- 1Indian Council of Agricultural Research (ICAR)-National Bureau of Plant Genetic Resources, Regional Station, Shimla, India
- 2Indian Council of Agricultural Research (ICAR)-National Bureau of Plant Genetic Resources, New Delhi, India
- 3Indian Council of Agricultural Research (ICAR)-Indian Institute of Pulses Research, Kanpur, India
- 4Alliance of Bioversity International and International Center for Tropical Agriculture (CIAT), India Office, National Agricultural Science Complex, New Delhi, India
Moth bean (Vigna aconitifolia) is an orphan legume of Vigna genus, exhibiting wide adaptability and has the potential to grow well in arid and semi-arid areas, predominantly across different eco-geographical regions of Asia, particularly the Indian subcontinent. The inherent adaptive attributes of this crop have made it more tolerant towards a diverse array of abiotic and biotic stresses that commonly restrain yield among other Vigna species. Additionally, the legume is recognized for its superior nutritional quality owing to its high protein content as well as amino acid, mineral and vitamin profile and is utilized as both food and fodder. Moth bean can play a vital role in sustaining food grain production, enhancing nutritional security as well as provide a source of income to resource-poor farmers amid rise in global temperatures and frequent drought occurrences, particularly in rain-fed cropping systems which accounts for about 80% of the world’s cultivated land. However, this minor legume has remained underutilized due to over-exploitation of major staple crops. With the exception of a few studies involving conventional breeding techniques, crop improvement in moth bean for traits such as late maturity, indeterminate growth habit, shattering and anti-nutritional factors has not garnered a lot of attention. Recent advances in sequencing technologies, modern breeding approaches and precision phenotyping tools, in combination with the available crop gene pool diversity in gene banks, can accelerate crop improvement in moth bean and lead to the development of improved cultivars. Considering the recent surge in awareness about the development of climate-smart crops for sustainable agricultural future, collective effort towards effective utilization of this hardy, neglected legume is the need of the hour.
1 Introduction
Agricultural sustainability is stymied by excessive reliance on major staple crops, climate change as well as deterioration of cultivable land. These limitations extend a challenge to global food security while simultaneously accentuating rural poverty and malnutrition in developing and under-developed nations. Many crops are missing from agricultural fields while others have seen a slump in both cultivation and usage. Evolution of new pests and pathogens along with enhanced crop uniformity in farmers’ field are posing a serious threat to crop production. Tackling global food demand and hidden hunger warrants a radical shift from current unsustainable agricultural practices and call attention to alternative potential future crops. Introduction of new crop species in cropping systems and enhancing crop diversity through the utilization of diverse germplasm can play vital role in global food and nutritional security. Underutilized plants are the potential genetic resources to be exploited to address the decreasing nutritional quality and stress resilience in the already mainstreamed cultivars. ‘Underutilized’ plant species are those that had been grown widely in the past or have the capability to be grown extensively in the future, however, are cultivated restrictively owing to agronomic, economic or genetic causes (Gruère et al., 2006). They are also known as ‘orphan’ or ‘neglected’ crops owing to insufficient information available about them as a result of sparse consideration from research and development (Eyzaguirre et al., 1999). These crops remain ‘underexploited’ or ‘minor’ since their economic potential has not been ascertained (Padulosi and Hoeschle-Zeledon, 2004).
Pulse crops are exceptional sources of quality protein, in addition to being utilized for their medicinal properties, as fodder for livestock, for enriching soil as a result of symbiotic relationship with nitrogen-fixing bacteria and mitigating greenhouse emissions (Lemke et al., 2007; Singh et al., 2007; Stagnari et al., 2017). The current scenario of booming food insecurity signals an urgency to reformulate and steer crop improvement and production agronomy strategies, in the coming decades, towards grain legumes to successfully determine climate-resilient species having enhanced grain attributes (Considine et al., 2017). Crop diversification, alternative cropping systems and value-added products’ development are significant contributions made by underutilized legumes, to the life of local communities. Mostly, these minor pulses are adapted to marginal lands. The genetic resources of these orphan plants face rapid destruction owing to changes in traditional food habits and depreciation of traditional farming culture along with the introduction and acclimatization of high yielding crops. Therefore, mainstreaming of these underutilized crop plants, essentially minor legumes, should be set in motion in order to impede global food concerns.
Moth bean [Vigna aconitifolia (Jacq.) Maréchal syn. Phaseolus aconitifolius Jacq. (2n= 2x=22)] is an underutilized, minor grain legume. It is also known as mat bean, math, mattenbohne, matki, dew bean, Turkish gram or haricot papillon (Heuzé et al., 2020). Moth bean is an annual herbaceous trailing legume of warm and dry habitats, particularly the semi-arid and arid regions of Indian subcontinent. It is principally grown for its protein-rich seeds, sprouts and green pods that are used as vegetable, apart from being equally important as feed and fodder for livestock and as a cover crop as well as green manuring, hence serves as multipurpose crop (Saravanan and Ignacimuthu, 2015) (Figure 1). Integrated consumption of moth bean like pulse legume crops with cereals is expedient as they have a complementary relationship, for moth is rich in lysine and leucine and cereals supply sulphur-containing amino acids, thereby supplementing mutual amino acid deficiencies.
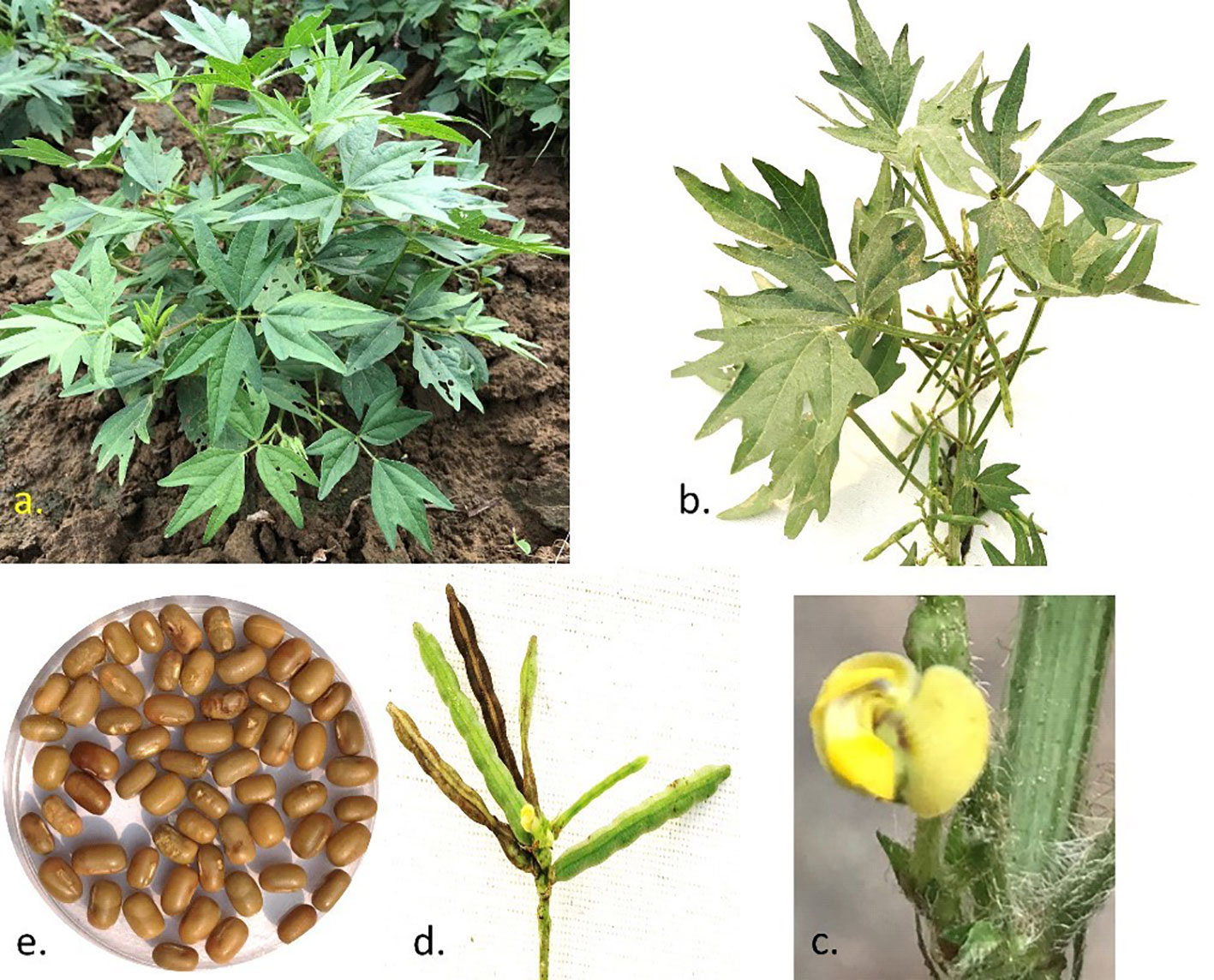
Figure 1 Highlights of the morphological features of Moth bean: A typical plant type with central branch and trailing primary branches (A), Inflorescence and pods in a branch (B), papilionaceous flower (C), semi-erect pods arrangement in peduncle (D) and seed shape and size (E). Seeds are placed in a 2.5 cm dish.
As a prospective major legume crop, Vigna aconitifolia is quite notable for being the most drought hardy and heat tolerant pulse among Asian Vignas (Tomooka et al., 2011). It is usually grown on marginal and sub-marginal lands with poor moisture holding capacity. Having a deep, fast penetrating tap root system and a dense low-lying leaf cover that resembles a mat, it can withstand lack of water and drying, hot temperatures (∼ 40°C), by keeping the soil moist as well as lowering soil temperature, thereafter also reducing the possibility of soil erosion. The multi-adaptive nature of moth bean, in addition to requiring little to no care and input, has led it to being recognized as an arid legume. Notwithstanding the attributes of moth bean that makes it a future legume for sustainable agriculture, it is only grown limitedly and there has been a precipitous reduction in the cultivable area and production of the legume over the past decade (Figure 2) owing to agronomic bottlenecks viz., low variability, slow growth, longer maturation duration, substandard yields due to poor seed set and below par response to fertilizer treatments (Harsh et al., 2016). Its competence, with respect to agronomy, genetics and nutrition has been vastly overlooked. This review attempts to encapsulate the work done in Vigna aconitifolia, in an effort to harness its potentialities to curb the unprecedented challenges to global agriculture.
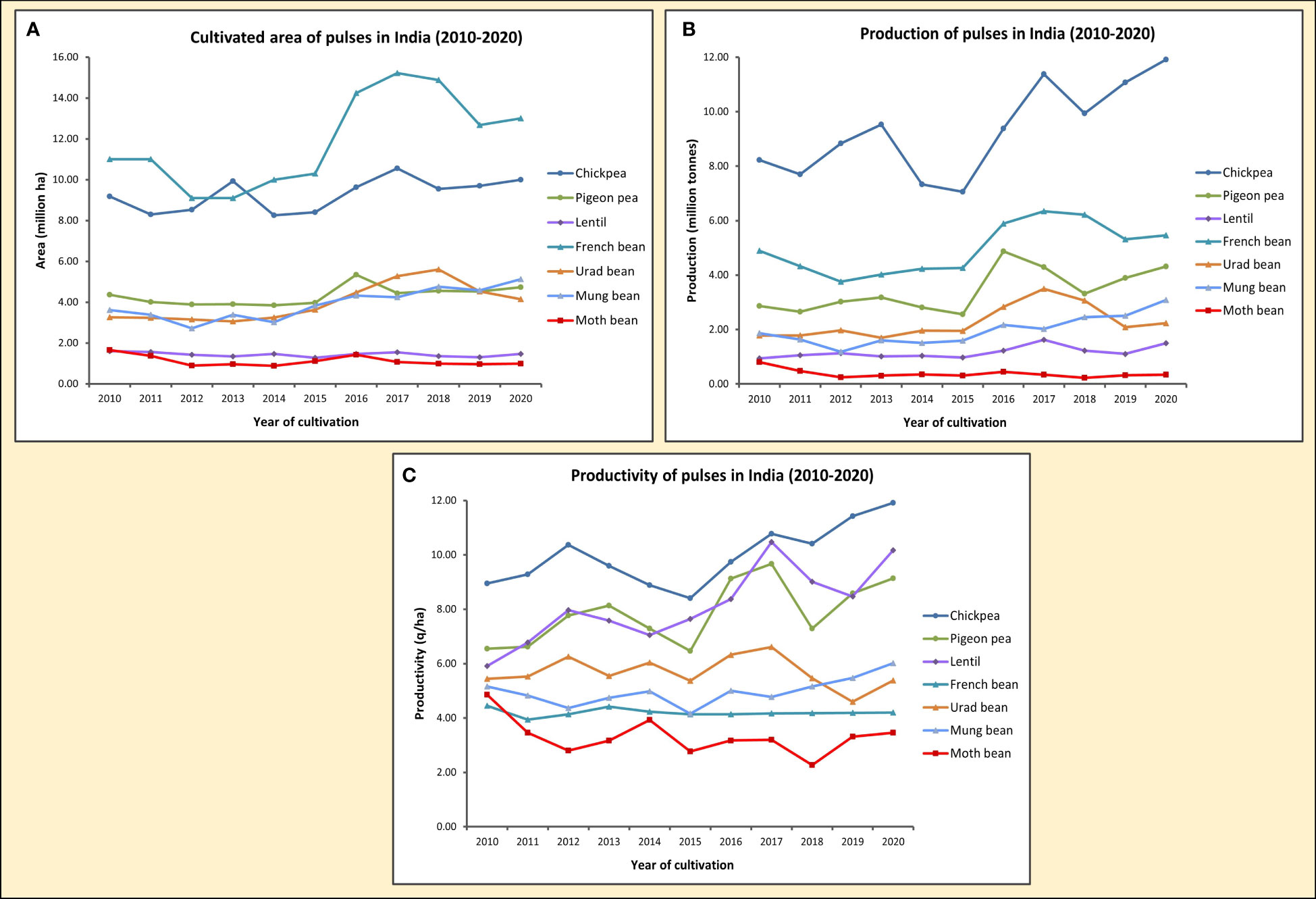
Figure 2 Graphs showing the cultivated area in million ha (A), production in million tonnes (B) and productivity in q/ha (C) of seven pulse crops, including Moth bean, in India from 2010-2020. [Source: Directorate of Economics and Statistics, India (eands.dacnet.nic.in); Food and Agriculture Organization Corporate Statistical Database (FAOSTAT) (www.fao.org/faostat)].
