- 1Graduate School of Bioresource and Bioenvironmental Sciences, Kyushu University, Fukuoka, Japan
- 2Faculty of Agriculture, Kyushu University, Fukuoka, Japan
- 3Graduate School of Agricultural and Life Science, University of Tokyo, Tokyo, Japan
- 4Biological Resources and Post-harvest Division, Japan International Research Center for Agricultural Sciences, Tsukuba, Japan
- 5Graduate School of Agriculture, Kindai University, Nara, Japan
- 6Biologie des Semences, Unité Mixte de Recherche (UMR) 7622, The Institut de Biologie Paris-Seine (IBPS), Sorbonne Université, Paris, France
Climate change due to global warming is now affecting agricultural production worldwide. In rice, one of the most important crops, water limitation due to irregular rainfall in rainfed lowlands during crop growth limits yield. Dry direct-sowing has been proposed as a water-efficient approach to cope with water stress during rice growth, but poor seedling establishment due to drought during germination and emergence is a problem. Here, we germinated indica rice cultivars Rc348 (drought tolerant) and Rc10 (drought sensitive) under osmotic stress induced by PEG to elucidate mechanisms of germination under drought. Rc348 had higher germination rate and germination index under severe osmotic stress of −1.5 MPa, above those of Rc10. Rc348 showed up-regulated GA biosynthesis, down-regulated ABA catabolism, and up-regulated α-amylase gene expression in imbibed seeds under PEG treatment compared to that of Rc10. During germination, reactive oxygen species (ROS) play important roles in antagonism between gibberellic acid (GA) and abscisic acid (ABA). Embryo of Rc348 treated with PEG had significantly greater expression of NADPH oxidase genes and higher endogenous ROS levels, together with significantly increased endogenous GA1, GA4 and ABA contents compared to that of Rc10. In aleurone layers treated with exogenous GA, expression of α-amylase genes was higher in Rc348 than in Rc10, and expression of NADPH oxidase genes was enhanced with significantly higher ROS content in Rc348, suggesting higher sensitivity of GA to ROS production and starch degradation in aleurone cells of Rc348. These results suggest that the osmotic stress tolerance of Rc348 is due to enhancement of ROS production, GA biosynthesis, and GA sensitivity, resulting in a higher germination rate under osmotic stress.
1 Introduction
Rice (Oryza sativa L.) is one of the most important staple crops, feeding a third of the world’s population. It is produced mainly in Asia, largely in flooded conditions. Increasing demand for rice production and diminishing rainfall due to climate change profoundly affect rice production, for which reliable irrigation is crucial (Vries et al., 2010). Rainfed lowland covers more than 30% of rice cultivation areas globally and in major rice producing countries (Matloob et al., 2015; Gadal et al., 2019). However, weather fluctuations due to climate change in recent years and the irregular rainfall in rainfed lowlands, often delay transplantation (Ohno et al., 2018). Prolonged water stress during the transition from vegetative stage to reproductive stage delays heading and significantly reduces yield (Pantuwan et al., 2002). To cope with water stress in rice, dry direct-sowing (DDS) has been proposed as a water-efficient approach, since it uses much less water than transplantation into puddled fields (Haefele et al., 2016). DSS method has been adopted in many countries (Shekhawat et al., 2020), which more than 25% of total rice production in tropical regions in Asia, and more than 90% of rice cultivated areas in the United States and Sri Lanka depend on DDS (Kumar and Ladha, 2011; Subedi et al., 2019). DDS is a promising approach for rainfed rice cropping, using less labor and having no need for irrigation or seedling preparation (Hayashi et al., 2007; Kato and Katsura, 2014). However, it faces problems of weed infestation and poor seedling establishment if drought occurs during germination and emergence (Yamane et al., 2017; Ohno et al., 2018).
Germination is a crucial developmental stage and is regulated by many factors, including the phytohormones gibberellic acid (GA), which induces germination, and abscisic acid (ABA), which suppresses germination (Liu et al., 2010; Ishibashi et al., 2012; Jacobsen et al., 2020). Biosynthesis of GA involves many catalytic enzymes, including ent-kaurene acid oxidase (KAO), GA 20-oxidase (GA20ox), and GA 3-oxidase (GA3ox) (Hedden and Phillips, 2000). ABA is synthesized by the enzyme 9-cis epoxycarotenoid dioxygenase (NCED) and is biodegraded by a cytochrome P450 monooxygenase or ABA 8’-hydroxylase (ABA8′OH) (Millar et al., 2006). Reactive oxygen species (ROS) as developmental and stress-signaling molecules are also involved via an ‘oxidative window’, wherein ROS homeostasis regulates germination (Bailly et al., 2008). ROS produced by NADPH oxidases during seed imbibition induce the production of GA and inhibit ABA to promote germination, which ROS homeostasis is important for abiotic stress responses via phytohormone signaling in many species (Oracz et al., 2007; Bailly et al., 2008; Ishibashi et al., 2010; Ishibashi et al., 2012; Ye et al., 2012; El-Maarouf-Bouteau et al., 2015; Shi et al., 2020; Wu et al., 2020). To degrade stored starch, the production of α-amylases, starch-hydrolyzing enzymes, are regulated by GA and ABA signaling factors such as GAMYB (GA-induced MYB-like transcription factor) and PKABA (ABA-induced protein kinase ABA-responsive protein kinase) which induces and inhibits expression of α-amylases, respectively, in cereal aleurone layers (Gubler et al., 1999; Gomez-Cadenas et al., 2001; Kaneko et al., 2002; Woodger et al., 2003; Ishibashi et al., 2012). Under osmotic stress caused by polyethylene glycol (PEG), α-amylase activity is inhibited, and germination is impaired (Bialecka and Kepczynski, 2010; Muscolo et al., 2013).
Indica rice Rc348 is a newly released drought-tolerant DDS cultivar that has a higher seedling emergence rate than the common and widely grown drought-sensitive cultivar Rc10, resulting in higher yield under drought stress on farm experiments in the Philippines (Yamane et al., 2017; Ohno et al., 2018). Both germination ability and seedling establishment are crucial for later growth and development (Yamane et al., 2017; Ohno et al., 2018). Although many studies have suggested drought-tolerant traits and cultivars for DDS cropping, the molecular mechanisms underlying drought responses of tolerant cultivars, especially in germination, are not yet well studied.
Here, we focused on germination ability of Rc348 under osmotic stress. We aimed at elucidating how different rice cultivars respond to osmotic pressure, an important component of drought stress, at the transcriptional, hormonal, and ROS levels during seed imbibition.
2 Materials and methods
2.1 Plant materials and growth conditions
Three-week-old seedlings of indica rice (Oryza sativa L.) cvv. Rc348, Rc10, Rc420, Rc222, and Dular were transplanted into 1/2000-a Wagner pots (5 plants per pot) with 32.8 g of basal dressing compound fertilizer (N–P–K: 4%–4%–4%) and 3.2 g of sigmoid-type controlled-release coated urea. Topdressing of 1.88 g of ammonium sulfate (21% N) per pot was applied during the tiller development stage and the panicle booting stage. Plants were grown under natural conditions at Kyushu University, Fukuoka, Japan, from mid-May to late-October in 2019. Anthesis, the day when spikelets on the upper primary rachis branches flowered on >50% of the population, was set as the day of flowering (0 DAF; days after flowering). Plants were harvested at 49 DAF. Harvested seeds were dried at room temperature for 1 week and stored at −30°C to maintain dormancy. Seed morphology of all cultivars are shown in Supplemental Figure 1.
2.2 Seed germination test under osmotic stress and exogenous chemical treatments
Seeds of all cultivars underwent dormancy break treatment at 45°C in the dark for 2 weeks to ensure a uniform degree of seed dormancy. Seeds were rested at room temperature for 1 h, sterilized in 0.2% NaClO for 20 min and washed thoroughly in sterilized distilled water; 30 seeds were placed in 9-cm Petri dishes with 10 mL of sterilized distilled water (control) or −0.5, −1.0, or −1.5 MPa of PEG 4000 solution (Nacalai Tesque inc., Kyoto, Japan) to germinate at 28°C in the dark. Germination rates were recorded every 6 h until 144 h after imbibition (HAI). A seed was recorded as germinated when shoot length was ≥0.2 cm. The germination index of each sample was calculated as described by Coolbear and Grierson (1984).
In the experiment with exogenous GA and ABA, embryoless half-seeds were imbibed in 10 mL of 1 µM GA3 in −1.5-MPa PEG solution on a filter paper in a Petri dish at 28°C in the dark, with or without 5 µM ABA, and transcript levels of GAMYB, SAPK, α-amylase, and NADPH oxidase genes were analyzed at 24 HAI and endogenous ROS content was measured at 36 HAI.
In the GA sensitivity experiment, embryoless half-seeds were imbibed in 10 mL of 1 µM GA3 in −1.5-MPa PEG solution on a filter paper in a Petri dish at 28°C in the dark and sampled at 36 HAI for endogenous hydrogen peroxide content measurement.
In the experiment with exogenous sodium ascorbate, seeds were imbibed in 10 mL of -1.5 MPa PEG or 5, 15 and 25 mM of sodium ascorbate dissolved in -1.5MPa PEG solution. Germination percentage, gene expression and endogenous hormonal levels were analyzed at 84 HAI.
Seeds were imbibed with 6 mL of -1.5 MPa PEG supplied with exogenous 100 μM Diphenyleneiodonium chloride (DPI) or 10, 20, and 50 mM H2O2 with equal amount of DMSO to that of DPI solution. Germination rates were recorded with the same methods above.
