- Graduate School of Integrative Science and Engineering, Tokyo City University, Setagata, Tokyo, Japan
Farfugium japonicum (L.) Kitam. var. japonicum grows mainly in the coastal areas of Japan. Meteorological recording data from natural habitats were used to investigate the factors associated with the laminas and petioles of radical leaves of F. japonicum var. japonicum to avoid or resist higher wind stress. Our morphological and mechanical results indicated that petiole length and petiole cross-sectional area had a weak correlation with wind speed and breaking strength, and the petiole second area moment of inertia did not differ significantly among populations. However, both lamina area and petiole length per petiole cross-sectional area decreased with increasing wind speed, indicating that F. japonicum var. japonicum resisted or avoided an increase in wind speed outdoors by reducing the lamina area and petiole length per petiole cross-sectional area without qualitative changes in their petioles. The results of this study indicated that densely distributed recording stations of the Automated Meteorological Data Acquisition System (AMeDAS) by the Japan Meteorological Agency can be used for environmental adaptation studies of plants in the field using nearby plant populations.
1 Introduction
The genus Farfugium Lindl. is a perennial herbaceous species in the Asteraceae family and consists of two species: F. hiberniflorum (Makino) Kitam. and F. japonicum (L.) Kitam. (Koyama, 1995). F. hiberniflorum is an endemic species of Japan that grows in the moist forests of Yakushima and Tanegashima Islands, subtropical islands off the southern coast of Kyushu and part of Kagoshima Prefecture. F. japonicum is distributed in Japan, Taiwan, and eastern China and occurs in the margins of forests, as well as roadsides, meadows, riversides, and coastal areas (Koyama, 1995). The four varieties of F. japonicum are: F. japonicum var. japonicum, F. japonicum var. luchuense (Masamune) Kitam., F. japonicum var. giganteum (Siebold et Zucc.) Kitam., and F. japonicum var. formosanum (Hayata) Kitam. These varieties have been used as antipyretics and ingredients in folk remedies for coughs, wound healing, bronchitis, and diarrhoea (Yun et al., 2015). As indicated by Kim et al. (2008), the F. japonicum essential oil exerted anti-inflammatory effects, and F. japonicum var. giganteum and F. japonicum var. formosanum have been identified as potential chemical and medicinal ingredients (Hsieh et al., 2012; Devkota et al., 2022). In contrast to these medicinal uses, investigation of F. japonicum var. luchuense has provided insights into the morphological, anatomical, and genetic mechanisms of local plant adaptation. The narrow leaves of F. japonicum var. luchuense subjected to flash floods after heavy rain showed mechanical selective pressure from water stream in flooded areas along rivers and have adapted to have a decreased number of leaf cells across the width of the leaf (Usukura et al., 1994). In addition, this species has significant intraspecific variation in leaf form, with a trend toward wider leaf base angles as one moves more distant from the riverside (Usukura et al., 1994). Plant groups, including F. japonicum var. luchuense, inhabiting riverbanks with these leaf morphological characteristics such as narrow leaves are called rheophytes (van Steenis, 1981), and some studies have reported the convergent evolution of many phylogenetically distinct taxa of ferns and angiosperms (Imaichi and Kato, 1992; Yamada et al., 2011; Ohga et al., 2012; Ueda et al., 2012; Yokoyama et al., 2012; Matsui et al., 2013; Shiba et al., 2021). Thus, varieties of F. japonicum have been studied for both pharmaceutical purposes and ecological research.
F. japonicum var. japonicum grows in the margin of inland forests near the coast, is frequently cultivated for ornamental purposes in gardens (Koyama, 1995), and has hybridised with F. hiberniflorum on Yakushima Island (Yamaguchi and Yahara, 1989). F. japonicum var. japonicum grows in a variety of environments, and it is possible that these ecological transitions from open habitats to coastal conditions have driven morphological and anatomical variation to adapt to different environments (Figure 1). In particular, coastal areas are characterised by open, sandy, or rocky habitats with weakly developed soils, which leads to morphological modification. Plant species growing in coastal regions need to adapt to an environment in which drought and wind strongly affect plant growth (Greenway and Munns, 1980). Therefore, it is of interest to clarify the adaptation patterns of F. japonicum var. japonicum in coastal areas.
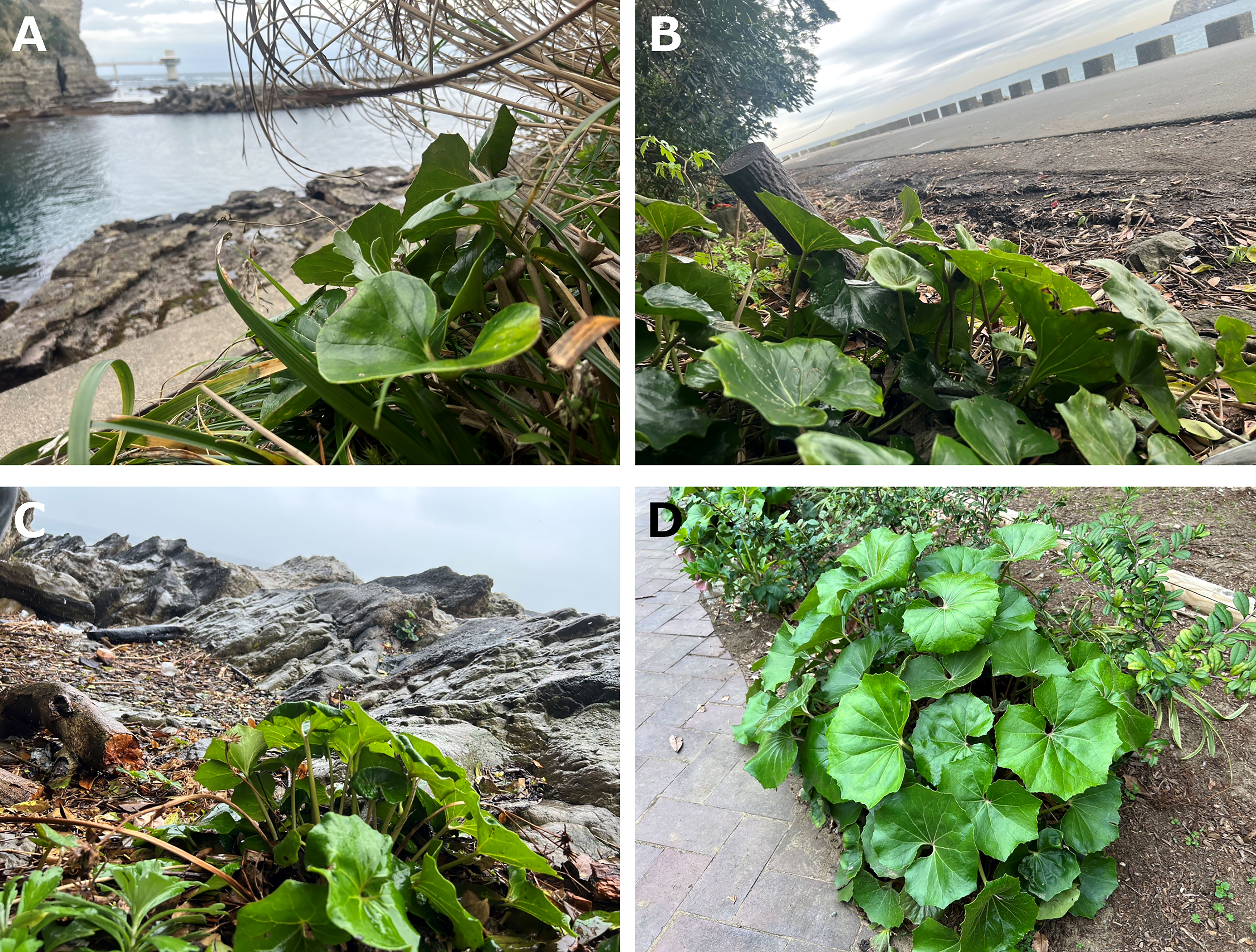
Figure 1 Farfugium japonicum (L.) Kitam. var. japonicum (Asteraceae). (A): coastal of Ubara; (B): coastal of Kyonan; (C): coastal of Arasaki; (D): inland of Kawasaki.
Analysing phenotypic plasticity in response to changes in environmental factors is complicated, partially because these conditions may have multiple effects on plants. Sodium (Na+) accumulates in the soil of coastal areas, causing osmotic stress between the soil and plant roots, which inhibits water uptake by coastal plants. Sodium (Na+) absorbed by the plant body is concentrated in the leaves, reducing the cell’s osmotic potential and causing dehydration or drought stress (Kaspari et al., 2009). Some studies on drought stress in coastal areas have been conducted on coastal adaptation based on morphological and anatomical analyses. Tunala Hayakawa et al. (2012) demonstrated that the epidermal cells of the coastal variety Aster hispidus Thunb. var. insularis (Makino) Okuyama (Asteraceae) are larger in size and less in number compared to As. hispidus var. hispidus, and they produce succulent leaves to store water. In this species, Kumekawa et al. (2013) and Sunami et al. (2013) reported that leaf hair on the abaxial side of leaves was correlated to the stomatal density of this variety, and the less hair on the leaf, the lower the stomatal density to avoid transpirational water loss. Ohga et al. (2013) suggested that the coastal population of Adenophora triphylla (Thunb.) A.DC. var. japonica (Regel) H. Hara (Campanulaceae) had evolved relatively thick leaves via a heterochronic process to store water. Shiba et al. (2022) pointed out that coastal populations of Eurya japonica Thunb. (Ternstroemiaceae) have small stomata and larger adaxial and abaxial epidermal cells to reduce transpiration during gas exchange and retain moisture within the leaves. Similar results regarding the differentiation between inland and coastal populations were obtained from Ligstrum japonicum Thunb. (Oleaceae) (Takizawa et al., 2022) and L. lucidum Aiton (Oleaceae) (Takizawa et al., 2023). However, coastal areas are subject to drought as well as wind stress; therefore, it is also necessary to consider morphological changes in response to strong wind stress when discussing adaptation to coastal areas.
