- State Key Laboratory of Agrobiotechnology and MOA Key Laboratory for Monitoring and Green Management of Crop Pests, China Agricultural University, Beijing, China
Lectin receptor-like kinases (LecRKs) locate on the cell membrane and play diverse roles in perceiving environmental factors in higher plants. Studies have demonstrated that LecRKs are involved in plant development and response to abiotic and biotic stresses. In this review, we summarize the identified ligands of LecRKs in Arabidopsis, including extracellular purine (eATP), extracellular pyridine (eNAD+), extracellular NAD+ phosphate (eNADP+) and extracellular fatty acids (such as 3-hydroxydecanoic acid). We also discussed the posttranslational modification of these receptors in plant innate immunity and the perspectives of future research on plant LecRKs.
Introduction
Plants constantly face challenges from bacteria, fungi, viruses, oomycetes, and nematodes. To fight against these threats, plants have evolved a sophisticated innate immune system. Conserved pathogen-/damage-/microbe-/herbivore-associated molecular patterns (PAMPs/DAMPs/MAMPs/HAMPs) are recognized by cell surface-localized pattern recognition receptors (PRRs), leading to the pattern-triggered immunity (PTI) responses (Jones and Dangl, 2006; Tanaka and Heil, 2021; Snoeck et al., 2022). Pathogens can secrete virulence effectors into plant cells through delivery devices, such as the type III secretion system (T3SS) in bacteria, haustoria in fungi and oomycetes, and stylets in aphids and nematodes, thereby inhibiting PTI responses and promoting infection (Dangl et al., 2013). To counteract the infection, the intracellular nucleotide-binding leucine-rich repeat receptors (NLRs) of plants recognize the cognate pathogen effectors, in many cases triggering a strong immune response, such as hypersensitive response (HR), referring to effector-triggered immunity (ETI) (Jones and Dangl, 2006). Recently, studies have demonstrated that PTI and ETI are not two independent immune pathways. In contrast, ETI enhances PTI immune response, and PTI, on the other hand, reinforces ETI-induced cell death (Ngou et al., 2021; Tian et al., 2021; Ngou et al., 2022).
PRRs, a group of cell surface receptors, consist of two different types of cell surface-localized proteins, receptor-like kinases (RLKs) and receptor-like proteins (RLPs) (Couto and Zipfel, 2016). RLKs possess three functional domains, including an N-terminal variable ligand-binding ectodomain, a single-pass transmembrane domain, and an intracellular kinase domain (Walker, 1994). Unlike RLKs, RLPs lack the intracellular kinase domain but have a short cytosolic tail (Jeong et al., 1999). The typical extracellular ligand-binding domains of RLKs and RLPs include the leucine-rich repeat (LRR), lysine motif (LysM), lectin domain, and an epidermal growth factor (EGF)-like domain. However, some RLKs, such as BAK1 and SERK1, lack functional extracellular domains. Instead, they have the functional kinase domains and act as coreceptors or scaffold proteins for the typical RLKs or RLPs that possess extracellular domains (Li et al., 2002; Stegmann et al., 2017; Liang and Zhou, 2018).
Lectin RLK (LecRK) is a large subfamily of RLKs and its N-terminal lectin domain reversibly binds to carbohydrates (Bouwmeester and Govers, 2009). Based on the characteristics of extracellular lectin domains, LecRKs are classified into three types: G-type, L-type, and C-type lectins (Vaid et al., 2013). The number of LecRKs varies dramatically among different species (Table 1). G-type LecRKs contain an α-mannose binding bulb lectin domain, an S-locus glycoprotein domain (SLG), and a PAN and/or Epidermal Growth Factor (EGF) domain (Vaid et al., 2013; Sun et al., 2020). L-type LecRKs contain a legume-lectin domain with a typical β-sandwich structure (Bellande et al., 2017). In plants, the number of C-type LecRKs, which are predicted to be calcium-dependent kinases, is quite small and their function remains unclear (Sun et al., 2020). The central transmembrane domain of LecRKs usually consists of approximately 18-25 amino acid residues. Kinase domains generally consist of 250-300 amino acid residues and contain various conserved phosphorylation sites that are responsible for transducing external signals to downstream pathways (Morillo and Tax, 2006).
Several excellent reviews have described the structures and functions of LecRKs in various abiotic and biotic processes, and in the process of plant development (Vaid et al., 2012; Singh and Zimmerli, 2013; Vaid et al., 2013; Sun et al., 2020). Wang et al. performed a systematic functional analysis to evaluate phenotypic changes in Arabidopsis LecRK T-DNA insertion lines in response to pathogen infection and abiotic stress treatment (Wang et al., 2014). We summarized the recent research progress of LecRKs in Table 2. In this review, we will mainly focus on summarizing the latest findings in ligand recognition, posttranslational modification, and natural variation of LecRKs.
Ligand perception by LecRKs
LecRKs are widely distributed in plant kingdoms, but interestingly they have no orthologs in yeast and human genomes (Navarro-Gochicoa et al., 2003). L-type LecRKs have a conserved hydrophobic cavity that can bind hydrophobic ligands like monosaccharides (glucose/fucose/mannose) or polypeptides, while G-type LecRKs exhibit a strong high binding affinity to α-D mannose. Due to the diversity of LecRKs and their target carbohydrates, it is difficult to identify the ligand-receptor interaction between them (Bellande et al., 2017). To date, only four ligands (eATP, eNAD+, eNADP+, and 3-OH-C10:0) of LecRKs have been identified.
ATP
ATP is not only an essential energy currency in nature, but also acts as an enzyme cofactor. Moreover, ATP is released into the extracellular matrix after wounding or other environmental stimulation (Kim et al., 2006; Khakh and Burnstock, 2009; Dark et al., 2011; Ramachandran et al., 2019). At present, eATP signaling has been widely studied in animals. Most mammals contain two families of ATP receptors, seven P2X receptors and eight P2Y receptors. P2X receptors are ion channels on the plasma membrane and are activated by the binding of eATP (Khakh and North, 2006). P2Y receptors are a family of metabotropic receptors that couple to intracellular second-messenger systems through heteromeric G-proteins (Van Der Giet et al., 2002). These receptors have diverse temporal and spatial expression patterns and respond differently to individual nucleotide ligands (Lustig et al., 1993; Webb et al., 1993; Ralevic and Burnstock, 1998; Abbracchio et al., 2006).
In contrast to mammals, the function of eATP in plants is poorly understood. During growth and in response to various biotic and abiotic stimuli, ATP can be released into the extracellular matrix. eATP plays fundamental roles in mediating plant defense against pathogens and herbivores, such as the production of reactive oxygen species (ROS), elevation of cellular Ca2+ concentration, activation of mitogen-activated protein kinase (MPK) phosphorylation, and indolic glucosinolate pathway (Figure 1) (Demidchik et al., 2003; Roux and Steinebrunner, 2007; Tanaka et al., 2010; Liu et al., 2012; Feng et al., 2015; Cho et al., 2017; Jewell et al., 2022). However, plant genomes do not contain potential orthologs of animal P2X and P2Y receptors (Fountain et al., 2008; Tanaka et al., 2010). Nevertheless, eATP did induce NADPH oxidase-mediated accumulation of superoxide (O2-), indicating that plants must possess a receptor for eATP (Song et al., 2006). Until 2014, the first eATP receptor, the DOes not Respond to Nucleotides 1 (DORN1), was identified in Arabidopsis by forward genetic screening approach (Choi et al., 2014a). DORN1 is an L-type lectin receptor kinase that binds ATP with high affinity (dissociation constant of 45.7 ± 3.1 nM). In addition, eight ethylmethanesulfonate (EMS) mutants and two T-DNA insertion lines, which are distributed in the entire gene of DORN1, including the extracellular lectin domain, the transmembrane domain and the intracellular serine/threonine kinase domain, all showed defects in eATP-induced calcium influx (Choi et al., 2014a; Choi et al., 2014b). Therefore, DORN1 is a bona fide receptor for eATP.
