyme sample, and D = dilution factor.
APX activity was measured by mixing 100 µl of enzyme extract with a buffer containing 0.05 mM sodium phosphate, 0.1 mM EDTA, 0.05 mM ascorbic acid, and ddH2O (Elevarthi and Martin, 2010). The enzymatic reaction was started by adding 0.8 ml of 3% (v/v) hydrogen peroxide (H2O2) solution, and the absorbance was measured for 3 min at 290 nm and calculated according to:
where ΔAbs = absorbance change during t (min), tV (ml) = total assay volume (buffer reagent + enzyme sample), Δt = time (3 min), extinction coefficient (2.8 mM−1 cm−1), V (ml) = volume of enzyme sample, L = cuvet diameter (1 cm).
CAT activity was measured according to a published protocol (Elevarthi and Martin, 2010) by reacting 200 µl of the enzyme extract with a buffer containing 50 mM sodium phosphate (pH 7.0). A solution of 3% H2O2 was added to start the reaction, followed by incubation at room temperature for 1 min. The absorbance was measured at 240 nm for 3 min and CAT activity was expressed as the decrease in H2O2 per minute per mg protein:
where ΔAbs = absorbance change during t, tV (ml) = total assay volume (buffer reagent + enzyme sample), Δt = time (3 min), = extinction coefficient (40 mM−1 cm−1), L = cuvet diameter (1 cm), FW = fresh weight (g).
The H2O2 content was measured using the method of Bouazizi et al. (2007). Leaf samples (0.25 g) were ground with 0.1% TCA. The supernatant (0.5 ml) was reacted with 10 mM ferrous ammonium sulfate, 2.5 M potassium thiocyanate, and 50% TCA, followed by incubation at room temperature for 1 min. The absorbance was measured at 390 nm. The hydrogen peroxide content was determined by comparing it with the standard H2O2 curve.
where FW = fresh weight (g) and D = dilution factor.
Morphological parameter analysis
The plant morphological parameters measured included plant height, number of tillers, number of leaves, and root length measured at 49 DAPs. Plant height was measured from the uppermost leaf to the base of the stem at the soil surface. The number of tillers was counted from the base above the soil surface that showed growth of more than two leaves. The total number of leaves measured was the leaves that had opened on each tiller. The longest root length was measured from the base near the soil surface to the longest root tip. The fresh weight of roots and shoots was measured separately using analytical scales. Each shoot and root part were then stored in an oven at 65°C until the weight became constant. The dry weight of each root and shoot was then measured.
Statistical analysis
The data obtained were analyzed for significant differences between treatments using a multivariate two-way ANOVA. Differences between groups were analyzed using Duncan’s multiple range test at a 95% confidence interval. Correlation between parameters was determined with Pearson’s r correlation analysis using SPSS (IBM-SPSS Ver 25.00.US). Data visualization was performed using GraphPad Prism 9.3.1.
Results
Expression of transcription factor and proline-related genes
In this study, osmoprotectants derived from the halophyte plant C. equisetifolia were extracted. From the test results that have been carried out, it can be observed at the expression level of TFs and genes related to the proline synthesis pathway as well as other physiological processes downstream of Pro accumulation.
Figure 1 shows the expression levels of drought-responsive transcription factors (OsWRKY45, OsNAC6, OsDREB1A, and OsDREB2A) as a result of drought stress treatment and exogenously applied osmoprotectant in the local rice cultivars BSM, GJ, and KM. Figure 1A shows that in the BSM cultivars, exposure to exogenous osmoprotectants at low and medium concentrations did not significantly increase OsWRKY45 expression in GJ cultivars, while in KM cultivars, an increase in the concentration of exogenous osmoprotectants led to an increased level of OsWRKY45 expressions, with the highest level shown in plants under drought stress.
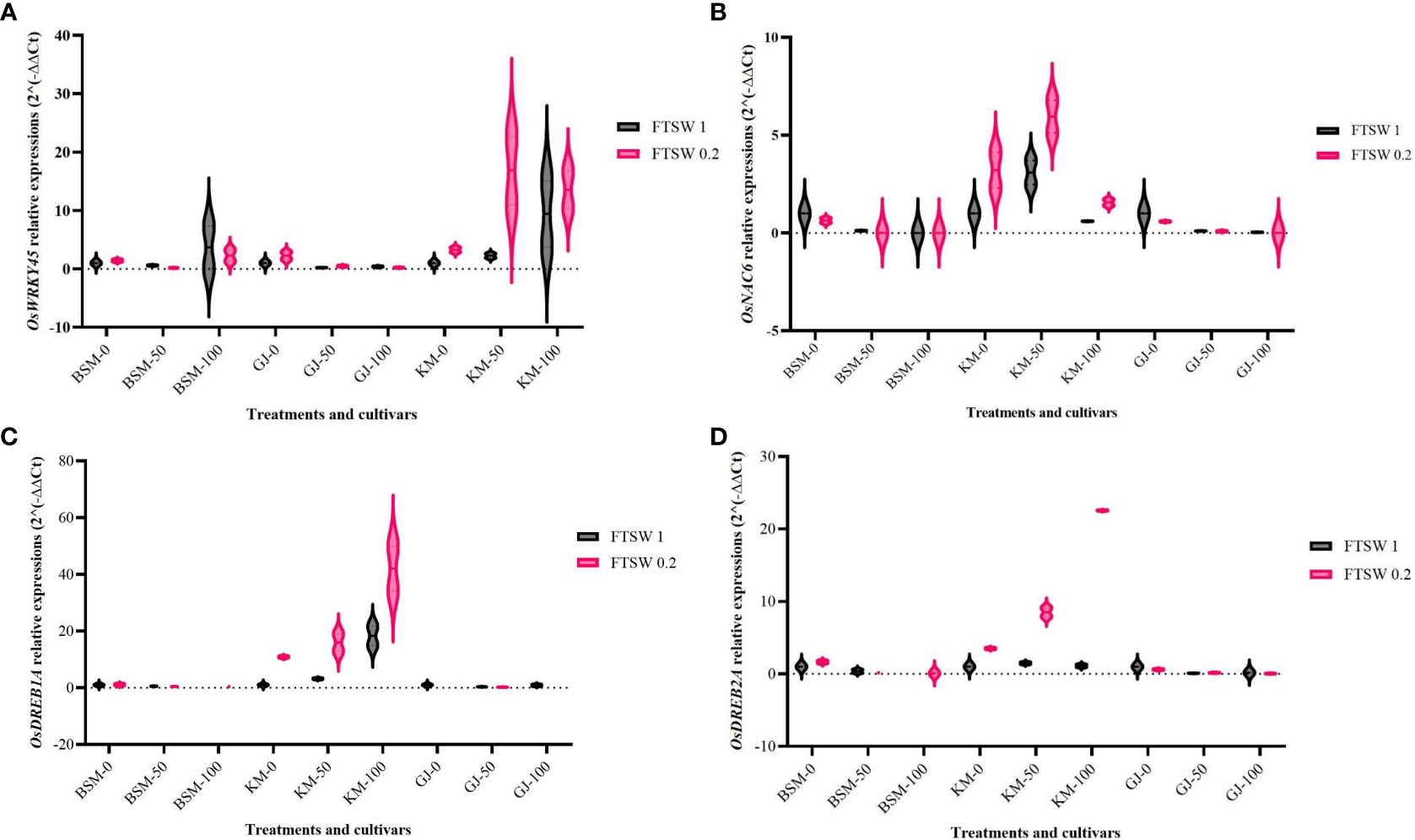
Figure 1 Expression level of (A) OsWRKY, (B) OsNAC6 (C) OsDREB1A, and (D) OsDREB2A in Oryza sativa leaves after treatment with two levels of FTSW (fraction of transpirable soil water), FTSW 1 (control) and FTSW 0.2 (drought stress), and three levels of exogenous osmoprotectant application, 0% (low), 50% (moderate), and 100% (high), in the cultivars BSM, KM, and GJ. A duplo–duplo experiment with three replications each was used to express the relative quantification of gene expression levels with three replications of each treatment group.
High levels of OsNAC6 expression was found in BSM and GJ cultivars treated with a lower concentration of osmoprotectants (Figure 1B). The gene’s expression in BSM and GJ decreased as the concentration of osmoprotectants increased. KM cultivars showed an increase in the expression level of OsNAC6 under drought stress and moderate osmoprotectants. The application of high concentrations of osmoprotectants caused a decrease in the expression level of OsNAC6 in KM.
Figure 1C shows the change in the expression level of OsDREB1A. KM cultivars showed an increase in the expression level of OsDREB1A in line with the increasing concentration of osmoprotectants under drought stress. The highest expression level of OsDREB1A was observed in KM plants subjected to drought stress. Figure 1D shows an increasing expression level of OsDREB2A in KM cultivar with the increase in the concentration of osmoprotectants but not with 100% osmoprotectant. In BSM and GJ, neither drought stress nor the application of osmoprotectant resulted in significant changes in the expression level of OsDREB2A.
The expression of the genes encoding TFs OsWRKY45, OsNAC6, OsDREB1A, and OsDREB2A is induced by drought stress independently whether osmoprotectants are applied or not. The application of exogenous osmoprotectants mainly increased the expression levels of these TFs in the KM cultivar under drought conditions. Furthermore, TFs play a role in activating gene expression in the proline metabolic pathway (Zhang et al., 2013).
Pro metabolism involves the activity of several functional genes. Increased proline accumulation requires activation of several genes involved in Pro synthesis—OsP5CS encodes P5CS (Δ 1-Pyrroline-5-carboxylate synthetase), while OsP5CR encodes P5CR (Δ 1-Pyrroline-5-carboxylate reductase), which functions in the proline synthesis pathway via glutamate (Kavi Kishor et al., 2005; Salsinha et al., 2022). Proline catabolism is carried out by PRODH (proline dehydrogenase), which is encoded by the OsProDH (Luo et al., 2020; Salsinha et al., 2022). We examined the transcript accumulation of the OsP5CS1, OsP5CR, and OsProDH genes in the rice cultivars under control and drought stress condition in combination with supplied osmoprotectants.
The expression levels of OsP5C1 increased only in KM treated with osmoprotectant during drought stress (Figure 2A). Analysis of variance showed that the expression of OsP5C1 was significantly different (p< 0.05) between KM cultivars and other cultivars. The OsP5CR expression of KM during drought stress was elevated in comparison to the control conditions and in comparison to the other cultivars during drought (Figure 2B). Drought stress combined with 50% exogenous osmoprotectant in KM showed the highest OsP5CR expression level (more than ninefold). The expression of the OsProDH gene in KM cultivar increases in both drought stress and 100% applied osmoprotectant (Figure 2C). Under control conditions, KM showed decreased expression of OsProDH when exposed to high concentrations of osmoprotectants.
