- 1Key Laboratory of Oasis Eco-Agriculture, College of Agriculture, Shihezi University, Shihezi, China
- 2Xinjiang Production and Construction Group Key Laboratory of Crop Germplasm Enhancement and Gene Resources Utilization, Cotton Research Institute, Xinjiang Academy of Agricultural and Reclamation Science, Shihezi, China
- 3College of Plant Science and Technology, Tarim University, Alar, China
WRKY transcription factors (TFs) play an important role in regulating the mechanism of plant self-defense. However, the function of most WRKY TFs in upland cotton (Gossypium hirsutum) is still unknown. Hence, studying the molecular mechanism of WRKY TFs in the resistance of cotton to Verticillium dahliae is of great significance to enhancing cotton disease resistance and improving its fiber quality. In this study, Bioinformatics has been used to characterize the cotton WRKY53 gene family. we analyzed the GhWRKY53 expression patterns in different resistant upland cotton cultivars treated with salicylic acid (SA) and methyl jasmonate (MeJA). Additionally, GhWRKY53 was silenced using a virus-induced gene silencing (VIGS) to determine the contribution of GhWRKY53 to V. dahliae resistance in cotton. The result showed that GhWRKY53 mediated SA and MeJA signal transduction pathways. After VIGS of the GhWRKY53, the ability of cotton to resist V. dahliae decreased, indicating that the GhWRKY53 could be involved in the disease resistance mechanism of cotton. Studies on the levels of SA and jasmonic acid (JA) and their related pathway genes demonstrated that the silencing of GhWRKY53 inhibited the SA pathway and activated the JA pathway, thereby reducing the resistance of plants to V. dahliae. In conclusion, GhWRKY53 could change the tolerance of upland cotton to V. dahliae by regulating the expression of SA and JA pathway-related genes. However, the interaction mechanism between JA and SA signaling pathways in cotton in response to V. dahliae requires further study.
1 Introduction
Upland cotton is an important economic crop in China and one of the major cultivars grown in the northwest of the country due to its high yield and good fiber quality. In recent years, Verticillium dahliae outbreaks were frequent in cotton due to changing climatic conditions, long-term monocultures, and the frequent introduction of new cotton varieties across the globe (Shaban et al., 2018). V. dahliae is a soil-borne, semi-living parasitic plant pathogenic fungus that causes verticillium wilt in cotton. It has the characteristics of wide distribution, harmful effects, strong infectivity, and is very hard to cure (Klosterman et al., 2009). Due to the absence of a targeted control agent for infected plants (Fradin et al., 2006), V. dahliae seriously affects cotton yield in China.
Recently, it was established that secondary metabolites and hormones were involved in cotton disease resistance. When plants sense signals of pathogenic fungus invasion, self-defense responses are regulated through hormonal signal transduction. Currently, salicylic acid (SA), jasmonic acid (JA), ethylene (ET), abscisic acid (ABA), gibberellins (GAs), and brassinosteroids (BRs) are widely studied. In Gossypium barbadense disease-resistant cultivars inoculated with V. dahliae, it was found that the content of SA increased, and the expression of SA synthesis genes (PAL, phenylalanine ammonia-lyase and ICS, Isochorismate synthase) and downstream reaction genes (NPR1, nonexpressor of pathogenesis-related genes 1 and PR1, polyadenylic acid 1) also increased (Zhang et al., 2013; Zhang et al., 2017). The exogenous application of methyl jasmonate (MeJA) to cotton activated the expression of PR genes in the jasmonate signaling pathway and enhanced plant disease resistance (Li et al., 2014). Similar results were also obtained after spraying with ET (Guo et al., 2016).
As a plant-specific class of transcriptional regulators, WRKY transcription factors (TFs) possess conserved domains that determine their functions and provide a basis for the classification of the WRKY family of TFs (Eulgem et al., 2000). Domain C of WRKY TFs has a characteristic zinc finger structure with seven amino acid residues of conserved WRKYGQK in the N-terminus. This helps in its specific binding to w-box (T/C) TGAC (T/C) sites in genes regulating phytohormone signaling, including ABA, ET, JA, and SA, and in the disease resistance mechanisms of cotton (Pandey and Somssich, 2009; Zhang et al., 2015). Studies showed that WRKY TFs specifically recognize w-box sites in the promoters of the PR of SA signaling pathways to promote disease resistance response (Rushton and Somssich, 1998). WRKY TFs, including those in Capsicum CaWRKYd (Huh et al., 2012), tobacco NtWRKY12 (Van et al., 2011), Populus trichocarpa PtrWRKY73 (Duan et al., 2015), OsWRKY53 (Zhang et al., 2015), etc have been shown to regulate plant resistance to pathogens by inducing the expression of the PR in the SA, ABA, and GA signaling pathways.
As a member of the WRKY family, the WRKY53 transcription factor was first discovered in Arabidopsis leaves and was found to be specifically expressed in the early stage of leaf senescence (Hinderhofer and Zentgraf, 2001). Wan et al. (2004) through transgenic expression experiments on tobacco, it was demonstrated that active mutant NtMEK2 induced the expression of WRKY33 and WRKY53, thereby confirming that this class of transcription factors played a role in signal transmission. Murray et al. (2007) identified that WRKY53 belonged to type III WRKY transcription factors and that most of the type III WRKY transcription factors were associated with plant resistance to pathogenic fungus. In addition, it had been reported that WRKY53 could be a major factor affecting JA and SA synergy (Miao and Zentgraf, 2007; Shang et al., 2011). Luna and Ton (2012) found that plant immune response to pathogen invasion could be inherited under disease pressure. Also, after plant infestation, a transgenerational defense phenotype was developed through the activation of SA-induced defense genes, including GENE1, WRKY 6, and WRKY53.
Upland cotton, the largest cultivated variety in China, strongly supports the country’s national economic development. However, the invasion of V. dahliae has brought great loss to cotton farmers. With no chemical agents available against V. dahliae, cotton farmers resort to the use of cultivation measures, including stubble rotation, mid cultivation, and deep turning, to alleviate the effects of verticillium wilting. Apart from cultivation measures, the most economical and effective measures are to cultivate and promote disease-resistant varieties. Research on disease-resistant genes is of great significance to breeding disease-resistant cotton varieties. In this study, we identified the GhWRKY53 and analyzed its expression patterns in different resistant upland cotton cultivars treated with SA and MeJA. GhWRKY53 was silenced using VIGS to determine the contribution of GhWRKY53 to V. dahliae resistance in cotton. Changes in the levels of SA and MeJA and their roles in the expression of GhWRKY53 to V. dahliae resistance in cotton were also studied. Our study provides a theoretical basis for investigating the signaling pathways and molecular mechanisms of cotton resistance to V. dahliae and the breeding of high-resistance cultivars.
2 Materials and methods
2.1 Identification of GhWRKY53 family genes
The genomes of G. raimondii, G. arboreum, G. hirsutum and G. barbadense were downloaded from CottonGen (https://www.cottongen.org/). Blastp (E-value ≤ 10-10) was carried out in cotton genome using GhWRKY53 encoded amino acid sequence as a probe to obtain WRKY53 protein sequence in each of the four cotton species. Multiple sequence alignment of GhWRKY53 was performed with DNAMAN (Kumar et al., 2016). Conserved motifs of the WRKY53 protein sequence were predicted with the use of online software, MEME (https://meme-suite.org/). RT-qPCR was used to analyze the gene expression level of GhWRKY53 in the roots, stems, and leaves of resistant and susceptible cotton varieties.
2.2 Virus-induced gene silencing of GhWRKY53
Tobacco rattle virus (TRV) derived vectors, pTRV1 and pTRV2, were used for VIGS (Liu et al., 2002). The VIGS vector construction and experimental procedure were carried out according to the protocol previously described (Lu et al., 2021). The fragment targeting GhWRKY53 was amplified using the forward primer of 5’-GAATTCGGGCAAAAAGACATCCTGGG-3’ and the reverse primer 5’-GGTACCGAAAGAAGTTGCCATCTCGGT-3’ (the underlined nucleotides in the forward and reverse primers represent the restriction sites of Eco R1 and Kpn I, respectively). The PCR cycles and confirmation of the fragment were done according to Lu et al. (2021). The cotton variety used in VIGS was Upland cotton ZZM2 and XLZ7. The method of V. dahliae infection of cotton was done according to Li et al. (2023). All primers used for the VIGS were designed with Primer3 software and are shown in Table S1.