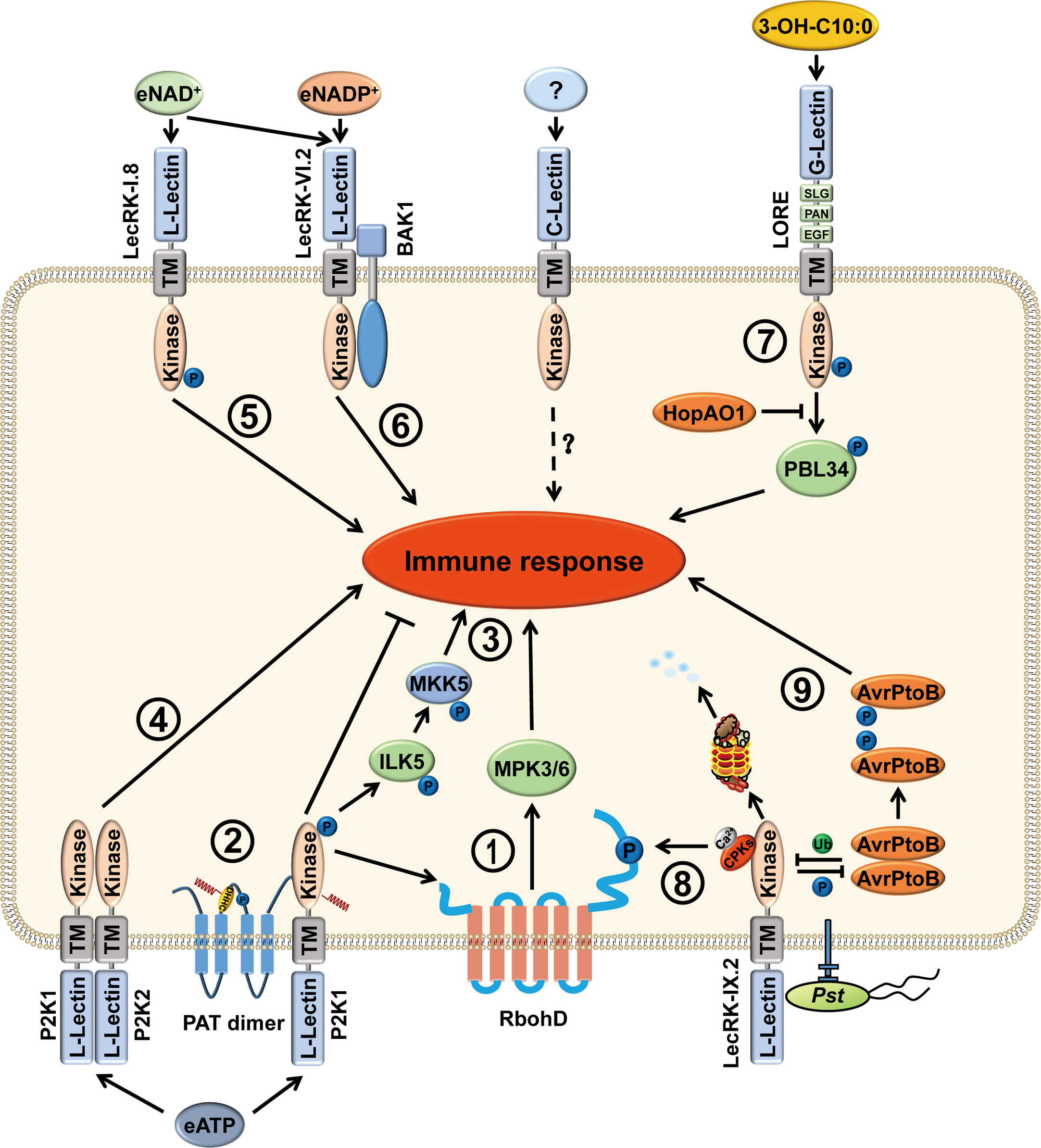
Figure 1 Functions of LecRKs in stress responses of plants. LecRKs are divided into three types, L-type, G-type and C-type, based on the extracellular lectin domain in plants. LecRKs can recognize specific extracellular signaling molecules and transduce signals into the cell, thus activating the plant immune response. (1) P2K1 (LecRK-I.9) perceives eATP and then phosphorylates RbohD and MPK3/6, causing increased production of ROS and stomatal closure to fight against pathogen infection. (2) In addition, P2K1 directly interacts with and phosphorylates PATs to activate their S-acylation ability. Activated PATs S-acylate P2K1 to inhibit the immune response and protect plant growth. (3) In the presence of eATP, P2K1 interacts with and phosphorylates ILK5. Then, ILK5 interacts with and phosphorylates the MKK5, leading to an activated immune response. (4) Upon eATP treatment, P2K1 and P2K2 (LecRK-I.5) interact with each other and may function as part of a heteromeric complex. (5) LecRK-I.8 can perceive eNAD+ and activate the plant immune response. (6) LecRK-VI.2 is a potential receptor for eNAD+ and eNADP+. Furthermore, LecRK-VI.2/BAK1 complex mediates systemic acquired resistance that is triggered by eNAD+. (7) G-type LecRK LONE is the receptor of 3-OH-C10:0. After perception of 3-OH-C10:0, the phosphorylation level of LORE Y600 is elevated and trans-phosphorylates PBL34 at T306 and T310. Phosphorylated PBL34 dissociates from LORE, activating immune response. However, the bacterial effector HopAO1 targets LORE Y600 and dephosphorylates the tyrosine-phosphorylated Y600 to suppress immune response. (8) When perceiving pathogen infection, LecRK-IX.2 recruits CPKs to phosphorylate RbohD, resulting in PTI activation, ROS production, and the subsequent ROS triggered SA accumulation. (9) Moreover, the bacterial effector AvrPtoB ubiquitinates LecRK-IX.2 to degrade LecRK-IX.2, thus suppressing LecRK-IX.2-mediated PTI. However, LecRK-IX.2 phosphorylates AvrPtoB at S335 which leads to AvrPtoB dimer dissociation and reduces its virulence.
Arabidopsis DORN1, also termed P2K1 or LecRK-I.9, belongs to the L-type LecRK subfamily, which contains 45 members. Most LecRK-encoded genes are differentially expressed in various tissues and at different developmental stages. Their expression can be induced by various elicitors and pathogen infections (Bouwmeester and Govers, 2009). dorn1 mutants completely lose the ability to respond to eATP in young seedlings. However, the diverse expression patterns of different LecRKs suggest the possibility that there are other LecRKs involved in the eATP-activated signaling pathway (Choi et al., 2014a; Choi et al., 2014b). In Arabidopsis, L-type LecRK family is divided into nine subclades; P2K1 belongs to clade I which consists of 11 members. Recently, by screening all members of the LecRK clade I, only P2K2 (LecRK-I.5) was able to partially restore the Ca2+ response in p2k1 mutant plants. Further experiments confirmed that P2K2 is a second purinergic receptor. P2K1 and P2K2 showed self-association and formed heterodimer with each other upon ATP treatment (Chen et al., 2017; Pham et al., 2020) The fact that the low expression of P2K2 and activation of P2K1 leads to transphosphorylation of P2K2 likely explains why P2K2 was not identified in the initial eATP response deficient mutant screening (Pham et al., 2020).
A previous study showed that by analyzing data from Genevestigator V3 web, different Arabidopsis LecRK genes had different expression profiles in response to hormone treatment, abiotic stress, elicitor treatment and pathogen infection (Bouwmeester and Govers, 2009). We analyzed and visualized the relative expression patterns of 45 L-type AtlLecRKs under both cold (4°C) and heat (38°C) stresses using Plant eFP database (Figure S1). Most AtlLecRKs showed obvious changes at 12 h after cold treatment and 1h after heat treatment. P2K1 did not show obvious changes after temperature treatment, however, P2K2 could be suppressed by cold treatment and induced by heat. Therefore, by changing specific growth conditions, such as high or low temperature, it may be possible to identify additional eATP receptors in plants in the future.
NAD+
NAD+ and NAD phosphate (NADP+) are electron carriers in most of metabolic reactions. In mammalian cells, NAD+ is released into the extracellular space upon cell death and inflammation, which could potentially activate immunity, but whether there is an eNAD+-recognition receptor remains elusive (Billington et al., 2006). In Arabidopsis, many experiments have revealed that NAD+ and NADP+ are released into the extracellular compartment during infection by the bacterial pathogens. NAD+ or NADP+ release leads to the activation of salicylic acid (SA) signaling pathway and the accumulation of ROS resulting in disease resistance (Zhang and Mou, 2009; Pétriacq et al., 2012; Zhang and Mou, 2012; Pétriacq et al., 2016). In addition, exogenously applied NAD+ moves systemically and induces systemic acquired resistance (SAR) (Wang et al., 2019). These studies suggest that there is an NAD+ or NADP+ receptor to sense these signals in Arabidopsis.
In 2017, Wang et al. analyzed the transcriptomic changes in Arabidopsis after eNAD+ treatments. The microarray data indicated that the expression of some receptor kinases was induced by NAD+. In the receptor kinase mutant lecrk I.8, NAD+-induced PR1 expression was significantly decreased and disease resistance to P. syringae pv. maculicola (Psm) ES4326 was largely compromised (Figure 1) (Wang et al., 2017). LecRK-I.8 specifically binds to NAD+, but not to NADP+, ATP, ADP, or AMP. In contrast, P2K1, LecRK-I.3, and LecRK-I.6 do not bind to NAD+. Moreover, although LecRK-I.8 null mutants do not respond to low concentration of NAD+, it does have response to high concentrations of NAD+, including induced Pathogenesis-Related gene (PR) expression and enhanced disease resistance. These data suggest that LecRK-I.8 is not the only receptor that perceives eNAD+ (Wang et al., 2017). In addition, LecRK-I.8 is also a potential cell surface receptor for Pieris brassicae egg-derived elicitors, where loss of LecRK-I.8 significantly reduced the immune response to the egg extract, suggesting that there is a potential egg-derived ligand for LecRK-I.8 (Gouhier-Darimont et al., 2019).
Although LecRK-I.8 is a potential receptor for NAD+, it does not bind NADP+ (Wang et al., 2017). Later, the same group found that LecRK-VI.2 is a potential receptor for eNADP+, where NADP+-induced disease resistance is clearly reduced in lecrk-VI.2 mutants. The recombinant extracellular domain of LecRK-VI.2 (eLecRK-VI.2) exhibited a typical saturation curve in binding with 32P-NAD+. Unlabeled NAD+ and NADP+ compete for binding of 32p-NAD+, but unlabeled ATP cannot. However, the binding affinity of NADP+ with eLecRK-VI.2 is slightly higher than that of NAD+ (Figure 1) (Wang et al., 2019). Notably, exogenous NAD(P)+-induced systemic PR1 transcription was only slightly reduced in lecrk-VI.2 mutants, suggesting that there are other receptors that can sense NAD(P)+.
Lipopolysaccharide and 3-OH-C10:0
Lipopolysaccharide (LPS), a major cell wall component of gram-negative bacteria, consists of three different functional moieties: lipophilic lipid A, a core oligosaccharide, and O-antigen (Ranf, 2016; Kagan, 2017). In mammals, LPS acts as MAMP and triggers strong immune responses, and the molecular mechanism for LPS recognition has been well studied. The event can be divided into intracellular and extracellular recognition in two different ways. In extracellular recognition, Toll-like receptor 4 (TLR4) forms a heterodimer with myeloid differentiation factor 2 (MD2) to recognize LPS and initiates intracellular signaling mediated by caspase-11 (Park et al., 2009; Kayagaki et al., 2011). Moreover, LPS-binding protein (LBP) and CD14 are also essential for LPS recognition (Tsukamoto et al., 2018). In intracellular recognition, human caspase-4 and its mouse homologue caspase-11 can directly bind to LPS and lipid A with high specificity and affinity, resulting in activation of caspases (Shi et al., 2014).
