- 1Institute of Plant Genetics and Developmental Biology, Zhejiang Normal University, Jinhua, China
- 2Department of Horticulture, Abdul Wali Khan University Mardan, Mardan, KP, Pakistan
- 3Department of Agronomy, Abdul Wali Khan University Mardan, Mardan, KP, Pakistan
- 4Department of Entomology, Abdul Wali Khan University Mardan, Mardan, KP, Pakistan
- 5Department of Botany and Microbiology, College of Science, King Saud University, Riyadh, Saudi Arabia
- 6Plant Production Department (Horticulture-Pomology) Faculty of Agriculture, Saba Basha, Alexandria University, Alexandria, Egypt
Under the changing climate due to global warming, various abiotic stresses including drought (D) and salinity (S) are expected to further trigger their devastating effects on the already vulnerable crop production systems. This experiment was designed to unravel and quantify the potential role of exogenous application of salicylic acid (SA) in mitigating both D and S stresses and their combination (D+S), with three replications using CRD (Completely Randomized Design). The obtained results of the current study demonstrated significant effects of all three types of stresses (D, S, and D+S) on various parameters in Brassica napus plants. Quantifying these parameters provides a more informative and precise understanding of the findings. Current results revealed that all three stress types (D, S, and D+S) resulted in a reduction in leaf area (13.65 to 21.87%), chlorophyll levels (30 to 50%), gaseous exchange rate (30 to 54%) and the concentration of mineral ions compared to non-stressed plants. However, application of SA helped in mitigating these stresses by ameliorating the negative effects of these stresses. Moreover, Malondialdehyde (MDA) contents, an indicator of lipid per-oxidation and oxidative stress, the levels of antioxidants, proline content, an osmolyte associated with stress tolerance, and sugar content in the leaves were elevated in response to all stress conditions. In addition, the ultra-structures within the leaves were negatively affected by the stresses, while an application of SA considerably minimized the deterioration of these structures thus providing protection to the brassica plants against the stresses. In a nutshell, the findings of this study suggest that SA application in S, D and S+ D stresses provides evasion to the plants by improving different physiological and growth indices. The application of Salicylic Acid (SA) mitigated the negative effects of the stresses on all the above parameters, reducing MDA contents (47%), antioxidants (11 to 20%), proline (28%), sugar contents (20.50%), and minimizing the deterioration of ultra-structures. The findings emphasize the potential mitigatory role of SA in mitigating D and S stresses and highlight the need for further research to understand the underlying mechanisms in detail and explore its practical application in farming practices.
1 Introduction
Agriculture activities are closely associated with the existing atmosphere, with majority of crops often effects with different biotic and abiotic stresses. Common abiotic stress conditions which immensely hinder the normal growth and development of agriculture produce includes drought, salinity, waterlogging, cold, heat, and temperature fluctuations (Raza et al., 2019a and Raza et al., 2020a). Drought and Salinity are rated as the most drastic and deadly stress conditions, affecting about 26% & 20% of the agriculture acreage, respectively (USDA-FAO, 2018). Arid And semi-arid agricultural land is mostly subjected with the issue of salt stress (Sabagh et al., 2019). In the prevailing scenario of climate change, normal crop production is becoming challenging with every day passes on as various kind of abiotic stresses are get severe (Anderson et al., 2020; Zulfiqar and Hancock, 2020). The extent of damage caused due to drought and salt stress are very high and therefore they are considered as lethal among the list of all abiotic stress factors (Pokhrel et al., 2021). These abiotic stress factors has increased with every bit of human development as the anthropogenic activities in the era of progressive industrial development has caused serious damages to the agricultural system and its produce in form of introducing and enhancing various stress factors such as raised temperature, UV-B radiation, ozone depletion, metal/metalloids, water scarcity, salinity and nutrient depletion or excess (Anjum et al., 2014a). Abiotic stressors, including drought and salinity, alter their growth and development, seriously threatening crop productivity (Zhang et al., 2022). Both natural and anthropogenic stressors can lead to environmental challenges to plant growth, such as drought and salinity. Among all the environmental and climatic stress factors, these two abiotic-natured stresses (drought and salinity) are reflected as the most significant limiting factor towards crop productivity and growth enhancement, influencing about 40% and 11% of the world irrigated land, respectively (FAO et al., 2020). These abiotic factors can cause serious damages to the overall physiology and internal biology of a crop, for instance drought condition can cause wide range of biochemical and physiological changes in plang body and also carry damages its metabolic system (Abid et al., 2018; Qaseem et al., 2019). These environmental challenges pose a serious threat to agricultural production. Major grain producers and exporters such as China, India and the United States are currently experiencing severe water shortages in several important agricultural regions. A survey conducted by China’s Ministry of Water Resources found that during the 15.5-year plan, drought stress affected more than 25.67 million hectares of farmland annually, resulting in a reduction of up to 35 million tons of agricultural production and economic losses of up to about $35 billion (Ahmed et al., 2018).
In general, the simultaneous presence of multiple abiotic stresses has a more severe effect on agricultural production than a single stress situation (Mittler, 2006). Studies on crops such as potatoes (Levy et al., 2013), wheat (Yousfi et al., 2012) and barley (Yousfi et al., 2010) have shown that the simultaneous effect of saltiness and dryness on production is more in respect the effects of each individual stress. Previous studies have mainly convergent on the outcome of individual emphasis on plants (Wu et al., 2013), with tiny knowledge available on the physical and molecular mechanics that contribute to plant acclimatization to accumulation of saltiness and dryness stress (Mittler, 2006). However, modern research suggests that the consequence of plants to multiple physical stresses is distinct and may not be straight away generalized from the result of plants towards all stress separately (Rollins et al., 2013). Nature can evolve itself to the prevailing atmosphere changes, plants can overcome the drastic effect of drought and salt stress by activating a conducive defensive mechanisms in form of producing secondary metabolites, glycine betaine (GB), antioxidant enzymes, osmolyte-linked proline (PRO), carbohydrates, and total soluble proteins (TSP) (Shafiq et al., 2014; Shahzad et al., 2019). It was recorded that osmotic adjustment decreased the osmotic potential in plants and exhibited multiple benefits to plants in terms of enhanced growth patterns, adequate cell turgor pressure, and also modulates suitable metabolic activities inside a plants cell (Mafakheri et al., 2010). Furthermore, it was also recorded that glycine betaine immensely accumulates in maize plants while plants were subjected to water scare condition, which can increase all the growth traits (Gou et al., 2015). Similarly, proline acts as a conducive osmolyte during osmoregulation in water-stressed cotton plants (Habib et al., 2016). Plants develop different responsive-strategies towards various abiotic stresses, reflecting their variant levels of tolerance capacities (Noman et al., 2018; Ahmad et al., 2021). Plants subjected with water stress can adversely affect the biochemical, physiological and metabolic mechanisms in plants thus limiting their growth rate and development and quality of produce (Raza et al., 2020a). Along with drought and salt stress, the drastic influence of various other abiotic factors such as high and low temperature ranges (Du et al., 2016), waterlogging and metal toxicity (Lv et al., 2016) on crop quality and quantitative attributes have been reported in various crops, such as rapeseed (Raza et al., 2020a), and Brassica napus (Hatzig et al., 2014). To maintain yield on marginal land subject to stress, the most effective approach is to breed stress-tolerant crops. Therefore, it is crucial to determine inherited resources with higher permissiveness to nonbiotic stresses, particularly that occur at the same time in the piece of land, such as salinity and drought, and to realize their mechanics.
Plant hormones and growth regulators are integrally involved in modulating plant developmental systems and signal pathways, thus highlighting their pivotal role in responsive- strategies of plants towards various abiotic and biotic factors (Asgher et al., 2015). Enlisted to other integral plant hormones, Salicylic acid (SA) is basically a phenolic compound which can regulate the growth and developmental spectrums of plant and their response to different stress conditions (Khan et al., 2013b). SA is known for its involvement in regulating essential physiological and biological mechanisms in a plant body, worth mentioning such as photosynthesis, antioxidant defense responses, nitrogen metabolism, glycinebetaine (GB) and proline metabolism, thus strenthing defensive mechanism in plants against various abiotic stress factors (Miura and Tada, 2014).Beside its association in regulating defense-related genes and adequate resistance towards different biotic stress conditions (Kumar, 2014), SA has also been reported for its involvement in tolerance mechanisms of some major plants towards wide range of abiotic stress factors such as drought (Fayez and Bazaid, 2014), salinity (Khan et al., 2014; Nazar et al., 2015), metal (Zhang et al., 2015), and heat stress (Khan et al., 2013b) and osmotic (Alavi et al., 2014). Exogenous application of SA to plants in various ways, either by seed soaking method, nutrient solution, spray method or in general irrigation, can activate different mechanisms to better equipped plants with abiotic stress tolerance (Khan et al., 2013b; Palma et al., 2013; Khan et al., 2014).
Salicylic acid (SA) a plant’s hormone plays a vital role in regulating several physicochemical processes and have shown a significant impact on plants responses to overcome biotic and abiotic stress (Syeed et al., 2011). Although high concentrations of AS can generate oxidative stress and cell death in plants due to H2O2 accumulation, low concentrations of AS have been found to promote resistance to most of these stresses (Horvath et al., 2007).
Salt stress acclimatization in plants with SA improves through mechanisms such as ion exclusion and/or categorization, osmoregulation, reduction of membrane lipid peroxidation, protein kinase synthesis, and regulation of the antioxidant defense system (Khalifa et al., 2016; Yadu et al., 2017). However, the impact of exogenous application of SA on the plant stress tolerance habit is influenced by several factors, including the concentration, the mode of application and the general conditions of the plant, such as its stage of development, the oxidative balance of the cells and the previous state exposure to biotic or abiotic stress (Horvath et al., 2007). Salicylic acid (SA) is one of the pivotal phenolic compound produced adequately inside the plant body and regulates its various functions (Arif et al., 2020). Water-deficit conditions influence the endogenous biosynthesis of SA thus numerous studies has recommended the exogenous application of SA to plants which can improve its performance under unfavorable abiotic stress conditions (Abbaszadeh et al., 2020).
In present era, where the world is facing evolving with wide spectrum changes in urbanization, industrialization and climate change, crop production is threatened with various kinds of abiotic stresses and environmental hazards. Rapeseed (Brassica napus L.), just like other oil seed crops, are very sensitive to abnormal abiotic stress condition which will immensely reduce its growth patterns and also predominantly reduce its oil quality and quantity (Luo et al., 2017). Thus increasing tolerance capacity of rapeseed for various abiotic stress factors is the basic principal towards enhanced oilseed production (Luo et al., 2015; Raza et al., 2020a). The objective of this study is to explicate the physiological and biochemical processes of Brassica napus L. subjected to water- deficit an salt stress which will eventually lead towards its adaptation strategies to climate change. We will also investigate the influence of exogenous application of Salicylic Acid (SA), alone or in combination on the defense mechanism of Brassica napus L. towards drought and salt stress conditions.
2 Materials and methods
2.1 Plant’s materials and treatments
The current experimental study was carried out at the Faculty of Agriculture, The University of Poonch, Rawalakot, AJK, Pakistan, employing both hydroponic and field methods. The experimental area lies a Longitude 73° 45’ 34.93” E, Latitude 33° 51’ 32.18” N and an altitude of 1638 m. Oilseed rape seedlings were cultivated in pots using a mixture comprising 1/4 vermiculite, 1/2 compost, and 1/4 sand (v/v/v) for one month under controlled environmental conditions during the end of October. Subsequently, the seedlings were transferred to an experimental screen house to acclimatize to natural light conditions. Healthy seedlings with similar morphological characteristics were then transplanted into a container/pot of 5 L in volume having a Basal Nutrient Solution (BNS) of 4.5 L. After one week, different treatments were applied to the respective containers, including the control (BNS), drought stress (PEG) 10%, salinity stress (NaCl) 100 mM, control with Salicylic Acid (SA) 50 µM, combined drought and salinity stress (D + S), and SA with D + S stress. Salicylic acid (SA) was applied every 5th day until the harvest. Fifteen days after treatment, samples were collected from the treated plants to assess various parameters.
2.2 Leaf and seedling architecture analysis
Morphological parameters, including leaf area (cm2) and seedling height (cm), were measured using a leaf area meter (CID, CI-202) and a measuring scale, respectively (Gil-Ortiz et al., 2020).
2.3 Photosynthetic pigments and photosynthesis-related parameter analysis
A method previously described by Lichtenthaler (1987), was used to measure the contents of photosynthetic pigments (Chlorophyll-a and chlorophyll-b, mg/g FW) in fresh leaves of Brassica napus. After seven (7) days of treatment, fresh leaves were collected and 0.5 g of the collected leaves was grinded and mixed in a 10 mL tubes containing 80% acetone. The tubes were thoroughly mixed with shaker and then incubated for one night in the dark at room temperature. The extract concentration was determined using an ultraviolet spectrophotometer (UV-2100, UNIC, Shanghai, China) with the wavelengths of 665 nm and 649 nm respectively.
