- 1Department of Biology, University of Patras, Patras, Greece
- 2Hellenic Centre for Marine Research, Institute of Marine Biological Resources and Inland Waters, Attiki, Greece
In addition to quantifying the taxonomic diversity of aquatic communities, understanding the patterns of alpha functional diversity (α-diversity) and exploring changes in functional dissimilarity (β-diversity) can improve our understanding on how ecosystems respond to environmental changes. In this study, we quantified functional alpha (α) and beta (β) diversity of macrophytic assemblages from river sites in Greece and then, examined relationships with water quality parameters and hydromorphological factors. We assigned 6 traits (Ellenberg nutrients indicator, Ellenberg light indicator, growth form, leaf size, leaf type, fruit size) to a total of 36 hydrophyte species and calculated three indices of functional diversity (functional richness, functional dispersion and functional evenness). We also estimated the total β-functional diversity and its’ main components, turnover and nestedness. To assess the effects of water quality (including physical and chemical variables) we used Generalized Additive Models (GAM) for alpha functional diversity indices and Generalized Dissimilarity Models (GDM) for beta functional diversity. We performed Kruskal-Wallis tests and permutational multivariate analysis of variance (PERMANOVA) to search for significant variations of α- and β-diversity among the hydromorphological factors. Our results showed that macrophyte growth form and light preference were important trait characteristics that explained a large share of the total variance of functional composition. We also found relatively low taxonomic and functional richness, whereas taxonomic and functional dissimilarity were mostly attributed to species turnover, which expresses the changes in taxonomic and functional composition. We found significant relationships between functional dispersion and functional evenness with pH and oxygen saturation, whereas functional dissimilarity was driven only by geographic distance, although the GDM explained a small portion of the total variance. Functional richness, dispersion and evenness were significantly higher at systems with fine substrates and deep waters with low or high flow compared to systems with coarser substrates and riffle habitats. We also found significant variation in functional dissimilarity among the hydromorphological factors, although much of the total variance remained unexplained. Overall, our study highlights the importance of considering the functional diversity of aquatic plant assemblages within the frame of freshwater monitoring and conservation plans.
Introduction
Aquatic macrophytes provide numerous functions in lotic ecosystems. For instance, plants that grow within the channel and along the banks are known to mediate nutrient and sediment transport from the land into the watercourse (Valkama et al., 2019; Walton et al., 2020), while at the same time they stabilize the channel and the banks preventing erosion. Most importantly, aquatic plants can provide foraging and reproduction habitats for fish, amphibians and invertebrates (Lind et al., 2019; Cole et al., 2020). They can also influence the physical, chemical and flow characteristics within the channel (Gurnell, 2015; Preiner et al., 2020), which in turn may affect fish and invertebrate communities.
Because of their importance for stream and riverine ecosystems, aquatic macrophytes have been widely used as indicators of ecosystem health and ecological integrity (Aguiar et al., 2014; Rodrigues et al., 2019; Szoszkiewicz et al., 2020). Aquatic macrophytes are one of the four biological quality elements that are used for the assessment of ecological status of streams and rivers of Europe, following the implementation of the Water Framework Directive 2000/60, and several national assessment approaches have been developed by the EU Member States (Birk and Willby, 2010). Most ecological assessment schemes consider the composition of aquatic macrophytic communities (Szoszkiewicz et al., 2020; Stefanidis et al., 2022) since the increased occurrence and abundance of certain plants is related with environmental factors that indicate anthropogenic degradation of aquatic ecosystems (e.g. eutrophication and hydromorphological alteration) (Szoszkiewicz et al., 2014; Stefanidis and Papastergiadou, 2019; Manolaki et al., 2020; Stefanidis et al., 2021). However, there are studies that have shown that the responses of macrophytes to environmental gradients can be complex and difficult to decipher (Steffen et al., 2014; O’Hare et al., 2018; Son et al., 2018; Gyosheva et al., 2020). Thus, freshwater ecologists have shown increased interest in studying multiple facets of aquatic biodiversity, including aquatic macrophytes (Fu et al., 2014a; Schneider et al., 2015; Alahuhta et al., 2017; Elo et al., 2018; Son et al., 2018; Stefanidis et al., 2019).
The functional diversity which Tilman (2001) defined as ‘those components of biodiversity that influence how an ecosystem operates or functions’, has emerged as a facet of biodiversity and a step beyond species richness. It has become a powerful tool to link community composition to ecosystem properties and then to ecosystem services by quantifying the value and range of functional characteristics and thus ecosystem functioning (Díaz et al., 2007). In addition, the impact of the global loss of biodiversity is increasingly attributed to the loss of functional rather than taxonomic groups (Bellwood et al., 2002). Further, species are not equal in their effects on ecosystem functioning since their functional traits matter to ecosystem processes (Mouchet et al., 2010). The study of trait distributions can be used as a more powerful conceptual model for understanding broad-scale patterns in assemblage structure since organisms with similar traits will share similar niche requirements and will select the same habitat (Olden et al., 2010).
Besides species richness (alpha diversity) and changes in species composition among communities (beta diversity), investigating patterns of functional alpha (α) and beta (β) diversity may provide invaluable information and a better understanding on how environmental gradients affect aquatic biodiversity processes (Zhang et al., 2018; Wang et al., 2021). Considering that assembly processes influencing natural communities may differ depending on the spatial scale considered, separating functional diversity in within-community (α) and among-community (β) components will improve the detection of all processes influencing community assembly. Furthermore, trait-based approaches are more likely to indicate an early response to environmental change than community-based approaches because a change in the functional structure is easier to detect than a change to community composition (Degen et al., 2018). In addition, such approaches can be applied to all species regardless of geographic region and location (Dolédec et al., 2006).
Previous studies have linked morphological and life history traits of aquatic plants with ecological and biogeochemical processes showing the response of aquatic plant functional structure to eutrophication and other environmental changes (Fu et al., 2014b; Baattrup-Pedersen et al., 2016; Stefanidis and Papastergiadou, 2019). Therefore, exploring the patterns of functional trait composition in relation to environmental change is crucial for a better understanding of the response of aquatic communities to pressure and identifying key trait characteristics that can serve as indicators of anthropogenic pressures. Although there is a general consensus that taxonomic diversity is shaped by environmental filters, including anthropogenic changes (Bornette and Puijalon, 2011; Dybkjaer et al., 2012), there are relatively few studies that have explored the patterns of functional α- and β- diversity of aquatic macrophytes in lotic ecosystems.
The main objective of this study is to investigate the patterns of functional alpha and beta diversity of aquatic macrophytic assemblages in Greek lotic ecosystems and to assess whether these patterns are subject to environmental change. Our hypotheses are that a) indices of a- functional diversity decline with increased levels of nutrient pollution and degraded water quality, b) the change of functional compositional structure increases with water quality impairment and c) α- and β- functional diversity show significant variations among different types of hydromorphological conditions and different degrees of hydromorphological modification.
Material and methods
Macrophyte samplings
Field samplings were conducted in summer of 2021 and 2022 as part of the ecological monitoring program for the assessment of the ecological status of rivers of Greece in line with the EU Water Framework Directive (WFD) (Skoulikidis et al., 2021). We used presence-absence data of hydrophytes, plants that grow exclusively in water, from 74 river sites that belong to the national monitoring network for the ecological quality assessment of inland waters. The sampled sites extend across several biogeographic regions of Greece ranging from 37°N to 41°N and 20°E to 25°E and at altitudes spanning from sea level to 765 m a.s.l (Figure 1).
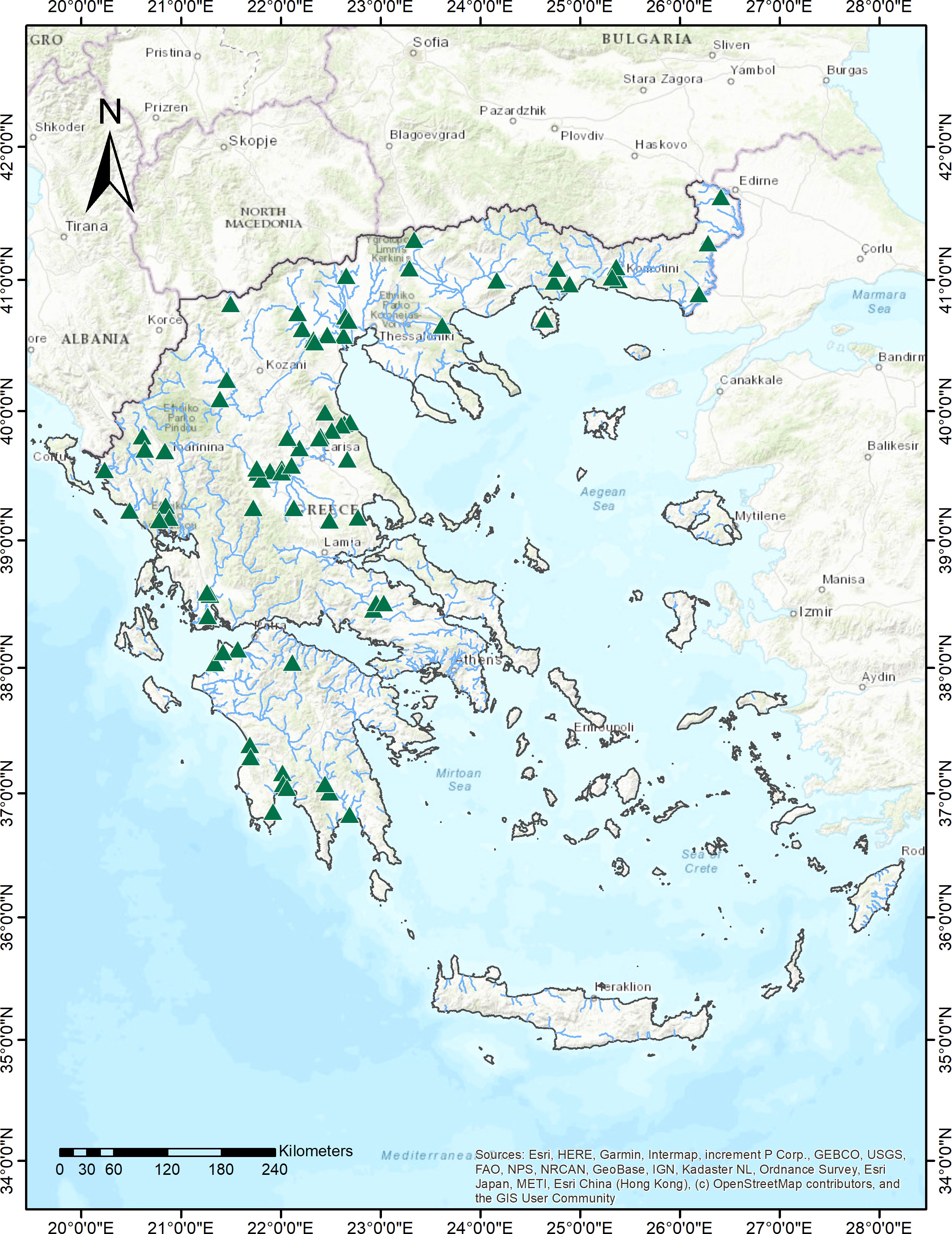
Figure 1 Map showing the location of sampling sites (N=74) of the National Monitoring network, across running waters of mainland Greece.
Macrophytes were sampled in accordance with national protocols harmonized with European standards (CEN, 2003; CEN, 2006). Sampling was conducted by wading into the water, following a zigzag pattern upstream for a 100 meter-long section of the river channel. Unidentified specimens were collected and transferred at the laboratory for identification.
