- 1Camphor Engineering Technology Research Center for National Forestry and Grassland Administration, Jiangxi Academy of Forestry, Nanchang, China
- 2College of Life Sciences, Nanjing Agricultural University, Nanjing, China
Drought stress (DS) is a potential abiotic stress that is substantially reducing crop productivity across the globe. Likewise, salinity stress (SS) is another serious abiotic stress that is also a major threat to global crop productivity. The rapid climate change increased the intensity of both stresses which pose a serious threat to global food security; therefore, it is urgently needed to tackle both stresses to ensure better crop production. Globally, different measures are being used to improve crop productivity under stress conditions. Among these measures, biochar (BC) has been widely used to improve soil health and promote crop yield under stress conditions. The application of BC improves soil organic matter, soil structure, soil aggregate stability, water and nutrient holding capacity, and the activity of both beneficial microbes and fungi, which leads to an appreciable increase in tolerance to both damaging and abiotic stresses. BC biochar protects membrane stability, improves water uptake, maintains nutrient homeostasis, and reduces reactive oxygen species production (ROS) through enhanced antioxidant activities, thereby substantially improving tolerance to both stresses. Moreover, BC-mediated improvements in soil properties also substantially improve photosynthetic activity, chlorophyll synthesis, gene expression, the activity of stress-responsive proteins, and maintain the osmolytes and hormonal balance, which in turn improve tolerance against osmotic and ionic stresses. In conclusion, BC could be a promising amendment to bring tolerance against both drought and salinity stresses. Therefore, in the present review, we have discussed various mechanisms through which BC improves drought and salt tolerance. This review will help readers to learn more about the role of biochar in causing drought and salinity stress in plants, and it will also provide new suggestions on how this current knowledge about biochar can be used to develop drought and salinity tolerance.
Introduction
Abiotic stresses are a serious threat to crop productivity and global food security. The intensity of abiotic stresses (drought, heat, heavy metals, and salinity) is continuously increasing, which is negatively affecting crop productivity (Hassan et al., 2021). Among these abiotic stresses, drought and salinity stress (SS) are serious abiotic stresses that are responsible for a substantial reduction in crop yields across the globe (Singh and Takhur, 2018). Drought stress (DS) is a serious abiotic stress responsible for a substantial reduction in crop productivity (Liang et al., 2020; Latif et al., 2022). Drought stress disturbs various functions from morphological levels to physiological and anatomical levels (Figure 1; Zhao et al., 2022). DS increases leaf senescence and decreases chlorophyll synthesis, which leads to a substantial decline in photosynthesis and crop productivity (Sunaina et al., 2019; Ma et al., 2021). DS also induces the overproduction of ROS (Vijayaraghavareddy et al., 2022), which damages proteins, lipids, DNA, and enzymatic reactions (Cui et al., 2017). Besides this, DS also negatively affects physiological processes and impacts agronomic traits, which cause a decline in grain productivity (Zhou et al., 2007). However, yield losses largely depend on the severity and duration of DS and plant species (Agarwal et al., 2016). Moreover, DS also causes stomata to close which decreases the conductance of stomata to the loss of water and negatively affects photosynthesis and transpiration rates (Mahmoud and Swaefy, 2020). Additionally, DS causes the destruction of enzymes and proteins along with a reduction in the synthesis of chlorophyll, which in turn causes a marked reduction in photosynthesis and, subsequently, plant productivity (Mahmoud et al., 2022a; Mahmoud et al., 2022b).
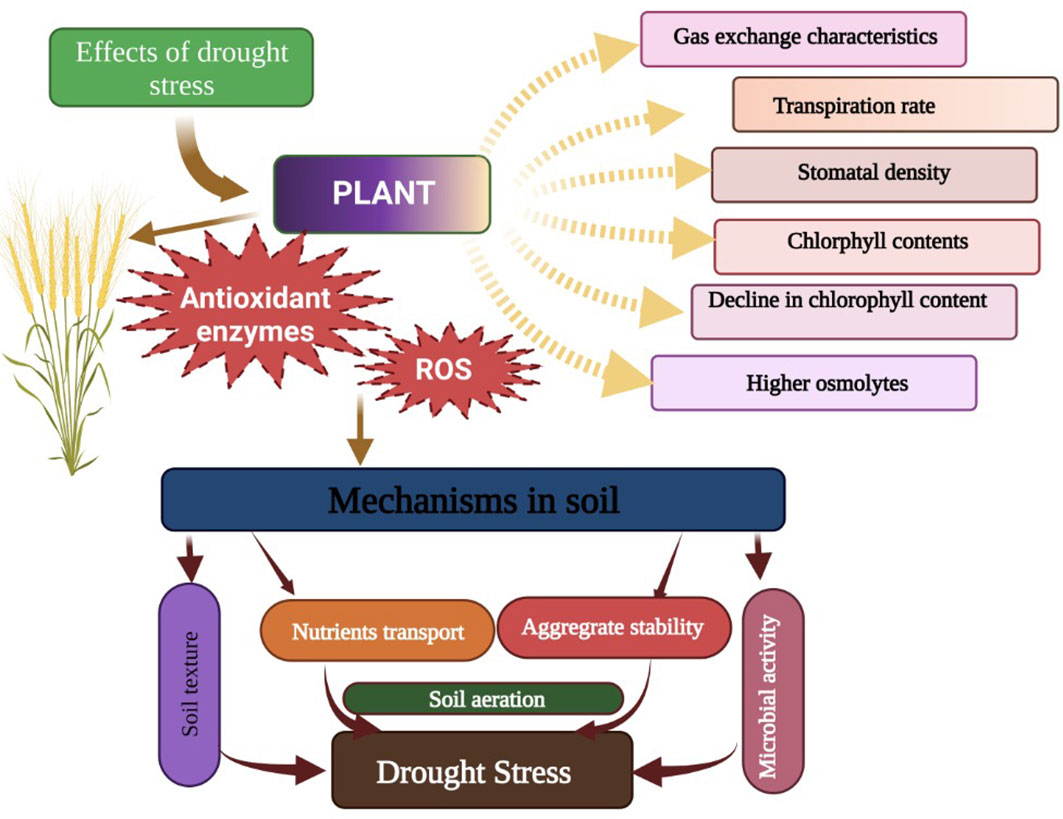
Figure 1 Effects of drought stress on plants. Drought stress disturbs the gas exchange characteristics, transpiration rate, stomata density, chlorophyll contents, soil microbial activities, soil aeration, and nutrients, thereby leadings to a significant reduction in plant growth.
Salinity stress is continuously increasing globally, and it has been reported that 900 million hectares around the globe are salt-affected (Hernández-Herrera et al., 2022). By the end of 2050, half of the arable land will be damaged by SS (Denaxa et al., 2022) owing to the continuous accumulation of salts due to fertilization, salty irrigation, and climate change (Denaxa et al., 2022). Salinity stress inhibits the germination, growth, development, and productivity of plants in both dry and irrigated regions (Sultan et al., 2021). Salinity stress is an intense abiotic stress that negatively affects plant physiological and biochemical processes and leads to a serious reduction in growth and yield (Sofy et al., 2020).
Salinity stress limits germination, growth, and development by inducing osmotic stress and pseudo-drought stress (Parida and Das, 2005). The increased concentration of salts in the root zone under SS causes metabolic disorders, affects the photosynthetic efficiency, and assimilates production, which resultantly affects plant growth rate (Ghaderi et al., 2018; Mushtaq et al., 2022; Raza et al., 2022). Salinity stress also disrupts the ionic balance in cells and leads to excessive production of reactive oxygen species (ROS) (Hasanuzzaman et al, 2021), which causes oxidation of crucial molecules such as membranes, lipids, proteins, and carbohydrates (Table 1), alters redox homeostasis, and hinders the plant growth (Aref et al., 2016; Sultan et al., 2021). Moreover, SS also increases electrolyte leakage and MDA accumulation, and it also disturbs nutrient uptake, thereby reducing plant growth (Sultan et al., 2021). Therefore, as a matter of global food security, solutions must be put forth to reclaim and treat salt-affected soils to support better plant growth and productivity under SS conditions (Hernández-Herrera et al., 2022).
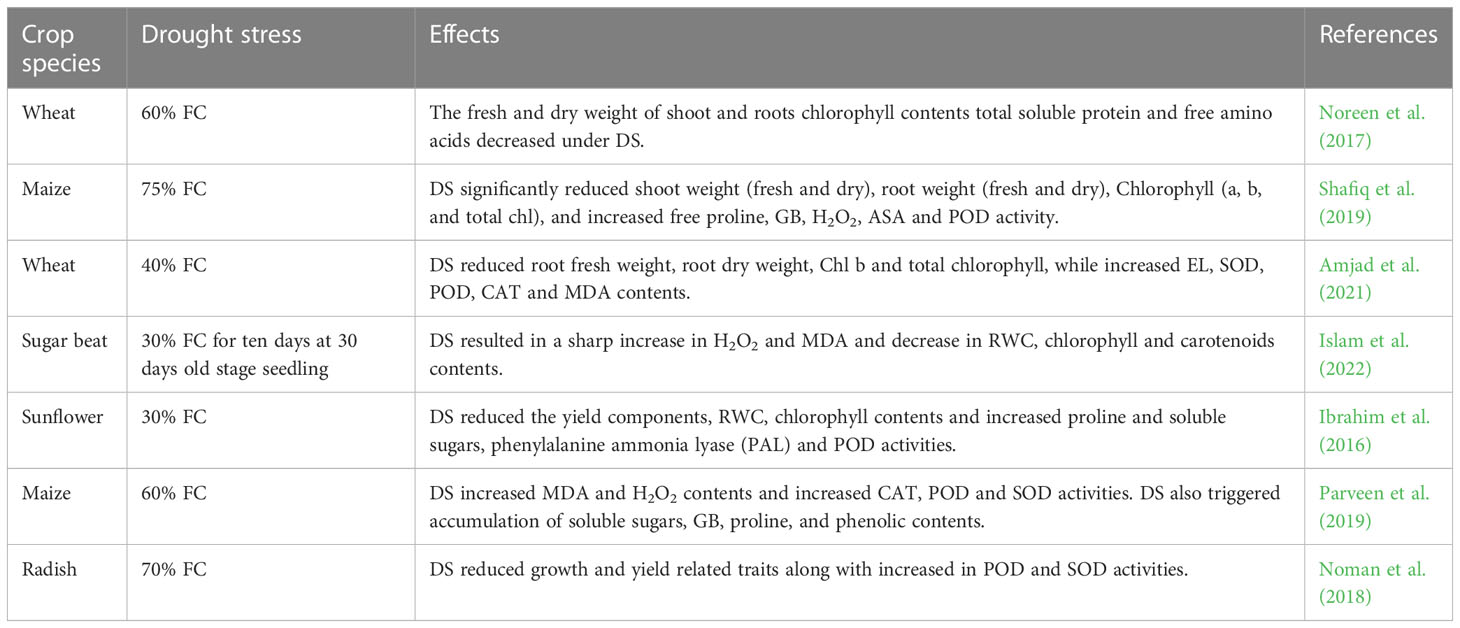
Table 1 Effect of drought stress on growth, physio-biochemical process, and antioxidant activities of various crops.
Biochar (BC) has emerged as an excellent tool to improve crop productivity (Palviainen et al., 2020; Moragues Saitua et al., 2023) and tolerance to abiotic stresses (Tang et al., 2022). It has a high cation exchange capacity and an alkaline nature, which makes it an important amendment for the reclamation of salt-affected soils (Lehmann and Joseph, 2009; Lashari et al., 2013). The application of BC reduced the harmful impacts of SS by improving soil physiochemical and biological processes and Na leaching (Dahlawi et al., 2018). Biochar addition to salt-affected soils improves potassium (K+) uptake and reduces Na+ uptake, which in turn improves plant performance under SS (Drake et al., 2016; Usman et al., 2016). Recently, BC has also been identified as an important tool to improve crop productivity and water use efficiency (WUE) (Singh et al., 2019). The application of BC-enhanced nutrient uptake, carbon assimilation, and antioxidant activities, therefore, leads to an appreciable increase in plant growth under DS (Sorrenti et al., 2016; Wang et al., 2018; Wang et al., 2020). Moreover, BC also improves chlorophyll synthesis, WUE, and stomata conductance, thereby improving plant growth under DS (Paneque et al., 2016; Ramzani et al., 2017; Langeroodi et al., 2019; Haider et al., 2020). Further, BC application also improves soil physiochemical and biological properties that also induce favorable impacts on plant growth under DS (Agbna et al., 2017). Therefore, in this review, we have presented information on various mechanisms of BC in mitigating drought and salinity stresses in plants. We also identified the various research gaps that must be filled to realize the promising future of biochar as a soil amendment. This is the first detailed review of the role of BC in mitigating salinity and drought stress, and it will provide better insights into existing knowledge of BC in improving plant tolerance to both SS and DS.
Why biochar is an important amendment
Biochar (BC) is a carbon-rich material that can be used as a soil conditioner to improve soil carbon sequestration and soil quality. Biochar is prepared from organic materials through a pyrolysis process (250–700°C; Rajakumar and Sankar, 2016), and it has various unique and special properties that make it an efficient, environment-friendly, and economical source of soil conditioner (Oliveira et al., 2017). Biochar is a porous and fine-grained material, and it has a similar appearance to charcoal; however, the only difference between the two is their utilitarian intention (Kapoor et al., 2022). The characteristics of BC depend on feedstock and pyrolysis conditions (Bird, 2015; Agegnehu et al., 2017). The pyrolysis temperature makes a difference in the properties of BC. For instance, Jindo et al. (2014) prepared the BC at different temperatures (400, 500, 600, 700, and 800°C), and they found that the BC obtained at 600°C has high recalcitrant characteristics as compared to the BC obtained at other temperatures (Kapoor et al., 2022). BC has a porous structure with many pores, which provides excellent habitat for soil microbes (bacteria, action-myocytes, and AMF) to colonize, grow, and reproduce, which in turn improves soil health and plant performance (Kapoor et al., 2022). Moreover, BC also improves soil nutrient holding capacity, water uptake efficiency, soil organic matter, and soil physiochemical and biological properties, which improve plant growth (Minamino et al., 2019; Ohtsuka et al., 2021; Kapoor et al., 2022; Qu et al., 2022).
Biochar production and important feed stocks for biochar preparation
Biochar is produced through the pyrolysis process, which involves the heating of biomass in the complete or near absence of oxygen. During the pyrolysis process, oil, char, and gases are produced; however, processing conditions largely affect the quantity of these materials (Kapoor et al., 2022). The chemical composition of feedstock is reflected in the composition of BC, which also defines BC behavior, function, and fate in soil (Kapoor et al., 2022). Secondly, the extent of physio-chemical properties undergone by biomass during pyrolysis also depends on pyrolysis conditions like residence time and temperature (Verheijen et al., 2009). Globally, different materials, including wood, nut shells, husks, manures, and crop residues, are being used as feedstock to prepare the BC (González et al., 2009). Similarly, other feedstocks, including sewage sludge and municipal wastes, are also being used to prepare the BC; however, a risk is associated with the use of these materials owing to the presence of heavy metals (HM) in these feedstocks (Kapoor et al., 2022). The biomass with high mineral contents, like grasses, grain husks, and straw residue, produce BC rich in ash (Demirbas, 2004). Additionally, pyrolysis of wood-based feedstocks produces coarser and more resistant BC with carbon contents up to 80% (Winsley, 2007). Therefore, BC application could be an effective approach to improving soil health and plant productivity (Brown, 2009).
Biochar a promising amendment to mitigate drought stress
Biochar has emerged as an excellent tool to mitigate the deleterious effects of drought stress (Siebielec et al., 2020). Biochar, being the black gold of agriculture, has received significant attention in recent times to offset the negative effects of DS (Zhang et al., 2019). The BC amendment increases SOC, soil moisture contents, water and nutrient uptake, CEC, and aggregate stability, which helps with drought tolerance (Zheng et al., 2018b; Odugbenro et al., 2020).
Biochar maintains membrane stability and plant water relations under drought stress
Drought stress induces harmful effects on plasma membranes, and it also causes dehydration of the cytoplasm (Figure 1), which consequently increases electrolyte leakage and lipid peroxidation (Table 2, Hassan et al., 2021). The BC and chitosan addition enhanced DS tolerance in barley plants by decreasing EL and lipid peroxidation by improving membrane stability, RWC, and water pressure (Hafez et al., 2020). In another study, Medicago ciliaris plants grown under DS treated with BC showed a significant reduction in MDA concentration. Likewise, Yildirim et al. (2021) also found that BC decreases MDA accretion in Brassica oleracea by increasing the activity of antioxidant enzymes (Yildirim et al., 2021). There is a controversial role of BC on plant water status; for example, it was noted that BC improved the WUE and biomass production, but did not improve the RWC (Table 2). The assumption is that BC improved the plant nutrition status and increased the K uptake, which enhanced the stress resistance (Mannan et al., 2021).
However, some authors noticed that BC application not only improved the WUE, but it also improved soil water holding capacity, and consequently plant water status (Laird et al., 2010; Licht and Smith, 2017). Likewise, another group of authors also noticed that BC amendment in sandy loam enhanced the plant available water (PAW) and improved RWC under DS (Aller et al., 2017). In another study, Licht and Smith (2017) found that BC application to frozen soil mitigated the adverse effect of DS by improving soil water contents, photosynthesis, leaf transpiration, and water under water stress conditions. Moreover, in maize plants, it was also reported that BC applications (2% and 3%) appreciably improved the leaf water potential and photosynthesis under DS (Ahmed et al., 2016). Haider et al. (2015) also found that BC application improved the RWC in poor sandy soil, whereas Lyu et al. (2016) revealed that BC addition strengthened antioxidant activities and plant water relations; however, they also found that BC response to DS varies according to plant species, soil, and BC type. In conclusion, BC application improves water uptake and RWC and thereby improves drought tolerance by increasing plant physiological functioning.
Biochar improves nutrient uptake and maintain nutrient homeostasis under drought stress
Drought stress disturbs nutrient homeostasis and causes significant growth and yield losses. However, BC, being a promising soil amendment, improves nutrient homeostasis and leads to an appreciable increase in plant growth. For instance, BC applications (0.75% and 1.5%) improved the N uptake, which could be due to a BC-mediated increase in N retention in soil (Muhammed et al., 2020). Similarly, Ibrahim et al. (2020b) suggested that BC effectively works as a slow-release N fertilizer and improves plant performance under DS. In addition, BC-mediated increase in N uptake is linked with improved CEC, as soil with a higher CEC has a better ability to NH4+ and N utilization (Liang et al., 2014). Glaser et al. (2002) also noted that BC application improves Ca, Mg, and K concentrations in soil solution, which in turn increases the availability of nutrients under DS.
Further, according to Van Zwieten et al. (2010), BC increases soil nutrient availability by affecting soil pH (Figure 2). In another study, it was found that BC applications (0.75% and 1.5%) improved the N uptake and mitigated the adverse effects of DS on peanuts by improving the N uptake (Zhang et al., 2021). Zoghi et al. (2019) found that BC application improves nutrient uptake, possibly by increasing soil WUC and WUE under DS conditions. Egamberdieva et al. (2017) reported that BC in combination with Bradyrhizobium substantially improved growth and NP uptake as compared to control conditions. Likewise, Liu et al. (2017) noted that BC (birch wood, 500°C) in combination with Rhizophagus irregularis increased the leaf area, N and P uptake, and WUE; however, BC in combination with R. irregularis had no significant impact on soil pH and root biomass under DS.