The parameters related to photosynthetic gas exchange, including leaf net photosynthetic rate (Pn, μmol m-2 s-1), internal CO2 concentration (Ci, μmol mol-1), stomatal conductance (gs, mol H2O m-2 s-1), and transpiration rate (Tr, mmol H2O m-2 s-1) were observed using an open type and portable photosynthesis system (LCA4. Bio-Scientific. Great Amwell. Herts. UK). The measurements were taken between 09:00 and 11:00 using the third top leaf. The CO2 concentration in the leaf chamber was maintained at 400 µmol mol-1, and the airflow speed was set at 500 µmol s-1. The photosynthetically active radiation (PAR) was maintained at 1000 µmol m-2 s-1. The air relative humidity was kept at 75 ± 5%, and the leaf temperature was maintained at 24 ± 2°C. The data were collected at intervals of 2-3 minutes with a minimum of three replicates.
2.4 Determination of osmo-regulatory substances analysis
The determination of malondialdehyde (MDA) content was conducted using a modified thiobarbituric acid (TBA) method (Kotula et al., 2020). Leaf samples (0.5 g) were homogenized with liquid nitrogen and 80% ethanol. The resulting mixture was centrifuged in 2 mL microcentrifuge tubes at 6000 rpm for 5 minutes. Aliquots of 0.7 mL of each supernatant were combined with 0.7 mL of 0.65% TBA in 20% trichloroacetic acid (TCA) and 0.01% butylated hydroxytoluene (BHT). Another set of 0.7 mL samples was mixed with 0.7 mL 20% TCA and 0.01% BHT. The microcentrifuge tubes were then incubated at 95°C for 25 minutes, followed by centrifugation at 6000 rpm for 5 minutes after cooling. The absorbance at 450 nm, 532 nm, and 600 nm was measured using a UV-Vis spectrophotometer (Evolution 210, Thermo Scientific), and the concentration of MDA (nM g-1 FW) was determined using an extinction coefficient of 157 mM cm-1. The content of proline was determined by a modified method based on Abdel and Tran (2016). Fresh shoots (0.5 g) were ground using a rapid automatic sample grinding instrument (JXFSTPRP24, Shanghai Jingxin Industrial Development, Shanghai, China) and digested in 5 mL of 3% aqueous sulfosalicylic acid. Afterwards, 2 mL of the extract solution was mixed with 2 mL ninhydrin reagent and 2 mL glacial acetic acid. The mixture was then boiled at 100°C for 60 minutes and cooled in an ice bath for 5 minutes. Subsequently, the mixture was extracted with 4 mL toluene, vigorously mixed using a vortex for 15-20 seconds, and cooled to room temperature. The free toluene was measured at 520 nm using a spectrophotometer (Beckman Coulter Inc., Fullerton, CA, USA). The amount of soluble sugar was determined by treating the sample with 80% ethanol according to the method described by Dubois et al. (1951). The sample was filtered, and the extract was heated in a water bath at 80°C for 1 h. Afterward, 2.5 mL of sulfuric acid (H2SO4) was added and mixed well. 0.5 mL of the mixture was mixed with 1 mL of 18% phenol, and the mixture was incubated at 37°C. The absorbance was recorded at 490 nm.
2.5 Quantification of antioxidant enzyme activities
To determine the enzyme activity, leaf samples weighing 0.5 g fresh weight (FW) were pulverized into a powdered form using a mortar and pestle. Subsequently, they were homogenized in 8 mL of 50 mM potassium phosphate buffer (PPB) with a pH of 7.8 under cooling conditions. The resulting homogenized solution was subjected to centrifugation at 10,000 rpm for 20 minutes at a temperature of 4°C, yielding a crude enzyme extract for the measurement of superoxide dismutase (SOD), peroxidase (POD), catalase (CAT), and ascorbate peroxidase (APX) activities (Hussain et al., 2015; Ahanger et al., 2018; Ahmad et al., 2020).
Superoxide dismutase (SOD; EC 1.15.1.1) activity was assessed by inhibiting the photochemical reduction of nitro blue tetrazolium (NBT). The reaction mixture consisted of 50 mM PPB (pH 7.8), 13 mM methionine, 75 mM NBT, 2 mM riboflavin, 0.1 mM EDTA, and 0.1 mL of the enzyme extract in a total volume of 3 mL. The unit of SOD activity was defined as the quantity of enzyme required to cause a 50% inhibition of NBT reduction, as measured at a wavelength of 560 nm.
For peroxidase (POD; EC 1.11.1.7) activity determination, 0.1 mL of the enzyme extract was combined with 50 mM PPB (pH 7.0), 1% (m/v) guaiacol, and 0.4% (v/v) H2O2. The absorbance was quantified at a wavelength of 470 nm.
Catalase (CAT; EC 1.11.1.6) activity was measured by employing H2O2 (with an extinction coefficient of 39.4 mM-1 cm-1). A reaction mixture of 3 mL was prepared, comprising 50 mM PPB (pH 7.0), 2 mM EDTA-Na2, 10 mM H2O2, and 0.1 mL of the enzyme extract. The measurement was performed at a wavelength of 240 nm.
Ascorbate peroxidase (APX; EC 1.11.1.11) activity was determined using a reaction mixture (3 mL) containing 100 mM phosphate buffer (pH 7), 0.1 mM EDTA-Na2, 0.3 mM ascorbic acid, 0.06 mM H2O2, and 0.1 mL of the enzyme extract. The change in absorption was monitored at 290 nm for a duration of 30 seconds after the addition of H2O2.
2.6 Ultrastructural analysis using transmission electron microscopy
Small leaf fragments, measuring approximately 1 mm^2, were collected from the fully expanded topmost leaves of the plant at 10 days after treatment (DAT) for the purpose of investigating intracellular ultrastructures. The samples were subjected to analysis using a JEOL TEM-1230EX transmission electron microscope. Initially, the leaf specimens were fixed overnight in a 2.5% glutaraldehyde solution (v/v) and subsequently washed three times with a 0.1 M Sodium Phosphate Buffer (SBP) at pH 7.0. Post-fixation was carried out by treating the specimens with 1% osmium tetraoxide (OsO4) for 1 hour, followed by washing with a 0.2 M Sodium Phosphate Buffer (SPB) at pH 7.2 for 1-2 hours. Dehydration of the samples was performed using a series of graded ethanol concentrations (50, 60, 70, 80, 90, 95, and 100%) and 100% acetone. The samples were then infiltrated and embedded in Spurr’s resin. Thin sections with a thickness of 80 nm were prepared, mounted on copper grids, and observed under a JEOL TEM-1230EX transmission electron microscope operating at an accelerating voltage of 60.0 kV.
2.7 Analysis of mineral nutrient content
Approximately 0.1 g of oven-dried shoot and root tissues, subjected to drying at 65-70°C for 72 hours, were pulverized to determine the concentrations of essential mineral nutrients, namely Magnesium (Mg), Calcium (Ca), Zinc (Zn), and Iron (Fe). Mineralization was performed by heating 5 ml of 65% nitric acid (HNO3) using a microwave digester (Microwave 3000; AntoonPaar). The volumes of the digested samples were adjusted to 10 ml by adding Milli-Q water. Subsequently, the specimens were analyzed using an inductively coupled plasma-optical emission spectrophotometer (ICP-OES; Optima 8000DV; PerkinElmer) to quantify the content of mineral nutrients.
2.8 Statistical analysis
Analysis of variance (ANOVA) was presented on the obtained data for various parameters, and appropriate completely randomized design was used for testing the treatments. Tukey test was used to calculated the probability level greater than 0.05. The software OriginPro7 was utilized to generate the figures.
3 Results and discussions
3.1 Leaf and seedling architecture analysis
Figure 1 illustrates the influence of drought and salinity stress alone and in combination on the shoot length and leaf area of Brassica napus plants. Both stress conditions exhibits a considerable decrease in shoot length and leaf area, with more prominent effect reflected in combine stress growing condition. Drought stress decreases the shoot length by (32.2%) and leaf area by (13.6%). Similarly, Shoot length and leaf area declined in salt stress by (33.4%) and (15.4%). Combined effect of both the stresses (D+S) results in severe decrease in shoot length (41.5%) and leaf area (21.8%) as compared to non-stressed plants. However, drought and salinity stressed-subjected plants while treated with SA showed enhanced shoot length (26.9%) and leaf area (7.7%) as compared to non-SA treated plants (Figure 1). This response showed that SA application could effectively improve Brassica napus plants growth under water and salinity stress. In current study, the decline growth pattern of Bassica napus L. seedlings under water-deficit and salinity was probably due negative affect on important metabolic functions linked with photosynthesis, chlorophyll biosynthesis rate, oxidative metabolism and cell division & enlargement. Cellular elongation and differentiation process drastically slow-down under salt and drought stress conditions (Abid et al., 2018; Sarabi et al., 2019). Also, vegetative growth features and traits of major crops considerably reduce under water-deficit stress (Damalas, 2019). Moreover, the retardant impact of water and salt stress on brassica napus plants were mitigated with SA application, with partial recovery in shoot and leaf growth and also boosting the photosynthesis synthesis rate and chlorophyll content leaves (Saudy et al., 2023b; Shaaban et al., 2023). Similar findings were also reported in various other crop species, including sweet basil (Damalas, 2019), maize (Bijanzadeh et al., 2019), sesame (Najafabadi and Ehsanzadeh, 2017), sunflower (Kosar et al., 2018), and squash (El-Mageed et al., 2016). Our results supplements the idea that SA is playing apivotal role in defense mechanism and protecting the photosynthetic apparatus in brassica plants (Kosar et al., 2018), with mitigating the photosynthetic and chlorophyll activities and thus encouraging overall plant growth traits in water deficit and saline stress conditions.
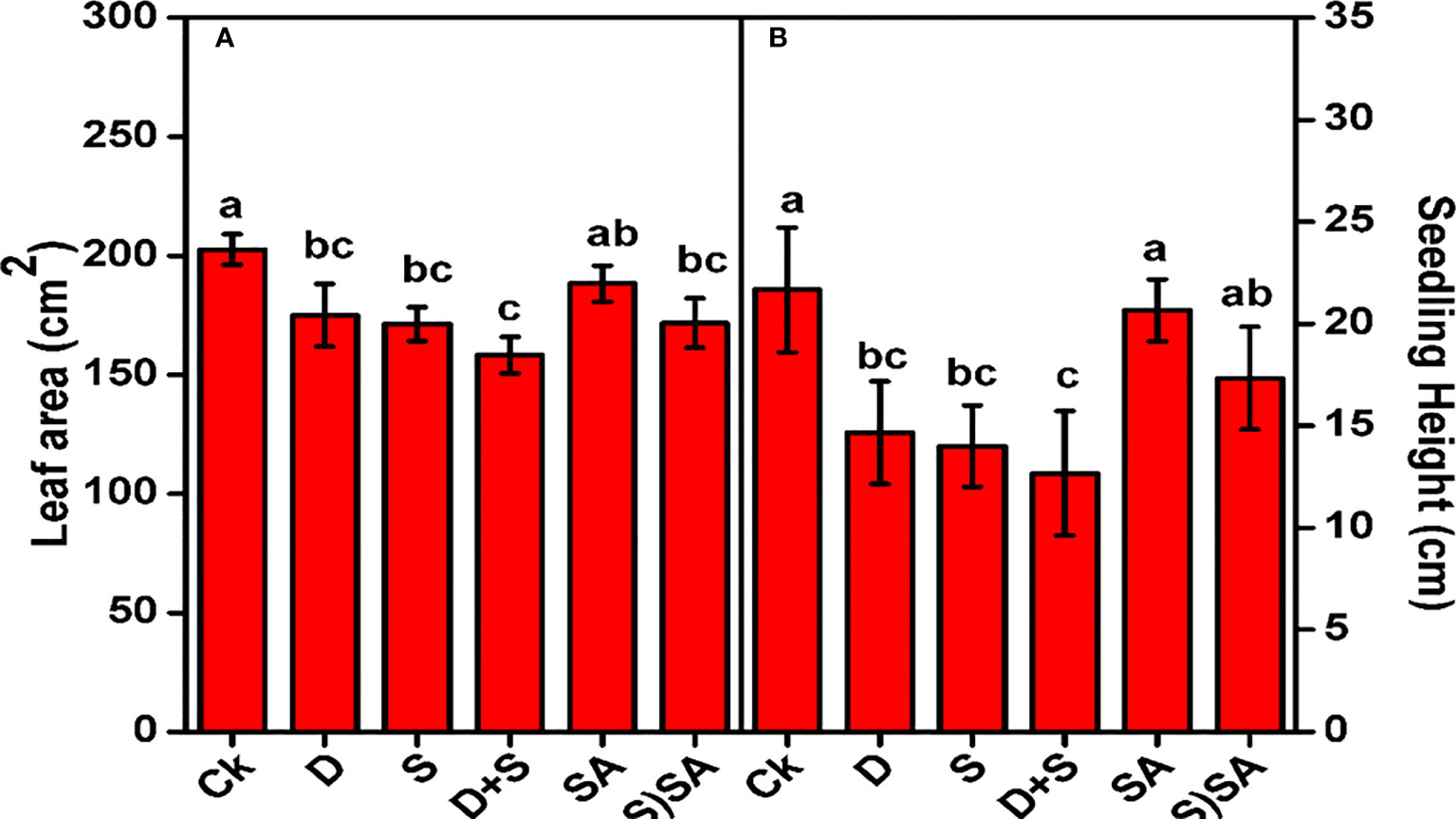
Figure 1 Effect of Drought, Salinity, and SA on leaf area (A) and seedling height (B) of Brassica napus genotype. Statistical differences at a 5% level exist between means labeled with different letters (a-e) for each attribute (mean ± SD; n = 3).
