- 1College of Bioscience and Biotechnology, Shenyang Agricultural University, Shenyang, China
- 2Department of Pharmacy, Taizhou Central Hospital (Taizhou University Hospital), Taizhou, China
Introduction: Solanum rostratum, an annual malignant weed, has seriously damaged the ecological environment and biodiversity of invasion area. This alien plant gains a competitive advantage by producing some new phytotoxic substances to inhibit the growth of native plants, thus achieving successful invasion. However, the chemical structures, inhibitory functions and action mechanisms of phytotoxic substances of S. rostratum remain unclear.
Methods: In this study, to clarify the chemical structures of phytotoxic substances from S. rostratum, we isolated phenylpropanoid amides from the plant. Their structures were identified by comprehensive HR-ESIMS, NMR and ECD data. And the inhibitory functions of isolated phenylpropanoid amides on one model plant (Arabidopsis thaliana) were also investigated. In addition, the action mechanisms of active phenylpropanoid amides were revealed by antioxidant-related enzymes [Catalase (CAT), Peroxidase (POD), Superoxide dismutase (SOD)] activities and corresponding molecular docking analyses.
Results and Discussion: Phytochemical research on the whole plant of S. rostratum led to the isolation and identification of four new phenylpropanoid amides (1−4), together with two known analogues (5−6). All the compounds showed phytotoxic effects with varying levels on the seed germination and root elongation of one model plant (Arabidopsis thaliana), especially compound 2 and 4. Likewise, compounds 2 and 4 displayed potent inhibitory effects on antioxidant-related enzyme (POD). In addition, compounds 2 and 4 formed common conventional hydrogen bonds with residues Ala34 and Ser35 in POD revealed by molecular docking analyses. These findings not only helped to reveal the invasion mechanism of S. rostratum from the perspective of “novel weapons hypothesis”, but also opened up new ways for the exploitation and utilization of S. rostratum.
Introduction
With the acceleration of world economic globalization and international trade liberalization, especially the rapid development of tourism, alien invasive plants have posed a great threat to the economy, ecology, social security and national interests of many countries in the world (Seebens et al., 2017; Bonnamour et al., 2021). In China, some representative invasive plants, such as Ageratina adenophora, Alternanthera philoxeroides, Ambrosia artemisiifolia and Mikania micrantha, caused immeasurable losses every year (Tu et al., 2021). Therefore, preventing the invasion of alien plants, containing their spread and harm, and achieving sustainable control of existing invasive plants have become a major national demand to ensure the coordinated development of social progress and nature. In order to effectively prevent and control the further damage of invasive plants, it is important to deeply understand and reveal the invasion mechanism of alien plants from the research level (Enders et al., 2020). In recent years, the “novel weapons hypothesis” has successfully explained the causes of some alien plant invasion, and has become a research hotspot (Bais, 2003). The hypothesis suggested that alien plants gain a competitive advantage by producing some new phytotoxic substances to inhibit the growth of native plants, thus achieving successful invasion (Callaway and Ridenour, 2004). These phytotoxic substances belong to the secondary metabolites of plants, including small molecules of phenols, organic acids, terpenoids and nitrogenous compounds (Macías et al., 2019). However, the chemical structures, inhibitory functions and action mechanisms of phytotoxic substances of most invasive plants remain unclear. Therefore, fully clarifying the contribution of phytotoxic substances in the process of alien plant invasion is not only of great significance to reveal the invasion mechanism of alien plants, but also to open up new ways for the exploitation and utilization of invasive plants.
Solanum rostratum Duna., also known as Huang Hua Ci Qie and Kan Sa Si Ji, is an annual malignant weed of Solanaceae. It originated from North America and has spread to sixteen countries and regions in Europe, Asia, Africa and Oceania. In China, S. rostratum was first found in Liaoning Province in 1981. Now it has spread to Xinjiang, Jilin, Hebei, Shanxi, Beijing and other places, and the spreading trend is still expanding (Zhong et al., 2009; Liu et al., 2021). The Ministry of Ecology and Environment of China has listed S. rostratum as the fourth batch of invasive quarantine species in 2016. The species reproduces rapidly and competes with native plants for sunlight, water and living space, seriously damaging the ecological environment and biodiversity of invasion area. In addition, the species could produce a neurotoxin (solanine) that has a paralyzing effect on the central nervous system of human or livestock, and has become an extremely dangerous invasive plant (Wei et al., 1985; Wei et al., 2010). For a long time, the invasion mechanism of S. rostratum has been studied in many aspects, including its physiological characteristics (seed propagation, growth and development, pollination) and chemical defense against herbivorous insects (Karen, 1975; Eminniyaz et al., 2013; Liu et al., 2020; Liu et al., 2021; Yu et al., 2021). However, it is neglected to reveal the invasion mechanism of S. rostratum from the perspective of “novel weapons hypothesis”.
Previous studies showed that extracts from different parts of S. rostratum had certain inhibitory effects on seed germination and seedling growth of Amaranthus retroflexus, Poa annua and Solanum lycopersicum (Ping et al., 2012; Shao, 2015). However, these studies only focused on the phytotoxic effects of S. rostratum extracts, and the chemical structures, inhibitory functions and action mechanisms of its phytotoxic substances remain unclear. Like other higher plants, S. rostratum contains abundant secondary metabolites, mainly nitrogenous compounds, such as steroid alkaloids, pyrrole alkaloids, and phenylpropanoid amides (Novruzov et al., 1973; Liu et al., 2020; Liu et al., 2021). Among them, phenylpropanoid amides compounds are biosynthesized from cinnamic acid and phenylethylamine derivatives, which has complex chemical structures and diverse biological activities (Sun et al., 2015; Hao et al., 2022). A large number of studies have shown that phenylpropanoid amides have significant inhibitory effects on plant growth (Vishnoi et al., 2009; Thi et al., 2014; Thi et al., 2017), and we speculated that these compounds may be the potential phytotoxic substances for S. rostratum.
In this study, to clarify the chemical structures of phytotoxic substances from S. rostratum, we isolated and identified phenylpropanoid amides from the plant. And the inhibitory functions of isolated phenylpropanoid amides on one model plant (Arabidopsis thaliana) were also investigated. In addition, the action mechanisms of active phenylpropanoid amides were revealed by antioxidant-related enzymes [Catalase (CAT), Peroxidase (POD), Superoxide dismutase (SOD)] activities and corresponding molecular docking analyses.