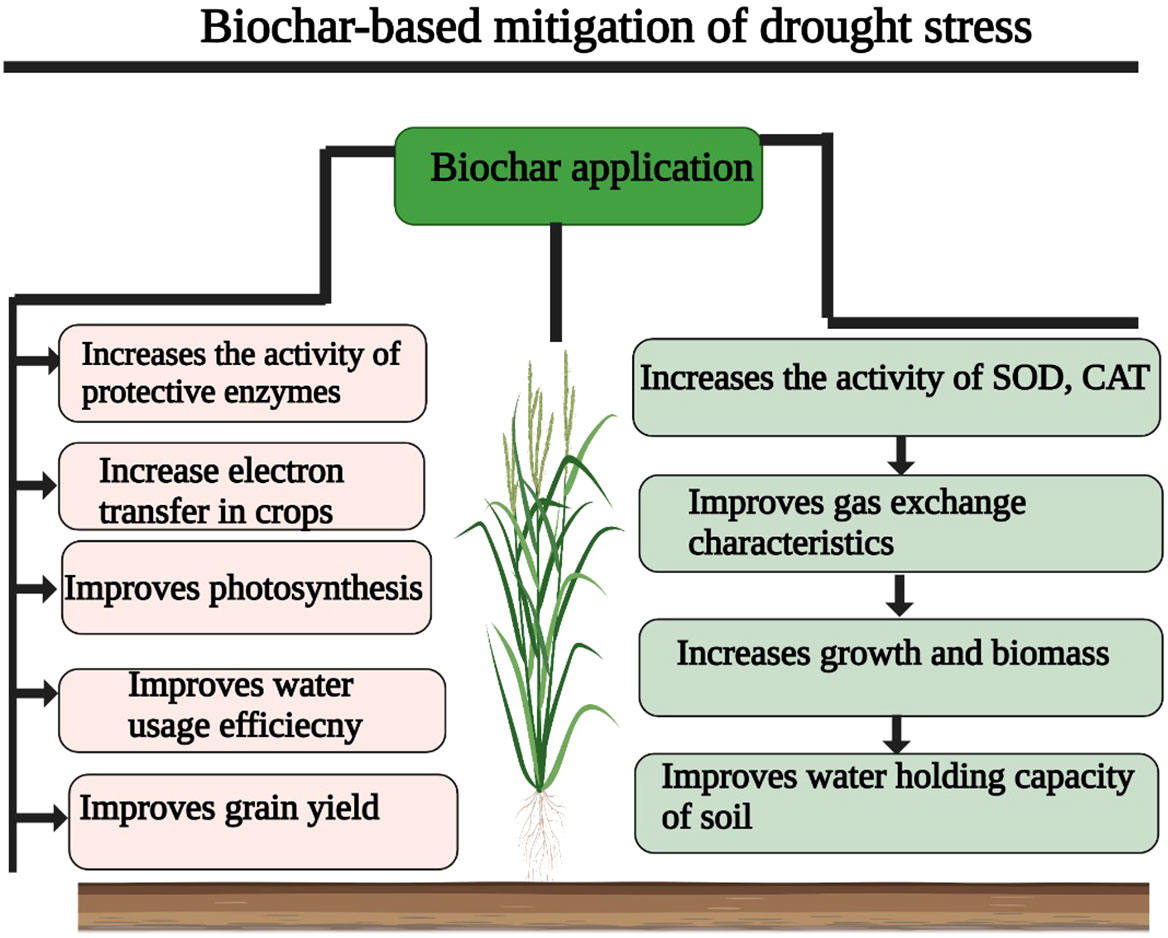
Figure 2 Biochar application increases antioxidant activities, membrane stability, photosynthesis, water use efficiency, gas exchange characteristics, water holding capacity, genes expression, and osmolytes accumulation and leads to a significant increase in drought tolerance.
Şahin et al. (2016) found that BC enhanced N concentration, whereas Durukan et al. (2020) exposed the sugar beet plants to DS and BC. They found a higher concentration of P under 100% FC (field capacity) with 0.5% BC; however, P concentration was decreased with a parallel increase in BC application, which indicates that the rate of BC plays a significant role in nutrient uptake under DS. Langeroodi et al. (2019) treated the pumpkin plants with different DS levels (45%, 60%, 75%, and 90%) and BC rates (0, 5, 10, and 20 t ha−1). They found that Mg concentration was increasing with BC rate, and higher Mg concentration was noted under medium DS with 20 t ha−1. In another study, Poormansour et al. (2019) reported that increasing the rate of BC application (0%, 1.25%, 2.5%, 3.75%, and 5%) to faba bean plants under diverse irrigation levels (100%, 75%, and 50% of the water requirement) increased the Ca and Mg uptake and concentration in soil. To summarize, BC increased the CEC and nutrient uptake, which in turn improved drought tolerance by favoring a substantial increase in antioxidant activity and physiological functioning.
Biochar protects photosynthetic apparatus and improves photosynthesis under drought stress
The devastating impact of DS on photosynthesis contributes to a reduction in chlorophyll synthesis, leaf area, and electron transport. It is well established that BC application improves the synthesis of growth-regulating substances, which improve plant functioning under DS (Manolikaki and Diamadopoulos, 2019). BC addition supports water deficit conditions by increasing chlorophyll contents and antioxidant activities (Lyu et al., 2016). Previously, it has been reported that BC amendment buffers the effects of DS on carbon assimilation and photosynthesis, which is linked with boosted chlorophyll synthesis and a less pronounced reduction in stomata conductance (Zainul et al., 2017; Wang et al., 2021).
Under DS conditions, BC application enhanced the WUE, which in turn improved the net photosynthetic rate and reduced non-stomatal limitations (Paneque et al., 2016). Likewise, BC application mitigated the adverse effect of DS by improving WUE, stomata conductance, and chlorophyll synthesis in cowpea, okra, and tomato plants (Farooq et al., 2021). Recently, it has also been reported that BC treatment increases productivity and reduces ROS damage by increasing chlorophyll synthesis and photosynthetic rate, and lowering electrolyte leakage (Gharred et al., 2022). Besides, this BC also brings ultra-structural changes to improve photosynthesis. For instance, BC application improved the stomata length, as well as the width and density of stomata, which significantly improved WUE and photosynthetic rate under DS conditions (Akhtar et al., 2014; Khan et al., 2021).
The application of BC increased the RWC, which improved the transpiration and photosynthetic rates as well as leaf osmotic potential under DS conditions (Haider et al., 2015). Kammann and Graber (2015) studied the impact of DS on drought tolerance in quinoa plants. They found that BC improved the soil properties, leaf water status, and photosynthesis and led to an appreciable increase in overall plant photosynthetic efficiency and assimilation production under water-scarce conditions (Haider et al., 2015; Ahmed et al., 2016; Lyu et al., 2016). The BC application to poor sandy soil improves plant water relations, photosynthesis, and growth by reducing toxic effects, or ROS (Haider et al., 2015; Table 3). In another study, Lyu et al. (2016) noted that BC application improves electron transport and enzymatic activities, by reducing the damaging effects of DS on the photosynthetic apparatus. In essence, BC protects the photosynthetic apparatus from drought-induced oxidative stress and increases the synthesis of photosynthetic pigments, thus resulting in a significant increase in photosynthesis under drought stress.
Biochar maintains osmolytes accumulation and hormonal balance under drought stress
Osmolytes play an indispensable role against DS; however, it has been reported that DS disturbs the hormonal balance and osmolyte accumulation. Proline produced under stress conditions works as an ROS scavenger as well as for osmotic adjustment. For instance, DS in M. ciliaris leaves significantly increased the proline accumulation; however, findings of Yildirim et al., 2021 showed that BC-treated plants reduced the proline contents, possibly due to less ROS production and reduced oxidative and osmotic stresses in BC-amended plants. In another study, BC in combination with chitosan markedly reduced the soluble sugars, sucrose, and starch contents in stressed and controlled barley plants (Hafez et al., 2020). In a study, Gullap et al. (2022) found that BC application and irrigation levels significantly affected the ABA, IAA, and GA contents of soybean plants. These authors noted that IAA and GA contents were reduced, whereas ABA contents were increased under DS. Nonetheless, BC also increased the IAA and GA and decreased the ABA contents of soybean-treated plants (Gullap et al., 2022). Thus, BC maintains hormones and osmolyte accumulation, which protect the plants from drought-induced oxidative stress and substantially improve drought tolerance.
Biochar improves antioxidant systems and detoxify ROS under drought stress
Drought stress induces oxidative stress by increasing ROS that damage the major molecules of plants. For instance, an increase in SOD activity in the water-stressed plant was linked with improved photoprotection and an increase in membrane stability (Gharred et al., 2022). BC application strengthens antioxidant activities; for instance, an increase in SOD and APX activity in control and water-stressed plants was observed with BC application (Gharred et al., 2022). BC application buffers the toxic effects of DS on the plant photosynthetic apparatus by regulating electron transport and antioxidant activity (Chaves et al., 2009). Foyer et al. (2009) found an increase in the AsA/DHAsA ratio and SOD, APX, GPX, and GR activities at DS, which was not sufficient to counter the effects of DS (Table 4). However, BC application (2%) under DS increased the AsA/DHAsA ratio, SOD, APX, GPX, and GR activities and encountered the toxic effects of DS through ROS scavenging (Foyer et al., 2009).
Khan et al. (2021) found that BC application markedly improved the TSS and TSP contents, while BC proline contents indicated that BC could reduce the harmful effects of DS (Khan et al., 2021). Moreover, it was observed that BC in combination with AMF enhanced drought tolerance by improving osmotic adjustments, hormonal balance, and antioxidant activity (Mickan et al., 2016). Biochar addition to soil improves CAT, POD, and SOD activity (Table 5) by improving plant metabolic functioning, cell growth, and reducing ROS production, which results in substantial improvement in plant performance under DS (Zulfiqar et al., 2022). Additionally, barley plants treated with BC and chitosan under DS showed a marked improvement in CAT, POD, and GR activities, which resultantly reduced the drought-induced oxidative damage on barley plants (Hafez et al., 2020). In conclusion, a BC-mediated increase in antioxidant activities reduces ROS and protects the plants from the deleterious impacts of drought stress, therefore improving plant growth under drought stress.
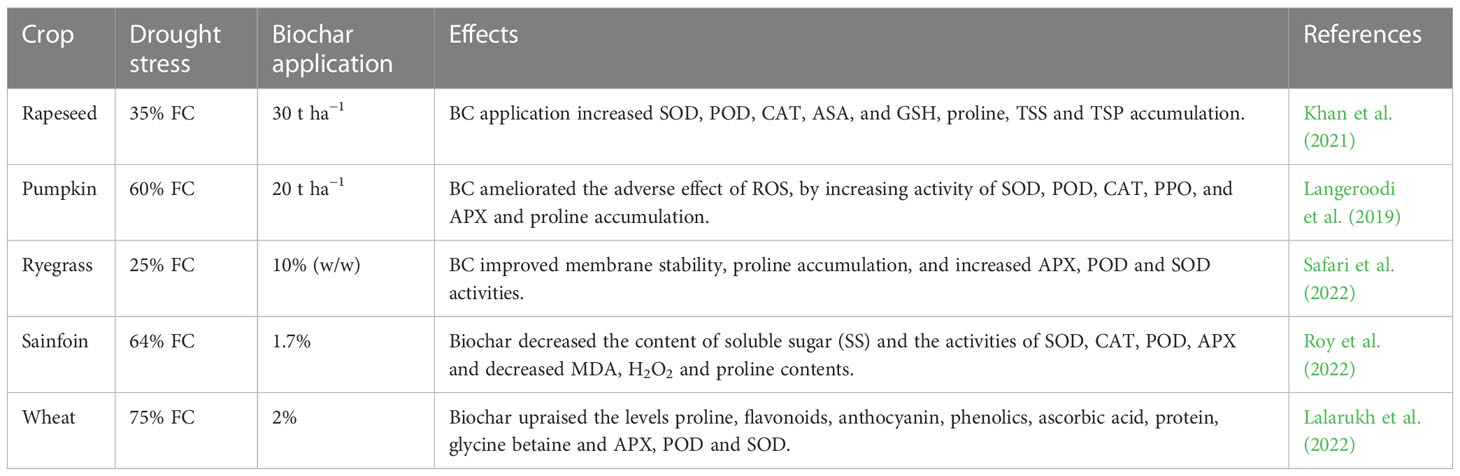
Table 5 Effect of biochar on accumulation of various osmolyte and antioxidant activities under drought stress.
Biochar improves genes expression and stress responsive proteins under drought stress
BC application also improves gene expression to induce drought stress in plants. For instance, plants showed increased expression of CAT, APX, and Mn-SOD genes under 50% FC as compared to 75% and 100% FC; nonetheless, BC and vermin-compost applications reduced the expression level of CAT, APX, and MnSOD genes under all irrigation levels (Hafez et al., 2021). Conversely, BC application increased the expression of all these genes under DS (Racioppi et al., 2019). BC application also activates the auxin-responsive growth-promoting pathway, which stimulates the germination and growth of wheat plants treated with BC (Vissenberg et al., 2005). Xyloglucan endotransglucosylase/hydrolase (XTH) genes are involved in controlling the extensibility of the cell wall during plant growth stimulated by GAs and IAA (Sánchez-Rodríguez et al., 2010). The application of BC increases the expression of the XTH gene in the Saragolla cultivar, which stimulates the plant synthesis of GAs after BC treatment (Racioppi et al., 2019). Viger et al. (2015) found that modifications in soil pH and increase in K+ availability in BC-treated soil activate Ca2+ and ROS-mediated cell signaling, which in turn stimulate the IAA and BR growth-promoting pathways.
Biochar nutrition improves plant growth, yield, and quality under drought stress
Drought stress reduces the growth of plants by decreasing photosynthesis, nutrient uptake, and increasing ROS production. However, BC application improves growth and biomass production by improving plant nutrition, antioxidant activities, and osmolytes accumulation (Gharred et al., 2022). BC application increased the leaf area of okra and maize plants (Batool et al., 2015; Haider et al., 2015) under DS and it also increased the biomass of wheat grown in the semi-arid Mediterranean (Olmo et al., 2014). BC-mediated increases in plant leaf area do good and constant supply of nutrients to plants by alleviating DS (Laird et al., 2010; Zhang et al., 2021).
Plants use different mechanisms including signaling pathways, gene expression, and accumulation of proteins and enzymes to cope with DS. It has been reported that DS increased the protein of M. ciliaris (Gharred et al., 2022) and they were further increased by the application of BC. BC application also increased the vegetative growth, seed production, and quality of sunflower plants grown under DS (Paneque et al., 2016). Likewise, another group of authors also found that BC application improved the growth, yield, and quality of tomato and rapeseed plants under DS (Bamminger et al., 2016; Agbna et al., 2017). Haider et al. (2015) noted that BC could improve the growth of water stresses plants by increasing soil-plant water relationships and photosynthesis. Egamberdieva et al. (2017) found that BC with Bradyrhizobium showed a significant increase in growth and biomass production and N and P concentration in lupin plants.
In another study, it was found that BC application (0–30 t ha−1) improved biomass and yield while BC application at the rate of 60 t ha-1 had adverse impacts and it negatively affected the rapeseed growth and seed production under DS. Similarly, BC application (0–30 t ha−1) improved the biomass, pods/plant, and 1,000 seed weight by 56%, 26%, and 15% and control conditions while BC application improves biomass, pods/plant, and 1,000 seed weight by 23%, 32% and 21% in drought conditions (Khan et al., 2021). DS also negatively affected the oil and protein contents; however, BC treatment led to a marked improvement in oil and protein contents under DS (Khan et al., 2021). Drought also increased the erucic acid contents, while BC application significantly decreased the erucic acid contents. Drought stress also led to a significant decrease in oleic acid; nonetheless, biochar appreciably improved oleic acid concentration under DS conditions. Similarly, compared to control conditions BC application (0–30 t ha−1) also showed a significant increase in linoleic acid under normal and DS conditions (Khan et al., 2021). Thus, the BC-mediated increase in growth and yield is linked with an increase in photosynthetic performance, antioxidant activities, gene expression, and osmolyte accumulation.
Biochar improves soil properties to induce drought tolerance
Biochar has emerged as an excellent tool to improve soil health and crop productivity. It has been reported that BC improves soil physical properties, including soil density, soil moisture content, and aggregate stability under DS (Ye et al., 2016; Bamminger et al., 2016; Zhang et al., 2017). Zhang et al. (2017) reported that BC improved the soil properties and abundance of bacteria, which contributed to a substantial increase in the stress tolerance ability of tobacco plants. Likewise, other authors also noted that BC application improved soil bulk density, WHC, and water retention, which led to a significant increase in drought tolerance (Abel et al., 2013; Liang et al., 2014). Moreover, BC also improves aggregate stability in coarse-textured soils and soil WHC; both factors play a critical role in soil plant growth (Lehmann et al., 2011; Foster et al., 2016).
BC-mediated increase in soil WHC is due to the porous structure of BC and the higher CEC of BC (Laghari et al., 2016). Soil microbial biomass (SMB) plays an imperative role in OM decomposition. Higher SMB improves nutrient availability and soil fertility, and it also works as the linkage between the source and sink of soil nutrients (Marschner et al., 2015). Drought stress induces osmotic stress, which causes microbial death and a reduction in SMB (Sanaullah et al., 2011). Drought-mediated decrease in SMB decreases the decomposition of OM under DS Hailegnaw et al. (2019); however, BC has been reported to increase microbial activity, OM, and nutrient contents, therefore improving the SMB, which in turn improves soil fertility and plant growth (Cornelissen et al., 2018). Moreover, BC also increases soil organic carbon, which increases soil microflora and soil enzymatic activities that positively affect plant performance (Rahman et al., 2018; Rahman et al., 2021). DS imposes negative effects on soil biological properties, and BC substantially offsets these negative impacts and improves soil biochemical properties. For instance, BC application (38 g kg−1) appreciably improved the soil P (18.72%), K (7.44%), soil carbon (11.86%), nitrogen mineralization (16.35%), and soil respiration (6.37%), which in turn increased the soil microbial activities compared to the control and lower rates (28 g kg−1) of BC application (Zaheer et al., 2021). Therefore, a BC-mediated increase in OM, CEC, WHC, water retention, and bulk improves drought stress by bringing about favorable changes in plant functioning.
How does biochar improve salinity tolerance?
Soil salinity represents the most critical constraint to crop productivity and global food security (Farouk et al., 2020; Sofy et al., 2020). Soil salinization is continuously increasing, which is posing a serious threat to crop productivity and food security (FAO, 2017). The area under soil salinity is significantly increasing, and salinity stress causes 27.2 billion USD annual losses in irrigated agriculture (Munns and Gilliham, 2015). It has been documented that 20% of cultivated and 33% of irrigated land globally is affected by salinity stress (Machado and Serralheiro, 2017). Further, soil salinized area is increasing at a rate of 10% annually owing to anthropogenic activities, which pose a serious threat to food productivity (Jamil et al., 2011; Dadshani et al., 2019). Biochar is a carbon-rich product used as an important soil conditioner to improve soil quality and plant performance (Ali et al., 2021). Further, BC application brought favorable changes in soil and plant functioning to improve SS tolerance (Ibrahim et al., 2020; Ali et al., 2021). The various mechanisms by which BC improves salinity tolerance are presented below.
