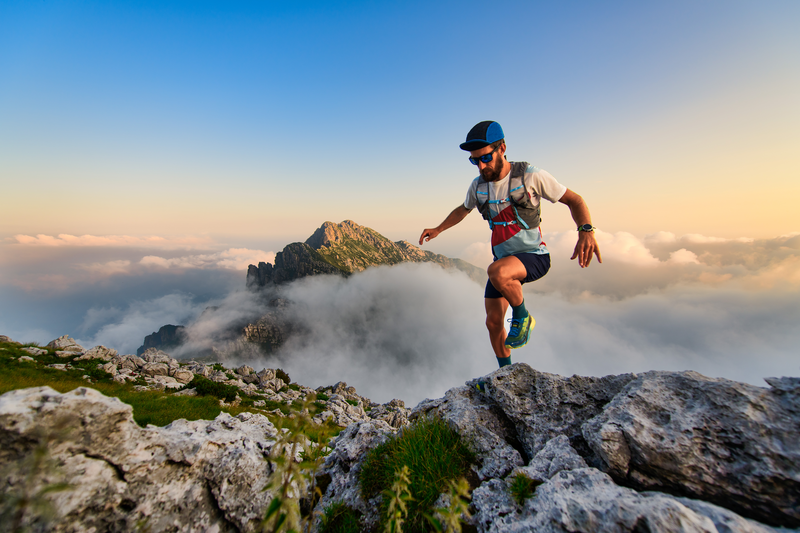
94% of researchers rate our articles as excellent or good
Learn more about the work of our research integrity team to safeguard the quality of each article we publish.
Find out more
REVIEW article
Front. Plant Sci. , 10 July 2023
Sec. Plant Pathogen Interactions
Volume 14 - 2023 | https://doi.org/10.3389/fpls.2023.1156869
This article is part of the Research Topic Detection, characterization, and management of plant pathogens View all 29 articles
A correction has been applied to this article in:
Corrigendum: Ganoderma boninense: general characteristics of pathogenicity and methods of control
Ganoderma boninense (G. boninense) is a soil-borne fungus threatening oil palm at the present. It causes basal stem rot disease on oil palm. Within six months, this fungus can cause an oil palm plantation to suffer a significant 43% economic loss. The high persistence and nature of spread of G. boninense in soil make control of the disease challenging. Therefore, controlling the pathogen requires a thorough understanding of the mechanisms that underlie pathogenicity as well as its interactions with host plants. In this paper, we present the general characteristics, the pathogenic mechanisms, and the host’s defensive system of G. boninense. We also review upcoming and most promising techniques for disease management that will have the least negative effects on the environment and natural resources.
Basal stem rot (BSR), which has resulted in significant losses in oil palm plantations, is caused by Ganoderma boninense (G. boninense), a basidiomycete that is a member of the Polyporaceae family. However, other Ganoderma species have been linked to BSR (Chong et al., 2016). One of the major plantation crops in Malaysia and Indonesia is oil palm (Elaeis guineensis Jacq. Dura x Pisifera), and these two nations together account for 85 to 90% of global export (Kadarusman and Herabadi, 2018; Varkkey et al., 2018). According to studies by Stichnothe et al. (2014) and Kurnia et al. (2016), palm oil, which is widely recognised as one of the major sources of edible oil, also serves as a feedstock for oleochemicals and a precursor for biodiesel fuel. Nearly $24.66 billion in total export revenues from palm oil and palm oil products were reported for Malaysia (Parveez et al., 2022).
Despite the substantial export earnings from this product, oil palm plantations are hampered by the basal stem rot (BSR) disease, which severely reduces oil palm productivity and life cycle. For more than eight decades, BSR has been regarded as a serious threat to the oil palm sector. According to estimation by Olaniyi and Szulczyk (2020), G. boninense can wreak havoc on Malaysia’s oil palms by 2040, wiping out 860,610 ha of mature oil palm trees in the process. However, to date there is no published data on the exact damage areas caused by BSR. The most frequent species of fungus found to be the causal pathogen of BSR is G. boninense (Moncalvo, 2000; Susanto et al., 2005; Rees et al., 2007). In addition to mature oil palm trees, BSR also affects seedlings and younger plants, where the disease manifests quickly and severely (Susanto et al., 2005). BSR is characterised by the gradual decay of roots, bole and trunk tissue, which prevents water and nutrients from reaching the upper portion of adult oil palm trees. As a result, frond wilting, frond yellowing, un-opening of spear leaves, and eventually stand collapse occur (Chung, 2011). Unfortunately, oil palms infected with BSR are asymptomatic in the early stages of the infection, with the earliest symptom frequently being noticed on foliage when the infection has developed by 60–70% (Chong et al., 2017a). Young oil palm plants that exhibit disease symptoms typically die within 1 to 2 years, while mature trees only have a 3-to-5-year life expectancy (Corley and Tinker, 2003).
G. boninense management strategies continue to be difficult to implement despite extensive research efforts to control the diseases. In fact, interactions between the host plant, the pathogen, and the biotic and abiotic elements of the environment lead to diseases brought on by this soil pathogen are less explored. Therefore, our goals in this review are to (1) describe the general characteristics of G. boninense, (2) report the most recent findings regarding the mechanisms underlying pathogenicity as well as the interactions between the fungal pathogen and its host plants and/or other microorganisms, and (3) review the most effective current and upcoming control methods.
G. boninense, a white rot fungus that degrade the lignin component of wood, belongs to the Basidiomycetes class under Ganodermataceae family (Paterson, 2007). Several Ganoderma spp. viz. G. applanatum, G. boninense, G. chalceum, G. lucidum, G. miniatocinctum, G. pseudoferreu and G. tornatum have been reported related to the oil palm basal stem rot in early investigations (Turner, 1981). However, G. boninense is the virulent species causing high incidence of BSR disease (Idris, 1999), and also a major species harmful to the oil palm (Moncalvo, 2000).
The colonies of G. boninense were distinguished morphologically as having a white colour on the surface and dark pigment on the reverse. Under the dark condition, G. boninense cultures developed an undulating surface that buckled the agar. The ideal temperature for G. boninense growth is 30°C, and it can grow there at pH 3-8.5. Growth is severely hampered at 15°C and 35°C, and it is impossible above 40°C (Idris et al., 2000). After one to three weeks of incubation on rubber wood block (RWB), a white mycelium that later transformed into a small, white, button-like structure served as the initial indicator that a basidiomata of G. boninense had been artificially produced. When the bracket-like structures first emerged, they were typically white. However, as their length and width rapidly extended, their upper surfaces took on a variety of yellowish-brown colours with concentric zonations (Idris, 2009). Large, woody basidiocarps that are perennial are a characteristic of G. boninense. On the stems of infected palms, their fruiting bodies often develop into a fan-like pattern and contain double-walled, truncate spores with inner layers that range in colour from yellow to brown (Chong et al., 2017a).
Contact between roots and a source of inoculum, such as stumps, trunks, and diseased oil palms, is related to the spread of BSR to healthy palms (Ariffin et al., 2000). Animals, insects, and the wind are all examples of vectors that can disperse the basidiospores that G. boninense fruiting bodies produce (Pilotti et al., 2003). Mycelia (monokaryotic form), which the basidiospore germinates into, is not pathogenic to the oil palm tree (Turner, 1965). The pathogenic dikaryotic mycelia are produced through mating between suitable mates of choice (monokaryon/dikaryon) (Freihorst et al., 2016). Then, infection occurred when the oil palm tree’s root and basal stem are colonised by the dikaryotic mycelia (Ariffin et al., 1996; Rees et al., 2009). In order to create a needle-like structure that will help them penetrate the host cells, dikaryotic mycelia will go through hyphae morphogenesis (Govender and Wong, 2017; Govender et al., 2020). Basidiocarps will form on the infected tree’s basal stem. As a result, the oil palm tree is infected with G. boninense (Rees et al., 2007). The disease cycle is summarised in Figure 1.