2 Origin and distribution
Moth bean is considered to be a native of India, Pakistan, Myanmar and Sri Lanka (Piper and Morse, 1914; Purseglove, 1974; Rachie and Roberts, 1974; Jain and Mehra, 1980). Vavilov (1926) and De Candolle (1986) reported India as the center of origin for wild and cultivated forms of V. aconitifolia (Jacq), and Maréchal (1978) proposed that Sri Lanka and Pakistan are the centers of diversity of this minor legume. Dana (1976) proffered that the wild forms of moth bean were distributed from Sonoran Desert of Mexico to Tapachula, near the Guatemalan border in Central America.
Vigna aconitifolia is principally cultivated in India. It is also grown in Sri Lanka, Myanmar, China, Pakistan, Malaysia, Africa and is also grown in the South-Western states of the USA (Jain and Mehra, 1980; Munro and Small, 1998; Brink and Jansen, 2006; Kochhar, 2016). In India, it is distributed from the North-West Himalayas (up to the height of 6000 m) to Karnataka in south and from the foot hills of North-East Himalayas in the east to Saurashtra in the west (Arora, 1985). Moth bean is essentially grown in the arid and semi-arid regions, particularly in the North-Western states. Rajasthan, Maharashtra, Gujarat, Punjab, Haryana, Jammu and Kashmir, Madhya Pradesh and Uttar Pradesh are the prime moth bean growing states (Arora, 1985) (Figure 3). Rajasthan, the driest state in India, contributes the most, both in terms of production as well as area, at national level (Gupta et al., 2016; Viswanatha et al., 2016).
3 Taxonomy and phylogenetic relationship
Moth bean belongs to the genus Vigna and sub-family Papilionoideae which comes under the family Fabaceae. It is considered as one of the most primitive species among the Vigna genus, with respect to its evolution (Smartt, 1985). Earlier, moth bean or Dew gram (Phaseolus aconitifolius Jacq. syn. Vigna aconitifolia (Jacq.) Marechal) was a part of the genus Phaseolus. Thereafter, it was shifted to the Vigna genus of the tribe Phaseolae. Vigna and Phaseolus, together, form a very elaborate taxonomic group called the Phaseolus-Vigna complex. Verdcourt (1970) proposed that the genus Phaseolus be restricted to exclusively include those American species that have a tightly coiled style and pollen grains without course reticulation, thereby significantly promoting the concept of Vigna. In the taxonomic revision made by Verdcourt (1970) in Phaseolus Linn. and Vigna savi, species with yellow-colored flowers, under subgenus Ceratotropis Piper, were transferred to Vigna genus, Ceratotropis Verdc. Subgenus. This led to an increase in the number of species included in the genus Vigna. Maréchal (1978) followed Verdcourt by presenting a monograph on the Phaseolus-Vigna complex and also proposed nomenclatural changes, following which, Phaseolus aconitifolius (Jacq.) became Vigna aconitifolia (Jacq.) Marechal. This taxonomic system is generally accepted now.
The wild, conspecific forms as well as cultigens of moth bean are not recognized (Maréchal, 1978). The precursor of moth bean is thought to be Phaseolus trilobata (L.) (syn. Phaseolus trilobus), which is a wild species endemic to India, with both being diploid and having chromosome number 2n=22 (Darlington and Wylie, 1955; Biswas and Dana, 1976a). Phaseolus trilobata and Phaseolus aconitifolia were considered to be equivalent by some while others believed Phaseolus trilobata to be the wild form of Phaseolus aconitifolia, however, studies have established both of them to be different (Sampson, 1936; Whyte et al., 1953; Klozová, 1965; West and Garber, 1967). Similarly, Vigna aconitifolia was classified with Vigna radiata and Vigna mungo, nonetheless, RAPD analysis to study genetic variability showed interspecific differences among them (Dana, 1980; Kaga et al., 1996). Takahashi et al. (2016) have performed molecular phylogenetic analysis of Vigna species using rDNA-ITS and atpB-rbcL sequences which divided the Aconitifoliae section into various branches, of which V. aconitifolia is one (Figure 4).
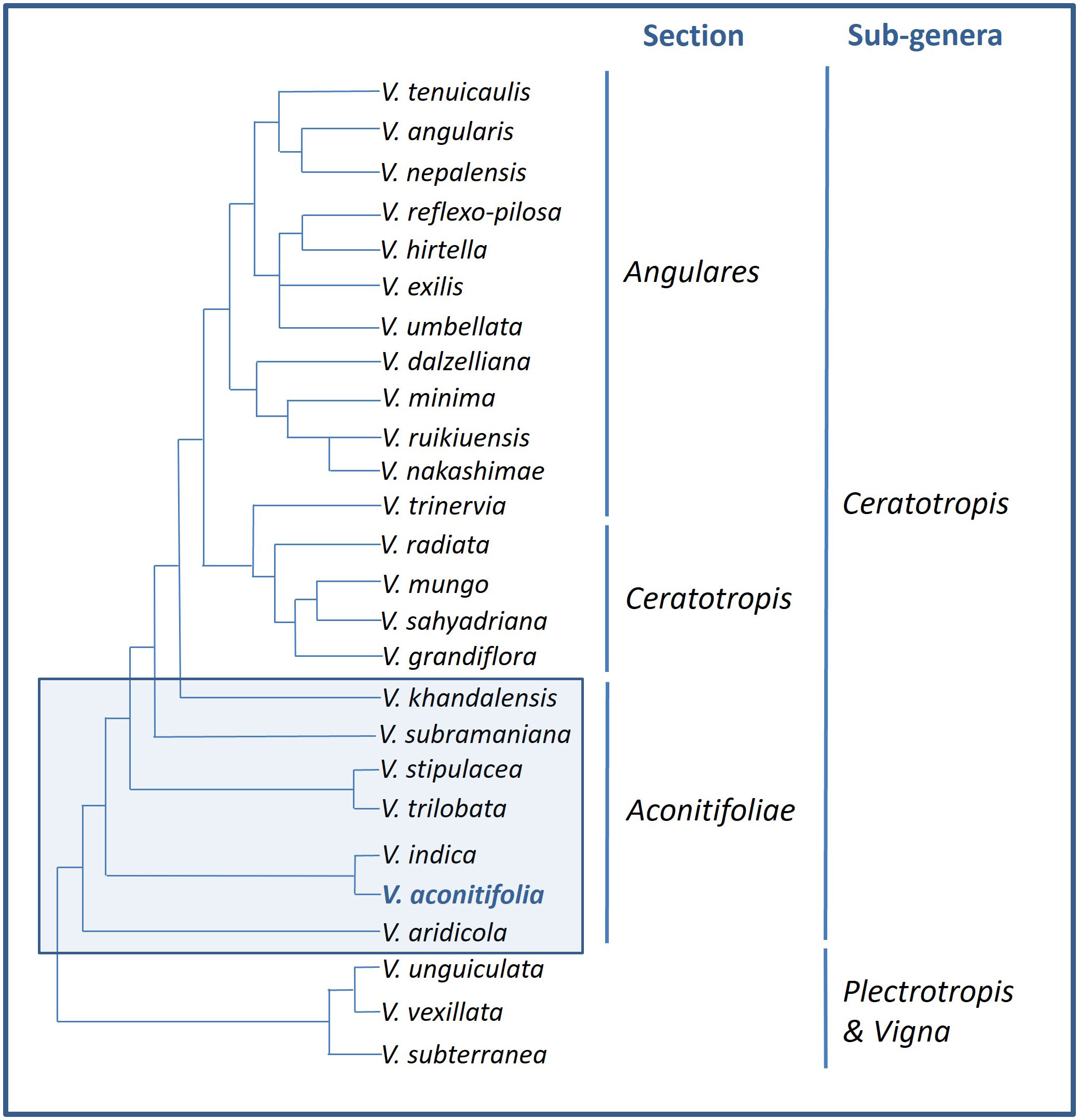
Figure 4 Taxonomic relationships among moth bean and other species of the genus Vigna [Source: Adapted from Takahashi et al. (2016)].
4 Nutritional profile
Moth bean is primarily consumed by people in the arid and semi-arid regions of South Asian countries, particularly India (Adsule, 1996; Takahashi et al., 2016). Ripe seeds are often consumed whole or split and can be cooked, roasted or fried. In India, a popular use of moth bean seeds is in the form of ‘dal’ (bean stew) or ‘bhujia’ (crispy snack), in addition to being used in the preparation of papad, mangori, rabri and vada (moth bean fritters) (Senthilkumar and Ngadi, 2020). The seeds are also ground into flour and often mixed with other flours to make unleavened bread (Brink and Jansen, 2006). Sprouted as well as cooked seeds are a popular breakfast dish while fried, split moth bean can be readily consumed (Medhe et al., 2019). Boiled immature green pods are consumed as a vegetable. As a medical use, the seeds are used to treat fevers. The roots are found to be narcotic (Brink and Jansen, 2006). Other pharmacological properties of Moth bean include anti-hypertensive, anti-oxidant, anti-cancerous, anti-bacterial, diuretic and hypocholesteromic activities (Adsule, 1996; Ma et al., 2010; Saravanan and Ignacimuthu, 2015; Panicker and Hamdule, 2021; Verma et al., 2021). Moth bean also contributes indirectly to human nutrition by being used as fodder for livestock.
In terms of the nutritional aspects of moth bean, it is considered as a good and inexpensive source of protein in cereal-based vegetarian diets among the people of developing nations. The protein content in moth bean seeds ranges from 20 to 24% (Gopalan et al., 1989; Adsule, 1996; Longvah et al., 2017). It is rich in the essential amino acids, lysine (6.63 g/100 g protein) and leucine (7.85 g/100 g) that are deficient in cereals and thus complements the amino acid profile of cereals. Among the total soluble protein present in moth, the glutelin fraction was found to be the highest at 63.93 g/100 g of total soluble protein, followed by glutelin, albumin and prolamin (Sathe and Venkatachalam, 2007). Additionally, the total carbohydrate content in moth bean was found to range from 52 to 68%, the total fat ranged from 1.1 to 3.9% while the crude fiber content ranged from 3.9 to 4.5%. Moth bean is also found to be rich in minerals such as calcium, magnesium, iron, phosphorus and potassium as well as vitamins like niacin, thiamine and riboflavin (Longvah et al., 2017; USDA FoodData Central, 2019). A comparison of the nutritional profile of moth bean with other pulses is illustrated in Table 1.
Moth bean, like other pulses, also contains flatulence-producing oligosaccharides such as raffinose, stachyose and verbascose. Also, like other legumes, it contains anti-nutritional and phytonutrient factors that affect the nutrient bioavailability as well as palatability and digestibility of moth bean. These factors include trypsin inhibitors, phytic acid, saponins and phyto-haemagglutinins (lectins). Trypsin and chymotrypsin inhibitors have been involved in reducing protein digestibility and thereafter in pancreatic hypertrophy (Liener, 1976). Phytic acid interferes with the bioavailability and absorption of minerals in the body and inhibits proteolytic enzymes and amylases while saponins are gastric irritants (Davies and Nightingale, 1975; Erdman, 1979; Singh and Krikorian, 1982; Deshpande and Cheryan, 1984; Pugalenthi et al., 2005; Bouchenak and Lamri-Senhadji, 2013). However, food preparation techniques, such as soaking, sprouting, boiling, pressure cooking as well as fermentation of moth, aid in improving the taste as well as the bioavailability of nutrients as they deactivate these anti-nutritional factors besides allowing the digestion and assimilation of its starch and protein (Khokhar and Chauhan, 1986; Kigel, 1999; Xu and Chang, 2008; Deshmukh and Pawar, 2020).
Regardless of being nutritionally dense, the present consumption levels of moth bean are rather low which can be attributed to its underutilized status. While there are indirect evidences that support its role in reduction of diseases, they are more based on its nutritional composition, therefore, focused research on the clarification of the health effects of moth bean should be undertaken.