Compared to the studies of the responses to LPS in mammals, there are few studies of the mechanisms involved in LPS recognition and downstream signal transduction in plants. LPS-elicited defense-related responses include programmed cell death, nitric oxide (NO) production, PR gene expression, and ROS burst in plants (Zeidler et al., 2004; Desaki et al., 2006; Sun et al., 2012; Proietti et al., 2014; Shang-Guan et al., 2018). Using a forward-genetic approach to screen Arabidopsis mutants that are insensitive to LPS, Ranf et al. reported that a G-type LecRK LORE (lipooligosaccharide-specific reduced elicitation) is required for LPS -mediated PTI responses (Ranf et al., 2015). Moreover, they found that lipid A moiety is indispensable and sufficient for LORE-dependent sensing of LPS. Removal of the ester-linked acyl chains from lipid A abolishes its ability to trigger plant immunity (Ranf et al., 2015). In Arabidopsis, miR393 regulates one L-type LecRK and two G-type LecRKs following the perception of bacterial LPS to initiate immunity and basal resistance (Djami-Tchatchou and Dubery, 2019). These results suggest that LPS and acylated Lipid A are essential for immune sensing in Arabidopsis.
However, the same group in 2019 found that medium-chain 3-hydroxy fatty acids (mc-3-OH-FAs) rather than LPS, produced during LPS biosynthesis, are sensed by LORE (Figure 1). mc-3-OH-FAs can be copurified with LPS and lipid A. mc-3-OH-FA-depleted LPS does not activate the immune responses (Kutschera et al., 2019). The immune strength elicited by mc-3-OH-FAs depends on the chain length and the specificity of hydroxylation. Free 3-OH-C10:0 is the strongest immune molecule and has a strong binding affinity with the LORE ectodomain. In addition, LORE can sense the (R)-3-hydroxyalkanoate precursors HAAs which are involved in bacterial surface dissemination and biofilm development (Schellenberger et al., 2021). These results indicate that plants can also utilize LecRK to perceive pathogens by sensing the conserved features of microbial metabolites.
Modification of LecRKs
Protein phosphorylation and ubiquitination are two major posttranslational modifications in eukaryotes (Mithoe and Menke, 2018). These modifications play important roles in the turnover of proteins, signal transduction, and cellular homeostasis.
Phosphorylation modification
Phosphorylation and autophosphorylation of LecRKs
Phosphorylation is required for signal transduction and for maintaining cellular homeostasis upon receptor complex activation. Previous studies have shown that phosphorylation regulation is critical for the function of protein kinase RLKs (Couto and Zipfel, 2016; Tang et al., 2017; Mithoe and Menke, 2018). PnLPK, a lectin-like protein kinase in Populus nigra var. italica, is the first identified LecRK in plants, and exhibits autophosphorylation activity on serine and threonine residues in the protein kinase domain but not on tyrosine residues (Nishiguchi et al., 2002). Further studies proved that the autophosphorylation of LecRKs plays important roles in signal transduction in Arabidopsis and rice. AtLecRK-VI.2 has autophosphorylation activity in vitro in the presence of divalent metal cations and positively modulates early bacterium-mediated MPK signaling (Singh et al., 2012; Singh et al., 2013). P2K1 has autophosphorylation activity and this activity is essential for ATP-induced plant responses (Choi et al., 2014a; Choi et al., 2014b). Mass spectrometry (MS) and biochemistry assays revealed that autophosphorylation of S391, S440, and S451 is critical for P2K1 kinase domain-mediated eATP recognition. Phosphomimetic S391D, S440D, and S451D mutants exhibited a significant increase in Ca2+ cyt concentration after ATP addition (Chen et al., 2017). Overexpression of LecRK-IX.2 leads to spontaneous cell death in Arabidopsis (Wang et al., 2015b). In contrast, three kinase-dead LecRK-IX.2 mutant variants, LecRK-IX.2K379R, LecRK-IX.2K477E, and LecRK-IX.2D532N, not only have no autophosphorylation activity, but also lost the ability to elicit cell death in N. benthamiana (Luo et al., 2017).
In addition to the serine/threonine phosphorylation, recent studies have demonstrated that the tyrosine phosphorylation of LRR-RLKs and LysM-RLKs is also essential for immune activation (Liu et al., 2018a; Perraki et al., 2018). Although plants do not have tyrosine kinases discovered in animals, many RLKs, including BAK1, have been shown to possess dual specificity kinases, auto-phosphorylating in vitro on serine/threonine as well as tyrosine residues (Perraki et al., 2018). Luo et al. showed that LORE is also a dual-specificity protein kinase (Figure 1) (Luo et al., 2020). Y600 in LORE is a conserved residue in many immunity-related RLK orthologs, including G-type lectin kinases, EFR, CERK1, and BAK1 (Macho et al., 2014; Liu et al., 2018a; Perraki et al., 2018; Luo et al., 2020). Substitution of Y600 with phenylalanine markedly reduces the kinase activity of LORE and LORE-mediated 3-OH-C10:0 recognition in Arabidopsis.
The phosphorylation targets of LecRKs
In addition to autophosphorylation, LecRKs can also phosphorylate other proteins to transmit the signal to downstream pathways. In rice, a G-type LecRK OsPID2 is able to phosphorylate OsPUB15, a U-box/ARM repeat protein. Phosphorylated OsPUB15 exhibits an active E3 ligase activity. In addition, overexpression of OsPUB15 in rice leads to a spontaneous cell death phenotype as well as a constitutive activation of plant basal defense responses (Chen et al., 2006; Wang et al., 2015a). The L-type LecRK Salt Intolerance 1 (SIT1) phosphorylates MPK3/6 to mediate salt sensitivity and regulates ethylene homeostasis in rice (Li et al., 2014). In Arabidopsis, upon perception of eATP, P2K1 directly phosphorylates the NADPH oxidase RBOHD and the Raf-like MAP kinase kinase kinase INTEGRIN-LINKED KINASE 5 (ILK5), which subsequently elevates the production of ROS, induces stomatal closure and triggers innate immune response (Figure 1) (Chen et al., 2017; Kim et al., 2023). Although LecRK-IX.2 interacts with RBOHD, it is unable to directly phosphorylate RBOHD. Instead, LecRK-IX.2 likely recruits the calcium-dependent protein kinases (CPKs) to phosphorylate and activate RBOHD, which leads to the ROS burst and the enhanced ROS-triggered SA biosynthesis (Figure 1) (Luo et al., 2017). Recent phosphoproteomics biochemical results showed that cGMP-dependent protein kinase is a potential substrate of PtLecRLK1 (Shrestha et al., 2023).
PRRs often trans-phosphorylate RLCKs to activate immune signaling from extracellular ligand perception into downstream signaling in plants (Monaghan et al., 2014; Liang and Zhou, 2018). Our recent study showed that LORE-mediated immune activation follows this rule. After perception of the ligand 3-OH-C10:0, LORE is autophosphorylated at Y600, which further transphosphorylates the receptor-like cytoplasmic kinases PBL34, PBL35, and PBL36 to activate plant immunity. However, Pseudomonas syringe pv. tomato (Pst) effector HopAO1, which is a phosphatase, targets LORE to dephosphorylate tyrosine-phosphorylated Y600 and suppresses immune responses (Luo et al., 2020). Whether other LecRKs also rely on RLCKs to activate the immune signaling deserves further investigation.
Ubiquitination and palmitoylation modifications
Ubiquitination plays an important role in maintaining cellular homeostasis and activating the immune receptor complex in plants. For example, Arabidopsis LRR-RLK FLAGELLIN-SENSING 2 (FLS2) can be polyubiquitinated by two U-box E3 ligases, PUB12 and PUB13, to attenuate immune signaling upon flagellin recognition (Lu et al., 2011). The large-scale identification of ubiquitination sites in Arabidopsis reveals that over 100 protein kinases that are associated with or integrated into the plasma membrane are ubiquitinated, including L-type LecRKs, LecRK-VII.2 (K339), LecRK-VIII.1 (K374), RLK/LecRK-IV.1 (K350, K370), and a C-type lectin receptor kinase (K265, K278, K292) (Grubb et al., 2021). We recently found that Pst effector AvrPtoB, a 553-amino-acid protein with a functional E3 ligase domain, targets and degrades LecRK-IX.2 to suppress LecRK-IX.2-mediated PTI. In contrast, LecRK-IX.2 phosphorylates AvrPtoB at serine site 335 to interrupt AvrPtoB self-association and reduce its E3 ligase activity (Figure 1) (Xu et al., 2020). Very recently, OsPID2, a key protein in the resistance of rice to Magnaporthe oryzae strain ZB15, was shown to interact with U-box E3 ubiquitin ligase OsPIE3 (PID2-interacting E3). This interaction leads to the intracellular kinase domain of PID2 entering the nucleus and reducing blast disease resistance (Wang et al., 2023). These findings indicate that ubiquitination is important in maintaining the function of LecRKs.
