- 1College of Forestry, Northwest A & F University, Yangling, Shaanxi, China
- 2Shaanxi Key Laboratory of Economic Plant Resources Development and Utilization, College of Forestry, Northwest Agriculture and Forestry University, Yangling, Shaanxi, China
Juniperus sabina L. is used in the traditional Chinese medicine (TCM) system to prevent or treat various diseases. However, only the leaves and branches are used as medicinal parts. The aim of this study was to compare the chemical characteristics of different tissues (leaves, branches, stems, and roots) of J. sabina at different ages by HPLC-MS and to evaluate the biological activity (enzyme inhibition, anti-drug-resistant bacteria). Total phenol (TPC) and total lignan (TLC) contents in J. sabina were determined by Folin-Ciocalteu method and UV spectrophotometry, respectively. High levels of total phenols (87.16 mg GAE/g dry weight) and total lignans (491.24 mg PPT/g dry weight) were detected in fifteen annual J. sabina roots and current year leaves, respectively. Eleven compounds, of which six were phenolic compounds and five were lignans, were identified and quantified by HPLC/HPLC-MS. Statistical analysis showed that the distribution and content of the detected compounds showed considerable variation among ages and tissues, and that the current year leaves of fifteen annual J. sabina could be used as a potential application site for the source of podophyllotoxin. Acetylcholinesterase (AChE) inhibitory activity was found to be the highest on the extracts of fifteen annual J. sabina current year leaves (47.37 μg/mL), while the highest inhibition towards butyrylcholinesterase (BChE) was observed for the extracts of seven annual J. sabina previous year leaves (136.3 μg/mL). And the second annual J. sabina current year stem’s extracts showed the best antidiabetic activity (anti-α-glucosidase, 62.59 μg/mL). In addition, the extracts of fifteen annual J. sabina roots (47.37 μg/mL) showed the highest anti-MRSA activity (31.25 μg/mL). Redundancy analysis (RDA) was conducted to clarify the factors affecting the biological activity of J. sabina, and its results showed that epicatechin and matairesinol showed positive promotion. This study provides a new perspective for understanding the chemical differences and comprehensive utilization of different tissues of J. sabina.
1 Introduction
Juniperus L, represented by 70 species in the world and 23 species in China, belongs to the Cupressaceae family (Esteban et al., 2023; Kavaz and Faraj, 2023; Lim et al., 2023). The wood, bark and leaves of the juniper genus are rich sources of natural products that can be used as fragrances in alcoholic beverages and cosmetics, with high commercial application value (Rangel et al., 2018). Previous phytochemical studies of Juniperus species showed a rich chemical content, including diterpenes, triterpenes, phenolic acids, flavonoids and lignans (Tavares and Seca, 2018; Lim et al., 2023). The relevant in vitro and in vivo studies indicated that many Juniperus species possess anti-inflammatory, antioxidant, antiviral, antibacterial, hypotensive, anticancer, antidiabetic, and neuroprotective properties (Al-Snafi, 2018a; Al-Snafi, 2018b; Gonçalves et al., 2022; Rehman et al., 2022; Spengler et al., 2022; Kavaz and Faraj, 2023).
Juniperus sabina L. is an evergreen coniferous shrub in the Juniperus genus, widely cultivated as a horticultural plant and used for decoration in China (Wang et al., 2014). J. sabina is rich in volatile oils, phenols and lignans (Xu et al., 2022). In particular, J. sabina is rich in podophyllotoxin, a synthetic precursor of the first-line anticancer drug etoposide (Ivanova et al., 2023). However, current studies on the genus Juniperus have mainly focused on Juniperus communis L. and Juniperus oxycedrus L. (Majewska et al., 2017; Spengler et al., 2022). Although data on the chemical composition of phenols and lignans from Juniperus species cones and leaves have been published, most studies focus on the isolation and characterization of single compounds (Xu et al., 2022). Furthermore, the content of phenols and lignans in plants and their biological activity depend on age, tissues, and ontogeny stage (Dossou et al., 2021; Butnaru et al., 2022). The phytochemical and molecular characteristics of perennials, especially medicinal plants, vary significantly with age and this must be taken into account (Bielecka et al., 2019). To our knowledge, there is currently no literature data on the composition of compounds or their biological activities or medicinal properties in different tissues of different ages J. sabina. Considering the importance of multiple enzymes, such as cholinesterase and α-glucosidase, in the development of many chronic diseases, it is worthwhile to study the enzyme-inhibitory activity of J. sabina. Moreover, almost all Juniperus species show antibacterial activity, but the studies are only for common strains, and the research on the inhibition of drug-resistant bacteria is still lacking.
Based on this, this study aims to analyze chemical composition of different tissues (leaves, branches, stems, roots) of J. sabina at different ages and evaluated their biological activities (enzyme inhibition, antibacterial activity) to address the key and difficult issues in J. sabina applications. These results can promote the comprehensive utilization of J. sabina plant resources.
2 Material and methods
2.1 Plant materials
In this study, three different tree ages (biennial, seven annual and fifteen annual) of J. sabina were selected as test materials. The test samples were identified by Prof. Kunliang Dang of the College of Forestry, Northwest A&F University, and kept in the herbarium of the College of Forestry, Northwest A&F University. Five plants with good growth and healthy trees were selected as target trees before sampling. Samples were collected in July 2020 from two experimental sites (Northwest A&F University School of Forestry Nursery and Northwest A&F University Expo Park). According to the physiological and morphological characteristics of the collected samples (Cheng et al., 2005), the current year leaves, previous year leaves, branches, current year stem, previous year stem and roots (Figure 1) were separated. The samples were prepared according to the method of “freeze-drying to constant weight, crushing and sieving (Pharmacopoeia standard sieve No. 4)”, and stored at -20°C.
2.2 Extraction procedure
The samples were extracted according to the method of Xu et al. (2022). Samples of different tissues (5 g each) of J. sabina were weighed into triangular flasks, and 200 mL of a 90% methanol-water solution was added. The mixture was treated in an ultrasonic bath (HH-6, Shanghai High Precision Instrument Co., Ltd., Shanghai, China) for 7 min, followed by homogenization-assisted extraction at 55°C for 90 min using a constant temperature magnetic stirrer (XMTD203, Jiangsu Keyi Instrument Co., Ltd., Nanjing, China). The extracts were filtered and evaporated under vacuum at 45°C until dryness. The crude extracts obtained were kept at 4°C prior to the activity and phytochemical experiments. The yields (%, w/w) of the crude extracts were given in SM-Table 1 (Supplementary Material). Extracts of plant material were performed in triplicate.
