- 1Cereal Institute, Henan Academy of Agricultural Sciences, Zhengzhou, China
- 2State Key Laboratory of North China Crop Improvement and Regulation/Key Laboratory of Crop Growth Regulation of Hebei Province, College of Agronomy, Hebei Agricultural University, Baoding, China
- 3Key Laboratory of North China Water-saving Agriculture, Ministry of Agriculture and Rural Affairs, Baoding, Hebei, China
Introduction: High rainfall and excessive urea application are counterproductive to summer maize growth requirements and lower grain yield and water/nitrogen (N) use efficiency. The objective of this study was to determine whether ETc irrigation based on summer maize demand and reduced nitrogen rate in the Huang Huai Hai Plain increased water and nitrogen use efficiency without sacrificing yield.
Methods: To achieve this, we conducted an experiment with four irrigation levels [ambient rainfall (I0) and 50% (I1), 75% (I2), and 100% (I3) of actual crop evapotranspiration (ETc)] and four nitrogen rates [no nitrogen fertilizer (N0), recommended nitrogen rate of urea (NU), recommended nitrogen rate of blending controlled-release urea with conventional urea fertilizer (BCRF) (NC), and reduced nitrogen rate of BCRF (NR)] in 2016–2018.
Results: The results show that reduced irrigation and nitrogen rate reduced Fv/Fm, 13C-photosynthate, and nitrogen accumulation both in the kernel and plant. I3NC and I3NU accumulated higher 13C-photosynthate, nitrogen, and dry matter. However, 13C-photosynthate and nitrogen distribution to the kernel was decreased from I2 to I3 and was higher in BCRF than in urea. I2NC and I2NR promoted their distribution to the kernel, resulting in a higher harvest index. Compared with I3NU, I2NR increased root length density by 32.8% on average, maintaining considerable leaf Fv/Fm and obtaining similar kernel number and kernel weight. The higher root length density of I2NR of 40–60 cm promoted 13C-photosynthate and nitrogen distribution to the kernel and increased the harvest index. As a result, the water use efficiency (WUE) and nitrogen agronomic use efficiency (NAUE) in I2NR increased by 20.5%–31.9% and 11.0%–38.0% than that in I3NU, respectively.
Discussion: Therefore, 75%ETc deficit irrigation and BCRF fertilizer with 80% nitrogen rate improved root length density, maintained leaf Fv/Fm in the milking stage, promoted 13C-photosynthate, and distributed nitrogen to the kernel, ultimately providing a higher WUE and NAUE without significantly reducing grain yield.
1 Introduction
Population expansion and climate change are generating water scarcity worldwide. As 70% of the fresh water supply is used by agriculture, water scarcity is a threat to the sustainability of agriculture (Mishra et al., 2021; Salehi, 2022). Increasing agricultural water use efficiency is a priority for food security and an effective way to mitigate water scarcity (Wang et al., 2019a). This problem is particularly serious in China, which hosts 6% of the world freshwater resources and feeds a significant amount of the world population.
With the greatest total output and acreage in China, maize is the most widely planted crop in the Huang-Huai-Hai Plain (Figure 1A), accounting for 35% of the national maize planting acreage and more than 40% of the corn grain output in China (Shu et al., 2021). Grown in the rainy season, summer maize received 310.0–536.4 mm of rainfall from 1961 to 2015 but only 115.5–166.0 mm of effective rainfall, which is significantly less than it requires (312.7–389.1 mm). Due to this misalignment between the rainfall and the maize’s water demand (Tuan et al., 2011), local farmers must irrigate their crops two or three times each year to increase the maize yield. Flood irrigation averaging 90–100 mm of water increases evaporation loss and nitrogen leaching, reducing water and nitrogen use efficiencies while polluting the environment (Guo et al., 2010; Eekhout et al., 2018; Yu et al., 2019; Hou et al., 2021).
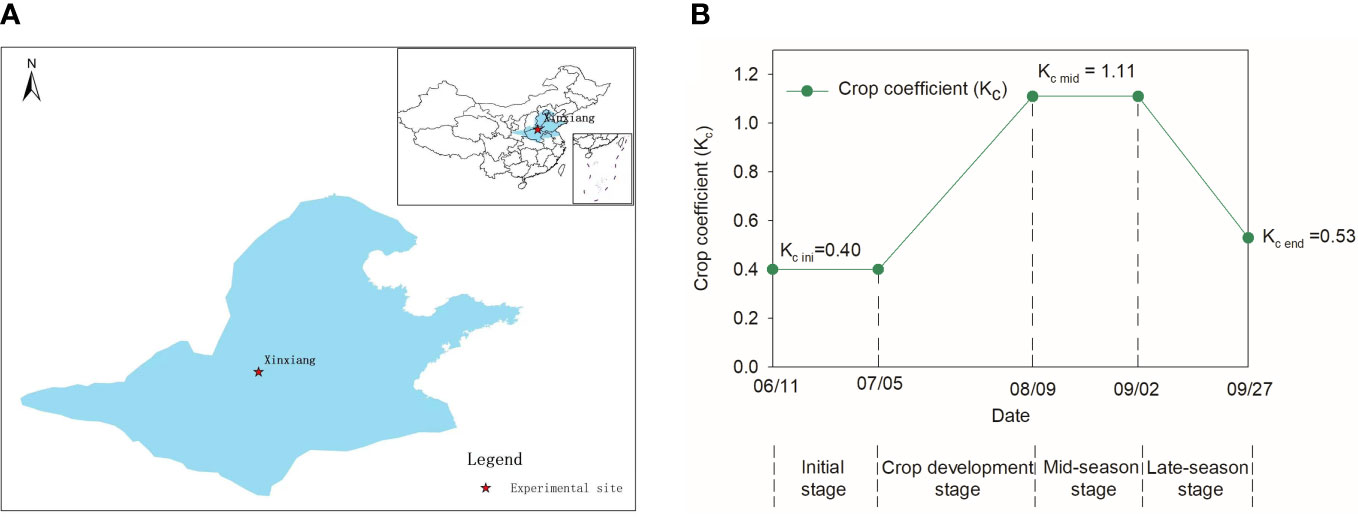
Figure 1 The experimental site in Huang-Huai-Hai maize region (blue area) of China (A) and the single crop coefficients Kc for the various development stages for summer maize in this experiment (B).
Deficit irrigation maximizes water productivity and achieves water delivery equal to or better than full irrigation cultivation (Zhang, 2003b; Geerts and Raes, 2009; Comas et al., 2019; Sandhu et al., 2019). Deficit irrigation reduces soil evaporation and regulates leaf stomatal opening to reduce transpiration water loss, maintaining high photosynthetic efficiency (Ullah et al., 2019; Jovanovic et al., 2020). Understanding maize water requirements is the basis of deficit irrigation strategy. Preliminary research demonstrated that maize water requirements varied by variety, weather, and soil conditions and that all these variables should be addressed when making irrigation decisions (Peng et al., 2019; Masupha and Moeletsi, 2020; Mirhashemi and Panahi, 2021). Nevertheless, the current irrigation strategy is mainly based on the field capacity (Guo et al., 2015; Liu et al., 2022a), ignoring crop requirements and meteorological conditions. Local stress irrigation based on surface irrigation (i.e., border irrigation) has a high single irrigation volume (approximately 60–120 mm) but low irrigation frequency, which is performed by two irrigations at the sowing and flowering stages (Wang et al., 2020). The high irrigation level induces higher soil evaporation between plants and the water leachate (Srivastava et al., 2020; Yi et al., 2022). Currently, the most widely used conventional irrigation system is not compatible with advanced and efficient irrigation equipment, such as drip irrigation, sprinkler irrigation, or low-pressure pipe irrigation methods necessary to cover an area of 23,191×103 ha, 30.1% of the total irrigated area in 2020 in China (Ministry of Water Resources, 2020). It is critical, therefore, to investigate modern agricultural irrigation systems that are geared to water conservation, high land efficiency, and labor efficiency.
The crop evapotranspiration (ETc) metric based on the FAO56 whitepaper is commonly used to make irrigation decisions, since it takes both crop requirements (growth phases) and climatic circumstances into account (Pereira et al., 2020). It also provides a precise and flexible irrigation schedule for an automatic or digital irrigation system, which is an excellent approach to cut labor expenses and water loss, and is accepted and employed by a growing number of farmers (Cancela et al., 2015). The majority of irrigation decision-making research has been conducted using models (Mancosu et al., 2016; López-Urrea et al., 2020), and the field performance of irrigation based on ETc needs additional investigation. ETc irrigation applied at 100% increases maize growth, net photosynthetic rate, and accumulation of dry matter (Guo et al., 2022). However, the effect of ETc irrigation on photosynthetic transport, root length density, and water-saving potential of deficit ETc irrigation on maize are currently unknown.
Numerous studies have demonstrated that soil moisture content and nitrogen availability have a complicated effect on crop yield (Sandhu et al., 2019). Irrigation that is appropriate for the soil could improve nitrogen use efficiency (NUE) by increasing nitrogen accumulation, translocation, and distribution (Yan et al., 2019). Blending controlled-release urea with conventional urea (BCRF) is both environment and economic friendly and has a wide range of applications (Vejan et al., 2021; Guo et al., 2022). Compared with urea, BCRF reduces N2O emissions (Lyu et al., 2021) and nitrogen leachate (Adu-Gyamfi et al., 2019), meets crop nitrogen demands (Zheng et al., 2020), and increases photosynthetic efficiency (Guo et al., 2022), crop yield, and NUE (Zheng et al., 2016; Zhu and Zhang, 2016; Vejan et al., 2021). As a result, the optimal nitrogen rate for BCRF and ETc irrigation levels may differ from the optimal nitrogen rate under standard irrigation and nitrogen management methods. While earlier research was focused on crop grain yield, water use efficiency (WUE), and NUE, the interplay between ETc irrigation and BCRF on maize performance is less studied.
The purpose of this study was to (1) examine how reduced water and nitrogen input increase water and nitrogen use efficiency without compromising yield, (2) investigate the effect of deficit irrigation based on ETc and BCRF on photosynthate accumulation and distribution, and (3) investigate the interaction between deficit ETc irrigation and BCRF-dependent reduced nitrogen rates on WUE and NUE, to lay the groundwork for more precise irrigation and fertilization maize crop management strategies.
2 Materials and methods
2.1 Site and weather description
From 2016 to 2018, studies were conducted in the Henan Academy of Agricultural Sciences experimental base in Yuanyang, Henan Province, China (113°42′28.7′′N, 35°0′13.3′′E), 78 m above sea level). The regional climate is subhumid, warm temperate, continental, monsoon, and features four distinct seasons. This is a typical location on the Huang-Huai-Hai Plain (Figure 1A). The ETc was calculated using weather data from 1983 to 2013 received from China’s National Meteorological Information Center (climate data, Figure 2). Precipitation totaled 349.4, 193.6, and 239.4 mm during the maize growth period in 2016, 2017, and 2018, respectively.
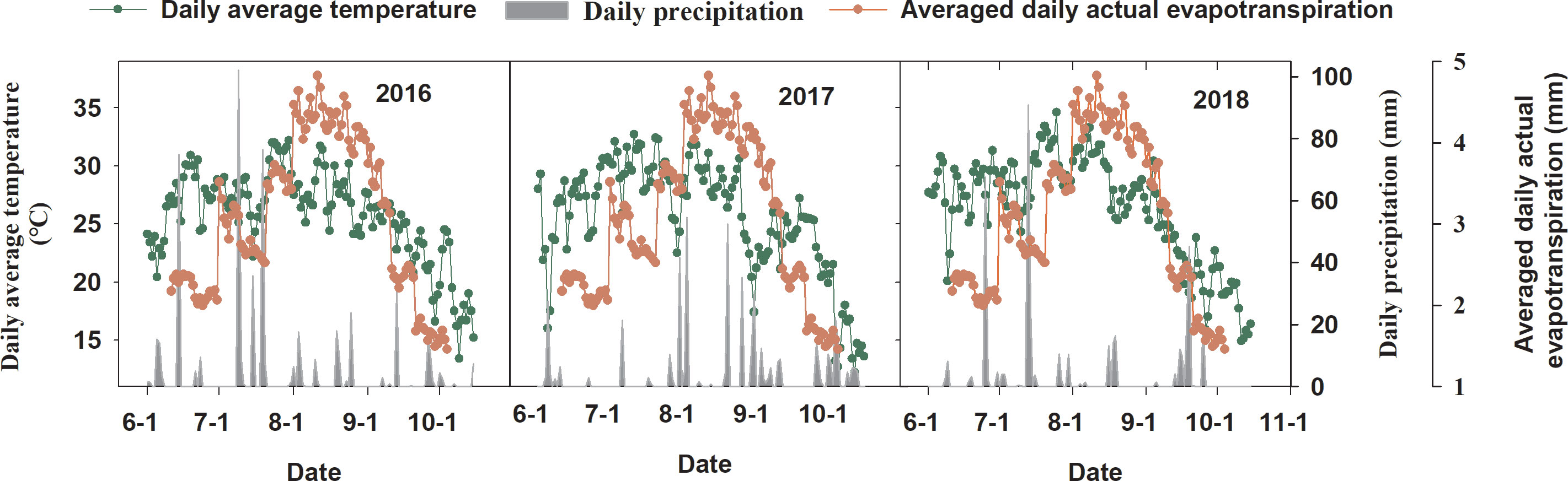
Figure 2 Daily mean temperature (°C) and precipitation (mm) during the summer maize season in 2016–2018 and the average daily actual evapotranspiration from 1983 to 2013.
The regional soil is fluvo-aquic, with 13.44 g kg−1 organic matter, 73.8 g kg−1 available N, 50.2 mg kg−1 available phosphate, and 134.5 mg kg−1 available potassium under a rainproof shelter and 13.86 g kg−1 organic matter, 86.13 g kg−1 available N, 53.5 mg kg−1 available phosphate, and 146.0 mg kg−1 available potassium in the field.