5 Crop improvement
5.1 Plant genetic resources (PGR): status and trait discovery
Major moth bean ex-situ collections are maintained by the Indian National Gene bank, ICAR-National Bureau of Plant Genetic Resources, New Delhi, India. In India, systematic exploration and collection of the germplasm work commenced as back early as the 1940s, with the establishment of the Plant Introduction unit in the Division of Botany, IARI (Indian Agricultural Research Institute), New Delhi. Intensive collection efforts, carried out by National Bureau of Plant Genetic Resources, from 1971 till date, have resulted in the assembly of 3422 accessions of moth bean. These accessions include primitive cultivars/landraces, primarily from the states of Rajasthan, Gujarat, Maharashtra, Karnataka, western Uttar Pradesh, Punjab, Haryana and Madhya Pradesh. Variations in growth habit, leaf location as well as pod and seed color were observed in major collections of V. aconitifolia. The collections made from Rajasthan and Gujarat have turned out to be more promising and are currently being utilized in extensive characterization and evaluation programmes, to identify superior genotypes (Bisht and Singh, 2013). Besides germplasm collections from within the country, NBPGR further plays a crucial role in the augmentation of the germplasm from other countries as well. 41 accessions of moth bean have been introduced from Sri Lanka, USA (19), Mexico, USSR, Thailand (5) and others (14). Other small collections are being held by institutions worldwide are University of Georgia, USDA (56 accessions), VIR (48), Australian Grain Gene bank (35), AVRDC-The World Vegetable Centre (26), CIAT, Columbia (8) and Leibniz Institute of Plant Genetics and Crop Plant Research (6) (Table 2; Figure 3). Moth bean has orthodox seeds that can be dried and stored for a longer period with minimum to no loss of viability. A limited range of germplasm accessions can also be found in countries such as Bangladesh, Belgium and Kenya. Active collections are being maintained at NBPGR, Regional Station, Jodhpur (Rajasthan). The working collections of a range of Vigna species are also maintained at the Indian Institute of Pulses Research (IIPR), Kanpur, India and its coordinating centers (Asthana, 1998). More than 2000 accessions of moth bean have been characterized and evaluated at NBPGR, Regional Station, Jodhpur (Singh et al., 2001). Yield and other growth characteristics have shown a wide range of variation, as demonstrated in Table 3. Nodulation and nitrogenase activity as well as resistance to insect pests and diseases have shown extensive varietal divergence in moth bean (Rao et al., 1984; Dabi and Gour, 1988). Quality characters have also demonstrated a substantial amount of variability (Figure 5).
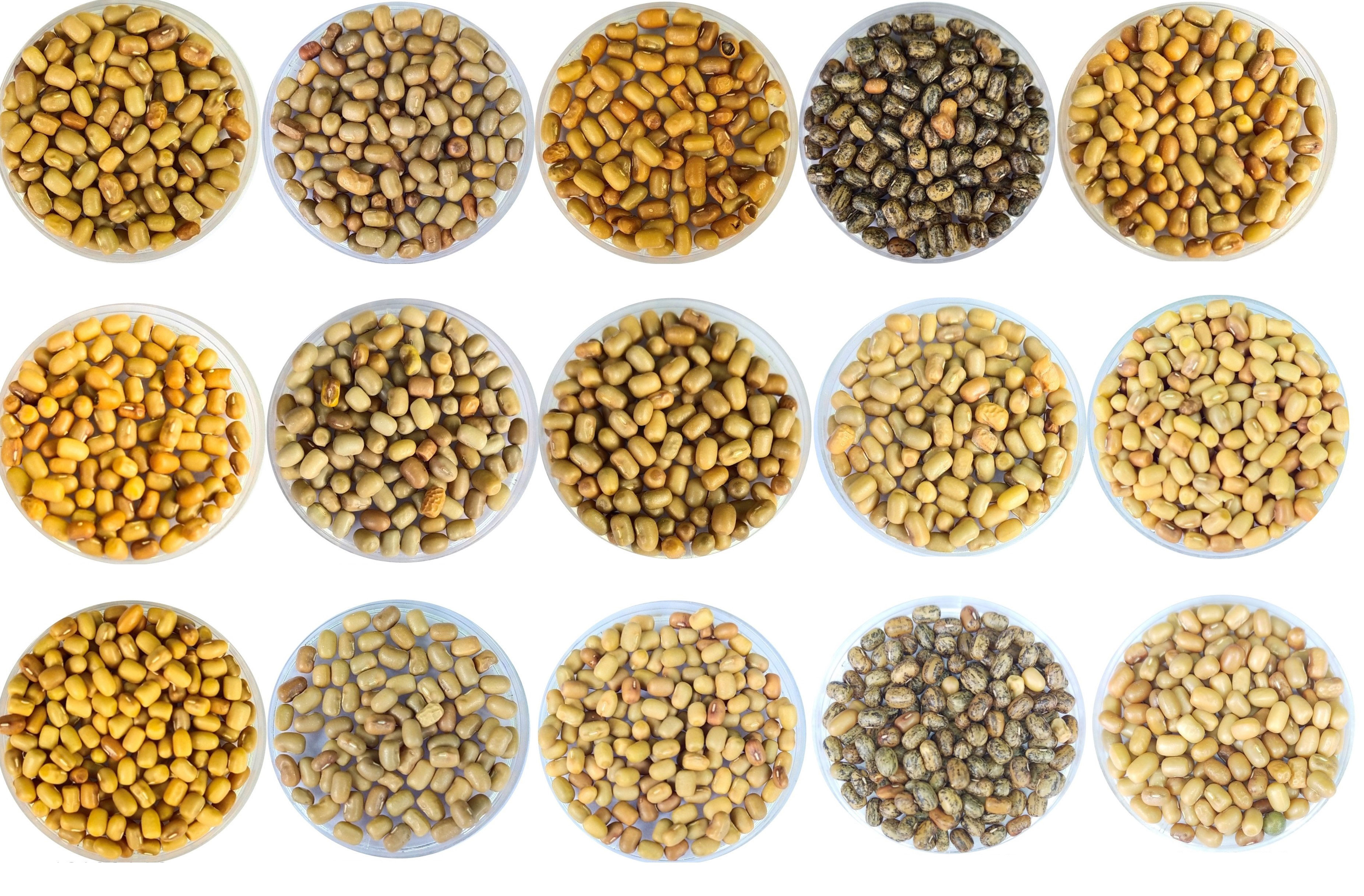
Figure 5 Variability in the shape, size and color of Vigna aconitifolia seeds conserved in the Indian National Gene bank, New Delhi.
5.2 Conventional breeding
Initially, moth bean breeders focused mainly on the maintenance of crop genetic resources, germplasm evaluation, and genetic diversity assessment (Cullis and Kunert, 2017). Owing to the difficulty in handling the tiny flowers that drop off during crossing, hybridization programmes were unsuccessful in minor legumes like moth bean resulting in lack in genetic studies. However, studies on germplasm evaluation resulted in the identification of promising genotypes in addition to providing information on diversity, heritability, and other genetic parameters as well as the correlation between traits and environmental influences that could be useful in expediting genetic gains (Vir and Singh, 2015; Kohakade et al., 2017; Sahoo et al., 2019; Pal et al., 2020; Chaudhary et al., 2021). A few promising accessions identified through germplasm evaluation, are IC36245, IC36555, IC36667, IC36577 and IC36604 (Vir and Singh, 2015). Likewise, Sihag et al. (2004) reported that in terms of the traits studied, MH 65, MH 34/66, MH 45, and MH 66 were the most promising genetic stocks, and their use in the moth bean varietal improvement programme could be propitious. Similarly, Kohakade et al. (2017) identified five promising genotypes (DHMB-32, DHMB-26, DHMB-31, DHMB-30 and DHMB-16) under the hybridization programme based on inter-cluster distances, cluster mean and per se performance. Systematic germplasm evaluation studies by various workers have identified valuable trait-specific accessions for moth bean improvement (Table 4). In moth bean breeding programmes, these accessions could be a valuable resource for improving moth bean resistance to major biotic and abiotic stresses and for enhancement of grain yield. Variety development programme in moth bean was probably initiated in the late 1960 or early 1970s (Singh and Mann, 1976). Traditional germplasm-selected varieties were of spreading types that covered the ground like a mat, grew slowly, and matured in 100-120 days. These varieties (Type-I, Type-3, MG-l, Baleswar-12, and so on) were introduced prior to 1970. However, under ideal conditions, these fodder types barely give about 200-300 kg/ha of yield (Kumar, 2002). Further selections from the germplasm resulted in identification of high yielding varieties with long and medium duration (Maru moth) maturity before the inception of AICRP on Arid legumes in 1992 (Table 5). Work on arid legumes viz., cluster bean, moth bean, cowpea and horse gram started in 1992. High-yielding varieties that mature in 75-90 days have thus been developed as a result of continuous, sincere, and deliberate efforts to improve this crop’s genetics and yield. As a result, the newer cultivars (Jadia, Jawala, IPCMO-912, and CAZRI moth-1) could produce higher yield while also withstanding the extreme heat and limiting soil moisture conditions. These varieties were also better suited to areas with high levels of rain (250- 350 mm) (Kumar, 2002). In 2003, CAZRI moth-2 (CZM-2) was developed by Central Arid Zone Research Institute, which was the first variety to be released through hybridization and has gained wide popularity in recent years owing to its high yield potential and considerable podding (Solanki et al., 2018). In addition to these, its introduction to the marginal irrigated farming system, development of early maturing, photosynthetically efficient plants, breed of input responsive genotypes, development of varieties suitable for inter/mixed cropping system, identification of ‘dual types’ (high grain as well as fodder yielder) and development of varieties resistant to biotic and abiotic factors are some of the priority areas which should be taken care of in future genetic improvement programmes on moth bean.
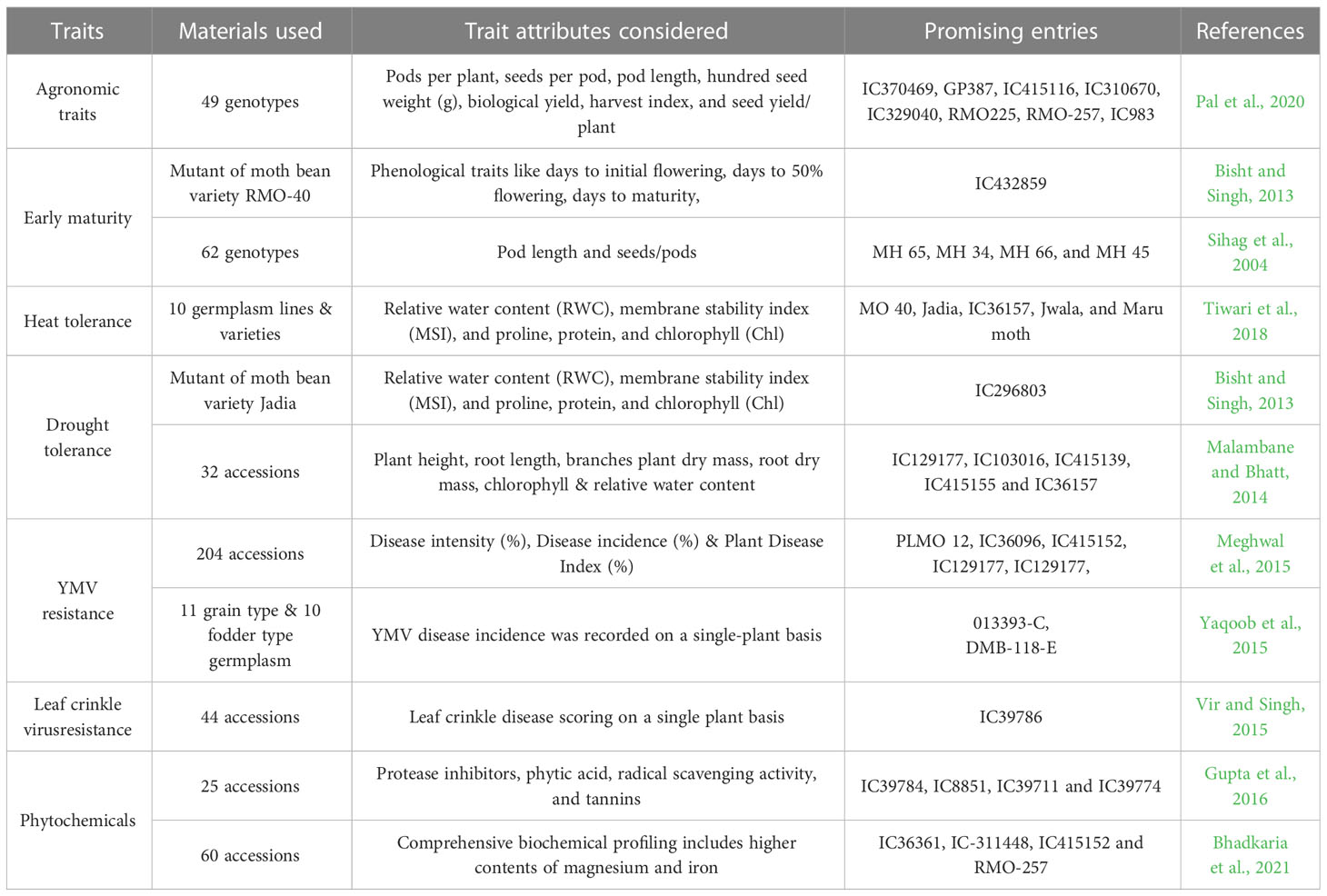
Table 4 Potential trait-specific accessions identified in germplasm for utilization in moth bean improvement.
5.3 Interspecific hybridization
In India and other Asian countries, Vigna species constitute an economically significant group of 10 cultivated crops (domesticated species) and more than 100 wild species (Schrire et al., 2005; Bisht and Singh, 2013; Takahashi et al., 2016). Moth bean is the most interesting of the Asian Vigna species that have been domesticated as it is highly resistant to drought and heat (Tomooka et al., 2014). The wild ancestor of the moth bean hasn’t been precisely described, but it is said to have occurred in India (Arora and Nayar, 1984). Understanding the genetic basis of crop domestication aids in the identification of beneficial genes (or genes) in wild relatives of cultivated crops that can be used in plant breeding. In addition, as a reliable strategy for expanding a crop species’ genetic base, interspecific hybridization has successfully introduced beneficial traits from wild relatives into closely related crops (Singh, 1990). Varying degrees of success in interspecific hybridization of Vigna has been reported (Chen et al., 1983; Singh, 1990; Pandiyan et al., 2012; Tomooka et al., 2014; Basavaraja et al., 2019). Cross-compatibility between V. aconitifolia and other relative species hasn’t been studied much, but it has been crossed as a seed parent with V. trilobata, and V. trilobata has been crossed with success, as a pollen parent, with V. mungo, V. radiata, and V. aconitifolia, but the reciprocal hasn’t reportedly worked (Bisht et al., 2005). The interspecific hybrids between V. aconitifolia and V. trilobata produced viable seeds. The F1 hybrids had 5.7% pollen fertility, but complete seed sterility. Colchicine-induced amphiploids had 89.7% pollen fertility and such plants had fairly regular meiosis, suggesting the possibility of such crosses. Hybrid sterility is segregational in nature (Biswas and Dana, 1976b).