3.2 Photosynthetic pigments and photosynthesis-related parameter analysis
Drought and salt stress both individually and in combination predominantly affect the photosynthetic pigments in Brassica napus plants (Figure 2). Drought stress drastically reduced Chl-a contents by (30.5%) and Chl-b contents by (32.6%) as compared to plants without stress. Similarly, salt stress condition also decreases Chl-a by (36.1%) and Chl-b by (26%). Moreover, the combined impact of D+S depicts drastic decline in concentrations of Chl-a (52%) and Chl-b (49%). However, brassica plants subjected with SA predominantly elevated the photosynthetic pigments Chl-a (29%) and Chl-b (28%), as compared with non-SA treated plants (Figure 2). A reduction in chlorophyll content reflects as a characteristic indication of oxidative stress and may be due to the pigment photo-oxidation and chlorophyll degradation under drought stress environments. Decline in concentration of Chlorophyll pigments under water deficit condition might be due to disruption in chlorophyll biosynthesis which can decrease the chlorophyll contents in leaves (Ashraf and Harris, 2013), and thus cause a cosiderbale decline in photosynthesis rate in plants (Amirjani and Mahdiyeh, 2013). Similar kind of findings where a substantial decline in chlorophyll content and photosynthesis rate were also observed in wheat (Habib et al., 2020) and cucumber (Naz et al., 2016) grown under drought stress condition. Moreover, Ahmed et al. (2013b) and Saudy et al. (2021) also found a noticeable reduction in the chlorophyll contents (Chl-a and Chl-b) in barley plants raised in drought and salinity stress environment. The beneficial influence of SA in relation to the damaging effects of water-deficit and salt stress on production of chlorophyll pigments in brassica plants as displayed in Figure 2, could be accredited to its regulatory effects on Rubisco activity, and thus eventually, to enhance the photosynthesis process. SA probably plays its role in the Cellular membrane defense mechanism and also in scavenging the toxic ROS species produced during the oxidative stress condition. Another supplementary effect of SA could be attributed to stimulating the biosynthesis of protein kinases which plays a pivotal role in regulating cell division process at different stages of cell development (Ali et al., 2013). Few other reports further describes that photosynthesis process and stomatal conductance elevates due to SA treatment under growing condition prone with various abiotic stresses (Kosar et al., 2018). Similar kind of impact created by SA treatment was also recorded in Triticum aestivum L. (Maghsoudi et al., 2019).
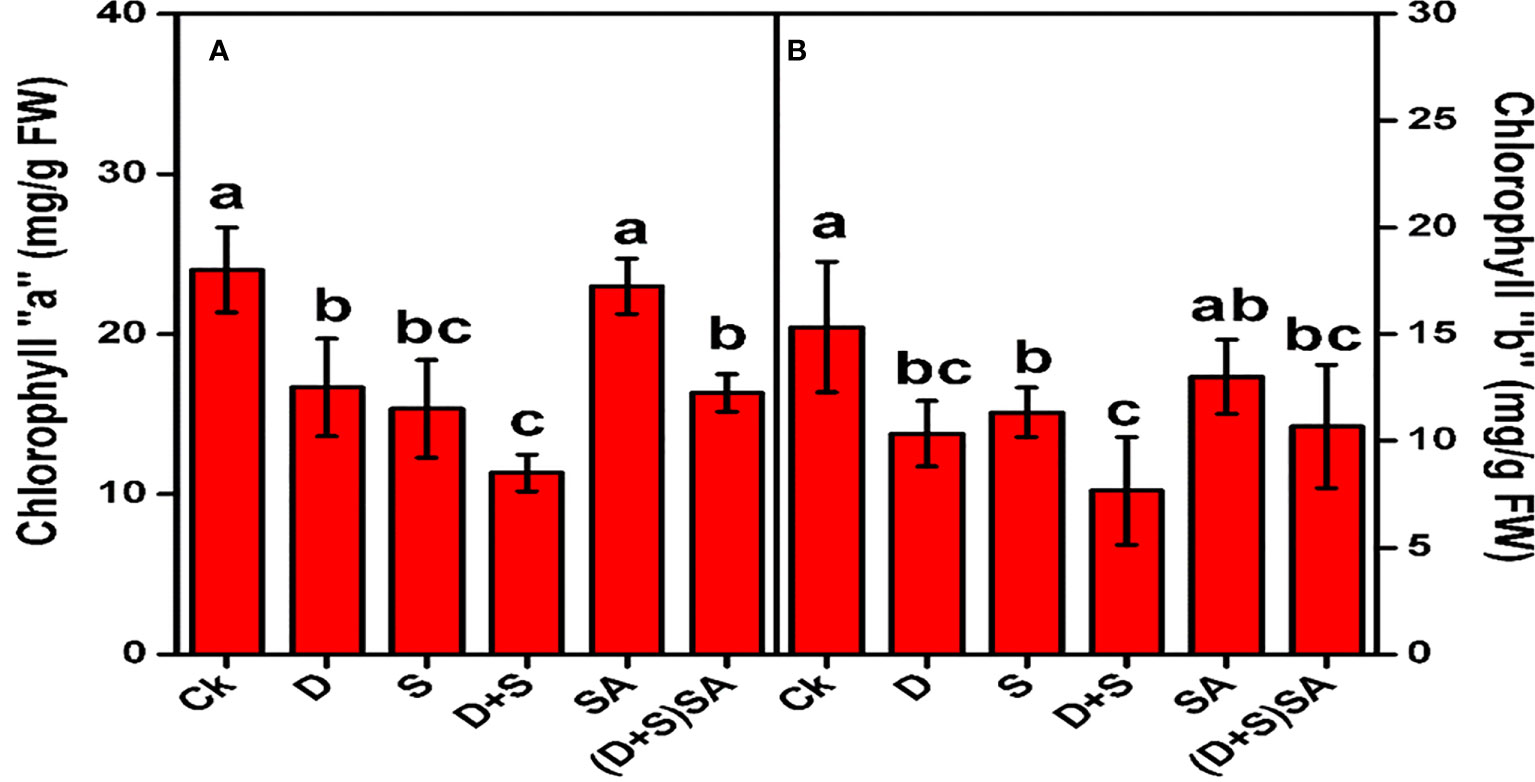
Figure 2 Effect of Drought, Salinity, and SA on Chlorophyll “a” (A) and Chlorophyll “b” (B) of Brassica napus leaves. Statistical differences at a 5% level exist between means labeled with different letters (a-e) for each attribute (mean ± SD; n = 3).
3.3 Gas exchange parameters
The results presented in Figure 3 depict the impact of water deficit and saline stress alone or in combination on all the traits associated with photosynthesis. Both the stress conditions significantly reduce various kinds of leaf exchange characteristics in Brassica napus plants including Pn, Ci, Tr, and Gs. Drought stress cause a decreases by Pn (28%), Ci (12.4%), Tr (26.3%) and Gs (29.6%) as compare to non-stress plants. Likewise, a declining pattern for Pn (29.2%), Ci (11.3%), Tr (29.8%) and Gs (31.6%) due to salt stress. Combined effect of D+S was severe and reflected with much declined values of leaf exchange catalyses’ Pn (52.4%), Ci (21.2%), Tr (47.3%) and Gs (54.3%) as compare to non-stress plants. However SA applied to plants subjected to combined stress condition can significantly increase all the aforementioned photosynthetic parameters such as Pn by (23.5%), Ci (6%), Tr (36.1%) and Gs (19.4%) as compare to its influence of control plants. These results indicate that SA can restore the negative effects of stress on plant overall growth and development. Both drought and salinity stresses have a significant negative impact on photosynthesis, which is among the initial step towards biological degradation of plant physiology and vitality (Sarabi et al., 2019). These effects may be direct, for instance lower CO2 availability due to limitations in diffusion through stomata and mesophyll or possible changes in photosynthetic metabolism, or they may depict as secondary effects such as oxidative stress. Drought among all the abiotic stress factors drastically stimulates the photo-oxidative degradation of photosystems in various plants (Hu et al., 2018), thus causing a decline in Pn concentration (Shao et al., 2016). Our findings also reflected that water deficit stress results also degraded the cellular membrane system in Brassica napus plants and caused decline in various leaf exchange characteristics Pn, Ci, Tr, and Gs. Disruption and ineffectiveness in chloroplast membrane system, due to swelling and damages caused to the thylakoid membranes (either stromal or granal) were observed in wheat and maize under water deficit stress factors. Salt stress is subjected with a significant decline in photosynthetic rate in many plant species, which may be due to decreased stomatal conductance, decline in photochemical capacity, and reduce metabolic processes during carbon uptake (Pérez-López et al., 2012). In another study, a predominant decline in transpiration rate, photosynthetic rate, stomatal conductance and inter-cellular CO2 were recorded for maize plants grown in water stress condition (Anjum et al., 2011). Moreover, numerous reports have reported that exogenously application of SA retained the membrane integrity of chloroplast & thylakoid structures in plants confronted with drought stress (Hu et al., 2018). In support with these earlier reports, our findings also depicted that exogenous application of SA stabilizes the integrity of cellular membrane structures, reducing the Pn, Ci, Gs, and Tr concentrations, and decreases the electrical conductivity in leaves of Brassica napus plants during both water-deficit and saline stress conditions (Figure 2).
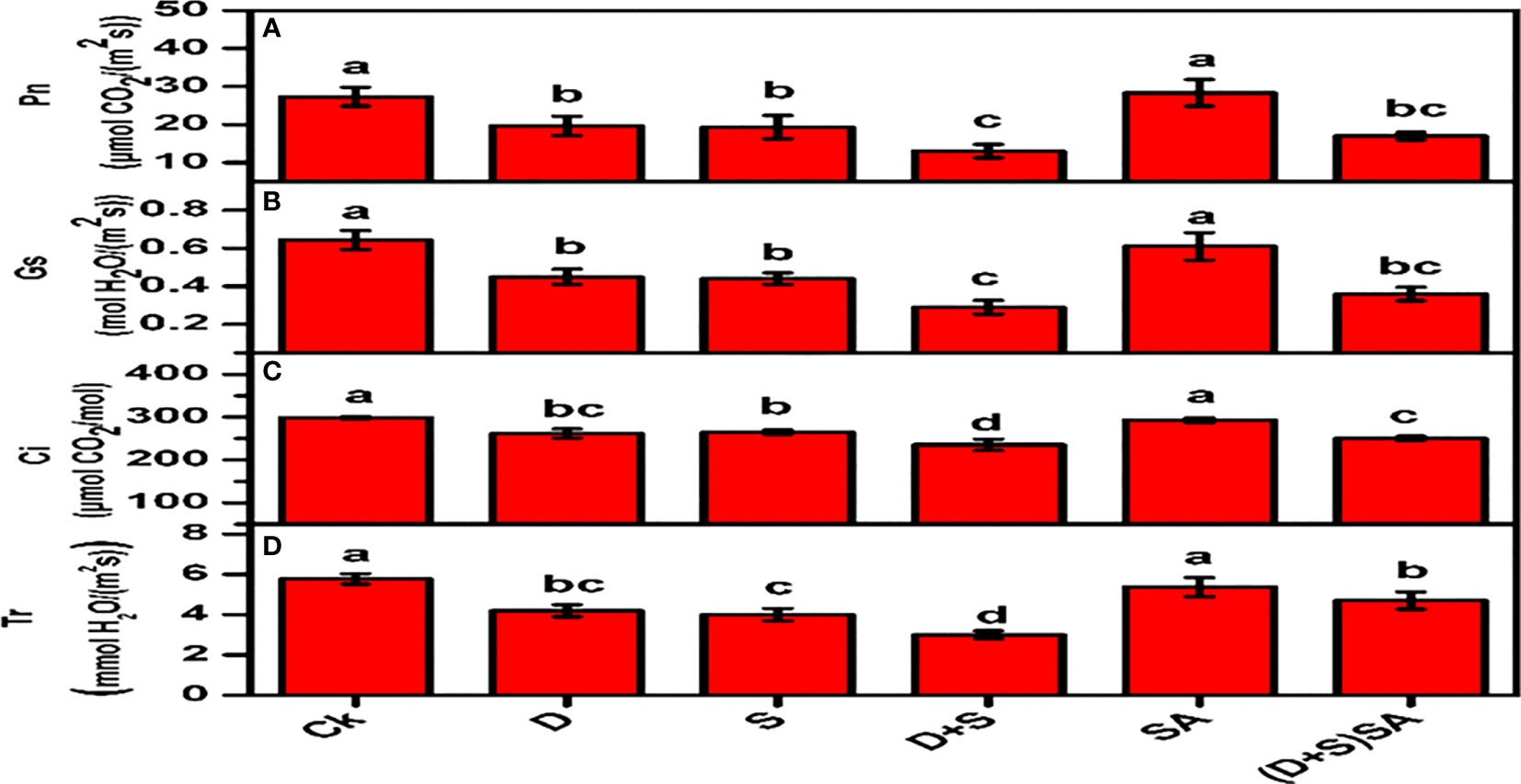
Figure 3 Effect of Drought, Salinity and SA on gas exchange parameters; Photosynthetic rate (Pn) (A), stomatal conductance (Gs) (B), internal CO2 concentration (Ci) (C), and transpiration rate (Tr) (D) in leaves of Brassica napus genotypes. Statistical differences at a 5% level exist between means labeled with different letters (a-e) for each attribute (mean ± SD; n = 3).
