- 1Shandong Key Laboratory of Eco-Environmental Science for Yellow River Delta, Binzhou University, Binzhou, China
- 2College of Forestry, Shandong Agricultural University, Taian, China
Introduction: Human activities have increased the nitrogen (N) and phosphorus (P) supply ratio of the natural ecosystem, which affects the growth of plants and the circulation of soil nutrients. However, the effect of the N and P supply ratio and the effect of plant on the soil microbial community are still unclear.
Methods: In this study, 16s rRNA sequencing was used to characterize the response of bacterial communities in Phragmites communis (P.communis) rhizosphere and non-rhizosphere soil to N and P addition ratio.
Results: The results showed that the a-diversity of the P.communis rhizosphere soil bacterial community increased with increasing N and P addition ratio, which was caused by the increased salt and microbially available C content by the N and P ratio. N and P addition ratio decreased the pH of non-rhizosphere soil, which consequently decreased the a-diversity of the bacterial community. With increasing N and P addition ratio, the relative abundance of Proteobacteria and Bacteroidetes increased, while that of Actinobacteria and Acidobacteria decreased, which reflected the trophic strategy of the bacterial community. The bacterial community composition of the non-rhizosphere soil was significantly affected by salt, pH and total carbon (TC) content. Salt limited the relative abundance of Actinobacteria, and increased the relative abundance of Bacteroidetes. The symbiotic network of the rhizosphere soil bacterial community had lower robustness. This is attributed to the greater selective effect of plants on the bacterial community influenced by nutrient addition.
Discussion: Plants played a regulatory role in the process of N and P addition affecting the bacterial community, and nutrient uptake by the root system reduced the negative impact of N and P addition on the bacterial community. The variations in the rhizosphere soil bacterial community were mainly caused by the response of the plant to the N and P addition ratio.
1 Introduction
Atmospheric N deposition has continuously increased caused by human activities and fossil fuel burning. The soil N content in Chinese natural ecosystems increased by 8 Kg N ha-1yr-1 from 1980 to 2000 (Liu et al., 2013). According to the current trend, N deposition will increase to double the current level (Peñuelas et al., 2012; Lu et al., 2021). P is mainly from the rock, which is different from N mainly stored in the atmosphere (Dong et al., 2019). In addition, ecosystems obtain P mainly through the decomposition and mineralization of soil organic matter (SOM) by soil extracellular enzymes (Bell et al., 2014; Dong et al., 2019). The lagged and low input of P compared to multiple pathways of N input (e.g., atmospheric N deposition, microbial mineralization) has resulted in a gradual imbalance of the N and P input ratio (Deng et al., 2017; Dong et al., 2019). N and P input must be coordinated and synergistic because organisms need a relatively constant ratio of elements to metabolize (Elser et al., 2007). Imbalance of N and P input may cause soil degradation (Zhu et al., 2016), which may affect the regular function of the bacterial community and thus cause structural changes and dysfunction of the ecosystem (Cusack et al., 2011; Tian and Niu, 2015).
Microorganisms are the most diverse and abundant organisms on the planet and are essential to the nutrient cycle (Bennett et al., 2017). Extensive reports have simulated the effects of N deposition on soil microbial communities through N addition experiments, but the conclusions are controversial. On the one hand, the increase in soil N content due to N addition promotes the growth of the bacterial community and increases the diversity of the bacterial community (Fierer et al., 2012; Redding et al., 2016). On the other hand, soil acidification and low pH caused by N addition makes the bacterial community in an unfavorable environment for growth (Sha et al., 2021). Compared to N addition, there are relatively few P addition studies. P addition had a significant effect on the α-diversity and composition of the soil bacterial community (Ma et al., 2021). However, it has also been found that the effect of P input on the bacterial community is negligible (Widdig et al., 2020; Ran et al., 2021). These works have focused on the effect of single nutrient input on the soil bacterial community. Wang et al. (2018b) found that N and P input played different roles in influencing soil bacterial richness and community. The negative effects of N and P imbalance have been observed in the study of plant community structure and diversity (Liu et al., 2019). Li et al. (2022a) found that N and P imbalance input changed microbial biomass. However, the effect of the N and P addition ratio on α-diversity and structure of the bacterial community is poorly understood. The N addition can lead to the P limitation (Zhu et al., 2016; Mao et al., 2017). As imbalances continue to tilt in the same direction, the effects of imbalances will continue to increase (Penuelas and Sardans, 2022). Therefore, the effect of the N and P addition ratio on the bacterial community is more important to study than single nutrient.
Previous studies have found that the effect of N addition on the bacterial community is regulated by the plant. Wang et al. (2022a) found that the plant rhizosphere soil bacterial community differed from the non-rhizosphere bacterial community in response to N addition. Nutrient addition stimulates the release of more C content from plant roots (Liu et al., 2016), which facilitates the growth of bacterial community (Fierer et al., 2009). However, it was also found that although nutrient addition increased the C content released by the root system (Peng et al., 2017), it also increased the proportion of recalcitrant C, which was detrimental to the acquisition and utilization of the bacterial community (Freedman et al., 2013; Liu et al., 2016). Therefore, the study of the effect of nutrient input on the bacterial community should consider the role of plants. It is reasonable to study the plant rhizosphere and non-rhizosphere soil bacterial community separately (Miki et al., 2010).
The Yellow River Delta, located on the southern coast of Bohai Bay, is the youngest and most complete coastal wetland ecosystem in the warm zone, which has important value in maintaining biodiversity, purifying pollutants and regulating microclimate (Liu et al., 2021). During the growing season (MayN-November) in 2012, the nitrogen deposition in the Yellow River Delta was relatively high in the country, at 22.64 Kg ha-1 (Yu et al., 2014). Due to the fragility of coastal wetland ecosystems, imbalances of N and P input may be more damaging to the ecosystem. To investigate the effects of N and P addition on bacterial community, control experiments with different N and P addition ratios were designed to research (a) the effect of N and P input ratios on bacterial community, (b) the effect of plants in the process of bacterial community response to N and P ratios, and (c) the coupling correlation between soil and bacterial community by 16s sequencing technology.
2 Materials and methods
2.1 Study area
This experimental study area is located in the Yellow River Delta wetlands (E 118°44’2”, N 38°1’18”). The annual precipitation is 550-640mm, and the average annual temperature is 12.6°C. The plants are mainly dominated by halophytes, including Limonium bicolor, P.communis, Suaeda salsa, Tamarix chinensis Lour, etc. Based on the distribution of vegetation and growth conditions in the study area, etc., we selected the P.communis distribution area with the widest distribution range for the experiment. The total area of the experimental area is approximately 120 m2.
2.2 Experimental design
The N-P ratio was referred to previous studies (Liu et al., 2019; Li et al., 2022a). Five N and P addition treatments were set up, N0P0 (0 g/m2 N, 0 g/m2 P), N0P1 (0 g/m2 N, 1 g/m2 P), N1P1 (5 g/m2 N, 1 g/m2 P), N2P1 (15 g/m2 N, 1 g/m2 P), N3P1 (45 g/m2 N, 1 g/m2 P). Use urea and KH2PO4 as N and P fertilizers respectively (; Li et al., 2019a). Urea and KH2PO4 were dissolved in pure water and then divided equally into 4 parts. From May to August 2021, N and P addition were added at the beginning of each month. Three replicates were set up for each treatment. A 1 m buffer interval was set up to prevent nutrient flow.
2.3 Soil sampling
Soil collection was conducted in September 2021. Dig out the P.communis completely, shake off the loose soil and then collect the soil attached to the root with the brush, which is considered as rhizosphere soil. In treatment samples, the soil of 5-10 cm depth with no plant growth was collected as non-rhizosphere soil. The collected soil was divided into two parts. One part was used for measuring soil physical and chemical properties, and the other part was used for microbial sequencing.
2.4 Measurements of soil physical and chemical properties
Total nitrogen (TN) and total carbon (TC) content were measured by elemental analyzer (Vario EL III, Elementar, Germany). Total phosphorus (TP) and available phosphorus (AP) content were measured by the ultraviolet-visible spectrophotometer. Soil pH was measured by the pH meter. SOM content was measured by the potassium dichromate volumetric method. The salt content was measured by the conductivity method.
2.5 Soil DNA extraction, sequencing and data analysis
Genomic DNA was extracted from the samples using the soil DNA kit (Omega, USA), followed by agarose gel electrophoresis to detect the purity and concentration of DNA, and the appropriate amount of sample was taken in a centrifuge tube and diluted with sterile water. 16S rRNA genes of distinct regions (V3-V4) were amplified used 341F (5’-CCTAYGGGRBGCASCAG-3’) and 806R (5’-GGACTACNNGGGTATCTAAT-3’) primers. PCR products were detected by electrophoresis using agarose gels with 2% concentration and then were purified with Qiagen Gel Extraction Kit (Qiagen, Germany). The MiSeqPE300 platform of Illumina company (Wekemo Tech Group Co., Ltd. Shenzhen China) was used for high-throughput sequencing.
2.6 Statistical analysis
The effects of N and P addition ratio on α-diversity, soil environmental factor and dominant bacterial phyla were evaluated by one-way ANOVA analysis followed by LSD multiple range tests. Principal coordinate analysis (PCoA) based on the Bray-Curtis distance of OTUs was performed the difference of bacterial community structure. PERMANOVA was conducted to test the effect of N, P and soil position on bacterial community structure. Aggregated boosted tree (ABT) was used to assess the importance of environmental factors on the α-diversity of the bacterial community. OTUs with relative abundance less than 0.1% are filtered out during network construction. The threshold of edge in the network is absolute value of correlation coefficient (Corr) > 0.6 and P < 0.01. The network hubs were selected based on Within-module connectivity (Zi) > 2.5 or Among-module connectivity (Pi) > 0.62 (Wang et al., 2022a). We observe the rate of network stability degradation by removing a certain percentage of OTUs. The structural equation model (SEM) has been revised several times and has the perfect fit. Redundancy analysis (RDA) was used to analyze the effect of soil on the bacterial community.