2.3 Determination of total phenols and lignans
The phytochemical analysis of extracts was performed according to the method described by Gómez-Martínez et al. (2020) with some modifications. The total phenol content (TPC) of the extracts was determined according to the Folin-Ciocalteau method. After pipetting 200 μL of extracts of J. sabina (1 mg/mL) into a test tube, 2 mL of Folin-Ciocalteu reagent was added, followed by a 7% Na2CO3 solution. The mixture was shaken well and left to react for 6 min before reading the absorbance at 760 nm on an ultraviolet spectrophotometer (UV-1800, Shimadzu Manufacturing Co., Kyoto, Japan). A standard curve (SM-Figure 1A) was drawn with gallic acid dilutions, and the results of TPC were expressed as mg gallic acid equivalents (GAE) per g of sample dry weight (mg GAE/g DW). The results were expressed as mg of gallic acid equivalents (mg GAE) per g of dry weight (DW).
The total lignan content (TLC) of the extracts was calculated by UV spectrophotometry. Briefly, serial dilutions of podophyllotoxin (PPT) were used to prepare standard calibration curves. The extracts (1.25 mg/mL) and podophyllotoxin dilutions were measured for absorbance at 290 nm with a UV-Vis spectrophotometer (UV mini-1240, Shimadzu Manufacturing Co., Kyoto, Japan). A standard curve (SM-Figure 1B) was drawn with podophyllotoxin dilutions, and the results of TLC were expressed as mg podophyllotoxin equivalents per g of sample dry weight (mg PPT/g DW).
2.4 Identification of phenols and lignans by HPLC/HPLC-MS analysis
2.4.1 HPLC-MS analysis
The samples (leaves, branches, stems, and roots) of J. sabina were used for metabolomic analysis, with three biological replicates per group. The HPLC separation was carried out using an EXIONLC System (SCIEX, USA), and the column was a Waters Acquity UPLC HSS T3 (2.1 mm x 100 mm, 1.8 μm, Waters, USA). The mobile phases were 0.1% formic acid-water solution (A) and acetonitrile (B) with the following gradients: 0-0.5 min, 2% B; 0.5-10 min, 2%-50% B; 10-11 min, 50%-95% B; 11-13 min, 95% A; 13-13.1 min, 95%-2% B; 13.1-15 min, 2% B. The column temperature was set at 40 °C and the flow rate was 0.4 mL/min. The auto-sampler temperature was set at 4 °C and the injection volume was 2 μL.
A SCIEX 6500 QTRAP+ triple quadrupole mass spectrometer equipped with an IonDrive Turbo V ESI ion source was used for the mass spectrometry analysis in multiple reaction monitoring (MRM) modes. The ion source parameters were as follows: IonSpray Voltage: +5500/-4500 V, Curtain Gas: 35 psi, Temperature: 400 °C, Ion Source Gas 1:60 psi, Ion Source Gas 2: 60 psi, DP: ± 100 V. Data acquisition is performed in full scan mode in the range of m/z 50-1000. Analyst Work Station Software (Version 1.6.3, SCIEX, USA) was used for all mass spectrometry data acquisition and quantitative analysis of target compounds. The MS conventer software was used to convert the raw mass spectra into TXT format. Then the R program package was used for peak extraction and annotation in combination with a self-created database. MRM transitions and MS conditions for the identification of compounds were shown in SM-Table 2.
2.4.2 HPLC quantitative analysis
The determination was performed by high-performance liquid chromatography (HPLC, Agilent 1260, Agilent Technologies Inc., Santa Clara, CA, USA) using a C18 (250 mm×4.6 mm, 5 μm; Agilent Technologies Inc., Santa Clara, CA, USA) column. The mobile phases were methanol (A) and 2% formic acid-water solution (B), and the gradient was as follows: 0-10 min, 10%-30% A; 10-20 min, 30%-55% A; 20-35 min, 55%-80% A; 35-40 min, 80%-84% A; 40-45 min, 84%-100% A; 45-50 min, 100% A; 50-56 min, 100% A. The column temperature was 35°C, the flow rate was 0.8 mL/min, the injection volume was 20 μL, and the UV detection wavelength was set to 290 nm. The contents of 11 compounds identified in this study were calculated by the external standard method, that is, by referring to the peak area of standard compounds. The standards for the 11 compounds in this study were purchased from Yuanye Bio-Technology Co., Ltd. (Shanghai, China). Standard solutions were prepared in methanol with an initial concentration of 1 mg/mL, and a standard curve was drawn after serial dilution (SM-Table 3, SM-Figure 2).
2.5 Enzyme activity inhibition test
2.5.1 Cholinesterase inhibitory activity assays
A modified Ellman’s method was used to assess the AChE and BChE enzyme inhibitory activities of J. sabina extracts (Ellman et al., 1961). The extracts were dissolved as gradient dilutions using 2% DMSO water for activity determination. The extract gradient dilutions (1000, 500, 250, 125, 62.5, and 31.25 µg/mL) 20 μL were incubated with AChE (0.28 U/mL, 20 μL) and BChE (0.28 U/mL, 20 μL) in phosphate buffer (PBS, pH 7.8, 140 µL) for 15 min in 25°C. Afterwards, acetylthiocholine iodide and butyrylthiocholine chloride were added as the substrates (10 µL) for AChE and BChE, respectively. Simultaneously, the coloring reagent 5,5-dithio-bis (2-nitrobenzoic) acid (DTNB, 10 µL) was added and incubated at 37°C for 30 minutes. The absorbance values of the sample wells were measured at 412 nm. PBS buffer was used as a blank, and galanthamine was used as the reference drug. Analyses were performed in triplicate. The cholinesterase inhibitory was calculated according to the following equation:
Cholinesterase inhibitory (%) = (Blank group -Experimental group)/Blank group *100%
AChE (electric eel), BChE (horse serum), and galanthamine were all purchased from Sigma-Aldrich (Sigma, St. Louis, MO, USA).
2.5.2 α-Glucosidase inhibitory activity assay
The α-glucosidase (α-Glu) inhibition assay was performed according to the method of Zaharudin et al. (2019) with a slight modification. The extract gradient dilutions (1000, 500, 250, 125, 62.5, and 31.25 µg/mL) 10 µL, α-glucosidase (2.0 U/mL, 10 µL) and PBS (pH 6.8, 80 µL) were added to a 96-well microtiter plate. The mixture was incubated for 10 min at 37°C. The p-nitrophenyl-α-d-glucopyranoside (20 µL) was mixed into the solution and incubated at 37°C for 20 min to initiate the reaction. The reaction was aborted by the addition of sodium carbonate solution (100 μL). Acarbose was used as the reference drug, and 2% DMSO water was used as the solvent control. Enzyme inhibitory was quantified by measuring the release of p-nitrophenol at 405 nm.
All experiments were performed in triplicate. α-Glucosidase inhibitory effect was calculated by the following equation:
α-Glucosidase inhibitory (%) = Among them, AS = sample absorbance value, AK = control (2% DMSO water) absorbance value, and the background absorbance value of the reaction system was subtracted without enzyme in AS0 and AK0.