3.4 Lipid peroxidation (MDA)
An increase in ROS accumulation is a major consequence of drought and/or salinity stress conditions in plant cells. The application of salinity, dearth, and D + S treatments triggered a major rise in MDA production in the leaves of Brassica napus as compared to untreated plants (Figure 4). Water deficit and salinity stress caused an elevation in MDA by 42.3% and 47.5%, respectively. The impact was more pronounced in the D + S treatment increasing by 60.1% as compare to either drought or salinity stress alone and control plants. The application of SA caused a decrease in MDA content by 47.3% in B. napus leaves under D + S stresses as compare to plants without SA treatments (Figure 4). Water deficit-stress condition results in ROS accumulation due to decreased light absorption and minimum photosynthetic electron transport, which can cause photo-oxidative damages to the photosystems (Dalal and Tripathy, 2018; Yudina et al., 2020). Oxidative stress markers including H2O2, Electrolyte leakage, and MDA are found elevated in plants during abnormal environmental condition (Shao et al., 2016). Brassica napus plants leaves subjected to salt and water stress displayed an elevated levels for MDA concentrations as compare to non-stress plants. Our findings were similar to reports studied in E. globulus (Jesus et al., 2015) and Carthamus tinctorius L. (Bijanzadeh et al., 2019) and. Also, application of SA reduced the MDA concentrations in Brassica anpus L. plants grown in saline and drought- stressed conditions, reflecting that SA mitigated the oxidative stress inked with these abiotic stress conditions. Similar kind of findings were also reported for Brassica rapa L. (La et al., 2018) and Triticum aestivum L. (Maghsoudi et al., 2019), where MDA concentration and electrolyte leakages and H2O2 displayed a declining patterns with introduction of SA under saline and drought stress. Another study also recorded a novel feature in SA-subjected sweet basil plant that the relative water content (RWC) was also elevated in leaves, indicating that SA increases the membrane integrity during stress conditions (Damalas, 2019).
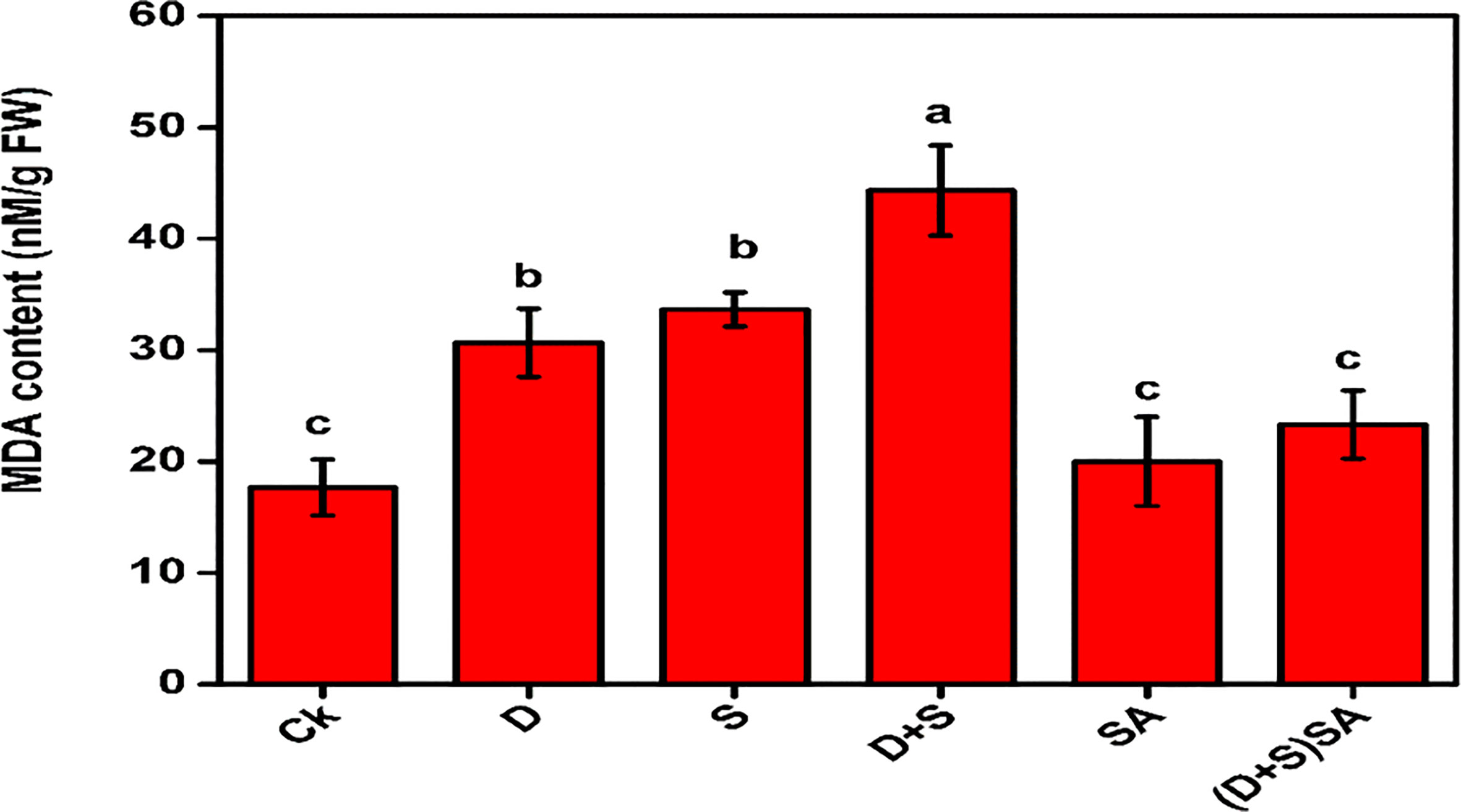
Figure 4 Effect of Drought, Salinity and SA on MDA content in leaves of Brassica napus genotypes. Statistical differences at a 5% level exist between means labeled with different letters (a-e) for each attribute (mean ± SD; n = 3).
3.5 Antioxidant enzymes activities
A substantial raise in the concentrations of various antioxidant enzymes SOD, POD, APX and CAT were recorded for brassica napus plants while confronted with water-deficit and salt stress (Figure 5). The Combined influence of both the stress factors (D+S) was further severe as compare to single stress condition. Drought stress induced CAT, SOD, POD and APX activities by 57%, 15%, and 20.3%, 17% respectively, compared to well-watered brassica plants. Similarly, salinity stress also exhibited elevation in CAT (57%), SOD (12.7%), POD (26.8%) and APX (20.5%) as compared to unstressed (Figure 5). Similarly, combined stress (D+S) influence was highly significant with CAT (73%), SOD (25%), POD (54.5%) and APX (38.2%) increasing concentrations as contrast to control. However, when leaves were treated with SA, all the enzyme activities were found to decrease. Foliar application of SA decreases CAT, SOD, and POD activities by 35.4%, 11.1%, 17.4% and 22.2% respectively, as compared to non-SA treated plants (Figure 5). Reports confirm that water-deficit conditions leads to abnormal and harmful effects in plant body, majorly distortion of cellular membrane and structures, ion-leakages, inactivation of enzymes, and decreased osmotic regulation, due to increasing ROS accumulation inside infected plant cells (Shafiq et al., 2014). In present study, the rising activities of antioxidant enzymes in brassica napus plants due to drought and salt stress conditions can be linked to the activation of plant defense mechanism in order to safeguard plants against water deficit-induced ROS accumulation. The activation of antioxidant systems is a robust process in order to counter the oxidative stress under stress conditions, which involves different kind of enzymes mainly superoxide dismutase (SOD), peroxidase (POD), catalase (CAT) and ascorbate peroxidase (APX), ascorbate peroxidases and few non enzymatic molecules i.e phenols, glutathione, osmolytes and ascorbates (Cirulis et al., 2013; Ali et al., 2018). In this study, we observed the instance activation of these antioxidant enzymes in response to salt and water stress and further decrease with SA application. Similar results were reported earlier in different crops, with activation in antioxidants enzymes such as SOD, POD and CAT were observed under drought stress in grapes (Wang et al., 2014). Furthermore, several more studies further elaborated that SOD plays an integral role during detoxification, whereas enzymes such as CAT and POD were engaged in countering numerous latent oxidants in order to restore stress tolerance (Ashraf, 2009; Akram et al., 2018). An important indicator for plant stress tolerance is the up-regulation and activation of antioxidant enzymes during stress conditions (Yadav et al., 2016). The accumulation of antioxidants helps in minimizing the adverse effects of oxidative stress and to normalize the metabolic activities under stress condition. SOD converts O2− to H2O2, which is further converted to water by ascorbate peroxidase (APX) utilizing ascorbate as an electron donor (Scandalios, 2005). Higher plants, when confronted with drought stress exhibits an increased buildup of antioxidant enzymes, including SOD, POD, CAT and APX, which protect against oxidative injury (Ozkur et al., 2009). In contrast, Yang et al. (2010) reported that at 25% field capacity, the activities of CAT, POD, SOD, GR and APX were elevated relative to 100% field capacity. In another study, Ozkur et al. (2009) reported opposite trends in activities of SOD, POD, CAT, GR and APX in response to NaCl stress condition in H. marinum and H. vulgare plants Several reports reflected that exogenous SA induction alleviate the activation of different enzymatic antioxidants especially during salt and water-deficit stress-induced Brassica rapa (La et al., 2019), safflower (Bijanzadeh et al., 2019) and wheat (Maghsoudi et al., 2019).
