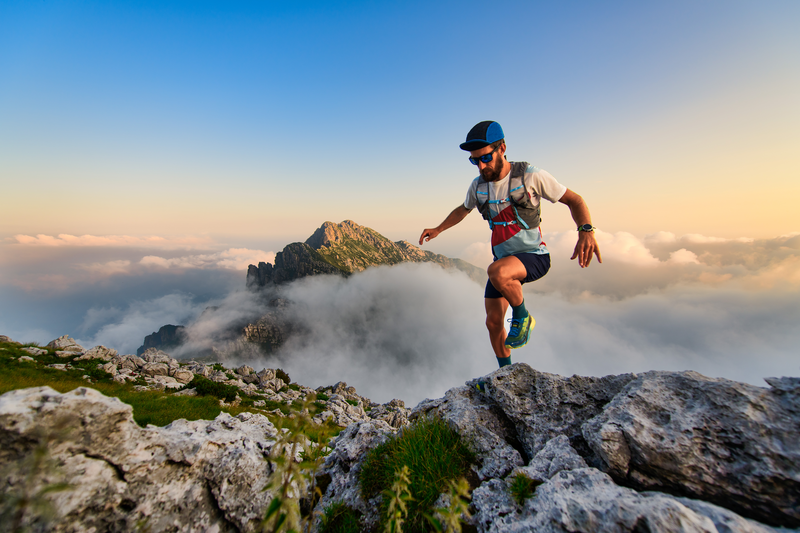
95% of researchers rate our articles as excellent or good
Learn more about the work of our research integrity team to safeguard the quality of each article we publish.
Find out more
ORIGINAL RESEARCH article
Front. Plant Sci. , 14 August 2023
Sec. Plant Symbiotic Interactions
Volume 14 - 2023 | https://doi.org/10.3389/fpls.2023.1184489
This article is part of the Research Topic Plant Growth Promoting Bacteria, Application in Biofertilizers and Biocontrol of Pathogens View all 9 articles
Endophytic bacterial microbiomes of plants contribute to the physiological health of the host and its adaptive evolution and stress tolerance. Wild rice possesses enriched endophytic bacteria diversity, which is a potential resource for sustainable agriculture. Oryza officinalis is a unique perennial wild rice species in China with rich genetic resources. However, endophytic bacterial communities of this species and their plant growth-promoting (PGP) traits remain largely unknown. In this study, endophytic bacteria in the root, stem, and leaf tissues of O. officinalis were characterized using 16S rRNA gene Illumina sequencing. Culturable bacterial endophytes were also isolated from O. officinalis tissues and characterized for their PGP traits. The microbiome analysis showed a more complex structure and powerful function of the endophytic bacterial community in roots compared with those in other tissue compartments. Each compartment had its specific endophytic bacterial biomarkers, including Desulfomonile and Ruminiclostridium for roots; Lactobacillus, Acinetobacter, Cutibacterium and Dechloromonas for stems; and Stenotrophomonas, Chryseobacterium, Achromobacter and Methylobacterium for leaves. A total of 96 endophytic bacterial strains with PGP traits of phosphate solubilization, potassium release, nitrogen fixation, 1-aminocyclopropane-1-carboxylate (ACC) deaminase secretion, and siderophore or indole-3-acetic acid (IAA) production were isolated from O. officinalis. Among them, 11 strains identified as Enterobacter mori, E. ludwigii, E. cloacae, Bacillus amyloliquefaciens, B. siamensis, Pseudomonas rhodesiae and Kosakonia oryzae were selected for inoculation of perennial rice based on their IAA production traits. These strains showed promising PGP effects on perennial rice seedlings. They promoted plants to form a strong root system, stimulate biomass accumulation, and increase chlorophyll content and nitrogen uptake, which could fulfil the ecologically sustainable cultivation model of perennial rice. These results provide insights into the bacterial endosphere of O. officinalis and its application potential in perennial rice. There is the prospect of mining beneficial endophytic bacteria from wild rice species, which could rewild the microbiome of cultivated rice varieties and promote their growth.
Oryza officinalis, with a diploid CC genome, is one of the native wild rice resources in South China. It has a suite of desirable agronomic traits similar to those of C4 plants, e.g., strong growth potential, high photosynthetic efficiency and large biomass accumulation (Kiran et al., 2013). This species exhibits both high tolerance to abiotic stresses (Hirabayashi et al., 2015; Liu et al., 2015; Kitazumi et al., 2018) and strong resistance to biotic stresses (Velusamy et al., 1990; Devanna et al., 2014; Zhang et al., 2014; Chen et al., 2022). Genetic resources of agronomic traits and resistance in O. officinalis have been utilized successfully for cultivated rice variety breeding and improvement.
In addition to their hereditary potential, wild plants utilize the plant-associated microbiome to expand their environmental adaptability, nutrient utilization efficiency and tolerance to biotic and abiotic stresses (Cordovez et al., 2019; White et al., 2019; Liu et al., 2022a). The main members of the phytomicrobiome originate from the endosphere, phyllosphere and rhizosphere (Singh et al., 2020). Despite the different microbial communities, plant plays the main role in the plant holobiont and determine the composition and function of the microbiome (Sanchez-Canizares et al., 2017). Previous studies have demonstrated that host genotype and phenotype have an active impact on microbiome assembly (Bulgarelli et al., 2015; Perez-Jaramillo et al., 2017; Zhang et al., 2019). Thus, wild plants harbour a higher abundance and more diverse microbiome than their domesticated cultivars (Zachow et al., 2014; Mommer et al., 2018). O. officinalis may harbour a largely unexplored functional microbiome, which will offer an understanding of its synergetic adaptive evolution and reinstate the microbiome diversity of cultivated rice that has been reduced through domestication.
Endophytes, as nonpathogenic microorganisms, are pivotal components of plant-associated microbiomes that colonize every accessible host plant tissue (Wani et al., 2015). Among them, endophytic bacteria are attracting much attention for their direct or indirect positive effects on plant development and health (Afzal et al., 2019; White et al., 2019). Endophytic bacteria have PGP mechanisms similar to those of rhizobacteria, and both are recognized as plant growth promoting bacteria (PGPB) (Afzal et al., 2019). Most PGPB convert macronutrients (potassium, phosphorous and zinc) in soil and atmospheric nitrogen into available nutrients for plant uptake. A large number of PGPB synthesize phytohormones to regulate plant metabolism and nutrient accumulation, e.g., indole-3-acetic acid (IAA), gibberellins and ethylene. Some PGPB release 1-aminocyclopropane-1-carboxylate (ACC) deaminase to lower endogenous ethylene levels and promote plant growth under abiotic stress. Moreover, some of them also generate volatile organic compounds (VOCs) to interfere with quorum sensing of phytopathogens, synthesize siderophores for iron competition with phytopathogens, produce hydrolytic enzymes and antibiotics to kill phytopathogens, and induce systematic-acquired resistance (SAR) to strengthen the host immune system. Furthermore, some PGPB are able to produce antiherbivore compounds (e.g., alkaloids) and toxins to deter feeding by insects and other herbivores (Wani et al., 2015; White et al., 2019; Kumar and Nautiyal, 2022). Some endophytic bacteria also protect their host from metal toxicities and remediate metal-contaminated fields (Franco-Franklin et al., 2021). Due to their effective endophytic colonization, endophytic bacteria usually expand more significant PGP effects on plants than rhizobacteria, especially under stress conditions (Chanway et al., 2000; Afzal et al., 2019).
Most endophytic bacteria have a wide host range. When isolated and inoculated into host plants or nonhost plants, many of them have been shown to promote plant health, enhance crop productivity and improve stress responses (Sun et al., 2016; Lopes et al., 2018; Wang et al., 2019; Wang et al., 2020b). Due to their environmentally friendly and sustainable nature, endophytic bacteria can be exploited as biofertilizers, biocontrol and bioremediation agents to improve agricultural management and add value to the agricultural market.