2.6 Antibacterial activity determination
Bacterial strains of methicillin-resistant Staphylococcus aureus (MRSA, ATCC4300) and vancomycin-resistant Escherichia coli (VREC) were provided by the Key Laboratory of Synthetic and Natural Functional Molecule Chemistry of Ministry of Education, Chemical Biology Innovation Laboratory, College of Chemistry and Materials Science, Northwest University (Chen and Yang, 2019). The extracts of J. sabina were dissolved in a 2% DMSO-water solution to prepare the samples to be tested at the corresponding concentrations and passed through 0.22 μm Millipore Express (Bio-protocol LLC., China) for later use. Antimicrobial resistance tests were performed according to CLSI microbiological test standards (CLSI, 2010). Specific experimental methods were referred to the adapted test method of Falcão et al. (2018). Individual colonies of clinical isolates MRSA and VREC on LB agar plates were transferred to 5 mL Mueller-Hinton (MH) liquid medium and grown overnight at 37°C. Bacterial cells were collected by centrifugation (4000 rpm, 10 min). After discarding the supernatant, the pelleted cells were resuspended in MH medium and diluted to an OD600 of 0.5. The sample solution (20 µL) and bacterial suspension (105 CFU/mL, 180 µL) were mixed and incubated at 37°C for 18 h. Vancomycin hydrochloride and colistin sulfate were used as positive controls for MRSA and VREC, respectively. The microdilution minimum inhibitory concentration (MIC) was interpreted as the lowest drug concentration that completely inhibited the visible growth of bacteria (OD600< 0.1) after incubating the plates for at least 16 h at 37°C. Each inhibitor was tested in triplicate.
2.7 Statistical analysis
The assays described were performed in triplicate for all experiments and the results are expressed as mean ± standard deviation (SD). All experiments were repeated three times. Statistical analysis was made by one-way analysis of variance (ANOVA) in SPSS 18.0 software. Redundancy analysis (RDA) was carried out in R (version 4.1.2) using vegan package.
3 Results and discussion
3.1 Variation of TPC and TLC in J. sabina during ages
This study evaluated the content of total phenols and lignans in different tissue extracts from different ages of J. sabina. Table 1 showed that among the tissues, the roots were richer in phenolic substances. The highest TPC (487.16 mg GAE/g DW) was found in root extracts from fifteen annual J. sabina. The total phenol content in leaves was also considerable, and it showed an increasing trend with the increase in tree age. The determination results of total lignans showed that TLC in J. sabina leaves was the highest (306.09-491.24 mg PPT/g DW), and it showed an increasing trend with the increase in tree age (Table 1). The highest total lignan content was found in the current year’s leaf extracts of fifteen annual J. sabina. TPC and TLC showed considerable differences in the age and tissues of J. sabina. The roots and leaves are the dominant tissues at different ages for the distribution of total phenols and total lignans, respectively.
Previous studies have shown that the main active substances in J. sabina were found to be phenols and lignans, and phenols are important secondary metabolites for plant resistance to biotic and abiotic stresses (Sarkar and Shetty, 2014; Tavares and Seca, 2018). In this study, the TPC of J. sabina was higher than that reported by Gonçalves et al. (2022). In addition, the lignans and their derivatives in J. sabina leaves exhibited excellent insecticidal and acaricidal activities (Yang et al., 2017; Yang et al., 2021). The diversity and heterogeneity of total lignans enriched in J. sabina leaves and roots provided broad-spectrum protective defenses against a variety of pathogens and pests. The lignans (podophyllotoxin, deoxypodophyllotoxin, etc.) in J. sabina were also shown to have anti-tumor and antimicrobial activity (Liu et al., 2021).
3.2 Identification of phenols and podophyllotoxins by HPLC/HPLC-MS
The HPLC characterization of J. sabina extracts allowed the identification of a total of 11 characteristic compounds, separated by their molecular mass and according to their retention time (Table 2) using the MS analyst Work Station Software (Version 1.6.3). Positive ionisation was used as the MS operating condition for the identification of the compounds. The present study showed that most phenols were more abundant in the tissues of adult J. sabina compared to juvenile J. sabina. The most common taxonomic substances included catechin (SM-Figure 3A), epicatechin and curcumin, followed by apigenin and amentoflavone, which were only found in tissues other than the roots of J. sabina, and luteolin, which was only found in the leaves of adult J. sabina. Compound 11 was identified as curcumin by comparison with the standards (SM-Figure 3B). In the (+) ESI-MS/MS analysis, the product ion m/z 177.1 was observed as the dominant peak (SM-Figure 3B; SM-Figure. 4), which is consistent with the characteristic ion peak of curcumin previously reported (Jia et al., 2017). This is the first time that curcumin has been found in juniper. The second most common group of compounds were the podophyllotoxins, which belong to the aryl naphthalene group of lignans, such as podophyllotoxin (SM-Figure 3C) (deoxypodophyllotoxin, matairesinol, α-Peltatin, and podophyllotoxinone). These compounds were identified in all ages of J. sabina leaves.
The results of the quantitative analysis (Table 2) showed significant differences in the age and tissue distribution of individual phenol and podophyllotoxin compounds in J. sabina. The total distribution of 11 compounds in J. sabina of different ages was in the order: fifteen annual > seven annual > biennial (Figure 2), among which the more abundant substances were catechin, curcumin, podophyllotoxin and α-peltatin. The highest curcumin content (6.49 mg/g DW) was obtained in previous year’s leaves of fifteen annual J. sabina. The highest contents of the other three compounds were obtained from the current year leaves of fifteen annual J. sabina, which were catechin (21.66 mg/g DW), podophyllotoxin (7.58 mg/g DW) and α-peltatin (5.31 mg/g DW). The content of most of the tested compounds in the different tissues of J. sabina was in decreasing order: current year leaves > previous year leaves > branch > current year stem > previous year stem > root (Figure 2). The current data suggests that the content of phenols and podophyllotoxins may be highly dependent on the growth process of secondary metabolite transformation and biosynthesis in the organs of J. sabina, as in earlier studies on other plants (Bai et al., 2017; Feng et al., 2014; Kim et al., 2020).