Wind flow exerts drag forces on plants causing mechanical stress, and plant responses to wind typically include inhibition of stem elongation and increased stem diameter (Jaffe and Forbes, 1993; Telewski and Pruyn, 1998; Coutand et al., 2000; Anten et al., 2010; Bang et al., 2010). However, wind can also induce responses that are different or even opposite to those induced by pure mechanical stress, such as the production of thinner, more elongated stems under wind loading (Henry and Thomas, 2002; Smith and Ennos, 2003). Wind is a particularly complex environmental factor that has several effects on plants (Ennos, 1997). Wind speeds are stronger in coastal areas than inland areas, and wind-induced stress plays an important role in the speciation of plants adapted to coastal areas (Shiba et al., 2022; Takizawa et al., 2022; Takizawa et al., 2023). However, limited studies have been conducted on plant adaptation to wind-induced mechanical stress in coastal areas. Meguro and Miyawaki (1994) reported that the strain energy per unit volume of branches increased in individuals grown under strong winds compared to those grown under weak winds using the coastal tree species Celtis sinensis Pers. (Cannabaceae), Ilex integra Thunb. (Aquifoliaceae), E. japonica, Pittosporum tobira (Thunb.) W.T.Aiton (Pittosporaceae), Euonymus japonicus Thunb. (Celastraceae), and Cinnamomum japonicum H.Ohba (Lauraceae). However, F. japonicum var. japonicum may have different responses to the wind because it is a herbaceous species. In general, the most optimised leaf would have a large, flat, and stiff lamina to maximise light capture and a flexible petiole to avoid fractures (Niinemets and Fleck, 2002). The ability to deploy leaves to sunlight regardless of external factors, such as wind, is crucial for plant survival (Roden and Pearcy, 1993; Takenaka, 1994; de Langre, 2008; Vogel, 2009). Niklas (1996) reported that the comparison of sugar maple (Acer saccharum Marshall [Aceraceae]) leaves sampled from young trees growing in two locations with different ambient wind speeds showed that individuals in wind-exposed areas had smaller leaf blades and more flexible petioles than those in protected areas. This indicated that wind affected both the leaves and petioles in the sugar maple. Louf et al. (2018) indicated that the ability of the leaf to reduce wind stress at the stem-petiole junction could be achieved by locating the twisting area closer to the lamina; even if the stress is constant throughout the petiole, the strain decreases closer to the stem. This hypothesis is supported by wind-induced bending and twisting stress in red oak (Quercus rubra L. [Fagaceae]), American sycamore (Platanus occidentalis L. [Platanaceae]), yellow poplar (Liriodendron tulipifera L. [Magnoliaceae]), and sugar maple. In addition, some studies have shown that avoidance strategies reduce stem or petiole thickness and flexural rigidity in response to mechanical stress in Arabidopsis thaliana (L.) Heynh., Cecropia schreberiana Miq. (Urticaceae), Potentilla reptans L. (Rosaceae), and Phaseolus vulgaris L. (Fabaceae) (Jaffe et al., 1984; Cordero, 1999; Liu et al., 2007; Paul-Victor and Rowe, 2011). Although these studies are important for considering the mechanism by which leaves are not detached from the junction between the lamina and petiole and the stem and petiole, they cannot be directly applied to F. japonicum var. japonicum; this species has radical leaves that grow perpendicular to the aboveground area from the rhizome, so the static load on the petiole itself may be different from that of plants that extend the petiole horizontally from the stem. In fact, the petioles of F. japonicum var. japonicum are approximately 30 cm long and carries a leaf which is approximately 10 cm long and 20 cm wide at the tip of the petiole (Figure 1). In other plants with radical leaves, comparisons of morphological and mechanical traits under wind stress using Plantago major L. (Plantaginaceae) showed that the length of petioles was shorter, but the diameter was not different from that of controls (Anten et al., 2010). This result is important for understanding F. japonicum var. japonicum adaptations; petioles could also shorten in windy coastal areas and the strength could vary with wind speed.
Understanding the wind-induced motion of plants is of interest in many fields of plant science and engineering (Roden and Pearcy, 1993; Endalew et al., 2011), and many studies have been conducted in laboratories using wind tunnels (Niinemets and Fleck, 2002; Poulsen and Nordal, 2005; Sack et al., 2008; Jones and Kang, 2015; Ray and Jones, 2018; Wunnenberg et al., 2021). Thorough analysis is key for comparing with the realistic outdoor motion of petioles, as petioles are the most common flexible structure. The Japan Meteorological Agency publishes data and maps of wind speed at the recording stations of the Automated Meteorological Data Acquisition System (AMeDAS). Densely distributed recording stations (1,300 locations) of AMeDAS can approximate field meteorological data without artificial weather manipulation by analysing nearby populations. Because F. japonicum var. japonicum grows in various places along the coast, the analysis of populations near recording stations of AMeDAS was thought to enable characterisation of differences in laminas and petioles due to wind stress. Using populations as close as possible was preferred because other environmental factors must be considered when the distance between populations is large. Populations of F. japonicum var. japonicum were found within areas of the east and west sides of Boso Peninsula and the nearby Miura Peninsula of Kanto District in Japan that had different wind speeds, such as average annual wind speed, maximum wind speed, and maximum instantaneous wind speed, as well as the number of occurrences per year with a wind speed of 10 m/s and 20 m/s, according to the AMeDAS data of the Japan Meteorological Agency (Figures 2, 3). The aim of this study was to clarify the effects of wind speed on the laminas and petioles of F. japonicum var. japonicum in the field based on morphological and mechanical analyses.
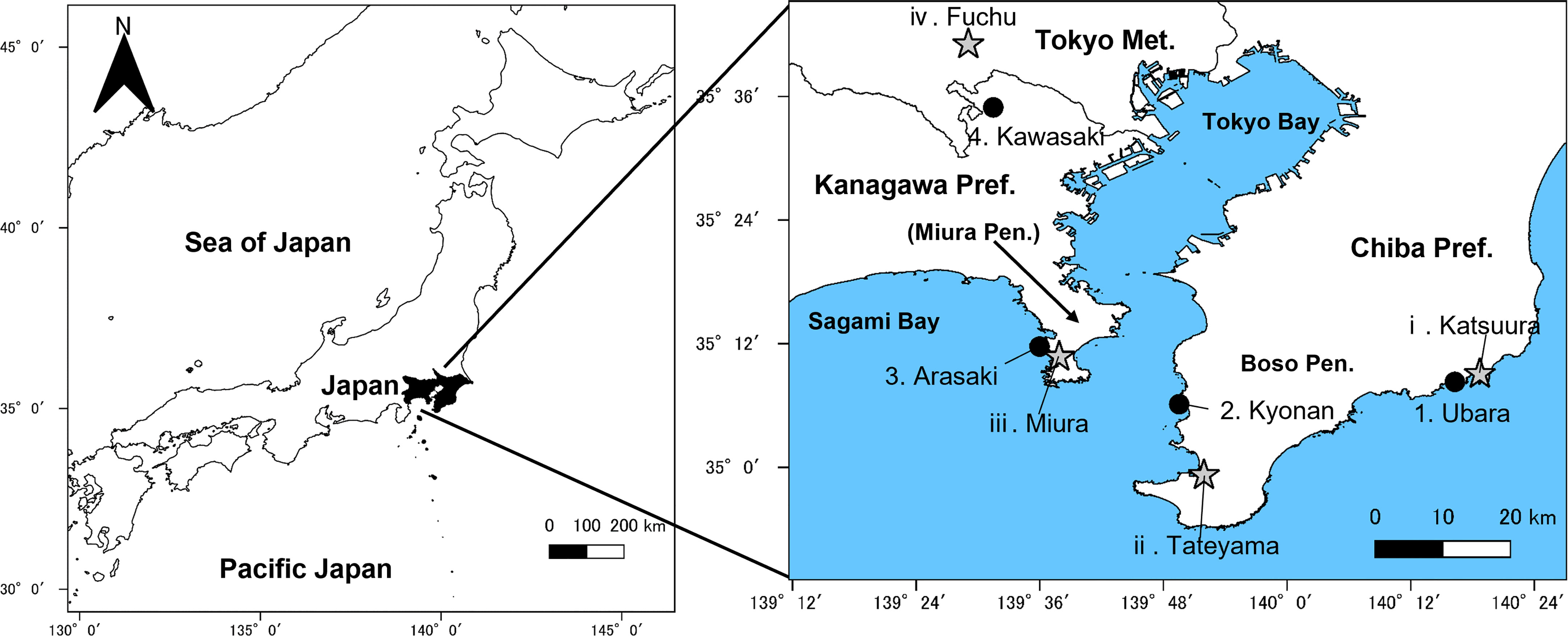
Figure 2 Sampling localities (●) and recording stations of Automated Meteorological Data Acquisition System (AMeDAS) (★). Number corresponds to that given in Table 1.