Perennial rice is a new type of rice variety with the capacity to survive and be harvested for consecutive years (Yao et al., 2022; Zhang et al., 2022). Perennial rice production saves seed and labour costs by eliminating sowing or planting after the first growth seasons. It has extended application in China, South and Southeast Asia and Africa (Zhang et al., 2017; Huang et al., 2018). Due to long-term cultivation, ecological intensification strategies should be adopted for perennial rice to maintain sustainable production and soil health. Microbial fertilizers are a multi-win solution for perennial rice management without the environmental and food safety concerns caused by chemical products. During continuous cultivation, microbial fertilizers may autonomously and continuously remedy the loss of host-associated microbes for perennial rice. However, there are no effective microbial fertilizers specifically for perennial rice. Therefore, endophytic bacterial composition and diversity in the root, stem and leaf tissues of O. officinalis were analysed using 16S rRNA gene Illumina sequencing technology in this study. Endophytic bacteria of O. officinalis were subsequently isolated and identified. Moreover, their PGP traits in vitro were screened and PGP effects on perennial rice were also investigated. Our results provide a basic understanding of the endophytic micro-ecosystem in O. officinalis. Endophytic bacterial strain resources collected in this study can be developed as biofertilizers in compliance with the simplified and sustainable cultivation model of perennial rice.
In April 2020, germinated seeds of O. officinalis were grown for 20 days in a tray with sterilized soil and three healthy seedlings were transplanted equidistantly into a pot (15 cm in length, 15 cm in width and 30 cm in height) filled with 3 kg of sterilized field soil. Seedlings of O. officinalis were planted in six pots with four replicates, and a total of 18 plants were used for sampling. The pots were maintained under controlled conditions with 14 h daylight at 28°C and a 10 h dark period at 25°C in the greenhouse of Yunnan University (Kunming city, Yunnan Province, 24° 82′ 76″ N, 102° 84′ 52″ E). They were well watered with tap water to ensure plant health and maintain the soil under flooded conditions. The roots, stems, and leaves from 6 individuals at the flowering stage were sampled as one replicate, and 3 replicates were used for further study. Samples were washed successively in 70% ethanol for 2~5 min and 2.5% sodium hypochlorite for 2~3 min to remove microorganisms on the surface of tissues. Finally, they were rinsed with sterile water 1~3 times, and the residual water was absorbed from the tissue surface with sterile filter paper (Zhang et al., 2013). The final rinse water was cultured on Luria broth (LB) medium plates to verify the surface sterilization effect. One batch of samples was flash frozen in liquid nitrogen and stored at -80°C for DNA extraction, and the other batch was prepared for the isolation of endophytic bacteria.
DNA was extracted from tissue samples of O. officinalis using the OMEGA Soil DNA Kit (Omega Bio-Tek, Norcross, GA, USA); quantity and quality were measured using a NanoDrop NC2000 spectrophotometer (Thermo Fisher Scientific, Waltham, MA, USA) and agarose gel electrophoresis, respectively. PCR amplification of the V5-V7 region was performed using the forwards primer 799F (5’- AACMGGATTAGATACCCKG-3’) and the reverse primer 1193R (5’- ACGTCATCCCCACCTTCC -3’). PCR amplicons were purified with Vazyme VAHTSTM DNA Clean Beads (Vazyme, Nanjing, China) and quantified using the Quant-iT PicoGreen dsDNA Assay Kit (Invitrogen, Carlsbad, CA, USA). Paired-end sequencing was performed using the Illumina NovaSeq-PE250 platform at Shanghai Personal Biotechnology Co., Ltd. (Shanghai, China).
Raw sequences were processed to demultiplex, quality filter, denoise, merge, remove chimaeras and taxonomically annotate using QIIME2 (version 2019.4) (Bolyen et al., 2019). All raw sequences are publicly accessible in the Genome Sequence Archive (GSA) under accession number CRA008714 (https://ngdc.cncb.ac.cn/gsa/browse/CRA008714). After removing the sequences related to chloroplasts or mitochondria, amplicon sequence variants (ASVs) were generated for further analysis using the QIIME2 and R packages (version 3.2.0). Biomarkers were predicted with random forest classification. Alpha diversity (Shannon index, Chao1, Pielou index, Faith-PD, observed species and Good’s coverage) was analysed based on the normalized ASVs table and Wilcoxon rank-sum test. Beta diversity was visualized via nonmetric multidimensional scaling (NMDS) based on Bray-Curtis distance and ANOSIM similarity tests. Metacyc pathway analysis was conducted by the PICRUSt2 package (Douglas et al., 2020). Co-occurrence networks were constructed using SparCC and visualized using the R packages igraph and ggraph.
Surface-sterilized tissue samples were ground gently in a mortar using sterile distilled water. Tenfold serial dilutions (10-1-10-5) of ground suspension (100 μL) were plated on nutrient agar (NA) medium. After 1-7 d of inoculation at 30°C, morphologically unique bacterial colonies were selected and purified on fresh NA plates by repeated streaking.
The PGP traits of inorganic phosphate solubilization, organic phosphate solubilization, potassium release, siderophore production and nitrogen fixation were determined on Pikovskaya’s agar medium (Pikovskaya, 1948), Mongina organophosphorus culture medium (Hu et al., 2009), potassium bacteria agar medium (Yaghoubi et al., 2021), chrome azurol S (CAS) agar medium (Balasjin et al., 2022) and nitrogen-free Ashby’s medium (Patil et al., 2010), respectively. The presence of a transparent zone around the bacterial colony was considered an indicator of a positive reaction for phosphate solubilization or potassium release. A yellow halo formed around the bacterial colony was considered an indicator of a positive reaction for siderophore production. The ratio of the halo diameter to the colony diameter was defined as the mp value. Strains that grew well in nitrogen-free Ashby’s medium were recognized as nitrogen-fixing bacteria. The yields of IAA produced by bacteria cultured in precursor L-tryptophan medium were quantified using chloride perchloric acid reagent (FeCl3-HClO4) by colorimetric methods (Balasjin et al., 2022). ACC deaminase was induced on Dworkin & Foster (DF) salt minimal medium containing 3 mM ACC as the sole nitrogen source (Penrose and Glick, 2003). ACC deaminase activity was quantified by the amount of α-ketobutyrate produced by the cleavage of ACC. It was defined as the amount of α-ketobutyrate (μmol) produced by per mg of enzyme per hour. All traits were tested in triplicate and repeated three times. The nifH gene (PolF primer, 5’- TGCGAYCCSAARGCBGACTC-3’ and PolR primer, 5’- ATSGCCATCATYTCRCCGGA-3’) and acdS gene encoding ACC deaminase (acdSf3 primer, 5’-ATCGGCGGCATCCAGWSNAAYCANAC-3’ and acdSr4 primer, 5’ -GGCACGCCGCCCARRTGNRCRTA-3’) were amplified and sequenced (Pang et al., 2020).
Colony morphology and bacterium size of isolates were observed based on purified cultures on NA plates and Gram staining, respectively. The full-length 16S rRNA sequences of isolates were amplified using the following primers (27 forwards, 5’- AGAGTTTGATCCTGGCTCAG-3’ and 1492 reverse, 5’-GGTTACCTTGTTACGACTT-3’). The PCR amplification reaction (25 L) contained 2.5 μL of 10×Ex Taq Buffer (Mg2+ plus) (Takara, Beijing, China), 2 μL of dNTP mixture, 50 ng of the bacterial genomic DNA, 200 nM of each primer, 1.25 U of Ex Taq (Takara, Beijing, China) and 18.25 μL of H2O. The PCR amplification conditions were as follows: denaturation at 94°C for 2 min, followed by 35 cycles of 94°C for 30 s, 55°C for 30 s, 72°C for 1.5 min and final extension at 72°C for 5 min. After purification and sequencing, amplicons were analysed using the BLASTn algorithm in the NCBI GenBank database and then deposited in the GenBase database under the accession numbers presented in Table S4, which are publicly accessible at https://ngdc.cncb.ac.cn/genbase/. The phylogenetic tree was constructed using MEGA software (version 11) with the neighbour-joining method.
