- 1Department of Agriculture, Faculty of Environmental Science, King Abdulaziz University, Jeddah, Saudi Arabia
- 2Department of Plant Pathology, Faculty of Agriculture, University of Assiut, Assiut, Egypt
- 3Department of Vegetable Crops, Faculty of Agriculture, Assiut University, Assiut, Egypt
- 4DARWIN21, Center for Desert Agriculture, Biological and Environmental Sciences & Engineering Division (BESE), King Abdullah University of Science and Technology (KAUST), Thuwal, Saudi Arabia
Introduction: Alternaria solani is a challenging pathogen in the tomato crop globally. Chemical control is a rapid approach, but emerging fungicide resistance has become a severe threat. The present study investigates the use of culture filtrates (CFs) of three species of Trichoderma spp. to control this disease.
Methods: Highly virulent A. solani strain and three Trichoderma fungal strains viz., T. harzianum (Accession No: MW590687), T. atroviride (Accession No: MW590689) and T. longibrachiatum (Accession No: MW590688) previously isolated by authors were used in this study. The efficacy of culture filtrates (CFs) to mitigate early blight disease were tested under greenhouse and field conditions, experiments were conducted in different seasons of 2020 using a tomato variety “doucen”.
Results and discussion: The CFs of T. harzianum, T. longibrachiatum, and T. atroviride significantly inhibited the in vitro mycelial growth of A. solani (62.5%, 48.73%, and 57.82%, respectively, followed by control 100%). In the GC–MS analysis of Trichoderma CF volatile compounds viz., harzianic acid (61.86%) in T. harzianum, linoleic acid (70.02%) in T. atroviride, and hydroxymethylfurfural (68.08%) in the CFs of T. longibrachiatum, were abundantly present. Foliar application of CFs in the greenhouse considerably reduced the disease severity (%) in all treatments, viz., T. harzianum (18.03%), T. longibrachiatum (31.91%), and T. atroviride (23.33%), followed by infected control (86.91%), and positively affected the plant biomarkers. In the greenhouse, the plants treated with CFs demonstrated higher flavonoids after 6 days of inoculation, whereas phenolic compounds increased after 2 days. The CF-treated plants demonstrated higher antioxidant enzymes, i.e., phenylalanine ammonia-lyase (PAL) and peroxidase (POD), after 4 days, whereas polyphenol oxidase (PPO) was higher after 6 days of inoculation, followed by healthy and infected controls. In open field conditions, disease severity in CF-treated plants was reduced in both seasons as compared to naturally infected plants, whereas CF-treated plants exhibited a higher fruit yield than controls. The present results conclude that CFs can be a potential biocontrol candidate and a promising alternative to the early blight pathogen for sustainable production.
1 Introduction
Tomato (Solanum lycopersicum L.) is a very significant crop that is widely grown worldwide, including Saudi Arabia (Imran et al., 2021), and is considered a major contributor to the fruit and vegetable diet of humans (Kapsiya et al., 2015). Tomato plants are vulnerable to various biotic factors, including viruses, nematodes, fungus, and bacteria (Imran et al., 2022a), under favorable growth conditions in this region, but the growth yield and production of tomatoes are mainly affected by fungal phytopathogens as various fungal diseases on plants have been reported during different growth stages, which leads to significant pre- and post-harvest yield losses (Gondal et al., 2012; Kolomiets et al., 2017; Tomazoni et al., 2017; González-Fernández et al., 2021; Abo-Elyousr et al., 2022). Among the fungal diseases of tomatoes, early blight disease caused by a pathogen called Alternaria solani is one of the most destructive diseases reported worldwide (Koley et al., 2015; Camlica and Tozlu, 2019; Mazrou et al., 2020; Zhang et al., 2020a; Imran et al., 2022b). Due to its adverse effects, this pathogen has drawn great attention over the years due to extensive yield losses in crops (Bauske et al., 2017). Various fungicides of different modes of action have been commonly used to control this disease, but in severe disease outbreaks, multiple applications of fungicides with a higher dose rate are required, which leads to the development of resistance in fungal pathogens (Pasche et al., 2004; Zhang et al., 2017; Zhang et al., 2020b) and ultimately may increase the toxicity of soil, which affects the microbiota population. The development of resistance in fungal pathogens is mainly associated with the detection of point mutations (Senthil et al., 2008; Edin et al., 2019) in the genetic material of fungal pathogens, which aids endurance (Sriwati et al., 2019; Wang W et al., 2021; Zhang et al., 2021). Except for emerging resistance, the multiple applications also pollute the environment, which has a negative impact on human health. Therefore, alternative approaches must be adopted to overcome the resistance problems in the pathogen of early blight disease in tomatoes.
Most recent studies reported the use of various endophytic microorganisms isolated from the rhizospheric zone of plants and screened for their inhibitory antagonistic potential against various fungal pathogens, including A. solani (Imran et al., 2022a; Imran et al., 2022b). Among the fungal bioagents, Trichoderma harzianum, T. atroviride, T. longibrachiatum, T. gamsii, and T. asperellum has been widely reported and used as the best biocontrol candidates for potential antagonistic activity against a variety of phytopathogens (Mazrou et al., 2020; Stracquadanio et al., 2020; Castro-Restrepo et al., 2022; Imran et al., 2022b). Trichoderma species as cell suspensions or culture filtrates (by acting as protective barriers) can effectively assist the plant to survive microbial competition as well as environmental stresses (Rahman et al., 2018). The results of a study by Alka et al. represent a significant in vitro and in vivo reduction of a fungal pathogen, Rhizopus oryzae, causing Rhizopus rot of tomatoes (Alka and Prajapati, 2017), when culture filtrates of Trichoderma species were applied. Additionally, the culture filtrates of various Trichoderma species as biofungicides against various fungal pathogens, viz., Colletotrichum gloeosporioides (Nurbailis et al., 2019), against anthracnose of great millet (Manzar et al., 2021), Pythium species, and Phytophthora species, demonstrated inordinate antifungal potential (Ben M'henni et al., 2022). The use of various Trichoderma species has widely been reported as an eco-friendly and safe approach to the control of plant diseases (Alka and Prajapati, 2017; Nurbailis et al., 2019; Manzar et al., 2022). The application of culture filtrates (CFs) of Trichoderma species as biocontrol not only acts as biostimulants against the inhibition of pathogens but also induces systemic or localized resistance in plants to biotic stresses and, ultimately, as a growth regulator, increases plant biomass (Abbas et al., 2019; Guzmán-Guzmán et al., 2019). The inhibition of fungal pathogens by Trichoderma species implicates various mechanisms, including specific metabolite and phenolic compound production, fibrolytic enzymes, various antimicrobial substances, direct parasitization, and competition for food by nutrients (Cavallo et al., 2020; Dini and Laneri, 2021; Dini et al., 2021a; Iannaccone et al., 2022). Moreover, Trichoderma species stimulate the production of phenolic compounds, which increase the nutraceutical value and defense system of plants (Cavallo et al., 2020; Dini and Laneri, 2021), causing the degradation of polysaccharides, chlorophenols, hydrocarbons, and xenobiotic pesticides (Zafra and Cortés-Espinosa, 2015; Dini et al., 2021b). During infection by a pathogen, Trichoderma species activate systemic resistance through multiple hormonal signaling pathways, which act as the primary barrier to the plant defense system (Mendoza-Mendoza et al., 2018). In the defense system of plants, the activation of defense enzymes, viz., polyphenol oxidase (PPO), peroxidase (POD), phenylalanine ammonia lyase (PAL), chitinase, β-1,3-glucanase, and various antioxidants such as catalase, flavonoids, and phenolics, has a significant role in inducing resistance (Anand et al., 2007; Almagro et al., 2009), along with microbial volatile elicitor compounds (Kashyap et al., 2022). Various studies reported a significant increase in the production of defense enzymes following the application of culture filtrates and/or cell suspensions of Trichoderma species to plants (Akram et al., 2021; Heflish et al., 2021; Mahmoud et al., 2021; Tripathi et al., 2021; Vukelić et al., 2021). Phenolic compounds are well known as they contain a wide group of chemicals (phenocarbonic acids, flavonoids, phenolic acids, lignans, polymeric lignans, and stilbenes) and, as plant growth regulators, modulate physiological processes such as vesicle trafficking, membrane permeability, signal transduction, and gene transcription in plants (Quideau et al., 2011; Babenko et al., 2019). Generally, phenylalanine ammonia lyase (PAL) has an imperative role in the biosynthesis of phenolic compounds as it catalyzes the non-oxidative eradication of the -NH2 group from L-phenylalanine (Phe) to construct trans-cinnamate, which is a starting molecule for the synthesis of other phenolic compounds (Pereira et al., 2009; Jun et al., 2018). While polyphenol oxidase (PPO) catalyzes the O2-dependent oxidation of ortho (o)-phenolics to o-quinones, which condensed the nutritive importance of protein (Constabel and Barbehenn, 2008). Peroxidase (POD) utilizes O2 or H2O2 to oxidize various molecules, which are used in diagnosis and immune assays (Yoshida et al., 2003). The induction of defense enzymes, antioxidants, phenols, and flavonoids is mainly responsible for the activation of the defense system in plants, which protects them from the infection of pathogens (Schulz-Bohm et al., 2017; Zehra et al., 2017; Kaur et al., 2022). Previously, the effect of foliar application of Trichoderma as cell suspension on disease severity and fruit yield under greenhouse and open field conditions was studied (Imran et al., 2022a; Imran et al., 2022b). Considering the emerging fungicide resistance risks and their impact on the environment, some eco-friendly approaches are needed to adopt for the control of early blight pathogen, and to the author’s knowledge, no study so far has been conducted to use the culture filtrates (CFs) of Trichoderma for the control of early blight disease in this region. Therefore, the aim of the present study was to investigate the effect of foliar application of Trichoderma culture filtrates (CFs) in greenhouses on disease severity and plant biomass; to study the effect of CFs on defense enzymes and antioxidant production in tomato plants; and to study the effect of CFs in open fields under natural infection conditions and their influence on fruit yield.
2 Materials and methods
2.1 Collection of the fungal pathogen, bioagents, and growth conditions
A highly virulent A. solani strain was previously isolated, screened, and identified by Imran et al. (2022b) was collected from the fungal stock culture of the Laboratory of Plant Pathology, Department of Agriculture, King Abdulaziz University, Jeddah, Saudi Arabia. The pathogenic strain was subcultured on a potato dextrose agar (PDA) medium plate at 27°C for 5–7 consecutive days, and the actively grown strain was preserved at 4°C for further use.
Three Trichoderma fungal strains, viz., T. harzianum (Accession No.: MW590687), T. atroviride (Accession No.: MW590689), and T. longibrachiatum (Accession No.: MW590688) previously isolated and identified by Imran et al. (2022a), were obtained from the fungal stock culture of the Laboratory of Plant Pathology, Department of Agriculture, King Abdulaziz University, Jeddah, Saudi Arabia. The Trichoderma strains were subsequently subcultured on PDA medium plates at 27°C for 3 days, and the active colony culture was preserved at 4°C.
2.2 In vitro assay
2.2.1 Preparation of culture filtrates and mycelial growth inhibition
Culture filtrates of Trichoderma strains were initially prepared by transferring 5-mm mycelial discs (3-day-old previously grown on PDA) to 50 ml sterile conical flasks containing 25 ml potato dextrose broth (PDA lacking agar). Mycelial discs of uniform diameter (8–10 discs/flask) were carefully placed (in floating positions) in PDB containing flasks that were subsequently incubated at 27°C in a shaker (125×g) for 7–10 days. The resulting suspension was centrifuged (10,000×g for 10 min at 4°C) and filtered through Whatman No. 1 filter paper (Sigma-Aldrich, USA), followed by a final filtration through a Manifolds vacuum filtration unit (MFA-1S SS316L, 100 µm; Bioevopeak, Shandong, China) for the purity of culture filtrates. The obtained supernatant (100% purity) was preserved at 4°C.
To assess in vitro mycelial growth inhibition, previously prepared culture filtrates (CFs) of Trichoderma strains were supplemented with PDA medium to evaluate their effect on A. solani. Briefly, PDA plates supplemented with culture filtrate (CF) suspension containing 20 ml of PDA/petri plate (1 ml of CF suspension was thoroughly mixed with 9 ml of PDA) were inoculated with a 5-mm mycelial disc (placed face-down in the middle) excised from the edge of a 5-day-old A. solani culture previously grown on PDA. Four replicates were used for each strain, whereas six plates were used as a replicate. The plates lacking culture filtrate suspension were used as a control. Plates were incubated at 27°C for 7–10 days until the completion of the control plate with the growth of the pathogen. Then, the colony diameter was measured on each plate and compared with the control.
2.3 Assessment and characterization of volatile compounds in fungal bioagents by gas chromatography–mass spectrometry analysis
2.3.1 Extraction of metabolites from culture filtrates
Metabolites from the culture filtrates were extracted with the method described by Stracquadanio et al. (2020). Briefly, the culture filtrates of Trichoderma strains were extracted three times by using ethyl acetate solvent (1:1). To dry the combined organic fraction, magnesium sulfate (MgSO4) was used and evaporated at 35°C under reduced pressure. The residues having a red-brown color were recovered and dissolved in 10% methanol (CH3OH) or dimethylsulfoxide (DMSO), and extracts were stored at −20°C to perform further analysis.
2.3.2 GC–MS analysis
The metabolites obtained from the culture filtrates of selected fungal bioagents were used to perform GC–MS analysis for the detection of active biomolecule components. The volatile compounds in fungal metabolites were identified with a single quadrupole mass spectrometer (GC–MS) detector (Stoppacher et al., 2010; Siddiquee et al., 2012). The identification of the volatile compounds in the culture filtrate of Trichoderma species was conducted by GC–MS analysis. This chemical identification of the compounds was determined by injecting the standard compounds into GC–MS or by comparison to library mass spectra from the NIST database. The energy for electron impact was 70eV, whereas the ion source temperature was adjusted to 250°C. The electron impact (EI) mass scan (m/s) range was 40–450 Da in fully scan acquisition mode (Hernádez-Rodrίguez et al., 2008; Khan et al., 2021). The spectra of the identified compounds were compared with the available spectra of compounds in the GC–MS of the National Institute of Standards and Technology (NIST) database. More than 90% resemblance was considered a threshold for detection.