2.3 Determination of endogenous SA and MEJA contents in cotton after V. dahliae infection
For the SA and MeJA treatment experiments, two-leaf stage seedlings of cotton were sprayed with 1mmolL−1 SA or 100 µmol L−1 MeJA (Xiong et al., 2019). Patients treated for 0 hours were selected as the control group. Collect root samples of cotton seedlings at 0, 6, 12, 24, and 48 hours after treatment. Roots of TRV::00 and TRV::GhWRKY53 inoculated with V. dahliae for 0 and 48 h were selected to determine the endogenous levels of SA and MEJA. Moreover, the roots from five individual seedlings of cottons at two-leaf stage were collected to measure the expression profiles of GhPAL, GhPR1, GhAOS1, GhPDF1.2 and GhLOX after V.dahliae infection in TRV::00 and TRV::GhWRKY53 plants by qRT-PCR. The differences between groups were compared using Student’s t-test (* P < 0.05; ** P < 0.01).
2.4 qRT-PCR analysis
Total RNA was extracted from leaves of TRV1/TRV2::00 or TRV1/TRV2::GhWRKY53 treated ZZM2 and XLZ7 plants (both control and V.dahliae treated), and then reverse transcribed into cDNA to be used in qRT-PCR to analyze the effect of inoculation with V.dahliae treatment on gene expression changes. qRT-PCR was carried out by the SYBR Green (Roche, Rotkreuz, Switzerland) on a Light Cycler 480II (Roche, Germany) with default parameters. All primers used for the validation experiments were designed with Primer3 software and are shown in Table S1. The GhUBQ7 gene served as an internal control to normalize differences between samples. The relative expression levels of genes from three biologically independent experiments were calculated using the 2-ΔΔCTmethod (Livak and Schmittgen, 2001).
2.5 Statistical analysis
SPSS 26.0 (SPSS, Chicago, USA) was used for data processing and analysis of variance. The data were analyzed by a one-way analysis of variance (ANOVA) and the significance of the difference was tested using the Duncan multiple comparison method. Origin 2022 (OriginLab, Northampton, USA) was used to plot the figures.
3 Results
3.1 Sequence alignment and phylogenetic analysis of GhWRKY53
The 2, 2, 4, and 4 WRKY53 genes were identified in G. arboreum, G. raimondii, G. barbadense, and G. hirsutum, respectively. Further, the conserved domains of GhWRKY53 were demonstrated by multiple protein sequence alignments of GhWRKY53. Almost all proteins contained the WRKY domain and zinc finger structure in the form of CX7C23HXC (Figure 1A). Based on the results of a motif analysis of GhWRKY53 proteins using MEME suite, a total of ten conserved motifs were identified. GrWRKY53-2, GbWRKY53-2D and GhWRKY53 contained nine motifs, while the others contained ten motifs (Figure 1B). Additionally, the promoter region of WRKY53 in each cotton species contained at least two plant hormone cis-acting elements (Figure 1C). Of them, an ETH cis-responsive element was found in the promoter region of each WRKY53 gene. And a MeJA cis-responsive element and an SA were found in four WRKY53 (GaWRKY53-2, GbWRKY53-2A, GbWRKY53-1D and GbWRKY53-2D). The results also showed that the GaWRKY53-1, GhWRKY53-1A and GhWRKY53-1D exclude MeJA and SA cis-responsive element (Figure 1C). The above results indicated that cotton WRKY53 was regulated by a variety of hormones and was involved in different types of hormone response.
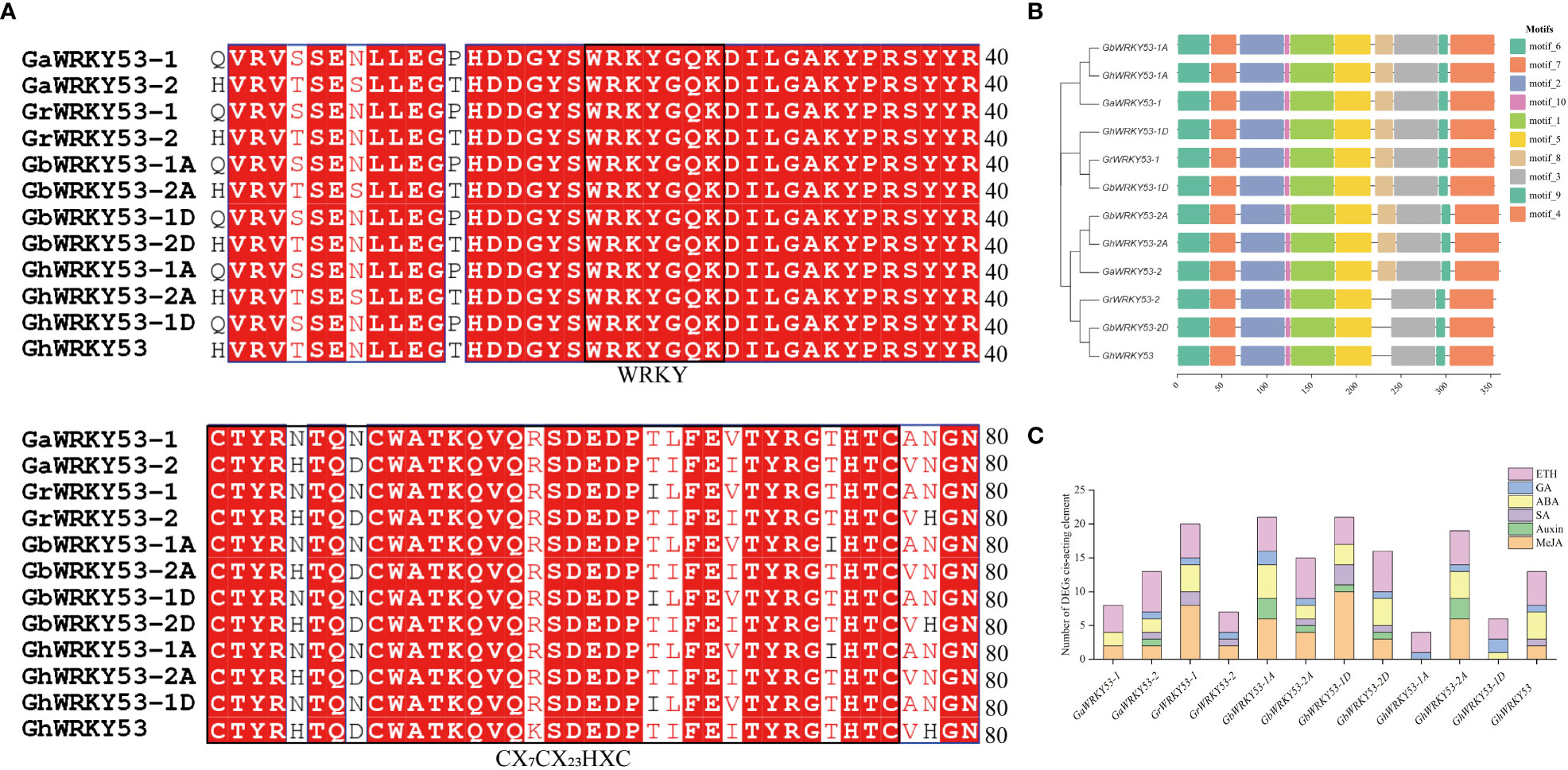
Figure 1 Sequence alignment, motifs, cis-acting elements analysis of WRKY53. (A) Multiple sequence alignment of cotton WRKY53 gene. (B) Motif of 12 WRKY53 proteins. Ten motifs were investigated using the MEME online tool. (C) Statistical analysis of the types and quantity of cis-acting elements in the promoter of WRKY53. GaWRKY53-1 (Ga08G1308), GaWRKY53-2 (Ga12G0445), GrWRKY53-1 (Gorai.004G134600), GrWRKY53-2 (Gorai.008G253300), GbWRKY53-1A (GB_A08G1300), GbWRKY53-2A (GB_A12G2642), GbWRKY53-1D (GB_D08G1397), GbWRKY53-2D (GB_D12G2648), GhWRKY53-1A (GH_A08G1141), GhWRKY53-2A (GH_A12G2543), GhWRKY53-1D (GH_D08G1341), GhWRKY53 (GH_D12G2563).