Environmental parameters
We used geographical variables (latitude, longitude and altitude), physical and chemical parameters (electrical conductivity, total dissolved solids, pH, dissolved oxygen concentration, dissolved oxygen saturation and biochemical oxygen demand, nitrate, nitrite, ammonium, total nitrogen and total phosphorus) and hydromorphological characteristics in order to identify links between facets of plant diversity and environmental characteristics. Water physicochemical parameters and water samples for the determination of nutrients were obtained during samplings that were conducted during the same period with the macrophyte surveys. Hydromorphological features (e.g. type of channel substrate, effects of hydrological and morphological modifications, bed stability, channel shade) were assessed during the plant sampling according to Stefanidis et al. (2022). A description of the environmental variables that were considered in this study is listed in Table 1. For further details on field samplings, characteristics of river reaches, macrophyte identification and quantification of physicochemical and geomorphological variables see our publications (Stefanidis et al., 2021; Papastergiadou, 2022; Stefanidis et al., 2022).
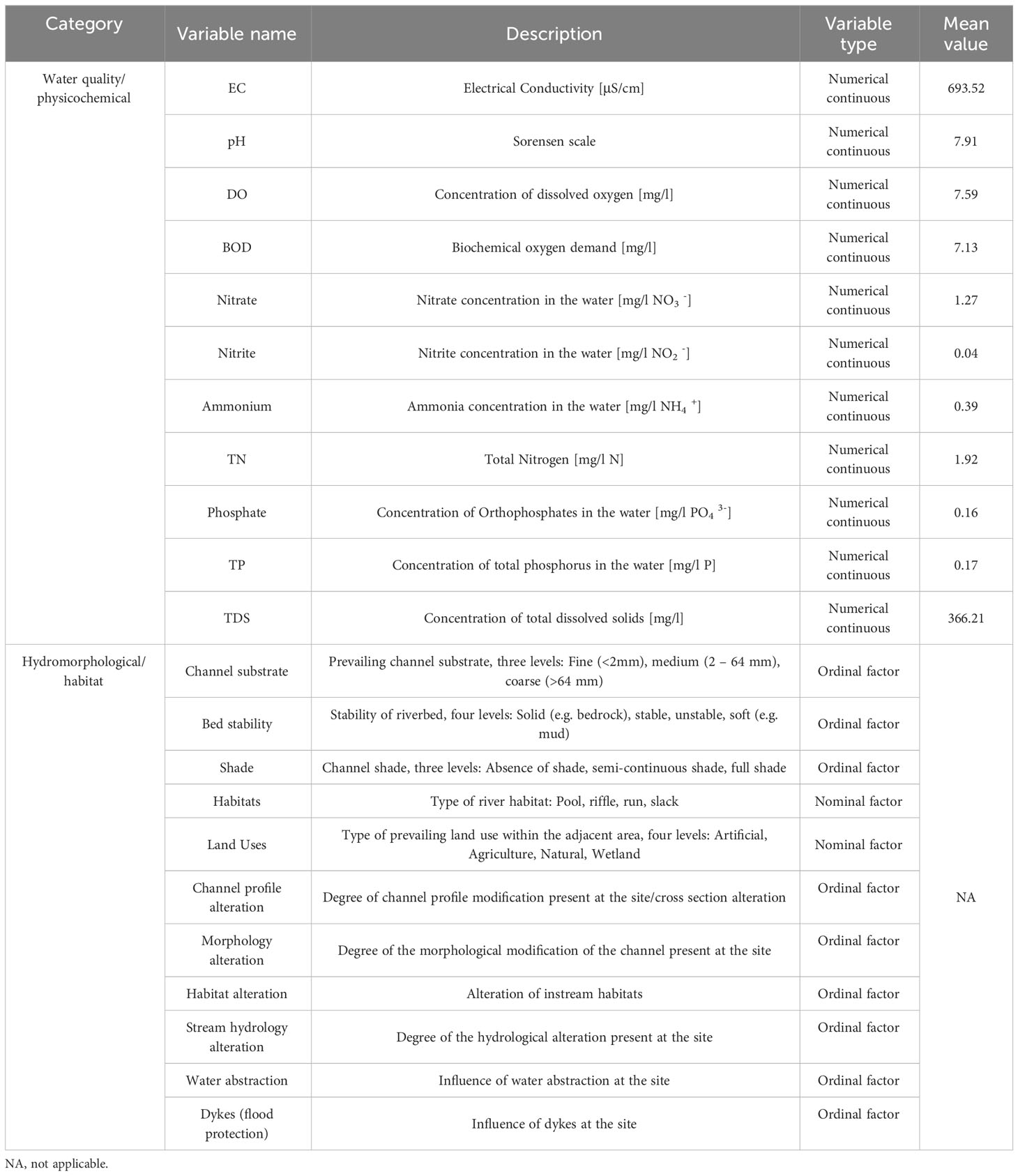
Table 1 Details and brief description of the environmental variables that were considered in this study.
Trait-based analysis and calculation of functional diversity indices
Patterns of functional composition and diversity of aquatic macrophytes were investigated following a methodological framework that is based on the construction of multidimensional functional space using community and trait data (Magneville et al., 2022). First, we created a matrix with presence-absence data of macrophyte species for the 74 river sites. Then, we constructed a matrix with functional community characteristics based on six nominal and ordinal plant traits allocated to a total of 36 hydrophyte species (Table 2). The traits that we used were the Ellenberg indicator values for nitrogen and light preference (Tichý et al., 2023), the life-form according to Wilby et al. (Willby et al., 2000), the leaf size and fruit size classified into three categories (small, moderate, and large) (Willby et al., 2000), and the leaf type classified into three types (entire, capillary and tubular) (Willby et al., 2000). Table 3 includes a list with the allocated traits that we used.
A functional distance matrix that contains the functional distances for each pair of species was calculated using the Gower distance, since all traits are categorical. Then, a hierarchical cluster analysis was conducted on the distance matrix to obtain groups of plants with similar functional assemblages. Following Kelley et al. (1996), we employed the Kelley–Gardner–Sutcliffe penalty function (KGS) to identify distinct clusters of the dendrogram. This method maximises differences between groups and cohesiveness within groups. The minimum of the KGS function corresponds to the optimal number of clusters. Functional space was then constructed based on the functional dissimilarity matrix using a principal coordinates analysis (PCoA) and functional diversity indices were calculated using the species coordinates on the first three principal components (Maire et al., 2015). Correlations between traits and the functional axes were identified with a Kruskal-Wallis test to help understand how plant groups are distributed across the functional space with regard to their trait composition.
Concerning the functional indices, Mouillot et al. (Mouillot et al., 2013) has proposed the use of several indices that act complementary and can provide useful insights about the functional community structure. Here we calculated three of these indices. Functional dispersion, which shows the deviation of species traits values from the center of the functional space filled by the assemblage, the functional richness which represents the amount of functional space occupied by a species assemblage, and the functional evenness which corresponds to how regularly species abundances are distributed in the functional space (Mason et al., 2005; Mouillot et al., 2013).
Beta (β) diversity was assessed as differences between all pairs of sites using the Sørensen index (βsor). The taxonomic and functional β dissimilarities are consistent with two additive components: the turnover component (replacement of species or functional space not shared by communities) and the nestedness-resultant component (difference in species or functional space filled by communities) (Baselga, 2010; Villéger et al., 2013). The turnover (βsim) and nestedness (βsne) components were quantified in accordance with the β-diversity partitioning framework proposed by Baselga (2010) and Villéger et al. (2013). The indices of β-diversity were calculated using the R package betapart (Baselga and Orme, 2012). These indices range from 0 to 1, where higher values indicate greater dissimilarities among sites.
By examining both taxonomic and functional β-diversity we intended to draw useful conclusions about the changes in taxonomic and functional composition and to associated them further with environmental characteristics. For the calculation of all functional diversity indices, we used the package “mFD” in R environment (Magneville et al., 2022). The correlations between taxonomic and functional β-diversity as well as between their respective components were tested using Mantel permutational tests.
Functional diversity and environmental parameters
We used Generalized Additive Models (GAM) to investigate the relationships between the alpha functional diversity indices, taxonomic diversity (species richness) and the water quality predictors (physical and chemical). GAMs have been commonly used in ecology for fitting non-linear relationships between species and environmental predictors (Guisan et al., 2002; Leathwick et al., 2006). Models were fitted with the “mgcv” package in R environment (Wood, 2020) using cubic smoothing splines. In order to assess the variation of alpha diversity indices among the levels of hydromorphological factors, we conducted Kruskall-Wallis tests.
Prior to the model fitting, predictors were tested for collinearity by calculating the variance inflation factor (VIF) with the vifstep function of the “usdm” package (Naimi et al., 2014) in R environment. Environmental variables with VIF > 3 were excluded from further analysis (Vittinghoff et al., 2012). The other variables were used for fitting full models with functional richness, functional dispersion and functional evenness as response variables. Then, using the dredge function from package “MuMIN” (Barton, 2020), the model with the lowest Akaike information criterion (AICc) value was selected as the final model.
Furthermore, we calculated Moran’s coefficients based on the geographical coordinates of the sites, to evaluate the spatial autocorrelation in each final model. Calculations were made with R package “ape” (Paradis and Schliep, 2019).
To investigate how β-diversity changes across water quality gradients, we used Generalized Dissimilarity models. Generalized Dissimilarity Models (GDMs) model the dissimilarity in species composition as a function of environmental and geographical parameters using dissimilarity matrices (Fitzpatrick et al., 2013). In our analysis, we used the default three I-spline basis functions per predictor was used and we plotted the I-splines to visually assess how magnitudes and rates of the total functional dissimilarity, functional turnover and nestedness change along the environmental gradients. The variable importance and significance of each environmental predictor were estimated based on matrix permutation. Specifically, the environmental data were permutated 50 times and for each permutated matrix a new model was fitted. The model significance was estimated by comparing the global deviance of the GDM fit to un-permutated data with that of permutated data. Then the same process was repeated for each predictor separately to assess the variable significance and importance. The GDMs were fitted with the package “gdm” in R environment (Fitzpatrick et al., 2020). Finally, we conducted a permutational multivariate analysis of variance (PERMANOVA) to assess whether functional dissimilarity differs among levels of hydromorphological factors. PERMANOVA was run with the adonis2 function of the “vegan” package in R environment.
Results
Patterns of trait composition
The hierarchical cluster analysis based on the functional distance matrix revealed five distinct groups of plants (Figure 2). The first group (I) consists of nine species of macrophytes, four of which are rooted floating leaved, another four are rooted submerged and one (Sparganium emersum) can be found with either emergent leaves or floating on the surface. The second (II) functional group of macrophytes consists of four species that are exclusively free-floating and Trapa natans which is a floating-leaved plant usually anchored at the sediment. The third group includes emergent macrophytes growing their stems and leaves above the water surface (e.g. Alisma plantago-aquatica, Mentha aquatica, Veronica anagalis-aquatica). The two remaining groups (IV, V) include submerged species that seem to vary because of their leaf type. Group IV consists of plants that are fine-leaved (e.g. Myriophyllum spicatum, Ranunculus trichophyllus, Ceratophyllum demersum), whereas Group V is characterized by a mix of aquatic macrophytes that can have submerged leaves (fine-leaves or entire leaves), floating leaves or both.