Materials and methods
General
Column chromatography was carried out using silica gel (3.0 × 30 cm, Qingdao Marine, China), CHP20P (3.0 × 25 cm, Mitsubishi, Japan), octadecylsilyl silica (ODS) (YMC, Japan) and Sephadex LH-20 (1.5 × 180 cm, GE Healthcare, Sweden). Semi-preparative high performance liquid chromatography (HPLC) was conducted on a 1260 system (Agilent, USA) equipped with an XDB-C18 column (9.4 × 250 mm, 5 μm, Agilent, USA). Ultra violet (UV) spectra were determined using a 241 spectrophotometer (Perkin Elmer, USA). High resolution electrosparay ionization mass spectrum (HR-ESIMS) spectra were recorded on a 6545 Q-TOF instrument (Agilent, USA). Nuclear magnetic resonance (NMR) spectra were acquired using an AV-600 instrument (Bruker, Germany). Electronic circular dichroism (ECD) spectra were obtained using a MOS-450 instrument (Bio-Logic, France). The solvents used in column chromatography were analytical grade (Tianjin Hengxing, China), the solvents used in HPLC, HR-ESIMS, ECD and activity assays were chromatographic grade (Sigma-Aldrich, USA), and the solvent used in NMR was methanol-d4 (Cambridge Isotope Laboratories, USA).
Plant material
The whole plant of S. rostratum was collected from Baicheng, Jilin Province, China (123° 31′ E, 45° 98′ N) in September 2017 and identified by Professor Meini Shao (Shenyang Agricultural University). A voucher specimen (NO.ZW20170906) was deposited in the herbarium of Shenyang Agricultural University. The seeds of A. thaliana (Col-0) were obtained from Professor Xianling Wang (Shenyang Agricultural University) and stored in a 4°C refrigerator.
Extraction and isolation
The air-dried S. rostratum (20 kg) was extracted with 70% ethanol (150 L × 2 times × 10 d, at room temperature). The concentrated extract (800 g) was successively partitioned with petroleum ether (8 L × 5 times) and ethyl acetate (8 L × 5 times). The ethyl acetate fraction (210 g) was subjected to silica gel column chromatography eluted with gradient dichloromethane: methanol (99: 1–50: 50, v/v) to obtain four subfractions (Fr. A–Fr. D). Fr. B (27 g) was subjected to silica gel column chromatography eluted with gradient dichloromethane: methanol (98: 2–50: 50, v/v) to obtain six subfractions (Fr. B-1–Fr. B-6). Fr. B-2 (3.5 g) was subjected to CHP20P column chromatography eluted with gradient methanol: H2O (10: 90–90: 10, v/v) to obtain five subfractions (Fr. B-2-1–Fr. B-2-5). Fr. B-2-2 (800 mg) was subjected to Sephadex LH-20 column chromatography eluted with isocratic methanol to yield four subfractions (Fr. B-2-2-1–Fr. B-2-2-4). Fr. B-2-2-1 (186 mg) was purified using semi-preparative HPLC (210 nm, 3.0 mL/min) eluted with isocratic methanol: H2O (15: 85, v/v) to obtain compounds 1 (3.5 mg, tR = 47.5 min), 2 (8.4 mg, tR = 32.1 min) and 3 (19.8 mg, tR = 68.6 min), respectively, see Figure S29. Similarly, Fr. B-2-2-2 (172 mg) was purified using semi-preparative HPLC (210 nm, 3.0 mL/min) eluted with isocratic methanol: H2O (10: 90, v/v) to obtain compound 4 (4.6 mg, tR = 37.2 min), see Figure S29. Fr. C (34 g) was subjected to silica gel column chromatography eluted with gradient dichloromethane: methanol (90: 10–50: 50, v/v) to obtain six subfractions (Fr. C-1–Fr. C-6). Fr. C-3 (4.1 g) was subjected to ODS column chromatography eluted with gradient methanol: H2O (10: 90–100: 0, v/v) to obtain seven subfractions (Fr. C-3-1–Fr. C-3-7). Fr. C-3-4 (1.2 g) was subjected to silica gel column chromatography eluted with gradient dichloromethane: methanol (90: 10–50: 50, v/v) to obtain seven subfractions (Fr. C-3-4-1–Fr. C-3-4-7). Fr. C-3-4-7 (67 mg) was purified using semi-preparative HPLC (210 nm, 5.0 mL/min) eluted with isocratic methanol: H2O (15: 85, v/v) to obtain compounds 5 (3.2 mg, tR = 28.5 min) and 6 (2.2 mg, tR = 21.4 min), respectively, see Figure S29. All the obtained compounds (1−6) were dried by a drying incubator and stored in a 4°C refrigerator.
Phenylpropylcyclamide A (1): yellowish brown oil; ECD (methanol) λmax (△ε) 217 (-11.96), 227 (+13.90), 301 (-21.65) nm; HR-ESIMS at m/z 304.0947 [M+Na]+ (calcd for C17H15NO3Na, 304.0950); 1H and 13C NMR data, see Table 1.
Phenylpropylcyclamide B (2): yellowish brown oil; HR-ESIMS at m/z 316.0953 [M+Na]+ (calcd for C18H15NO3Na, 316.0950); 1H and 13C NMR data, see Table 1.
Phenylpropylcyclamide C (3): yellowish brown oil; HR-ESIMS at m/z 330.1109 [M+Na]+ (calcd for C19H17NO3Na, 330.1106); 1H and 13C NMR data, see Table 1.
Phenylpropylcyclamide D (4): yellowish brown oil; HR-ESIMS at m/z 332.0873 [M+Na]+ (calcd for C18H15NO4Na, 332.0899); 1H and 13C NMR data, see Table 1.
N-trans-p-coumaroyl-3′,4′-dihydroxyphenylethylamine (5): yellowish amorphous powder; 1H and 13C NMR data, see Figures S25, S26.
trans-N-coumaroyltyramine (6): yellowish amorphous powder; 1H and 13C NMR data, see Figures S27, S28.
ECD calculations
The ECD calculations were performed as a previously described method with little modifications (Liu et al., 2021). Firstly, the conformational searches were carried out using Spartan 14.0 program under MMFF94 force field. Then, the possible conformations were optimized using Gaussian 09 program at the B3LYP/6-31G (d) level. Subsequently, the theoretical calculations of ECD were calculated using time-dependent density functional theory (TDDFT) at the B3LYP/6-311 + G (2d, p) level in methanol. Finally, the ECD curves were obtained using SpecDis 1.60 program based on the Boltzmann weighting of each conformation.