5.4 Tissue culture approach
Through interspecific hybridization, efforts have been made in the recent past to develop disease-resistant as well as high-yielding varieties of moth bean. However, interspecific cross incompatibility and hybrid sterility are some significant hurdles for moth bean variety development programme. Consequently, it is conceivable that moth bean quality and yield can be improved by using a combination of tissue culture techniques and traditional breeding methods. Tissue culture is a potent method that enables scientists to cultivate and manipulate plants in a sterile environment. It is an advantageous method for plant breeding. Therefore, cell and tissue culture application to moth bean assumes a special significance. However, the lack of or low rate of plant differentiation from cultured cells is one of the major obstacles towards the utilization of cell and tissue culture techniques in grain legume breeding programmes (Bajaj and Gosal, 1981; Gresshoff and Mohapatra, 1982; Mroginski and Kartha, 1984; Saxena, 2004). Several studies have reported that many legumes have now been successfully cultured and regenerated as whole plants. Bhargava and Chandra (1983) initiated callus cultures of two cultivars of Vigna aconitifolia (moth bean) (IPCM0-926, RDM-120) and studied their growth and differentiation. Likewise, Godbole et al. (1984) optimized the cultural conditions for whole moth bean plant regeneration from explant shoot apices and cotyledons, callus cultures derived from explant shoot apices, and field transfer of rooted plantlets. As a practical issue, the development of shoots and subsequent plantlets from excised roots is of interest because it provides a new source of somaclonal variation. Gill and Eapen (1986) attempted to develop cell and tissue cultures in moth bean, whereby a successful attempt was made in moth bean plant regeneration from hypocotyl protoplasts. Eapen and Gill (1986) were able to be regenerate plants from root explants of moth bean by culturing on Murashiage and Skoog’s basal medium without any phytohormone, but the addition of cytokinins increased the frequency of plant regeneration.
Protoplasts, on the other hand, are also an excellent source of inducing variation in Vigna. Protoplasts from this important group of economic crops have been isolated and regenerated into cell colonies and callus tissues. Callus cultures derived from shoot apices of moth bean provided a high yield of viable moth bean protoplasts as a starting point for the development of an efficient and reproducible moth bean protoplast isolation and regeneration method (Krishnamurthy et al., 1984). Similarly, Arya et al. (1990) regenerated plants from leaves’ protoplasts of Vigna aconitifolia ‘Jadia’ and reported that protoplasts from highly homozygous legumes are of application in moth bean improvement. Kumar et al. (1988) demonstrated successful plant regeneration from cell suspension cultures lines of Vigna aconitifolia in L-6 medium containing 44.5 M 2,4-D, which resulted in the highest growth rate. Till date, no systematic study has been reported in moth bean on the effects of cultivar, basal medium, different medium combinations (Murashiage and Skoog medium (MS), 2,4-D & 6-Benzylaminopurine (BAP) and interactions on callus induction, callus propagation and subsequent plant regeneration (Bhargava and Chandra, 1983; Bhargava and Chandra, 1989). Furthermore, Jangid et al. (2010) studied factors affecting callus induction in moth bean and found significant differences, for both callus initiation days and callus fresh weight, between the varieties, explants, medium combinations, and their two- and three-way interactions while also reporting that MS medium supplemented with 1.0 mg-1, 2-D was the best medium for maintaining callus in all of the tested types. There have been numerous attempts made to develop moth bean regeneration protocols in vitro (Arya et al., 1990; Chandra and Pental, 2003; Kaviraj et al., 2006). When tested on Indian moth varieties, the in vitro regeneration techniques developed by these researchers failed to deliver the expected outcomes. Hence, Choudhary et al. (2009) developed an effective method for regenerating moth bean using somatic embryos and in vitro-grown plantlets that resulted in well-formed roots being successfully hardened and established in soil. Recently, regeneration potential, gene expression, and genetic stability were found to be affected by heat treatment in moth bean (Jangid et al., 2010). Additionally, the response of tissue to callus formation was studied in explants that had been heated at 37, 42, or 47°C for 10 minutes (Sharma et al., 2018). The results showed that most of the heat treatments slowed down regeneration, and a few polypeptides in the protein profile of the callus were both up-regulated and down-regulated by the heat treatments. Understanding the mechanism of flowering regulation in in-vitro cultures is practical and theoretically relevant. The effect of growth regulators such as abscisic acid and proline on in vitro flowering manipulation in moth bean has been investigated by Saxena et al., 2008. On the contrary, moth bean presents an efficient system to be used for in vitro mutagenesis because it exhibits an efficient regenerability from various explants and bear seeds under in vitro conditions (Saxena et al., 2006). Likewise, Narayan et al. (2015) demonstrated that gamma radiation exposure increased the number of shoot bud primordia and the number of shoots per explant in callus generated from primary leaves of seedlings of moth bean seeds. Such explants also resulted in a higher number of flowering plantlets under in vitro growth conditions. Considerable success has been achieved in moth bean in vitro culture plant regeneration, and this progress offers an alternative option, over conventional breeding or interspecific hybridization, for the improvement of moth bean.
5.5 Mutation breeding
Mutation is a highly effective technique to generate the desired variation in crop plants. It has been extensively used in many of the world’s most important crops, including wheat, rice, pulses, millets, and oilseeds, and several original and review studies show its effectiveness (Khadke and Kothekar, 2011; Raina et al., 2016; Jegadeesan and Reddy, 2018; Pandit et al., 2021). Work on the varietal improvement of moth bean crop is minimal; a mutation breeding programme in moth bean was taken up in 1983, to isolating mutants with high yield potential, early maturing and at the same time possessing tolerance to drought conditions. Henry and Daulay (1983) researchers examined the performance of induced mutants in moth bean, treating seeds of the Jadia variety with aqueous solutions of EMS at doses ranging from 0 to 3 percent. In the M2 generation, they found 25 mutants with higher pods than their parents. The mutants were further carried forward up to M5 and M6 generation and tested under dry land conditions for yield performance, along with other high-yielding varieties. On the contrary, to improve arid legumes, a mutation breeding technique utilizing chemical and physical mutagens was undertaken, (Ramkrishna et al., 2008; Jain et al., 2013). The most commonly used mutagens, namely, Ethyl methane sulphoanate (EMS), Methyl methane sulphonate (MMS), Sodium azide (SA) and Hydroxylamine (HA) were used in moth mutation breeding and the effectiveness of the mutagens was tested on three different bean crops. Moth bean genotypes had the highest efficiency, followed by cluster bean, while mung bean genotypes had the lowest efficiency (Sharma and Kakani, 2002; Ramkrishna et al., 2008). Four moth bean varieties, RMO-40, RMO-257, Jwala and CZM-1, were tested for their response to EMS, MMS, SA, and HA, induced biological damage, polygenic variability, and comparative mutagenic effectiveness and efficiency. This was performed to better understand how different moth bean varieties respond to different types and treatments of chemical mutagens. Subsequently, the mutagenic progenies, concerning yield and yield attributes in M2 and M3 generation, were evaluated. Treatment efficacy declined in the following order: MMS > SA> EMS > HA. The moth bean variety, RMO-40, was shown to have the maximum mutagenesis effectiveness with a concentration of 1.0 mM Sodium azide. In M3 generation, 15 progenies showed higher seed yield with superior magnitude of yield contributing traits than the best check, RMO-225 (Jain et al., 2013).
Many national and state agricultural universities, including Bhabha Atomic Research Centre (BARC), Mumbai (India), have been doing intensive mutation research since decades by employing X-rays, gamma rays, fast and thermal neutrons in order to generate genetic variability in these arid legume crops (D’Souza et al., 2009). As a result, research on induced mutations in oilseeds and legumes has remained at the leading edge of Indian agricultural research for developing popular varieties with higher productivity in these crops. Khadke and Kothekar (2018) studied the effect of EMS and SA on moth bean trypsin inhibitor content. On electrophoresis, various viable and micro mutants of moth bean were found to have between three and seven iso-inhibitors of trypsin. Some viable mutants had considerable differences in their TI profiles when compared to the control. The TI content of these mutants reduced by 25 to 45 percent, and the seed protein content of micro mutants and viable mutants differed significantly. In 1994, the release of the first early maturing mutant variety, RMO-40, sparked an interest in mutagenesis, leading to the development of a series of short-duration varieties, including RMO-257, RMO-225, RMO-435, RMO-423, and RMO-2251 (Table 6). All of these cultivars were early maturing (60-67 days), exhibited completely transformed plant types, were semi-erect to erect, and had synchronous maturation. Due to short growing seasons, these varieties could successfully fend off drought problems and circumvent devastating diseases like YMD (yellow mosaic disease) and Cercospora leaf spot. Such varieties are more suited to low rainfall (200-250 mm) and shorter growing season. Varieties developed through mutation breeding could be direct mutants with good agro-morphological traits or the resultants of hybridization with mutants for mutant trait introgression in more adaptable and elite genetic backgrounds Pulses with narrow genetic bases have enormous potential for increasing genetic variability through a combination of mutation breeding and conventional breeding approaches. This could lead to the development of elite varieties that are suitable for various agro-climatic zones, thereby improving pulses production subsequently leading to nutritional security.
6 Abiotic and biotic stress tolerance and adaptive traits in moth bean
6.1 Heat stress tolerance
Moth bean is a highly adaptable annual legume that thrives in arid and warm climates, making it one of the most temperature-resistant legumes in arid climates (Sachdeva et al., 2016). It has a deep and fast-penetrating root system, can thrive in open fields for up to 30-40 days, and can withstand temperatures of more than 45°C. By virtue of its adaptive features, whereby it can sustain harsh environmental conditions, moth bean is widely recognized as an arid legume. To mitigate heat stress, moth bean has developed morphological accommodations such prostrate development thereby shading the soil to reduce temperature, large leaves with dense canopy for transpirational cooling, higher biomass but poor partitioning, indeterminate growth, and a fast-penetrating tap root system (Sharma et al., 2014; Harsh et al., 2016). The crop appears to have a genetic buffer that allows it to quickly adapt to rapidly changing moisture conditions as well as deprived and hot environments. Temperature stress, contrarily, has been shown to speed up plant growth in moth bean begetting reduction in both vegetative and grain yields (Khadke et al., 2011; Sharma et al., 2014). Similar results were observed in a study based on the induction of thermo-tolerance for deciphering the efficaciousness of heat acclimation in nine different varieties of moth bean, which showed that all nine varieties could tolerate a sudden temperature rise of 42°C, and that the development of thermo-tolerance was linked to the induction of peroxidase (POX), ascorbic peroxidase (APOX), and catalase (CAT) enzymes (Sharma et al., 2014; Harsh et al., 2016). Likewise, the accumulation of total sugar and proline, as well as an increase in the activity of CAT, GPOX (glutathione peroxidase), and SOD (superoxide dismutase), was seen in thirty-seven genotypes of moth bean under heat stress in earlier investigations. (Harsh et al., 2016; Tiwari et al., 2018) and by that mutagenesis was suggested as an effective means of improving thermo-tolerance by altering the osmo-protectants and anti-oxidative enzymes that go along with it.
6.2 Drought tolerance
As a drought-tolerant crop, moth bean has had a long history of success in rain-fed arid environments. Pre-meditated and need-based efforts have been made for decades to increase the productivity and adaptability of this drought-resistant crop in harsher and more hostile conditions. There has been paucity in systematic research on the physiological basis of yield in rain-fed conditions and the mechanism for drought resistance in this crop, despite its economic importance. Physiological investigations of yielding ability in moth bean types under rain-fed conditions established that the crop is a poor seed producer despite producing enough dry matter. Leaf water potential, osmotic potential, and pressure potential were measured at regular intervals over a period of time. There were noticeable changes in transpiration amongst the different varieties (Srivastava and Soni, 1995). It is quite difficult to predict the outcome of crop production in arid and semi-arid areas. However, the choice of proper plant type and adopting an appropriate cropping system may provide yield stability in the region. The importance of the adoption of suitable genotypes and cropping systems has been emphasized by several investigators (Singh and Reddy, 1988; Lawn, 1989). Higher photosynthetic rates and more effective water use have been linked to the increased performance of mixed crops under low-rainfall conditions. Kathju et al. (2003) suggested that under both low and high rainfall situations, planting early and late genotypes in alternate rows at a 1:1 ratio is an ideal strategy for maintaining the production stability of moth bean. Priyanka et al. (2011) investigated the effect that a low water potential, caused by PEG (polyethylene glycol), has on growth, sugar content, and enzymes related to stress (such as catalase, GPOX). Additionally, SDS-PAGE (Sodium dodecyl-sulfate polyacrylamide gel electrophoresis) analysis was performed on seedlings of twelve moth bean genotypes and traits such as seed set and abscisic acid concentration in the pods as well as root traits have been proven to increase the tolerance of moth bean to drought (Kumar et al., 2019). Accordingly, Garg et al. (2001) reported that on rise in water stress in three moth bean genotypes (RMO-40, Maru moth, and CZM-32 E), net photosynthetic rate and starch and soluble protein content dropped besides a marked decline in the nitrate reductase activity of the plant. Discordantly, there was a build-up of reducing and soluble sugars as well as proline content at pre-flowering phase of the genotypes under study. A germplasm collection comprising of 32 genotypes was evaluated under both dry (terminal drought) and wet (rain-fed) environments. The results exhibited significant differences for genotypes, treatment (stress), and the GxS effects. Also, the differences in the amount of chlorophyll were significant for genotype and combination of genotype and stress treatment. The treatment effect showed a significant difference and led to the identification of two varieties (Maru moth and Jadia) and five accessions (IC 129177, IC 103016, IC 415139, IC 415155, and IC 36157) that were drought tolerant (Malambane and Bhatt, 2014). In another study, Sachdeva et al. (2016) reported that three cultivars (IC103016, IC36011 and IC36157) were more drought tolerant because they sustained greater RWC (Relative Water Content) and MSI (Membrane Stability Index) along with plant height, leaf area, seed weight and plant dry mass besides higher levels of proline accumulation. Recognized as having a primitive plant type, moth bean has evolved to survive but not for increased productivity. In this context, early partitioning, early maturation, and semi-erect to erect growth habit types may be preferred to the conventional sort. Consequently, such advantageous plant traits will not only lead to an increase in yield but also encourage the cultivation of this crop in new and unexplored areas.