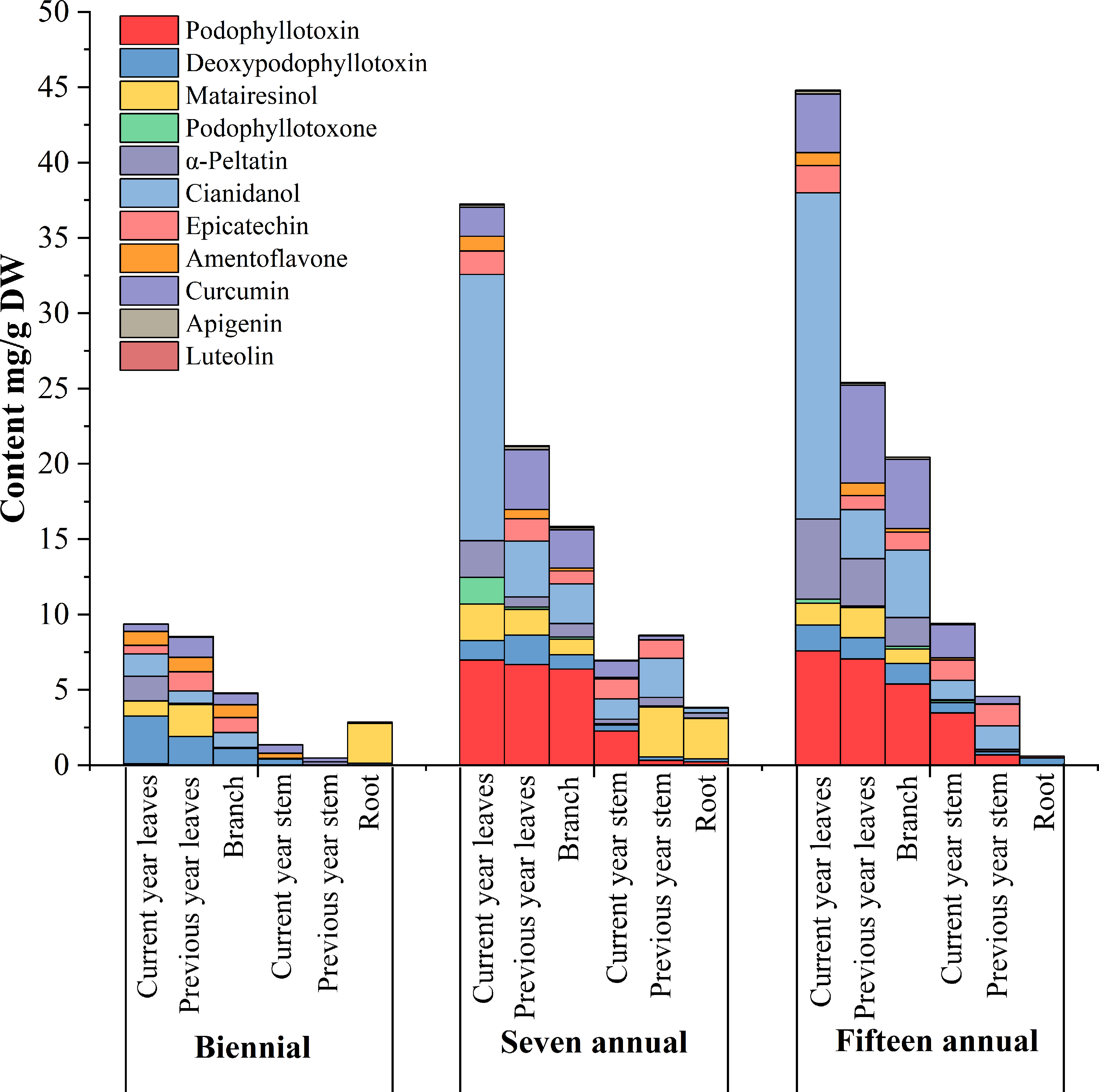
Figure 2 Accumulation analysis of compounds in different tissues extracts of different ages J. sabina.
The results of the clustering (Figure 3) of the 11 identified components in J. sabina showed that the extracts were divided into 3 groups: the current year leaves of seven and fifteen annual J. sabina were divided into Group I; the previous year leaves and branches of seven and fifteen annual J. sabina were divided into Group II; the remaining other tissues were divided into Group III. Figure 3 showed that 11 compounds had higher content in Group I and Group II, mainly in the leaves and branches of adult J. sabina. The leaves and branches of J. sabina were the main medicinal tissues in traditional ethnopharmacological applications, which also showed consistency with our clustering results (Kavaz and Faraj, 2023). Significantly, the compounds detected in this study were low in young J. sabina, so age was probably a key factor in the quality of the medicinal tissues of J. sabina (Ghasemzadeh et al., 2014).
The bioactive compounds in J. sabina are of economic and health importance. The phenol catechin belongs to a family of polyphenols that have been reported to have anti-inflammatory (Monika et al., 2023), anti-tumour (Rawangkan et al., 2018; Shin et al., 2018), anti-diabetic (Silva et al., 2018; Spínola et al., 2019) and neuroprotective effects (Ortiz-López et al., 2016). Podophyllotoxins have received much attention for their good in vitro anticancer activity (Kumari et al, 2017). The Juniperus genus is a potential source of podophyllotoxins (Tavares and Seca, 2018). As many as 22 species of podophyllotoxins and their derivatives have been reported to be isolated and identified in J. sabina (Li et al., 2006). However, in the present manuscript, only a small number of podophyllotoxins were identified, including podophyllotoxin, deoxypodophyllotoxin, and podophyllotoxinone. The synthesis of podophyllotoxins is affected by factors such as growth environment, genetics, sampling time and location (Yang et al., 2016; Li et al., 2018). At the same time, this also shows the complexity of natural active products.
3.3 Validation of three enzyme inhibitory activities in vitro
This study analyzed the AChE, BChE, and α-glucosidase inhibitory activities of J. sabina extracts from various tissues (Table 3). The results revealed that the best inhibition results of AChE (47.37 μg/mL) and BChE (136.3 μg/mL) were shown in the leaves of adult J. sabina. However, extracts from J. sabina seedlings as well as branches and roots of adult J. sabina showed no significant inhibitory activity (>1000 μg/mL). In addition, all J. sabina extracts tested in this study were seen to have an inhibitory effect (62.59-412.43 μg/mL) on α-glucosidase (Table 3). Compared with cholinesterase, the stem (IC50 62.59 μg/mL) and branch (IC50 93.46 μg/mL) of biennial J. sabina showed the best α-glucosidase inhibitory activities, and they were only a few times higher than the positive control’s values (26.60 μg/mL). The stems and branches of young J. sabina appear to be more promising application tissues for α-glucosidase inhibitory activity.
In the present study, it was found that the natural active constituents of J. sabina, along with other juniper plants, play an important role in the inhibitory properties of AChE, BChE, and α-glucosidase (Jung et al., 2015; Orhan et al., 2017; Tavares and Seca, 2018). The extracts of J. sabina in this study caused similar levels of AChE and BChE inhibition, comparable to extracts from other Juniperus leaves (Orhan et al., 2017; Tavares and Seca, 2018). And the inhibitory activity of J. sabina leaves on AChE and BChE may come from their lignans and phenolic compounds alone or in combination. Additionally, the results of α-glucosidase inhibitory activity in J. sabina extracts are higher than those of other Juniperus extracts (Kim et al., 2014; Orhan et al., 2017). At present, α-glucosidase inhibitors have become an important strategy for the treatment of diabetes (type II) (Cardullo et al., 2020). Flavonoids and biflavonoids in juniper seem to be the main factors to exert their hypoglycemic activity (Hee-Sung et al., 2023).
3.4 Anti-drug resistant bacteria activity in vitro
In this study, the microdilution method was used to determine the antibacterial activity of J. sabina extracts. The two clinically resistant strains were MRSA and VREC. Table 4 showed that the antibacterial range of J. sabina extracts was 31.25-2000 μg/mL, and the antibacterial range of the positive control drug was 0.39-0.58 μg/mL. The order of antibacterial activity of the different tissues was root > previous year stem > current year stem > branch and leaves. The root extracts of fifteen annual J. sabina showed the best antibacterial activity against MRSA (MIC 31.25 μg/mL). However, the J. sabina extracts did not show significant inhibitory activity against VREC (MIC ≥500 μg/mL).