3 Results
3.1 α-diversity analysis
The response of α-diversity of the bacterial community in P.communis rhizosphere soil and non-rhizosphere soil differed with changes in N and P addition ratio (Figure 1). The α-diversity of the rhizosphere soil bacterial community increased with N and P ratio increasing and reached a maximum at the N3P1 treatment. The Chao1 index significantly increased by 16.7% (P < 0.05) and 15.4% (P < 0.05) compared to N0P0 and N0P1 treatments, respectively, and the Shannon index significantly increased by 5.1% compared to N0P0 treatment. In the non-rhizosphere soil, bacterial community α-diversity decreased with increasing N and P ratio. Compared to the N0P0 treatment, the Chao1 index decreased by 8.7% in the N3P1 treatment (P < 0.05). The α-diversity of the two soil bacterial communities was significantly different in the N3P1 treatment (Table S1, P < 0.05). The analysis combining soil position, N and P addition revealed that the α-diversity of the bacterial community was significantly affected by soil position (Table S2, P < 0.01). The interaction between N addition and soil position also had a significant effect on bacterial community α-diversity (Table S2, P < 0.01), indicating that the effect of N addition on bacterial community α-diversity was related to soil position.
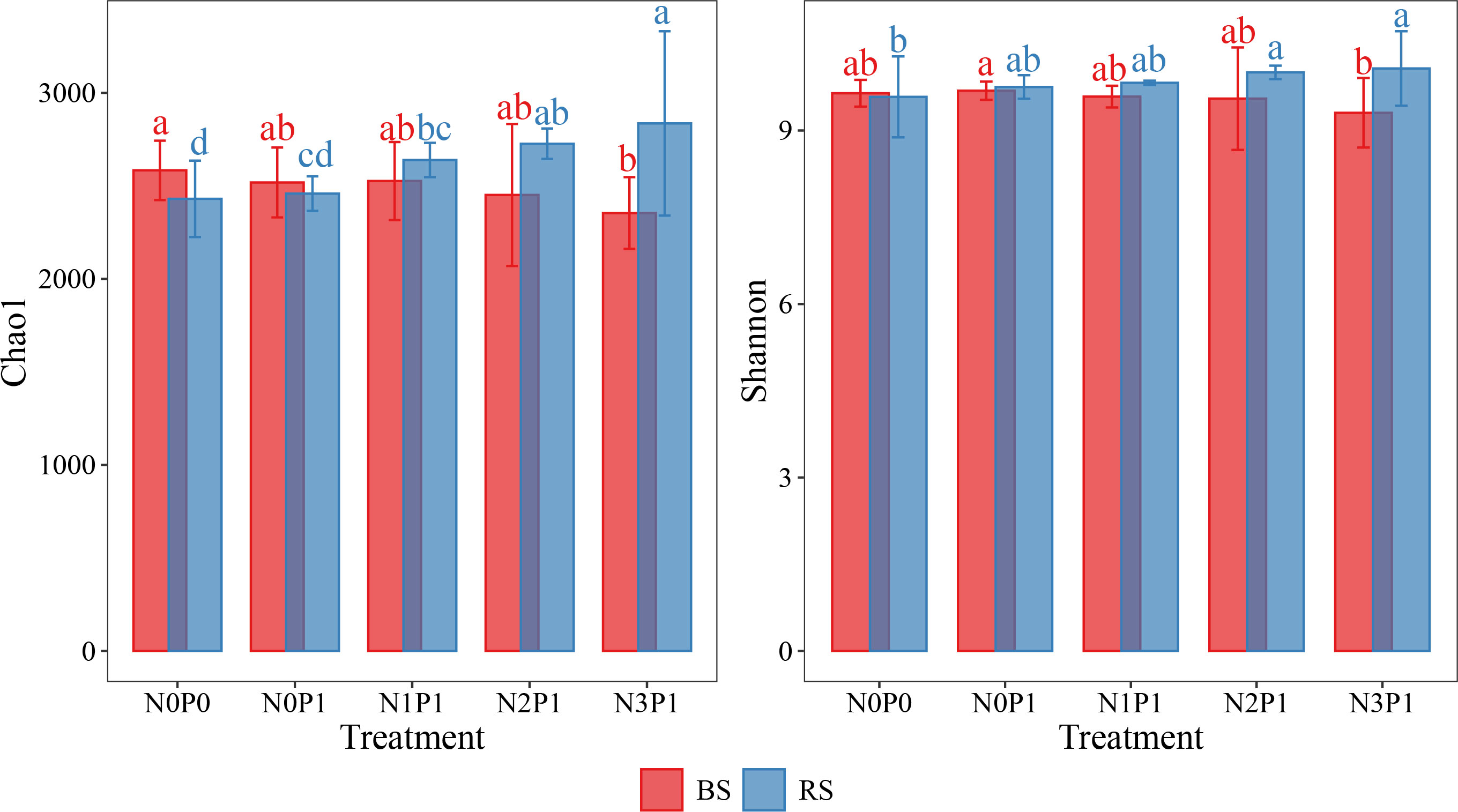
Figure 1 The α-diversity in different N and P addition ratio treatments. BS, non-rhizosphere soil. RS, rhizosphere soil. Different letters with the same color represent significant differences between treatments.
3.2 PCoA analysis
PCoA analysis of bacterial community structure is shown in Figure 2. The first and second axes explained 21.5% and 13.9% of the variations in the bacterial community of rhizosphere soil, respectively. In the rhizosphere soil, N0P0, N1P1 and N2P1 treatments clustered together, indicating that the bacterial community structure of these three treatments was similar and differed from N3P1 and N0P1 treatments. In non-rhizosphere soil, the first two axes explained 26.7% and 17.7% of the variation in the bacterial community, respectively. The bacterial community was clustered according to treatment, indicating that the N and P addition ratio had a significant effect on the bacterial community structure of non-rhizosphere soil. N and P addition ratio increased the differences in bacterial community structure. N and P had a significant effect on the bacterial community structure of non-rhizosphere soil (Table S3, P < 0.05). Nitrogen, phosphorus, and soil position as well as the interaction of all three had significant effects on bacterial community structure (Table S4, P < 0.05). Soil position and the interaction of N and soil position had the greatest effect on bacterial community structure, explaining 15.1% and 15.3% of the variation in bacterial community, respectively (P < 0.001).
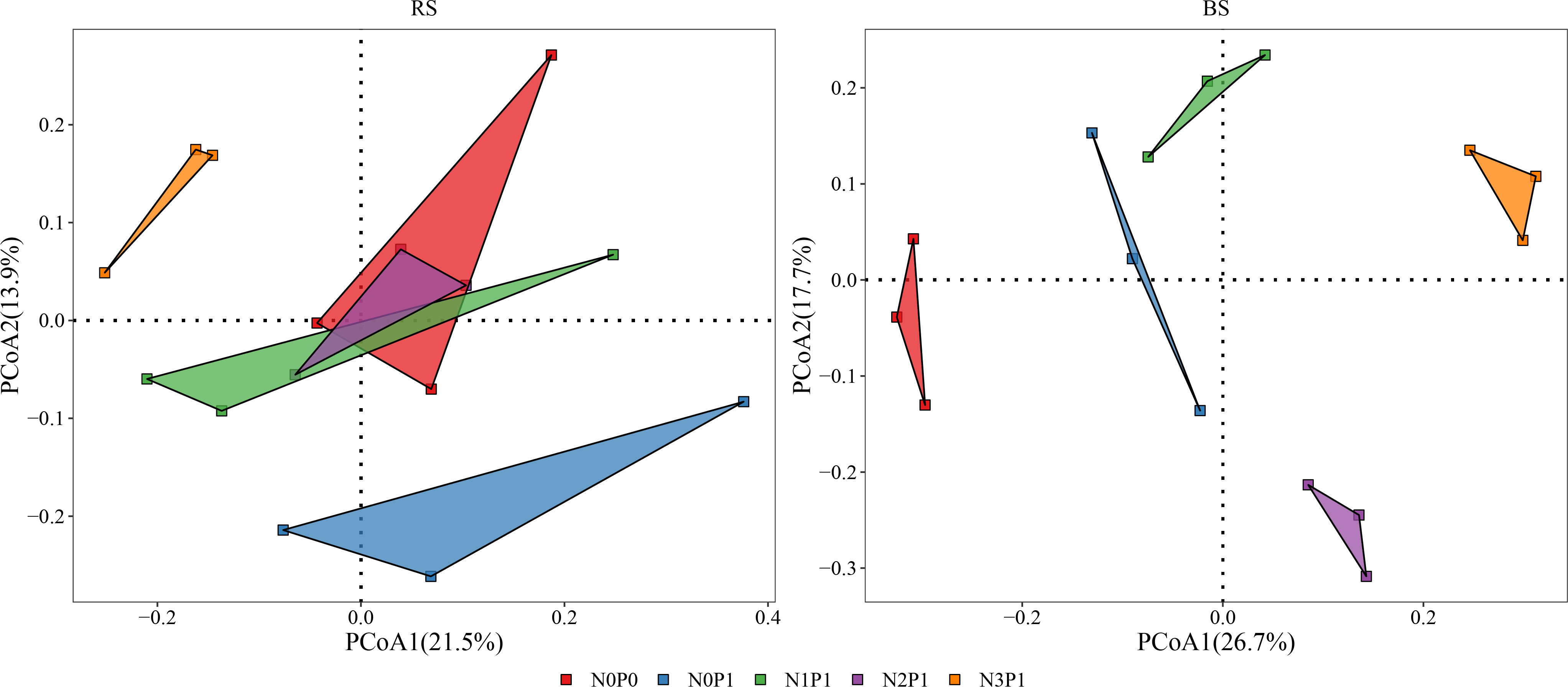
Figure 2 The PCoA analysis in different N and P addition ratio treatments. BS, non-rhizosphere soil. RS, rhizosphere soil.