Healthy seeds of perennial rice (Geng/japonica cultivar PR23 and Xian/indica cultivar PR107) were surface-sterilized with 70% ethanol (v/v) for 1 min, followed by 30 min in 50% (v/v) commercial bleach with shaking at 180 rpm. Seeds were then washed 8-10 times with sterile distilled water and dried on autoclaved Whatman paper (3 mm) for 5 min. Then the surface sterilized seeds were soaked in 1×108 cfu/mL strain suspension for 12 h, whereas the control seeds were soaked in LB medium. After germination in the dark for 1 d at 35°C, 9 seeds were planted in a pot filled with sterile conventional nutrient soil (weakly acidic soil contained enough nitrogen, phosphorus and potassium) and placed in a growth chamber under controlled conditions with 14 h daylight at 28°C and a 10 h dark period at 25°C. Each treatment was repeated with three pots in triplicate. At the 4-leaf seedling stage of 30 days after germination, chlorophyll (SPAD) and nitrogen content were measured using a TYS-4 N leaf metre (Jinkelida, Beijing, China). Seedling height, root length, fresh weight and dry weight were then determined at the seedling stage.
Data are presented as the mean ± SD and were compared with SPSS software (version 20.0) using a one-way analysis of variance (ANOVA) according to Duncan’s multiple range test.
A total of 1,280,955 high-quality sequences were generated from 12 samples and assigned to 4,565 ASVs of root endophytic bacteria (REB), 850 ASVs of stem endophytic bacteria (SEB) and 719 ASVs of leaf endophytic bacteria (LEB) (Figure S1A). The number of ASVs, specific ASVs and taxa were highest in REB, followed by those in SEB, whereas the lowest numbers were observed in LEB (Figures S1B, C). Proteobacteria (39.30-79.12%) and Gammaproteobacteria (14.94-46.28%) were the dominant phylum and class, respectively, across all tissue endospheres of O. officinalis (Figures 1A, B). The average relative abundance of the top 10 dominant bacterial classes exceeded 1% (Figure 1B).
Figure 1 Relative abundances of the endophytic bacterial dominant phyla (A) and classes (B) in Oryza officinalis. The heatmap shows the top 20 relative abundances of the dominant endophytic bacterial genera in each tissue (C). The importance of the top 10 dominant endophytic bacterial genera is identified based on random forest classification models and their relative abundance in each tissue (D).
In addition, the relative abundance of the taxa at each taxonomic level exhibited considerable variation among the different tissue types. Among the top 20 relative abundances of genera, WCHB1-41, Bacteroidetes_vadin HA17, Syntrophorhabdus, Haliangium, Treponema, Christensenellaceae R-7 group and SB-5 were dominant in the root endosphere; Aquabacterium, SJA-15 and Aminicenantales were dominant in the stem endosphere; and Rubrobacter, Allorhizobium-Neorhizobium-Pararhizobium-Rhizobium, Alteribacillus, Pseudolabrys, Sphingomonas, Ochrobactrum, and Methylobacterium were dominant in the leaf endosphere (Figure 1C).
Based on random forest classification model analysis, 10 bacterial genera were predicted as biomarkers in the endospheres of O. officinalis, and their relative abundances also differed among tissue types (Figure 1D). For example, the relative abundances of Desulfomonile and Ruminiclostridium were highest in the REB; the relative abundances of Lactobacillus, Acinetobacter, Cutibacterium and Dechloromonas were highest in the SEB; and the relative abundances of Stenotrophomonas, Chryseobacterium, Achromobacter and Methylobacterium were highest in the LEB, suggesting that the bacterial endospheres of O. officinalis have tissue-specific biomarkers. However, no common taxa were found in all three compartments These results indicate that the differentiation and growth of O. officinalis tissues shape the discriminative endophytic bacterial communities.
The alpha diversity indices (Shannon, Chao1, Pielou, Faith-PD and observed species) of REB were significantly higher than those of SEB and LEB (p < 0.05), indicating that species diversity, richness and evenness as well as the phylogenetic diversity of REB were highest across all tissue endospheres of O. officinalis (Figure 2A). The Good’s coverage index of the REB, SEB and LEB samples was greater than 99.9%, suggesting that the data were highly representative of the actual endophytic bacterial communities of O. officinalis (Figure 2A). Beta-diversity analysis using NMDS ordinations and ANOSIM also confirmed the significant differences among REB, SEB and LEB (p < 0.01) (Figure 2B). These results indicate that the roots of O. officinalis may harbor a distinct endophytic bacterial community structure with the greatest diversity, whereas the stems and leaves may share homologous endophytic bacterial community structures.
Figure 2 Shannon index (species diversity), Chao1 (species richness), Pielou index (species evenness), Faith-PD (phylogenetic diversity), observed species and Good’s coverage of endophytic bacterial communities in different tissues of Oryza officinalis are presented in box plots of alpha diversity. The p value of the Kruskal-Wallis test is shown under the alpha diversity index label (A). Nonmetric multidimensional scaling plot (NMDS) analysis for endophytic bacterial communities associated with O. officinalis was estimated using Bray-Curtis distance and ANOSIM similarity tests (B).
A total of 59 MetaCyc pathways were predicted in the endophytic bacterial communities of O. officinalis (Figure 3A). The relative abundance of ASVs involved in biosynthesis was highest in comparison with those involved in other metabolic pathways, suggesting that endophytic bacteria of O. officinalis may produce various metabolites to interact with their communities and host. Thirty-four, eleven and two significant differential MetaCyc pathways were enriched in the comparison groups of root vs. leaf, root vs. stem and stem vs. leaf, respectively, indicating that the root endosphere had more features and functionality of biosynthesis, degradation and biology; furthermore, the stem and leaf endospheres shared various common functions (Figure 3B and Table S1).
Figure 3 MetaCyc pathways of endophytic bacteria in Oryza officinalis (A) and the significantly differential MetaCyc pathways enriched in the root vs. leaf, root vs. stem and stem vs. leaf comparison groups (B).
The endophytic bacterial co-occurrence network of O. officinalis, with an average degree of 64.81, had three putative modules that might be reassembled for these specific ecological functions (Figure S2A). A total of 178 ASVs constituted the network nodes among which 50 ASVs coacted in tight Module 1 and 74 and 54 ASVs coacted in loose Module 2 and Module 3, respectively (Table S2). The genera SJA-15, Methylobacterium, Alteribacillus, Burkholderia-Caballeronia-Paraburkholderia, Ochrobactrum, Aquabacterium, Prauserella, WCHB1-41, Bradyrhizobium, and Bacteroidetes vadin HA17 were the top nodes in this network (Figure S2B). There was no obvious difference in the number of nodes and edges and the average degree among subnetworks for REB, SEB and LEB, which is perhaps related to the small sample size of each subnetwork (Figure S2C).
A total of 99 endophytic bacterial strains were isolated from the root, stem and leaf tissues of O. officinalis, including 42 REB, 26 SEB and 31 LEB (Table S3). Among them, 96 endophytic bacterial strains had at least one PGP trait of phosphate solubilization, potassium release, nitrogen fixation, ACC deaminase activity, or siderophore/IAA production (Figure 4, Figure S3 and Table S3). In addition, nifH and acdS genes were detected in 19 and 34 strains with nitrogen fixating abilities and ACC deaminase activity, respectively, indicating that they had the genetic potential for N2 fixation and ethylene regulation (Figure S3). Thus, these strains were preliminarily characterized as PGPB. The number of PGPB isolated from root tissues was greater than that isolated from stem and leaf tissues. However, their PGP abilities in vitro were not significantly different among the REB, SEB and LEB (Figure 4A). These results suggest that O. officinalis may assemble a large number of endophytic bacteria with PGP traits in each tissue compartment to aid in the acquisition of nutrients from the environment, phytohormone production and stress tolerance.
Figure 4 Plant growth-promoting bacteria isolated from O. officinalis were determined in vitro. Each PGP ability value of the strains isolated from the root, stem and leaf tissues are represented by green, brown and blue dots, respectively (A). Phylogenetic tree, morphological characteristics and PGP traits of 11 strains selected for inoculation of perennial rice (B).
Eleven strains were selected for further study of their effects on perennial rice based on their IAA production abilities (yields >3 mg/L) (Figure 4B and Table S3). Based on morphological observations and full-length 16S rRNA sequence analysis, these strains were identified as Enterobacter mori (strain OOR1-8), Enterobacter ludwigii (strains OOR3-15, OOL2-1 and OOL3-11), Enterobacter cloacae (strain OOL3-3), Bacillus amyloliquefaciens (strains OOR3-4 and OOS2-5), Bacillus siamensis (strains OOS3-4 and OOL2-3), Pseudomonas rhodesiae (strain OOR2-11), and Kosakonia oryzae (strains OOR3-1) (Figure 4B and Table S4).