Biochar maintains membrane stability and plant water relations under salinity stress
Salinity stress negatively affects cellular membranes, and it damages the membranes by increasing MDA accumulation (Guo et al., 2019). The membrane damage due to SS also leads to the loss of important solutes (Mata-Pérez et al., 2015). The concentration and composition of fatty acids and lipids have a strong impact on membrane functioning, stability, and fluidity. For instance, unsaturated fatty acids play a critical role in membrane protein activity and membrane protection (Mikami and Murata, 2003). The application of BC has been reported to increase the concentration of unsaturated fatty acids, which in turn improves the membrane stability under SS (Ndiate et al., 2021). The application of BC also improves antioxidant activities (APX, CAT, POD, SOD, and GR), which also protects the membranes from the damaging effects of SS (Kim et al., 2016).
Lashari et al. (2015) noted that the application of manure and BC application appreciably improved the membrane stability by decreasing MDA concentration (Figure 3). The application of BC also reduced electrolyte leakage and increased the relative water content (RWC) by making membranes stronger (Table 6) and protecting membranes from the toxic effects of Na+ (Ran et al., 2020). In another study, it was observed that BC addition improved membrane integrity by improving the concentration of unsaturated fatty acids and increasing the activities of antioxidant enzymes, which protect membranes from the damaging effects of oxidative stress (Ndiate et al., 2022). Biochar application also improves the leaf water status and protects the plants from the damaging effects of SS. For instance, it has been recorded that BC improved the leaf water status of rice at the heading and grain-filling stages as compared to no BC application (Ran et al., 2020). These authors further stated that BC application prevents membrane damage by decreasing Na+ content and increasing K+, which therefore improves the leaf water status under SS (Ran et al., 2020). In fact, BC improves the leaf water status by increasing K concentration, as K is considered an important osmoprotectant in plant tissues (Ran et al., 2020). The leaf RWC of plants is improved after BC application, resulting in an increase in WUE efficiency by plants (Naeem et al., 2017; Shabbir et al., 2021).
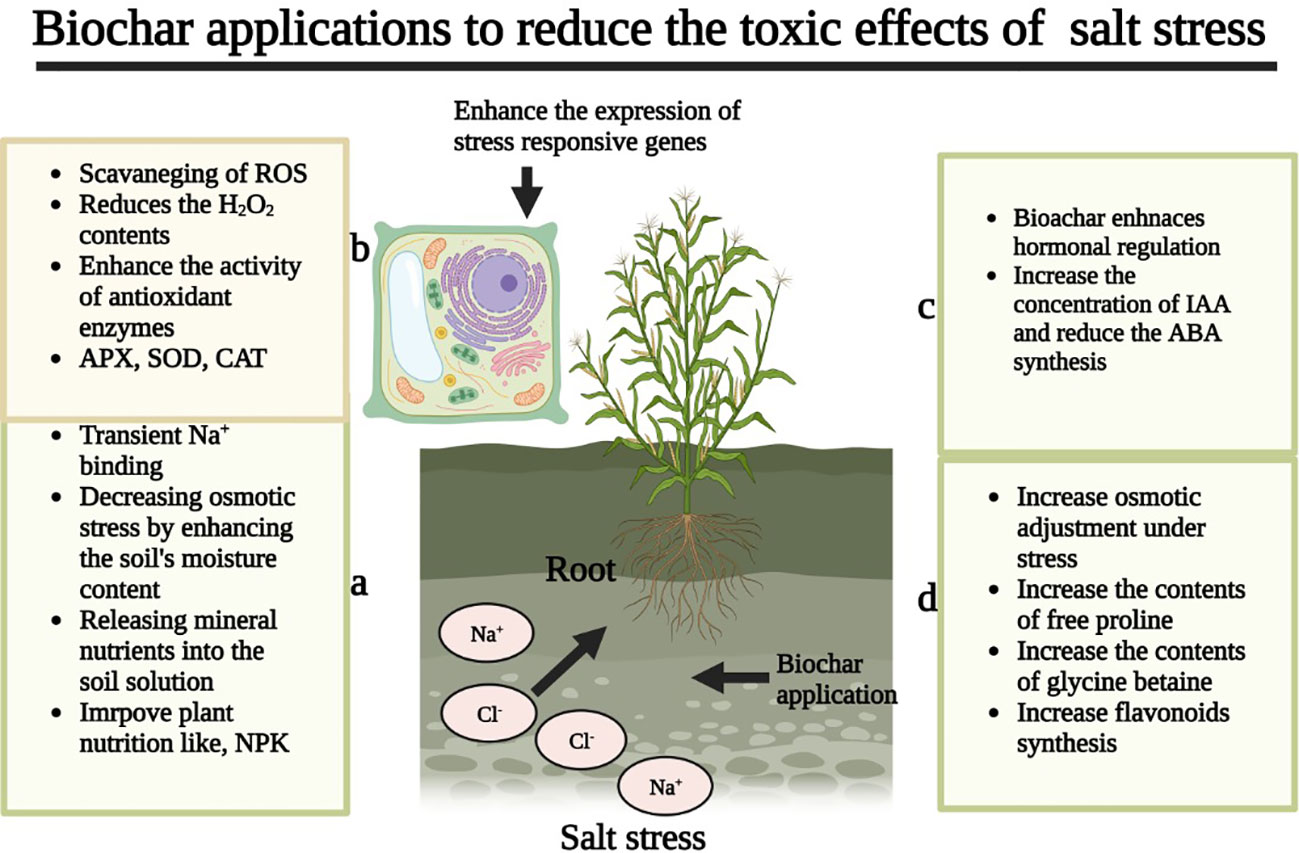
Figure 3 Biochar reduces ROS production, and increaseds membrane stability, antioxidant activities, osmolytes accumulation, gas exchange characteristics, soil properties, and nutrient uptake, and decreases the accumulation of toxic ions, thereby improve improving salinity tolerance.
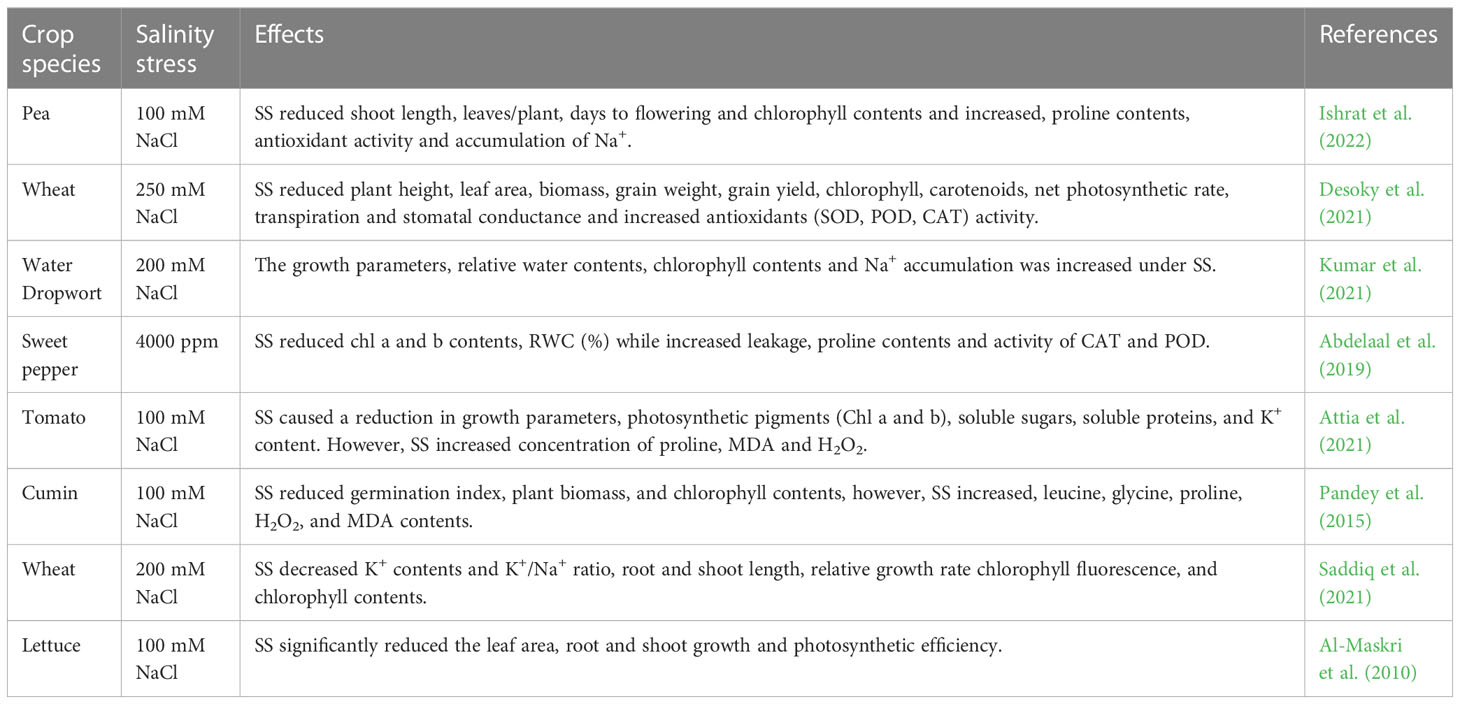
Table 6 Effect of salinity stress on growth, physio-biochemical process, and antioxidant activities of various crops.
Other authors also found that BC is an excellent strategy to improve the leaf RWC under SS. The application of BC improves water uptake and maintains the osmotic potential, which in turn improves the leaf RWC of plants growing under SS (Torabian et al., 2018; Soliman et al., 2022). Aquaporins play an imperative role in the transportation of water; for instance, PIPs not only improve the uptake and transportation of water to plant leaves and roots, but they also increase CO2 diffusion and ensure an abundant supply of substrates for photosynthesis as well as carbohydrate assimilation (Groszmann et al., 2017; Cui et al., 2021; Saibi, 2021). BC application increased carbon assimilation and upregulated AQP genes, which improved water uptake and leaf water status (Kayum et al., 2017). BC application has been reported to improve the activity of water transporter genes and improve the water-holding capacity of the soil, thereby improving the leaf water status under SS (Shashi et al., 2018; Soliman et al., 2022). Thus, BC reduces ROS, which ensures membrane protection and maintains better plant water relations under SS.
Biochar improves nutrient uptake and maintain nutrient homeostasis under salinity stress
Soil salinity negatively affects the accumulation and uptake of various macro and micronutrients. For instance, it was reported that salt-affected plants contained 35%, 61%, 59%, 12%, and 11% lower N, P, K, Ca, and Mg in leaf tissues as compared to the control treatment (Soliman et al., 2022). The application of BC promoted the uptake of nutrients under controlled and SS conditions. BC application improves Ca2+ content, which improves SS tolerance through modification of cellular signaling pathways (Qin et al., 2019). As a result, BC application increased the K+/Na+ ratio and Ca2+ by the Ca2+- dependent SOS pathway (Soliman et al., 2022). In another study, it was reported that BC application improved P and Mn concentrations in lettuce plants (Hammer et al., 2015).
In another study, it was reported that BC increased tissue P contents in a dose-dependent way (Kim et al., 2016), whereas Usman et al. (2016) also found that BC substantially increased the P, K, Fe, Mn, Zn, and Cu concentrations (Table 7) in tomato plants growing under SS. Nonetheless, wheat straw-based BC increased P precipitation and reduced the P concentration in plants growing under sodic soils (Xu et al., 2016). Similarly, Na+ uptake by plants was also reduced after BC application (Hammer et al., 2015), which indicates that ionic homeostasis in plants is improved after the application of BC (Akhtar et al., 2015a; Mansoor et al., 2021). Hence, BC application is an effective approach to reduce Na+ absorption and increase plant growth in saline soils (Mansoor et al., 2021).
The application of BC also leads to a slight increase in the EC of both normal and sodic soils, and an increase in this EC is linked with the release of various nutrients (Ca, K, and Mg) after the application of BC (Mehdizadeh et al., 2020). The higher quantity of Ca and mg released by BC displaces Na+ on soil exchange sites, which reduces the availability of Na+ for plants (Huang et al., 2019). The addition of BC hinders the uptake and accumulation of Na+ owing to the fact BC has a higher surface area, CEC, and porosity. Besides this, BC also has a high adsorption capacity, which reduces the negative effects of SS by increasing Na+ adsorption and increasing the release of beneficial nutrients (Ca, Mg, and K) (Iqbal et al., 2019). Further BC also improved the K/Na+ ratio and increased the water holding capacity, plant available water, and WUE, which reduced salinity-induced osmotic stress (Akhtar et al., 2015b; Naeem et al., 2017). The studies indicate that BC application increases the uptake of minerals under saline soils; nonetheless, more research is required to understand the mechanism of BC-mediated increase in nutrient uptake.
Biochar protects photosynthetic apparatus and improves photosynthesis under salinity stress
Salinity stress predominantly decreases RWC and chlorophyll synthesis and leads to a substantial reduction in photosynthesis (Manan et al., 2016). BC application has been reported to increase the chlorophyll contents owing to an increase in N (Akhtar et al., 2015a). Under SS, BC has been shown to improve stomata conductance and the synthesis of chlorophyll and lead to an increase in photosynthetic efficiency (Feng et al., 2018; Shabbir et al., 2021). The application of BC to saline soils improves stomata density and stomata conductance, which improve leaf gas exchange characteristics, resulting in substantial increase in photosynthetic efficiency under SS (Thomas et al., 2013; Akhtar et al., 2015a). Biochar-mediated improvement in photosynthetic pigments is linked with nutrient uptake and availability (K, P, Mg, Ca, and S) and improvements in the physiochemical and biological properties of soil (Farhangi-Abriz and Torabian, 2018). The plants growing under SS exhibited more damage to photosynthetic pigments (Kaya et al., 2018); however, BC application appreciably improved the antioxidant activities, which prevent the oxidative damage to photosynthetic pigments and photosynthetic apparatus of plants growing under SS (Rasheed et al., 2019).
Biochar addition leads to a significant increase in chlorophyll and carotenoid contents under normal and stressful conditions, leading to the maintenance of greenish leaves (Ran et al., 2020). The increased nutrient uptake and assimilation in BC-treated soils improved the enzymatic activity, chlorophyll synthesis, photosynthesis, and tolerance against stress conditions (Soliman et al., 2022). The impact of BC on chlorophyll and carotenoid contents under SS is linked with accelerated antioxidant activity and the building of antioxidant activity. BC supplementation also provokes Mg2+ uptake, which is considered a building block in the synthesis of chlorophyll (Farouk and Al-Huqail, 2022). Moreover, BC also positively improves transpiration and stomata conductance, which leads to a substantial improvement in photosynthetic efficiency under SS as compared to no BC application (Akhtar et al., 2015b; Ghassemi-Golezani and Farhangi-Abriz, 2021). The deficiency of K affects chlorophyll fluorescence through an increase in thermal dissipation and a reduction in the efficiency of electron transport. The reduction in electron transport efficiency decreases NADPH and ATP concentrations, which decreases the activity of ATPase and increases NADP reduction under SS conditions (Ghassemi-Golezani and Rahimzadeh, 2022). BC application decreases the rate of NADP reduction through negative feedback from increasing NADPH in the plant. BC treatments provide more energy (NADPH, ATP) for photosynthetic activities by increasing the Hill reaction and decreasing the rate of NADP reduction, thereby increasing photosynthesis under SS (Lu et al., 2020). ATPases are considered as essential enzymes for the formation of adenosine diphosphate (ADP) and carbon assimilation during photosynthesis (Lu et al., 2020). BC application increases the activity of the Hill reaction, the functional integrity of PS-II, and electron transport efficiency, which is useful to decrease ROS production and increase plant photosynthetic efficiency (Ghassemi-Golezani and Rahimzadeh, 2022). Thus, BC-mediated increase in photosynthesis is linked to better synthesis of photosynthetic pigments, increased nutrient uptake, and improvements in soil physicochemical properties.
Biochar strengthens antioxidant systems and detoxify ROS under salinity stress
Salt stress induces the excessive production of ROS that impose devastating impacts on plants’ membranes, proteins, and lipids (Abbas et al., 2022). BC treatment improved redox homeostasis and prevented the overproduction of ROS. For instance, it was recorded that BC application reduced the H2O2 and TBARS concentration at both levels of BC (1% and 2%) in saline-sodic soil. However, the application of 2% BC markedly reduced the H2O2 concentration (Table 8) as compared to 1%, which indicates that the rate of BC has a strong influence on mitigating salinity-induced oxidative stress (Abbas et al., 2022). BC application enhanced antioxidant activities (CAT, POD, and SOD; Table 4); however, this increase was sufficient to reduce the toxic effects of ROS (Abbas et al., 2021). The improved function of the AsA–GSH cycle prevents H2O2-mediated oxidative impacts by maintaining a redox balance, which protects the metabolic pathways, photosynthesis, and enzyme functions (Alam et al., 2020).
BC supplementation improves AsA–GSH activity and the activities of other antioxidant enzymes that prevent the production and accumulation of ROS (Soliman et al., 2022). Likewise, Rasheed et al (2019) also noted that BC application decreased ROS production and consequently reduced lipid peroxidation with the help of enhanced antioxidant enzyme activity. However, some authors found a reduction in antioxidant activities (APX and GR) by using BC, possibly due to less uptake of Na+ in BC-treated plants (Kim et al., 2016). Recently, it has also been observed that BC application reduced the activity of antioxidant enzymes and oxidative stress in bean seedlings compared to control (Farhangi-Abriz and Torabian, 2017). The application of BC improved the AsA and GSH contents and reduced the DHA and GSSG content, therefore, improving the capability for detoxification of ROS under SS (Hasanuzzaman et al., 2021; Turan, 2021).
The increase in APX activity was also reported in soybean-treated plants following BC application (Mehmood et al., 2020); on the other hand, it was also reported that BC supply led to a substantial increase in APX, MDHAR, DHAR, and GR activity in jute plants growing under SS (Hasanuzzaman et al., 2021). Sofy et al. (2021) also found that BC supplementation improves the activities of SOD, CAT, and POD, reducing ROS accumulation under SS. Moreover, the BC amendment also improved the Gly-1 and Gly-II activity in SS plants, which therefore reduced the MG-induced cellular damage to the plant growing under SS (Hasanuzzaman et al., 2021). Thus, BC assisted in an increase in antioxidant activities to protect the plant from salinity-induced deleterious impacts, therefore improving plant performance under SS.
Biochar improves genes expression and stress responsive proteins under salinity stress
BC application also improves gene expression to induce salt tolerance. For instance, a BC-mediated increase in biomass and carbon assimilation in wheat plants under SS was linked with the up-regulation of water transporter genes (Soliman et al., 2022). Aquaporins play an imperative role in water transportation, particularly PIPs; they not only improve water uptake and transportation to roots and leaves (Cui et al., 2021), but also boost CO2 diffusion that ensures abundant substrate supply for photosynthesis and carbohydrate assimilation (Groszmann et al., 2017). It has been reported that BC in combination with Se-NPs upregulates the aquaporin and ion transporter genes, which regulate ionic homeostasis under SS (Santander et al., 2021). Moreover, BC + Se-NP mediated increase in Ca2+ concentration induced salinity tolerance by modifying cellular signaling pathways (Qin et al., 2019).