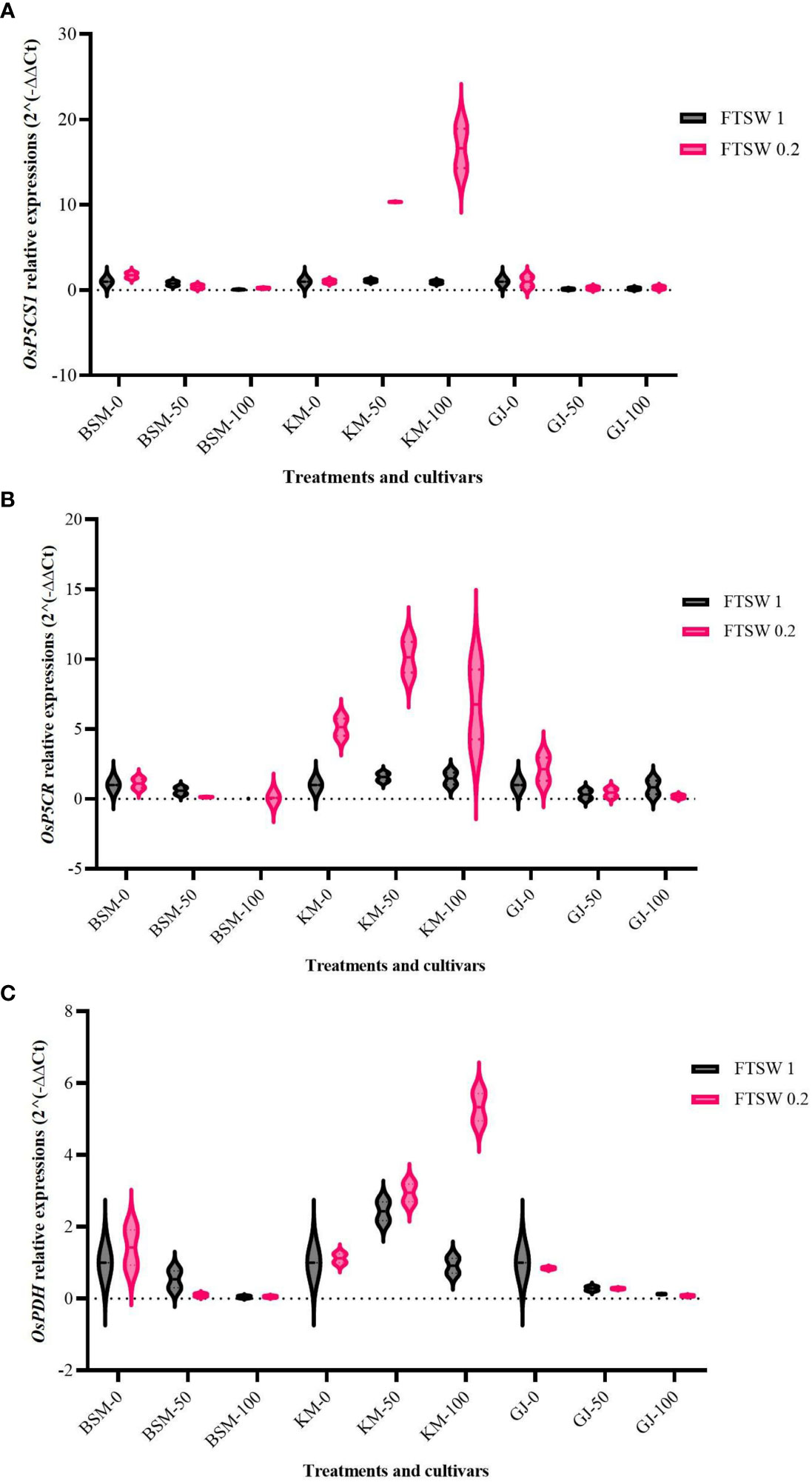
Figure 2 Expression levels of (A) OsP5CS1, (B) OsP5CR, and (C) OsProDH, in the three cultivars BSM, KM, and GJ grown at one of the two levels of FTSW and treated with three different concentrations of exogenous osmoprotectant. A duplo–duplo experiment with three replications each was used to express the relative quantification of gene expression levels with three replications of each treatment group.
Pro accumulation
Pro accumulation is induced by several processes during drought stress. In this study, proline contents were measured in plants after drought stress treatment and application of osmoprotectants. In this phase, the plants showed significant differences in proline accumulation (p< 0.05) in response to the different treatments (Figure 3). BSM cultivars exposed to drought stress were detected to have higher proline levels than the other cultivars at low concentrations of osmoprotectant exposure. The application of high amount of osmoprotectant under drought gives a significant difference of proline accumulation in BSM cultivars. In high concentrations of osmoprotectant treatment, BSM cultivars also showed the highest proline levels.
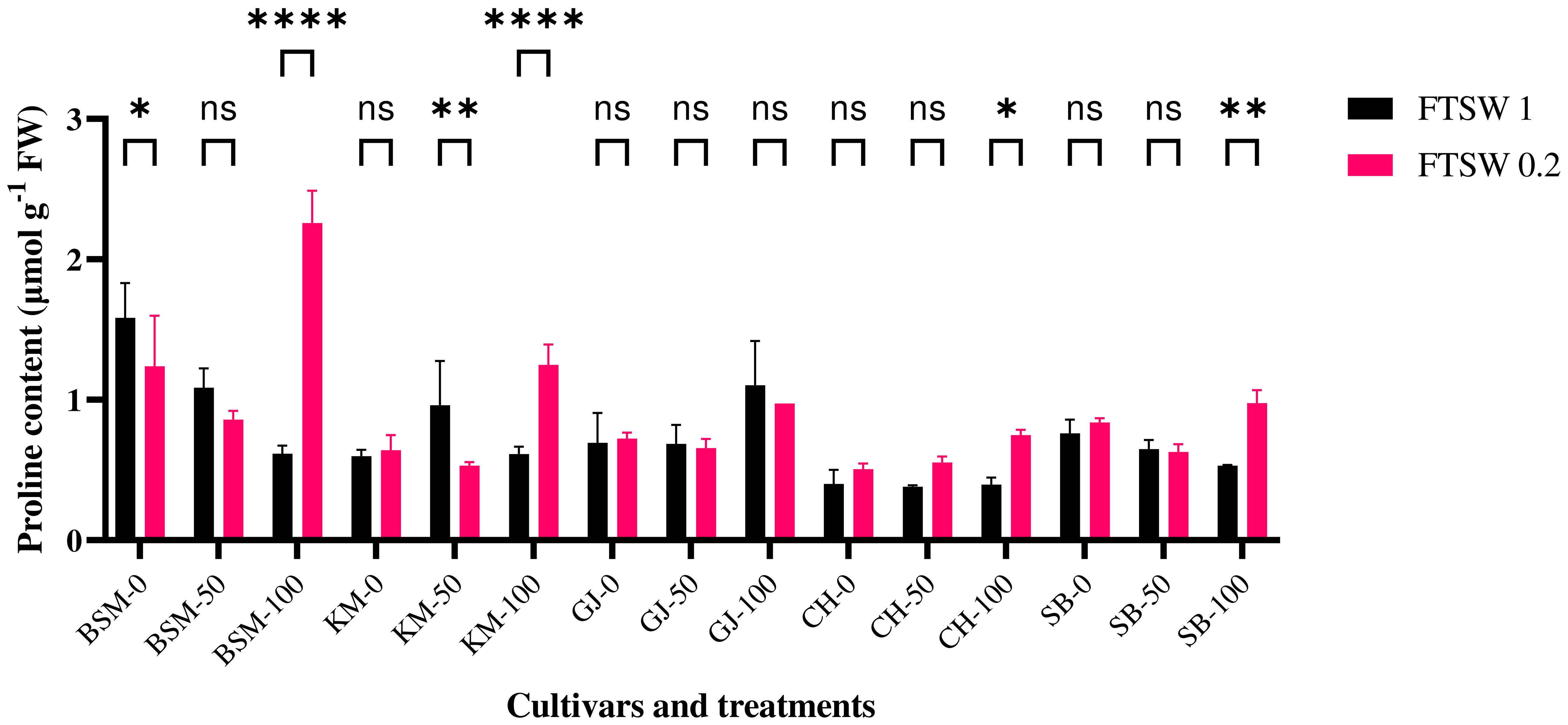
Figure 3 Differences in Pro levels at the end of drought stress treatment with FTSW 1 (control) and FTSW 0.2 (drought stress) in rice cultivars GJ, KM, BSM, CH (drought-susceptible control), and SB (drought-tolerant control) exposed to osmoprotectant concentrations of 0% (low), 50% (moderate), and 100% (high). ns=no significant difference with p value>0.05, * p value < 0.05, ** p value=0.02, **** p value < 0.0001 based on 95% confidence level in ANOVA two-way test with three replications each.
Oxidative responses of plants
Drought stress affects changes in the physiological characters of rice plants. At the same time, the application of osmoprotectants also has an impact on plant resistance to drought. Changes in the oxidative stress response of plants as a result of drought stress and osmoprotectant treatment are depicted in Figure 4. On exposure to low concentrations of osmoprotectants, BSM and SB cultivars showed a significantly different decrease in SOD activity (p< 0.05), while other cultivars showed an increase in activity in the exposure to the 50% osmoprotectant treatments.
