- 1Biology Department, College of Science and Arts at Khulis, University of Jeddah, Jeddah, Saudi Arabia
- 2Department of Biochemistry, College of Science, University of Jeddah, Jeddah, Saudi Arabia
- 3Integrated Molecular Plant Physiology Research, Department of Biology, University of Antwerp, Antwerp, Belgium
Elevating CO2 (eCO2) levels will change behavior and the effect of soil fertilizers and nutrients. Selenium NPs (SeNPs) have arisen as an alternative to conventional Se fertilizers to enrich crops. However, it remains unclear whether eCO2 will change the biological effects of soil SeNPs on plant growth and metabolism. The current study aimed to shed new light on the interactive impacts of eCO2 and SeNPs on wheat plants. Accordingly, the attempts were to reveal whether the application of SeNPs can modulate the eCO2 effects on wheat (Triticum aestivum L.) physiological and biochemical traits. With this goal, a pot experiment was carried out where the seeds were primed with SeNPs and plants were grown under two levels of CO2 concentrations (ambient CO2 (aCO2, 410 μmol CO2 mol−1; and eCO2 (710 μmol CO2 mol−1)) during six weeks after sowing. Although SeNPs+eCO2 treatment resulted in the highest accumulation of photosynthetic pigment content in leaves (+49-118% higher than control), strong evidence of the positive impacts on Rubisco activity (~+23%), and stomatal conductance (~+37%) was observed only under eCO2, which resulted in an improvement in photosynthesis capacity (+42%). When photosynthesis parameters were stimulated with eCO2, a significant improvement in dry matter production was detected, in particular under SeNPs+eCO2 which was 1.8 times higher than control under aCO2. The highest content of antioxidant enzymes, molecules, and metabolites was also recorded in SeNPs+eCO2, which might be associated with the nearly 50% increase in sodium content in shoots at the same treatment. Taken together, this is the first research documenting the effective synergistic impacts of eCO2 and SeNPs on the mentioned metabolites, antioxidants, and some photosynthetic parameters, an advantageous consequence that was not recorded in the individual application of these treatments, at least not as broadly as with the combined treatment.
1 Introduction
Carbon dioxide (CO2) emissions are gradually increasing due to expanding industrial activities and fossil fuel use, conversion of natural ecosystems, and poor agricultural and forestry management, from 280 μmol CO2 mol–1 in pre-industrial times to the current 416 μmol CO2 mol–1 and more than 700 μmol CO2 mol–1 by the end of this century (Fang et al., 2022; Shabbaj et al., 2022). This CO2 rise is the primary cause of climate change and global warming, resulting in increasing severity and iteration of climate-inordinate phenomena such as flooding, environmental stress (e.g., salinity, drought, high temperature, etc.), forest fires, heavy rain, and heat waves (AbdElgawad et al., 2014). As a result, elevated CO2 (eCO2) is increasingly recognized as a main factor affecting global crop production, because an increase in primary carbon sources influences crop growth, biomass production, the photosynthesis cycle, and metabolite profiling differently and significantly, even in non-stressed plants (Jing et al., 2016; Kaur et al., 2023). In recent years, there has been an increasing amount of literature on the responses of wheat plants to elevated atmospheric CO2 through improving photosynthesis capacity, declining transpiration and water use, and resulting in a productive advantage and higher grain yield (Ainsworth and Rogers, 2007). Accordingly, previous research has reported analyses of field experiments on wheat in different growing areas that demonstrated the increment in grain yield and yield components at high CO2 levels under non-environmental stress conditions, suggesting more boost in wheat grain number per unit surface area than the increase in grain weight (Wang et al., 2013; Broberg et al., 2019; Blandino et al., 2020). Likewise, data from several sources have identified the increased wheat productive response to elevated CO2 is associated with physiological mechanisms (Wang et al., 2013; Dubey et al., 2015), especially synthesizing bioactive compounds and antioxidant metabolites in non-stressed wheat plants (Blandino et al., 2020). Sreeharsha et al. (2019) considered the carbon supply under eCO2 as a factor for better plant growth because of its affecting sink capacity and mediating better N assimilation. Improved photosynthesis capacity and photosystem-II efficiency under eCO2 conditions have also reported through increasing chlorophyll a fluorescence characteristics (Sekhar et al., 2015).
Selenium (Se) deficiency, as an essential trace element for human health, is one of the foremost concerns in the rising risks of numerous illnesses and cancers (Liu et al., 2021). The nutritional deficit in staple foods is typically caused by plant production in mineral -poor soils, resulting in nearly two billion people worldwide suffering from nutritional deficiencies (Lanza and dos Reis, 2021). Agronomic biofortification is an agricultural technique employed to enrich crops with target nutrients, such as selenium, to ultimately improve crop-based food quality (Reis et al., 2017). Since the high mobility and availability of Se conventional chemical fertilizers have become a major concern in environmental pollution (Rosenfeld et al., 2017), there has been a significant increase in the potential applications of nanotechnology in Se supplements with significantly higher efficiency and lower toxicity (Liu et al., 2021). Selenium nanoparticles (SeNPs) have recently gained prominence due to their beneficial effects on plant growth, photosynthesis, and metabolite biosynthesis, as well as their association with plant oxidative stress tolerance (Lanza and dos Reis, 2021). Application of SeNPs as foliar (Ikram et al., 2020), seed priming (Nawaz et al., 2013), soil treated (Lyu et al., 2022) and under hydroponic conditions (Hu et al., 2018) resulted in improved nutritional values and physiological traits of wheat plants under both stress and non-stress conditions. Moreover, it has already been reported that eCO2 can increase the allocation of photosynthates to the roots (Habeeb et al., 2020). Also, it has been suggested that root exudates and CO2 produced via root respiration can acidify the rhizosphere, consequently making some nutrients more available to plants (Duval et al., 2012). Thus, eCO2 could change the behaviour of soil nutrients and thus modify their availability and biological impact on plants (Du et al., 2017). Similarly, eCO2 improved the potential for roots to uptake more nutrients such as Se, from the soil (Du et al., 2023), which consequently led to further growth and development in plants under eCO2.
SeNPs have arisen as an alternative to conventional Se fertilizers to enrich crops.
Although much research has been carried out on eCO2 and NPs, it remains unclear whether eCO2 will change the biological effects of soil SeNPs on plant growth and metabolism. It has already been reported that diverse metabolic processes can be modified under eCO2 (Mishra et al., 2013; AbdElgawad et al., 2016; Du et al., 2023). In Despite the research summarized above, the present study aimed to determinehow eCO2 can affect the biological impact of SeNPs on the biochemical and physiological shifts in eCO2-treated plants. We hypothesized that the application of SeNPs could synergistically and positively affect plant growth, photosynthesis parameters, and biochemistry in eCO2-treated wheat plants, as compared to those in control plants grown under ambient CO2 conditions.
2 Materials and methods
2.1 Experimental setup
Eight healthy wheat seeds (var. Giza 119) were sterilized by drenching in sodium hypochlorite solution (1% v/v) for 10 min and were planted in potting mix (Tref EGO substrates, Moerdijk, The Netherlands) in pots (15 cm diameter × 15 cm height) filled with a mixture (1:1, v/v) of loamy soil and organic compost at a humidity of 300 mg water g–1 dry soil. Plants were grown for six weeks after planting at controlled-environment reach-in growth chambers with a constant regime of 20°C air temperature, 14/10 h day/night photoperiod, 150 μmol m−2 s−1 photosynthetically active radiation until the tillering stage and then 550 μmol m−2 s−1 until jointing stage, and ~65% soil water content.
Three replications and a fully randomized design were used in the experiment, which contained four treatments, including two levels of selenium nanoparticles (SeNPs and control), and two levels of air CO2 concentrations (ambient CO2 (aCO2, 410 μmol CO2 mol−1; and elevated CO2 (eCO2, 710 μmol CO2 mol−1)). To apply the SeNPs treatment, the prepared seeds were treated in a suspension with 25 mg L-1 of SeNPs (purity of 99.99%; density of 4.79 g cm−3; American Elements, Los Angeles, CA, USA) at room temperature for 10 h in an ongoing shaking (IKA KS 501 shaker, Staufen, Germany), and washed thrice with distilled water. Soils were also mixed with 200 mL of 25 mg L-1 of SeNPs, however the control soils were mixed with 200 mL of distilled water. The rough aggregates in the aqueous suspension of SeNPs were sidestepped by sonication (Selim et al., 2022). The morphological characteristics of the SeNPs structure were also validated using a scanning electron microscope (SEM manufactured by JEOL JSM-6510, LA, Japan). The applied dose of Se-NPs was recommended in the previous experiments examining the effect of various concentrations of SeNPs (0, 10, 25, 50, and 75 mg L−1) on plants (Albqmi et al., 2023). SeNPs concentration was chosen based on the results of preliminary assay, in which the impact of target SeNPs dose induce 25% induction in plant dry weight. The CO2 was also emitted into the airflow of the chamber and was maintained at the target CO2 level using a CO2 analyzer (WMA-4, PP Systems, Hitchin, UK). Seeds used for control treatments (aCO2 and eCO2) were treated with distilled water for 10 h.
2.2 Sampling
2.2.1 Plant biomass
Plant shoot tissues were harvested six weeks after planting, and a part of them was used to measure the plant’s fresh and dry matter, and the rest for following physiological and biochemical analysis.
2.2.2 Determination of photosynthesis parameters
Chlorophyll and carotenoids in the leaves were measured spectrophotometrically based on the modified Porra (Porra, 2002) technique by reading the absorbance of extracted samples at the wavelengths of 665.2, 652.4 and 470 nm using a microplate reader (Synergy Mx, Biotek Instruments Inc., Vermont, VT, USA) (Yaghoubi Khanghahi et al., 2019). High-performance liquid chromatography (HPLC; Shimadzu, Hertogenbosch, The Netherlands) was used to measure β-carotene, zeaxanthin (Gilmore and Yamamoto, 1991), and lutein (Fratianni et al., 2015) in leaves, as fully described by previous studies.