2.4 In planta assay
2.4.1 Effect of culture filtrates on disease severity
To study the efficacy of culture filtrates (CFs) to mitigate early blight disease, experiments were conducted in different seasons of 2020 under the greenhouse using the tomato variety “doucen.” Briefly, tomato seedlings were grown in 18-cm plastic pots containing peat moss (1:3), and at three- to four-leaf stage, seedlings were transplanted to the new pots containing an identical amount of growth medium. Previously prepared culture filtrates (100%) of Trichoderma strains were used as foliar application after twelve days of transplanting (30 ml plant−1), whereas, after 24 h, the cell suspension of a virulent pathogenic strain (previously grown on PDA for 7 days adjusted with a hemocytometer) was sprayed (104 spores/ml; 30 ml plant−1). Plants sprayed with sterile distilled water were treated as healthy controls whereas plants inoculated with the suspension of pathogens were treated as infected controls. Plants were covered with sterile polythene bags for three days to retain humidity for the initiation of infection by pathogens. The humidity (75%–80%) and optimal temperature (27 ± 1°C) inside the greenhouse were maintained. The experiment was performed with six replicates for each treatment, and six plants were subjected to each replicate. The experiment was repeated twice, and disease severity was measured with a reported 0–5 disease rating scale as follows: 0—[no infection on leaves]; 1—[0%–5% infection on leaves]; 2—[6%–20% infection on leaves]; 3—[21%–40% infection on leaves]; 4—[41%–70% infection on leaves], and 5—[>70% infection on leaves] (Gondal et al., 2012). Disease severity (%) was recorded as: disease severity = Σ (no. of infected plants × no. scale)/total no. of plants × higher no. scale × 100 (Akhtar et al., 2016).
2.4.2 Effect of culture filtrates on plant biomass
The biomass of tomato plants, viz., plant height and fresh and dry weight of roots and shoots, were measured after the determination of disease severity. Plant height was calculated inside the greenhouse within the pots, and subsequently, plants were harvested to measure the fresh weight. Then, the plants were placed in a moisture dryer chamber at 60°C for 5 days to ensure the complete drying of the moisture contents. The dry weight of the plants was calculated, and the means were compared among the treatments.
2.5 Effect of culture filtrates on secondary metabolite production
2.5.1 Preparation of leaf aliquot and estimation of secondary metabolites
In the greenhouse experiment, leaf samples from each treatment were randomly collected from randomly selected replicates at 0, 2, 4, 6, and 8 days after inoculation. Samples were immersed in liquid nitrogen to obtain the fine powder that was stored at −80°C to measure the secondary metabolites. viz., total phenol and flavonoids contents.
2.5.1.1 Total phenol contents
Total phenol contents in the samples were assayed by using the Folin–Ciocalteu reagent with the method of Meda et al. (2005) with trivial modifications. Briefly, 1 g of powdered sample was dissolved in 5 ml of methanol (80%). The aliquot was centrifuged at 10,000 rpm for 10 min at 4°C. The supernatant was transferred to new 2 ml tubes, followed by storage at −80°C before further analysis. Subsequently, 100 µl of the methanol-extracted sample was assorted with 750 µl of 1 N Folin–Ciocolteu reagent (1:10), followed by incubation for 5 min at room temperature. Thereafter, 60 µl of sodium carbonate (Na2CO3) (7.5%) was added, and the mixture was incubated for 30 min at room temperature. The absorbance of the mixture was recorded at 750 nm using a UV–Vis spectrophotometer. The phenolic contents in the reaction mixture were determined by using a standard curve obtained from gallic acid (0–5 mg) and expressed as mg/g plant material. Six replicates were used for each treatment.
2.5.1.2 Flavonoid contents
Flavonoid contents in the sample were determined according to the method of Chang et al. (2002) using an aluminum chloride (AlCl3) reagent. Briefly, 250 μl of the methanol-extracted samples were mixed into 75 μl sodium nitrite (5%) followed by the addition of 1,250 μl distilled H2O followed by incubation for 5 min at room temperature. Afterward, 150 μl aluminum chloride (10%), 500 μl sodium hydroxide (1M), and 275 μl distilled H2O were added to the reaction mixture. The mixture was incubated for 5 min at room temperature, and the absorbance was recorded at 510 nm and expressed as mg/g plant material. Six replicates were used for each treatment.
2.6 Antioxidant enzyme assay
2.6.1 Protein assays
The concentration of protein in leaf extract was determined by Bradford’s (1976) method using bovine serum albumin (BSA) as a standard. Protein contents were determined by a UV–Vis spectrophotometer (Thermo Fisher Scientific™) at an absorbance of 595 nm, and a standard curve was developed. The standard curve was used to determine secondary metabolites and antioxidants in leaf extract.
2.6.1.1 Preparation of crude enzyme
To prepare crude enzymes, previously ground (powder) leaf tissues were used to estimate antioxidant enzymes, viz., phenylalanine ammonia-lyase (PAL), peroxidase (POD), and polyphenol oxidase (PPO). Briefly, 500 mg of fine powder from each replicate was suspended in 2 ml of extraction buffer containing 20 ml of phenylmethyl sulfonyl fluoride (1 mM) and polyvinylpyrrolidone (0.1%). To determine PAL activity, sodium borate buffer (0.1 M, pH 8.7) was used, while for PO and PPO activity, sodium phosphate buffer (0.1 M, pH 7.0) was used. The extraction was conducted at 4°C, and samples were centrifuged at 12,000×g for 20 min at 4°C. The supernatant (the crude enzyme source) was transferred to a new centrifuge tube and preserved at −80°C to determine the enzymatic assay.
2.6.1.2 Phenylalanine ammonia-lyase assay
Phenylalanine ammonia-lyase (PAL) activity in treated tomato leaves was determined according to the method of Dickerson et al. (1984). Briefly, the reaction containing 200 µl of crude enzyme extract, 1,300 µl sodium borate buffer (0.1 M, pH 8.7) and 500 µl of L-phenyl alanine (12 mM) was incubated at 37°C in a water bath for 30 min. Subsequently, the PAL activity was determined at 290 nm absorbance with a UV–Vis spectrophotometer, whereas cinnamic acid (0–5 mg, nM) was used as a standard, and the PAL activity was expressed as min/g protein. Six replicates were used for each treatment.
2.6.1.3 Peroxidase assay
Peroxidase (POD) activity in treated tomato leaves was assayed using the method of Hemeda and Klein (1990). Briefly, the substrate was prepared by dissolving 5 ml of H2O2 (0.3%) in 5 ml of guaiacol (1%) and then adding 50 ml of sodium phosphate buffer (0.05 M, pH 6.5). The reaction mixture was prepared by suspending 1,475 µl of the substrate in 25 µl of crude enzyme extract. The change in absorption was recorded at the absorbance of 470 nm, and PO activity was calculated by the increase in absorbance caused by the oxidation of guaiacol. PO activity was expressed as µmol/min/mg of protein (E = 26.6/mM/cm).
2.6.1.4 Polyphenol oxidase assay
Polyphenol oxidase (PPO) activity was determined using the method of Kumar and Khan (1982). The reaction mixture containing 250 µl crude enzyme extract, 1,000 µl sodium phosphate buffer (0.1 M, pH 6.5), and 500 µl catechol (0.1 M) was incubated at room temperature for 10 min. Then, 500 µl of H2SO4 (2.5N) was added to stop the reaction. The purpurogallin-formed absorption was observed at an absorbance of 495 nm. While the initial addition of H2SO4 to the reaction mixture was used as a blank, PPO activity was calculated and expressed as U/min/mg protein (U = change in 0.1 absorbance/min/mg protein).
2.7 Open field trails
The efficiency of Trichoderma culture filtrates in two consecutive seasons [early (January–March) and late (September–December)] of 2020 was determined in open fields under natural infection conditions. The experimental site was prepared at the “Hada Al Sham” field station of King Abdulaziz University. In the early season, seedlings of the tomato variety “Doucen” were grown in a plastic seedling growing tray (50 holes) containing peat moss (1:3). At the three- to four-leaf stage, seedlings were transplanted in an agricultural field previously prepared (maintaining a 60-cm distance between rows and 45 cm within plants) (Supplementary Figure 1). Culture filtrates of fungal bioagents (previously prepared) were used as foliar applications. After one week of transplanting, the suspension of culture filtrates (100%) was applied as a foliar spray (50 ml/plant) to tomato plants. For healthy control, the plants were sprayed with sterile distilled water, whereas the untreated plants (lacking any of the treatment) were subjected to infected control. All treatments were applied in the evening, and plants were left for natural infection in an open field. Plants were irrigated properly as per requirements with a drip irrigation system, and standard agronomic practices were carried out throughout the experiment. For each treatment, including control, plants were randomly selected, and disease severity was recorded with a reported disease rating scale as previously mentioned (Gondal et al., 2012; Ismail et al., 2016). Fruit yield was recorded by harvesting the ripened fruit regularly from all replicates of all treatments, and the total yield for each treatment was calculated and compared.
The experiment was conducted in a completely randomized block design with six replicates, each containing nine plants. All recommended agronomic practices were adopted in the experimental field, and each treatment was applied randomly to plants. An experiment with identical parameters was performed in the late season. Disease severity and fruit yield in both seasons were recorded and compared with controls to observe the efficacy of culture filtrates against the natural infection of the early blight pathogen.
2.8 Statistical analysis
All in vitro experiments were conducted in triplicate, while field experiments were conducted in quadruplicate. Experiments were performed in a complete randomized design, and all collected data was analyzed by using Statistix 8.1 (Analytical Software, Statistix; Tallahassee, FL, USA, 1985–2003). The data from disease severity was transformed into arcsine values, and a one-way analysis of variance (ANOVA) was performed. The means of replicates in all treatments were compared using Fisher’s least significant difference test at p = 0.05 (Steel et al., 1996).
3 Results
3.1 In vitro mycelial growth inhibition by culture filtrates
The in vitro application of Trichoderma culture filtrates (CFs) demonstrated significant (p = 0.05) mycelial growth suppression of the A. solani pathogen. However, the CFs of T. harzianum suppressed (62.5%) mycelial growth as lower (31.5 mm) mycelial growth was recorded, followed by the control (84 mm). Meanwhile, mycelial growth inhibited by CFs of T. longibrachiatum and T. atroviride (48.7% and 57.8%, respectively) was relatively lower than that of T. harzianum followed by the control (Figure 1A). Apparently, the in vitro application of all Trichoderma CFs significantly altered the growth pattern of the A. solani colony, followed by the control (Figure 1B). The results herein presented indicate that even lower volumes (1 ml of CFs to 9 ml of PDA) of CFs of these Trichoderma strains showed better growth inhibition of the A. solani pathogen.
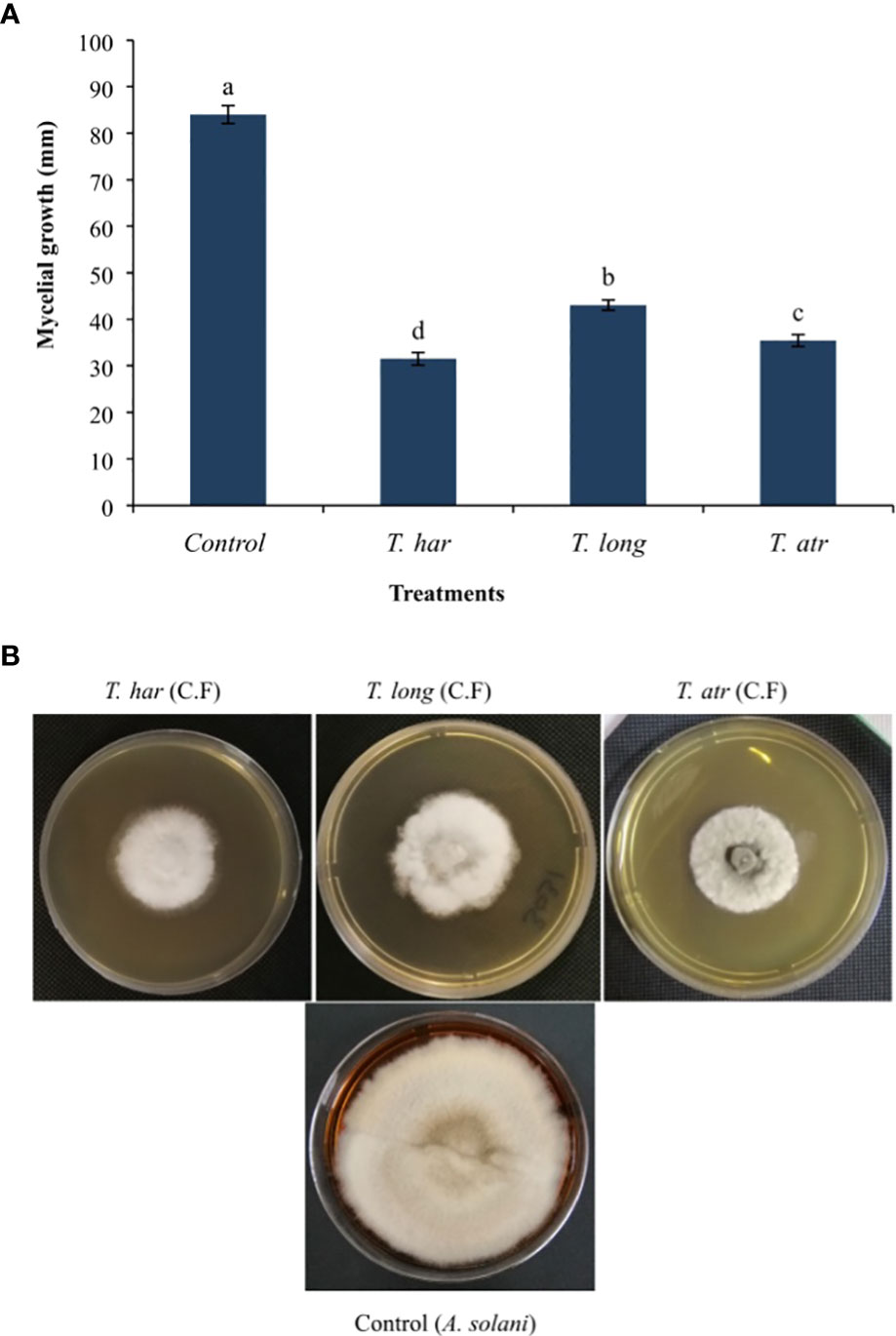
Figure 1 (A) In vitro effect of Trichoderma culture filtrates (CFs) on the mycelial growth of the A. solani pathogen. Values followed by different letters indicate that means are significantly different from each other according to Fisher’s least significant difference at p = 0.05. T. har, Trichoderma harzianum; T. long, Trichoderma longibrachiatum; T. atr, Trichoderma atroviride. (B) The in vitro effect of Trichoderma culture filtrates on mycelial growth inhibition of the A. solani pathogen on PDA medium.
3.2 GC–MS analysis of Trichoderma culture filtrates
The GC–MS chromatogram of T. harzianum demonstrated the presence of 10 peaks (Figure 2A) with 27 volatile compounds (Table 1A), whereas 25 volatile compounds (Table 1B) with eight peaks (Figure 2B) in T. atroviride and nine peaks (Figure 2C) with 32 volatile compounds (Table 1C) were detected in T. longibrachiatum.