The extracts from Juniperus branches, leaves, and berries showed broad-spectrum antibacterial properties, especially for S. aureus (Taviano et al., 2013; Dziedzinski et al., 2020). In addition, as key phenols and podophyllotoxins of the extracts, catechin, curcumin, and podophyllotoxin were previously shown to have inhibitory activity against S. aureus and E. coli in previous studies (Rocha et al., 2018; Muniz et al., 2021). However, the content of the above compounds in the roots of the best inhibiting tissues was low. Accordingly, the antibacterial activity of the different tissues of J. sabina cannot be simply attributed to the high content of phenolic and podophyllotoxins in the extracts. The antibacterial activity of J. sabina may be influenced by other podophyllotoxin, phenol and undetected components, or by synergistic effects between them.
3.5 Chemometrics analysis of J. sabina samples
In order to investigate the main factors influencing the biological activity of the extracts of J. sabina, the values of the inhibition rates of the three enzymes and the MIC values of the antibacterial activity were selected for analysis in relation to the content and composition of the extract compounds. As the content of podophyllotoxin and catechin showed a significant linear correlation (VIF > 10) with the bioactivity of J. sabina, nine compounds other than them were selected for Redundancy analysis (RDA) with bioactivity in this study. The RDA1 and RDA2 explained 90.80% and 6.61% of the variance, respectively. Obtuse angles indicate a negative correlation, and acute angles indicate a positive correlation. The results of the RDA analysis (Figure 4) showed that the living activity of the extract of J. sabina showed a close correlation with epicatechin and matairesinol. Studies have shown that epicatechin and matairesinol have positive effects on antibacterial, anti-acetylcholinesterase, anti-α-amylase, anti-butyrylcholinesterase and anti-α-glucosidase activities (Kim et al., 2016; Bursal et al., 2019). In addition, the clustering results of samples showed that the biological activity of J. sabina samples was closely related to their age, and adult J. sabina seemed to have better application potential than younger J. sabina, which might be related to the rich accumulation and composition of active compounds in adult J. sabina.
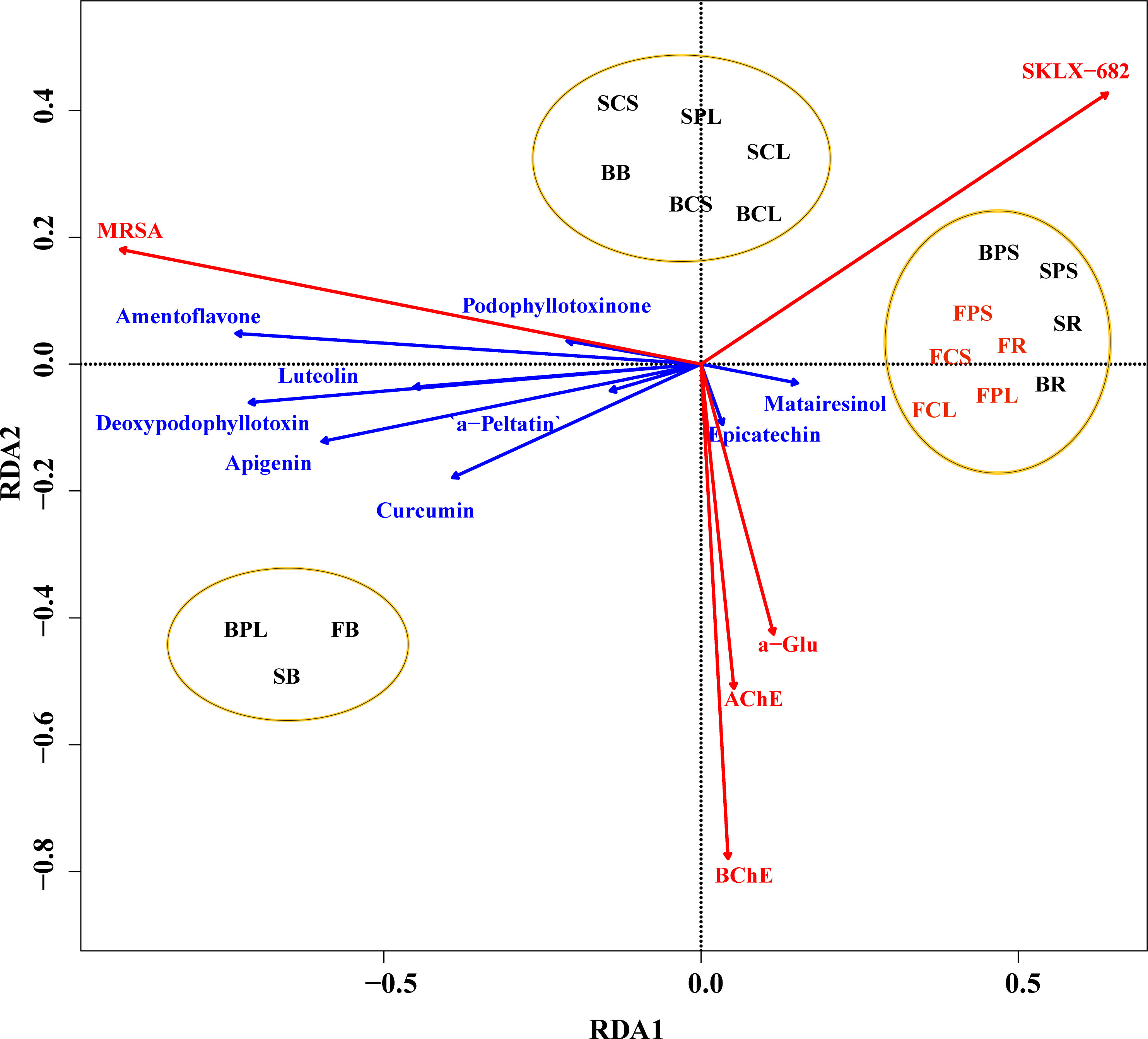
Figure 4 RDA analysis of J. sabina samples. Reaction variables (biological activity of the extract, marked by red arrow), explanatory variables (compound content, marked by blue arrow), sample classification cluster (marked by ellipse).
4 Conclusions
The present study reports for the first time the phenolic and lignan content, compositional characteristics, and biological profile of the methanol extract of J. sabina. The leaves and roots of fifteen annual J. sabina contain the highest levels of total lignans and total phenols. High levels of TPC (87.16 mg GAE/g DW) and TLC (491.24 mg PPT/g DW) were detected in fifteen annual J. sabina. Eleven phenols and lignans were identified by HPLC/HPLC-MS, which were mainly distributed in the leaves of adult J. sabina. Studies on the biological activities found that the extracts of seven annual J. sabina roots and fifteen annual J. sabina current year leaves had the potential to be anti-MRSA and neuroprotective agents, respectively. In addition, the extract of biennial J. sabina branches possessed excellent anti-diabetic activity, and its exact mechanism could be further investigated. The results of the RDA analysis showed that the biological activity of the extract of J. sabina showed a close correlation with epicatechin and matairesinol. The results of this study support the potential application value of J. sabina in neuroprotection, diabetes, and anti-drug resistance bacteria. And it will help facilitate the application of J. sabina resources in the functional food, pharmaceutical, and nutraceutical industries.