2.2 Experimental design
The experiment used a randomized complete block design with 16 treatments (4 irrigation levels and 4 nitrogen fertilizer treatments) in triplicate. The four irrigation levels were ambient rainfall (I0), 50% ETc (I1), 75% ETc (I2), and 100% ETc (I3). The four nitrogen fertilizer treatments were as follows: no fertilizer (N0), urea with the recommended nitrogen rate (240 kg N ha−1, NU), BCRF with the recommended nitrogen rate (240 kg N ha−1, NC), and BCRF with a reduced nitrogen rate (200 kg N ha−1, NR).
To avoid the potential impact of unforeseen rainfall on the experiment, maize, the experiment of I1, I2, and I3 was performed in microplots beneath an autonomously triple-folding rainproof shelter in 2016 and 2017. When it rained, the rainproof shelter was opened to cover the plots and keep the rain off. At other times, the rainproof shelter was stored adjacent to the experimental plots in an unoccupied location. The plants in I0 treatment received only rainfall with no supplemental irrigation from 2016 to 2018. In 2018, the experiment was conducted in a field 300 m away. That is, the water input in I0 was only rainfall in three maize seasons; the water input in I1, I2, and I3 in 2016 and 2017 under rainproof shelter was only irrigation; while water input in I1, I2, and I3 in field in 2018 was the sum of irrigation and rainfall.
The microplot was 2.9 m × 1.9 m in size, and the field plot was 4.2 m × 6.7 m in size. In 2016, 2017, and 2018, summer maize (Zhengdan 309, a national maize variety suitable for harvesting grain mechanically) was planted on June 16, June 16, and June 17 and harvested on September 30, October 3, and October 5, respectively. A precision irrigation equipment irrigated the maize. The previous crop, winter wheat, was irrigated fully and fertilized with no nitrogen to achieve identical soil moisture and nitrogen concentrations between microplots before planting maize. The maize was planting in 75,000 plans ha−1 with 60 cm plant row spacing. Pest, disease, and weed control strategies were similar to those used regionally.
2.2.1 Irrigation regime
Actual crop evapotranspiration (ETc) was determined by the following formula:
ET0 is averaged daily reference evapotranspiration (mm), calculated using daily meteorological data from 1983 to 2013 (from the National Meteorological Information Center of China) with the ET0 calculator (Food and Agriculture Organization (FAO56). Kc is the crop coefficient, determined by the FAO56 guidelines. The lengths of the crop development phases for the initial stage, development stage, mid-season stage, and late-season stage were 26, 34, 24, and 20 days, respectively, according to FAO56. The Kc values for the initial stage, mid-season stage, and end of the late-season stage were 0.4, 1.11, and 0.53, respectively (Figure 1B).
The irrigation amounts for I1, I2, and I3 were calculated as follows:
Pe is the effective precipitation amount (mm), and ETc is the actual crop evapotranspiration. P = 0 for I1, I2, and I3 in 2016 and 2017 under rain shelter when it rains. Pe was calculated as Pe=a×P, in which a was 0, 1.0, and 0.75 when the precipitation<5 mm, 5 mm≤precipitation ≤ 50 mm, and precipitation >50 mm, respectively.
For both the microplot and field tests, 44.1 mm of water was irrigated after sowing to enable maize seedling emergence. Aside from the sowing irrigation, the plants were irrigated at the V6, V12, VT, R2, and R4 stages. The irrigation amounts and the precipitation levels at different irrigation levels in 2016–2017 and 2018 are shown in Tables 1, 2.
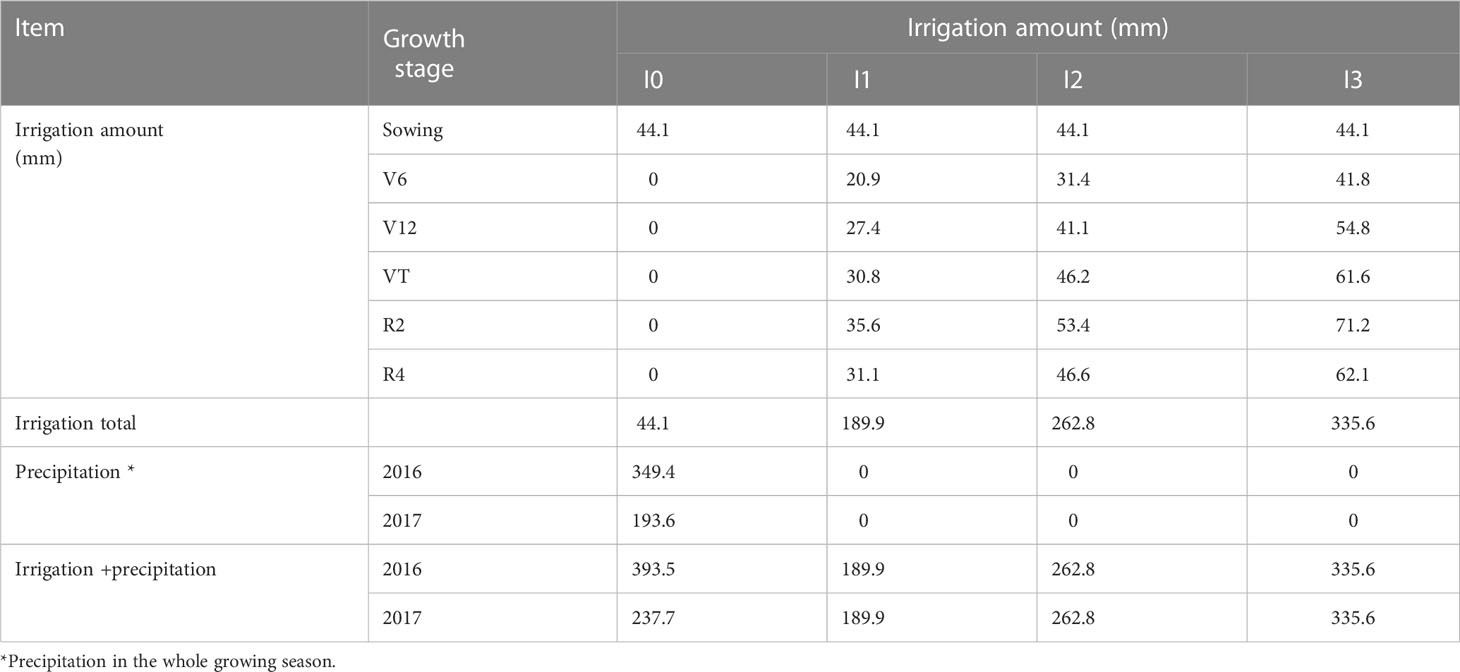
Table 1 Irrigation volume of different irrigation levels at various summer maize growth stages in 2016 and 2017.
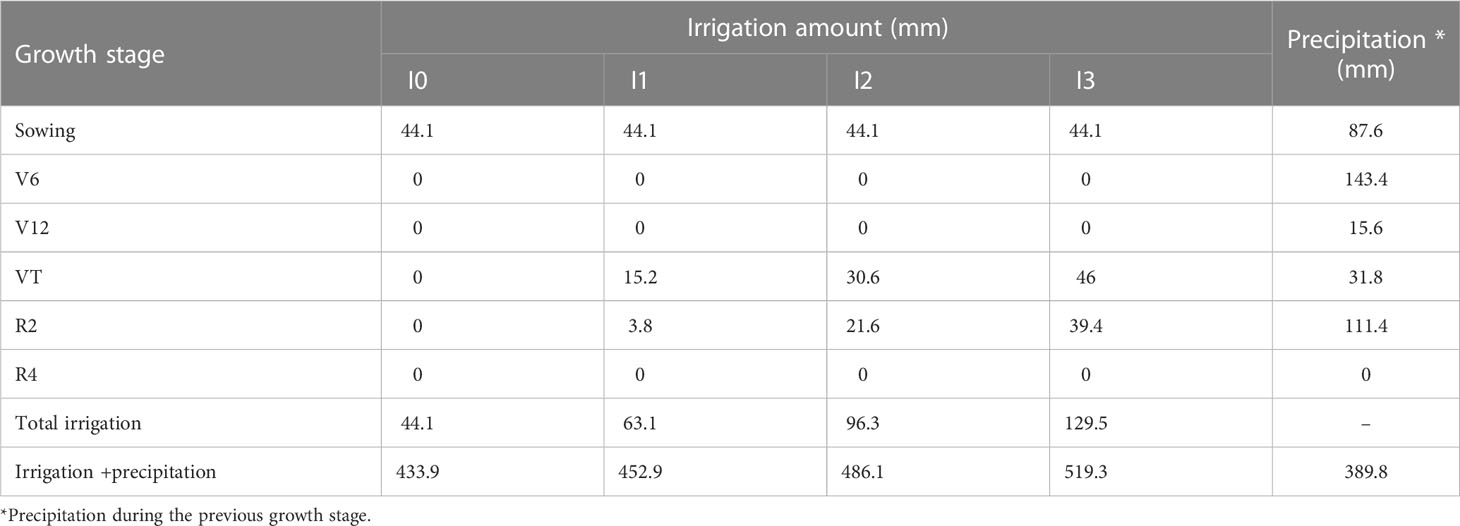
Table 2 Precipitation and irrigation volume of different irrigation levels at various summer maize growth stages in 2018.
2.2.2 Fertilizer management
Four fertilizer treatments were tested: (i) a quick-release urea (46% N) with a 240 kg N kg−1 application rate, (ii) a BCRF (26% N, 10% P2O5, and 9% K2O; Kingenta; controlled-release fertilizer: quick-acting fertilizer = 1:1) with a 240 kg N kg−1 application rate (NC), (iii) a BCRF with a 200 kg N kg−1 application rate (NR), and (Srivastava et al.) no nitrogen fertilizer (N0). All plots had the same phosphate and potassium rates of 150 kg P2O5 kg−1 and 120 kg K2O kg−1, respectively. Calcium superphosphate (12.0% P2O5) and potassium chloride (52.0% K2O) fertilizers were used to compensate for the deficiency of phosphate and potassium. As a basal fertilizer, 100% BCRF, 50% urea, 100% calcium superphosphate, and potassium chloride fertilizer were applied. At the tasseling stage (VT), urea (50%) was applied as a topdressing fertilizer. Winter wheat was planted prior to maize, and it was fertilized with nitrogen-free fertilizer to maintain a same soil nitrogen content prior to planting maize.
2.3 Sampling, measurements, and calculations
2.3.1 Meteorological data
Meteorological data, including rainfall, temperature, air humidity, and wind speed, were obtained automatically at a station 200 m from the trial site.
2.3.2 Labeling of selected plants with 13CO2
Six representative plants from each plot were selected and labeled with 13CO2 on silking stage. Ear leaves of each plant were covered in 0.1-mm thick mylar plastic bags, which permitted up to 95% of natural sunlight intensity. After sealing the bags at the base with Plasticine, 50 ml of 13CO2 was injected. After 60 min, the 13CO2 in each bag was extracted using a KOH washer to remove any residual radioactive 13CO2, and the plastic bag was removed (Liu et al., 2015).
2.3.3 Dry matter, 13C-photosynthate distribution ratio, and nitrogen distribution ratio among plant organs
The labeled plants were collected at physiological maturity and dissected into leaves, stem, sheath, ear bract, cob, and grain. The harvest index was calculated as the ratio of grain dry matter to total plant dry matter. All separated components were oven-dried to a constant weight at 80°C, weighed to determine dry matter (g plant−1), and then milled into a powder. Subsamples of 4 mg were used to determine the isotopic abundance using an Isoprime 100 instrument (Isoprime 100, Cheadle, UK). Subsamples were digested using an H2SO4–H2O2 method (Thomas et al., 1967), and total nitrogen was measured using a continuous flow autoanalyzer (AA3, SEAL Analytical, Germany). The 13C-photosynthate accumulation (13C-AC) and distribution ratio (13C-DR) among different plant organs at physiological maturity (%/plant) and the nitrogen distribution accumulation (N-AC) and distribution ratio (N-DR) were calculated.
2.3.4 Ear leaf Fv/Fm and root length density
Using a continuous excitation fluorometer Pocket Plant Efficiency Analyzer (PEA, Hansatech, UK), the Fv/Fm of six representative ear leaves at the silking stage (R1) and milk stage (R3) were determined under dark conditions for 15 min. Three maize plants in each treatment were selected to evaluate root length density at the anthesis stage. In the 0–60-cm soil layer, a block of soil surrounding the plant (60 cm long, 22 cm wide, 20 cm deep; 26,400 cm3) was removed for each plant sample. The root samples were carefully cleaned of non-root material. Root lengths were measured using WinRHIZO (Regent Instruments Inc., Canada) after the fresh roots were scanned using an Epson Perfection V800 scanner. Root length density was calculated by dividing root length by soil volume (26,400 cm3).
2.3.5 Yield and harvest index
All ears in each plot were collected at the physiological maturity to investigate the yield and yield components. For each harvested ear, the kernel number per plant (KNP) was counted. Three 1,000-kernel samples were oven-dried at 80°C to a consistent weight and weighed to determine the kernel weight (KW). To determine grain yield, all kernels were air-dried, and grain yield was expressed at 14% moisture content.
2.3.6 Nitrogen agronomic use efficiency and water use efficiency
The nitrogen agronomic use efficiency (NAUE, kg kg N−1) was calculated as follows:
Y Fertilizer N is the grain yield (kg ha−1) for the nitrogen fertilizer treatment (NC, NR, and NU), and YN0 is the grain yield for the N0 treatment. The nitrogen rate was the nitrogen fertilizer applied for the nitrogen fertilizer treatment.