Seeds from the perennial rice varieties PR23 (japonica subspecies) and PR107 (indica subspecies) were inoculated individually with each of the eleven strains. Generally, inoculated PR23 and PR107 grew faster and stronger than the noninoculated control plants (Figure 5). Except for B. amyloliquefaciens OOS2-5, most strains significantly promoted the seedling height, fresh weight, chlorophyll and nitrogen content of PR23, and E. mori OOL3-11 and E. ludwigii OOR3-15, OOR1-8 and OOL2-1 also promoted the root length of PR23 (Table 1). All strains significantly promoted the fresh weight and chlorophyll content of PR107 and most promoted the root length and nitrogen content of PR107. Only P. rhodesiae OOR2-11 and B. siamensis OOL2-3 promoted the seedling height of PR107 (Table 2). These results suggest that E. mori, E. ludwigii, E. cloacae, B. amyloliquefaciens, B. siamensis, P. rhodesiae and K. oryzae with IAA production and other PGP traits could promote perennial rice seedling development and enhance their biomass accumulation, chlorophyll content, and nitrogen uptake.
Figure 5 Effects of 11 bacteria strains isolated from Oryza officinalis on perennial rice growth. PR23 is a japonica subspecies (A), and PR107 is an indica subspecies (B).
Table 1 Growth-promoting effects of 11 endophytic bacterial strains isolated from Oryza officinalis on perennial rice PR23.
Table 2 Growth-promoting effects of 11 endophytic bacterial strains isolated from Oryza officinalis on perennial rice PR107.
Wild rice, including 22 species of genome types (AA, BB, CC, EE, FF, GG, BBCC, CCDD, HHJJ and KKLL), possesses much higher genetic diversity than domesticated rice (O. sativa) (Kajiya-Kanegae et al., 2021). Their genotypes mainly determine the assembly of plant-associated microbiome, suggesting that wild rice recruits more powerful host-associated microbiomes to regulate environmental adaptation than domesticated rice (Yin et al., 2023). Rhizosphere and phyllosphere microbial communities have been characterized in O. rufipogon and O. longistaminata, while the endophytic microbiome has rarely been reported in most wild rice relatives (Peng et al., 2021; Xie et al., 2021; Chang et al., 2022; Xu et al., 2022). Our study provided insight into the endophytic bacterial community in O. officinalis. Compared to previous studies, the endophytic bacterial composition of wild rice and cultivated rice is consistent at the phylum level and class level regardless of genetic and ecological distance. Proteobacteria and Gammaproteobacteria are typically the dominant phylum and class in rice, respectively (Moronta-Barrios et al., 2018; Kim and Lee, 2020; Lin et al., 2020; Chu et al., 2021; Kunda et al., 2021; Peng et al., 2021).
Endophytic bacterial microbiomes exhibit high variation in genus composition and abundance patterns among different rice species and varieties, resulting in different host-specific biomarkers. For instance, Desulfomonile, Lactobacillus, Stenotrophomonas, etc., are biomarkers of the endophytic bacterial microbiome of O. officinalis, compared with Methylobacteriaceae and Streptococcus in African wild rice (O. longistaminata) and Pseudomonas and Ralstonia in cultivated rice (Chu et al., 2021; Peng et al., 2021).
The microbial interaction network with high correlations between microbial groups maintains a stable and sustainable ecological network to respond to environmental changes, which are directly beneficial for the host (Coyte et al., 2015). The key set of microbial species in the cooperative network could play a critical role in driving the formation of communities and promoting microecological stability. O. officinalis uses Methylobacterium, Alteribacillus, Burkholderia-Caballeronia-Paraburkholderia, Ochrobactrum, Aquabacterium, etc., to establish linkages between endophytic bacteria. The endophytic bacterial network structure of O. officinalis showed a different connectivity pattern than those of O. longistaminata and cultivated rice (Chu et al., 2021; Peng et al., 2021; Singha et al., 2021; Xie et al., 2021). Thus, rice genotype is the critical influencing factor of their endophytic bacterial community structure and interaction.
Due to the differentiation of structure, function and nutrient environment in tissues, rice plants shape distinctive ecological niches for endophytes in the different compartments. The root endosphere has received more attention than the stem and leaf endospheres in most previous studies because of its importance for interactions between roots and soil (Beckers et al., 2017; Yu and Hochholdinger, 2018; Carrion et al., 2019; Kim and Lee, 2020; Sun et al., 2021). The frequent exchange of microbiota between the root endosphere and rhizosphere results in a larger and more effective endophytic microbiota being recruited by rice roots than by aboveground compartments.
In this study, the bacterial composition, abundance, diversity, and functional profile of the root endosphere in O. officinalis were significantly different from those of the leaf and stem endospheres. Similar results were found in the endospheres of O. longistaminata, black rice and other plants (Sun et al., 2020; Kim and Lee, 2021; Peng et al., 2021; Singha et al., 2021; Lin et al., 2022; Sun et al., 2022). Desulfomonile and Ruminiclostridium, with the capability of nitrogen fixation and cellulose degradation (Ju et al., 2007; Kampik et al., 2020), are REB-specific biomarkers of O. officinalis. Lactobacillus, Acinetobacter, and Dechloromonas are SEB-specific biomarkers of O. officinalis and are involved in inhibition against phytopathogenic bacteria, nitrogen removal, biodegradation and iron oxidation (Chakraborty and Picardal, 2013; Silambarasan and Vangnai, 2016; Tsuda et al., 2016; Ke et al., 2022). Stenotrophomonas, Chryseobacterium, Achromobacter and Methylobacterium, as LEB-specific biomarkers of O. officinalis, can promote plant growth and nutrient uptake, suppress plant pathogens and reduce arsenic accumulation in rice grains (Suckstorff and Berg, 2003; Kim et al., 2010; Sang and Kim, 2012; Wang et al., 2020a). These results indicated that endophytic bacteria with PGP potential assemble and colonize different compartments of O. officinalis, whose functions may evolve compatibly with the functions of the host tissues. Moreover, tissue-specific endophytic bacterial biomarkers are firmly controlled by rice genotype. For instance, Rhizobium, AB2 and Leptonema are LEB-specific biomarkers of O. longistaminata; Methylobacterium and Nevskia are SEB-specific biomarkers; while Enhydrobacter, YS2 and Roseburia are rhizome-specific biomarkers (Peng et al., 2021), which are completely different from O. officinalis.
Many endophytic bacteria with PGP traits have been isolated from wild rice species such as O. alta, O. brachyantha, O. eichingeri, O. latifolia, O. longiglumis, O. meridionalis, O. minuta, O. officinalis, O. punctata, O. ridley and O. rufipogon (Borah et al., 2021; Zhang et al., 2021; Liu et al., 2022b). Only a few species have been applied to cultivated rice, and even fewer have been applied to perennial rice. Microbacterium strain B24 with cell wall degradation enzyme activities and Herbaspirillum strain B501 with the ability to fix nitrogen in plants were isolated and functionally identified from O. officinalis (Elbeltagy et al., 2000; Elbeltagy et al., 2001). In this study, 96.97% of endophytic bacterial strains isolated from O. officinalis had at least one PGP trait of phosphate solubilization, potassium release, nitrogen fixation, ACC deaminase, or siderophore/IAA production. The PGPB isolation efficiency is remarkably higher from endophytic bacteria of O. officinalis than from those of O. rufipogon (28.48%) (Zhang et al., 2021). Plant genomic diversity positively regulates the composition and diversity of phytomicrobiome (Sutherland et al., 2022; Zhang et al., 2023). Previous studies showed that the O. officinalis genome (average 10.26 Gbp) was larger than O. rufipogon genome (average 6.83 Gbp), indicating that O. officinalis has higher genetic diversity than O. rufipogon (Kajiya-Kanegae et al., 2021). Thus, O. officinalis might also have higher microbiome diversity than O. rufipogon, indicating more endophytic bacteria with the abilities of stress resistance and environmental adaptability in O. officinalis.