3.2 Analysis of GhWRKY53 expression pattern
qRT-PCR analysis showed that GhWRKY53 was expressed in roots, stems and leaves of the ZZM2 and XLZ7, especially in the roots and stems. And its expression levels in leaves were relatively low. For XLZ7, a susceptible variety, GhWRKY53 expression levels in the stems were significantly higher than roots. However, GhWRKY53 expression levels were higher in the roots of disease-resistant variety ZZM2 (Figure 2). To further analyze the function of GhWRKY53 in cotton verticillium wilt resistance, the two-leaf stage seeding was inoculated with V. dahliae by the root irrigation method (Xiong et al., 2019). The results showed that GhWRKY53 expression levels were significantly up-regulated in the root and stem tissues of ZZM2 after 12 h of V. dahliae inoculated (Figures 3A, B). The GhWRKY53 showed significantly up-regulated after 24 h of V. dahliae inoculated in the root and stem of XLZ7 (Figures 3A, B). However, the up-regulated of GhWRKY53 expression levels of XLZ7 in roots and stems was not significant than ZZM2. In the resistant variety ZZM2, GhWRKY53 had higher expression levels in the root and was more sensitive to the stress response of V. dahliae. It also indicated that GhWRKY53 may play an important role in cotton resistance to verticillium wilt.
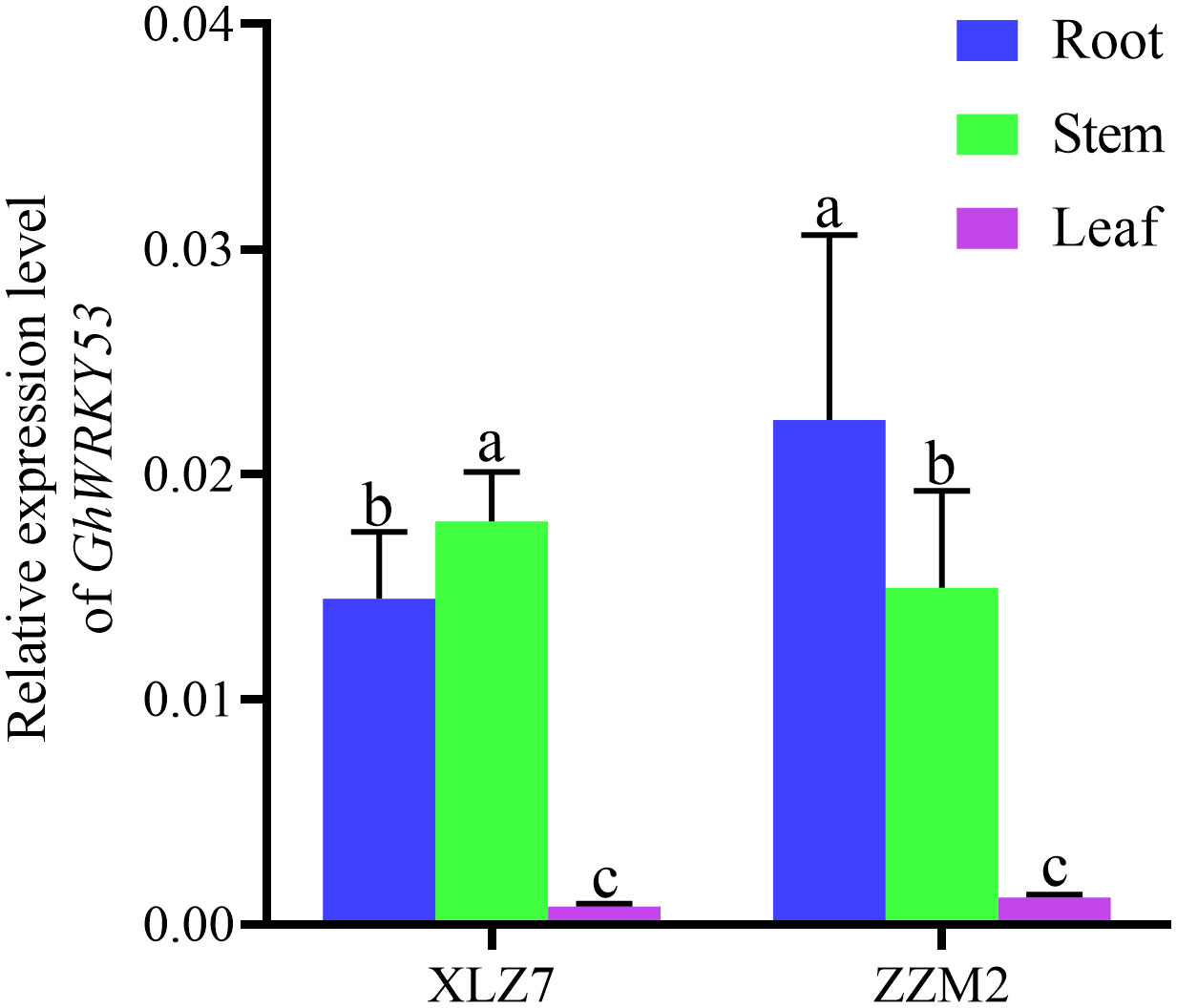
Figure 2 Expression levels of GhWRKY53 in cotton roots, stems and leaves. XLZ7: V. dahliae-susceptible cotton vatiety; ZZM2:V. dahliae-resistant cotton vatiety. GhUBQ7 is an internal reference gene. The data are three independent biological replicates. Different lowercase letters indicate significant differences (P < 0.05) between groups determined using Duncan’s multiple range test.
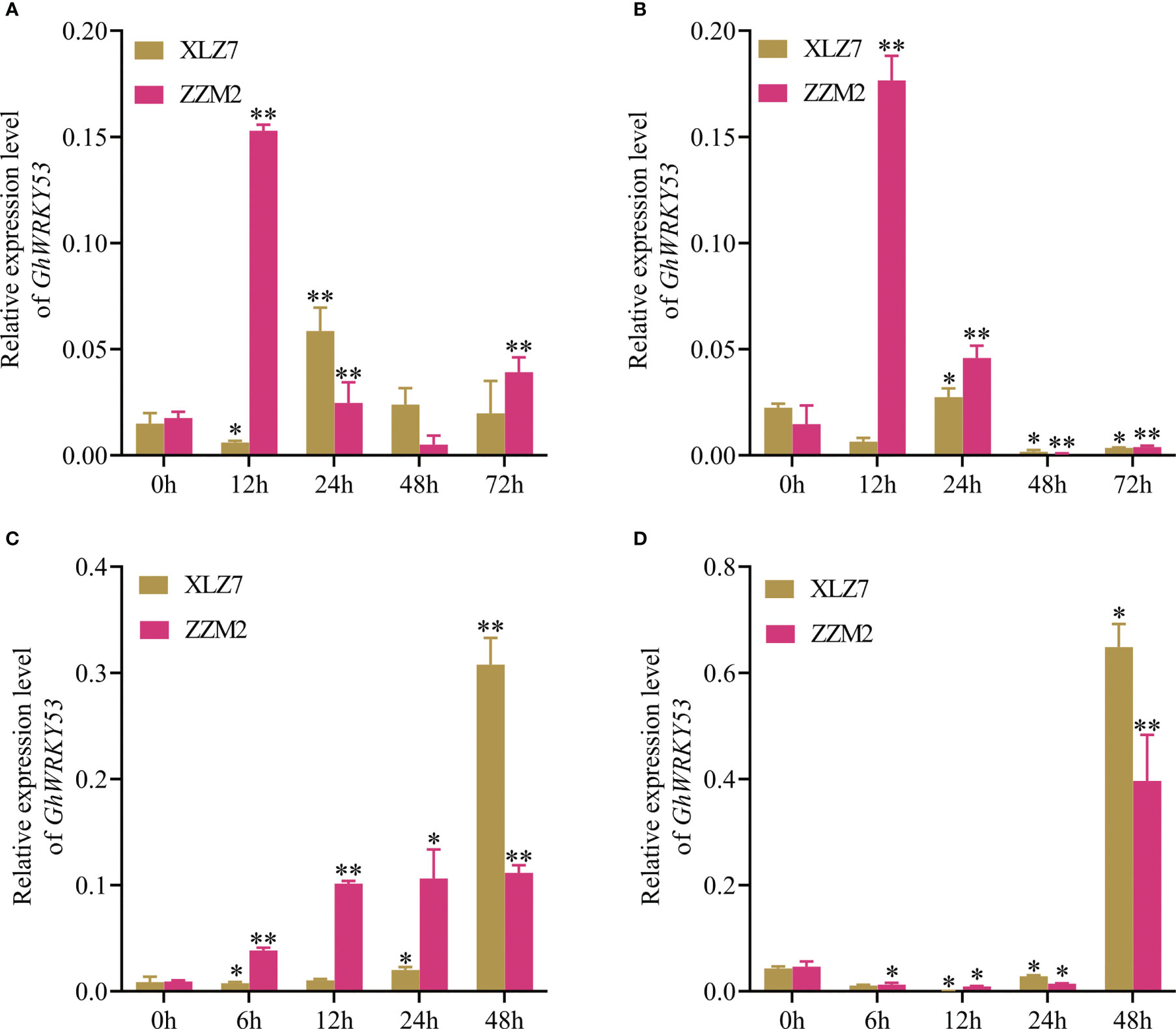
Figure 3 Expression levels of GhWRKY53 after V.dahliae inoculation and SA, MeJA treatment. (A) The relative expression levels of GhWRKY53 in cotton roots with V.dahliae inoculation at 0, 12, 24, 48 and 72 h. (B) The relative expression level of GhWRKY53 in cotton stems with V.dahliae inoculation at 0, 12, 24, 48 and 72 h. (C) The relative expression levels of GhWRKY53 in cotton roots with SA at 0, 12, 24, 48 and 72 h. (D) The relative expression level of GhWRKY53 in cotton stems with MeJA at 0, 12, 24, 48 and 72 h. XLZ7: V. dahliae-susceptible cotton vatiety; ZZM2: V. dahliae-resistant cotton vatiety. GhUBQ7 is an internal reference gene. The data are three independent biological replicates, Significance analysis using T test (*P < 0.05; **P < 0.01).