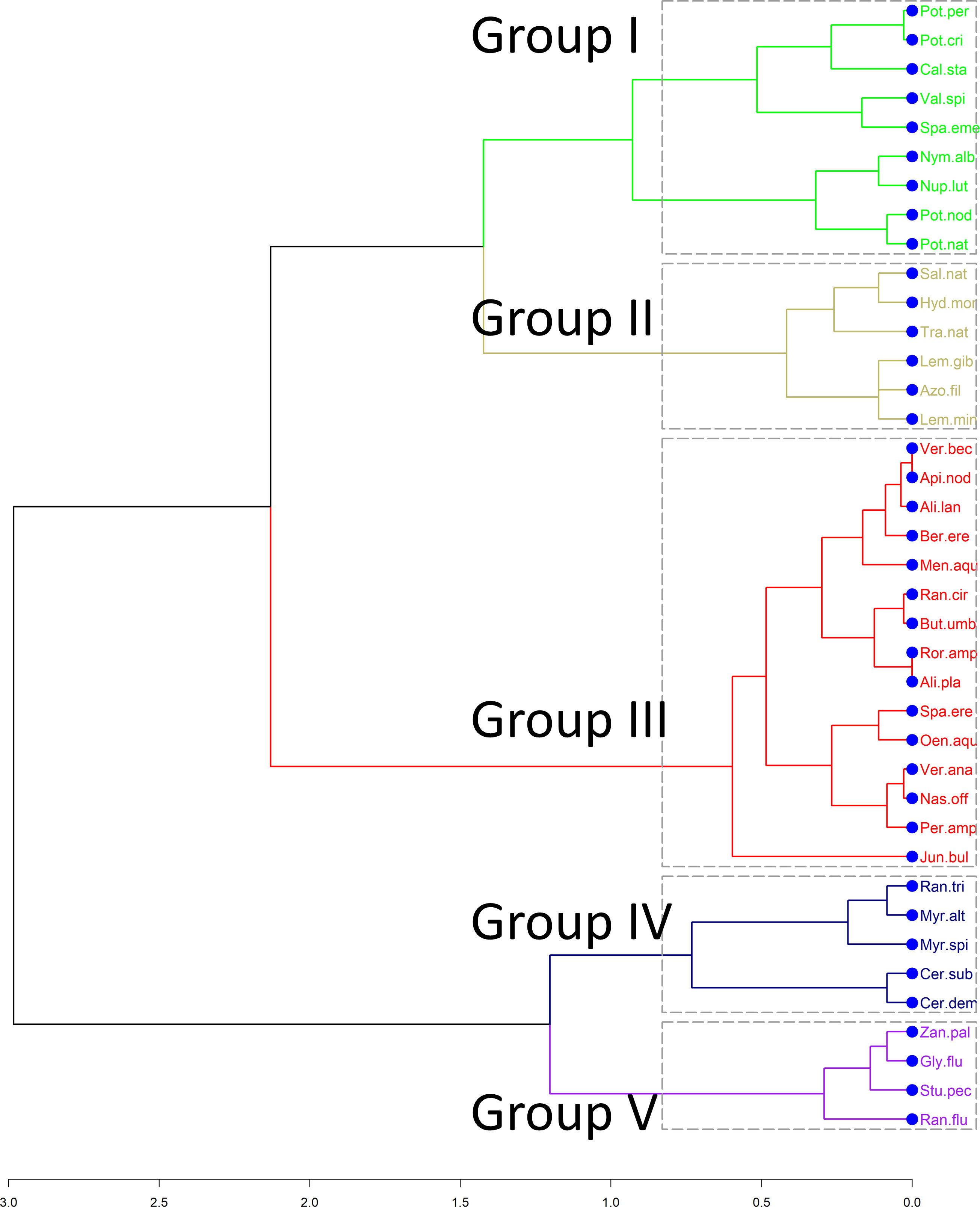
Figure 2 Dendrogram obtained from hierarchical clustering based on the species distances. Colors correspond to the five functional groups (I, II, III, IV, V) derived after the calculation of the Kelley-Gardner-Sutcliffe penalty.
The first two principal components of the PCA performed on trait data accounted for more than 60% of the variance among species characteristics (Figure 3). The first component (PC1) explained 53% of the total variance of the data, while the PC2 contributed with another 15%. Based on the boxplots of Figure 4, the first PC is mostly related with the traits Ellenberg light (EIV L), growth form (GF), leaf type (LT) and leaf size (LS), whereas the second PC is correlated significantly with Ellenberg light (EIV L), growth form (GF) and fruit size (FS), (Figure 4). Hence, the position of aquatic plants and functional groups along the two axes can indicate a strong affinity with specific functional traits and reflect specific ecological preferences. Plants that are more shade tolerant (lower values of EL) are positioned across the right part of the PCA plot (Groups IV and V) whereas plants that prefer good light conditions are clustered across the left part of the PC1 (mostly Group III), Figure 3. Macrophytes from Groups IV and V are also characterized by small and fine leaves opposed to Group III which consists of plants that have larger leaves and can be either emergent or floating-leaved. The position of the macrophytes across PC2 appears to relate mostly with the fruit size and the floating-leaved growth form, since macrophytes with floating leaves and larger fruits are positioned at the top part of the plot (Figures 3, 4).
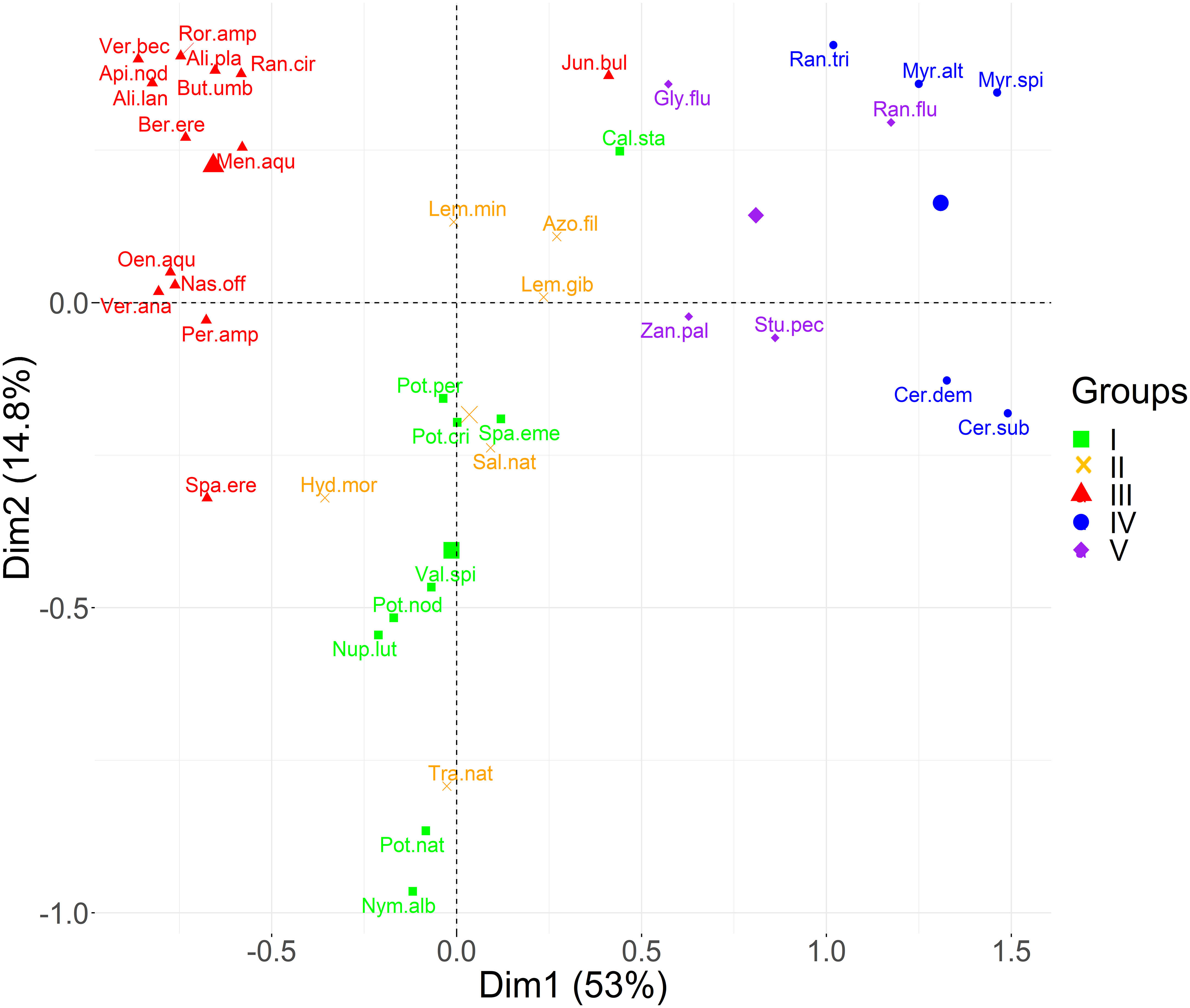
Figure 3 Principal component analysis plot of the species scores for the first two components. Colors correspond to the five functional groups (I, II, III, IV, V) derived after the calculation of the Kelley-Gardner-Sutcliffe penalty.
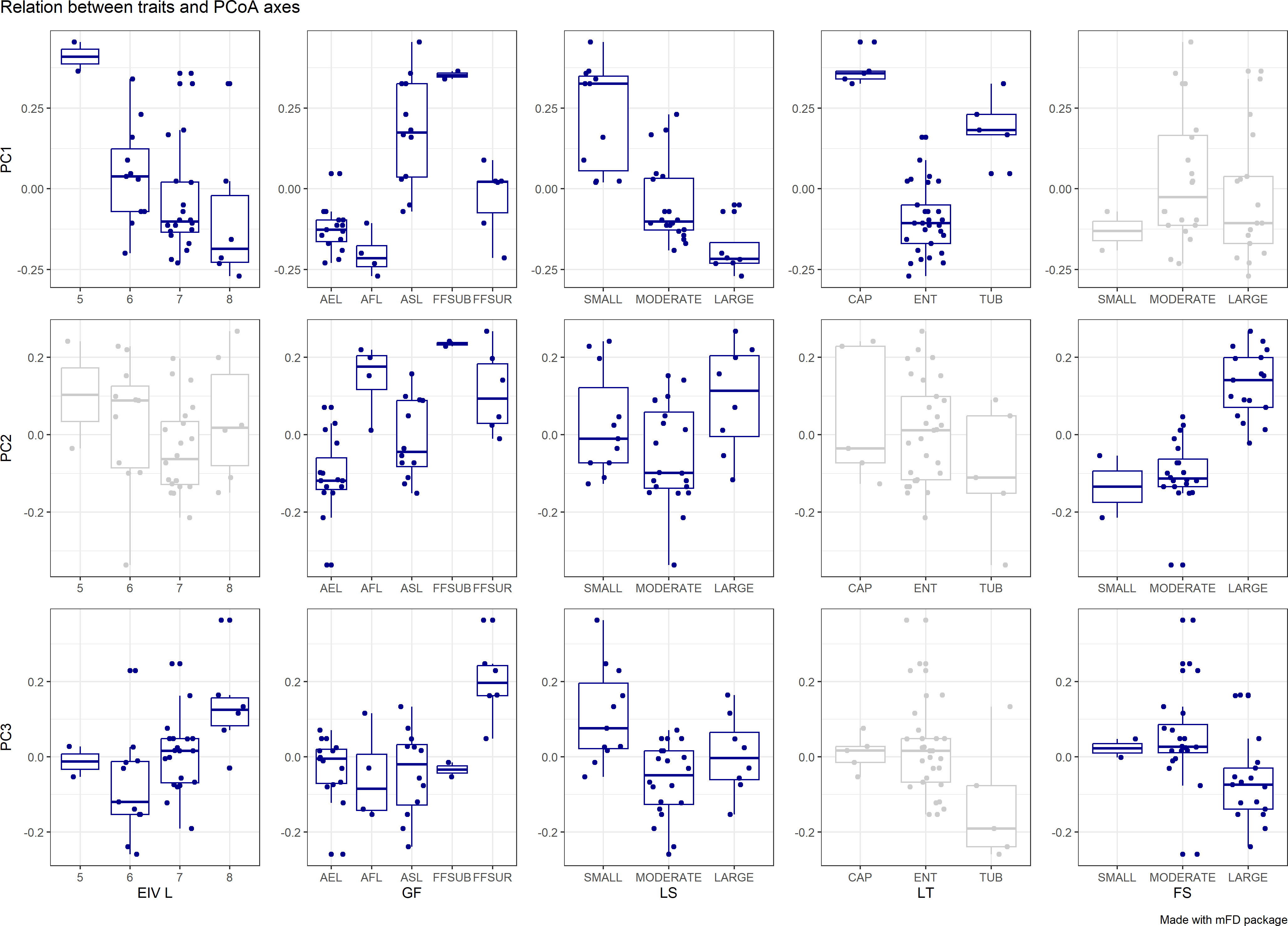
Figure 4 Boxplots show the relationship of the macrophyte species traits (EIV L: Ellenberg Light, GF: Growth Form, LS: Leaf Size, LT: Leaf Type, FS: Fruit Size) with the first three principal components. Dark blue plots indicate significant relationships (p ≤ 0.05).