6.3 Salinity tolerance
Regulation of proline biosynthesis as well as its degradation, uptake and transport are one of the key mechanisms of the plant defense system, which plays a compelling role in the survival of osmotically stressed plants (Stewart and Lee, 1981; Verma et al., 1992). The gene encoding the enzyme, ornithine delta-aminotransferase (delta-OAT) that is involved in proline biosynthesis pathway, was isolated from moth bean cDNA library by complementation of Escherichia coli proBA mutant auxotroph (Delauney et al., 1993). Zhu et al. (1998) transformed rice var. Nipponbare with P5SC gene obtained from moth bean and increased biomass in salt and water stress conditions. Similarly, when the rice cultivar, ADT 43, was transformed with Δ1-pyrroline-5-carboxylate synthetase (P5CS) gene obtained from moth bean and it was observed that under 200 mM NaCl, the transformed plants showed better plant growth and biomass production while the control plants died within 10 days (Karthikeyan et al., 2011). In another study, the overexpression of P5CS gene derived from moth bean in rice cultivar IR-50, showed tolerance to high salt (200 mM NaCl) conditions (Anoop and Gupta, 2003). Surekha et al. (2014) used P5CSF129A, a mutagenized P5CS gene obtained from moth bean, in combination with CaMV35S, a constitutive promoter, for the genetic transformation of Cajanus cajan to enhance proline accumulation and salt tolerance. Moreover, the P5CS gene from moth bean was transplanted into the hybrid larch (Larix x leptoeuropaea (Dengler)) to improve the productivity of Larch, a tree species that thrives under stresses like cold, salt, and frost, by which the transgenic tissues exhibited a thirtyfold increase in proline concentration (Gleeson et al., 2005).
6.4 YMV disease resistance
Biotic stresses such as Yellow Mosaic Disease (YMD) and the pod borer extend major production challenges for various Vigna crops. Like other Vigna crops, moth bean has not received the proper scientific attention towards management of biotic stresses, and among many, the crop requires particular consideration of YMV and Bacterial Leaf Spot disease. The Mung bean yellow mosaic virus (MYMV) is resistant to mung bean’s morphological and biochemical diversity, according to recent investigations. In terms of resistance, morphological characteristics such as leaf thickness and trichome density are varied. Leaf thickness was found to be higher in the MYMV-resistant genotypes compared to the highly sensitive ones. Similarly, high levels of trichome density were found in resistant genotypes, compared to low levels seen in the most susceptible ones (Patel et al., 2013; Mantesh et al., 2020). In another study, 204 moth bean lines were screened for resistance to the Mung bean yellow mosaic virus (MYMV). Thirteen of the 204 accessions studied showed resistance or tolerance to MYMV in the field (Meghwal et al., 2015). Stress tolerance genes must be identified which requires substantial information on the control of gene expression as well as the biochemical activity of specific proteins involved in the process of tolerance. According to Tiwari et al. (2018), the genes for stress-enzymes such as Catalase, Cyt P450 monooxygenase, heat shock proteins (HSP 90 and HSP 70), oxidoreductase, protein kinases, dehydration-responsive protein (DRP), universal stress proteins, and ferridoxin NADH oxidase were over-expressed in stressed samples. Additionally, expression profiling revealed ten transcripts to be up-regulated and 41 to be down-regulated, whereas 490 exhibited no significant change under moisture stress (Tiwari et al., 2018).
6.5 Insect resistance
Specific management measures to reduce yield losses due to insect pests, such as Jassids, white flies, pulse beetles, white grubs, and other storage pests is imperative Callosobruchus chinensis L. and Callosobruchus maculatus L. are major bruchid pests of most Vigna species, particularly cultivated ones. In light of this, bruchid resistance enhancement is prioritized in all Vigna crop breeding programmes. Plant breeders have long aimed to improve the bruchid resistance of cowpeas, mung beans, adzuki beans, and black gram. These Vigna crops have sources of bruchid resistance, but the resistant germplasm is limited. A wild moth bean accession, TN67, was recently discovered to be extremely resistant to C. chinensis (Somta et al., 2018). For Vigna crop breeding, wild moth bean’s resistance gene(s), including moth bean with C. chinensis resistance could be highly favorable (Somta et al., 2018).
7 Biotechnological interventions
7.1 Genetic linkage map and QTL mapping for agronomic traits
The work done in moth bean, with respect to breeding, is exiguous, therefore, mapping populations, core sets or trait specific reference sets are lacking in the crop. In addition, studies analyzing the genetic variability in V. aconitifolia germplasm, by way of molecular techniques like DNA markers, have been sparse (Sharma et al., 2021). Nevertheless, recently, there has been some progress. Despite having the potentiality to be a new food crop of the future owing to being a source of genes for resistance against biotic and abiotic stresses, the genetics of the domestication process in moth bean is not known. Its domestication will principally involve phenotypic changes, including reduction of seed dormancy and pod shattering, increased organ size and earlier flowering and maturity, which needs extensive knowledge of its genomic resources that are currently scarce. Recently, Yundaeng et al. (2019) constructed a genetic linkage map for moth bean, based on an F2 population of 188 individuals produced from a cross of wild moth bean (TN67) and cultivated moth bean (ICPMO056), and utilized it for the identification of quantitative trait loci (QTL) for domestication-related traits that can be used for genetic improvement of the moth bean and related Vigna species. The genetic linkage map comprised of 11 linkage groups (LGs) of 172 simple sequence repeat (SSR) markers that spanned a total length of 1016.8 centimorgan (cM), with an average marker distance of 7.34 cM. Additionally, a high genome synteny was observed between moth bean and other orphan legumes; mung bean (Vigna radiata), adzuki bean (Vigna angularis), rice bean (Vigna umbellata), and yard long bean (Vigna unguiculata) based on comparative genome analysis. A total of 50 QTLs and 3 genes associated with 20 domestication-related traits were identified. Most of the QTLs belonged to five LGs (1, 2, 4, 7, and 10). Pod shattering, trailing plant canopy, seed dormancy, poor yield potential, less responsive to input resources, late flowering and maturity are the key attributes of moth bean which indicates that the crop is still under active domestication. In another study, Somta et al. (2018) developed QTL mapping in F2 population (188 plants) of moth bean by crossing resistant accession, ‘TN67’ (male parent) and susceptible accession, ‘IPCMO056’ (female parent) for seed resistance to adzuki bean weevil (Callosobruchus chinensis L.). Segregation analysis suggested that C. chinensis resistance in TN76 is controlled by a single dominant gene, designated as Rcc. QTL analysis revealed one principal and one modifying QTL for the resistance, named qVacBrc2.1 and qVacBrc5.1 respectively. qVacBrc2.1 was located on linkage group 2 between simple sequence repeat markers CEDG261 and DMB-SSR160 and accounted for 50.41 to 64.23% of resistance-related traits, depending on the trait and population, while qVacBrc5.1 was detected in only one population. Somta et al. (2018), suggested that markers CEDG261 and DMB-SSR160 should be useful for marker-assisted selection for C. chinensis resistance in moth bean. A list of genetic linkage maps in Vigna aconitifolia is depicted in Table 7. Oliveira et al. (2020) presented the first physical map of moth bean and compared chromosomes with other Vigna and Phaseolus species. As with Yundaeng et al. (2019), a high magnitude of genome synteny was observed between moth bean and other related pulse crops such as mung bean (Vigna radiata), adzuki bean (V. angularis), rice bean (V. umbellata), yard long bean (V. unguiculata) as well as common bean (Phaseolus vulgaris) (Oliveira et al., 2020). As a result, molecular markers and genomic resources developed in these crops will be highly useful in moth bean crop improvement programmes.
7.2 Transcriptome analysis
An extensive study was done to identify genes associated with moisture stress tolerance utilizing differential transcriptome assembly under stressed and non-stressed environments (Tiwari et al., 2018). They have identified 51 differentially expressing transcripts along with 1287 useful SSRs (Simple sequence repeats). Another study was done to identify genes associated with heat stress utilizing forward suppression subtractive hybridization (SSH) cDNA library of heat tolerant cultivar RMO-40 (Gurjar et al., 2014). A total 125 unigenes, out of which 21 were novel to moth bean, were identified. Functional annotation of ESTs (expressed sequence tags) led to the identification of enzymes and heat-shock proteins involved in plant defense against heat stress. In a first ever attempt to identify heat stress responsive genes in moth bean, an elevated temperature of 42°C for 5 min was given to a heat tolerant moth bean cultivar RMO-40 (Rampuria et al., 2012). Utilizing SSH cDNA library, 488 unigenes were identified that were constructed from 738 ESTs. Annotation and semi-quantitative PCR analysis indicated 20 signaling genes and 16 transcription factors associated with heat stress in moth bean. Recently, a number of transcriptome studies in other Vigna species have been added to the public data bases. Transcriptome derived resources, particularly EST-SSR markers, from other Vigna species can be utilized in genetic mapping, marker trait association and marker assisted selection for improvement programmes in moth bean.
7.3 Genetic transformation
Direct transformation was found to be an efficient transformation method in moth bean (Köhler et al., 1987a). Protoplast was used to transform moth bean by using PEG (polyethylene glycol) treatment and electroporation method. The plant genotype also has the role in the transformation success rate (Köhler et al., 1987b). In another transformation method, 60% plating efficiency was observed using co-cultivation of protoplast cells with Agrobacterium tumefaciens containing the Ti plasmid derivative pGV38501103 (Eapen et al., 1987). Particle bombardment method was also successfully used for direct gene transfer in moth bean using mature embryos (Bhargava and Smigocki, 1994) and hypocotyl explants (Kamble et al., 2003). A stable transformation was reported by co-cultivation of primary leaves and cotyledonary nodes, as well as vacuum infiltration into cotyledonary nodes of moth bean (cultivars Jadia and Jawala), with Agrobacterium tumefaciens strain EHA105 accommodating the binary vector p35SGUSINT (Kumar et al., 2008; Kumar et al., 2010). Unlike the earlier studies, genotypic effect was not observed in transformation frequency in this study. However, other studies show that the methodology and genotypes influence the transformation frequency (Kamble et al., 2003).
8 Future perspectives
The impact of drastic changes in global environment on crop productivity has been recognized by the agricultural sector, resulting in increased awareness about Climate Smart Agriculture (CSA) approach in order to increase food security and mitigate the effects of climate change. Orphan crops, though neglected, are well acclimatized to current conditions. Moth bean has evolved and is adapted to hot arid and semi-arid agro-climatic conditions, which makes it a relevant crop amid such challenging situations, with the potential to serve as an alternative to major legume crops. However, owing to its agronomic constraints, cultivation of the crop is still confined to traditional growing areas. Moth bean has not been fully exploited and has also lagged behind other pulses in terms of the quantum of developed genetic and genomic resources. Since there is limited number of genetic resources, considerable efforts are needed to carry out extensive explorations as well as characterization and evaluation of the collected moth bean germplasm for employing the genetic variability for high yield potential, yield stability, improved salinity tolerance, multi-disease and pest resistance as well as enhanced nutrition. With respect to genomic resources, extensive efforts are needed for development of reference gold standard genome since it is fundamental to understand the genome composition and gene repertory of the crop. Further, whole genome resequencing (WGRS), transcripts and genome-wide SSR and SNP markers are required for the identification and annotation of novel genetic polymorphism/candidate genes which are responsible for different agro-morphological, biotic and abiotic stresses as well as biofortification traits, along with being crucial for the construction of genetic linkage maps and physical maps in the future. Therefore, by utilizing recent advances in breeding methods such as haplotype-based breeding, precision-based phenotyping and high throughput genotyping, accompanied with bioinformatic resources, in association with available genetic diversity can help in accelerating domestication in moth bean and expediting its productivity. Identifying pan-genome sequence variation associated with advantageous traits and utilizing them for development of varieties will have a greater impact. Neo-domestication of stress adapted wild species using mutation breeding and TILLING and de novo domestication through new breeding techniques (NBTs) including genome editing tools like CRISPR/Cas could also help in development of novel plants with desired traits (Tomooka et al., 2014; Zsögön et al., 2018). Additionally, utilization of innovative value-added agricultural practices besides adopting effective marketing strategies can be instrumental in the promotion of moth bean as a potential major legume crop (Figure 6). Concentrated, continuous and coordinated efforts are required, from both the scientific community and policy-makers, for implementing these approaches which will not only aid in the development of high yielding and climate-resilient varieties, but also in the mainstreaming of moth bean for re-diversification of global food systems. Finally, when these challenges will be addressed, moth bean, being nutritionally sound and environmentally hardy, is going to be a hopeful climate-smart legume crop for sustainable food and nutritional security across the globe.
Author contributions
Conceptualization: KC and RC. Writing – Original draft preparation: KC, G, BT, and RC. Writing – Review and editing: RC and JR. All authors contributed to the article and approved the submitted version.