Data availability statement
The original contributions presented in the study are included in the article/Supplementary Material. Further inquiries can be directed to the corresponding author.
Author contributions
SX: Methodology, Software, Formal analysis, Writing - Original Draft. QC: Software, Formal analysis, Resources, Writing - Review & Editing. NL: Resources, Data Curation. JY: Formal analysis, Investigation, Supervision. DL: Conceptualization, Methodology, Supervision. All authors contributed to the article and approved the submitted version.
Funding
This work was supported by the special fund for the Key Research and Development Program of Shaanxi Province (2023-YBNY-001) and the program from the National Natural Science Foundation of China (No. 31570655).
Conflict of interest
The authors declare that the research was conducted in the absence of any commercial or financial relationships that could be construed as a potential conflict of interest.
Publisher’s note
All claims expressed in this article are solely those of the authors and do not necessarily represent those of their affiliated organizations, or those of the publisher, the editors and the reviewers. Any product that may be evaluated in this article, or claim that may be made by its manufacturer, is not guaranteed or endorsed by the publisher.
Supplementary material
The Supplementary Material for this article can be found online at: https://www.frontiersin.org/articles/10.3389/fpls.2023.1174922/full#supplementary-material
References
Al-Snafi, A. E. (2018a). Medical importance of JuniPerus communis-a review. Indo Am. J. Pharm. Sci. 5 (3), 1779–1792. doi: 10.5281/zenodo.1210529
Al-Snafi, A. E. (2018b). Pharmacological and therapeutic effects of JuniPerus oxycedrus-A review. Indo Am. J. Pharm. Sci. 5, 2198–2205. doi: 10.5281/zenodo.1214996
Bai, Y., Xu, Y., Wang, B., Li, S., Gou, F., Hua, H., Zhao, Y., Yu, Z. (2017). Comparison of phenolic compounds, antioxidant and antidiabetic activities between selected edible beans and their different growth periods leaves. J. Funct. Foods 35, 694–702. doi: 10.1016/j.jff.2017.06.036
Bielecka, M., Zielińska, S., Pencakowski, B., Stafiniak, M., Ślusarczyk, S., Prescha, A., et al. (2019). Age-related variation of polyphenol content and expression of phenylpropanoid biosynthetic genes in Agastache rugosa. Ind. Crop Prod. 141, 111743. doi: 10.1016/j.indcrop.2019.111743
Bursal, E., Aras, A., Kılıç, Ö., Taslimi, P., Gören, A. C., Gülçin, İ. (2019). Phytochemical content, antioxidant activity, and enzyme inhibition effect of Salvia eriophora Boiss. & Kotschy against acetylcholinesterase, α-amylase, butyrylcholinesterase, and α-glycosidase enzymes. J. Food Biochem. 43 (3), e12776. doi: 10.1038/sj.ijo.080292610.1111/jfbc.12776
Butnaru, E., Pamfil, D., Stoleru, E., Brebu, M. (2022). Characterization of bark, needles and cones from silver fir (Abies alba Mill.) towards valorization of biomass forestry residues. Biomass Bioenerg. 159, 106413. doi: 10.1016/j.biombioe.2022.106413
Cardullo, N., Muccilli, V., Pulvirenti, L., Cornu, A., Pouységu, L., Deffieux, D., et al. (2020). Tringali, C. C-glucosidic ellagitannins and galloylated glucoses as potential functional food ingredients with anti-diabetic properties: A study of α-glucosidase and α-amylase inhibition. Food Chem. 313, 126099. doi: 10.1016/j.foodchem.2019.126099
Chen, C., Yang, K. (2019). Ebselen bearing polar functionality: Identification of potent antibacterial agents against multidrug-resistant Gram-negative bacteria. Bioorganic Chem. 93, 103286. doi: 10.1016/j.bioorg.2019.103286
Cheng, J. X., Su, S. P., Yang, Y. Z., Chen, C. G., Zhang, X. (2005). SFE-HPLC analysis of podophyllotoxin content in the stem and leave of Sabina vulgaris of different habitats and ages. J. Northwest A F Univ. (Natural Sci. Edition) 04), 57–60. doi: 10.13207/j.cnki.jnwafu.2005.04.013
CLSI (2010). Performance standards for antimicrobial susceptibility testing: 20th informational supplement, CLSI document M100-S20 (Wayne, PA: Clinical and Laboratory Standards Institute).
Dossou, S. S. K., Xu, F., Cui, X., Sheng, C., Zhou, R., You, J., et al. (2021). Comparative metabolomics analysis of different sesame (Sesamum indicum L.) tissues reveals a tissue-specific accumulation of metabolites. BMC Plant Biol. 21 (1), 1–14. doi: 10.1186/s12870-021-03132-0
Dziedzinski, M., Kobus-Cisowska, J., Szymanowska, D., Stuper-Szablewska, K., Baranowska, M. (2020). Identification of polyphenols from coniferous shoots as natural antioxidants and antimicrobial compounds. Molecules 25 (15), 3527. doi: 10.3390/molecules25153527
Ellman, G. L., Courtney, K. D., Andres, J. V., Featherstone, R. M. (1961). A new and rapid colorimetric determination of acetylcholinesterase activity. Biochem. Pharmacol. 7, 88–90. doi: 10.1016/0006-2952(61)90145-9
Esteban, L. S., Mediavilla, I., Xavier, V., Amaral, J. S., Pires, T. C. S. P., Calhelha, R. C., et al. (2023). Yield, chemical composition and bioactivity of essential oils from common Juniper (JuniPerus communis L.) from different spanish origins. Molecules 28, 4448. doi: 10.3390/molecules28114448
Falcão, S., Bacém, I., Igrejas, G., Rodrigues, P. J., Vilas-Boas, M., Amaral, J. S. (2018). Chemical composition and antimicrobial activity of hydrodistilled oil from juniper berries. Ind. Crop Prod. 124, 878–884. doi: 10.1016/j.indcrop.2018.08.069
Feng, S., Luo, Z., Zhang, Y., Zhong, Z., Lu, B. (2014). Phytochemical contents and antioxidant capacities of different parts of two sugarcane (Saccharum officinarum L.) cultivars. Food Chem. 151, 452–458. doi: 10.1016/j.foodchem.2013.11.057
Ghasemzadeh, A., Nasiri, A., Jaafar, H. Z., Baghdadi, A., Ahmad, I. (2014). Changes in phytochemical synthesis, chalcone synthase activity and pharmaceutical qualities of Sabah snake grass (Clinacanthus nutans L.) in relation to plant age. Molecules 19 (11), 17632–17648. doi: 10.3390/molecules191117632