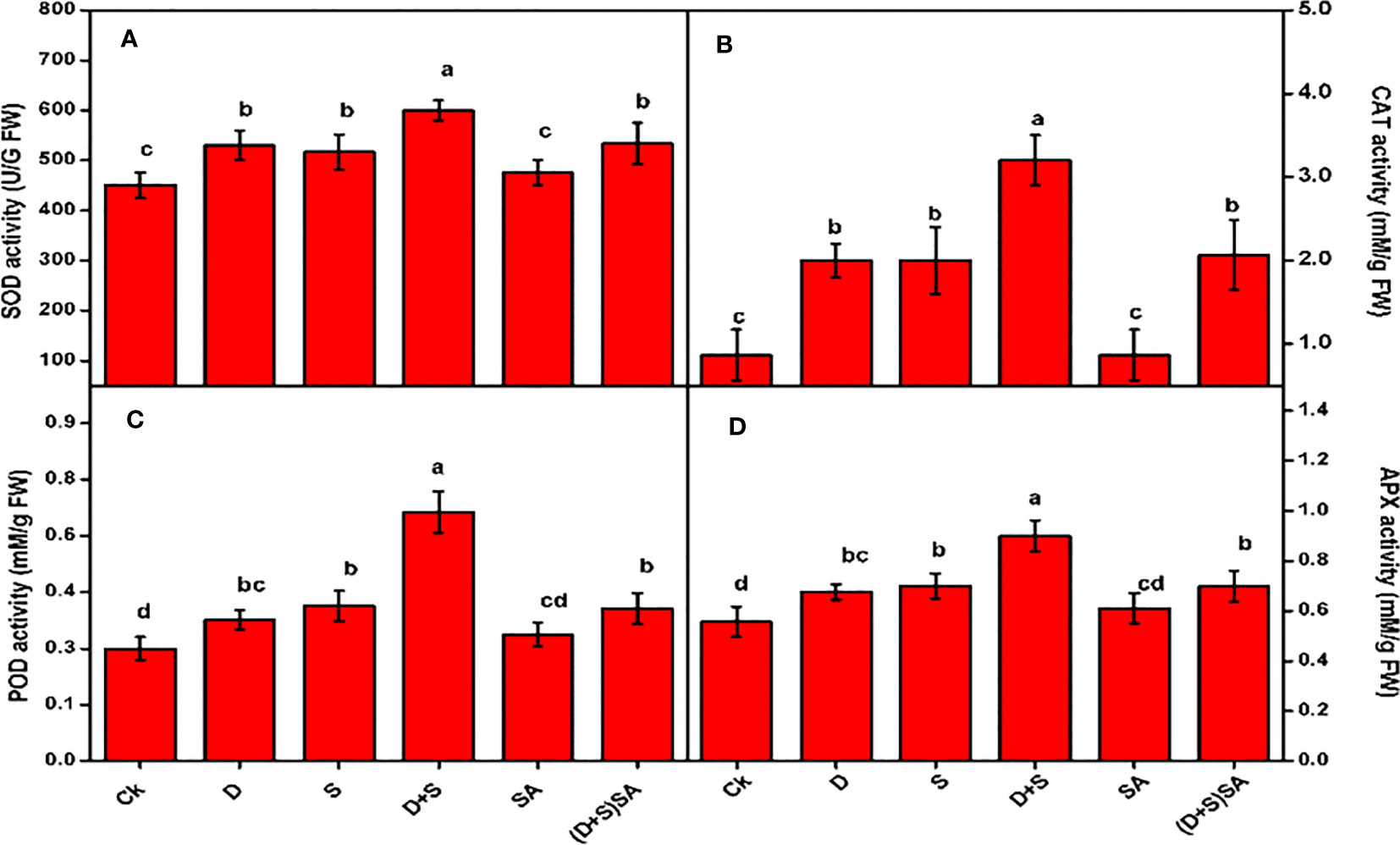
Figure 5 Effect of Drought, Salinity and SA on the activities of Antioxidant Enzymes in leaves of Brassica napus genotype; activities for SOD (A), CAT (B), POD (C) and APX (D) were measured separately. Statistical differences at a 5% level exist between means labeled with different letters (a-e) for each attribute (mean ± SD; n = 3).
3.6 Buildup of proline and soluble sugars
Figure 6 illustrates that proline and soluble sugar levels in Brassica napus leaves were considerably increased under D + S stress conditions as compared to control. Drought stress elevated the leaf Proline and soluble sugars concentrations by 44.7% and 34.9% respectively, as compare to the plants without stress. Likewise the proline and soluble sugar in salt stress situation also raised by 41.6% and 28.7%, respectively. Similarly, Combine stressed effect (D+S) reflected a much higher elevation of 72% in proline and 64.6% in soluble sugars as compare to non-stressed plants. However, when SA was applied, the levels of proline decreased under D + S stress conditions, though no substantial effects were found in sugar contents (Figure 6). Foliar application of SA decreases Proline concentration by 28% and soluble sugar concentration by 20.5% relative to unsprayed stressed plants. Leaf free Proline and soluble sugars are the multifunction molecules found in plants that protect the plant cellular membranes against oxidation and dehydration conditions (Per et al., 2017; Annunziata et al., 2019). In present experiments, we will found an elevated pattern of proline for Brassica napus plants subjected to drought and saline stress conditions. Proline has been known for its multiple regulatory functions especially during abnormal growing conditions, with more profound roles are to safeguard the photosynthetic system, cellular membranes and enzymatic regulations, prevent cellular dehydration and act as a molecule. Therefore, proline may be closely linked with stress-tolerance mechanism in plants mainly protecting the cellular structures and regulating physiological processes inside plant body. Furthermore, Pro also decreases the oxidative stress in various plants by alleviating the activities of ROS-scavenging antioxidants which will reduce the accumulation of ROS species (Ibrahim and Abdellatif, 2016). Compatible solutes are typically small in size and easily dissolve in water, and they are generally considered safe even when found in high concentrations within a cell. Plants collect compatible solutes, including Proline and sugars, in order to aid in water absorption as a response to drought and salinity conditions (Ashraf and Foolad, 2007; El-Metwally and Saudy, 2021; El-Metwally et al., 2022). Apart from regulating osmotic pressure, these compatible solutes have been advised to play a key character in shielding cells from ROS accumulation that occurs during stress conditions. While K+ is the primary contributor to osmotic adjustment during the primary phases of stress, molecules like Pro, GB and glucose become more important during later stages (Nio et al., 2011; El-Metwally et al., 2021). In the present study, Pro and soluble sugars displayed elevation in brassica napus plants subjected to water and salt stress, and these levels decreased further after SA treatments alone or in combination. In another study, sweet basil (Damalas, 2019) and maize (Bijanzadeh et al., 2019) were reported for enhanced Pro and GB levels after SA treatments under water-deficit stress.
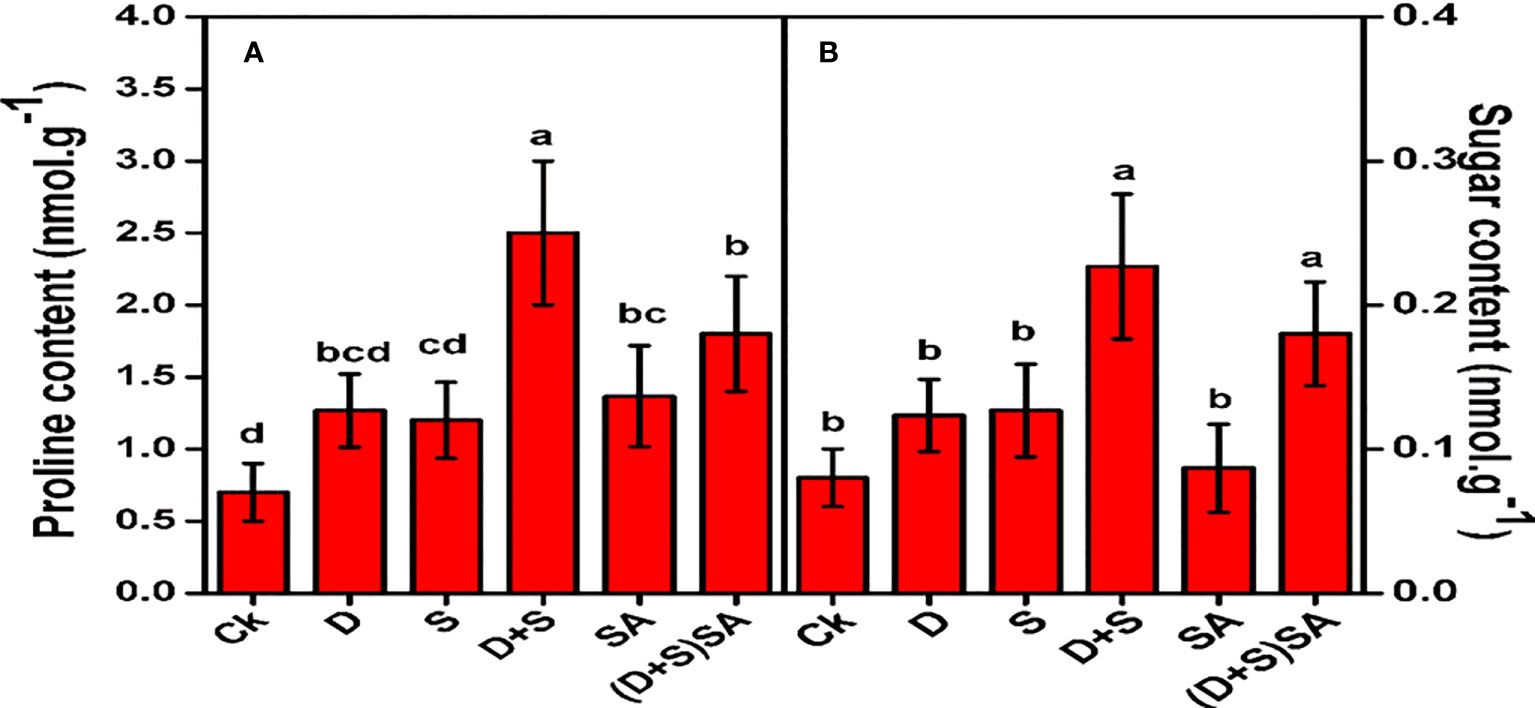
Figure 6 Effect of Drought, Salinity and SA on Proline (A) and sugar content (B) in leaves of Brassica napus genotype. Statistical differences at a 5% level exist between means labeled with different letters (a-e) for each attribute (mean ± SD; n = 3).
3.7 Accumulation of nutrient elements
Table 1 illustrates the influence of individual and combination of both drought and salinity stress condition on the concentration of nutrient elements. Both drought (D) and salinity (S), either single or in combination, significantly reduced the levels of Ca, Mg, Fe, and Zn. Drought stress decreases the mineral concentration by Ca (40.6%), Mg (27.6%), Fe (34.4%) and Zn (28.1%) as compare to unstressed plants. Similarly, mineral conc. for Ca (36.3%), Mg (33.5%), Fe (26.5%) and Zn (23.1%) also reduced in saline stress condition. Combined influence of D+S was immense and cause a decrease Ca conc. by (53.7%), Mg (54.8%), Fe (14%) and Zn (43.7%) as contrast to unstressed plants. However, when SA was administered alone or in combination with salinity and drought, it resulted in an increase in the levels of the aforementioned elements, Ca (19.4%), Mg (20.6%), Fe (40%) and Zn (36%) as compare to non-SA applied plants, indicating the beneficial effects of SA during stressful conditions (Table 1). Limited water availability due to drought and salinity generally results in a reduced uptake of nutrients and lower tissue concentrations in crops (Saudy and El-Metwally, 2019; Mubarak et al., 2021; Salem et al., 2021; Abd–Elrahman et al., 2022). One of the most notable effects of water scarcity is on the capacity of roots to soak up nutrients and their mobility to upper portion of the crop/plant as shoots (Farooq et al., 2009; Saudy et al., 2020; Salem et al., 2022). In another study it was reported that drought and salinity stress predominantly reduce the Zn, Fe, and Mn content found in shoot and roots of Linseed plants and further that SA application could regulate this declining patterns (Mohsen et al., 2019). SA plays very important and necessary function in regulating various nutrient content inside the plants under different various stresses conditions (Khan et al., 2010). For instance, the application of SA enhanced the inhibition of Calcium (Ca), Zinc (Zn) and Magnesium (Mg) absorption caused due to excessive Manganese (Mn) in and important vegetable as cucumber (Shi and Zhu, 2008), while it also mitigated the Cd-induced inhibition of Calcium (Ca), Magnesium (Mg) and Iron (Fe) absorption in Linum usitatissimum (Khan et al., 2010).
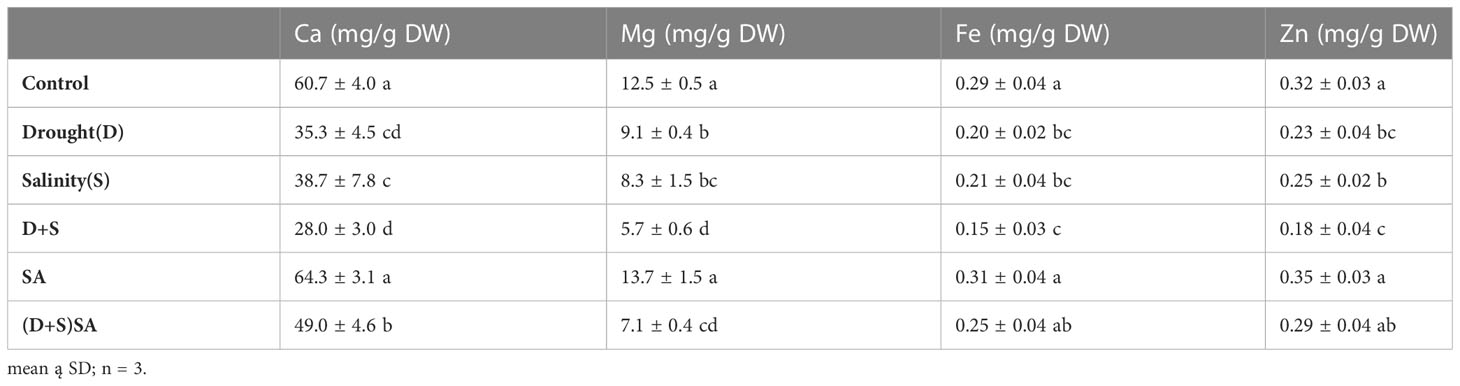
Table 1 Effect of drought (D), salinity (S), and SA on mineral nutrients; calcium (Ca), magnesium (Mg), zinc (Zn), and iron (Fe) in the leaves of Brassica napus genotypes.