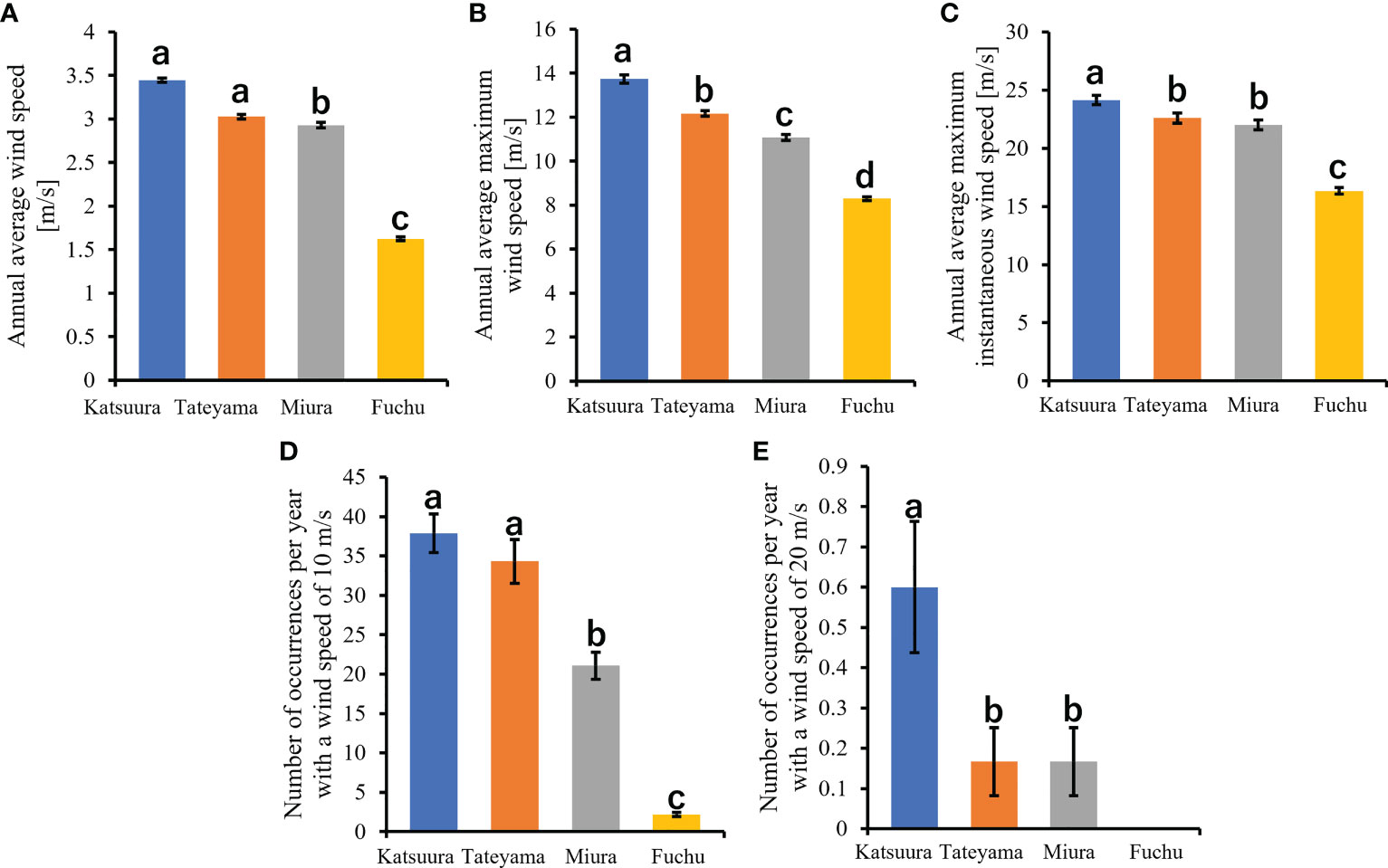
Figure 3 Comparisons of wind speeds of Boso Peninsula and the nearby Miura Peninsula of Kanto District in Japan. (A): annual average wind speed; (B): annual average maximum wind speed; (C): annual average maximum instantaneous wind speed; (D): number of occurrences per year with a wind speed of 10m/s; (E): number of occurrences per year with a wind speed of 20m/s.
2 Materials and methods
In this study, wind speed data were obtained from Katsuura, Tateyama, Miura, and Fuchu AMeDAS recording stations near our collection locations (Figure 2 and Table 1). For the analysis of AMeDAS data from the Japan Meteorological Agency, data for the past 30 years in Katsuura, Tateyama, Miura, and Fuchu were used for average annual wind speed, average annual maximum wind speed, and number of occurrences per year with wind speeds of 10 and 20 m/s. Data for the past 10 years were used for the average annual maximum instantaneous wind speed; data from the past 30 years could not be used because there was a difference in the amount of AMeDAS data accumulated among Katsuura, Tateyama, Miura, and Fuchu.
Individuals of F. japonicum var. japonicum examined in this study were collected from the field locations (Figure 2 and Table 1). Leaves were collected one per individual. The inland populations near each coastal area were used as controls. Samples were collected from a total of 126 individuals representing four populations in three coastal areas (Ubara, Kyonan, and Arasaki) and one inland area (Kawasaki) (Table 1). For morphological analysis, we measured lamina length, thickness, and area, as well as petiole length, diameter and cross-sectional area for each sample. ImageJ is a widely used application for the analysis of biological images (Bakr, 2005; Igathinathane et al., 2006) and can be used to analyse the area and lamina dimensions. Therefore, we used ImageJ software to measure the lamina length, and area. Leaf thickness was measured using a digimatic outside micrometer (MDC-SB, Mitutoyo, Japan). The petiole cross-section of F. japonicum var. japonicum at each collection site was sectioned using a razor and observed to be circular, so the elliptical formula was used henceforth (Figures 4A–D). Petiole length and diameter were measured using a digimatic caliper (CD-15CXR, Mitutoyo, Japan). The petiole cross-sectional area was calculated according to Eq. 1.
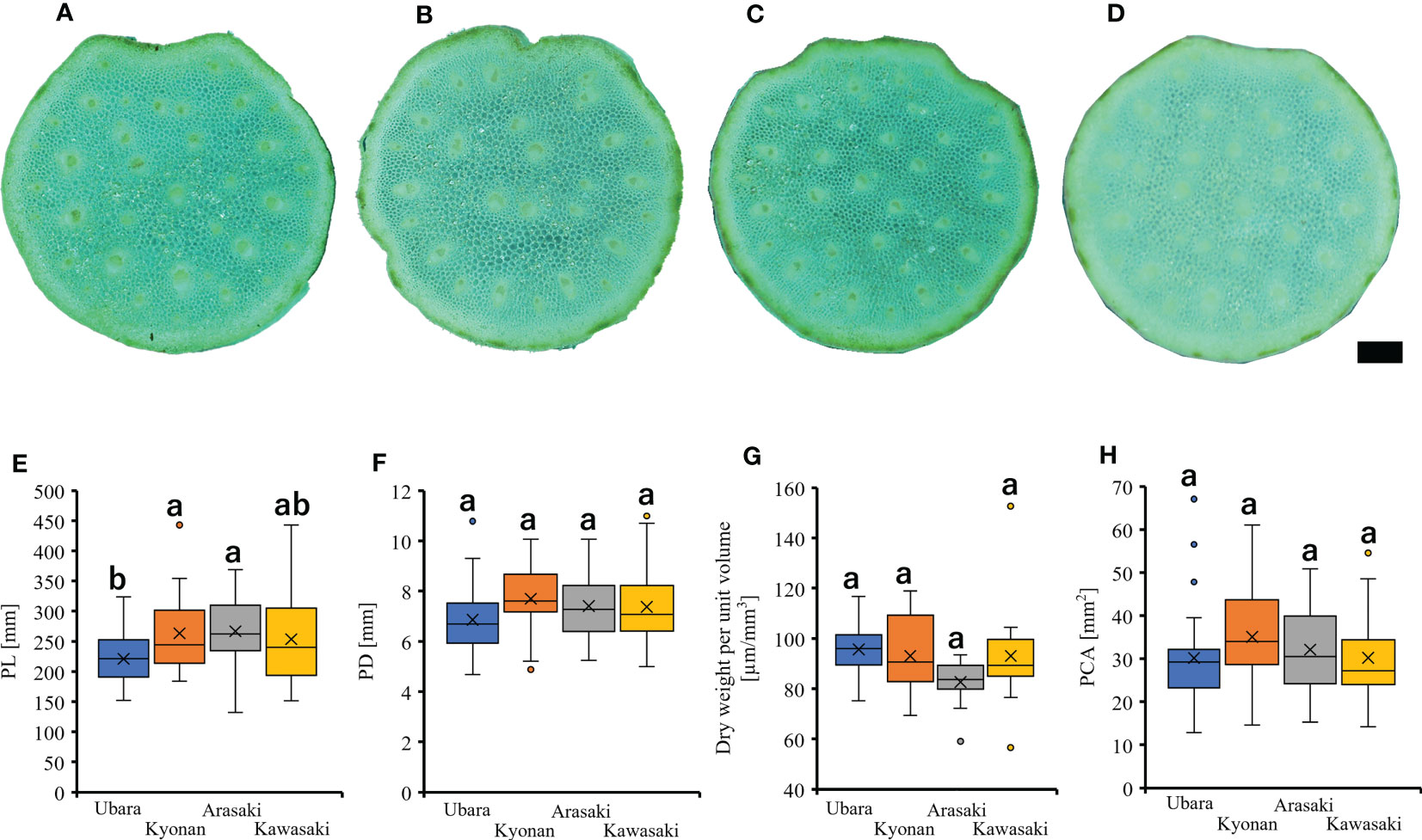
Figure 4 Petiole cross-section of F. japonicum var. japonicum. (A): Ubara; (B): Kyonan; (C): Arasaki; (D): Kawasaki; Bar = 1 mm. The comparison of petiole morphologies among populations. (E): petiole length; (F): petiole diameter; (G): dry weight per unit volume using mechanical analysis with petiole; (H): petiole cross-sectional area.
where a = petiole long diameter, and b = petiole short diameter. After measuring the lamina area, the sample was dried at 100°C for 72 hours in an incubator (FS-405, Advantec, Japan), and the dried weight was measured using an electronic balance (ATX224R, Shimadzu, Japan).
For anatomical analyses, the adaxial surface of the fully expanded lamina was used Suzuki’s Universal Micro-Printing (SUMP) method (Kijima, 1962). Three measurements were taken along the midrib of the lamina, and the average value was calculated. ImageJ software was used to measure the size of epidermal cells.
For mechanical analysis, the bending strength of the petiole was measured for each sample using a three-point bending test. The three-point bending test does not require a clamp; instead, the petiole is placed on two supports and a point load is applied to the petiole while the upper support descends at a constant speed to measure displacement and load in bending (Figure 5A). A force tester (MCT-1150, A&D, Japan) equipped with a load cell (USM-500N, A&D, Japan) with a resolution of 0.1 N was used in this study. In addition, a three-point bending test was run by attaching an optional jig (JM-B1-500N, A&D, Japan). The span length of the two lower supports was set at 50 cm, so that they were centered in the span relative to the upper supports. Sampling was performed while the upper support was moved downward at a constant speed (10 mm/min). Before conducting the test, a, b and length of the petiole used for the test were measured as described above and the volume was calculated. The bending strength (σ) was calculated from the results of the three-point bending test using Eq. (2),
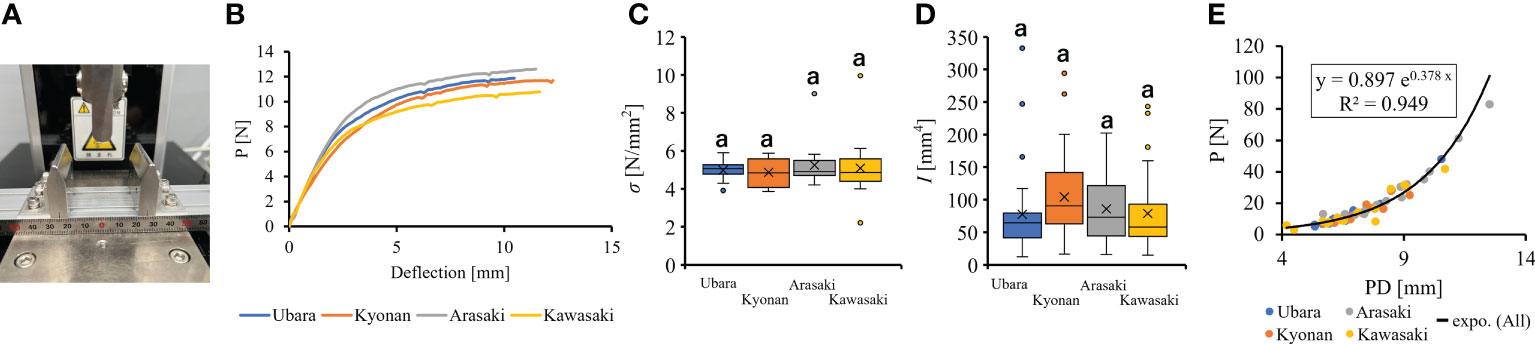
Figure 5 The comparison of petiole mechanical properties among populations. (A): three point bending tester; (B): Representative force-displacement curves of petiole at each location. Measurements approximately 6 mm in petiole diameter are plotted; (C): bending strength; (D): second area moment of inertia; (E): The relationship of petiole diameter and breaking load.
where P = breaking load point, and L = span (In this experiment, 50 mm fixed). After the test, dry weight was measured on petiole as described above, and dry weight per unit volume was determined. The second area moment of inertia (I) was calculated using Eq. 3,
Due to sample availability, the number of samples used in the mechanical analysis was performed on 15 individuals at all locations.