Failure to identify symptoms and signs of infection at an early stage of disease development is a practical barrier to control of G. boninense disease in oil palm. The pathogen typically destroys a significant amount of a palm’s base before signs of infection appear (Darmono, 2000). In recent decades, many diagnostics tools have been developed and introduced to detect G. boninense. Milestones achieved in the development of diagnostic tools for detection of G. boninense are listed in Table 1.
Table 1 Achievement of milestones in the development of diagnostic tools for detection of G. boninense.
The state of the art of CRISPR–Cas system has revolutionized nucleic acid detection. A recent study has adopted CRISPR-Cas12a as a diagnostic tool for detection of wheat fungi (Mu et al., 2022) and Leptosphaeria maculans (Lei et al., 2022). The advantages of robust nucleic acid detection make CRISPR-Cas12 widely used in plant disease and pest management (Karmakar et al., 2022). Based on those achievement, CRISPR-Cas12a is potentially to be developed as Ganoderma detection kit used in the field.
Amplification fragment length polymorphisms (AFLPs) has been combined with mitochodrial (mtDNA) profiling to provide a more comprehensive view of the molecular variation among the Ganoderma isolates (Rolph et al., 2000). After 7 years, microsatellite markers were used to study more about the genetic variation and population structure of G. boninense in Malaysia and Indonesia. The isolates of G. boninense from Peninsular Malaysia and Sumatra, Indonesia, showed a high level of genetic variation based on information from 11 microsatellite markers. The lack of genetic difference between G. boninense isolates from these two regions suggested that airborne spores may be a cause in the spread of G. boninense in these two regions (Mercière et al., 2017).
Based on the molecular characteristics of these Ganoderma species, the studies have revealed a high level of genetic variation among monokaryons of G. boninense, indicating that this species is genetically heterogeneous and may have developed from the same species with widespread genetic variation or from closely related species (Zakaria et al., 2005). This genetic heterogeneity may have been caused by different geographic locations or outcrossing of the isolates over generations or generation of new genetically distinct individuals through spore dispersal in the process of disease spread (Miller et al., 1999; Pilotti et al., 2003; Mohd Rakib et al., 2014). According to Pilotti (2005), G. boninense possesses a tetrapolar mating system, numerous alleles at both mating type loci, and is heterothallic, which encourages out-breeding within a population (Sanderson and Pilloti, 1997; Pilotti, 2005). Therefore, the extensive genetic variety exhibited in the G. boninense population on oil palm is caused by this potential to outcross.
G. boninense in Peninsular Malaysia, Sarawak, Malaysia, and Sumatra, Indonesia showed a significant degree of genetic variation when using 16 polymorphic microsatellite markers (Wong et al., 2021). In contrast to the low genetic variation reported in previous studies, due to G. boninense’s basidiospores’ outcrossing, high genetic variation was seen among isolates from the same population as well as between populations. G. boninense populations showed high levels of gene flow, but no genetic difference was found, suggesting that the BSR pathogen populations in three oil palm growing locations were homogeneous. Conclusively, Peninsular Malaysia, Sarawak, Malaysia, and Sumatra, Indonesia all have three admixed genetic clusters of the pathogen population (Wong et al., 2021). Conspecificity of G. boninense isolates from Indonesia, Malaysia, Papua New Guinea, and the Solomon Islands was shown based on mating studies, ITS sequences, and microsatellite genotyping (Pilotti et al., 2021). G. boninense isolates found in eight coastal sites in the Malaysian state of Sarawak showed low genetic variation. Using sequences from the internal transcribed spacer (ITS) region, low genetic variation was found in 117 isolates. The plantation was a first-generation planting that was formerly a secondary forest, which may be related to low disease pressure, which may account for the minimal genetic variation. First-generation plantation isolates of G. boninense are thought to be founder populations that eventually adapted to oil palm as the host (Midot et al., 2019).
Numerous studies have uncovered defense-related genes involved in G. boninense infection of oil palm. Several genes related to oil palm defensive mechanisms were discovered by Tee et al. (2013), including genes associated to phytoalexin synthesis and genes associated to plant signal transduction. When the oil palm was infected by G. boninense, these genes were either upregulated to strengthen defence mechanisms or downregulated to weaken them. Ho et al. (2016) examined the transcriptomes of oil palm seedlings that had been infected and those that had not in order to deduce the molecular reactions of oil palm roots to the infection with G. boninense. Reactive oxygen species (ROS) such as hydrogen peroxide, which play a role in the oxidation of lipids and lignification of plant cells as well as acting as a signalling molecule inside the plant, are produced when enzymes like chitinases and glucanases are up-regulated (Ho et al., 2016).
Bahari et al. (2018) detected upregulated and downregulated gene sequences related to the change from the biotrophic to the necrotrophic phases of G. boninense in another transcriptome research. ROS elicitors, transcription factors EgERF113 and EgMYC2, defense-related genes (pathogenesis-related proteins, protease inhibitors, and chitinase), and defense-related genes were all differentially elevated (peroxidase and NADPH oxidase).
In another study, upon G. boninense infection, the regulation of a number of oil palm genes changes. Receptor-like kinases and proteins (RLKs and RLPs), which are important in recognising the pathogen, are extensively produced during the early stages of infection (Rosli et al., 2018).
In 2021, through RNA-sequencing (RNA-seq) transcriptomic libraries of oil palm roots infected with G. boninense, seven differentially expressed genes (DEGs) have been detected. These seven DEGs are anthocyanidin synthase, chalcone synthase-like, transcription factor 1B-like, leucoanthocyanidin reductase-like, mannose-specific lectin-like, putative senescence-associated protein, thaumatin-like protein. During G. boninense infection, seedlings and mature plants consistently displayed upregulation of seven putative defense-related DEGs. These seven genes may be developed into biomarkers for the early detection of BSR in oil palm (Mohd Zuhar et al., 2021).
An earlier study revealed that various methods, viz. debris, root contact and basidiospores, are used by Ganoderma sp. for colonisation in the oil palm plantation (Turner, 1965; Pilotti et al., 2003; Pilotti et al., 2018; Govender et al., 2020). Turner (1965) showed that G. boninense can continue to spread via debris from colonised oil palm trees after new plants are planted. Besides, mycelia can colonise on healthy, damaged or dead roots (Miller et al., 1999; Govender et al., 2020). The continuous grow of the oil palm roots to the first four planting rows lead to the root-to-root contact between palms, which facilitates the spread of pathogen (Soepena et al., 2000). The increasing number of patches caused by BSR infection over time support the theory stated the roots are the main source of inoculum in the field (Miller et al., 1999). Infective dikaryotic mycelia continue to form when germinated basidiospores mating with the mycelia (Pilotti et al., 2003; Pilotti et al., 2018).
The process of G. boninense colonising the root surface is crucial for the pathogen to later penetrate between host cells and finally cause host harm (Rees et al., 2009). The root cortex becomes colonised by G. boninense during the biotrophic phase’s initial infection, with many hyphae filling the host cells’ completely intact cell walls (Rees et al., 2009). The epidermal surface of the oil palm root tissue served as the entry point for G. boninense’s infection, which then led to the xylem vessels (Alexander et al., 2017a). Pathogen-associated molecular patterns (PAMPs) from G. boninense may bind to pattern recognition receptors (PRRs) that are extracellular or located at the plasma membrane to begin the process of pathogen recognition by oil palm (Ho et al., 2016). In oil palm-Ganoderma pathosystems, chitin, glucans, and ergosterol, components of the fungal cell wall or cell membrane, have been identified as PAMPs (Ho et al., 2016).