The water use efficiency (WUE, kg m-3) was calculated as follows:
Y is the grain yield (kg ha−1), ET is evapotranspiration (mm), ρW is variation in soil water storage in the 0–100-cm soil layer between planting and maturity, I is the water input (the sum of irrigation, mm), and P is precipitation (i.e., rain in our study). R is the water lost to runoff from the ground surface, which was zero in this experiment due to the borders for each plot in both canopied (2016–2017) and in open-field (2018) microplots. D is deep percolation from the soil, which was ignored due to the low amount of irrigation in 2016 and 2017.
2.4 Statistical analyses
Data were analyzed using Microsoft Excel 2016 and mapped using Sigma Plot 10.0 and Origin 2021. The SAS software system for Windows 9.0 (SAS Institute, Cary, NC, USA) was used to perform analyses of variance (ANOVAs). An ANOVA was performed among all the irrigation and nitrogen treatments for grain yield, yield components, dry matter, harvest index, WUE, and NAUE (p<0.05). The multiple comparison procedure (SSR) test with Bonferroni correction for all treatments was used for multiple comparisons.
3 Results
3.1 Yield, dry matter, and harvest index
Irrigation, nitrogen fertilizer, and their interaction all had a substantial impact on the kernel number per ear, kernel weight, grain yield, dry matter, and harvest index (Table 3). The yield and dry matter increased with irrigation level, but there was no significant difference between the yields of I2 and I3. Compared with I3, I0 and I1 significantly decreased the kernels number per ear and kernel weight. I2 had a lower KNP but higher kernel weight than I3. For the same irrigation levels in I2 or I3, the kernels number per ear and kernel weight of three nitrogen fertilizers show the trend that NC>NR>NU, but there was no significant difference either between NC and NR or between NR and NU.
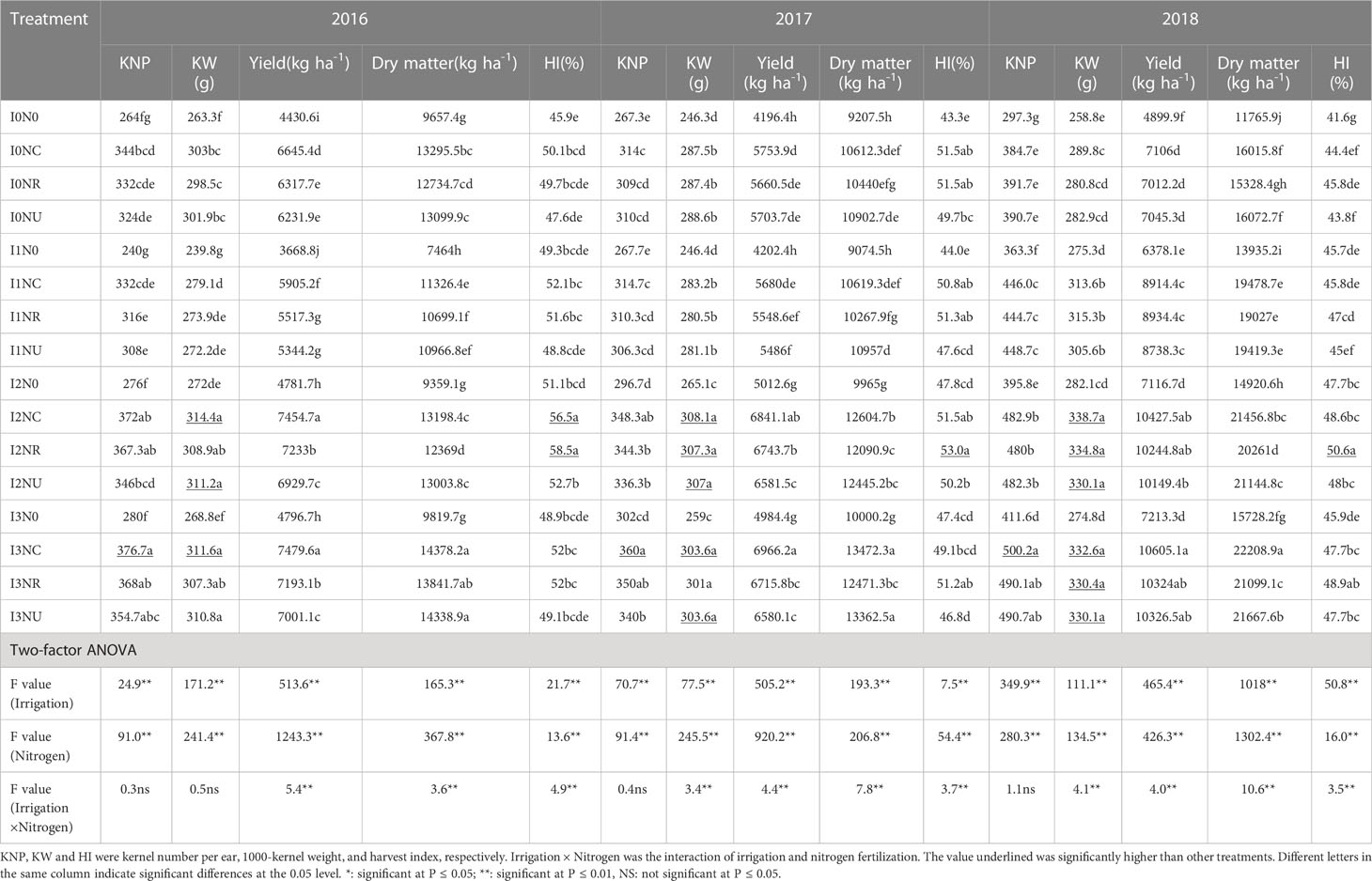
Table 3 Effect of irrigation and nitrogen fertilizer on the grain yield, yield components, dry matter, and harvest index for summer maize in 2016–2018.
The harvest index rose with irrigation level, then subsequently fell with I2 representing the peak. The HI was highest in NR and lowest in NU. Over three seasons, the plants in I2NR produced a similar yield to those in I3NC treatments despite having a lower dry matter but a higher harvest index.
3.2 The 13C-photosynthate/nitrogen accumulation and distribution ratio
The irrigation, nitrogen fertilizer, and their interaction had a significant effect on 13C-photosynthate/nitrogen accumulation and distribution ratio (Supplementary Table S1). The 13C-AC and N-AC of the plant and kernel increased with the irrigation level from I1 to I3, and nitrogen rate from 0 to 240 kg N ha−1 in the three growth seasons (Figure 3). Although there was no discernible difference between NU and NC in 2016, I3NC had the greatest 13C-AC and N-AC of the plant and kernel, followed by I3NU, I2NC, and I2NU.
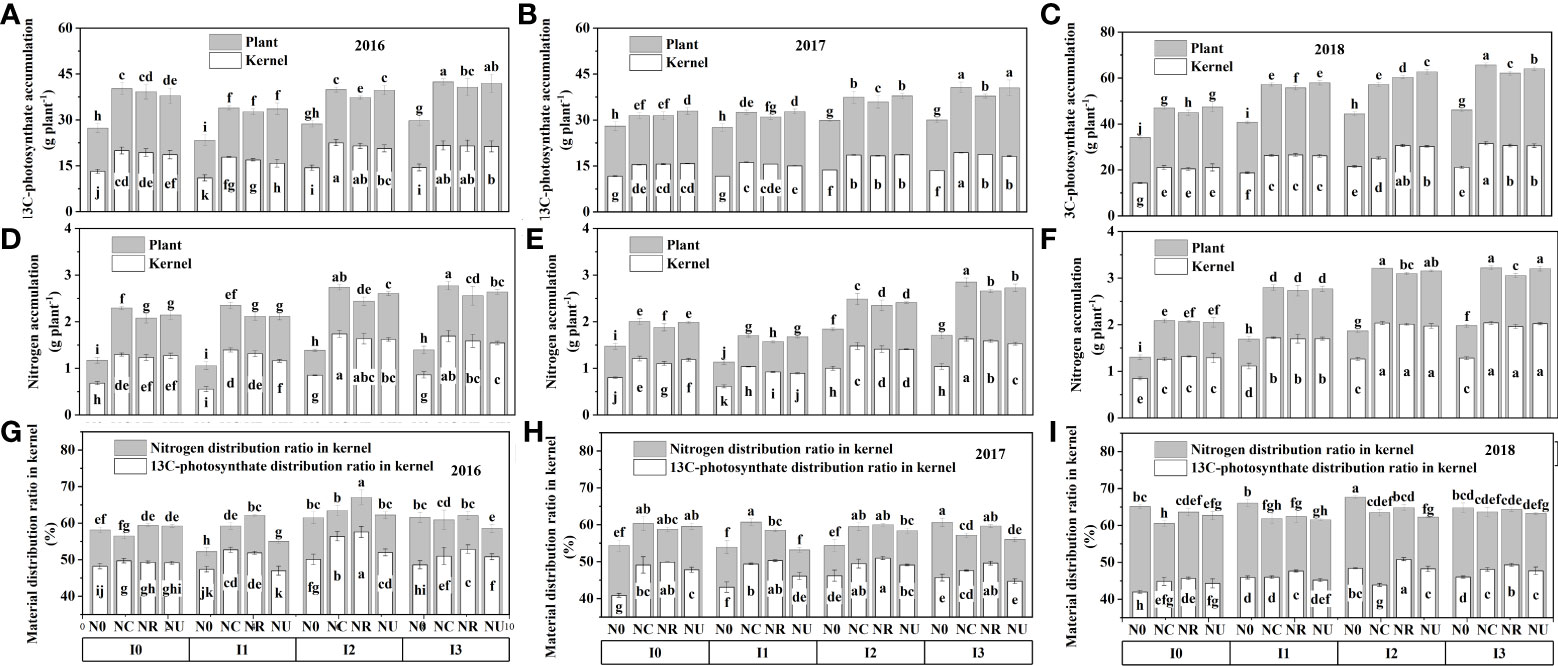
Figure 3 The effect of irrigation and nitrogen fertilizer on the 13C-photosynthate accumulation (A–C), nitrogen accumulation (D–F), and 13C-photosynthate distribution ratio and nitrogen distribution ratio (G–I) in the kernel at physiological maturity. The relative data in 2016 is shown in panels (A, D, G); in 2017, panels (B, E, H); and in 2018, (C, F, I). Different letters in the same figure indicate significant differences at the 0.05 level.
13C-DR and N-DR both increased when irrigation level increased from I1 to I2, while they decreased from I2 to I3. The 13C-DR of N0 was higher than that of NC, NU, and NR. Whereas the N-DR of N0 was lower than NC, NU, and NR. For the nitrogen fertilized treatments, the 13C-DR and N-DR in the kernels was higher in I2NR in 2016 and 2017 with no significant difference between I2NC, I2NR, and I3NC; that is, the BCRF fertilizer promoted the distribution of 13C-photosynthate and nitrogen to the kernel. Thus, a higher 13C-AC and N-AC were obtained in the I3NC and I3NU treatments, and the highest 13C-DR and N-DR in the kernel were obtained in I2NR.
3.3 Root length density
There was a significant effect of irrigation treatment and nitrogen fertilizer on root length density (Supplementary Table S1). The root length density of 0–20 cm, 20–40 cm, and 40–60 cm accounted for 68.4%–76.4%, 18.8%–24.3%, and 4.3%–7.5% of total root length density (Figure 4). Root length density of either soil layer was increased with irrigation level from I0 to I2 and decreased from I2 to I3. In 0–60 cm, root length density was higher in NC and NR than in NU under the same irrigation level, while the difference was not statistically significant. There was no significant difference between root length density of NR and NC, except that NR was higher than NC under I2 and I1 irrigation levels at 40–60-cm soil layer. The I2NR treatment achieved the maximum root length density in the 0–20-cm and 40–60-cm soil layers, whereas I2NR, I2NC, and I2NU treatments achieved the highest root length density in the 20–40-cm soil layer. These results imply that (1) compared with urea, BCRF could improve maize root length density, and (2) Root length density of I2NR in the 0-60-cm soil layer, particularly in the 40-60-cm layer, was much higher than that of I3NC or I3NR.
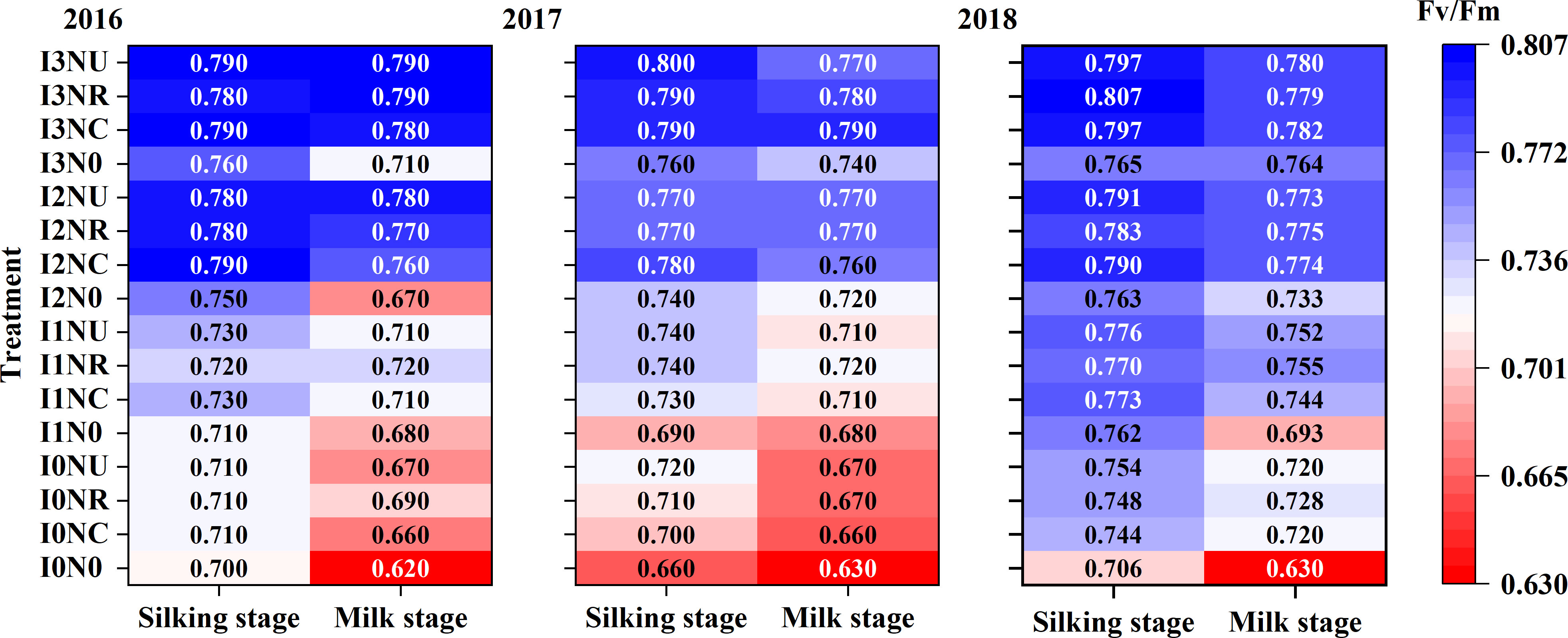
Figure 4 Effect of irrigation and nitrogen fertilizer on Fv/Fm at the silking stage and milk stage in 2016, 2017 and 2018.
