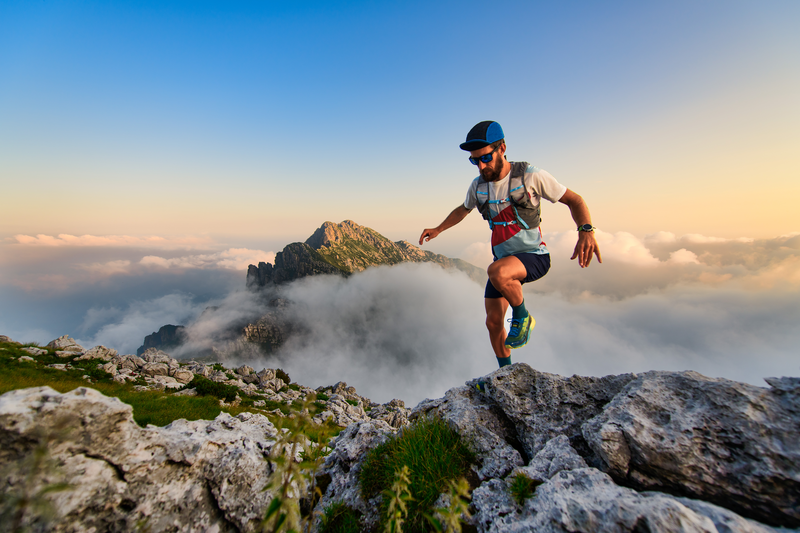
94% of researchers rate our articles as excellent or good
Learn more about the work of our research integrity team to safeguard the quality of each article we publish.
Find out more
OPINION article
Front. Plant Sci. , 31 May 2023
Sec. Plant Development and EvoDevo
Volume 14 - 2023 | https://doi.org/10.3389/fpls.2023.1187634
This article is part of the Research Topic Hormone Metabolism and Function in Plant Developmental Plasticity View all 3 articles
Plants adjust their growth rapidly in response to various stimuli, thus improving their fitness to ever-changing environments. In most cases, including plasma membrane (PM) depolarization, H+-pump activation, transient cytosolic Ca2+, and pH changes, are regulated by the plant phytohormone auxin (Friml, 2022). Moreover, auxin also induces fast (within 2 min) and reversible primary root elongation inhibition, which particularly requires the cytoplasm localized AFB1 receptor together with TIR1 and other AFBs, to operate in an unexpected non-transcriptional manner (Fendrych et al., 2018; Prigge et al., 2020; Dubey et al., 2021; Li et al., 2021; Serre et al., 2021; Chen et al., 2023; Dubey et al., 2023).
The influx auxin carrier AUX1 imports IAA− into cells with two H+, and passive diffusion also delivers auxin into the cells. On one hand, TIR1/AFB receptors perceive auxin to promote cellular H+ influx across the PM through so far undiscovered channels or transporters/antiporters, resulting in rapid apoplast alkalinization. The Ca2+ transient contributes to H+ influx (Li et al., 2021; Li et al., 2022a); however, it remains elusive whether undefined Ca2+ channels are required for H+ influx. On the other hand, the auxin-TIR1/AFB module acts through CYCLIC NUCLEOTIDE-GATED CHANNEL 14 (CNGC14), a Ca2+ channel, to rapidly stimulate PM depolarization (Serre et al., 2021; Serre et al., 2022). As a consequence, primary root elongation is repressed rapidly (Fendrych et al., 2018; Li et al., 2021; Serre et al., 2021; Serre et al., 2022). Recently, it has been suggested that adenylate cyclase (AC) activity is essential for TIR1/AFB receptor function and auxin perception (Qi et al., 2022). Auxin stimulates the AC activity to produce cAMP, thus executing its long-term effect on primary root elongation. Notably, AC activity is not required for auxin-TIR1/AFB signaling-mediated fast primary root elongation inhibition (Qi et al., 2022). Notably, the involvement of cGMP signaling in the auxin-induced fast primary root elongation inhibition requires further investigation.