3.3 Bacterial community composition
The bacterial phylum with relative abundance more than 1% in the rhizosphere and non-rhizosphere soil of P.communis is shown in Figure 3. In the non-rhizosphere soil, N3P1 treatment significantly increased the bacterial community Proteobacteria by 39.9% and 20.6% relative to N0P0 and N0P1 treatment, respectively (P < 0.05). The relative abundance of Actinobacteria, Acidobacteria and Gemmatimonadetes was highest in N0P0 treatment and lowest in N2P1, N3P1 and N3P1 with a decrease of 50.3, 42.3% and 62.6%, respectively (P < 0.05). The Firmicutes had the highest relative abundance in the N0P1 treatment, which was significantly higher than the N0P0 treatment (P < 0.05). There was insignificant variation in the Proteobacteria and Bacteroidetes in the rhizosphere soil. The relative abundance of Acidobacteria and Actinobacteria was highest in the N0P1 treatment. The relative abundance of both of Acidobacteria and Actinobacteria decreased with increasing N and P addition ratio, decreasing by 33.6% and 45.2% in the N3P1 treatment, respectively (P < 0.05). The relative abundance of Chloroflexi and Firmicutes showed contrasting results, with the highest and lowest in N0P0 treatment, respectively. The relative abundance of both decreased and increased with increasing N and P addition ratio, reaching the minimum and maximum value at N3P1 treatment, decreasing and increasing by 46.7% and 178.9%, respectively (P < 0.05).
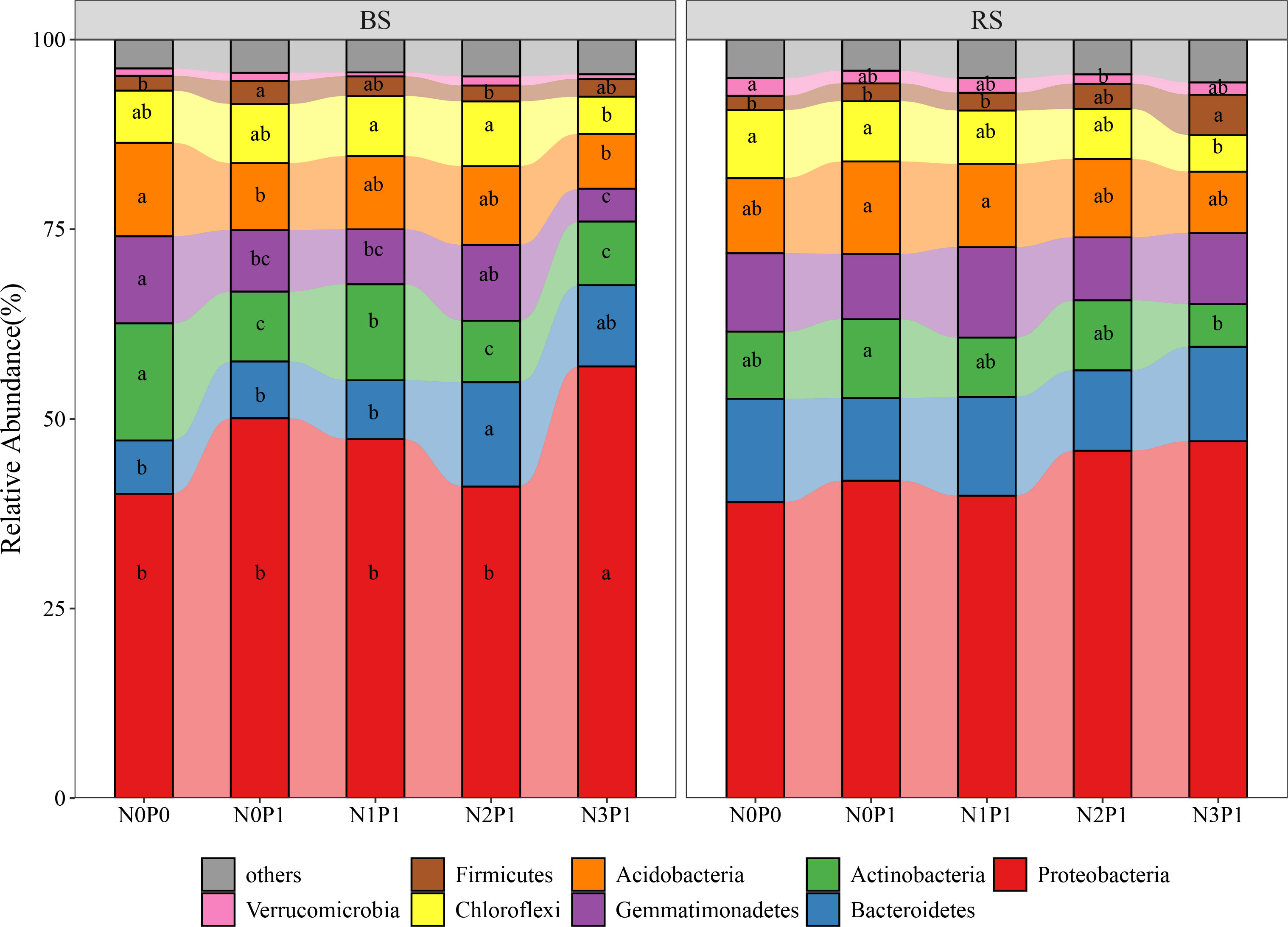
Figure 3 The relative abundance of dominant bacterial phyla in different N and P addition ratio treatments. BS, non-rhizosphere soil. RS, rhizosphere soil. Different lowercase letters represent significant differences between treatments in the same soil position.
3.4 Symbiotic network analysis
The bacterial community symbiotic network is shown in Figure 4A, and the bacterial community network was divided into 7 modules. Seven key nodes were screened, including five connectors and two module bubs (Figure 4B). In addition, we constructed sub-networks for rhizosphere and non-rhizosphere soil, and the number of nodes, number of edges, and complexity of non-rhizosphere soil were higher than those of rhizosphere soil (Table S5). To further compare the stability of the networks, we calculated the network robustness based on the natural connectivity. The results showed that the natural connectivity decreased with the removal of more OTU nodes. The network connectivity of rhizosphere soil was significantly lower than that of non-rhizosphere soil (Figure 4C). Annotated analysis of key OTUs in the microbial interactions network (Figure S1) revealed that seven key OTUs belonged to four phyla, which identified the Proteobacteria, Bacteroidetes, Gemmatimonadetes, and Actinobacteria. Longimicrobiales order, Pseudomonas order, Cyclobacteriaceae family and MND1 genera were significantly higher in rhizosphere soil than in non-rhizosphere soil (Figure S1, P < 0.05).
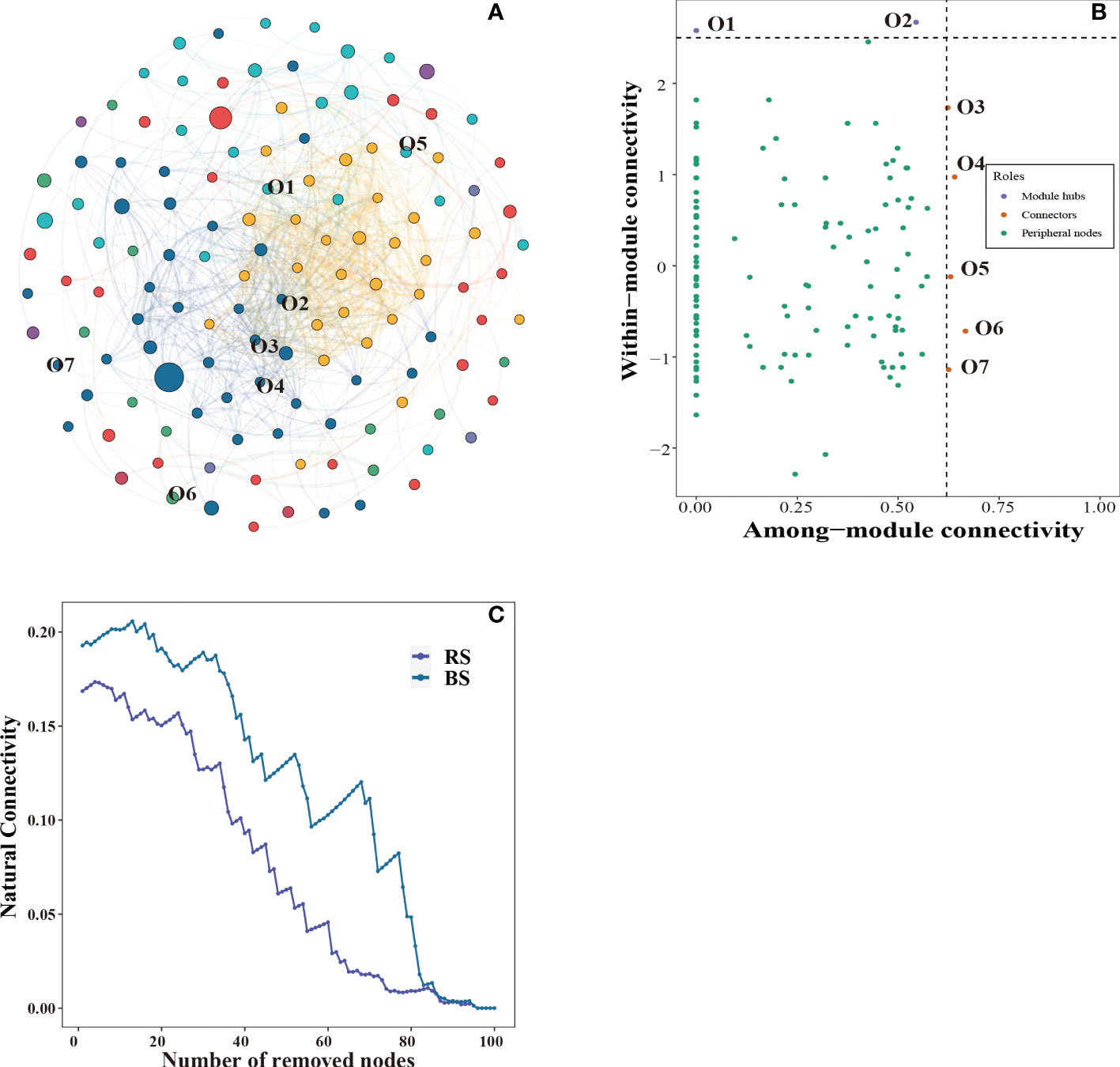
Figure 4 Co-occurrence networks of the bacterial community (A). Putative keystone OTUs (B). The robustness of microbial network for rhizosphere soil and non-rhizosphere soil (C). BS, non-rhizosphere soil. RS, rhizosphere soil.