3.4 Maximum photochemical efficiency of photosystem II (Fv/Fm)
Irrigation and nitrogen fertilizer management both had a significant impact on the Fv/Fm ratio (Supplementary Table S1). There was no significant difference between the Fv/Fm of NC, NR, and NU treatments, but the Fv/Fm for NC, NR, and NU were significantly higher than that for N0 (Figure 5). With the application of nitrogen fertilizer, the I3 treatment had the highest Fv/Fm at the silking stage growth stage, followed by I2, I1, and I0. The Fv/Fm decreased significantly from silking stage to the milk stage, with average decreases of 6.2%, 3.1%, 2.6%, and 1.7% for I3, I2, I1, and I0, respectively. At the milk stage, Fv/Fm values were increased with irrigation level, but there was no significant difference between Fv/Fm values of I3 and I2. It may be concluded that irrigation at I3 and I2 benefited for maintaining a higher Fv/Fm of the ear leaves.
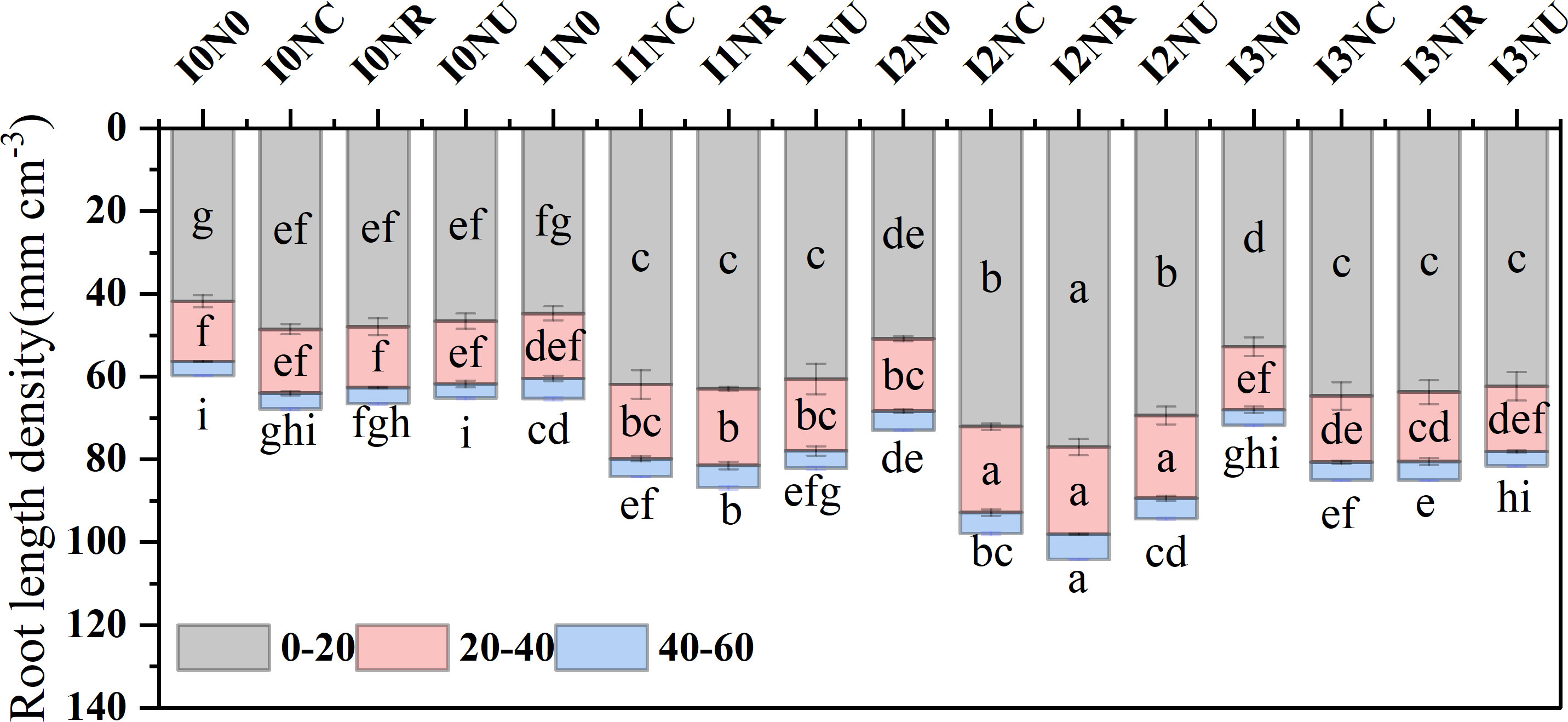
Figure 5 Effect of irrigation and nitrogen fertilizer on root length density. Different letters on the gray, pink, and blue histograms indicate significant differences at the 0.05 level.
3.5 Evapotranspiration and water use efficiency
Along with the amount of rainfall, ET was highest in 2018 (442.8–532.8 mm) and lowest in 2017 (180.4–336.0 mm) (Figure 6). Both irrigation and nitrogen fertilizer and their interaction had an impact on ET, but the effect of irrigation was higher than that of nitrogen fertilizer. The ET was increased with irrigation or rainfall showing increased from I1 to I3 (Figure 6). The ET of nitrogen fertilizer treatments was highest in NC, followed by NR and NU, and was lowest in N0 (Figure 6). Maize grain yield showed a parabolic trend of opening downward as ET increased. The WUE was highest obtain in I1 in 2016 and 2017 but highest in I2 in 2018 (Table 4). Similar to yield, the WUE was highest in NC, but the difference between NC with NR or NU was significant in 2016.
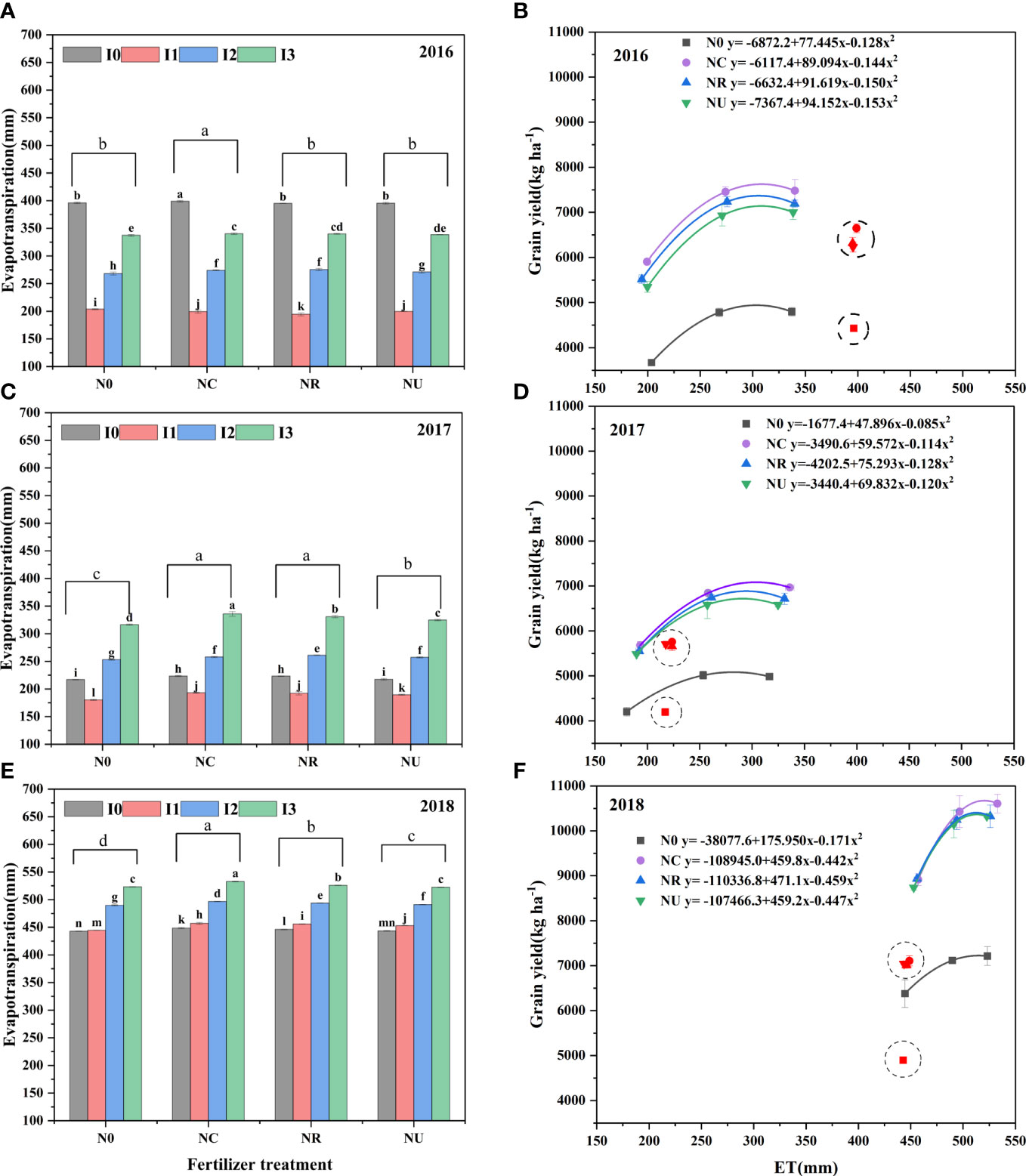
Figure 6 Effect of irrigation and nitrogen fertilizer on evapotranspiration and the relationship between grain yield and evapotranspiration. The data of I1, I2, and I3 were used to fit curves. Different letters on the gray, pink, and blue histograms indicate significant differences at the 0.05 level (A, C, E). The curve with lines in blank, purple, blue, and green color was fitted with data of N0, NC, NR, and NU, (B, D, F) respectively.
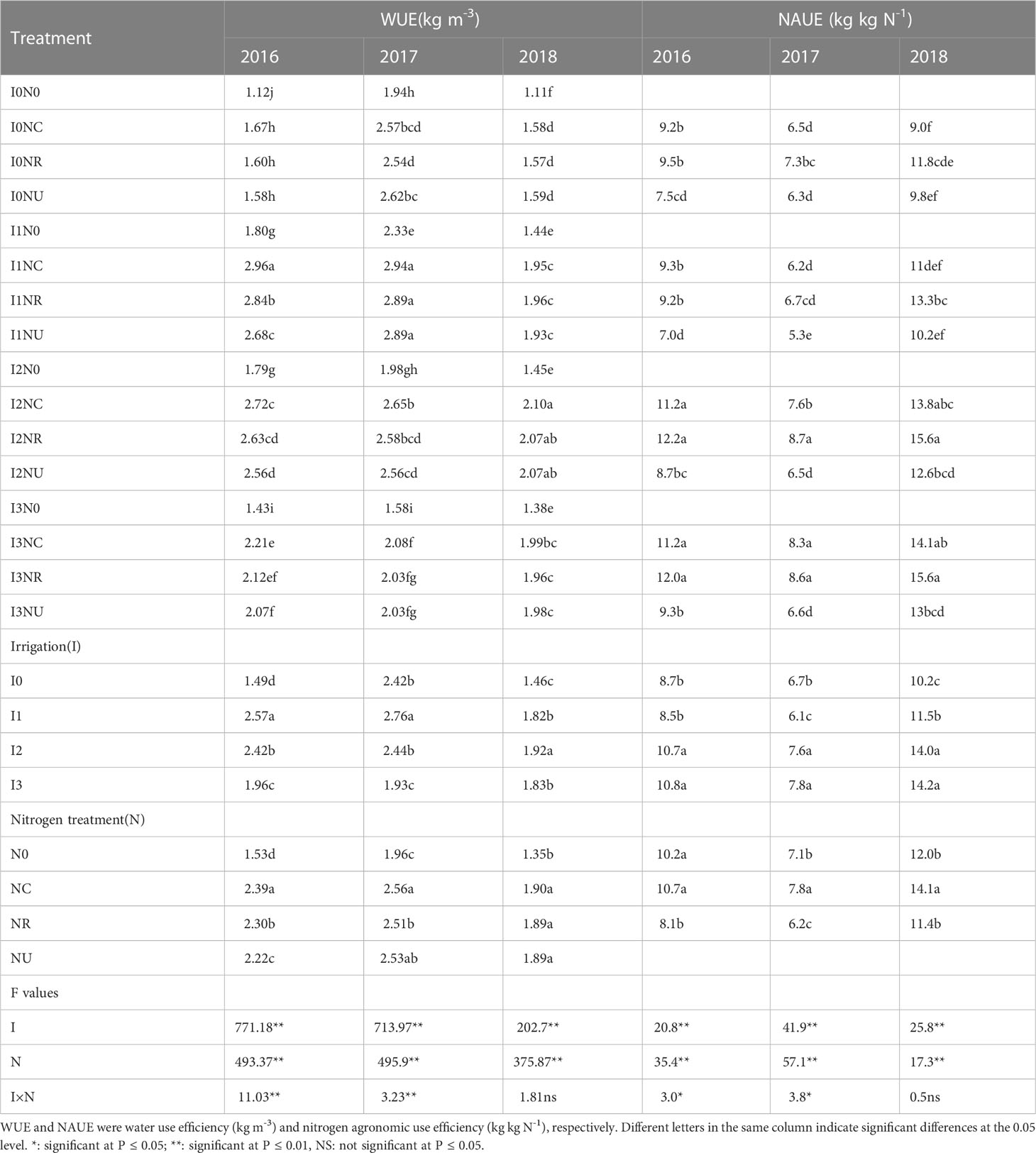
Table 4 Effect of irrigation and fertilizer treatment on water use efficiency and nitrogen agronomic use efficiency for summer maize in 2016–2018.