The photosynthesis rate (PN) and stomatal conductance (gs) of the last developed leaves were measured using the LI-COR portable photosynthesis system (LI-COR 6400/XT, USA). The maximum efficiency of photosystem II in dark-adapted leaves (Fv/Fm) was also determined using a Pulse Amplitude Modulated fluorometer (PAM–2500, Walz, Germany), in which Fm and Fv are the maximum fluorescence and the variable fluorescence, respectively, as fully described by Genty et al. (1989). Briefly, the leaves were acclimated to the dark for 15 min using the dark leaf clips (DLC-8) to measure the basal and maximum fluorescence in response to low-intensity light (<0.1 μmol photons m−2 s−1, red light) and a saturating light pulse (>8,000 μmol photons m−2 s−1, white light) was turned on for 1 s (Yaghoubi Khanghahi et al., 2019).
Ribulose-1,5-biphosphate carboxylase: oxygenase (Rubisco) activity of leaves was determined spectrophotometrically based on the oxidation of nicotinamide adenine dinucleotide (NADH) at 340 nm (Lobo et al., 2015). Briefly, Rubisco activity was measured in as assay reaction mixture containing bicine (100 mM; pH 8.0), KHCO3 (25 mM), MgCl2 (20 mM), ATP (3.5 mM), phosphocreatine (5 mM), glyceraldehyde 3-phosphate dehydrogenase (80 nkat), 3-phosphoglyceric phosphokinase (80 nkat), creatine phosphokinase (80 nkat), and NADH (0.25 mM). The extract was incubated at 25 °C in the assay buffer for 15 min to complete the activation of Rubisco in the assay solution (Lobo et al., 2015).
2.2.3 Determination of mineral content in plant and soil
Total-reflection X-ray fluorescence spectrometry (TXRF; Bruker Nano GmbH, Berlin, Germany) and an ion chromatograph (Shimadzu, Japan) were used to measure the concentration of selenium (Se), calcium (Ca), phosphorus (P), sodium (Na), potassium (K), magnesium (Mg), zinc (Zn) and sulfur (S) in plants and Se in soil (Yoshida et al., 2016; Bamrah et al., 2019).
2.2.4 Assessment of antioxidant metabolites and enzymes
Samples were homogenized in 1 ml of buffer [50 mM potassium phosphate, pH 7.0, 10% (w/v) polyvinyl pyrrolidone (PVP), 0.25% (v/v) Triton X-100, 1 mM phenylmethylsulfonyl fluoride (PMSF), 1 mM ASC] and centrifuged to get a clear supernatant for measuring the activity of the antioxidant enzymes. Accordingly, superoxide dismutase (SOD) was measured based on the inhibition of nitroblue tetrazolium (NBT) reduction at 560 nm (Dhindsa et al., 1982). Peroxidase (POX) activity was determined according to the pyrogallol oxidation, as fully described by Kumar and Khan (1982). Catalase activity was assessed based on the breakdown of H2O2 at 240 nm (Aebi, 1984). The estimation of ascorbate peroxidase (APX) and glutathione reductase (GR) activities was fully explained by Murshed et al. (2008). Glutathione peroxidase (GPX) activity was determined based on the reduction in NADPH absorption at 340 nm (Drotar et al., 1985). Reduced ascorbate (ASC) content was assessed based on the method of Foyer et al. (1983).
Total antioxidant capacity (TAC) was measured through the ferric-reducing antioxidant power (FRAP) method by employing Trolox as a reference base (Selim et al., 2022). Briefly, the samples were extracted in ethanol (80% v/v) and centrifuged (at 14,000× g for 20 min at 4°C). The FRAP reagent was prepared by adding FeCl3 (20 mM) to the acetate buffer (0.25 M). The FRAP reagent (FeCl3 (20 mM) and acetate buffer (0.25 M)) was mixed with extracts, and the reading was measured at 593 nm. The quantification of flavonoids and polyphenols content was carried out using aluminum chloride calorimetric and Folin–Ciocalteu assays, based on the method of AbdElgawad et al. (2020) and Zhang et al. (2006), respectively. Tocopherol content was measured using HPLC, in which Dimethyl tocol (DMT; 5 ppm) was used as an internal standard, as fully described by AbdElgawad et al. (2015). The quantification of organic acids in soil extracted from the rhizosphere of wheat plants was done using HPLC (Lawongsa et al., 1987).
2.3 Statistical analysis
The SigmaPlot software package was used to perform all statistical calculations, including a two-way analysis of variance (ANOVA), the Tukey’s HSD (honestly significant difference) test, Pearson correlation, and graph creation. The results were expressed as the mean ± standard deviation. The significance level (α) for Tukey test and correlation analysis was set at 0.05.
3 Results
3.1 Photosynthetic parameters
The results of some photosynthetic parameters are presented in Figure 1. Accordingly, the highest concentrations of Chl a, Chl b, β-carotene, lutein, and zeaxanthin belonged to the selenium nanoparticle treatment in eCO2-treated plants (SeNPs+eCO2), which were about 13–41% and 49–118% higher than those in control plants (P < 0.05) under eCO2 and aCO2 conditions, respectively (Figure 1A). The improvement of the accumulation of pigments in response to the treatments led us to investigate their effect on some of the most important photosynthetic parameters of the plant, as shown in Figure 1B. Interestingly, for those treated with SeNPs, no changes were found in photosynthesis rate (PN), Rubisco activity, stomatal conductance (gs) and the maximum quantum yield of photosystem II (Fv/Fm) as compared to control plants under both eCO2 and aCO2 conditions (Figure 1B). Moreover, although, strong evidence of the positive impacts of elevated CO2 on PN, gs, and Rubisco activity were observed (P < 0.05), Fv/Fm was not obviously affected by the treatments (P > 0.05).
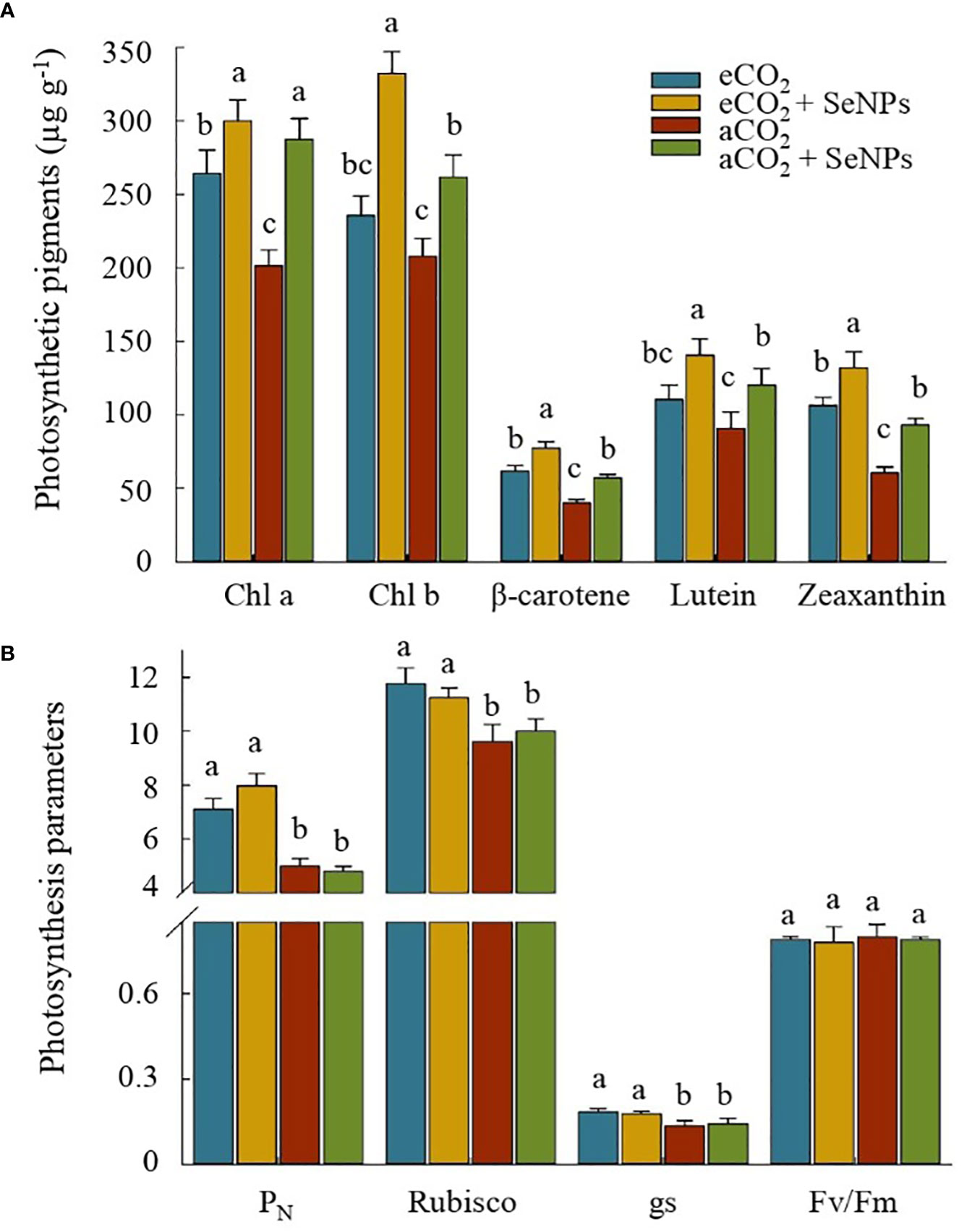
Figure 1 The effect of selenium nanoparticles (SeNPs) on the photosynthetic pigments (A) and photosynthesis parameters (µmol CO2 m-2 s-1 for PN. mmol CO2 m-2 s-1 for gs, and mg g-1 protein for Rubisco) (B) in plants under ambient CO2 (aCO2) and elevated CO2 (eCO2). Means in each parameter followed by similar letter(s) are not significantly different at 5% probability level (Tukey test). PN, Photosynthesis rate; gs, Stomatal conductance; Chl a, Chlorophyll a; Chl b, Chlorophyll b; Fv/Fm, maximum efficiency of PSII photochemistry in dark-adapted leaves.