Colonization of PGPB in the rhizosphere and phyllosphere is a necessary prerequisite for their successful application under field conditions (Rilling et al., 2019). IAA production activity is the first useful trait for selecting promising endophytic bacteria with more PGP potential and a high colonization rate in rice plants (Etesami et al., 2015). Eleven strains identified as Enterobacter, Bacillus, Pseudomonas and Kosakonia species were selected for inoculation of perennial rice based on their IAA yields ranging from 3.02 to 23.2 mg/L. Enterobacter, Bacillus and Pseudomonas were detected from three tissues using 16S rRNA sequencing, but Kosakonia was not captured. The possible reason is either the extremely low abundance of Kosakonia species in O. officinalis or the low amplification efficiency for these species using 16S rRNA (V5-V7) sequence primers.
Using the method of soaking seeds, all taxa encourage perennial rice to form a strong root system, stimulate biomass accumulation, and increase chlorophyll content and nitrogen uptake, which are promising features of biofertilizers specifically targeted at the successive planting of perennial rice. Therefore, IAA trait in vitro is effective and time-saving for screening PGPB for perennial rice. Moreover, several Enterobacter, Bacillus, Pseudomonas and Kosakonia species have been reported to have potential for growth promotion and stress tolerance in rice (Sherpa et al., 2021; Raturi et al., 2022; Shukla et al., 2023; Yang et al., 2023). Interestingly, E. ludwigii OOL3-11 with only IAA production activity had a positive effect on perennial rice seedling growth, which was comparable with those strains with more PGP traits. This is probably because the PGP effects of those strains with more PGP traits are limited under normal perennial rice growth conditions. However, they may exert their PGP effects (e.g., ACC deaminase and siderophore production) sufficiently under nutrient deficiency and stress conditions (Sharma and Johri, 2003; Moon and Ali, 2022).
B. amyloliquefaciens is a commercially successful biocontrol agent that can also enhance the yield of crop plants (Chowdhury et al., 2015). Interestingly, B. amyloliquefaciens OOS2-5 with the PGP traits of IAA production and organic-phosphate solubilization promotes PR107 (indica subspecies) growth but does not affect PR23 (japonica subspecies). The endophytic colonization and PGP effect of this strain has some differences in the japonica and indica subspecies. However, B. amyloliquefaciens OOR3-4 with more PGP traits of IAA production, organic-phosphate solubilization, potassium release, siderophore production and ACC deaminase activity can promote the growth of both perennial rice varieties. It is suggested that other PGP traits of B. amyloliquefaciens isolates are also important for endophytic colonization and the in-plant promoting effect on different hosts.
Four bacterial strains, Pseudomonas mosselii, Microvirga, Paenibacillus rigui, and P. graminis isolated from Asian rice species (O. sativa) showed a rice genotype-dependent manner of rice growth promotion (Balasjin et al., 2022), which is similar to B. amyloliquefaciens OOS2-5. These results also reveal that endophytic bacterial behavior with regard to rice growth is affected by rice genotype, which cannot be predicted by bacterial taxa and their PGP traits in vitro. It also reminds us that the application of microbial fertilizers for different perennial rice varieties needs to consider the bacterial isolate-specific and rice genotype-specific PGP effects.
The composition, diversity and function of endophytic bacterial communities in the root, stem and leaf tissues of O. officinalis were first characterized in this work, revealing the tissue-specific endophytic bacterial microbiomes in O. officinalis. A number of endophytic bacteria with PGP traits were isolated from O. officinalis. Among them, 11 bacterial strains belonging to the Enterobacter, Bacillus, Pseudomonas and Kosakonia species promoted the growth of perennial rice seedlings and have utilization potential as biofertilizers for the sustained productivity of perennial rice. The stable and long-lasting PGP effects on perennial rice should be further evaluated in the field. Moreover, it is worth considerable research on the mechanism of interaction between these strains and different perennial rice varieties, which will make good use of bacteria to improve the agronomic traits of perennial rice.
The datasets presented in this study can be found in online repositories. The names of the repository/repositories and accession number(s) can be found in the article/Supplementary Material.
SQ conceived and designed the research. QT and YG conducted the experiments and set up the data under SQ guidance. YG, SL and DK isolated and identified the endophytic bacteria. QT and MJ performed the bacterial phenotyping analysis. ZX, RT and LW performed in-plant phenotyping analysis under LH and FH guidance. FH provided rice materials. SQ and LH wrote the manuscript.
This work was supported by funding from the National Natural Science Foundation of China (32060593, 32272079 and 32060474), the Yunnan Provincial Science and Technology Department (202101AT070021, 202101AS070001 and 202201BF070001-011) and the Yunnan Provincial Department of Education Science Research Fund Project (2023J0006).
The authors declare that the research was conducted in the absence of any commercial or financial relationships that could be construed as a potential conflict of interest.
All claims expressed in this article are solely those of the authors and do not necessarily represent those of their affiliated organizations, or those of the publisher, the editors and the reviewers. Any product that may be evaluated in this article, or claim that may be made by its manufacturer, is not guaranteed or endorsed by the publisher.
The Supplementary Material for this article can be found online at: https://www.frontiersin.org/articles/10.3389/fpls.2023.1184489/full#supplementary-material
Afzal, I., Shinwari, Z. K., Sikandar, S., Shahzad, S. (2019). Plant beneficial endophytic bacteria: Mechanisms, diversity, host range and genetic determinants. Microbiological Res. 221, 36–49. doi: 10.1016/j.micres.2019.02.001
Balasjin, N. M., Maki, J. S., Schläppi, M. R., Marshall, C. W. (2022). Plant growth-promoting activity of bacteria isolated from Asian rice (Oryza sativa L.) depends on rice genotype. Microbiol. Spectrum. 10, e02787–e02721. doi: 10.1128/spectrum.02787-21
Beckers, B., De Beeck, M. O., Weyens, N., Boerjan, W., Vangronsveld, J. (2017). Structural variability and niche differentiation in the rhizosphere and endosphere bacterial microbiome of field-grown poplar trees. Microbiome. 5 (1), 25. doi: 10.1186/s40168-017-0241-2
Bolyen, E., Rideout, J. R., Dillon, M. R., Bokulich, N. A., Abnet, C. C., Al-Ghalith, G. A., et al. (2019). Reproducible, interactive, scalable and extensible microbiome data science using QIIME 2. Nat. Biotechnol. 37, 852–857. doi: 10.1038/s41587-019-0209-9
Borah, M., Das, S., Bora, S. S., Boro, R. C., Barooah, M. (2021). Comparative assessment of multi-trait plant growth-promoting endophytes associated with cultivated and wild Oryza germplasm of Assam, India. Arch. Microbiol. 203, 2007–2028. doi: 10.1007/s00203-020-02153-x