Seed germination bioassay
The seed germination assay was performed as a previously described method with little modifications (Liu et al., 2023). Firstly, A. thaliana seeds were sterilized with ethanol: H2O (75: 25, v/v) and rinsed with distilled water (500 mL × 3 times × 2 min), respectively. The sterilized seeds were stored in a 4°C refrigerator for 3 d before use. Then, the measured compounds were dissolved with methanol and added to different volumes of 1/2 MS medium, respectively, to obtain the medium containing different concentrations of compounds (100 µg/mL, 50 µg/mL, and 25 µg/mL). The medium with the same volume of methanol was used as blank control. A sulfonylurea herbicide (Triasulfuron) (100 µg/mL, 50 µg/mL, and 25 µg/mL) was used as positive control. Subsequently, the 3.0 mL of medium was transferred to each of the 6 cm petri dishes, respectively. After the medium was naturally cooled to a solid state, thirty sterilized seeds were evenly arranged and cultured in an illumination incubator (day 25°C 16 h, night 20°C 8 h, light intensity 100 µM m-2 s-1). Finally, the number of germinated seeds were checked when the most of blank control seeds (≥ 90%) had germinated (three replicates each concentration). Inhibitory rate (%) was calculated as (Sc-ST)/Sc × 100 (Sc, the number of germinated seeds of blank control; ST, the number of germinated seeds of treatment).
Root elongation assay
The root elongation assay was performed as a previously described method with little modifications (Liu et al., 2022). Firstly, A. thaliana seeds and the medium containing different concentrations of compounds were pretreated as described above. Then, the 1.5 mL of medium was transferred to each of the 6-well plates, respectively. Subsequently, after the medium was naturally cooled to a solid state, ten sterilized seeds were evenly arranged in a row and cultured vertically in an illumination incubator (day 25°C 16 h, night 20°C 8 h, light intensity 100 µM m-2 s-1). Finally, the roots lengths were measured when the roots of blank control reached the bottom of the 6-well plate (three replicates each concentration). Inhibitory rate (%) was calculated as (Rc-RT)/Rc × 100 (Rc, the average lengths of blank control; RT, the average lengths of treatment).
Antioxidant-related enzymes assay
The extraction of enzymes was performed as a previously described method with little modifications (Chen et al., 2009). Firstly, A. thaliana seeds and the medium containing 100 μg/mL of compounds 2 and 4 were pretreated as described above. Then, the 1.5 mL of medium was transferred to each of the 6-well plates, respectively. After the medium was naturally cooled to a solid state, ten sterilized seeds were evenly arranged in a row and cultured vertically in an illumination incubator (day 25°C 16 h, night 20°C 8 h, light intensity 100 µM m-2 s-1). Subsequently, the roots lengths were measured when the roots of blank control reached the bottom of the 6-well plate. After measuring root length, we weighed 0.2 g of A. thaliana for antioxidant-related enzymes assay. Finally, the treated A. thaliana (0.2 g) were homogenized with 5.0 mL phosphate buffer saline (PBS) (0.05 M, pH = 7.0) in cold mortar. The mixture was centrifuged at 12,000 rpm for 10 min at 4°C, and the supernatant was used for the antioxidant-related enzymes assay. The same volume of PBS was used as blank control.
The CAT assay was performed as ultraviolet absorption method with little modifications (He et al., 2009). The 0.1 mL enzyme solution was mixed with 1.0 mL H2O2 (0.2 mM, pH = 7.0) and 3.9 mL PBS (0.05 M, pH = 7.0). The absorbance of mixture was measured at 240 nm. One unit of CAT activity was defined as a decrease in absorbance of 0.1 per milligram of protein per minute. The POD assay was performed as guaiacol method with little modifications (Chen et al., 2009). The 0.1 mL enzyme solution was mixed with 1.0 mL H2O2 (0.2 mM, pH = 7.0), 2.9 mL PBS (0.05 M, pH = 7.0), and 1.0 mL guaiacol (0.05 M). The absorbance of mixture was measured at 470 nm. One unit of POD activity was defined as an increase in absorbance of 0.1 per milligram of protein per minute. The SOD assay was performed as nitro blue tetrazolium (NBT) method with little modifications (Chen et al., 2009). The 0.1 mL enzyme solution was mixed with 2.9 mL PBS (0.05 M, pH = 7.0) containing 2.0 µM riboflavin, 75.0 µM NBT, 10.0 µM ethylenediaminetetraacetic acid, and 13.0 µM methionine. The mixture was irradiated by fluorescent lamp (4000 lx) for 20 min. The absorbance was measured at 560 nm. One unit of SOD activity was defined as the amount of enzyme required to inhibit the reduction of NBT by 50% per milligram of protein. The above experiments were repeated three times.
Molecular docking analyses
Firstly, the structure of receptor (POD) was obtained from RCSB PDB (https://www.rcsb.org/) with code: 1PA2 (Ostergaard et al., 2000) and further optimized by SYBYL-X 2.0 program. Then, the structures of ligands (compouds 2 and 4) were optimized by the ChemDraw-3D 14.0 program. Subsequently, an active pocket centered on x (1.42), y (35.11), z (34.72) was created based on the binding sites of the original ligand and protein. Finally, the Molegro Virtual Docker 4.0 program was performed for the docking studies.
Statistical analyses
All data were presented as means ± SD of three independent experiments. Statistical comparisons between group means were performed using One-way ANOVA. A p value < 0.05 was considered statistically significant.
Results and discussion
Phenylpropylcyclamide A (1) was isolated as yellowish brown oil with C17H15NO3 (eleven degrees of unsaturation) according to HR-ESIMS data (m/z 304.0947 [M+Na]+, calcd for C17H15NO3Na, 304.0950). Its 1D-NMR spectra (Table 1) showed one carbonyl group [δC 175.3 (C-2)], one double bond group [δH 7.38 (1H, s, H-6); δC 134.2 (C-6), 130.6 (C-3)], two para-oxy-substituted phenyl groups [δH 7.19 (2H, d, J = 8.6 Hz, H-2′, H-6′), 7.05 (2H, d, J = 8.5 Hz, H-2′′, H-6′′), 6.70 (2H, d, J = 8.5 Hz, H-3′′, H-5′′, 6.61 (2H, d, J = 8.6 Hz, H-3′, H-5′); δC 161.5 (C-4′), 157.5 (C-4′′), 135.1 (C-1′′), 133.3 (C-2′, C-6′), 129.0 (C-2′′, C-6′′), 126.4 (C-1′), 116.9 (C-3′, C-5′), 116.7 (C-3′′, C-5′′)], respectively. Comparison of its 1D NMR spectra with a known compound Tamaractam showed that they were very similar except for two missing methoxy groups (Yao et al., 2017), which indicated that 1 possessed a phenylpropanoid amides skeleton. In the heteronuclear multiple bond correlation (HMBC) spectrum (Figure 1), the key correlations of H-4/C-2, C-5, C-6′′; H-6/C-2, C-4, C-2′; H-5′/C-1′, C-3′, C-4′; H-5′′/C-1′′, C-3′′, C-4′′ established the planar structure of compound 1. In the nuclear overhauser and exchange spectroscopy (NOESY) spectrum (Figure 2), the key correlation of H-4/H-2′ and the lack of correlation of H-4/H-6 indicated that the configuration of double bond between C-3 and C-6 was E. Furthermore, the computed ECD curve of (4S)-1a matched well with the experimental result (Figure 3), which established the stereoscopic structure of compound 1. Thus, the structure of 1 was identified and named Phenylpropylcyclamide A (Figure 4).