3.5 Soil environmental factor analysis
Salt, pH, SOM and TC content were significantly higher in rhizosphere soil than in non-rhizosphere soil (Table 1, P < 0.05), while TN and TP showed the opposite (P < 0.05). The soil SOM content increased with increasing N and P addition ratio and was lowest in the N0P1 treatment. In the rhizosphere soil, soil salt content increased with increasing N and P addition ratio, reaching a maximum in the N3P1 treatment, increasing by 57.2% and 40.8% compared to N0P0 and N0P1 treatment, respectively (P < 0.05). The N3P1 treatment decreased pH by 0.19 units compared to the N0P0 treatment (P < 0.05). The pH decreased significantly with increasing N and P ratio in the non-rhizosphere soil (P < 0.05).
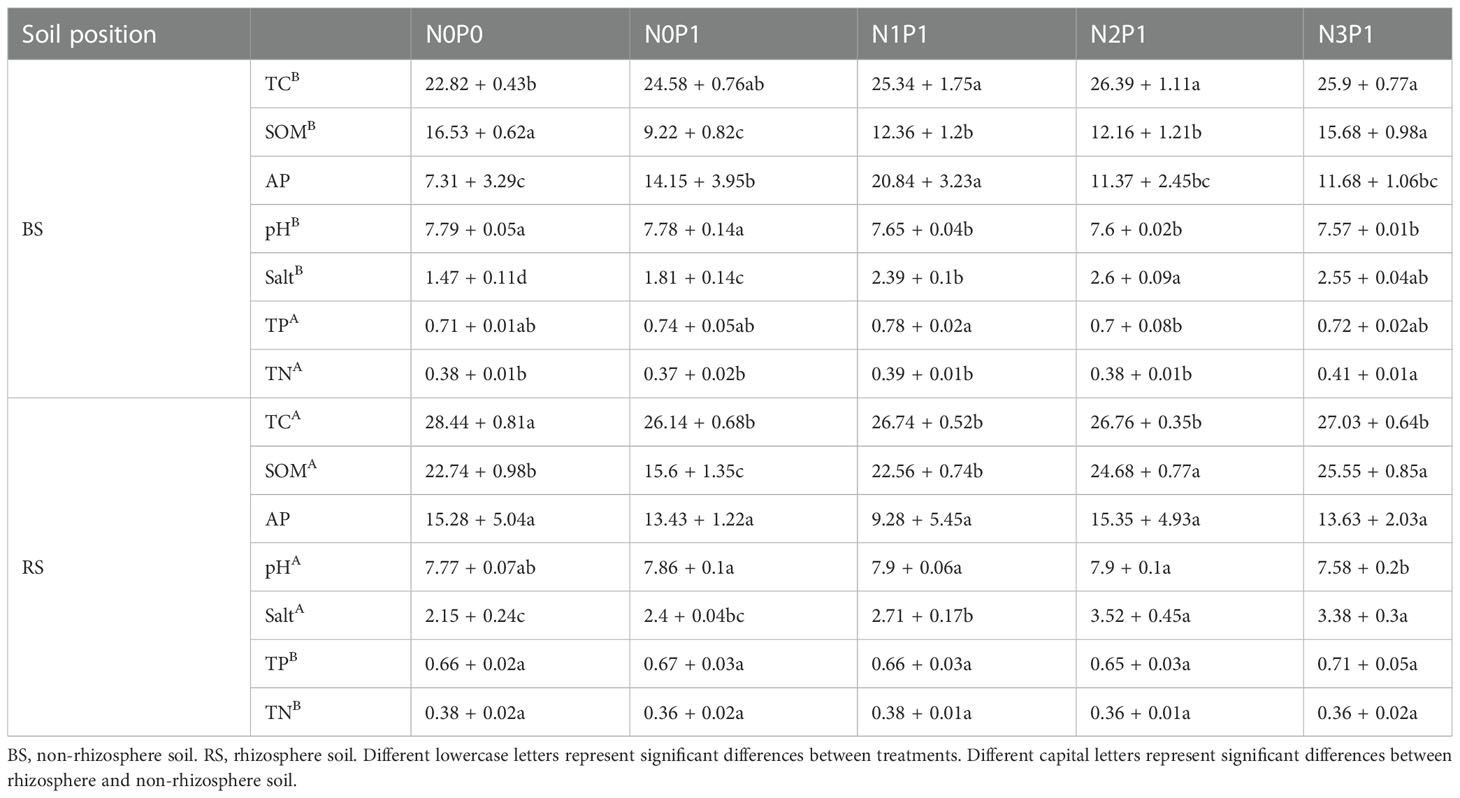
Table 1 The soil environmental factors of bacterial community in different N and P addition ratio treatments.
3.6 Correlation analysis of bacterial community and soil environmental factors
The ABT analysis (Table S6) showed that SOM, TN and salt content had the greatest effect on bacterial community diversity. There was a significant positive correlation between the Shannon index of soil bacterial community and soil salt and SOM (Figure 5), and significant negative correlation with TN (P < 0.05). In the rhizosphere soil, the Shannon index of the bacterial community increased with increasing soil salt and SOM content, but decreased with increasing pH. The Shannon index of the bacterial community was negatively correlated with the TN content in the non-rhizosphere soil.
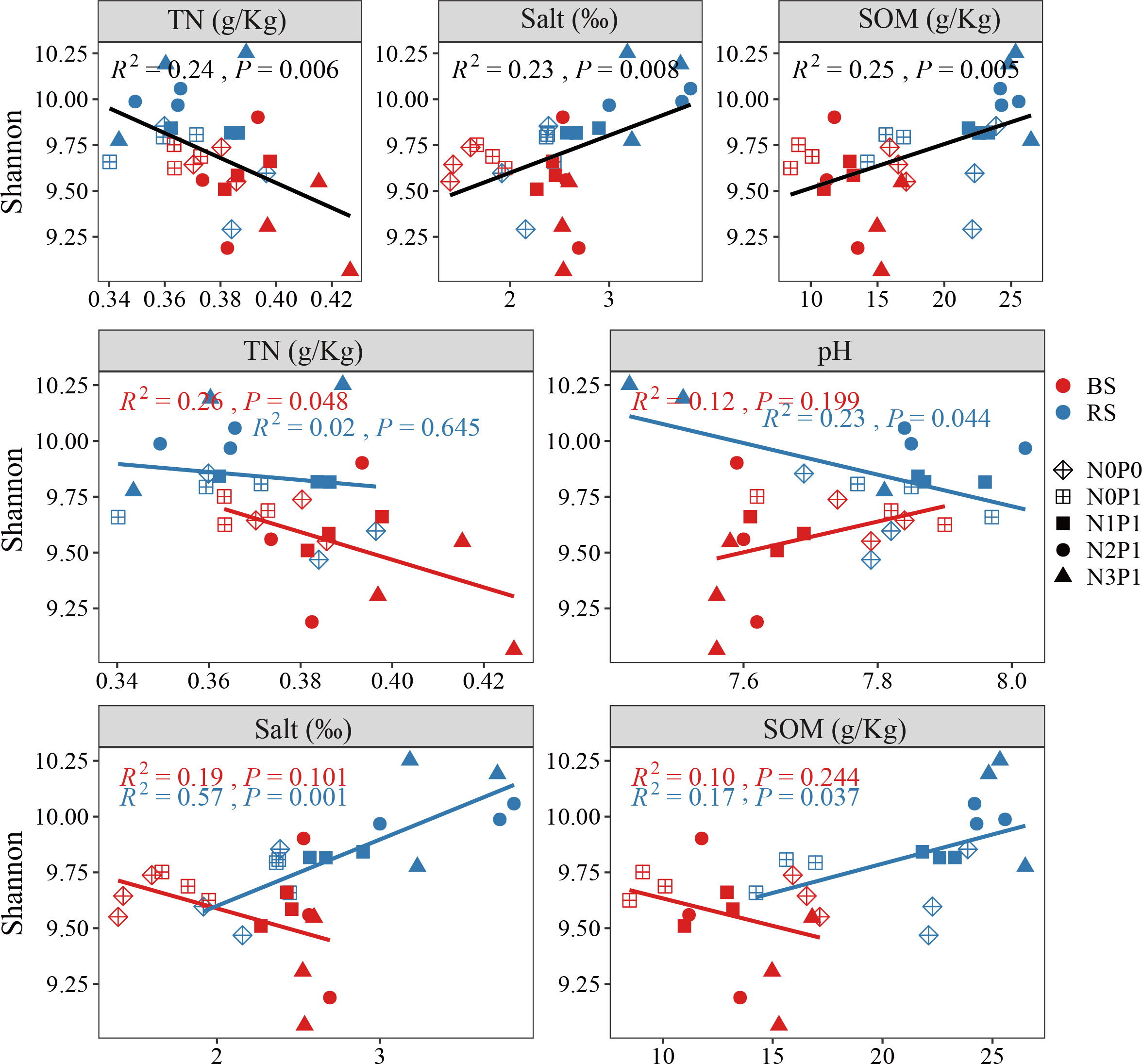
Figure 5 Correlation analysis of Shannon index with soil environmental factors BS, non-rhizosphere soil. RS, rhizosphere soil.