3.2 Antioxidant enzymes and molecules
The potential of modifications in the antioxidant defense system was assessed by measuring the concentration of antioxidant enzymes and metabolites in plants in response to the treatments. The highest activity of POX, CAT, and SOD enzymes was found in the combined treatment (SeNPs+eCO2), in which the activity of these antioxidant direct-scavenging enzymes significantly (P < 0.05) increased up to 103-131% and 117-247% higher than the control plants under eCO2 and aCO2 conditions, respectively (Figure 2). Similarly, the individual applications of SeNPs and eCO2 treatments did not affect the biochemical components involved in the ascorbate-glutathione (ASC/GSH) pathways (P > 0.05). Accordingly, only the combined treatment (SeNPs+eCO2) caused a significant (P < 0.05) boost in the content of the antioxidant enzymes and metabolites, including ASC, GSH, APX, DHAR, MDHAR, GR, and GPX, by 90–190% higher than eCO2 and 105–298% greater than the aCO2 treatment (Figure 2).
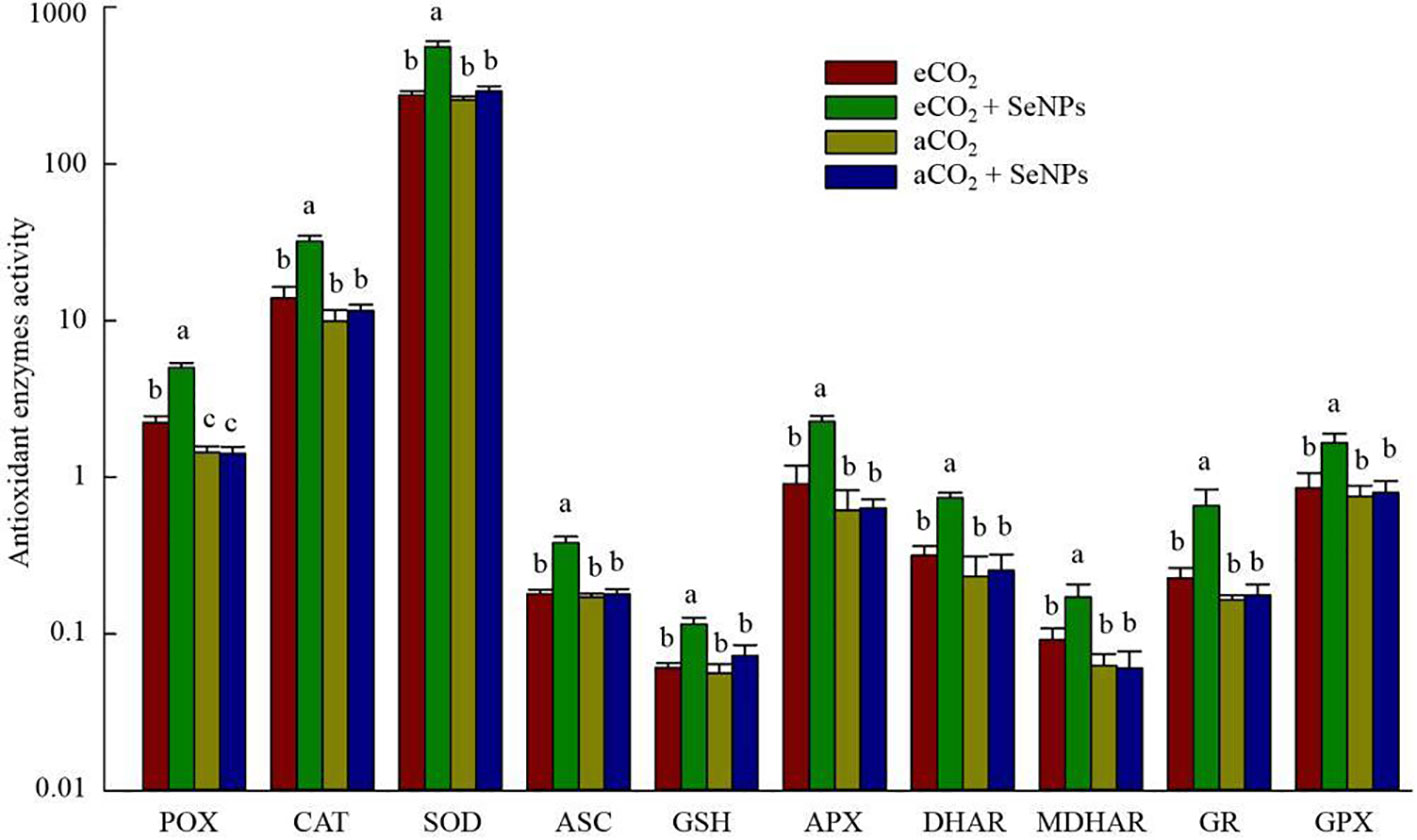
Figure 2 The effect of selenium nanoparticles (SeNPs) on the antioxidant enzymes (μmol min-1 mg-1 protein) and metabolites (µmol g-1 FW) in plants under ambient CO2 (aCO2) and elevated CO2 (eCO2). Means in each parameter followed by similar letter(s) are not significantly different at 5% probability level (Tukey test). GR, Glutathione reductase; GPX, Glutathione peroxidase; POX, Proxidase; CAT, Catalase; SOD, superoxide dismutase; DHAR, Dehydroascorbate reductase; MDHAR, Monodehydroascorbate reductase; ASC, Ascorbate; APX, Ascorbate peroxidase; GSH, Glutathione.
The beneficence of SeNPs+eCO2 treatment in improving the content of total antioxidant capacity, polyphenols, flavonoids, and total tocopherols (including α-, β-, γ-, and σ-tocopherols) in plants was recorded as equal to 171.3 µmol Trolox g–1, 7.6 µg g–1, 3.5 µg g–1, and 8.4 ng g–1, which were about 83, 122, 110, and 102% higher than those in control eCO2-treated plants (Figure 3). Similarly, the highest concentrations of polyphenols (92.5 mg g–1) and organic acids (191.3 mM) in soil were recorded in SeNPs+eCO2 treatment, which were significantly higher than other treatments (P < 0.05) (Figure 4).
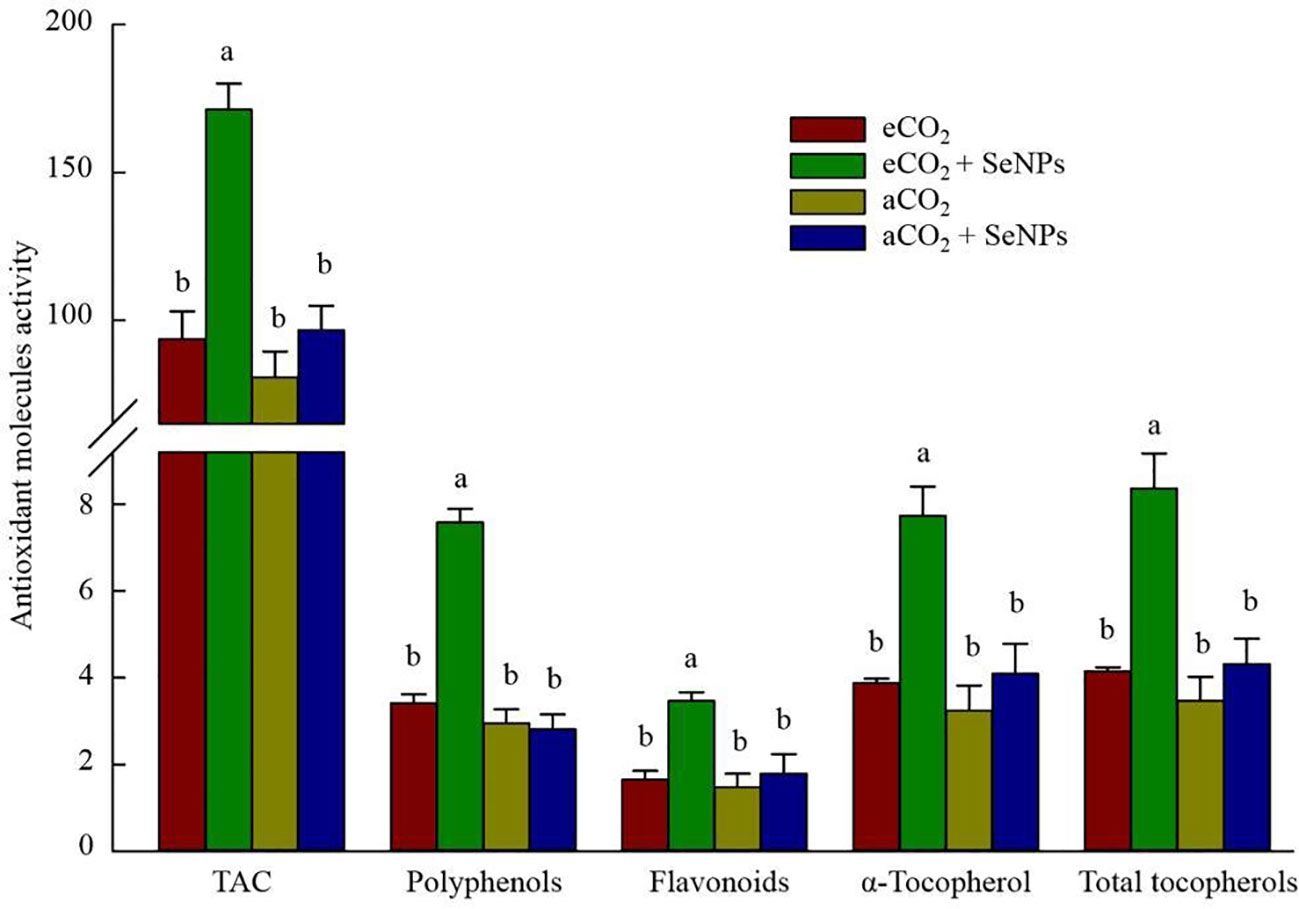
Figure 3 The effect of selenium nanoparticles (SeNPs) on the antioxidant molecules (µmol Torolex g-1 FW for TAC; mg GAE g-1 FW for polyphenols; mg Quercetin g-1 FW for flavonoids; ng g-1 for tocopherols) in plants under ambient CO2 (aCO2) and elevated CO2 (eCO2). Means in each parameter followed by similar letter(s) are not significantly different at 5% probability level (Tukey test). TAC, Total antioxidant capacity.