Bulgarelli, D., Garrido-Oter, R., Munch, P. C., Weiman, A., Droge, J., Pan, Y., et al. (2015). Structure and function of the bacterial root microbiota in wild and domesticated Barley. Cell Host Microbe 17, 392–403. doi: 10.1016/j.chom.2015.01.011
Carrion, V. J., Perez-Jaramillo, J., Cordovez, V., Tracanna, V., De Hollander, M., Ruiz-Buck, D., et al. (2019). Pathogen-induced activation of disease-suppressive functions in the endophytic root microbiome. Science. 366 (6465), 606–612. doi: 10.1126/science.aaw9285
Chakraborty, A., Picardal, F. (2013). Neutrophilic, nitrate-dependent, Fe(II) oxidation by a Dechloromonas species. World J. Microbiol. Biotechnol. 29, 617–623. doi: 10.1007/s11274-012-1217-9
Chang, J. J., Tian, L., Leite, M. F. A., Sun, Y., Shi, S. H., Xu, S. Q., et al. (2022). Nitrogen, manganese, iron, and carbon resource acquisition are potential functions of the wild rice Oryza rufipogon core rhizomicrobiome. Microbiome. 10, 196. doi: 10.1186/s40168-022-01360-6
Chanway, C. P., Shishido, M., Nairn, J., Jungwirth, S., Markham, J., Xiao, G., et al. (2000). Endophytic colonization and field responses of hybrid spruce seedlings after inoculation with plant growth-promoting rhizobacteria. Forest Ecology and Management 133, 81-88. doi: 10.1016/S0378-1127(99)00300-X
Chen, L., Yin, F., Zhang, D., Xiao, S., Zhong, Q., Wang, B., et al. (2022). Unveiling a novel source of resistance to bacterial blight in medicinal wild rice. Oryza officinalis. Life-Basel. 12 (6), 872. doi: 10.3390/life12060827
Chowdhury, S. P., Hartmann, A., Gao, X. W., Borriss, R. (2015). Biocontrol mechanism by root-associated Bacillus amyloliquefaciens FZB42-a review. Front. Microbiol. 6. doi: 10.3389/fmicb.2015.00780
Chu, C., Fan, M., Song, C., Li, N., Zhang, C., Fu, S., et al. (2021). Unveiling endophytic bacterial community structures of different rice cultivars grown in a cadmium-contaminated paddy field. Front. Microbiol. 12. doi: 10.3389/fmicb.2021.756327
Cordovez, V., Dini-Andreote, F., Carrion, V. J., Raaijmakers, J. M. (2019). Ecology and evolution of plant microbiomes. Annu. Rev. Microbiol. 73, 69. doi: 10.1146/annurev-micro-090817-062524
Coyte, K. Z., Schluter, J., Foster, K. R. (2015). The ecology of the microbiome: Networks, competition, and stability. Science. 350, 663–666. doi: 10.1126/science.aad2602
Devanna, N. B., Vijayan, J., Sharma, T. R. (2014). The blast resistance gene Pi54 of cloned from Oryza officinalis interacts with Avr-Pi54 through its novel non-LRR domains. PloS One 9, e104840. doi: 10.1371/journal.pone.0104840
Douglas, G. M., Maffei, V. J., Zaneveld, J., Yurgel, S. N., Brown, J. R., Taylor, C. M., et al. (2020). PICRUSt2: An improved and customizable approach for metagenome inference. Cold Spring Harbor Laboratory. 672295. doi: 10.1101/672295
Elbeltagy, A., Nishioka, K., Sato, T., Suzuki, H., Ye, B., Hamada, T., et al. (2001). Endophytic colonization and in planta nitrogen fixation by a Herbaspirillum sp. isolated from wild rice species. Appl. Environ. Microbiol. 67, 5285–5293. doi: 10.1128/AEM.67.11.5285-5293.2001
Elbeltagy, A., Nishioka, K., Suzuki, H., Sato, T., Sato, Y.-I., Morisaki, H., et al. (2000). Isolation and characterization of endophytic bacteria from wild and traditionally cultivated rice varieties. Soil Sci. Plant Nutr. 46, 617–629. doi: 10.1080/00380768.2000.10409127
Etesami, H., Alikhani, H. A., Hosseini, H. M. (2015). Indole-3-acetic acid (IAA) production trait, a useful screening to select endophytic and rhizosphere competent bacteria for rice growth promoting agents. MethodsX. 2, 72–78. doi: 10.1016/j.mex.2015.02.008
Franco-Franklin, V., Moreno-Riascos, S., Ghneim-Herrera, T. (2021). Are endophytic bacteria an option for increasing heavy metal tolerance of plants? A meta-analysis of the effect size. Front. Environ. Science. 8. doi: 10.3389/fenvs.2020.603668
Hirabayashi, H., Sasaki, K., Kambe, T., Gannaban, R. B., Miras, M. A., Mendioro, M. S., et al. (2015). qEMF3, a novel QTL for the early-morning flowering trait from wild rice, Oryza officinalis, to mitigate heat stress damage at flowering in rice, O. sativa. J. Exp. Botany. 66, 1227–1236. doi: 10.1093/jxb/eru474
Hu, J., Lin, X., Wang, J., Chu, H., Yin, R., Zhang, J. (2009). Population size and specific potential of P-mineralizing and -solubilizing bacteria under long-term P-deficiency fertilization in a sandy loam soil. Pedobiologia. 53, 49–58. doi: 10.1016/j.pedobi.2009.02.002
Huang, G. F., Qin, S. W., Zhang, S. L., Cai, X. L., Wu, S. K., Dao, J. R., et al. (2018). Performance, economics and potential impact of perennial rice PR23 relative to annual rice cultivars at multiple locations in Yunnan Province of China. Sustainability. 10, 1086. doi: 10.3390/su10041086
Ju, X., Zhao, L., Sun, B. (2007). Nitrogen fixation by reductively dechlorinating bacteria. Environ. Microbiol. 9, 1078–1083. doi: 10.1111/j.1462-2920.2006.01199.x
Kajiya-Kanegae, H., Ohyanagi, H., Ebata, T., Tanizawa, Y., Onogi, A., Sawada, Y., et al. (2021). OryzaGenome2.1: database of diverse genotypes in wild oryza species. Rice. 14 (1), 1–8. doi: 10.1186/s12284-021-00468-x
Kampik, C., Denis, Y., Pagès, S., Perret, S., Tardif, C., Fierobe, H.-P., et al. (2020). A novel two-component system, XygS/XygR, positively regulates xyloglucan degradation, import, and catabolism in Ruminiclostridium cellulolyticum. Appl. Environ. Microbiol. 86, e01357–e01320. doi: 10.1128/AEM.01357-20
Ke, X., Liu, C., Tang, S. Q., Guo, T. T., Pan, L., Xue, Y. P., et al. (2022). Characterization of Acinetobacter indicus ZJB20129 for heterotrophic nitrification and aerobic denitrification isolated from an urban sewage treatment plant. Bioresource Technology. 347, 126423. doi: 10.1016/j.biortech.2021.126423
Kim, H., Lee, Y. H. (2020). The rice microbiome: A model platform for crop holobiome. Phytobiomes J. 4, 5–18. doi: 10.1094/pbiomes-07-19-0035-rvw
Kim, H., Lee, Y. H. (2021). Spatiotemporal assembly of bacterial and fungal communities of seed-seedling-adult in rice. Front. Microbiol. 12. doi: 10.3389/fmicb.2021.708475
Kim, K., Yim, W., Trivedi, P., Madhaiyan, M., Deka Boruah, H. P., Islam, M. R., et al. (2010). Synergistic effects of inoculating arbuscular mycorrhizal fungi and Methylobacterium oryzae strains on growth and nutrient uptake of red pepper (Capsicum annuum L.). Plant Soil. 327, 429–440. doi: 10.1007/s11104-009-0072-4
Kiran, T. V., Rao, Y. V., Subrahmanyam, D., Rani, N. S., Bhadana, V. P., Rao, P. R., et al. (2013). Variation in leaf photosynthetic characteristics in wild rice species. Photosynthetica. 51, 350–358. doi: 10.1007/s11099-013-0032-3
Kitazumi, A., Pabuayon, I. C. M., Ohyanagi, H., Fujita, M., Osti, B., Shenton, M. R., et al. (2018). Potential of Oryza officinalis to augment the cold tolerance genetic mechanisms of Oryza sativa by network complementation. Sci. Rep. 8, 16346. doi: 10.1038/s41598-018-34608-z
Kumar, V., Nautiyal, C. S. (2022). Plant abiotic and biotic stress alleviation: From an endophytic microbial perspective. Curr. Microbiol. 79, 311. doi: 10.1007/s00284-022-03012-2
Kunda, P., Mukherjee, A., Dhal, P. K. (2021). Insights into endophytic bacterial diversity of rice grown across the different agro-ecological regions of West Bengal, India. World J. Microbiol. Biotechnol. 37, 184. doi: 10.1007/s11274-021-03153-9