2.3 RNA extraction and quantitative real-time PCR analysis
Total RNA from whole seeds, embryos, and embryoless half-seeds was extracted from frozen materials by the SDS/phenol/LiCl method (Chirgwin et al., 1979). cDNA was synthesized from extracted RNA with ReverTra Ace reverse transcriptase (Toyobo co., Ltd., Osaka, Japan) according to the manufacturer’s instructions. Quantitative real-time PCR was performed on a CFX Connect Optics Module Real-time PCR detector system (Bio-Rad) with SYBR Green dye (Toyobo) as described in the manufacturer’s instructions. PCR thermal cycling conditions were as follows: initial denaturation at 94°C for 2 min; 40 cycles of denaturation at 94°C for 20 s, annealing at a primer-specific temperature for 20 s (Table S1), and extension at 72°C for 20 s; followed by melting and plate reading. The data were normalized to the expression of OsActin.
2.4 Endogenous GA and ABA contents
Endogenous GA1, GA4, and ABA contents in embryos imbibed in −1.5-MPa PEG at 72 HAI were analyzed by LC-MS/MS (Exion LC and X500B, AB Sciex) as described by Xin et al. (2020). Three biological replicates were measured, each comprising embryos from 300 seeds. Isotope internal standards of GA1, GA4, and ABA were purchased from OlChemIm (Olomouc, Czech Republic).
2.5 NADPH oxidase enzyme activity
Embryos of 30 seeds from Rc348 and Rc10 (−1.5 MPa PEG at 48 HAI) were ground into fine power with liquid nitrogen. Ice cold 2 mL of Na-phosphate buffer (pH 8.0) was added to the sample and the contents were mixed and sonicate for 15 s prior to centrifugation at 16,000 g for 15 min at 4°C. Crude embryo homogenates were precipitated with acetone (9:1, acetone:homogenate) at −30°C for 15 min. Precipitated proteins were collected from centrifugation at 12,500 rpm for 10 min at 4°C. Protein pallets were resuspended in reaction buffer (50 mM Tris-HCl pH 8.0, 0.1 mM MgCl2, 0.25 M sucrose and 0.1% Triton-X100) and used for enzyme activity assay. The reaction of NADPH-dependent superoxide generation was measured using NBT (nitro blue tetrazolium chloride) at 530 nm in a spectrophotometer (Gynesys 40, Thermofisher Scientific) as previously described (Van Gestelen et al., 1997; Sarath et al., 2007; Ishibashi et al., 2010). Monoformazan concentrations were calculated using an extinction coefficient of 12.8 mM-1 cm-1.
2.6 Endogenous hydrogen peroxide content
Embryos of 20 seeds imbibed in −1.5-MPa PEG and embryoless half-seeds imbibed in 1 µM GA1 in −1.5-MPa PEG were sampled at 24 and 36 HAI, respectively. Samples were snap-frozen in liquid nitrogen and stored at −80°C before analysis. Samples were homogenized in 2 mL of 0.2 M perchloric acid on ice and centrifuged at 13,000 rpm at 4°C for 15 min. Supernatant (0.5 mL) was mixed with 0.5 mL of 4 M KOH, and samples were centrifuged at 1000× g at 4°C for 5 min. The H2O2 content was measured by peroxidase-based assay as described by Ishibashi et al. (2015) and O’Kane et al. (1996).
2.7 Statistical analysis
Statistical analyses in this study were performed in SPSS statistical software version 28.0.0.0 (IBM). Differences among treatments were analyzed by one-tailed Student’s t-test and Tukey’s test with biological replications described in figure legends.
3 Results
3.1 Delayed germination under osmotic stress
Imbibition of seeds of all five cultivars in PEG suppressed germination in a concentration-dependent manner (Figure 1A). Under severe osmotic pressure of −1.5 MPa, Rc348 had the fastest germination and the highest final germination rate of about 50%, whereas those of the other cultivars were ≤20%. Rc348 had a significantly higher GI than the other cultivars at all PEG concentrations, and about double that of the other cultivars at −1.5 MPa (Figure 1B). Therefore, we used this PEG concentration in all other experiments.
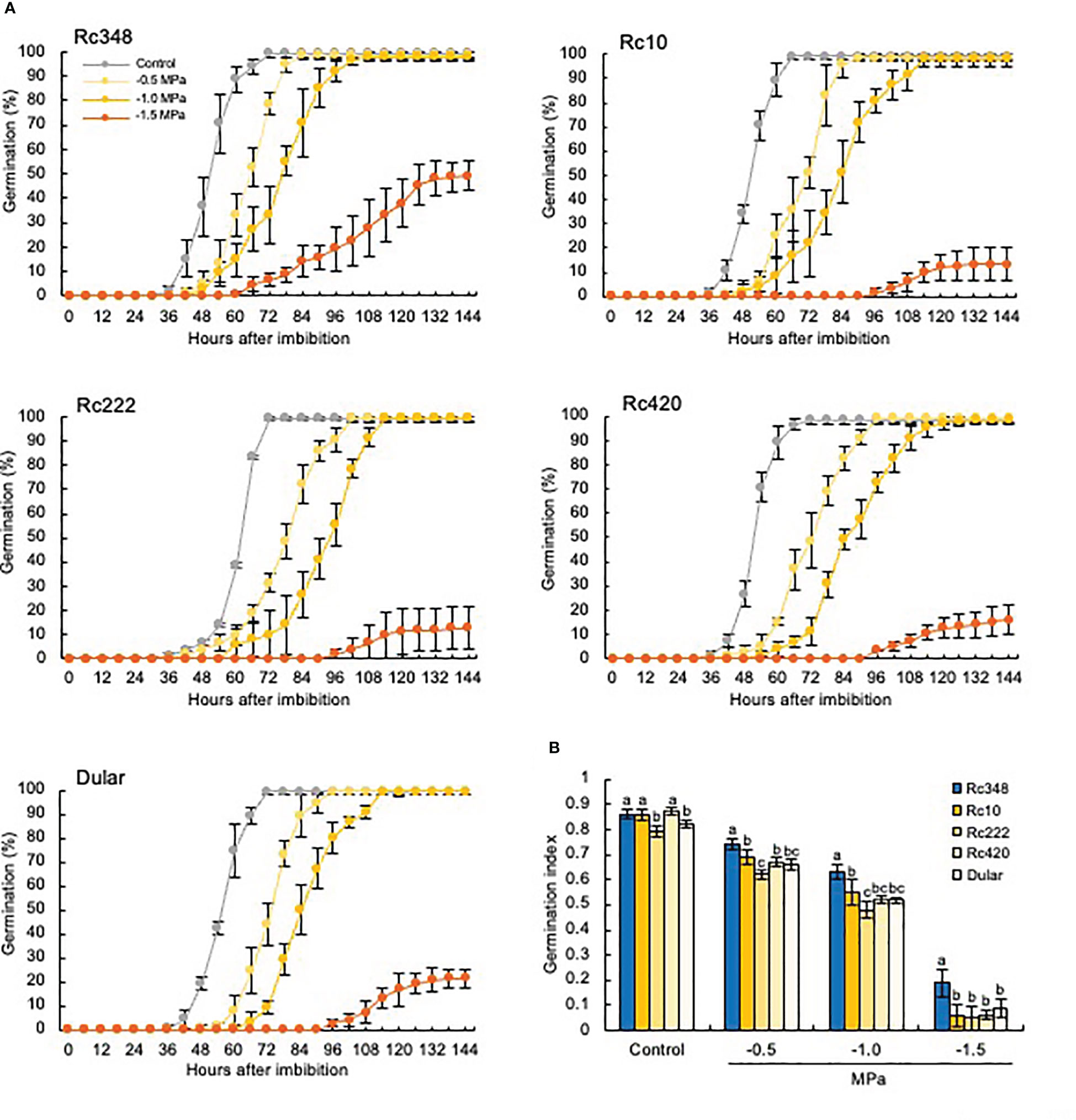
Figure 1 (A) Germination rates and (B) germination index of five indica rice cultivars under osmotic stress imposed by PEG. Control, germination in distilled water. Values with the same letter within a treatment are not significantly different at P < 0.05 by Tukey’s test (n = 5).
3.2 Expression of GA and ABA related genes in imbibed seeds under osmotic stress
As a reference, we chose the widely grown drought-tolerant cultivar Rc348 and drought-sensitive cultivar Rc10 within all examined cultivars (Supplemental Figure 1). We analyzed transcript levels of GA- and ABA-metabolism-related genes and contents of GA and ABA in seeds during imbibition at 24, 48 and 72 HAI. Among genes for GA biosynthesis, despite the significantly lower expression of OsKAO in Rc348 at 48 HAI (1/1.9×), and no significant difference in OsGA3ox2 expression, Rc348 had significantly higher OsGA20ox1 expression at 48 HAI (3.0× that of Rc10), and marginally higher at 24 HAI. Significantly higher OsGA3ox1 expression of Rc348 compared to that of Rc10 at 24 HAI (2.1×) and 48 HAI (2.1×) was also observed (Figure 2). Transcript levels of OsGA3ox2 remained stable and showed no significant difference over time in both cultivars.