Alpha (α-) and beta (β-) functional diversity patterns
Species richness was relatively low, ranging by a minimum of 4 to a maximum of 15 species per site. Alpha functional diversity was described by three indices: functional richness, dispersion and evenness. The average value of functional richness for all sites was 0.11, with a maximum of 0.39. Functional dispersion and evenness were higher ranging from 0.23 to 0.6 and 0.38 to 0.71 respectively. Additionally, we found that functional diversity was positively correlated (r=0.72) to taxonomic diversity (Figure 5). However, we have to note here that this result was influenced by a community found in a relatively pristine site, which showed remarkably higher species and functional richness than most communities. Excluding this community from our dataset yields a correlation coefficient r=0.59 (significant at p-value ≤ 0.001), which is still relatively high, but considerably lower than 0.72.
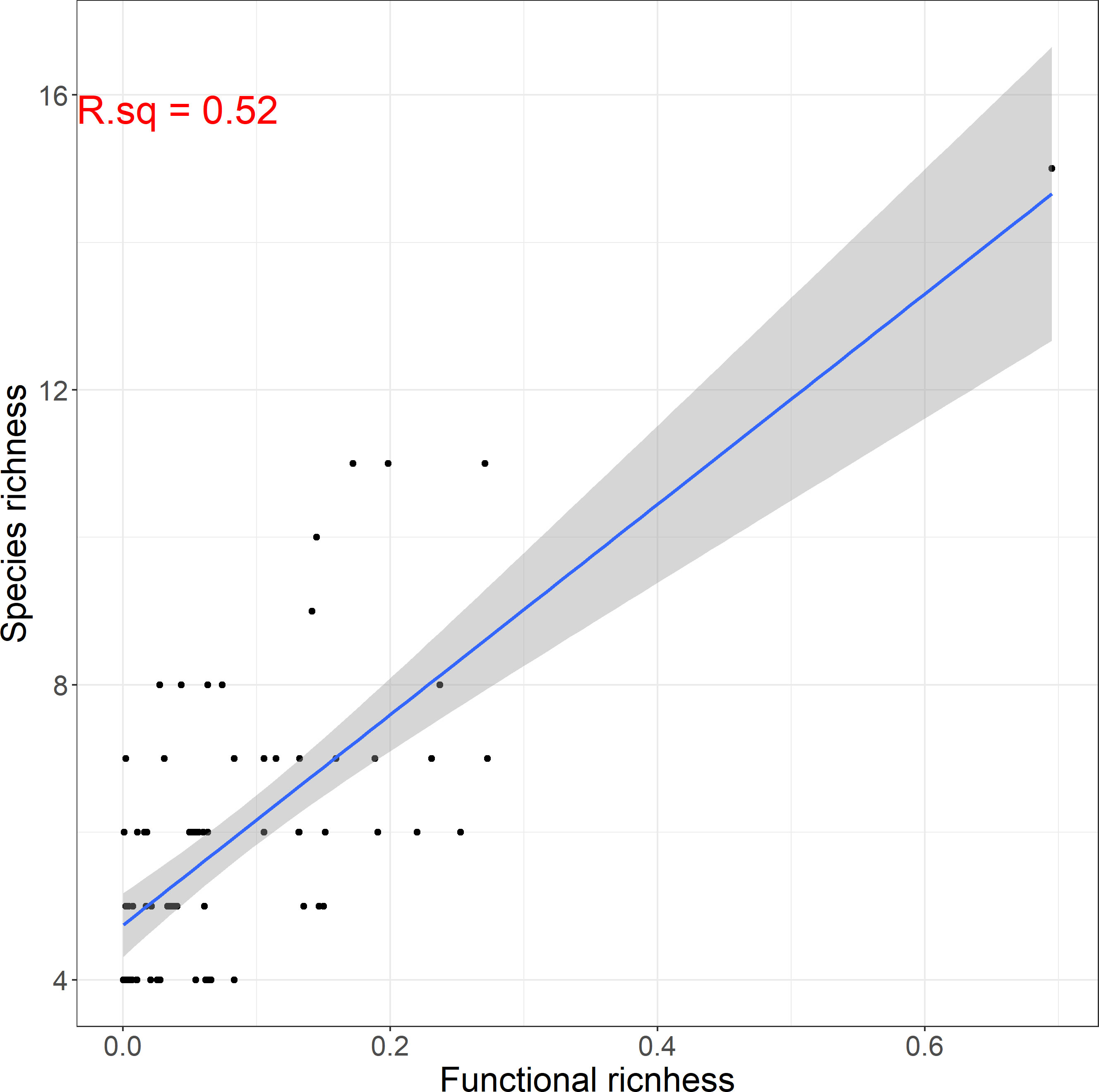
Figure 5 Linear relationship between functional and taxonomic macrophyte species richness among sites.
Taxonomic β diversity ranged from 0.08 to 1 with a mean value of 0.72 (± 0.19). The turnover (βsim, 0.65± 0.23) was higher than the nestedness-resultant component (βsne, 0.07). Functional dissimilarity based on Sørensen among the aquatic plant communities was high (0.85 ± 0.20), with the functional turnover (i.e. the communities host different functional strategies) accounting for 62% (0.62 ± 0.34) and dissimilarity due to difference in functional richness (nestedness) accounting for 23% of the total variation.
Functional β diversity was significantly correlated with taxonomic β diversity (Mantel test, r = 0.48, p < 0.001), with functional turnover and taxonomic turnover being also strongly correlated (r = 0.54, p < 0.001). Nestedness-resultant components of taxonomic and functional β diversity were also correlated (r = 0.48, P < 0.001).
Relationships between functional diversity indices and environmental descriptors
We tested for relationships between α functional diversity indices and water quality variables with the use of GAMs (Figure 6). The results of the best model fits showed that a relatively small share of variance of functional dispersion and functional evenness (25.6 and 25.3%) was explained by environmental variables. For functional richness the percentage of variance explained by the environment was even lower (approximately 11%). The best model for functional dispersion retained four predictors, with altitude, pH and oxygen saturation being significant (Table 4). For functional evenness the model retained five predictors with pH and oxygen saturation being significant (Table 4). The best model for functional richness, which had the lowest R2 among the three functional indicators, retained two variables (altitude and BOD) with neither being statistically significant.
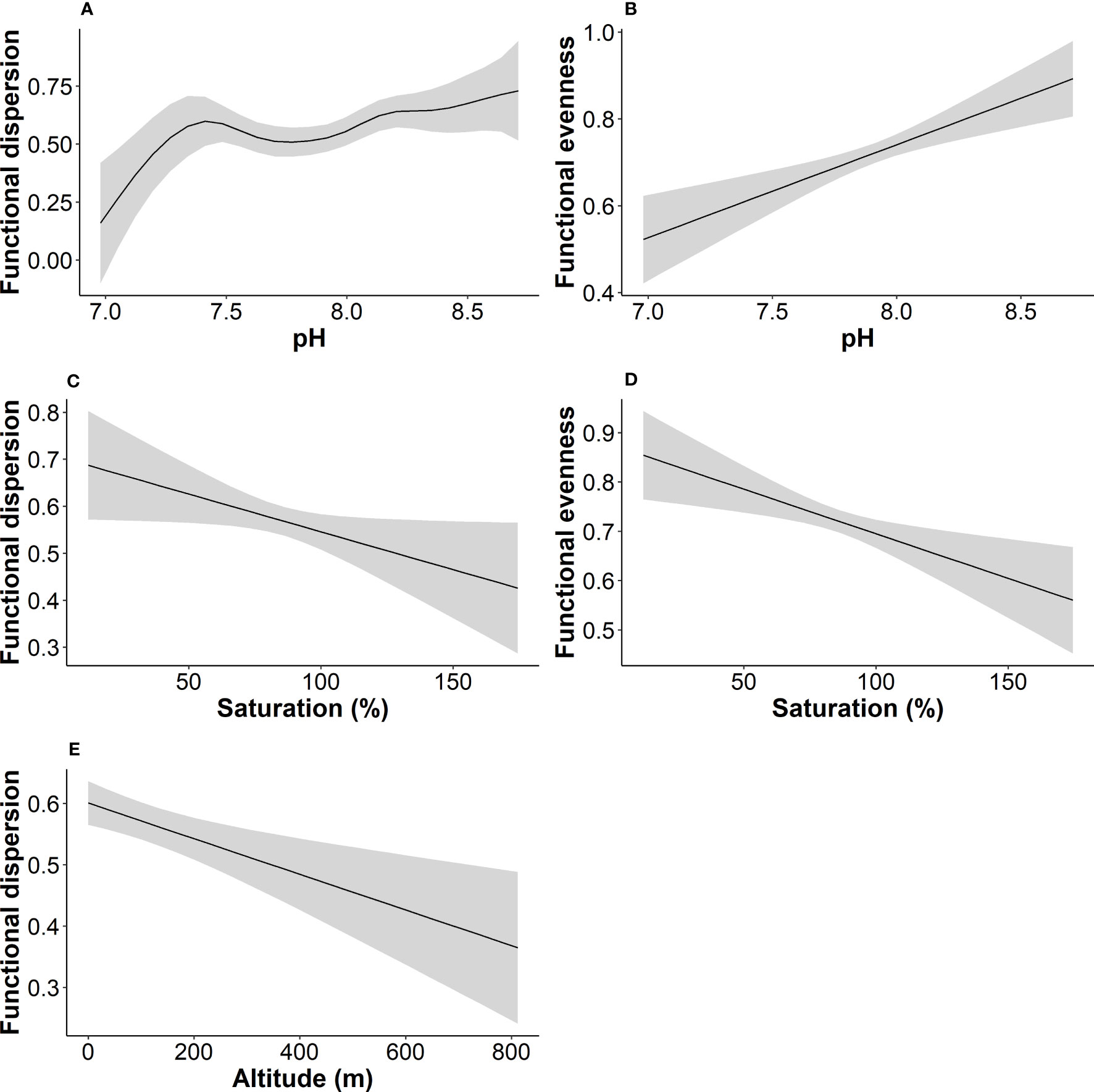
Figure 6 Fitted functions of functional dispersion (A, C, E), and functional evenness (B, D) to partial effects of statistically significant environmental variables. Line represents GAM smoothing curve while the grey area depicts the standard error.