3.8 Ultra-morphology of plants
In current study, the salt and water deficit stress resulted in obvious changes to the shape and size of chloroplasts in Brassica napus L. plants (Figure 7). Image further showed that the Chloroplast for Brassica napus plants subjected with stress conditions became oblate and spherical. Also an irregular and deformed shape of stroma thylakoids was observed, with grana lamella, and teas and faults decrease due to salt and drought stress (Figure 7). Chloroplast is the main power house for Photosynthesis process and the photoreaction is present inside the internal chloroplast membranes i.e., thylakoid. Drought and salt stress can disturb the sensitive formation of chloroplasts, destabilization of pigment-protein complexes, and breakdown of chlorophyll molecules, while also affect both quantity and makeup of carotenoids (Amirjani and Mahdiyeh, 2013). Our results are parallel to another study conducted by Ma et al. (2017) indicating that increasing abiotic stress levels especially salt stress in Dianthus superbus L. can induce the deformation in chloroplast size and shape, resulting into a oblate and spherical shape chloroplast, irregular grana with shrinked lamella and distorted thylakoid membrane structure. Another study reported that osmiophilic globules were found plastoglobuli in chloroplasts and linked with membrane breakdown, aging or senescence and may be stress factors (Lichtenthaler, 2013). Our results are also supported by another study reporting that saline stress may results in elevated ROS (H2O2, O2−) accumulation and damage the thylakoids membrane, thus reducing the photosynthetic rate (Ma et al., 2017). Elevated levels of NaCl lead to swelling of thylakoid membranes and reduction in chlorophyll fluorescence in the barley seedlings (Zahra et al., 2014). Both organelles, chloroplasts and mitochondria, are influenced differently in different cultivars under all given treatments. The drought-resistant wheat cultivar Katya demonstrates improved organelle preservation as compared to the sensitive variety Sadovo. Some studies have suggested that salicylic acid can alleviate stresses in different crops (Ali et al., 2018).
4 Conclusion
Based on findings of the study, it is concluded that applying Salicylic Acid (SA) during Salinity (S) and drought (D) stress conditions alone and their combinations (Salinity Drought) can help B. napus plants avoid damage by enriching different physiological as well as growth elements. The results on current study also convey important implication as they exhibit vigorous perspectives of SA applications while alleviating the ill-effects of D and S stress conditions. Further research is fortified to explore the specific utilizations involved and discover how SA can be applied in different farming practices.
Data availability statement
The raw data supporting the conclusions of this article will be made available by the authors, without undue reservation.
Author contributions
EA, SH, FJ, MAK, MIm, FSa, AAH, MAA, and FSh designed the experiments. EA carried out the field trials and collected the data. SH, MIs, SK, HMA, WFAM, and FSh helped in data analysis and article preparation. All authors contributed to the article and approved the submitted version.
Funding
This research work has been sponsored by the researchers supporting project number (RSP2023R316) sponsored by King Saud University (KSU), Riyadh, Saudi Arabia.
Acknowledgments
The authors extend their appreciation to the researchers supporting project number (RSP2023R316) at King Saud University (KSU), Riyadh, Saudi Arabia.
Conflict of interest
The authors declare that the research was conducted in the absence of any commercial or financial relationships that could be construed as a potential conflict of interest.
Publisher’s note
All claims expressed in this article are solely those of the authors and do not necessarily represent those of their affiliated organizations, or those of the publisher, the editors and the reviewers. Any product that may be evaluated in this article, or claim that may be made by its manufacturer, is not guaranteed or endorsed by the publisher.
References
Abbaszadeh, B., Layeghhaghighi, M., Azimi, R., Hadi, N. (2020). Improving water use efficiency through drought stress and using salicylic acid for proper production of Rosmarinus officinalis l. Ind. Crop Prod. 144, 111893. doi: 10.1016/j.indcrop.2019.111893
Abdel, L. A., Tran, L. S. (2016). Impacts of priming with silicon on the growth and tolerance of maize plants to alkaline stress. Front. Plant Sci. 7, 243–252. doi: 10.3389/fpls.2016.00243
Abd–Elrahman, S. H., Saudy, H. S., Abd El–Fattah, D. A., Hashem, F. A. (2022). Effect of irrigation water and organic fertilizer on reducing nitrate accumulation and boosting lettuce productivity. J. Soil Sci. Plant Nutr. 22, 2144–2155. doi: 10.1007/s42729-022-00799-8
Abid, M., Ali, S., Qi, L. K., Zahoor, R., Tian, Z., Jiang, D., et al. (2018). Physiological and biochemical changes during drought and recovery periods at tillering and jointing stages in wheat (Triticum aestivum L.). Sci. Rep. 8 (1), 4615. doi: 10.1038/s41598-018-21441-7
Ahanger, M. A., Alyemeni, M. N., Wijaya, L., Alamri, S. A., Alam, P., Ashraf, M., et al. (2018). Potential of exogenously sourced kinetin in protecting solanum lycopersicum from nacl-induced oxidative stress through up-regulation of the antioxidant system, ascorbate-glutathione cycle and glyoxalase system. PloS One 13, e0202175–e0202195. doi: 10.1371/journal.pone.0202175
Ahmad, P., Alyemeni, M. N., Al-Huqail, A. A., Alqahtani, M. A., Wijaya, L., Ashraf, M., et al. (2020). Zinc oxide nanoparticles application alleviates arsenic (as) toxicity in soybean plants by restricting the uptake of as and modulating key biochemical attributes, antioxidant enzymes, ascorbate-glutathione cycle and glyoxalase system. Plants 9, 825. doi: 10.3390/plants9070825
Ahmad, H. T., Hussain, A., Aimen, A., Jamshaid, M. U., Ditta, A., Asghar, H. N., et al. (2021). “Improving resilience against drought stress among crop plants through inoculation of plant growth-promoting rhizobacteria,” in Harsh environment and plant resilience: molecular and functional aspects, 1st, vol. 1 . Eds. Husen, A., Jawaid, M. (Cham, Switzerland: Springer), 387–408.
Ahmed, I. M., Cao, F., Zhang, M., Chen, X., Zhang, G., Wu, F. (2013b). Difference in yield and physiological features in response to drought and salinity combined stress during anthesis in Tibetan wild and cultivated barleys. PloS One 8 (10), e77869. doi: 10.1371/journal.pone.0077869
Ahmed, I. M., Nadira, U. A., Qiu, C. W., Cao, F., Zhang, G., Holford, P., et al. (2018). Tolerance to drought, low pH and Al combined stress in Tibetan wild barley is associated with improvement of ATPase and modulation of antioxidant defense system. Int. J. Mol. Sci. 19 (11), 3553. doi: 10.3390/ijms19113553
Akram, N. A., Iqbal, M., Muhammad, A., Ashraf, M., Al-Qurainy, F., Shafiq, S. (2018). Aminolevulinic acid and nitric oxide regulate oxidative defense and secondary metabolisms in canola (Brassica napus l.) under drought stress. Protoplasma 255, 163–174. doi: 10.1007/s00709-017-1140-x
Alavi, S. M. N., Arvin, M. J., Kalantari, K. M. (2014). Salicylic acid and nitric oxide alleviate osmotic stress in wheat (Triticum aestivum l.) seedlings. J. Plant Interac. 9, 683–688. doi: 10.1080/17429145.2014.900120
Ali, Q., Anwar, F., Ashraf, M., Saari, N., Perveen, R. (2013). Ameliorating effects of exogenously applied proline on seed composition, seed oil quality and oil antioxidant activity of maize (Zea mays l.) under drought stress. Int. J. Mol. Sci. 14, 818–835. doi: 10.3390/ijms14010818
Ali, S., Ganai, B. A., Kamili, A. N., Bhat, A. A., Mir, Z. A., Bhat, J. A., et al. (2018). Pathogenesis-related proteins and peptides as promising tools for engineering plants with multiple stress tolerance. Microbiol. Res. 212, 29–37. doi: 10.1016/j.micres.2018.04.008
Ali, Q., Javed, M. T., Noman, A., Haider, M. Z., Waseem, M., Iqbal, N., et al. (2018). Assessment of drought tolerance in mung bean cultivars/lines as depicted by the activities of germination enzymes, seedling’s antioxidative potential and nutrient acquisition. Arch. Agron. Soil Sci. 64, 84–102. doi: 10.1080/03650340.2017.1335393
Amirjani, M. R., Mahdiyeh, M. (2013). Antioxidative and biochemical responses of wheat to drought stress. J. Agric. Biol. Sci. 8, 291–301.
Anderson, R. E., Bayer, P., Edwards, D. (2020). Climate change and the need for agricultural adaptation. Curr. Opin. Plant Biol. 56, 197–202. doi: 10.1016/j.pbi.2019.12.006
Anjum, N. A., Gill, S. S., Gill, R. (2014a). Plant adaptation to environmental change: significance of amino acids and their derivatives. 1st (Wallingford: CABI).
Anjum, S. A., Xie, X., Wang, L., Saleem, M. F., Man, C., Lei, W. (2011). Morphological, physiological and biochemical responses of plants to drought stress. Afr. J. Agric. Res. 6, 2026–2032. doi: 10.5897/AJAR10.027
Annunziata, M. G., Ciarmiello, L. F., Woodrow, P., Dell’Aversana, E., Carillo, P. (2019). Spatial and temporal profile of glycine betaine accumulation in plants under abiotic stresses. Front. Plant Sci. 10. doi: 10.3389/fpls.2019.00230
Arif, Y., Sami, F., Siddiqui, H., Bajguz, A., Hayat, S. (2020). Salicylic acid in relation to other phytohormones in plant: a study towards physiology and signal transduction under challenging environment. Environ. Exp. Bot. 175, 104040. doi: 10.1016/j.envexpbot.2020.104040
Asgher, M., Khan, M. I. R., Anjum, N. A., Khan, N. A. (2015). Minimizing toxicity of cadmium in plants–role of plant growth regulators. Protoplasma 252, 399–413. doi: 10.1007/s00709-014-0710-4
Ashraf, M. (2009). Biotechnological approach of improving plant salt tolerance using antioxidants as markers. Biotechnol. Adv. 27, 84–93. doi: 10.1016/j.biotechadv.2008.09.003
Ashraf, M., Foolad, M. (2007). Roles of glycine betaine and proline in improving plant abiotic stress resistance. Environ. Exp. Bot. 59, 206–216. doi: 10.1016/j.envexpbot.2005.12.006
Ashraf, M., Harris, P. J. C. (2013). Photosynthesis under stressful environments: an overview. Photosynthetica 51, 163–190. doi: 10.1007/s11099-013-0021-6
Bijanzadeh, E., Naderi, R., Egan, T. P. (2019). Exogenous application of humic acid and salicylic acid to alleviate seedling drought stress in two corn (Zea mays l.) hybrids. J. Plant Nutr. 42, 1483–1495. doi: 10.1080/01904167.2019.1617312
Cirulis, J. T., Scott, J. A., Ross, G. M. (2013). Management of oxidative stress by microalgae. Can. J. Physiol. Pharmacol. 91, 15–21. doi: 10.1139/cjpp-2012-0249
Dalal, V. K., Tripathy, B. C. (2018). Water-stress induced downsizing of light-harvesting antenna complex protects developing rice seedlings from photo-oxidative damage. Sci. Rep. 8 (1), 5955. doi: 10.1038/s41598-017-14419-4.