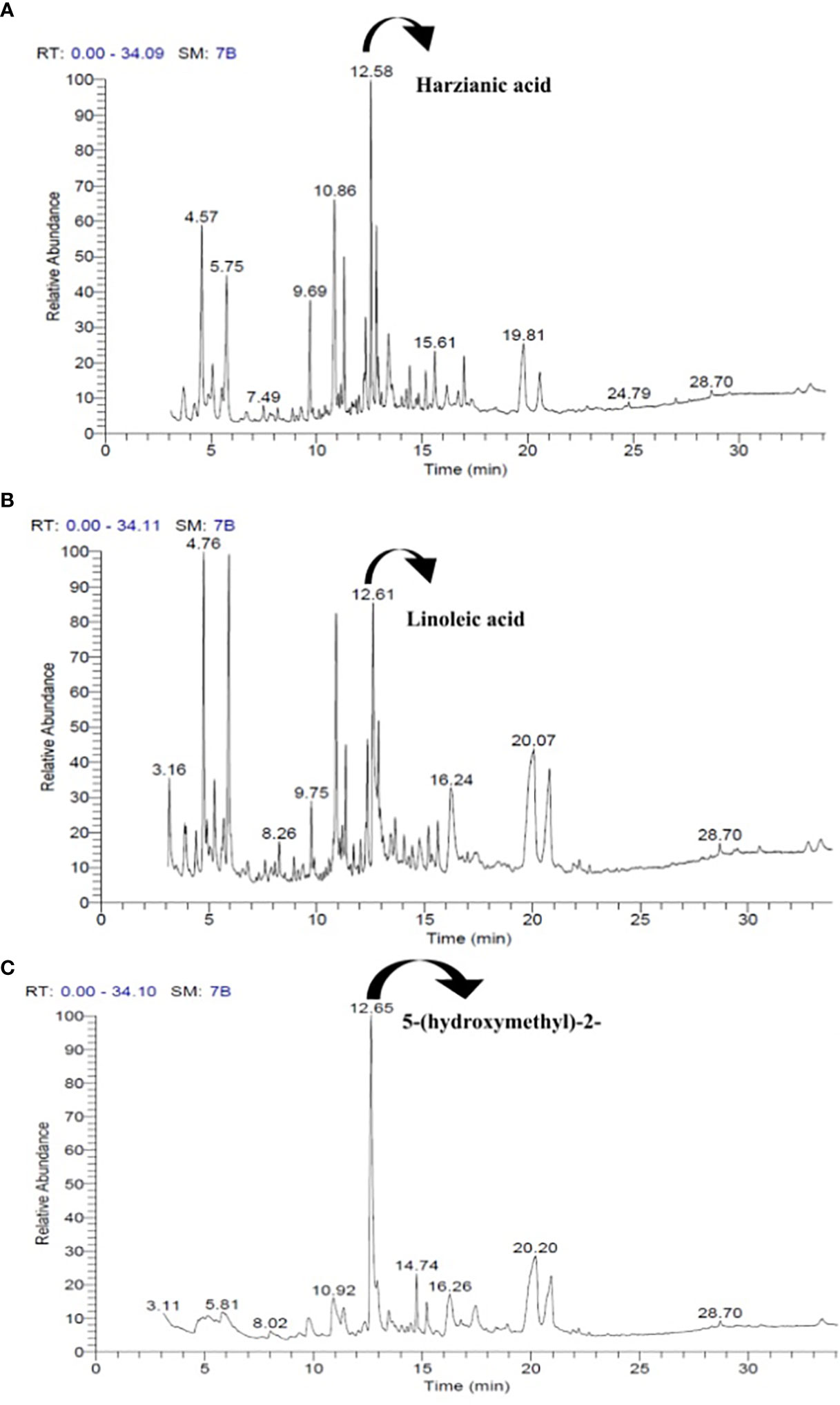
Figure 2 The standard GC–MS chromatograms of T. harzianum (A), T. atroviride (B), and T. longibrachiatum (C) strains and the peaks of volatile organic compounds (VOCs) detected from their culture filtrates.
In the CFs of T. harzianum, various groups of chemical compounds, viz., aldehydes, hydroxyls, different acids, glycerin, esters, and many hydroxyl groups containing compounds, were detected. The most abundant compound (61.86%) present in CFs of T. harzianum was harzianic acid (C19H27NO6), detected at a retention time (Rt) of 12.58 (Table 1A; Figure 2A). Glycerin was also observed in a higher amount (9.98%), followed by other compounds that ranged between 5% and 8%. The other prominent constituents in the CFs of T. harzianum were dl-glyceraldehyde (8.21%), 2-Propanone, 1,3-dihydroxy (6.23%), 3-Deoxy-d-mannoic lactone (5.34%), 1,2,3-Propanetriol, monoacetate (5.10%), 4H-Pyran-4-one,2,3-dihydro-3,5-dihydroxy-6-methyl- (4.94%), and D-Alanine, N-propargyloxycarbonyl-,isohexyl ester (4.41%), whereas other detected compounds were in lower amounts (%).
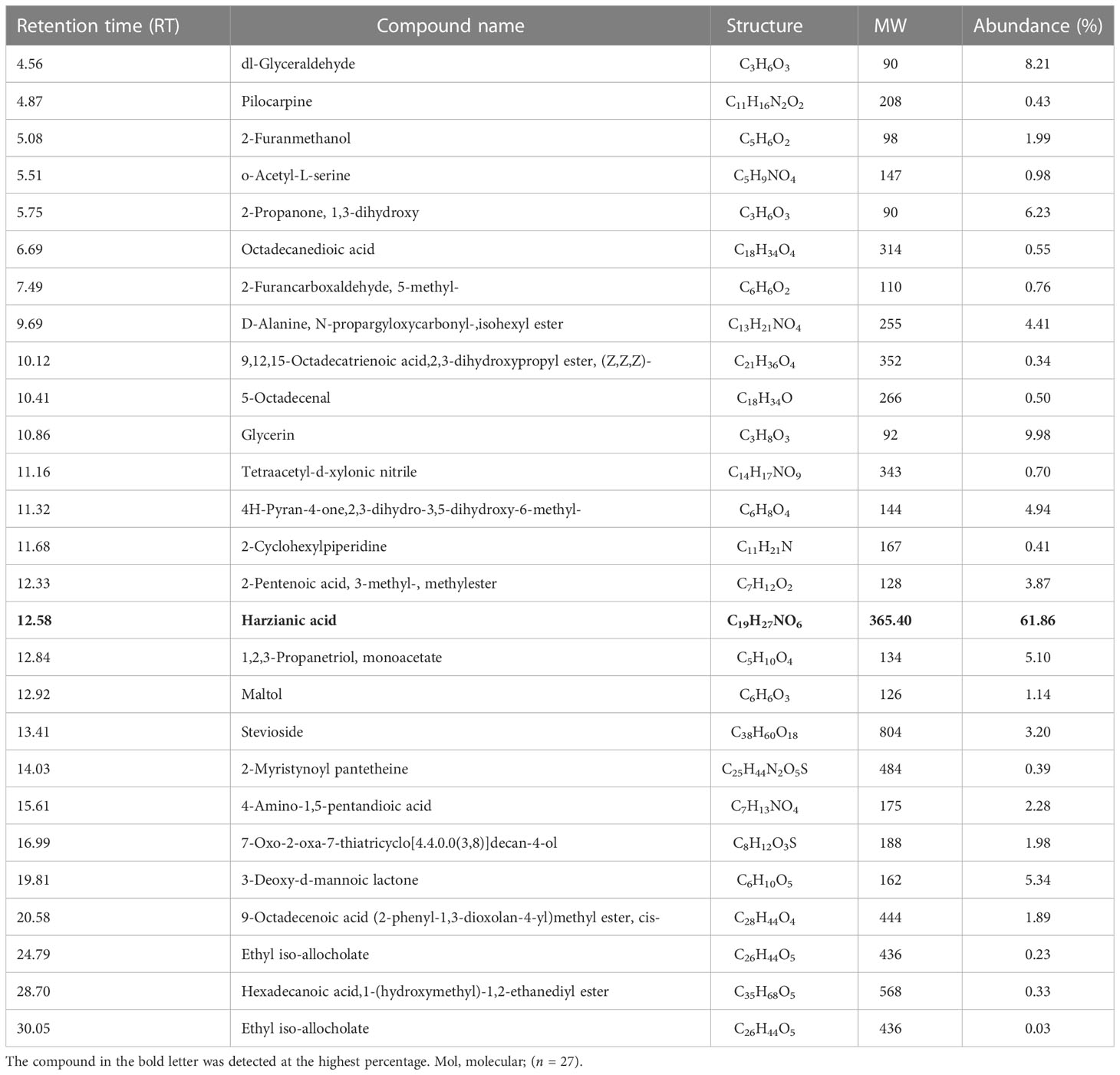
Table 1A Volatile organic compounds (VOCs) obtained from the culture filtrates of T. harzianum strain identified by GC–MS analysis. The bold letters indicates the compound which is abundantly present in the culture filtrates of T. harzianum.
Various groups of chemical compounds, including methyl, esters, sugar, glycerin, compounds containing an acetyl group, and compounds with a hydroxyl group, were detected in the CFs analysis of T. atroviride. The most significant and abundant compound (70.02%) in T. atroviride was 9,12-octadecadienoic acid (Z,Z)—which is commonly known as “linoleic acid” at the retention time (Rt) of 12.61 (Table 1B; Figure 2B). The chemical compounds 3-deoxy-d-mannoic lactone (10.15%), 2-propanone, 1,3-dihydroxy- (8.66%), dl-glyceraldehyde (8.67%), and glycerin (7.2%) were present, whereas the other chemical compounds were present in a lower amount <5%.
The results of GC–MS analysis of T. longibrachiatum culture filtrates (CFs) demonstrated the presence of various groups, viz., nitrile, methyl, hydroxyl groups, monosaccharides, glycosides, alcohols, ketoses, and some unknown compounds. The compound 2-furancarboxaldehyde,5-(hydroxymethyl)—commonly known as HMF or 5-(hydroxymethyl)-2—was detected at a higher level (68.08%) at the retention time (Rt) of 12.65 (Table 1C; Figure 2C). The compounds 3-deoxy-d-mannoic lactone (18.94%) and à-D-glucopyranoside,O-à-D-glucopyranosyl-(1.fwdarw.3)-á-D-fructofuranosyl (5.78%) were present in higher amounts, whereas other compounds were also detected in subordinate amounts (<5%). In these results, the CFs of these Trichoderma demonstrated the presence of various chemical compounds in higher amounts, including some common hydroxyl group-containing compounds (Tables 1A–C).
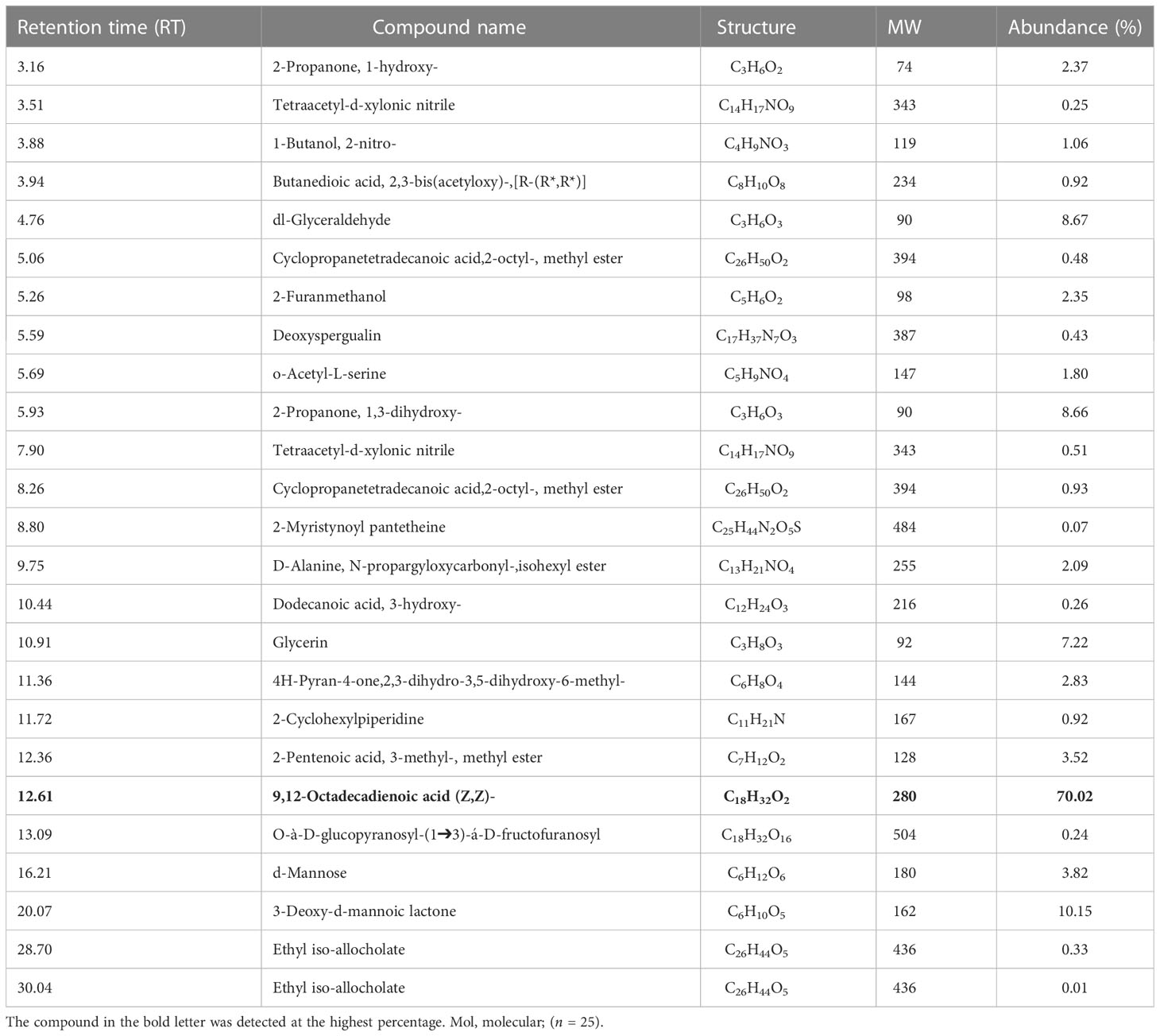
Table 1B Volatile organic compounds (VOCs) obtained from the culture filtrates of T. atroviride strain identified by GC–MS analysis. The bold letters indicates the compound which is abundantly present in the culture filtrates of T. atroviride.