Phenylpropylcyclamide B (2) was isolated as yellowish brown oil with C18H15NO3 (twelve degrees of unsaturation) according to HR-ESIMS data (m/z 316.0953 [M+Na]+, calcd for C18H15NO3Na, 316.0950). Comparison of its 1D NMR spectra with compound 1 showed that they were very similar except for one additional methyl group [δH 2.26 (3H, s, 5-CH3); δC 14.8 (5-CH3)] and one additional double bond group [δC 122.6 (C-5), 115.1 (C-4)], which indicated that 2 also possessed a phenylpropanoid amides skeleton. In the HMBC spectrum (Figure 1), the key correlations of H-5-CH3/C-4, C-5; H-6/C-2, C-4 revealed that the methyl group was connected to Phenylpropylcyclamide A by C-5 and the double bond group formed between C-4 and C-5. In the NOESY spectrum (Figure 2), the lack of correlation of H-6/H-2′′ indicated that the configuration of double bond between C-3 and C-6 was E. Thus, the structure of 2 was identified and named Phenylpropylcyclamide B (Figure 4).
Phenylpropylcyclamide C (3) was isolated as yellowish brown oil with C19H17NO3 (twelve degrees of unsaturation) according to HR-ESIMS data (m/z 330.1109 [M+Na]+, calcd for C19H17NO3Na, 330.1106). Comparison of its 1D NMR spectra with compound 2 showed that they were very similar except for one additional methoxy group [δH 3.82 (3H, s, 4′-OCH3); δC 55.7 (4′-OCH3)], which indicated that 3 also possessed a phenylpropanoid amides skeleton. In the HMBC spectrum (Figure 1), the key correlations of H-4′-OCH3/C-4′, C-5′ revealed that the methoxy group was connected to Phenylpropylcyclamide B by C-4′. In the NOESY spectrum (Figure 2), the lack of correlation of H-6/H-2′′ indicated that the configuration of double bond between C-3 and C-6 was E. Thus, the structure of 3 was identified and named Phenylpropylcyclamide C (Figure 4).
Phenylpropylcyclamide D (4) was isolated as yellowish brown oil with C18H15NO4 (twelve degrees of unsaturation) according to HR-ESIMS data (m/z 332.0873 [M+Na]+, calcd for C18H15NO4Na, 332.0899). Comparison of its 1D NMR spectra with compound 2 showed that they were very similar except for one additional 3, 4-dioxy-substituted phenyl group [δH 7.02 (1H, d, J = 2.1 Hz, H-2′), 6.93 (1H, dd, J = 8.6, 2.1 Hz, H-6′), 6.75 (1H, d, J = 8.6 Hz, H-5′); δC 148.5 (C-4′), 145.3 (C-3′), 127.6 (C-1′), 123.7 (C-6′), 115.3 (C-5′), 114.5 (C-2′)] and one missing para-oxy-substituted phenyl group, which indicated that 4 also possessed a phenylpropanoid amides skeleton. In the HMBC spectrum (Figure 1), the key correlations of H-6/C-2, C-4, C-2′, C-6′ revealed that the 3, 4-dioxy-substituted phenyl group was connected to Phenylpropylcyclamide B by C-6. In the NOESY spectrum (Figure 2), the lack of correlation of H-6/H-2′′ indicated that the configuration of double bond between C-3 and C-6 was E. Thus, the structure of 4 was identified and named Phenylpropylcyclamide D (Figure 4).
The two other compounds 5 and 6 were known and identified as N-trans-p-coumaroyl-3′,4′-dihydroxyphenylethylamine and trans-N-coumaroyltyramine, respectively, based on the NMR data and literature comparison (Liu et al., 2020).
A large number of studies have shown that phenylpropanoid amides have significant inhibitory effects on plant growth (Vishnoi et al., 2009; Thi et al., 2014; Thi et al., 2017), and we speculated that these compounds may be the potential phytotoxic substances for S. rostratum. In addition, A. thaliana is an important model plant for evaluating phytotoxic effects, which has been reported in many literatures (Liu et al., 2013; Chen et al., 2016; Li et al., 2019). Therefore, phenylpropanoid amides (1−6) were then assessed for their phytotoxic effects on the seed germination and root elongation of A. thaliana. As shown in Figure 5, these compounds showed phytotoxic effects with varying levels. Compounds 1, 3, 5 and 6 exhibited weak to moderate phytotoxic effects at 100 μg/mL with seed germination and root elongation inhibitory rates ranged from 21.37 to 40.05% and 23.56 to 43.31%, respectively. Among them, compounds 5 and 6 had slight promotion effects on the seed germination and root elongation of A. thaliana at 25 μg/mL. On the whole, compounds 2 and 4 exhibited stronger phytotoxic effects compared with other compounds. It is notable that compound 4 exhibited potent phytotoxic effects at 100 μg/mL (78.69% on seed germination and 75.56% on root elongation), which even comparable to Triasulfuron (75.32% on seed germination and 82.98% on root elongation).