3.3 Expression pattern analysis of GhWRKY53 under different hormone treatments
The upstream promoter sequence of GhWRKY53 contains cis-acting elements of SA and MeJA, suggesting that GhWRKY53 may be involved in disease resistance related to hormone response. Cotton seedlings treated with different hormones were sampled to detect the changes in GhWRKY53 expression levels in the roots of the ZZM2 and XLZ7 after SA and MeJA treatment. The results show that the GhWRKY53 expression levels were significantly up-regulated after 6, 12, and 24 h after SA treatment in ZZM2 (Figure 3C). However, the expression levels of GhWRKY53 in sensitive variety XLZ7 showed a slow upward trend from 6 to 24 h after SA treatment (Figure 3C). After MeJA treatment, GhWRKY53 expression levels in both XLZ7 and ZZM2 showed a trend of first downregulation and then upregulation, and GhWRKY53 expression levels in both cotton varieties were significantly up-regulated at 48 h (Figure 3D). These results indicated that GhWRKY53 was specifically regulated by SA and MeJA in different verticillium wilt resistant varieties.
3.4 Effect of silencing GhWRKY53 on V. dahliae resistance of cotton
To study the function of GhWRKY53 on the cotton resistance to V. dahliae, VIGS was used to silence GhWRKY53. Seedlings infiltrated with A. tumefaciens carrying pTRV1/pTRV2::GhCHLI (plants-TRV:: GhCHLI) that targeted a gene encoding a ChlI subunit of magnesium chelatase were used as a positive control. After about ten days of injection, plants-TRV::GhCHLI showed a yellowing phenotype, indicating that VIGS system was functioning properly (Figures 4A, 5A). Compared to the control plant (plants-TRV2::00), GhWRKY53 expression levels in ZZM2 and XLZ7 respectively infiltrated with A. tumefaciens carrying pTRV1/pTRV2::GhWRKY53, decreased significantly, indicating that GhWRKY53 was effectively silenced in XLZ7 and ZZM2 (Figures 4B, 5B). And then cotton seedlings were inoculated with V. dahliae to verify plants resistance to V. dahliae after silencing GhWRKY53. At 14 days after inoculation with V.dahliae, GhWRKY53-silenced plants were more sensitive to V. dahliae infection than control plants in XLZ7. XLZ7-pTRV2::GhWRKY53 also showed severe leaf yellowing and wilting, with more shedding of leaves (Figure 4C). The longitudinal section of stems after 21 d pathogen infection showed that the browning degree of the stems in the XLZ7-pTRV2::GhWRKY53 was more significant (Figure 4D). Additionally, the pathogen colonies isolated from the stems of XLZ7-pTRV2::GhWRKY53 were larger and darker than that of XLZ7 (Figure 4E). At 14 d and 21 d, the disease index of XLZ7-pTRV2::GhWRKY53 plants were 47 ± 2.04 and 85.67 ± 3.70, respectively, significantly higher than that of TRV::00 plants at each time point (Figure 4F). Meanwhile, the relative contents of pathogen in the stem tissues and the recovery of pathogen were detected. The results showed that the biomass of pathogen in the stems of XLZ7-pTRV2::GhWRKY53 was significantly higher than that of control plants, and its biomass was about three times that of XLZ7(Figure 4G). These demonstrated that silencing GhWRKY53 further reduces the resistance of susceptible cotton variety XLZ7 to V. dahliae. In ZZM2, after 14 d inoculation, GhWRKY53-silenced plants (ZZM2-pTRV2::GhWRKY53) exhibited poor growth with severe disease and yellowing of leaves compared with control plants ZZM2. Compared with the control plant, leaves of ZZM2-pTRV2::GhWRKY53 had significant withering (Figure 5C). The longitudinal section of stem showed that the accumulation of pathogen in ZZM2-pTRV2::GhWRKY53 with severe browning was more than that in control plants, and the stem browning was severe (Figure 5D). The results of the pathogen recovery experiment showed that the colonies isolated from the stems of ZZM2-pTRV2::GhWRKY53 were larger and had a denser villous shape than that of control plants (Figure 5E). The disease index of the ZZM2-pTRV2::GhWRKY53 on the 14 d and 21d was 13.17 ± 2.02 and 47.17 ± 3.01 respectively, and far higher than the control plant(Figure 5F). Compared to the control plants, the stems of ZZM2-pTRV2::GhWRKY53 accumulated more pathogen, and its biomass was about five times that of XLZ7 (Figure 5G). These were similar to the results observed in XLZ7, indicating that the silencing of GhWRKY53 also significantly reduced the resistance of cotton to V. dahliae.
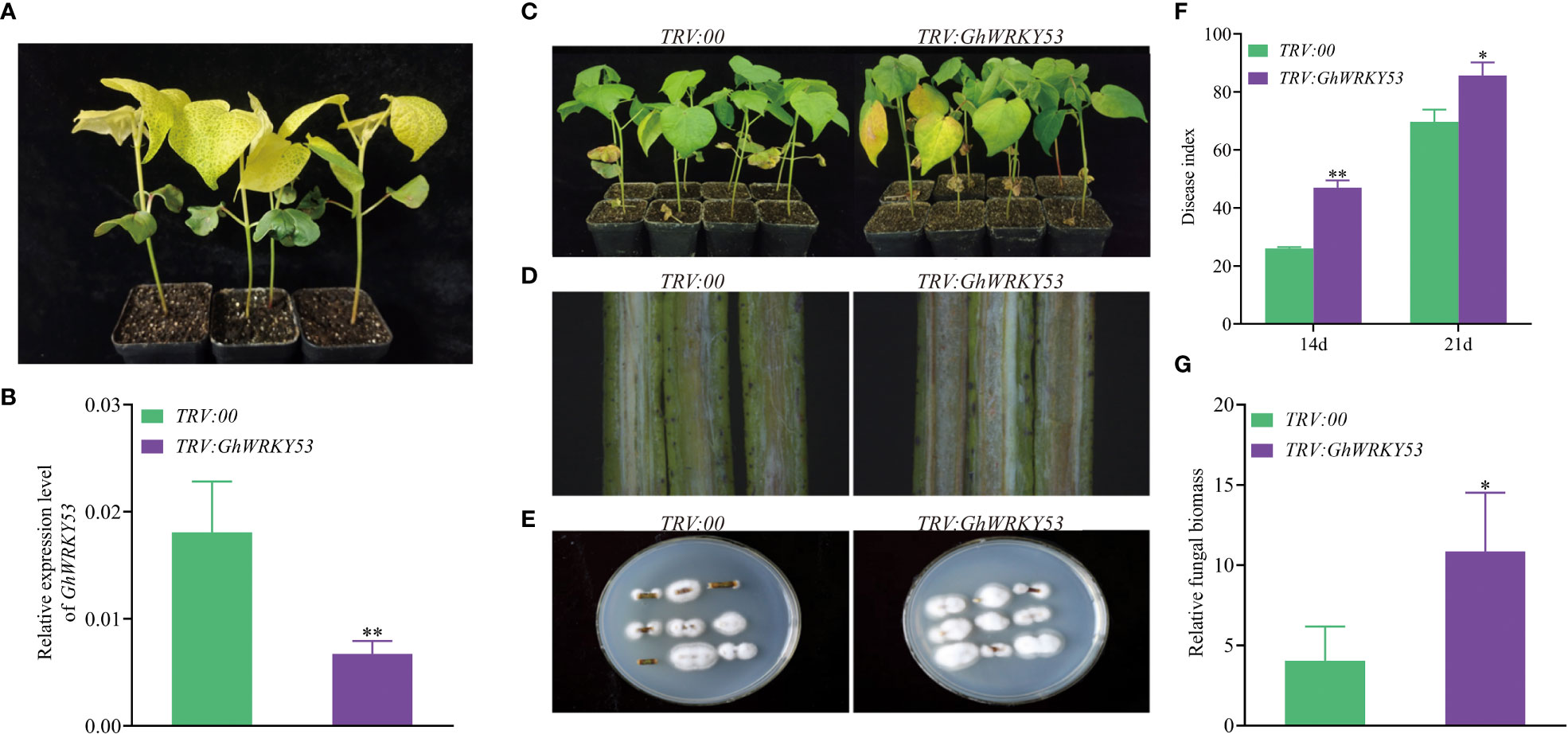
Figure 4 Silencing of GhWRKY53 in V. dahliae- susceptible XLZ7. (A) Observation of the expected yellowing leaf phenotype in TRV::GhCHLI plants. (B) GhWRKY53 expression in the TRV::00 and TRV::GhWRKY53 plants. (C) Disease symptoms of the TRV::00 and TRV::GhWRKY53 plants at 14 day post inoculation. (D) Comparison of vascular browning between TRV::00 and TRV::GhWRKY53 plants at 14 day. (E) Fungal isolation in the stem sections from TRV::00 and TRV::GhWRKY53 plants at 14 dpi. (F) Disease index for the TRV::00 and TRV::GhWRKY53 plants at 14 and 21-day post-inoculating. (G) Quantification of the relative fungal biomass in stems of the TRV::00 and TRV::GhWRKY53 plants at 21 day post inoculation. The data are three independent biological replicates, Significance analysis using T test (*P < 0.05; **P < 0.01).