In addition to TIR1/AFB perception machinery, apoplastic and PM-localized auxin signaling components have been proposed for years; AUXIN BINDING PROTEIN 1 (ABP1) and TRANSMEMBRANE KINASEs (TMKs) are excellent candidates (Cao et al., 2019; Lin et al., 2021; Friml et al., 2022). Remarkably, TMK1 transmits the auxin signal in an unraveled manner to phosphorylate AHA2, a H+-ATPase, at the well-known Thr947 activation site, resulting in apoplast acidification and promotion of primary root elongation (Li et al., 2021). Conversely, ABP1 has recently been reported to mediate auxin-induced fast (2 min) phospho-response together with TMK1 (Friml et al., 2022). However, the loss-of-function abp1 mutant responds normally to auxin in fast primary root elongation inhibition (Li et al., 2021). Nonetheless, further analysis of abp1 tmk1 double mutant in the fast auxin response is further needed (Rodriguez et al., 2022). Collectively, the TIR1/AFB and cell surface TMK1 kinase mediate antagonistic auxin signaling to fine-tune primary root elongation rapidly, but the mechanisms are still beyond our current understanding (Figure 1).
Figure 1 Auxin and RALF1 mediate rapid Arabidopsis primary root elongation inhibition via distinct routes. (A) The AUX1 auxin influx transporter and passive diffusions deliver auxin into the cells, then intracellular auxin is mainly perceived by cytoplasm AFB1 receptor, together with other SCFTIR1/AFB receptors in the nucleus to trigger a rapid CNGC14-mediated Ca2+ influx through unknown factors. The Ca2+ transient contributes to the H+ influx into cells across the PM via so far unidentified channels or transporters/antiporters, which ultimately leads to apoplast alkalinization and PM depolarization. As a result, primary root elongation is inhibited rapidly. In contrast, cell surface localized TMK1 kinase recognizes auxin via an undefined manner to phosphorylate AHA2, a H+-ATPase, to pump H+ to apoplast, resulting in apoplast acidification and promotion of primary root elongation. Hence, the SCFTIR1/AFB receptor and TMK1 kinase antagonize the auxin-dependent rapid primary root elongation inhibition. (B) RALF1 and its corresponding receptor FER may act through undefined channels or transporters/antiporters to trigger apoplast alkalinization, resulting in rapid and reversible primary root elongation inhibition. (C) The RALF1-FER module promotes auxin biosynthesis via increasing YUCCAs expression, thus inducing the canonical nucleus SCFTIR1/AFB transcriptional pathway for its long-term effect on primary root elongation inhibition. (D) Unmapped Ca2+ channels and signaling pathways that may be involved in RALF1-FER mediated both rapid and sustained effect on primary root elongation inhibition. (E) RALF1 acts via FER receptor to regulate undefined channels or transporters/antiporters to trigger PM depolarization in an unknown manner for the regulation of rapid response in primary root. AHA2, H+-ATPase 2; ARF, AUXIN RESPONSE FACTOR; CNGC14, Cyclic NUCLEOTIDE-GATED CHANNEL 14; PM, plasma membrane; TIR1/AFB, TRANSPORT INHIBITOR RESPONSE1/AUXIN-SIGNALLING F-BOX PROTEIN; TMK1, TRANSMEMBRANE KINASE 1; AUX1, AUXIN RESISTANT 1; CaM, CALMODULIN; CMLs, CaM-LIKE PROTEINs; CDPKs, Ca2+-DEPENDNT PROTEIN KINASEs; CBLs, CALCINEURIN B-LIKE PROTEINs; CIPKs, CBL-INTERACTING PROTEIN KINASEs; CPK9, CALCIUM-DEPENDENT PROTEIN KINASE 9; Pi, phosphorylation.