After establishing robust biotrophic growth and defeating the host defenses, the fungus enters a nectrotropic phase, which is characterised by significant cell wall lysis and the death and maceration of host tissues (Whalen, 2005; Alexander et al., 2017a). As a result of G. boninense infection, several oil palm chitinases and glucanases were in fact up-regulated in oil palm roots. The fungal cell wall may be broken down, loosen, and soften by these cell wall degrading enzymes (CWDEs) viz. cellulase, laccase, manganese peroxidase and polygalacturonase, releasing chitins and glucans (Breton et al., 2009). As an alternative, ergosterol, which has been implicated in pathogen virulence (Doehlemann et al., 2008), may similarly induce PAMP-triggered immunity (PTI) in oil palm. The oxidative degradation of lignin and the white rot status attributed to G. boninense may be detected by the production of melanized mycelium (Adaskaveg et al., 1990; Paterson, 2007). At last, the tissues of the oil palm roots lost their integrity and seemed to be a decaying mass covered in thick fungus mycelium. As the roots rot, the pathogens act as a source of inoculum and survive for a long time in the soil (Hasan and Turner, 1994). Once this inoculum found an appropriate host with a favourable environment, it began to actively spread (Chong et al., 2017c).
To date, there is no vertical resistance to BSR that inhibits or limits infection (Rozlianah et al., 2015). Instead, there are the horizontal and partial resistance that do not limit infection but reduces or compensates the damages, and as a result, the effects on the fitness of oil palm (Idris et al., 2004b; Durand-Gasselin et al., 2005; Breton et al., 2006; Wening et al., 2016). Breeding program has used South American E. oleifera and African E. guineensis species as genetic resources (Soh et al., 2017) because different BSR susceptibilities were found in these two species (de Franqueville et al., 2001; Durand-Gasselin et al., 2005).
In the past few decades, Ariffin et al. (1999) has discovered the tolerant progeny of oil palm to BSR viz. Cameroon x Cameroon, Cameroon x AVROS and Nigeria x Nigeria. Then, in 2004, Idris et al. (2004b) has discovered the sources of tolerance genes in Zaire x Cameroon bred, and the sources of partial resistance gene in Congo x Cameroon bred. A year later, the source of partial resistance from the Magenot line of E. oleifera. E. guineensis of Deli origin from Malaysia and Indonesia was found to be more susceptible to BSR than African material in North Sumatra, Indonesia (Durand-Gasselin et al., 2005). In the study of Chong et al. (2012), AVROS has been reported less susceptible to BSR compared to Calabar and Ekona. Two years later, Turnbull et al. (2014) has identified the potential resistance sources in La Mé A x Deli Dabou B and Yangambi IRHO A x Deli Socfindo. At the same time, ‘D x P Socfindo Moderate Gano Resistant’ which is a G. boninense partial resistance material has been released by PT Socfindo (Tan et al., 2018). In 2016, another partial resistance material namely ‘Yangambi Ganoderma-Tolerant 1’ has been released by FELDA Global Ventures (Tan et al., 2018). Despite the breeding program’s promise, it is unlikely to quickly produce tolerant or resistant Ganoderma planting materials.
Oil palms that are tolerant or resistant to BSR may offer a long-term approach of BSR control. However, the lack of quick and efficient screening procedures makes it difficult to generate resistant or tolerant varieties (Soh, 2017). Recently, the study of Ganoderma resistance in oil palm have been explored at the genetic level. The introduction of QTL (Quantitative Trait Loci) mapping in breeding program has efficiently and flexibly generated valuable data for the identification of resistance loci (Tisné et al., 2017). There were found to be four Ganoderma resistance loci, of which two affected the occurrence of BSR symptoms and two affected the rate at which oil palm trees died (Tisné et al., 2017). The ability to combine known favorable genomic regions, such as those for disease and yield related traits, will help with multicriteria improvement of oil palm by preventing unintentional unfavorable selection on the others. This will be made possible by the localization of Ganoderma resistance loci (Tisné et al., 2017).
Through the rapid multiplication of plantlets with uniformity and desired features, tissue culturing outperforms conventional breeding (Ngando-Ebongue et al., 2012). Lignin has been associated with the protection of oil palm lignin’s cell wall structure and function, which play a key part in protecting plants. Increase the amount of lignin of oil palm has been considered using tissue culture technique. However, the palm tissue culture is a long process and time is required for parameters of the transformation to be optimized (Sahebi et al., 2015).
Increasing accumulated Si in oil palm using genetical manipulation methods has been proposed. The TEM images demonstrate that intracellular regions of the root and leaf cells create a protective layer of cellulose-Si membrane when oil palm is subjected to Si treatment. The accessibility of the fungal mycelium to the middle lamella component of the cell wall is thought to be affected by the Si deposition in the intercellular regions of the cell (Sahebi et al., 2015).
Mineral fertilizers play a vital role in enhancing plant and soil health, and microbial activity (Singh and Ryan, 2015). Rahman and Othman (2020) have applied calcium carbonate to oil palm seedlings, and the best soil pH for suppressing BSR was pH 6. Integration of mineral fertilizers with other chemicals or living organisms have been performed in Ganoderma research. Purba et al. (1994) has demonstrated the integration of sulphur, cover crops and tridemorph fungicide has reduce the incidence of BSR after 5 years of application. Calcium nitrate and the biological control agent (BCA), Trichoderma sp., reduced illness, according to Sariah and Zakaria (2000) and Naher et al. (2018). Silicon oxide, potassium silicate, calcium silicate, sodium silicate, and sodium meta-silicate, according to Najihah et al. (2015), reduced the severity of BSR in oil palm seedlings. Silicon has positive impacts on growth, yield, and disease resistance in plants (Wang et al., 2017). Continuous calcium, copper, and SA supplementation may be essential for improving disease resistance in oil palm (Rahamah Bivi et al., 2016), and greater lignin content was also noticeable, suggesting a possible mechanism for infection resistance.
Oil palm plants infected with BSR have been treated with fungicides via trunk injection, soil drenching, combo treatments using both methods, pressure injection, soil injection, and soil fumigation (Ariffin and Idris, 1991; Ariffin et al., 2000; Idris et al., 2002; Idris et al., 2004a). Initially, diseased palms have been painted with carbolineum (fungicidal paste) after discarding basidiomata (Turner, 1981). Issue with pollution that poses a health risk persists after using carbolineum (Osterloh and Nusser, 2014).
Later, Ganoderma infected palms in the field has been reported to be highly inhibited by organic mercury formulations after trunk injection, however the residue issue made them unusable for commercial application (Turner, 1981). In 1990, Khairudin (1990) found out that injecting systemic fungicides like fusilazol, hexaconazole, cyproconazole, flutriafol, triadimenol, tridemorph, and oxycardoxin slowed the spread of BSR. Application of hexaconazole via pressure injection, trunk injection, soil drenching, and soil injection, into diseased oil palms reduced the spread of BSR in the infected oil palms (Idris et al., 2002; Idris et al., 2004a). Pressure injection has been used to accurately apply the hexaconazole to the infected area. Idris et al. (2004a) reported that after 5 years, 70% of treated oil palms produced fruit bunches. In Jee and Chong (2015) study, despite being given hexaconazole, ergosterol was found in the diseased tissues both before and after the treatments. But, at least, hexaconazole treatment has prolonged the period of productivity in Ganoderma-infected palms (Idris et al., 2004a).
Besides, Ariffin and Idris (1991) have reported that the formation of fruiting bodies might be inhibited by injecting the fumigant fungicide dazomet into the stem lesion which further reduced the spread of BSR. Idris and Maizatul (2012a) also reported that Ganoderma inoculum in infected stumps at three years after dazomet treatment has been eradicated. Later, Idris and Maizatul (2012b) observed that dazomet increased oil palm productivity by reducing the spread of BSR by eradicating Ganoderma inoculum.
The effectiveness of pyraclostrobin, a broad-spectrum fungicide with curative, preventative, and long-lasting characteristics, in preventing the growth of G. boninense has been assessed. Pyraclostrobin proved successful in reducing BSR in oil palm seedlings while also having beneficial physiological benefits on seedlings, such as increased height, bole diameter, root mass, photosynthetic rate, PSII quantum efficiency, and relative leaf chlorophyll content (Said et al., 2019). However, this fungicide’s efficacy on mature oil palms has not yet been investigated.