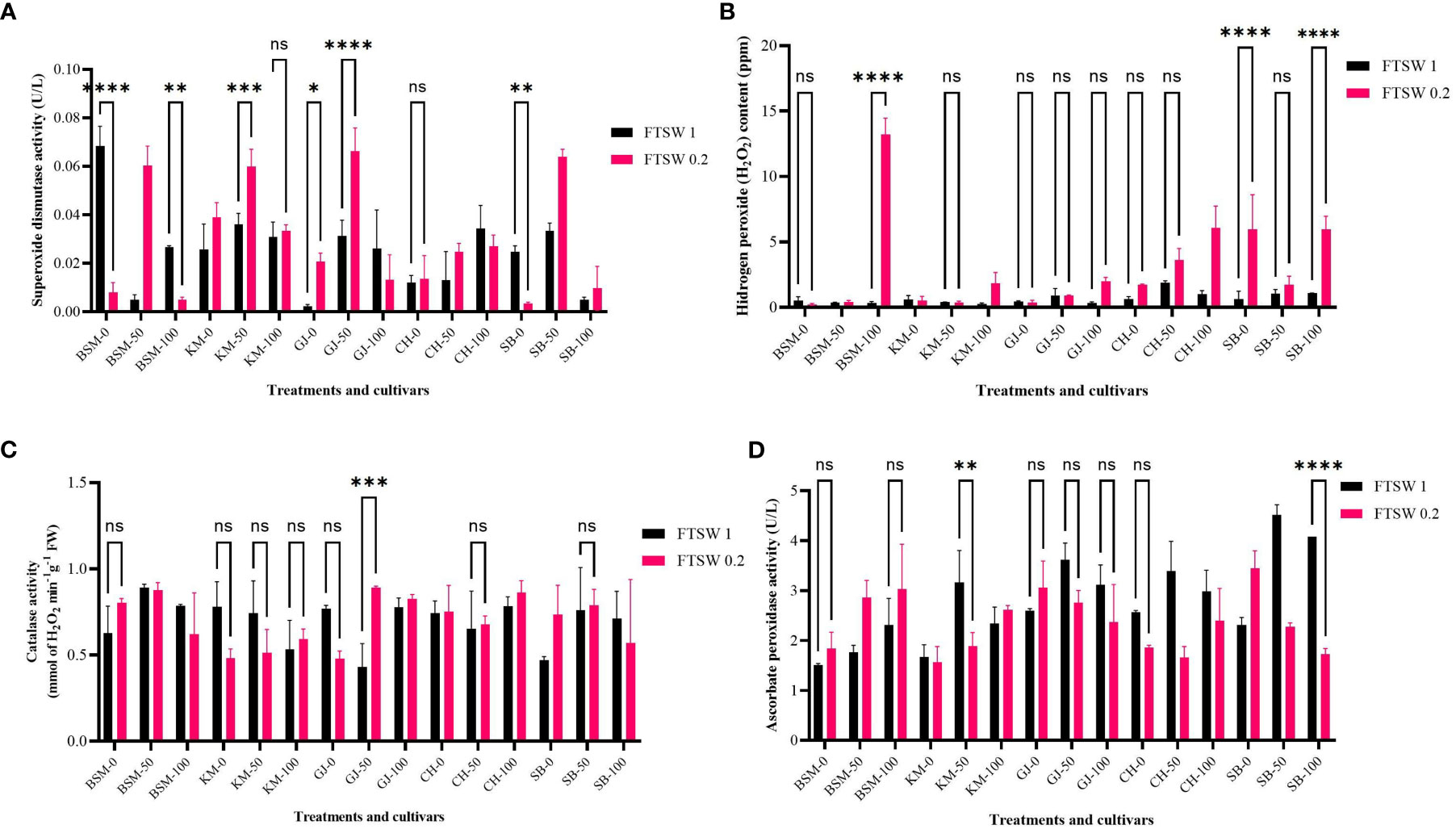
Figure 4 Enzymatic antioxidant activity of (A) SOD, (B) the product of SOD catalysis in the form of H2O2, and the catalytic activity of (C) APX and (D) CAT at the end of the drought stress treatment indicated by FTSW 0.2 and control (FTSW 1) in the rice cultivars GJ, KM, BSM, CH (drought-susceptible control), and SB (drought-tolerant control). The cultivars were exposed to osmoprotectant concentrations of 0% (low), 50% (moderate), and 100% (high). ns=no significant difference with p value>0.05, * p value < 0.05, ** p value=0.02, *** p value= 0.0001, **** p value < 0.0001 based on 95% confidence level in ANOVA two-way test with three replications each.
Figure 4B shows the different levels of H2O2 content. Exposure to low concentrations of osmoprotectants did not give a significant effect (p > 0.05) on H2O2 level changes in most of the cultivars. Non-significant differences (p > 0.05) were found in all cultivars on exposure to 50% osmoprotectant treatments. Exposure to high concentrations of osmoprotectants caused a substantial increase in H2O2 levels in BSM, CH, and SB.
ROS scavenging activity is also carried out by enzymes such as CAT and APX in all major H2O2 production compartments in the internal cell environment such as peroxisomes, mitochondria, cytosol, and chloroplast (You et al., 2019). Figure 4C significantly shows differences in APX activity among rice cultivars under drought stress and after osmoprotectant application. When exposed to 50% osmoprotectant, most cultivars showed significant differences (p< 0.05) between control and severe drought stress. The SB cultivars showed significant differences (p< 0.05) when exposed to high concentrations of osmoprotectants, while the other cultivars showed no significant differences between control and drought stress conditions.
CAT activity in Indonesia’s local rice exposed to drought stress and osmoprotectant application is shown in Figure 4D. On exposure to low concentrations of osmoprotectants, GJ and BSM showed a decrease in CAT activity. The BSM, CH, and SB cultivars showed a non-significant increase of CAT activity (p< 0.05) between control and drought stress. GJ plants on moderate osmoprotectant exposure displayed a significant increase (p< 0.05) in CAT activity. At 100% concentrations of osmoprotectants, there was no significant difference (p > 0.05) between control and drought stress conditions in any cultivar. Changes in enzymatic antioxidant activity affect the physiological performance of plants, which is reflected in changes in photosynthetic pigments.
Photosynthetic responses
The photosynthetic response of plants to environmental conditions correlates with the content of photosynthetic pigments. Figure 5 shows the content of the photosynthetic pigments chlorophyll a, chlorophyll b, carotenoids, and anthocyanins as an antioxidant compound. Chlorophyll a (Figure 5) generally decreased when plants were exposed to drought conditions in all cultivars. The deeper the loss of chlorophyll content between control and drought conditions, the stronger the susceptibility of a cultivar to drought stress.
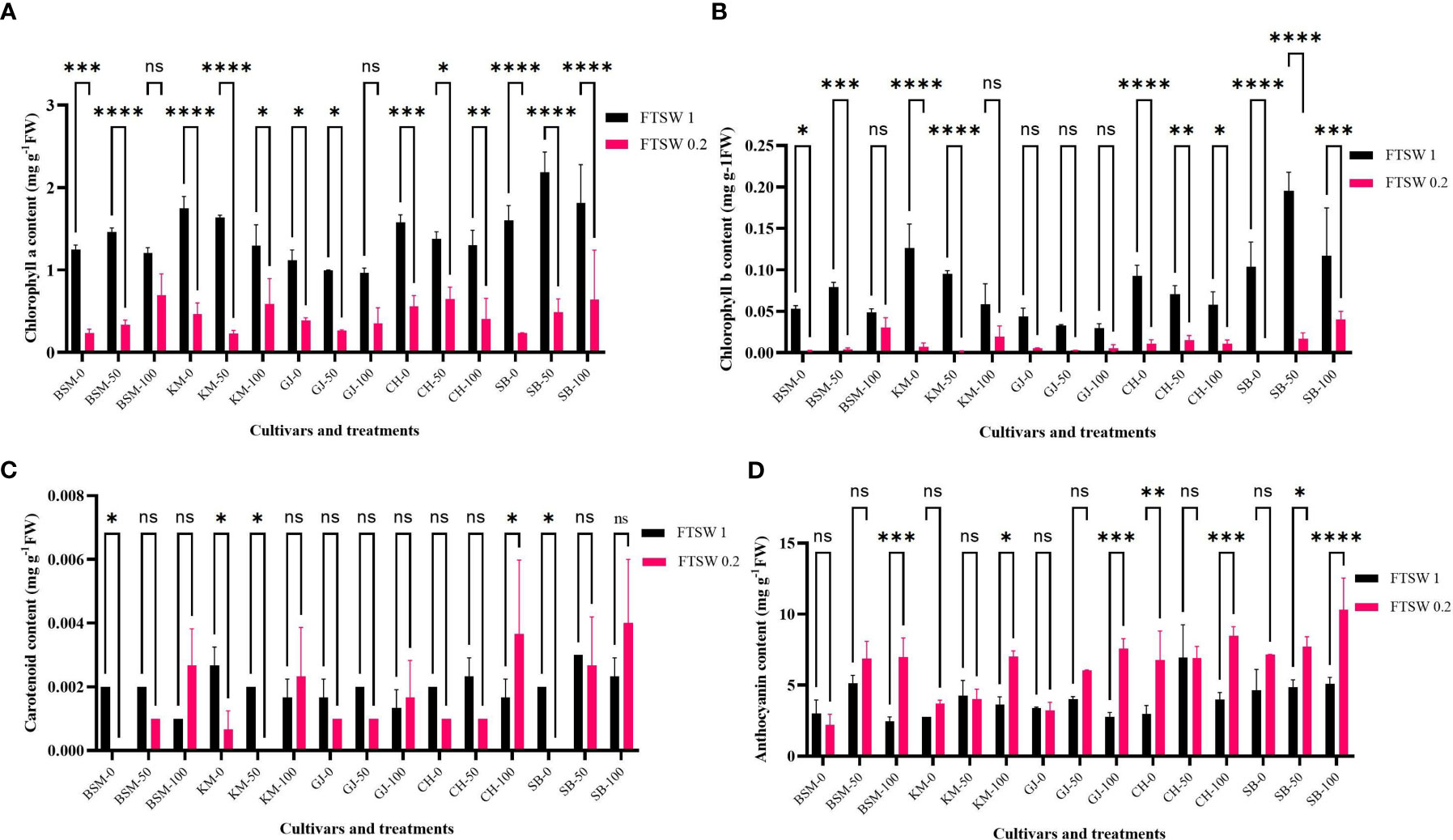
Figure 5 Differences in levels of photosynthetic pigments (A) chlorophyll a, (B) chlorophyll b, (C) carotenoids, and antioxidant pigment (D) anthocyanins of the rice cultivars GJ, KM, BSM, CH (drought-susceptible control), and SB (drought-tolerant control) at the end of the drought stress treatment with FTSW 1 (control) and FTSW 0.2 (drought stress). The cultivars were exposed to osmoprotectant concentrations of 0% (low), 50% (moderate), and 100% (high). Ns, no significant difference, *p< 0.05 based on 95% confident level in ANOVA two-way test with 3 replications each.