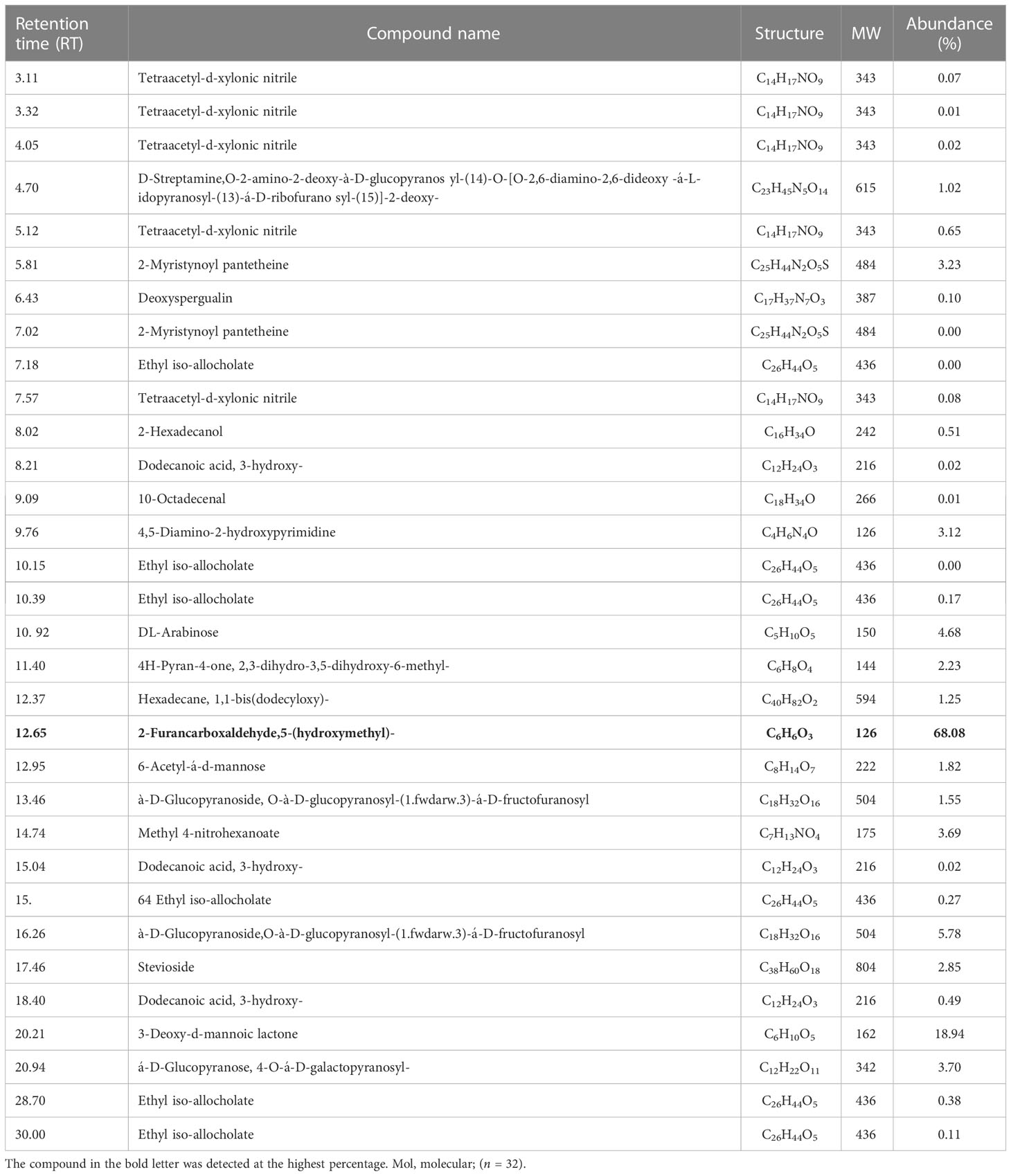
Table 1C Volatile organic compounds (VOCs) obtained from the culture filtrates of T. longibrachiatum strain were identified by GC–MS analysis. The bold letters indicates the compound which is abundantly present in the culture filtrates of T. longibrachiatum.
3.3 In vivo planta assay
3.3.1 Effect of Trichoderma CFs on disease severity
The foliar application of Trichoderma CFs demonstrated favorable effects on the suppression of early blight disease under greenhouse conditions. The disease severity in T. harzianum CF-treated plants was relatively lower (18.03%) than in T. longibrachiatum (31.91%) and T. atroviride (23.33%)-treated plants, followed by control (86.91%) (Figure 3). These results indicate that the foliar application of Trichoderma CFs drastically protected the plants from the infection of early blight pathogens under greenhouse conditions, as significant protection (79.25%) was observed in T. harzianum CF-treated plants, which was relatively higher than T. longibrachiatum (63.28%) and T. atroviride (73.15%) treated plants. These results illustrate that the foliar application of T. harzianum CFs remarkably protected the plants more than T. longibrachiatum and T. atroviride under greenhouse conditions, even after the artificial inoculation of A. solani. These results clearly indicate that the application of Trichoderma CFs has a transpicuous ability to attenuate the A. solani infection, and even a lower volume of CFs provided better control of the early blight pathogen.
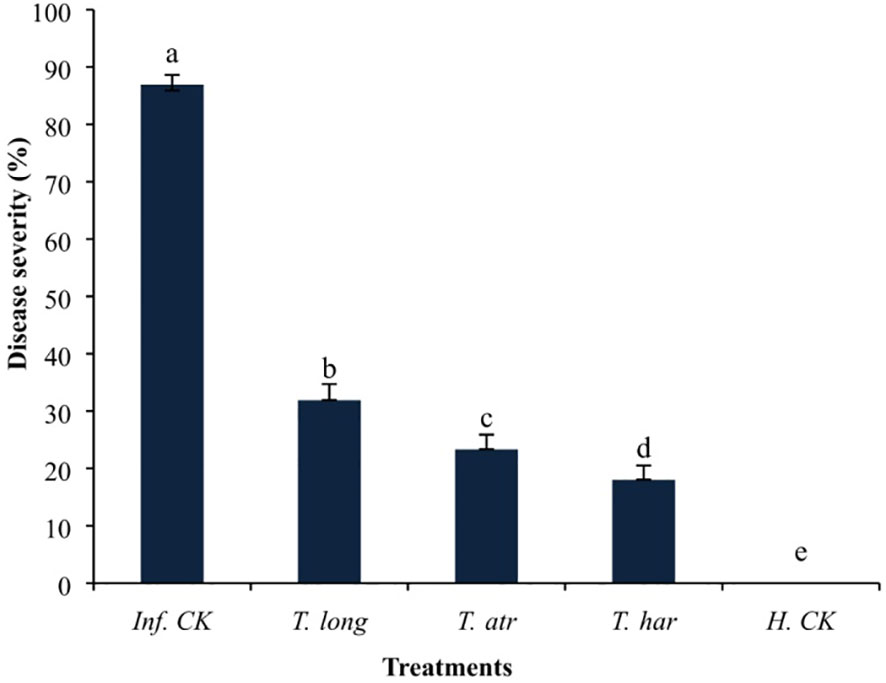
Figure 3 The effect of foliar application of Trichoderma culture filtrates (CFs) on the disease severity of A. solani on tomato plants in a greenhouse. Values followed by different letters indicate that means are significantly different from each other according to Fisher’s least significant difference at p = 0.05. T. har, Trichoderma harzianum; T. long, Trichoderma longibrachiatum; T. atr, Trichoderma atroviride; Inf. CK, infected control (pathogen inoculation); H.CK, healthy control (distilled water inoculation).
3.3.2 Effect of Trichoderma CFs on plant biomass
Foliar application of Trichoderma CFs not only alleviates the early blight infection on plants but also increases biomass, viz., the fresh and dry weight of roots and shoots. In all CF treatments, a considerable increase in the fresh and dry weight of plants was recorded. However, T. harzianum CFs instigated a more significant increase in fresh weight, branches, and leaves followed than other treatments (Table 2). In these results, T. harzianum CFs were found to be more responsive than T. longibrachiatum and T. atroviride. Thus, increased plant height allows more branches to emerge, which results in an increase in the weight of the plant and ultimately confers the plant growth promoter potential of these Trichoderma strains. Whereas no significant disparity between T. longibrachiatum and T. atroviride was recorded, although these treatments also demonstrated an increase in the growth parameters, followed by infected and healthy controls. In the present results, a significant reduction in the plant height, weight, branches, and number of leaves was recorded in the infected control (Table 2), which clearly demonstrates the catastrophic behavior of the early blight pathogen on plants. Results revealed that CFs of T. harzianum not only increase the plant height, weight, branches, and leaves of plants but also enhance the root development, which strengthens the plants to combat.
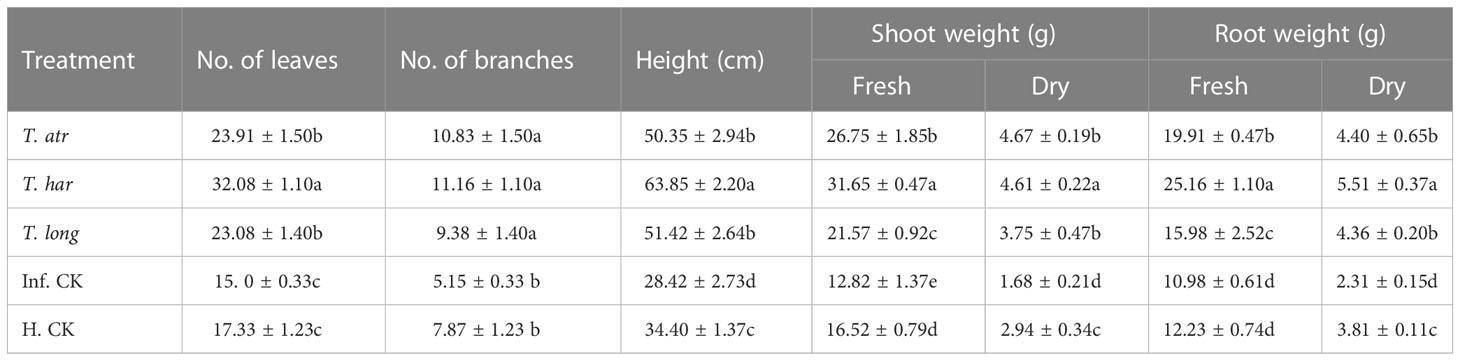
Table 2 Greenhouse effect of Trichoderma culture filtrates (CFs) on plant biomarkers post-treatment with A. solani. T. atr, Trichoderma atroviride; T. har, Trichoderma harzianum; T. long, Trichoderma longibrachiatum; Inf. CK, infected control (pathogen); H.CK, healthy control (water).
3.4 Effect of culture filtrates on phenolic compounds
3.4.1 Total phenol contents
Initially, the total phenol contents (TPCs) in all treatments were constant and slightly increased after 2 days in Trichoderma CF-treated plants. However, this increase in TPC remained constant (0.77 mg g−1 plant material) until 6 days, which decreased to 0.41 mg g−1 plant material after 8 days of inoculation. In these results, the TPCs in T. harzianum CF-treated plants were significantly higher than those in T. longibrachiatum and T. atroviride (Figure 4A). Comparatively, the increase in TPCs in T. harzianum treatments (0.73–0.77 mg g−1 plant material) was recorded, which remained superior to other Trichoderma treatments. However, lower TPC in infected (0.39 mg g−1 plant material) and healthy control (0.35 mg g−1 plant material) plants were observed, which slightly increased (0.49 and 0.46 mg g−1 plant material, respectively) after 6 days and then declined after 8 days (0.26 mg g−1 plant material). These results indicate that the foliar application of Trichoderma CFs, particularly T. harzianum (in this study), escalated the phenolic compound production in tomato leaves, which perhaps activated the defense system of plants to combat A. solani infection.
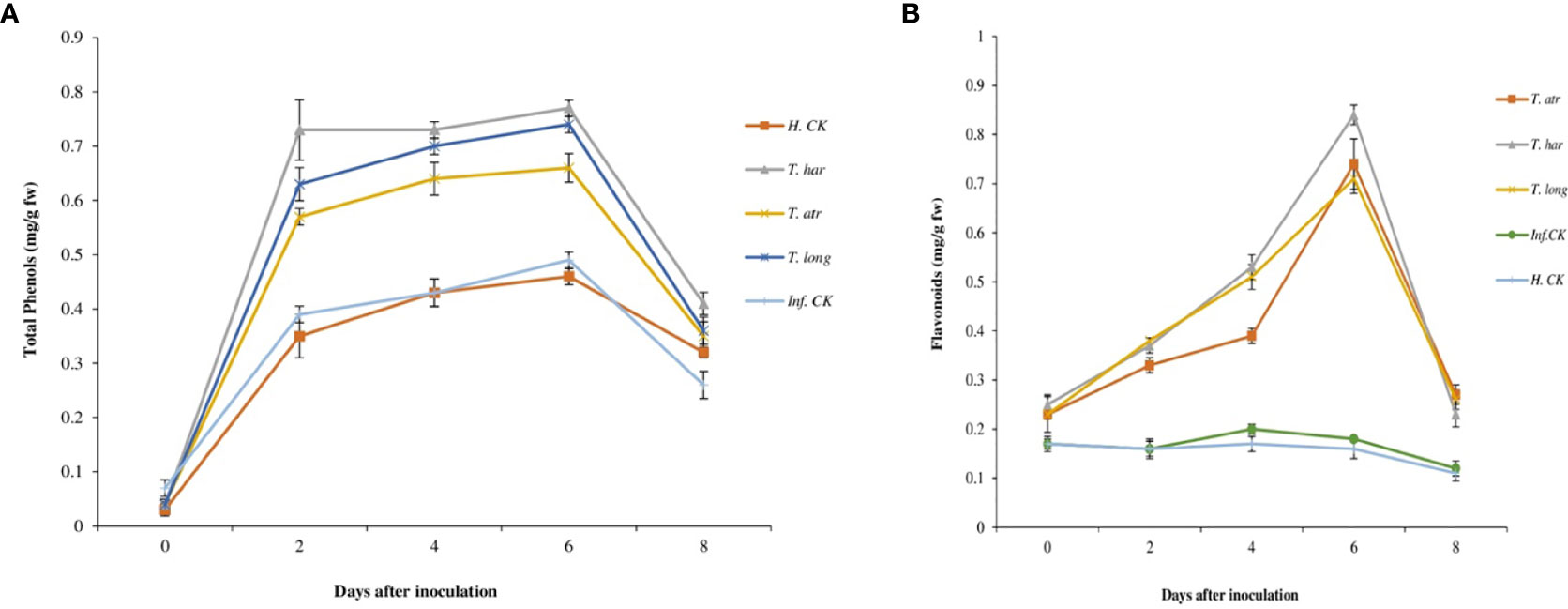
Figure 4 Effect of Trichoderma culture filtrates on (A) total phenol contents (mg gallic acid/g plant material), (B) flavonoids contents (mg/g plant material) in treated tomato leaves collected from greenhouse. T. har, Trichoderma harzianum; T. atr, Trichoderma atroviride; T. long, Trichoderma longibrachiatum; Inf. CK, infected control (plants treated with A. solani pathogen); H.CK, healthy control (plants treated with sterilized distilled water). Error bars on each graph represent the mean ± SE according to Fisher’s least significant difference (LSD) test at p = 0.05.