Nanomaterials are being applied as carrier for fungicides delivery. Hexaconazole-zinc and Dazomet-zinc/aluminum layered double hydroxide nanocomposite has performed better than pure dazomet and hexaconazole as a fungicide and a micro-nutrient (Mustafa et al., 2018a; Mustafa et al., 2018b). Hexaconazole-zinc/aluminum layered double hydroxide nanocomposite exhibits superior growth inhibition against G. boninense than pure hexaconazole (Mustafa et al., 2018a). Hexaconazole and dazomet were successfully delivered to G. boninense cells using a nanodelivery system that used chitosan nanoparticles as the carrier for the fungicides. Low phytotoxicity and high activity against the pathogen were displayed by the nanodelivery system (Maluin et al., 2019a; Maluin et al., 2019b).
Despite the fact that some effective fungicides are available, utilizing fungicides in control approaches might cause environmental contamination and the emergence of fungicide resistance (Shcherbakova et al., 2020). For the effective control of Ganoderma, cultural control techniques such as field hygiene or the prevention of pest immigration are essential. Since it is hard to control all pest species using biological control, it is crucial for commercial application to combine the use of counterpart of Ganoderma with other control strategies, such as cultural control and the use of chemicals.
In established oil palm plantations, it is frequently advised to manage BSR through good cultural practises. To stop the spread of disease, cultural methods often involve eliminating and decreasing the pathogen inoculums to provide oil palm with short-term relief. These include clean clearance, sanitation, soil mounding, surgery, trenching, and windrowing.
Excision and removal of all leftover fragments from an infected palm area is known as “clean clearance,” and it involves digging holes that are 1.5 m2 and 60 cm deep from both untreated vacant points and infected palm points (Singh, 1990). Despite the practice of clean clearance during the replanting of second- and third-generation palms, residual root pieces can play a significant role in the emergence of BSR. The ability of the root fragments to form G. boninense basidiomata, which are occasionally found on their cut ends, indicates that they still possess adequate inoculum potential to spread disease (Ariffin et al., 2000). Normally, all of the still-present fragments of the infected palm are brought to the surface and either removed or burned. However, this strategy is expensive, and many nations that produce palm oil restrict open burning. As a result, it is usual practise to shred all palm pieces, which can then be either dispersed across the entire field or stacked in rows and covered with a legume cover crop to speed up decomposition (Chong et al., 2017b). Although these procedures typically reduce the occurrence of BSR in newly planted oil palms, infection can nonetheless gradually take place due to debris left in the soil (Singh, 1991).
When an appropriate disease inoculum is present, diseased stumps are the tissues that are most contagious. The oil palm will be attacked by the Ganoderma inoculums in these stumps if there is a contact. Therefore, it is crucial to maintain field sanitation and the removal of diseased tissues in order to keep the plantation free of pathogen sources (Chong et al., 2017b). In this sanitation operation, a sizable hole measuring 2 m x 2 m x 1 m is dug (Idris et al., 2004a). The infected materials are removed, crushed into little pieces, and placed in the interrow spaces to decompose naturally. In the majority of sizable plantations, this technique is frequently used while replanting (Chung, 2012). Prior to replanting, sanitation had greatly reduced the prevalence of BSR on the replanted palms by removing soil and old stumps, ploughing, and rotovating. Ten years after replanting, the BSR incidence was lower in places with sanitation than it was in areas without sanitation, at less than 1% as opposed to more than 13% (Ahmad et al., 2012).
By preventing weakened boles from being blown over by wind, soil mounding, which involves gathering dirt from nearby regions and piling it up to a height of 0.75 m up and 1 m radius wide around the trunk, inhibits the progress of BSR in infected trees (Ho and Khairudin, 1997; Susanto et al., 2009). However, the spread of Ganoderma was not slowed down by this strategy, but it did aid to extend the economic life of yielding palms that were afflicted by BSR (Chong et al., 2017b).
In some plantations, surgery is performed by mechanically removing infected tissues using a backhoe blade (Singh, 1991) or manually removing them with a hand-held chisel (Turner, 1981). Following surgery, palms have shown improved survival and production (Hasan and Turner, 1994). However, surgery frequently failed because of late identification, a huge disease lesion, and a lesion that extended below ground, including infected root masses, leading treated palms to fall at the end (Chung, 2011).
Digging trenches to prevent palm-to-palm contact is another cultural practise that has been adopted. Compared to windrowing and open clearing, it might be a preferable choice (Chong et al., 2017b). Wakefield (1920) recommended digging trenches around infected palm trees as a control measure to stop the pathogen from spreading to nearby healthy trees. Oil palm on peat field trials in Indonesia have demonstrated that the establishment of isolation trenches measuring 4 x 4 x 0.75 m deep can effectively reduce the transmission of BSR infection to nearby healthy oil palms (Lim and Udin, 2010). According to a field trial by Lim and Udin (2010), isolation of trenches around old stumps is successful in preventing BSR infection for oil palm planted between the old stands for up to 14 years. However, trenches have not been effective because they were either not maintained or their depths were insufficient to stop roots from passing underneath (Turner, 1981; Ariffin et al., 2000). This control measure must also be regularly maintained because erosion of the trench edges will cause partial filling (Ariffin et al., 2000). In general, this measure is not frequently used (Chong et al., 2017b). Surgery and trenching are not widely practiced nonetheless being recorded earlier.
Oil palm trunks that have been felled and root tissues that have been removed are put along the previous rows using the windrowing technique. This method has proven to be virtually as effective at limiting losses in the cultivated crop as clean clearing yet involving less labour (Chong et al., 2017b). But according to a comparative study by Hashim (1991), windrow treatment (27.3-17.6%) and clean clearing (from 27.3% in the prior stand to 14% in the replanted stand after 15 years) were the most successful in reducing the incidence of BSR.
Biological control agents (BCAs) are becoming more popular as a potential replacement for chemical fungicides for controlling BSR in oil palm fields (Sujarit et al., 2020). Many BCAs have been studied to control Ganoderma in the last few decades. Most of the BCAs have been conducted in vitro as reviewed by Supramani et al. (2022). In in vitro studies, Ganoderma boninense has been inhibited to some extent by some BCAs viz. actinomycetes, arbuscular mycorrhizal fungi, Bacillus spp., Diaporthe spp., mycoparasite, Penicillium spp., Streptomyces spp. and Trichoderma spp. (Bakhtiar et al., 2010; Sundram et al., 2011; Azizah et al., 2015; Goh et al., 2015; Chow et al., 2017; Lim et al., 2018; Chow et al., 2019; Sujarit et al., 2020). Inhibition happened attributed to some potential antimicrobials compound such as eudistomin I, Gly-Met-OH, halstoctacosanolide A, lovastatin, naringenin, N-acetyl-leu-leu-tyr-amide, N-methyl-a-aminoisobutyric acid, penipanoid A, phenazine and phenazine-1-carboxylic acid, phenylethyl alcohol, phenyl alkenoic acids, pyrene-1,6-dione, 4-O-8’,5”-5’-dehydrotriferulic acid, 7-O-beta-D-glucoside, 12-deoxyaklanonic acid, and 12-oxo-10Z-dodecenoic acid (Angel et al., 2016; Alexander et al., 2017b; Lee et al., 2018; Ahmad et al., 2020; Sujarit et al., 2020). However, in-vitro studies conducted in nursery and field trials are required to validate the efficiency of those BCAs. Some examples of BCAs which have showed their efficiency in BSR control in in vivo are listed in Table 2.