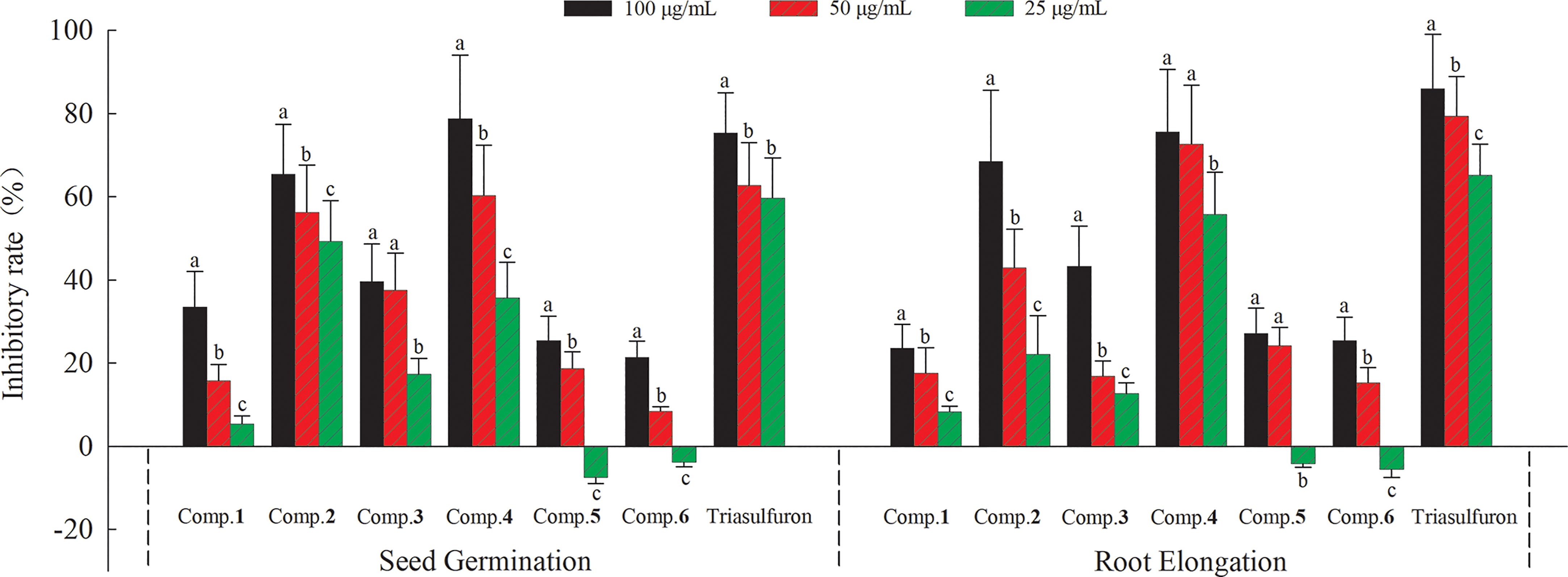
Figure 5 Phytotoxic effects of compounds 1−6 on the seed germination and root elongation of A. thaliana. Different letters indicate significant differences between concentration treatments (p < 0.05).
Plants could produce reactive oxygen species (ROS) when subjected to biological or abiotic stress. The accumulation of ROS could induce membrane injury and lipid peroxidation to damage cell structure, thus inhibiting plant growth. Antioxidant-related enzymes (CAT, POD and SOD) could effectively prevent the accumulation of high concentration ROS to reduce plant damage (Arora et al., 2002). Therefore, the action mechanisms of active phenylpropanoid amides (2 and 4) were revealed by antioxidant-related enzymes activities. The effects of compounds 2 and 4 on CAT, POD and SOD activities of A. thaliana at different time were shown in Figure 6. As a whole, the CAT and POD activities of compounds (2 and 4) and blank control treatment decreased with the extension of time, and the SOD activities of compounds (2 and 4) and blank control treatment increased with the extension of time. It is notable that POD activities decreased significantly by 40.63%, 45.23% and 68.40% under compound 2 treatment, and 34.27%, 58.04% and 76.68% under compound 4 treatment compared with the blank control at 12, 24 and 48 h, respectively, indicating that compounds 2 and 4 could inhibit plant growth probably by inhibiting POD enzyme effectively. It is appeared that POD may be a key target for compounds 2 and 4 to exert their phytotoxic effects.
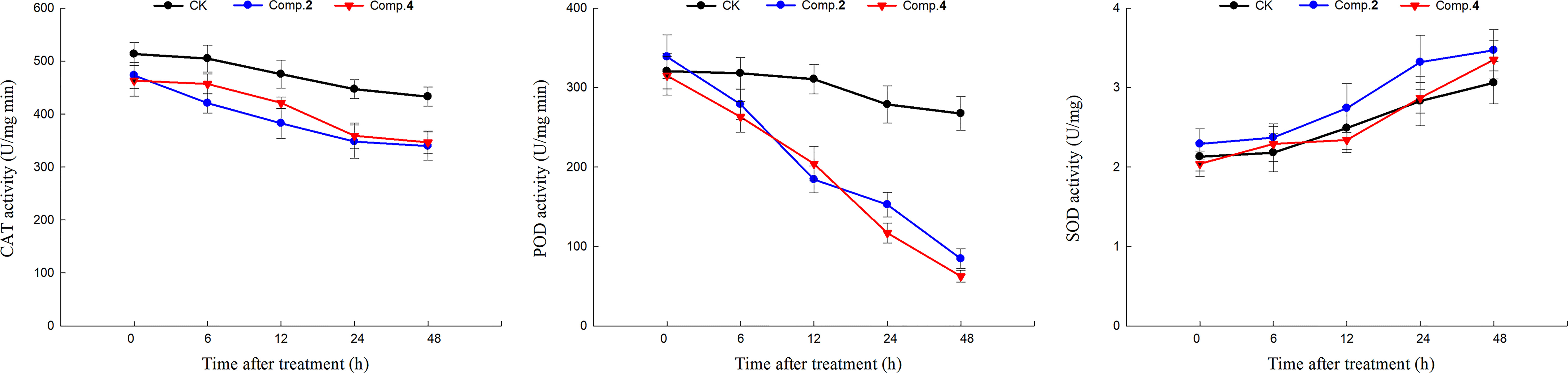
Figure 6 The effects of compounds 2 and 4 (100 μg/mL) on antioxidant-related enzymes (CAT, POD, SOD) activities of A. thaliana.