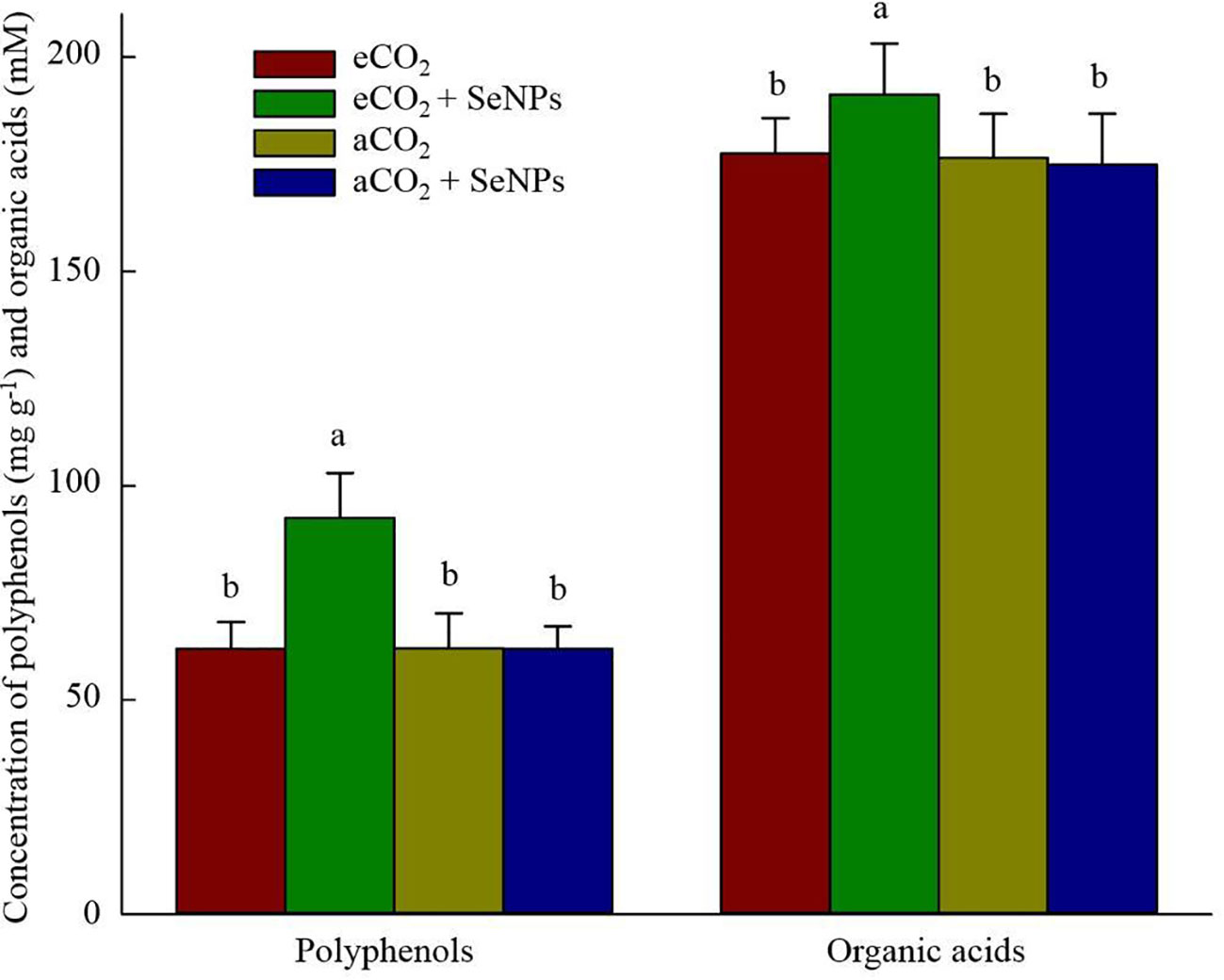
Figure 4 The effect of selenium nanoparticles (SeNPs) on the concentration of organic acids and polyphenols in soil under ambient CO2 (aCO2) and elevated CO2 (eCO2). Means in each parameter followed by similar letter(s) are not significantly different at 5% probability level (Tukey test).
3.3 Nutrient concentrations in plant
The nutrient status of the shoot samples was determined in order to assess the effectiveness of the selenium nanoparticle treatment and its interaction with CO2 levels. Accordingly, the concentration of sulfur, potassium, magnesium, calcium, and zinc in shoots was positively affected under elevated CO2 treatment, which was more pronounced when SeNPs was applied (+ 34-53%) compared to control eCO2-treated plants (Figure 5). The results were slightly different for phosphorus and sodium contents which were significantly higher only under the combined treatment (SeNPs+eCO2) as compared to other treatments, equal to +47-70% and +51-88%, respectively (Figure 5). Interestingly, the individual application of SeNPs did not cause a significant increase (P > 0.05) in the concentration of the studied nutrient in plants (Figure 5).
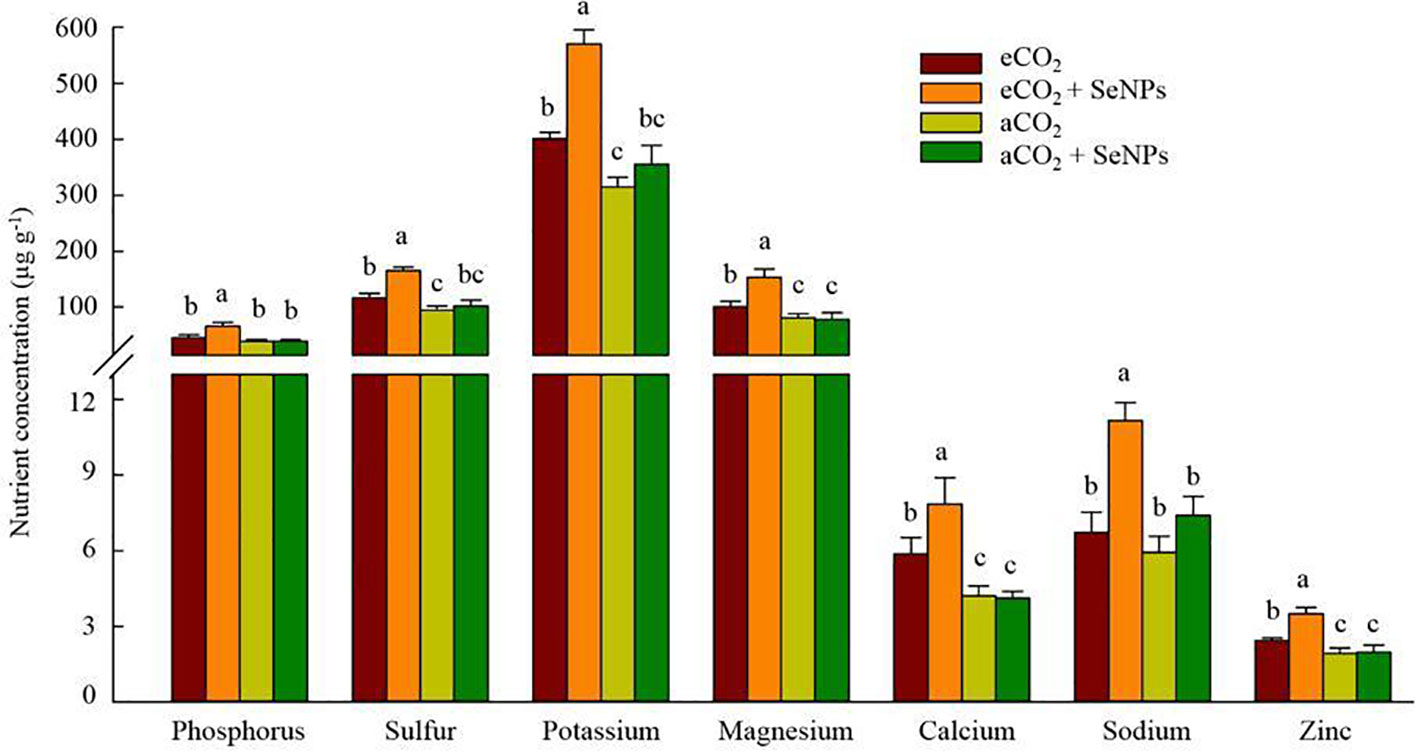
Figure 5 The effect of selenium nanoparticles (SeNPs) on the nutrient concentrations in plant under ambient CO2 (aCO2) and elevated CO2 (eCO2). Means in each parameter followed by similar letter(s) are not significantly different at 5% probability level (Tukey test).
3.4 Biomass production and selenium content in plant and soil
Although elevated CO2 did not affect the accumulation of selenium in soil and plant shoot tissues, the application of of SeNPs significantly enriched the concentration of Se in soil and plants (Figure 6A). This remarkable increment in soils treated by SeNPs under eCO2 and aCO2 conditions were about 4318 and 1783 times higher than that of the control plants at the same CO2 level, respectively (Figure 6A). The increase in soil Se content resulted in a significant enhancement in the Se accumulation in the plant (P < 0.05), which in SeNPs+eCO2 and SeNPs+aCO2 were 21 and 19 times more concentrated than eCO2 and eCO2 conditions, respectively (Figure 6A). A higher fresh weight belonged to the SeNPs-containing treatments, including SeNPs+eCO2 and SeNPs+aCO2 which were 20% and 72% higher than the eCO2 and aCO2 treatments, respectively (Figure 6B). This is while the highest dry weight was recorded from eCO2-containing treatments, SeNPs+eCO2 (2.15 g plant–1) and eCO2 (1.78 g plant–1), which were about +80% and +49% higher than that in control plants under aCO2 treatment, respectively (Figure 6B).