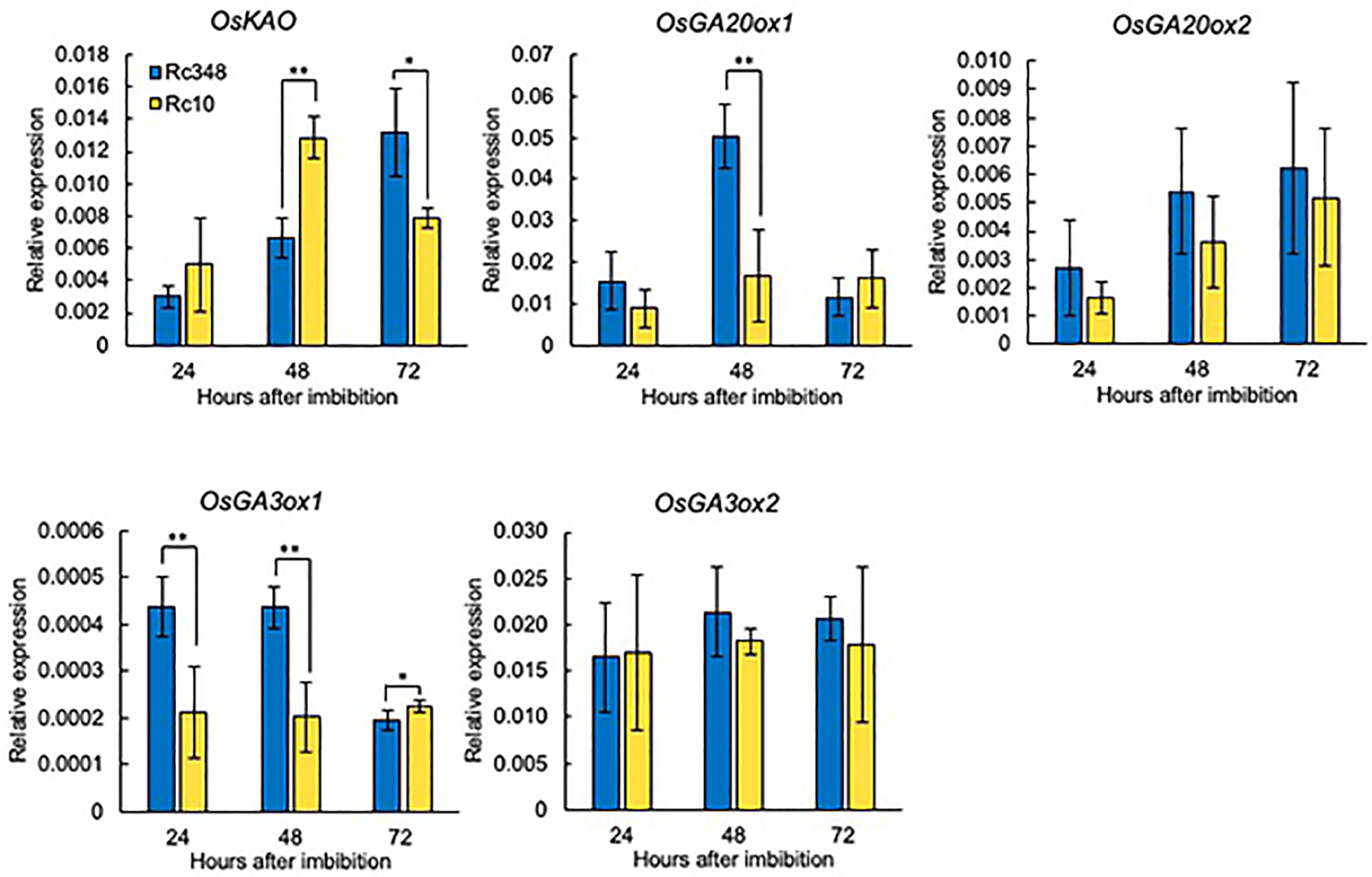
Figure 2 Relative expression of GA biosynthesis genes at 24, 48, and 72 HAI during seed imbibition under osmotic stress imposed by −1.5 MPa PEG. Significant differences: *P < 0.05, **P < 0.01 by Student’s t-test (n = 3).
Among genes for ABA biosynthesis (OsNCEDs), OsNCED1 and OsNCED3 expression gradually increased from 24 to 72 HAI in both Rc10 and Rc348 under osmotic stress. On the other hands, changes of OsNCED5 expression overtime from 24 to 72 HAI were barely observed. Rc10 had significantly higher OsNCED1 and OsNCED5 expression at 24 HAI. Rc348 showed higher expression of OsNCED3 and OsNCED5 at 48 HAI, together with marginally higher expression of OsNCED1 and OsNCED3 at 72 HAI (Figure 3A). Despite no change in OsABA8’OH1 expression, Rc348 had significantly lower OsABA8’OH3 expression at 24 HAI (1/2.4×) and 72 HAI (1/2.2×). Overall, with fluctuations during germination time course of ABA biosynthesis genes, significant downregulation of OsABA8’OH3 for ABA catabolism in Rc348 was observed (Figure 3B).
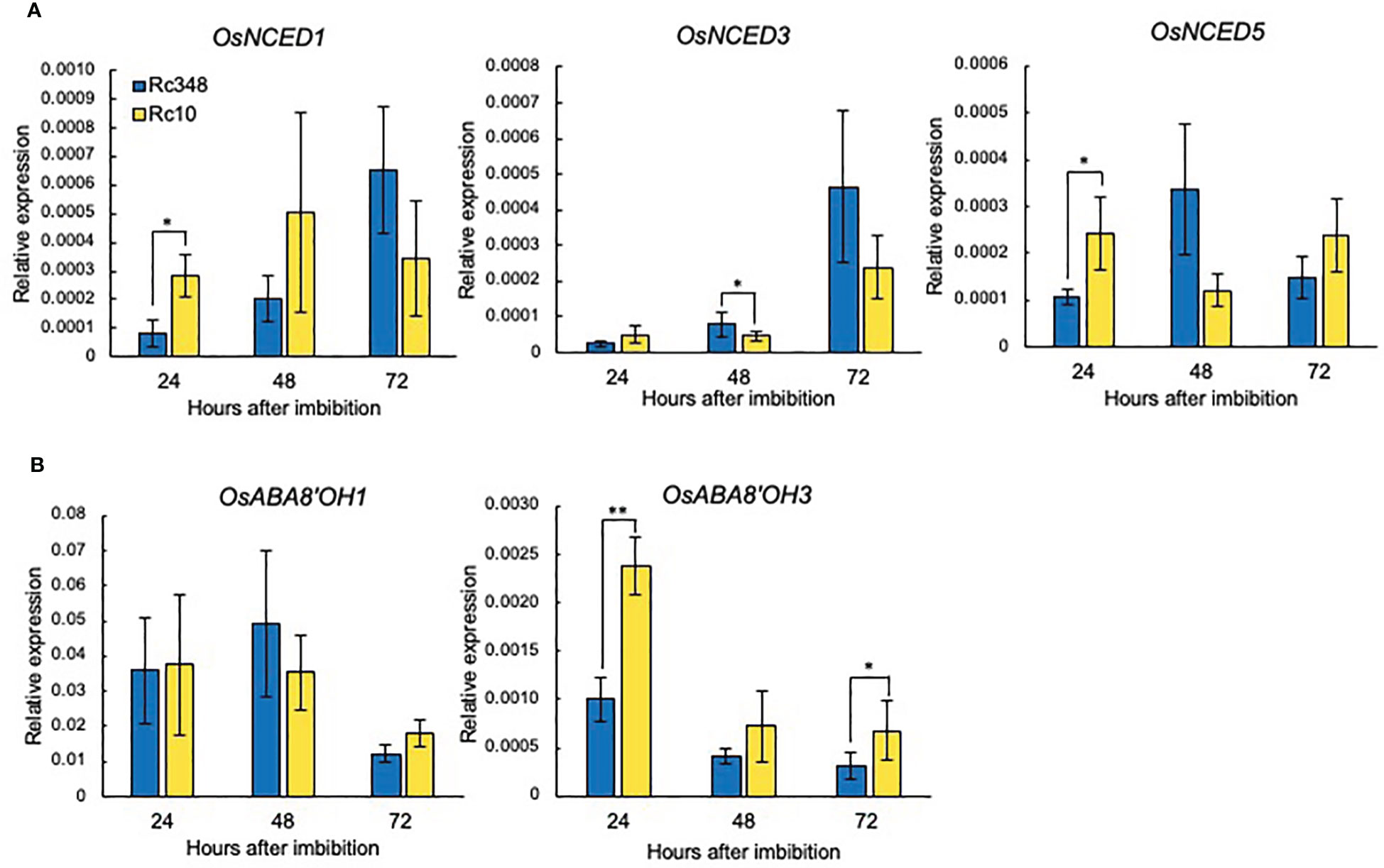
Figure 3 Expression of (A) ABA biosynthesis and (B) ABA catabolism genes at 24, 48, and 72 HAI during imbibition under osmotic stress imposed by −1.5 MPa PEG. Significant differences: *P < 0.05, **P < 0.01 by Student’s t-test (n = 3).