Recently, virus and viroid-like RNAs have been detected in fungi (Dong et al., 2022; Liu et al., 2022). A mycovirus called SlAV1 from the plant pathogen Stemphylium lycopersici that causes pigmentation loss and hypovirulence by preventing the fungus from biosynthesising the phytotoxin Altersolanol A. The pathogen becomes a BCA and improves plant resistance to virulent strains when its genome is integrated and a crucial SlAV1 gene is expressed in the fungal host (Liu et al., 2022). Besides, Botryosphaeria dothidea RNAs (BdcRNAs), a viroid-like RNAs similar to those of viroids have exhibited pathogenic effects on hosts (Dong et al., 2022). Their hypovirulence have conferred them the ability as potential BCAs to control fungi. Considering the current aforementioned findings, there is a possibility that G. boninense might have mycovirus or viroid-like RNA to control it and improve oil palm resistance, but yet to be discovered in the future. To date, only Coconut cadang-cadang viroid (CCCVd) has been reported to cause orange spotting on fronds of oil palm (Vadamalai et al., 2009). This finding may raise the question of whether CCCVd-infected oil palm would have better resistance against G. boninense. If yes, what is the mechanism behind this viroid-fungi-host relationship? Peptidases are secreted by both pathogenic fungi to break down a range of (poly)peptides in their environment (Amselem et al., 2011). In the finding of Dong et al. (2022), for example, BdcRNA 1 transfectants have down regulated the DEGs related to peptidase activities, and thus attenuated the fungi virulence. This finding has provided the novel insight on understanding the relationship of mycoviroid and fungi in their host.
Elicitors are compounds that elicit various plant defensive responses (Thakur and Sohal, 2013). Elicitors have been shown to induce hypersensitive response (HR) and systemic acquired resistance (SAR) in plants to fend off infections from bacteria, fungi, and viruses (Zhang et al., 2004; Pawlowski et al., 2016). The effectiveness of elicitors amino acid (AA), benzoic acid (BZA), caffeic acid (CA), chitosan, fulvic acid (FA), humic acid (HA), jasmonic acid (JA), salicylic acid (SALA), syringic acid (SA) and 4-hydroxybenzoic acid (4-HBA) have been examined on G. boninense in-vitro or in-vivo (Ommelna et al., 2012; Jee and Chong, 2015; Rahamah Bivi et al., 2016; Chong et al., 2017b; Ong et al., 2018; Surendran et al., 2018). In this subsection, elicitors are reviewed and recommended (Table 3).
It has been noted that chitosan, a natural polymer can be found in the shells of insects, fungus, and crustaceans have protected various crops from harmful fungus by triggering their natural defense response (Bhaskarareddy et al., 1999; Hadwiger, 1999; Takeshi et al., 2000). In the study of effectiveness of chitosan against G. boninense, the findings implied the fungal sterol of G. boninense in oil-palm root was suppressed and the lowest percentage mean disease severity was recorded when treated with chitosan at a minimum concentration of 0.5% (w/v). Result also showed that there was no discernible difference between any of the chitosan concentrations for bole tissue infection as no infection was found. Compared to seedlings that were pre-treated with chitosan and later infected with G. boninense at the same concentration, Ganoderma-infected seedlings that were treated with chitosan (0.1%, w/v) displayed slightly less severe illness (Ommelna et al., 2012).
Salicylic acid (SALA), a naturally occurring plant hormone, gained interest after it was discovered that it can increase plants’ tolerance to abiotic stress and disease resistance (Alonso-Ramírez et al., 2009; Gautam and Singh, 2009; Pieterse et al., 2009). Effectiveness of SALA has been examined in vitro on G. boninense growing in potato dextrose agar (PDA). The finding indicated that mycelial growth recovery was seen starting on 9 day-after-inoculation for SALA levels of 150 ppm and above (Ong et al., 2018). In a glasshouse experiment, Rahamah Bivi et al. (2016) shown that SA decreased disease incidence and severity brought on by G. boninense in oil palms. The findings are in contrast to those of Ho et al. (2020), who discovered that the disease symptoms of infected oil palm seedlings treated with SALA and the untreated oil palm seedlings were not significantly different. However, Mohan et al. (2022) showed that during the biotrophic phase of Ganoderma infection, SALA was involved in the early defense response from infected oil palm.
Jasmonic acid (JA) has become important signaling molecules linked to stress resistance and plant development (Ghorbel et al., 2021). JA has been reported to promote the growth of G. boninense in PDA starting on 7 day-after-inoculation regardless of different concentrations (Ong et al., 2018). Ho et al. (2020), who demonstrated that the illness symptoms of infected oil palm seedlings treated with JA and the untreated oil palm seedlings were not substantially different, provide additional support for the findings (Ong et al., 2018). The results are contrast to the current study (Mohan et al., 2022). During the necrotrophic phase of Ganoderma infection, JA mediated the defensive response from the root lesions of Ganoderma-infected oil palm root tissues.
Benzoic acid (BZA) plays a crucial role in the composition of defensive chemicals (Widhalm and Dudareva, 2015). Benzoic acid at a dose of 15 mM has been shown by Surendran et al. (2018) to be an effective control against BSR disease in oil palm. More than 80% of the growth of G. boninense was inhibited by treatment with benzoic acid. The mycelia of G. boninense were severely structurally damaged by the benzoic acid treatment (5.00 mM). G. boninense mycelia that had been treated had distinct holes and ruptures and were thinner, less dense, deformed, and shriveled. However, demonstration of field and glasshouse studies are scarce (Fernanda et al., 2021).
Besides benzoic acid, other phenolic acids such as caffeic acid (CA), syringic acid (SA) and 4-hydroxybenzoic acid (4-HBA) have been applied to control G. boninense in the estate. The combination of 4 g of each phenolic acid is the concentration that suppresses G. boninense the most effectively, leading to the greatest decrease in Ganoderma ergosterol in infected tissues (Jee and Chong, 2015).
Fulvic acid (FA) is a type of plant growth regulator that enhance plant growth and plant stress resistance (Peiris et al., 2011; Sun et al., 2020). Humic acid (HA) is an organic product that control several biochemical effects, such as cell membrane permeability, photosynthetic rate, cell elongation, and increase water use efficiency (Zhang et al., 2013). Combination of FA and HA with multiple biological control agents (MBCA) have been demonstrated to control G. boninense. By adding HAs and FAs, the soil becomes naturally equipped to fend off many fungi that are capable of causing soil-borne diseases (Chong et al., 2017b). Oil palm seedlings in nursery were used to assess the effects of adding multi-BCAs, chitin, and AA.
In response to a number of environmental variables, plants generate a numerous of primary and secondary metabolites. Certain metabolites have significant roles in plants’ defensive systems against fungi, bacteria, and other pests, according to secondary metabolite profiling.
These metabolites play a role in defensive processes during interactions between plants and pathogens (Bennett and Wallsgrove, 1994; Pedras and Ahiahonu, 2005; Hasegawa et al., 2010). As metabolites of plant defence against infection with G. boninense, several steroid molecules have been discovered. The crude root extracts of infected tolerant and susceptible oil palm progenies included the three primary steroids sitosterol, -sitosterol, and stigmasterol. Stigmast-5-en-3-ol, campesterol, cholestan, and an ergosterol (- and -tocopherol) were also found (Nusaibah et al., 2011). Other metabolites related to the early defence mechanism of the oil palm included lipids like fucosterol, betasitosterol, gamma-sitosterol, palmitic acid, and linoleic acid, antioxidants like -tocopherol and -tocopherol as well as heterocyclic aromatic organic compounds like pyridine, benzo[h]quinoline, and pyrimidine. These metabolites were observed in the root extract of infected samples, and it was discovered that resistant oil palm progeny had higher quantities of these metabolites than susceptible oil palm progeny (Nusaibah et al., 2011; Nusaibah et al., 2016). Based on the current findings, the resistant progeny increased the number and concentration of metabolites in the roots that had antifungal properties during the early stages of G. boninense infection. Because of this, the resistant progeny can exhibit greater levels of resistance than the susceptible progeny (Nusaibah et al., 2016). These biomarkers were found in G. boninense-infected oil palm, which indicates that they are part of the oil palm’s defence mechanism against the pathogen. Therefore, an early detection could help to extend the palm’s economic life (Nusaibah et al., 2016).