In the rhizosphere soil (Figure 6A), the first two axes explained 42.9% of the variation in the bacterial community dominant phyla. The effect of TP, salt and SOM content on the structure of the bacterial community dominant phyla was significant, explaining 49.25%, 44.28% and 31.38% of the variation, respectively (P < 0.05). The bacterial community dominant phyla composition in non-rhizosphere soil (Figure 6B) was significantly affected by soil salt, TC and pH (P < 0.05). In both soils, TC, TP, salt and SOM content significantly affected the bacterial community (Figure 6C), explaining 39.68%, 20.91%, 26.74% and 29.77% of the variation in the bacterial community dominant phyla composition, respectively (P < 0.05). Soil salt had a significant effect on the bacterial community in all three RDA analyses (Figure 6D, P < 0.05). RDA analysis showed a negative correlation between soil salt and both Actinobacteria and Acidobacteria.
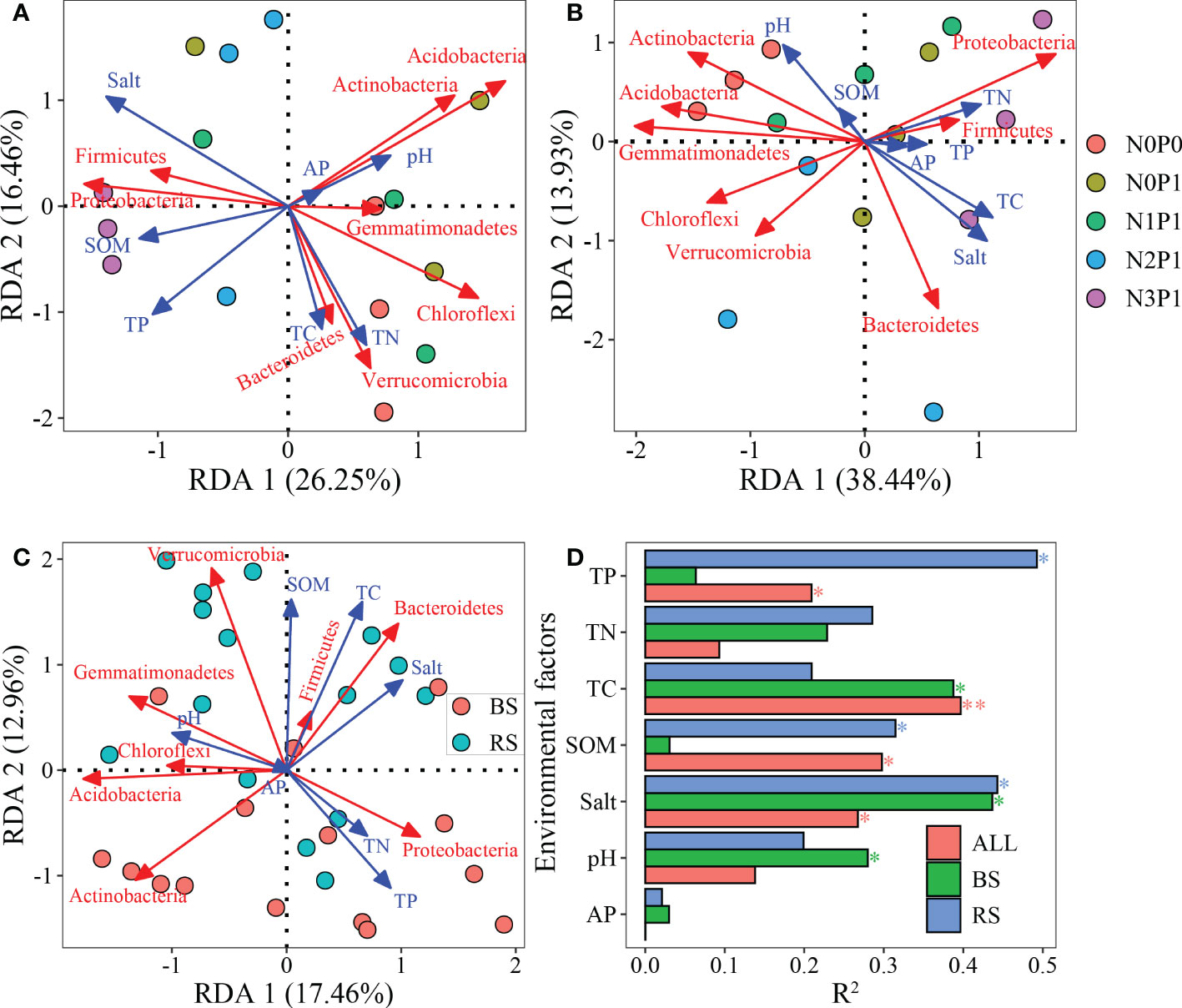
Figure 6 RDA analysis of dominant phyla composition with soil environmental factors. (A), rhizosphere soil. (B), non-rhizosphere soil, (C), both of rhizosphere and non-rhizosphere soil. (D), Contribution of soil environmental factors to variation in bacterial community. BS, non-rhizosphere soil. RS, rhizosphere soil. * and **indicate that the effect of environmental factors is significant at 0.05 and 0.01 level, respectively. RS: rhizosphere soil. BS: non-rhizosphere soil. ALL: rhizosphere and non-rhizosphere soils.
To further investigate the effect of the N and P addition ratio on the bacterial community, we constructed SEM with good fitness (Figure 7). In rhizosphere soil, SEM explained 41.2% of the variation in the Shannon index of the bacterial community (Figure 7A). Shannon diversity was directly influenced by pH, Salt and TN content with path coefficients of -0.38, 0.728 and 0.392, respectively. There was a significant positive effect of N and P addition ratio on soil TC, SOM and soil salinity, and a negative effect on pH (P < 0.05). The N and P addition ratio increased bacterial community α-diversity by decreasing pH and increasing soil salinity, with a total effect of 0.275 and 0.468 for the two pathways, respectively. In non-rhizosphere soil, SEM explained 40.4% of the variation in the bacterial community (Figure 7B). The Shannon index is directly affected by TN and pH, with path coefficients of -0.385 and 0.731, respectively. The N and P addition ratio indirectly decreased the α-diversity by directly and indirectly decreasing soil pH, with a total effect of -0.518.
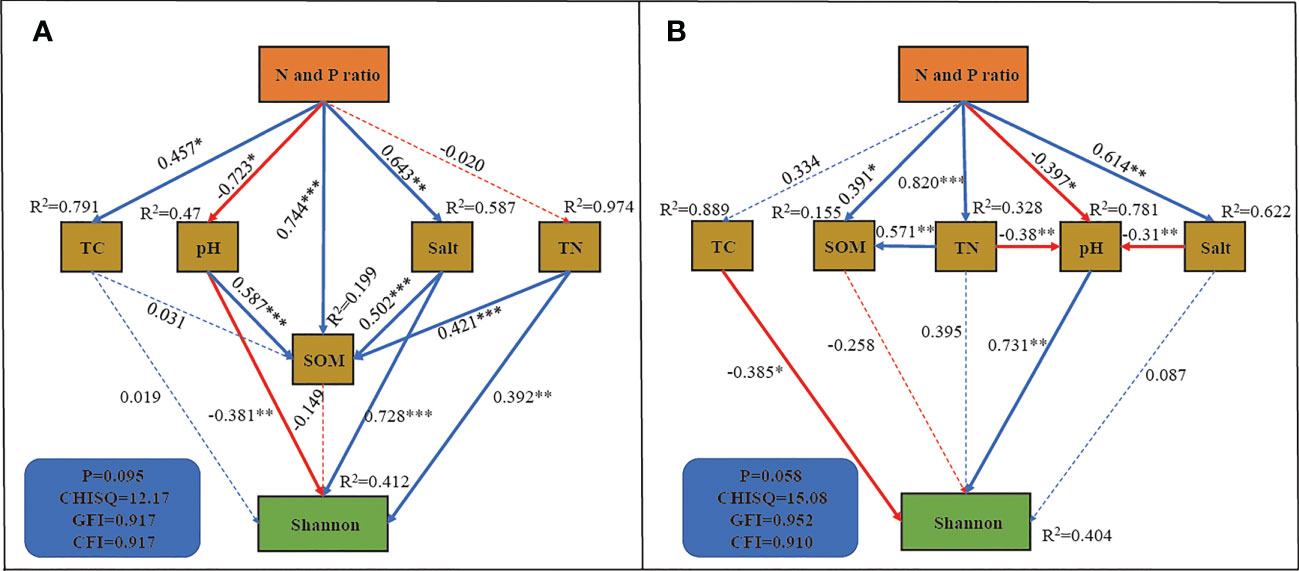
Figure 7 SEM analysis of N and P addition ratio on Shannon index. (A), rhizosphere soil. (B), non-rhizosphere soil. *, **, *** indicate that the path coefficient is significant at 0.05, 0.01 and 0.001 level, respectively.
4 Discussion
4.1 Effect of N and P addition ratio on the α-diversity
Plant-bacterial community interactions are regulated by several factors. N and P are among the important regulatory factors (Wang et al., 2018b). Previous studies have found differences in the effects of N addition on the bacterial community without separating rhizosphere and non-rhizosphere soils (Ling et al., 2017; Zhang et al., 2017). In this work, the rhizosphere and non-rhizosphere soil were studied separately and differences were found in the response of α-diversity of the two soils bacterial community to the N-P addition ratio, which is consistent with the results of Wang et al. (2022a) and Ran et al. (2021) et al. On the one hand, plants uptake soil nutrients and buffer the direct effect of fertilization on bacterial community (Wang et al., 2019). On the other hand, plants are influenced by N and P addition and the increase of rhizosphere secretion released indirectly affects the bacterial community (Liu et al., 2016).