3 Results
In this study, wind speed data were obtained from Katsuura, Tateyama, Miura, and Fuchu AMeDAS recording stations near our collection locations. For the analysis of AMeDAS data from the Japan Meteorological Agency, data for the past 30 years in Katsuura, Tateyama, Miura, and Fuchu were used for average annual wind speed, average annual maximum wind speed, and number of occurrences per year with wind speeds of 10 and 20 m/s. Data for the past 10 years were used for the average annual maximum instantaneous wind speed; data from the past 30 years could not be used because there was a difference in the amount of AMeDAS data accumulated among Katsuura, Tateyama, Miura, and Fuchu. The results of AMeDAS data analysis showed that average wind speeds from 1991 to 2020 of Katsuura and Tateyama were significantly larger than that of Miura, and all three were significantly larger than Fuchu (Figure 3A and Table 2). The maximum wind speed from 1991 to 2020 was significantly different in all locations, with the highest in Katsuura, followed by Tateyama, Miura, and Fuchu (Figure 3B and Table 2). The maximum instantaneous wind speed (2011-2020) was significantly larger in Katsuura than in Tateyama, Miura, and Fuchu (Figure 3C and Table 2). The number of occurrences per year (1991-2020) with a wind speed of 10 m/s was significantly larger in Katsuura and Tateyama than in Miura and Fuchu (Figure 3D and Table 2), and wind speed of 20 m/s was significantly larger in Katsuura than in Tateyama, Miura, and Fuchu (Figure 3E and Table 2).
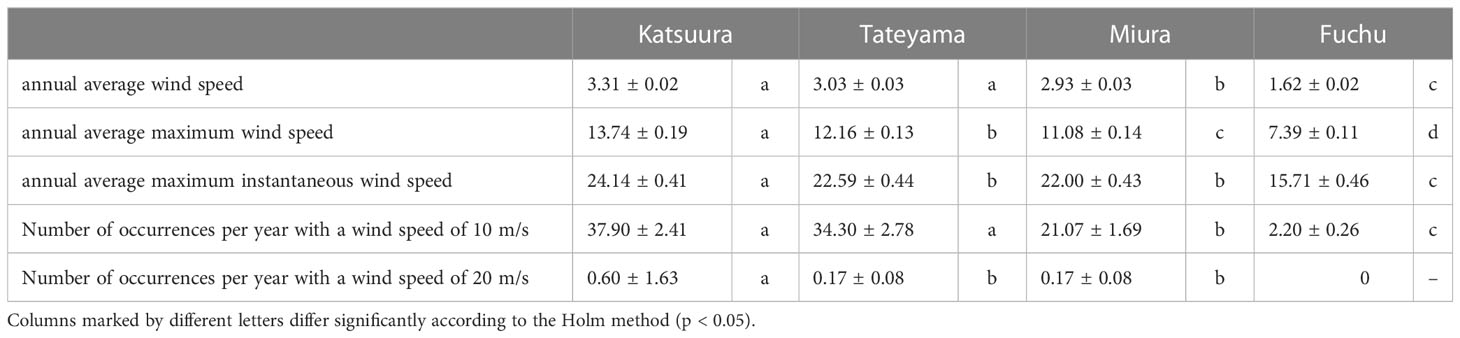
Table 2 Comparison of wind speeds (average ± standard error) of Boso Peninsula and the nearby Miura Peninsula of Kanto District in Japan.
Comparison of the lamina morphologies of F. japonicum var. japonicum among the populations showed that lamina length was significantly shorter in the population of Ubara than in those of Kyonan, Arasaki, and Kawasaki (Figure 6A and Table 3). Lamina thickness in populations of Ubara and Kyonan were significantly larger than those of Arasaki and Kawasaki (Figure 6B and Table 3). Lamina area was smallest in Ubara, followed by Arasaki, Kyonan, and Kawasaki (Figure 6C and Table 3). In addition, a strong correlation (R2 = 0.614) was observed between lamina area and dry weight (Figure 6D). Our anatomical analyses were indicated that there was no significant difference among Ubara, Kyonan, Arasaki, and Kawasaki (Figure 6E and Table 3).
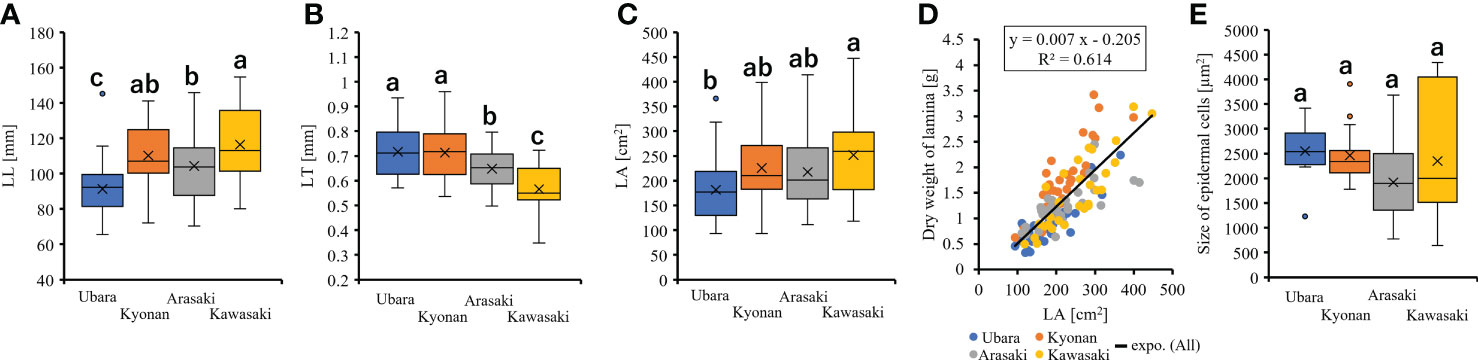
Figure 6 The comparison of lamina morphologies and anatomical among populations. (A): lamina length; (B): lamina thickness; (C): lamina area; (D): the relationship of lamina area and dry weight of lamina; (E): size of epidermal cells.
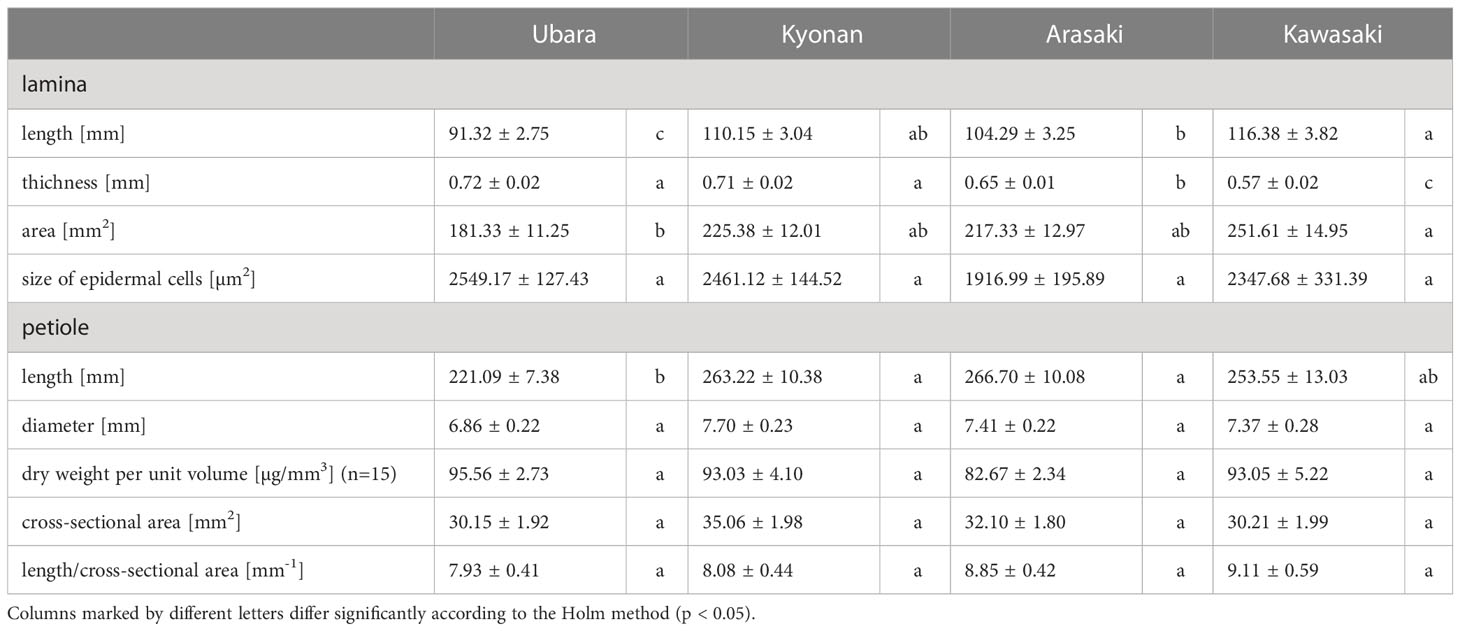
Table 3 Morphological and anatomical measurements (average ± standard error) of lamina and petiole in Farfugium japonicum.