It is well known that molecular docking provides visual analyses to explore the binding sites and modes of action of protein and ligand (Ferreira et al., 2015). In addition, molecular docking is generally meaningful only when combined with actual enzyme activity experiments. However, after testing the activities, there were no enough compounds 1, 5 and 6 to measure antioxidant-related enzymes assay. Compounds 2 and 4 were finally selected for molecular docking because of their significant effects on seed germination, root elongation and POD enzyme activities of Arabidopsis thaliana. As shown in Table S1, compound 4 (MolDock scores of −135.462 kcal/mol) had a lower binding energy with POD than compound 2 (MolDock scores of −128.591 kcal/mol), indicating that compound 4 binds to POD more stable than compound 2. It was also in good agreement with the phytotoxic activities and POD enzyme assay of compounds 2 and 4. In addition, phenolic hydroxyl groups and phenyl groups of compounds bound to neighboring amino acid residues though conventional hydrogen bonds and Pi-Alkyl interactions, respectively, indicating that the phenol structural fragment of the compounds 2 and 4 played a crucial role in their POD activities (Figure 7). On the other hand, studies have shown that conventional hydrogen bonds are more important than other interactions (Panigrahi and Desiraju, 2007). Compound 2 formed two conventional hydrogen bonds with residues Ala34 (distance 2.22 Å) and Ser35 (distance 1.48 Å) in POD. Meanwhile, compound 4 formed three conventional hydrogen bonds with residues Ala168 (distance 2.09 Å), Ala34 (distance 2.46 Å) and Ser35 (distance 1.63 Å) in POD (Figure 7). The above not only proved that more hydrogen bonds lead to more stable bonding of protein and ligand, but also suggested that the common residues Ala34 and Ser35 may be key active sites interacting with 2 and 4.
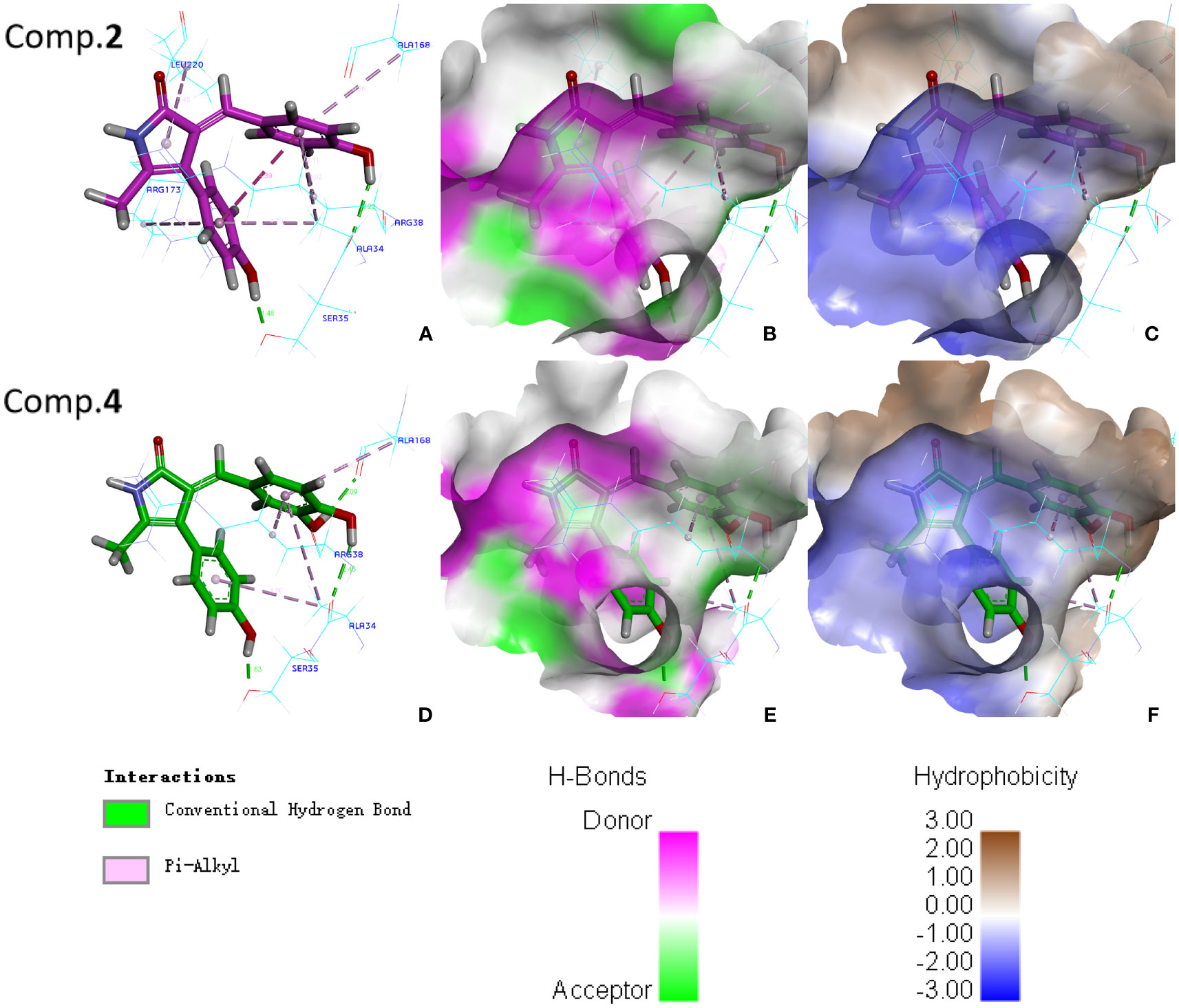
Figure 7 Molecular docking analyses of compounds 2 and 4 with POD: (A) Interactions between compound 2 and amino acid residues of POD; (B) H-bonds between compound 2 and active pocket of POD; (C) Hydrophobic property between compound 2 and active pocket of POD; (D) Interactions between compound 4 and amino acid residues of POD; (E) H-bonds between compound 4 and active pocket of POD; (F) Hydrophobic property between compound 4 and active pocket of POD.
In recent years, alien invasive plants have posed a great threat to the economy, ecology, social security and national interests of many countries in the world (Seebens et al., 2017; Bonnamour et al., 2021). Studies have shown that the “novel weapons hypothesis” could successfully explain the causes of some alien plant invasion (Bais, 2003). The hypothesis suggested that alien plants gain a competitive advantage by producing some new phytotoxic substances that negatively affect on the growth of native plants (Callaway and Ridenour, 2004). Phenylpropanoid amides are the potential phytotoxic substances, which have significant inhibitory effects on plant growth (Vishnoi et al., 2009; Thi et al., 2014; Thi et al., 2017). In our study, four new phenylpropanoid amides (1−4), together with two known analogues (5−6) were isolated and identified from S. rostratum. Among them, compounds 2 and 4 showed potent phytotoxic effects on the seed germination and root elongation of A. thaliana. These two active compounds could inhibit plant growth probably by inhibiting POD enzyme effectively, thereby indirectly improving the competitive advantage of S. rostratum. We speculated that due to the lack of co-evolution between native and alien plants, antioxidant enzymes of A. thaliana have not adapted to the new compounds (2 and 4) from S. rostratum. However, further research of more native companion plants (non-model) is needed to confirm this. From another perspective, compounds 2 and 4 are expected to be the lead active substances with potential application of plant-derived herbicides. It will realize the transformation of S. rostratum waste into treasure, and also provide ideas for the exploitation and utilization of other invasive plants.