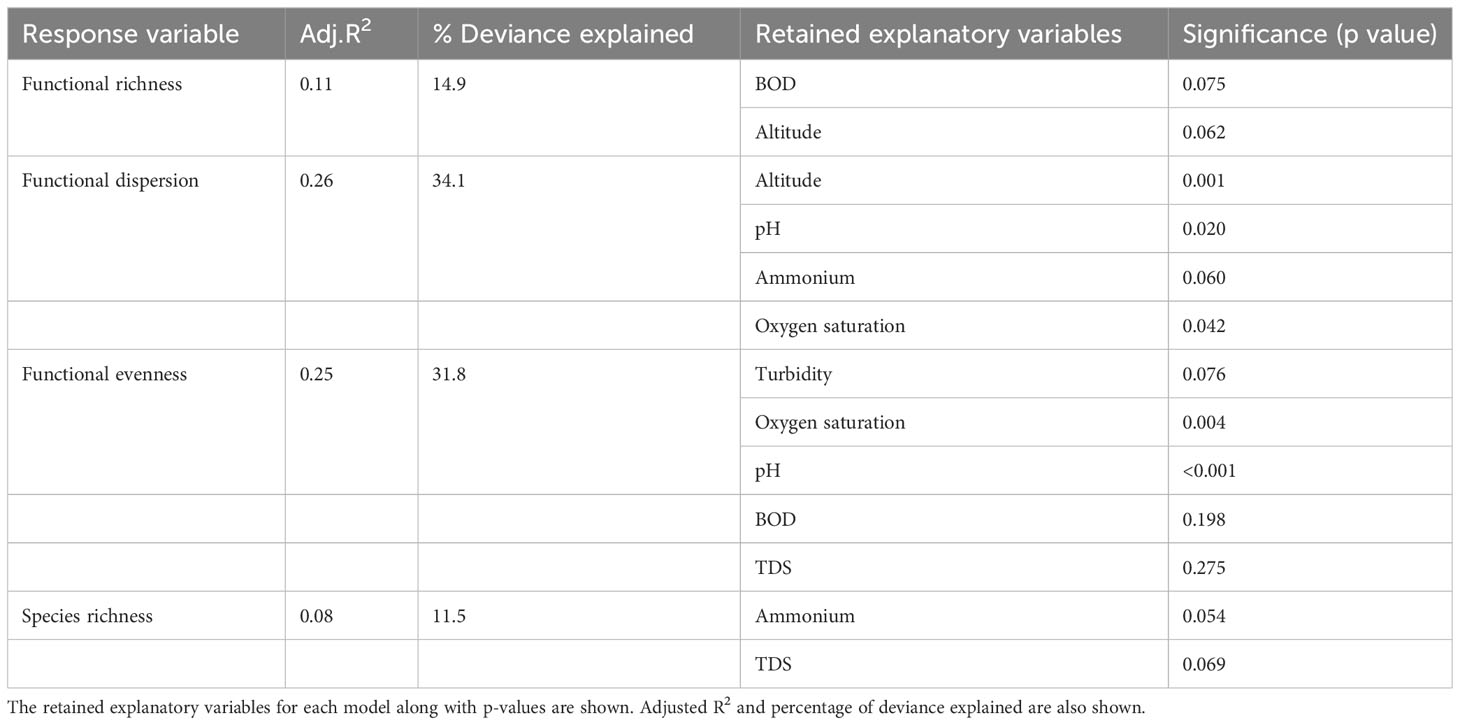
Table 4 Summary of GAMs fitted to the functional richness, functional dispersion, functional evenness and species richness.
The results of the Kruskal-Wallis tests indicated significant variations of α functional diversity indices (Table 5) among the channel bed stability (stable, solid, soft, unstable) and habitat type (pool, rifle, run, slack) (Figure 7). In addition, functional evenness and functional dispersion showed statistically significant differences among the channel substrate and the degree of hydrological alteration respectively. Concerning the species richness, we found less significant differences (p ≤ 0.1) among the habitat type, the adjacent land uses and the effect of water abstraction (Table 5).
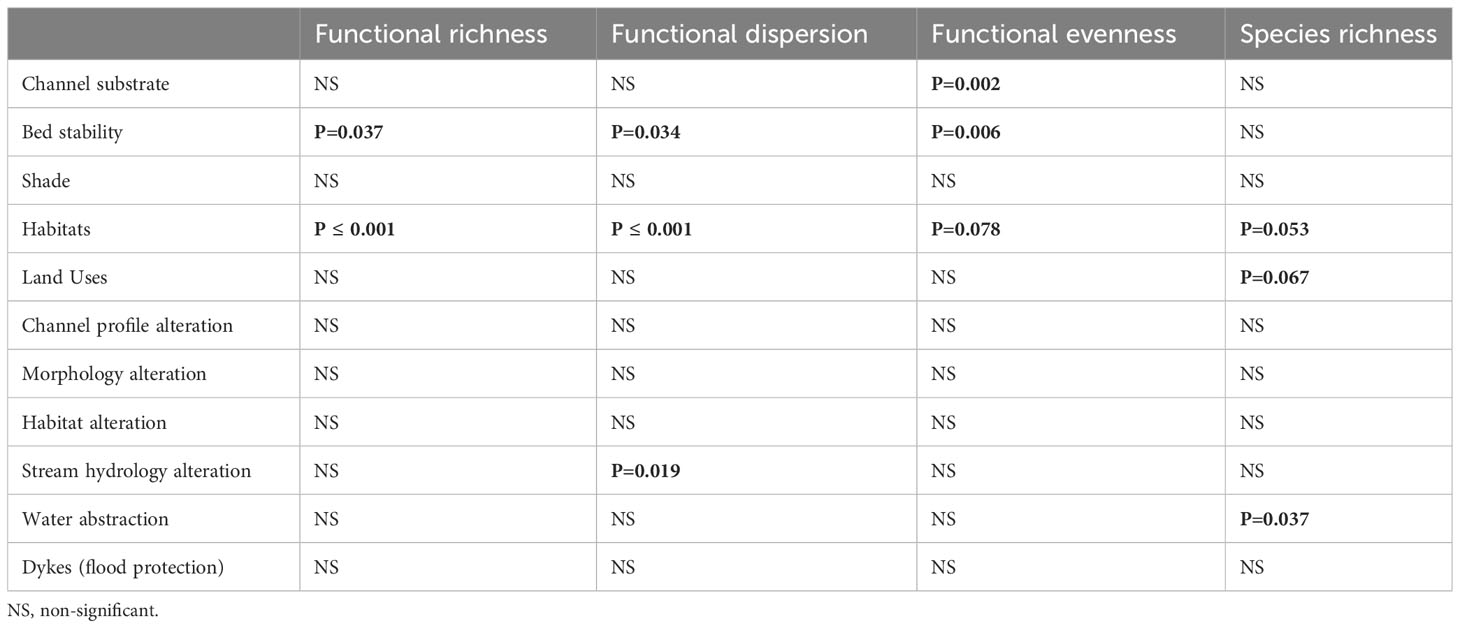
Table 5 Results of Kruskal-Wallis tests showing significant differences (values in bold) of the functional diversity indices among the levels of hydromorphological factors.
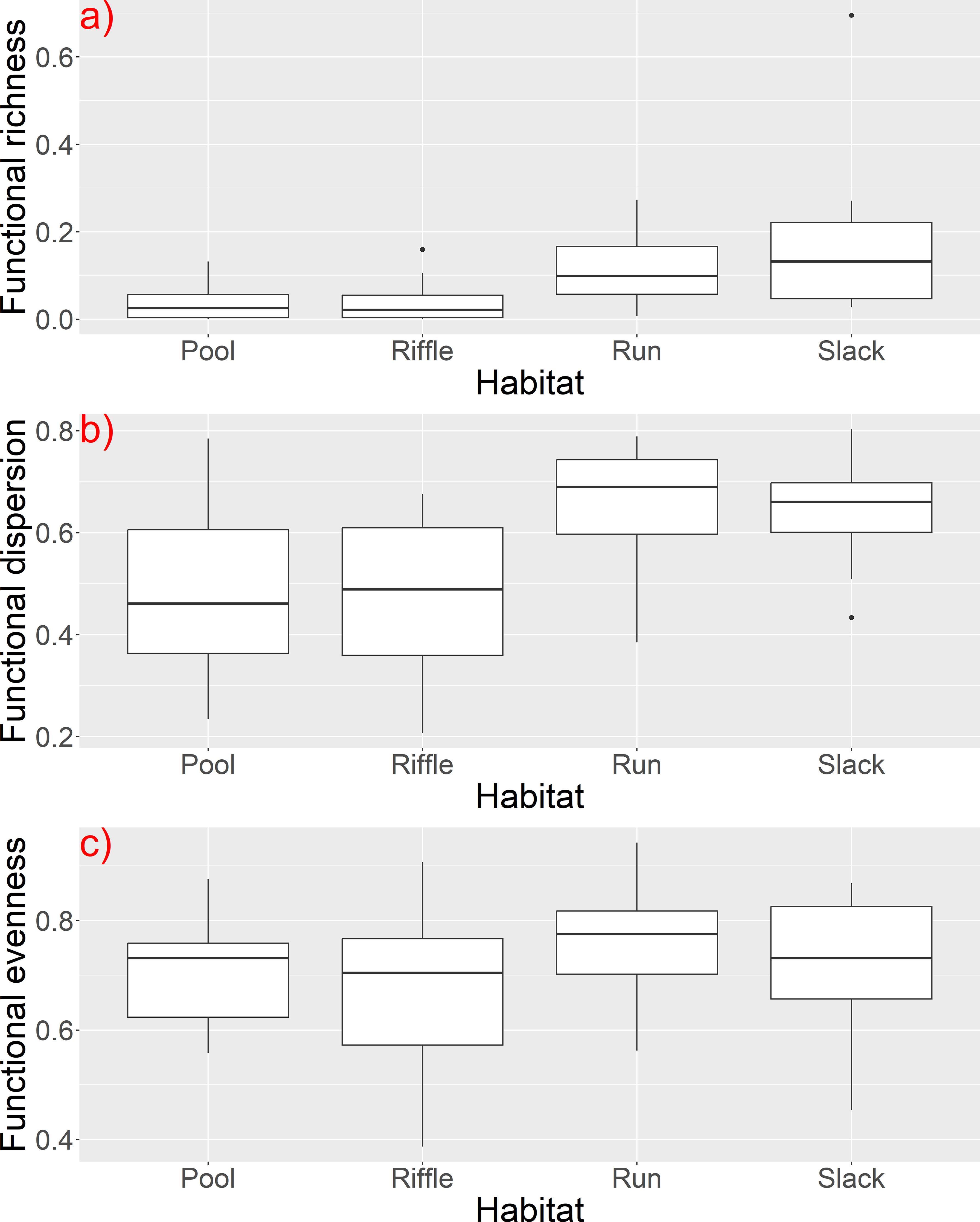
Figure 7 Boxplots of (A) functional richness, (B) dispersion and (C) evenness among river habitat types.
The results of the generalized dissimilarity modelling showed that geographical distance was the sole significant predictor for the Sørensen functional dissimilarity, although the model explained just the 8.5% of the total deviance. The models for the components turnover and nestedness explained even lower shares of total deviance (3.9 and 2.1) with none of the environmental variables being significant. We found similar results for the taxonomic dissimilarity with geographic distance being a significant predictor for Sørensen dissimilarity and the turnover component, but both models explained 6.9 and 7.4 of the total deviance respectively. The GDM for the taxonomic nestedness explained 3.2% of the total deviance, with none of the environmental variables being significant.
PERMANOVA showed significant variations of the Sørensen functional dissimilarity and the turnover component among most hydromorphological factors (p ≤ 0.1), but with low R2 ranging between 0.05 and 0.15 (Table 6).