Comparison of the petiole morphologies of F. japonicum var. japonicum among the populations showed that petiole length was significantly longer in populations of Kyonan and Arasaki than in Ubara, while no significant difference was observed with the population of Kawasaki (Figure 4E and Table 3). No significant differences were observed in the petiole diameter (Figure 4F and Table 3), dry weight per unit volume using mechanical analyses of petiole (Figure 4G and Table 3), or petiole cross-sectional area (Figure 4H and Table 3). The force-displacement curves at the petiole of each location obtained in this study are shown in Figure 5B. Comparisons of the petiole mechanical properties of F. japonicum var. japonicum showed there were no significant differences bending strength (Figure 5C and Table 4) and second area moment of inertia (Figure 5D and Table 4). A strong correlation (R2 = 0.949) was observed between petiole diameter and breaking load (Figure 5E).
The relationships between various morphological traits of F. japonicum var. japonicum were then examined. Lamina length had a weak correlation (R2 = 0.196) with petiole length (Figure 7A), but lamina area had a moderate correlation (R2 = 0.584) with petiole cross-sectional area (Figure 7B). Examining the relationship between wind speed and the various morphological traits of F. japonicum var. japonicum showed that the lamina area was strongly correlated with the average annual wind speed (R2 = 0.744), maximum wind speed (R2 = 0.827), and maximum instantaneous wind speed (R2 = 0.763) (Figures 8A–C). However, both petiole length and cross-sectional area had little correlation with any wind speed measurements (Supplementary Figure 1). While there was no significant difference in the ratio of petiole length per unit petiole cross-sectional area among populations (Figure 9A), there was a strong correlation of the ratio with average annual wind speed (R2 = 0.669), maximum wind speed (R2 = 0.818), and maximum instantaneous wind speed (R2 = 0.690) (Figures 9B–D).
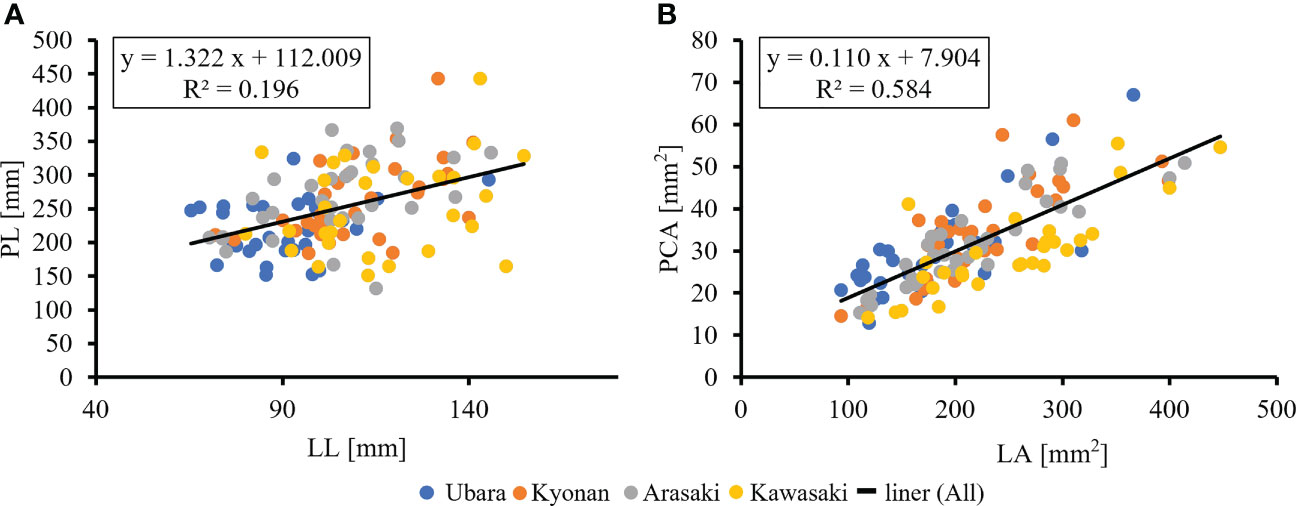
Figure 7 (A): The relationship of lamina length and petiole length. (B): The relationship of lamina area and petiole cross-sectional areas.
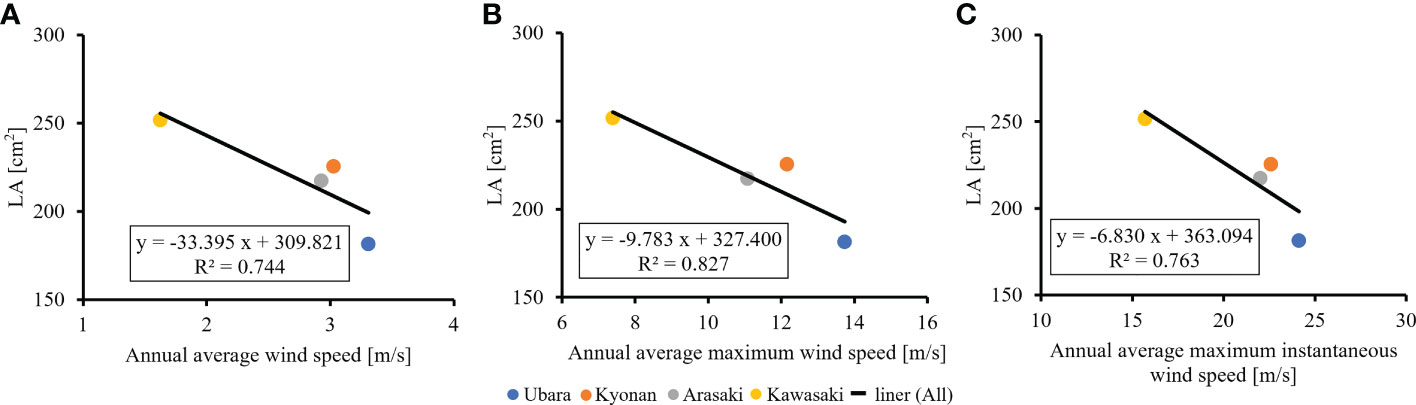
Figure 8 The relationship of lamina area and wind speeds. (A): annual average wind speed; (B): annual average maximum wind speed; (C): annual average maximum instantaneous wind speed.
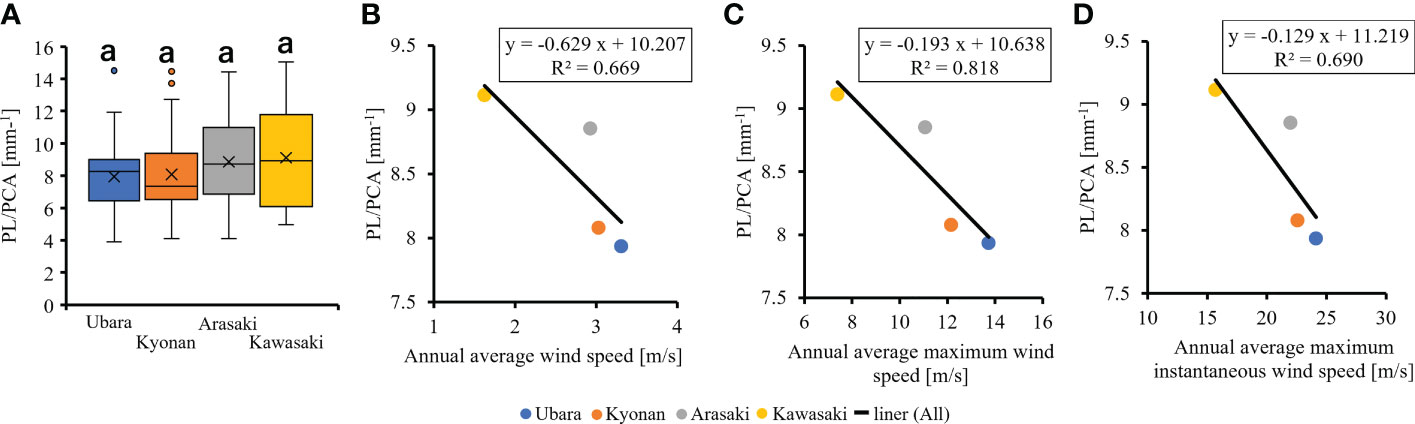
Figure 9 (A): The comparison of petiole length per unit petiole cross-sectional areas among populations. The relationship of petiole length per unit petiole cross-sectional area and wind speeds. (B): annual average wind speed; (C): annual average maximum wind speed; (D): annual average maximum instantaneous wind speed.
4 Discussion
Among plant architectures, petioles serve as conduits for the transport of nutrients and sap (Yamada et al., 1999), providing mechanical support for the lamina (Yamada et al., 1999), and adjusting leaf angle or leaf orientation to adapt to variations in the environment (Falster and Westoby, 2003). Petiole lodging of radical leaves can lead to the physical collapse of photosynthetic capacity and can occur spontaneously due to mechanical instability of the leaf structure through external forces such as wind. Wind is one of main environmental factors in petiole lodging. Petiole lodging occurs when a plant is subjected to wind forces greater than the maximum force it can withstand before the petiole breaks, and is the result of wind, lamina area, weight, length, diameter, and mechanical properties of the petiole. Therefore, an understanding all these factors are required to evaluate the petiole lodging resistance of F. japonicum var. japonicum in different coastal environments. Although our locations were close, the wind speeds in each area, including average annual wind speed, maximum wind speed, maximum instantaneous wind speed, and number of occurrences per year with wind speeds of 10 m/s and 20 m/s, were shown to be different. Our analysis indicated that these wind values were the largest in Katsuura according to the Japan Meteorological Agency records for the past 30 years. Katsuura is located in south-eastern Japan, and low-pressure systems moving from west to east in the Pacific Ocean south of the Japanese archipelago often send southerly to easterly winds directly counter clockwise, especially during typhoons. However, the wind weakens to indirectly reach Tateyama and Miura over the Boso Hills, including the mountains on Mt. Atago, Mt. Kiyosumi-myouken, Mt. Nokogiri, and Mt. Iyogatake on the Boso Peninsula; therefore, there was sufficient difference in the strength of the wind between Katsuura and other locations, even in the coastal area.