It has also been recorded that BC + Se-NPs increase the K+/Na+ ratio and Ca2+ concentration via the Ca2+dependent SOS pathway. Similarly, ion transport proteins such as NHX1, HKT1, and SOS1 also play a key role in regulating salinity tolerance (Assaha et al., 2017; Li et al., 2022), and it has been reported that BC in combination with Se-NPs improved the expression of these genes, leading to a significant increase in salinity tolerance (Soliman et al., 2022). The findings of these authors also suggest a synergy between BC and Se-NPs for stabilizing membrane potential for optimal functioning of H + ATPase, favoring K uptake, and protecting wheat plants from salt-induced injury (Soliman et al., 2022). Further, SS also upregulates AQPs (P1P1, NIP, and N1P1), and these proteins are further upregulated by BC + Se-NPs, which promote water status equilibrium in plant cells and adjust their position to ROS-induced membrane damage (Soliman et al., 2022).
Biochar maintains osmolytes accumulation and hormonal balance under salinity stress
Secondary metabolites, i.e., phenols and flavonoids, play an important role in ROS scavenging by improving antioxidant activities (Austen et al., 2019). According to Soliman et al. (2022), BC application markedly improved the concentration of phenols and flavonoids in salinity-stressed plants, with a parallel increase in the accumulation of different osmolytes (glycine betaine, proline, and carbohydrates). The BC-mediated increase in phenols and flavonoids strengthened the antioxidant defense system that prevents salinity-induced oxidative damage (Soliman et al., 2022). Some studies also documented that BC decreased the SS in plants and lowered the production of some hormones (Lashari et al., 2015; Akhtar et al., 2015a). For instance, BC application decreased the ABA contents of maize leaf sap growing under SS conditions (Lashari et al., 2015). In another study, authors also noted that BC application alone or in combination with entophytic bacteria decreased the xylem ABA contents of maize and wheat plants growing under SS as compared to controls (Akhtar et al., 2015b). The improvement in soil properties, i.e., soil moisture and Na+ binding in BC-amended soil decreases the root’s sensitivity to osmotic stress (Akhtar et al., 2015b). The incorporation of BC into salt-affected soils mitigates the effects of SS by decreasing Na+ uptake, which leads to a reduction in the ABA contents of cabbage plants grown under SS (Farhangi-Abriz and Torabian, 2018). BC application also increases the concentration of osmolytes that protect the plants from the damaging effects of SS. For instance, BC-mediated increase in GB and proline results in better osmotic adjustments under SS owing to the upregulation of antioxidant activity (Rasheed et al., 2019). However, some authors also noted that BC application reduced the accumulation of osmolytes. For instance, BC addition leads to a significant decrease in osmolytes accumulation owing to a reduction in exchangeable sodium under SS (Ghassemi-Golezani et al., 2020). Further BC treatments decrease the ABA, SA, and JA contents by decreasing the Na+ uptake and accumulation in plants (Ghassemi-Golezani and Rahimzadeh, 2022). Conversely, BC application increased the IAA synthesis by improving plant nutrient (Zn) uptake, owing to the fact Zn triggers tryptophan synthesis which is an essential amino acid for IAA production (Castillo-González et al., 2018). The reduction in ABA concentration following BC application substantially mitigated the adverse effects of SS (Ghassemi-Golezani and Rahimzadeh, 2022). The BC application improves water uptake and turgor pressure, which cause a reduction in ABA concentration (Ghassemi-Golezani and Rahimzadeh, 2022). The endogenous JA and SA are stress hormones, and they are directly linked with sodium concentration in plant parts (Ryu and Cho, 2015). Thus, BC has the potential to reduce oxidative stress and, therefore, reduce the synthesis of these hormones under SS (Ghassemi-Golezani and Rahimzadeh, 2022). In conclusion, BC maintains the accumulation of favorable hormones and osmolytes that protect plants from the toxic effects of SS and ensures better plant performance under SS.
Biochar nutrition improves plant growth, yield, and quality under salinity stress
BC improves the growth and quality of plants grown under SS through various mechanisms (Akhtar et al., 2015c). BC amendment increased maize growth as well as biomass yield in soils containing a high concentration of Na+ and exchangeable salts (Kim et al., 2016). Likewise, BC supplementation improved the growth and biomass of tomatoes under SS (3.6 dS m−1) as compared to control conditions (Usman et al., 2016). In a field experiment, Lashari et al. (2013) found that BC application for six weeks in saline soil substantially improved the yield of wheat as compared to the control, whereas again in a 2-year field study, Lashari et al. (2015) found that BC amendments improved the plant height, leaf area, root density, photosynthesis, and grain yield under SS (Lashari et al., 2015).
Akhtar et al, 2015b and Akhtar et al, 2015c found that BC application improved the photosynthetic rate, root and shoot growth, leaf area, and yield of maize and wheat plants under SS as compared to the control treatment. Similarly, other authors also reported the same trend of increased in plant growth and biomass with BC application in saline-sodic soil (Lashari et al., 2015; Akhtar et al., 2015b). BC-mediated increase in growth and yield under SS is linked with improved soil physio-chemical and biological properties, improved CEC, nutrient and water uptake, microbial population, and a reduction in the uptake of Na+ (Akhtar et al., 2015a; Farhangi-Abriz and Torabian, 2018; Mansoor et al., 2021). BC-mediated increase in mineral and nutrient assimilation regulate chlorophyll synthesis, photosynthesis, and stress tolerance, which in turn improve plant growth (Soliman et al., 2022).
Soliman et al (2022) found that an increase in the growth of beans following BC application is linked with an improvement in soil pH and nutrient availability. Other authors also found that BC amendment (3%) increased nutrient concentration, which is the main factor responsible for the increase in plant growth; further, BC-mediated reduction in H2O2 production also leads to a substantial increase in plant growth under SS (Kaya et al., 2018). BC application has no impact on the essential oil contents of inflorescences and seeds in saline soils; however, an increase in the oil content of vegetative plant parts was observed with the application of BC (Ghassemi-Golezani and Rahimzadeh, 2022). Other authors also found that BC application improves essential oil contents by increasing nutrient availability to plants (Yadegari, 2017). Salinity stress modifies the composition of borage oil; however, BC + melatonin application appreciably increased palmitoleic acid (0.24%), stearic acid (4.01%), oleic acid (19.58%), linoleic acid (36.16%), α-linolenic-ω6 acid (19.52%), α-linolenic-ω3 acid (0.17%), and arachidic acid (0.27 wt.%) as compared to the control treatment (Farouk and Al-Huqail, 2022). Thus, a BC-mediated increase in plant performance is linked with an increase in soil properties, membrane stability, and antioxidant activities.
Biochar improves soil properties to induce salinity tolerance
BC application plays an appreciable role in nutrient homeostasis under SS, and it has been recorded that BC application markedly decreased Na+ concentrations in potato xylem sap while BC application increased the K+ in xylem sap (Lashari et al., 2015; Akhtar et al., 2015a). Similarly, in another study, BC application reduced the Na+ uptake in lettuce and maize and increased the K uptake and accumulation (Hammer et al., 2015; Kim et al., 2016). Likewise, BC also increased K, N, and P contents and decreased the Na and Na+/K ratios of maize xylem sap (Lashari et al., 2015). BC application is very effective in reducing the Na+ uptake, and salt-affected soils can be cultivated by adapting the application of BC (Ding et al., 2020).
The use of organic amendments has emerged as an excellent tool to improve plant growth by changing the soil’s physio-chemical properties. Recently, the addition of BC to salt-affected soils has gained considerable attention across the globe (Amini et al., 2016; Ding et al., 2020). The application of BC to saline increases nutrient concentration owing to a concomitant increase in CEC, surface area, structure, porosity, and stability of soil structure (Zheng et al., 2018a). In salt-affected soils, higher Na+ concentration impairs K+ uptake; however, BC application significantly improved the K+ uptake as compared to the control (Lin et al., 2015). Biochar application also improves the NUE of crops owing to its porous structure and large surface area, which are conducive to an increase in NH4+ and a reduction in microbial de-nitrification (Liu et al., 2019).
Researchers have reported that BC with a high pH (9.6–10.8) increased the NH3 volatilization from salt-affected soil (Esfandbod et al., 2017; Sun et al., 2017); therefore, BC application effectively reduces the NH3 losses from saline and sodic soils. P availability is higher at pH 5.5–7; however, at pH >7, P availability substantially decreases. Nonetheless, BC addition increased the P availability in saline soils because of its inherent P fertilizer value and increased the growth of favorable bacteria (Flavobacterium, Pseudomonas, and Thiobacillus) that solubilize the unavailable P in soils (Ding et al., 2020). Similarly, BC application also improved nutrient availability, soil quality, and soil organic matter. All the studied BC rates increased the soil organic carbon (SOC); however, the application of 2.5% BC significantly improved the SOC by 10% as compared to other rates (Abo-Elyousr et al., 2022). Similarly, BC application also improved dehydrogenase activity, enhanced soil microbial biomass and organic matter stability, and led to a significant increase in nutrient absorption in saline soil (Abo-Elyousr et al., 2022).
Biochar as an important amendment to improve soil fertility
Biochar application has been reported to improve soil fertility by incrassating soil pH, WHC, and CEC, retaining soil nutrients, and stimulating the activity of beneficial bacteria and fungi (Warnock et al., 2007). The incorporation of BC into soil alters soil properties including soil texture, structure, pore size, WHC soil bulk density, soil pore volume soil, soil porosity, WHC, and saturated hydraulic conductivity and water retention in soil (Figure 4, Downie et al., 2009; Abel et al., 2013; Zhang et al., 2017; Adekiya et al., 2020; Kapoor et al., 2022). The soil’s physical as well as hydraulic properties, directly and indirectly, affect the services provided by soil. For example, these properties also include root growth, soil aeration, soil compaction, and nutrient and water uptake. Biochar has also been reported to increase soil pH, soil organic carbon, CEC, and NUE and lead to an appreciable increase in plant growth after BC application (Van Zwieten et al., 2010; Agegnehu et al., 2017). Yamato et al. (2006) also noted that BC made from Acacia magnum increased soil pH, soil calcium, CEC, and base saturation, and according to Novak et al. (2009), BC application to acidic coastal soil substantially increased the soil pH, SOM, soil Ca, and Mg concentrations while BC decreased soil sulfur and Zn concentrations.
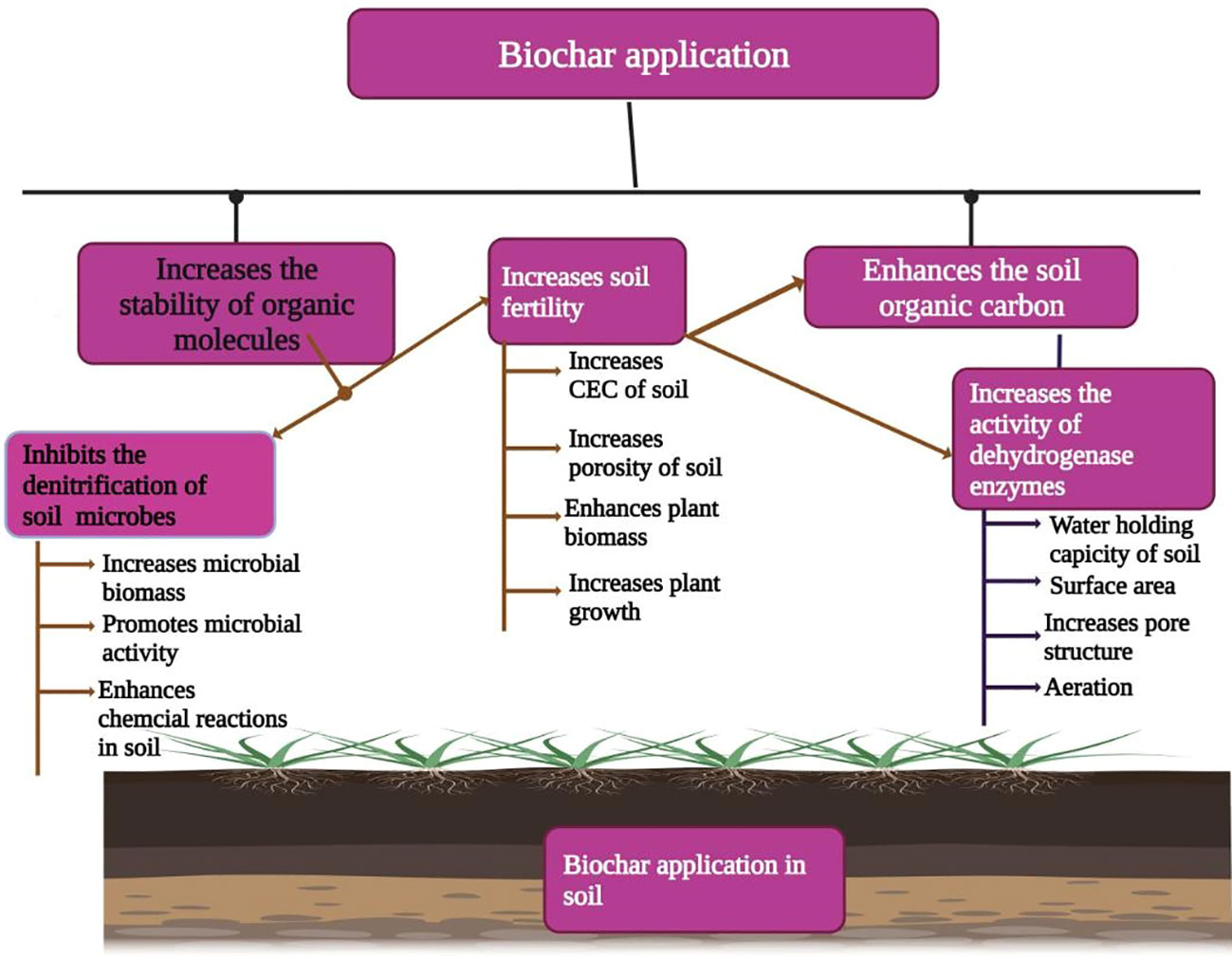
Figure 4 Biochar application improves soil fertility by increasing aggregate stability, microbial biomass, microbial activity, soil CEC, soil porosity, soil organic carbon, water holding capacity, and soil aeration.
Researchers have also documented that BC did not equally increase the soil porosity when applied at the same rate due to the difference in soil type and soil class (Alghamdi, 2018). Generally, BC appreciably improved the soil porosity of coarse-textured soils as compared to fine-textured soils (Alghamdi, 2018). BC particles have porosity and intra-pore space that provide additional space for water retention; therefore, the addition of BC (30 Mg ha−1) significantly increased the moisture contents and water infiltration (Adekiya et al., 2020). However, improvement in soil moisture is linked with the rate of BC application (Kätterer et al., 2019).
El-Naggar et al. (2018) found that BC made from rice straw and crop residues substantially improved SOM, whereas Adekiya et al. (2020) found that BC made from hardwood (30 Mg ha−1) substantially improved SOM by 18% as compared to the unamended control. In another study, Yang and Lu (2020) noted a substantial increase in SOM after applying BC to rice as well as rapeseed straws. In a series of lab studies, a maximum decrease of 31% in soil BD was reported after BC application in sandy soil, whereas in coarse and fine-texture soils, BD was decreased by 14.2% and 9.2%, respectively, following BC application (Blanco-Canqui, 2017; Liu et al., 2017). El-Naggar et al. (2018) noted a sharp increase in soil pH following the application of BC made from umbrella tree residues and rice straw, respectively. Moreover, Chathurika et al. (2016) amended soil with 1% and 2% acer woodchip BC and found a significant increase in soil pH after 75 days as compared to control un-amended soil.
Zhang et al. (2017) noted an increase of 21% in CEC after BC application, whereas El-Naggar et al. (2018) found that BC made from rice straw, silver-grass residues, and umbrella trees to sandy soils increased the CEC by 906, 180, and 130%, respectively. Ndor et al. (2015) also found an increase of 21% in CEC after the application of 5 Mg ha−1 made from rice husk and sawdust, whereas they found an increase of 44% and 57% in CEC after the application of BC at rates of 10 Mg ha−1. Rodríguez-Vila et al. (2016) and Adekiya et al. (2020) found a substantial increase in soil N, P, K, S, Ca, and Mg status following BC application, whereas Yao et al. (2017) found a substantial increase in soil N concentration and decrease in total soil P after addition of maize straw BC (50–200 Mg ha−1). BC structure provides a refuge for beneficial microbes (bacteria and AMF), and it increases soil enzymatic activities. For instance, Demisie et al. (2014) found that BC application improved the activity of urease and b- glucosidase as compared to the control. Likewise, BC also activates the Rhizobium, which in turn increases the nodulation and nitrogenase activity and colonizes Azotobactor and Azospirillum (Gabhane et al., 2020). Karimi et al. (2020) observed a substantial increase in SMB after BC application, whereas Yao et al. (2017) noted an increase from 6.6% to 31.2% in fungal abundance after maize stalk BC application (50, 100, and 200 Mg ha−1) as compared to control.
Biochar as an important amendment to improve crop growth and yield
Many authors have reported that BC application significantly improved the growth as well as biomass of different plant species (Seleiman et al., 2019). For example, Khan et al. (2017) found an increase of 49% in rapeseed yield following BC addition, whereas Rafique et al. (2020) noted that soil amended with BC showed an increase of 50%–55% in maize fresh and dry weights. Similarly, in sunflowers, BC improved growth and oil yield under water deficit conditions (Seleiman et al., 2019), while Zhang et al. (2020) noted higher cotton physiological activity in cotton plants amended with BC. Raboin et al. (2016) noted that maize yield in acid soil increased yield from 48% to 56% after the application of BC at 50 Mg ha−1. Agegnehu et al. (2015) also found an increase of 22% and 24% in seed and pod yield of peanuts after the application of BC (25 Mg ha−1) with inorganic fertilizers as compared to the control, while according to Xu et al. (2015), BC application increased the kernel quality of peanut.
Palansooriya et al., 2019 found a substantial increase in sweet potato yield after BC application, whereas, Gholizadeh et al. (2020) found no differences in yield between BC amended plots and control plots. Also, Mclennon et al. (2020) found that BC in combination with N fertilizer had no significant impacts on the growth and biomass productivity of Schedonorus arundinacea and Poa pratensis plants. These contradictory results could be due to differences in the physiochemical properties of BC. For instance, BC produced at a pyrolysis temperature of ≥600°C can absorb plant nutrients, therefore decreasing nutrient uptake. Moreover, Agegnehu et al. (2015) noted an increase in maize yield from 98% to 150% due to a parallel increase in WUE between 91% and 139%. Generally, increased crop yields and nutrient uptake might be due to the direct addition of nutrients from applied BC, which therefore increases the crop yield. Though crop responses to BC largely depend on BC type, soil type, and plant species. Asai et al. (2009) studied the impact of BC on the grain yield of rice grown in northern Laos, and they found a double increase in rice yield following BC application at 8 t ha−1. The experiments conducted by Abd-Elwahed et al. (2019) found that BC application (300 mg/L) enhanced wheat yield. Moreover, Salama et al. (2021) found the highest yields of 2.04 and 2.01 t ha−1 following the application of BC.