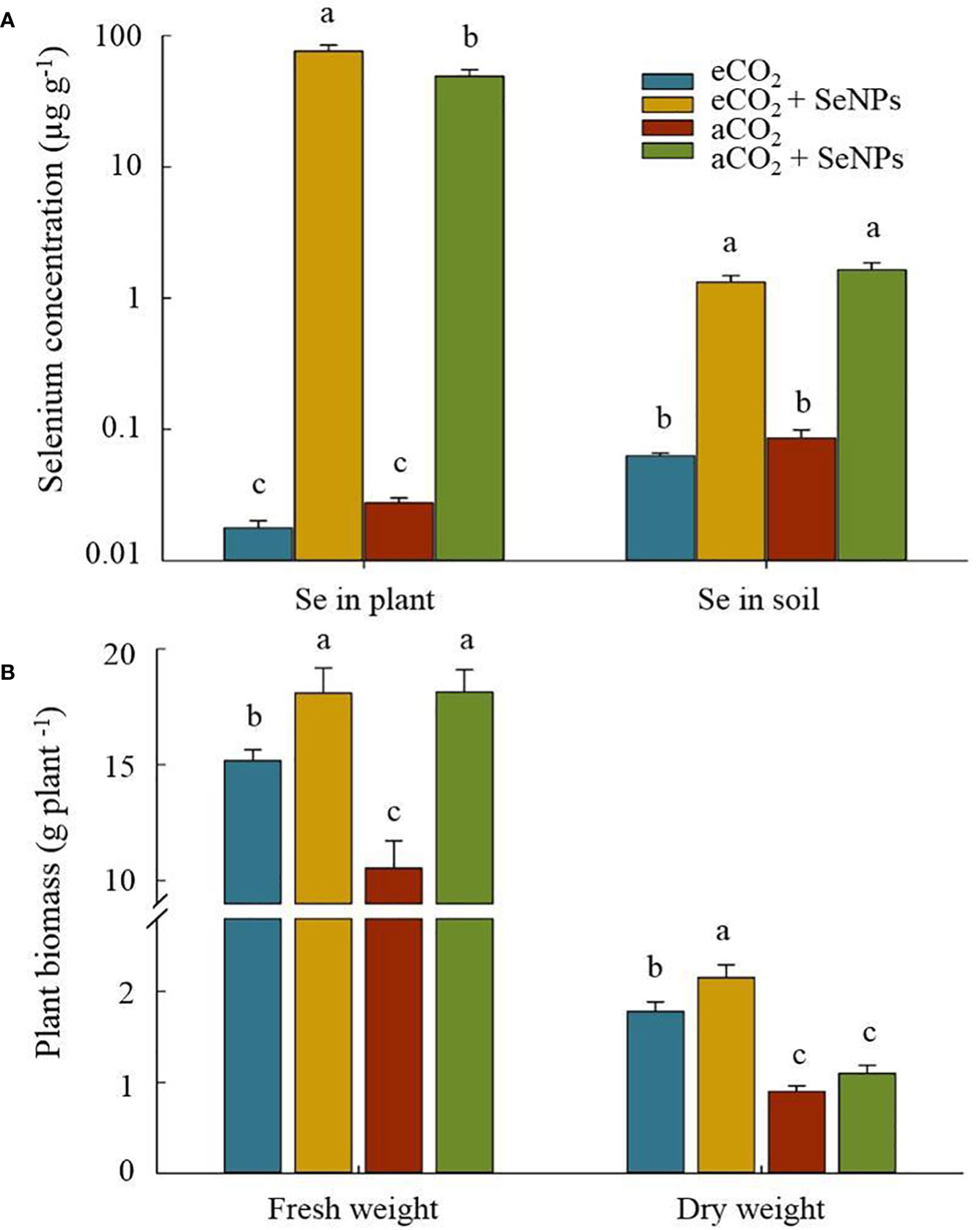
Figure 6 The effect of selenium nanoparticles (SeNPs) on the selenium concentration (A) and plant biomass (B) under ambient CO2 (aCO2) and elevated CO2 (eCO2). Means in each parameter followed by similar letter(s) are not significantly different at 5% probability level (Tukey test).
3.5 Correlation analysis
Figure 7 provides the Pearson correlation analysis relationship between plant biomass, selenium concentration in plants and some key studied traits. In this regard, plant dry weight had a positive correlation with Chl a+b (r = 0.62; P < 0.05), PN (r = 0.94; P < 0.01), gs (r = 0.89; P < 0.01), TAC (r = 0.75; P < 0.01), polyphenols (r = 0.81; P < 0.01), flavonoids (r = 0.71; P < 0.01) and total tocopherols (r = 0.81; P < 0.01). In contrast, there was no significant relationship between plant dry weight and Se concentration in soil (r = 0.17; P > 0.05). Also, we didn’t find a correlation between Se concentration in shoot tissues and photosynthesis parameters, including PN and gs (P > 0.05). Nevertheless, the Se concentration in the plant not only was correlated to soil Se content (r = 0.87; P < 0.01), but also had a positive relationship to Chl a+b (r = 0.74; P < 0.05), TAC (r = 0.80; P < 0.01), polyphenols (r = 0.73; P < 0.01), flavonoids (r = 0.80; P < 0.01) and total tocopherols (r = 0.79; P < 0.01) (Figure 7).
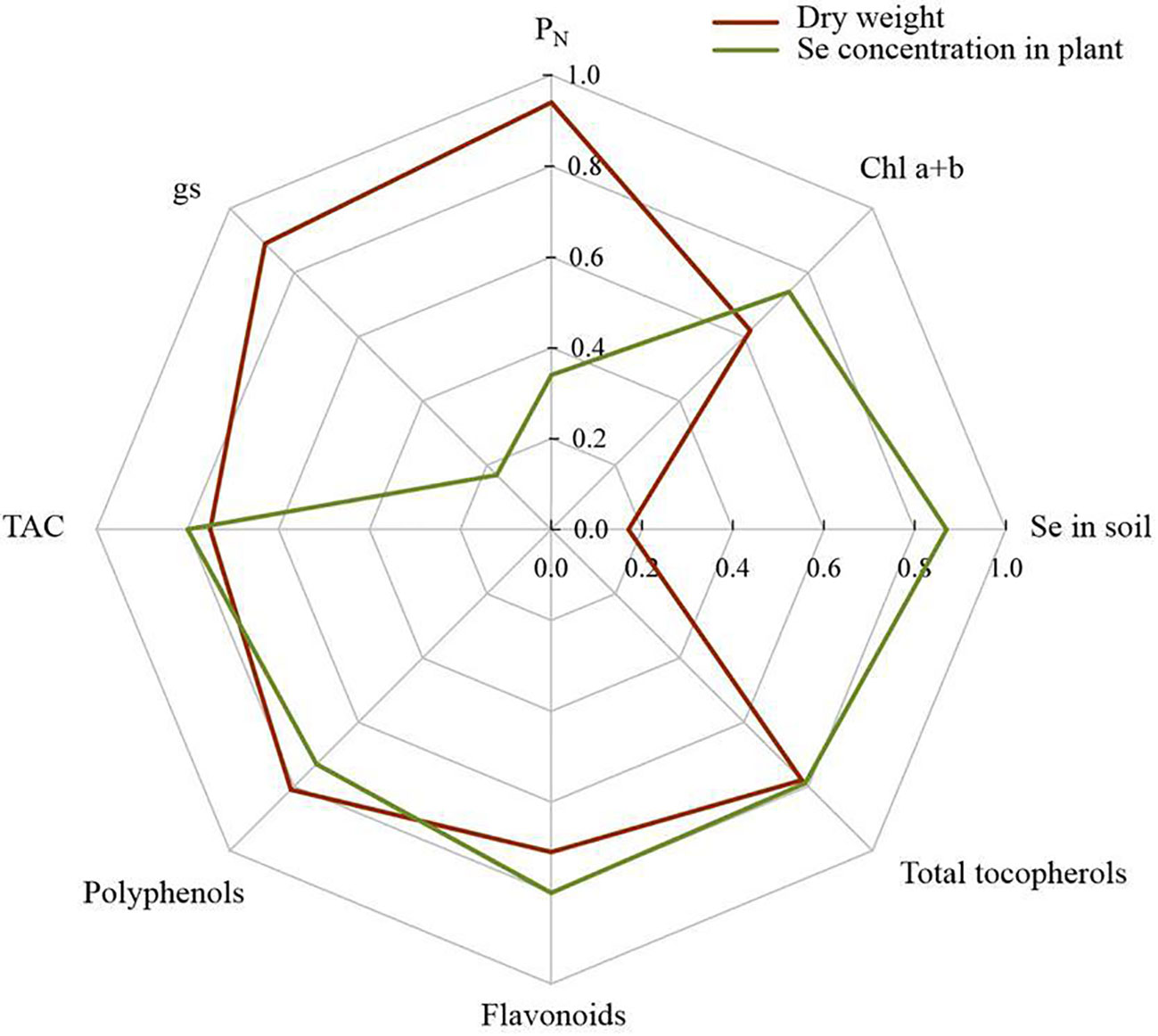
Figure 7 Pearson’s pair-wise correlation coefficients between plant dry matter and selenium content in pant and some other studied variables in this study.
4 Discussion
The present study attempted to unravel some of the unanswered questions surrounding the impacts of climate change on crops, one of which is the increase in the atmospheric CO2 level. Accordingly, the wheat plant’s responses to the application of selenium nanoparticles were investigated to show whether they can act synergistically and more effectively with elevated CO2 on some of the plant’s physiological and biochemical characteristics. eCO2 was already found to be influencing wheat physiological and agronomic traits and has been explored in several studies as a factor enhancing wheat biomass and improving net CO2 assimilation rate (Broberg et al., 2019; Wang and Liu, 2021). Moreover, agronomic factors (e.g. nutrient supply) were suggested to modulate the eCO2 effects on wheat responses (Wang and Liu, 2021). Therefore, SeNPs treatment in the present research can act as a factor in optimizing growth conditions to maximize the eCO2-induced benefit in crop production systems.