3.6 Nitrogen use efficiency
Irrigation and nitrogen fertilizer management have a considerable impact on NAUE (5.3–15.6 kg N−1) (Table 4). The NAUE increased with irrigation level from I1 to I2, but there was no significant difference between I2NC, I2NR, I3NC, and I3NR in 2017. The NAUE of the NC and NR was significantly higher than the NU by 15.25% and 27.20% averagely, where there was no significant difference between the NAUE of NC and NR.
3.7 Correlation analysis
Correlation analysis (Figure 7) revealed that the 13C-AC, N-AC, Fv/Fm, and root length density were all significantly and positively linked with grain yield, KNP, 1,000 KW, and WUE (p<0.05). The 13C-AC and N-AC of kernel were linearly related to kernel number per kernel, kernel weight, ET, and NAUE (Figure 8). The harvest index was increased linearly with the increase in 13C-DR and N-DR of kernel, except harvest index versus N-DR in 2018.
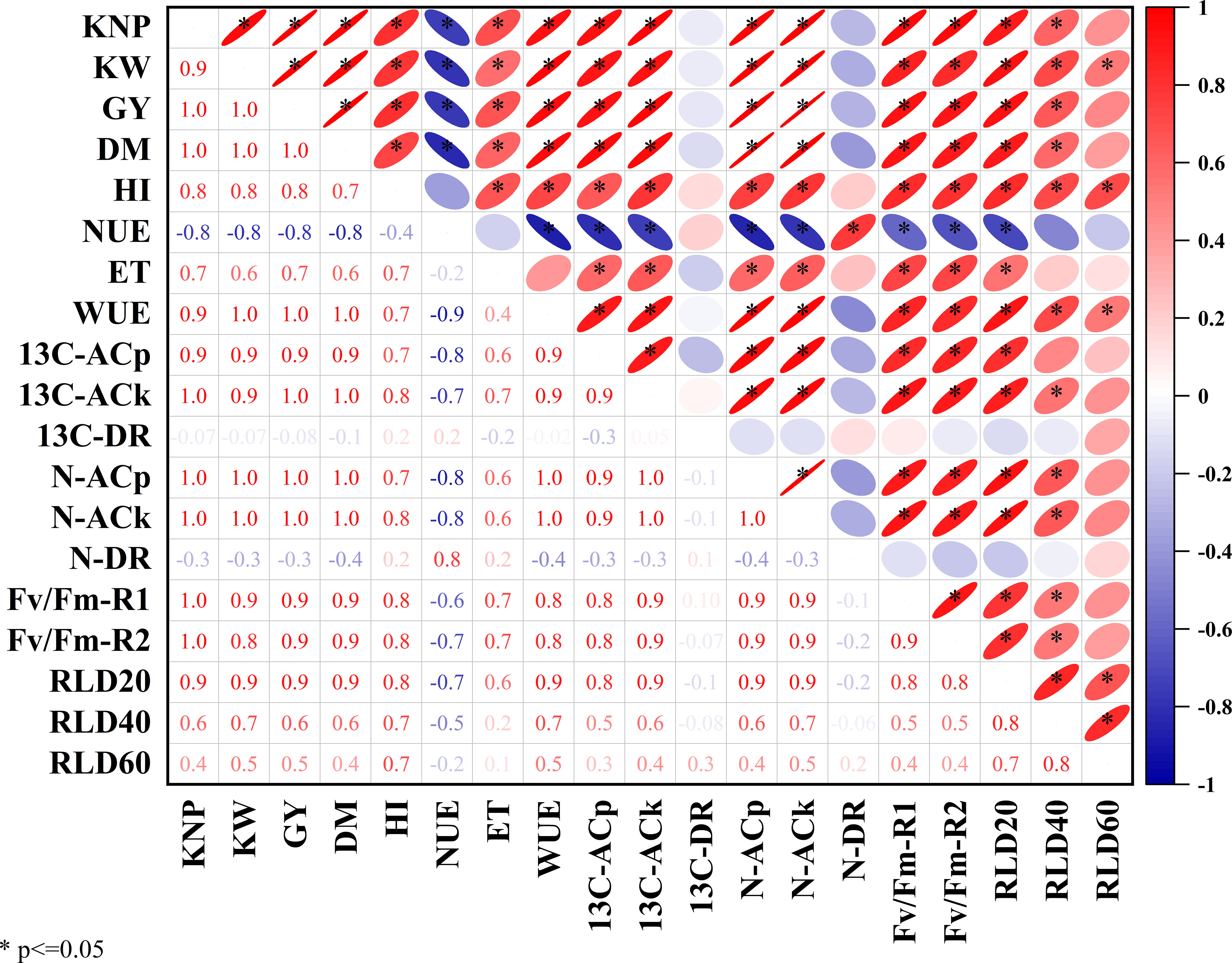
Figure 7 Correlation coefficients between maize grain yield, dry matter, 13C-photosynthate and nitrogen accumulation and distribution characteristics, leaf maximum photochemical efficiency of photosystem II, root length density, and nitrogen and water use efficiency. KNP, kernel number per ear; KW, kernel weight; GY, grain yield; NI, harvest index; NUE, nitrogen agronomic use efficiency; ET, evapotranspiration; WUE, water use efficiency; 13C-ACp, 13C-photosynthate accumulation in plant; 13C-ACk, 13C-photosynthate accumulation in kernel; 13C-DR, 13C-photosynthate distribution ratio in kernel; N-ACp, nitrogen accumulation in plant; N-ACk, nitrogen accumulation in kernel; N-DR, nitrogen distribution ratio in kernel; Fv/Fm-R1 and Fv/Fm-R2, maximum photochemical efficiency of photosystem II (Fv/Fm) in silking stage and milk stage, respectively; RLD20, RLD40 and RLD60, root length density for 0-20cm, 20-40cm and 40-60cm, respectively.
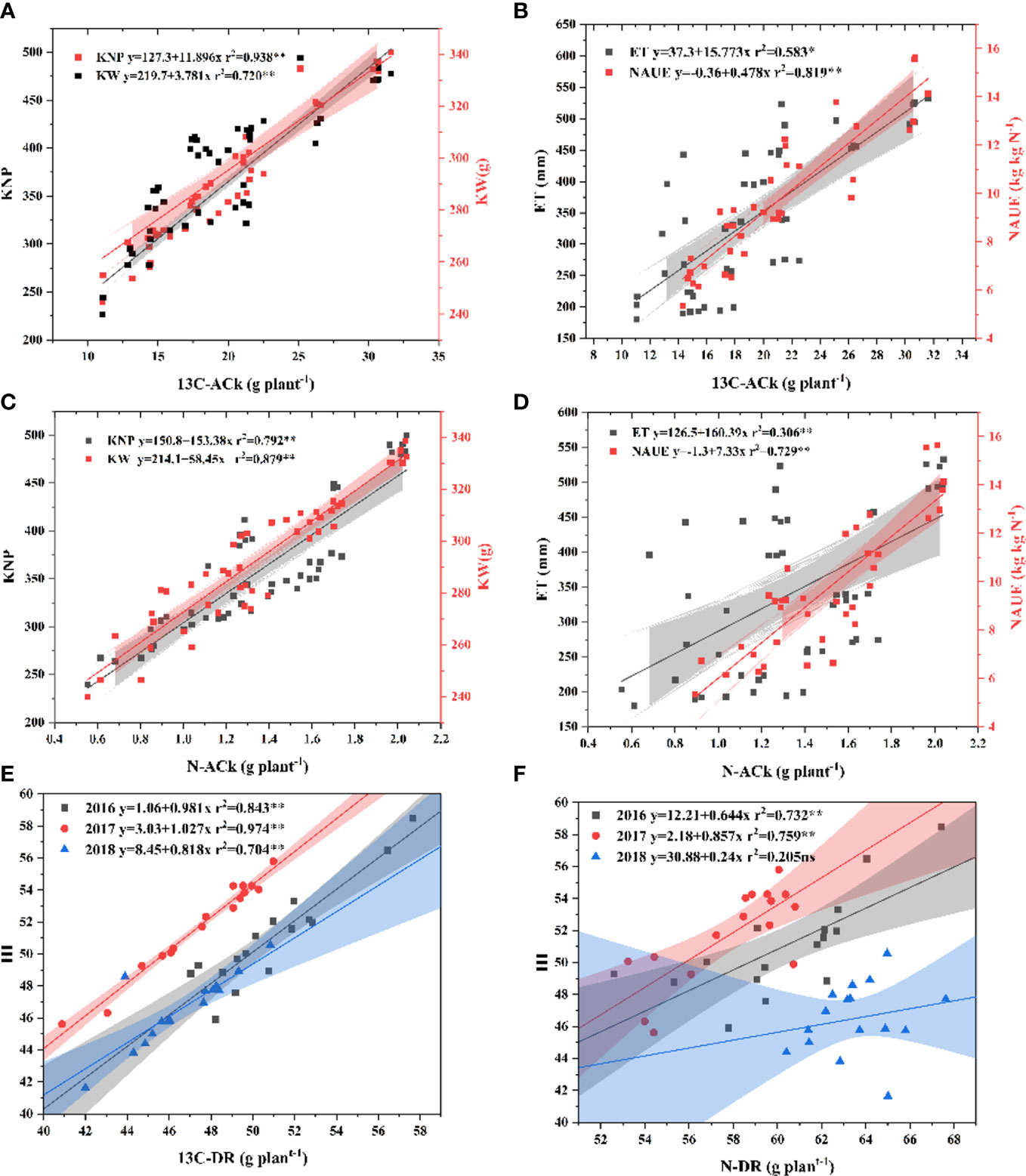
Figure 8 Scatterplots of kernel number per spike (KNP), kernel weight (KW), evapotranspiration (ET), and nitrogen agronomic use efficiency (NAUE) versus 13C-photosynthate accumulation in kernel (13C-ACk) (A, B) and nitrogen accumulation in kernel (N-ACk) (C, D), 13C-photosynthate distribution ratio in kernel (13C-DR), and nitrogen distribution ratio in kernel (N-DR) versus harvest index (HI) E, F), respectively. The colored areas indicate the 95% confidence intervals of the fitted curves.
4 Discussion
4.1 Effect of irrigation and nitrogen fertilizer on 13C-photosynthate and nitrogen accumulation and distribution and harvest index
Maize grain yield is determined by dry matter and harvest index. The accumulation of dry matter is mostly determined by photosynthesis production, while the harvest index is primarily determined by the partitioning of photosynthate and biomass to kernels (Sinclair, 1998; Allison, 2010). 13C-labeled CO2 is an effective method for studying the accumulation and distribution characteristics of photosynthetic assimilate (Nouchi et al., 1995; Tremblay et al., 2012; Ren et al., 2021). 13C-photosynthate allocation to grain is positive for kernel weight and grain yield (Liu et al., 2015; Ren et al., 2021). In this study, both 13C-photosynthate and nitrogen accumulation in the kernel was positively related to kernel number per spike, kernel weight, and grain yield. Their distribution ratio to the kernel were found to be linearly related to harvest index. Increasing the harvest index was the primary strategy to increase maize grain yield at lower yield levels (<15 Mg ha−1) (Liu et al., 2020). Thus, management that increases the accumulation and distribution of 13C-photosynthate and nitrogen to the kernel could ultimately improve grain yield.
Researchers have demonstrated that 13C-photosynthate allocation to grain increased with nitrogen fertilizer (Wei et al., 2019; Ren et al., 2021) but decreased when the nitrogen rate exceeded the acceptable rate (Zhang et al., 2021c). In this study, the nitrogen fertilization improved the 13C-photosynthate and nitrogen accumulation and nitrogen distribution ratio to the kernel but reduced the 13C-photosynthat distribution ratio to the kernel Figure 3).
In addition, 13C-photosynthate and nitrogen accumulation and distribution characteristics were influenced by fertilizer type. Compared with common urea, controlled-release fertilizers and BCRF increased dry matter and nitrogen accumulation per plant and promoted its distribution to kernel (Zhao et al., 2010; Vejan et al., 2021). In this study, The NC treatment had a higher LAI and SPAD (not shown in this paper) (higher source) and a similar Fv/Fm value to the NU, resulting in a higher 13C-AC and N-AC. The increased DM, 13C-AC, N-AC, 13C-DR, and N-DR in the BCRF treatments showed that the BCRF facilitated carbohydrate and nitrogen accumulation in plant tissue and subsequent remobilization to the kernels, resulting in an increased kernel per spike, kernel weight, and harvest index (Figure 8). These results are consistent with previous research (Qu et al., 2020; Guo et al., 2022). Compared with urea, plants grown with BCRF fertilizers had a similar “sink” (similar KNP and 1000 KW) but a greater “source” (Fv/Fm, 13C-AC, and N-AC) and higher “flow” (13C-DR, N-DR, and HI), resulting in more carbohydrate and nitrogen accumulation in the kernels and a higher yield (Graphical abstract).