Funding
This research was conducted with funding provided under the UN Environment implanted GEF project ‘Mainstreaming agricultural biodiversity conservation and utilization in agricultural sector to ensure ecosystem services and reduce vulnerability in India (Project Code: A 1265)’ being executed jointly by Alliance of Bioversity International and CIAT, and the Indian Council of Agricultural Research (ICAR).
Acknowledgments
The authors duly acknowledge the ICAR-National Bureau of Plant Genetic Resources, New Delhi; the ICAR-Indian Institute of Pulses Research, Kanpur, India; and the Alliance of Biodiversity International and CIAT, India Office, NAS Complex, New Delhi for providing the necessary support.
Conflict of interest
The authors declare that the research was conducted in the absence of any commercial or financial relationships that could be construed as a potential conflict of interest.
Publisher’s note
All claims expressed in this article are solely those of the authors and do not necessarily represent those of their affiliated organizations, or those of the publisher, the editors and the reviewers. Any product that may be evaluated in this article, or claim that may be made by its manufacturer, is not guaranteed or endorsed by the publisher.
References
Adsule, R. N. (1996). Moth bean (Vigna aconitifolia (Jacq.) marechal). Food feed legumes oilseeds (New York, NY: Springer), 203–205. doi: 10.1007/978-1-4613-0433-3_21
Agrawal, A., Rajkumar, S., Rana, J. C., Tyagi, R. K., Yadav, O. P., Singh, K., Paroda, R. S. (Eds.) (2019). Proceedings and recommendations of the satellite symposium on ‘Dryland agrobiodiversity for adaptation to climate change Vol. 87 (Jodhpur, Rajasthan, India: Indian Society of Plant Genetic Resources, New Delhi, Bioversity International, New Delhi, Asia Pacific Association of Agricultural Research Institutes, Bangkok).
Anoop, N., Gupta, A. K. (2003). Transgenic indica rice cv IR-50 over-expressing Vigna aconitifolia Δ1-pyrroline-5-carboxylate synthetase DNA shows tolerance to high salt. J. Plant Biochem. Biotechnol. 12 (2), 109–116. doi: 10.1007/BF03263170
Arora, R. K. (1985). Diversity and collection of wild vigna species in India. Plant Genet. Resour. Newslett. (IBPGR/FAO). (63), 26–33.
Arora, R. K., Nayar, E. R. (1984). Wild relatives of Crop Plants in India. New Delhi: National Bureau of Plant Genetic Resources.
Arya, I. D., Arya, S., Rao, D. V., Shekhawat, N. S. (1990). Variation amongst protoplast-derived moth bean Vigna aconitifolia plants. Euphytica 47 (1), 33–38. doi: 10.1007/BF00040360
Bajaj, Y. P., Gosal, S. S. (1981). “Induction of genetic variability in grain-legumes through tissue culture,” in Tissue culture of economically important plants: proceedings of the international symposium held at the botany department, national university of Singapore (Singapore: COSTED and ANBS (Asian Network for Biological Sciences), 25–41.
Baptista, A., Pinho, O., Pinto, E., Casal, S., Mota, C., Ferreira, I. M. (2017). Characterization of protein and fat composition of seeds from common beans (Phaseolus vulgaris l.), cowpea (Vigna unguiculata l. walp) and bambara groundnuts (Vigna subterranea l. verdc) from Mozambique. J. Food Meas. Charact. 11, 442–450. doi: 10.1007/s11694-016-9412-2
Basavaraja, T., Murthy, N., Kumar, L. V., Mallikarjun, K. (2019). Studies on cross compatibility in interspecific crosses of Vigna radiata × Vigna umbellata species. Legume Res. 42 (5), 699–704.
Bhadkaria, A., Srivastava, N., Bhagyawant, S. S. (2021). A prospective of underutilized legume moth bean (Vigna aconitifolia (Jacq.) marechàl): phytochemical profiling, bioactive compounds and in vitro pharmacological studies. Food Biosci. 42, 101088.
Bhargava, S., Chandra, N. (1983). In vitro differentiation in callus cultures of moth bean, Vigna aconitifolia (JACQ) marechal. Plant Cell Rep. 2 (1), 47–50. doi: 10.1007/BF00269235
Bhargava, S., Chandra, N. (1989). Factors affecting regeneration from leaf explants of moth bean Vigna aconitifolia (Jacq.) marechal. Indian J. Exp. Biol. 27 (1), 55–57.
Bhargava, S. C., Smigocki, A. C. (1994). Transformation of tropical grain legumes using particle bombardment. Curr. Sci. 66 (6), 439–442.
Bisht, I. S., Bhat, K. V., Lakhanpaul, S., Latha, M., Jayan, P. K., Biswas, B. K., et al. (2005). Diversity and genetic resources of wild vigna species in India. Genet. Resour. Crop Evol. 52 (1), 53–68. doi: 10.1007/s10722-005-0286-0
Bisht, I. S., Singh, M. (2013). “Asian Vigna,” in Genetic and genomic resources of grain legume improvement (London, United Kingdom: Elsevier), 237–267.
Biswas, M. R., Dana, S. (1976a). Phaseolus aconitifolius x Phaseolus trilobatus. Indian J. Genet. 36, 125–131.
Biswas, M. R., Dana, S. (1976b). Allopolyploid of tetraploid Phaseolus species× P. calcaratus cross. Biol. Plant 18 (5), 335–338. doi: 10.1007/BF02922458
Bouchenak, M., Lamri-Senhadji, M. (2013). Nutritional quality of legumes, and their role in cardiometabolic risk prevention: a review. J. Med. Food. 16 (3), 185–198. doi: 10.1089/jmf.2011.0238
Brink, M., Jansen, P. C. M. (2006). “(Jacq.) maréchal,” in PROTA (Plant resources of tropical Africa/Ressources végétales de l’Afriquetropicale). Eds. Brink, M., Belay, G.(Wageningen, Netherlands: PROTA Foundation).
Chandra, A., Pental, D. (2003). Regeneration and genetic transformation of grain legumes: an overview. Curr. Sci. 84 (3), 381–387.
Chaudhary, N. B., Patel, A. M., Chaudhari, R. H., Viradiya, Y. A., Patel, H. S. (2021). Genetic analysis for yield and quality related traits in moth bean [Vigna aconitifolia (Jacq.) marechal]. Int. J. Chem. Stud. 9 (1), 2658–2661. doi: 10.22271/chemi.2021.v9.i1ak.11629
Chen, N. C., Baker, L. R., Honma, S. (1983). Interspecific crossability among four species of vigna food legumes. Euphytica 32 (3), 925–937. doi: 10.1007/BF00042175
Choudhary, K., Singh, M., Rathore, M. S., Shekhawat, N. S. (2009). Somatic embryogenesis and in vitro plant regeneration in moth bean [Vigna aconitifolia (Jacq.) marechal]: a recalcitrant grain legume. Plant Biotechnol. Rep. 3 (3), 205–211.
Considine, M. J., Siddique, K. H., Foyer, C. H. (2017). Nature’s pulse power: legumes, food security and climate change. J. Exp. Bot. 68 (8), 1815–1818. doi: 10.1093/jxb/erx099
Cullis, C., Kunert, K. J. (2017). Unlocking the potential of orphan legumes. J. Exp. Bot. 68 (8), 1895–1903.
Dabi, R. K., Gour, H. N. (1988). Field screening of moth bean (Vigna aconitifolia) for susceptibility to insect pests and diseases. Indian J. Agric. Sci. 58 (11), 843–844.
Dana, S. (1976). Origin, evolution and distribution of some grain legumes. Indian J. Genet. 36, 125–131.
Dana, S. (1980). Genomic relationship in the genus vigna and its implications in the breeding programme. In Breeding methods for the improvement of pulse crops (Ludhiana, India: Punjab Agr Univ), 357–367.
Darlington, C. D., Wylie, A. P. (1955). Chromosome atlas of flowering plants. chromosome atlas of flowering plants, 2nd Ed. (London: Allen & Unwin)
Davies, N. T., Nightingale, R. (1975). The effects of phytate on intestinal absorption and secretion of zinc, and whole-body retention of zn, copper, iron and manganese in rats. Br. J. Nutr. 34 (2), 243–258. doi: 10.1017/S0007114575000293
Delauney, A. J., Hu, C. A., Kishor, P. B., Verma, D. P. (1993). Cloning of ornithine delta-aminotransferase cDNA from Vigna aconitifolia by trans-complementation in Escherichia coli and regulation of proline biosynthesis. J. Biol. Chem. 268 (25), 18673–18678. doi: 10.1016/S0021-9258(17)46682-8
Deshmukh, B. A., Pawar, V. S. (2020). Effects of different pretreatments on physicochemical and anti-nutritional quality of moth bean. J. Pharmacogn. phytochem. 9 (1), 1965–1968.
Deshpande, S. S., Cheryan, M. (1984). Effects of phytic acid, divalent cations, and their interactions on α-amylase activity. J. Food Sci. 49 (2), 516–519. doi: 10.1111/j.1365-2621.1984.tb12456.x
Directorate of Economics & Statistics, DAC&FW India. (Accessed Accessed: July 16, 2022). eands.dacnet.nic.in.
D’Souza, S. F., Reddy, K. S., Badigannavar, A. M., Manjaya, J. G., Jambhulkar, S. J. (2009). Mutation breeding in oilseeds and grain legumes in India: accomplishments and socio-economic impact. In Induced plant mutations in the genomics era. Proceedings of an International Joint FAO/IAEA Symposium (Rome, Italy: Food and Agriculture Organization of the United Nations), 55–57.
Eapen, S., Gill, R. (1986). Regeneration of plants from cultured root explants of moth bean (Vigna aconitifolia l. jacq. marechal). Theor. Appl. Genet. 72 (3), 384–387. doi: 10.1007/BF00288577
Eapen, S., Köhler, F., Gerdemann, M., Schieder, O. (1987). Cultivar dependence of transformation rates in moth bean after co-cultivation of protoplasts with Agrobacterium tumefaciens. Theor. Appl. Genet. 75 (1), 207–210. doi: 10.1007/BF00249165
Erdman, J. W. (1979). Oilseed phytates: nutritional implications. J. Am. Oil Chem. Soc 56 (8), 736–741. doi: 10.1007/BF02663052
Eyzaguirre, P. B., Padulosi, S., Hodgkin, T. (1999). IPGRI’s strategy for neglected and underutilized species and the human dimension of agrobiodiversity. Priority-setting underutilized Negl. Plant species Mediterr. region. Report of the IPGRI Conference 9-11 February 1998 – ICARDA, Aleppo, Syria. (Rome, Italy: International Plant Genetic Resources Institute), 1–19.
Food and Agriculture Organization of the United Nations Statistics Division. Available at: www.fao.org/faostat/en (Accessed Accessed: July 16, 2022).
Garg, B. K., Kathju, S., Burman, U. (2001). Influence of water stress on water relations, photosynthetic parameters and nitrogen metabolism of moth bean genotypes. Biol. Plant 44 (2), 289–292. doi: 10.1023/A:1010215812791
Genebank Project, NARO (National Agriculture and Food Research Organization). Available at: www.gene.affrc.go.jp (Accessed Accessed: September 30, 2022).
Genesys. Available at: www.genesys-pgr.org (Accessed Accessed: September 30, 2022).
Gill, R., Eapen, S. (1986). Plant regeneration from hypocotyl protoplasts of moth bean (Vigna aconitifolia). Curr. Sci. 55 (2), 100–102.
Gleeson, D., Lelu-Walter, M. A., Parkinson, M. (2005). Overproduction of proline in transgenic hybrid larch (Larix x leptoeuropaea (Dengler)) cultures renders them tolerant to cold, salt and frost. Mol. Breed. 15 (1), 21–29. doi: 10.1007/s11032-004-1363-3
Godbole, D. A., Kunachgi, M. N., Potdar, U. A., Krishnamurthy, K. V., Mascarenhas, A. F. (1984). Studies on a drought resistant legume: the moth bean, Vigna aconitifolia (Jacq) marechal. II. morphogenetic studies. Plant Cell Rep. 3 (2), 75–78. doi: 10.1007/BF00270976
Gopalan, C., Ramasastri, B. V., Balasubramanian, S. C. (1989). Nutritive Value of Indian Foods. (Hyderabad: National Institute of Nutrition)
Gresshoff, P. M., Mohapatra, S. S. (1982). “Legume cell and tissue culture,” in Tissue culture of economically important plants: proceedings of the international symposium held at the botany department, national university of Singapore, Singapore, 28-30 April 1981/edited by AN rao (Bangalore: COSTED and ANBS (Asian Network for Biological Sciences).
Gruère, G., Giuliani, A., Smale, M. (2006). Marketing underutilized plant species for the benefit of the poor: a conceptual framework (No. 154). International Food Policy Research Institute (IFPRI), 1–36.
Gupta, N., Shrivastava, N., Singh, P. K., Bhagyawant, S. S. (2016). Phytochemical evaluation of moth bean (Vigna aconitifolia l.) seeds and their divergence. Biochem. Res. Int. 2016, 128–133.
Gurjar, K., Rampuria, S., Joshi, U., Palit, P., Bhatt, K. V., Mohapatra, T., et al. (2014). Identification of heat-related ESTs in moth bean through suppression subtraction hybridization. Appl. Biochem. Biotechnol. 173 (8), 2116–2128. doi: 10.1007/s12010-014-1011-2
Hardy, Z. (2016). Functional and nutritional characteristics of bambara groundnut milk powder as an ingredient in yoghurt [Doctoral dissertation] (Cape Town Western Province: Cape Peninsula University of Technology).