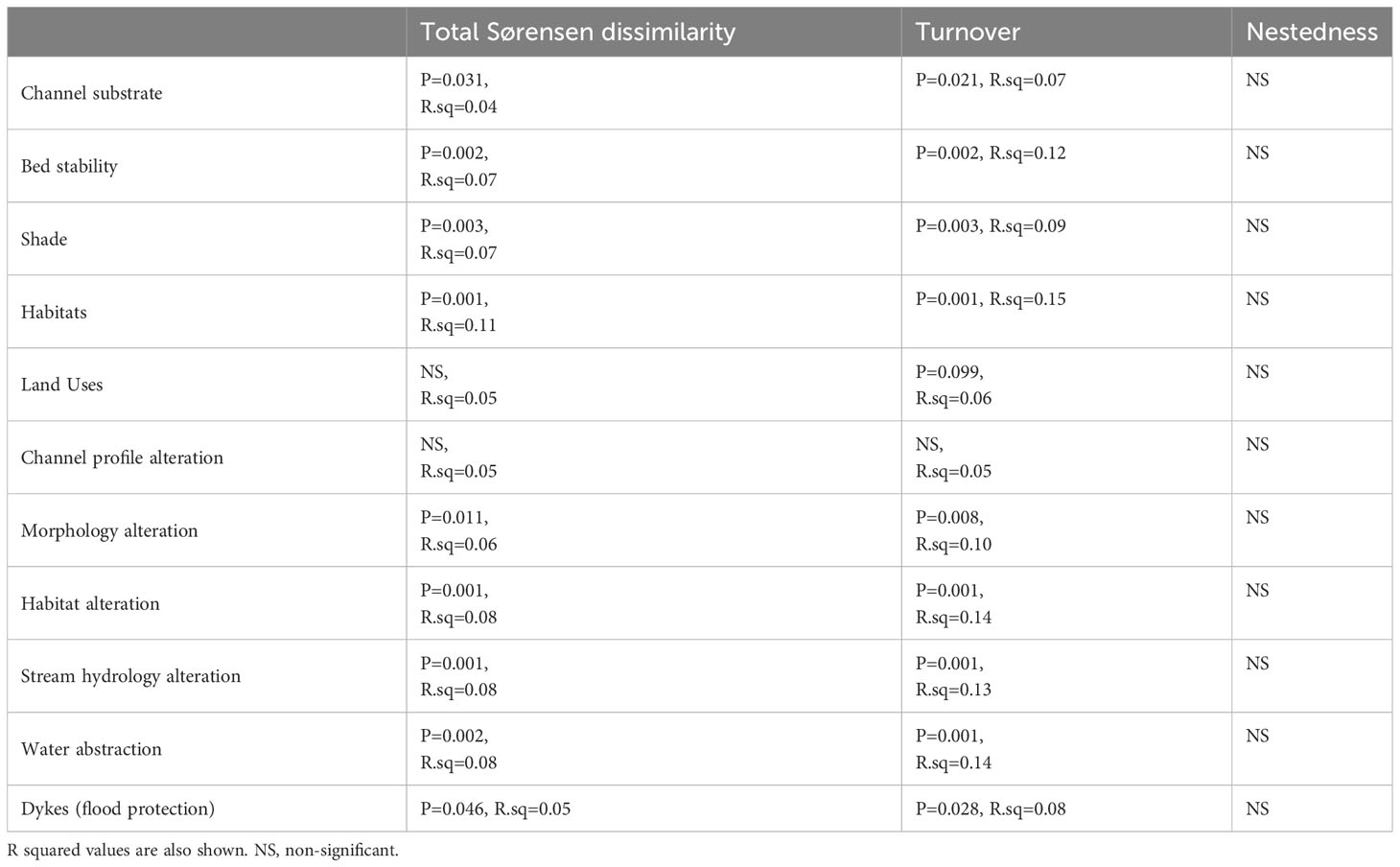
Table 6 Results of PERMANOVA showing significant variations of the functional dissimilarity matrices among the levels of hydromorphological factors.
Discussion
The study of plant ecomorphological traits attempts to link morphological characteristics (e.g. habitus size, shape and morphology) with species functions. In this article, we assessed functional diversity patterns and changes of functional composition of aquatic macrophytic communities of riverine ecosystems in Greece. We identified notable variations of the functional composition in terms of key trait characteristics, such as life growth form and preference to light conditions. We also managed to distinguish a few significant relationships between functional diversity (alpha and functional dissimilarity) and environmental variables.
Our results indicated that growth form and light preference are key trait characteristics that grouped the aquatic macrophytes into five discrete groups based on their functional trait composition. Macrophytes were distinguished along a gradient that reveals light availability as a key driver that shapes macrophytic communities in the investigated river reaches. The PC1 has a high affinity with rooted submerged macrophytes and macrophytes with high EIV L value (which indicates preference to higher light intensity) and thus plants that were positioned at the left part of the PCA plot are those that could require waters with high clarity and undisturbed conditions (e.g. oxygenated waters and less turbidity). Previous studies have highlighted the role of light availability as an important environmental filter that restricts certain functional traits of aquatic macrophytes (Fu et al., 2014b; Su et al., 2019) and promoting others that can help plants to persist to the environmental conditions. However, when we examined the relationship between functional diversity indices and environmental variables, including water quality features that are related with light availability (e.g. turbidity and nutrient concentrations), we did not find evidence that could explain a possible effect of water clarity on functional diversity. Specifically, we did not find any significant effect of water quality parameters on functional richness, but only a significant effect of pH and oxygen saturation on both functional dispersion and evenness. Similarly, we did not find any significant effect of water quality variables on the taxonomic richness. Previous studies in Greek rivers have noted relatively moderate and high levels of nutrients in several sites, indicating signs of eutrophication (Stefanidis et al., 2021). Narrow gradients of nutrient concentrations and high occurrence of macrophytes that can be found in a wide range of trophic conditions (e.g. Myriophyllum spicatum, Potamogeton nodosus, Stuckenia pectinata) may explain the difficulty in finding specific patterns of responses along water quality gradients. These results are similar with those published by Zelnik et al. (2021), who also found Myriophyllum spicatum as the most common aquatic macrophyte species in watercourses in Slovenia, despite relatively different environmental conditions from those in Greece. Among the submerged macrophytes or macrophytes with floating leaves, the most common species were: Myriophyllum spicatum, Potamogeton nodosus, Potamogeton perfoliatus, Elodea canadensis, Potamogeton crispus, Stuckenia pectinata. This is another evidence for wide ecological amplitudes of these species, which make the interpretations more difficult. Furthermore, excessive nutrients are related with eutrophication processes that reduce light availability and may have substantial effects on taxonomic and functional diversity in standing waters (Stefanidis and Papastergiadou, 2019; Lindholm et al., 2020). However, water transparency in rivers can be affected by geohydromorphological factors such as erosion, sediment load, geology, land uses and rainfall intensity (Chalov and Prokopeva, 2022; Lu et al., 2023) that are not necessarily related with water chemistry (e.g. nutrients). Our findings also showed a significant differentiation of the functional diversity indices among the types of bed stability (stable, solid, soft and unstable) and the types of habitat (pool, rifle, run and slack), which suggests that hydromorphological conditions (e.g. substrate rigidness, depth and flow type) are important drivers of certain plant traits. Functional richness, dispersion and evenness were higher at slack and run habitats, which are characterized by deeper waters and slow or fast water flow respectively. All three indices were also higher at soft substrates (mostly sand, silt and mud) than more stable substrates (e.g. bedrock, gravel, cobbles and boulders). Similarly, functional evenness was higher at fine substrates (p=0.002) which confirms a possible association of increased functional diversity with soft and fine river substrates. Previous studies have shown that geomorphological features such as river bottom type, substrate structure and riverbank stability are important factors for explaining macrophyte composition in lotic ecosystems (Peternel et al., 2022). Rooted aquatic plants in particular, prefer fine sediments (Willby et al., 2000; Hrivnák et al., 2010) while bryophytes usually occur at coarser substrates, such as boulders and cobbles. Thus, life forms are differentiated among various types of substrates. Moreover, macrophytes are considered to be ecological engineers because macrophyte assemblages have a positive impact on fine sediment accumulation on the river bottom, modifying the channel bed and facilitating plant colonization (Jones et al., 2012). We did not find any other significant differentiation of α diversity indices among the remainder hydromorphological factors, except for a significant effect of hydrological alteration and water abstraction on functional dispersion and evenness respectively. Although aquatic plants are known to respond to hydromorphological changes (Szoszkiewicz et al., 2014; Baattrup-Pedersen et al., 2016; Turunen et al., 2016; Birk et al., 2020; Gyosheva et al., 2020), only a few studies have highlighted the role of hydromorphology as an important driver of aquatic macrophytic diversity, including functional diversity (Manolaki et al., 2020; Stefanidis et al., 2021; Vukov et al., 2022). Besides substrate, hydrology plays a major role in promoting species with traits that enable them to persist droughts and low flow conditions in Mediterranean rivers (Manolaki et al., 2020). In our case, we used nominal and ordinal hydromorphological variables that limited our capability to fully explore how functional diversity changes across hydromorphological gradients (e.g. hydrological alteration). Still, we were able to capture the effect of different substrates and river habitats and extract useful conclusions on the conditions that favor increased functional diversity.
Another issue that might explain why we did not find significant relationships between certain environmental parameters and diversity indices, is the intraspecific trait variability that many plants exhibit, including aquatic plants (Fu et al., 2014b). Some plants may show high phenotypic plasticity that provides them with various adaptations to large environmental changes (Fu et al., 2018; Lindholm et al., 2020). For instance, a submerged macrophyte in China, Potamogeton maackianus, can form large monospecific beds across wide environmental gradients (Fu et al., 2018). Similar macrophyte assemblages with a few species that occur across wide ranges of nutrients and physico-chemical gradients are common in Greece (Stefanidis et al., 2019), such as Myriophyllum spicatum and Potamogeton nodosus. Other plants can show adaptations to water level fluctuations showing various growth forms (e.g. emergent, rooted with floating-leaved plants, or rooted submerged) that can help them offset environmental limitations such as limited light availability. It is likely that the inclusion of intraspecific trait variability in trait-based studies could further elucidate the functional responses to environment gradients.
An additional finding of the current research concerns the relationship between taxonomic and functional diversity. The relationship between taxonomic and functional richness has been previously used to investigate the functional redundancy of communities (Ricotta et al., 2016; da Silva Camilo et al., 2018). In this study, we found a positive significant relationship between functional and taxonomic richness that indicates low functional redundancy. This means that the loss of a few species from the macrophyte communities is more likely to lead to loss of certain functions that are strictly related with those species. This finding is particularly important for conservation scientists and environmental managers because it emphasizes the need to include the monitoring of functional diversity (besides taxonomic diversity) in order to better assess the impact of environmental changes (Ricotta et al., 2016; Biggs et al., 2020).
Separating turnover and nestedness-resultant contributions to the overall β-diversity could provide further insights into mechanisms shaping community composition with respect to β-diversity. We found that the taxonomic β-diversity was mainly governed by turnover, i.e., replacement of disappearing species by new emerging species along the gradient, which is a typical finding in freshwater studies (Perez Rocha et al., 2019). Studies that explore the relationship between taxonomic and functional beta diversity in freshwaters are not common, and their results are rather ambiguous (Perez Rocha et al., 2019; Teittinen and Virta, 2021). In the current research, we found that functional β-diversity was higher than taxonomic β-diversity. The high functional dissimilarity may partly stem from low number of taxonomic species versus considerably higher number of functional traits or functions. A discrepancy was also revealed in the decomposition of functional β-diversity, where the higher levels of functional β-diversity were mainly due to a higher nestedness-resultant component compared to taxonomic β-diversity decomposition, where the taxonomic and functional β-diversity turnover were at similar levels. Thus, replaced species in functionally poor assemblages held traits already included in the functional space of functionally rich ones, resulting in increased functional nestedness. The results of the GDM did not show strong indications of environmental effects on the taxonomic and functional dissimilarity rate and thus our study does not provide support for the role of environmental filtering as a driver of neither functional nor community dissimilarities. The PERMANOVA results showed significant variation of the overall β-diversity and the turnover among the majority of hydromorphological factors but the R2 values were quite low indicating a large share of unexplained variation. It is likely that regional spatial processes (dispersal limitation with increasing geographic distance) are more important factors than local environmental descriptors for aquatic macrophyte taxonomic and functional composition changes among sites (Oikonomou and Stefanidis, 2020; Stefanidis et al., 2021).
Conclusions
This article provides new information filling the gap of knowledge of the functional responses of aquatic macrophytic assemblages to environmental gradients in an extended network of running waters from mainland Greece. With this article we quantified the a- and β- functional diversity of aquatic macrophytic communities of river reaches and we attempted to look for significant responses to environmental parameters related with water quality gradients and hydromorphological factors. We found that the trait characteristics that contributed most to explaining the total variance of the functional space were the macrophyte growth form and the preference to light conditions which indicates that light availability plays a major role in filtering traits of aquatic plants. We did not find any clear indication of strong relationships between functional diversity and water quality gradients. We found significant variations of alpha and beta functional diversity among hydromorphological factors - mainly substrate and river habitat - which suggested that lotic systems with fine substrates and deep waters (run and slack habitats) promoted functional diversity. We consider likely that further studies to explore the effects of additional hydromorphological gradients could reveal significant responses of functional plant communities. An important finding was the positive relationship between species richness and functional richness which implies that the loss of taxonomic richness could lead to a loss of functions. Overall, our study provides useful insights and recommendations concerning the study of functional diversity of aquatic plant assemblages within the frame of freshwater monitoring and conservation.