In order to clarify the mechanisms employed by plants, we first discussed the reactions of photosynthetic pigments and parameters. As shown in Figure 1, both SeNPs and eCO2 increased the concentrations of Chl a, Chl b, -carotene, lutein, and zeaxanthin, with the combined treatment (SeNPs+eCO2) having a greater effect. Although no single study exists that investigates the photosynthesis process in response to SeNPs in eCO2-treated plants, extensive research has been carried out on the effects of the individual application of eCO2 or selenium biofortification on crops. In this regard, it has already been reported that the protection of chlorophyll structure and function from disruption and degradation was due to the rising concentration of photosynthetic pigments induced by the application of SeNPs (Lanza and dos Reis, 2021; Albqmi et al., 2023) or eCO2 conditions (Habeeb et al., 2020) under both stress and non-stress conditions. These findings are relevant to reveal plant status under eCO2 once the photochemical reactions are saturated and disperse the excess excitation energy deactivating singlet molecular oxygen (Approbato et al., 2023). eCO2 can stimulate a boost in pigment content, mirroring the improved size of the PSII light-harvesting complex by transferring excitation energy favoring the PSII activity (Wang et al., 2015).
Similarly, Silva et al. (2020) considered the improvement of chlorophyll content as one of the main factors for better regulation of the antenna complex, which is associated with an increase in carotenoid content. Lanza and dos Reis (2021) proved that the biosynthesis of such metabolites (e.g., carotenoids, xanthophylls (lutein and zeaxanthin), and β-carotene) in SeNPs-treated plants can improve the energy efficiency of photosynthesis for the better functioning of the photosynthetic system. Since one of the effects of elevated CO2 is a deficiency in supplied energy from the light reactions centers, which leads to a decrease in ribulose-1,5-bisphosphate carboxylase/oxygenase (Rubisco) enzyme activity (Jensen, 2000), the possible improvement in the energy efficiency of photosynthesis in SeNPs-treated plants can be one of the explanations for the increase in Rubisco activity at least under eCO2 conditions (Figure 1B). Nevertheless, improvements in photosynthetic pigments induced by SeNPs could not result in further improvements in the photochemistry of photosynthesis under ambient CO2 conditions, despite the obvious amelioration that was recorded for photosynthesis rate (PN) and stomatal conductance (gs) in eCO2-treated plants (Figure 1B). These results showed that the boost in photosynthetic capacity in eCO2-treated plants in our research was more related to the enhancement of Rubisco enzyme activity than to photosynthetic pigment content, especially since Rubisco activation is the principal limitation to CO2 fixation during photosynthesis under elevated CO2 conditions (Jensen, 2000). Also, it has already been reported that increases in the stomatal conductance in plants are associated with more activation of Rubisco, as one of the non-stomatal and active parameters in the Calvin cycle (Acosta-Motos et al., 2017; Yaghoubi Khanghahi et al., 2021) which in turn is one of the reasons for improving the photosynthetic capacity under eCO2 in the present study. On the other hand, Fv/Fm ratios in the current research were 0.79-0.80 and were not affected by the studied treatments (Figure 1B). It has previously been proven that this parameter, as the expression of the maximum efficiency of PSII in dark-adapted leaves, usually ranges from 0.79 to 0.85 under non-stress conditions (Yaghoubi Khanghahi et al., 2019). Similar to our results, recent evidence suggests that the Fv/Fm ratio is less sensitive to non-stress conditions or even mild environmental stress and can remain unchanged (Yaghoubi Khanghahi et al., 2021).
Similar to photosynthetic, the combined treatment (SeNPs + eCO2) had the highest content of antioxidant enzymes and metabolites (Figures 2, 3), which are known for their involvement in direct or indirect reactive oxygen species (ROS) scavenging (Albqmi et al., 2023). In accordance with the present results (except for POX), previous studies have demonstrated no significant increase in antioxidant enzymes and metabolites in control plants under eCO2 compared to aCO2 conditions (Mishra et al., 2013; AbdElgawad et al., 2016) and linked it to the lack of a significant increase in ROS content (AbdElgawad et al., 2016). Although the stress biomarkers that could confirm oxidative stress were not investigated in the current study, it seems that there was no increase in the ROS content in eCO2-treated control plants. If we accept this explanation, then the sharp increase in the content of antioxidant enzymes (POX, CAT, SOD, APX, DHAR, MDHAR, GR, and GPX) and metabolites (ASC, GSH, TAC, polyphenols, flavonoids, and tocopherols) in SeNPs-treated plants under eCO2 conditions (Figures 2, 3) should be interpreted with caution. It is difficult to explain this result, but it might be related to nearly 50% increase in sodium concentration in the combined (SeNPs+eCO2) treatment compared to other treatments (Figure 5). So, this response may explain the relatively greater content of antioxidant components in plants treated by SeNPs under eCO2. The increase in Na concentration in shoots did not have an antagonistic relationship with the uptake of ions K+ and Ca2+ (Yu and Assmann, 2016), because the concentration of K and Ca was also increased in SeNPs-treated plants under eCO2 (Figure 5); therefore it seemingly independently caused oxidative stress in these plants (Lanza and dos Reis, 2021). It has already been proposed that Se can individually enter the root system via passive diffusion (through the aquaporin membrane channels) to be rapidly assimilated as selenite and/or organic Se, followed by transportation to the shoot (Lyu et al., 2022), and consequently, Se in the plant has been suggested to have dual impacts on the nutrient uptake by plants, not merely inhibitory impacts (Gui et al., 2022). It was suggested that Se ions can also change the permeability coefficients of some ions (e.g. Na+) in biomembranes and influencing the transportation of those ions via the membrane (Kaur et al., 2014; Bano et al., 2021). Therefore, a 50% increase in Na concentration in SeNPs+eCO2 treatment may be explained by the fact that it is the quantitative content of Se and essential nutrients in plants that can specify the content of Na and the interaction between Se and Na in plants, resulting in antagonistic and/or synergistic effects (Bano et al., 2021).
Elkelish et al. (2019) considered the stimulation of such enzymatic and non-enzymatic antioxidant activity in plant cells as a mechanism for transporting Na+ to the vacuoles to prevent the adverse effects of excess Na-induced damage. Lanza and dos Reis (2021) and Habibi (2017) also reported the positive effects of Se through compartmentalizing Na+ in vacuoles and increasing the bond with the cell wall in high Na-containing plants. Better nutrient absorption (Figure 5) and higher biomass production by the plants in SeNPs+eCO2 treatment (Figure 6B) can indicate the high efficiency of the plant’s defense system, which indeed prevents the disruption of the plants’ mineral balance and various physiological processes under high Na content (Bano et al., 2021). Moreover, the higher uptake of minerals (Figure 5) and dry matter production (Figure 6B) in SeNPs+eCO2 treatment in our study mirror those of the previous research, which reported an improved photosynthesis rate and increased photosynthates allocation to the roots in response to eCO2 and SeNPs (Habeeb et al., 2020; Albqmi et al., 2023), which in turn, resulted in strengthening the capacity of roots to uptake more nutrients from the soil and produce biomass (Albqmi et al., 2023; Du et al., 2023).
There was a clear increase in the accumulation of selenium in rhizospheric soil and plant shoot tissues in response to SeNPs application, a result that was not recorded in eCO2-treated plants (Figure 6A). Nonetheless, the results of the correlational analysis didn’t show a significant correlation between Se concentration and dry matter production in the plant (Figure 7), one of the reasons for which might be the lack of significant effect of SeNPs on the photochemical parameters of the photosynthesis process, such as Rubisco activity, stomatal conductance and photosynthetic capacity (Figure 1B). Consequently, elevated CO2 could be a principal factor, if not the only one, causing the positive changes in photosynthetic sink-source balance in plants by overcoming one of the main limitations of photosynthesis (Jensen, 2000), which in turn yielded a contribution to plant growth and biomass production (Yaghoubi Khanghahi et al., 2021).
The current study is based on a recommended dose of 25 mg L–1 SeNPs (Albqmi et al., 2023) and the consequent Se content in plant tissues (49-76 µg g–1) and rhizospheric soil (1.3-1.6 µg g–1) in SeNPs-treated plants was in the normal range that was previously reported (0.005-5500 µg g–1 for plants and 0.02-100 µg g–1 for soil) to be safe for plants and humans (Gupta and Gupta, 2000; Lanza and dos Reis, 2021). Nevertheless, considering the intense increase in Se content in rhizospheric soil (19-21 times higher than control) and plant (1783-4318 times higher than control), our results may not be applicable to the long-term application of SeNPs, especially under elevated CO2. In other words, an important unanswered question that arises here is whether the application of SeNPs in the long term can break the border of toxic and beneficial effects of Se on plants, especially in the soil that will face the gradual accumulation of Se over time. Moreover, although, there has been considerable discussion about the low risks of the SeNPs in the agricultural section so far (Morales-Espinoza et al., 2019; Lanza and dos Reis, 2021), it seems that caution should be applied to extrapolate those previous findings to plants grown under elevated CO2 conditions since the Na was significantly absorbed by SeNPs-treated plants in our study. So, it seems that further research is needed to propose optimal doses of SeNPs, in particular under elevated CO2 conditions.
5 Conclusion
The obtained results showed that the synergistic effects of selenium nanoparticles and elevated CO2 conditions are better than those of only eCO2. The benefits of SeNPs+eCO2 treatment in improving the content of total antioxidant capacity, polyphenols, flavonoids, and total tocopherols in plants were recorded. SeNPs+eCO2 treatment resulted in the highest accumulation of photosynthetic pigment content in leaves. Under eCO2 only, positive impacts on Rubisco activity and stomatal conductance were observed. Further research is needed to propose optimal doses of SeNPs, in particular under elevated CO2 conditions.