Besides apoplast alkalinization, other cellular processes such as cortical microtubule re-orientation (Adamowski et al., 2019) and vacuolar expansion (Dünser et al., 2019; Dünser et al., 2022) have been implicated in cell elongation. In elongating cells, the orientation of microtubules can either limit or allow cell expansion in a certain direction. Vacuoles are unique plant organelles, and their dimensions play essential roles in defining plant cell expansion rates. During cellular elongation, the size of the vacuole and its relative occupancy of the cell dramatically increase to 80%–90% of the cellular volume, which leads to rapid cellular growth (Dünser et al., 2019; Dünser et al., 2022). Therefore, microtubule re-orientation and vacuolar expansion may potentially be part of the machinery in rapid primary root elongation inhibition. Evidence has been shown that auxin does not rapidly induce microtubule re-orientation or vacuolar expansion (Li et al., 2021).
Ca2+ signaling consists of an array of receptors that perceive extracellular cues and Ca2+ channels that transport Ca2+ into the cells, formulating a specific Ca2+ signature (Tang et al., 2020). CALMODULIN (CaM), CaM-LIKE PROTEINs (CMLs), Ca2+-DEPENDNT PROTEIN KINASEs (CDPKs), and CALCINEURIN B-LIKE PROTEINs (CBLs) have been identified in land plants as Ca2+ sensors. Plant-specific CBL-INTERACTING PROTEIN KINASEs (CIPKs) act as major downstream signaling components of the CBL sensors to orchestrate central CBL-CIPK signaling networks and fine-tune plant adaptive growth in response to developmental and environmental cues (Tang et al., 2020). Auxin immediately triggers ion fluxes across the PM in root cells. In particular, transient increased cytosolic Ca2+ and increased apoplastic pH are detected less than 30 s after auxin treatment (Li et al., 2021; Serre et al., 2022). Therefore, the Ca2+ transient and external pH changes represent very early responses to auxin. Most likely, auxin acts via TIR1/AFB receptors to activate CNGC14 channel (Shih et al., 2015; Dindas et al., 2018; Li et al., 2021; Serre et al., 2022). The increased Ca2+ thus transiently initiates fast H+ influx and apoplast alkalinization. Consequently, primary root elongation is rapidly inhibited (Figure 1) (Li et al., 2021; Serre et al., 2022). How auxin regulates the well-known Ca2+ signaling pathways to mediate fast root elongation regulation (Tang et al., 2020), however, is largely unknown. On the other hand, it is unclear whether so far undiscovered channels or transporters/antiporters are involved in auxin-mediated fast H+ influx into the cells, as auxin induces the K+ efflux (Li et al., 2021; Li et al., 2022a).
Auxin rapidly arrests primary root elongation, which is too quick to be associated with transcriptional regulation, suggesting that this rapid elongation regulation may occur at the protein level (Fendrych et al., 2018; Li et al., 2021; Serre et al., 2021). Protein (de)phosphorylation is a prominent post-translational modification involved in many signaling pathways (Vu et al., 2018), and auxin has been reported to provoke a fast (2 min) protein phosphorylation through the receptors, TIR1/AFB, ABP1, and TMK1 (Han et al., 2021; Lin et al., 2021; Friml et al., 2022; Roosjen et al., 2022; Kuhn et al., 2022). Indeed, this fast protein phosphorylation analysis in root tips has successfully led to the identification of AHA2 that antagonizes TIR1/AFB-mediated rapid primary root elongation modulation (Han et al., 2021; Li et al., 2021). Additionally, a RAF-like protein kinase was identified as a central mediator of fast auxin-mediated phosphorylation, but its role in the fast responses of primary root needs further investigation in the future (Kuhn et al., 2022). Together with the fast phospho-response in the auxin receptor mutants (Lin et al., 2021; Friml et al., 2022; Kuhn et al., 2022; Roosjen et al., 2022), it will provide new access to identify unprecedented auxin-mediated machinery that enables plants to adjust primary root elongation rapidly through the non-canonical auxin signaling cascade. We also have to note that auxin may also trigger the rapid flow of K+ and other ions across the membrane (Ward et al., 2009; Li et al., 2021) and cell wall extensibility (Du et al., 2020), which will ultimately lead to the primary root elongation inhibition. A further investigation of ion exchange and cell wall plasticity in the auxin-mediated fast responses of primary root at the translational or post-translational level needs to be carried out.