Data availability statement
The raw data supporting the conclusions of this article will be made available by the authors, without undue reservation.
Author contributions
KS, AO and EP contributed to the conception and design of the study. EP, GD, MS, DT and KS contributed to data compilation. KS and AO analysed the data and wrote the manuscript. KS, AO and EP authors contributed to the final version of the manuscript.
Funding
This research was funded by European and National grants from the Hellenic Centre for Marine Research under the “Monitoring of ecological quality of Greek rivers for the Implementation of Article 8 of WFD 2000/60/EE: samplings and analyses of aquatic macrophytes” research project. The publication of this article has been financed by the Research Committee of the University of Patras, funding number 873/25.07.2023.
Acknowledgments
We would like to thank Dionysios Mermygkas, Maria Sarika, Konstantina Christopoulou, Ionna Xynogala, and the staff of Patras Laboratory of Ecology for field work assistance and collection of the samples. We greatly appreciate the help of Angeliki Tsanta for the linguistic revision of the article, and reviewers for their invaluable comments and recommendations that helped us improve our article. We would also like to express our gratitude to the Research Committee of the University of Patras for the support on managing the project and related activities.
Conflict of interest
The authors declare that the research was conducted in the absence of any commercial or financial relationships that could be construed as a potential conflict of interest.
Publisher’s note
All claims expressed in this article are solely those of the authors and do not necessarily represent those of their affiliated organizations, or those of the publisher, the editors and the reviewers. Any product that may be evaluated in this article, or claim that may be made by its manufacturer, is not guaranteed or endorsed by the publisher.
References
Aguiar, F. C., Segurado, P., Urbanič, G., Cambra, J., Chauvin, C., Ciadamidaro, S., et al. (2014). Comparability of river quality assessment using macrophytes: A multi-step procedure to overcome biogeographical differences. Sci. Total Environ. 476–477, 757–767. doi: 10.1016/j.scitotenv.2013.10.021
Alahuhta, J., Toivanen, M., Hjort, J., Ecke, F., Johnson, L. B., Sass, L., et al. (2017). Species richness and taxonomic distinctness of lake macrophytes along environmental gradients in two continents. Freshw. Biol. 62, 1194–1206. doi: 10.1111/fwb.12936
Baattrup-Pedersen, A., Göthe, E., Riis, T., O’Hare, M. T. (2016). Functional trait composition of aquatic plants can serve to disentangle multiple interacting stressors in lowland streams. Sci. Total Environ. 543, 230–238. doi: 10.1016/j.scitotenv.2015.11.027
Baselga, A. (2010). Partitioning the turnover and nestedness components of beta diversity. Global Ecol. Biogeogr. 19, 134–143. doi: 10.1111/j.1466-8238.2009.00490.x
Baselga, A., Orme, C. D. L. (2012). Betapart: An R package for the study of beta diversity. Methods Ecol. Evol. 3, 808–812. doi: 10.1111/j.2041-210X.2012.00224.x
Bellwood, D. R., Wainwright, P. C., Fulton, C. J., Hoey, A. (2002). Assembly rules and functional groups at global biogeographical scales. Funct. Ecol. 16, 557–562. doi: 10.1046/j.1365-2435.2002.00655.x
Biggs, C. R., Yeager, L. A., Bolser, D. G., Bonsell, C., Dichiera, A. M., Hou, Z., et al. (2020). Does functional redundancy affect ecological stability and resilience? A review and meta-analysis. Ecosphere 11, e03184. doi: 10.1002/ecs2.3184
Birk, S., Chapman, D., Carvalho, L., Spears, B. M., Andersen, H. E., Argillier, C., et al. (2020). Impacts of multiple stressors on freshwater biota across spatial scales and ecosystems. Nat. Ecol. Evol. 4, 1060–1068. doi: 10.1038/s41559-020-1216-4
Birk, S., Willby, N. (2010). Towards harmonization of ecological quality classification: Establishing common grounds in European macrophyte assessment for rivers. Hydrobiologia 652, 149–163. doi: 10.1007/s10750-010-0327-3
Bornette, G., Puijalon, S. (2011). Response of aquatic plants to abiotic factors: A review. Aquat. Sci. 73, 1–14. doi: 10.1007/s00027-010-0162-7
CEN. (2003). EN 14184:2003 - Water quality - Guidance standard for the surveying of aquatic macrophytes in running waters (European Committee for Standardization (CEN)).
CEN. (2006). EN 14996:2006 - Water quality - Guidance on assuring the quality of biological and ecological assessments in the aquatic environment. Available at: https://standards.iteh.ai/catalog/standards/cen/804ddce8-966c-4ee9-99ea-b18798e71526/en-14996-2006 (Accessed October 6, 2020).
Chalov, S., Prokopeva, K. (2022). Sedimentation and erosion patterns of the Lena river anabranching channel. Water (Switzerland) 14, 3845. doi: 10.3390/w14233845
Cole, L. J., Stockan, J., Helliwell, R. (2020). Managing riparian buffer strips to optimise ecosystem services: A review. Agric. Ecosyst. Environ. 296, 106891. doi: 10.1016/j.agee.2020.106891
da Silva Camilo, G., de Freitas Terra, B., Araújo, F. G. (2018). Using the relationship between taxonomic and functional diversity to assess functional redundancy in streams of an altered tropical watershed. Environ. Biol. Fishes 101, 1395–1405. doi: 10.1007/s10641-018-0786-3
Degen, R., Aune, M., Bluhm, B. A., Cassidy, C., Kędra, M., Kraan, C., et al. (2018). Trait-based approaches in rapidly changing ecosystems: A roadmap to the future polar oceans. Ecol. Indic. 91, 722–736. doi: 10.1016/j.ecolind.2018.04.050
Díaz, S., Lavorel, S., De Bello, F., Quétier, F., Grigulis, K., Robson, T. M. (2007). Incorporating plant functional diversity effects in ecosystem service assessments. Proc. Natl. Acad. Sci. U.S.A. 104, 20684–20689. doi: 10.1073/PNAS.0704716104/SUPPL_FILE/DIAZ_FINAL.MP3
Dolédec, S., Phillips, N., Scarsbrook, M., Riley, R. H., Townsend, C. R. (2006). Comparison of structural and functional approaches to determining landuse effects on grassland stream invertebrate communities. J. North Am. Benthol. Soc. 25, 44–60. doi: 10.1899/0887-3593(2006)25[44:COSAFA]2.0.CO;2
Dybkjaer, J. B., Baattrup-Pedersen, A., Kronvang, B., Thodsen, H. (2012). Diversity and distribution of riparian plant communities in relation to stream size and eutrophication. J. Environ. Qual. 41, 348–354. doi: 10.2134/jeq2010.0422
Elo, M., Alahuhta, J., Kanninen, A., Meissner, K. K., Seppälä, K., Mönkkönen, M. (2018). Environmental characteristics and anthropogenic impact jointly modify aquatic macrophyte species diversity. Front. Plant Sci. 9. doi: 10.3389/fpls.2018.01001
Fitzpatrick, M., Mokany, K., Manion, G., Lisk, M., Fer-rier, S., Nieto-Lugilde, D., et al. (2020). Package “gdm”: Generalized Dissimilarity Modeling.
Fitzpatrick, M. C., Sanders, N. J., Normand, S., Svenning, J. C., Ferrier, S., Gove, A. D., et al. (2013). Environmental and historical imprints on beta diversity: Insights from variation in rates of species turnover along gradients. Proc. R. Soc. B: Biol. Sci. 280, 20131201. doi: 10.1098/rspb.2013.1201
Fu, H., Yuan, G., Lou, Q., Dai, T., Xu, J., Cao, T., et al. (2018). Functional traits mediated cascading effects of water depth and light availability on temporal stability of a macrophyte species. Ecol. Indic. 89, 168–174. doi: 10.1016/j.ecolind.2018.02.010
Fu, H., Zhong, J., Yuan, G., Ni, L., Xie, P., Cao, T. (2014a). Functional traits composition predict macrophytes community productivity along a water depth gradient in a freshwater lake. Ecol. Evol. 4, 1516–1523. doi: 10.1002/ece3.1022
Fu, H., Zhong, J., Yuan, G., Xie, P., Guo, L., Zhang, X., et al. (2014b). Trait-based community assembly of aquatic macrophytes along a water depth gradient in a freshwater lake. Freshw. Biol. 59, 2462–2471. doi: 10.1111/fwb.12443
Guisan, A., Edward, T. C., Hastie, T. (2002). Generalized linear and generalized additive models in studies of species distributions: setting the scene. Ecol. Modell. 157, 89–100. doi: 10.1016/S0304-3800(02)00204-1
Gurnell, A. (2015). Plants as river system engineers: Further comments. Earth Surf. Process Landf 40, 135–137. doi: 10.1002/esp.3671
Gyosheva, B., Kalchev, R., Beshkova, M., Valchev, V. (2020). Relationships between macrophyte species, their life forms and environmental factors in floodplain water bodies from the Bulgarian Danube River Basin. Ecohydrol. Hydrobiol. 20, 123–133. doi: 10.1016/j.ecohyd.2019.06.003
Hrivnák, R., Ot’ahel’ová, H., Valachovič, M., Pal’ove-Balang, P., Kubinská, A. (2010). Effect of environmental variables on the aquatic macrophyte composition pattern in streams: A case study from Slovakia. Fundam. Appl. Limnol. 177, 115–124. doi: 10.1127/1863-9135/2010/0177-0115
Jones, J. I., Collins, A. L., Naden, P. S., Sear, D. A. (2012). The relationship between fine sediment and macrophytes in rivers. River Res. Appl. 28, 1006–1018. doi: 10.1002/rra.1486
Kelley, L. A., Gardner, S. P., Sutcliffe, M. J. (1996). An automated approach for clustering an ensemble of NMR-derived protein structures into conformationally related subfamilies. Protein Eng. Des. Sel. 9, 1063–1065. doi: 10.1093/protein/9.11.1063
Leathwick, J. R., Elith, J., Hastie, T. (2006). Comparative performance of generalized additive models and multivariate adaptive regression splines for statistical modelling of species distributions. Ecol. Modell. 199, 188–196. doi: 10.1016/j.ecolmodel.2006.05.022
Lind, L., Hasselquist, E. M., Laudon, H. (2019). Towards ecologically functional riparian zones: A meta-analysis to develop guidelines for protecting ecosystem functions and biodiversity in agricultural landscapes. J. Environ. Manage 249, 109391. doi: 10.1016/j.jenvman.2019.109391
Lindholm, M., Alahuhta, J., Heino, J., Hjort, J., Toivonen, H. (2020). Changes in the functional features of macrophyte communities and driving factors across a 70-year period. Hydrobiologia 847 (18), 3811–3827. doi: 10.1007/s10750-019-04165-1
Lu, Y., Chen, J., Xu, Q., Han, Z., Peart, M., Ng, C. N., et al. (2023). Spatiotemporal variations of river water turbidity in responding to rainstorm-streamflow processes and farming activities in a mountainous catchment, Lai Chi Wo, Hong Kong, China. Sci. Total Environ. 863, 160759. doi: 10.1016/J.SCITOTENV.2022.160759
Magneville, C., Loiseau, N., Albouy, C., Casajus, N., Claverie, T., Escalas, A., et al. (2022). mFD: an R package to compute and illustrate the multiple facets of functional diversity. Ecography 2022. doi: 10.1111/ecog.05904
Maire, E., Grenouillet, G., Brosse, S., Villéger, S. (2015). How many dimensions are needed to accurately assess functional diversity? A pragmatic approach for assessing the quality of functional spaces. Global Ecol. Biogeogr. 24, 728–740. doi: 10.1111/geb.12299
Manolaki, P., Guo, K., Vieira, C., Papastergiadou, E., Riis, T. (2020). Hydromorphology as a controlling factor of macrophytes assemblage structure and functional traits in the semi-arid European Mediterranean streams. Sci. Total Environ. 703, 134658. doi: 10.1016/j.scitotenv.2019.134658
Mason, N. W. H., Mouillot, D., Lee, W. G., Wilson, J. B. (2005). Functional richness, functional evenness and functional divergence: the primary components of functional diversity. Oikos 111, 112–118. doi: 10.1111/J.0030-1299.2005.13886.X
Mouchet, M. A., Villéger, S., Mason, N. W. H., Mouillot, D. (2010). Functional diversity measures: an overview of their redundancy and their ability to discriminate community assembly rules. Funct. Ecol. 24, 867–876. doi: 10.1111/J.1365-2435.2010.01695.X
Mouillot, D., Graham, N. A. J., Villéger, S., Mason, N. W. H., Bellwood, D. R. (2013). A functional approach reveals community responses to disturbances. Trends Ecol. Evol. 28, 167–177. doi: 10.1016/J.TREE.2012.10.004
Naimi, B., Hamm, N. A. S., Groen, T. A., Skidmore, A. K., Toxopeus, A. G. (2014). Where is positional uncertainty a problem for species distribution modelling? Ecography 37, 191–203. doi: 10.1111/j.1600-0587.2013.00205.x
O’Hare, M. T., Baattrup-Pedersen, A., Baumgarte, I., Freeman, A., Gunn, I. D. M., Lázár, A. N., et al. (2018). Responses of aquatic plants to eutrophication in rivers: A revised conceptual model. Front. Plant Sci. 9. doi: 10.3389/fpls.2018.00451
Oikonomou, A., Stefanidis, K. (2020). α- and β-diversity patterns of macrophytes and freshwater fishes are driven by different factors and processes in lakes of the unexplored Southern Balkan biodiversity hotspot. Water (Basel) 12, 1984. doi: 10.3390/w12071984
Olden, J. D., Kennard, M. J., Leprieur, F., Tedesco, P. A., Winemiller, K. O., García-Berthou, E. (2010). Conservation biogeography of freshwater fishes: recent progress and future challenges. Divers. Distrib. 16, 496–513. doi: 10.1111/J.1472-4642.2010.00655.X
Papastergiadou, E. (2022). Samplings and analyses of aquatic macrophytes in Greek rivers (in Greek).