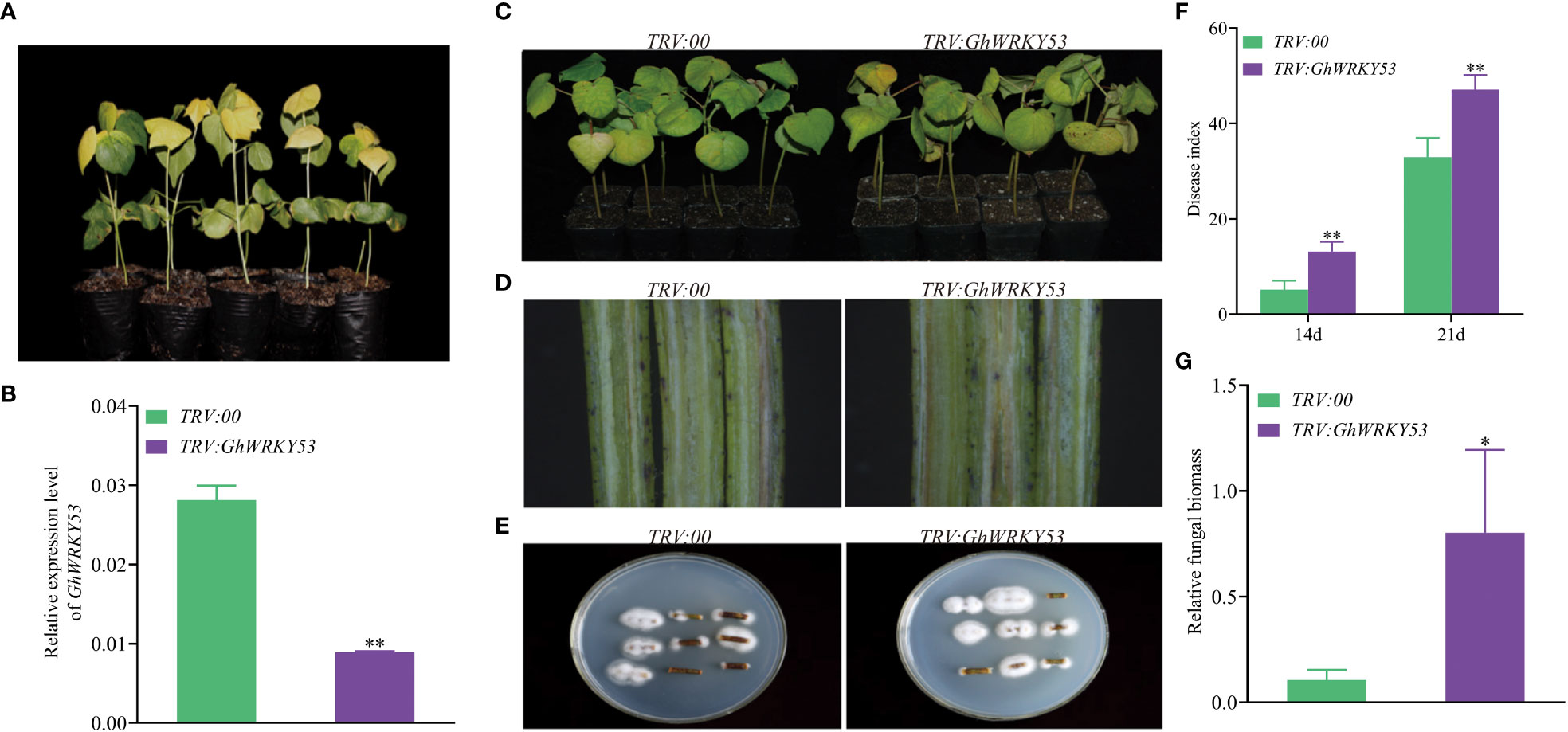
Figure 5 Silencing of GhWRKY53 in V. dahliae- resistance ZZM2. (A) Observation of the expected yellowing leaf phenotype in TRV::GhCHLI plants. (B) GhWRKY53 expression in the TRV::00 and TRV::GhWRKY53 plants. (C) Disease symptoms of the TRV::00 and TRV::GhWRKY53 plants at 14 day post inoculation. (D) Comparison of vascular browning between TRV::00 and TRV::GhWRKY53 plants at 14-day. (E) Fungal isolation in the stem sections from TRV::00 and TRV::GhWRKY53 plants at 14 dpi. (F) Disease index for the TRV::00 and TRV::GhWRKY53 plants at 14 and 21-day post-inoculating. (G) Quantification of the relative fungal biomass in stems of the TRV::00 and TRV::GhWRKY53 plants at 21-day post-inoculating. The data are three independent biological replicates, Significance analysis using T test (*P < 0.05; **P < 0.01).
3.5 Effect of silencing GhWRKY53 on SA and JA signaling pathways
To explore whether the deterioration of V. dahliae resistance in GhWRKY53-silenced plants is mediated by JA and SA signaling pathways, we measured JA and SA concentrations in the roots of the GhWRKY53-silenced plants and control plants, and the expression of signaling pathway-related genes also were analyzed. The results showed that SA content was up-regulated in both the control and silenced plants after inoculation with V. dahliae for 48 h in ZZM2 and XLZ7. For XLZ7, at 0 h after V. dahliae infected, the content of SA in GhWRKY53-silenced plants and control plants were 52.16 and 113 15 ng/g, respectively. And at 48 h, the content of SA increased to 122.51 and 130.67 ng/g, respectively (Figure 6A). Simultaneously, the content of JA in TRV::GhWRKY53 and TRV::00 were 8.05 and 3.46 ng/g at o h, respectively. And increased to 11.75 and 9.59 ng/g at 48 h, respectively (Figure 6B). However, it was noted that the SA content in the GhWRKY53-silenced plants of XLZ7 was lower compared to the control plants at 0 h after V. dahliae inoculation (Figure 6A). Consistent with this result, SA biosynthesis gene (GhPAL) and response gene (GhPRI) were significantly up-regulated at 48 h (Figures 6C, D). And JA biosynthesis gene (GhLOX) and JA response gene (GhPDF1.2) also followed a similar trend (Figures 6E, F). For ZZM2, the SA content of TRV::GhWRKY53 (68.92 ng/g) was significantly lower than TRV::00 (120.32 ng/g) at 48 h post-inoculation (hpi) (Figure 7A), with a similar trend being seen in the expression levels of SA biosynthesis gene (GhPAL) and response gene (GhPRI) (Figures 7C, D). The content of JA in TRV::GhWRKY53 plants and TRV::00 plants at 48 hpi increased 9.43 and 2.67 ng/g than 0 hpi after V. dahliae inoculation (Figure 7B), while the expression levels of JA biosynthesis genes (GhAOS and GhLOX) were also significantly induced at 48 hpi (Figures 7E, F). These results were support the notion that GhWRKY53 plays a positive role in V. dahliae resistance by activating JA signaling pathway and repressing SA signaling pathway.