Conclusion
In summary, four new phenylpropanoid amides (1−4), together with two known analogues (5−6) were isolated from the whole plant of S. rostratum and their structures were identified by HR-ESIMS, NMR and ECD data. All the compounds showed phytotoxic effects with varying levels on one model plant (A. thaliana), especially compound 2 and 4, which exhibited potent phytotoxic effects on the seed germination and root elongation at 100 μg/mL. Likewise, compounds 2 and 4 displayed potent inhibitory effects on antioxidant-related enzyme (POD). In addition, compounds 2 and 4 formed common conventional hydrogen bonds with residues Ala34 and Ser35 in POD revealed by molecular docking analyses. Our findings not only helped to reveal the invasion mechanism of S. rostratum from the perspective of “novel weapons hypothesis”, but also opened up new ways for the exploitation and utilization of S. rostratum.
Data availability statement
The original contributions presented in the study are included in the article/Supplementary Material, further inquiries can be directed to the corresponding author/s.
Author contributions
ZXL designed the research and wrote the manuscript. XQM, NZ, LLY, HRY and LLZ performed the experiments and analyzed the data. TA and YBX reviewed and revised the draft. All authors contributed to the article and approved the submitted version.
Funding
This work was supported by the National Natural Science Foundation of China (32271741).
Acknowledgments
We thank Shenyang Pharmaceutical University for the ECD, NMR and molecular docking analyses.
Conflict of interest
The authors declare that the research was conducted in the absence of any commercial or financial relationships that could be construed as a potential conflict of interest.
Publisher’s note
All claims expressed in this article are solely those of the authors and do not necessarily represent those of their affiliated organizations, or those of the publisher, the editors and the reviewers. Any product that may be evaluated in this article, or claim that may be made by its manufacturer, is not guaranteed or endorsed by the publisher.
Supplementary material
The Supplementary Material for this article can be found online at: https://www.frontiersin.org/articles/10.3389/fpls.2023.1174844/full#supplementary-material
References
Arora, A., Sairam, R. K., Srivastava, G. C. (2002). Oxidative stress and antioxidative system in plants. Curr. Sci. 82, 1227–1238. doi: 10.1073/pnas.112147699
Bais, H. P. (2003). Allelopathy and exotic plant invasion: From molecules and genes to species interactions. Science 327, 1377–1380. doi: 10.1126/science.1083245
Bonnamour, A., Gippet, J. M. W., Bertelsmeier, C., Gurevitch, J. (2021). Insect and plant invasions follow two waves of globalisation. Ecol. Lett. 24, 2418–2426. doi: 10.1111/ele.13863
Callaway, R. M., Ridenour, W. M. (2004). Novel weapons: invasive success and the evolution of increased competitive ability. Front. Ecol. Environ. 2, 436–443. doi: 10.1890/1540-9295(2004)002[0436:nwisat]2.0.co;2
Chen, X. T., Lin, J., Shao, X. F., Ou, X. M., Wang, Z. H., Lu, G. D. (2009). The physiological and molecular responses of Arabidopsis thaliana to the stress of oxalic acid. Agr. Sci. China 8, 828–834. doi: 10.1016/S1671-2927(08)60284-9
Chen, J., Zheng, G. W., Zhang, Y., Aisa, H. A., Hao, X. J. (2016). Phytotoxic terpenoids from Ligularia cymbulifera roots. Front. Plant Sci. 7. doi: 10.3389/fpls.2016.02033
Eminniyaz, A., Qiu, J., Tan, D. Y., Baskin, C. C., Baskin, J. M., Nowak, R. S. (2013). Dispersal mechanisms of the invasive alien plant species buffalobur (Solanum rostratum) in cold desert sites of northwest China. Weed Sci. 61, 557–563. doi: 10.1614/WS-D-13-00011.1
Enders, M., Havemann, F., Ruland, F., Bernard-Verdier, M., Catford, J. A., Gómez-Aparicio, L., et al. (2020). A conceptual map of invasion biology: Integrating hypotheses into a consensus network. Global Ecol. Biogeogr. 29, 978–991. doi: 10.1111/geb.13082
Ferreira, L. G., Dos Santos, R. N., Oliva, G., Andricopulo, A. D. (2015). Molecular docking and structure-based drug design strategies (Review). Molecules 20, 13384–13421. doi: 10.3390/molecules200713384
Hao, J. F., Li, H. Y., Zhang, N., Ma, X. Q., Yuan, L. L., Zhang, T., et al. (2022). Phenylpropanoid amides from physalis pubescens and their chemotaxonomic significance. Biochem. Syst. Ecol. 100, 104361. doi: 10.1016/j.bse.2021.104361
He, H. B., Wang, H. B., Fang, C. X., Lin, Y. Y., Zeng, C. M., Wu, L. Z. (2009). Herbicidal effect of a combination of oxygenic terpenoids on Echinochloa crus-galli. Weed Res. 49, 183–192. doi: 10.1111/j.1365-3180.2008.00675.x
Karen, A. W. B. (1975). The pollination ecology of Solanum rostratum (Solanaceae). Am. J. Bot. 62, 633–638. doi: 10.1002/j.1537-2197.1975.tb14094.x
Li, Y. L., Zhu, R. X., Li, G., Wang, N. N., Liu, C. Y., Zhao, Z. T., et al. (2019). Secondary metabolites from the endolichenic fungus Ophiosphaerella korrae. RSC Adv. 9, 4140–4149. doi: 10.1039/c8ra10329a
Liu, C., Tian, J. L., An, T., Lyu, F. N., Jia, P. F., Zhou, M. J., et al. (2020). Secondary metabolites from Solanum rostratum and their antifeedant defense mechanisms against Helicoverpa armigera. J. Agric. Food Chem. 68, 88–96. doi: 10.1021/acs.jafc.9b06768