The small cysteine-rich polypeptide, RAPID ALKALINIZXATION FACTOR1 (RALF1), has also been shown to rapidly (within 2 min) arrest primary root elongation in a similar way to auxin (Li et al., 2021; Li et al., 2022b). The application of the synthetic RALF1 peptide may also generate a rapid net H+ influx across the PM into cells; nevertheless, this rapid net H+ influx and primary root elongation are abolished in FERONIA (FER) receptor mutant (Gjetting et al., 2020; Li et al., 2022b). Their result further suggests that the RALF1-FER module functions in a non-transcriptional manner to actuate rapid apoplast alkalinization and root elongation inhibition (Li et al., 2022b). It is implausible for RALF1 to directly regulate AHAs proton pump, as the tested aha mutants show normal responses to RALF1 peptide treatment within a time scale of 0 to 6 h, suggesting that the existence of so far undiscovered channels or transporters/antiporters is responsible for RALF1-inhibited rapid primary root elongation (Li et al., 2021; Li et al., 2022b). Unexpectedly, RALF1 and auxin independently suppress primary root elongation rapidly, supported by the fact that fer responds normally to short-term auxin treatment, and tir triple and tmk mutants respond normally to RALF1 peptide (Li et al., 2021; Li et al., 2022b). Notably, RALF1-FER modulates the biosynthesis of auxins via elevating the expression of auxin biosynthesis gene YUCCAs, and the accumulated auxin then inhibits primary root elongation acts through TIR1/AFB signaling in a long-term dimension (Li et al., 2022b). Thus, RALF1 exhibits both fast and sustained effects on primary root elongation through different mechanisms (Figure 1).
FER plays multiple roles in plant development, including cortical microtubule re-orientation (Malivert et al., 2021; Lin et al., 2022; Tang et al., 2022) and intracellular expansion of the vacuole (Dünser et al., 2019). However, whether microtubule re-orientation or vacuole expansion is involved in RALF1-FER module-mediated rapid response requires further investigation. Additionally, it is also necessary to examine whether RALF1-FER initiates rapid PM depolarization in parallel with apoplast alkalinization and, if so, what the underlying mechanisms are (Figure 1).
A fast RALF1-induced PM protein phosphorylation assay has led to the identification of CALCIUM-DEPENDENT PROTEIN KINASE 9 (CPK9) as a strong candidate to enhance cytoplasmic calcium concentrations (Haruta et al., 2014). NORTIA (NTA) functions as a Ca2+ sensor in FER-mediated pollen tube reception (Gao et al., 2022). Therefore, it is an open question to test the involvement of CNGC14, NTA, or other unmapped Ca2+ channels and signaling components (Tang et al., 2020) in RALF1-FER-dependent fast and sustained primary root elongation inhibition (Figure 1).
FER is a Ser/Thr receptor kinase that phosphorylates itself or substrates in a context-specific manner (Zhu et al., 2021). RALF1 induces FER phosphorylation within 5 min, indicating uncharacterized rapid protein phosphorylation networks to operate the rapid effect on primary root elongation through RALF1-FER signaling (Haruta et al., 2014). The newly developed timsTOF Pro mass spectrometer, powered by parallel accumulation serial fragmentation (PASEF) acquisition mode and trapped ion mobility spectrometry (TIMS) technology, paves the way to 4D-proteomics (Loginov et al., 2021). This approach will provide essential information on fast protein phosphorylation and will contribute to identify undefined robust protein networks involved in auxin or RALF1-FER module in both fast and sustained primary root elongation inhibition.