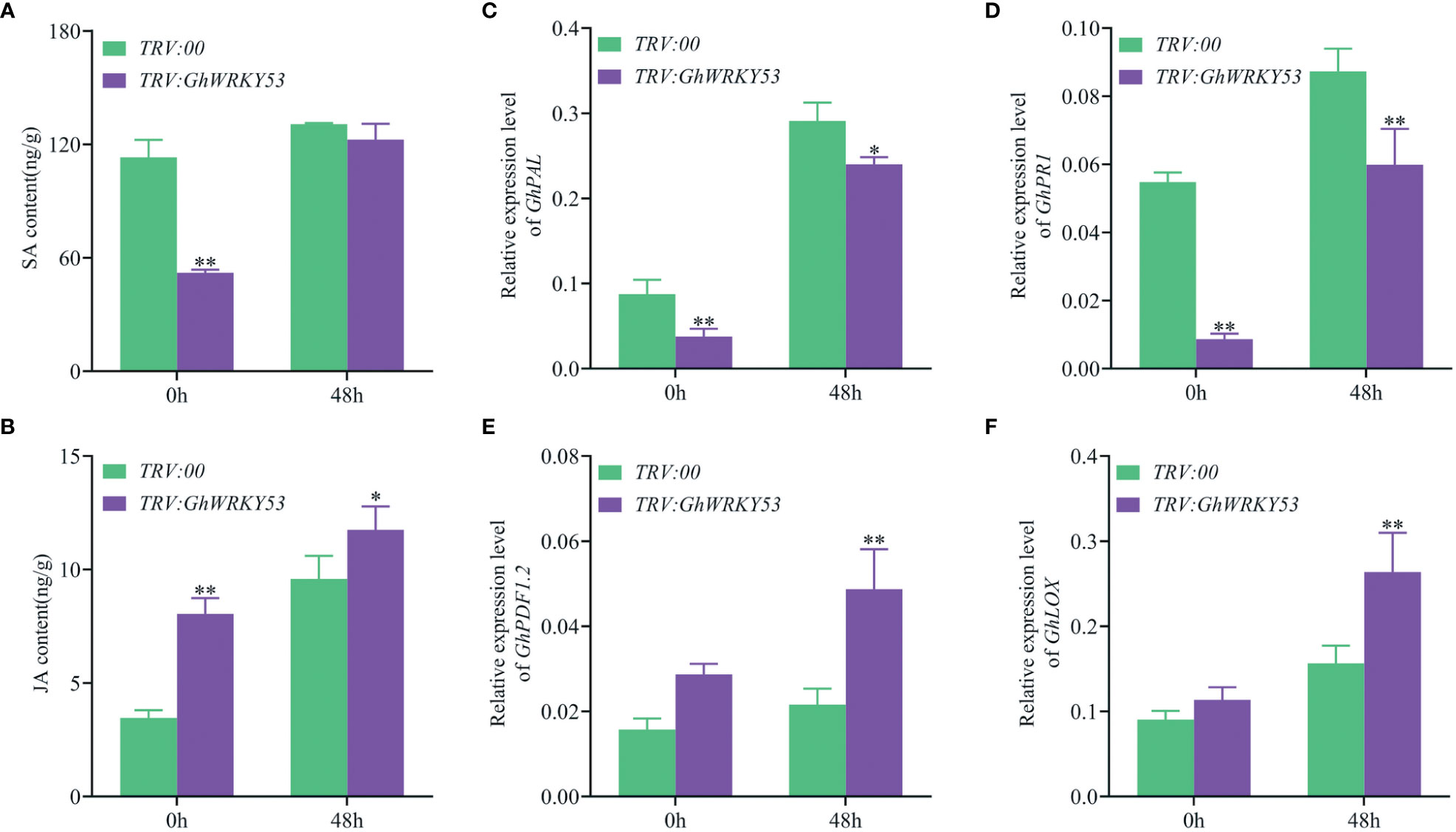
Figure 6 Changes in SA and JA content and the expression levels of related pathway genes in XLZ7 after V. dahliae inoculation. (A) Changes in SA content of TRV::00 and TRV::GhWRKY53 plants after V. dahliae inoculation. (B) Changes in MEJA content of TRV::00 and TRV::GhWRKY53 plants after V. dahliae inoculation. (C) qRT-PCR detects the expression changes of GhPAL after V. dahliae inoculation in TRV::00 and TRV::GhWRKY53 plants. (D) GhPR1. (E) GhPDF1.2. (F) GhLOX. Significance analysis using T test (*P < 0.05; ** P< 0.01).
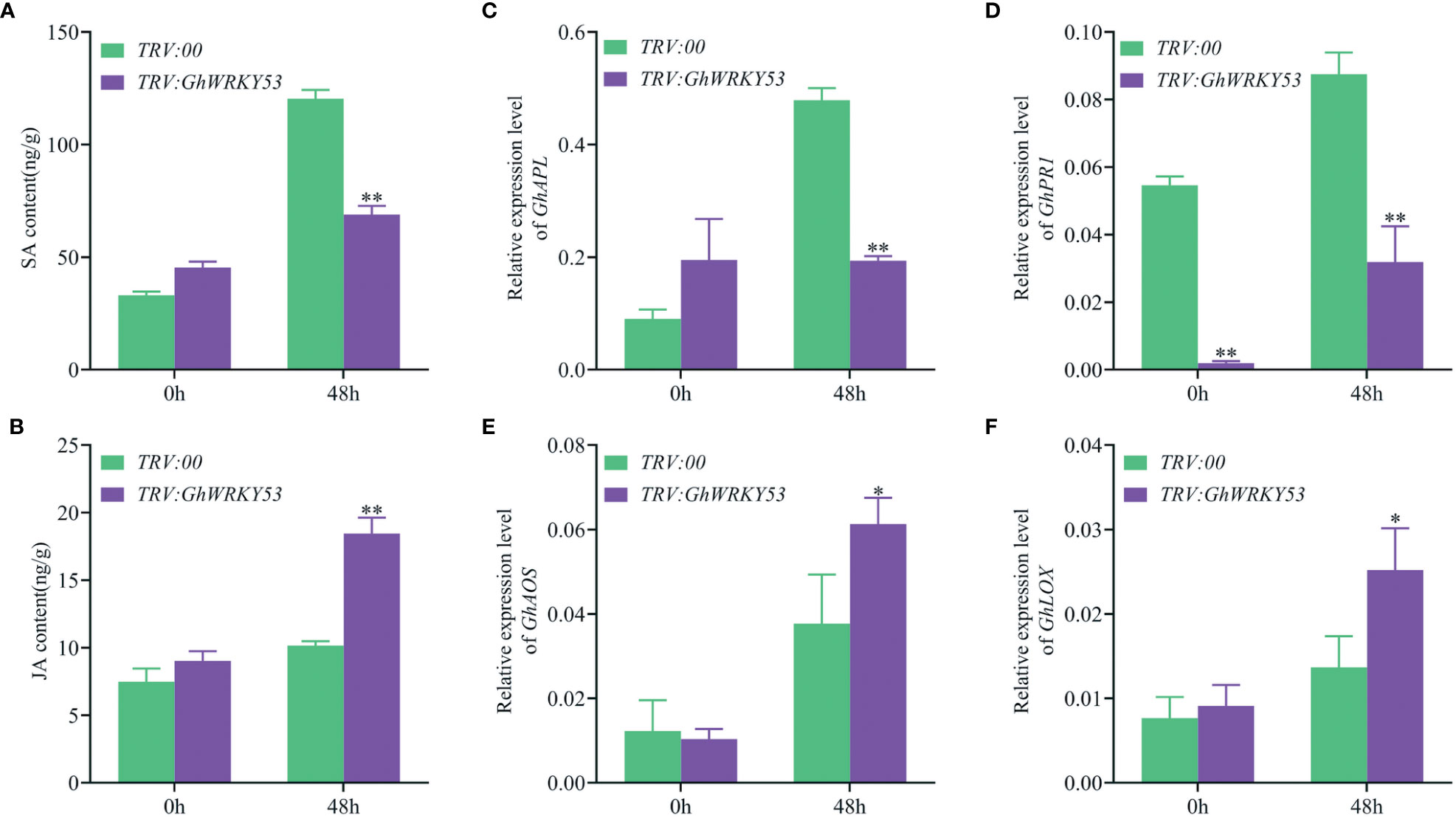
Figure 7 Changes in SA and JA content and the expression levels of related pathway genes in ZZM2 after V. dahliae inoculation. (A) Changes in SA content of TRV::00 and TRV::GhWRKY53 plants after V. dahliae inoculation. (B) Changes in MEJA content of TRV::00 and TRV::GhWRKY53 plants after V. dahliae inoculation. (C) qRT-PCR detects the expression changes of GhPAL after V. dahliae inoculation in TRV::00 and TRV::GhWRKY53 plants. (D) GhPR1. (E) GhPDF1.2. (F) GhLOX. Significance analysis using T test (*P < 0.05; **P < 0.01).