Future implications
The use of biochar can reduce the negative impacts of abiotic stress depending on its biomass properties, feedstock type, and processing conditions. Biochar can be used to absorb air and water pollutants and salts, and it can also be used as a soil conditioner, compost additive, and carbon sequestration source to mitigate the adverse effects of climate change. Besides this, biochar can also contribute to the circular economy through its use in agriculture and horticulture. Moreover, different benefits of biochar have an appreciable potential for emerging bioenergy production systems. As the application of biochar has significantly increased in recent times, there is a need to develop guidelines and standards to produce this black gold. For instance, crop productivity can be decreased or increased by BC application depending on fertilizer management and soil type, and the chemical attitude of BC is also inconsistent with heavy metals. The interaction mechanisms, productive technologies, applications, and properties between soil, plant, and biochar are very critical and yet not thoroughly discovered. Therefore, more efforts are needed to underpin all these things for the promising future of biochar.
Conclusion
Drought and salinity stress induce serious alterations in plant growth and development by disturbing various biochemical, physiological, and molecular processes, BC application improves membrane stability, nutrient uptake, and nutrient homeostasis, thereby improving plant performance under drought and salinity stress. The BC amendment also improves photosynthetic efficiency and antioxidant activity, which maintains hormonal balance and protects the plant from drought and salinity-induced oxidative and osmotic stresses and improves plant performance. In the case of salinity stress, BC also restricted the entry of noxious Na+ and increased the entry of K+, which regulates stomata movements and improves the leaf gas exchange characteristics under salinity stress.
Yet many unanswered questions exist regarding the role of BC in different plant processes under drought and salinity stresses. For instance, the role of seed germination under both stresses has not been studied yet; therefore, future studies must be conducted to determine the role of BC in the different mechanisms involved in seed germination. More research studies are required to explore the effect of BC on nutrient signaling via ionic transporters and nutrient channels under both stresses. The role of BC in protecting the photosynthetic apparatus from oxidative stress and the effect of BC on stomatal signaling and the regulation of guard cells must be explored. The role of BC on plant reproductive characteristics hass not been studied yet; therefore, it is crucial to study the effect of BC on this aspect under both stresses. The role of BC on hormones and osmolytes accumulation is poorly studied, so it is mandatory to explore the role of BC in the accumulation of different osmolytes and hormones under drought and salinity stress. It would also be fascinating to determine the effect of BC on the complex relationship between salicylic acid, IAA, gibberellic acid, cytokinin, and ethylene at the transcriptomic level.
The role of BC under drought and salinity stresses is less studied in field conditions; therefore, it is suggested to conduct long-term field studies under a wide range of climate conditions to increase understanding about the role of BC in mediating drought as well as salinity stresses. The use of BC in combination with microbes would enhance the plant’s tolerance to both stresses. However, detailed studies are needed to determine the effectiveness of BC and microbes in improving growth under water deficiency and salinity stress. Moreover, pilot scale studies are also needed to develop models to recommend the rates of BC application based on soil, plant, and climatic conditions.
Author contributions
YW and YZ: conceptualization. YW, LZ, and XW: writing original draft. XW, YZha, and XL: writing-review and editing. All authors have read and agreed to the published version of the manuscript.
Funding
This work was supported by the Science and Technology Planning Project of Jiangxi Province (20203BBF62W010 and 20202BBF63006) and the Forestry Science and Technology Innovation Special Project of Jiangxi Forestry Department (CXZX) (2021)15 and JXTG (2021)16).
Conflict of interest
The authors declare that the research was conducted in the absence of any commercial or financial relationships that could be construed as a potential conflict of interest.
Publisher’s note
All claims expressed in this article are solely those of the authors and do not necessarily represent those of their affiliated organizations, or those of the publisher, the editors and the reviewers. Any product that may be evaluated in this article, or claim that may be made by its manufacturer, is not guaranteed or endorsed by the publisher.
Abbreviations
ABA, abscisic acid; AMF, arbuscular mycorrhiza; APX, ascorbate peroxidase; AQP, aquaporins; BC, biochar; CAT, catalase; CEC, cation exchange capacity; DNA, deoxyribonucleic acid; DS, drought stress; EL, electrolyte leakage; GA, gibberellic acid; GPX, glutathione peroxidase; GR, glutathione reductase; FC, field capacity; HM, heavy metals; IAA, indole-3-acetic acid; MDA, malondialdehyde; NADPH, reduced nicotinamide adenine dinucleotide phosphate; RWC, relative water contents; SOC, soil organic carbon; SOD, superoxide dismutase; SMB, Soil microbial biomass; SS, salinity stress; WUE, water use efficiency.
References
Abbas, G., Abrar, M. M., Naeem, M. A., Siddiqui, M. H., Ali, H. M., Li, Y., et al. (2022). Biochar increases salt tolerance and grain yield of quinoa on saline-sodic soil: multivariate comparison of physiological and oxidative stress attributes. J. Soil Sediment. 22, 1446–1459. doi: 10.1007/s11368-022-03159-2
Abbas, G., Amjad, M., Saqib, M., Murtaza, B., Asif Naeem, M., Shabbir, A., et al. (2021). Soil sodicity is more detrimental than salinity for quinoa (Chenopodium quinoa willd.): A multivariate comparison of physiological, biochemical and nutritional quality attributes. J. Agron. Crop Sci. 207, 59–73. doi: 10.1111/jac.12451
Abdelaal, K. A., EL-Maghraby, L. M., Elansary, H., Hafez, Y. M., Ibrahim, E. I., El-Banna, M., et al. (2019). Treatment of sweet pepper with stress tolerance-inducing compounds alleviates salinity stress oxidative damage by mediating the physio-biochemical activities and antioxidant systems. Agron. 10, 26. doi: 10.3390/agronomy10010026
Abd-Elwahed, M. S., Abd-El-Aziz, M. E., Shaaban, E. A., Salama, D. M. (2019). New trend to use biochar as foliar application for wheat plants (Triticum aestivum). J. Plant Nutr. 42, 1180–1191. doi: 10.1080/01904167.2019.1609503
Abel, S., Peters, A., Trinks, S., Schonsky, H., Facklam, M., Wessolek, G. (2013). Impact of biochar and hydrochar addition on water retention and water repellency of sandy soil. Geoderma. 202, 183–191. doi: 10.1016/j.geoderma.2013.03.003
Abideen, Z., Koyro, H. W., Huchzermeyer, B., Ansari, R., Zulfiqar, F., Gul, B. J. P. B. (2020). Ameliorating effects of biochar on photosynthetic efficiency and antioxidant defence of phragmites karka under drought stress. Plant Biol. 22, 259–266. doi: 10.1111/plb.13054
Abo-Elyousr, K. A., Mousa, M. A., Ibrahim, O. H., Alshareef, N. O., Eissa, M. A. (2022). Calcium-rich biochar stimulates salt resistance in pearl millet (Pennisetum glaucum l.) plants by improving soil quality and enhancing the antioxidant defense. Plant 11, 1301.
Adekiya, A. O., Agbede, T. M., Olayanju, A., Ejue, W. S., Adekanye, T. A., Adenusi, T. T., et al. (2020). Effect of biochar on soil properties, soil loss, and cocoyam yield on a tropical sandy loam alfisol. Sci. World J. 2020, 9391630.
Agarwal, P., Parida, S. K., Raghuvanshi, S., Kapoor, S., Khurana, P., Khurana, J. P., et al. (2016). Rice improvement through genome-based functional analysis and molecular breeding in India. Rice. 9, 1–17. doi: 10.1186/s12284-015-0073-2
Agbna, G. H., Dongli, S., Zhipeng, L., Elshaikh, N. A., Guangcheng, S., Timm, L. C. (2017). Effects of deficit irrigation and biochar addition on the growth, yield, and quality of tomato. Sci. Hortic. 222, 90–101. doi: 10.1016/j.scienta.2017.05.004
Agegnehu, G., Bass, A. M., Nelson, P. N., Muirhead, B., Wright, G., Bird, M. I. (2015). Biochar and biochar-compost as soil amendments: effects on peanut yield, soil properties and greenhouse gas emissions in tropical north Queensland, australia. agric. Ecosyst. Environ. 213, 72–85. doi: 10.1016/j.agee.2015.07.027
Agegnehu, G., Srivastava, A. K., Bird, M. I. (2017). The role of biochar and biochar-compost in improving soil quality and crop performance: A review. Appl. Soil Ecol. 119, 156–170. doi: 10.1016/j.apsoil.2017.06.008
Ahmed, F., Arthur, E., Plauborg, F., Andersen, M. N. (2016). Biochar effects on maize physiology and water capacity of sandy subsoil. Mech. Agric. Conserv. Resour. 62, 8–13.
Akhtar, S. S., Andersen, M. N., Liu, F. (2015a). Residual effects of biochar on improving growth, physiology and yield of wheat under salt stress. agric. Water Manage. 158, 61–68. doi: 10.1016/j.agwat.2015.04.010
Akhtar, S. S., Andersen, M. N., Liu, F. (2015c). Biochar mitigates salinity stress in potato. J. Agron. Crop Sci. 201, 368–378. doi: 10.1111/jac.12132
Akhtar, S. S., Andersen, M. N., Naveed, M., Zahir, Z. A., Liu, F. (2015b). Interactive effect of biochar and plant growth-promoting bacterial endophytes on ameliorating salinity stress in maize. Funct. Plant Biol. 42, 770–781. doi: 10.1071/FP15054
Akhtar, S. S., Li, G., Andersen, M. N., Liu, F. (2014). Biochar enhances yield and quality of tomato under reduced irrigation. Agric. Water Manag. 138, 37–44.
Alam, M. J., Ahmed, K. S., Nahar, M. K., Akter, S., Uddin, M. A. (2020). Effect of different sowing dates on the performance of maize. J. Krishi Vigyan. 8, 75–81. doi: 10.5958/2349-4433.2020.00015.X
Alghamdi, A. G. (2018). Biochar as a potential soil additive for improving soil physical properties–a review. Arab. J. Geosci. 11, 1–16. doi: 10.1007/s12517-018-4056-7
Alharbi, K., Alaklabi, A. (2022). Alleviation of salinity induced growth and photosynthetic decline in wheat due to biochar and jasmonic acid application involves up-regulation of ascorbate-glutathione pathway, glyoxylase system and secondary metabolite accumulation. Rhizosphere. 24, 100603. doi: 10.1016/j.rhisph.2022.100603
Ali, E. F., Al-Yasi, H. M., Kheir, A., Eissa, M. A. (2021). Effect of biochar on CO2 sequestration and productivity of pearl millet plants grown in saline sodic soils. J. Soil Sci. Plant Nutri. 21, 897–907. doi: 10.1007/s42729-021-00409-z
Aller, D., Rathke, S., Laird, D., Cruse, R., Hatfield, J. (2017). Impacts of fresh and aged biochars on plant available water and water use efficiency. Geoderma. 307, 114–121. doi: 10.1016/j.geoderma.2017.08.007
Al-Maskri, A., Al-Kharusi, L., Al-Miqbali, H., Khan, M. M. (2010). Effects of salinity stress on growth of lettuce (Lactuca sativa) under closed-recycle nutrient film technique. Int. J. Agric. Biol. 12, 377–380.
Amini, S., Ghadiri, H., Chen, C., Marschner, P. (2016). Salt-affected soils, reclamation, carbon dynamics, and biochar: a review. J. Soil Sediment. 16, 939–953. doi: 10.1007/s11368-015-1293-1
Amjad, S. F., Mansoora, N., Din, I. U., Khalid Iqbal, R., Jatoi, G. H., Murtaza, G., et al. (2021). Application of zinc fertilizer and mycorrhizal inoculation on physio-biochemical parameters of wheat grown under water-stressed environment. Sustain. 13, 11007. doi: 10.3390/su131911007
Aref, I. M., Khan, P. R., Khan, S., El-Atta, H., Ahmed, A. I., Iqbal, M. (2016). Modulation of antioxidant enzymes in juniperus procera needles in relation to habitat environment and dieback incidence. Tree. 30, 1669–1681. doi: 10.1007/s00468-016-1399-0
Asai, H., Samson, B. K., Stephan, H. M., Songyikhangsuthor, K., Homma, K., Kiyono, Y., et al. (2009). Biochar amendment techniques for upland rice production in northern Laos: 1. soil physical properties, leaf SPAD and grain yield. Field Crops Res. 111, 81–84. doi: 10.1016/j.fcr.2008.10.008
Assaha, D. V., Ueda, A., Saneoka, H., Al-Yahyai, R., Yaish, M. W. (2017). The role of na+ and k+ transporters in salt stress adaptation in glycophytes. Front. Physiol. 8, 509. doi: 10.3389/fphys.2017.00509
Attia, M. S., Osman, M. S., Mohamed, A. S., Mahgoub, H. A., Garada, M. O., Abdelmouty, E. S., et al. (2021). Impact of foliar application of chitosan dissolved in different organic acids on isozymes, protein patterns and physio-biochemical characteristics of tomato grown under salinity stress. Plant. 10, 388. doi: 10.3390/plants10020388
Austen, N., Walker, H. J., Lake, J. A., Phoenix, G. K., Cameron, D. D. (2019). The regulation of plant secondary metabolism in response to abiotic stress: interactions between heat shock and elevated CO2. Front. Plant Sci. 10, 1463. doi: 10.3389/fpls.2019.01463
Bamminger, C., Poll, C., Sixt, C., Högy, P., Wüst, D., Kandeler, E., et al. (2016). Short-term response of soil microorganisms to biochar addition in a temperate agroecosystem under soil warming. Agric. Ecosys. Environ. 233, 03–10. doi: 10.1016/j.agee.2016.09.016
Batool, A., Taj, S., Rashid, A., Khalid, A., Qadeer, S., Saleem, A. R., et al. (2015). Potential of soil amendments (biochar and gypsum) in increasing water use efficiency of abelmoschus esculentus l. Moench. Front. Plant Sci. 6, 1–13. doi: 10.3389/fpls.2015.00733
Bird, M. I. (2015). “Test procedures for biochar analysis in soils,” in Biochar for environmental management: Science, technology and implementation. Eds. Lehmann, J., Joseph, S. (London: Routledge), 677–714.
Blanco-Canqui, H. (2017). Biochar and soil physical properties. Soil Sci. Soc Am. J. 81, 687–711. doi: 10.2136/sssaj2017.01.0017
Brown, R. (2009). “Biochar production technology,” in Biochar for environmental management: Science and technology. Eds. Lehmann, J., Joseph, S. (London: Earthscan), 127–139.
Castillo-González, J., Ojeda-Barrios, D., Hernández-Rodríguez, A., Cecilia-González-Franco, A., Robles-Hernández, L., Rogelio-López-Ochoa, G. (2018). Zinc metalloenzymes in plants. Interciencia. 43, 242–248.
Chathurika, J. A. S., Kumaragamage, D., Zvomuya, F., Akinremi, O. O., Flaten, D. N., Indraratne, S. P., et al. (2016). Woodchip biochar with or without synthetic fertilizers affects soil properties and available phosphorus in two alkaline, chernozemic soils. Can. J. Soil Sci. 96, 472–484. doi: 10.1139/cjss-2015-0094
Chaves, M. M., Flexas, J., Pinheiro, C. (2009). Photosynthesis under drought and salt stress: Regulation mechanisms from whole plant to cell. Ann. Bot. 103, 551–560. doi: 10.1093/aob/mcn125
Cornelissen, G., Jubaedah, G., Nurida, N. L., Hale, S. E., Martinsen, V., Silvani, L., et al. (2018). Fading positive effect of biochar on crop yield and soil acidity during five growth seasons in an Indonesian ultisol. Sci. Total Environ. 634, 561–568. doi: 10.1016/j.scitotenv.2018.03.380
Cui, G., Zhao, X., Liu, S., Sun, F., Zhang, C., Xi, Y. (2017). Beneficial effects of melatonin in overcoming drought stress in wheat seedlings. Plant Physiol. Biochem. 118, 138–149. doi: 10.1016/j.plaphy.2017.06.014
Cui, Y., Zhao, Y., Lu, Y., Su, X., Chen, Y., Shen, Y., et al. (2021). In vivo single-particle tracking of the aquaporin AtPIP2, 1 in stomata reveals cell type-specific dynamics. Plant Physiol. 185, 1666–1681. doi: 10.1093/plphys/kiab007
Dadshani, S., Sharma, R. C., Baum, M., Ogbonnaya, F. C., Léon, J., Ballvora, A. (2019). Multi-dimensional evaluation of response to salt stress in wheat. PloS One 14, e0222659. doi: 10.1371/journal.pone.0222659
Dahlawi, S., Naeem, A., Rengel, Z., Naidu, R. (2018). Biochar application for the remediation of salt-affected soils: Challenges and opportunities. Sci. Total Environ. 625, 320–335. doi: 10.1016/j.scitotenv.2017.12.257
Demirbas, A. (2004). Effects of temperature and particle size on bio-char yield from pyrolysis of agricultural residues. J. Anal. Appl. Pyrolysis. 72, 243–248. doi: 10.1016/j.jaap.2004.07.003
Demisie, W., Liu, Z., Zhang, M. (2014). Effect of biochar on carbon fractions and enzyme activity of red soil. Catena. 121, 214–221. doi: 10.1016/j.catena.2014.05.020
Denaxa, N.-K., Nomikou, A., Malamos, N., Liveri, E., Roussos, P. A., Papasotiropoulos, V. (2022). Salinity effect on plant growth parameters and fruit bioactive compounds of two strawberry cultivars, coupled with environmental conditions monitoring. Agron. 12, 2279. doi: 10.3390/agronomy12102279
Desoky, E. S. M., Merwad, A. R. M., Abo El-Maati, M. F., Mansour, E., Arnaout, S. M., Awad, M. F., et al. (2021). Physiological and biochemical mechanisms of exogenously applied selenium for alleviating destructive impacts induced by salinity stress in bread wheat. Agron. 11, 926. doi: 10.3390/agronomy11050926
Ding, Z., Kheir, A. S., Ali, O. A., Hafez, E., Elshamey, E. A., Zhou, Z., et al. (2020). Vermicompost and deep tillage system to improve saline-sodic soil quality and wheat productivity. J. Environ. Manage. 277, 111388. doi: 10.1016/j.jenvman.2020.111388
Downie, A., Crosky, A., Munroe, P. (2009). “Physical properties of biochar,” in Biochar for environmental management: Science and technology. Eds. Lehmann, J., Joseph, S. (London: Earthscan), 13–32.
Drake, J. A., Cavagnaro, T. R., Cunningham, S. C., Jackson, W. R., Patti, A. F. (2016). Does biochar improve establishment of tree seedlings in saline sodic soils? land degrad. Develop. 27, 52–59.