Unlike animals, plants cannot run when they meet environmental stressors. Rapidly adjusting their growth is one of the most effective strategies to avoid the negative effects of diverse stressors on development and growth. The disclosed fast (within 2 min) and reversible effects of auxin and RALF1 peptide provide mechanistic insights into rapid primary root elongation regulation. Nevertheless, open questions remain to be unanswered. The key unresolved question is how TIR1/AFB and TMK1 kinase confer primary root rapid reactions, and which downstream players function in non-canonical auxin actions. Phosphoproteomics analysis has revealed that many proteins are rapidly phosphorylated independently of the TIR1/AFB pathway (Han et al., 2021). Only a small portion of the identified proteins overlaps with auxin-mediated transcriptional regulation (Han et al., 2021; Friml et al., 2022; Kuhn et al., 2022; Roosjen et al., 2022). Therefore, an in-depth analysis of fast phosphorylation assays in related receptor mutants (Han et al., 2021; Friml et al., 2022; Kuhn et al., 2022; Roosjen et al., 2022) will help identify the downstream players that are responsible for auxin-mediated fast primary root elongation regulation. On the other hand, it would be appealing to uncover the kinase cascades that endow the rapidity of the TIR1/AFB-TMK-dependent non-canonical auxin signaling or the RALF1-FER dependent signaling, although only a few kinases have been confirmed in these fast signaling pathways (Haruta et al., 2014; Kubeš and Napier, 2019; Kuhn et al., 2022). We have to note that the rapid elongation inhibition of Arabidopsis primary root depends not only on the AFB1 subcellular localization but also on specific AFB1 protein properties (Prigge et al., 2020; Chen et al., 2023; Dubey et al., 2023). Furthermore, the auxin effect on endocytic PIN trafficking is also a rapid, non-transcriptional, and TIR1/AFB-independent biological process (Friml, 2022). Hence, auxin may also regulate its target protein dynamics through endocytic trafficking to execute its rapid inhibitory effect on primary root elongation.
RALF1 also regulates dynamics and partitioning of FER at PM via clathrin-mediated endocytosis (CME), and the clc2-1/3-1 mutant, impaired in CME, shows resistance to RALF1 peptide in root growth inhibition (Yu et al., 2020). It is likely that RALF1 may trigger a rapid dynamic change of FER and FER-targeted PM proteins to initiate the early signals (such as pH, ROS, Ca2+) and MITOGEN-ACTIVATED PROTEIN KINASE (MAPK) cascades to exert its rapidness (Zhu et al., 2021), but this requires detailed investigation. Moreover, FER shows differential affinity to RALF peptides, and the involvement of other RALFs in this rapid primary root response remains unknown. The RALF1-FER signaling in the plant kingdom has been revealed, and RALFs and their (co)receptors co-evolved from bryophytes to seed plants (Zhu et al., 2021). Hence, it is intriguing to reveal that whether the RALF1-FER signaling in plant rapid primary root elongation is evolutionary conserved. Additionally, it is worth examining whether the FER interacting proteins (Du et al., 2016) are required for both fast and long-term effects of RALF1. Another crucial unanswered question is how RALF1 regulates FER kinase activity under different conditions or in diverse cell types, thus coordinating its biphasic effect on primary root elongation. Its potential players and the underlying mechanisms for the rapid activation or inactivation of RALF1-FER signaling remain largely unclear. It is also puzzling why plants utilize two independent pathways to rapidly inhibit primary root elongation via regulating H+ influx (Figure 1). Also, it is not clear at which physiological circumstances plants awaken RALF1-FER or auxin signaling, and how these independent pathways are integrated to adjust Arabidopsis primary root elongation rapidly. The answers to these fundamental but mysterious questions would assist in better elucidating the unprecedented auxin and RALF1-FER regulatory networks and provide a novel toolbox to improve agronomic traits.
All authors listed have made a substantial, direct, and intellectual contribution to the work and approved it for publication.
This work is supported by funding from Jiangxi Agricultural University (9232308314), the Education Department of Jiangxi Province (GJJ210409), and the Science and Technology Department of Jiangxi Province (20223BCJ25037) to HH.
We thank Dr. Jakub Hajný (Palacký University) and Prof. Yuzhou Zhang (Northwest A&F University) for their critical comments on this manuscript. We also thank you the editor and reviewer for their constructive comments to improve our manuscript.
The authors declare that the research was conducted in the absence of any commercial or financial relationships that could be construed as a potential conflict of interest.
All claims expressed in this article are solely those of the authors and do not necessarily represent those of their affiliated organizations, or those of the publisher, the editors and the reviewers. Any product that may be evaluated in this article, or claim that may be made by its manufacturer, is not guaranteed or endorsed by the publisher.