Recently, LecRK S-acylation has been reported. S-acylation is a reversible and cycling posttranslational modification, in which cysteine residues are conjugated with a variety of acyl chains through a thioester bond; however, how to regulate this process is still poorly understood in plants. Arabidopsis plant encodes 24 S-acyl transferases (PATs) which contain a conserved Asp-His-His-Cys (DHHC) catalytic domain; however, the specific substrates of these AtPATs are barely identified (Batistic, 2012). A previous study has identified two proteins from the CLAVATA2 (CLV2)-like AtRLP subfamily and eight RLCK subfamily members that are S-acylated, indicating that S-acylation may play an important role in the LRR-RLK/AtRLP/RLCK signaling pathway (Hemsley et al., 2013). Although subsequent study suggests that juxta-membrane S-acylation of plant RLKs is likely fortuitous, S-acylation of FLS2 adjacent to the transmembrane domain is not required for FLS2-mediated immune signaling (Hurst et al., 2019). A recent study discovered that two DHHC-PAT proteins AtPAT5 and AtPAT9 negatively regulate the plant immune receptor P2K1 via S‐acylation. Upon perception of eATP, P2K1 is rapidly autophosphorylated, which then in turn phosphorylates PATs to activate PAT mediated S-acylation. Activation of PATs can S-acylate P2K1 to reduce P2K1 phosphorylation, dampen the immune response and protect growth (Figure 1) (Chen et al., 2021). These works highlight the important roles of S-acylation in plant innate immunity.
Natural variation of LecRKs
Due to technical advances in genetic mapping, resequencing, and genome-wide association study (GWAS), some LecRKs have been identified to regulate many biological processes in plants. A recent GWAS using a set of 295 natural Arabidopsis accessions showed that LecRK-I.1, a close homolog of LecRK-I.8, is specifically involved in large white butterfly Pieris brassicae egg extract (EE)-triggered HR-like response (Groux et al., 2021). There are five most significant single nucleotide polymorphisms (SNPs) in the LecRK-I.1 gene. One SNP is located in the carbohydrate-binding lectin-like domain. Two are located in the kinase domain, while the other two are silent mutations. Further analysis found that two main haplotypes of LecRK-I.1 segregated at the population level and the SNP in the kinase domain was significantly associated with the EE-triggered HR-like responses (Groux et al., 2021). Moreover, three novel QTLs were determined by genetic analysis of EE-induced HR-like cell death in 56 Brassica rapa accessions. These three QTLs include many candidate genes that are involved in plant immunity processes One QTL contains a cluster of LecRK-I genes, which are homologous genes of AtLecRKI.1 Another QTL includes LecRK-V.5, which negatively modulates plant immunity against necrotrophic bacteria (Arnaud et al., 2012; Bassetti et al., 2022). Further fine-mapping of these identified QTLs will help to identify the key genes. By GWAS mapping of ca. 1,000 resequenced Populus trichocarpa trees individually challenged with an invasive fungal pathogen Sphaerulina musiva, one L-type LecRK was associated with resistance and one G-type LecRK was a susceptibility-associated locus. The L-type LecRK had a mutant frequency of 10% and predicted to have a high impact on protein translation. The G-type LecRK showed only two high-impact mutations at frequency 1.5% and 8.0%. Biochemical results demonstrated that both LecRKs lection domain bound to cell wall preparations of S. musiva (Muchero et al., 2018). Intriguingly, Lin et al. used another advanced technology named RLP/KSeq and successfully fine-mapped the SCR74 (an apoplastic effector of Phytophthora infestans) receptor to a 43-kbp G-LecRK locus in Solanum microdontum plants (Lin et al., 2020).
Recently, some LecRKs have been identified to respond to abiotic stress treatments. GWAS and epigenome wide association studies (EWAS) for the 60 Populus tomentosa hub genes identified that LecRK-VIII.2 is associated with chlorophyll content among different methylotypes (Zhou et al., 2023). Using in-field overwinter survival as a trait, another GWAS and population differentiation analysis in Lotus japonicas revealed that LjLecRK is required for non-acclimated freezing tolerance and haplotype dependent cold responses (Shah et al., 2020; Mustamin et al., 2023). As there is tremendous natural variation in LecRKs, more LecRKs may be identified by GWAS analysis combined with different biotic or abiotic treatments in the future.
Conclusions and future perspectives
In the past decade, our knowledge about plant LecRKs has greatly advanced. Some new ligands have been found. Accordingly, new modifications and natural variations of LecRKs have been discovered. Although many LecRKs have been identified in plants, very few LecRKs have been functionally characterized. Of these LecRKs, most have been discovered in Arabidopsis. Future studies should explore the ligand and signal transduction of LecRKs in other plant species.
Ligand identification
By forward genetic screen approach and transcriptomics, a few ligands of LecRKs have been identified. However, the highly variable lectin domains of LecRKs imply that a wide range of ligands have not been identified in plants. Self-molecules, such as eATP, eNAD+ and lipids from pathogens are known ligands that can be recognized by LecRKs. The current challenge is that although LecRKs have sugar-binding residues, they are poorly conserved and unlikely to bind monosaccharide molecules (Bouwmeester and Govers, 2009). 3-OH-C10:0 is hydrophobic ligand and the main egg-derived elicitors which can activate the expression of PR1 in Arabidopsis are enriched in the fraction containing total egg lipids (Bruessow et al., 2010; Kutschera et al., 2019). This indicates that some LecRKs may recognize some hydrophobic compounds from insect egg. In addition, metabolomic analysis of apoplast wash fluid is a powerful tool for discovering novel ligands. Suspension cells can also be used to search for potential ligands after stress treatment. Some small signaling peptides have been identified from chitin-treated rice suspension cells combined with metabolomic analysis (Wang et al., 2020). Recently, glycan array assays have emerged as an excellent tool for high-throughput screening of the interaction between proteins and glycans (Malik et al., 2014; Nikiforova et al., 2022). By using of functionalized sugar nucleotide donors on glycan microarrays, a few plant glycosyltransferases have been characterized (Ruprecht et al., 2020). Moreover, nuclear magnetic resonance followed by computational modeling to study the 3D structure of LecRKs and glycans is another ascendant method to mine novel ligands. The newly developed RLP/KSeq technology is assisting us in rapidly identifying the novel immune receptors and helps to genetically map the genes responsible for ligand- triggered immune responses in plants (Lin et al., 2020).
Signal transduction
As a kind of RLK protein kinase, autophosphorylation and transphosphorylation play important roles in LecRKs. At present, some LecRKs have been proven to function in autophosphorylation and some substrates have been identified. However, compared to the large number of LecRKs, these results only show the tip of the iceberg. Future researches should continue to focus on the identification of downstream substrates of LecRKs. Yeast two-hybrid and co-immunoprecipitation combined with MS assays are frequently-used means to identify the protein-protein interactions (PPIs). In addition, the recently developed proximity labeling (PL) approach has been used to screen PPIs. PL can detect weak, transient, hydrophobic, low-abundance and membrane-localized PPIs by exploring an unprecedented spatial and temporal protein interaction network in their native state (Yang et al., 2021). The application of PL methods may help to identify more interacting proteins of LecRKs in the future.
Applications in crop improvement
LysM-RLKs control different stages of symbioses with beneficial mycorrhizal fungi and nitrogen-fixing bacteria (Chiu and Paszkowski, 2020). In addition to LysM-RLKs, a recent report has shown that G-type LecRK mediates the symbiotic interaction between Populus and the ectomycorrhizal fungus Laccaria bicolor. Moreover, genetic transformation of PtLecRLK1 in Arabidopsis (Labbé et al., 2019) and switchgrass (Qiao et al., 2021; Shrestha et al., 2023) facilitated the colonization of L. bicolor with non-host plants. This work implied that LecRKs can be used to promote plant-microbe symbioses in crops. In addition to symbioses, LecRKs are also widely involved in resistance to pathogens and insects. Different LecRKs of different species might sense different ligands from multitudinous pathogens. Thus, transferring AtLORE to crops, such as rice, might enable the engineering of resistance traits to bacteria, such as Xanthomonas species, or constructing transgenic plants with chimeric LecRKs will achieve a potentially important step toward generating pathogen resistant crops (He et al., 2019). Meanwhile, LjLecRK of Lotus japonicas, plays important roles in freezing tolerance (Mustamin et al., 2023). SIT1 mediates ethylene production and salt-induced ethylene signaling (Li et al., 2014). These LecRKs can be used for engineering cold and salt-tolerant crops in the future.
Author contributions
LL and NX drafted the manuscript. JL revised the manuscript. All authors contributed to the article and approved the submitted version.
Acknowledgments
We apologize to the authors whose contributions could not be directly cited due to limited space. This work is supported by the National Natural Science Foundation of China (32270297).
Conflict of interest
The authors declare that the research was conducted in the absence of any commercial or financial relationships that could be construed as a potential conflict of interest.
Publisher’s note
All claims expressed in this article are solely those of the authors and do not necessarily represent those of their affiliated organizations, or those of the publisher, the editors and the reviewers. Any product that may be evaluated in this article, or claim that may be made by its manufacturer, is not guaranteed or endorsed by the publisher.