Paradis, E., Schliep, K. (2019). Ape 5.0: An environment for modern phylogenetics and evolutionary analyses in R. Bioinformatics 35, 526–528. doi: 10.1093/bioinformatics/bty633
Perez Rocha, M., Bini, L. M., Grönroos, M., Hjort, J., Lindholm, M., Karjalainen, S. M., et al. (2019). Correlates of different facets and components of beta diversity in stream organisms. Oecologia 191, 919–929. doi: 10.1007/s00442-019-04535-5
Peternel, A., Gaberščik, A., Zelnik, I., Holcar, M., Germ, M. (2022). Long-term changes in macrophyte distribution and abundance in a lowland river. Plants 11, 1–14. doi: 10.3390/plants11030401
Preiner, S., Dai, Y., Pucher, M., Reitsema, R. E., Schoelynck, J., Meire, P., et al. (2020). Effects of macrophytes on ecosystem metabolism and net nutrient uptake in a groundwater fed lowland river. Sci. Total Environ. 721, 137620. doi: 10.1016/j.scitotenv.2020.137620
Ricotta, C., de Bello, F., Moretti, M., Caccianiga, M., Cerabolini, B. E. L., Pavoine, S. (2016). Measuring the functional redundancy of biological communities: a quantitative guide. Methods Ecol. Evol. 7, 1386–1395. doi: 10.1111/2041-210X.12604
Rodrigues, C., Alves, P., Bio, A., Vieira, C., Guimarães, L., Pinheiro, C., et al. (2019). Assessing the ecological status of small Mediterranean rivers using benthic macroinvertebrates and macrophytes as indicators. Environ. Monit. Assess. 191, 1–23. doi: 10.1007/s10661-019-7766-8
Schneider, B., Cunha, E. R., Marchese, M., Thomaz, S. M. (2015). Explanatory variables associated with diversity and composition of aquatic macrophytes in a large subtropical river floodplain. Aquat. Bot. 121, 67–75. doi: 10.1016/j.aquabot.2014.11.003
Skoulikidis, N. T., Karaouzas, I., Amaxidis, Y., Lazaridou, M. (2021). Impact of EU environmental policy implementation on the quality and status of Greek rivers. Water 13, 1858. doi: 10.3390/W13131858
Son, D., Cho, H., Lee, E. J. (2018). Determining factors for the occurrence and richness of submerged macrophytes in major Korean rivers. Aquat. Bot. 150, 82–88. doi: 10.1016/j.aquabot.2018.07.003
Stefanidis, K., Dimitrellos, G., Sarika, M., Tsoukalas, D., Papastergiadou, E. (2022). Ecological quality assessment of Greek lowland rivers with aquatic macrophytes in compliance with the EU water framework directive. Water (Basel) 14, 2771. doi: 10.3390/w14182771
Stefanidis, K., Oikonomou, A., Papastergiadou, E. (2021). Responses of different facets of aquatic plant diversity along environmental gradients in Mediterranean streams : Results from rivers of Greece. J. Environ. Manage 296, 113307. doi: 10.1016/j.jenvman.2021.113307
Stefanidis, K., Papastergiadou, E. (2019). Linkages between macrophyte functional traits and water quality: insights from a study in freshwater lakes of Greece. Water (Basel) 11, 1047. doi: 10.3390/w11051047
Stefanidis, K., Sarika, M., Papastegiadou, E. (2019). Exploring environmental predictors of aquatic macrophytes in water-dependent Natura 2000 sites of high conservation value: Results from a long-term study of macrophytes in Greek lakes. Aquat. Conserv. 29, 1133–1148. doi: 10.1002/aqc.3036
Steffen, K., Leuschner, C., Müller, U., Wiegleb, G., Becker, T. (2014). Relationships between macrophyte vegetation and physical and chemical conditions in northwest German running waters. Aquat. Bot. 113, 46–55. doi: 10.1016/j.aquabot.2013.10.006
Su, H., Chen, J., Wu, Y., Chen, J., Guo, X., Yan, Z., et al. (2019). Morphological traits of submerged macrophytes reveal specific positive feedbacks to water clarity in freshwater ecosystems. Sci. Total Environ. 684, 578–586. doi: 10.1016/j.scitotenv.2019.05.267
Szoszkiewicz, K., Ciecierska, H., Kolada, A., Schneider, S. C., Szwabińska, M., Ruszczyńska, J. (2014). Parameters structuring macrophyte communities in rivers and lakes – results from a case study in North-Central Poland. Knowl. Manag. Aquat. Ecosyst. (415), 08. doi: 10.1051/kmae/2014034
Szoszkiewicz, K., Jusik, S., Pietruczuk, K., Gebler, D. (2020). The macrophyte index for rivers (MIR) as an advantageous approach to running water assessment in local geographical conditions. Water (Switzerland) 12, 108. doi: 10.3390/w12010108
Teittinen, A., Virta, L. (2021). Exploring multiple aspects of taxonomic and functional diversity in microphytobenthic communities: effects of environmental gradients and temporal changes. Front. Microbiol. 12. doi: 10.3389/fmicb.2021.668993
Tichý, L., Axmanová, I., Dengler, J., Guarino, R., Jansen, F., Midolo, G., et al. (2023). Ellenberg-type indicator values for European vascular plant species. J. Vegetation Sci. 34, e13168. doi: 10.1111/jvs.13168
Tilman, D. (2001). Functional diversity. Encyclopedia Biodiver., 109–120. doi: 10.1016/B0-12-226865-2/00132-2
Turunen, J., Muotka, T., Vuori, K. M., Karjalainen, S. M., Rääpysjärvi, J., Sutela, T., et al. (2016). Disentangling the responses of boreal stream assemblages to low stressor levels of diffuse pollution and altered channel morphology. Sci. Total Environ. 544, 954–962. doi: 10.1016/j.scitotenv.2015.12.031
Valkama, E., Usva, K., Saarinen, M., Uusi-Kämppä, J. (2019). A meta-analysis on nitrogen retention by buffer zones. J. Environ. Qual. 48, 270–279. doi: 10.2134/jeq2018.03.0120
Villéger, S., Grenouillet, G., Brosse, S. (2013). Decomposing functional β-diversity reveals that low functional β-diversity is driven by low functional turnover in European fish assemblages. Global Ecol. Biogeogr. 22, 671–681. doi: 10.1111/GEB.12021
Vittinghoff, E., Glidden, D., Shiboski, S. C., McCulloch, C. E. (2012). Regression Methods in Biostatistics (Boston, MA: Springer US). doi: 10.1007/978-1-4614-1353-0
Vukov, D., Ilić, M., Ćuk, M., Igić, R. (2022). The effect of hydro-morphology and habitat alterations on the functional diversity and composition of macrophyte communities in the large river. Front. Environ. Sci. 10. doi: 10.3389/fenvs.2022.863508
Walton, C. R., Zak, D., Audet, J., Petersen, R. J., Lange, J., Oehmke, C., et al. (2020). Wetland buffer zones for nitrogen and phosphorus retention: Impacts of soil type, hydrology and vegetation. Sci. Total Environ. 727, 138709. doi: 10.1016/j.scitotenv.2020.138709
Wang, H., Fu, H., Wen, Z., Yuan, C., Zhang, X., Ni, L., et al. (2021). Seasonal patterns of taxonomic and functional beta diversity in submerged macrophytes at a fine scale. Ecol. Evol. 11, 9827–9836. doi: 10.1002/ece3.7811
Willby, N. J., Abernethy, V. J., Demars, B. O. L. (2000). Attribute-based classification of European hydrophytes and its relationship to habitat utilization. Freshw. Biol. 43, 43–74. doi: 10.1046/J.1365-2427.2000.00523.X
Wood, S. (2020). Package “mgcv”: Mixed GAM Computation Vehicle with Automatic Smoothness Estimation. doi: 10.1201/9781315370279
Zelnik, I., Kuhar, U., Holcar, M., Germ, M., Gaberščik, A. (2021). Distribution of vascular plant communities in Slovenian watercourses. Water (Switzerland) 13, 1071. doi: 10.3390/w13081071
Keywords: aquatic macrophytes, functional richness, functional beta diversity, generalized dissimilarity models, rivers
Citation: Stefanidis K, Oikonomou A, Dimitrellos G, Tsoukalas D and Papastergiadou E (2023) Beyond taxonomic diversity patterns – investigating how α and β components of macrophyte functional diversity respond to environmental gradients in lotic ecosystems of Greece. Front. Plant Sci. 14:1204383. doi: 10.3389/fpls.2023.1204383
Received: 12 April 2023; Accepted: 20 July 2023;
Published: 08 September 2023.
Edited by:
Rodolfo Gentili, University of Milano-Bicocca, ItalyReviewed by:
Agnieszka Kolada, Institute of Environmental Protection (IOS), PolandIgor Zelnik, University of Ljubljana, Slovenia
Copyright © 2023 Stefanidis, Oikonomou, Dimitrellos, Tsoukalas and Papastergiadou. This is an open-access article distributed under the terms of the Creative Commons Attribution License (CC BY). The use, distribution or reproduction in other forums is permitted, provided the original author(s) and the copyright owner(s) are credited and that the original publication in this journal is cited, in accordance with accepted academic practice. No use, distribution or reproduction is permitted which does not comply with these terms.
*Correspondence: Eva Papastergiadou, ZXZhcGFwQHVwYXRyYXMuZ3I=; Konstantinos Stefanidis, a3N0ZWZhbmlAdXBhdHJhcy5ncg==