Adamowski, M., Li, L., Friml, J. (2019). Reorientation of cortical microtubule arrays in the hypocotyl of arabidopsis thaliana is induced by the cell growth process and independent of auxin signaling. Int. J. Mol. Sci. 20, 3337. doi: 10.3390/ijms20133337
Cao, M., Chen, R., Li, P., Yu, Y., Zheng, R., Wang, X., et al. (2019). TMK1-mediated auxin signalling regulates differential growth of the apical hook. Nature 568, 240–243. doi: 10.1038/s41586-019-1069-7
Chen, H., Li, L., Qi, L., Friml, J. (2023). Distinct functions of TIR1 and AFB1 receptors in auxin signalling. Preprint at bioRxiv. doi: 10.1101/2023.01.05.522749
Dindas, J., Scherzer, S., Roelfsema, M. R. G., von Meyer, K., Müller, H. M., Al-Rasheid, K. A. S., et al. (2018). AUX1-mediated root hair auxin influx governs SCFTIR1/AFB-type Ca2+ signaling. Nat. Commun. 9, 1174. doi: 10.1038/s41467-018-03582-5
Du, C., Li, X., Chen, J., Chen, W., Li, B., Li, C., et al. (2016). Receptor kinase complex transmits RALF peptide signal to inhibit root growth in arabidopsis. Proc. Natl. Acad. Sci. U. S. A. 113, E8326–E8334. doi: 10.1073/pnas.1609626113
Du, M., Spalding, E. P., Gray, W. M. (2020). Rapid auxin-mediated cell expansion. Annu. Rev. Plant Biol. 71, 379–402. doi: 10.1146/annurev-arplant-073019-025907
Dubey, S. M., Han, S., Stutzmanm, N., Prigge, M. J., Medvecká, E., Platre, M. P., et al. (2023). The AFB1 auxin receptor controls the cytoplasmic auxin response pathway in. Preprint at bioRxiv. doi: 10.1101/2023.01.04.522696
Dubey, S. M., Serre, N. B. C., Oulehlová, D., Vittal, P., Fendrych, M. (2021). No time for transcription-rapid auxin responses in plants. Cold spring harb. Perspect. Biol. 13, a039891. doi: 10.1101/cshperspect.a039891
Dünser, K., Gupta, S., Herger, A., Feraru, M. I., Ringli, C., Kleine-Vehn, J. (2019). Extracellular matrix sensing by FERONIA and leucine-rich repeat extensins controls vacuolar expansion during cellular elongation in Arabidopsis thaliana. EMBO J. 38, e100353. doi: 10.15252/embj.2018100353
Dünser, K., Schöller, M., Rößling, A. K., Löfke, C., Xiao, N., Pařízková, B., et al. (2022). Endocytic trafficking promotes vacuolar enlargements for fast cell expansion rates in plants. Elife 11, e75945. doi: 10.7554/eLife.75945
Fendrych, M., Akhmanova, M., Merrin, J., Glanc, M., Hagihara, S., Takahashi, K., et al. (2018). Rapid and reversible root growth inhibition by TIR1 auxin signalling. Nat. Plants 4, 453–459. doi: 10.1038/s41477-018-0190-1
Friml, J. (2022). Fourteen stations of auxin. Cold Spring Harb. Perspect. Biol. 14, a039859. doi: 10.1101/cshperspect.a039859
Friml, J., Gallei, M., Gelová, Z., Johnson, A., Mazur, E., Monzer, A., et al. (2022). ABP1-TMK auxin perception for global phosphorylation and auxin canalization. Nature 609, 575–581. doi: 10.1038/s41586-022-05187-x
Gao, Q., Wang, C., Xi, Y., Shao, Q., Li, L., Luan, S. A. (2022). Receptor-channel trio conducts Ca2+ signalling for pollen tube reception. Nature 607 (7919), 534–539. doi: 10.1038/s41586-022-04923-7
Gjetting, S. K., Mahmood, K., Shabala, L., Kristensen, A., Shabala, S., Palmgren, M., et al. (2020). Evidence for multiple receptors mediating RALF-triggered Ca2+ signaling and proton pump inhibition. Plant J. 104, 433–446. doi: 10.1111/tpj.14935