Supplementary material
The Supplementary Material for this article can be found online at: https://www.frontiersin.org/articles/10.3389/fpls.2023.1201805/full#supplementary-material
References
Abbracchio, M. P., Burnstock, G., Boeynaems, J. M., Barnard, E. A., Boyer, J. L., Kennedy, C., et al. (2006). International union of pharmacology LVIII: update on the P2Y G protein-coupled nucleotide receptors: from molecular mechanisms and pathophysiology to therapy. Pharmacol. Rev. 58, 281–341. doi: 10.1124/pr.58.3.3
Arnaud, D., Desclos-Theveniau, M., Zimmerli, L. (2012). Disease resistance to pectobacterium carotovorum is negatively modulated by the arabidopsis lectin receptor kinase LecRK-V.5. Plant Signal Behav. 7, 1070–1072. doi: 10.4161/psb.21013
Balagué, C., Gouget, A., Bouchez, O., Souriac, C., Haget, N., Boutet-Mercey, S., et al. (2017). The arabidopsis thaliana lectin receptor kinase LecRK-I.9 is required for full resistance to pseudomonas syringae and affects jasmonate signalling. Mol. Plant Pathol. 18, 937–948. doi: 10.1111/mpp.12457
Bassetti, N., Caarls, L., Bukovinszkine'kiss, G., El-Soda, M., Van Veen, J., Bouwmeester, K., et al. (2022). Genetic analysis reveals three novel QTLs underpinning a butterfly egg-induced hypersensitive response-like cell death in brassica rapa. BMC Plant Biol. 22, 140. doi: 10.1186/s12870-022-03522-y
Batistic, O. (2012). Genomics and localization of the arabidopsis DHHC-cysteine-rich domain s-acyltransferase protein family. Plant Physiol. 160, 1597–1612. doi: 10.1104/pp.112.203968
Bellande, K., Bono, J. J., Savelli, B., Jamet, E., Canut, H. (2017). Plant lectins and lectin receptor-like kinases: how do they sense the outside? Int. J. Mol. Sci. 18, 1164. doi: 10.3390/ijms18061164
Billington, R. A., Bruzzone, S., De Flora, A., Genazzani, A. A., Koch-Nolte, F., Ziegler, M., et al. (2006). Emerging functions of extracellular pyridine nucleotides. Mol. Med. 12, 324–327. doi: 10.2119/2006-00075.Billington
Bouwmeester, K., Govers, F. (2009). Arabidopsis l-type lectin receptor kinases: phylogeny, classification, and expression profiles. J. Exp. Bot. 60, 4383–4396. doi: 10.1093/jxb/erp277
Bouwmeester, K., Han, M., Blanco-Portales, R., Song, W., Weide, R., Guo, L. Y., et al. (2014). The arabidopsis lectin receptor kinase LecRK-I.9 enhances resistance to phytophthora infestans in solanaceous plants. Plant Biotechnol. J. 12, 10–16. doi: 10.1111/pbi.12111
Bruessow, F., Gouhier-Darimont, C., Buchala, A., Metraux, J. P., Reymond, P. (2010). Insect eggs suppress plant defence against chewing herbivores. Plant J. 62, 876–885. doi: 10.1111/j.1365-313X.2010.04200.x
Chen, D., Cao, Y., Li, H., Kim, D., Ahsan, N., Thelen, J., et al. (2017). Extracellular ATP elicits DORN1-mediated RBOHD phosphorylation to regulate stomatal aperture. Nat. Commun. 8, 2265. doi: 10.1038/s41467-017-02340-3
Chen, D., Hao, F., Mu, H., Ahsan, N., Thelen, J. J., Stacey, G. (2021). S-acylation of P2K1 mediates extracellular ATP-induced immune signaling in arabidopsis. Nat. Commun. 12, 2750. doi: 10.1038/s41467-021-22854-1
Chen, X., Shang, J., Chen, D., Lei, C., Zou, Y., Zhai, W., et al. (2006). A b-lectin receptor kinase gene conferring rice blast resistance. Plant J. 46, 794–804. doi: 10.1111/j.1365-313X.2006.02739.x
Cheng, X., Wu, Y., Guo, J., Du, B., Chen, R., Zhu, L., et al. (2013). A rice lectin receptor-like kinase that is involved in innate immune responses also contributes to seed germination. Plant J. 76, 687–698. doi: 10.1111/tpj.12328
Chiu, C. H., Paszkowski, U. (2020). Receptor-like kinases sustain symbiotic scrutiny. Plant Physiol. 182, 1597–1612. doi: 10.1104/pp.19.01341
Cho, S. H., Nguyen, C. T., Choi, J., Stacey, G. (2017). Molecular mechanism of plant recognition of extracellular ATP. Adv. Exp. Med. Biol. 1051, 233–253. doi: 10.1007/5584_2017_110
Choi, J., Tanaka, K., Cao, Y., Qi, Y., Qiu, J., Liang, Y., et al. (2014a). Identification of a plant receptor for extracellular ATP. Science 343, 290–294. doi: 10.1126/science.343.6168.290
Choi, J., Tanaka, K., Liang, Y., Cao, Y., Lee, S. Y., Stacey, G. (2014b). Extracellular ATP, a danger signal, is recognized by DORN1in arabidopsis. Biochem. J. 463, 429–437. doi: 10.1042/BJ20140666
Couto, D., Zipfel, C. (2016). Regulation of pattern recognition receptor signalling in plants. Nat. Rev. Immunol. 16, 537–552. doi: 10.1038/nri.2016.77
Dangl, J. L., Horvath, D. M., Staskawicz, B. J. (2013). Pivoting the plant immune system from dissection to deployment. Science 341, 746–751. doi: 10.1126/science.1236011
Dark, A., Demidchik, V., Richards, S. L., Shabala, S., Davies, J. M. (2011). Release of extracellular purines from plant roots and effect on ion fluxes. Plant Signal Behav. 6, 1855–1857. doi: 10.4161/psb.6.11.17014
Demidchik, V., Nichols, C., Oliynyk, M., Dark, A., Glover, B. J., Davies, J. M. (2003). Is ATP a signaling agent in plants? Plant Physiol. 133, 456–461. doi: 10.1104/pp.103.024091
Deng, K., Wang, Q., Zeng, J., Guo, X., Zhao, X., Tang, D., et al. (2009). A lectin receptor kinase positively regulates ABA response during seed germination and is involved in salt and osmotic stress response. J. Plant Biol. 52, 493–500. doi: 10.1007/s12374-009-9063-5
Desaki, Y., Miya, A., Venkatesh, B., Tsuyumu, S., Yamane, H., Kaku, H., et al. (2006). Bacterial lipopolysaccharides induce defense responses associated with programmed cell death in rice cells. Plant Cell Physiol. 47, 1530–1540. doi: 10.1093/pcp/pcl019
Desclos-Theveniau, M., Arnaud, D., Huang, T. Y., Lin, G. J., Chen, W. Y., Lin, Y. C., et al. (2012). The arabidopsis lectin receptor kinase LecRK-V.5 represses stomatal immunity induced by pseudomonas syringae pv. tomato DC3000. PloS Pathog. 8, e1002513. doi: 10.1371/journal.ppat.1002513
Djami-Tchatchou, A. T., Dubery, I. A. (2019). miR393 regulation of lectin receptor-like kinases associated with LPS perception in arabidopsis thaliana. Biochem. Biophys. Res. Commun. 513, 88–92. doi: 10.1016/j.bbrc.2019.03.170
Feng, H., Guan, D., Bai, J., Sun, K., Jia, L. (2015). Extracellular ATP: a potential regulator of plant cell death. Mol. Plant Pathol. 16, 633–639. doi: 10.1111/mpp.12219
Fountain, S. J., Cao, L., Young, M. T., North, R. A. (2008). Permeation properties of a P2X receptor in the green algae ostreococcus tauri. J. Biol. Chem. 283, 15122–15126. doi: 10.1074/jbc.M801512200
Gouhier-Darimont, C., Stahl, E., Glauser, G., Reymond, P. (2019). The arabidopsis lectin receptor kinase LecRK-I.8 is involved in insect egg perception. Front. Plant Sci. 10, 623. doi: 10.3389/fpls.2019.00623
Groux, R., Stahl, E., Gouhier-Darimont, C., Kerdaffrec, E., Jimenez-Sandoval, P., Santiago, J., et al. (2021). Arabidopsis natural variation in insect egg-induced cell death reveals a role for LECTIN RECEPTOR KINASE-I.1. Plant Physiol. 185, 240–255. doi: 10.1093/plphys/kiaa022
Grubb, L. E., Derbyshire, P., Dunning, K. E., Zipfel, C., Menke, F. L. H., Monaghan, J. (2021). Large-Scale identification of ubiquitination sites on membrane-associated proteins in arabidopsis thaliana seedlings. Plant Physiol. 185, 1483–1488. doi: 10.1093/plphys/kiab023
Guo, L., Qi, Y., Mu, Y., Zhou, J., Lu, W., Tian, Z. (2022). Potato StLecRK-IV.1 negatively regulates late blight resistance by affecting the stability of a positive regulator StTET8. Horticulture Res. 9, uhac010. doi: 10.1093/hr/uhac010
Han, H., Mu, X., Wang, P., Wang, Z., Fu, H., Gao, Y. G., et al. (2021). Identification of LecRLK gene family in cerasus humilis through genomic-transcriptomic data mining and expression analyses. PloS One 16, e0254535. doi: 10.1371/journal.pone.0254535
He, J., Zhang, C., Dai, H., Liu, H., Zhang, X., Yang, J., et al. (2019). A LysM receptor heteromer mediates perception of arbuscular mycorrhizal symbiotic signal in rice. Mol. Plant 12, 1561–1576. doi: 10.1016/j.molp.2019.10.015
He, Z., Zou, T., Xiao, Q., Yuan, G., Liu, M., Tao, Y., et al. (2021). An l-type lectin receptor-like kinase promotes starch accumulation during rice pollen maturation. Development 148, dev196378. doi: 10.1242/dev.196378