3.4.2 Flavonoids contents
Initially, the flavonoids contents were lower and constant in all treatments, including control, which showed a slight increase after 2 days (Figure 4B) in Trichoderma CF-treated plants, but no elevation in infected or healthy control plants was recorded. A continuous increase in flavonoids up to 6 days was observed, while higher flavonoids production in T. harzianum, T. atroviride, and T. longibrachiatum-treated plants (0.84, 0.74, and 0.71 mg g−1 plant material, respectively) was recorded that gradually declined after 6 days. Comparatively, T. harzianum demonstrated a higher and more significant increase (0.84 mg g−1 plant material) in flavonoids after 6 days, followed by T. atroviride and T. longibrachiatum (Figure 4B). Overall, Trichoderma treatments remained supercilious, which evidently confers the positive response of these Trichoderma strains on flavonoid production in tomato plants.
3.5 Effect of culture filtrates on antioxidant enzyme
3.5.1 Phenylalanine ammonia-lyase assay
Initially, in all treated plants, the phenylalanine ammonia-lyase (PAL) activity remained lower and consistent. Subsequently, a gradual increase in PAL activity was recorded after 2 days, which reached its maximum after 4 days in T. longibrachiatum, T. atroviride, and T. harzianum (0.53, 0.52, and 0.47 nmol of cinnamic acid min−1 g−1 protein, respectively) treated plants (Figure 5A). Though the PAL activity in infected and healthy control plants demonstrated a considerable increase after 4 days (0.21 and 0.22 nmol of cinnamic acid min−1 g−1 protein, respectively), this increase was not significant compared to Trichoderma CF-treated plants. While no significant difference between T. longibrachiatum and T. atroviride-treated plants was recorded (Figure 5A). On the other hand, the results herein presented concluded that the PAL activity in T. longibrachiatum and T. atroviride-treated plants was considerably higher than that in T. harzianum, and these Trichoderma strains positively affected the PAL activity in tomato plants.
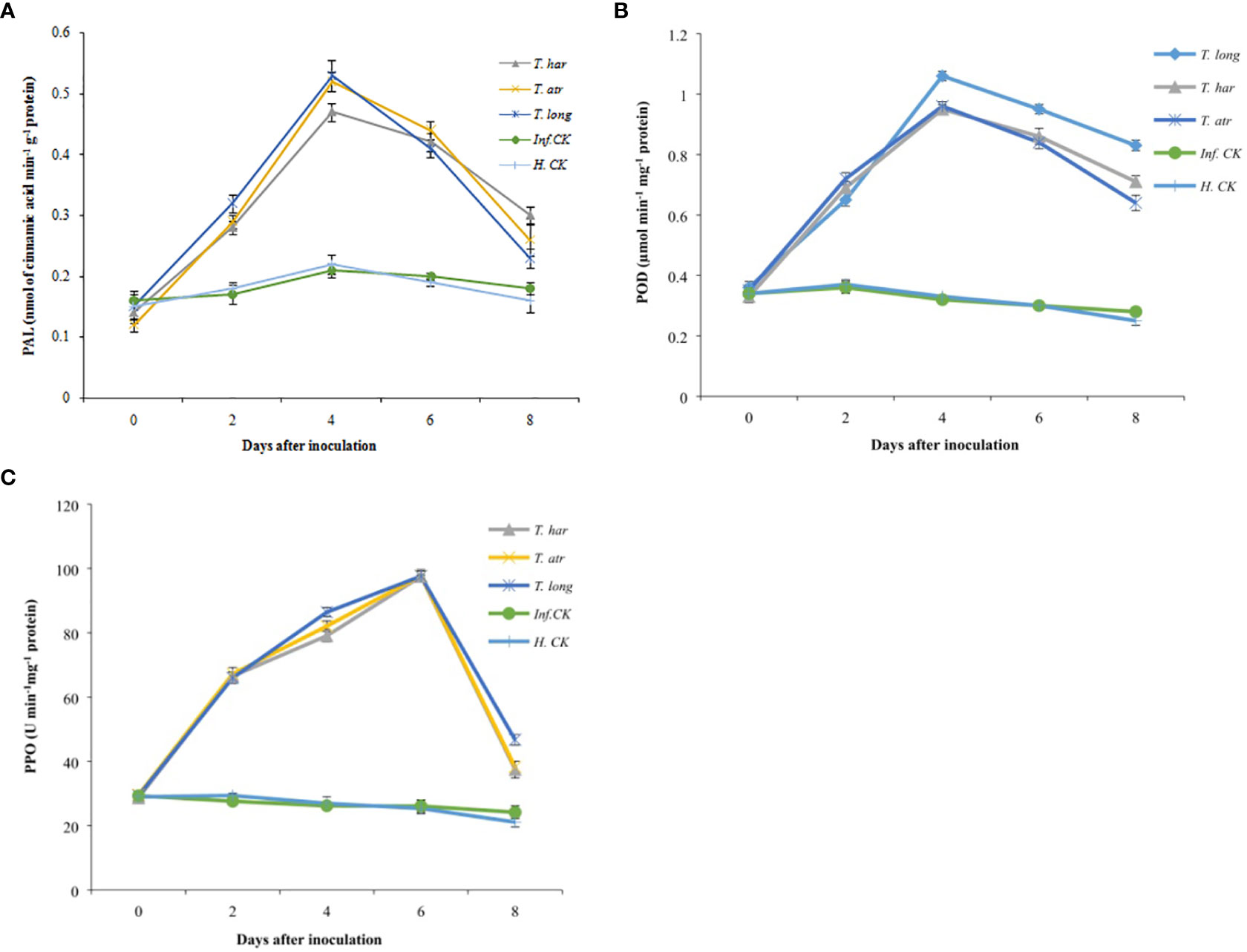
Figure 5 Effect of Trichoderma culture filtrates on (A) phenylalanine ammonia-lyase (nmol of cinnamic acid min−1 g−1 protein) activity, (B) peroxidase activity (µmol min−1 mg−1 protein), (C) polyphenol oxidase activity (U min−1 mg−1 protein) in inoculated tomato leaves collected from greenhouse. T. har, Trichoderma harzianum; T. atr, Trichoderma atroviride; T. long, Trichoderma longibrachiatum; Inf. CK, infected control (plants treated with A. solani pathogen); H.CK, healthy control (plants treated with sterilized distilled water). Error bars on each graph represent the mean ± SE according to Fisher’s least significant difference (LSD) test at p = 0.05.
3.5.2 Peroxidase activity assay
Peroxidase (POD) activity in all treatments, including healthy and infected control plants, was relatively lower, and a slight increase in POD activity in Trichoderma CF-treated plants was observed after 2 days of inoculation (Figure 5B). However, the momentous increase in Trichoderma CF-treated plants after 4 days was recorded and remained higher in T. longibrachiatum (1.06 µmol min−1 mg−1 protein), followed by T. harzianum (0.95 µmol min−1mg−1 protein) and T. atroviride (0.96 µmol min−1mg−1 protein). Generally, no significant increase in POD activity in control plants was observed, though a persistent decrease was recorded. Comparatively, the POD activity in healthy and infected controls was lower than in Trichoderma treatments, whereas, among Trichoderma strains, T. longibrachiatum demonstrated a promising increase, which confers the positive effect of T. longibrachiatum on POD induction.
3.5.3 Polyphenol oxidase activity
Polyphenol oxidase (PPO) activity in all treatments was lower, primarily increasing after 2 days of inoculation, and no significant difference was observed among Trichoderma treatments as PPO activity remained uniform (66–67.24 U min−1 mg−1 protein) but higher than healthy (29.39 U min−1 mg−1 protein) and infected control (27.62 U min−1 mg−1 protein). A significant increase in PPO activity after 4 days was recorded, with a significantly higher value after 6 days (97 ± 0.76 U min−1 mg−1 protein) and a decline after 8 days (Figure 5C). PPO activity in infected and healthy control plants was relatively lower than in Trichoderma-treated plants. In these results, all Trichoderma strains positively responded to significant PPO production in plants.
3.6 Open field trails
3.6.1 Effect of culture filtrates on disease severity
The efficacy of Trichoderma culture filtrates (CFs) to mitigate the early blight disease under natural infection conditions was monitored, and the field application of Trichoderma CFs significantly reduced the early blight infection in the open field. In these results, significant reduction in disease severity (%) after the application of T. harzianum (SI-12.18%; SII-12.56%), followed by naturally infected control plants (SI-37.87%; SII-36.83%) in both seasons (Figure 6A). However, the application of T. atroviride and T. longibrachiatum also demonstrated vital control of early blight infection in both seasons (SI-21.17%; SII-20.75%) and (SI-16.62%; SII-19.12%), respectively. In the present study, the field application of Trichoderma CFs exhibited considerable protection to tomato plants even after natural infection with the early blight pathogen. Foliar application of T. harzianum CFs substantially protected the tomato plants from the natural infection of early blight disease in both seasons (SI-67.83%; SII-65.89%), while the application of T. longibrachiatum and T. atroviride CFs also provided significant protection. These results revealed that the applications of these Trichoderma CFs are capable of diminishing the early blight infection in open fields, and even lower volumes (50 ml/plant) can effectively control the early blight pathogen in open fields. Thus, it can be predicted that the increased volume of these Trichoderma CFs may provide exceptional management of this pathogen in fields. Additionally, multiple applications of CFs at different plant growth stages in a season may also help to reduce early blight infection. As in the present study, the application of these Trichoderma CFs in open fields provided a significant reduction in early blight.
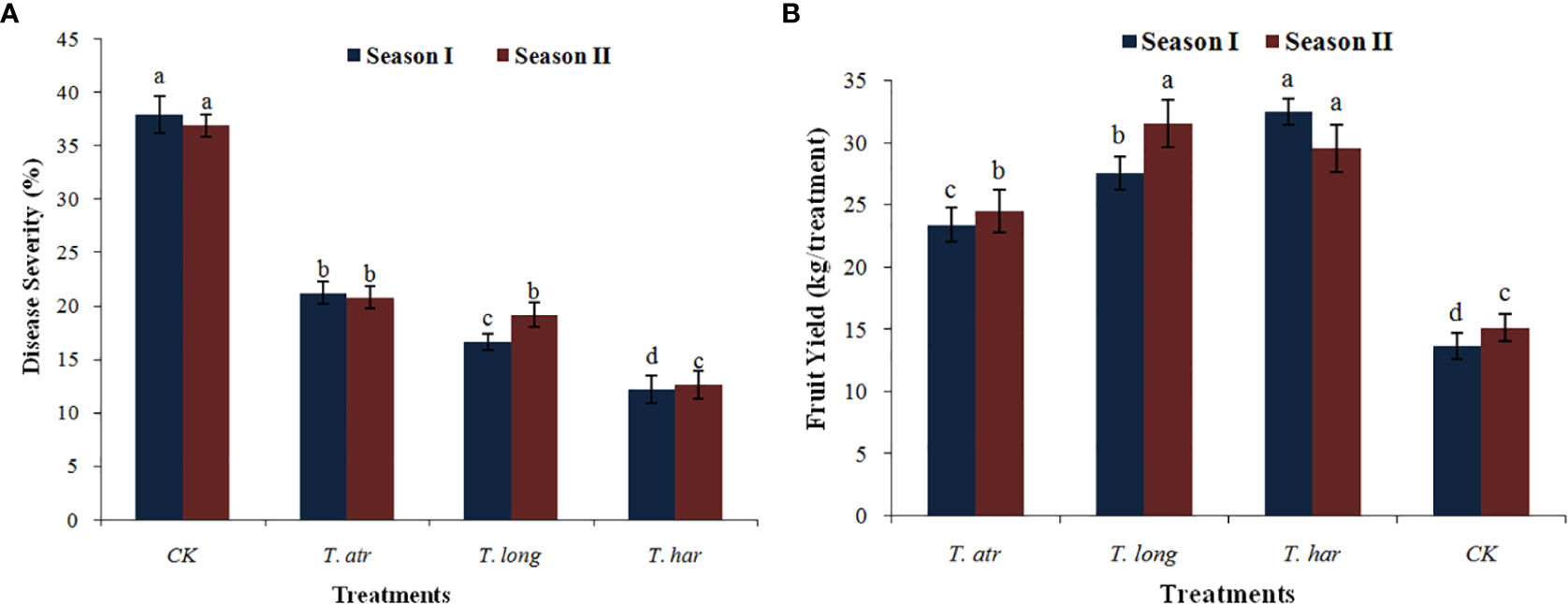
Figure 6 The effect of foliar application of Trichoderma culture filtrates (CF) on (A) disease severity (%) of early blight and (B) fruit yield in an open field under natural infection. Values followed by different letters indicate that means are significantly different from each other according to Fisher’s least significant difference at p = 0.05. T. har, Trichoderma harzianum; T. long, Trichoderma longibrachiatum; T. atr, Trichoderma atroviride; CK, naturally infected control (untreated plants).
3.6.2 Effect of culture filtrates on fruit yield
The foliar application of Trichoderma CFs in field conditions not only curtailed the early blight disease severity but also improved the fruit yield in both seasons, as a significant increase in yield was observed. The fruit yield in T. atroviride, T. longibrachiatum, and T. harzianum was considerably higher than that in untreated/naturally infected control plants during season I, which increased in season II (Figure 6B). The Trichoderma treatments, viz., T. atroviride and T. longibrachiatum, exhibited lower fruit production (23.26 kg and 27.54 kg, respectively) during season I (Figure 6B), which increased in season II (24.48 kg and 31.54 kg, respectively). In the field application of T. harzianum CFs, higher (32.29 kg) fruit production was recorded in season I, which was slightly reduced in season II (29.58 kg). These results illustrate that the application of CFs of these Trichoderma strains in open fields not only minimized early blight infection but also improved yield, which symbolizes the positive effect of Trichoderma culture filtrates.