Gómez-Martínez, M., Ascacio-Valdés, J. A., Flores-Gallegos, A. C., González-Domínguez, J., Gómez-Martínez, S., Aguilar, C. N., et al. (2020). Location and tissue effects on phytochemical composition and in vitro antioxidant activity of Moringa oleifera. Ind. Crop Prod. 151, 112439. doi: 10.1016/j.indcrop.2020.112439
Gonçalves, A. C., Flores-Félix, J. D., Coutinho, P., Alves, G., Silva, L. R. (2022). Zimbro (JuniPerus communis L.) as a promising source of bioactive compounds and biomedical activities: a review on recent trends. Int. J. Mol. Sci. 23 (6), 3197. doi: 10.3390/ijms23063197
Hee-Sung, C., Olivia, D., Tahir, M. M., Mohammad, K. A., Bharathi, A., Larry, A. W., et al. (2023). Juniper berries regulate diabetes and obesity markers through modulating PPARα, PPARγ, and LXR: in vitro and in vivo effects. J. Med. Food., 26(5), 307–318. doi: 10.1089/jmf.2022.0146
Ivanova, D., Nedialkov, P., Tashev, A., Kokanova-Nedialkova, Z., Olech, M., Nowak, R., et al. (2023). Anticancer podophyllotoxin recovery from juniper leaves at atmospheric and high pressure using eco-friendly solvents. Plants 12, 1526. doi: 10.3390/plants12071526
Jia, S. L., Du, Z. F., Song, C. W., Jin, S. N., Zhang, Y., Feng, Y. L., et al. (2017). Identification and characterization of curcuminoids in turmeric using ultra-high performance liquid chromatography-quadrupole time of flight tandem mass spectrometry. J. Chromatogr. A. 1521, 110–122. doi: 10.1016/j.chroma.2017.09.032
Jung, H. J., Jung, H. A., Min, B. S., Choi, J. S. (2015). Anticholinesterase and β-site amyloid precursor protein cleaving enzyme inhibitory compounds from the heartwood of JuniPerus chinensis. Chem. Pharm. Bull. 63 (11), 955–960. doi: 10.1248/cpb.c15-00504
Kavaz, D., Faraj, R. E. (2023). Investigation of composition, antioxidant, antimicrobial and cytotoxic characteristics from JuniPerus sabina and Ferula communis extracts. Sci. Rep. 13, 7193. doi: 10.1038/s41598-023-34281-x
Kim, J. H., Lee, S. H., Lee, H. W., Sun, Y. N., Jang, W. H., Yang, S. Y., et al. (2016). (-)-Epicatechin derivate from Orostachys japonicus as potential inhibitor of the human butyrylcholinesterase. Int. J. Biol. Macromol. 91, 1033–1039. doi: 10.1016/j.ijbiomac.2016.06.069
Kim, J. H., Lee, S. Y., Park, J. M., Park, J. H., Kwon, O. J., Lee, J. Y. (2014). Antioxidant activity and inhibition activity against α-amylase and α-glucosidase of JuniPerus rigida Sieb extracts. Korean J. Food Preservation 21 (3), 396–403. doi: 10.11002/kjfp.2014.21.3.396
Kim, D. H., Yang, W. T., Cho, K. M., Lee, J. H. (2020). Comparative analysis of isoflavone aglycones using microwave-assisted acid hydrolysis from soybean organs at different growth times and screening for their digestive enzyme inhibition and antioxidant properties. Food Chem. 305, 125462. doi: 10.1016/j.foodchem.2019.125462
Kumari, A., Singh, D., Kumar, S. (2017). Biotechnological interventions for harnessing podophyllotoxin from plant and fungal species: current status, challenges, and opportunities for its commercialization. Crit. Rev. Biotechnol. 37 (6), 739–753. doi: 10.1080/07388551.2016.1228597
Li, G. Z., He, J., Yan, H. Y., Feng, R. H., Feng, J. T. (2006). Advances in the studies on chemical constituents and bioactivities of Sabina vulgaris Ant. J. Northwest A F Univ. (Natural Sci. Edition). 34 (10), 133–139. doi: 10.13207/j.cnki.jnwafu.2006.10.027
Li, M., Sun, P., Kang, T., Xing, H., Yang, D., Zhang, J., et al. (2018). Mapping podophyllotoxin biosynthesis and growth-related transcripts with high elevation in Sinopodophyllum hexandrum. Ind. Crop Prod. 124, 510–518. doi: 10.1016/j.indcrop.2018.08.007
Lim, D. J., Song, J. S., Lee, B. H., Son, Y. K., Kim, Y. (2023). Qualitative and quantitative analysis of the major bioactive components of JuniPerus chinensis L. Using LC-QTOF-MS and LC-MSMS and investigation of antibacterial activity against pathogenic bacteria. Molecules 28, 3937. doi: 10.3390/molecules28093937
Liu, W., Yin, D. X., Tang, N., Zhang, T., Wang, J., Qin, D. H., et al. (2021). Quality evaluation of Sinopodophyllum hexandrum (Royle) Ying based on active compounds, bioactivities and RP-HPLC fingerprint. Ind. Crop Prod. 174, 114159. doi: 10.1016/j.indcrop.2021.114159
Majewska, E., Kozłowska, M., Kowalska, D., Gruczynska, E. (2017). Characterization of the essential oil from cone-berries of JuniPerus communis L. (Cupressaceae). Herba Polonica 63 (3), 48–55. doi: 10.1515/hepo-2017-0018
Monika, P., Chandraprabha, M. N., Murthy, K. N. C. (2023). Catechin, epicatechin, curcumin, garlic, pomegranate peel and neem extracts of Indian origin showed enhanced anti-inflammatory potential in human primary acute and chronic wound derived fibroblasts by decreasing TGF-β and TNF-α expression. BMC Complement Med. Ther. 23, 181. doi: 10.1186/s12906-023-03993-y
Muniz, I. P. R., Galantini, M. P. L., Ribeiro, I. S., Gonçalves, C. V., Dos Santos, D. P., Moura, T. C., et al. (2021). Antimicrobial photodynamic therapy (aPDT) with curcumin controls intradermal infection by Staphylococcus aureus in mice with type 1 diabetes mellitus: a pilot study. J. Photochem. Photobiol. B-Biol. 224, 112325. doi: 10.1016/j.jphotobiol.2021.112325
Orhan, N., Orhan, D. D., Gökbulut, A., Aslan, M., Ergun, F. (2017). Comparative analysis of chemical profile, antioxidant, in-vitro and in-vivo antidiabetic activities of JuniPerus foetidissima Willd. and JuniPerus sabina L. Iran. J. Pharm. Res. 16 (Suppl), 64. doi: 10.22037/IJPR.2017.1962
Ortiz-López, L., Márquez-Valadez, B., Gómez-Sánchez, A., Silva-Lucero, M. D. C., Torres-Pérez, M., Téllez-Ballesteros, R. I., et al. (2016). Green tea compound epigallo-cathechin-3-gallate (EGCG) increases neuronal survival in adult hippocampal neurogenesis in vivo and in vitro. Neuroscience 322, 208–220. doi: 10.1016/j.neuroscience.2016.02.040