Hemsley, P. A., Weimar, T., Lilley, K. S., Dupree, P., Grierson, C. S. (2013). A proteomic approach identifies many novel palmitoylated proteins in arabidopsis. New Phytol. 197, 805–814. doi: 10.1111/nph.12077
Hurst, C. H., Wright, K. M., Turnbull, D., Leslie, K., Jones, S., Hemsley, P. A. (2019). Juxta-membrane s-acylation of plant receptor-like kinases is likely fortuitous and does not necessarily impact upon function. Sci. Rep. 9, 12818. doi: 10.1038/s41598-019-49302-x
Jeong, S., Trotochaud, A. E., Clark, S. E. (1999). The arabidopsis CLAVATA2 gene encodes a receptor-like protein required for the stability of the CLAVATA1 receptor-like kinase. Plant Cell 11, 1925–1934. doi: 10.1105/tpc.11.10.1925
Jewell, J. B., Berim, A., Tripathi, D., Gleason, C., Olaya, C., Pappu, H. R., et al. (2022). Activation of indolic glucosinolate pathway by extracellular ATP in arabidopsis. Plant Physiol. 190, 1574–1578. doi: 10.1093/plphys/kiac393
Jones, J. D., Dangl, J. L. (2006). The plant immune system. Nature 444, 323–329. doi: 10.1038/nature05286
Kagan, J. C. (2017). Lipopolysaccharide detection across the kingdoms of life. Trends Immunol. 38, 696–704. doi: 10.1016/j.it.2017.05.001
Kayagaki, N., Warming, S., Lamkanfi, M., Vande Walle, L., Louie, S., Dong, J., et al. (2011). Non-canonical inflammasome activation targets caspase-11. Nature 479, 117–121. doi: 10.1038/nature10558
Khakh, B. S., Burnstock, G. (2009). The double life of ATP. Sci. Am. 301, 84–90,92. doi: 10.1038/scientificamerican1209-84
Khakh, B. S., North, R. A. (2006). P2X receptors as cell-surface ATP sensors in health and disease. Nature 442, 527–532. doi: 10.1038/nature04886
Kim, D., Chen, D., Ahsan, N., Jorge, G. L., Thelen, J. J., Stacey, G. (2023). The raf-like MAPKKK INTEGRIN-LINKED KINASE 5 regulates purinergic receptor-mediated innate immunity in arabidopsis. Plant Cell 35, 1572–1592. doi: 10.1093/plcell/koad029
Kim, S. Y., Sivaguru, M., Stacey, G. (2006). Extracellular ATP in plants. visualization, localization, and analysis of physiological significance in growth and signaling. Plant Physiol. 142, 984–992. doi: 10.1104/pp.106.085670
Kutschera, A., Dawid, C., Gisch, N., Schmid, C., Raasch, L., Gerster, T., et al. (2019). Bacterial medium-chain 3-hydroxy fatty acid metabolites trigger immunity in arabidopsis plants. Science 364, 178–181. doi: 10.1126/science.aau1279
Labbé, J., Muchero, W., Czarnecki, O., Wang, J., Wang, X., Bryan, A. C., et al. (2019). Mediation of plant-mycorrhizal interaction by a lectin receptor-like kinase. Nat. Plants 5, 676–680. doi: 10.1038/s41477-019-0469-x
Li, C. H., Wang, G., Zhao, J. L., Zhang, L. Q., Ai, L. F., Han, Y. F., et al. (2014). The receptor-like kinase SIT1 mediates salt sensitivity by activating MAPK3/6 and regulating ethylene homeostasis in rice. Plant Cell 26, 2538–2553. doi: 10.1105/tpc.114.125187
Li, J., Wen, J., Lease, K. A., Doke, J. T., Tax, F. E., Walker, J. C. (2002). BAK1, an arabidopsis LRR receptor-like protein kinase, interacts with BRI1 and modulates brassinosteroid signaling. Cell 110, 213–222. doi: 10.1016/S0092-8674(02)00812-7
Liang, X., Zhou, J. M. (2018). Receptor-like cytoplasmic kinases: central players in plant receptor kinase-mediated signaling. Annu. Rev. Plant Biol. 69, 267–299. doi: 10.1146/annurev-arplant-042817-040540
Lin, X., Armstrong, M., Baker, K., Wouters, D., Visser, R. G. F., Wolters, P. J., et al. (2020). RLP/K enrichment sequencing; a novel method to identify receptor-like protein (RLP) and receptor-like kinase (RLK) genes. New Phytol. 227, 1264–1276. doi: 10.1111/nph.16608
Liu, P. L., Huang, Y., Shi, P. H., Yu, M., Xie, J. B., Xie, L. (2018b). Duplication and diversification of lectin receptor-like kinases (LecRLK) genes in soybean. Sci. Rep. 8, 5861. doi: 10.1038/s41598-018-24266-6
Liu, J., Liu, B., Chen, S., Gong, B. Q., Chen, L., Zhou, Q., et al. (2018a). A tyrosine phosphorylation cycle regulates fungal activation of a plant receptor Ser/Thr kinase. Cell Host Microbe 23, 241–253.e246. doi: 10.1016/j.chom.2017.12.005
Liu, S., Wang, J., Chen, K., Zhang, Z., Zhang, P. (2016). The l-type lectin receptor-like kinase (PnLecRLK1) from the Antarctic moss pohlia nutans enhances chilling-stress tolerance and abscisic acid sensitivity in arabidopsis. Plant Growth Regul. 81, 409–418. doi: 10.1007/s10725-016-0217-4
Liu, X., Wu, J., Clark, G., Lundy, S., Lim, M., Arnold, D., et al. (2012). Role for apyrases in polar auxin transport in arabidopsis. Plant Physiol. 160, 1985–1995. doi: 10.1104/pp.112.202887
Lu, D., Lin, W., Gao, X., Wu, S., Cheng, C., Avila, J., et al. (2011). Direct ubiquitination of pattern recognition receptor FLS2 attenuates plant innate immunity. Science 332, 1439–1442. doi: 10.1126/science.1204903
Luo, X., Wu, W., Liang, Y., Xu, N., Wang, Z., Zou, H., et al. (2020). Tyrosine phosphorylation of the lectin receptor-like kinase LORE regulates plant immunity. EMBO J. 39, e102856. doi: 10.15252/embj.2019102856
Luo, X., Xu, N., Huang, J., Gao, F., Zou, H., Boudsocq, M., et al. (2017). A lectin receptor-like kinase mediates pattern-triggered salicylic acid signaling. Plant Physiol. 174, 2501–2514. doi: 10.1104/pp.17.00404
Lustig, K. D., Shiau, A. K., Brake, A. J., Julius, D. (1993). Expression cloning of an ATP receptor from mouse neuroblastoma cells. Proc. Natl. Acad. Sci. U.S.A. 90, 5113–5117. doi: 10.1073/pnas.90.11.5113
Macho, A. P., Schwessinger, B., Ntoukakis, V., Brutus, A., Segonzac, C., Roy, S., et al. (2014). A bacterial tyrosine phosphatase inhibits plant pattern recognition receptor activation. Science 343, 1509–1512. doi: 10.1126/science.1248849
Malik, A., Lee, J., Lee, J. (2014). Community-based network study of protein-carbohydrate interactions in plant lectins using glycan array data. PloS One 9, e95480. doi: 10.1371/journal.pone.0095480
Micol-Ponce, R., Garcia-Alcazar, M., Lebron, R., Capel, C., Pineda, B., Garcia-Sogo, B., et al. (2023). Tomato POLLEN DEFICIENT 2 encodes a G-type lectin receptor kinase required for viable pollen grain formation. J. Exp. Bot. 74, 178–193. doi: 10.1093/jxb/erac419
Mithoe, S. C., Menke, F. L. (2018). Regulation of pattern recognition receptor signalling by phosphorylation and ubiquitination. Curr. Opin. Plant Biol. 45, 162–170. doi: 10.1016/j.pbi.2018.07.008
Monaghan, J., Matschi, S., Shorinola, O., Rovenich, H., Matei, A., Segonzac, C., et al. (2014). The calcium-dependent protein kinase CPK28 buffers plant immunity and regulates BIK1 turnover. Cell Host Microbe 16, 605–615. doi: 10.1016/j.chom.2014.10.007
Morillo, S. A., Tax, F. E. (2006). Functional analysis of receptor-like kinases in monocots and dicots. Curr. Opin. Plant Biol. 9, 460–469. doi: 10.1016/j.pbi.2006.07.009
Muchero, W., Sondreli, K. L., Chen, J. G., Urbanowicz, B. R., Zhang, J., Singan, V., et al. (2018). Association mapping, transcriptomics, and transient expression identify candidate genes mediating plant-pathogen interactions in a tree. Proc. Natl. Acad. Sci. U.S.A. 115, 11573–11578. doi: 10.1073/pnas.1804428115
Mustamin, Y., Akyol, T. Y., Gordon, M., Manggabarani, A. M., Isomura, Y., Kawamura, Y., et al. (2023). FER and LecRK show haplotype-dependent cold-responsiveness and mediate freezing tolerance in lotus japonicus. Plant Physiol. 191, 1138–1152. doi: 10.1093/plphys/kiac533
Navarro-Gochicoa, M. T., Camut, S., Timmers, A. C., Niebel, A., Herve, C., Boutet, E., et al. (2003). Characterization of four lectin-like receptor kinases expressed in roots of medicago truncatula. structure, location, regulation of expression, and potential role in the symbiosis with sinorhizobium meliloti. Plant Physiol. 133, 1893–1910. doi: 10.1104/pp.103.027680
Ngou, B. P. M., Ahn, H. K., Ding, P., Jones, J. D. G. (2021). Mutual potentiation of plant immunity by cell-surface and intracellular receptors. Nature 592, 110–115. doi: 10.1038/s41586-021-03315-7
Ngou, B. P. M., Ding, P., Jones, J. D. G. (2022). Thirty years of resistance: zig-zag through the plant immune system. Plant Cell 34, 1447–1478. doi: 10.1093/plcell/koac041
Nikiforova, A. V., Golovchenko, V. V., Mikshina, P. V., Patova, O. A., Gorshkova, T. A., Bovin, N. V., et al. (2022). Plant polysaccharide array for studying carbohydrate-binding proteins. Biochem. (Mosc) 87, 890–902. doi: 10.1134/S0006297922090036