In this work, P.communis rhizosphere bacterial community α-diversity increased with increasing N and P addition ratios, while non-rhizosphere bacterial community α-diversity decreased. SEM analysis showed that N and P addition ratio indirectly decreased α-diversity by lowering pH in non-rhizosphere soil, which is consistent with previous studies (Chen et al., 2021; Niu et al., 2021). Although the N and P addition ratio increased the non-rhizosphere soil TC content, it did not have a significant increase on bacterial community α-diversity. Ning et al. (2021) found that N addition increased soil TC but reduced the effectiveness of soil microbial available C, leading to increased microbial C limitation and inhibition of microbial activity. Unlike in non-rhizosphere soil, N and P addition decreased soil pH and increased soil salt to increase rhizosphere soil bacterial α-diversity. Yao et al. (2021) found that soil salt and pH dominated the soil bacterial community diversity in saline agricultural fields, which is consistent with our study. pH was significantly lower in the N3P1 treatment, but was higher and less variable compared to non-interstitial soil. Brigham et al. (2022) found that N addition only resulted in lower soil pH when the plant was removed, which is consistent with our study.
Salt is an important factor in changing the bacterial community (Kamble et al., 2014; Wang et al., 2018a). Increased salt content affects plant growth and bacterial community diversity and structure(Cheng et al., 2021), negatively affecting ecosystem stability, while lower soil salinity favors bacterial community richness and diversity in coastal wetland ecosystems(Li et al., 2021). The increase in rhizosphere soil bacterial community α-diversity was mainly due to the increase in soil salt, which was inconsistent with most previous studies. P.communis are typically salt-rejecting plants (Zhao et al., 2022a), and the root can filter salt ions to reduce the damage. The N and P ratio increased water uptake, leading to an increase in rhizosphere soil salt. Contrary to conventional wisdom, increasing salt does not simply decrease bacterial diversity, but increases microbial abundance and diversity at moderate salt levels (Ji et al., 2019). This indicates that despite the increase in P.communis rhizosphere soil salt, it did not reach the threshold for suppression of bacterial community α-diversity. The TN content of non-rhizosphere soil increased with the increase of the N-P input ratio, and there was a significant negative correlation with bacterial community diversity. However, in rhizosphere soil, TN content did change insignificantly with increasing N and P ratios. Zhao et al. (2022b) found that P.communis absorb as much soil N as possible and stores it in their body to provide for their growth. SEM showed a significant positive effect of TN content on α-diversity, which may be caused by the N limitation of the rhizosphere bacterial community due to the uptake of soil N with P.communis.
4.2 Response of bacterial community structure to N and P addition ratio
Soil and plant properties and plant-microbe interactions affect bacterial community composition(Weand et al., 2010). PCoA analysis showed that the N and P addition ratio had insignificant effects on the bacterial community structure of the rhizosphere soil and significant effect on that of non-rhizosphere soil. The difference in variance between treatments increased with increasing N and P addition ratio, which is similar to the previous study(Ling et al., 2017). While in the rhizosphere soil, only the N3P1 and N0P1 treatments were different from the N0P0. Plant growth in the Yellow River Delta is mainly N-limited (Zhang et al., 2015), and P addition exacerbates the N-limitation of P.communis growth. Response of plants to N limitation altered bacterial community structure. In addition, the P addition decreases the N/P of the root secretion, thus causing major variations in the bacterial community structure(Ma et al., 2023). PERMANOVA analysis revealed differences in the effects of both N and P on the bacterial community structure of the two soils, which may be due to P.communis buffering soil nutrient changes (Wang et al., 2019).
4.3 Response of bacterial community phylum composition to N and P addition ratio
In the present study, the highest relative abundance of Proteobacteria was found in both soils, which is consistent with previous reports (Li et al., 2018; Shao et al., 2019), suggesting that Proteobacteria is more widely adapted. The relative abundance of Acidobacteria and Actinobacteria was highest in the N0P0 treatment, while the relative abundance decreased with the increase of the N-P addition ratio. The N and P addition ratio increased the relative abundance of the non-rhizosphere soil Proteobacteria and Bacteroidetes, which was similar to the previous studies (Ma et al., 2022; Ma et al., 2023). Proteobacteria and Bacteroidetes are eutrophic taxa (Wang et al., 2022b), and soil nutrients increase the relative abundance of both. The Acidobacteria belongs to oligotrophic taxa, and increased nutrients hurt their abundance (Sha et al., 2021). Actinobacteria belong to eutrophic taxa, however, nutrient addition had a significant negative effect on their abundance (Li et al., 2019b). The RDA analysis showed negative correlation between Actinobacteria and salt. Actinobacteria are susceptible to salt limitation (Zhao et al., 2020). In the rhizosphere soil, the relative abundance of the Chloroflexi decreased with increasing N and P ratios. A study (Hu et al., 2022) showed that N addition reduced the relative abundance of Chloroflexi. The Chloroflexi uses CO2 as a C source for photosynthesis (Klatt et al., 2013). Elevated N and P ratio increases SOM content, which may be at a disadvantage in competition with other bacteria (Yang et al., 2022b). The relative abundance of Bacteroidetes of rhizosphere soil was higher than that of Actinobacteria because Bacteroidetes have stronger salt tolerance and are more adaptable to the high salt environment of P.communis rhizosphere soil (Zhao et al., 2020).
4.4 Effect of plants on bacterial community
Symbiotic networks are increasingly used to explore potential microbial interactions (Wang et al., 2022a). In this study, it was found that the number of nodes, the number of edges and the complexity of the symbiotic network of rhizosphere soil were lower than those of non-rhizosphere soil, which is consistent with the previous study (Ling et al., 2022). Modularity of the rhizosphere soil bacterial network was higher than that of the non-rhizosphere soil, but the bacterial symbiotic network in the rhizosphere soil was less stable (Ling et al., 2022). Wang et al. (2022a) found that network complexity was significantly influenced by plant root secretions. Compared to non-rhizosphere soil, the rhizosphere environment is influenced by plant secretions and has a stronger ability to select for the bacterial community. In addition, the increase of N and P addition ratio may strengthen the ability of the rhizosphere environment to select bacterial community, which is detrimental to the robustness of the network. Annotation of key nodes in the symbiotic network revealed that Longimicrobiales order, Pseudomonas order, Cyclobacteriaceae family and MND1 genera were significantly higher in the rhizosphere than in non-rhizosphere soil. Qian et al. (2022) found that the abundance of Longimicrobiales order was higher in rhizosphere soil than in non-rhizosphere soil, due to more dodecanoic acid in rhizosphere soil. Yang et al. (2022a) found that the Longimicrobiales order is mainly associated with root apoplast through the modular function of the co-occurrence network, which further corroborates its close connection with plant roots. Pseudomonas has been shown to suppress plant pathogens and promote plant growth (Tao et al., 2020). In addition, Pseudomonas has a strong N fixation capacity (Li et al., 2022b) and can provide N for P.communis, which may also be an important reason why P.communis can adapt to N-deprived habitat. Both the Cyclobacteriaceae family and MND1 genera were also found to promote plant growth (Li et al., 2020; Cheng et al., 2022), which is consistent with our study.
5 Conclusion
Increased N and P input ratio reduced the non-rhizosphere bacterial communityα-diversity, which was caused by the decrease of pH. The rhizosphere soil bacterial community α-diversity increased with increasing N and P ratios. This was due to the absorption of N from the soil by the P.communis, which reduced the direct effect of N and P addition on the bacterial community. Variation in rhizosphere soil bacterial community α-diversity is due to plant response to N and P addition ratio. The effect of imbalance N and P addition ratio on the community structure of rhizosphere bacteria was less than that of non-rhizosphere soil. N and P additions increased the relative abundance of Bacteroidetes and Proteobacteria in non-rhizosphere soil and decreased the relative abundance of Acidobacteria and Actinobacteria. Salt is a key influencing factor in the composition of both soil bacterial communities. These results can deepen our understanding of the relationship between plant and bacterial community and provide theoretical guides for the restoration of degraded soil in coastal wetlands under the background of N deposition.
Data availability statement
The datasets presented in this study can be found in online repositories. The names of the repository/repositories and accession number(s) can be found below: https://www.ncbi.nlm.nih.gov/, PRJNA935937.
Author contributions
ZZ completed data analysis and wrote the manuscript. JS conceived and designed the study, and fund support. TL and PS were in charge of the modification of the manuscript. JM and KD conducted sample collection. All authors contributed to the article and approved the submitted version.
Funding
The funding was supported by the National Natural Science Foundation of China (42171059, 41971119 and 41871089), the Natural Science Foundation of Shandong Province (ZR2019MD024, ZR2020QD004) and the Science and Technology Support Plan for Youth Innovation of Colleges and Universities (2019KJD010).
Conflict of interest
The authors declare that the research was conducted in the absence of any commercial or financial relationships that could be construed as a potential conflict of interest.
Publisher’s note
All claims expressed in this article are solely those of the authors and do not necessarily represent those of their affiliated organizations, or those of the publisher, the editors and the reviewers. Any product that may be evaluated in this article, or claim that may be made by its manufacturer, is not guaranteed or endorsed by the publisher.