Lin, G. Y., Lin, C. Y., Chang, S. J., Lin, W. Y. (2020). The dynamics of endophytic bacterial community structure in rice roots under different field management systems. Agronomy. 10 (11), 1623–1643. doi: 10.3390/agronomy10111623
Lin, H., Liu, C., Peng, Z., Tan, B., Wang, K., Liu, Z. (2022). Distribution pattern of endophytic bacteria and fungi in tea plants. Front. Microbiol. 13. doi: 10.3389/fmicb.2022.872034
Liu, D., Lin, L., Zhang, T., Xu, Q., Wang, M. L., Gao, M. H., et al. (2022a). Wild Panax plants adapt to their thermal environment by harboring abundant beneficial seed endophytic bacteria. Front. Ecol. Evol. 10. doi: 10.3389/fevo.2022.967692
Liu, L. H., Yuan, T., Zhang, J. Y., Tang, G. X., Lu, H. X., Zhao, H. M., et al. (2022b). Diversity of endophytic bacteria in wild rice (Oryza meridionalis) and potential for promoting plant growth and degrading phthalates. Sci. Total Environ. 806, 150310. doi: 10.1016/j.scitotenv.2021.150310
Liu, R., Zhang, H. H., Chen, Z. X., Shahid, M. Q., Fu, X. L., Liu, X. D. (2015). Drought-tolerant rice germplasm developed from an Oryza officinalis transformation-competent artificial chromosome clone. Genetice Mol. Res. 14, 13667–13678. doi: 10.4238/2015.october.28.29
Lopes, R., Tsui, S., Goncalves, P., De Queiroz, M. V. (2018). A look into a multifunctional toolbox: endophytic Bacillus species provide broad and underexploited benefits for plants. World J. Microbiol. Biotechnol. 34, 94. doi: 10.1007/s11274-018-2479-7
Mommer, L., Cotton, T. E. A., Raaijmakers, J. M., Termorshuizen, A. J., Van Ruijven, J., Hendriks, M., et al. (2018). Lost in diversity: the interactions between soil-borne fungi, biodiversity and plant productivity. New Phytologist. 218, 542–553. doi: 10.1111/nph.15036
Moon, Y. S., Ali, S. (2022). Possible mechanisms for the equilibrium of ACC and role of ACC deaminase-producing bacteria. Appl. Microbiol. Biotechnol. 106, 877–887. doi: 10.1007/s00253-022-11772-x
Moronta-Barrios, F., Gionechetti, F., Pallavicini, A., Marys, E., Venturi, V. (2018). Bacterial microbiota of rice roots: 16S-based taxonomic profiling of endophytic and rhizospheric diversity, endophytes isolation and simplified endophytic community. Microorganisms. 6 (1), 14–34. doi: 10.3390/microorganisms6010014
Pang, Z., Zhao, Y., Xu, P., Yu, D. (2020). Microbial diversity of upland rice roots and their influence on rice growth and drought tolerance. Microorganisms. 8, 1329. doi: 10.3390/microorganisms8091329
Patil, S. V., Salunkhe, R. B., Patil, C. D., Patil, D. M., Salunke, B. K. (2010). Bioflocculant exopolysaccharide production by Azotobacter indicus using flower extract of Madhuca latifolia L. Appl. Biochem. Biotechnol. 162, 1095–1108. doi: 10.1007/s12010-009-8820-8
Peng, X., Xie, J., Li, W., Xie, H., Cai, Y., Ding, X. (2021). Comparison of wild rice (Oryza longistaminata) tissues identifies rhizome-specific bacterial and archaeal endophytic microbiomes communities and network structures. PloS One 16, e0246687. doi: 10.1371/journal.pone.0246687
Penrose, D., Glick, B. (2003). Methods for isolating and characterizing ACC deaminase-containing plant growth-promoting rhizobacteria. Physiologia plantarum. 118, 10–15. doi: 10.1034/j.1399-3054.2003.00086.x
Perez-Jaramillo, J. E., Carrion, V. J., Bosse, M., Ferrao, L. F. V., De Hollander, M., Garcia, A., et al. (2017). Linking rhizosphere microbiome composition of wild and domesticated Phaseolus vulgaris to genotypic and root phenotypic traits. ISME J. 11, 2244–2257. doi: 10.1038/ismej.2017.85
Pikovskaya, R. I. (1948). Mobilization of phosphorus in soil in connection with the vital activity of some microbial species. Mikrobiologiya. 17, 362–370.
Raturi, G., Sharma, Y., Mandlik, R., Kumawat, S., Rana, N., Dhar, H., et al. (2022). Genomic landscape highlights molecular mechanisms involved in silicate solubilization, stress tolerance, and potential growth-promoting activity of Bacterium Enterobacter sp. LR6. Cells. 11, 3622. doi: 10.3390/cells11223622
Rilling, J. I., Acuña, J. J., Nannipieri, P., Cassan, F., Maruyama, F., Jorquera, M. A. (2019). Current opinion and perspectives on the methods for tracking and monitoring plant growth-promoting bacteria. Soil Biol. Biochem. 130, 205–219. doi: 10.1016/j.soilbio.2018.12.012
Sanchez-Canizares, C., Jorrin, B., Poole, P. S., Tkacz, A. (2017). Understanding the holobiont: the interdependence of plants and their microbiome. Curr. Opin. Microbiol. 38, 188–196. doi: 10.1016/j.mib.2017.07.001
Sang, M. K., Kim, K. D. (2012). Plant growth-promoting rhizobacteria suppressive to Phytophthora blight affect microbial activities and communities in the rhizosphere of pepper (Capsicum annuum L.) in the field. Appl. Soil Ecology. 62, 88–97. doi: 10.1016/j.apsoil.2012.08.001
Sharma, A., Johri, B. N. (2003). Growth promoting influence of siderophore-producing Pseudomonas strains GRP3A and PRS9 in maize (Zea mays L.) under iron limiting conditions. Microbiological Res. 158, 243–248. doi: 10.1078/0944-5013-00197
Sherpa, M. T., Sharma, L., Bag, N., Das, S. (2021). Isolation, characterization, and evaluation of native rhizobacterial consortia developed from the rhizosphere of rice grown in organic state Sikkim, India, and their effect on plant growth. Front. Microbiol. 12. doi: 10.3389/fmicb.2021.713660
Shukla, A., Gupta, A., Srivastava, S. (2023). Bacterial consortium (Priestia endophytica NDAS01F, Bacillus licheniformis NDSA24R, and Priestia flexa NDAS28R) and thiourea mediated amelioration of arsenic stress and growth improvement of Oryza sativa L. Plant Physiol. Biochem. 195, 14–24. doi: 10.1016/j.plaphy.2022.12.022
Silambarasan, S., Vangnai, A. S. (2016). Biodegradation of 4-nitroaniline by plant-growth promoting Acinetobacter sp. AVLB2 and toxicological analysis of its biodegradation metabolites. J. Hazardous Materials. 302, 426–436. doi: 10.1016/j.jhazmat.2015.10.010
Singh, B. K., Liu, H. W., Trivedi, P. (2020). Eco-holobiont: A new concept to identify drivers of host-associated microorganisms. Environ. Microbiol. 22, 564–567. doi: 10.1111/1462-2920.14900
Singha, K. M., Singh, B., Pandey, P. (2021). Host specific endophytic microbiome diversity and associated functions in three varieties of scented black rice are dependent on growth stage. Sci. Rep. 11, 12259. doi: 10.1038/s41598-021-91452-4
Suckstorff, I., Berg, G. (2003). Evidence for dose-dependent effects on plant growth by Stenotrophomonas strains from different origins. J. Appl. Microbiol. 95, 656–663. doi: 10.1046/j.1365-2672.2003.02021.x
Sun, N., Gu, Y., Jiang, G., Wang, Y., Wang, P., Song, W., et al. (2022). Bacterial communities in the endophyte and rhizosphere of white radish (Raphanus sativus) in different compartments and growth conditions. Front. Microbiol. 13. doi: 10.3389/fmicb.2022.900779
Sun, A., Jiao, X., Chen, Q., Wu, A., Hu, H. (2020). Microbial communities in crop phyllosphere and root endosphere are more resistant than soil microbiota to fertilization. Soil Biol. Biochem. 153, 108113. doi: 10.1016/j.soilbio.2020.108113
Sun, L. N., Wang, X. H., Li, Y. (2016). Increased plant growth and copper uptake of host and non-host plants by metal-resistant and plant growth-promoting endophytic bacteria. Int. J. Phytoremediation. 18, 494–501. doi: 10.1080/15226514.2015.1115962
Sun, A. Q., Jiao, X. Y., Chen, Q. L., Wu, A. L., Zheng, Y., Lin, Y. X. (2021). Microbial communities in crop phyllosphere and root endosphere are more resistant than soil microbiota to fertilization. Soil Biology & Biochemistry 153, 108113. doi: 10.1016/j.soilbio.2020.108113