Rangel, M. L., Guerrero-Analco, J. A., Monribot-Villanueva, J. L., Kiel-Martínez, A. L., Avendaño-Reyes, S., Abad, J. P. D., et al. (2018). Anatomical and chemical characteristics of leaves and branches of JuniPerus deppeana var. deppeana (Cupressaceae): A potential source of raw materials for the perfume and sweet candies industries. Ind. Crop Prod. 113, 50–54. doi: 10.1016/j.indcrop.2017.12.046
Rawangkan, A., Wongsirisin, P., Namiki, K., Iida, K., Kobayashi, Y., Shimizu, Y., et al. (2018). Green tea catechin is an alternative immune checkpoint inhibitor that inhibits PD-L1 expression and lung tumor growth. Molecules 23, 2071. doi: 10.3390/molecules23082071
Rehman, N. U., Shah, M., Ullah, S., Khan, M., Khan, A., Ullah, O., et al. (2022). Enzymes inhibition and antioxidant potential of medicinal plants growing in Oman. BioMed. Res. Int. 2022, 7880387–7880387. doi: 10.1155/2022/7880387
Rocha, M. P., Campana, P. R. V., Scoaris, D. D. O., Almeida, V. L. D., Lopes, J. C. D., Shaw, J. M. H., et al. (2018). Combined in vitro studies and in silico target fishing for the evaluation of the biological activities of Diphylleia cymosa and Podophyllum hexandrum. Molecules 23 (12), 3303. doi: 10.3390/molecules23123303
Sarkar, D., Shetty, K. (2014). Metabolic stimulation of plant phenolics for food preservation and health. Annu. Rev. Food Sci. Technol. 5 (1), 395–413. doi: 10.1146/annurev-food-030713-092418
Shin, C. M., Lee, D. H., Seo, A. Y., Lee, H. J., Kim, S. B., Son, W. C., et al. (2018). Green tea extracts for the prevention of metachronous colorectal polyps among patients who underwent endoscopic removal of colorectal adenomas: A randomized clinical trial. Clin. Nutr. 37 (2), 452–458. doi: 10.1016/j.clnu.2017.01.014
Silva, C. P., Sampaio, G. R., Freitas, R. A. M. S., Torres, E. A. F. S. (2018). Polyphenols from guaraná after in vitro digestion: evaluation of bioacessibility and inhibition of activity of carbohydrate-hydrolyzing enzymes. Food Chem. 267, 405–409. doi: 10.1016/j.foodchem.2017.08.078
Spengler, G., Gajdács, M., Donadu, M. G., Usai, M., Marchetti, M., Ferrari, M., et al. (2022). Evaluation of the antimicrobial and antivirulent potential of essential oils isolated from JuniPerus oxycedrus L. ssp. macrocarpa aerial parts. Microorganisms 10 (4), 758. doi: 10.3390/microorganisms10040758
Spínola, V., Pinto, J., Llorent-Martínez, E. J., Tomas, H., Castilho, P. C. (2019). Evaluation of Rubus grandifolius L. (wild blackberries) activities targeting management of type-2 diabetes and obesity using in vitro models. Food Chem. Toxicol. 123, 443–452. doi: 10.1016/j.fct.2018.11.006
Tavares, W. R., Seca, A. M. (2018). The current status of the pharmaceutical potential of JuniPerus L. metabolites. Medicines 5 (3), 81. doi: 10.3390/medicines5030081
Taviano, M. F., Marino, A., Trovato, A., Bellinghieri, V., Melchini, A., Dugo, P., et al. (2013). JuniPerus oxycedrus L. subsp. oxycedrus and JuniPerus oxycedrus L. subsp. macrocarpa (Sibth. & Sm.) Ball.”berries” from Turkey: comparative evaluation of phenolic profile, antioxidant, cytotoxic and antimicrobial activities. Food Chem. Toxicol. 58, 22–29. doi: 10.1016/j.fct.2013.03.049
Wang, Y. S., Dang, H. Z., Wang, L. H., Zhang, G. S., Li, Q. X. (2014). Preliminary analysis on the geographical differences of growth characteristics of JuniPerus sabina Ant. in China. J. Arid Land Resour. Environ. 28 (11), 98102. doi: 10.13448/j.cnki.jalre.2014.11.017
Xu, S., Li, X., Liu, S., Tian, P., Li, D. (2022). JuniPerus sabina L. as a source of podophyllotoxins: extraction optimization and anticholinesterase activities. Int. J. Mol. Sci. 23 (18), 10205. doi: 10.3390/ijms231810205
Yang, R., Huang, X., Che, Z., Zhang, Y., Xu, H. (2017). Application of sustainable natural resources in crop protection: Podophyllotoxin-based botanical pesticides derived from Podophyllum hexandrum for controlling crop-threatening insect pests. Ind. Crop Prod. 107, 45–53. doi: 10.1016/j.indcrop.2017.05.033
Yang, R., Ma, M., Lv, M., Zhang, S., Xu, H. (2021). Non-food bioactive products for pesticides candidates (III): Agricultural properties of isoxazole esters from the plant product podophyllotoxin as botanical pesticides. Ind. Crop Prod. 174, 114181. doi: 10.1016/j.indcrop.2021.114181
Yang, D. L., Sun, P., Li, M. F. (2016). Chilling temperature stimulates growth, gene over-expression and podophyllotoxin biosynthesis in Podophyllum hexandrum Royle. Plant Physiol. Biochem. 107, 197–203. doi: 10.1016/j.plaphy.2016.06.010
Keywords: Juniperus sabina L., phenols, lignans, neuroprotection and antidiabetic activities, anti-bacteria activities
Citation: Xu S, Chen Q, Luo N, Yang J and Li D (2023) Effects of age and tissue of Juniperus sabina L. on its phytochemical characteristics, anti-cholinesterase, antidiabetes, and anti-drug resistant bacteria activities. Front. Plant Sci. 14:1174922. doi: 10.3389/fpls.2023.1174922
Received: 27 February 2023; Accepted: 21 July 2023;
Published: 04 September 2023.
Edited by:
Marta Olech, Medical University of Lublin, PolandReviewed by:
Filipa Mandim, Centro de Investigação de Montanha (CIMO), PortugalNajeeb Ur Rehman, University of Nizwa, Oman
Copyright © 2023 Xu, Chen, Luo, Yang and Li. This is an open-access article distributed under the terms of the Creative Commons Attribution License (CC BY). The use, distribution or reproduction in other forums is permitted, provided the original author(s) and the copyright owner(s) are credited and that the original publication in this journal is cited, in accordance with accepted academic practice. No use, distribution or reproduction is permitted which does not comply with these terms.
*Correspondence: Dengwu Li, ZGVuZ3d1bGlAMTYzLmNvbQ==