Nishiguchi, M., Yoshida, K., Sumizono, T., Tazaki, K. (2002). A receptor-like protein kinase with a lectin-like domain from lombardy poplar: gene expression in response to wounding and characterization of phosphorylation activity. Mol. Genet. Genomics 267, 506–514. doi: 10.1007/s00438-002-0683-4
Park, B. S., Song, D. H., Kim, H. M., Choi, B. S., Lee, H., Lee, J. O. (2009). The structural basis of lipopolysaccharide recognition by the TLR4-MD-2 complex. Nature 458, 1191–1195. doi: 10.1038/nature07830
Peng, X., Wang, M., Li, Y., Yan, W., Chang, Z., Chen, Z., et al. (2020). Lectin receptor kinase OsLecRK-S.7 is required for pollen development and male fertility. J. Integr. Plant Biol. 62, 1227–1245. doi: 10.1111/jipb.12897
Perraki, A., Defalco, T. A., Derbyshire, P., Avila, J., Séré, D., Sklenar, J., et al. (2018). Phosphocode-dependent functional dichotomy of a common co-receptor in plant signalling. Nature 561, 248–252. doi: 10.1038/s41586-018-0471-x
Pétriacq, P., De Bont, L., Hager, J., Didierlaurent, L., Mauve, C., Guérard, F., et al. (2012). Inducible NAD overproduction in arabidopsis alters metabolic pools and gene expression correlated with increased salicylate content and resistance to pst-AvrRpm1. Plant J. 70, 650–665. doi: 10.1111/j.1365-313X.2012.04920.x
Pétriacq, P., Ton, J., Patrit, O., Tcherkez, G., Gakière, B. (2016). NAD acts as an integral regulator of multiple defense layers. Plant Physiol. 172, 1465–1479. doi: 10.1104/pp.16.00780
Pham, A. Q., Cho, S. H., Nguyen, C. T., Stacey, G. (2020). Arabidopsis lectin receptor kinase P2K2 is a second plant receptor for extracellular ATP and contributes to innate immunity. Plant Physiol. 183, 1364–1375. doi: 10.1104/pp.19.01265
Proietti, S., Giangrande, C., Amoresano, A., Pucci, P., Molinaro, A., Bertini, L., et al. (2014). Xanthomonas campestris lipooligosaccharides trigger innate immunity and oxidative burst in arabidopsis. Plant Physiol. Biochem. 85, 51–62. doi: 10.1016/j.plaphy.2014.10.011
Qiao, Z., Yates, T. B., Shrestha, H. K., Engle, N. L., Flanagan, A., Morrell-Falvey, J. L., et al. (2021). Towards engineering ectomycorrhization into switchgrass bioenergy crops via a lectin receptor-like kinase. Plant Biotechnol. J. 19, 2454–2468. doi: 10.1111/pbi.13671
Ralevic, V., Burnstock, G. (1998). Receptors for purines and pyrimidines. Pharmacol. Rev. 50, 413–492. doi: 10.1007/978-3-642-28863-0_5
Ramachandran, S. R., Kumar, S., Tanaka, K. (2019). Quantification of extracellular ATP in plant suspension cell cultures. Methods Mol. Biol. 1991, 43–54. doi: 10.1007/978-1-4939-9458-8_5
Ranf, S. (2016). Immune sensing of lipopolysaccharide in plants and animals: same but different. PloS Pathog. 12, e1005596. doi: 10.1371/journal.ppat.1005596
Ranf, S., Gisch, N., Schäffer, M., Illig, T., Westphal, L., Knirel, Y. A., et al. (2015). A lectin s-domain receptor kinase mediates lipopolysaccharide sensing in arabidopsis thaliana. Nat. Immunol. 16, 426–433. doi: 10.1038/ni.3124
Roux, S. J., Steinebrunner, I. (2007). Extracellular ATP: an unexpected role as a signaler in plants. Trends Plant Sci. 12, 522–527. doi: 10.1016/j.tplants.2007.09.003
Ruprecht, C., Bartetzko, M. P., Senf, D., Lakhina, A., Smith, P. J., Soto, M. J., et al. (2020). A glycan array-based assay for the identification and characterization of plant glycosyltransferases. Angew Chem. Int. Ed Engl. 59, 12493–12498. doi: 10.1002/anie.202003105
Saidou, M., Zhang, Z. (2022). The l-type lectin-like receptor kinase gene TaLecRK-IV.1 regulates the plant height in wheat. Int. J. Mol. Sci. 23, 8208. doi: 10.3390/ijms23158208
Schellenberger, R., Crouzet, J., Nickzad, A., Shu, L. J., Kutschera, A., Gerster, T., et al. (2021). Bacterial rhamnolipids and their 3-hydroxyalkanoate precursors activate arabidopsis innate immunity through two independent mechanisms. Proc. Natl. Acad. Sci. U.S.A. 118, e2101366118. doi: 10.1073/pnas.2101366118
Shah, N., Wakabayashi, T., Kawamura, Y., Skovbjerg, C. K., Wang, M. Z., Mustamin, Y., et al. (2020). Extreme genetic signatures of local adaptation during lotus japonicus colonization of Japan. Nat. Commun. 11, 253. doi: 10.1038/s41467-019-14213-y
Shang-Guan, K., Wang, M., Htwe, N., Li, P., Li, Y., Qi, F., et al. (2018). Lipopolysaccharides trigger two successive bursts of reactive oxygen species at distinct cellular locations. Plant Physiol. 176, 2543–2556. doi: 10.1104/pp.17.01637
Shi, J., Zhao, Y., Wang, Y., Gao, W., Ding, J., Li, P., et al. (2014). Inflammatory caspases are innate immune receptors for intracellular LPS. Nature 514, 187–192. doi: 10.1038/nature13683
Shrestha, H., Yao, T., Qiao, Z., Muchero, W., Hettich, R. L., Chen, J. G., et al. (2023). Lectin receptor-like kinase signaling during engineered ectomycorrhiza colonization. Cells 12, 1082. doi: 10.3390/cells12071082
Shumayla, S. S., Pandey, A. K., Singh, K., Upadhyay, S. K. (2016). Molecular characterization and global expression analysis of lectin receptor kinases in bread wheat (Triticum aestivum). PloS One 11, e0153925. doi: 10.1371/journal.pone.0153925
Singh, P., Chien, C. C., Mishra, S., Tsai, C. H., Zimmerli, L. (2013). The arabidopsis LECTIN RECEPTOR KINASE-VI.2 is a functional protein kinase and is dispensable for basal resistance to botrytis cinerea. Plant Signal Behav. 8, e22611. doi: 10.4161/psb.22611
Singh, P., Kuo, Y. C., Mishra, S., Tsai, C. H., Chien, C. C., Chen, C. W., et al. (2012). The lectin receptor kinase-VI.2 is required for priming and positively regulates arabidopsis pattern-triggered immunity. Plant Cell 24, 1256–1270. doi: 10.1105/tpc.112.095778
Singh, P., Mishra, A. K., Singh, C. M. (2021). Genome-wide identification and characterization of lectin receptor-like kinase (LecRLK) genes in mungbean (Vigna radiata l. wilczek). J. Appl. Genet. 62, 223–234. doi: 10.1007/s13353-021-00613-8
Singh, P., Zimmerli, L. (2013). Lectin receptor kinases in plant innate immunity. Front. Plant Sci. 4, 124. doi: 10.3389/fpls.2013.00124
Snoeck, S., Guayazán-Palacios, N., Steinbrenner, A. D. (2022). Molecular tug-of-war: plant immune recognition of herbivory. Plant Cell 34, 1497–1513. doi: 10.1093/plcell/koac009
Song, C. J., Steinebrunner, I., Wang, X., Stout, S. C., Roux, S. J. (2006). Extracellular ATP induces the accumulation of superoxide via NADPH oxidases in arabidopsis. Plant Physiol. 140, 1222–1232. doi: 10.1104/pp.105.073072
Stegmann, M., Monaghan, J., Smakowska-Luzan, E., Rovenich, H., Lehner, A., Holton, N., et al. (2017). The receptor kinase FER is a RALF-regulated scaffold controlling plant immune signaling. Science 355, 287–289. doi: 10.1126/science.aal2541
Sun, A., Nie, S., Xing, D. (2012). Nitric oxide-mediated maintenance of redox homeostasis contributes to NPR1-dependent plant innate immunity triggered by lipopolysaccharides. Plant Physiol. 160, 1081–1096. doi: 10.1104/pp.112.201798
Sun, Y., Qiao, Z., Muchero, W., Chen, J. G. (2020). Lectin receptor-like kinases: the sensor and mediator at the plant cell surface. Front. Plant Sci. 11, 596301. doi: 10.3389/fpls.2020.596301
Sun, Y., Zhao, X., Gao, Y., Jiao, J., Sun, Y., Zhu, D., et al. (2022). Genome-wide analysis of lectin receptor-like kinases (LecRLKs) in sweet cherry (Prunus avium) and reveals PaLectinL16 enhances sweet cherry resistance with salt stress. Environ. Exp. Bot. 194, 104751. doi: 10.1016/j.envexpbot.2021.104751
Tanaka, K., Gilroy, S., Jones, A. M., Stacey, G. (2010). Extracellular ATP signaling in plants. Trends Cell Biol. 20, 601–608. doi: 10.1016/j.tcb.2010.07.005
Tanaka, K., Heil, M. (2021). Damage-associated molecular patterns (DAMPs) in plant innate immunity: applying the danger model and evolutionary perspectives. Annu. Rev. Phytopathol. 59, 53–75. doi: 10.1146/annurev-phyto-082718-100146
Tang, D., Wang, G., Zhou, J. M. (2017). Receptor kinases in plant-pathogen interactions: more than pattern recognition. Plant Cell 29, 618–637. doi: 10.1105/tpc.16.00891
Tian, H., Wu, Z., Chen, S., Ao, K., Huang, W., Yaghmaiean, H., et al. (2021). Activation of TIR signalling boosts pattern-triggered immunity. Nature 598, 500–503. doi: 10.1038/s41586-021-03987-1