Figure 5 shows the differences in chlorophyll b between control and drought stress conditions in some cultivars exposed to osmoprotectants. In all cultivars, exposure to low concentrations of osmoprotectants caused a decrease in chlorophyll b level under drought stress. Under 100% concentrations of osmoprotectants, some cultivars showed changes in chlorophyll b level between control and drought conditions. All cultivars under the control condition showed a gradual decrease of chlorophyll b content except for SB, in which the content was higher concentration at the 100% concentration of exogenous osmoprotectant.
In addition to chlorophyll a and b, the levels of carotenoids also changed when plants were exposed to drought conditions and when the osmoprotectant was applied. On exposure to low concentrations of osmoprotectants (Figure 5), KM, BSM, and SB cultivars showed significant differences (p< 0.05) between control and drought stress conditions. CH showed a significant difference (p< 0.05) in carotenoid levels between control and drought stress conditions on exposure to 100% concentrations of osmoprotectants, while KM with 50% osmoprotectant also showed a significant difference (p< 0.05).
Anthocyanin is a plant pigment that plays a role in ROS scavenging. Anthocyanin levels (Figure 5) increased in cultivars exposed to drought stress and osmoprotectants. Exposure to osmoprotectants at low and 50% concentration levels did not affect anthocyanin levels of BSM, GJ, and KM cultivars under drought compared to control, except for CH. On exposure to high concentrations of osmoprotectants, cultivars GJ, KM, BSM, CH, and SB experienced an increase in anthocyanin levels relative to drought stress compared to control.
Morphological changes
Morphological characters are the result of the accumulation of plant physiological processes over time. Several studies have shown that root morphological characters play an important role in adaptation to drought (e.g., Liu et al., 2017). Plants with good adaptation mechanisms have high root biomass and dense root systems. Under drought stress conditions (Figure 6), KM as a drought-susceptible cultivar showed increased tolerance when exogenous osmoprotectant was applied with elevated plant height, number of leaves, and root length. In contrast, the number of tillers did not change in any cultivar when exposed to drought stress conditions and subjected to exogenous osmoprotectants at various concentrations (p > 0.05). Without exogenous osmoprotectants, KM showed a significant decrease (p< 0.05) in plant height, number of leaves, and total root length. BSM and SB showed similar patterns but with a non-significant decrease between drought stress and control conditions when no exogenous osmoprotectant was applied.
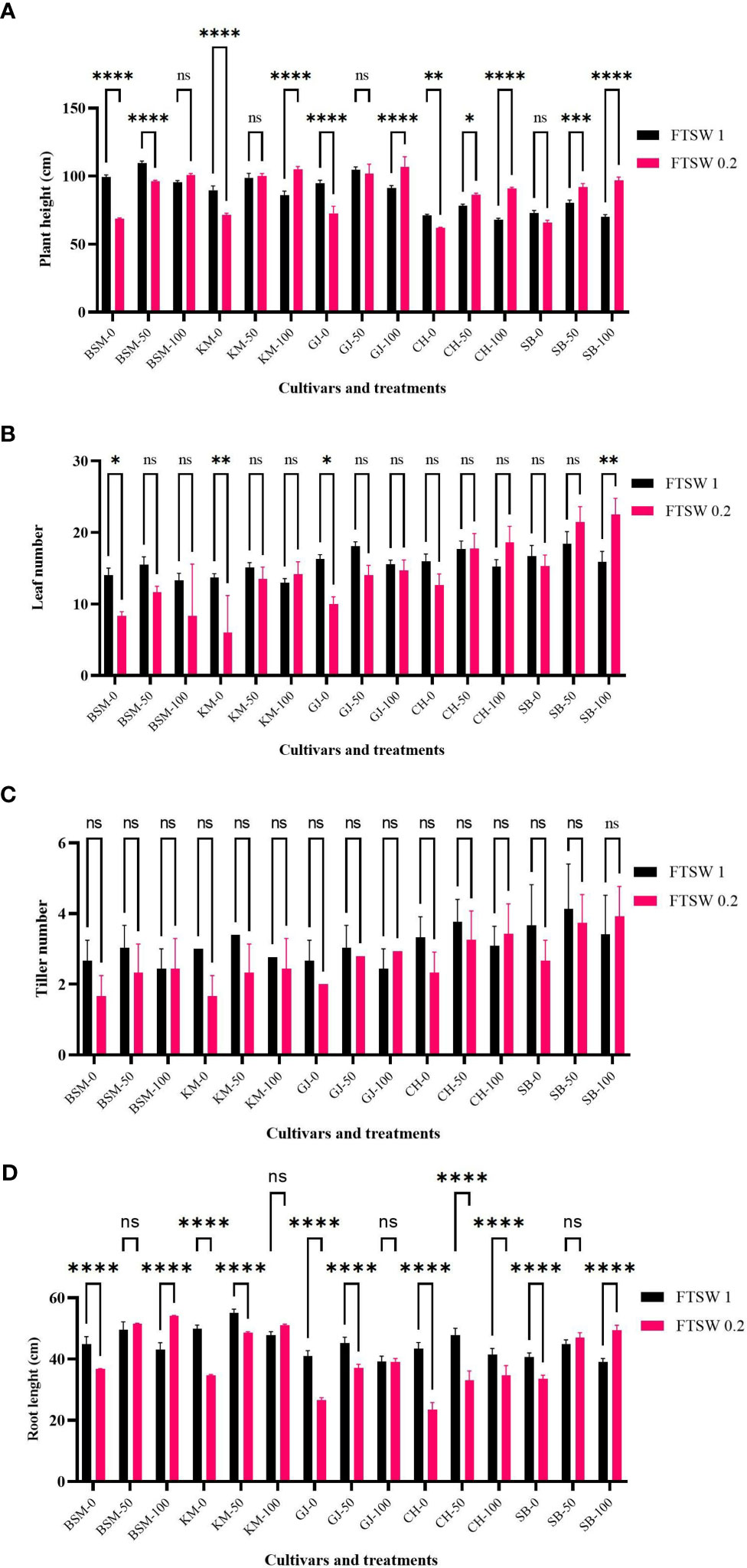
Figure 6 Growth parameters of (A) plant height, (B) leaf number, (C) tiller number, and (D) root length of the drought stress treatment rice cultivars GJ, KM, BSM, CH (drought-susceptible control), and SB (drought-tolerant control) at the end of the drought stress treatment with FTSW 1 (control) and FTSW 0.2 (drought stress). The cultivars were exposed to osmoprotectant concentrations of 0% (low), 50% (moderate), and 100% (high). Ns, no significant difference with p value>0.05, * p value< 0.05, ** p value=0.02, *** p value= 0.0001, **** p value < 0.0001 based on 95% confident level in ANOVA two-way test with three replications each.
Figure 7 shows changes in plant biomass when treated with drought stress and exogenous osmoprotectant treatment. Overall, in conditions without exogenous osmoprotectants (0%), plants showed a decrease in root fresh weight, root dry weight, and shoot fresh weight and dry weight (Figures 7A–D, respectively) between control and stress treatment. A significant decrease shown by KM and CH. Several cultivars such as BSM and GJ did not show significant changes in biomass (p > 0.05) under drought stress compared with controls even though they were exposed to moderate and high concentrations of exogenous osmoprotectants.
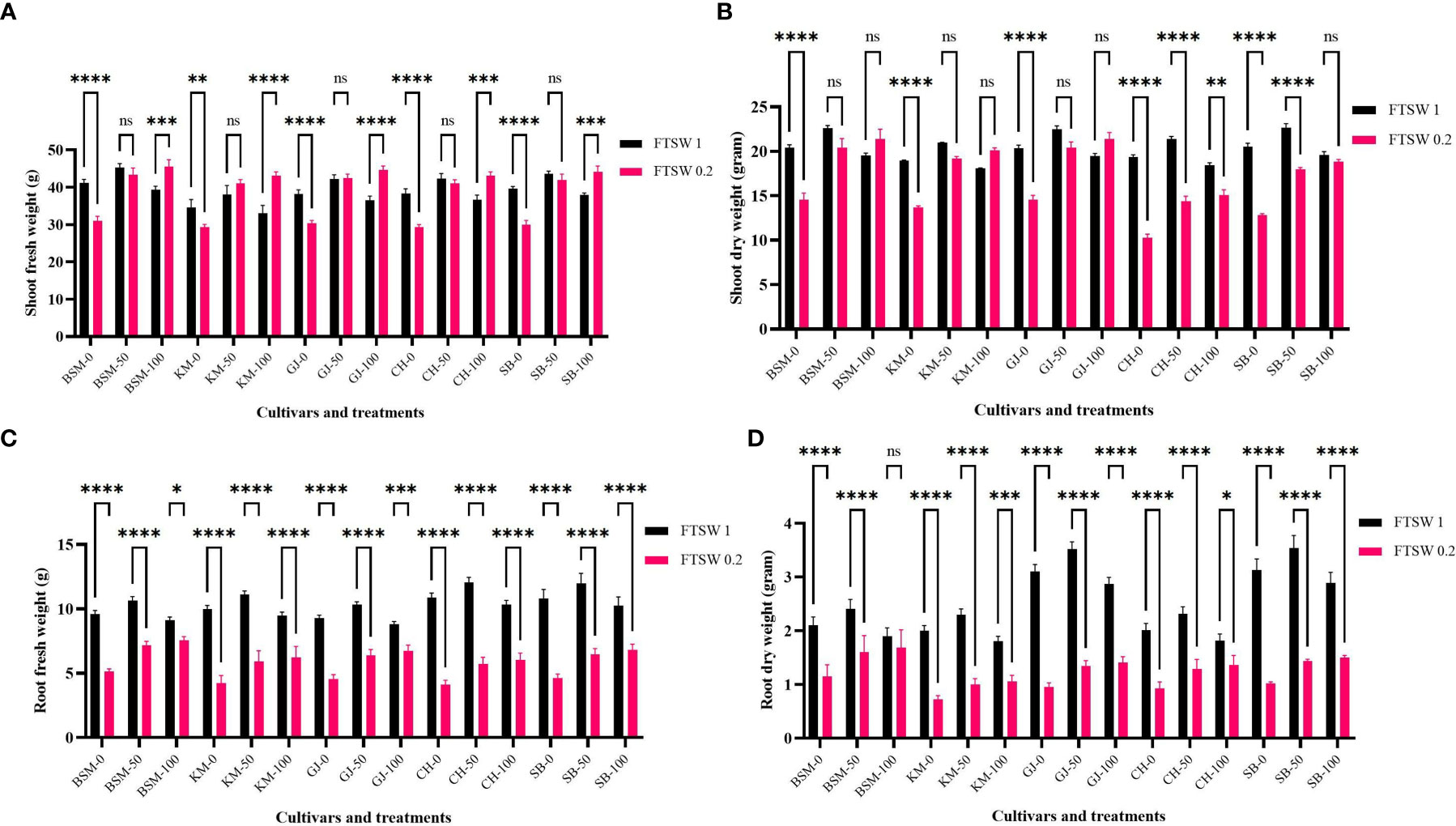
Figure 7 The biomass parameters of (A) root fresh weight, (B) root dry weight, (C) shoot fresh weight, and (D) shoot dry weight after drought stress treatment with FTSW 1 (control) and FTSW 0.2 (severe drought stress) in rice cultivars GJ, KM, BSM, CH (drought-susceptible control), and SB (drought-tolerant control) at the end of the drought stress treatment with FTSW 1 (control) and FTSW 0.2 (drought stress). The cultivars were exposed to osmoprotectant concentrations of 0% (low), 50% (moderate), and 100% (high). Ns, no significant difference, *p< 0.05 based on 95% confident level in ANOVA two-way test with three replications each. ns=no significant difference with p value>0.05, * p value < 0.05, ** p value=0.02, *** p value= 0.0001, **** p value < 0.0001 based on 95% confidence level in ANOVA two-way test with three replications each.