Harsh, A., Sharma, Y. K., Joshi, U., Rampuria, S., Singh, G., Kumar, S., et al. (2016). Effect of short-term heat stress on total sugars, proline and some antioxidant enzymes in moth bean (Vigna aconitifolia). Ann. Agric. Sci. 61 (1), pp.57–pp.64. doi: 10.1016/j.aoas.2016.02.001
Henry, A., Daulay, H. S. (1983). Performance of induced mutants in moth bean. Indian J. Genet. Plant Breed. 43 (3), 342–344.
Heuzé, V., Tran, G., Lebas, F. (2020). Moth bean (Vigna aconitifolia). Feedipedia, a programme by INRAE, CIRAD, AFZ and FAO. Available at: https://www.feedipedia.org/node/237 (Accessed: July 16, 2022).
Jain, H. K., Mehra, K. L. (1980). Evolution, adaptation, relationships, and uses of the species of vigna cultivated in India. (Richmond: Royal Botanical Gardens, Kew) 459–468.
Jain, U. K., Ramkrishana, K., Jain, S. K. (2013). Comparative mutagenic efficiency, effectiveness and induced polygenic variability in mothbean (Vigna aconitifolia l.). Indian J. Genet. Plant Breed. 73 (1), 57–63. doi: 10.5958/j.0019-5200.73.1.008
Jangid, R. K., Sharma, R., Sudarsan, Y., Eapen, S., Singh, G., Purohit, A. K. (2010). Microwave treatment induced mutations and altered gene expression in Vigna aconitifolia. Biol. Plant 54 (4), 703–706. doi: 10.1007/s10535-010-0124-x
Jegadeesan, S., Reddy, S. K. (2018). Radiation Induced Mutations in Genetic Enhancement and Development of New Varieties in Black Gram [Vigna mungo (L.) Hepper] (No. IAEA-CN--263). Austria: International Atomic Energy Agency (IAEA)
Kaga, A., Tomooka, N., Egawa, Y., Hosaka, K., Kamijima, O. (1996). Species relationships in the subgenus ceratotropis (genus vigna) as revealed by RAPD analysis. Euphytica 88 (1), 17–24. doi: 10.1007/BF00029261
Kamble, S., Misra, H. S., Mahajan, S. K., Eapen, S. (2003). A protocol for efficient biolistic transformation of mothbean (Vigna aconitifolia l. jacq. marechal). Plant Mol. Biol. Rep. 21 (4), 457–458. doi: 10.1007/BF02772595
Karthikeyan, A., Pandian, S. K., Ramesh, M. (2011). Transgenic indica rice cv. ADT 43 expressing a Δ1-pyrroline-5-carboxylate synthetase (P5CS) gene from Vigna aconitifolia demonstrates salt tolerance. Plant Cell Tissue Organ Cult. 107 (3), 383–395.
Kathju, S., Garg, B. K., Vyas, S. P., Lahiri, A. N. (2003). Sustainable production of moth bean through genotype management under arid environments. J. Arid Environ. 53 (1), 137–143. doi: 10.1006/jare.2002.1026
Kaviraj, C. P., Kiran, G., Venugopal, R. B., Kishor, P. K., Rao, S. (2006). Somatic embryogenesis and plant regeneration from cotyledonary explants of green gram [Vigna radiata (L.) Wilezesk.]–a recalcitrant grain legume. In Vitro Cell. Dev. Biol. Plant 42, 134–138. doi: 10.1079/IVP2005739
Khadke, S. G., Kothekar, V. S. (2011). Genetic improvement of moth bean (Vigna acconitifolia (Jacq.) marechal) through mutation breeding. In: Khan, S., Kozgar, M. I. (Eds.), Breeding of Pulse Crops (Ludhiana, India: Kalyani Publishers), 34–54.
Khadke, S., Kothekar, V. (2018). Effect of EMS and SA on Trypsin Inhibitor Content in Moth Bean (Vigna aconitifolia (Jacq.) Marechal), (No. IAEA-CN–263). Austria: International Atomic Energy Agency (IAEA)
Khokhar, S., Chauhan, B. M. (1986). Antinutritional factors in moth bean (Vigna aconitifolia): varietal differences and effects of methods of domestic processing and cooking. J. Food Sci. 51 (3), 591–594. doi: 10.1111/j.1365-2621.1986.tb13887.x
Kigel, J. (1999). Culinary and nutritional quality of phaseolus vulgaris seeds as affected by environmental Biotechnol. Agron. Soc. Environ 3(4):205–209 .
Klozová, E. (1965). Interrelations among several Asiatic species of the genus Phaseolus studied by immunochemical methods. In Proceedings of the Symposium on the Mutational Process, 9-11 August 1965 - Prague, Czech Republic. (Prague, Czech Republic: Academia), 485–487.
Kochhar, S. L. (2016). “Mat or moth bean [Phaseolus aconitifolius],” in Economic botany: a comprehensive study, 5th edition, vol. 430 . Ed. Kochhar (Cambridge Univ. Press), 167–195.
Kohakade, S. N., Bhavsar, V. V., Pawar, V. Y. (2017). Research article genetic divergence in moth bean (Vigna aconitifolia (Jacq) marechal). Electron. J. Plant Breed. 8 (4), 1148–1158. doi: 10.5958/0975-928X.2017.00167.3
Köhler, F., Golz, C., Eapen, S., Kohn, H., Schieder, O. (1987a). Stable transformation of moth bean vigna aconitifolia via direct gene transfer. Plant Cell Rep. 6 (4), 313–317. doi: 10.1007/BF00272007
Köhler, F., Golz, C., Eapen, S., Schieder, O. (1987b). Influence of plant cultivar and plasmid-DNA on transformation rates in tobacco and moth bean. Plant Sci. 53 (1), 87–91. doi: 10.1016/0168-9452(87)90182-8
Krishnamurthy, K. V., Godbole, D. A., Mascarenhas, A. F. (1984). Studies on a drought resistant legume: the moth bean, Vigna aconitifolia (Jacq) marechal. i. protoplast culture and organogenesis. Plant Cell Rep. 3 (1), 30–32.
Kumar, D. (2002). Production technology for moth bean in India (Jodhpur, India: Indian Turisum Publication).
Kumar, D. (2005). Status and direction of arid legumes research in India. Indian J. Agric. Sci. 75, 375–391.
Kumar, J., Choudhary, A. K., Gupta, D. S., Kumar, S. (2019). Towards exploitation of adaptive traits for climate-resilient smart pulses. Int. J. Mol. Sci. 20 (12), 2971. doi: 10.3390/ijms20122971
Kumar, A. S., Gamborg, O. L., Nabors, M. W. (1988). Plant regeneration from cell suspension cultures of Vigna aconitifolia. Plant Cell Rep. 7 (2), 138–141. doi: 10.1007/BF00270124
Kumar, S., Saxena, S. N., Jat, R. S., Sharma, R. (2008). Agrobacterium tumefaciens mediated genetic transformation of moth bean (Vigna aconitifolia (Jacq) marechal). Indian J. Genet. Plant Breed. 68 (3), 327.
Kumar, S., Singh, G., Jat, R. S., Saxena, S. N., Sharma, R. (2010). Transformation of moth bean (Vigna aconotifolia) cotyledonary nodes through vacuum infiltration of. Agrobacterium tumefaciens. Green Farm. 1(6), 551–554.
Lawn, R. J. (1989). Agronomic and physiological constraints to the productivity of tropical grain legumes and prospects for improvement. Exp. Agric. 25(4), 509–528. doi: 10.1017/S0014479700015143
Lemke, R. L., Zhong, Z., Campbell, C. A., Zentner, R. (2007). Can pulse crops play a role in mitigating greenhouse gases from north American agriculture? Agron. J. 99 (6), 1719–1725.
Liener, I. E. (1976). Legume toxins in relation to protein digestibility-a review. J. Food Sci. 41 (5), 1076–1081. doi: 10.1111/j.1365-2621.1976.tb14391.x
Longvah, T., An̲antan̲, I., Bhaskarachary, K., Venkaiah, K., Longvah, T. (2017). Indian Food composition tables (Hyderabad: National Institute of Nutrition, Indian Council of Medical Research), 2–58.
Ma, D. Z., Sun, J., Zhang, G. Q., Wang, H. X., Ng, T. B. (2010). A storage protein-like trypsin inhibitor from the moth bean (Phaseolus acutifolius) with antiproliferative activity toward lymphoma cells. Protein Pept. Lett. 17 (6), 782–788. doi: 10.2174/092986610791190408
Malambane, G., Bhatt, K. V. (2014). Agro-morphological performance of moth bean (Vigna aconitifolia) lines in severe drought stressed and rain-fed condition. Direct Res. J. Agric. Food Sci. (DRJAFS) 2, 107–112.
Mantesh, M., Venkatesh, Pankaja, N. S. (2020). The studies on the morphological variability and biochemical changes induced by mungbean yellow mosaic virus (MYMV) in mungbean [Vigna radiata (L.) wilczek]. Indian Phytopathol. 73 (3), 543–553. doi: 10.1007/s42360-020-00238-7
Maréchal, R. (1978). Taxonomic study of a complex group of species of the genera Phaseolus and Vigna (Papilionaceae) on the basis of morphological and pollen data, processed by computer analysis. Boissiera 28, 1–273.
Medhe, S., Jain, S., Anal, A. K. (2019). Effects of sprouting and cooking processes on physicochemical and functional properties of moth bean (Vigna aconitifolia) seed and flour. J. Food Sci. Technol. 56, 2115–2125. doi: 10.1007/s13197-019-03692-y
Meghwal, R., Joshi, U., Kumar, S., Sharma, R. (2015). Screening of moth bean (Vigna aconitifolia) core collection against yellow mosaic virus. Indian J. Agric. Sci. 854, 571–575.
Mroginski, L. A., Kartha, K. K. (1984). Tissue culture of legumes for crop improvement: phaseolus. Plant Breed. Rev. 2, 215–264. doi: 10.1002/9781118060995.ch7
Narayan, S., Saxena, S. N., Jakhar, M. L., Sharma, R. (2015). Enhancement of regeneration in moth bean [Vigna aconitifolia (Jacq) marechal] through gamma irradiation. Legume Res. 38 (4), 519–523. doi: 10.5958/0976-0571.2015.00086.7
N’Dri Yao, D., Kouassi, K. N., Erba, D., Scazzina, F., Pellegrini, N., Casiraghi, M. C. (2015). Nutritive evaluation of the bambara groundnut Ci12 landrace [Vigna subterranea (L.) verdc. (Fabaceae)] produced in côte d’Ivoire. Int. J. Mol. Sci. 16 (9), 21428–21441.
Oliveira, A. R. D. S., Martins, L. D. V., Bustamante, F. D. O., Muñoz-Amatriaín, M., Close, T., da Costa, A. F., et al. (2020). Breaks of macrosynteny and collinearity among moth bean (Vigna aconitifolia), cowpea (V. unguiculata), and common bean (Phaseolus vulgaris). Chromosome Res. 28 (3), 293–306.
Oyeleke, G. O., Afolabi, O., Isola, A. D. (2012). Some quality characteristics and carbohydrate fractions of bambara groundnut (Vigna subterranea l.) seed flour. J. Appl. Chem. 2 (4), 16–19.
Padulosi, S., Hoeschle-Zeledon, I. (2004). Underutilized plant species: what are they? Leisa-Leusden 20, 5–6.
Pal, S., Sharma, N. K., Sharma, A. K., Kumar, A., Meena, S. (2020). Study of genetic variability parameters for seed yield and component traits in mothbean [vigna aconitifolia (jacq) marechal]. Int. J. Chem. Stud. 8, 2638–2641. doi: 10.22271/chemi.2020.v8.i4ae.10038
Pandit, R., Bhusal, B., Regmi, R., Neupane, P., Bhattarai, K., Maharjan, B., et al. (2021). Mutation breeding for crop improvement: a review. Rev. Food Agr. 2 (1), 31–35. doi: 10.26480/rfna.01.2021.31.35
Pandiyan, M., Senthil, N., Suersh, R., Chakravarthy, N., Packiaraj, D., Jagadeesh, S. (2012). Interspecific hybridization of Vigna radiata× Vigna trilobata. Euphytica 35, 1017–1022.
Panicker, S., Hamdule, A. (2021). Analysis of medicinal properties of Vigna aconitifolia (Matki). Asian J. Pharm. Clin. Res. 14(5), 78–81. doi: 10.22159/ajpcr.2021.v14i5.41186
Patel, H., Kalaria, R., Mahatma, M., Chauhan, D. A., Mahatma, L. (2013). Physiological and biochemical changes induced by mungbean yellow mosaic virus (MYMV) in mungbean [Vigna radiata (L.) wilczek]. Cell Tissue Res. 13 (3), 3927.
Piper, C. V., Morse, W. J. (1914). Five Oriental Species of Beans. U.S. Dept. Agric. Bull. 119, 1–32.
Priyanka, S., Rizwan, M., Bhatt, K. V., Mohapatra, T., Govind, S. (2011). In-vitro response of Vigna aconitifolia to drought stress induced by peg 6000. J. Stress Physiol. Biochem. 7 (3), 108–121.
Pugalenthi, M., Vadivel, V., Siddhuraju, P. (2005). Alternative food/feed perspectives of an underutilized legume Mucuna pruriens var.utilis–a review. Plant foods Hum. Nutr. 60 (4), 201–218. doi: 10.1007/s11130-005-8620-4
Rachie, K. O., Roberts, L. M. (1974). Grain legumes of the lowland tropics. Adv. Agron. 26, 1–132. doi: 10.1016/S0065-2113(08)60869-X
Raina, A., Laskar, R. A., Khursheed, S., Amin, R., Tantray, Y. R., Parveen, K., et al. (2016). Role of mutation breeding in crop improvement-past, present and future. Asian Res. J. Agric. 2 (2), 1–13. doi: 10.9734/ARJA/2016/29334
Ramkrishna, K., Jain, U., Kumawat, S. C., Balai, O. P. (2008). Mutation breeding in arid legumes in India (No.IAEA-CN–167). Austria: International Atomic Energy Agency (IAEA).