Data availability statement
The raw data supporting the conclusions of this article will be made available by the authors, without undue reservation.
Author contributions
All authors have contributed equally to the research and analysis of the various results sections within the review. All have corrected and modified the different versions of the manuscript as prepared by the corresponding and senior authors. All authors contributed to the article and approved the submitted version.
Acknowledgments
The authors extend their appreciation to the Deputyship for Research & Innovation, Ministry of Education in Saudi Arabia for funding this research work through the project number MoE-IF-UJ-22-4220070-3.
Conflict of interest
The authors declare that the research was conducted in the absence of any commercial or financial relationships that could be construed as a potential conflict of interest.
Publisher’s note
All claims expressed in this article are solely those of the authors and do not necessarily represent those of their affiliated organizations, or those of the publisher, the editors and the reviewers. Any product that may be evaluated in this article, or claim that may be made by its manufacturer, is not guaranteed or endorsed by the publisher.
References
AbdElgawad, H., Abuelsoud, W., Madany, M. M., Selim, S., Zinta, G., Mousa, A. S. (2020). Actinomycetes enrich soil rhizosphere and improve seed quality as well as productivity of legumes by boosting nitrogen availability and metabolism. Biomolecules 10, 1675. doi: 10.3390/biom10121675
AbdElgawad, H., De Vos, D., Zinta, G. (2015). Grassland species differentially regulate proline concentrations under future climate conditions: an integrated biochemical and modelling approach. New Phytolgist 208, 354–369. doi: 10.1111/nph.13481
AbdElgawad, H., Peshev, D., Zinta, G., Van den Ende, W., Janssens, I. A., Asard, H. (2014). Climate extreme effects on the chemical composition of temperate grassland species under ambient and elevated CO2: a comparison of fructan and non-fructan accumulators. PLoS One 9 (3), e92044. doi: 10.1371/journal.pone.0092044
AbdElgawad, H., Zinta, G., Beemster, G. T. S., Janssens, I. A., Asard, H. (2016). Future climate CO2 levels mitigate stress impact on plants: increased defense or decreased challenge? Front. Plant Sci. 7. doi: 10.3389/fpls.2016.00556
Acosta-Motos, J. R., Ortuño, M. F., Bernal-Vicente, A., Diaz-Vivancos, P., Sanchez-Blanco, M. J., Hernandez, J. A. (2017). Plant responses to salt stress: adaptive mechanisms. Agronomy 7, 18. doi: 10.3390/agronomy7010018
Aebi, H. (1984). Catalase in vitro. Methods Enzymology 105, 121–126. doi: 10.1016/S0076-6879(84)05016-3
Ainsworth, E. A., Rogers, A. (2007). The response of photosynthesis and stomatal conductance to rising [CO2]: mechanisms and environmental interactions. Plant Cell Environ. 30, 258–270. doi: 10.1111/j.1365-3040.2007.01641.x
Albqmi, M., Yaghoubi Khanghahi, M., Selim, S., Al-Sanea, M. M., Alnusaire, T. S., Almuhayawi, M. S., et al. (2023). Positive interaction of selenium nanoparticles and olive solid waste on vanadium-stressed soybean plant. Agriculture 13 (2), 426. doi: 10.3390/agriculture13020426
Approbato, A. U., Contin, D. R., de Oliveira, E. A. D., Habermann, E., Cela, J., Pintó-Marijuan, M., et al. (2023). Adjustments in photosynthetic pigments, PS II photochemistry and photoprotection in a tropical C4 forage plant exposed to warming and elevated [CO2]. Plant Physiol. Biochem. 194, 345–360. doi: 10.1016/j.plaphy.2022.11.033
Bamrah, R. K., Vijayan, P., Karunakaran, C., Muir, D., Hallin, E., Stobbs, J., et al. (2019). Evaluation of X-ray fluorescence spectroscopy as a tool for nutrient analysis of pea seeds. Crop Sci. 59, 2689–2700. doi: 10.2135/cropsci2019.01.0004
Bano, I., Skalickova, S., Sajjad, H., Skladanka, J., Horky, P. (2021). Uses of selenium nanoparticles in the plant production. Agronomy 11, 2229. doi: 10.3390/agronomy11112229
Blandino, M., Badeck, F. W., Giordano, D., Marti, A., Rizza, F., Scarpino, V., et al. (2020). Elevated CO2 impact on common wheat (Triticum aestivum l.) yield, wholemeal quality, and sanitary risk. J. Agric. Food Chem. 68 (39), 10574–10585. doi: 10.1021/acs.jafc.0c02975
Broberg, M. C., Högy, P., Feng, Z., Pleijel, H. (2019). Effects of elevated CO2 on wheat yield: non-linear response and relation to site productivity. Agronomy 9, 243. doi: 10.3390/agronomy9050243
Dhindsa, R. S., Plumb-Dhindsa, P. L., Reid, D. M. (1982). Leaf senescence and lipid peroxidation, effects of some phytohormones, and scavengers of free radicals and singlet oxygen. Physiologia Plantarum 56, 453–457. doi: 10.1111/j.1399-3054.1982.tb04539.x
Drotar, A., Phelps, P., Fall, R. (1985). Evidence for glutathione peroxidase activities in cultured plant cells. Plant Sci. 42, 35–40. doi: 10.1016/0168-9452(85)90025-1
Du, W., Gardea-Torresdey, J. L., Xie, Y., Yin, Y., Zhu, J., Zhang, X., et al. (2017). Elevated CO2 levels modify TiO2 nanoparticle effects on rice and soil microbial communities. Sci. Total Environ. 578, 408–416. doi: 10.1016/j.scitotenv.2016.10.197
Du, B., Shukla, M. K., Yang, X., Du, T. (2023). Enhanced fruit yield and quality of tomato by photosynthetic bacteria and CO2 enrichment under reduced irrigation. Agricult. Water Manage. 277, 108106. doi: 10.1016/j.agwat.2022.108106
Dubey, S. K., Tripathi, S. K., Pranuthi, G. (2015). Effect of elevated CO2 on wheat crop: mechanism and impact. Crit. Rev. Environ. Sci. Technol. 45 (21), 2283–2304. doi: 10.1080/10643389.2014.1000749
Duval, B. D., Blankinship, J. C., Dijkstra, P. (2012). CO2 effects on plant nutrient concentration depend on plant functional group and available nitrogen: a meta-analysis. Plant Ecol. 213, 505–521. doi: 10.1007/s11258-011-9998-8
Elkelish, A. A., Soliman, M. H., Alhaithloul, H. A., El-Esawi., M. A. (2019). Selenium protects wheat seedlings against salt stress-mediated oxidative damage by up-regulating antioxidants and osmolytes metabolism. Plant Physiol. Biochem. 137, 144–153. doi: 10.1016/j.plaphy.2019.02.004
Fang, R., Li, Y., Yu, Z., Xie, Z., Wang, G., Liu, X., et al. (2022). Warming offsets the beneficial effect of elevated CO2 on maize plant-carbon accumulation in particulate organic carbon pools in a mollisol. Catena 213, 106219. doi: 10.1016/j.catena.2022.106219
Foyer, C., Rowell, J., Walker, D. (1983). Measurements of the ascorbate content of spinach leaf protoplasts and chloroplasts during illumination. Planta 157, 239–244.