Plant and alga extracts have been studied to inhibit the growth of G. boninense in vitro. Plant extracts such as carboxylic acid, ester, fatty acid ester, phenol and steroid caused the inhibition growth of G. boninense in vitro (Tay and Chong, 2016). According to Abdul Aziz et al. (2019), extracts from algae Caulerpa racemosa, C. racemosa var. lamouroxii and Halimeda macrophysa have been reported to exhibited antifungal activities on G. boninense at the lowest concentration tested. Further research can be conducted to apply these bioactive compounds to confer resistance to oil palm in the field.
Phenolic compounds such as Gallic acid (GA) and Thymol (THY) have been extracted from bee-produced products. These compounds have showed their significant inhibition of the mycelial growth of G. boninense. Gallic acid at a dose of 8 mg/mL provided the best inhibition of G. boninense with a PIRG of 94%, followed by THY at a concentration of 0.25 mg/mL with an inhibition of 87.13% (Ganapathy et al., 2021). Humic acid (HA) has been reported to increase plant membrane permeability (Lotfi et al., 2018). Similar to function of HA, but in different organisms, Gallic acid (GA) and Thymol (THY) have been reported to increase G. boninense membrane permeability and cause the depletion of components essential to fungal survival (Ganapathy et al., 2021). In the future, GA and THY had the potential to be used as a green control strategy and further developed as a natural antifungal agent to suppress G. boninense.
Genetic modification has been applied to confer resistance to oil palm against BSR infection. In 2020, oil palm embryogenic calli that co-bombarded with a construct carrying the AGLU1 and RCH10 genes have been reported to exhibited resistance to G. boninense. The results demonstrated that G. boninense resistance oil palm could be produced through biolistic bombardment (Hanin et al., 2020).
A cutting-edge CRISPR/Cas9 has been applied to introduce the genes in the oil palm calli (OPC). When delivered into oil palm callus, CRISPR/Cas9 constructs carrying sgRNA with targeted isoflavone reductase (IFR) and methallotioneine like protein (MT) genes produced mutations in both targeted DNA areas, altering the sequences of the amino acid residues (Budiani et al., 2018). A method like this might open up new possibilities for research into the defence genes responsible for disease resistance in oil palm. In 2021, effective and efficient CRISPR/Cas9 gene editing in oil palm have been achieved through maximising the choice of efficient gRNA and DNA delivery techniques. The genetic enhancement of the oil palm will be made possible by this newly developed technique (Yeap et al., 2021).
Control strategies is always the priority to be considered of to curb or control G. boninense. Albeit many control methods have been showed positive results in the in-vitro tests, but none of the methods can totally eradicate G. boninense in the field.
Diseased oil palm stumps are the tissues that are most contagious when G. boninense inoculum is present. To keep the plantation free of sources of pathogens, it is vital to remove unhealthy tissues (Chong et al., 2017b). This has led to a great deal of interest in the use of white-rot fungi (WRF), an environmentally friendly and biodegradable Ganoderma management strategy, to destroy the diseased stumps. According to Naidu et al. (2017), Pycnoporus sanguineus FBR and Grammothele fuligo ST2 are the strongest biodegraders of wood blocks that have been colonized by G. boninense. The findings have provided novel insights on the potential of the WRF to eradicate Ganoderma inoculums in the future.
Gene technology has been thought of as a potential improvement for the oil palm. The Malaysian Palm Oil Board (MPOB) and their American collaborators have sequenced and published the oil-palm genomes of the South American oil-palm E. oleifera and the African oil-palm E. guineensis to aid in the improvement study of oil palm (Singh et al., 2013). Through gene technology, genetically modified (GM) oil palm with traits such as oleic acid for healthy low unsaturated oil and high PHBV (polyhydroxybutyrate-co-valerate) for bioplastics production have been produced in the laboratory and biosafety containment houses (Yunus et al., 2008; Nurfahisza et al., 2014). The studies have proved that gene technology can improve the oil palm. However, GM oil palm faced technical and commercial problems, and public sensitivity (Murphy, 2017; Soh, 2018). Even if these obstacles in the way of technique and commerce can be addressed, public aversion to GM crops, which has existed in various regions of the world since 1999, means that new transgenic cultivars continue to encounter difficulties. Up until the present, this opposition has been particularly strong in Europe. As a result, many local regions in the EU implemented legal restrictions on the production of all GM crops in late 2015, even varieties that had received European Commission approval (Nelson, 2015).
In present era, the controversy of GM crops has been viewed as being resolved by the genome editing (GE) approach, which permits targeted in situ DNA change without or temporarily using transgenes (Mao et al., 2013). Integration of GE with tissue-culture-free methods would avoid the crops being labelled as genetically modified organism (GMO) (Hickey et al., 2019). The ability to do single or multiplex edits has been widely established (Zhang et al., 2016); this capability can now be put into practise using the following tissue-culture-free methods. For an instance, Clustered, regularly interspaced short palindromic repeat/CRISPR-associated protein (CRISPR/Cas9) ribonucleoproteins (RNPs) complexes can be used for GE. CRISPR-based GE enables precise target modifications in the DNA of an organism (Adli, 2018). This has been done for a variety of species, including potato, wheat (Liang et al., 2017), and maize (Andersson et al., 2018). Target tissues have included protoplasts or immature embryos; ideally, this technique would be enhanced for mature seeds or sprouting seedlings (Hamada et al., 2017). Current GMO rules in some countries may be addressed by CRISPR/Cas9 RNPs (Kanchiswamy, 2016; Lu et al., 2017); nevertheless, this technology can also be used to control oil palm diseases and pests (Khan et al., 2022).
Since the interactions between G. boninense and oil palm are so complicated, it is crucial to fully comprehend them in order to improve disease control measures. As there is no single effective control approach that can stop the spread of this disease, integrated disease management is necessary to manage BSR. Chemical fungicides, cultural practices, biological techniques, and fertilizer application are all examples of current management approaches. All current and potential management strategies are summarised in Figure 2. Reduced BSR incidence following replanting is the goal of BSR integrated disease management. Since the impact of this soilborne fungus lies in both the losses it causes and the effects it has on the environment as a result of unsustainable management practices, it is crucial to handle BSR brought on by G. boninense responsibly.
Conceptualization, YK and KC; Writing-original draft preparation, YK; Writing-Review & Editing, KC; Supervision, KC. Both authors contributed to the article and approved the submitted version.
The authors wish to thank Universiti Malaysia Sabah for the financial support through grant UMSG2655.
The authors declare that the research was conducted in the absence of any commercial or financial relationships that could be construed as a potential conflict of interest.
All claims expressed in this article are solely those of the authors and do not necessarily represent those of their affiliated organizations, or those of the publisher, the editors and the reviewers. Any product that may be evaluated in this article, or claim that may be made by its manufacturer, is not guaranteed or endorsed by the publisher.
Abdul Aziz, S. D., Jafarah, N. F., Sabri, S., Wahab, M. A. A., Yusof, Z. N. B. (2019). Antifungal activities against oil palm pathogen Ganoderma boninense from seaweed sources. Asia-Pacific J. Mol. Biol. Biotechnol. 27, 75–83. doi: 10.35118/apjmbb.2019.027.1.08
Adaskaveg, J. E., Gilbertson, R. L., Blanchette, R. A. (1990). Comparative studies of delignification caused by Ganoderma species. Appl. Environ. Microbiol. 56, 1932–1943. doi: 10.1128/aem.56.6.1932-1943.1990
Adli, M. (2018). The CRISPR tool kit for genome editing and beyond. Nat. Commun. 9, 1–13. doi: 10.1038/s41467-018-04252-2
Ahmad, M. N., Ali, S. R. A., Seman, I. A., Kamaruddin, N. (2012). Mechanization of pest & disease control in meeting of the palm mech, bangi, selangor, Malaysia (Bangi, Malaysia: Malaysian Palm Oil Board). 1–18.