Sutherland, J., Bell, T., Trexler, R. V., Carlson, J. E., Lasky, J. R. (2022). Host genomic influence on bacterial composition in the switchgrass rhizosphere. Mol. Ecology. 31, 3934–3950. doi: 10.1111/mec.16549
Tsuda, K., Tsuji, G., Higashiyama, M., Ogiyama, H., Umemura, K., Mitomi, M., et al. (2016). Biological control of bacterial soft rot in Chinese cabbage by Lactobacillus plantarum strain BY under field conditions. Biol. Control. 100, 63–69. doi: 10.1016/j.biocontrol.2016.05.010
Velusamy, R., Thayumanavan, B., Sadasivam, S. (1990). Effect of steam distillate extracts of selected resistant cultivated and wild rices on behavior of leaffolder Cnaphalocrocis medinalis (Guenée) (Lepidoptera: Pyralidae). J. Chem. Ecology. 16, 2291–2296. doi: 10.1007/BF01026938
Wang, Q., Ge, C. F., Xu, S. A., Wu, Y. J., Sahito, Z. A., Ma, L. Y., et al. (2020b). The endophytic bacterium Sphingomonas SaMR12 alleviates Cd stress in oilseed rape through regulation of the GSH-AsA cycle and antioxidative enzymes. BMC Plant Biol. 20, 63. doi: 10.1186/s12870-020-2273-1
Wang, K., Li, Y., Wu, Y., Qiu, Z., Ding, Z., Wang, X., et al. (2020a). Improved grain yield and lowered arsenic accumulation in rice plants by inoculation with arsenite-oxidizing Achromobacter xylosoxidans GD03. Ecotoxicology Environ. Safety. 206, 111229. doi: 10.1016/j.ecoenv.2020.111229
Wang, Q., Ma, L. Y., Zhou, Q. Y., Chen, B., Zhang, X. C., Wu, Y. J., et al. (2019). Inoculation of plant growth promoting bacteria from hyperaccumulator facilitated non-host root development and provided promising agents for elevated phytoremediation efficiency. Chemosphere. 234, 769–776. doi: 10.1016/j.chemosphere.2019.06.132
Wani, Z. A., Ashraf, N., Mohiuddin, T., Riyaz-Ul-Hassan, S. (2015). Plant-endophyte symbiosis, an ecological perspective. Appl. Microbiol. Biotechnol. 99, 2955–2965. doi: 10.1007/s00253-015-6487-3
White, J. F., Kingsley, K. L., Zhang, Q., Verma, R., Obi, N., Dvinskikh, S., et al. (2019). Review: Endophytic microbes and their potential applications in crop management. Pest Manage. Science. 75, 2558–2565. doi: 10.1002/ps.5527
Xie, J., Wang, X. Q., Xu, J. W., Xie, H. W., Cai, Y. H., Liu, Y. Z., et al. (2021). Strategies and structure feature of the aboveground and belowground microbial community respond to drought in wild rice (Oryza longistaminata). Rice. 14, 79. doi: 10.1186/s12284-021-00522-8
Xu, S. Q., Chang, J. J., Chang, C. L., Tian, L., Li, X. J., Tian, C. J. (2022). Rhizospheric microbiomes help dongxiang common wild rice (Oryza rufipogon Griff.) rather than Leersia hexandra Swartz survive under cold stress. Arch. Agron. Soil Science. 68, 76–88. doi: 10.1080/03650340.2020.1827232
Yaghoubi, M., Strafella, S., Allegretta, I., Crecchio, C. (2021). Isolation of bacteria with potential plant-promoting traits and optimization of their growth conditions. Curr. Microbiol. 78, 1–15. doi: 10.1007/S00284-020-02303-W
Yang, R., Shi, Q., Huang, T., Yan, Y., Li, S., Fang, Y., et al. (2023). The natural pyrazolotriazine pseudoiodinine from Pseudomonas mosselii 923 inhibits plant bacterial and fungal pathogens. Nat. Commun. 14, 734. doi: 10.1038/s41467-023-36433-z
Yao, F., Hu, Q., Yu, Y., Yang, L., Jiao, S., Huang, G., et al. (2022). Regeneration pattern and genome-wide transcription profile of rhizome axillary buds after perennial rice harvest. Front. Plant Science. 13. doi: 10.3389/fpls.2022.1071038
Yin, Y., Wang, Y., Cui, H., Zhou, R., Li, L., Duan, G., et al. (2023). Distinctive structure and assembly of phyllosphere microbial communities between wild and cultivated rice. Microbiol. Spectrum. 11, e04371–e04322. doi: 10.1128/spectrum.04371-22
Yu, P., Hochholdinger, F. (2018). The role of host genetic signatures on root-microbe interactions in the rhizosphere and endosphere. Front. Plant Science. 9. doi: 10.3389/fpls.2018.01896
Zachow, C., Muller, H., Tilcher, R., Berg, G. (2014). Differences between the rhizosphere microbiome of Beta vulgaris ssp maritima-ancestor of all beet crops-and modern sugar beets. Front. Microbiol. 5. doi: 10.3389/fmicb.2014.00415
Zhang, W. L., Dong, Y., Yang, L., Ma, B. J., Ma, R. R., Huang, F. D., et al. (2014). Small brown planthopper resistance loci in wild rice (Oryza officinalis). Mol. Genet. Genomics 289, 373–382. doi: 10.1007/s00438-014-0814-8
Zhang, X., Gao, J., Cao, Y., Ma, X., He, J.-Z. (2013). Long-term rice and green manure rotation alters the endophytic bacterial communities of the rice root. Microbial Ecology. 66, 917–926. doi: 10.1007/s00248-013-0293-1
Zhang, S. L., Hu, J., Yang, C. D., Liu, H. T., Yang, F., Zhou, J. H., et al. (2017). Genotype by environment interactions for grain yield of perennial rice derivatives (Oryza sativa L./Oryza longistaminata) in southern China and Laos. Field Crops Res. 207, 62–70. doi: 10.1016/j.fcr.2017.03.007
Zhang, S., Huang, G., Zhang, Y., Lv, X., Wan, K., Liang, J., et al. (2022). Sustained productivity and agronomic potential of perennial rice. Nat. Sustainability. 6, 28–38. doi: 10.1038/s41893-022-00997-3
Zhang, J., Liu, W., Bu, J., Lin, Y., Bai, Y. (2023). Host genetics regulate the plant microbiome. Curr. Opin. Microbiol. 72, 102268. doi: 10.1016/j.mib.2023.102268
Zhang, J. Y., Liu, Y. X., Zhang, N., Hu, B., Jin, T., Xu, H. R., et al. (2019). NRT1.1B is associated with root microbiota composition and nitrogen use in field-grown rice. Nat. Biotechnol. 37, 676–684. doi: 10.1038/s41587-019-0104-4
Keywords: Oryza officinalis, endophytic bacteria, plant growth-promoting trait, perennial rice, biofertilizer
Citation: Tian Q, Gong Y, Liu S, Ji M, Tang R, Kong D, Xue Z, Wang L, Hu F, Huang L and Qin S (2023) Endophytic bacterial communities in wild rice (Oryza officinalis) and their plant growth-promoting effects on perennial rice. Front. Plant Sci. 14:1184489. doi: 10.3389/fpls.2023.1184489
Received: 11 March 2023; Accepted: 24 July 2023;
Published: 14 August 2023.
Edited by:
Sofia I.A. Pereira, Universidade Católica Portuguesa, PortugalReviewed by:
Becky Nancy Aloo, University of Eldoret, KenyaCopyright © 2023 Tian, Gong, Liu, Ji, Tang, Kong, Xue, Wang, Hu, Huang and Qin. This is an open-access article distributed under the terms of the Creative Commons Attribution License (CC BY). The use, distribution or reproduction in other forums is permitted, provided the original author(s) and the copyright owner(s) are credited and that the original publication in this journal is cited, in accordance with accepted academic practice. No use, distribution or reproduction is permitted which does not comply with these terms.
*Correspondence: Fengyi Hu, aGZlbmd5aUB5bnUuZWR1LmNu; Liyu Huang, bHlodWFuZ0B5bnUuZWR1LmNu; Shiwen Qin, c2hpd2VucWluQHludS5lZHUuY24=
†These authors have contributed equally to this work
Disclaimer: All claims expressed in this article are solely those of the authors and do not necessarily represent those of their affiliated organizations, or those of the publisher, the editors and the reviewers. Any product that may be evaluated in this article or claim that may be made by its manufacturer is not guaranteed or endorsed by the publisher.
Research integrity at Frontiers
Learn more about the work of our research integrity team to safeguard the quality of each article we publish.