4 Discussion
Biological control approaches, particularly using fungal and bacterial microorganisms as biocontrol agents, are being considered as an emerging tool to combat fungicide resistance challenges. A variety of Bacillus and Trichoderma species with strong biocontrol potential, along with salt compounds and nanoparticles, have extensively been used to control plant diseases, including early blight on tomatoes (Mazrou et al., 2020; Stracquadanio et al., 2020; Castro-Restrepo et al., 2022; Imran et al., 2022b; Narware et al., 2023). Presently, the use of culture filtrates (CFs) in agriculture systems is considered an emerging method to control plant diseases and is getting a lot of attention due to its effective potential to inhibit plant pathogens. In the present study, the in vitro application of Trichoderma culture filtrates (CFs) revealed significant suppression of the mycelial growth of A. solani. The CFs of T. harzianum demonstrated strong inhibition of mycelial growth as lower mycelial growth (31.5 mm) was recorded, followed by T. longibrachiatum (43.06 mm) and T. atroviride (35.43 mm). The results herein presented illustrate the strong inhibitory potential of Trichoderma CFs. In a most recent study, dominant mycelial growth inhibition of Pythium undulatum and Phytophthora inundata by the CFs of T. simmonsii and T. asperellum was recorded (Ben M'henni et al., 2022). In another study, the CFs of various Trichoderma species, including T. harzianum (29.88 mm) and T. viride (27.13 mm), demonstrated remarkable suppression of R. oryzae (Alka and Prajapati, 2017). Additionally, the CFs of five Trichoderma species (T. viride, Trichoderma PP3, T. harzianum, Trichoderma PP2, and T. koningii) as biofungicides exhibited strong hindrance to C. gloesporioides growth (Nurbailis et al., 2019). Various studies have reported the significant mycelial growth inhibition of various fungal pathogens (Filizola et al., 2019; Mulatu et al., 2022), which sturdily confers the biocontrol potential of Trichoderma CFs, and these evidently support the results of the present study. Trichoderma species as biocontrol agents against fungal pathogens mainly involve various mechanisms, i.e., competition for food, space, and nutrients, resulting in the production of various metabolites that depreciate the mycelium of fungal pathogens (Alabouvette et al., 2006). There are ample pieces of evidence that report the abundant metabolite production by Trichoderma species, which showed significant inhibition of a broad spectrum of fungal pathogens from different taxonomic groups (Abbas et al., 2019; Edin et al., 2019; Mazrou et al., 2020; Mahmoud et al., 2021). In the present study, GC–MS analysis of Trichoderma species CFs demonstrated the abundant production of volatile organic compounds containing various classes of compounds. The chemical compounds and metabolites in the CFs of Trichoderma species identified from the known spectra in the National Institute of Standards and Technology (NIST) database exhibited the presence of harzianic acid (C19H27NO6) in T. harzianum, linoleic acid (C18H32O2) as 9,12-octadecadienoic acid (Z,Z)—in T. atroviride and hydroxymethylfurfural (C6H6O3) as 2-furancarboxaldehyde,5-(hydroxymethyl)—in T. longibrachiatum as the main components that were observed in relatively higher abundance (%). The abundance (%) and quantity of active compounds/metabolites in Trichoderma CFs can differ depending upon the method of compound extraction and the species of Trichoderma. Various researchers reported identical compounds during the GC–MS analysis of T. harzianum, T. atroviride, and T. longibrachiatum culture filtrates (Reithner et al., 2007; Anita et al., 2012; Stracquadanio et al., 2020), while dissimilarity in active metabolic compounds, viz., 6-pentyl-α-pyrone (Yassin et al., 2022), harzianic acid (Xie et al., 2021), acetic acid (Yassin et al., 2021), glacial acetic acid, and ethanoic acid (Siddiquee et al., 2012), was reported in the CFs of T. harzianum in GC–MS analysis. In the greenhouse application of CFs, T. harzianum demonstrated a promising effect for the reduction of disease severity that might be due to the excessive production of harzianic acid because harzianic acid is an important secondary metabolite from T. harzianum that has been widely reported to have strong antimicrobial and plant growth promoter potential against various phytopathogens such as Rhizoctonia solani, Sclerotinia sclerotiorum, and Pythium irregulare and also capable of chelating soil iron (Fe3+) in plants (Vinale et al., 2013; Vukelić et al., 2021; Xie et al., 2021). During GC–MS analysis, various volatile compounds, including esters, hydrocarbons, ethers, alcohols, aldehydes, ketones, and different acids, were found. Various studies reported the presence of similar compounds with considerable yield (%) differentiation in metabolites of Trichoderma CFs (Anita et al., 2012; Sarsaiya et al., 2020; Sornakili et al., 2020; Stracquadanio et al., 2020; Rajani et al., 2021). In greenhouses, the foliar application of Trichoderma CFs demonstrated a significant reduction in disease severity (%), as lower disease severity (%) was recorded in the plants inoculated with T. harzianum CFs (18.03%) as compared to T. longibrachiatum (31.91%) and T. atroviride (23.33%), followed by infected control (86.91%). Besides the alleviation of disease severity, the CFs of Trichoderma strains also exhibited a momentous increase in the biomass of plants, viz., plant leaves and branches, and the fresh and dry weight of roots and shoots, which confers the growth promoter potential of Trichoderma species. However, various studies reported the optimistic influence of Trichoderma species on plant biomass. The application of T. harzianum and T. asperellum positively increased the plant biomass of Diplotaxis tenuifolia (Caruso et al., 2020) and Mentha spicata, respectively (Castro-Restrepo et al., 2022). In another study, the combined application of Trichoderma simmonsii and Aspergillus westerdijkiae also exhibited an increase in the growth of apple trees (Ben M'henni et al., 2022). These studies sturdily confer the plant growth promoter potential of Trichoderma species, which strappingly supports the findings of the present study. Thus, an increase in plant biomass and reduction in disease after the application of synthetic pathogenic infection might be associated with the stimulation of antioxidants and the production of polyphenolic secondary metabolites in plants that ultimately induce resistance against pathogen infection. Secondary metabolites such as lignin and phenolic acid significantly medicate the defense activity by strengthening the rigidity of the cell wall, which eventually prevents the invasion of pathogens into plants (El-Khallal, 2007; Abo-Elyousr et al., 2008). Various pathogenesis-related (PR) proteins are coded by the host and have a significant role in the defense system of plants induced by the infection of various pathogens as well as abiotic stress (Jiang et al., 2015). In the present study, a significant increase in total phenol (TP) contents, flavonoids, phenylalanine ammonia lyase (PAL), peroxidases (POD), and polyphenol oxidase (PPO) in Trichoderma-treated plants was recorded that was relatively higher than untreated/control plants. However, a significant increase in TP contents was recorded after two days of inoculation, while flavonoids contents increased after 6 days of inoculation in Trichoderma-treated plants followed by healthy and infected controls. Flavonoids play a significant role in plants and act as detoxifying agents, phytoalexins, signal molecules, and allelochemical agents (Fawe et al., 1998; McNally et al., 2003; Peer and Murphy, 2006). The increase in TP and flavonoids in plants demonstrates that these Trichoderma species robustly induce systemic resistance by releasing not only proteins but also secondary metabolites. The results reported by EL-Tanany et al. (2018) and Mayo-Prieto et al. (2019) showed a considerable increase in flavonoids and total phenolic contents when the tomato plants were inoculated with T. viride or T. hamatum after the inoculation with A. solani and T. velutinum after the inoculation with R. solani, respectively, and these documented findings are in support of our results and strongly underpin these findings. In another study, the highest increase in enzyme activity was recorded when the tomato plants were inoculated with T. harzianum and R. solani (Youssef et al., 2016). In accordance with Mahmoud et al. (2021), tomato plants treated with A. cerealis and T. harzianum extensively increase the total phenol and flavonoid contents, and our results are in agreement with the previously reported findings and substantiate that Trichoderma species accumulate PR-proteins and phenolic compounds, which hinder the invasion of the A. solani pathogen in plants. Furthermore, tomato leaves contain solavetivone, flavonoids, lubimin, phytuberol, phytuberin, glutinosone, and rishitin, which are toxic and antimicrobial agents that act as rebellious compounds against various phytopathogens (Kim et al., 2019).
Phenylalanine ammonia-lyase (PAL), peroxidases (POD), and polyphenol oxidase (PPO) are the major enzymes for the induction of resistance in plants against biotic and abiotic stresses. Phenolic compounds are endogenous growth regulators, whereas PAL is a key enzyme for the biosynthesis of phenolic compounds from the shikimic acid pathway, which may amend the defense response by regulating the biosynthesis of phenolic compounds after different stresses (Caretto et al., 2015; Abo-Elyousr et al., 2020). The activation of PAL is mainly associated with distinct signal transduction pathways with respect to apoptotic cell death and oxidative burst (Sasabe et al., 2000), and the induction of PAL by pathogen-derived elicitors can provide significant insights into signal transduction mechanism that activates the plant response. Further, the stimulation of PAL may also enable a quantitative appraisal of both plant responses and the effectiveness of protein. Generally, tomato plants, not only fruits but roots, stems, and leaves, are also considered rich sources of antioxidants and phenolic compounds, and in this study, a significant increase in PAL contents in tomato leaves was recorded after 4 days of inoculation followed by healthy and infected control plants. A study by Kumar et al. documented that the application of T. harzianum and T. viride increased the induction of defense enzymes, including total phenol and PAL, after 48 h of inoculation against early blight infection (Kumar et al., 2022). Various studies reported a significant increase in PAL activity in tomato plants after inoculation with the suspension of Trichoderma species (Kashyap et al., 2020; Tripathi et al., 2021; Kumar et al., 2022), which confers the positive impact of Trichoderma species on plants, and these results strongly support our findings. Peroxidases (POD) widely contribute to physiological processes such as the formation of suberin and lignin phytoalexin synthesis, cross-linking of cell wall components, and/or also participate in reactive nitrogen species (RNS) and reactive oxygen species (ROS) metabolism, which activate the hypersensitive response by limiting the expansion of pathogens (Peer and Murphy, 2006). While PPO implicates the lignification of plant cells and the oxidation of polyphenols into quinines (antimicrobial compounds) during the infection of pathogens. In this study, a significant increase in POD and PPO activity after the application of Trichoderma CFs was recorded; thus, the increase in PPO and POD activity that restricted the pathogen growth may be due to the oxidation of phenolic compounds to quinone, which increases the antimicrobial activity and ultimately strengthens the defense system of the plant. Various researchers documented the identical findings, which showed an astronomical increase in phenolic contents in response to various treatments (Pawel et al., 2020), which affirm that the increase in phenolic compounds activates the defense system of plants against the fungal infection. It is to be believed that phytotoxic chemical compounds engender the overproduction of ROS in plants and ultimately elevate the level of phenolic compounds, which may act as sufficient antioxidants to prevent the formation of cellular deterioration due to oxidative stress. Thus, the higher activity of PPO, POD, and PAL increased the oxidation of phenolic compounds, which led to distortion of cell wall structure and restricted pathogen growth.
In the present study, the open field trials under natural infection of early blight revealed that the CFs of these Trichoderma strains not only mitigated the infection of the pathogen but also escalated the yield production, which epitomizes the positive effect of CFs and their capability to promote fruit production in open field conditions even during natural infection. A study by Soesanto et al. (2022) documented that the culture filtrates of T. harzianum not only improved the growth and yield components of tomato plants but also increased the phenolic compounds, number of fruits, and total yield under in vivo conditions (Soesanto et al., 2022). In another study, a significant increase in yield and growth-related parameters of Celosia cristata was recorded after the inoculation of Trichoderma strains (Wang et al., 2021). Similar findings were also reported by Alka and Prajapati (2017); Vukelić et al. (2021), and Sriwati et al. (2019) when the culture filtrates (CFs) of different Trichoderma strains were used against various fungal plant diseases of tomato, and these results are in agreement with the findings of the present study, which positively support these results. In this study, the beneficial effect of Trichoderma species was confirmed, and a safe approach using culture filtrates (CFs) to diminish the early blight disease in open fields in this region was deployed.
5 Conclusion
These results of the present study suggested that the foliar application of Trichoderma culture filtrates (CFs) considerably reduced the mycelia growth of A. solani under in vitro conditions, which confers the strong inhibitory potential of CFs against fungal pathogens. Moreover, the CFs of these Trichoderma strains not only decreased the early blight infection in greenhouses, but also improved plant growth-related parameters and, in field conditions, also promoted fruit production. The most important aspect of CFs is the induction of antioxidants in plants, which act as the first barrier against pathogen infection. Significantly increased antioxidant production firmly restricts the further invasion of pathogens. Therefore, an appropriate number of CFs at different growth stages of tomato plants may ameliorate plant vigor, disease severity, and fruit yield by acting as plant growth promoters. We believe that it would be of great interest to carry out identical practices in other regions to minimize the fungicide resistance risk of early blight pathogens, which are becoming more serious concerns in sustainable agricultural production.
Data availability statement
The original contributions presented in the study are included in the article/Supplementary Material, further inquiries can be directed to the corresponding authors.
Author contributions
MI: Conceptualization, experimentation, methodology, formal analysis, and writing—original draft. KA-E: Supervision and review and editing. MM: Supervision, conceptualization, formal analysis, and review and editing. MS: Supervision, revision, and formal analysis. All authors contributed to the article and approved the submitted version.
Acknowledgments
We thank the Deanship of Graduate Studies for providing the scholarship to complete the research. The authors would like to thank the Growbiom research and development team and The Center for Desert Agriculture (CDA) for their financial support and help in many aspects of this work. The authors are thankful and acknowledge the Deanship of Graduate Studies (DGS) King Abdulaziz University, Jeddah Saudi Arabia for providing International Post-graduate Scholarship to conduct this research.
Conflict of interest
The authors declare that the research was conducted in the absence of any commercial or financial relationships that could be construed as a potential conflict of interest.
Publisher’s note
All claims expressed in this article are solely those of the authors and do not necessarily represent those of their affiliated organizations, or those of the publisher, the editors and the reviewers. Any product that may be evaluated in this article, or claim that may be made by its manufacturer, is not guaranteed or endorsed by the publisher.
Supplementary material
The Supplementary Material for this article can be found online at: https://www.frontiersin.org/articles/10.3389/fpls.2023.1192818/full#supplementary-material
References
Abbas, A., Ullah, S. K., Ullah, W. K., Saleh, T., Ullah, M. K., Ullah, S., et al. (2019). Antagonist effects of strains of Bacillus spp. against Rhizoctonia solani for their protection against several plant diseases, alternatives to chemical pesticides. Comptes Rendus Biol. 342, 124–135. doi: 10.1016/j.crvi.2019.05.002
Abo-Elyousr, K. A. M., Almasoudi, N. M., Abdelmagid, A. W. M., Roberto, S. R., Youssef, K. (2020). Plant extract treatments induce resistance to bacterial spot by tomato plants for a sustainable system. Horticulturae 6 (2), 36. doi: 10.3390/horticulturae6020036
Abo-Elyousr, K. A. M., Hussein, M. A. M., Allam A.D.A. and Hassan, M. H. A. (2008). Enhanced onion resistance against stemphylium leaf blight disease, caused by Stemphylium vesicarium, by di-potassium phosphate and benzothiadiazole treatments. Plant Pathol. J. 24, 171–177. doi: 10.5423/PPJ.2008.24.2.171
Abo-Elyousr, K. A. M., Imran, M., Almasoudi, N. M., Ali, E. F., Hassan, S., Sallam, N., et al. (2022). Controlling of Xanthomonas axonopodis pv. phaseoli by induction of phenolic compounds in bean plants using salicylic and benzoic acids. J. Plant Pathol. 104 (3), 947–957. doi: 10.1007/s42161-022-01102-5
Akhtar, K. P., Saleem, M. Y., Iqbal, Q., Asghar, M., Hameed, A., Sarwar, N. (2016). Evaluation of tomato genotypes for late blight resistance using low tunnel assay. J. Plant Pathol. 421-428.
Akram, W., Ahmad, A., Yasin, N. A., Anjum, T., Ali, B., Fatima, S., et al. (2021). Mechanical strengthening and metabolic re-modulations are involved in protection against Fusarium wilt of tomato by B. subtilis IAGS174. J. Plant Inter. 16 (1), 411–421. doi: 10.1080/17429145.2021.1966107
Alabouvette, C., Olivain, C., Steinberg, C. (2006). Biological control of plant diseases, the European situation. Eur. J. Plant Pathol. 114 (3), 329–341. doi: 10.1007/s10658-005-0233-0
Alka, R. K., Prajapati, B. K. (2017). Effect of Trichoderma spp. and its culture filtrate antagonists on growth and management of rhizopus rot of tomato fruit in vitro and in vivo. J. Pharmacogn. Phytochem. 6 (4), 394–398.