It is best to study the effect of plants on the wind using AMeDAS meteorological data at the recording stations, but F. japonicum var. japonicum could not occur in them. Therefore, we performed the analysis using the closest population from them. AMeDAS meteorological data were commonly used in Japan to assess both the impact of climate change on crop production and crop management practices in the neighboring areas of the recording stations (Seino, 1993; Hasegawa et al., 2011; Kuwagata et al., 2011; Fukuoka and Yoshimoto, 2012; Ohno et al., 2016), suggesting that there is no significant difference in AMeDAS meteorological data used in this study between the neighboring areas and the AMeDAS recording stations. Comparisons were made to clarify the relationship between the wind effects and the lamina area of F. japonicum var. japonicum. These indicated that the Ubara population near the Katsuura AMeDAS recording station had the smallest lamina area, and there were correlations between wind effects and lamina area. In addition, our results indicated that smaller lamina area was correlated with lighter leaf weight. We found significant differences in lamina area among the populations; lamina area at the lowest wind speed was approximately 1.5 times greater than that at the highest wind speed. Moreover, our results revealed that the lamina area of F. japonicum var. japonicum showed strong correlations with the average annual wind speed, maximum wind speed, and maximum instantaneous wind speed. Whitehead (1962) showed that the lamina area was greater at the lowest wind speed compared with the highest speed, and our results supported with a small effect at lower wind speeds and much greater effect at higher wind speeds. In addition, our anatomical results indicated that variations in lamina area had not involved changes in cell size because there were no significant differences in cell size among localities. Is it possible for F. japonicum var. japonicum to vary the lamina area without changing the cell size? Usukura et al. (1994) reported that F. japonicum var. luchuense had adapted to riverside as a rheophyte by creating narrow lamina without changing cell size, indicating that this report was similar to our results. These indicated that cell size was not involved in the differences in lamina area in F. japonicum group, and therefore, we considered that F. japonicum var. japonicum had adapted to the wind stress by varying the lamina area without changing the cell size.
The petioles of F. japonicum var. japonicum were related to changes in lamina area between locations. In general, petiole growth is one of the most obvious changes to diminish self-shading at the expense of support; lamina can be sent to a higher position as well as adjusting the angle of the lamina on a branch to avoid overlapping with its neighbours (King and Maindonald, 1999; Bell and Galloway, 2007; Sarlikioti et al., 2011; Zhong et al., 2019). Tsukaya et al. (2002) and Tsukaya (2005) suggested that there is expected to be a positive relationship between petiole and lamina lengths because of a higher proportion of overlap of longer laminas in a given space, which is under genetic control. However, our results indicated that F. japonicum var. japonicum showed a weak correlation between petiole and lamina length. The pipe-model theory (Shinozaki et al., 1964a; Shinozaki et al., 1964b) indicates that lamina area increases proportionally with increasing petiole cross-sectional area because the large total cross-sectional area of vascular conduits can meet the high demand for photosynthesis or respiration for large laminas (reviewed in Lehnebach et al., 2018). This is supported by several field studies (Li et al., 2008; Ray and Jones, 2018). Our results also revealed a strong correlation between petiole cross-sectional area and lamina area of F. japonicum var. japonicum. In general, large lamina is not only heavy itself, but also more susceptible to the physical effects of wind, resulting in a greater moment of the upper part of the petiole, which increases the risk of petiole breakage and lodging. In particular, in windy areas, the influence of the wind on the lamina is greater, so the moment of the upper part of the petiole is even greater. Reducing lamina size in strong windy areas is linked to avoid the effects of wind and could adapt without morphological and mechanical changes in petioles, however, smaller lamina reduces the amount of resources obtained through photosynthesis. Conversely, in order to maintain the lamina size in windy areas, it is necessary to increase the strength or the diameter of petioles to withstand the moment at the upper part of them. This is a dilemma between lamina and petiole in windy areas, and our study indicated that F. japonicum var. japonicum had adapted to windy areas by avoiding the influence of the wind by sacrificing the lamina size without changing the petiole.
Some plant species show resistance to mechanical stress by increasing their stem cross-sectional area and tissue strength (Biddington, 1986; Smith and Ennos, 2003; Anten et al., 2010). This quantifies the distribution of mass in a cross-section with respect to the centre of inertia, which describes the important effect of the size and geometry of the cross-section in mechanics (Mahley et al., 2018). However, our mechanical results for F. japonicum var. japonicum showed that the bending strength and second moment of inertia were not significantly different among populations, and breaking load increased with thicker petioles. This shows that the petiole becomes thicker by increasing similar supportive tissues such as xylem, sclerenchyma, and collenchyma. These findings implied that the petiole of F. japonicum var. japonicum would be thicker in the most wind stress locality (Ubara) in our study, but our results did not support this scenario. Some studies have indicated avoidance can occur by a reduction in stem or petiole thickness and flexural rigidity (Jaffe et al., 1984; Cordero, 1999; Liu et al., 2007; Wang et al., 2008; Anten et al., 2010; Paul-Victor and Rowe, 2011). In fact, large plant species, such as trees, are restricted in their ability to bend as they carry heavy loads (Smith and Ennos, 2003; Anten et al., 2009), but small plants are less restricted in this respect and it may be a better strategy to avoid stress (Niklas, 1996; Paul-Victor and Rowe, 2011). F. japonicum var. japonicum is relatively large herbaceous plant and may be adapted to the highest wind environments through stress-avoidance strategies. Therefore, additional anatomical research on F. japonicum var. japonicum plays an important role in linking our results with anatomical characteristics.
How plants adapt to fluctuating external forces depends on the strength, frequency, and structure of the plant. Moderate dwarfing and elongation can enhance resistance to wind and rain, and increase lodging resistance in plant species (Jaffe et al., 1984; Cooper and Mendiola, 2004; Liu et al., 2007). Furthermore, plants exposed to wind are suggested to have an adaptive advantage of reducing wind damage due to their lower height compared to plants developed in still air (Russell and Grace, 1978; Telewski and Jaffe, 1986). Zhang et al. (2021) reported changes in the overall morphology of four plant species, Artemisia ordosica Krasch (Asteraceae), Caragana intermedia Kuang (Fabaceae), Agriophyllum squarrosum (L.) Moq. (Chenopodiaceae), and Salsola ruthenica Iljin (Chenopodiaceae), among three wind treatments in a field experiment under wind conditions with a monthly average wind speed of approximately 2 m/s and a maximum wind speed of 14.4 m/s in May. In this study, F. japonicum var. japonicum was shown to adapt to high wind environments by reducing lamina area and the length/cross-sectional area ratio of the leaf stalk as wind speed increased. Three-point bending tests were conducted assuming lodging due to strong winds in this study. However, the petiole affected by wind also experience twisting in addition to bending (Langer et al., 2022). In the future, it will be possible to clarify how petiole of F. japonicum var. japonicum avoid lodging when subjected to wind loads by evaluating mechanical properties other than bending strength.
F. japonicum var. japonicum is widely distributed along the coastal areas of Japan (Koyama, 1995), and there are meteorological recording stations for AMeDAS at various locations in these areas. Further studies of F. japonicum var. japonicum populations at sites with similar wind speeds in Japan are required to clarify morphological changes. This study only partly investigated the strategies used by plants against wind stress by linking the leaf morphology of F. japonicum var. japonicum and meteorological information in the field using populations near the meteorological recording stations of AMeDAS. Evaluation of morphological traits other than leaves is also important for understanding the environmental adaptations of this plant. F. japonicum var. japonicum have long scapes, from 30 to 75 cm, that support the inflorescence (Koyama, 1995). Although their radical leaves remain exposed to wind stress for a long period, their scapes appear for a shorter period than the petioles. Investigation into whether differences in the emergence period reflect the differences in adaptive morphology between radical leaves and scapes is required. In addition, Khan et al. (2016) reported that root architecture plays an important role in plant anchorage capability to withstand toppling and overturning by wind loading. Wind could induce thigmomorphogenesis in plants by altering root architecture (Chehab et al., 2009), and the effects of strong winds could increase root biomass allocation (Onoda and Anten, 2011). Whether strong winds also affect the root architecture of F. japonicum var. japonicum requires further investigation. Addressing these two areas would provide a greater understanding of the morphological and mechanical traits of scapes and roots in F. japonicum var. japonicum among populations with different wind stresses in the coastal areas.
Data availability statement
The original contributions presented in the study are included in the article/Supplementary Material. Further inquiries can be directed to the corresponding author.
Author contributions
MS, TM, and TF contributed to conception and design of the study. MS and TM organized the database. MS performed the statistical analysis. TF wrote the first draft of the manuscript. All authors contributed to the article and approved the submitted version.
Funding
This work was partly supported by JSPS KAKENHI Grant Number 21K06324.
Acknowledgments
We thank Drs. M. Iijima, Y. Nakajima and S. Yoshizaki for advising of our study. We also thank two reviewers for kindly comments.
Conflict of interest
The authors declare that the research was conducted in the absence of any commercial or financial relationships that could be construed as a potential conflict of interest.
Publisher’s note
All claims expressed in this article are solely those of the authors and do not necessarily represent those of their affiliated organizations, or those of the publisher, the editors and the reviewers. Any product that may be evaluated in this article, or claim that may be made by its manufacturer, is not guaranteed or endorsed by the publisher.