Liu, Z. X., Wang, M. Q., Tian, M. X., Yuan, L. L., Yu, B. M., Qu, B., et al. (2021). Pyrrole alkaloids from Solanum rostratum and their chemical defense function against Henosepilachna vigintioctomaculata. Fitoterapia 155, 105031. doi: 10.1016/j.fitote.2021.105031
Liu, Q., Xu, R., Yan, Z. Q., Jin, H., Cui, H. Y., Lu, L. Q., et al. (2013). Phytotoxic allelochemicals from roots and root exudates of Trifolium pratense. J. Agric. Food Chem. 61, 6321–6327. doi: 10.1021/jf401241e
Liu, Z. X., Zhang, N., Ma, X. Q., Zhang, T., Li, X., Tian, G., et al. (2022). Sesquiterpenes from Ambrosia artemisiifolia and their allelopathy. Front. Plant Sci. 13. doi: 10.3389/fpls.2022.996498
Liu, Y., Zhou, Y. Y., Luo, S. H., Guo, K., Zhang, M. W., Jing, S. X., et al. (2023). Labdane diterpenoids from the heartwood of Leucosceptrum canum that impact on root growth and seed germination of Arabidopsis thaliana. Phytochemistry 206, 113531. doi: 10.1016/j.phytochem.2022.113531
Macías, F. A., Mejías, F. J. R., Molinillo, J. M. G. (2019). Recent advances in allelopathy for weed control: from knowledge to applications. Pest Manage. Sci. 75, 2413–2436. doi: 10.1002/ps.5355
Novruzov, E. N., Aslanov, S. M., Ismailov, N. M. (1973). Glycosidic alkaloids of Solanum rostratum. Chem. Nat. Compd. 9, 659. doi: 10.1007/bf00564416
Ostergaard, L., Teilum, K., Mirza, O., Mattsson, O., Petersen, M., Welinder, K. G., et al. (2000). Arabidopsis ATP A2 peroxidase. expression and high-resolution structure of a plant peroxidase with implications for lignification. Plant Mol. Biol. 44, 231–243. doi: 10.1023/a:1006442618860
Panigrahi, S. K., Desiraju, G. R. (2007). Strong and weak hydrogen bonds in the protein-ligand interface. Proteins 67, 128–141. doi: 10.1002/prot.21253
Ping, Y. F., Zhu, J. W., Zhang, Z. G. (2012). Effects of Solanum rostratum on seed germination and seedling growth of tomato. Agric. Res. Arid Area. 30, 176–179.
Seebens, H., Blackburn, T. M., Dyer, E. E., Genovesi, P., Hulme, P. E., Jeschke, J. M., et al. (2017). No saturation in the accumulation of alien species worldwide. nat. Commun. 8, 14435. doi: 10.1038/ncomms14435
Sun, J., Song, Y. L., Zhang, J., Huang, Z., Huo, H. X., Zheng, J., et al. (2015). Characterization and quantitative analysis of phenylpropanoid amides in eggplant (Solanum melongena l.) by high performance liquid chromatography coupled with diode array detection and hybrid ion trap time-of-flight mass spectrometry. J. Agric. Food Chem. 63, 3426–3436. doi: 10.1021/acs.jafc.5b00023
Thi, H. L., Lin, C. H., Smeda, R. J., Leigh, N. D., Wycoff, W. G., Fritschi, F. B. (2014). Isolation and identification of an allelopathic phenylethylamine in rice. Phytochemistry 108, 109–121. doi: 10.1016/j.phytochem.2014.08.019
Thi, H. L., Zhou, H. Y., Lin, C. H., Liu, S. J., Berezin, M. Y., Smeda, R., et al. (2017). Synthesis and plant growth inhibitory activity of N-trans-cinnamoyltyramine: its possible inhibition mechanisms and biosynthesis pathway. J. Plant Interact. 12, 51–57. doi: 10.1080/17429145.2016.1278050
Tu, W. Q., Xiong, Q. L., Qiu, X. P., Zhang, Y. M. (2021). Dynamics of invasive alien plant species in China under climate change scenarios. Ecol. Indic. 129, 107919. doi: 10.1016/j.ecolind.2021.107919
Vishnoi, S., Agrawal, V., Kasana, V. K. (2009). Synthesis and structure-activity relationships of substituted cinnamic acids and amide analogues: a new class of herbicides. J. Agric. Food Chem. 57, 3261–3265. doi: 10.1021/jf8034385
Wei, S. H., Zhang, C. X., Chen, X. Z., Li, X. J., Sui, B. F., Huang, H. J., et al. (2010). Rapid and effective methods for breaking seed dormancy in buffalobur (Solanum rostratum). Weed Sci. 58, 141–146. doi: 10.1614/WS-D-09-00005.1
Yao, Y., Jiang, C. S., Sun, N., Li, W. Q., Niu, Y., Han, H. Q., et al. (2017). Tamaractam, a new bioactive lactam from tamarix ramosissima, induces apoptosis in rheumatoid arthritis fibroblast-like synoviocyte. Molecules 22, 96. doi: 10.3390/molecules22010096
Yu, H. L., Zhao, X. Y., Huang, W. D., Zhan, J., He, Y. Z. (2021). Drought stress influences the growth and physiological characteristics of Solanum rostratum dunal seedlings from different geographical populations in China. Front. Plant Sci. 12. doi: 10.3389/fpls.2021.733268
Keywords: invasive plant, isolate, phenylpropanoid amides, phytotoxic activities, Solanum rostratum, Arabidopsis thaliana
Citation: Liu Z, Ma X, Zhang N, Yuan L, Yin H, Zhang L, An T and Xu Y (2023) Phenylpropanoid amides from Solanum rostratum and their phytotoxic activities against Arabidopsis thaliana. Front. Plant Sci. 14:1174844. doi: 10.3389/fpls.2023.1174844
Received: 27 February 2023; Accepted: 27 March 2023;
Published: 12 April 2023.
Edited by:
Maciej Strzemski, Medical University of Lublin, PolandReviewed by:
Anna Rysiak, Marie Curie-Sklodowska University, PolandWeronika Kozlowska, Wroclaw Medical University, Poland
Jan Sawicki, Medical University of Lublin, Poland
Copyright © 2023 Liu, Ma, Zhang, Yuan, Yin, Zhang, An and Xu. This is an open-access article distributed under the terms of the Creative Commons Attribution License (CC BY). The use, distribution or reproduction in other forums is permitted, provided the original author(s) and the copyright owner(s) are credited and that the original publication in this journal is cited, in accordance with accepted academic practice. No use, distribution or reproduction is permitted which does not comply with these terms.
*Correspondence: Tong An, m18602463916@163.com; Yubin Xu, xuyubin1988@126.com