3.3 NADPH oxidase gene expression, ROS and hormone contents in embryos under osmotic stress
Since GA and ABA are known to be regulated by ROS in embryos, we then analyzed the transcript levels of nine NADPH oxidase genes (Respiratory burst oxidase homologs, OsRbohs) in embryos during imbibition in −1.5-MPa PEG at 48 HAI (Figure 4A). Expression of OsRbohA, OsRbohC, OsRbohF, OsRbohG, OsRbohH, and OsRbohI was significantly higher in Rc348 than in Rc10 (Figure 2A); OsRbohH had the highest transcript level in OsRbohs (2.8× that in Rc10). NADPH oxidase activity in embryos of Rc348 was also 2.0× significantly higher than that in Rc10 (Figure 4B), resulting in significantly enhanced endogenous ROS content in Rc348 embryos for 3.1× that in Rc10 (Figure 4C). These results show that osmotic stress enhanced NADPH oxidase gene expression and increased ROS content in Rc348 embryos. We also showed that inhibition of NADPH oxidase by DPI significantly reduced germination rate of Rc348, where exogenous H2O2 significantly improved Rc10 seed germination under −1.5-MPa PEG (Supplemental Figure 2), suggesting the role of ROS on seed germination under osmotic stress. We also analyzed endogenous GA1, GA4 and ABA in imbibed embryos at 72 HAI. Rc348 had significantly higher content of endogenous GA1 (1.9×), GA4 (1.9×), and ABA (2.0×) than Rc10, which is explained by upregulated GA biosynthesis and downregulated ABA catabolism transcript levels during imbibition in Rc348 seeds under osmotic stress (Table 1). Since enhanced endogenous ROS stimulated GA production without decreasing ABA content to promote germination in Rc348, exogenous sodium ascorbate (AsA), an antioxidant to decrease endogenous ROS, was applied to elucidate the role of ROS on GA and ABA production under osmotic stress in Rc348 (Supplemental Figure 3). As a result, exogenous AsA significantly reduced germination rate in dose dependent manner under −1.5 MPa PEG (Supplemental Figure 1A). Despite no obvious change in ABA metabolism gene expression, Rc348 seeds imbibed with 25 mM AsA showed significantly reduced expression of GA biosynthesis, OsGA20ox1 (1/4.3×) and OsGA3ox2 (1/33.8×) compared to that of −1.5 MPa PEG only during imbibition (Supplemental Figure 3B−C). Consequently, endogenous GA1 content was significantly reduced by exogenous AsA, while ABA and GA4 content remained unchanged (Supplemental Figure 3D−F). Thus, these results suggest that enhancement of ROS rather induce GA production than inhibiting ABA to promote seed germination in Rc348 under osmotic stress.
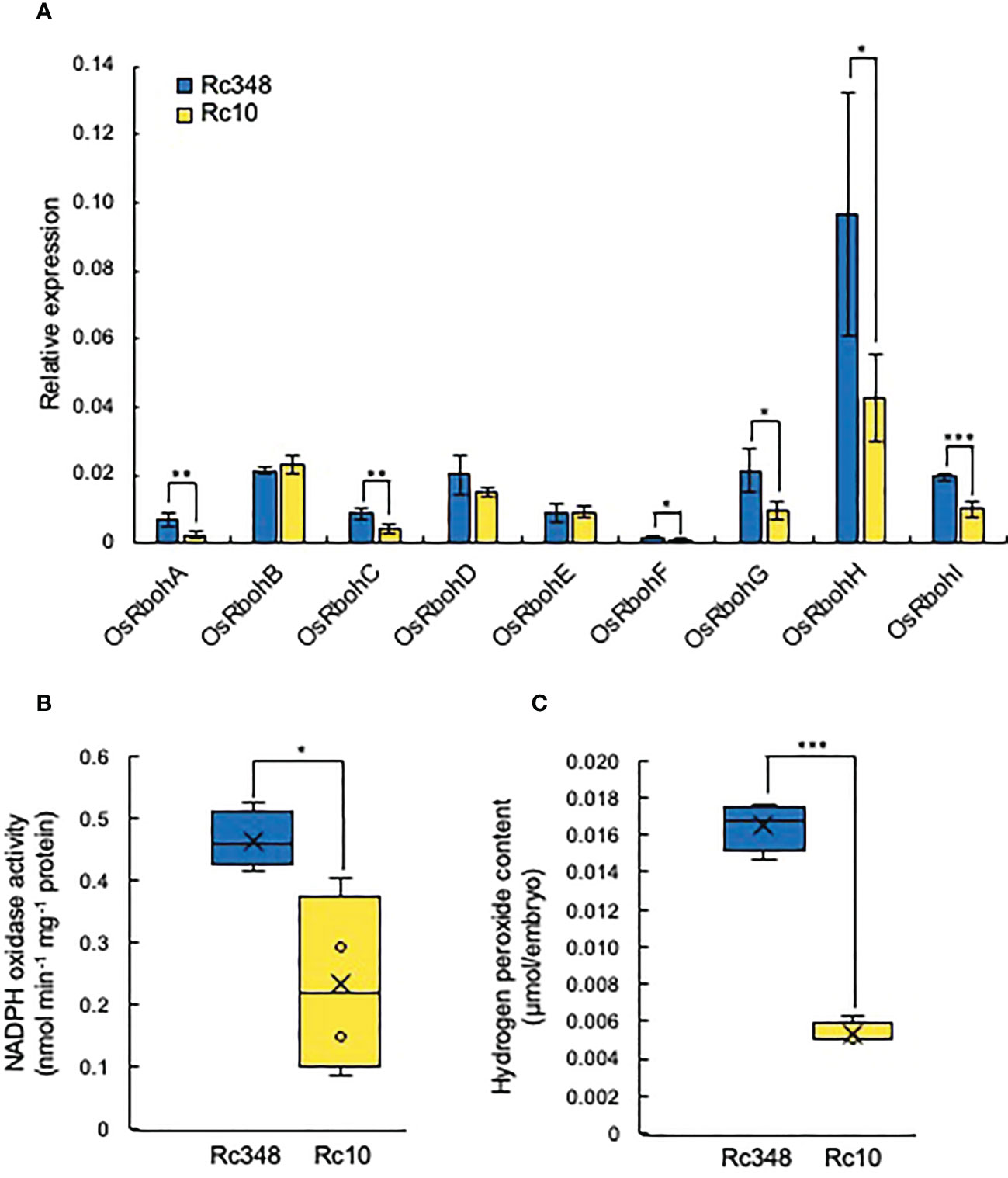
Figure 4 Expression of rice NADPH oxidases, NADPH oxidase enzyme activity, and endogenous hydrogen peroxide content in embryos in −1.5 MPa PEG. (A) Relative expression of OsRbohA to OsRbohI at 48 HAI. (B) Endogenous NADPH oxidase activity and (C) hydrogen peroxide content in embryo at 48 HAI. Significant differences: *P < 0.05, **P < 0.01, ***P < 0.001 by Student’s t-test [n = 3 for A, n = 4 for (B, C)].

Table 1 Endogenous GA1, GA4, and ABA contents in imbibed seeds under osmotic stress imposed by −1.5 MPa PEG.
3.4 α-Amylase gene expression in imbibed seeds under osmotic stress
α-Amylase is induced by GA and suppressed by ABA in cereal aleurone cells (Woodger et al., 2003). During seed imbibition, the expression of α-amylase genes (OsAmy1A, OsAmy1C, OsAmy3B, and OsAmy3E) is induced by GA, and they are highly expressed in rice endosperm after imbibition (Chen, 2006). We analyzed the expression of these genes in imbibed seeds during germination under osmotic stress (Figure 5). Rc348 had significantly higher α-amylase gene expression than Rc10 at 48 HAI (OsAmy3B, 4.8×; OsAmy3E, 2.6×) and 72 HAI (OsAmy1A, 1.6×; OsAmy1C, 3.4×; OsAmy3B, 1.8×; OsAmy3E, 1.5×). These results suggest that α-amylase upregulation during imbibition of Rc348 seeds facilitates germination under osmotic stress.
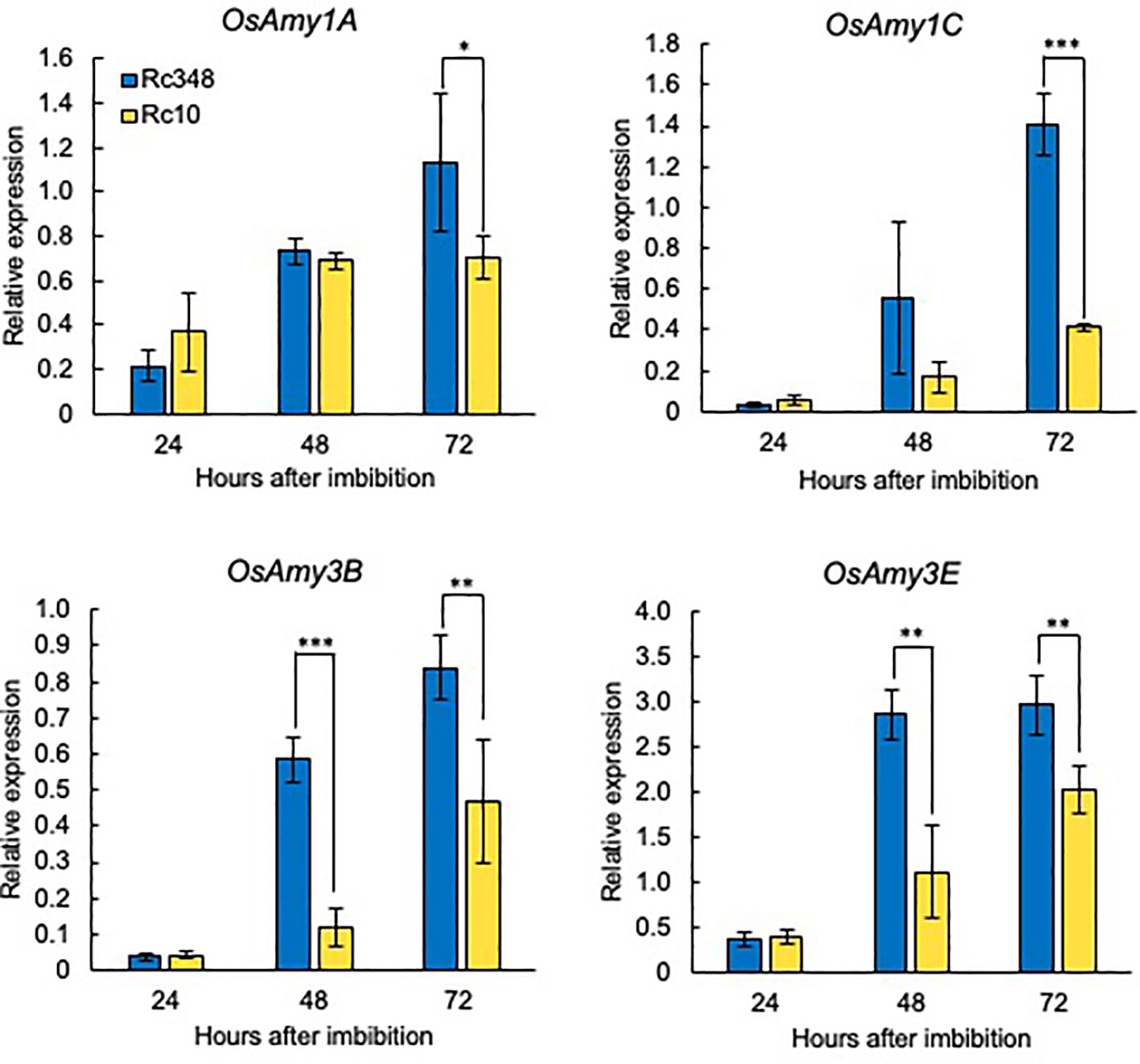
Figure 5 Relative expression of α-amylase genes at 24, 48, and 72 HAI during imbibition under osmotic stress imposed by −1.5 MPa PEG. Significant differences: *P < 0.05, **P < 0.01, ***P < 0.001 by Student’s t-test (n = 3).