4.2 Effect of irrigation and nitrogen fertilizer on root length density
Irrigation and nitrogen supply are the two important factors affecting the formation and development of the maize root system (Ning et al., 2015; Chilundo et al., 2017), and an active and deep rooting system was found to be favorably associated with water and nitrogen extraction and grain yield (Aina and Fapohunda, 1986). Root development rates were critical in enhancing plant biomass and cob yield under conditions of deficit irrigation (Flynn et al., 2020). In maize, a mild soil water deficit (50%–60%) resulted in the development of longer lateral roots and an increased root to shoot ratio (Kang et al., 2000), but severe water stress had an adverse effect on lateral root spread (Sampathkumar et al., 2012). In this study, root length density increased from I1 to I2 and decreased from I2 to I3. Compared with I2, the I1 and I3 treatments reduced root length density by 9.0%–14.6% and 10.6%–24.4%, respectively. In other words, both excessive and deficient irrigation amount may inhibit root elongation. In this study, maize plants in deficit irrigation increased root depth (increased root length density) and water extraction from deeper soil profiles (Li et al., 2022) while simultaneously decreasing leaf area to minimize transpiration, resulting in lower water consumption (Pandey et al., 2000).
In the cold–dry season, the effect of irrigation on root density was weaker than fertilizer type, and slow-release fertilization resulted in overall higher root density, above-ground biomass, and grain yield than quick-release fertilization (Chilundo et al., 2017). In this study, nitrogen fertilizer had a significant impact on root length density, increasing from 0 kg N ha−1 to 200–240 kg N ha−1, but the effect was less than that observed with changes in irrigation. Compared with I3NU treatment, I2NR treatment increased root length density by 26.4% in 0–60-cm soil layer and by 41.0% in 40–60 cm. The result was consistent with other studies (Flynn et al., 2020; Halli et al., 2021). Appropriate water and nitrogen deficiency induced root elongation in search of more water and nutrients. The moderate water-stress and low nitrogen rate treatments resulted in an optimal root distribution defined by increased root length density and a bigger and deeper penetration scale throughout the soil layers, resulting in fewer drought responses and the best WUE and NUE (Wang et al., 2019b). Additionally, the root length density of BCRF fertilizers was greater than that of urea in this investigation, both at the same and reduced nitrogen rates. The blend product consistently supplies sufficient nitrogen to maize crops (Zheng et al., 2020), demonstrating that BCRF can alter the abundance of microbial colonies and improve soil nitrate content, root growth, and nitrogen uptake throughout the maize growing season (Li et al., 2021; Zhang et al., 2021b). Root length density was positive to photosynthesis (Fv/Fm) on the milk stage, harvest index, 13C-photosynthate, and nitrogen distribution ratio. Suitable root length is beneficial to optimize root–shoot ratio and increase dry matter accumulation in aboveground and underground parts simultaneously (Elazab et al., 2016; Ordonez et al., 2020). In this study, the first increased and then decreased with the increase in irrigation level, which was consistent to previous studies (Elazab et al., 2016; Gao et al., 2022). Furthermore, the higher root length density treatment, with higher water and nitrogen assimilating capacity, delayed the leaf senescence process with higher photosynthetic rate (Figure 4) and ultimately increased photosynthate and nitrogen accumulation and distribution in kernel (Chilundo et al., 2017). In this study, a higher root length density in I2NR maintains higher maize photosynthesis (Fv/Fm) in the milk stage, delayed leaf senescence in the later stage, and results in similar kernel weights as with I3NU.
4.3 Effect of irrigation and nitrogen fertilizer on grain yield, NAUE, and WUE
Water shortage is worsening, and droughts are becoming more common in the Huang-Huai-Hai Plain, China’s key summer maize-producing region (Kang and Zhang, 2016; Chen et al., 2021). Effective irrigation practice is critical for maintaining high summer maize yields while improving WUE. Deficit irrigation is preferable to full irrigation for eco-agriculture (Zhang, 2003a; Tavakkoli and Oweis, 2004; Geerts and Raes, 2009). Researchers have reported that 75% ETc in winter wheat (Lu et al., 2021) and 80% ETc in maize (Guo et al., 2022) produced higher yield and WUE due to higher net photosynthetic efficiency and leaf area index. In this study, the plants in I1 were severely drought stressed and had the lowest Fv/Fm, dry matter, kernel number, kernel weight, and yield but the highest WUE. Fv/Fm, optimal/maximal quantum yield of PSII, was the indicator for adjusting leaf growth status under water deficit (Song et al., 2018; Li et al., 2019). The I1 significantly decreased the maximum light energy absorption and capture efficiency and accumulated low energy for photosynthesis, limiting the maize dry matter accumulation. When compared with I3, the maize growth in I1 was severely limited, with yield losses of 10.9%–32.0%; hence, this treatment is not recommended for maize production. I2 maize had lower dry matter, 13C-AC, and N-AC than I3, but it produced the same yield due to higher root length density, HI, 13C-DR, and N-DR. This finding was consistent with previous reports (Tolk et al., 1999; Oktem et al., 2003; Payero et al., 2006; Imma and Maria, 2007; Lu et al., 2021; Guo et al., 2022) that appropriate deficit irrigation optimizes yield and WUE. As a result, a mild water deficit of 75% ETc promoted deeper root growth (40–60 cm), maintaining long-term Fv/Fm benefits for leaf photosynthesis, and promoted more photosynthate and nitrogen from other organs to kernel tissues, resulting in increased grain yield and WUE.
The tolerance of maize to drought stress varied depending on the stage of growth. Drought from the tasseling stage to the milk stage had the largest impact on maize output, followed by drought from the seventh leaf stage to the tasseling stage and drought from the sowing to the seventh leaf stage (Zhang et al., 2021a; Zhu et al., 2021). A hypothetical lower degree of drought stress in the sensitive period and a higher degree of drought stress in the non-sensitive period could further improve the yield and WUE than drought stress during the entire growth period. The degree of drought stress based on ETc criteria during the maize growing season needs to be further studied (Mansouri-Far et al., 2010).
Clarifying the relationship between ET and maize grain yield, WUE and NAUE could improve our understanding of regulatory mechanisms when facing persistent water scarcity and climate change (Chen et al., 2021). The trend of grain yield, WUE, and ET in 2018 was consistent to previous studies (Grassini et al., 2009; Hernández et al., 2015). The lower ET for highest grain and WUE in 2016 and 2017 may be attributed to the higher productivity for limited irrigation when rainfall was prevented. Increased with ET, the grain yield were quadratic (Figure 6). Without taking soil evaporation into account, larger daily ET rates could be the result of increased root capacity for water and nitrogen extraction (Canales et al., 2021) and/or a greater canopy capacity, resulting in higher photosynthate accumulation (Hernández et al., 2015). While photosynthesis increased initially and then stayed consistent as leaf stomatal opening increased, excessive stomatal opening resulted in excessive water loss and decreased the leaf immediate water use efficiency. Furthermore, the grain yield in 2016 and 2017 under rainproof shelter was significantly lower than that in 2018 and the farmer field in this region. With rainproof shelter, the irrigation effect on maize performance could be studied clearly, reducing the risk of unforeseen rainfall affecting (Kundel et al., 2018). However, the temperature was higher than the field, resulting in higher evapotranspiration, shorter growth period, and lower yield. The maize performance under rainproof shelter could provide referential value for dryland or dry years.
The actual average ET (526.2 mm) for I3 in 2018 was much higher than the average ETc (335.9 mm, calculated by FAO56) from 1981 to 2015, which was higher than the precipitation in 2016 and 2017 but lower than the precipitation in 2018 (349.4, 193.6, and 389.8 mm in the three growing seasons, respectively). The discrepancy between real ET and estimated ETc was partly related to an imbalance in the timing and quantity of rainfall and maize need (Liu et al., 2022b). The ineffective evaporation was compounded by more precipitation prior to the V12 stage (231 mm) but a lower maize demand (94.6 mm). Rainfall exceeds maize demand by a substantial margin, resulting in significant water losses through soil evaporation (Jia et al., 2021) and leachate (Nouchi et al., 1995; Li et al., 2020), whereas the rainfall (15.6 mm) was much lower than the maize demand (61.6 mm) during V12 stage to anthesis stage, the water critical period. The low yield and WUE of I0 treatment throughout the three seasons indicated that additional irrigation was required for the summer maize season, despite the fact that rainfall was greater than ETc in 2018 (Ren et al., 2022).
Improved grain yield and NAUE requires better coordination of crop nitrogen requirements and multiple-source availability (Cui et al., 2010; Ciampitti and Vyn, 2012; Zhang et al., 2012; Meng et al., 2016). A larger LAI and SPAD (not shown) and a similar Fv/Fm value (for the same nitrogen rate treatment) in the NC treatment resulted in increased 13C-AC and N-AC. The higher dry matter, 13C-AC, N-AC, 13C-DR, and N-DR in the BCRF treatments indicate that the BCRF enhanced the carbohydrate and nitrogen accumulation in plant tissue and subsequent remobilization to the kernels, ultimately resulting in greater yield. These results are consistent with previous research (Qu et al., 2020; Guo et al., 2022). Compared with plants grown using urea, plants grown with BCRF fertilizer had the same “sink” (similar kernel number per spike) and higher “source” (13C-AC and N-AC) and “flow” (13C-DR, N-DR and HI), resulting in more carbohydrate and nitrogen accumulation in the kernels and a higher yield Graphical abstract.
The coupling effect of irrigation amount and nitrogen management was significant. Blending control-release fertilizer and urea could dramatically alleviate grain yield loss due to water stress (Guo et al., 2022). In this study, the grain yield of NC was 6.6%–10.6%, 0.9%–3.5%, and 0.9%–2.0% higher than that of NU in the 2016, 2017, and 2018 growing seasons, respectively. However, the difference between NU and NC was greater under I2 and I3 treatments. In addition, drought stress was lessened because of the nitrogen fertilizer (Tilling et al., 2007; Sandhu et al., 2019). In this study, the nitrogen-fertilized treatments, especially the I1 and I0 treatments, significantly increased the Fv/Fm, 13C-AC, N-AC, and grain yield compared with the N0 treatments. Drought-stressed maize had a lower root density (Chilundo et al., 2017; Gheysari et al., 2017), excessive nutrients remaining in the soil (Ge et al., 2012), and a reduced nitrogen uptake (Xiao et al., 2021). Proper irrigation (I2 in this study) helped to enhance N-AC and 13C-AC in the kernels and NAUE. Excessive irrigation resulted in ineffective plant development and decreased N-DR content in kernels and raised the danger of nutrient leaching (Ren et al., 2016; Srivastava et al., 2020).
5 Conclusion
Compared with the I3NU treatment, the I2NC and I2NR treatments increased the kernel number per ear (sink size), maintained a higher Fv/Fm in the milk stage (Ministry of Water Resources), increased 13C-photosynthate and nitrogen accumulation, and promoted 13C-photosynthate and nitrogen transport from nutritive organs to the kernels (flow), resulting in a higher harvest and a comparable yield. Meanwhile, I2NC and I2NR had a reduced irrigation input and topdressing cost while synchronously increasing the WUE and NAUE. Due to its balanced “source-flow-sink” characteristics, the 75% ETc-based irrigation combined with 200 kg N ha−1 of BCRF is an effective treatment in terms of yield, WUE, and NAUE. Additional field experiments on a 75% ETc irrigation treatment with different water deficits in the water-sensitive and water-insensitive stages of the plants should be undertaken to optimize the potential for greater yields and resource use efficiency.
Data availability statement
The original contributions presented in the study are included in the article/Supplementary Material. Further inquiries can be directed to the corresponding authors.
Author contributions
LG: data curation, writing—original draft, visualization, investigation, and funding acquisition. XM: writing—review and editing. JQ: supervision. BT: funding acquisition and supervision. WZ: conceptualization and writing—review and editing. LX: conceptualization and writing—review and editing. All authors contributed to the article and approved the submitted version.
Funding
This work was supported by the National Natural Science Foundation of China (31601259), Project of Corn Industry Technology System Construction in Henan (HARS-22-02-G2), scientific and technological innovation team project of Henan Academy of Agricultural Sciences (2023TD37), and Special Fund for Agro-scientific Research in the Public Interest (201503130). We thank LetPub (www.letpub.com) for linguistic assistance and pre-submission expert review.
Conflict of interest
The authors declare that the research was conducted in the absence of any commercial or financial relationships that could be construed as a potential conflict of interest.
Publisher’s note
All claims expressed in this article are solely those of the authors and do not necessarily represent those of their affiliated organizations, or those of the publisher, the editors and the reviewers. Any product that may be evaluated in this article, or claim that may be made by its manufacturer, is not guaranteed or endorsed by the publisher.
Supplementary material
The Supplementary Material for this article can be found online at: https://www.frontiersin.org/articles/10.3389/fpls.2023.1180734/full#supplementary-material
Abbreviations
WUE, water use efficiency; NAUE, nitrogen agronomic use efficiency; 13C-AC, 13C-photosynthate accumulation; 13C-DR, 13C-photosynthate distribution ratio; N-AC, nitrogen distribution accumulation; N-DR, nitrogen distribution ratio.