Fratianni, A., Mignogna, R., Niro, S., Panfili, G. (2015). Determination of lutein from fruit and vegetables through an alkaline hydrolysis extraction method and HPLC analysis. J. Food Sci. 80 (12), C2686–C2691. doi: 10.1111/1750-3841.13122
Genty, B., Briantais, J. M., Baker, N. R. (1989). The relationship between the quantum yield of photosynthetic electron transport and quenching of ChlF. Biochimica et Biophysica Acta (BBA) - General Subjects 99, 87–92. doi: 10.1016/s0304-4165(89)80016-9
Gilmore, A. M., Yamamoto, H. Y. (1991). Zeaxanthin formation and energy-dependent fluorescence quenching in pea chloroplasts under artificially mediated linear and cyclic electron transport. Plant Physiol. 96 (2), 635–643. doi: 10.1104/pp.96.2.635
Gui, J. Y., Rao, S., Huang, X., Liu, X., Cheng, S., Xu, F. (2022). Interaction between selenium and essential micronutrient elements in plants: a systematic review. Sci. Total Environment. 853, 158673. doi: 10.1016/j.scitotenv.2022.158673
Gupta, U. C., Gupta, S. C. (2000). Selenium in soils and crops, its deficiencies in livestock and humans: implications for management. Commun. Soil Sci. Plant Anal. 31, 1791–1807. doi: 10.1080/00103620009370538
Habeeb, T. H., Abdel-Mawgoud, M., Yehia, R. S., Khalil, A. M. A., Saleh, A. M., AbdElgawad, H. (2020). Interactive impact of arbuscular mycorrhizal fungi and elevated CO2 on growth and functional food value of Thymus vulgare. J. Fungi 6, 168. doi: 10.3390/jof6030168
Habibi, G. (2017). Physiological, photochemical and ionic responses of sunflower seedlings to exogenous selenium supply under salt stress. Acta Physiol. Plant 39, 1–9. doi: 10.1007/s11738-017-2517-3
Hu, T., Li, H., Li, J., Zhao, G., Wu, W., Liu, L., et al. (2018). Absorption and bio-transformation of selenium nanoparticles by wheat seedlings (Triticum aestivum l.). Front. Plant Sci. 9. doi: 10.3389/fpls.2018.00597
Ikram, M., Raja, N. I., Javed, B., Mashwani, Z. R., Hussain, M., Hussain, M., et al. (2020). Foliar applications of bio-fabricated selenium nanoparticles to improve the growth of wheat plants under drought stress. Green Process. Synthesis 9, 706–714. doi: 10.1515/gps-2020-0067
Jensen, R. G. (2000). Activation of rubisco regulates photosynthesis at high temperature and CO2. Proc. Natl. Acad. Sci. U.S.A. 97 (24), 12937–12938. doi: 10.1073/pnas.97.24.12937
Jing, L., Wang, J., Shen, S., Wang, Y., Zhu, J., Wang, Y., et al. (2016). The impact of elevated CO2 and temperature on grain quality of rice grown under open-air field conditions. J. Sci. Food Agric. 96, 3658–3667. doi: 10.1002/jsfa.7545
Kaur, H., Kumar, A., Choudhary, A., Sharma, S., Choudhary, D. R., Mehta, S. (2023). “Chapter 3– effect of elevated CO2 on plant growth, active constituents, and production,” in Plants and their interaction to environmental pollution. Ed. Husen, A. (Elsevier), 61–77. doi: 10.1016/B978-0-323-99978-6.00016-9
Kaur, N., Sharma, S., Kaur, S., Nayyar, H. (2014). Selenium in agriculture: a nutrient or contaminant for crops? Arch. Agron. Soil Sci. 60, 1593–1624. doi: 10.1080/03650340.2014.918258
Kumar, K. B., Khan, P. A. (1982). Peroxidase and polyphenol oxidase in excised ragi (Eleusine coracana cv.PR 202) leaves during senescence. Ind. J. Exp. Bot. 20, 412–416. doi: 10.1104/pp.57.2.315
Lanza, M. D. G. B., dos Reis, A. R. (2021). Roles of selenium in mineral plant nutrition: ROS scavenging responses against abiotic stresses. Plant Physiol. Biochem. 164, 27–43. doi: 10.1016/j.plaphy.2021.04.026
Lawongsa, P., Inubushi, K., Wada, H. (1987). Determination of organic acids in soil by high performance liquid chromatography. Soil Sci. Plant Nutr. 33, 299–302. doi: 10.1080/00380768.1987.10557575
Liu, J., Qi, W. Y., Chen, H., Song, C., Li, Q., Wang, S. G. (2021). Selenium nanoparticles as an innovative selenium fertilizer exert less disturbance to soil microorganisms. Front. Microbiol. 12. doi: 10.3389/fmicb.2021.746046
Lobo, A. K. M., de Oliveira Martins, M., Neto, M. C. L., Machado, E. C., Ribeiro, R. V., Silveira, J. A. G. (2015). Exogenous sucrose supply changes sugar metabolism and reduces photosynthesis of sugarcane through the down-regulation of rubisco abundance and activity. J. Plant Physiol. 179, 113–121. doi: 10.1016/j.jplph.2015.03.007
Lyu, L., Wang, H., Liu, R., Xing, W., Li, J., Man, Y. B., et al. (2022). Size-dependent transformation, uptake, and transportation of SeNPs in a wheat–soil system. J. Hazardous Materials 424, 127323. doi: 10.1016/j.jhazmat.2021.127323
Mishra, A. K., Rai, R., Agrawal, S. (2013). Individual and interactive effects of elevated carbon dioxide and ozone on tropical wheat (Triticum aestivum l.) cultivars with special emphasis on ROS generation and activation of antioxidant defence system. Indian J. Biochem. Biophysics 50, 139–149.
Morales-Espinoza, M. C., Cadenas-Pliego, G., Pérez-Alvarez, M., Hernández-Fuentes, A. D., de la Fuente, M. C., Benavides-Mendoza, A., et al. (2019). Se Nanoparticles induce changes in the growth, antioxidant responses, and fruit quality of tomato developed under NaCl stress. Molecules 24, 3030. doi: 10.3390/molecules24173030
Murshed, S. M. S., Tan, S. H., Nguyen, N. T. (2008). Temperature dependence of interfacial properties and viscosity of nanofluids for droplet-based microfluidics. J. Phys. D. Appl. Phys. 41, 85502. doi: 10.1088/0022-3727/41/8/085502
Nawaz, F., Ashraf, M. Y., Ahmad, R. (2013). Selenium (Se) seed priming induced growth and biochemical changes in wheat under water deficit conditions. Biol. Trace Elem Res. 151, 284–293. doi: 10.1007/s12011-012-9556-9
Porra, R. J. (2002). The chequered history of the development and use of simultaneous equations for the accurate determination of chlorophylls a and b. Photosynthesis Res. 73, 149–156. doi: 10.1023/A:1020470224740
Reis, A. R., El-Ramady, H., Santos, E. F., Gratão, P. L., Schomburg, L. (2017). Overview of selenium deficiency and toxicity worldwide: affected areas, selenium-related health issues, and case studies (Springer), 209–230. doi: 10.1007/978-3-319-56249-0_13
Rosenfeld, C. E., Kenyon, J. A., James, B. R., Santelli, C. M. (2017). Selenium (IV,VI) reduction and tolerance by fungi in an oxic environment. Geobiology 15, 441–452. doi: 10.1111/gbi.12224
Sekhar, K. M., Sreeharsha, R. V., Reddy, A. R. (2015). Differential responses in photosynthesis, growth and biomass yields in two mulberry genotypes grown under elevated CO2 atmosphere. J. Photochem. Photobiol. B: Biol. 151, 172–179. doi: 10.1016/j.jphotobiol.2015.08.008
Selim, S., Akhtar, N., El Azab, E., Warrad, M., Alhassan, H. H., Abdel-Mawgoud, M., et al. (2022). Innovating the synergistic assets of β-amino butyric acid (BABA) and selenium nanoparticles (SeNPs) in improving the growth, nitrogen metabolism, biological activities, and nutritive value of Medicago interexta sprouts. Plants 11, 306. doi: 10.3390/plants11030306
Shabbaj, I. I., Madany, M. M. Y., Balkhyour, M. A., Tammar, A., AbdElgawad, H. (2022). CO2 enrichment differentially upregulated sugar, proline, and polyamine metabolism in young and old leaves of wheat and sorghum to mitigate indium oxide nanoparticles toxicity. Front. Plant Sci. 13. doi: 10.3389/fpls.2022.843771
Silva, V. M., Rimoldi Tavanti, R. F., Gratão, P. L., Alcock, T. D., dos Reis, A. R. (2020). Selenate and selenite affect photosynthetic pigments and ROS scavenging through distinct mechanisms in cowpea (Vigna unguiculata (L.) walp) plants. Ecotoxicology Environ. Saf. 201, 110777. doi: 10.1016/j.ecoenv.2020.110777
Sreeharsha, R. V., Mudalkar, S., Sengupta, D. (2019). Mitigation of drought-induced oxidative damage by enhanced carbon assimilation and an efficient antioxidative metabolism under high CO2 environment in pigeonpea (Cajanus cajan l.). Photosynth Res. 139, 425–439. doi: 10.1007/s11120-018-0586-9
Wang, M., Dong, C., Fu, Y., Liu, H. (2015). Growth, morphological and photosynthetic characteristics, antioxidant capacity, biomass yield and water use efficiency of Gynura bicolor DC exposed to super-elevated CO2. Acta Astronaut. 114, 138–146. doi: 10.1016/j.agee.2013.06.013
Wang, L., Feng, Z., Schjoerring, J. K. (2013). Effects of elevated atmospheric CO2 on physiology and yield of wheat (Triticum aestivum l.): a meta-analytic test of current hypotheses. Agric. Ecosyst. Environ. 178, 57–63. doi: 10.1016/j.agee.2013.06.013
Wang, X., Liu, F. (2021). Effects of elevated CO2 and heat on wheat grain quality. Plants 10, 1027. doi: 10.3390/plants10051027
Yaghoubi Khanghahi, M., Leoni, B., Crecchio, C. (2021). Photosynthetic responses of durum wheat to chemical/microbiological fertilization management under salt and drought stresses. Acta Physiologiae Plantarum 43, 123. doi: 10.1007/s11738-021-03289-z
Yaghoubi Khanghahi, M., Pirdashti, H., Rahimian, H., Nematzadeh, G. A., Ghajar Sepanlou, M., Salvatori, E., et al. (2019). Evaluation of leaf photosynthetic characteristics and photosystem II photochemistry of rice (Oryza sativa l.) under potassium soloubilizing bacteria (KSB) inoculation. Photosynthetica 57, 500–511. doi: 10.32615/ps.2019.065
Yoshida, Y., Marubodee, R., Ogiso-Tanaka, E., Iseki, K., Isemura, T., Takahashi, Y., et al. (2016). Salt tolerance in wild relatives of adzuki bean, Vigna angularis (Willd.) ohwi et ohashi. Genet. Resour. Crop Evol. 63, 627–637. doi: 10.1007/s10722-015-0272-0
Yu, Y., Assmann, S. M. (2016). The effect of NaCl on stomatal opening in arabidopsis wild type and agb1 heterotrimeric G-protein mutant plants. Plant Signaling Behav. 11, e1085275. doi: 10.1080/15592324.2015.1085275
Keywords: antioxidant, elevated CO2, metabolite, selenium nanoparticles, wheat
Citation: Alsherif EA, Hajjar D, Aldilami M and AbdElgawad H (2023) Physiological and biochemical responses of wheat to synergistic effects of selenium nanoparticles and elevated CO2 conditions. Front. Plant Sci. 14:1183185. doi: 10.3389/fpls.2023.1183185
Received: 09 March 2023; Accepted: 07 June 2023;
Published: 13 July 2023.
Edited by:
Pawan Shukla, Seri-Biotech Research Laboratory (SBRL), IndiaReviewed by:
Anirban Guha, Indian Institute of Science Education and Research, IndiaBarbara Hawrylak-Nowak, University of Life Sciences of Lublin, Poland
Rachapudi V. Sreeharsha, Indian Institute of Chemical Technology (CSIR), India
Copyright © 2023 Alsherif, Hajjar, Aldilami and AbdElgawad. This is an open-access article distributed under the terms of the Creative Commons Attribution License (CC BY). The use, distribution or reproduction in other forums is permitted, provided the original author(s) and the copyright owner(s) are credited and that the original publication in this journal is cited, in accordance with accepted academic practice. No use, distribution or reproduction is permitted which does not comply with these terms.
*Correspondence: Emad A. Alsherif, ZWFhbHNoZXJpZkB1ai5lZHUuc2E=