Pearson’s r correlation analysis
A positive value indicates a positive correlation between the two parameters being compared, meaning that an increase in the value of one parameter is accompanied by an increase in the other parameter. Positive correlations were found in comparisons between expression level of TFs (OsWRKY45, OsNAC6, OsDREB1A, and OsDREB2A) and Pro-related genes (OsP5CS1, OsP5CR, and OsProDH) (Figure 8A). Mostly, the gene expression level and the activity of APX and CAT were found negatively correlated.
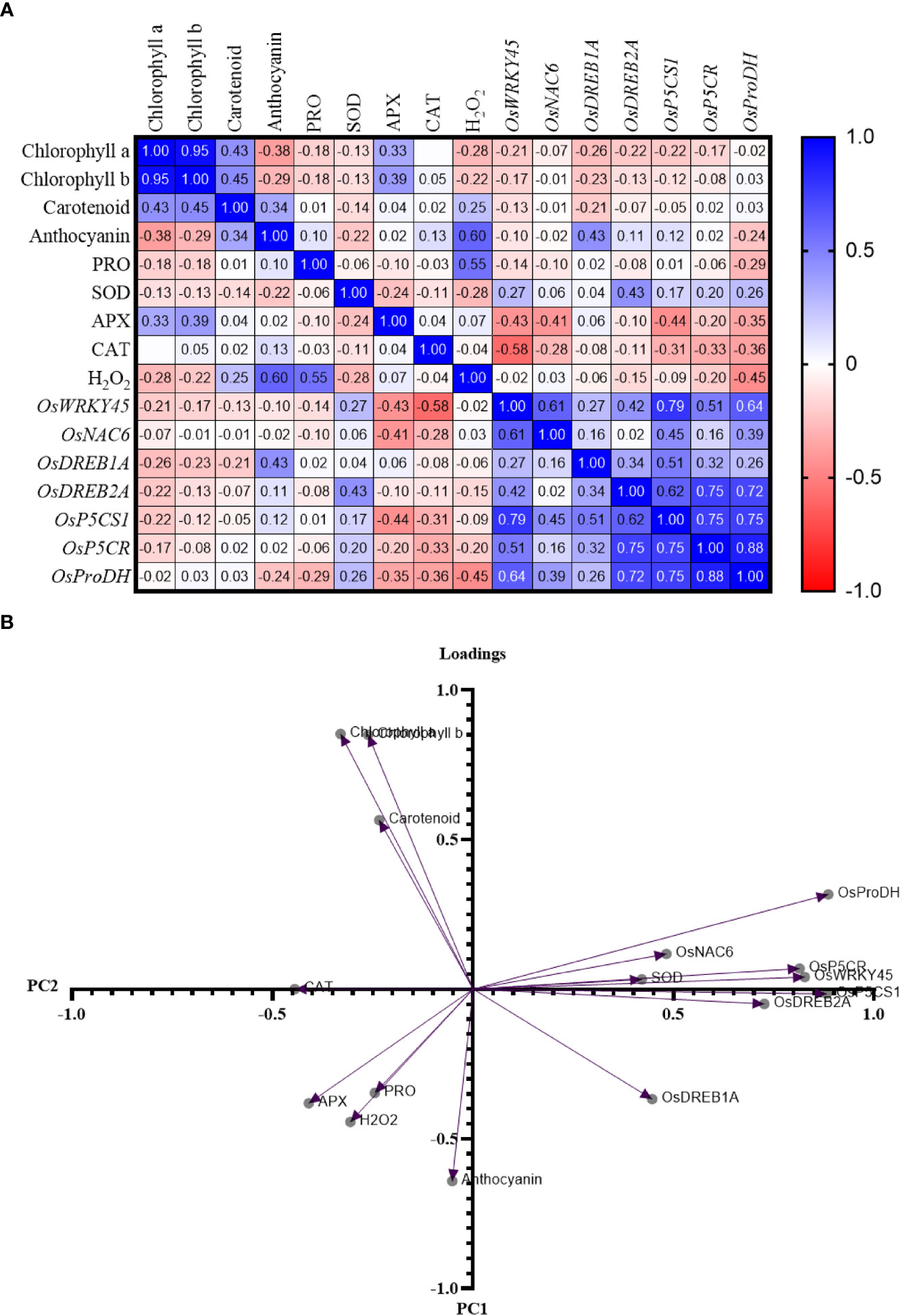
Figure 8 (A) Pearson’s r correlation matrix and (B) principal component analysis of parameters in rice cultivars GJ, KM, BSM, CH (drought-susceptible control), and SB (drought-tolerant control) at the end of the drought stress treatment with FTSW 1 (control) and FTSW 0.2 (drought stress). The cultivars were exposed to osmoprotectant concentrations of 0% (low), 50% (moderate), and 100% (high).
The results of the principal component analysis revealed a grouping based on Eigenvalue (Figure 8B). Based on the analysis with principal component (PC) I, the characters OsNAC6, OsProDH, OsP5CR, OsDREB1A, OsDREB2A, OsWRKY45, and OsP5CS1 were found to contrast with the characters of chlorophyll a, chlorophyll b, carotenoids, Pro, anthocyanins, H2O2, APX, CAT, and SOD. Based on PC II, the characters of chlorophyll A, B, carotenoids, SOD, OsProDH, OsNAC6, OsP5CR, and OsWRKY45, and the expression of OsP5CS1 contrast with the character of the activity of APX, H2O2, Pro, anthocyanin, and CAT, and the expression of OsDREB1A.
Discussion
One of the widely known osmolytes that act as metabolic markers under abiotic stress conditions is Pro. The accumulation of Pro in plants is closely related to its function in osmotic adjustment. This process allows the plant to conserve water by reducing the rate of water loss from subcellular compartments to the extracellular environment (Farahani et al., 2013). In rice, the increased accumulation of Pro is proportional to the level of drought stress (Raye et al., 2018). In stress-susceptible plants (KM), the addition of exogenous osmoprotectants acts as a trigger that plays a role in the activation of TFs and Pro metabolism regulatory genes, while in drought-tolerant cultivars (BSM and GJ), the addition of exogenous osmoprotectants acts as a negative regulatory signal to stop endogenous proline biosynthesis in cells.
In this study, an analysis of TFs and the expression levels of genes in Pro synthesis pathways and their relation to physiological characteristics related to Pro accumulation has been carried out. The TFs involved in the Pro regulatory pathways are OsDREB1A, OsDREB2A, OsNAC6, and OsWRKY45. These TFs provide molecular switches for gene expression in the response to drought stress. In this study, the drought stress response was observed through the expression level of targeted genes in the Pro synthesis pathway including OsP5CS1, OsP5CR, and OsProDH.
Based on Figure 9, there are two Pro regulatory pathways, namely, ABA-dependent and ABA-independent pathways. In the ABA-dependent pathway, Pro accumulation is regulated by the AREB/ABF, bZIP, bHLH, and WKRY TFs group. These TFs activate MYB in glutamate catalysis as a Pro precursor to P5C. In the reduction step of P5C to Pro, the TFs involved is a member of the WRKY family, which regulates the synthesis of the P5CR enzyme. In the ABA-independent pathway, proline accumulation is regulated by the AREB/ABF and NAC TFs that are involved in activating P5CS1 and P5CS2 synthesis and P5C anabolism through the catalytic activity of P5CR.