References
Adu-Gyamfi, R., Agyin-Birikorang, S., Tindjina, I., Manu, Y., Singh, U. (2019). Minimizing nutrient leaching from maize production systems in northern Ghana with one-time application of multi-nutrient fertilizer briquettes. Sci. Total Environ. 694, 133667. doi: 10.1016/j.scitotenv.2019.133667
Aina, P. O., Fapohunda, H. O. (1986). Root distribution and water uptake patterns of maize cultivars field-grown under differential irrigation. Plant Soil 94 (2), 257–265. doi: 10.1007/BF02374349
Allison, J. (2010). Effect of plant population on the production and distribution of dry matter in maize. Ann. Appl. Biol. 63 (1), 135–144. doi: 10.1111/j.1744-7348.1969.tb05474.x
Canales, F. J., Rispail, N., García-Tejera, O., Arbona, V., Pérez-de-Luque, A., Prats, E. (2021). Drought resistance in oat involves ABA-mediated modulation of transpiration and root hydraulic conductivity. Environ. Exp. Bot. 182, 104333. doi: 10.1016/j.envexpbot.2020.104333
Cancela, J. J., Fandiño, M., Rey, B. J., Martínez, E. M. (2015). Automatic irrigation system based on dual crop coefficient, soil and plant water status for vitis vinifera (cv godello and cv mencía). Agric. Water Manage. 151, 52–63. doi: 10.1016/j.agwat.2014.10.020
Chen, L., Chang, J., Wang, Y., Guo, A., Liu, Y., Wang, Q., et al. (2021). Disclosing the future food security risk of China based on crop production and water scarcity under diverse socioeconomic and climate scenarios. Sci. Total Environ. 790, 148110. doi: 10.1016/j.scitotenv.2021.148110
Chilundo, M., Joel, A., Wesström, I., Brito, R., Messing, I. (2017). Response of maize root growth to irrigation and nitrogen management strategies in semi-arid loamy sandy soil. Field Crops Res. 200, 143–162. doi: 10.1016/j.fcr.2016.10.005
Ciampitti, I. A., Vyn, T. J. (2012). Physiological perspectives of changes over time in maize yield dependency on nitrogen uptake and associated nitrogen efficiencies: a review. Field Crops Res. 133, 48–67. doi: 10.1016/j.fcr.2012.03.008
Comas, L. H., Trout, T. J., DeJonge, K. C., Zhang, H., Gleason, S. M. (2019). Water productivity under strategic growth stage-based deficit irrigation in maize. Agric. Water Manage. 212, 433–440. doi: 10.1016/j.agwat.2018.07.015
Cui, Z., Chen, X., Zhang, F. (2010). Current nitrogen management status and measures to improve the intensive wheat–maize system in China. Ambio 39 (5/6), 376–384. doi: 10.1007/s13280-010-0076-6
Eekhout, J. P. C., Hunink, J. E., Terink, W., de Vente, J. (2018). Why increased extreme precipitation under climate change negatively affects water security. Hydrol. Earth System Sci. 22 (11), 5935–5946. doi: 10.5194/hess-22-5935-2018
Elazab, A., Serret, M. D., Araus, J. L. (2016). Interactive effect of water and nitrogen regimes on plant growth, root traits and water status of old and modern durum wheat genotypes. Planta 244 (1), 125–144. doi: 10.1007/s00425-016-2500-z
Flynn, N. E., Stewart, C. E., Comas, L. H., Fonte, S. J. (2021). Deficit irrigation drives maize root distribution and soil microbial communities with implications for soil carbon dynamics. Soil Sci. Soc. America J. 85, 412–422. doi: 10.1002/saj2.20201
Gao, Y., Chen, J., Wang, G., Liu, Z., Sun, W., Zhang, Y., et al. (2022). Different responses in root water uptake of summer maize to planting density and nitrogen fertilization. Front Plant Sci. 13, 918043. doi: 10.3389/fpls.2022.918043
Ge, T., Sun, N., Bai, L., Tong, C., Sui, F. (2012). Effects of drought stress on phosphorus and potassium uptake dynamics in summer maize (Zea mays) throughout the growth cycle. Acta Physiol. Plant. 34 (6), 2179–2186. doi: 10.1007/s11738-012-1018-7
Geerts, S., Raes, D. (2009). Deficit irrigation as an on-farm strategy to maximize crop water productivity in dry areas. Agric. Water Manage. 96 (9), 1275–1284. doi: 10.1016/j.agwat.2009.04.009
Gheysari, M., Sadeghi, S.-H., Loescher, H. W., Amiri, S., Zareian, M. J., Majidi, M. M., et al. (2017). Comparison of deficit irrigation management strategies on root, plant growth and biomass productivity of silage maize. Agric. Water Manage. 182, 126–138. doi: 10.1016/j.agwat.2016.12.014
Grassini, P., Yang, H., Cassman, K. G. (2009). Limits to maize productivity in Western corn-belt: a simulation analysis for fully irrigated and rainfed conditions. Agric. For. Meteorol. 149 (8), 1254–1265. doi: 10.1016/j.agrformet.2009.02.012
Guo, J., Fan, J., Xiang, Y., Zhang, F., Yan, S., Zhang, X., et al. (2022). Maize leaf functional responses to blending urea and slow-release nitrogen fertilizer under various drip irrigation regimes. Agric. Water Manage. 262, 107396. doi: 10.1016/j.agwat.2021.107396
Guo, J. H., Liu, X. J., Zhang, Y., Shen, J. L., Han, W. X., Zhang, W. F., et al. (2010). Significant acidification in major Chinese croplands. Science 327 (5968), 1008–1010. doi: 10.1126/science.1182570
Guo, Z., Shi, Y., Yu, Z., Zhang, Y. (2015). Supplemental irrigation affected flag leaves senescence post-anthesis and grain yield of winter wheat in the Huang-Huai-Hai plain of China. Field Crops Res. 180, 100–109. doi: 10.1016/j.fcr.2015.05.015
Halli, H. M., Angadi, S., Kumar, A., Govindasamy, P., Madar, R., El-Ansary, D. O., et al. (2021). Influence of planting and irrigation levels as physical methods on maize root morphological traits, grain yield and water productivity in semi-arid region. Agronomy 11 (2), 294. doi: 10.3390/agronomy11020294
Hernández, M., Echarte, L., Della Maggiora, A., Cambareri, M., Barbieri, P., Cerrudo, D. (2015). Maize water use efficiency and evapotranspiration response to n supply under contrasting soil water availability. Field Crops Res. 178, 8–15. doi: 10.1016/j.fcr.2015.03.017
Hou, P., Jiang, Y., Yan, L., Petropoulos, E., Wang, J., Xue, L., et al. (2021). Effect of fertilization on nitrogen losses through surface runoffs in Chinese farmlands: a meta-analysis. Sci. Total Environ. 793, 148554. doi: 10.1016/j.scitotenv.2021.148554
Imma, F., Maria, F. J. (2007). Comparative response of maize (Zea mays l.) and sorghum (Sorghum bicolor l. moench) to deficit irrigation in a Mediterranean environment. Agric. Water Manage. 83 (1), 135–143. doi: 10.1016/j.agwat.2005.11.001
Jia, Q., Shi, H., Li, R., Miao, Q., Feng, Y., Wang, N., et al. (2021). Evaporation of maize crop under mulch film and soil covered drip irrigation: field assessment and modelling on West liaohe plain, China. Agric. Water Manage. 253, 106894. doi: 10.1016/j.agwat.2021.106894
Jovanovic, N., Pereira, L. S., Paredes, P., Pôças, I., Cantore, V., Todorovic, M. (2020). A review of strategies, methods and technologies to reduce non-beneficial consumptive water use on farms considering the FAO56 methods. Agric. Water Manage. 239, 106267. doi: 10.1016/j.agwat.2020.106267
Kang, S., Shi, W., Zhang, J. (2000). An improved water-use efficiency for maize grown under regulated deficit irrigation. Field Crops Res. 67 (3), 207–214. doi: 10.1016/S0378-4290(00)00095-2
Kang, L., Zhang, H. (2016). A comprehensive study of agricultural drought resistance and background drought levels in five main grain-producing regions of China. Sustainability 8 (4), 346. doi: 10.3390/su8040346
Kundel, D., Meyer, S., Birkhofer, H., Fliessbach, A., Mäder, P., Scheu, S., et al. (2018). Design and manual to construct rainout-shelters for climate change experiments in agroecosystems. Front. Environ. Sci. 6, 14. doi: 10.3389/fenvs.2018.00014
Li, R., Gao, Y., Chen, Q., Li, Z., Gao, F., Meng, Q., et al. (2021). Blended controlled-release nitrogen fertilizer with straw returning improved soil nitrogen availability, soil microbial community, and root morphology of wheat. Soil Tillage Res. 212, 105045. doi: 10.1016/j.still.2021.105045
Li, Y., Huang, G., Chen, Z., Xiong, Y., Huang, Q., Xu, X., et al. (2022). Effects of irrigation and fertilization on grain yield, water and nitrogen dynamics and their use efficiency of spring wheat farmland in an arid agricultural watershed of Northwest China. Agric. Water Manage. 260, 107277. doi: 10.1016/j.agwat.2021.107277
Li, Y., Song, H., Zhou, L., Xu, Z., Zhou, G. (2019). Vertical distributions of chlorophyll and nitrogen and their associations with photosynthesis under drought and rewatering regimes in a maize field. Agric. For. Meteorol. 272-273, 40–54. doi: 10.1016/j.agrformet.2019.03.026
Li, Z., Wen, X., Hu, C., Li, X., Li, S., Zhang, X., et al. (2020). Regional simulation of nitrate leaching potential from winter wheat-summer maize rotation croplands on the north China plain using the NLEAP-GIS model. Agricult. Ecosyst. Environ. 294, 106861. doi: 10.1016/j.agee.2020.106861
Liu, T., Gu, L., Dong, S., Zhang, J., Liu, P., Zhao, B. (2015). Optimum leaf removal increases canopy apparent photosynthesis, 13C-photosynthate distribution and grain yield of maize crops grown at high density. Field Crops Res. 170, 32–39. doi: 10.1016/j.fcr.2014.09.015
Liu, W., Hou, P., Liu, G., Yang, Y., Guo, X., Ming, B., et al. (2020). Contribution of total dry matter and harvest index to maize grain yield–a multisource data analysis. Food Energy Secur. 9 (4), e256. doi: 10.1002/fes3.256
Liu, Y., Lin, Y., Huo, Z., Zhang, C., Wang, C., Xue, J., et al. (2022b). Spatio-temporal variation of irrigation water requirements for wheat and maize in the yellow river basin, china 1974–2017. Agric. Water Manage. 262, 107451. doi: 10.1016/j.agwat.2021.107451
Liu, S., Lin, X., Wang, W., Zhang, B., Wang, D. (2022a). Supplemental irrigation increases grain yield, water productivity, and nitrogen utilization efficiency by improving nitrogen nutrition status in winter wheat. Agric. Water Manage. 264, 107505. doi: 10.1016/j.agwat.2022.107505
López-Urrea, R., Domínguez, A., Pardo, J. J., Montoya, F., García-Vila, M., Martínez-Romero, A. (2020). Parameterization and comparison of the AquaCrop and MOPECO models for a high-yielding barley cultivar under different irrigation levels. Agric. Water Manage. 230, 105931. doi: 10.1016/j.agwat.2019.105931
Lu, J., Hu, T., Geng, C., Cui, X., Fan, J., Zhang, F. (2021). Response of yield, yield components and water-nitrogen use efficiency of winter wheat to different drip fertigation regimes in Northwest China. Agric. Water Manage. 255, 107034. doi: 10.1016/j.agwat.2021.107034
Lyu, X., Wang, T., Song, X., Zhao, C., Rees, R. M., Liu, Z., et al. (2021). Reducing N2O emissions with enhanced efficiency nitrogen fertilizers (EENFs) in a high-yielding spring maize system. Environ. pollut. 273, 116422. doi: 10.1016/j.envpol.2020.116422
Mancosu, N., Spano, D., Orang, M., Sarreshteh, S., Snyder, R. L. (2016). SIMETAW# - a model for agricultural water demand planning. Water Resour. Manage. 30 (2), 541–557. doi: 10.1007/s11269-015-1176-7
Mansouri-Far, C., Modarres Sanavy, S. A. M., Saberali, S. F. (2010). Maize yield response to deficit irrigation during low-sensitive growth stages and nitrogen rate under semi-arid climatic conditions. Agric. Water Manage. 97 (1), 12–22. doi: 10.1016/j.agwat.2009.08.003
Masupha, T. E., Moeletsi, M. E. (2020). The use of water requirement satisfaction index for assessing agricultural drought on rain-fed maize, in the luvuvhu river catchment, south Africa. Agric. Water Manage. 237, 106142. doi: 10.1016/j.agwat.2020.106142
Meng, Q. F., Yue, S. C., Hou, P., Cui, Z. L., Chen, X. P. (2016). Improving yield and nitrogen use efficiency simultaneously for maize and wheat in China: a review. Pedosphere 26 (2), 137–147. doi: 10.1016/S1002-0160(15)60030-3
Ministry of Water Resources (2020) 2020 statistic bulletin on China water activities (China: Water and Power Press). Available at: http://www.mwr.gov.cn/sj/tjgb/slfztjgb/202202/t20220209_1561588.html (Accessed 9 2021).