Han, H., Verstraeten, I., Roosjen, M., Mazur, E., Rýdza, N., Hajný, J., et al. (2021). Rapid auxin-mediated phosphorylation of myosin regulates trafficking and polarity in arabidopsis. Preprint at bioRxiv. doi: 10.1101/2021.04.13.439603
Haruta, M., Sabat, G., Stecker, K., Minkoff, B. B., Sussman, M. R. (2014). A peptide hormone and its receptor protein kinase regulate plant cell expansion. Science 343, 408–411. doi: 10.1126/science.1244454
Kubeš, M., Napier, R. (2019). Non-canonical auxin signalling: fast and curious. J. Exp. Bot. 70, 2609–2614. doi: 10.1093/jxb/erz111
Kuhn, A., Roosjen, M., Mutte, S., Dubey, S. M., Carrasco, P. C., Monzer, A., et al. (2022). A RAF-like kinase mediates a deeply conserved, ultra-rapid auxin response. Preprint at bioRxiv. doi: 10.1101/2022.11.25.517951
Li, L., Chen, H., Alotaibi, S. S., Pěnčík, A., Adamowski, M., Novák, O., et al. (2022b). RALF1 peptide triggers biphasic root growth inhibition upstream of auxin biosynthesis. Proc. Natl. Acad. Sci. U. S. A. 119, e2121058119. doi: 10.1073/pnas.2121058119
Li, L., Gallei, M., Friml, J. (2022a). Bending to auxin: fast acid growth for tropisms. Trends Plant Sci. 27, 440–449. doi: 10.1016/j.tplants.2021.11.006
Li, L., Verstraeten, I., Roosjen, M., Takahashi, K., Rodriguez, L., Merrin, J., et al. (2021). Cell surface and intracellular auxin signalling for h+ fluxes in root growth. Nature 599, 273–277. doi: 10.1038/s41586-021-04037-6
Lin, W., Tang, W., Pan, X., Huang, A., Gao, X., Anderson, C. T., et al. (2022). Arabidopsis pavement cell morphogenesis requires FERONIA binding to pectin for activation of ROP GTPase signaling. Curr. Biol. 32, 497–507. doi: 10.1016/j.cub.2021.11.030
Lin, W., Zhou, X., Tang, W., Takahashi, K., Pan, X., Dai, J., Ren, H., et al. (2021). TMK-based cell-surface auxin signalling activates cell-wall acidification. Nature 599, 278–282. doi: 10.1038/s41586-021-03976-4
Loginov, D. S., Fiala, J., Chmelik, J., Brechlin, P., Kruppa, G., Novak, P. (2021). Benefits of ion mobility separation and parallel accumulation-serial fragmentation technology on timsTOF pro for the needs of fast photochemical oxidation of protein analysis. ACS Omega 6, 10352–10361. doi: 10.1021/acsomega.1c00732
Malivert, A., Erguvan, Ö., Chevallier, A., Dehem, A., Friaud, R., Liu, M., et al. (2021). FERONIA and microtubules independently contribute to mechanical integrity in the arabidopsis shoot. PloS Biol. 19, e3001454. doi: 10.1371/journal.pbio.3001454
Prigge, M. J., Platre, M., Kadakia, N., Zhang, Y., Greenham, K., Szutu, W., et al. (2020). Genetic analysis of the arabidopsis TIR1/AFB auxin receptors reveals both overlapping and specialized functions. Elife 9, e54740. doi: 10.7554/eLife.54740.sa2
Qi, L., Kwiatkowski, M., Chen, H., Hoermayer, L., Sinclair, S., Zou, M., et al. (2022). Adenylate cyclase activity of TIR1/AFB auxin receptors in plants. Nature 611, 133–138. doi: 10.1038/s41586-022-05369-7
Rodriguez, L., Fiedler, L., Zou, M., Giannini, C., Monzer, A., Gelová, Z., et al. (2022). Cell surface auxin signalling directly targets PIN-mediated auxin fluxes for adaptive plant development. Preprint at bioRxiv. doi: 10.1101/2022.11.30.518503
Roosjen, M., Kuhn, A., Mutte, S. K., Boeren, S., Krupar, P., Koehorst, J., et al. (2022). An ultra-fast, proteome-wide response to the plant hormone auxin. Preprint at bioRxiv. doi: 10.1101/2022.11.25.517949