Ahmad, R., Lim, C. K., Marzuki, N. F., Goh, Y. K., Azizan, K. A., Goh, Y. K., et al. (2020). Metabolic profile of Scytalidium parasiticum-Ganoderma boninense co-cultures revealed the alkaloids, flavonoids and fatty acids that contribute to anti-Ganoderma activity. Molecules 25, 5965–5984. doi: 10.3390/molecules25245965
Ahmadi, P., Muharam, F. M., Ahmad, K., Mansor, S., Abu Seman, I. (2017). Early detection of ganoderma basal stem rot of oil palms using artificial neural network spectral analysis. Plant Dis. 101, 1009–1016. doi: 10.1094/PDIS-12-16-1699-RE
Alexander, A., Abdullah, S., Rossall, S., Chong, K. P. (2017b). Evaluation of the efficacy and mode of action of biological control for suppression of Ganoderma boninense in oil palm. Pak. J. Bot. 49, 1193–1199.
Alexander, A., Chong, K. P. (2014a). Combination of biological agents in suppressing colonization of Ganoderma boninense of basal stem rot. Am.-Eurasian J. Sustain. Agric. 8 (Special), 1–7.
Alexander, A., Chong, K. P. (2014b). The impact of biological control agents on soil microbial communities in oil palm plantation soils. Am.-Eurasian J. Sustain. Agric. 8 (Special), 8–14.
Alexander, A., Sipaut, C. S., Dayou, J., Chong, K. P. (2017a). Oil palm roots colonisation by Ganoderma boninense: an insight study using scanning electron microscopy. J. Oil Palm Res. 29, 262–266. doi: 10.21894/jopr.2017.2902.1
Alizadeh, F., Abdullah, S. N. A., Khodavandi, A. (2013). Influence of oil palm-fungi interactions on soil microfungal community and growth profile of plant. J. Pure Appl. Microbio. 7, 2577–2590.
Alonso-Ramírez, A., Rodríguez, D., Reyes, D., Jiménez, J. A., Nicolás, G., López-Climent, M., et al. (2009). Evidence for a role of gibberellins in salicylic acid-modulated early plant responses to abiotic stress in Arabidopsis seeds. Plant Physiol. 150, 1335–1344. doi: 10.1104/pp.109.139352
Amselem, J., Cuomo, C. A., van Kan, J. A., Viaud, M., Benito, E. P., Couloux, A., et al. (2011). Genomic analysis of the necrotrophic fungal pathogens Sclerotinia sclerotiorum and Botrytis cinerea. PLoS Genet. 7, e1002230. doi: 10.1371/journal.pgen.1002230
Andersson, M., Turesson, H., Olsson, N., Fält, A., Ohlsson, P., Gonzalez, M. N., et al. (2018). Genome editing in potato via CRISPR-Cas9 ribonucleoprotein delivery. Physiol. Plant 164, 378–384. doi: 10.1111/ppl.12731
Angel, L. P. L., Yusof, M. T., Ismail, I. S., Ping, B. T. Y., Mohamed Azni, I. N. A., Kamarudin, N. H., et al. (2016). An in vitro study of the antifungal activity of Trichoderma virens 7b and a profile of its non-polar antifungal components released against Ganoderma boninense. J. Microbiol. 54, 732–744. doi: 10.1007/s12275-016-6304-4
Ariffin, D., Idris, A. S. (1991). “Investigation on the control of Ganoderma with dazomet,” in Proceeding of the PIPOC International Palm Oil Congress-Agriculture Conference 2016, PORIM, Bangi, Selangor; Publisher: Palm Oil Research Institute of Malaysia. 424–429.
Ariffin, D., Idris, A., Azahari, M. (1996). “Spread of Ganoderma boninense and vegetative compatibility studies of a single field palm isolates,” in Proceedings of the PORIM international palm oil congress 1996, Bangi, Selangor; Publisher: Palm Oil Research Institute of Malaysia. 317–329.
Ariffin, D., Idris, A. S., Kushairi, A., Watt, T. A., Swinburne, T. R. (1999). “Screening of oil palm for resistance to Ganoderma,” in Colloquium on Advances in Oil Palm Research, Bangi, Malaysia. 12–17.
Ariffin, D., Idris, A. S., Singh, G. (2000). Status of ganoderma in oil palm. Eds. Flood, J., Bridge, P. D., Holderness, M. (Wallingford, UK: CABI Publishing), 49–68. doi: 10.1079/9780851993881.0049
Azizah, S. N., Mubarik, N. R., Sudirman, L. I. (2015). Potential of chitinolytic Bacillus amyloliquefaciens SAHA 12.07 and Serratia marcescens KAHN 15.12 as biocontrol agents of Ganoderma boninense. Res. J. Microbiol. 10, 452–465. doi: 10.3923/jm.2015.452.465
Bahari, M. N. A., Sakeh, N. M., Abdullah, S. N. A., Ramli, R. R., Kadkhodaei, S. (2018). Transcriptome profiling at early infection of Elaeis guineensis by Ganoderma boninense provides novel insights on fungal transition from biotrophic to necrotrophic phase. BMC Plant Biol. 18, 377–401. doi: 10.1186/s12870-018-1594-9
Bakhtiar, Y., Yahya, S., Sumaryono, W., Sinaga, M. S., Budi, S. W., Tajudin, T. (2010). Isolation and identification of mycorrhizosphere bacteria and their antagonistic effects towards Ganoderma boninense in vitro. Microbiol. Indones. 4, 96–102. doi: 10.5454/mi.4.2.9
Bennett, R., Wallsgrove, R. (1994). Secondary metabolites in plant defence mechanisms. New Pyhtopathol. 127, 617–633. doi: 10.1111/j.1469-8137.1994.tb02968.x
Bhaskarareddy, M. V., Arul, J., Angers, P., Couture, L. (1999). Chitosan treatment of wheat seeds induces resistance to Fusarium graminearum and improves seed quality. J. Agric. Food Chem. 47, 1208–1216. doi: 10.1021/jf981225k
Breton, F., Hasan, Y., Lubis, Z., de Franqueville, H. (2006). Characterization of parameters for the development of an early screening test for basal stem rot tolerance in oil palm progenies. J. Oil Palm Res. 24–36.
Breton, F., Rahmaningsih, M., Lubis, Z., Syahputra, I., Setiawati, U., Flori, A., et al. (2009). “Early screening test: a routine work to evaluate resistance/susceptibility level of oil palm progenies to basal stem rot disease,” in Proceedings of International Palm Oil Congress MPOB, Kuala Lumpur, Malaysia. 549–561.
Budiani, A., Putranto, R. A., Riyadi, I., Sumaryono, Minarsih, H., Faizah, R. (2018). Transformation of oil palm calli using CRISPR/Cas9 system: toward genome editing of oil palm. IOP Conf. Ser.: Earth Environ. Sci. 183, 12003. doi: 10.1088/1755-1315/183/1/012003
Chong, K. P., Alexander, A., Abdullah, S. (2016). Early detection and management of Ganoderma basal stem rot disease: a special report from sabah. Trans. Sci. Technol. 3, 517–523.
Chong, K. P., Dayou, J., Alexander, A. (2017a). Pathogenic nature of ganoderma boninense and basal stem rot disease. Eds. Chong, K. P., Dayou, J., Alexander, A. (Cham, Switzerland: Springer), 5–12. doi: 10.1007/978-3-319-54969-9_2
Chong, K. P., Dayou, J., Alexander, A. (2017b). Control methods of g. boninense in oil palm industry. Eds. Chong, K. P., Dayou, J., Alexander, A. (Cham, Switzerland: Springer), 21–30. doi: 10.1007/978-3-319-54969-9_4
Chong, K. P., Dayou, J., Alexander, A. (2017c). Control of g. boninense using multi-biological agents. Eds. Chong, K. P., Dayou, J., Alexander, A. (Cham, Switzerland: Springer), 31–46. doi: 10.1007/978-3-319-54969-9_5
Chong, K. P., Elda, P. A., Dayou, J. (2014). Relation of Ganoderma ergosterol content to basal stem rot disease severity index. Adv. Environ. Biol. 8 (Special), 14–19.