Mirhashemi, S. H., Panahi, M. (2021). Investigation and prediction of maize water requirements in four growth stages under the influence of natural factors (Case study: qazvin plain, Iran). Environ. Technol. Innovation 24, 102062. doi: 10.1016/j.eti.2021.102062
Mishra, B. K., Kumar, P., Saraswat, C., Chakraborty, S., Gautam, A. (2021). Water security in a changing environment: concept, challenges and solutions. Water 13 (4), 490. doi: 10.3390/w13040490
Ning, S., Shi, J., Zuo, Q., Wang, S., Ben-Gal, A. (2015). Generalization of the root length density distribution of cotton under film mulched drip irrigation. Field Crops Res. 177, 125–136. doi: 10.1016/j.fcr.2015.03.012
Nouchi, I., Ito, O., Harazono, Y., Kouchi, H. (1995). Acceleration of 13C-labelled photosynthate partitioning from leaves to panicles in rice plants exposed to chronic ozone at the reproductive stage. Environ. pollut. 88 (3), 253. doi: 10.1016/0269-7491(95)93437-5
Oktem, A., Simsek, M., Oktem, G. A. (2003). Deficit irrigation effects on sweet corn (Zea mays saccharata sturt) with drip irrigation system in a semi-arid region: i. water-yield relationship. Agric. Water Manage. 61 (1), 63–74. doi: 10.1016/S0378-3774(02)00161-0
Ordonez, R. A., Archontoulis, S. V., Martinez-Feria, R., Hatfield, J. L., Wright, E. E., Castellano, M. J. (2020). Root to shoot and carbon to nitrogen ratios of maize and soybean crops in the US Midwest. J. Eur. J. Agron. 120 (1), 123130. doi: 10.1016/j.eja.2020.126130
Pandey, R. K., Maranville, J. W., Chetima, M. M. (2000). Deficit irrigation and nitrogen effects on maize in a sahelian environment: II. shoot growth, nitrogen uptake and water extraction. Agric. Water Manage. 46 (1), 15–27. doi: 10.1016/S0378-3774(00)00074-3
Payero, J. O., Melvin, S. R., Irmak, S., Tarkalson, D. D. (2006). Yield response of corn to deficit irrigation in a semiarid climate. Agric. Water Manage. 84 (1), 101–112. doi: 10.1016/j.agwat.2006.01.009
Peng, Y., Xiao, Y., Fu, Z., Dong, Y., Zheng, Y., Yan, H., et al. (2019). Precision irrigation perspectives on the sustainable water-saving of field crop production in China: water demand prediction and irrigation scheme optimization. J. Cleaner Product. 230, 365–377. doi: 10.1016/j.jclepro.2019.04.347
Pereira, L. S., Paredes, P., Jovanovic, N. (2020). Soil water balance models for determining crop water and irrigation requirements and irrigation scheduling focusing on the FAO56 method and the dual kc approach. Agric. Water Manage. 241, 106357. doi: 10.1016/j.agwat.2020.106357
Qu, Z., Qi, X., Shi, R., Zhao, Y., Hu, Z., Chen, Q., et al. (2020). Reduced n fertilizer application with optimal blend of controlled-release urea and urea improves tomato yield and quality in greenhouse production system. J. Soil Sci. Plant Nutr. 20 (4), 1741–1750. doi: 10.1007/s42729-020-00244-8
Ren, P., Huang, F., Li, B. (2022). Spatiotemporal patterns of water consumption and irrigation requirements of wheat-maize in the Huang-Huai-Hai plain, China and options of their reduction. Agric. Water Manage. 263 (H032649), 107468. doi: 10.1016/j.agwat.2022.107468
Ren, H., Jiang, Y., Zhao, M., Qi, H., Li, C. (2021). Nitrogen supply regulates vascular bundle structure and matter transport characteristics of spring maize under high plant density. Front. Plant Sci. 11. doi: 10.3389/fpls.2020.602739
Ren, B., Zhu, Y., Zhang, J., Dong, S., Liu, P., Zhao, B. (2016). Effects of spraying exogenous hormone 6-benzyladenine (6-BA) after waterlogging on grain yield and growth of summer maize. Field Crops Res. 188, 96–104. doi: 10.1016/j.fcr.2015.10.016
Salehi, M. (2022). Global water shortage and potable water safety; today’s concern and tomorrow’s crisis. Environ. Int. 158, 106936. doi: 10.1016/j.envint.2021.106936
Sampathkumar, T., Pandian, B. J., Mahimairaja, S. (2012). Soil moisture distribution and root characters as influenced by deficit irrigation through drip system in cotton–maize cropping sequence. Agric. Water Manage. 103 (103), 43–53. doi: 10.1016/j.agwat.2011.10.016
Sandhu, O. S., Gupta, R. K., Thind, H. S., Jat, M. L., Sidhu, H. S., Yadvinder, S. (2019). Drip irrigation and nitrogen management for improving crop yields, nitrogen use efficiency and water productivity of maize-wheat system on permanent beds in north-west India. Agric. Water Manage. 219, 19–26. doi: 10.1016/j.agwat.2019.03.040
Shu, G., Cao, G., Li, N., Wang, A., Wei, F., Li, T., et al. (2021). Genetic variation and population structure in China summer maize germplasm. Sci. Rep. 11 (1), 8012. doi: 10.1038/s41598-021-84732-6
Sinclair, T. R. (1998). Historical changes in harvest index and crop nitrogen accumulation. Crop Sci. 38 (3), 638–643. doi: 10.1016/j.agwat.2011.10.016
Song, H., Li, Y., Zhou, L., Xu, Z., Zhou, G. (2018). Maize leaf functional responses to drought episode and rewatering. Agric. For. Meteorol. 249, 57–70. doi: 10.1016/j.agrformet.2017.11.023
Srivastava, R. K., Panda, R. K., Chakraborty, A. (2020). Quantification of nitrogen transformation and leaching response to agronomic management for maize crop under rainfed and irrigated condition. Environ. pollut. 265, 114866. doi: 10.1016/j.envpol.2020.114866
Tavakkoli, A. R., Oweis, T. Y. (2004). The role of supplemental irrigation and nitrogen in producing bread wheat in the highlands of Iran. Agric. Water Manage. 65 (3), 225–236. doi: 10.1016/j.agwat.2003.09.001
Thomas, R. L., Sheard, R. W., Moyer, J. R. (1967). Comparison of conventional and automated procedures for nitrogen, phosphorus, and potassium analysis of plant material using a single digestion. Agron. J. 59 (3), 240–243. doi: 10.2134/agronj1967.00021962005900030010x
Tilling, A. K., O’Leary, G. J., Ferwerda, J. G., Jones, S. D., Fitzgerald, G. J., Rodriguez, D., et al. (2007). Remote sensing of nitrogen and water stress in wheat. Field Crops Res. 104 (1-3), 77–85. doi: 10.1016/j.fcr.2007.03.023
Tolk, J. A., Howell, T. A., Evett, S. R. (1999). Effect of mulch, irrigation, and soil type on water use and yield of maize. Soil Tillage Res. 50 (2), 137–147. doi: 10.1016/S0167-1987(99)00011-2
Tremblay, P., Grover, R., Maguer, J. F., Legendre, L., Ferrier-Pages, C. (2012). Autotrophic carbon budget in coral tissue: a new 13C-based model of photosynthate translocation. J. Exp. Biol. 215 (8), 1384–1393. doi: 10.1242/jeb.065201
Tuan, N. T., Qiu, J.-j., Verdoodt, A., Li, H., Van Ranst, E. (2011). Temperature and precipitation suitability evaluation for the winter wheat and summer maize cropping system in the Huang-Huai-Hai plain of China. Agric. Sci. China 10 (2), 275–288. doi: 10.1242/jeb.06520110.1016/s1671-2927(11)60005-9
Ullah, H., Santiago-Arenas, R., Ferdous, Z., Attia, A., Datta, A. (2019). “Chapter two - improving water use efficiency, nitrogen use efficiency, and radiation use efficiency in field crops under drought stress: a review,” in Advances in agronomy. Ed. Sparks, D. L. (Academic Press), 109–157.
Vejan, P., Khadiran, T., Abdullah, R., Ahmad, N. (2021). Controlled release fertilizer: a review on developments, applications and potential in agriculture. J. Controlled Release 339, 321–334. doi: 10.1016/j.jconrel.2021.10.003
Wang, S., Hu, Y., Yuan, R., Feng, W., Pan, Y., Yang, Y. (2019a). Ensuring water security, food security, and clean water in the north China plain – conflicting strategies. Curr. Opin. Environ. Sustain. 40, 63–71. doi: 10.1016/j.cosust.2019.09.008
Wang, Y., Li, S., Qin, S., Guo, H., Yang, D., Lam, H.-M. (2020). How can drip irrigation save water and reduce evapotranspiration compared to border irrigation in arid regions in northwest China. Agric. Water Manage. 239, 106256. doi: 10.1016/j.agwat.2020.106256
Wang, Y., Zhang, X., Chen, J., Chen, A., Wang, L., Guo, X., et al. (2019b). Reducing basal nitrogen rate to improve maize seedling growth, water and nitrogen use efficiencies under drought stress by optimizing root morphology and distribution. Agric. Water Manage. 212, 328–337. doi: 10.1016/j.agwat.2018.09.010
Wei, S., Wang, X., Li, G., Jiang, D., Dong, S. (2019). Maize canopy apparent photosynthesis and (13)C-photosynthate reallocation in response to different density and n rate combinations. Front. Plant Sci. 10. doi: 10.3389/fpls.2019.01113
Xiao, C., Zou, H., Fan, J., Zhang, F., Li, Y., Sun, S., et al. (2021). Optimizing irrigation amount and fertilization rate of drip-fertigated spring maize in northwest China based on multi-level fuzzy comprehensive evaluation model. Agric. Water Manage. 257, 107157. doi: 10.1016/j.agwat.2021.107157
Yan, S., Wu, Y., Fan, J., Zhang, F., Qiang, S., Zheng, J., et al. (2019). Effects of water and fertilizer management on grain filling characteristics, grain weight and productivity of drip-fertigated winter wheat. Agric. Water Manage. 213, 983–995. doi: 10.1016/j.agwat.2018.12.019
Yi, J., Li, H., Zhao, Y., Shao, M.a., Zhang, H., Liu, M. (2022). Assessing soil water balance to optimize irrigation schedules of flood-irrigated maize fields with different cultivation histories in the arid region. Agric. Water Manage. 265, 107543. doi: 10.1016/j.agwat.2022.107543
Yu, C., Huang, X., Chen, H., Godfray, H. C. J., Wright, J. S., Hall, J. W., et al. (2019). Managing nitrogen to restore water quality in China. Nature 567 (7749), 516–520. doi: 10.1038/s41586-019-1001-1
Zhang, H. (2003a). Improving water productivity through deficit irrigation: examples from Syria, the north China plain and Oregon, USA (International Water Management Institute).
Zhang, H. (2003b). Production and quality of the sugar beet (Beta vulgaris l.) cultivated under controlled deficit irrigation conditions in a semi-arid climate. Agric. Water Manage. 62 (3), 215–227. doi: 10.1079/9780851996691.0301
Zhang, F., Cui, Z., Chen, X., Ju, X., Shen, J., Chen, Q., et al. (2012). Chapter one – integrated nutrient management for food security and environmental quality in China. Adv. Agron. 116, 1–40. doi: 10.1016/B978-0-12-394277-7.00001-4
Zhang, L., Liang, Z., Hu, Y., Schmidhalter, U., Zhang, W., Ruan, S., et al. (2021b). Integrated assessment of agronomic, environmental and ecosystem economic benefits of blending use of controlled-release and common urea in wheat production. J. Cleaner Product. 287, 125572. doi: 10.1016/j.jclepro.2020.125572
Zhang, H., Ma, L., Douglas-Mankin, K. R., Han, M., Trout, T. J. (2021a). Modeling maize production under growth stage-based deficit irrigation management with RZWQM2. Agric. Water Manage. 248, 106767. doi: 10.1016/j.agwat.2021.106767
Zhang, Z., Yu, Z., Zhang, Y., Shi, Y. (2021c). Split nitrogen fertilizer applicationimproved grain yield in winter wheat (Triticum aestivumL.) via modulating antioxidant capacity and13C photosynthate mobilization under water-saving irrigation conditions. Ecol. Processes 10 (1), 21. doi: 10.1186/S13717-021-00290-9
Zhao, B., Shuting, D., Zhang, J.W.J.A.A.S. (2010). Effects of controlled-release fertilizer on yield and nitrogen accumulation and distribution in summer maize. Acta Agronom. Sin. 10 (2), 301–304. doi: 10.2966/scrip.100213.301
Zheng, W., Wan, Y., Li, Y., Liu, Z., Chen, J., Zhou, H., et al. (2020). Developing water and nitrogen budgets of a wheat-maize rotation system using auto-weighing lysimeters: effects of blended application of controlled-release and un-coated urea. Environ. pollut. 263, 114383. doi: 10.1016/j.envpol.2020.114383
Zheng, W., Zhang, M., Liu, Z., Zhou, H., Lu, H., Zhang, W., et al. (2016). Combining controlled-release urea and normal urea to improve the nitrogen use efficiency and yield under wheat-maize double cropping system. Field Crops Res. 197, 52–62. doi: 10.1016/j.fcr.2016.08.004
Zhu, X., Xu, K., Liu, Y., Guo, R., Chen, L. (2021). Assessing the vulnerability and risk of maize to drought in China based on the AquaCrop model. Agric. Syst. 189, 103040. doi: 10.1016/j.agsy.2020.103040
Keywords: blending controlled-release urea with conventional urea, deficit irrigation, summer maize, 13C-photosynthate distribution, nitrogen agronomic use efficiency, water use efficiency
Citation: Gu L, Mu X, Qi J, Tang B, Zhen W and Xia L (2023) Nitrogen reduction combined with ETc irrigation maintained summer maize yield and increased water and nitrogen use efficiency. Front. Plant Sci. 14:1180734. doi: 10.3389/fpls.2023.1180734
Received: 06 March 2023; Accepted: 06 April 2023;
Published: 22 June 2023.
Edited by:
Jiwang Zhang, Shandong Agricultural University, ChinaReviewed by:
Dalei Lu, Yangzhou University, ChinaCongfeng Li, Institute of Crop Sciences (CAAS), China
Copyright © 2023 Gu, Mu, Qi, Tang, Zhen and Xia. This is an open-access article distributed under the terms of the Creative Commons Attribution License (CC BY). The use, distribution or reproduction in other forums is permitted, provided the original author(s) and the copyright owner(s) are credited and that the original publication in this journal is cited, in accordance with accepted academic practice. No use, distribution or reproduction is permitted which does not comply with these terms.
*Correspondence: Laikun Xia, xialaikun@126.com; Wenchao Zhen, wenchao@hebau.edu.cn
†These authors have contributed equally to this work