4 Discussion
As one of the most prominent transcription factor families in plants, members of the WRKY gene family play an essential role in many plant life processes, such as plant response to biotic and abiotic stresses, secondary metabolism of plants, and plant growth and development. Verticillium wilt caused by Verticillium dahliae is a destructive cotton disease causing severe yield and quality losses worldwide. WRKY transcription factors play important roles in plant defense against pathogen infection. However, little has been reported on the functions of WRKYs in cotton’s resistance to V. dahliae. Overexpression of GhWRKY27a reduced the tolerance of transgenic plants to drought stress and resistance to Rhizoctonia solani (Yan et al., 2020). In 2020, the reported that GhWRKY70D13 negatively regulates cotton’s resistance to V. dahliae mainly through its effect on ET and JA biosynthesis and signaling pathways (Xiong et al., 2020). In this study, we explored the regulation of GhWRKY53 in the V. dahliae resistance of cotton by silencing GhWRKY53. The gene-silenced plants had severe disease conditions and higher fungal contents, thereby indicating the positive regulatory role of GhWRKY53 in the resistance mechanism of G. hirsutum to V. dahliae.
Studies have shown that WRKY TFs are involved in hormonal signal transduction processes (Zhang et al., 2015). At present, a large number of cis-acting elements related to SA and JA hormones have been identified in the promoter regions of WRKY TFs in Arabidopsis, tomato, wheat, and rice (Liu et al., 2007; Miao and Zentgraf, 2007; Murray et al., 2007; Van et al., 2014). In Arabidopsis, AtWRKY18, AtWRKY40, and AtWRKY60 act as negative regulators of ABA signaling for seed germination and development (Chen et al., 2010). This study demonstrated multiple hormone-related cis-acting elements in the promoter regions of WRKY53 genes in four cotton species by predicting the cis-acting elements of cotton WRKY53. Among them, the cis-acting elements of MeJA and SA were ubiquitously present in the promoter region of the WRKY53 gene. In response to cotton hormone treatment, the results showed that GhWRKY53 was induced by SA and MeJA at 24 and 48 h. Similar to the WRKY53 gene in G. barbadense, GbWRKY53 expression increased after SA and MeJA induction, with SA responding more compared to MeJA (Li et al., 2017). We speculated that GhWRKY53 might act as a regulatory factor in the SA and MeJA signaling processes of cotton.
Researchers found that WRKY53 was specifically induced by SA following Arabidopsis infection to improve the plant’s defense against pathogenic fungus (Hu et al., 2012). In Arabidopsis, WRKY53 enhanced the disease resistance of plants by inhibiting the MeJA signaling pathway and activating the SA signaling pathway. In this study, both silenced and control plants were inoculated with V. dahliae, and the expression levels of SA and MEJA were measured. The results showed that the SA content in the silenced plants was significantly lower in different resistance varieties, while the MEJA content in the silenced plants was significantly higher at 48 h of inoculation. GhPAL and GhPRI have been confirmed to be involved in plant resistance to fungal infection and can be used as innate immunity markers in plants (Zhang et al., 2023). To further explore the function of GhWRKY53 in defense against V. dahliae, the relative expression levels of the SA biosynthesis gene (GhPAL) and response gene (GhPRI) were monitored before and after V. dahliae treatment in gene-silenced plants. Additionally, the relative expression levels of the JA biosynthesis genes GhLOX and the JA signal response genes GhPDF1.2 were monitored. Expression of the related pathway genes also followed a similar trend. The results suggest which further confirmed that GhWRKY53 might act as a positive regulator in resistance to V. dahliae. It was speculated that the silencing of GhWRKY53 inhibited the signaling pathway of SA and activated the signaling pathway of MEJA in cotton, thereby reducing the resistance of cotton to V. dahliae. These factors work together to enhance the resistance of cotton plants to V. dahliae infection. However, the interaction mechanism between MEJA and SA signaling pathways in cotton in response to V. dahliae requires further study.
5 Conclusion
In this study, We identified and obtained the gene encoding type III WRKY transcription factor GhWRKY53 in upland cotton. Expression of GhWRKY53 and the contents of SA and MeJA in different resistant varieties inoculated with V. dahliae showed that GhWRKY53 mediated SA and MeJA signal transduction. Silencing of GhWRKY53 inhibited the SA pathway and activated the MEJA pathway, thereby reducing the resistance of plants to V. dahliae. Our study demonstrated that GhWRKY53 can change the tolerance of upland cotton to V. dahliae by regulating the expression of SA and MEJA pathway-related genes.
Data availability statement
The original contributions presented in the study are included in the article/Supplementary Material. Further inquiries can be directed to the corresponding authors.
Author contributions
YZ, YL, and FL designed and supervised the research. HC, FL, and YZ revised the manuscript. JS and YW guided the content of the article. YL, XZ, and JZ performed the data analysis. YL and HC finished the writing of the manuscript. All authors contributed to the article and approved the submitted version.
Funding
This study was supported by grants from the Youth Technology Leading Talents Project in the Corps (No. 2022CB002-06), International S&T Cooperation Projects of BINGTUAN (No. 2021BC001), the Key Research and Development Program of Shihezi City (No. 2021NY01), and the Science and Technology Cooperation Promotion Plan of Shihezi University (GJHZ202201).
Conflict of interest
The authors declare that the research was conducted in the absence of any commercial or financial relationships that could be construed as a potential conflict of interest.
Publisher’s note
All claims expressed in this article are solely those of the authorsand do not necessarily represent those of their affiliated organizations, or those of the publisher, the editors and the reviewers. Any product that may be evaluated in this article, or claim that may be made by its manufacturer, is not guaranteed or endorsed by the publisher.
Supplementary material
The Supplementary Material for this article can be found online at: https://www.frontiersin.org/articles/10.3389/fpls.2023.1203695/full#supplementary-material
References
Chen, H., Lai, Z., Shi, J., Xiao, Y., Chen, Z., Xu, X. (2010). Roles of arabidopsis WRKY18, WRKY40 and WRKY60 transcription factors in plant responses to abscisic acid and abiotic stress. BMC Plant Biol. 10, 281. doi: 10.1186/1471-2229-10-281
Duan, Y., Jiang, Y., Ye, S., Karim, A., Ling, Z., He, Y., et al. (2015). PtrWRKY73, a salicylic acid-inducible poplar WRKY transcription factor, is involved in disease resistance in arabidopsis thaliana. Plant Cell Rep. 34 (5), 831–841. doi: 10.1007/s00299-015-1745-5
Eulgem, T., Rushton, P. J., Robatzek, S., Somssich, I. E. (2000). The WRKY superfamily of plant transcription factors. Trends Plant Sci. 5 (5), 199–206. doi: 10.1016/S1360-1385(00)01600-9
Fradin, E., Thomma, B. (2006). Physiology and molecular aspects of Verticillium wilt diseases caused by V. dahliae and V. albo-atrum. Mol Plant Pathol. 7 (2), 71–86. doi: 10.1111/j.1364-3703.2006.00323.x
Guo, W., Jin, L., Miao, Y., He, X., Hu, Q., Guo, K., et al. (2016). An ethylene response-related factor, GbERF1-like, from Gossypium barbadense improves resistance to Verticillium dahliae via activating lignin synthesis. Plant Mol. Biol. 91 (3), 305–318. doi: 10.1007/s11103-016-0467-6