Almagro, L., Gómez-Ros, L. V., Belchi-Navarro, S., Bru, R., Ros-Barceló, A., Pedreño, M. A. (2009). Class III peroxidases in plant defence reactions. J. Exp. Bot. 60, 377–390. doi: 10.1093/jxb/ern277
Anand, T., Chandrasekaran, A., Kuttalam, S., Raguchander, T., Prakasam, V., Samiyappan, R. (2007). Association of some plant defense enzyme activities with systemic resistance to early leaf blight and leaf spot induced in tomato plants by azoxystrobin and Pseudomonas fluorescens. J. Plant Inter. 2 (4), 233–244. doi: 10.1080/17429140701708985
Anita, S., Ponmurugan, P., Babu, R. G. (2012). Significance of secondary metabolites and enzymes secreted by Trichoderma atroviride isolates for the biological control of Phomopsis canker disease. Afr. J. Biotechnol. 11 (45), 10350–10357. doi: 10.5897/AJB12.599
Babenko, L. M., Smirnov, O. E., Romanenko, K. O., Trunova, O. K., Kosakivska, I. V. (2019). Phenolic compounds in plants, biogenesis and functions. Ukr. Biochem. J. 91, 5–18. doi: 10.15407/ubj91.03.005
Bauske, M. J., Mallik, I., Yellareddygari, S. K. R., Gudmestad, N. C. (2017). Spatial and temporal distribution of mutations conferring QoI and SDHI resistance in Alternaria solani across the united states. Plant Dis. 102, 349–358. doi: 10.1094/PDIS-06-17-0852-RE
Ben M'henni, Y., Salem, I. B., Souli, M., Tounsi, S., Debieu, D., Fillinger, S., et al. (2022). Biocontrol and growth promotion potential of combined application of Trichoderma simmonsii and Aspergillus westerdijkiae against apple tree dieback disease. PhytoFrontiers. 2 (3), 268–279. doi: 10.1094/PHYTOFR-01-22-0005-R
Bradford, M. M. (1976). A rapid and sensitive method for the quantification of microgram quantities of protein utilizing the principle of protein-dye binding. Anal. Biochem. 72, 248–254. doi: 10.1016/0003-2697(76)90527-3
Camlica, E., Tozlu, E. (2019). BIological control of Alternaria solani in tomato. Fresenius Env. Bull. 28, 7092–7100.
Caretto, S., Linsalata, V., Colella, G., Mita, G., Lattanzio, V. (2015). Carbon fluxes between primary metabolism and phenolic pathway in plant tissues under stress. Int. J. Mol. Sci. 16, 26378–26394. doi: 10.3390/ijms161125967
Caruso, G., El-Nakhel, C., Rouphael, Y., Comite, E., Lombardi, N., Cuciniello, A., et al. (2020). Diplotaxis tenuifolia (L.) DC. yield and quality as influenced by cropping season, protein hydrolysates, and Trichoderma applications. Plants 9 (6), 697. doi: 10.3390/plants9060697
Castro-Restrepo, D., Dominguez, M. I., Gaviria-Gutiérrez, B., Osorio, E., Sierra, K. (2022). Biotization of endophytes Trichoderma asperellum and Bacillus subtilis in Mentha spicata microplants to promote growth, pathogen tolerance and specialized plant metabolites. Plants. 11, 1474. doi: 10.3390/plants11111474
Cavallo, P., Dini, I., Sepe, I., Galasso, G., Fedele, F. L., Sicari, A., et al. (2020). An innovative olive pâté with nutraceutical properties. Antioxidants. 9, 581. doi: 10.3390/antiox9070581
Chang, C. C., Yang, M. H., Wen, H. M., Chern, J. C. (2002). Estimation of total flavonoid content in propolis by two complementary colorimetric methods. J. Food Drug Anal. 10, 178–182. doi: 10.38212/2224-6614.2748
Constabel, C. P., Barbehenn, R. (2008). Defensive roles of polyphenol oxidase in plants. in induced plant resistance to herbivory. Ed. Schaller, A. (Dordrecht, The Netherlands: Springer), 253–270. doi: 10.1007/978-1-4020-8182-8_12
Dickerson, D. P., Pascholati, S. F., Hagerman, A. E., Butler, L. G., Nicholson, R. L. (1984). Phenylalanine ammonia-lyase and hydroxycinnamate, CoA ligase in maize mesocotyls inoculated with Helminthosporium maydis or Helminthosporium carbonum. Physiol. Plant Pathol. 25, 111–123. doi: 10.1016/0048-4059(84)90050-X
Dini, I., Laneri, S. (2021). Spices, condiments, extra virgin olive oil and aromas as not only flavorings, but precious allies for our wellbeing. Antioxidants. 10, 868. doi: 10.3390/antiox10060868
Dini, I., Marra, R., Cavallo, P., Pironti, A., Sepe, I., Troisi, J., et al. (2021a). Trichoderma strains and metabolites selectively increase the production of volatile organic compounds (VOCs) in olive trees. Metabolites. 11, 213. doi: 10.3390/metabo11040213
Dini, I., Pascale, M., Staropoli, A., Marra, R., Vinale, F. (2021b). Effect of selected Trichoderma strains and metabolites on olive drupes. Appl. Sci. 11, 8710. doi: 10.3390/app11188710
Edin, E., Liljeroth, E., Andersson, B. (2019). Long term field sampling in Sweden reveals a shift in occurrence of cytochrome b genotype and amino acid substitution F129L in Alternaria solani, together with a high incidence of the G143A substitution in Alternaria alternata. Eur. J. Plant Pathol. 155, 627–641. doi: 10.1007/s10658-019-01798-9
El-Khallal, S. M. (2007). Induction and modulation of resistance in tomato plants against fusarium wilt disease by bioagent fungi (Arbuscular mycorrhiza) and/or hormonal elicitors (Jasmonic acid & salicylic acid), 1-changes in the antioxidant enzymes, phenolic compounds and pathogen related-proteins. Aust. J. Basic Appl. Sci. 1, 717–732.
EL-Tanany, M. M., Hafez, M. A., Ahmed, G. A., El-Mageed, M. A. (2018). Efficiency of biotic and abiotic inducers for controlling tomato early blight. Middle East J. 7, 650–670.
Fawe, A., Abou-Zaid, M., Menzies, J. G., Belanger, R. R. (1998). Silicon-mediated accumulation of flavonoid phytoalexins in cucumber. Phytopathol. 88 (5), 396–401. doi: 10.1094/PHYTO.1998.88.5.396
Filizola, P. R. B., Luna, M. A. C., de Souza, A. F., Coelho, I. L., Laranjeira, D., Campos-Takaki, G. M. (2019). Biodiversity and phylogeny of novel Trichoderma isolates from mangrove sediments and potential of biocontrol against Fusarium strains. Microb. Cell fact. 18 (1), 1–14. doi: 10.1186/s12934-019-1108-y
Gondal, A. S., Ijaz, M., Riaz, K., Khan, A. R. (2012). Effect of different doses of fungicide mancozeb) against alternaria leaf blight of tomato in tunnel. J. Plant Pathol. Microb. 3 (125), 3. doi: 10.4172/2157-7471.1000125
González-Fernández, E., Kennedy, R., Osborn, R., Fernández-González, M., Rodríguez-Rajo, F. J. (2021). Botrytis cinerea airborne conidia and their germination ability assessed by immunological methods in a NW Spain vineyard. Agronomy. 11, 1441. doi: 10.3390/agronomy11071441
Guzmán-Guzmán, P., Porras-Troncoso, M. D., Olmedo-Monfil, V., Herrera-Estrella, A. (2019). Trichoderma species, versatile plant symbionts. Phytopathol. 109, 6–16. doi: 10.1094/PHYTO-07-18-0218-RVW
Heflish, A. A., Abdelkhalek, A., Al-Askar, A. A., Behiry, S. I. (2021). Protective and curative effects of Trichoderma asperelloides Ta41 on tomato root rot caused by Rhizoctonia solani Rs33. Agronomy. 11, 1162. doi: 10.3390/agronomy11061162
Hemeda, H. M., Klein, B. P. (1990). Effects of naturally occurring antioxidants on peroxidase activity of vegetable extracts. J. Food Sci. 55, 184–185. doi: 10.1111/j.1365-2621.1990.tb06048.x
Hernádez-Rodrίguez, A., Heydrich-Pérez, M., Acebo-Guerrero, Y., Velazquez-Del, V. M. G., Hernandez-Lauzardo, A. N. (2008). Antagonistic activity of Cuban native rhizobacteria against Fusarium verticillioides (Sacc.) Nirenb. in maize (Zea mays l.). Appl. Soil Ecol. 39 (2), 180–186. doi: 10.1016/j.apsoil.2007.12.008
Iannaccone, F., Alborino, V., Dini, I., Balestrieri, A., Marra, R., Davino, R., et al. (2022). ). In vitro application of exogenous fibrolytic enzymes from Trichoderma spp. to improve feed utilization by ruminants. Agriculture. 12, 573. doi: 10.3390/agriculture12050573
Imran, M., Abo-Elyousr, K. A. M., Mousa, M. A., Saad, M. M. (2022a). Screening and biocontrol evaluation of indigenous native Trichoderma spp. against early blight disease and their field assessment to alleviate natural infection. Egyptian J. Biol. Pest Control. 32 (1), 40. doi: 10.1186/s41938-022-00544-4
Imran, M., Abo-Elyousr, K. A. M., Mousa, M. A., Saad, M. M. (2022b). A study on the synergetic effect of Bacillus amyloliquefaciens and dipotassium phosphate on Alternaria solani causing early blight disease of tomato. Eur. J. Plant Pathol. 162 (1), 63–77. doi: 10.1007/s10658-021-02384-8
Imran, M., Ali, E. F., Abo-Elyousr, K. A. M., Sallam, N. M. A., Khan, M. M. M., Younas, M. W. (2021). Characterization and sensitivity of Botrytis cinerea to benzimidazole and SDHI fungicides and illustration of resistance profile. Aus. Plant Pathol. 50 (5), 589–601. doi: 10.1007/s13313-021-00803-2
Ismail, A. W., Sidkey, N. M., Arafa, R. A., Fathy, R. M., El-Batal, A. I. (2016). Evaluation of in vitro antifungal activity of silver and selenium nanoparticles against Alternaria solani caused early blight disease on potato. Br. Biotechnol. J. 12 (3), 1. doi: 10.9734/BBJ/2016/24155
Jiang, L., Wu, J., Fan, S., Li, W., Dong, L., Cheng, Q., et al. (2015). Isolation and characterization of a novel pathogenesis-related protein gene (gmprp) with induced expression in soybean (Glycine max) during infection with Phytophthora sojae. PloS One 10, e0129932. doi: 10.1371/journal.pone.0129932
Jun, S. Y., Sattler, S. A., Cortez, G. S., Vermerris, W., Sattler, S. E., Kang, C. H. (2018). Biochemical and structural analysis of substrate specificity of a phenylalanine ammonia-lyase. Plant Physiol. 176, 1452–1468. doi: 10.1104/pp.17.01608
Kapsiya, J., Gungula, D. T., Tame, V. T., Bukar, N. (2015). Effects of storage chemicals and packaging systems on physicochemical characteristics of tomato (Solanum lycopersicum l.) fruits. Aascit J. Biosci. 1, 41–46.
Kashyap, A. S., Manzar, N., Nebapure, S. M., Rajawat, M. V. S., Deo, M. M., Singh, J. P., et al. (2022). Unraveling microbial volatile elicitors using a transparent methodology for induction of systemic resistance and regulation of antioxidant genes at expression levels in chili against bacterial wilt disease. Antioxidants 11, 404. doi: 10.3390/antiox11020404
Kashyap, P. L., Solanki, M. K., Kushwaha, P., Kumar, S., Srivastava, A. K. (2020). Biocontrol potential of salt-tolerant Trichoderma and Hypocrea isolates for the management of tomato root rot under saline environment. J. Soil Sci. Plant Nutr. 20, 160–176. doi: 10.1007/s42729-019-00114-y
Kaur, T., Bala, M., Kumar, G., Vyas, A. (2022). Biosynthesis of zinc oxide nanoparticles via endophyte Trichoderma viride and evaluation of their antimicrobial and antioxidant properties. Arch. Microbiol. 204 (10), 1–23. doi: 10.1007/s00203-022-03218-9
Khan, M. R., Chonhenchob, V., Huang, C., Suwanamornlert, P. (2021). Antifungal activity of propyl disulfide from neem (Azadirachta indica) in vapor and agar diffusion assays against anthracnose pathogens (Colletotrichum gloeosporioides and Colletotrichum acutatum) in mango fruit. Microorganisms. 9 (4), 839. doi: 10.3390/microorganisms9040839
Kim, D. S., Kwack, Y., Lee, J. H., Chun, C. (2019). Antimicrobial activity of various parts of tomato plants varied with different solvent extracts. Plant Pathol. J. 35 (2), 149–155. doi: 10.5423/PPJ.OA.07.2018.0132
Koley, S., Mahapatra, S. S., Kole, P. C. (2015). In vitro efficacy of bio-control agents and botanicals on the growth inhibition of Alternaria solani causing early leaf blight of tomato. Int. J. Bio-res Env. Agric. Sci. 1 (3), 114–118.
Kolomiets, J. V., Grygoryuk, I. P., Butsenko, L. M. (2017). Bacterial diseases of tomato plants in terms of open and covered growing of Ukraine. Annal. Agr. Sci. 15, 213–216. doi: 10.1016/j.aasci.2017.05.010
Kumar, S., Chandra, R., Behera, L., Keswani, C., Sansinenea, E. (2022). Dual Trichoderma consortium mediated elevation of systemic defense response against early blight in potato. Eur. J. Plant Pathol. 162, 681–696. doi: 10.1007/s10658-021-02431-4
Kumar, K., Khan, P. (1982). Peroxidase and polyphenol oxidase in excised ragi (Eleusine corocana cv PR 202) leaves during senescence. Ind. J. Exp. Biol. 20, 412–416.
Mahmoud, G. A. E., Abdel-Sater, M. A., Al-Amery, E., Hussein, N. A. (2021). Controlling alternaria cerealis MT808477 tomato phytopathogen by Trichoderma harzianum and tracking the plant physiological changes. Plants. 10 (9), 1846. doi: 10.3390/plants10091846
Manzar, N., Kashyap, A. S., Goutam, R. S., Rajawat, M. V. S., Sharma, P. K., Sharma, S. K., et al. (2022). Trichoderma: advent of versatile biocontrol agent, its secrets and insights into mechanism of biocontrol potential. Sustainability 14, 12786. doi: 10.3390/su141912786
Manzar, N., Singh, Y., Kashyap, A. S., Sahu, P. K., Rajawat, S. M. V., Bhowmik, A., et al. (2021). Biocontrol potential of native Trichoderma spp. against anthracnose of great millet (Sorghum bicolour l.) from tarai and hill regions of India. Biol. Control. 152, 104474.