3.5 Responses of starch degradation and ROS accumulation in aleurone cells to exogenous GA and ABA
Rc348 imbibed seeds had significantly higher expression of α-amylase genes (Figure 5). In aleurone cells, the expression of GAMYB and its downstream target α-amylase is induced by GA and suppressed by ABA through PKABA induction (Gomez-Cadenas et al., 2001; Woodger et al., 2003; Ishibashi et al., 2012). It has been shown that rice SAPK8 and SAPK10 of SAPK family genes in rice are orthologous to PKABA1 in barley, which expression of both is induced by ABA (Li et al., 2007). We investigated the effects of exogenous GA with/without of ABA on GAMYB, PKABA and α-amylase gene expression in aleurone cells at 24 HAI (Figure 6). Exogenous GA alone significantly increased expression of OsGAMYB (1.3×) relative to level in Rc10 (Figure 6A). Presence of exogenous ABA inhibited the expression of OsGAMYB in both Rc348 and Rc10, however, the expression was significantly increased in Rc348 (1.4×) relative to levels in Rc10. Exogenous ABA induced the expression of OsSAPK8 and OsSAPK10 in aleurone cells. Despite no change between Rc348 and Rc10 in OsSAPK10 expression, Rc348 showed significantly reduced expression of OsSAPK8 (1/1.5×) compared to level in RC10. Exogenous GA alone significantly increased expression of α-amylase genes (OsAmy1A, 4.9×; OsAmy1C, 4.2×; OsAmy3B, 9.5×; OsAmy3E, 6.1×) relative to levels in Rc10 (Figure 6A). α-amylase gene expression was also increased by GA even in the presence of ABA in Rc348 (3.9×, 4.1×, 7.3×, and 4.6×, respectively). Therefore, the suppressive effect of ABA was countered by the inductive effect of GA on signaling and starch degradation in aleurone cells of Rc348. GA induces RboH gene expression to regulate α-amylase activity in barley aleurone cells (Ishibashi et al., 2015). We analyzed the transcript levels of NADPH oxidase genes in aleurone cells treated with exogenous GA and found that OsRbohA, OsRbohD, OsRbohE, OsRbohG, and OsRbohI expression (Figure 6B) and endogenous ROS levels in aleurone cells (Figure 6C) were significantly higher in Rc348 than in Rc10. These results suggest that Rc348 also had higher sensitivity to exogenous GA in terms of ROS induction in aleurone cells.
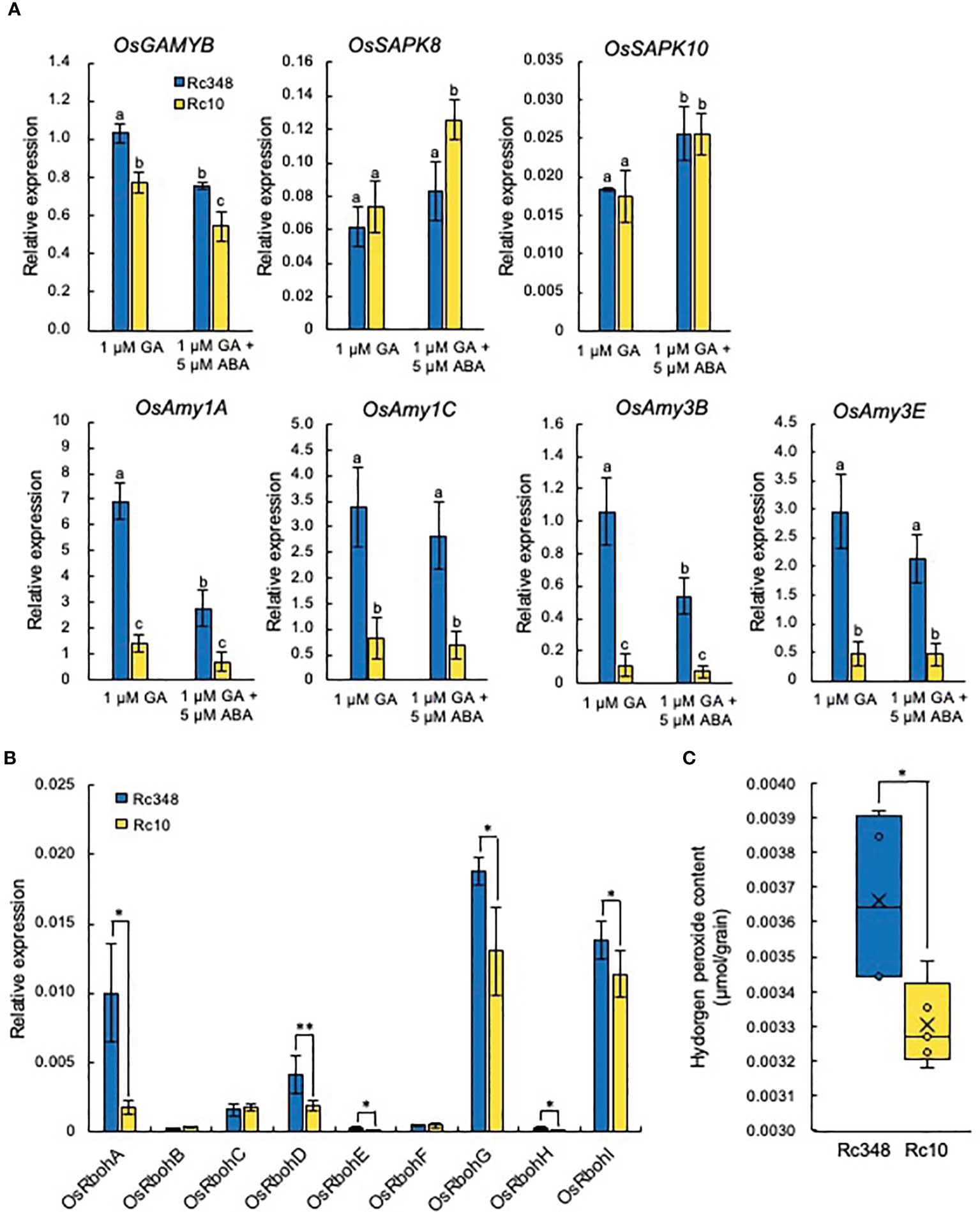
Figure 6 Induction of GA and ABA signaling and NADPH oxidase genes in aleurone layers under osmotic stress. (A) Relative expression of OsGAMYB, OsSAPK8,10, and α-amylase genes at 24 HAI in aleurone layers of embryoless seeds in −1.5 MPa PEG + 1 µM GA or −1.5 MPa PEG + 1 µM GA + 5 µM ABA. (B) Relative expression of NADPH oxidase genes at 24 HAI in −1.5 MPa PEG + 1 µM GA. (C) Endogenous hydrogen peroxide content in aleurone layers of embryoless seeds at 36 HAI in −1.5 MPa PEG + 1 µM GA. In A, values with the same letter are not significantly different at P < 0.05 by Tukey’s test (n = 3). (B, C) Significant differences: *P < 0.05, **P < 0.01 by Student’s t-test (n = 3 for A, B, n = 4 for C).