Supplementary material
The Supplementary Material for this article can be found online at: https://www.frontiersin.org/articles/10.3389/fpls.2023.1168111/full#supplementary-material
References
Bell, C., Carrillo, Y., Boot, C. M., Rocca, J. D., Pendall, E., Wallenstein, M. D. (2014). Rhizosphere stoichiometry: are c : N : P ratios of plants, soils, and enzymes conserved at the plant species-level? New Phytol. 201, 505–517. doi: 10.1111/nph.12531
Bennett, J. A., Maherali, H., Reinhart, K. O., Lekberg, Y., Hart, M. M., Klironomos, J. (2017). Plant-soil feedbacks and mycorrhizal type influence temperate forest population dynamics. Science 355, 181–184. doi: 10.1126/science.aai8212
Brigham, L. M., De Mesquita, C. P. B., Smith, J. G., Sartwell, S. A., Schmidt, S. K., Suding, K. N. (2022). Do plant-soil interactions influence how the microbial community responds to environmental change? Ecology 103, e03554. doi: 10.1002/ecy.3554
Chen, L., Li, K. K., Shi, W. J., Wang, X. L., Wang, E. T., Liu, J. F., et al. (2021). Negative impacts of excessive nitrogen fertilization on the abundance and diversity of diazotrophs in black soil under maize monocropping. Geoderma 393, 114999. doi: 10.1016/j.geoderma.2021.114999
Cheng, Q., Chang, H., Yang, X., Wang, D., Wang, W. (2021). Salinity and nutrient modulate soil bacterial communities in the coastal wetland of the yellow river delta, China. Environ. Sci. pollut. Res. Int. 28, 14621–14631. doi: 10.1007/s11356-020-11626-x
Cheng, H., Mai, Z. M., Wang, Y. S., Liu, D. X., Sun, Y. T. (2022). Role of extracellular polymeric substances in metal sequestration during mangrove restoration. Chemosphere 306, 135550. doi: 10.1016/j.chemosphere.2022.135550
Cusack, D. F., Silver, W. L., Torn, M. S., Burton, S. D., Firestone, M. K. (2011). Changes in microbial community characteristics and soil organic matter with nitrogen additions in two tropical forests. Ecology 92, 621–632. doi: 10.1890/10-0459.1
Deng, Q., Hui, D., Dennis, S., Reddy, K. C., Xu, X. (2017). Responses of terrestrial ecosystem phosphorus cycling to nitrogen addition: A meta-analysis. Global Ecol. Biogeography 26, 713–728. doi: 10.1111/geb.12576
Dong, C. C., Wang, W., Liu, H. Y., Xu, X. T., Zeng, H. (2019). Temperate grassland shifted from nitrogen to phosphorus limitation induced by degradation and nitrogen deposition: Evidence from soil extracellular enzyme stoichiometry. Ecol. Indic. 101, 453–464. doi: 10.1016/j.ecolind.2019.01.046
Elser, J. J., Bracken, M. E., Cleland, E. E., Gruner, D. S., Harpole, W. S., Hillebrand, H., et al. (2007). Global analysis of nitrogen and phosphorus limitation of primary producers in freshwater, marine and terrestrial ecosystems. Ecol. Lett. 10, 1135–1142. doi: 10.1111/j.1461-0248.2007.01113.x
Fierer, N., Lauber, C. L., Ramirez, K. S., Zaneveld, J., Bradford, M. A., Knight, R. (2012). Comparative metagenomic, phylogenetic and physiological analyses of soil microbial communities across nitrogen gradients. ISME J. 6, 1007–1017. doi: 10.1038/ismej.2011.159
Fierer, N., Strickland, M. S., Liptzin, D., Bradford, M. A., Cleveland, C. C. (2009). Global patterns in belowground communities. Ecol. Lett. 12, 1238–1249. doi: 10.1111/j.1461-0248.2009.01360.x
Freedman, Z., Eisenlord, S. D., Zak, D. R., Xue, K., He, Z. L., Zhou, J. Z. (2013). Towards a molecular understanding of n cycling in northern hardwood forests under future rates of n deposition. Soil Biol. Biochem. 66, 130–138. doi: 10.1016/j.soilbio.2013.07.010
Hu, Y., Chen, M., Yang, Z. L., Cong, M. F., Zhu, X. P., Jia, H. T. (2022). Soil microbial community response to nitrogen application on a swamp meadow in the arid region of central Asia. Front. Microbiol. 12. doi: 10.3389/fmicb.2021.797306
Ji, M. K., Kong, W. D., Yue, L. Y., Wang, J. B., Deng, Y., Zhu, L. P. (2019). Salinity reduces bacterial diversity, but increases network complexity in Tibetan plateau lakes. FEMS Microbiol. Ecol. 95, fiz190. doi: 10.1093/femsec/fiz190
Kamble, P. N., Gaikwad, V. B., Kuchekar, S. R., Baath, E. (2014). Microbial growth, biomass, community structure and nutrient limitation in high pH and salinity soils from pravaranagar (India). Eur. J. Soil Biol. 65, 87–95. doi: 10.1016/j.ejsobi.2014.10.005
Klatt, C. G., Liu, Z. F., Ludwig, M., Kuhl, M., Jensen, S. I., Bryant, D. A., et al. (2013). Temporal metatranscriptomic patterning in phototrophic chloroflexi inhabiting a microbial mat in a geothermal spring. Isme J. 7, 1775–1789. doi: 10.1038/ismej.2013.52
Li, X. M., Chen, Q. L., He, C., Shi, Q., Chen, S. C., Reid, B. J., et al. (2019b). Organic carbon amendments affect the chemodiversity of soil dissolved organic matter and its associations with soil microbial communities. Environ. Sci. Technol. 53, 50–59. doi: 10.1021/acs.est.8b04673
Li, J., Han, G., Wang, G., Liu, X., Zhang, Q., Chen, Y., et al. (2022a). Imbalanced nitrogen–phosphorus input alters soil organic carbon storage and mineralisation in a salt marsh. Catena 208, 105720. doi: 10.1016/j.catena.2021.105720
Li, Y., Kang, E., Song, B., Wang, J., Zhang, X., Wang, J., et al. (2021). Soil salinity and nutrients availability drive patterns in bacterial community and diversity along succession gradient in the yellow river delta. Estuarine Coast. Shelf Sci. 262, 107621. doi: 10.1016/j.ecss.2021.107621
Li, L., Liu, B., Gao, X., Li, X., Li, C. (2019a). Nitrogen and phosphorus addition differentially affect plant ecological stoichiometry in desert grassland. Sci. Rep. 9, 18673. doi: 10.1038/s41598-019-55275-8
Li, W., Lv, X., Ruan, J., Yu, M., Song, Y. B., Yu, J., et al. (2018). Variations in soil bacterial composition and diversity in newly formed coastal wetlands. Front. Microbiol. 9. doi: 10.3389/fmicb.2018
Li, Y. B., Yang, R., Haggblom, M. M., Li, M. Y., Guo, L. F., Li, B. Q., et al. (2022b). Characterization of diazotrophic root endophytes in Chinese silvergrass (Miscanthus sinensis). Microbiome 10, 186. doi: 10.1186/s40168-022-01379-9
Li, X. N., Yao, S., Bian, Y. R., Jiang, X., Song, Y. (2020). The combination of biochar and plant roots improves soil bacterial adaptation to PAH stress: Insights from soil enzymes, microbiome, and metabolome. J. Hazardous Materials 400, 123227. doi: 10.1016/j.jhazmat.2020.123227
Ling, N., Chen, D., Guo, H., Wei, J., Bai, Y., Shen, Q., et al. (2017). Differential responses of soil bacterial communities to long-term n and p inputs in a semi-arid steppe. Geoderma 292, 25–33. doi: 10.1016/j.geoderma.2017.01.013
Ling, N., Wang, T. T., Kuzyakov, Y. (2022). Rhizosphere bacteriome structure and functions. Nat. Commun. 13, 836. doi: 10.1038/s41467-022-28448-9
Liu, J., Li, A., Sun, J., Song, A., Xia, J. (2021). Foliar c, n, and p stoichiometry of dominant shrubs in the chenier wetland of the yellow river delta. China. Acta Ecologica Sin. 41 (10), 3805–3815. doi: 10.5846/stxb202007091789
Liu, X., Wang, G., Ran, Y., Qi, D., Han, G., Guan, B., et al. (2019). Overall supply level, not the relative supply of nitrogen and phosphorus, affects the plant community composition of a supratidal wetland in the yellow river delta. Sci. Total Environ. 695, 133866. doi: 10.1016/j.scitotenv.2019.133866
Liu, J., Wu, N. N., Wang, H., Sun, J. F., Peng, B., Jiang, P., et al. (2016). Nitrogen addition affects chemical compositions of plant tissues, litter and soil organic matter. Ecology 97, 1796–1806. doi: 10.1890/15-1683.1
Liu, X. J., Zhang, Y., Han, W. X., Tang, A. H., Shen, J. L., Cui, Z. L., et al. (2013). Enhanced nitrogen deposition over China. Nature 494, 459–462. doi: 10.1038/nature11917
Lu, G., Xie, B., Cagle, G. A., Wang, X., Han, G., Wang, X., et al. (2021). Effects of simulated nitrogen deposition on soil microbial community diversity in coastal wetland of the yellow river delta. Sci. Total Environ. 757, 143825. doi: 10.1016/j.scitotenv.2020.143825
Ma, S. H., Chen, G. P., Tang, W. G., Xing, A. J., Chen, X., Xiao, W., et al. (2021). Inconsistent responses of soil microbial community structure and enzyme activity to nitrogen and phosphorus additions in two tropical forests. Plant Soil 460, 453–468. doi: 10.1007/s11104-020-04805-9
Ma, X. Y., Wang, T. X., Shi, Z., Chiariello, N. R., Docherty, K., Field, C. B., et al. (2022). Long-term nitrogen deposition enhances microbial capacities in soil carbon stabilization but reduces network complexity. Microbiome 10, 112. doi: 10.1186/s40168-022-01309-9
Ma, X. M., Zhou, Z., Chen, J., Xu, H., Ma, S. H., Dippold, M. A., et al. (2023). Long-term nitrogen and phosphorus fertilization reveals that phosphorus limitation shapes the microbial community composition and functions in tropical montane forest soil. Sci. Total Environ. 854, 158709. doi: 10.1016/j.scitotenv.2022.158709