Mayo-Prieto, S., Marra, R., Vinale, F., Rodríguez-González, Á., Woo, S. L., Lorito, M., et al. (2019). Effect of Trichoderma velutinum and Rhizoctonia solani on the metabolome of bean plants (Phaseolus vulgaris l.). Int. J. Mol. Sci. 20 (3), 549. doi: 10.3390/ijms20030549
Mazrou, Y. S., Makhlouf, A. H., Elseehy, M. M., Awad, M. F., Hassan, M. M. (2020). Antagonistic activity and molecular characterization of biological control agent Trichoderma harzianum from Saudi Arabia. Egyptian J. Biol. Pest Control. 30 (1), 1–8. doi: 10.1186/s41938-020-0207-8
McNally, D. J., Wurms, K. V., Labbe, C., Belanger, R. R. (2003). Synthesis of c-glycosyl flavonoids phytoalexins as a site-specific response to fungal penetration in cucumber. Physiol. Mol. Plant Pathol. 63 (6), 293–303. doi: 10.1016/j.pmpp.2004.03.005
Meda, A., Lamien, C. E., Romito, M., Millogo, J., Nacoulma, O. G. (2005). Determination of the total phenolic, flavonoid and proline contents in Burkina fasan honey, as well as their radical scavenging activity. Food Chem. 91 (3), 571–577. doi: 10.1016/j.foodchem.2004.10.006
Mendoza-Mendoza, A., Zaid, R., Lawry, R., Hermosa, R., Monte, E., Horwitz, B. A., et al. (2018). Molecular dialogues between Trichoderma and roots, role of the fungal secretome. Fungal Biol. Rev. 32 (2), 62–85. doi: 10.1016/j.fbr.2017.12.001
Mulatu, A., Megersa, N., Tolcha, T., Alemu, T., Vetukuri, R. R. (2022). Antifungal compounds, GC-MS analysis and toxicity assessment of methanolic extracts of Trichoderma species in an animal model. PLOSONE 17 (9), e0274062. doi: 10.1371/journal.pone.0274062
Narware, J., Singh, S. P., Manzar, N., Kashyap, A. S. (2023). Biogenic synthesis, characterization, and evaluation of synthesized nanoparticles against the pathogenic fungus Alternaria solani. Front. Microbiol. 14. doi: 10.3389/fmicb.2023.1159251
Nurbailis, N., Djamaan, A., Rahma, H., Liswarni, Y. (2019). Potential of culture filtrate from trichoderma spp. as biofungicide to Colletotrichum gloeosporioides causing anthracnose disease in chili. J. Biol. Divers. 20 (10), 2915–2920. doi: 10.13057/biodiv/d201020
Pasche, J., Wharam, C., Gudmestad, N. (2004). Shift in sensitivity of Alternaria solani in response to QoI fungicides. Plant Dis. 88 (2), 181–187. doi: 10.1094/PDIS.2004.88.2.181
Pawel, S., Urszula, K., Bederska-Błaszczyk, M., Agnieszka, G. (2020). Canavanine increases the content of phenolic compounds in tomato (Solanum lycopersicum l.) roots. Plants. 9 (11), 1595. doi: 10.3390/plants9111595
Peer, W. A., Murphy, A. S. (2006). “Flavonoids as signal molecules, targets of flavonoid action,” in The science of flavonoids. Eds. Peer, W. A., Murphy, A. S. (New York: Springer), 239–268. doi: 10.1007/978-0-387-28822-2_9
Pereira, D. M., Valentão, P., Pereira, J. A., Andrade, P. B. (2009). Phenolics, from chemistry to biology. Molecules. 14 (6), 2202–2211. doi: 10.3390/molecules14062202
Quideau, S., Deffieux, D., Douat-Casassus, C., Pouységu, L. (2011). Plant polyphenols, chemical properties, biological activities, and synthesis. Angew Chem. Int. Ed. 50, 586–621. doi: 10.1002/anie.201000044
Rahman, M., Sabir, A., Akter, J., Khan, M., Mohi-Ud-Din, M., Miah, G., et al. (2018). Plant probiotic bacteria Bacillus and Paraburkholderia improve growth, yield and content of antioxidants in strawberry fruit. Sci. Rep. 8 (1), 2504. doi: 10.1038/s41598-018-20235-1
Rajani, P., Rajasekaran, C., Vasanthakumari, M. M., Olsson, S. B., Ravikanth, G., Shaanker, R. U. (2021). Inhibition of plant pathogenic fungi by endophytic Trichoderma spp. through mycoparasitism and volatile organic compounds. Microbiol. Res. 242, 126595. doi: 10.1016/j.micres.2020.126595
Reithner, B., Schuhmacher, R., Stoppacher, N., Pucher, M., Brunner, K., Zeilinger, S. (2007). Signaling via the Trichoderma atroviride mitogen-activated protein kinase Tmk1 differentially affects mycoparasitism and plant protection. Fungal Genet. Biol. 44, 1123–1133. doi: 10.1016/j.fgb.2007.04.001
Sarsaiya, S., Jain, A., Fan, X., Jia, Q., Xu, Q., Shu, F., et al. (2020). New insights into detection of a dendrobine compound from a novel endophytic Trichoderma longibrachiatum strain and its toxicity against phytopathogenic bacteria. Front. Microbiol. 11. doi: 10.3389/fmicb.2020.00337
Sasabe, M., Takeuchi, K., Kamoun, S., Ichinose, Y., Govers, F., Toyoda, K., et al. (2000). Independent pathways leading to apoptotic cell death, oxidative burst and defense gene expression in response to elicitin in tobacco cell suspension culture. Eur. J. Biochem. 267 (16), 5005–5013. doi: 10.1046/j.1432-1327.2000.01553.x
Schulz-Bohm, K., Martín-Sánchez, L., Garbeva, P. (2017). Microbial volatiles, small molecules with an important role in intra-and inter-kingdom interactions. Front. Microbiol. 8. doi: 10.3389/fmicb.2017.02484
Senthil, K. A., Kannathasan, K., Venkatesalu, V. (2008). Chemical constituents and larvicidal property of the essential oil of Blumea mollis (D. don) merr. against Culex quinquefasciatus. Parasitol. Res. 103, 959–962. doi: 10.1007/s00436-008-1085-2
Siddiquee, S., Cheong, B. E., Taslima, K., Kausar, H., Hasan, M. M. (2012). Separation and identification of volatile compounds from liquid cultures of Trichoderma harzianum by GC-MS using three different capillary columns. J. Chromatogr. Sci. 50 (4), 358–367. doi: 10.1093/chromsci/bms012
Soesanto, L., Sastyawan, M. W. R., Nurliana, N., Mugiastuti, E. (2022). Potency of two Trichoderma harzianum isolates in liquid and solid organic formula for controlling bacterial wilt on tomato in the field. J. Trop. Plant Pests Dis. 22, 116–125. doi: 10.23960/jhptt.222116-125
Sornakili, A., Thankappan, S., Sridharan, A. P., Nithya, P., Uthandi, S. (2020). Antagonistic fungal endophytes and their metabolite-mediated interactions against phytopathogens in rice. Physiol. Mol. Plant Pathol. 112, 101525. doi: 10.1016/j.pmpp.2020.101525
Sriwati, R., Chamzurn, T., Soesanto, L., Munazhirah, M. (2019). Field application of Trichoderma suspension to control cacao pod rot (Phytophthora palmivora). Agrivita. J. Agr. Sci. 41 (1), 175–182. doi: 10.17503/agrivita.v41i1.2146
Steel, R. G. D., Torrie, J. H., Dickey, D. A. (1996). Principles and procedures of statistics, a biometric approach. 3rd ed (New York, NY, USA: McGraw Hill Book Co. Inc.).
Stoppacher, N., Kluger, B., Zeilinger, S., Krska, R., Schuhmacher, R. (2010). Identification and profiling of volatile metabolites of the biocontrol fungus Trichoderma atroviride by HS-SPME-GC-MS. J. Microbiol. Methods 81 (2), 187–193. doi: 10.1016/j.mimet.2010.03.011
Stracquadanio, C., Quiles, J. M., Meca, G., Cacciola, S. O. (2020). Antifungal activity of bioactive metabolites produced by Trichoderma asperellum and Trichoderma atroviride in liquid medium. J. Fungi. 6 (4), 263. doi: 10.3390/jof6040263
Tomazoni, E. Z., Pauletti, G. F., da Silva Ribeiro, R. T., Moura, S., Schwambach, J. (2017). In vitro and in vivo activity of essential oils extracted from Eucalyptus staigeriana, Eucalyptus globulus and Cinnamomum camphora against Alternaria solani sorauer causing early blight in tomato. Scientia Hortic. 223, 72–77. doi: 10.1016/j.scienta.2017.04.033
Tripathi, R., Keswani, C., Tewari, R. (2021). Trichoderma koningii enhances tolerance against thermal stress by regulating ROS metabolism in tomato (Solanum lycopersicum l.) plants. J. Plant Inter. 16 (1), 116–125. doi: 10.1080/17429145.2021.1908634
Vinale, F., Nigro, M., Sivasithamparam, K., Flematti, G., Ghisalberti, E. L., Ruocco, M., et al. (2013). Harzianic acid, a novel siderophore from Trichoderma harzianum. FEMS Microbiol. Lett. 347 (2), 123–129. doi: 10.1111/1574-6968.12231
Vukelić, I. D., Prokić, L. T., Racić, G. M., Pešić, M. B., Bojović, M. M., Sierka, E. M., et al. (2021). Effects of Trichoderma harzianum on photosynthetic characteristics and fruit quality of tomato plants. Int. J. Mol. Sci. 22 (12), 6961. doi: 10.3390/ijms22136961
Wang, W., Fang, Y., Imran, M., Hu, Z., Zhang, S., Huang, Z., et al. (2021). Characterization of the field fludioxonil resistance and its molecular basis in Botrytis cinerea from shanghai province in China. Microorganisms. 9 (2), 266. doi: 10.3390/microorganisms9020266
Xie, L., Zang, X., Cheng, W., Zhang, Z., Zhou, J., Chen, M., et al. (2021). Harzianic acid from Trichoderma afroharzianum is a natural product inhibitor of acetohydroxyacid synthase. J. Am. Chem. Soc. 143 (25), 9575–9584. doi: 10.1021/jacs.1c03988
Yassin, M. T., Mostafa, A. A. F., Al-Askar, A. A. (2022). In vitro antagonistic activity of Trichoderma spp. against fungal pathogens causing black point disease of wheat. J. Taibah Uni. Sci. 16 (1), 57–65. doi: 10.1080/16583655.2022.2029327
Yassin, M. T., Mostafa, A. A. F., Al-Askar, A. A., Sayed, S. R., Rady, A. M. (2021). Antagonistic activity of Trichoderma harzianum and Trichoderma viride strains against some fusarial pathogens causing stalk rot disease of maize, in vitro. J. King Saud Uni. Sci. 33 (3), 101363. doi: 10.1016/j.jksus.2021.101363
Yoshida, K., Kaothien, P., Matsui, T., Kawaoka, A., Shinmyo, A. (2003). Molecular biology and application of plant peroxidase genes. App. Microbiol. Biotechnol. 60 (6), 665–670. doi: 10.1007/s00253-002-1157-7
Youssef, S. A., Tartoura, K. A., Abdelraouf, G. A. (2016). Evaluation of Trichoderma harzianum and Serratia proteamaculans effect on disease suppression, stimulation of ROS-scavenging enzymes and improving tomato growth infected by Rhizoctonia solani. Biol. Control. 100, 79–86. doi: 10.1016/j.biocontrol.2016.06.001
Zafra, G., Cortés-Espinosa, D. V. (2015). Biodegradation of polycyclic aromatic hydrocarbons by Trichoderma species, a mini review. Environ. Sci. Pollut. Res. Int. 22, 19426–19433. doi: 10.1007/s11356-014-3357-y
Zehra, A., Meena, M., Dubey, M. K., Aamir, M., Upadhyay, R. S. (2017). Activation of defense response in tomato against fusarium wilt disease triggered by Trichoderma harzianum supplemented with exogenous chemical inducers (SA and MeJA). Braz. J. Bot. 40 (3), 651–664. doi: 10.1007/s40415-017-0382-3
Zhang, C., Diao, Y., Wang, W., Hao, J., Imran, M., Duan, H., et al. (2017). Assessing the risk for resistance and elucidating the genetics of Colletotrichum truncatum that is only sensitive to some DMI fungicides. Front. Microbiol. 8. doi: 10.3389/fmicb.2017.01779
Zhang, C., Imran, M., Liu, M., Li, Z., Gao, H., Duan, H., et al. (2020b). Two point mutations on CYP51 combined with induced expression of the target gene appeared to mediate pyrisoxazole resistance in Botrytis cinere. Front. Microbiol. 11. doi: 10.3389/fmicb.2020.01396
Zhang, C., Imran, M., Xiao, L., Hu, Z., Li, G., Zhang, F., et al. (2021). Difenoconazole resistance shift in Botrytis cinerea from tomato in China associated with inducible expression of CYP51. Plant Dis. 105 (2), 400–407. doi: 10.1094/PDIS-03-20-0508-RE
Keywords: Alternaria solani, culture filtrates, antioxidants, volatile metabolites, biocontrol
Citation: Imran M, Abo-Elyousr KAM, Mousa MAA and Saad MM (2023) Use of Trichoderma culture filtrates as a sustainable approach to mitigate early blight disease of tomato and their influence on plant biomarkers and antioxidants production. Front. Plant Sci. 14:1192818. doi: 10.3389/fpls.2023.1192818
Received: 23 March 2023; Accepted: 19 May 2023;
Published: 17 July 2023.
Edited by:
Sara Álvarez, Instituto Tecnológico Agrario de Castilla y León, SpainReviewed by:
Elsherbiny A. Elsherbiny, Mansoura University, EgyptAbhijeet Shankar Kashyap, National Bureau of Agriculturally Important Microorganisms (ICAR), India
Copyright © 2023 Imran, Abo-Elyousr, Mousa and Saad. This is an open-access article distributed under the terms of the Creative Commons Attribution License (CC BY). The use, distribution or reproduction in other forums is permitted, provided the original author(s) and the copyright owner(s) are credited and that the original publication in this journal is cited, in accordance with accepted academic practice. No use, distribution or reproduction is permitted which does not comply with these terms.
*Correspondence: Muhammad Imran, aW1yYW5wYXRob2xvZ2lzdEBjYXUuZWR1LmNu; Kamal A. M. Abo-Elyousr, a2FhYm9lbHlvdXNyQGFnci5hdS5lZHUuZWc=; Magdi A. A. Mousa, bWFtb3VzYUBrYXUuZWR1LnNh; Maged M. Saad, bWFnZWQuc2FhZEBrYXVzdC5lZHUuc2E=