Rampuria, S., Joshi, U., Palit, P., Deokar, A. A., Meghwal, R. R., Mohapatra, T., et al. (2012). Construction and analysis of an SSH cDNA library of early heat-induced genes of Vigna aconitifolia variety RMO-40. Genome 55 (10), 783–796.
Rao, A. V., Venkateswarlu, B., Henry, A. (1984). Genetic variation in nodulation and nitrogenase activity in guar and moth [bean]. Indian J. Genet. Plant Breed. 44, 425–428.
Sachdeva, S., Sharma, V., Bhat, K. V., Sen, S. (2016). Morphological and physiological response studies of moth bean (Vigna aconitifolia l.) genotypes under drought stress. Int. J. IT Eng. Appl. Sci. Res. 5 (7), 2319–4413.
Sahoo, S., Sanadya, S. K., Kumari, N., Baranda, B. (2019). Estimation of the various genetic variability parameters for seed yield and its component traits in mothbean germplasm [Vigna aconitifolia (Jacq.) marechal]. J. Pharmacognosy Phytochem. 8 (3S), 49–52.
Sampson, H. C. (1936). Cultivated crop plants of the British empire and the Anglo-Egyptian Sudan tropical and sub-tropical. Kew Bulletin. London: H.M. Stationery Office.
Saravanan, M., Ignacimuthu, S. (2015). Hypocholesterolemic effect of Indian medicinal plants-a review. Med. Chem. 5 (1), 040–049. doi: 10.4172/2161-0444.1000241
Sathe, S. K., Venkatachalam, M. (2007). Fractionation and biochemical characterization of moth bean (Vigna aconitifolia l.) proteins. LWT-Food Sci. Technol. 40 (4), 600–610. doi: 10.1016/j.lwt.2006.03.021
Saxena, S. N. (2004). Physio-molecular analysis of somaclonal variation in cultures of moth bean (Vigna aconitifolia) derived from different levels of cytokinins [Doctoral dissertation] (Jobner (RAJ)]: Sri Karan Narendra Agriculture University).
Saxena, S. N., Kaushik, N., Sharma, R. (2008). Effect of abscisic acid and proline on in vitro flowering in Vigna aconitifolia. Biol. Plant 52 (1), 181–183. doi: 10.1007/s10535-008-0040-5
Saxena, S. N., Sharma, R., Mahla, H. R. (2006). Analysis of induced and naturally available genetic diversity in moth bean (Vigna aconitifolia (Jacq) merachal) using RAPD markers. J. Arid Environ. 3 (1), 60–64.
Schrire, B. D., Lavin, M. A. T. T., Lewis, G. P. (2005). “Global distribution patterns of the leguminosae: insights from recent phylogenies,” in Plant diversity and complexity patterns: local, regional and global dimensions. proceedings of an international symposium held at the royal Danish academy of sciences and letters in Copenhagen, Denmark, 25-28 may 2003 (Copenhagen, Denmark: The Royal Danish Academy of Sciences and Letters), 375–422.
Senthilkumar, T., Ngadi, M. (2020). “Moth bean,” in Pulses: Processing and Product Development (Switzerland: Springer Nature), pp.205–pp.212.
Sharma, R., Jain, M., Kumar, S., Kumar, V. (2014). Evaluation of differences among Vigna aconitifolia varieties for acquired thermotolerance. Agric. Res. 3 (2), 104–112. doi: 10.1007/s40003-014-0108-8
Sharma, R. C., Kakani, R. K. (2002). “Moth bean genetic improvement,” in Nat. Symp.on Arid Legume for Sustainable Agriculture and Trade (Jodhpur, India: Scientific Publishers), 9–30.
Sharma, R., Kumar, S., Mahla, H. R., Khandelwal, V., Roy, P. K., Sundria, M. M. (2021). “Moth bean,” in The beans and the peas (Cambridge, United Kingdom: Woodhead Publishing), 67–88.
Sharma, N. K., Kumawat, N., Panwar, P. K. (2015). Evaluation of high yielding varieties and demonstration of production technologies in moth bean at farmers’ fields in Western rajasthan. Ann. Arid Zone. 54(1&2), 51–53.
Sharma, R., Sharma, P., Kumar, S., Saxena, S. N., Khandelwal, V., Rizwan, M. (2018). Heat treatment affects regeneration, protein expression and genetic make-up of Vigna aconitifolia (Jacq.) marechal. Ann. Agrar. Sci. 16 (2), 116–120. doi: 10.1016/j.aasci.2017.12.001
Sihag, S. K., Khatri, R. S., Joshi, U. N. (2004). Genetic variability and heritability for grain yield and its components in mothbean [Vigna aconitifolia (Jacq.) marechal]. Ann. Biol. Res. 20, 219–222.
Singh, D. P. (1990). Distant hybridization in genus vigna-a review. Indian J. Genet. Plant Breed. 50 (3), 268–276.
Singh, M., Bisht, I. S., Sardana, S., Gautam, N. K., Husain, Z., Gupta, S., et al. (2006). Asiatic Vigna. Plant Genetic Resources: Food Grain Crops. (New Delhi, India: Narosa Publishing House), 275–301.
Singh, R. J., Chung, G. H., Nelson, R. L. (2007). Landmark research in legumes. Genome 50 (6), 525–537. doi: 10.1139/G07-037
Singh, M., Krikorian, A. D. (1982). Inhibition of trypsin activity in vitro by phytate. J. Agric. Food Chem. 30 (4), 799–800. doi: 10.1021/jf00112a049
Singh, M., Kumar, G., Abraham, Z., Phogat, B. S., Sharma, B. D., Patel, D. P. (2001). “Germplasm evaluation,” in National bureau of plant genetic resources: a compendium of achievements. Eds. Dhillon, B. S., Varaprasad, K. S., Singh, M., Srivastava, S. A. U., Sharma, G. D. (New Delhi: NBPGR), 116–165.
Singh, R. P., Mann, H. S. (1976). Improved dryland agriculture for Western rajasthan (Hisar, India: Haryana Agricultural University Press).
Singh, R. P., Reddy, G. S. (1988). Identifying crops and cropping systems with greater production stability in water-deficit environments. Drought Res. priorities dryland tropics. 77, 85.
Smartt, J. (1985). Evolution of grain legumes. III. pulses in the genus Vigna. Exp. Agric. 21 (2), 87–100.
Solanki, R. K., Mahla, H. R., Kakani, R. K., Choudhary, K. B., Sharma, R. (2018). Stress tolerant crop varieties of major arid zone crops to promote resilience to climatic stresses. Indian Farm. 68(09), 41–45.
Somta, P., Jomsangawong, A., Yundaeng, C., Yuan, X., Chen, J., Tomooka, N., et al. (2018). Genetic dissection of azuki bean weevil (Calloso bruchuschinensis l.) resistance in moth bean (Vigna aconitifolia [Jaqc.] maréchal). Genes 9 (11), 555.
Srivastava, J. P., Soni, C. P. (1995). Physiological basis of yield in moth bean [Vigna aconitifolia (Jacq.) marechal] under rainfed conditions. J. Agron. Crop Sci. 174 (2), 73–77.
Stagnari, F., Maggio, A., Galieni, A., Pisante, M. (2017). Multiple benefits of legumes for agriculture sustainability: an overview. Chem. Biol. Technol. Agric. 4 (1), 1–13. doi: 10.1186/s40538-016-0085-1
Stewart, C. R., Lee, J. A. (1981). “Proline accumulation: biochemical aspects,” in Physiology and biochemistry of drought resistance in plants, vol. 243. (Sydney, Australia: Academic Press), 243–259.
Surekha, C. H., Kumari, K. N., Aruna, L. V., Suneetha, G., Arundhati, A., Kavi Kishor, P. B. (2014). Expression of the Vigna aconitifolia P5CSF129A gene in transgenic pigeonpea enhances proline accumulation and salt tolerance. Plant Cell Tissue Organ Cult. 116 (1), 27–36. doi: 10.1007/s11240-013-0378-z
Takahashi, Y., Somta, P., Muto, C., Iseki, K., Naito, K., Pandiyan, M., et al. (2016). Novel genetic resources in the genus Vigna unveiled from gene bank accessions. PloS One 11 (1), p.e0147568. doi: 10.1371/journal.pone.0147568
Tiwari, B., Kalim, S., Bangar, P., Kumari, R., Kumar, S., Gaikwad, A., et al. (2018). Physiological, biochemical, and molecular responses of thermotolerance in moth bean (Vigna aconitifolia (Jacq.) marechal). Turk. J. Agric. For. 42 (3), 176–184. doi: 10.3906/tar-1709-1
Tomooka, N., Kaga, A., Isemura, T., Vaughan, D. (2011). Vigna. In Wild Crop Relatives: Genomic and Breeding Resources: Legume Crops and Forages (Heidelberg, Berlin: Springer), 291–311.
Tomooka, N., Naito, K., Kaga, A., Sakai, H., Isemura, T., Ogiso-Tanaka, E., et al. (2014). Evolution, domestication and neo-domestication of the genus Vigna. Plant Genet. Resour. 12 (S1), S168–S171. doi: 10.1017/S1479262114000483
United States Department of Agriculture (USDA). Food data central (Accessed Accessed: July 16, 2022). fdc.nal.usda.gov [fdc Published: April 1.
Vavilov, N. L. (1926). Centers of origin of cultivated plants. Inst. Appl. Bot. Plant Breed. 16 (2), 1–248.
Verdcourt, B. (1970). Studies in the leguminosae-papillionoideae for the ‘Flora of tropical East africa’. IV. Kew Bull. 24, 507–570. doi: 10.2307/4102859
Verma, D. P. S., Hu, C. A., Delauney, A. J. (1992). Genetic manipulation for proline overproduction and the control of osmoregulation in plants. In AVRDS Symposium (Tainan, Taiwan: Asian Vegetable Research and Development Center), 47–57.
Verma, N., Sehrawat, K. D., Sehrawat, A. R., Pandey, D. (2021). Effective green synthesis, characterization and antibacterial efficacy of silver nanoparticles from seaweed treated sprouts of moth bean (Vigna aconitifolia jacq.). Regen. Eng. Transl. Med. 8, 152–165.
Vir, O., Singh, A. K. (2015). Moth bean [Vigna aconitifolia (Jacq.) marechal] germplasm: evaluation for genetic variability and inter characters relationship in hot arid climate of western rajasthan, India. Legume Res. 38 (6), 748–752.
Viswanatha, K. P., Kumar, D., Ramavtar, S., Kumar, D. (2016). Improvement of minor pulses and their role in alleviating malnutrition. Indian J. Genet. Plant Breed. 76 (4), 593–607. doi: 10.5958/0975-6906.2016.00075.4
West, N. B., Garber, E. D. (1967). Genetic studies of variant enzymes. i. an electrophoretic survey of esterases and leucine aminopeptidases in the genus phaseolus. Can. J. Genet. Cytol. 9 (3), 640–645.
Whyte, R. O., Nilsson-Leissner, G., Trumble, H. C. (1953). Legumes agriculture. Soil Sci. 76 (5), 403. doi: 10.1097/00010694-195311000-00017
Xu, B. J., Chang, S. K. C. (2008). Total phenolic content and antioxidant properties of eclipse black beans (Phaseolus vulgaris l.) as affected by processing methods. J. Food Sci. 73 (2), H19–H27.
Yaqoob, M., Khan, N. U., Mansoor, M., Gul, S., Khalil, I. A., Afridi, K. (2015). Moth bean germplasm screening against yellow mosaic virus, II. developmentof moth bean high-yielding seed and fodder cultivars. Turk. J. Agric. For. 39(2), 212–226.
Yundaeng, C., Somta, P., Amkul, K., Kon gjaimun, A., Kaga, A., Tomooka, N. (2019). Construction of genetic linkage map and genome dissection of domestication-related traits of moth bean (Vigna aconitifolia), a legume crop of arid areas. Mol. Genet. Genom. 294 (3), 621–635. doi: 10.1007/s00438-019-01536-0
Zahoor, A. (2007). Country report on plant genetic resources for food and agriculture. Pakistan Agric. Res. Council. 86, 27–34.
Zhu, B., Su, J., Chang, M., Verma, D. P. S., Fan, Y. L., Wu, R. (1998). Overexpression of a Δ1-pyrroline-5-carboxylate synthetase gene and analysis of tolerance to water-and salt-stress in transgenic rice. Plant Sci. 139 (1), 41–48. doi: 10.1016/S0168-9452(98)00175-7
Keywords: moth bean, genetic diversity, crop improvement, stress tolerance, climate change, sustainable agriculture
Citation: C. KR, G, T. B, Chandora R and Rana JC (2023) Moth bean (Vigna aconitifolia): a minor legume with major potential to address global agricultural challenges. Front. Plant Sci. 14:1179547. doi: 10.3389/fpls.2023.1179547
Received: 04 March 2023; Accepted: 02 May 2023;
Published: 06 June 2023.
Edited by:
Omena Bernard Ojuederie, Kings University, NigeriaReviewed by:
Oluwaseyi Samuel Olanrewaju, North-West University, South AfricaChandra Mohan Singh, Banda University of Agriculture and Technology, India
Copyright © 2023 C., Gayacharan, T., Chandora and Rana. This is an open-access article distributed under the terms of the Creative Commons Attribution License (CC BY). The use, distribution or reproduction in other forums is permitted, provided the original author(s) and the copyright owner(s) are credited and that the original publication in this journal is cited, in accordance with accepted academic practice. No use, distribution or reproduction is permitted which does not comply with these terms.
*Correspondence: Rahul Chandora, rahul1@icar.gov.in; Jai Chand Rana, j.rana@cgiar.org