4 Discussion
We propose that Rc348, a newly developed drought-stress-tolerant cultivar bred for DDS (Yamane et al., 2017; Ohno et al., 2018), gains its capacity for a high germination rate under osmotic stress via the regulation of ROS and phytohormones. Loss of function of NADPH oxidase of osrbohb mutant results in reduced osmotic stress tolerance due to lower levels of ROS and ABA contents in rice seedlings and resulted in impaired seed germination (Shi et al., 2020). In plants, Rboh genes not only function in responses to stress signaling and development (Kaur and Pati, 2016; Suriyasak et al., 2017; Chapman et al., 2019), but also promote germination (Muller et al., 2009; Ishibashi et al., 2010; Ishibashi et al., 2015; Kai et al., 2016; Ishibashi et al., 2017). After imbibition, ROS produced in seeds induce GA and inhibit ABA production to initiate germination (Liu et al., 2010; Ishibashi et al., 2015). We showed that Rc348 had the highest ability to germinate under a severe osmotic stress of -1.5 MPa, when compared to other cultivars tested. ROS induce production of GA (which promotes germination) and inhibit production of ABA (which suppresses germination) (Oracz et al., 2007; Ishibashi et al., 2012; El-Maarouf-Bouteau et al., 2015; Ishibashi et al., 2015). In barley embryos treated with diphenylene iodonium chloride (DPI), an NADPH oxidase inhibitor, endogenous GA was significantly reduced while ABA was enhanced resulting in inhibited germination (Ishibashi et al., 2015). In our results, we observed increased ROS content together with higher endogenous GA1, GA4 and ABA contents in Rc348, which were due to up-regulated OsGA20ox1, OsGA3ox1 and OsNCED3, and down-regulated OsABA8’OH3. For ABA biosynthesis, previous studies have reported that OsNCED1 plays a role in salinity stress response (Zhang et al., 2022) and heat stress tolerance (Zhou et al., 2022), where OsNCED3 expression is highly induced by PEG and other osmotic stresses, contributing to ABA accumulation for stress responses (Huang et al., 2018). Here, we observed overall increase in OsNCED1 and OsNCED3 expression overtime upon germination under osmotic stress in both cultivars. This suggests the possibility of ABA accumulation due to osmotic stress response in both cultivars. For OsNCED5, we could not observe obvious difference between cultivars from 24 to 72 HAI, which might be due to that its expression drops rapidly after imbibition and stays at the same basal level from 18 HAI onward (Suriyasak et al., 2020). Additionally, OsABA8’OH3 expression in Rc348 was lower than that in Rc10 under osmotic stress. High endogenous ABA level in RC348 under osmotic stress might be attributed to the OsABA8’OH3 expression. For GA biosynthesis, we did not observe change in OsGA3ox2 expression over time, which might be due to its rapid peak at the very early stage of imbibition as reported in previous study (Kaneko et al., 2002), whereas OsGA20ox1 expression peaks at the later phase of germination (Liu et al., 2014). A previous study has shown that expression of GA biosynthetic genes, including OsGA20ox1 and OsGA3ox1, was suppressed by ABA in rice (Ye et al., 2012). In our study, enhancement of endogenous ABA did not inhibit expression of OsGA20ox1 and OsGA3ox1 in Rc348 under osmotic stress. Our results showed that enhanced ROS rather promote GA than act to suppress ABA in Rc348. To explain this phenomenon, we observed that exogenous sodium ascorbate (AsA), an antioxidant, significantly inhibited seed germination of Rc348 via suppressing GA biosynthesis genes, OsGA20ox1 and OsGA3ox2, rather than affecting ABA metabolism genes (Supplemental Figure 3A−C). Consequently, endogenous GA1 level in seeds was not detected by AsA treatment, without affecting endogenous ABA and GA4 contents (Supplemental Figure 3D−F), which confirmed our results that enhancement of ROS mainly induces GA production to promote germination under osmotic stress of Rc348. Additionally, drought-tolerant maize and Medicago sativa L. seedlings accumulated more endogenous ABA in leaves under osmotic stress induced by PEG than drought-intolerant seedlings (Yao et al., 2019; Liu et al., 2022). Since ABA is known to accumulate under osmotic stress and enhance stress responses (Kuromori et al., 2018), in this study also, enhancement of ABA under osmotic stress in Rc348 might be involved in osmotic stress tolerance, with better seedling establishment under drought, as described in our previous study (Yamane et al., 2017).
In cereal aleurone cells, GAMYB is a transcription factor that is upregulated by GA and downregulated by ABA (Gomez-Cadenas et al., 2001; Washio, 2003; Woodger et al., 2003; Ishibashi et al., 2012), and binds to GARE boxes in α-amylase promoters to induce starch degradation (Kaneko et al., 2002). Osmotic stress reduces α-amylase activity and thus impairs germination (Muscolo et al., 2013). Here, we showed that Rc348 gains its osmotic tolerance via upregulation of α-amylase gene expression in aleurone cells due to higher endogenous bioactive GA levels in embryos under osmotic stress. In aleurone cells of Rc348, expression of both GAMYB and α-amylase genes was highly induced by exogenous GA, suggesting its higher responses to GA than Rc10’s. In barley aleurone cells, PKABA induced by ABA inhibits GAMYB and α-amylase expression (Ishibashi et al., 2012). Here we showed that ABA induction of SAPK8 was significantly lower in Rc348 aleurone cells. Consequently, GAMYB and α-amylase induction in aleurone cells was still significantly higher in Rc348 than in Rc10 even in the presence of exogenous ABA, suggesting the importance of enhanced GA signaling in Rc348 for starch degradation to fuel germination. We previously showed that GA stimulates NADPH oxidase gene expression for ROS production in aleurone layer of barley seed, which inhibits PKABA to promote α-amylase expression (Ishibashi et al., 2012; Ishibashi et al., 2015). Rc348 also had higher sensitivity than Rc10 to exogenous GA in terms of higher Rboh expression and endogenous ROS content in aleurone cells, which consequently led to α-amylase induction in aleurone cells via up-regulation of GAMYB and down-regulation of PKABA. Despite an increase in endogenous ROS contents in both embryos and aleurone cells, expression patterns of OsRboh genes differed between the two: OsRbohH was expressed mainly in embryos, while OsRbohA, OsRbohG, and OsRbohI were highly expressed in GA-treated aleurone. In summary, the osmotic stress tolerance in seed germination of Rc348 is caused by enhancement of ROS production, GA biosynthesis, and GA sensitivity (Figure 7).
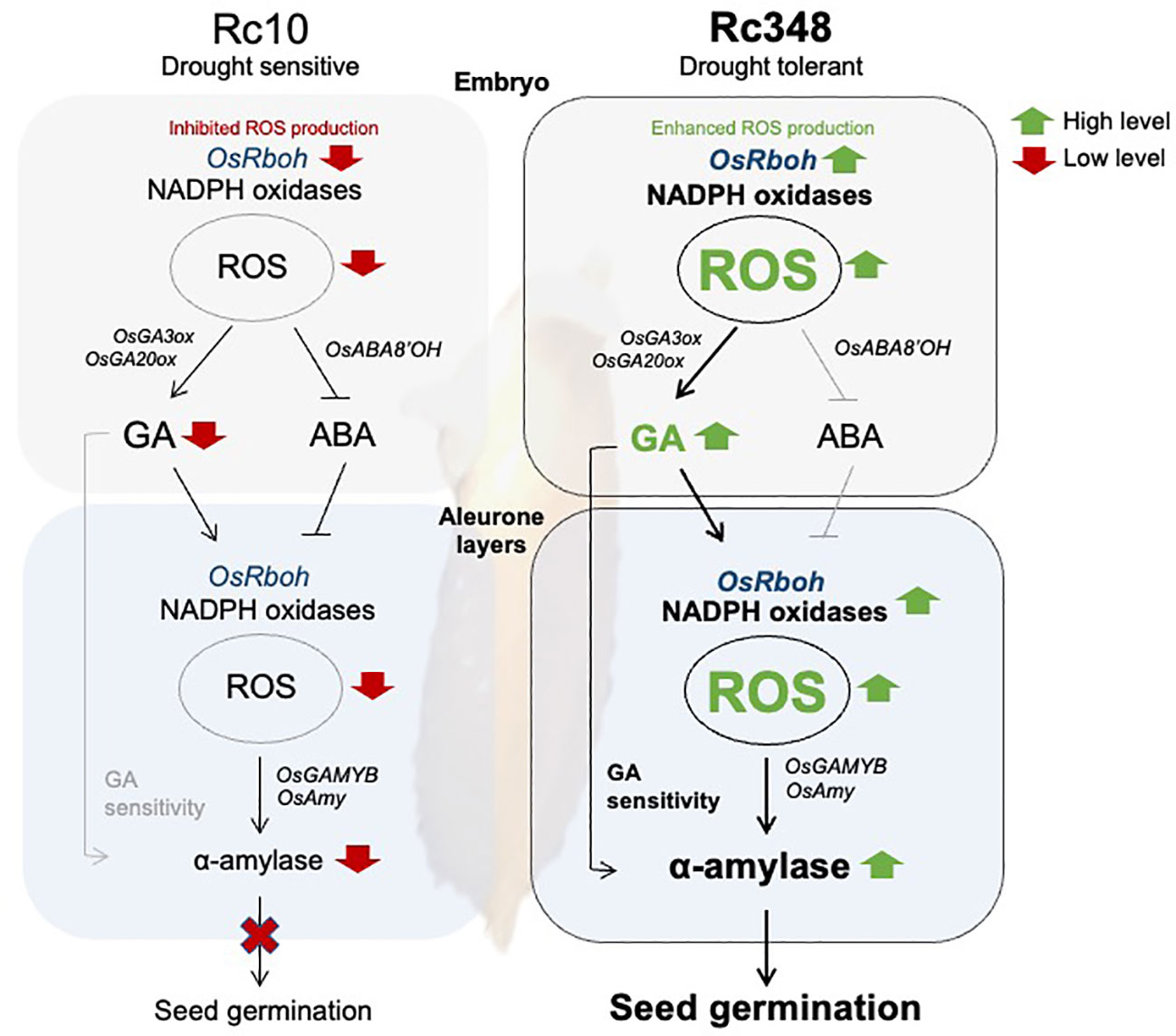
Figure 7 Scheme of regulations of reactive oxygen species in osmotic stress tolerance during seed germination suggested in this study. Pathways in black lines are shown to be more effective than that in grey lines in this study.