Supplementary material
The Supplementary Material for this article can be found online at: https://www.frontiersin.org/articles/10.3389/fpls.2023.1182266/full#supplementary-material
References
Anten, N. P. R., Alcala-Herrera, R., Schieving, F., Onoda, Y. (2010). Wind and mechanical stimuli differentially affect leaf traits in Plantago major. New Phytol. 188, 554–564. doi: 10.1111/j.1469-8137.2010.03379.x
Anten, N. P. R., Pawlowski, M., von Wettberg, E., Huber, H. (2009). Interactive effects of spectral shading and mechanical stress on the expression and costs of shade avoidance. Am. Nat. 173, 241–255. doi: 10.1086/595761
Bakr, E. M. (2005). A new software for measuring leaf area, and area damaged by tetranychus urticae Koch. J. Appl. Entomol. 129, 173–175. doi: 10.1111/j.1439-0418.2005.00948.x
Bang, C., Sabo, J. L., Faeth, S. H. (2010). Reduced wind speed improves plant growth in a desert city. PloS One 5, 8. doi: 10.1371/journal.pone.0011061
Bell, D. L., Galloway, L. F. (2007). Plasticity to neighbour shade: fitness consequences and allometry. Funct. Ecol. 21, 1146–1153. doi: 10.1111/j.1365-2435.2007.01327.x
Biddington, N. L. (1986). The effects of mechanically-induced stress in plants–a review. Plant Growth Regul. 4, 103–123. doi: 10.1007/BF00025193
Chehab, E. W., Eich, E., Braam, J. (2009). Thigmomorphogenesis: a complex plant response to mechano-stimulation. J. Exp. Bot. 60, 43–56. doi: 10.1093/jxb/ern315
Cooper, R. L., Mendiola, T. (2004). Registration of 10 determinate semidwarf soybean germplasm lines. Crop Sci. 44, 699–700. doi: 10.2135/cropsci2004.6990
Cordero, R. A. (1999). Ecophysiology of Cecropia schreberiana saplings in two wind regimes in an elfin cloud forest: growth, gas exchange, architecture and stem biomechanics. Tree Physiol. 19, 153–163. doi: 10.1093/treephys/19.3.153
Coutand, C., Julien, J. L., Moulia, B., Mauget, J. C., Guitard, D. (2000). Biomechanical study of the effect of a controlled bending on tomato stem elongation: global mechanical analysis. J. Exp. Bot. 51, 1813–1824. doi: 10.1093/jexbot/51.352.1813
de Langre, E. (2008). Effects of wind on plants. Annu. Rev. Fluid Mech. 40, 141–168. doi: 10.1146/annurev.fluid.40.111406.102135
Devkota, P. H., Tsushiro, K., Watanabe, T. (2022). Bioactive phenolic compounds from the flowers of Farfugium japonicum (L.) kitam. var. giganteum (Siebold et zucc.) kitam. (Asteraceae). Nat. Prod. Res. 36, 4036–4039. doi: 10.1080/14786419.2021.1903004
Endalew, A. M., Debaer, C., Rutten, N., Vercammen, J., Delele, M. A., Ramon, H., et al. (2011). Modelling the effect of tree foliage on sprayer airflow in orchards. Boundary Layer Meteorol. 138, 139–162. doi: 10.1007/s10546-010-9544-6
Ennos, A. R. (1997). Wind as an ecological factor. Trends Ecol. Evol. 12, 108–111. doi: 10.1016/S0169-5347(96)10066-5
Falster, D. S., Westoby, M. (2003). Leaf size and angle vary widely across species: what consequences for light interception? New Phytol. 158, 509–525. doi: 10.1046/j.1469-8137.2003.00765.x
Fukuoka, M., Yoshimoto, M. (2012). Observation and use of air temperature above and within canopy in crop cultivation experiments. J. Jpn. Crop Sci. 81, 363–371. doi: 10.1626/jcs.81.363
Greenway, H., Munns, R. (1980). Mechanisms of salt tolerance in non-halophytes. Annu. Rev. Plant Physiol. 22, 131–160. doi: 10.1146/annurev.pp.31.060180.001053
Hasegawa, T., Ishimaru, T., Kondo, M., Kuwagata, T., Yoshimoto, M., Fukuoka, M. (2011). Spikelet sterility of rice observed in the record hot summer of 2007 and the factors associated with its variation. J. Agric. Meteorol. 67, 225–232. doi: 10.2480/agrmet.67.4.3
Henry, H. A. L., Thomas, S. C. (2002). Interactive effects of lateral shade and wind on stem allometry, biomass allocation and mechanical stability in Abutilon theophrasti (Malvaceae). Amer. J. Bot. 89, 1609–1615. doi: 10.3732/ajb.89.10.1609
Hsieh, S. F., Hsieh, T. J., El-Shazly, M., Du, Y. C., Wu, C. C., Hwang, T. L., et al. (2012). Chemical constituents from Farfugium japonicum var. formosanum. Nat. Prod. Commun. 7, 435–440. doi: 10.1177/1934578X1200700405
Igathinathane, C., Prakash, V. S. S., Padma, U., Babu, R. G., Womac, A. R. (2006). Interactive computer software development for leaf area measurement. Comput. Electron. Agric. 51, 1–16. doi: 10.1016/j.compag.2005.10.003
Imaichi, R., Kato, M. (1992). Comparative leaf development of Osmunda lancea and O. japonica (Osmundaceae) : Heterochronic origin of rheophytic stenophylly. Bot. Mag. 105, 199–213. doi: 10.1007/BF02489415
Jaffe, M. J., Forbes, S. (1993). Thigmomorphogenesis: the effect of mechanical perturbation on plants. Plant Growth Regul. 12, 313–324. doi: 10.1007/BF00027213
Jaffe, M. J., Telewski, F. W., Cooke, P. W. (1984). Thigmomorphogenesis: on the mechanical properties of mechanically perturbed bean plants. Physiol. Plant 62, 73–78. doi: 10.1111/j.1399-3054.1984.tb05925.x
Jones, A., Kang, J. (2015). Development of leaf lobing and vein pattern architecture in the genus Ipomoea (morning glory). Int. J. Plant Sci. 176, 820–831. doi: 10.1086/683277
Kaspari, M., Yanoviak, S. P., Dudley, R., Yuan, M., Clay, N. A. (2009). Sodium shortage as a constraint on the carbon cycle in an inland tropical rainforest. Proc. Natl. Acad. Sci. U.S.A. 106, 19405–19409. doi: 10.1073/pnas.0906448106
Khan, M. A., Gemenet, D. C., Villordon, A. (2016). Root system architecture and abiotic stress tolerance: current knowledge in root and tuber crops. Front. Plant Sci. 7.1584 doi: 10.3389/fpls.2016.01584
Kim, J. Y., Oh, T. H., Kim, B. J., Kim, S. S., Lee, N. H., Hyun, C. G. (2008). Chemical composition and anti-inflammatory effects of essential oil from Farfugium japonicum flower. J. Oleo Sci. 57, 623–628. doi: 10.5650/jos.57.623
King, D. A., Maindonald, J. H. (1999). Tree architecture in relation to leaf dimensions and tree stature in temperate and tropical rain forests. J. Ecol. 87, 1012–1024. doi: 10.1046/j.1365-2745.1999.00417.x
Koyama, H. (1995). “Farfugium,” in Flora of Japan IIIb. Eds. Iwatsuki, K., Yamazaki, T., Boufford, D. E., Ohba, H.(Tokyo: Kodansha), 37–38.
Kumekawa, Y., Miyata, H., Ohga, K., Hayakawa, H., Yokoyama, J., Ito, K., et al. (2013). Comparative analyses of stomatal size and density among ecotypes of Aster hispidus (Asteraceae). Amer. J. Plant Sci. 4, 524–527. doi: 10.4236/ajps.2013.43067
Kuwagata, T., Yoshimoto, M., Ishigooka, Y., Hasegawa, T., Utsumi, M., Nishmori, M., et al. (2011). MeteoCrop DB: an agro-meteorological database coupled with crop models for studying climate change impacts on rice in Japan. J. Agric. Meteorol. 67, 297–306. doi: 10.2480/agrmet.67.4.9
Langer, M., Hegge, E., Speck, T., Speck, O. (2022). Acclimation to wind loads and/or contact stimuli? a biomechanical study of peltate leaves of Pilea peperomioides. J. Exp. Bot. 73, 1236–1252. doi: 10.1093/jxb/erab541
Lehnebach, R., Beyer, R., Letort, V., Heuret, P. (2018). The pipe model theory half a century on: a review. Ann. Bot. 121, 773–795. doi: 10.1093/aob/mcx194
Li, G., Yang, D., Sun, S. (2008). Allometric relationships between lamina area, lamina mass and petiole mass of 93 temperate woody species vary with leaf habit, leaf form and altitude. Funct. Ecol. 22, 557–564. doi: 10.1111/j.1365-2435.2008.01407.x
Liu, Y., Schieving, F., Stuefer, J. F., Anten, N. P. R. (2007). The effects of mechanical stress and spectral shading on the growth and allocation of ten genotypes of a stoloniferous plant. Ann. Bot. 99, 121–130. doi: 10.1093/aob/mcl230
Louf, J. F., Nelson, L., Kang, H., Song, P. N., Zehnbauer, T., Jung, S. (2018). How wind drives the correlation between leaf shape and mechanical properties. Sci. Rep. 8, 16314. doi: 10.1038/s41598-018-34588-0
Mahley, J. N., Pittermann, J., Rowe, N., Baer, A., Watkins, J. E., Schuettpelz, E., et al. (2018). Geometry, allometry and biomechanics of fern leaf petioles: their significance for the evolution of functional and ecological diversity within the pteridaceae. Front. Plant Sci. 9. doi: 10.3389/fpls.2018.00197
Matsui, R., Takei, S., Ohga, K., Hayakawa, H., Yoshida, M., Yokoyama, J., et al. (2013). Morphological and anatomical variations in rheophytic ecotype of violet, Viola mandshurica var. ikedaeana (Violaceae). Amer. J. Plant Sci. 4, 859–865. doi: 10.4236/ajps.2013.44106
Meguro, S., Miyawaki, A. (1994). A study of the relationship between mechanical characteristics and the coastal vegetation among several broad-leaf trees in miura peninsula in Japan. Vegetatio 112, 101–111. doi: 10.1007/BF00044685
Niinemets, Ü., Fleck, S. (2002). Petiole mechanics, leaf inclination, morphology, and investment in support in relation to light availability in the canopy of Liriodendron tulipifera. Oecologia 132, 21–33. doi: 10.1007/s00442-002-0902-z
Niklas, K. J. (1996). Differences between Acer saccharum leaves from open and wind-protected sites. Ann. Bot. 78, 61–66. doi: 10.1006/anbo.1996.0096
Ohga, K., Muroi, M., Hayakawa, H., Yokoyama, J., Ito, K., Tebayashi, S., et al. (2012). Comparative morphology and anatomy of non-rheophytic and rheophytic types of Adenophora triphylla var. japonica (Campanulaceae). Amer. J. Plant Sci. 3, 805–809. doi: 10.4236/ajps.2012.36097