Durukan, H., Demirbas, A., Turkekul, I. (2020). Effects of biochar rates on yield and nutrient uptake of sugar beet plants grown under drought stress. Commu. Soil Sci. Plant Anal. 51, 2735–2745. doi: 10.1080/00103624.2020.1849257
Ebrahimi, M., Souri, M. K., Mousavi, A., Sahebani, N. (2021). Biochar and vermicompost improve growth and physiological traits of eggplant (Solanum melongena l.) under deficit irrigation. Chem. Biol. Tech. Agric. 8, 1–14. doi: 10.1186/s40538-021-00216-9
Egamberdieva, D., Reckling, M., Wirth, S. (2017). Biochar-based bradyrhizobium inoculum improves growth of lupin (Lupinus angustifolius l.) under drought stress. Eur. J. Soil Biol. 78, 03–10. doi: 10.1016/j.ejsobi.2016.11.007
El-Naggar, A., Lee, S. S., Awad, Y. M., Yang, X., Ryu, C., Rizwan, M., et al. (2018). Influence of soil properties and feedstocks on biochar potential for carbon mineralization and improvement of infertile soils. Geoderma. 332, 100–108. doi: 10.1016/j.geoderma.2018.06.017
El Nahhas, N., AlKahtani, M. D., Abdelaal, K. A., Al Husnain, L., AlGwaiz, H. I., Hafez, Y. M., et al. (2021). Biochar and jasmonic acid application attenuates antioxidative systems and improves growth, physiology, nutrient uptake and productivity of faba bean (Vicia faba l.) irrigated with saline water. plant physiol. Biochem. 166, 807–817. doi: 10.1016/j.plaphy.2021.06.033
Esfandbod, M., Phillips, I. R., Miller, B., Rashti, M. R., Lan, Z. M., Srivastava, P., et al. (2017). Aged acidic biochar increases nitrogen retention and decreases ammonia volatilization in alkaline bauxite residue sand. Ecol. Eng. 98, 157–165. doi: 10.1016/j.ecoleng.2016.10.077
FAO (2017). The future of food and agriculture: Trends and challenges, food and agriculture organization of the united nations (Rome, Italy).
Farhangi-Abriz, S., Torabian, S. (2017). Antioxidant enzyme and osmotic adjustment changes in bean seedlings as affected by biochar under salt stress. Ecotoxicol. Environ. Saf. 137, 64–70. doi: 10.1016/j.ecoenv.2016.11.029
Farhangi-Abriz, S., Torabian, S. (2018). Effect of biochar on growth and ion contents of bean plant under saline condition. Environ. Sci. pollut. Res. 25, 11556–11564. doi: 10.1007/s11356-018-1446-z
Farooq, M., Rehman, A., Al-Alawi, A. K., Al-Busaidi, W. M., Lee, D. J. (2020). Integrated use of seed priming and biochar improves salt tolerance in cowpea. Sci. Hortic. 272, 109507. doi: 10.1016/j.scienta.2020.109507
Farooq, M., Romdhane, L., Rehman, A., Al-Alawi, A. K., Al-Busaidi, W. M., Asad, S. A., et al. (2021). Integration of seed priming and biochar application improves drought tolerance in cowpea. J. Plant Growth Regul. 40, 1972–1980. doi: 10.1007/s00344-020-10245-7
Farouk, S., Al-Huqail, A. A. (2022). Sustainable biochar and/or melatonin improve salinity tolerance in borage plants by modulating osmotic adjustment, antioxidants, and ion homeostasis. Plant. 11, 765. doi: 10.3390/plants11060765
Farouk, S., Elhindi, K. M., Alotaibi, M. A. (2020). Silicon supplementation mitigates salinity stress on ocimum basilicum l. via improving water balance, ion homeostasis, and antioxidant defense system. Ecotoxicol. Environ. Saf. 206, 111396.
Fazal, A., Bano, A. (2016). Role of plant growth-promoting rhizobacteria (PGPR), biochar, and chemical fertilizer under salinity stress. Commun. Soil Sci. Plant Anal. 47, 1985–1993. doi: 10.1080/00103624.2016.1216562
Feng, J. I. N., Cheng, R. A. N., Qul, A. A., Yan, Q. G., Li, Y. G., Jian, B. L., et al. (2018). Effects of biochar on sodium ion accumulation, yield and quality of rice in saline-sodic soil of the west of songnen plain, northeast China. Plant Soil Environ. 64, 612–618. doi: 10.17221/359/2018-PSE
Foster, E. J., Hansen, N., Wallenstein, M., Cotrufo, M. F. (2016). Biochar and manure amendments impact soil nutrients and microbial enzymatic activities in a semi-arid irrigated maize cropping system. Agric. Ecosyst. Environ. 233, 03–10. doi: 10.1016/j.agee.2016.09.029
Foyer, C. H., Bloom, A. J., Queval, G., Noctor, G. (2009). Photorespiratory metabolism: genes, mutants, energetics, and redox signaling. Annu. Rev. Plant. Biol. 60, 455–484.
Gabhane, J. W., Bhange, V. P., Patil, P. D., Bankar, S. T., Kumar, S. (2020). Recent trends in biochar production methods and its application as a soil health conditioner: a review. SN Appl. Sci. 2, 1–21. doi: 10.1007/s42452-020-3121-5
Ghaderi, N., Hatami, M., Mozafari, A., Siosehmardeh, A. (2018). ). change in antioxidant enzymes activity and some morpho-physiological characteristics of strawberry under long-term salt stress. Physiol. Mol. Biol. Plant 24, 833–843. doi: 10.1007/s12298-018-0535-2
Gharred, J., Derbali, W., Derbali, I., Badri, M., Abdelly, C., Slama, I., et al. (2022). Impact of biochar application at water shortage on biochemical and physiological processes in medicago ciliaris. Plant. 11, 2411. doi: 10.3390/plants11182411
Ghassemi-Golezani, K., Abdoli, S. (2022). Alleviation of salt stress in rapeseed (Brassica napus l.) plants by biochar-based rhizobacteria: new insights into the mechanisms regulating nutrient uptake, antioxidant activity, root growth and productivity. Arch. Agron. Soil Sci. 1–18. doi: 10.1080/03650340.2022.2103547
Ghassemi-Golezani, K., Farhangi-Abriz, S. (2021). Biochar-based metal oxide nanocomposites of magnesium and manganese improved root development and productivity of safflower (Carthamus tinctorius l.) under salt stress. Rhizosphere. 19, 100416. doi: 10.1016/j.rhisph.2021.100416
Ghassemi-Golezani, K., Farhangi-Abriz, S., Abdoli, S. (2020). How can biochar-based metal oxide nanocomposites counter salt toxicity in plants? Environ. Geochem. Health 43, 2007–2023. doi: 10.1007/s10653-020-00780-3
Ghassemi-Golezani, K., Rahimzadeh, S. (2022). Biochar-based nutritional nanocomposites: a superior treatment for alleviating salt toxicity and improving physiological performance of dill (Anethum graveolens). Environ. Geochem. Health 68, 1–23. doi: 10.1007/s10653-022-01397-4
Gholizadeh, M., Li, C., Zhang, S., Wang, Y., Niu, S., Li, Y., et al. (2020). Progress of the development of reactors for pyrolysis of municipal waste. Sustain. Energy Fuels 4, 5885–5915. doi: 10.1039/D0SE01122C
Glaser, B., Lehmann, J., Zech, W. (2002). Ameliorating physical and chemical properties of highly weathered soils in the tropics with charcoal–a review. Biol. Fertil. Soil. 35, 219–230. doi: 10.1007/s00374-002-0466-4
González, J. F., Román, S., Encinar, J. M., Martínez, G. (2009). ). pyrolysis of various biomass residues and char utilization for the production of activated carbons. J. Anal. Appl. Pyrolysis. 85, 134–141. doi: 10.1016/j.jaap.2008.11.035
Groszmann, M., Osborn, H. L., Evans, J. R. (2017). Carbon dioxide and water transport through plant aquaporins. Plant Cell Environ. 40, 938–961. doi: 10.1111/pce.12844
Gullap, M. K., Severoglu, S., Karabacak, T., Yazici, A., Ekinci, M., Turan, M., et al. (2022). Biochar derived from hazelnut shells mitigates the impact of drought stress on soybean seedlings. New Z. J. Crop Hortic. Sci. 1–19. doi: 10.1080/01140671.2022.2079680
Guo, Q., Liu, L., Barkla, B. J. (2019). Membrane lipid remodeling in response to salinity. Int. J. Mol. Sci. 20, 4264. doi: 10.3390/ijms20174264
Hafeez, Y., Iqbal, S., Jabeen, K., Shahzad, S., Jahan, S., Rasul, F. (2017). Effect of biochar application on seed germination and seedling growth of glycine max (l.) merr. under drought stress. Pak. J. Bot. 49, 7–13.
Hafeez, F., Rizwan, M., Saqib, M., Yasmeen, T., Ali, S., Abbas, T., et al. (2019). Residual effect of biochar on growth, antioxidant defence and cadmium (Cd) accumulation in rice in a cd contaminated saline soil. Pak. J. Agric. Sci. 56 (1), 197–204.
Hafez, Y., Attia, K., Alamery, S., Ghazy, A., Al-Doss, A., Ibrahim, E., et al. (2020). Beneficial effects of biochar and chitosan on antioxidative capacity, osmolytes accumulation, and anatomical characters of water-stressed barley plants. Agron. 10, 630. doi: 10.3390/agronomy10050630
Hafez, E. M., Omara, A. E. D., Alhumaydhi, F. A., El-Esawi, M. A. (2021). Minimizing hazard impacts of soil salinity and water stress on wheat plants by soil application of vermicompost and biochar. Physiol. Plant 172, 587–602. doi: 10.1111/ppl.13261
Haider, G., Koyro, H. W. W., Azam, F., Steffens, D., Müller, C., Kammann, C. (2015). Biochar but not humic acid product amendment affected maize yields via improving plant-soil moisture relations. Plant Soil. 395, 03–10. doi: 10.1007/s11104-014-2294-3
Haider, I., Raza, M. A. S., Iqbal, R., Aslam, M. U., Habib-ur-Rahman, M., Raja, S., et al. (2020). Potential effects of biochar application on mitigating the drought stress implications on wheat (Triticum aestivum l.) under various growth stages. J. Saudi Chem. Soc 24, 974–981. doi: 10.1016/j.jscs.2020.10.005
Hailegnaw, N. S., Mercl, F., Pracke, K., Száková, L., Tlustoš, P. (2019). Mutual relationships of biochar and soil pH, CEC, and exchangeable base cations in a model laboratory experiment. J. Soils Sediments. 19, 2405–2416. doi: 10.1007/s11368-019-02264-z
Hammer, E. C., Forstreuter, M., Rillig, M. C., Kohler, J. (2015). Biochar increases arbuscular mycorrhizal plant growth enhancement and ameliorates salinity stress. Appl. Soil Ecol. 96, 114–121. doi: 10.1016/j.apsoil.2015.07.014
Hasanuzzaman, M., Raihan, M. R. H., Khojah, E., Samra, B. N., Fujita, M., Nahar, K. (2021). Biochar and chitosan regulate antioxidant defense and methylglyoxal detoxification systems and enhance salt tolerance in jute (Corchorus olitorius l.). Antioxidants. 10, 2017. doi: 10.3390/antiox10122017
Hashem, A., Kumar, A., Al-Dbass, A. M., Alqarawi, A. A., Al-Arjani, A. B. F., Singh, G. (2019). Arbuscular mycorrhizal fungi and biochar improves drought tolerance in chickpea. Saudi J. Biol. Sci. 26, 614–624. doi: 10.1016/j.sjbs.2018.11.005
Hassan, M. U., Chattha, M. U., Khan, I., Chattha, M. B., Barbanti, L., Aamer, M., et al. (2021). Heat stress in cultivated plants: Nature, impact, mechanisms, and mitigation strategies–a review. Plant Biosystems-An Int. J. Dealing all Aspects Plant Biol. 155 (2), 211–234. doi: 10.1080/11263504.2020.1727987
Hernández-Herrera, R. M., Sánchez-Hernández, C. V., Palmeros-Suárez, P. A., Ocampo-Alvarez, H., Santacruz-Ruvalcaba, F., Meza-Canales, I. D., et al. (2022). Seaweed extract improves growth and productivity of tomato plants under salinity stress. Agron 12. doi: 10.3390/agronomy12102495
Hoang, T. L. H., Jang, D. C., Nguyen, Q. T., Na, W. H., Kim, I. S., Vu, N. T. (2021). Biochar-improved growth and physiology of ehretia asperula under water-deficit condition. Appl. Sci. 11, 10685. doi: 10.3390/app112210685
Huang, M., Zhang, Z., Zhu, C., Zhai, Y., Lu, P. (2019). Effect of biochar on sweet corn and soil salinity under conjunctive irrigation with brackish water in coastal saline soil. Sci. Hortic. 250, 405–413. doi: 10.1016/j.scienta.2019.02.077
Hussien Ibrahim, M. E., Adam Ali, A. Y., Zhou, G., Ibrahim Elsiddig, A. M., Zhu, G., Ahmed Nimir, N. E., et al. (2020). Biochar application affects forage sorghum under salinity stress. Chil. J. Agric. Res. 80, 317–325. doi: 10.4067/S0718-58392020000300317
Ibrahim, M. E. H., Ali, A. Y. A., Zhou, G., Elsiddig, A. M. I., Zhu, G., Nimir, N. E. A., et al. (2020). ). biochar application affects forage sorghum under salinity stress. Chil. J. Agric. Res. 80, 317–325. doi: 10.4067/S0718-58392020000300317
Ibrahim, M. F. M., Faisal, A., Shehata, S. A. (2016). Calcium chloride alleviates water stress in sunflower plants through modifying some physio-biochemical parameters. Am. Eur. J. Agric. Environ. Sci. 16, 677–693.
Ibrahim, M. M., Tong, C., Hu, K., Zhou, B., Xing, S., Mao, Y. (2020b). Biochar-fertilizer interaction modifies n-sorption, enzyme activities and microbial functional abundance regulating nitrogen retention in rhizosphere soil. Sci. Total Environ. 739, 140065. doi: 10.1016/j.scitotenv.2020.140065
Iqbal, S., Basra, S. M., Afzal, I., Wahid, A., Saddiq, M. S., Hafeez, M. B., et al. (2019). Yield potential and salt tolerance of quinoa on salt-degraded soils of Pakistan. J. Agron. Crop Sci. 205, 13–21. doi: 10.1111/jac.12290
Ishrat, F., Afrasiab, H., Chaudhury, F. A. (2022). Effects of salinity stress on growth and physio-biochemical parameters of three pea (Pisum sativum l.) cultivars of different maturity duration. Adv. Life Sci. 9, 380–391.
Islam, M. J., Uddin, M. J., Hossain, M. A., Henry, R., Begum, M. K., Sohel, M. A. T., et al. (2022). Exogenous putrescine attenuates the negative impact of drought stress by modulating physio-biochemical traits and gene expression in sugar beet (Beta vulgaris l.). PloS One 17, e0262099. doi: 10.1371/journal.pone.0262099
Jamil, A., Riaz, S., Ashraf, M., Foolad, M. R. (2011). Gene expression profiling of plants under salt stress. CRC. Crit. Rev. Plant Sci. 30, 435–458. doi: 10.1080/07352689.2011.605739
Jindo, K., Mizumoto, H., Sawada, Y., Sanchez-Monedero, M. A., Sonoki, T. (2014). Physical and chemical characterization of biochars derived from different agricultural residues. Biogeosci. 11, 6613–6621. doi: 10.5194/bg-11-6613-2014
Kammann, C., Graber, E. R. (2015). “Biochar effects on plant ecophysiology,” in Biochar for environmental management: Science, technology and implementation. Ed. Lehmann, J. (Routledge, Abingdon: Routledge, Milton Park, Abingdon, Oxfordshire).
Kanwal, S., Ilyas, N., Shabir, S., Saeed, M., Gul, R., Zahoor, M., et al. (2018). Application of biochar in mitigation of negative effects of salinity stress in wheat (Triticum aestivum l.). J. Plant Nutr. 41, 526–538. doi: 10.1080/01904167.2017.1392568
Kapoor, A., Sharma, R., Kumar, A., Sepehya, S. (2022). Biochar as a means to improve soil fertility and crop productivity: a review. J. Plant Nutri. 45, 1–9. doi: 10.1080/01904167.2022.2027980
Karimi, A., Moezzi, A., Chorom, M., Enayatizamir, N. (2020). Application of biochar changed the status of nutrients and biological activity in a calcareous soil. J. Soil Sci. Plant Nutr. 20, 450–459. doi: 10.1007/s42729-019-00129-5
Kätterer, T., Roobroeck, D., Andrén, O., Kimutai, G., Karltun, E., Kirchmann, H., et al. (2019). Biochar addition persistently increased soil fertility and yields in maize-soybean rotations over 10 years in sub-humid regions of Kenya. Field Crops Res. 235, 18–26. doi: 10.1016/j.fcr.2019.02.015
Kaya, C., Akram, N. A., Ashraf, M., Sonmez, O. (2018). Exogenous Appl. Humic Acid mitigates salinity Stress maize (Zea mays L.) plants by improving some key physico-biochemical attributes. Cereal Res. Commun. 46, 67–78. doi: 10.1556/0806.45.2017.064
Kayum, M. A., Park, J. I., Nath, U. K., Biswas, M. K., Kim, H. T., Nou, I. S. (2017). Genome-wide expression profiling of aquaporin genes confer responses to abiotic and biotic stresses in brassica rapa. BMC Plant Biol. 17, 1–18. doi: 10.1186/s12870-017-0979-5
Khan, K. Y., Ali, B., Cui, X., Feng, Y., Yang, X., Stoffella, P. J. (2017). Impact different feedstocks derived biochar amendment cadmium low uptake affinity cultivar pak choi (Brassica Rapa Ssb. Chinensis L.) phytoavoidation Cd to reduce potential dietary toxicity. Ecotoxicol. Environ. Saf. 141, 129–138. doi: 10.1016/j.ecoenv.2017.03.020
Khan, Z., Khan, M. N., Zhang, K., Luo, T., Zhu, K., Hu, L. (2021). The application of biochar alleviated the adverse effects of drought on the growth, physiology, yield and quality of rapeseed through regulation of soil status and nutrients availability. Ind. Crops Prod. 171, 113878. doi: 10.1016/j.indcrop.2021.113878
Khan, W. U. D., Shaukat, R., Farooq, M. A., Ashraf, M. N., Nadeem, F., Tanveer, M., et al. (2022). Iron-doped biochar regulated soil nickel adsorption, wheat growth, its physiology and elemental concentration under contrasting abiotic stresses. Sustain. 14, 7852. doi: 10.3390/su14137852
Kim, H. S., Kim, K. R., Yang, J. E., Ok, Y. S., Owens, G., Nehls, T. (2016). Effect of biochar on reclaimed tidal land soil properties and maize (Zea mays l.) response. Chemosphere. 142, 153–159. doi: 10.1016/j.chemosphere.2015.06.041
Kul, R., Arjumend, T., Ekinci, M., Yildirim, E., Turan, M., Argin, S. (2021). Biochar as an organic soil conditioner for mitigating salinity stress in tomato. Soil Sci. Plant Nutr. 67, 693–706. doi: 10.1080/00380768.2021.1998924
Kumar, S., Li, G., Yang, J., Huang, X., Ji, Q., Liu, Z., et al. (2021). Effect of salt stress on growth, physiological parameters, and ionic concentration of water dropwort (Oenanthe javanica) cultivars. Front. Plant Sci. 12, 660409. doi: 10.3389/fpls.2021.660409
Laghari, M., Naidu, R., Xiao, B., Hu, Z., Mirjat, M. S., Hu, M., et al. (2016). Recent developments in biochar as an effective tool for agricultural soil management: a review. J. Sci. Food Agric. 96 (15), 4840–4849. doi: 10.1002/jsfa.7753
Laird, D. A., Fleming, P., Davis, D. D., Horton, R., Wang, B., Karlen, D. L. (2010). Impact of biochar amendments on the quality of a typical Midwestern agricultural soil. Geoderma. 158, 443–449. doi: 10.1016/j.geoderma.2010.05.013
Lalarukh, I., Amjad, S. F., Mansoora, N., Al-Dhumri, S. A., Alshahri, A. H., Almutari, M. M., et al. (2022). Integral effects of brassinosteroids and timber waste biochar enhances the drought tolerance capacity of wheat plant. Sci. Rep. 12, 1–10. doi: 10.1038/s41598-022-16866-0
Langeroodi, A. R. S., Campiglia, E., Mancinelli, R., Radicetti, E. (2019). Can biochar improve pumpkin productivity and its physiological characteristics under reduced irrigation regimes? Sci. Hortic. 247, 195–204. doi: 10.1016/j.scienta.2018.11.059
Lashari, M. S., Liu, Y., Li, L., Pan, W., Fu, J., Pan, G., et al. (2013). Effects of amendment of biochar-manure compost in conjunction with pyroligneous solution on soil quality and wheat yield of a salt-stressed cropland from central China great plain. Field Crops Res. 144, 113–118. doi: 10.1016/j.fcr.2012.11.015
Lashari, M. S., Ye, Y., Ji, H., Li, L., Kibue, G. W., Lu, H., et al. (2015). Biochar–manure compost in conjunction with pyroligneous solution alleviated salt stress and improved leaf bioactivity of maize in a saline soil from central China: a 2-year field experiment. J. Sci. Food Agric. 95, 1321–1327. doi: 10.1002/jsfa.6825
Latif, M., Bukhari, S. A. H., Alrajhi, A. A., Alotaibi, F. S., Ahmad, M., Shahzad, A. N., et al. (2022). Inducing drought tolerance in wheat through exopolysaccharide-producing rhizobacteria. Agron. 12, 1140. doi: 10.3390/agronomy12051140
Lehmann, J., Joseph, S. (2009). “Biochar for environmental management: an introduction,” in Biochar for environmental management: Science and technology. Eds. Lehmann, J., Joseph, S. (London: Earthscan), 1–12.