Serre, N. B. C., Kralík, D., Yun, P., Slouka, Z., Shabala, S., Fendrych, M. (2021). AFB1 controls rapid auxin signalling through membrane depolarization in Arabidopsis thaliana root. Nat. Plants 7, 1229–1238. doi: 10.1038/s41477-021-00969-z
Serre, N. B. C., Wernerová, D., Vittal, P., Dubey, S. M., Medvecká, E., Jelínková, A., et al. (2022). The AUX1-AFB1-CNGC14 module establishes longitudinal root surface pH profile. Preprint at bioRxiv. doi: 10.1101/2022.11.23.517700
Shih, H. W., DePew, C. L., Miller, N. D., Monshausen, G. B. (2015). The cyclic nucleotide-gated channel CNGC14 regulates root gravitropism in Arabidopsis thaliana. Curr. Biol. 25, 3119–3125. doi: 10.1016/j.cub.2015.10.025
Tang, W., Lin, W., Zhou, X., Guo, J., Dang, X., Li, B., et al. (2022). Mechano-transduction via the pectin-FERONIA complex activates ROP6 GTPase signaling in arabidopsis pavement cell morphogenesis. Curr. Biol. 32, 508–517. doi: 10.1016/j.cub.2021.11.031
Tang, R. J., Wang, C., Li, K., Luan, S. (2020). The CBL-CIPK calcium signaling network: unified paradigm from 20 years of discoveries. Trends Plant Sci. 25, 604–617. doi: 10.1016/j.tplants.2020.01.009
Vu, L. D., Gevaert, K., De Smet, I. (2018). Protein language: post-translational modifications talking to each other. Trends Plant Sci. 23, 1068–1080. doi: 10.1016/j.tplants.2018.09.004
Ward, J. M., Mäser, P., Schroeder, J. I. (2009). Plant ion channels: gene families, physiology, and functional genomics analyses. Annu. Rev. Physiol. 71, 59–82. doi: 10.1146/annurev.physiol.010908.163204
Yu, M., Li, R., Cui, Y., Chen, W., Li, B., Zhang, X., et al. (2020). The RALF1-FERONIA interaction modulates endocytosis to mediate control of root growth in arabidopsis. Development 147, dev189902. doi: 10.1242/dev.189902
Keywords: auxin, TMK, RALF1, FERONIA, fast root growth inhibition
Citation: Qiu Z, Zeng C, Deng H, Shen Z and Han H (2023) How plants cope with fast primary root elongation inhibition. Front. Plant Sci. 14:1187634. doi: 10.3389/fpls.2023.1187634
Received: 16 March 2023; Accepted: 09 May 2023;
Published: 31 May 2023.
Edited by:
Federica Brunoni, Palacký University Olomouc, CzechiaReviewed by:
Nelson Serre, UMR5667 Laboratoire Reproduction et Développement des Plantes (RDP), FranceCopyright © 2023 Qiu, Zeng, Deng, Shen and Han. This is an open-access article distributed under the terms of the Creative Commons Attribution License (CC BY). The use, distribution or reproduction in other forums is permitted, provided the original author(s) and the copyright owner(s) are credited and that the original publication in this journal is cited, in accordance with accepted academic practice. No use, distribution or reproduction is permitted which does not comply with these terms.
*Correspondence: Huibin Han, aHVpYmluaGFuQGp4YXUuZWR1LmNu
Disclaimer: All claims expressed in this article are solely those of the authors and do not necessarily represent those of their affiliated organizations, or those of the publisher, the editors and the reviewers. Any product that may be evaluated in this article or claim that may be made by its manufacturer is not guaranteed or endorsed by the publisher.
Research integrity at Frontiers
Learn more about the work of our research integrity team to safeguard the quality of each article we publish.