Hinderhofer, K., Zentgraf, U. (2001). Identification of a transcription factor specifically expressed at the onset of leaf senescence. Planta 213 (3), 469–473. doi: 10.1007/s004250000512
Hu, Y., Dong, Q., Yu, D. (2012). Arabidopsis WRKY46 coordinates with WRKY70 and WRKY53 in basal resistance against pathogen Pseudomonas syringae. Plant Sci. 185-186, 288–297. doi: 10.1016/j.plantsci.2011.12.003
Huh, S. U., Choi, L. M., Lee, G. J., Kim, Y. J., Paek, K. H. (2012). Capsicum annuum WRKY transcription factor d (CaWRKYd) regulates hypersensitive response and defense response upon tobacco mosaic virus infection. Plant Sci. 197, 50–58. doi: 10.1016/j.plantsci.2012.08.013
Klosterman, S. J., Atallah, Z. K., Vallad, G. E., Subbarao, K. V. (2009). Diversity, pathogenicity, and management of verticillium species. Annu. Rev. Phytopathol. 47, 39–62. doi: 10.1146/annurev-phyto-080508-081748
Kumar, S., Stecher, G., Tamura, K. (2016). MEGA7: molecular evolutionary genetics analysis version 7.0 for bigger datasets. Mol. Biol. Evol. 33, 1870–1874. doi: 10.1093/molbev/msw054
Li, C., He, X., Luo, X., Xu, L., Liu, L., Min, L., et al. (2014). Cotton WRKY1 mediates the plant defense-to-development transition during infection of cotton by Verticillium dahliae by activating jasmonate zim-Domain1 expression. Plant Physiol. 166 (4), 2179–2194. doi: 10.1104/pp.114.246694
Li, J., Liu, S., Cai, L., Wang, Z., Dong, L., Liu, J., et al. (2017). Cloning and expression analysis of GbWRKY53 in G. barbadense. J. Agric. Sci. Technol. 19 (11), 7. (in Chinese). doi: 10.13304/j.nykjdb.2017.0200
Li, Y., Zhang, X., Lin, Z., Zhu, Q., Li, Y., Xue, F., et al. (2023). Comparative transcriptome analysis of interspecific CSSLs reveals candidate genes and pathways involved in verticillium wilt resistance in cotton (Gossypium hirsutum l.). Ind. Crops Products 197, 116560. doi: 10.1016/j.indcrop.2023.116560
Liu, X., Bai, X., Wang, X., Chu, C. (2007). OsWRKY71, a rice transcription factor, is involved in rice defense response. J. Plant Physiol. 164 (8), 969–979. doi: 10.1016/j.jplph.2006.07.006
Liu, Y., Schiff, M., Dinesh-Kumar, S. P. (2002). Virus-induced gene silencing in tomato. Plant J. 31, 777–786. doi: 10.1046/j.1365-313X.2002.01394.x
Livak, K. J., Schmittgen, T. D. (2001). Analysis of relative gene expression data using real-time quantitative PCR and the 2(T)(-delta delta c) method. Methods 25, 402–408. doi: 10.1006/meth.2001.1262
Lu, Y., Cheng, X., Jia, M., Zhang, X., Xue, F., Li, Y., et al. (2021). Silencing GhFAR3.1 reduces wax accumulation in cotton leaves and leads to increased susceptibility to drought stress. Plant Direct 5 (4), e00313. doi: 10.1002/pld3.313
Luna, E., Ton, J. (2012). The epigenetic machinery controlling transgenerational systemic acquired resistance. Plant Signal Behav. 7, 615–618. doi: 10.4161/psb.20155
Miao, Y., Zentgraf, U. (2007). The antagonist function of Arabidopsis WRKY53 and ESR/ESP in leaf senescence is modulated by the jasmonic and salicylic acid equilibrium. Plant Cell. 19 (3), 819–830. doi: 10.1105/tpc.106.042705
Murray, S. L., Ingle, R. A., Petersen, L. N., Denby, K. J. (2007). Basal resistance against Pseudomonas syringae in Arabidopsis involves WRKY53 and a protein with homology to a nematode resistance protein. Mol. Plant Microbe Interact. 20 (11), 1431–1438. doi: 10.1094/MPMI-20-11-1431
Pandey, S. P., Somssich, I. E. (2009). The role of WRKY transcription factors in plant immunity. Plant Physiol. 150 (4), 1648–1655. doi: 10.1104/pp.109.138990
Rushton, P. J., Somssich, I. E. (1998). Transcriptional control of plant genes responsive to pathogens. Curr. Opin. Plant Biol. 1 (4), 311–315. doi: 10.1016/1369-5266(88)80052-9
Shaban, M., Miao, Y., Ullah, A., Khan, A. Q., Menghwar, H., Khan, A. H., et al. (2018). Physiological and molecular mechanism of defense in cotton against Verticillium dahliae. Plant Physiol. Biochem. 125, 193–204. doi: 10.1016/j.plaphy.2018.02.011
Shang, J., Xi, D., Xu, F., Wang, S., Cao, S., Xu, M., et al. (2011). A broad-spectrum, efficient and nontransgenic approach to control plant viruses by application of salicylic acid and jasmonic acid. Planta 233 (2), 299–308. doi: 10.1007/s00425-010-1308-5
Van, E. L., Davidson, R. M., Wu, S., Zhao, B. Y., Botha, A. M., Leach, J. E., et al. (2014). The transcriptional network of WRKY53 in cereals links oxidative responses to biotic and abiotic stress inputs. Funct. Integr. Genomics 14 (2), 351–362. doi: 10.1007/s10142-014-0374-3
Van, V. M. C., Neeleman, L., Bol, J. F., Linthorst, H. J. (2011). Tobacco transcription factor NtWRKY12 interacts with TGA2.2 in vitro and in vivo. Front. Plant Sci. 2, 32. doi: 10.3389/fpls.2011.00032
Wan, J., Zhang, S., Stacey, G. (2004). Activation of a mitogen-activated protein kinase pathway in Arabidopsis by chitin. Mol. Plant Pathol. 5 (2), 125–135. doi: 10.1111/j.1364-3703.2004.00215.x
Xiong, X., Sun, S., Li, Y., Zhang, X., Sun, J., Xue, F. (2019). The cotton WRKY transcription factor GhWRKY70 negatively regulates the defense response against Verticillium dahliae. Crop J. 7 (3), 393–402. doi: 10.1016/j.cj.2018.10.005
Xiong, X., Sun, S., Zhang, X., Li, Y., Liu, F., Zhu, Q., et al. (2020). GhWRKY70D13 regulates resistance to Verticillium dahliae in cotton through the ethylene and jasmonic acid signaling pathways. Front. Plant Sci. 11, 69. doi: 10.3389/fpls.2020.00069
Yan, Y., Jia, H., Wang, F., Wang, C., Liu, S., Guo, X.. (2015). Overexpression of GhWRKY27a reduces tolerance to drought stress and resistance to Rhizoctonia solani infection in transgenic Nicotiana benthamiana. Front Physiol. 6, 265. doi: 10.3389/fphys.2015.00265
Zhang, S., Dong, L., Zhang, X., Fu, X., Zhao, L., Wu, L., et al. (2023). The transcription factor GhWRKY70 from gossypium hirsutum enhances resistance to verticillium wilt via the jasmonic acid pathway. BMC Plant Biol. 23, 141. doi: 10.1186/s12870-023-04141-x
Zhang, L., Gu, L., Ringler, P., Smith, S., Rushton, P. J., Shen, Q. J. (2015). Three WRKY transcription factors additively repress abscisic acid and gibberellin signaling in aleurone cells. Plant Sci. 236, 214–222. doi: 10.1016/j.plantsci.2015.04.014
Zhang, Y., Wang, X., Ding, Z., Ma, Q., Zhang, G. R., Zhang, S., et al. (2013). Transcriptome profiling of Gossypium barbadense inoculated with Verticillium dahliae provides a resource for cotton improvement. BMC Genomics 14, 637. doi: 10.1186/1471-2164-14-637
Zhang, Y., Wang, X., Rong, W., Yang, J., Li, Z., Wu, L., et al. (2017). Histochemical analyses reveal that stronger intrinsic defenses in Gossypium barbadense than in g. hirsutum are associated with resistance to Verticillium dahliae. Mol. Plant Microbe Interact. 30 (12), 984–996. doi: 10.1094/MPMI-03-17-0067-R
Keywords: Gossypium hirsutum, verticillium wilt, virus virus-induced gene silencing, GhWRKY53, jasmonic acid, salicylic acid
Citation: Li Y, Chen H, Wang Y, Zhu J, Zhang X, Sun J, Liu F and Zhao Y (2023) Function analysis of GhWRKY53 regulating cotton resistance to verticillium wilt by JA and SA signaling pathways. Front. Plant Sci. 14:1203695. doi: 10.3389/fpls.2023.1203695
Received: 11 April 2023; Accepted: 08 May 2023;
Published: 02 June 2023.
Edited by:
Hongjie Feng, Institute of Cotton Research (CAAS), ChinaCopyright © 2023 Li, Chen, Wang, Zhu, Zhang, Sun, Liu and Zhao. This is an open-access article distributed under the terms of the Creative Commons Attribution License (CC BY). The use, distribution or reproduction in other forums is permitted, provided the original author(s) and the copyright owner(s) are credited and that the original publication in this journal is cited, in accordance with accepted academic practice. No use, distribution or reproduction is permitted which does not comply with these terms.
*Correspondence: Feng Liu, liufeng@shzu.edu.cn; Yiying Zhao, zhaoyiying@shzu.edu.cn
†These authors have contributed equally to this work