Lehmann, J., Rillig, M. C., Thies, J., Masiello, C. A., Hockaday, W. C., Crowley, D. (2011). Biochar effects on soil biota–a review. Soil Biol. Biochem. 43, 03–10. doi: 10.1016/j.soilbio.2011.04.022
Li, S. J., Wu, G. Q., Lin, L. Y. (2022). AKT1, HAK5, SKOR, HKT1, 5, SOS1 and NHX1 synergistically control na+ and k+ homeostasis in sugar beet (Beta vulgaris l.) seedlings under saline conditions. J. Plant Biochem. Biotechnol. 31, 71–84. doi: 10.1007/s13562-021-00656-2
Liang, G., Liu, J., Zhang, J., Guo, J. (2020). Effects of drought stress on photosynthetic and physiological parameters of tomato. J. Am. Soc Hortic. Sci. 145, 12–17. doi: 10.21273/JASHS04725-19
Liang, C., Zhu, X., Fu, S., Méndez, A., Gascó, G., Paz-Ferreiro, J. (2014). Biochar alters the resistance and resilience to drought in a tropical soil. Environ. Res. Lett. 9, 03–10. doi: 10.1088/1748-9326/9/6/064013
Licht, J., Smith, N. (2017). The influence of lignocellulose and hemicellulose biochar on photosynthesis and water use efficiency in seedlings from a northeastern US pine-oak ecosystem. J. Sustain. For. 37, 25–37. doi: 10.1080/10549811.2017.1386113
Lin, X. W., Xie, Z. B., Zheng, J. Y., Liu, Q., Bei, Q. C., Zhu, J. G. (2015). Effects of biochar application on greenhouse gas emissions, carbon sequestration and crop growth in coastal saline soil. eur. J. Soil Sci. 66, 329–338. doi: 10.1111/ejss.12225
Liu, C., Liu, F., Ravnskov, S., Rubæk, G. H., Sun, Z., Andersen, M. N. (2017). Impact of wood biochar and its interactions with mycorrhizal fungi, phosphorus fertilization and irrigation strategies on potato growth. J. Agron. Crop Sci. 203, 03–10. doi: 10.1111/jac.12185
Liu, C., Wang, H., Li, P., Xian, Q., Tang, X. (2019). Biochar’s impact on dissolved organic matter (DOM) export from a cropland soil during natural rainfalls. Sci. Total Environ. 650, 1988–1995. doi: 10.1016/j.scitotenv.2018.09.356
Lu, X., Sun, D., Zhang, X., Hu, H., Kong, L., Rookes, J. E., et al. (2020). ). stimulation of photosynthesis and enhancement of growth and yield in arabidopsis thaliana treated with amine-functionalized mesoporous silica nanoparticles. Plant Physiol. Biochem. 156, 566–577. doi: 10.1016/j.plaphy.2020.09.036
Lyu, S., Du, G., Liu, Z., Zhao, L., Lyu, D. (2016). Effects of biochar on photosystem function and activities of protective enzymes in pyrus ussuriensis maxim. under drought stress. Acta Physiol. Plant 38, 03–10. doi: 10.1007/s11738-016-2236-1
Ma, S., Wang, Z., Guo, X., Wang, F., Huang, J., Sun, B., et al. (2021). Sourdough improves the quality of whole-wheat flour products: Mechanisms and challenges–a review. Food Chem. 360, 130038. doi: 10.1016/j.foodchem.2021.130038
Machado, R. M., Serralheiro, R. P. (2017). Soil salinity: Effect on vegetable crop growth. management practices to prevent and mitigate soil salinization. Horticulturae 3, 30.
Mahmoud, A. W. M., Esmail, S. E. A., El-Attar, A. B., Othman, E. Z., El-Bahbohy, R. M. (2022b). Prospective practice for compound stress tolerance in thyme plants using nanoparticles and biochar for photosynthesis and biochemical ingredient stability. Agron. 12, 1069. doi: 10.3390/agronomy12051069
Mahmoud, A. W. M., Samy, M. M., Sany, H., Eid, R. R., Rashad, H. M., Abdeldaym, E. A. (2022a). Nanopotassium, nanosilicon, and biochar applications improve potato salt tolerance by modulating photosynthesis, water status, and biochemical constituents. Sustainability. 14, 723. doi: 10.3390/su14020723
Mahmoud, A. W. M., Swaefy, H. M. (2020). Comparison between commercial and nano NPK in presence of nano zeolite on sage plant yield and its components under water stress. Agric. 66, 24–39.
Manan, A., Ayyub, C. M., Ahmad, R., Bukhari, M. A., Mustafa, Z. (2016). Salinity induced deleterious effects on biochemical and physiological processes of tomato. Pak. J. Life Soc. Sci. 14 (2), 83–90.
Mannan, M., Mia, S., Halder, E., Dijkstra, F. A. (2021). Biochar application rate does not improve plant water availability in soybean under drought stress. Agric. Water Manage. 253, 106940. doi: 10.1016/j.agwat.2021.106940
Manolikaki, I., Diamadopoulos, E. (2019). Positive effects of biochar and biochar-compost on maize growth and nutrient availability in two agricultural soils. Commun. Soil Sci. Plant Anal. 50, 512–526. doi: 10.1080/00103624.2019.1566468
Mansoor, S., Kour, N., Manhas, S., Zahid, S., Wani, O. A., Sharma, V., et al. (2021). Biochar as a tool for effective management of drought and heavy metal toxicity. Chemosphere. 271, 129458. doi: 10.1016/j.chemosphere.2020.129458
Marschner, P., Hatam, Z., Cavagnaro, T. (2015). Soil respiration, microbial biomass and nutrient availability after the second amendment are influenced by legacy effects of prior residue addition. Soil Biol. Biochem. 88, 169–177. doi: 10.1016/j.soilbio.2015.05.023
Mata-Pérez, C., Sánchez-Calvo, B., Padilla, M. N., Begara-Morales, J. C., Luque, F., Melguizo, M., et al. (2015). Nitro-fatty acids in plant signaling: Nitro-linolenic acid induces the molecular chaperone network in arabidopsis. Plant Physiol. 170, 686–701. doi: 10.1104/pp.15.01671
Mclennon, E., Solomon, J. K. Q., Neupane, D., Davison, J. (2020). Biochar and nitrogen application rates effect on phosphorus removal from a mixed grass sward irrigated with reclaimed wastewater. Sci. Total Environ. 715, 137012. doi: 10.1016/j.scitotenv.2020.137012
Mehdizadeh, L., Moghaddam, M., Lakzian, A. (2020). Amelioration of soil properties, growth and leaf mineral elements of summer savory under salt stress and biochar application in alkaline soil. Sci. Hortic. 267, 109319. doi: 10.1016/j.scienta.2020.109319
Mehmood, S., Ahmed, W., Ikram, M., Imtiaz, M., Mahmood, S., Tu, S., et al. (2020). Chitosan modified biochar increases soybean (Glycine max l.) resistance to salt-stress by augmenting root morphology, antioxidant defense mechanisms and the expression of stress-responsive genes. Plant. 9, 1173.
Mickan, B. S., Abbott, L. K., Stefanova, K., Solaiman, Z. M. (2016). : Interactions between biochar and mycorrhizal fungi in a water-stressed agricultural soil. Mycorrhiza. 26, 03–10. doi: 10.1007/s00572-016-0693-4
Mikami, K., Murata, N. (2003). Membrane fluidity and the perception of environmental signals in cyanobacteria and plants. Prog. Lipid Res. 42, 527–543. doi: 10.1016/S0163-7827(03)00036-5
Minamino, Y., Fujitake, N., Suzuki, T., Yoshitake, S., Koizumi, H., Tomotsune, M. (2019). Effect of biochar addition on leaf-litter decomposition at soil surface during three years in a warm-temperate secondary deciduous forest, Japan. Sci. Rep. 9, 16961. doi: 10.1038/s41598-019-53615-2
Moragues Saitua, L., Arias-González, A., Blanco, F., Benito-Carnero, G., Gartzia-Bengoetxea, N. (2023). Effects of biochar and wood ash amendments in the soil-water-plant environment of two temperate forest plantations. Front. Forests Global Change 5, 290.
Muhammed, M. I., Tong, C. X., Kun, H., Zhou, B. Q., Xing, S. H., Mao, Y. L. (2020). Biocharfertilizer interaction modifies n-sorption, enzyme activities and microbial functional abundance regulating nitrogen retention in rhizosphere soil. Sci. Total Environ. 739, 140065. doi: 10.1016/j.scitotenv.2020.140065
Munns, R., Gilliham, M. (2015). Salinity tolerance of crops–what is the cost? New Phytol. 208, 668–673.
Mushtaq, Z., Faizan, S., Gulzar, B., Mushtaq, H., Bushra, S., Hussain, A., et al. (2022). Changes in growth, photosynthetic pigments, cell viability, lipid peroxidation and antioxidant defense system in two varieties of chickpea (Cicer arietinum l.) subjected to salinity stress. Phyton Int. J. Exp. Bot. 91, 149. doi: 10.32604/phyton.2022.016231
Naeem, M. A., Khalid, M., Aon, M., Abbas, G., Tahir, M., Amjad, M., et al. (2017). Effect of wheat and rice straw biochar produced at different temperatures on maize growth and nutrient dynamics of a calcareous soil. Arch. Agron.Soil Sci. 63, 2048–2061. doi: 10.1080/03650340.2017.1325468
Ndiate, N. I., Saeed, Q., Haider, F. U., Liqun, C., Nkoh, J. N., Mustafa, A. (2021). Co-Application of biochar and arbuscular mycorrhizal fungi improves salinity tolerance, growth and lipid metabolism of maize (Zea mays l.) in an alkaline soil. Plant. 10, 2490. doi: 10.3390/plants10112490
Ndiate, N. I., Zaman, Q. U., Francis, I. N., Dada, O. A., Rehman, A., Asif, M., et al. (2022). Soil amendment with arbuscular mycorrhizal fungi and biochar improves salinity tolerance, growth, and lipid metabolism of common wheat (Triticum aestivum l.). Sustainability. 14, 3210. doi: 10.3390/su14063210
Ndor, E., Jayeoba, O. J., Asadu, C. L. A. (2015). Effect of biochar soil amendment on soil properties and yield of sesame varieties in lafia, Nigeria. J. Exp. Agric. Int. 9, 1–8. doi: 10.9734/AJEA/2015/19637
Noman, A., Ali, Q., Maqsood, J., Iqbal, N., Javed, M. T., Rasool, N., et al. (2018). Deciphering physio-biochemical, yield, and nutritional quality attributes of water-stressed radish (Raphanus sativus l.) plants grown from zn-lys primed seeds. Chemosphere. 195, 175–189. doi: 10.1016/j.chemosphere.2017.12.059
Noreen, S., Fatima, K., Athar, H. U. R., Ahmad, S., Hussain, K. (2017). Enhancement of physio-biochemical parameters of wheat through exogenous application of salicylic acid under drought stress. J. Anim. Plant Sci. 27 (1), 153–163.
Novak, J. M., Busscher, W. J., Laird, D. L., Ahmedna, M., Watts, D. W., Niandou, M. A. (2009). Impact of biochar amendment on fertility of a southeastern coastal plain soil. Soil Sci. 174, 105–112. doi: 10.1097/SS.0b013e3181981d9a
Odugbenro, G. O., Liu, Z., Sun, Y. (2020). Soil aggregate size distribution and total organic carbon in intra-aggregate fractions as affected by addition biochar and organic amendments. Pol. J. Soil Sci. 53, 41–54. doi: 10.17951/pjss.2020.53.1.41
Ohtsuka, T., Tomotsune, M., Ando, M., Tsukimori, Y., Koizumi, H., Yoshitake, S. (2021). Effects of the application of biochar to plant growth and net primary production in an oak forest. Forests 12, 152. doi: 10.3390/f12020152
Oliveira, F. R., Patel, A. K., Jaisi, D. P., Adhikari, S., Lu, H., Khanal, S. K. (2017). Environmental application of biochar: Current status and perspectives. Bioresour. Technol. 246, 110–122. doi: 10.1016/j.biortech.2017.08.122
Olmo, M., Alburquerque, J. A., Barrón, V., del Campillo, M. C., Gallardo, A., Fuentes, M., et al. (2014). Wheat growth and yield responses to biochar addition under Mediterranean climate conditions. Biol. Fert. Soil. 50, 1177–1187. doi: 10.1007/s00374-014-0959-y
Palansooriya, K. N., Ok, Y. S., Awad, Y. M., Lee, S. S., Sung, J. K., Koutsospyros, A., et al. (2019). Impacts of biochar application on upland agriculture: A review. J. Environ. Manage. 234, 52–64. doi: 10.1016/j.jenvman.2018.12.085
Palviainen, M., Aaltonen, H., Laurén, A., Köster, K., Berninger, F., Ojala, A., et al. (2020). Biochar amendment increases tree growth in nutrient-poor, young scots pine stands in Finland. For. Ecol. Manage. 474, 118362. doi: 10.1016/j.foreco.2020.118362
Pandey, S., Patel, M. K., Mishra, A., Jha, B. (2015). Physio-biochemical composition and untargeted metabolomics of cumin (Cuminum cyminum l.) make it promising functional food and help in mitigating salinity stress. PloS One 10, e0144469.
Paneque, M., José, M., Franco-Navarro, J. D., Colmenero-Flores, J. M., Knicker, H. (2016). Effect of biochar amendment on morphology, productivity and water relations of sunflower plants under non-irrigation conditions. Catena. 147, 280–287. doi: 10.1016/j.catena.2016.07.037
Parida, A. K., Das, A. B. (2005). Salt tolerance and salinity effects on plants: A review. Ecotoxicol. Environ. Saf. 60, 324–349. doi: 10.1016/j.ecoenv.2004.06.010
Parveen, A., Liu, W., Hussain, S., Asghar, J., Perveen, S., Xiong, Y. (2019). Silicon priming regulates morpho-physiological growth and oxidative metabolism in maize under drought stress. Plant. 8, 431. doi: 10.3390/plants8100431
Poormansour, S., Razzaghi, F., Sepaskhah, A. R. (2019). Wheat straw biochar increases potassium concentration, root density, and yield of faba bean in a sandy loam soil. commun. Soil Sci. Plant Anal. 50, 1799–1810. doi: 10.1080/00103624.2019.1635145
Qin, H., Wang, J., Chen, X., Wang, F., Peng, P., Zhou, Y., et al. (2019). Rice os DOF 15 contributes to ethylene-inhibited primary root elongation under salt stress. New Phytol. 223, 798–813. doi: 10.1111/nph.15824
Qu, Z. L., Li, X. L., Ge, Y., Palviainen, M., Zhou, X., Heinonsalo, J., et al. (2022). The impact of biochar on wood-inhabiting bacterial community and its function in a boreal pine forest. Environ. Microb. 17, 1–15. doi: 10.1186/s40793-022-00439-9
Raboin, L. M., Razafimahafaly, A. H. D., Rabenjarisoa, M. B., Rabary, B., Dusserre, J., Becquer, T. (2016). Improving the fertility of tropical acid soils: Liming versus biochar application? a long term comparison in the highlands of Madagascar. Field Crops Res. 199, 99–108. doi: 10.1016/j.fcr.2016.09.005
Racioppi, M., Tartaglia, M., de la Rosa, J. M., Marra, M., Lopez-Capel, E., Rocco, M. (2019). Response of ancient and modern wheat varieties to biochar application: effect on hormone and gene expression involved in germination and growth. Agron. 10, p.5. doi: 10.3390/agronomy10010005
Rafique, M., Ortas, I., Rizwan, M., Chaudhary, H. J., Gurmani, A. R., Munis, M. F. H. (2020). Residual effects of biochar and phosphorus on growth and nutrient accumulation by maize (Zea mays l.) amended with microbes in texturally different soils. Chemosphere 238, 124710. doi: 10.1016/j.chemosphere.2019.124710
Rahman, M. H., Ahmad, I., Wang, D., Fahad, S., Afzal, M., Ghaffar, A., et al. (2021). Influence of semi-arid environment on radiation use efficiency and other growth attributes of lentil crop. Environ. Sci. pollut. Res. 28, 13697–13711. doi: 10.1007/s11356-020-11376-w
Rahman, M. H., Ahmad, A., Wang, X., Wajid, A., Nasim, W., Hussain, M., et al. (2018). Multi-model projections of future climate and climate change impacts uncertainty assessment for cotton production in Pakistan. Agric. For. Meteorol. 253, 94–113. doi: 10.1016/j.agrformet.2018.02.008
Rajakumar, R., Sankar, J. (2016). Biochar for sustainable agriculture – a review. Int. J. Appl. Pure Sci. Agric. 2, 173–184.