Data availability statement
The original contributions presented in the study are included in the article/Supplementary Material. Further inquiries can be directed to the corresponding author.
Author contributions
YK, KY, KS, CS, and YI designed the experiments; RK, CS, RM, YSaw, YSak, NH, and YI performed the experiments; RK, CS, and YI performed data analysis; CS, CB, and YI wrote the manuscript. All authors contributed to the article and approved the submitted version.
Funding
This work was supported by JSPS KAKENHI (Grant Numbers: 18H02186 and 21KK0108).
Conflict of interest
The authors declare that the research was conducted in the absence of any commercial or financial relationships that could be construed as a potential conflict of interest.
Publisher’s note
All claims expressed in this article are solely those of the authors and do not necessarily represent those of their affiliated organizations, or those of the publisher, the editors and the reviewers. Any product that may be evaluated in this article, or claim that may be made by its manufacturer, is not guaranteed or endorsed by the publisher.
Supplementary material
The Supplementary Material for this article can be found online at: https://www.frontiersin.org/articles/10.3389/fpls.2023.1186960/full#supplementary-material
References
Bailly, C., El-Maarouf-Bouteau, H., Corbineau, F. (2008). From intracellular signaling networks to cell death: the dual role of reactive oxygen species in seed physiology. C. R. Biol. 331, 806–814. doi: 10.1016/j.crvi.2008.07.022
Bialecka, B., Kepczynski, J. (2010). Germination, α-, β-amylase and total dehydrogenase activities of Amaranthus caudatus seeds under water stress in the presence of ethephon or gibberellin A3. Acta Biol. Crac. Ser. Bot. 52, 7–12. doi: 10.2478/v10182-010-0001-0
Chapman, J. M., Muhlemann, J. K., Gayomba, S. R., Muday, G. K. (2019). RBOH-dependent ROS synthesis and ROS scavenging by plant specialized metabolites to modulate plant development and stress responses. Chem. Res. Toxicol. 32, 370–396. doi: 10.1021/acs.chemrestox.9b00028
Chen, P. W. (2006). Interaction between rice MYBGA and the gibberellin response element controls tissue-specific sugar sensitivity of α-amylase genes. Plant Cell 18, 2326–2340. doi: 10.1105/tpc.105.038844
Chirgwin, J. M., Przybyla, A. E., MacDonald, R. J., Rutter, W. J. (1979). Isolation of biologically active ribonucleic acid from sources enriched in ribonuclease. Biochemistry 18, 5294–5299. doi: 10.1021/bi00591a005
Coolbear, P., Grierson, D. (1984). The effect of low temperature pre-sowing treatment under the germination performance and membrane integrity of artificially aged tomato seeds. J. Exp. Bot. 35, 1609–1617. doi: 10.1093/jxb/35.11.1609
El-Maarouf-Bouteau, H., Sajjad, Y., Bazin, J., Langlade, N., Cristescu, S. M., Balzergue, S., et al. (2015). Reactive oxygen species, abscisic acid and ethylene interact to regulate sunflower seed germination. Plant Cell Env. 38, 364–374. doi: 10.1111/pce.12371
Gadal, N., Shrestha, J., Poudel, M. N., Pokharel, B. (2019). A review on production status of growing environments of rice in Nepal and in the world. AAES 4, 83–87. doi: 10.26832/24566632.2019.0401013
Gomez-Cadenas, A., Zentella, R., Walker-Simmons, M. K., Ho, T. H. D. (2001). Gibberellin/abscisic acid antagonism in barley aleurone cells: site of action of the protein kinase PKABA1 in relation to gibberellin signaling molecules. Plant Cell 13, 667–669. doi: 10.1105/tpc.13.3.667
Gubler, F., Racentos, D., Keys, M., Watts, R., Mundy, J., Jacobsen, J. V. (1999). Target genes and regulatory domain of the GAMYB transcriptional activator in cereal aleurone. Plant J. 17, 1–9. doi: 10.1046/j.1365-313X.1999.00346.x
Haefele, S. M., Kato, Y., Singh, S. (2016). Climate ready rice: augmenting drought tolerance with best management practices. Field Crops Res. 190, 60–69. doi: 10.1016/j.fcr.2016.02.001
Hayashi, S., Kamoshita, A., Yamagishi, J., Kotchasatit, A., Jongdee, B. (2007). Genotypic differences in grain yield of transplanted and direct-seeded rainfed lowland rice (Oryza sativa l.) in northeastern Thailand. Field Crops Res. 102, 9–21. doi: 10.1016/j.fcr.2007.01.001
Hedden, P., Phillips, A. L. (2000). Gibberellin metabolism: new insights revealed by the genes. Trends Plant Sci. 5, 523–530. doi: 10.1016/S1360-1385(00)01790-8
Hossain, M., Bhattacharjee, S., Armin, S–M., Qian, P., Xin, W., Li, H–Y., et al. (2015). Hydrogen peroxide priming modulates abiotic oxidative stress tolerance: insights from ROS detoxification and scavenging. Front. Plant Sci. 6. doi: 10.3389/fpls.2015.00420
Huang, Y., Guo, Y., Liu, Y., Zhang, F., Wang, Z., Wang, H., et al. (2018). 9-cis-Epoxycarotenoid dioxygenase 3 regulates plant growth and enhances multi-abiotic stress tolerance in rice. Front. Plant Sci. 9. doi: 10.3389/fpls.2018.00162
Ishibashi, Y., Aoki, N., Kasa, S., Sakamoto, M., Kai, K., Tomokiyo, R., et al. (2017). The interrelationship between abscisic acid and reactive oxygen species plays a key role in barley seed dormancy and germination. Front. Plant Sci. 8, 275. doi: 10.3389/fpls.2017.00275
Ishibashi, Y., Kasa, S., Sakamoto, M., Aoki, N., Kai, K., Yuasa, T., et al. (2015). A role for reactive oxygen species produced by NADPH oxidases in the embryo and aleurone cells in barley seed germination. PloS One 10, e0143173. doi: 10.1371/journal.pone.0143173
Ishibashi, Y., Tawaratsumida, T., Kondo, K., Kasa, S., Sakamoto, M., Aoki, N., et al. (2012). Reactive oxygen species are involved in gibberellin/abscisic acid signaling in barley aleurone cells. Plant Physiol. 158, 1705–1714. doi: 10.1104/pp.111.192740
Ishibashi, Y., Tawaratsumida, T., Zheng, S. H., Yuasa, T., Iwaya-Inoue, M. (2010). NADPH oxidases act as key enzyme on germination and seedling growth in barley (Hordeum vulgare l.). Plant Prod. Sci. 13, 45–52. doi: 10.1626/pps.13.45
Jacobsen, J. V., Pearce, D. W., Poole, A. T., Pharis, R. P., Mander, L. N. (2002). Abscisic acid, phaseic acid and gibberellin contents associated with dormancy and germination in barley. Physiol. Plant 115, 428–441. doi: 10.1034/j.1399-3054.2002.1150313.x
Jira-anunkul, W., Pattanakul, W. (2021). Effects of hydrogen peroxide application on agronomic traits of rice (Oryza sativa l.) under drought stress. Plant Soil Environ. 67, 221–229. doi: 10.17221/628/2020-PSE
Kai, K., Kasa, S., Sakamoto, M., Aoki, N., Watabe, G., Yuasa, T., et al. (2016). Role of reactive oxygen species produced by NADPH oxidase in gibberellin biosynthesis during barley seed germination. Plant Signal. Behav. 11, e1180492. doi: 10.1080/15592324.2016.1180492
Kaneko, M., Itoh, H., Ueguchi-Tanaka, M., Ashikari, M., Matsuoka, M. (2002). The alpha-amylase induction in endosperm during rice seed germination is caused by gibberellin synthesized in epithelium. Plant Physiol. 128, 1264–1270. doi: 10.1104/pp.010785
Kato, Y., Katsura, K. (2014). Rice adaptation to aerobic soils: physiological considerations and implications for agronomy. Plant Prod. Sci. 17, 1–12. doi: 10.1626/pps.17.1
Kaur, G., Pati, P. K. (2016). Analysis of cis-acting regulatory of respiratory burst oxidase homolog (Rboh) gene families in arabidopsis and rice provides clues for their diverse functions. Comput. Biol. Chem. 62, 104–118. doi: 10.1016/j.compbiolchem.2016.04.002
Kumar, V., Ladha, J. K. (2011). Chapter six – direct seeding of rice: recent developments and future research needs. Adv. Agron. 111, 297–413. doi: 10.1016/B978-0-12-387689-8.00001-1
Kuromori, T., Seo, M., Shinosaki, K. (2018). ABA transport and plant water stress responses. Trends Plant Sci. 23, 513–522. doi: 10.1016/j.tplants.2018.04.001
Li, G., Lin, F., Xue, H. W. (2007). Genome-wide analysis of the phospholipase d family in Oryza sativa and functional characterization of PLDβ1 in seed germination. Cell Res. 17, 881–894. doi: 10.1038/cr.2007.77