Ohga, K., Muroi, M., Hayakawa, H., Yokoyama, J., Ito, K., Tebayashi, S., et al. (2013). Coastal adaptation of Adenophora triphylla (Thunb.) a. DC. var. japonica (Regel) h. hara (Campanulaceae). Amer. J. Plant Sci. 4, 596–601. doi: 10.4236/ajps.2013.43078
Ohno, H., Sasaki, K., Ohara, G., Nakazono, K. (2016). Development of grid square air temperature and precipitation data compiled from observed, forecasted, and climatic normal data. Cli. Biosph. 16, 71–79. doi: 10.2480/cib.J-16-028
Onoda, Y., Anten, N. P. R. (2011). Challenges to understand plant responses to wind. Plant Signal. Behav. 6, 1057–1059. doi: 10.4161/psb.6.7.15635
Paul-Victor, C., Rowe, N. (2011). Effect of mechanical perturbation on the biomechanics, primary growth and secondary tissue development of inflorescence stems of Arabidopsis thaliana. Ann. Bot. 107, 209–218. doi: 10.1093/aob/mcq227
Poulsen, A. D., Nordal, I. A. (2005). A phenetic analysis and revision of guineo-congolean rain forest taxa of Chlorophytum (Anthericaceae). Bot. J. Linn. 148, 1–20. doi: 10.1111/j.1095-8339.2005.00386.x
Ray, D. M., Jones, C. S. (2018). Scaling relationships and vessel packing in petioles. Amer. J. Bot. 105, 1–10. doi: 10.1002/ajb2.1054
Roden, J. S., Pearcy, R. W. (1993). Effect of leaf flutter on the light environment of poplars. Oecologia 93, 201–207. doi: 10.1007/BF00317672
Russell, G., Grace, J. (1978). The effect of wind-speed on the growth of grasses. J. Appl. Ecol. 16507–514. doi: 10.2307/2402525
Sack, L., Dietrich, E. M., Streeter, C. M., Sánchez-Gómez, D., Holbrook, N. M. (2008). Leaf palmate venation and vascular redundancy confer tolerance of hydraulic disruption. Proc. Natl. Acad. Sci. U.S.A. 105, 1567–1572. doi: 10.1073/pnas.0709333105
Sarlikioti, V., de Visser, P. H. B., Marcelis, L. F. M. (2011). Exploring the spatial distribution of light interception and photosynthesis of canopies by means of a functional-structural plant model. Ann. Bot. 107, 875–883. doi: 10.1093/aob/mcr006
Seino, H. (1993). An estimation of distribution of meteorological elements using GIS and AMeDAS data. J. Agric. Meteorol. 48, 379–383. doi: 10.2480/agrmet.48.379
Shiba, M., Tate, T., Fukuda, T. (2021). Rheophytic adaptation of Eurya japonica thunb. (Ternstroemiaceae). Int. J. Biol. 13, 65–73. doi: 10.5539/ijb.v13n2p65
Shiba, M., Tate, T., Fukuda, T. (2022). Leaf sdaptation of Eurya japonica thunb. (Pentaphylacaceae) in coastal area. J. Plant Stud. 11, 31–41. doi: 10.5539/jps.v11n1p31
Shinozaki, K., Yoda, K., Hozumi, K., Kira, T. (1964a). A quantitative analysis of plant form - the pipe model theory. i. basic analyses. Jpn. J. Ecol. 14, 97–105. doi: 10.18960/seitai.14.3_97
Shinozaki, K., Yoda, K., Hozumi, K., Kira, T. (1964b). A quantitative analysis of plant form-the pipe model theory. II. further evidence of the theory and its application in forest ecology. Jpn. J. Ecol. 14, 133–139. doi: 10.18960/seitai.14.4_133
Smith, V. C., Ennos, A. R. (2003). The effects of air flow and stem flexure on the mechanical and hydraulic properties of the stems of sunflowers Helianthus annuus l. J. Exp. Bot. 54, 845–849. doi: 10.1093/jxb/erg068
Sunami, T., Ohga, K., Muroi, M., Hayakawa, H., Yokoyama, J., Ito, K., et al. (2013). Comparative analyses of hairless-leaf and hairy-leaf type individuals in Aster hispidus var. insularis (Asteraceae). J. Plant Stud. 2, 1–6. doi: 10.5539/jps.v2n1p1
Takenaka, A. (1994). Effects of leaf blade narrowness and petiole length on the light capture efficiency of a shoot. Ecol. Res. 9, 109–114. doi: 10.1007/BF02347485
Takizawa, E., Shiba, M., Yoshizaki, S., Fukuda, T. (2023). The stomatal study of introduced species, Ligustrum lucidum aiton (Oleaceae), spreads into coastal areas of Japan with adaptive features. J. Plant Stud. 12, 24–36. doi: 10.5539/jps.v12n1p24
Takizawa, E., Tate, T., Shiba, M., Ishii, C., Yoshizaki, S., Fukuda, T. (2022). Coastal adaptation of Ligustrum japonicum thunb. (Oleaceae): a case study of stomatal adaptation pattern into coastal forests. J. Jpn. Soc Coast. For. 21, 1–8.
Telewski, F. W., Jaffe, M. J. (1986). Thigmomorphogenesis: field and laboratory studies of Abies fraseri in response to wind or mechanical perturbation. Physiol. Plant 66, 211–218. doi: 10.1111/j.1399-3054.1986.tb02411.x
Telewski, F. W., Pruyn, M. L. (1998). Thigmomorphogenesis: a dose response to flexing in Ulmus americana l. seedlings. Tree Physiol. 18, 65–68. doi: 10.1093/treephys/18.1.65
Tsukaya, H. (2005). Leaf shape: genetic controls and environmental factors. Int. J. Dev. Biol. 49, 547–555. doi: 10.1387/ijdb.041921ht
Tsukaya, H., Kozuka, T., Kim, G. T. (2002). Genetic control of petiole length in Arabidopsis thaliana. Plant Cell Physiol. 43, 1221–1228. doi: 10.1093/pcp/pcf147
Tunala Hayakawa, H., Minamiya, Y., Gale, S. W., Yokoyama, J., Arakawa, R., Fukuda, T., et al. (2012). Foliar adaptations in Aster hispidus var. insularis (Asteraceae). J. Plant Stud. 1, 19–25. doi: 10.5539/jps.v1n2p19
Ueda, R., Minamiya, Y., Hirata, A., Hayakawa, H., Muramatsu, Y., Saito, M., et al. (2012). Morphological and anatomical analyses of rheophytic Rhododendron ripense makino (Ericaceae). Plant Species Biol. 27, 233–240. doi: 10.1111/j.1442-1984.2011.00345.x
Usukura, M., Imaichi, R., Kato, M. (1994). Leaf morphology of a facultative rheophyte, Farfugium japonicum var. luchuense (Compositae). J. Plant Res. 107, 263–267. doi: 10.1007/BF02344253
van Steenis, C. G. G. J. (1981). Rheophyte of the world (Alphen aan den Rijn: Sijthoff and Noordhoff).
Vogel, S. (2009). Leaves in the lowest and highest winds: temperature, force and shape. New Phytol. 183, 13–26. doi: 10.1111/j.1469-8137.2009.02854.x
Wang, Y. H., He, W. M., Dong, M., Yu, F. H., Zhang, L. L., Cui, Q. G., et al. (2008). Effects of shaking on the growth and mechanical properties of Hedysarum laeve may be independent of water regimes. Int. J. Plant Sci. 169, 503–508. doi: 10.1086/528750
Whitehead, F. H. (1962). Experimental studies of the effect of wind on plant growth and anatomy II. Helianthus annuus. New Phytol. 61, 59–62. doi: 10.1111/j.1469-8137.1962.tb06274.x
Wunnenberg, J., Rjosk, A., Neinhuis, C., Lautenschläger, T. (2021). Strengthening structures in the petiole-lamina junction of peltate leaves. Biomimetics 6, 25. doi: 10.3390/biomimetics6020025
Yamada, Y., Hayakawa, H., Minamiya, Y., Ito, K., Shibayama, Z., Arakawa, R., et al. (2011). Comparative morphology and anatomy of rheophytic Aster microcephalus (Miq.) franch. et sav. var. ripensis makino (Asteraceae). J. Phytogeogr. Taxon. 59, 35–42. doi: 10.24517/00053453
Yamada, T., Suzuki, E., Yamakura, T. (1999). Scaling of petiole dimensions with respect to leaf size for a tropical tree, Scaphium macropodum (Sterculiaceae), in Borneo. J. Plant Res. 112, 61–66. doi: 10.1007/PL00013859
Yamaguchi, H., Yahara, T. (1989). Analysis on pollen flow in a hybridizing population between Farfugium hiberniflorum and F. japonicum (Asteraceae: senecioneae): derivation of an empirical formula describing frequency of hybridization as a function of interspecific plant distance. Plant Species Biol. 4, 101–106. doi: 10.1111/j.1442-1984.1989.tb00051.x
Yokoyama, N., Hayakawa, H., Matsuyama, K., Muroi, M., Ohga, K., Ito, K., et al. (2012). Morphological and molecular analyses of rheophytic Rhododendron ripense and its allied dryland species R. macrosepalum (Ericaceae). Environ. Control Biol. 50, 305–312. doi: 10.2525/ecb.50.305
Yun, Y. H., Kwon, H. W., Ahn, H. S., Kim, S. H. (2015). First report of leaf rust caused by Puccinia caricis in Farfugium japonicum in Korea. Mycobiology 43, 351–353. doi: 10.5941/MYCO.2015.43.3.351
Zhang, S., Liu, G., Cui, Q., Huang, Z., Ye, X., Cornelissen, J. H. C. (2021). New field wind manipulation methodology reveals adaptive responses of steppe plants to increased and reduced wind speed. Plant Methods 17, 5. doi: 10.1186/s13007-020-00705-2
Keywords: AMeDAS, Farfugium japonicum var. japonicum, leaf, petiole, wind
Citation: Shiba M, Mizuno T and Fukuda T (2023) Effect of strong wind on laminas and petioles of Farfugium japonicum (L.) Kitam. var. japonicum (Asteraceae). Front. Plant Sci. 14:1182266. doi: 10.3389/fpls.2023.1182266
Received: 08 March 2023; Accepted: 27 April 2023;
Published: 29 June 2023.
Edited by:
Olga Speck, University of Freiburg, GermanyReviewed by:
Nicholas Peter Rowe, UMR5120 Botanique et modélisation de l’architecture des plantes et des végétations (AMAP), FranceAnita Roth-Nebelsick, Staatliches Museum für Naturkunde Stuttgart, Germany
Copyright © 2023 Shiba, Mizuno and Fukuda. This is an open-access article distributed under the terms of the Creative Commons Attribution License (CC BY). The use, distribution or reproduction in other forums is permitted, provided the original author(s) and the copyright owner(s) are credited and that the original publication in this journal is cited, in accordance with accepted academic practice. No use, distribution or reproduction is permitted which does not comply with these terms.
*Correspondence: Tatsuya Fukuda, dGZ1a3VkYUB0Y3UuYWMuanA=