Ramzani, P. M. A., Shan, L., Anjum, S., Ronggui, H., Iqbal, M., Virk, Z. A., et al. (2017). Improved quinoa growth, physiological response, and seed nutritional quality in three soils having different stresses by the application of acidified biochar and compost. Plant Physiol. Biochem. 116, 127–138. doi: 10.1016/j.plaphy.2017.05.003
Ran, C., Gulaqa, A., Zhu, J., Wang, X., Zhang, S., Geng, Y., et al. (2020). ). benefits of biochar for improving ion contents, cell membrane permeability, leaf water status and yield of rice under saline–sodic paddy field condition. J. Plant Growth Regul. 39, 370–377. doi: 10.1007/s00344-019-09988-9
Rasheed, R., Parveen, A., Riaz, M., Arif, M. S., Hussain, I., Firdous, S., et al. (2019). Soil biochar ameliorates salinity stress and improves nutrient uptake, biomass production and physiochemical parameters in sunflower. Int. J. Agric. Biol. 22, 1663–1674.
Raza, A., Tabassum, J., Fakhar, A. Z., Sharif, R., Chen, H., Zhang, C., et al. (2022). Smart reprograming of plants against salinity stress using modern biotechnological tools. Crit. Rev. Biotechnol. 15, 1–28. doi: 10.1080/07388551.2022.2093695
Rodríguez-Vila, A., Forján, R., Guedes, R. S., Covelo, E. F. (2016). Changes on the phytoavailability of nutrients in a mine soil reclaimed with compost and biochar. Water Air Soil pollut. 227, 453. doi: 10.1007/s11270-016-3155-x
Roy, R., Núñez-Delgado, A., Wang, J., Kader, M. A., Sarker, T., Hasan, A. K., et al. (2022). Cattle manure compost and biochar supplementation improve growth of onobrychis viciifolia in coal-mined spoils under water stress conditions. Environ. Res. 205, 112440. doi: 10.1016/j.envres.2021.112440
Ryu, H., Cho, Y. G. (2015). Plant hormones in salt stress tolerance. J. Plant Biol. 58, 147–155. doi: 10.1007/s12374-015-0103-z
Şahin, Ö., Taskin, M. B., Kaya, E. C., Taskin, H. (2016). Fosfor ile zenginleştirilmiş biyokömürün marul bitkisinin (Lactucasativa l. cv. maritima) gelişimi ve mineral element konsantrasyonu Üzerine etkisi. Çukurova Tarım Ve Gıda Bilimleri Dergisi 31, 101–107.
Saddiq, M. S., Iqbal, S., Hafeez, M. B., Ibrahim, A. M., Raza, A., Fatima, E. M., et al. (2021). Effect of salinity stress on physiological changes in winter and spring wheat. Agron. 11, 1193. doi: 10.3390/agronomy11061193
Safari, S., Nazari, F., Vafaee, Y., Teixeira da Silva, J. A. (2022). Impact of rice husk biochar on drought stress tolerance in perennial ryegrass (Lolium perenne l.). J. Plant Growth Regul. 42, 1–17.
Saibi, W. (2021). “Brini faiçal,” in Oxidative stress and antioxidant defense in brassicaceae plants under abiotic stresses, pp 232–pp 244.
Salama, D. M., Abd El-Aziz, M. E., El-Naggar, M. E., Shaaban, E. A., Abd EL-Wahed, M. S. (2021). Synthesis of an eco-friendly nanocomposite fertilizer for common bean based on carbon nanoparticles from agricultural waste biochar. Pedosphere 31, 923–933. doi: 10.1016/S1002-0160(21)60024-3
Sanaullah, M., Blagodatskaya, E., Chabbi, A., Rumpel, C., Kuzyakov, Y. (2011). Drought effects on microbial biomass and enzyme activities in the rhizosphere of grasses depend on plant community composition. Appl. Soil Ecol. 48, 38–44. doi: 10.1016/j.apsoil.2011.02.004
Sánchez-Rodríguez, C., Rubio-Somoza, I., Sibout, R., Persson, S. (2010). Phytohormones and the cell wall in arabidopsis during seedling growth. Trends Plant Sci. 15, 291–301. doi: 10.1016/j.tplants.2010.03.002
Santander, C., Aroca, R., Cartes, P., Vidal, G., Cornejo, P. (2021). Aquaporins and cation transporters are diferentially regulated by two arbuscular mycorrhizal fungi strains in lettuce cultivars growing under salinity conditions. Plant Physiol. Biochem. 158, 396–409. doi: 10.1016/j.plaphy.2020.11.025
Seleiman, M. F., Refay, Y., Al-Suhaibani, N., Al-Ashkar, I., El-Hendawy, S., Hafez, E. M. (2019). Integrative effects of rice-straw biochar and silicon on oil and seed quality, yield and physiological traits of helianthus annuus l. grown under water deficit stress. Agron. 9, 637.
Shabbir, A., Saqib, M., Murtaza, G., Abbas, G., Imran, M., Rizwan, M., et al. (2021). Biochar mitigates arsenic-induced human health risks and phytotoxicity in quinoa under saline conditions by modulating ionic and oxidative stress responses. Environ. pollut. 287, 117348. doi: 10.1016/j.envpol.2021.117348
Shafiq, S., Akram, N. A., Ashraf, M. (2019). Assessment of physio-biochemical indicators for drought tolerance in different cultivars of maize (Zea mays l.). Pak. J. Bot. 51, 1241–1247. doi: 10.30848/PJB2019-4(21)
Shashi, M. A., Mannan, M. A., Islam, M. M., Rahman, M. M. (2018). Impact of rice husk biochar on growth, water relations and yield of maize (Zea mays l.) under drought condition. Agric. 16, 93–101.
Siebielec, S., Siebielec, G., Klimkowicz-Pawlas, A., Gałązka, A., Grządziel, J., Stuczyński, T. (2020). Impact of water stress on microbial community and activity in sandy and loamy soils. Agron. 10, 1429. doi: 10.3390/agronomy10091429
Singh, M., Saini, R. K., Singh, S., Sharma, S. P. (2019). Potential of integrating biochar and deficit irrigation strategies for sustaining vegetable production in water-limited regions: A review. HortSci. 54, 1872–1878. doi: 10.21273/HORTSCI14271-19
Singh, J., Takhur, J. K. (2018). “Photosynthesis and abiotic stress in plants,” in Biotic and abiotic stress tolerance in plants. Ed. Vats, S. (Singapore: Springer Nature Singapore Private Ltd), 27–46.
Sofy, M. R., Elhawat, N., Alshaal, T. (2020). Glycine betaine counters salinity stress by maintaining high K+/Na+ ratio and antioxidant defense via limiting na+ uptake in common bean (Phaseolus vulgaris l.). Ecotoxicol. Environ. Saf. 200, 110732. doi: 10.1016/j.ecoenv.2020.110732
Sofy, M., Mohamed, H., Dawood, M., Abu-Elsaoud, A., Soliman, M. (2021). Integrated usage of trichoderma harzianum and biochar to ameliorate salt stress on spinach plants. Arch. Agron. Soil Sci. 68, 1–22.
Soliman, M. H., Alnusairi, G. S., Khan, A. A., Alnusaire, T. S., Fakhr, M. A., Abdulmajeed, A. M., et al. (2022). Biochar and selenium nanoparticles induce water transporter genes for sustaining carbon assimilation and grain production in salt-stressed wheat. J.Plant Growth Regul., 1–22.
Sorrenti, G., Masiello, C. A., Toselli, M. (2016). Biochar interferes with kiwifruit fe-nutrition in calcareous soil. Geoderma. 272, 10–19. doi: 10.1016/j.geoderma.2016.02.017
Sultan, I., Khan, I., Chattha, M. U., Hassan, M. U., Barbanti, L., Calone, R., et al. (2021). Improved salinity tolerance in early growth stage of maize through salicylic acid foliar application. Ital. J. Agron. 16 (3), 1–11. doi: 10.4081/ija.2021.1810
Sun, H., Lu, H., Chu, L., Shao, H., Shi, W. (2017). Biochar applied with appropriate rates can reduce n leaching, keep n retention and not increase NH3 volatilization in a coastal saline soil. Sci. Total Environ. 575, 820–825. doi: 10.1016/j.scitotenv.2016.09.137
Sunaina, B., Kumar, J. R., Rupak, K., Mahesh, R. A. (2019). Case study on soil fertility status and maize productivity in dang district, nepal. malays. J. Sustain. Agric. 3, 56–59. doi: 10.26480/mjsa.02.2019.56.59
Tang, H., Shubin, W., Ying, L., Hassan, M. U., Ying, S., Huang, G., et al. (2022). Biochar: A promising soil amendment to mitigate heavy metals toxicity in plants. Not. Bot. Hortic. Agrobot. Cluj-Napoca 50, 12778–12778. doi: 10.15835/nbha50312778
Thomas, S. C., Frye, S., Gale, N., Garmon, M., Launchbury, R., Machado, N., et al. (2013). Biochar mitigates negative effects salt additions two herbaceous Plant species. J. Environ. Manage. 129, 62–68. doi: 10.1016/j.jenvman.2013.05.057
Torabian, S., Farhangi-Abriz, S., Rathjen, J. (2018). Biochar and lignite affect H+-ATPase and H+-PPase activities in root tonoplast and nutrient contents of mung bean under salt stress. Plant Physiol. Biochem. 129, 141–149. doi: 10.1016/j.plaphy.2018.05.030
Turan, V. (2021). Arbuscular mycorrhizal fungi and pistachio husk biochar combination reduces Ni distribution in mungbean plant and improves plant antioxidants and soil enzymes. Physiol. Plant 173, 1–12. doi: 10.1111/ppl.13490
Usman, A. R. A., Al-Wabel, M. I., Abdulaziz, A. H., Mahmoud, W. A., EL-Naggar, A. H., Ahmad, M., et al. (2016). Conocarpus biochar induces changes in soil nutrient availability and tomato growth under saline irrigation. Pedosphere. 26, 27–38. doi: 10.1016/S1002-0160(15)60019-4
Van Zwieten, L., Kimber, S., Morris, S., Chan, K. Y., Downie, A., Rust, J., et al. (2010). Effects of biochar from slow pyrolysis of papermill waste on agronomic performance and soil fertility. Plant Soil. 327, 235–246. doi: 10.1007/s11104-009-0050-x
Verheijen, F., Jeffery, S., Bastos, A. C., van der Velde, M., Diafas, I., Parsons, C., et al. (2009). Biochar application to soils: a critical scientific review of effects on soil properties, processes and functions (Ispra, Italy: Institute for Environment and Sustainability).
Viger, M., Hancock, R. D., Miglietta, F., Taylor, G. (2015). More plant growth but less plant defence? first global gene expression data for plants grown in soil amended with biochar. GCB Bioenergy. 7, 658–672. doi: 10.1111/gcbb.12182
Vijayaraghavareddy, P., Lekshmy, S. V., Struik, P. C., Makarla, U., Yin, X., Sreeman, S. (2022). Production and scavenging of reactive oxygen species confer to differential sensitivity of rice and wheat to drought stress. Crop Environ. 1, 15–23. doi: 10.1016/j.crope.2022.03.010
Vissenberg, K., Oyama, M., Osato, Y., Yokoyama, R., Verbelen, J. P., Nishitani, K. (2005). Differential expression of AtXTH17, AtXTH18, AtXTH19 and AtXTH20 genes in arabidopsis roots. physiological roles in specification in cell wall construction. Plant Cell Physiol. 46, 192–200. doi: 10.1093/pcp/pci013
Wang, H., Ren, T., Feng, Y., Liu, K., Feng, H., Liu, G., et al. (2020). Effects of the application of biochar in four typical agricultural soils in China. Agron. 10, 1649. doi: 10.3390/agronomy10111649
Wang, Y., Xia, H., Yao, Z., Jiang, C. (2018). Effects of biochar and other amendments on the cabbage growth and soil fertility in yellow-brown soil and red soil. Sci. Agric. Sinica. 51, 4306–4315.
Wang, S., Zheng, J., Wang, Y., Yang, Q., Chen, T., Chen, Y., et al. (2021). Photosynthesis, chlorophyll fluorescence, and yield of peanut in response to biochar application. Front. Plant Sci. 12, 1000. doi: 10.3389/fpls.2021.650432
Warnock, D. D., Lehmann, J., Kuyper, T. W., Rillig, M. C. (2007). Mycorrhizal responses to biochar in soil – concepts and mechanisms. Plant Soil. 300, 9–20. doi: 10.1007/s11104-007-9391-5
Winsley, P. (2007). Biochar and bioenergy production for climate change mitigation. New Z. Sci. Rev. 64, 5–10.
Xu, C. Y., Bai, S. H., Hao, Y., Rachaputi, R. C. N., Xu, Z., Wallace, H. M. (2015). Peanut shell biochar improves soil properties and peanut kernel quality on a red ferrosol. J. Soils Sediments. 15, 2220–2231. doi: 10.1007/s11368-015-1242-z
Xu, G., Zhang, Y., Sun, J., Shao, H. (2016). Negative interactive effects between biochar and phosphorus fertilization on phosphorus availability and plant yield in saline sodic soil. Sci. Total Environ. 568, 910–915. doi: 10.1016/j.scitotenv.2016.06.079
Yadegari, M. (2017). Efects of zn, fe, Mn and Cu foliar application on essential oils and morpho-physiological traits of lemon balm (Melissa ofcinalis l.). J. Essent. Oil-Bear. Plant 20, 485–495. doi: 10.1080/0972060X.2017.1325010
Yamato, M., Okimori, Y., Wibowo, I. F., Anshori, S., Ogawa, M. (2006). ). effects of the application of charred bark of acacia mangium on the yield of maize, cowpea and peanut, and soil chemical properties in south Sumatra, Indonesia. Soil Sci. Plant Nutr. 52, 489–495. doi: 10.1111/j.1747-0765.2006.00065.x
Yang, C. D., Lu, S. G. (2020). Dynamic effects of direct returning of straw and corresponding biochar on acidity, nutrients, and exchangeable properties of red soil. Huan Jing Xue Huanjing Kexue. 41, 4246–4252.
Yao, Q., Liu, J., Yu, Z., Li, Y., Jin, J., Liu, X., et al. (2017). Three years of biochar amendment alters soil physiochemical properties and fungal community composition in a black soil of northeast China. Soil Biol. Biochem. 110, 56–67. doi: 10.1016/j.soilbio.2017.03.005
Ye, J., Zhang, R., Nielsen, S., Joseph, S. D., Huang, D., Thomas, T. (2016). A combination of biochar–mineral complexes and compost improves soil bacterial processes, soil quality, and plant properties. Front. Microbiol. 7, 372. doi: 10.3389/fmicb.2016.00372
Yildirim, E., Ekinci, M., Turan, M. (2021). Impact of biochar in mitigating the negative effect of drought stress on cabbage seedlings. J. Soil Sci. Plant Nutr. 21, 2297–2309. doi: 10.1007/s42729-021-00522-z
Zaheer, M. S., Ali, H. H., Soufan, W., Iqbal, R., Habib-ur-Rahman, M., Iqbal, J., et al. (2021). Potential effects of biochar application for improving wheat (Triticum aestivum l.) growth and soil biochemical properties under drought stress conditions. Land. 10, 1125.
Zainul, A., Koyro, H. W., Huchzermeyer, B., Gul, B., Khan, M. A. (2017). Impact of a biochar or a compost-biochar mixture on water relation, nutrient uptake and photosynthesis of phragmites karka. Pedosphere. 160, 1–22.
Zhang, Z., Dong, X., Wang, S., Pu, X. (2020). Benefits of organic manure combined with biochar amendments to cotton root growth and yield under continuous cropping systems in xinjiang, China. Sci. Rep. 10, 1–10. doi: 10.1038/s41598-020-61118-8
Zhang, C., Lin, Y., Tian, X., Xu, Q., Chen, Z., Lin, W. (2017). Tobacco bacterial wilt suppression with biochar soil addition associates to improved soil physiochemical properties and increased rhizosphere bacteria abundance. Appl. Soil Ecol. 112, 90–96. doi: 10.1016/j.apsoil.2016.12.005
Zhang, M., Riaz, M., Zhang, L., El-Desouki, Z., Jiang, C. (2019). Biochar induces changes to basic soil properties and bacterial communities of different soils to varying degrees at 25 mm rainfall: More effective on acidic soils. Front. Microbiol. 10, 1321. doi: 10.3389/fmicb.2019.01321
Zhang, Y., Wang, J., Feng, Y. (2021). The effects of biochar addition on soil physicochemical properties: A review. Catena 202, 105284. doi: 10.1016/j.catena.2021.105284
Zhao, Z. Y., Wang, P. Y., Xiong, X. B., Wang, Y. B., Zhou, R., Tao, H. Y., et al. (2022). Environmental risk of multi-year polythene film mulching and its green solution in arid irrigation region. J. Hazard. Mater. 435, 128981. doi: 10.1016/j.jhazmat.2022.128981
Zheng, H., Wang, X., Chen, L., Wang, Z., Xia, Y., Zhang, Y., et al. (2018a). Enhanced growth of halophyte plants in biochar-amended coastal soil: Roles of nutrient availability and rhizosphere microbial modulation. Plant Cell Environ. 41, 517–532. doi: 10.1111/pce.12944
Zheng, H., Wang, X., Luo, X., Wang, Z., Xing, B. (2018b). Biochar-induced negative carbon mineralization priming effects in a coastal wetland soil: Roles of soil aggregation and microbial modulation. Sci. Total Environ. 610, 951–960. doi: 10.1016/j.scitotenv.2017.08.166
Zhou, J., Wang, X., Jiao, Y., Qin, Y., Liu, X., Zhang, Q., et al. (2007). Global genome expression analysis of rice in response to drought and high-salinity stresses in shoot, flag leaf, and panicle. Plant Mol. Biol. 63, 591–608. doi: 10.1007/s11103-006-9111-1
Zoghi, Z., Hosseini, S. M., Kouchaksaraei, M. T., Kooch, Y., Guidi, L. (2019). The effect of biochar amendment on the growth, morphology and physiology of quercus castaneifolia seedlings under water-deficit stress. Eur. J. For. Res. 138, 967–979. doi: 10.1007/s10342-019-01217-y
Keywords: antioxidants, biochar, drought, osmotic stress, salinity, soil organic matter
Citation: Wu Y, Wang X, Zhang L, Zheng Y, Liu X and Zhang Y (2023) The critical role of biochar to mitigate the adverse impacts of drought and salinity stress in plants. Front. Plant Sci. 14:1163451. doi: 10.3389/fpls.2023.1163451
Received: 10 February 2023; Accepted: 23 March 2023;
Published: 08 May 2023.
Edited by:
Muhammad Umair Hassan, Jiangxi Agricultural University, ChinaReviewed by:
Mehmood Ali Noor, University of Okara, PakistanMuhammad Umer Chattha, University of Agriculture, Faisalabad, Pakistan
Copyright © 2023 Wu, Wang, Zhang, Zheng, Liu and Zhang. This is an open-access article distributed under the terms of the Creative Commons Attribution License (CC BY). The use, distribution or reproduction in other forums is permitted, provided the original author(s) and the copyright owner(s) are credited and that the original publication in this journal is cited, in accordance with accepted academic practice. No use, distribution or reproduction is permitted which does not comply with these terms.
*Correspondence: Yanfang Wu, eWFuZmFuZ3d1MjAxMkAxNjMuY29t