Liu, Y., Fang, J., Xu, F., Chu, J., Yan, C., Schlappi, M. R., et al. (2014). Expression patterns of ABA and GA metabolism genes and hormone levels during rice seed development and imbibition: a comparison of dormant and non-dormant rice cultivars. J. Genet. Genomics 41, 327–338. doi: 10.1016/j.jgg.2014.04.004
Liu, Y., Jiang, D., Yan, J., Wang, K., Lin, S., Zhang, W. (2022). ABA-insensitivity of alfalfa (Medicago sativa l.) during seed germination associated with plant drought tolerance. Env. Exp. Bot. 203, 105069. doi: 10.1016/j.envexpbot.2022.105069
Liu, Y., Ye, N., Liu, R., Chen, M., Zhang, J. (2010). H2O2 mediates the regulation of ABA catabolism and GA biosynthesis in Arabidopsis seed dormancy and germination. J. Exp. Bot. 61, 2979–2990. doi: 10.1093/jxb/erq125
Matloob, A., Khaliq, A., Chauhan, B. S. (2015). Weeds of rice in Asia: problems and opportunities. Adv. Agron. 130, 291–336. doi: 10.1016/bs.agron.2014.10.003
Millar, A. A., Jacobsen, J. V., Ross, J. J., Helliwell, C. A., Poole, A. T., Scofield, G., et al. (2006). Seed dormancy and ABA metabolism in Arabidopsis and barley: the role of ABA 8′-hydroxylase. Plant J. 45, 942–954. doi: 10.1111/j.1365-313X.2006.02659.x
Muller, K., Carstens, A. C., Linkies, A., Torres, M. A., Leubner-Metzger, G. (2009). The NADPH-oxidase AtrbohB plays a role in arabidopsis seed after-ripening. New Phytol. 184, 885–897. doi: 10.1111/j.1469-8137.2009.03005.x
Muscolo, A., Sidari, M., Anastasi, U., Santonoceto, C., Maggio, A. (2013). Effect of PEG-induced drought stress on seed germination of four lentil genotypes. J. Plant Interact. 9, 354–363. doi: 10.1080/17429145.2013.835880
Ohno, H., Banayo, N. P. M. C., Bueno, C., Kashiwagi, J., Nakashima, T., Iwama, K., et al. (2018). On-farm assessment of new early-maturing drought-tolerant rice cultivar for dry direct seeding in rainfed lowlands. Field Crops. Res. 219, 222–228. doi: 10.1016/j.fcr.2018.02.005
O’Kane, D., Gill, V., Burdon, R. (1996). Chilling, oxidative stress and antioxidant responses in Arabidopsis thaliana callus. Planta 198, 371–377. doi: 10.1007/BF00620053
Oracz, K., El-Maarouf-Bouteau, H., Farrant, J. M., Cooper, K., Belghazi, M., Claudette, J. (2007). ROS production and protein oxidation as a novel mechanism for seed dormancy alleviation. Plant J. 50, 452–465. doi: 10.1111/j.1365-313X.2007.03063.x
Pantuwan, G., Fukai, S., Cooper, M., Rajarasereekul, S., O’Toole, J. C. (2002). Yield response of rice (Oryza sativa l.) genotypes to different types of drought under rainfed lowlands part 1. grain yield and yield components. Field Crops Res. 73, 153–168. doi: 10.1016/S0378-4290(01)00187-3
Sarath, G., Hou, G., Baird, L. M., Mitchell, R. B. (2007). Reactive oxygen species, ABA and nitric oxide interactions on the germination of warm-season C4-grasses. Planta 226, 697–708. doi: 10.1007/s00425-007-0517-z
Shekhawat, K., Rathore, S. S., Chauhan, B. S. (2020). Weed management in dry direct-seeded rice: a review on challenges and opportunities for sustainable rice production. Agronomy 10, 1264. doi: 10.3390/agronomy10091264
Shi, Y., Chang, Y.-L., Wu, H.-T., Shalmani, A., Liu, W.-T., Li, W.-Q., et al. (2020). OsRbohB-mediated ROS production plays a crucial role in drought stress tolerance of rice. Plant Cell Rep. 39, 1767–1784. doi: 10.1007/s00299-020-02603-2
Subedi, S. R., Sandhu, N., Singh, V. K., Sinha, P., Kumar, S., Singh, S. P., et al. (2019). Genome-wide association study reveals significant genomic regions for improving yield, adaptability of rice under dry direct seeded cultivation condition. BMC Genomics 20, 471. doi: 10.1186/s12864-019-5840-9
Suriyasak, C., Harano, K., Tanamachi, K., Matsuo, K., Tamada, A., Iwaya-Inoue, M., et al. (2017). Reactive oxygen species induced by heat stress during grain filling of rice (Oryza sativa l.) are involved in occurrence of grain chalkiness. J. Plant Physiol. 216, 52–57. doi: 10.1016/j.jplph.2017.05.015
Suriyasak, C., Oyama, Y., Ishida, T., Mashiguchi, K., Yamaguchi, S., Hamaoka, N., et al. (2020). Mechanism of delayed seed germination caused by high temperature stress during grain filling in rice (Oryza sativa l.). Sci. Rep. 10, 17378. doi: 10.1038/s41598-020-74281-9
Van Gestelen, P., Asard, H., Caubergs, R. J. (1997). Solubilization and separation of a plant plasma membrane NADPH-O2- synthase from other NAD(P)H oxidoreductases. Plant Physiol. 115, 543–550. doi: 10.1104/pp.115.2.543
Vries, M. E. D., Rodenburg, J., Bado, B. V., Sow, A., Leffelaar, A., Giller, K. E. (2010). Rice production with less irrigation water is possible in a sahelian environment. Field Crops Res. 116, 154–164. doi: 10.1016/j.fcr.2009.12.006
Washio, K. (2003). Functional dissections between GAMYB and dof transcription factors suggest a role for protein-protein associations in the gibberellin-mediated expression of the RAmy1A gene in the rice aleurone. Plant Physiol. 133, 850–863. doi: 10.1104/pp.103.027334
Woodger, F. J., Millar, A., Murray, F., Jacobsen, J. V., Gubler, F. (2003). The role of GAMYB transcription factors in GA-regulated gene expression. J. Plant Growth Regul. 22, 176–184. doi: 10.1007/s00344-003-0025-8
Wu, J., Zhang, N., Liu, Z., Liu, S., Liu, C., Lin, J., et al. (2020). The AtGSTU7 gene influences glutathione-dependent seed germination under ABA and osmotic stress in arabidopsis. BBRC 528, 538–544. doi: 10.1016/j.bbrc.2020.05.153
Xin, P., Guo, Q., Li, B., Cheng, S., Yan, J., Chu, J. (2020). A tailored high-efficiency sample pretreatment method for simultaneous quantification of 10 classes of known endogenous phytohormones. Plant Commun. 1, 100047. doi: 10.1016/j.xplc.2020.100047
Yamane, K., Gracia, R., Imayoshi, K., Mabesa-Telosa, R. C., Banayo, N. P. M. C., Vergara, G., et al. (2017). Seed vigor contributes to yield improvement in dry direct-seeded rainfed lowland rice. Ann. Appl. Biol. 172, 100–110. doi: 10.1111/aab.12405
Yao, C., Zhang, F., Sun, X., Shang, D., He, F., Li, X., et al. (2019). Effects of s-abscisic acid (S-ABA) on seed germination, seedling growth, and Asi1 gene expression under drought stress in maize. J. Plant Growth. Reg. 38, 1300–1313. doi: 10.1007/s00344-019-09934-9
Ye, N., Zhu, G., Liu, Y., Zhang, A., Li, Y., Liu, R., et al. (2012). Ascorbic acid and reactive oxygen species are involved in the inhibition of seed germination by abscisic acid in rice seeds. J. Exp. Bot. 63, 1809–1822. doi: 10.1093/jxb/err336
Zhang, Q., Liu, Y., Jiang, Y., Li, A., Cheng, B., Wu, J. (2022). OsASR6 enhances salt stress tolerance in rice. Int. Mol. Sci. 23, 9340. doi: 10.3390/ijms23169340
Keywords: germination, reactive oxygen species, gibberellic acid, abscisic acid, osmotic stress, rice
Citation: Kawaguchi R, Suriyasak C, Matsumoto R, Sawada Y, Sakai Y, Hamaoka N, Sasaki K, Yamane K, Kato Y, Bailly C and Ishibashi Y (2023) Regulation of reactive oxygen species and phytohormones in osmotic stress tolerance during seed germination in indica rice. Front. Plant Sci. 14:1186960. doi: 10.3389/fpls.2023.1186960
Received: 15 March 2023; Accepted: 25 May 2023;
Published: 13 June 2023.
Edited by:
Ben Zhang, Shanxi University, ChinaReviewed by:
Nazim Hussain, Independent Researcher, Sharjah, United Arab EmiratesBaris Uzilday, Ege University, Türkiye
Copyright © 2023 Kawaguchi, Suriyasak, Matsumoto, Sawada, Sakai, Hamaoka, Sasaki, Yamane, Kato, Bailly and Ishibashi. This is an open-access article distributed under the terms of the Creative Commons Attribution License (CC BY). The use, distribution or reproduction in other forums is permitted, provided the original author(s) and the copyright owner(s) are credited and that the original publication in this journal is cited, in accordance with accepted academic practice. No use, distribution or reproduction is permitted which does not comply with these terms.
*Correspondence: Yushi Ishibashi, eXVzaGlAYWdyLmt5dXNodS11LmFjLmpw
†These authors have contributed equally to this work