Damalas, C. A. (2019). Improving drought tolerance in sweet basil (Ocimum basilicum) with salicylic acid. Sci. Hortic. 246, 360–365. doi: 10.1016/j.scienta.2018.11.005
Du, C., Hu, K., Xian, S., Liu, C., Fan, J., Tu, J., et al. (2016). Dynamic transcriptome analysis reveals AP2/ERF transcription factors responsible for cold stress in rapeseed (Brassica napus L.). Mol. Genet. Genomics 291 (3), 1053–1067. doi: 10.1007/s00438-015-1161-0
Dubois, M., Gilles, K., Hamilton, J. K., Rebers, P. A., Smith, F. A. (1951). Colorimetric method for the determination of sugars. Nature 168, 167. doi: 10.1038/168167a0
El-Mageed, T. A., Semida, W. M., Mohamed, G. F., Rady, M. M. (2016). Combined effect of foliar-applied salicylic acid and deficit irri-gation on physiological–anatomical responses, and yield of squash plants under saline soil. S. Afr. J. Bot. 106, 8–16. doi: 10.1016/j.sajb.2016.05.005
El-Metwally, I. M., Geries, L., Saudy, H. S. (2022). Interactive effect of soil mulching and irrigation regime on yield, irrigation water use efficiency and weeds of trickle–irrigated onion. Archiv. Agron. Soil Sci. 68, 1103–1116. doi: 10.1080/03650340.2020.1869723
El-Metwally, I. M., Saudy, H. S. (2021). Interactional impacts of drought and weed stresses on nutritional status of seeds and water use efficiency of peanut plants grown in arid conditions. Gesunde Pflanzen. 73, 407–416. doi: 10.1007/s10343-021-00557-3
El-Metwally, I. M., Saudy, H. S., Abdelhamid, M. T. (2021). Efficacy of benzyladenine for compensating the reduction in soybean productivity under low water supply. Ital. J. Agromet. 2, 81–90. doi: 10.36253/ijam-872
FAO, IFAD, UNICEF, WFP, WHO (2020). The state of food security and nutrition in the world, transforming food systems for affordable healthy diets (Rome: FAO).
Farooq, M., Wahid, A., Kobayashi, N., et al. (2009). Plant drought stress: effects, mechanisms and management. Agron. Sustain. Dev. 29, 185–212. doi: 10.1051/agro:2008021
Fayez, K. A., Bazaid, S. A. (2014). Improving drought and salinity tolerance in barley by application of salicylic acid and potassium nitrate. J. Saudi Soc Agric. Sci. 13, 45–55. doi: 10.1016/j.jssas.2013.01.001
Gil-Ortiz, R., Naranjo, M. A., Ruiz-Navarro, A., Caballero-Molada, M., Atares, S., García, C., et al. (2020). New eco-friendly polymeric-coated urea fertilizers enhanced crop yield in wheat. Agronomy 10, 438. doi: 10.3390/agronomy10030438
Gou, W., Tian, L., Ruan, Z., Zheng, P., Chen, F., Zhang, L., et al. (2015). Accumulation of choline and glycinebetaine and drought stress tolerance induced in maize (Zea mays) by three plant growth promoting rhizobacteria (PGPR) strains. Pak. J. Bot. 47, 581–586.
Habib, N., Akram, M. S., Javed, M. T., Azeem, M., Ali, Q., Shaheen, H. L., et al. (2016). Nitric oxide regulated improvement in growth and yield of rice plants grown under salinity stress: antioxidant defense system. Appl. Ecol. Environ. Res. 14, 91–105. doi: 10.15666/aeer/1405_091105
Habib, N., Ali, Q., Ali, S., Javed, M. T., Haider, M. Z., Perveen, R., et al. (2020). Use of nitric oxide and hydrogen peroxide for better yield of wheat (Triticum aestivum l.) under water deficit conditions: growth, osmoregulation, and antioxidative defense mechanism. Plants 9, 285. doi: 10.3390/plants9020285
Hatzig, S., Zaharia, L. I., Abrams, S., Hohmann, M., Legoahec, L., Bouchereau, A., et al. (2014). Early osmotic adjustment responses in drought-resistant and drought-sensitive oilseed rape. J. Integr. Plant Biol. 56, 797–809. doi: 10.1111/jipb.12199
Horvath, E., Szalai, G., Janda, T. (2007). Induction of abiotic stress tolerance by salicylic acid signaling. J. Plant Growth Regul. 26, 290–300. doi: 10.1007/s00344-007-9017-4
Hu, W. H., Yan, X. H., He, Y., Ye, X. L. (2018). Role of alternative oxidase pathway in protection against drought-induced photoinhibition in pepper leaves. Photosynthetica 56 (4), 1297–1303. doi: 10.1007/s11099-018-0837-1
Hussain, S., Liu, G., Liu, D., Hussain, N., Ahmad, M., Teng, Y. (2015). Study on the expression of dehydrin genes and activities of antioxidative enzymes in floral buds of two sand pear (Pyrus pyrifolia nakai) cultivars requiring different chilling requirements for bud break. Turkish J. Agric. Fores. 39, 930–939. doi: 10.3906/tar-1407-164
Ibrahim, H. A., Abdellatif, Y. M. (2016). Effect of maltose and trehalose on growth, yield and some biochemical components of wheat plant under water stress. Ann. Agric. Sci. 61, 267–274. doi: 10.1016/j.aoas.2016.05.002
Jesus, C., Meijón, M., Monteiro, P., Correia, B., Amaral, J., Escandón, M., et al. (2015). Salicylic acid application modulates physiological and hormonal changes in eucalyptus globulus under water deficit. Environ. Exp. Bot. 118, 56–66. doi: 10.1016/j.envexpbot.2015.06.004
Khalifa, G. S., Abdelrassoul, M., Hegazi, A. M., Elsherif, M. H. (2016). Attenuation of negative effects of saline stress in two lettuce cultivars by salicylic acid and glycine betaine. Gesunde Pflanzen. 68, 177–189. doi: 10.1007/s10343-016-0376-2
Khan, M. I. R., Asgher, M., Khan, N. A. (2014). Alleviation of salt-induced photosynthesis and growth inhibition by salicylic acid involves glycinebetaine and ethylene in mungbean (Vigna radiata l.). Plant Physiol. Biochem. 80, 67–74. doi: 10.1016/j.plaphy.2014.03.026
Khan, M. I. R., Iqbal, N., Masood, A., Per, T. S., Khan, N. A. (2013b). Salicylic acid alleviates adverse effects of heat stress on photosynthesis through changes in proline production and ethylene formation. Plant Signal. Behav. 8, e26374. doi: 10.4161/psb.26374
Khan, N. A., Syeed, S., Masood, A., Nazar, R., Iqbal, N. (2010). Application of salicylic acid increases contents of nutrients and antioxidative metabolism in mung bean and alleviates adverse effects of salinity stress. Inter. J. Plant Biol. 1 (1), e1. doi: 10.4081/pb.2010.e1
Kosar, F., Akram, N. A., Ashraf, M., Sadiq, M., Al-Qurainy, F. (2018). Trehalose-induced improvement in growth, photosynthetic characteristics and levels of some key osmoprotectants in sunflower (Helianthus annuus L.) under drought stress. Pak. J. Bot. 50, 955–961.
Kotula, L., Garcia-Caparros, P., Zörb, C., Colmer, T. D., Flowers, T. J. (2020). Improving crop salt tolerance using transgenic approaches: an update and physiological analysis. Plant Cell Environ 43 (12), 2932–2956. doi: 10.1111/pce.13865
Kumar, D. (2014). Salicylic acid signaling in disease resistance. Plant Sci. 228, 127–124. doi: 10.1016/j.plantsci.2014.04.014
La, V. H., Lee, B. R., Islam, M. T., Park, S. H., Jung, H. I., Bae, D. W., et al. (2019). Characterization of salicylic acid-mediated mod-ulation of the drought stress responses: reactive oxygen species, proline, and redox state in. Environ. Exp. Bot. 157, 1–10. doi: 10.1016/j.envexpbot.2018.09.013
La, V. H., Lee, B.-R., Zhang, Q., Park, S.-H., Islam, T., Kim, T. H. (2018). Salicylic acid improves drought-stress tolerance by regulating the redox status and proline metabolism in Brassica rapa. Hortic. Environ. Biotechnol. 60, 31–40. doi: 10.1007/s13580-018-0099-7
Levy, D., Coleman, W. K., Veilleux, R. E. (2013). Adaptation of potato to water shortage: irrigation management and enhancement of tolerance to drought and salinity. Amer. J. Potato. Res. 90, 1–21. doi: 10.1007/s12230-012-9291-y
Lichtenthaler, H. K. (1987). “Methods in enzymology,” in [34] chlorophylls and carotenoids: pigments of photosynthetic biomembranes, vol. 148. (Cambridge, MA, USA: Academic Press), 350–382.
Lichtenthaler, H. K. (2013). “Plastoglobuli, thylakoids, chloroplast structure and development of plastids,” in Plastid development in leaves during growth and senescence. Eds. Biswal, B., Krupinska, K., Biswal, U. C. (Dordrecht: Springer), 337–361.
Luo, J., Tang, S., Mei, F., Peng, X., Li, J., Li, X., et al. (2017). BnSIP1-1, a trihelix family gene, mediates abiotic stress tolerance and ABA signaling in Brassica napus. Front. Plant Sci. 8. doi: 10.3389/fpls.2017.00044
Luo, J., Tang, S., Peng, X., Yan, X., Zeng, X., Li, J., et al. (2015). Elucidation of cross-talk and specificity of early response mechanisms to salt and PEG-simulated drought stresses in brassica napus using comparative proteomic analysis. PloS One 10 (10), e0138974. doi: 10.1371/journal.pone.0138974
Lv, Y., Fu, S., Chen, S., Zhang, W., Qi, C. (2016). Ethylene response factor BnERF2-like (ERF2.4) from Brassica napus L. enhances submergence tolerance and alleviates oxidative damage caused by submergence in arabidopsis thaliana. Crop J. 4 (3), 199–211. doi: 10.1016/j.cj.2016.01.004
Ma, X., Zheng, J., Zhang, X., Hu, Q., Qian, R. (2017). Salicylic acid alleviates the adverse effects of salt stress on dianthus superbus (Caryophyllaceae) by activating photosynthesis, protecting morphological structure, and enhancing the antioxidant system. Front. Plant Sci. 8. doi: 10.3389/fpls.2017.00600
Mafakheri, A., Siosemardeh, A., Bahramnejad, B., Struik, P. C., Sohrabi, Y. (2010). Effect of drought stress on yield, proline and chlorophyll contents in three chickpea cultivars. Aust. J. Crop Sci. 4, 580–585.
Maghsoudi, K., Emam, Y., Ashraf, M., Arvin, M. J. (2019). Alleviation of field water stress in wheat cultivars by using silicon and salicylic acid applied separately or in combination. Crop Pasture Sci. 70, 36. doi: 10.1071/CP18213
Mittler, R. (2006). Abiotic stress, the field environment and stress combination. Trends Plant Sci. 11, 15–19. doi: 10.1016/j.tplants.2005.11.002
Miura, K., Tada, Y. (2014). Regulation of water, salinity, and cold stress responses by salicylic acid. Front. Plant Sci. 5. doi: 10.3389/fpls.2014.00004
Mohsen, M. D., Yaqoub, B., Nasrin, N., Raham, M. (2019). Salicylic acid mitigates the effects of drought and salinity on nutrient and dry matter accumulation of linseed. J. Plant Process Fun. 8, 31.
Mubarak, M., Salem, E. M. M., Kenawey, M. K. M., Saudy, H. S. (2021). Changes in calcareous soil activity, nutrient availability, and corn productivity due to the integrated effect of straw mulch and irrigation regimes. J. Soil Sci. Plant Nutr. 21, 2020–2031. doi: 10.1007/s42729-021-00498-w
Najafabadi, M. Y., Ehsanzadeh, P. (2017). Photosynthetic and antioxidative upregulation in drought-stressed sesame (Sesamum indicum L.) subjected to foliar-applied salicylic acid. Photosynthetica 55, 611–622. doi: 10.1007/s11099-017-0673-8
Naz, H., Akram, N. A., Ashraf, M. (2016). Impact of ascorbic acid on growth and some physiological attributes of cucumber (Cucumis sativus) plants under water-deficit conditions. Pak. J. Bot. 48, 877–883.