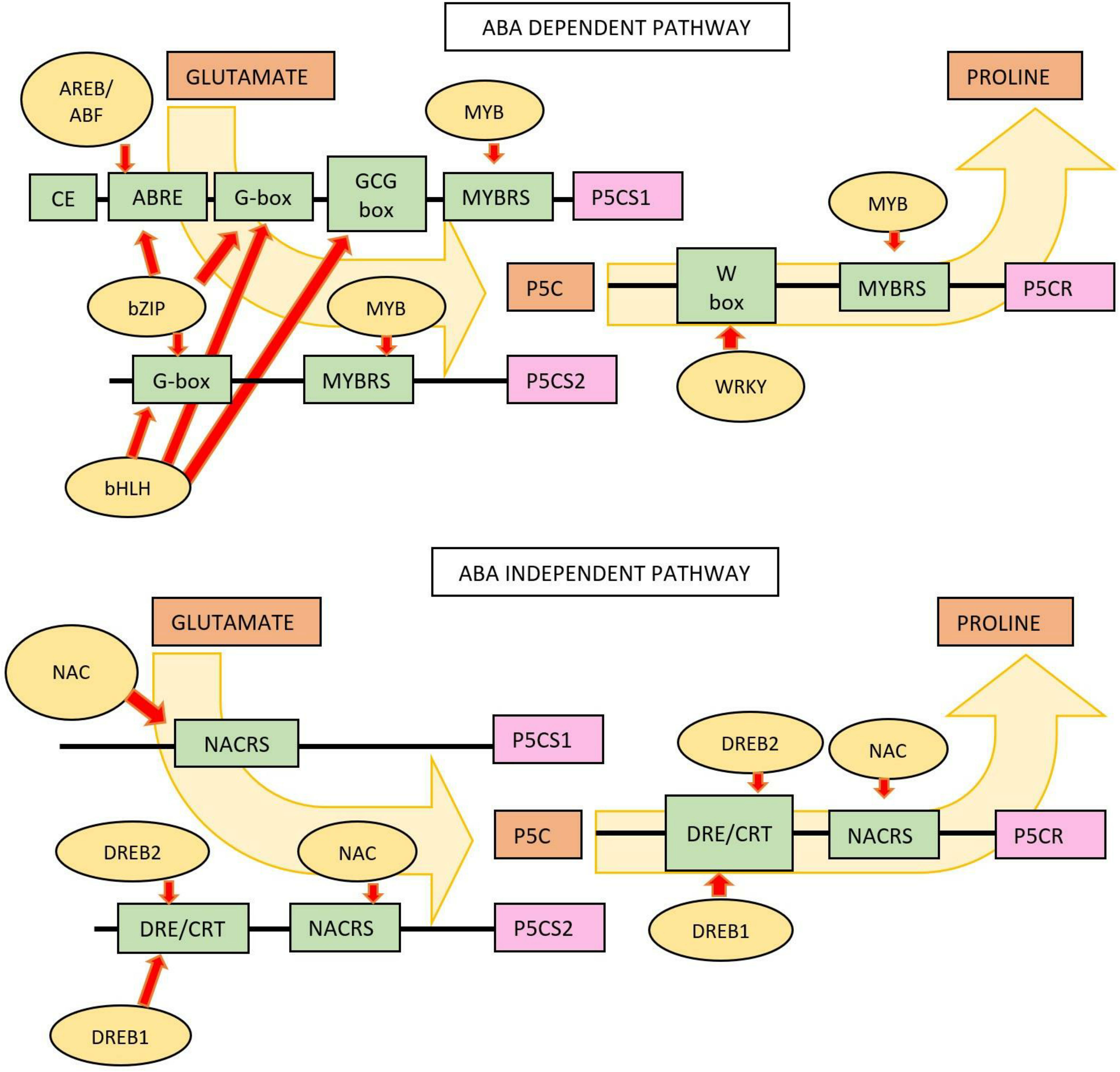
Figure 9 Pro synthesis pathways involving TFs and genes in the process of proline anabolism. Regulation of Pro accumulation by an ABA-dependent pathway. TFs included in this pathway are AREB/ABF (ABA-responsive element binding protein/ABRE-binding factor), bZIP (basic region, leucine zipper), bHLH (basic helix–loop–helix), MYB (v-Myb myeloblastosis viral oncogene homolog), and WKRY (a 60-amino-acid region that is defined by the conserved amino acid sequence WRKYGQK at its N-terminal end). Regulation of Pro accumulation by an ABA-independent pathway. The TFs involved are the NAC (no apical meristem—Petunia, ATAF1–2—Arabidopsis thaliana activating factor, and CUC2—cup-shaped cotyledon, Arabidopsis) and DREB1A and DREB2A (dehydration responsive element-binding) groups.
In this study, the drought-tolerant cultivars (BSM and GJ) did not show a significant increase in the expression level of OsWRKY45, while in the susceptible KM cultivar, an increase in the concentration of exogenous osmoprotectants also led to an increase in the expression level of OsWRKY45, with the highest levels shown in plants under drought stress.
The expressions level of OsNAC6 was found to be higher in the KM when treated with drought and osmoprotectant at the concentration level of 50%. In drought-tolerant cultivars (BSM and GJ), there was no significant differences in gene expressions at any level of exogenous osmoprotectant. Moreover, OsNAC6 expression decreased as the concentration of osmoprotectants increased. Previous studies (Nakashima et al., 2007; Parida et al., 2016; Karim et al., 2021) showed that NAC plays a role in growth and development, fluctuations in hormones, leaf senescence, and plant defense mechanisms against environmental stresses. This gene is induced by stress. Plants with higher NAC expression levels tend to have the potential to survive abiotic stresses (Baillo et al., 2019).
Under drought stress, the expression level of OsDREB1A in BSM and GJ rice decreased as the exogenous osmoprotectant concentration increased from 0% to 50%, but did not differ between 50% and 100% concentrations. The susceptible KM cultivar showed an increased level of OsDREB1A with 100% exogenous osmoprotectant application.
During drought stress, the expression level of OsDREB2A in drought-tolerant cultivars (BSM and GJ) increased at a 50% concentration of exogenous osmoprotectant but then decreased as the concentration rose to 100%. In drought-tolerant cultivars as above, an increase in the exogenous osmoprotectant concentration caused a decrease in the expression level of OsDREB2A. This increase in DREB TF activity at high concentrations of exogenous osmoprotectant treatment theoretically increases the transcription of P5CS1 and P5CS2 in the ABA-dependent pathway, as well as in the ABA-independent pathway through NAC activation. This whole process leads to an increase in endogenous Pro synthesis and its accumulation.
Pro is synthesized when plants experience drought. The mechanism of Pro biosynthesis can differ between species and even between cultivars. Previous research (Vendruscoloa et al., 2007) on Zea mays and Triticum aestivum found that Pro accumulation in plants is a defense mechanism through osmotic adjustment to prevent cell damage due to dehydration and the ROS scavenging mechanism in the oxidative response pathway. Our data show the expression of OsP5CS in NTT local rice leaves treated with drought and exogenous osmoprotectant. The expression levels of OsP5CS1 in KM with drought stress and control treatments increased when exposed to osmoprotectants at 50% and 100%.
In drought-tolerant cultivars (BSM, GJ, and SB), the application of 50% and 100% of osmoprotectant decreased the expression levels of OsP5CS1 in both the drought stress and the control conditions. In susceptible cultivars (KM), drought stress gave a significant increase in this gene expression at the osmoprotectant levels of 50% and 100%. Based on this study, KM showed increased expression levels of OsP5CS and OsP5CR with increasing exogenous osmoprotectant concentration, while GJ and BSM showed the opposite expression.
The same pattern was also found in the expression of OsProDH, which catalyzes the reduction of excess Pro into P5CS. In drought-tolerant cultivars, OsProDH expression increased as Pro accumulation levels increased, while in the drought-susceptible cultivar (KM), the opposite occurred. This may have happened because the accumulation of exogenous Pro became a feedback signal to reduce high OsProDH activity in drought-tolerant rice under drought. The expression of OsProDH1 and OsProDH2 increased during the senescence and recovery phase (Guo et al., 2020). This upregulation functions to reduce the level of toxicity of Pro/P5C.
Each cultivar accumulates Pro with different strategies. In control conditions without osmoprotectant, the drought-tolerant cultivar GJ showed the highest Pro content when treated with a high concentration of osmoprotectant. During drought, GJ and KM (drought susceptible) cultivars tend to show no significant differences between osmoprotectant level, while BSM (drought-tolerant) shows higher Pro accumulation with the application of osmoprotectant.
A study by Hossain et al, 2014 ; Shemi et al., 2021) has shown that exogenous Pro increases the antioxidant activity of plants against abiotic stress. This finding is in agreement with another report (Nounjan et al., 2012) that showed an increase in APX, POX, CAT, and APX activities under stress conditions and a faster recovery period with the application of exogenous Pro.
Foliar application of exogenous osmoprotectants derived from C.equisetifolia leaf extract causes accumulation of osmoprotectants in drought-tolerant plant organs. This accumulation leads to an inhibitory effect of OsP5CS and OsP5CR expression in drought-tolerant cultivars but instead activates the expression of OsP5CS and OsP5CR on drought-susceptible cultivars. Differences in the regulation of the two groups are presented in Figure 10. OsProDH gene expression increased in tolerant plants, but decreased in drought-susceptible plants (Figure 10). A decreased OsProDH expression level of drought-susceptible plants resulted in an accumulation of proline which induced cell membrane protection, which was observed through an increase in relative water content and higher cell membrane stability index.
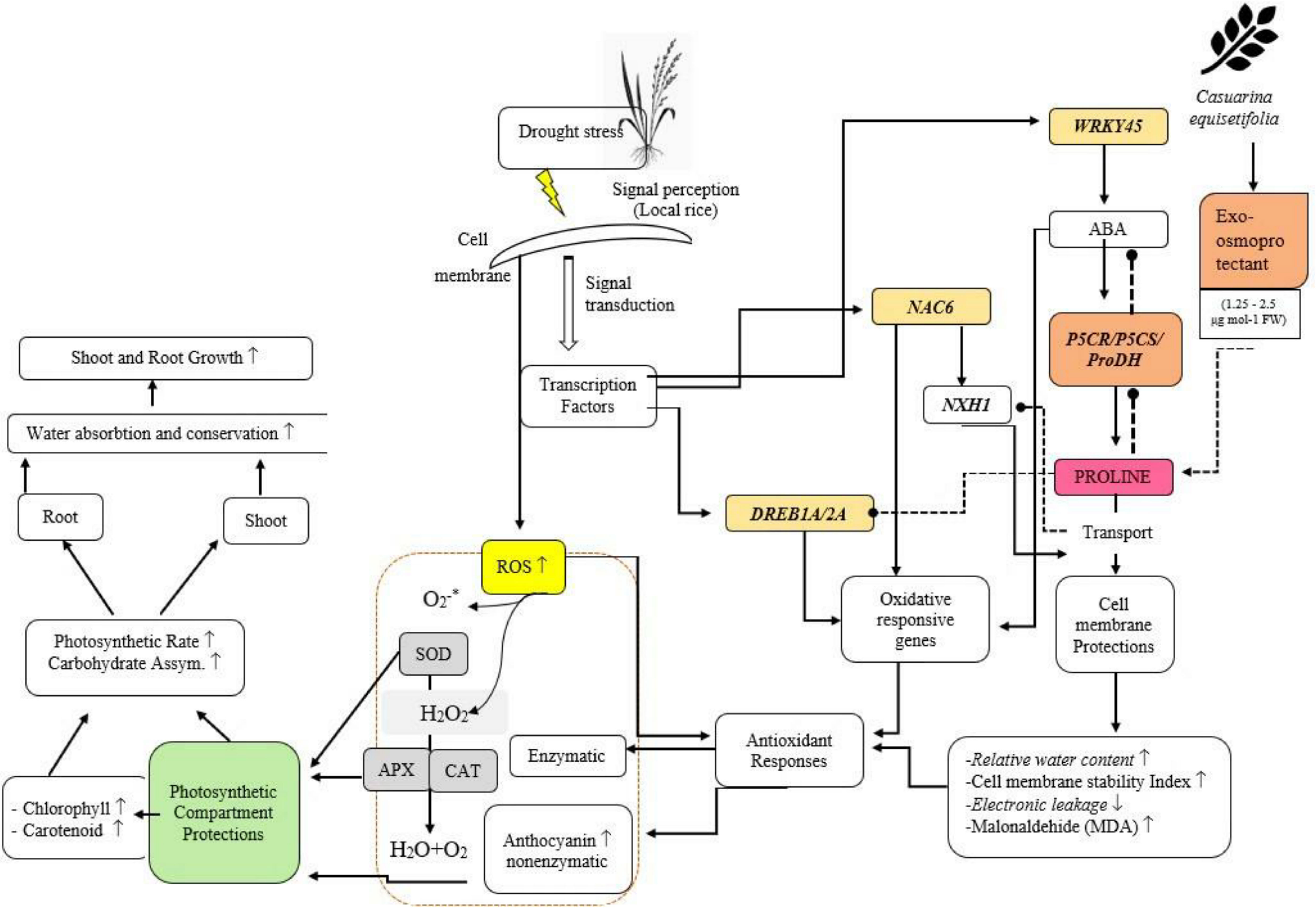
Figure 10 Schematic diagram of Pro regulation and physiological changes under drought stress in NTT local rice treated with exogenous osmoprotectants (containing 1.25–2.5 µg mol−1 FW). The image shows the interaction between drought stress treatment and physiological changes and the foliar application of exogenous osmoprotectants in NTT, Indonesia local rice plants.