Tsukamoto, H., Takeuchi, S., Kubota, K., Kobayashi, Y., Kozakai, S., Ukai, I., et al. (2018). Lipopolysaccharide (LPS)-binding protein stimulates CD14-dependent toll-like receptor 4 internalization and LPS-induced TBK1-IKKϵ-IRF3 axis activation. J. Biol. Chem. 293, 10186–10201. doi: 10.1074/jbc.M117.796631
Vaid, N., Macovei, A., Tuteja, N. (2013). Knights in action: lectin receptor-like kinases in plant development and stress responses. Mol. Plant 6, 1405–1418. doi: 10.1093/mp/sst033
Vaid, N., Pandey, P. K., Tuteja, N. (2012). Genome-wide analysis of lectin receptor-like kinase family from arabidopsis and rice. Plant Mol. Biol. 80, 365–388. doi: 10.1007/s11103-012-9952-8
Van Der Giet, M., Giebing, G., Tolle, M., Schmidt, S. (2002). The role of P2Y receptors in the control of blood pressure. Drug News Perspect. 15, 640–646. doi: 10.1358/dnp.2002.15.10.740237
Walker, J. C. (1994). Structure and function of the receptor-like protein kinases of higher plants. Plant Mol. Biol. 26, 1599–1609. doi: 10.1007/BF00016492
Wang, Y., Bouwmeester, K., Beseh, P., Shan, W., Govers, F. (2014). Phenotypic analyses of arabidopsis T-DNA insertion lines and expression profiling reveal that multiple l-type lectin receptor kinases are involved in plant immunity. Mol. Plant Microbe Interact. 27, 1390–1402. doi: 10.1094/MPMI-06-14-0191-R
Wang, Z., Cheng, J., Fan, A., Zhao, J., Yu, Z., Li, Y., et al. (2018). LecRK-V, an l-type lectin receptor kinase in haynaldia villosa, plays positive role in resistance to wheat powdery mildew. Plant Biotechnol. J. 16, 50–62. doi: 10.1111/pbi.12748
Wang, Y., Cordewener, J. H., America, A. H., Shan, W., Bouwmeester, K., Govers, F. (2015b). Arabidopsis lectin receptor kinases LecRK-IX.1 and LecRK-IX.2 are functional analogs in regulating phytophthora resistance and plant cell death. Mol. Plant Microbe Interact. 28, 1032–1048. doi: 10.1094/MPMI-02-15-0025-R
Wang, C., Huang, X., Li, Q., Zhang, Y., Li, J. L., Mou, Z. (2019). Extracellular pyridine nucleotides trigger plant systemic immunity through a lectin receptor kinase/BAK1 complex. Nat. Commun. 10, 4810. doi: 10.1038/s41467-019-12781-7
Wang, K., Li, S., Chen, L., Tian, H., Chen, C., Fu, Y., et al. (2023). E3 ubiquitin ligase OsPIE3 destabilises the b-lectin receptor-like kinase PID2 to control blast disease resistance in rice. New Phytol. 237, 1826–1842. doi: 10.1111/nph.18637
Wang, J., Qu, B., Dou, S., Li, L., Yin, D., Pang, Z., et al. (2015a). The E3 ligase OsPUB15 interacts with the receptor-like kinase PID2 and regulates plant cell death and innate immunity. BMC Plant Biol. 15, 49. doi: 10.1186/s12870-015-0442-4
Wang, Z., Ren, H., Xu, F., Lu, G., Cheng, W., Que, Y., et al. (2021). Genome-wide characterization of lectin receptor kinases in saccharum spontaneum l. and their responses to stagonospora tainanensis infection. Plants (Basel) 10, 322. doi: 10.3390/plants10020322
Wang, Y., Weide, R., Govers, F., Bouwmeester, K. (2015c). L-type lectin receptor kinases in nicotiana benthamiana and tomato and their role in phytophthora resistance. J. Exp. Bot. 66, 6731–6743. doi: 10.1093/jxb/erv379
Wang, P., Yao, S., Kosami, K. I., Guo, T., Li, J., Zhang, Y., et al. (2020). Identification of endogenous small peptides involved in rice immunity through transcriptomics- and proteomics-based screening. Plant Biotechnol. J. 18, 415–428. doi: 10.1111/pbi.13208
Wang, C., Zhou, M., Zhang, X., Yao, J., Zhang, Y., Mou, Z. (2017). A lectin receptor kinase as a potential sensor for extracellular nicotinamide adenine dinucleotide in arabidopsis thaliana. Elife 6, e25474. doi: 10.7554/eLife.25474.031
Webb, T. E., Simon, J., Krishek, B. J., Bateson, A. N., Smart, T. G., King, B. F., et al. (1993). Cloning and functional expression of a brain G-protein-coupled ATP receptor. FEBS Lett. 324, 219–225. doi: 10.1016/0014-5793(93)81397-I
Xiao, W., Hu, S., Zou, X., Cai, R., Liao, R., Lin, X., et al. (2021). Lectin receptor-like kinase LecRK-VIII.2 is a missing link in MAPK signaling-mediated yield control. Plant Physiol. 187, 303–320. doi: 10.1093/plphys/kiab241
Xu, N., Luo, X., Wu, W., Xing, Y., Liang, Y., Liu, Y., et al. (2020). A plant lectin receptor-like kinase phosphorylates the bacterial effector AvrPtoB to dampen its virulence in arabidopsis. Mol. Plant 13, 1499–1512. doi: 10.1016/j.molp.2020.09.016
Yang, Y., Labbe, J., Muchero, W., Yang, X., Jawdy, S. S., Kennedy, M., et al. (2016). Genome-wide analysis of lectin receptor-like kinases in populus. BMC Genomics 17, 699. doi: 10.1186/s12864-016-3026-2
Yang, X., Wen, Z., Zhang, D., Li, Z., Li, D., Nagalakshmi, U., et al. (2021). Proximity labeling: an emerging tool for probing in planta molecular interactions. Plant Commun. 2, 100137. doi: 10.1016/j.xplc.2020.100137
Yue, Z.-L., Tian, Z.-J., Zhang, J.-W., Zhang, S.-W., Li, Y.-D., Wu, Z.-M. (2022). Overexpression of lectin receptor-like kinase 1 in tomato confers resistance to fusarium oxysporum f. sp. radicis-lycopersici. Front. Plant Sci. 13. doi: 10.3389/fpls.2022.836269
Zeidler, D., Zähringer, U., Gerber, I., Dubery, I., Hartung, T., Bors, W., et al. (2004). Innate immunity in arabidopsis thaliana: lipopolysaccharides activate nitric oxide synthase (NOS) and induce defense genes. Proc. Natl. Acad. Sci. U.S.A. 101, 15811–15816. doi: 10.1073/pnas.0404536101
Zhang, W., Chen, Z., Kang, Y., Fan, Y., Liu, Y., Yang, X., et al. (2020a). Genome-wide analysis of lectin receptor-like kinases family from potato (Solanum tuberosum l.). PeerJ 8, e9310. doi: 10.7717/peerj.9310
Zhang, Y., Fang, Q., Zheng, J., Li, Z., Li, Y., Feng, Y., et al. (2022). GmLecRlk, a lectin receptor-like protein kinase, contributes to salt stress tolerance by regulating salt-responsive genes in soybean. Int. J. Mol. Sci. 23, 1030. doi: 10.3390/ijms23031030
Zhang, C., Li, J., Guo, X., Zhu, B., Xiao, W., Wang, P., et al. (2016). LecRK-VII.1, a lectin receptor-like kinase, mediates the regulation of salt stress and jasmonic acid response in arabidopsis. J. Plant Growth Regul. 36, 385–401. doi: 10.1007/s00344-016-9647-5
Zhang, X., Mou, Z. (2009). Extracellular pyridine nucleotides induce PR gene expression and disease resistance in arabidopsis. Plant J. 57, 302–312. doi: 10.1111/j.1365-313X.2008.03687.x
Zhang, X., Mou, Z. (2012). Expression of the human NAD(P)-metabolizing ectoenzyme CD38 compromises systemic acquired resistance in arabidopsis. Mol. Plant Microbe Interact. 25, 1209–1218. doi: 10.1094/MPMI-10-11-0278
Zhang, X., Zhao, G., Tan, Q., Yuan, H., Betts, N., Zhu, L., et al. (2020b). Rice pollen aperture formation is regulated by the interplay between OsINP1 and OsDAF1. Nat. Plants 6, 394–403. doi: 10.1038/s41477-020-0630-6
Zhao, W., Liu, Y.-W., Zhou, J.-M., Zhao, S.-P., Zhang, X.-H., Min, D.-H. (2016). Genome-wide analysis of the lectin receptor-like kinase family in foxtail millet (Setaria italica l.). Plant Cell Tissue Organ Culture (PCTOC) 127, 335–346. doi: 10.1007/s11240-016-1053-y
Keywords: plant, receptor-like kinases, lectin receptor-like kinases, ligand recognition, immunity
Citation: Liu L, Liu J and Xu N (2023) Ligand recognition and signal transduction by lectin receptor-like kinases in plant immunity. Front. Plant Sci. 14:1201805. doi: 10.3389/fpls.2023.1201805
Received: 07 April 2023; Accepted: 31 May 2023;
Published: 15 June 2023.
Edited by:
Yasuhiro Kadota, RIKEN Center for Sustainable Resource Science (CSRS), JapanReviewed by:
Yoshitake Desaki, Tokyo University of Science, JapanKiwamu Tanaka, Washington State University, United States
Jin-Gui Chen, Oak Ridge National Laboratory (DOE), United States
Copyright © 2023 Liu, Liu and Xu. This is an open-access article distributed under the terms of the Creative Commons Attribution License (CC BY). The use, distribution or reproduction in other forums is permitted, provided the original author(s) and the copyright owner(s) are credited and that the original publication in this journal is cited, in accordance with accepted academic practice. No use, distribution or reproduction is permitted which does not comply with these terms.
*Correspondence: Ning Xu, bmluZ3h1QGNhdS5lZHUuY24=