Mao, Qg, Lu, Xk., Zhou, Kj., Chen, H., Zhu, Xm, Mori, Tk. (2017). Effects of long-term nitrogen and phosphorus additions on soil acidification in an n-rich tropical forest. GEODERMA 285, 57–63. doi: 10.1016/j.geoderma.2016.09.017
Miki, T., Ushio, M., Fukui, S., Kondoh, M. (2010). Functional diversity of microbial decomposers facilitates plant coexistence in a plant-microbe-soil feedback model. Proc. Natl. Acad. Sci. U.S.A. 107, 14251–14256. doi: 10.1073/pnas.0914281107
Ning, Q. S., Hattenschwiler, S., Lu, X. T., Kardol, P., Zhang, Y. H., Wei, C. Z., et al. (2021). Carbon limitation overrides acidification in mediating soil microbial activity to nitrogen enrichment in a temperate grassland. Global Change Biol. 27, 5976–5988. doi: 10.1111/gcb.15819
Niu, G., Hasi, M., Wang, R., Wang, Y., Geng, Q., Hu, S., et al. (2021). Soil microbial community responses to long-term nitrogen addition at different soil depths in a typical steppe. Appl. Soil Ecol. 167, 104054. doi: 10.1016/j.apsoil.2021.104054
Peng, Y. F., Guo, D. L., Yang, Y. H. (2017). Global patterns of root dynamics under nitrogen enrichment. Global Ecol. Biogeography 26, 102–114. doi: 10.1111/geb.12508
Penuelas, J., Sardans, J. (2022). The global nitrogen-phosphorus imbalance. Science 375, 266–267. doi: 10.1126/science.abl4827
Peñuelas, J., Sardans, J., Rivasubach, A., Janssens, I. A. (2012). The human-induced imbalance between c, n and p in earth's life system. Global Change Biol. 18, 3–6. doi: 10.1111/j.1365-2486.2011.02568.x
Qian, F. H., Huang, X. J., Su, X. M., Bao, Y. Y. (2022). Responsese of microbial communities and metabolic profiles to the rhizosphere of tamarix ramosissima in soils contaminated by multiple heavy metals. J. Hazardous Materials 438, 129469. doi: 10.1016/j.jhazmat.2022.129469
Ran, J., Liu, X., Hui, X., Ma, Q., Liu, J. (2021). Differentiating bacterial community responses to long-term phosphorus fertilization in wheat bulk and rhizosphere soils on the loess plateau. Appl. Soil Ecol. 166, 104090. doi: 10.1016/j.apsoil.2021.104090
Redding, M. R., Shorten, P. R., Lewis, R., Pratt, C., Paungfoo-Lonhienne, C., Hill, J. (2016). Soil n availability, rather than n deposition, controls indirect N2O emissions. Soil Biol. Biochem. 95, 288–298. doi: 10.1016/j.soilbio.2016.01.002
Sha, M. H., Xu, J., Zheng, Z. C., Fa, K. Y. (2021). Enhanced atmospheric nitrogen deposition triggered little change in soil microbial diversity and structure in a desert ecosystem. Global Ecol. Conserv. 31, e01879. doi: 10.1016/j.gecco.2021.e01879
Shao, P., Liang, C., Rubert-Nason, K., Li, X., Xie, H., Bao, X. (2019). Secondary successional forests undergo tightly-coupled changes in soil microbial community structure and soil organic matter. Soil Biol. Biochem. 128, 56–65. doi: 10.1016/j.soilbio.2018.10.004
Tao, C. Y., Li, R., Xiong, W., Shen, Z. Z., Liu, S. S., Wang, B. B., et al. (2020). Bio-organic fertilizers stimulate indigenous soil pseudomonas populations to enhance plant disease suppression. Microbiome 8, 137. doi: 10.1186/s40168-020-00892-z
Tian, D. S., Niu, S. L. (2015). A global analysis of soil acidification caused by nitrogen addition. Environ. Res. Lett. 10, 24019. doi: 10.1088/1748-9326/10/2/024019
Wang, H., Gilbert, J. A., Zhu, Y., Yang, X. (2018a). Salinity is a key factor driving the nitrogen cycling in the mangrove sediment. Sci. Total Environ. 631, 1342–1349. doi: 10.1016/j.scitotenv.2018.03.102
Wang, J., Liao, L., Ye, Z., Liu, H., Zhang, C., Zhang, L., et al. (2022a). Different bacterial co-occurrence patterns and community assembly between rhizosphere and bulk soils under n addition in the plant–soil system. Plant Soil 471, 697–713. doi: 10.1007/s11104-021-05214-2
Wang, H., Liu, S., Zhang, X., Mao, Q., Li, X., You, Y., et al. (2018b). Nitrogen addition reduces soil bacterial richness, while phosphorus addition alters community composition in an old-growth n-rich tropical forest in southern China. Soil Biol. Biochem. 127, 22–30. doi: 10.1016/j.soilbio.2018.08.022
Wang, Q., Ma, M., Jiang, X., Guan, D., Wei, D., Zhao, B., et al. (2019). Impact of 36 years of nitrogen fertilization on microbial community composition and soil carbon cycling-related enzyme activities in rhizospheres and bulk soils in northeast China. Appl. Soil Ecol. 136, 148–157. doi: 10.1016/j.apsoil.2018.12.019
Wang, L., Zhang, H., Wang, J., Wang, J. D., Zhang, Y. C. (2022b). Long-term fertilization with high nitrogen rates decreased diversity and stability of diazotroph communities in soils of sweet potato. Appl. Soil Ecol. 170, 104266. doi: 10.1016/j.apsoil.2021.104266
Weand, M. P., Arthur, M. A., Lovett, G. M., Mcculley, R. L., Weathers, K. C. (2010). Effects of tree species and n additions on forest floor microbial communities and extracellular enzyme activities. Soil Biol. Biochem. 42, 2161–2173. doi: 10.1016/j.soilbio.2010.08.012
Widdig, M., Heintz-Buschart, A., Schleuss, P.-M., Guhr, A., Borer, E. T., Seabloom, E. W., et al. (2020). Effects of nitrogen and phosphorus addition on microbial community composition and element cycling in a grassland soil. Soil Biol. Biochem. 151, 108041. doi: 10.1016/j.soilbio.2020.108041
Yang, Y. Y., Chen, Q. Q., Yu, W., Shi, Z. (2022b). Estimating soil bacterial abundance and diversity in the southeast qinghai-Tibet plateau. Geoderma 416, 115807. doi: 10.1016/j.geoderma.2022.115807
Yang, G. W., Ryo, M., Roy, J., Lammel, D. R., Ballhausen, M. B., Jing, X., et al. (2022a). Multiple anthropogenic pressures eliminate the effects of soil microbial diversity on ecosystem functions in experimental microcosms. Nat. Commun. 13, 4260. doi: 10.1038/s41467-022-31936-7
Yao, R. J., Yang, J. S., Wang, X. P., Xie, W. P., Zheng, F. L., Li, H. Q., et al. (2021). Response of soil characteristics and bacterial communities to nitrogen fertilization gradients in a coastal salt-affected agroecosystem. Land Degradation Dev. 32, 338–353. doi: 10.1002/ldr.3705
Yu, Jb, Ning, K., Yz, Li, Sy, Du, Han, Gx. (2014). Wet and dry atmospheric depositions of inorganic nitrogen during plant growing season in the coastal zone of yellow river delta. World J. 2014, 949213. doi: 10.1155/2014/949213
Zhang, J., Ai, Z., Liang, C., Wang, G., Xue, S. (2017). Response of soil microbial communities and nitrogen thresholds of bothriochloa ischaemum to short-term nitrogen addition on the loess plateau. Geoderma 308, 112–119. doi: 10.1038/s41467-022-31936-7
Zhang, S., Liu, F., Liu, Q., Liu, Y., Li, D. (2015). Characteristics of N:P stoichiometry and the adaptation strategies for different coastal wetland communities in the yellow river delta. Chin. J. Ecol. 34, 2983–2989. doi: 10.13292/j.1000-4890.2015.0287
Zhao, Y. H., Li, T., Liu, J. H., Sun, J. K., Zhang, P. (2022b). Ecological stoichiometry, salt ions and homeostasis characteristics of different types of halophytes and soils. Front. Plant Sci. 13. doi: 10.3389/fpls.2022.990246
Zhao, Y., Li, T., Shao, P., Sun, J., Xu, W., Zhang, Z. (2022a). Variation in bacterial community structure in rhizosphere and bulk soils of different halophytes in the yellow river delta. Front. Ecol. Evol. 9. doi: 10.3389/fevo.2021.816918
Zhao, J., Xie, H., Zhang, J. (2020). Microbial diversity and physicochemical properties of rhizosphere microenvironment in saline-alkali soils of the yellow river delta. Acta Scientiae Circumstantiae 41 (03), 1449–1455. doi: 10.13227/j.hjkx.201908044
Keywords: Phragmites communis, bacterial community, Yellow River Delta, nitrogen to phosphorus ratio, rhizosphere soil
Citation: Zhang Z, Sun J, Li T, Shao P, Ma J and Dong K (2023) Plants changed the response of bacterial community to the nitrogen and phosphorus addition ratio. Front. Plant Sci. 14:1168111. doi: 10.3389/fpls.2023.1168111
Received: 17 February 2023; Accepted: 15 March 2023;
Published: 27 March 2023.
Edited by:
Xiao Guo, Qingdao Agricultural University, ChinaReviewed by:
Feng Li, Institute of Subtropical Agriculture (CAS), ChinaZhongsheng Zhang, Key Laboratory of Mollisols Agroecology, (CAS), China
Copyright © 2023 Zhang, Sun, Li, Shao, Ma and Dong. This is an open-access article distributed under the terms of the Creative Commons Attribution License (CC BY). The use, distribution or reproduction in other forums is permitted, provided the original author(s) and the copyright owner(s) are credited and that the original publication in this journal is cited, in accordance with accepted academic practice. No use, distribution or reproduction is permitted which does not comply with these terms.
*Correspondence: Jingkuan Sun, c3VuamluZ2t1YW5AMTI2LmNvbQ==