In drought-tolerant plants, there was no apparent difference in the level of Pro accumulation or SOD activity after the application of exogenous osmoprotectants. The increased protection of cell compartments in drought-susceptible cultivars led to a decrease in ROS in cells, which, in turn, led to an increase in physiological activities such as photosynthesis to support the formation of cell biomass.
Based on the present research, the regulatory pathway for exogenous osmoprotectants in Indonesia’s local upland rice involves several important components at the molecular, cellular, tissue, and organ level. In general, under normal conditions without exogenous osmoprotectant treatment, plants can be divided into groups that are tolerant and susceptible to drought. After the cultivars were given exogenous osmoprotectants, there were differences in the response to drought stress (tolerant and susceptible) and osmoprotectants applied (responsive and non-responsive).
Conclusion
Foliar application of exogenous osmoprotectants derived from C.equisetifolia caused accumulation of proline in drought-susceptible plants. The existence of these extracts stabilizes leaf cells and supports photosynthetic compartments and carbon assimilation in plants, leading to growth.
Data availability statement
The original contributions presented in the study are included in the article/Supplementary Material. Further inquiries can be directed to the corresponding author.
Author contributions
Conceptualization: YS, DR, and YP. Data curation: DSR and TA. Formal analysis: DR and DI. Funding acquisition: YP. Methodology: YS, DI, and YP. Software: YS, DSR, and TA. Validation: DR, DI, and TA. Visualization: YS and DSR. Writing—original draft: YS, DR, DI, YP, and DSR. Writing—review and editing: DR, TA, and YP. All authors contributed to the article and approved the submitted version.
Acknowledgments
The authors express their gratitude to Prof. Bernhard Grimm (Humboldt-Universität zu Berlin, Germany) and Dr. Ian Smith for assisting in preparing and writing of the manuscript, and to the Faculty of Biology, Universitas Gadjah Mada (UGM), the Research Center for Biotechnology under the Postdoctoral Program No. 13602/UN1.P.II/Dit-Lit/PT.01.04/2022 to YAP and The Research Center for Genetic Engineering, the National Research, and Innovation Agency of Indonesia (BRIN) under the Postdoctoral Research Program, for supporting this research and publication.
Conflict of interest
The authors declare that the research was conducted in the absence of any commercial relationship that could be constructed as a potential conflict of interest.
Publisher’s note
All claims expressed in this article are solely those of the authors and do not necessarily represent those of their affiliated organizations, or those of the publisher, the editors and the reviewers. Any product that may be evaluated in this article, or claim that may be made by its manufacturer, is not guaranteed or endorsed by the publisher.
Supplementary material
The Supplementary Material for this article can be found online at: https://www.frontiersin.org/articles/10.3389/fpls.2023.1210241/full#supplementary-material
References
Arsa, I. G. B. A., Ariffin, A., Aini, N., Lalel, H. J. D. (2016). Effectiveness of osmoprotectants in improving aroma quality and yield of Pare Wangi upland rice variety grown on two different soil types in East Nusa Tenggara. Agrivita 38, 231–241. doi: 10.17503/agrivita.v38i3.672
Aslam, M. M., Rashid, M. A. R., Siddiqui, M. A., Khan, M. T., Farhat, F., Yasmeen, S., et al. (2022). Recent insights into signaling responses to cope drought stress in rice. Rice Sci. 29, 105–117. doi: 10.1016/j.rsci.2021.08.001
Atteya, A. K. G., El-Serafy, R. S., El-Zabalawy, K. M., Elhakem, A., Genaidy, E. A. E. (2022). Exogenously supplemented proline and phenylalanine improve growth, productivity, and oil composition of salted moringa by up-regulating osmoprotectants and stimulating antioxidant machinery. Plants. 11 (12), 1553. doi: 10.3390/plants11121553
Baillo, E. H., Kimotho, R. N., Zhang, Z., Xu, P. (2019). Transcription factors associated with abiotic and biotic stress tolerance and their potential for crops improvement. Genes (Basel). 10, 1–23. doi: 10.3390/genes10100771
Basuki, T., Prajitno, D. (2014). Kontribusi senyawa kompatibel ekstrak daun tumbuhan halofit sebagai anti-salinitas dalam amandemen fisiologis padi (Oryza sativa L.) (Yogyakarta: Universitas Gadjah Mada).
Bates, L., Waldran, R., Teare, I. (1973). Rapid determination of free proline for water-stress studies. Plant Soil. 39, 205. doi: 10.1007/BF00018060
Bertolino, L. T., Caine, R. S., Gray, J. E. (2019). Impact of stomatal density and morphology on water-use efficiency in a changing world. Front. Plant Sci. 10 (March). doi: 10.3389/fpls.2019.00225
Bouazizi, H., Jouili, H., Ferjani, E. (2007). Effect of copper excess on growth, H2O2 production and peroxidase activities in maize seedlings (Zea mays L). Pak J. Biol. Sci. 10 (5), 751–756. doi: 10.3923/pjbs.2007.751.756
Cha-um, S., Samphumphuang, T., Kirdmanee, C. (2013). Glycinebetaine alleviates water deficit stress in indica rice using proline accumulation, photosynthetic efficiencies, growth performances and yield attributes. Aust. J. Crop Sci. 7 (2), 213–218.
Chen, R. J., Wang, L. X., Zhang, X. J., Wang, S. L., Li, H., Gao, S. J. (2020). Physiological and molecular basis of the effects of exogenous selenium application on wheat seedling performance under drought stress. Appl. Ecol. Environ. Res. 18 (1), 1227–1236. doi: 10.15666/aeer/1801_12271236
Darmadi, D., Junaedi, A., Sopandie, D., Supijatno, Lubis, I., Homma, K. (2021). Water-efficient rice performances under drought stress conditions. AIMS Agric. Food. 6 (3), 838–863. doi: 10.3934/agrfood.2021051
Dawe, D. (2013). Geographic determinants of rice self-sufficiency in Southeast Asia. ESA Work Pap. No. 13-03 (13), 1–17.
Elevarthi, S., Martin, B. (2010). “Spectrophotometric assays for antioxidant enzymes in plants,” in Plant stress tolerance-methods in molecular biology. Ed. Sunkar, R. (Heidelberg-London: Springer Science+ Business Media), 273–290.
Farahani, A., Lebaschi, H., Hussein, M., Hussein, S., Reza, V., Jahanfar, D. (2013). Effects of arbuscular mycorrhizal fungi, different levels of phosphorus and drought stress on water use efficiency, relative water content and proline accumulation rate of Coriander (Coriandrum sativum L.). J. Med. Plants Res. 2 (6), 125–131.
Farooq, M., Basra, S. M. A., Wahid, A., Cheema, Z. A., Cheema, M. A., Khaliq, A. (2008). Physiological role of exogenously applied glycinebetaine to improve drought tolerance in fine grain aromatic rice (Oryza sativa L.). J. Agron. Crop Sci. 194 (5), 325–333. doi: 10.1111/j.1439-037X.2008.00323.x
Farooq, M., Wahid, A., Kobayashi, N., Fujita, D. B. (2009). Plant drought stress : effects, mechanisms and management. Agron. Sustain Dev. 29 (1), 185–212. doi: 10.1051/agro:2008021
Guo, X., Xiaoning, Z., Qin, Y., Liu, Y. X., Zhang, J., Zhang, N., et al. (2020). Host-associated quantitative abundance profiling reveals the microbial load variation of root microbiome. Plant Commun. 1 (1), 1–17. doi: 10.1016/j.xplc.2019.100003
Harborne, J. (1984). Phytochemical methods: a guide to modern technique of plant analysis (London: Chapman and Hall).
Hasanuzzaman, M., Alam, M. M., Rahman, A., Nahar, K., Fujita, M. (2014). Exogenous proline and glycine betaine mediated upregulation of antioxidant defense and glyoxalase systems provides better protection against salt-induced oxidative stress in two rice (Oryza sativa L.) varieties. BioMed. Res. Int 2014, 757219. doi: 10.1155/2014/757219
Hassanein, A., Ibrahim, E., Ali, R. A., Hashem, H. (2021). Differential metabolic responses associated with drought tolerance in egyptian rice. J. Appl. Biol. Biotechnol. 9 (4), 37–46. doi: 10.7324/JABB.2021.9405
Herawati, R., Alnopri, Masdar, Simarmata, M., Sipriyadi, Sutrawati, M. (2021). Identification of drought tolerant markers, DREB2A and BADH2 genes, and yield potential from single-crossing varieties of rice in bengkulu, indonesia. Biodiversitas 22 (2), 785–793. doi: 10.13057/biodiv/d220232
Hong-zheng, S., Ting, P., Jing, Z., Jun-zhou, L., Yan-xiu, D., Quan-zhi, Z. (2017). Test of small RNA sequencing repeatability in rice. Rice Sci. 24 (1), 56–60. doi: 10.1016/j.rsci.2016.06.008
Hossain, M., Hoque, M., Burrit, D., Fujita, M. (2014). Proline protects plants against abiotic oxidative stress: biochemical and molecular mechanism, oxidative damage to plants (San Diego: Elsevier).