Nazar, R., Iqbal, N., Syeed, S., Khan, N. A. (2015). Salicylic acid alleviates decreases in photosynthesis under salt stress by enhancing nitrogen and sulfur assimilation and antioxidant metabolism differentially in two mungbean cultivars. J. Plant Physiol. 168, 807–815. doi: 10.1016/j.jplph.2010.11.001
Nio, S., Cawthray, G., Wade, L., Colmer, T. (2011). Pattern of solutes accumulated during leaf osmotic adjustment as related to duration of water deficit for wheat at the reproductive stage. Plant Physiol. Biochem. 49, 1126–1137. doi: 10.1016/j.plaphy.2011.05.011
Noman, A., Ali, Q., Maqsood, J., Iqbal, N., Javed, M. T., Rasool, N., et al. (2018). Deciphering physio-biochemical, yield, and nutritional quality attributes of water-stressed radish (Raphanus sativus l.) plants grown from zn-lys primed seeds. Chemosphere 195, 175–189. doi: 10.1016/j.chemosphere.2017.12.059
Ozkur, O., Ozdemir, F., Bor, M., Turkan, I. (2009). Physiochemical and antioxidant responses of the perennial xerophyte capparis ovata desf. to drought. Environ. Exp. Bot. 66, 487–492. doi: 10.1016/j.envexpbot.2009.04.003
Palma, F., López-Gómez, M., Tejera, N. A., Lluch, C. (2013). Salicylic acid improves the salinity tolerance of medicago sativa in symbiosis with sinorhizobium meliloti by preventing nitrogen fixation inhibition. Plant Sci. 208, 75–82. doi: 10.1016/j.plantsci.2013.03.015
Per, T. S., Khan, N. A., Reddy, P. S., Masood, A., Hasanuzzaman, M., Khan, M. I. R., et al. (2017). Approaches in modulating proline metabolism in plants for salt and drought stress tolerance: phytohormones, mineral nutrients and transgenics. Plant Physiol. Biochem. 115, 126–140. doi: 10.1016/j.plaphy.2017.03.018
Pérez-López, U., Robredo, A., Lacuesta, M., Mena-Petite, A., Muñoz-Rueda, A. (2012). Elevated CO2 reduces stomatal and metabolic limitations on photosynthesis caused by salinity in hordeum vulgare. Photosynth. Res. 111, 269–283. doi: 10.1007/s11120-012-9721-1
Pokhrel, Y., Felfelani, F., Satoh, Y., Boulange, J., Burek, P., Gädeke, A., et al. (2021). Global terrestrial water storage and drought severity under climate change. Nat. Clim. Change 11, 226–233. doi: 10.1038/s41558-020-00972-w
Qaseem, M. F., Qureshi, R., Shaheen, H. (2019). Effects of pre-anthesis drought, heat and their combination on the growth, yield and physiology of diverse wheat (Triticum aestivum L.) genotypes varying in sensitivity to heat and drought stress. Sci. Rep. 9 (1), 6955. doi: 10.1038/s41598-019-43477-z.
Raza, A. (2020a). Eco-physiological and biochemical responses of rapeseed (Brassica napus L.) to abiotic stresses: consequences and mitigation strategies. J. Plant Growth Regul. doi: 10.1007/s00344-020-10231-z
Raza, A., Ashraf, F., Zou, X., Zhang, X., Tosif, H. (2020a). “Plant adaptation and tolerance to environmental stresses: mechanisms and perspectives,” in Plant ecophysiology and adaptation under climate change: mechanisms and perspectives I. Ed. Hasanuzzaman, M. (Singapore: Springer), 117–145.
Raza, A., Razzaq, A., Mehmood, S. S., Zou, X., Zhang, X., Lv, Y., et al. (2019a). Impact of climate change on crops adaptation and strategies to tackle its outcome: a review. Plants 8, 34. doi: 10.3390/plants8020034
Rollins, J., Habte, E., Templer, S., Colby, T., Schmidt, J., von Korff, M. (2013). Leaf proteome alterations in the context of physiological and morphological responses to drought and heat stress in barley ( Hordeum vulgare l.). J. Exp. Bot. 64, 3201–3212. doi: 10.1093/jxb/ert158
Sabagh, A. E., Hossain, A., Barutçular, C., Islam, M. S., Ratnasekera., D., Kumar, N., et al. (2019). Drought and salinity stress management for higher and sustainable canola (‘Brassica napus’ L.) production: a critical review. Aust. J. Crop Sci. 13, 88. doi: 10.21475/ajcs.19.13.01.p1284
Salem, E. M. M., Kenawey, M. K. M., Saudy, H. S., Mubarak, M. (2021). Soil mulching and deficit irrigation effect on sustainability of nutrients availability and uptake, and productivity of maize grown in calcareous soils. Commun. Soil Sci. Plant Anal. 52, 1745–1761. doi: 10.1080/00103624.2021.1892733
Salem, E. M. M., Kenawey, M. K. M., Saudy, H. S., Mubarak, M. (2022). Influence of silicon forms on nutrient accumulation and grain yield of wheat under water deficit conditions. Gesunde Pflanzen. 74, 539–548. doi: 10.1007/s10343-022-00629-y\
Sarabi, B., Fresneau, C., Ghaderi, N., Bolandnazar, S., Streb, P., Badeck, F. W., et al. (2019). Stomatal and non-stomatal limitations are responsible in down-regulation of photosynthesis in melon plants grown under the saline condition: application of carbon isotope discrimination as a reliable proxy. Plant Physiol. Biochem. 141, 1–19. doi: 10.1016/j.plaphy.2019.05.010
Saudy, H. S., El-Bially, M. A., El-Metwally, I. M., Shahin, M. G. (2021). Physio–biochemical and agronomic response of ascorbic acid–treated sunflower (Helianthus annuus) grown at different sowing dates and under various irrigation regimes. Gesunde Pflanzen. 73, 169–179. doi: 10.1007/s10343-020-00535-1
Saudy, H. S., El-Metwally, I. M. (2019). Nutrient utilization indices of NPK and drought management in groundnut under sandy soil conditions. Commun. Soil Sci. Plant Anal. 50, 1821–1828. doi: 10.1080/00103624.2019.1635147
Saudy, H. S., El-Metwally, I. M., Abd El-Samad, G. A. (2020). Physio–biochemical and nutrient constituents of peanut plants under bentazone herbicide for broad–leaved weed control and water regimes in dry land areas. J. Arid. Land. 12 (4), 630–639. doi: 10.1007/s40333-020-0020-y
Saudy, H. S., Salem, E. M. M., Abd El–Momen, W. R. (2023b). Effect of potassium silicate and irrigation on grain nutrient uptake and water use efficiency of wheat under calcareous soils. Gesunde Pflanzen. 75, 647–654. doi: 10.1007/s10343-022-00729-9
Scandalios, J. (2005). Oxidative stress: molecular perception and transduction of signals triggering antioxidant gene defenses. Braz. J. Med. Biol. Res. 38, 995–1014. doi: 10.1590/S0100-879X2005000700003
Shaaban, A., Abd El-Mageed, T. A., Abd El-Momen, W. R., Saudy, H. S., Al-Elwany, O. A. A. I. (2023). The integrated application of phosphorous and zinc affects the physiological status, yield and quality of canola grown in phosphorus-suffered deficiency saline soil. Gesunde Pflanzen, 75. doi: 10.1007/s10343-023-00843-2
Shafiq, S., Akram, N. A., Ashraf, M., Arshad, A. (2014). Synergistic effects of drought and ascorbic acid on growth, mineral nutrients and oxidative defense system in canola (Brassica napus l.) plants. Acta Physiol. Plant 36, 1539–1553. doi: 10.1007/s11738-014-1530-z
Shahzad, H., Ullah, S., Iqbal, M., Bilal, H. M., Shah, G. M., Ahmad, S., et al. (2019). Salinity types and level-based effects on the growth, physiology and nutrient contents of maize (Zea mays). Ital. J. Agron. 14, 199–207. doi: 10.4081/ija.2019.1326
Shao, R. X., Xin, L. F., Zheng, H. F., Li, L. L., Ran, W. L., Mao, J., et al. (2016). Changes in chloroplast ultrastructure in leaves of drought-stressed maize inbred lines. Photosynthetica 54 (1), 74–80. doi: 10.1007/s11099-015-0158-6
Shi, Q. H., Zhu, Z. J. (2008). Effects of exogenous salicylic acid on manganese toxicity, element contents and antioxidative system in cucumber. Environ. Exp. Bot. 63, 317–326. doi: 10.1016/j.envexpbot.2007.11.003
Syeed, S., Nazar, A., Iqbal, R., Masood, A., Khan, N. A. (2011). Salicylic acid-mediated changes in photosynthesis, nutrients content and antioxidant metabolism in two mustard (Brassica juncea l.) cultivars differing in salt tolerance. Acta Physiol. Plant 33, 877–886. doi: 10.1007/s11738-010-0614-7
USDA-FAO (2018) American Geophysical union. Available at: https://sites.agu.org.
Wang, Y., Zhang, J., Li, J. L., Ma, X. R. (2014). Exogenous hydrogen peroxide enhanced the thermotolerance of festuca arundinacea and lolium perenne by increasing the antioxidative capacity. Acta Physiol. Plant 36, 2915–2924. doi: 10.1007/s11738-014-1661-2
Wu, D., Cai, S., Chen, M., Ye, L., Chen, Z., Zhang, H., et al. (2013). Tissue metabolic responses to salt stress in wild and cultivated barley. PloS One 8, e55431. doi: 10.1371/journal.pone.0055431
Yadav, N., Sharma, S., Samir, P. (2016). Research, reactive oxygen species, oxidative stress and ROS scavenging system in plants. J. Chem. Pharm. Res. 8, 595–604.
Yadu, S., Dewangan, T. L., Chandrakar, V., Keshavkant, S. (2017). Imperative roles of salicylic acid and nitric oxide in improving salinity tolerance in Pisum sativum l. Physiol. Mol. Biol. Plants. 23, 43–58. doi: 10.1007/s12298-016-0394-7
Yang, F., Xu, X., Xiao, X., Li, C. (2010). Responses to drought stress in two poplar species originating from water & nitrogen use efficiency in plants and crops (UK: Marston, Lincolnshire), 15–16.
Yousfi, S., Serret, M. D., Araus, J. L., Draye, X., Foulkes, J., Hawkesford, M., et al. (2010). A comparative effect of salinity and drought on growth, ion concentration and δ13C and δ15N in barley. Assoc. Appl. Biol. 105, 73–81.
Yousfi, S., Serret, M. D., Márquez, A., Voltas, J., Araus, J. L. (2012). Combined use of δ13C, δ18O and δ15N tracks nitrogen metabolism and genotypic adaptation of durum wheat to salinity and water deficit. New Phytol. 194, 230–244. doi: 10.1111/j.1469-8137.2011.04036.x
Yudina, L., Sukhova, E., Gromova, E., Nerush, V., Vodeneev, V., Sukhov, V. (2020). A light-induced decrease in the photochemical reflectance index (PRI) can be used to estimate the energy-dependent component of non-photochemical quenching under heat stress and soil drought in pea, wheat, and pumpkin. Photosynth. Res. 146 (1-3), 175–187. doi: 10.1007/s11120-020-00718-x
Zahra, J., Nazim, H., Cai, S., Han, Y., Wu, D., Zhang, B., et al. (2014). The influence of salinity on cell ultrastructures and photosynthetic apparatus of barley genotypes differing in salt stress tolerance. Acta Physiol. Planta. 36 (5), 1261–1269. doi: 10.1007/s11738-014-1506-z
Zhang, Y., Xu, S., Yang, S., Chen, Y. (2015). Salicylic acid alleviates cadmium-induced inhibition of growth and photosynthesis through upregulating antioxidant defense system in two melon cultivars (Cucumis melo l.). Protoplasma 252, 911–924. doi: 10.1007/s00709-014-0732-y
Zhang, H., Zhu, J., Gong, Z. Z. J. (2022). Abiotic stress responses in plants. Nat. Rev. Genet. 23, 104–119. doi: 10.1038/s41576-021-00413-0
Keywords: abiotic stressors, antioxidant enzymes, mineral nutrients, oilseed rape, osmo-regulatory substances, photosynthetic pigments
Citation: Ali E, Hussain S, Jalal F, Khan MA, Imtiaz M, Said F, Ismail M, Khan S, Ali HM, Hatamleh AA, Al-Dosary MA, Mosa WFA and Shah F (2023) Salicylic acid-mitigates abiotic stress tolerance via altering defense mechanisms in Brassica napus (L.). Front. Plant Sci. 14:1187260. doi: 10.3389/fpls.2023.1187260
Received: 15 March 2023; Accepted: 23 June 2023;
Published: 25 July 2023.
Edited by:
Jianfeng Zhang, Chinese Academy of Forestry, ChinaReviewed by:
Rehab Hafez, Cairo University, EgyptShahbaz Khan, Colorado State University, United States
Hani Saudy, Ain Shams University, Egypt
Copyright © 2023 Ali, Hussain, Jalal, Khan, Imtiaz, Said, Ismail, Khan, Ali, Hatamleh, Al-Dosary, Mosa and Shah. This is an open-access article distributed under the terms of the Creative Commons Attribution License (CC BY). The use, distribution or reproduction in other forums is permitted, provided the original author(s) and the copyright owner(s) are credited and that the original publication in this journal is cited, in accordance with accepted academic practice. No use, distribution or reproduction is permitted which does not comply with these terms.
*Correspondence: Sayed Hussain, sayedhussain@awkum.edu.pk; Farooq Shah, farooqshah@awkum.edu.pk