Hosseinifard, M., Stefaniak, S., Javid, M. G., Soltani, E., Wojtyla, Ł., Garnczarska, M. (2022). Contribution of exogenous proline to abiotic stresses tolerance in plants: a review. Int. J. Mol. Sci. 23 (9), 5186. doi: 10.3390/ijms23095186
Islam, M. J., Uddin, M. J., Hossain, M. A., Henry, R., Begum, K., Sohel, A. T., et al. (2022). Exogenous putrescine attenuates the negative impact of drought stress by modulating physio-biochemical traits and gene expression in sugar beet (Beta vulgaris L.). PloS One 17 (1 January), 1–25. doi: 10.1371/journal.pone.0262099
Jalal-ud-Din, Khan, S. U., Khan, A., Naveed, S. (2015). Effect of exogenously applied kinetin and glycinebetaine on metabolic and yield attributes of rice (Oryza sativa L.) Under drought stress. Emirates J. Food Agric. 27 (1), 75–81. doi: 10.9755/ejfa.v27i1.17950
Karim, M. F., Rosely, N. F. S. M., Kamil, N. A. M., Amri, C. N. A. C. (2021). Morpho-physiological and anatomical assessment of different rice varieties subjected to drought stress at early vegetative stage. Malaysian Appl. Biol. 50 (1), 55–64. doi: 10.55230/mabjournal.v50i1.12
Kavi Kishor, P. B., Sangam, S., Amrutha, R. N., Sri, L. P., Naidu, K. R., Rao, K. R. S. S., et al. (2005). Regulation of proline biosynthesis, degradation, uptake and transport in higher plants: Its implications in plant growth and abiotic stress tolerance. Curr. Sci. 88 (3), 424–438.
Kim, H., Lee, Y. (2020). The Rice Microbiome : A model platform for crop holobiome. Phyto. J. 4 (1), 5–18. doi: 10.1094/PBIOMES-07-19-0035-RVW
Kulkarni, M., Soolanayakanahally, R., Ogawa, S., Uga, Y., Selvaraj, M. G., Kagale, S. (2017). Drought response in wheat: Key genes and regulatory mechanisms controlling root system architecture and transpiration efficiency. Front. Chem. 5 (DEC), 13. doi: 10.3389/fchem.2017.00106
Lalarukh, I., Al-Dhumri, S. A., Al-Ani, L. K. T., Hussain, R., Al Mutairi, K. A., Mansoora, N., et al. (2022). A combined use of rhizobacteria and moringa leaf extract mitigates the adverse effects of drought stress in wheat (Triticum aestivum L.). Front. Microbiol. 13 (June), 12. doi: 10.3389/fmicb.2022.813415
Liu, Y., Li, P., Xu, G. C., Xiao, L., Ren, Z. P., Bin, L. (2017). Growth, morphological, and physiological responses to drought stress in bothriochloa ischaemum. Front. Plant Sci. 8 (February), 14. doi: 10.3389/fpls.2017.00230
Lotkowska, M., Tohge, T., Fernie, A., Xue, G., Balazadeh, S., Mueller-Roeber, B. (2015). The Arabidopsis transcription factor MYB112 promotes anthocyanin formation during salinity and under high light stress. Plant Physiol. 169 (3), 1862–1880. doi: 10.1104/pp.15.00605
Luo, H., Zhang, T., Zheng, A., He, L., Lai, R., Liu, J., et al. (2020). Exogenous proline induces regulation in 2-acetyl-1-pyrroline (2-AP) biosynthesis and quality characters in fragrant rice (Oryza sativa L.). Sci. Rep. 10 (1), 1–8. doi: 10.1038/s41598-020-70984-1
Marklund, S., Marklund, G. (1974). Involvement of the superoxide anion radical in the autoxidation of pyrogallol and a convenient assay for superoxide dismutase. Eur. J. Biochem. 47, 469–474. doi: 10.1111/j.1432-1033.1974.tb03714.x
Nakashima, K., Tran, L., Nguyen, D., Fujita, M., Maruyama, K., Todaka, D., et al. (2007). Functional analysis of a NAC-type transcription factor OsNAC6 involved in abiotic and biotic stress-responsive gene expression in rice. Plant J. 51 (4), 617–630. doi: 10.1111/j.1365-313X.2007.03168.x
Nounjan, N., Nghia, P. T., Theerakulpisut, P. (2012). Exogenous proline and trehalose promote recovery of rice seedlings from salt-stress and differentially modulate antioxidant enzymes and expression of related genes. J. Plant Physiol. 169 (6), 596–604. doi: 10.1016/j.jplph.2012.01.004
Pandey, V., Ansari, M. W., Tula, S., Sahoo, R. K., Bains, G., Kumar, J., et al. (2016). Ocimum sanctum leaf extract induces drought stress tolerance in rice. Plant Signal Behav. 11 (5), 1–9. doi: 10.1080/15592324.2016.1150400
Pandey, V., Shukla, A. (2015). Acclimation and tolerance strategies of rice under drought stress. Rice Sci. 22 (4), 147–161. doi: 10.1016/j.rsci.2015.04.001
Parida, A. K., Veerabathini, S. K., Kumari, A., Agarwal, P. K. (2016). Physiological, anatomical and metabolic implications of salt tolerance in the halophyte Salvadora persica under hydroponic culture condition. Front. Plant Sci. 7 (MAR2016), 18. doi: 10.3389/fpls.2016.00351
Raye, R., Tran, H., Xuan, T., Khank, T. (2018). Imposed water deficit after anthesis for the improvement of macronutrients, quality, phytochemical, and antioxidants in rice grain. Sustainability. 10, 4843–4846. doi: 10.3390/su10124843
Salsinha, Y. C. F., Maryani, Indradewa, D., Purwestri, Y., Rachmawati, D. (2021). Leaf physiological and anatomical characters contribute to drought tolerance of Nusa Tenggara Timur local rice cultivars. J. Crop Sci. Biotechnol. 24 (3), 337–348. doi: 10.1007/s12892-020-00082-1
Salsinha, Y. C. F., Nurbaiti, S., Sebastian, A., Indradewa, D., Purwestri, Y. A., Rachmawati, D. (2022). Proline-related gene expression contribute to physiological changes of East Nusa Tenggara (Indonesia) local rice cultivars during drought stress. Biodiversitas 23 (7), 3573–3583. doi: 10.13057/biodiv/d230734
Serraj, R., Liu, D., He, H., Sellamuthu, R., Impa, S., Cairns, J., et al. (2008). “Novel approaches for integration of physiology, genomics, and breeding for drought resistance improvement in rice,” in Drydown FTSW protoc (Manila: International Rice Research Institute).
Shahzad, A. N., Qureshi, M. K., Ullah, S., Latif, M., Ahmad, S., Bukhari, S. A. H. (2020). Exogenous Trehalose improves cotton growth by modulating antioxidant defense under salinity-induced osmotic stress. Pakistan J. Agric. Res. 33 (2), 270–279. doi: 10.17582/journal.pjar/2020/33.2.270.279
Shemi, R., Wang, R., Gheith, E. S. M. S., Hussain, H. A., Cholidah, L., Zhang, K., et al. (2021). Role of exogenous-applied salicylic acid, zinc and glycine betaine to improve drought-tolerance in wheat during reproductive growth stages. BMC Plant Biol. 21 (1), 1–15. doi: 10.1186/s12870-021-03367-x
Upadhyay, P. (2019). Climate change and adaptation strategies: a study of agriculture and livelihood adaptation by farmers in Bardiya District, Nepal. Adv. Agric. Environ. Sci. 2 (1), 47–52. doi: 10.30881/aaeoa.00022
Vendruscoloa, E., Schusterb, I., Pileggic, M., Scapimd, C., Molinarie, H., Marure, C., et al. (2007). Stress-induced synthesis of proline confers tolerance to water deficit in transgenic wheat. J. Plant Physiol. 164, 1367–1376. doi: 10.1016/j.jplph.2007.05.001
Wahyuni, S., Lande, M. L., Handayani, T. T. (2018). Efek ekstrak air daun kirinyuh cromolaena odorata [l.] r.m. king & h. rob) terhadap pertumbuhan padi sawah (Oryza sativa L.) varietas mekongga pada kondisi cekaman kekeringan effect of Kirinyuh leaf extract (Cromolaena odorata [L.] R.M. King & H. Rob) On. J. Penelit Pertan Terap 18 (2), 116–122.
Wu, J., Wang, J., Hui, W., Zhao, F., Wang, P., Su, C., et al. (2022). Physiology of plant responses to water stress and related genes: A Review. Forests 13 (2), 324. doi: 10.3390/f13020324
Yong, B., Xie, H., Li, Z., Li, Y. P., Zhang, Y., Nie, G., et al. (2017). Exogenous application of GABA improves PEG-induced drought tolerance positively associated with GABA-shunt, polyamines, and proline metabolism in white clover. Front. Physiol. 8 (DEC), 15. doi: 10.3389/fphys.2017.01107
You, J., Zhang, Y., Liu, A., Li, D., Wang, X., Dossa, K., et al. (2019). Transcriptomic and metabolomic profiling of drought-tolerant and susceptible sesame genotypes in response to drought stress. BMC Plant Biol. 19 (1), 1–16. doi: 10.1186/s12870-019-1880-1
Keywords: Casuarina equisetifolia, proline accumulation, exogenous osmoprotectant, rice, drought stress
Citation: Salsinha YCF, Rini DS, Indradewa D, Rachmawati D, Alam T and Purwestri YA (2023) Exogenously applied Casuarina equisetifolia leaf extracts act as an osmoprotectant on proline accumulation under drought stress in local rice from Indonesia. Front. Plant Sci. 14:1210241. doi: 10.3389/fpls.2023.1210241
Received: 22 April 2023; Accepted: 07 July 2023;
Published: 03 August 2023.
Edited by:
Muhamad Shakirin Mispan, University of Malaya, MalaysiaReviewed by:
Mohamed Magdy F. Mansour, Ain Sham University, EgyptElsayed Mansour, Zagazig University, Egypt
Copyright © 2023 Salsinha, Rini, Indradewa, Rachmawati, Alam and Purwestri. This is an open-access article distributed under the terms of the Creative Commons Attribution License (CC BY). The use, distribution or reproduction in other forums is permitted, provided the original author(s) and the copyright owner(s) are credited and that the original publication in this journal is cited, in accordance with accepted academic practice. No use, distribution or reproduction is permitted which does not comply with these terms.
*Correspondence: Yekti Asih Purwestri, eWVrdGlAdWdtLmFjLmlk