Chong, K. P., Markus, A., Rossall, S. (2012). The susceptibility of different varieties of oil palm seedlings to Ganoderma boninense infection. Pak. J. Bot. 44, 2001–2004.
Chong, K. P., Rossal, S., Markus, A. (2009). “A comparison on the susceptibility of varieties AVROS, calabar and ekona of oil palm seedlings to Ganoderma boninense,” in Proceedings of the Seminar on Science and Technology 2009, Universiti Malaysia Sabah, Kota Kinabalu, Malaysia (Kota Kinabalu, Sabah: Universiti Malaysia Sabah). 461–467.
Chow, Y. Y., Rahman, S., Ting, A. S. Y. (2017). Understanding colonization and proliferation potential of endophytes and pathogen in planta via plating, polymerase chain reaction and ergosterol assay. J. Adv. Res. 8, 13–21. doi: 10.1016/j.jare.2016.10.008
Chow, Y. Y., Rahman, S., Ting, A. S. Y. (2018). Interaction dynamics between endophytic biocontrol agents and pathogen in the host plant studied via quantitative real-time polymerase chain reaction (qPCR) approach. Biol. Control 125, 44–49. doi: 10.1016/j.biocontrol.2018.06.010
Chow, Y. Y., Rahman, S., Ting, A. S. Y. (2019). Evaluating the host defense responses in oil palm to complex biocontrol endophyte–pathogen–host plant interaction via fluidigm® real-time polymerase chain reaction (RT-PCR). Biol. Control 129, 148–157. doi: 10.1016/j.biocontrol.2018.10.011
Chung, G. F. (2012). Effect of pests and diseases on oil palm yield. Eds. Lai, O., Tan, C., Akoh, C. C. (Urbana, Il: AOCS Press), 163–210. doi: 10.1016/B978-0-9818936-9-3.50009-5
Corley, R. H. V., Tinker, P. B. (2003). Vegetative propagation and biotechnology. Eds. Corley, R. H. V., Tinker, P. B. (Oxford: Blackwell Science Ltd), 201–215. doi: 10.1002/9780470750971.ch6
Darmono, T. W. (2000). Ganoderma in oil palm in Indonesia: current status and prospective use of antibodies for the detection of infection. Eds. Flood, J., Bridge, P. D., Holderness, M. (Wallingford, UK: CABI Publishing), 249–266. doi: 10.1079/9780851993881.0249
de Franqueville, H., Asmad, Y. H., Jacquemard, J. C., Hayun, Z., Durand-Gasselin, T. (2001). “Indications on sources of oil palm (Elaeis guineensis jacq.) genetic resistance and susceptibility to ganoderma sp., the cause of basal stem rot,” in Proceeding 2001 International Palm Oil Congress – Agriculture, Kuala Lumpur, Malaysia. 420–431.
Doehlemann, G., Wahl, R., Horst, R. J., Voll, L. M., Usadel, B., Poree, F., et al. (2008). Reprogramming a maize plant: transcriptional and metabolic changes induced by the fungal biotroph Ustilago maydis. Plant J. 56, 181–195. doi: 10.1111/j.1365-313X.2008.03590.x
Dong, K., Xu, C., Kotta-Loizou, I., Jiang, J., Lv, R., Kong, L., et al. (2022). Novel viroid-like RNAs naturally infect a filamentous fungus. Adv. Sci. 10, 2204308. doi: 10.1002/advs.202204308
Durand-Gasselin, T., Asmady, H., Flori, A., Jacquemard, J. C., Hayun, Z., Breton, F., et al. (2005). Possible sources of genetic resistance in oil palm (Elaeis guineensis jacq.) to basal stem rot caused by Ganoderma boninense - prospects for future breeding. Mycopathol 159, 93–100. doi: 10.1007/s11046-004-4429-1
Dutse, S. W., Yusof, N. A., Ahmad, H., Hussein, M. Z., Hushiarian, R. (2013). DNA-Based biosensor for detection of Ganoderma boninense, an oil palm pathogen utilizing newly synthesized ruthenium complex [Ru(phen)2,(qtpy)]2+ based on a PEDOT-PSS/Ag nanoparticles modified electrode. Int. J. Electrochem. Sci. 8, 11048–11057. doi: 10.1016/S1452-3981(23)17979-X
Fernanda, R., Siddiqui, Y., Ganapathy, D., Ahmad, K., Surendran, A. (2021). Suppression of Ganoderma boninense using benzoic acid: impact on cellular ultrastructure and anatomical changes in oil palm wood. Forests 12, 1231–1248. doi: 10.3390/f12091231
Freihorst, D., Fowler, T. J., Bartholomew, K., Raudaskoski, M., Horton, J. S., Kothe, E. (2016). The mating-type genes of the basidiomycetes. Ed. Wendland, J. (Cham, Switzerland: Springer), 329–349. doi: 10.1007/978-3-319-25844-7_13
Ganapathy, D., Siddiqui, Y., Ahmad, K., Adzmi, F., Kong, L. L. (2021). Alterations in mycelial morphology and flow cytometry assessment of membrane integrity of Ganoderma boninense stressed by phenolic compounds. Biology 10, 930–949. doi: 10.3390/biology10090930
Gautam, S., Singh, P. K. (2009). Salicylic acid induced salinity tolerance incorn grown under NaCl stress. Acta Physiol. Plant 31, 1185–1190. doi: 10.1007/s11738-009-0338-8
Ghorbel, M., Brini, F., Sharma, A., Landi, M. (2021). Role of jasmonic acid in plants: the molecular point of view. Plant Cell Rep. 40, 1471–1494. doi: 10.1007/s00299-021-02687-4
Goh, Y. K., Goh, T. K., Marzuki, N. F., Tung, H. J., Goh, Y. K., Goh, K. J. (2015). Scytalidium parasiticum sp. nov., a new species parasitizing on Ganoderma boninense isolated from oil palm in peninsular Malaysia. Mycobiology 43, 107–117. doi: 10.5941/MYCO.2015.43.2.107
Goh, Y. K., Marzuki, N. F., Goh, T. K., Tan, S. Y., Goh, Y. K., Goh, K. J. (2016). Mycoparasitic Scytalidium parasiticum as a potential biocontrol agent against Ganoderma boninense basal stem rot in oil palm. Biocontrol Sci. Technol. 26, 1352–1365. doi: 10.1080/09583157.2016.1202192
Govender, N., Wong, M. Y. (2017). Detection of oil palm root penetration by agrobacterium-mediated transformed Ganoderma boninense, expressing green fluorescent protein. Phytopathology 107, 483–490. doi: 10.1094/PHYTO-02-16-0062-R
Govender, N., Wong, M. Y., Paterson, R. (2020). Opportunities for new-generation ganoderma boninense biotechnology. Ed. Nevalainen, H. (Cham, Switzerland: Springer), 477–500. doi: 10.1007/978-3-030-29541-7_17
Hadwiger, L. A. (1999). Host-parasite interactions: elicitation of defense responses in plants with chitosan. Eds. Jolles, P., Muzzarelli, R. A. A. (Switzerland: Birkhauser Verlag), 185–200. doi: 10.1007/978-3-0348-8757-1_13
Hamada, H., Linghu, Q., Nagira, Y., Miki, R., Taoka, N., Imai, R. (2017). An in planta biolistic method for stable wheat transformation. Sci. Rep. 7, 11443. doi: 10.1038/s41598-017-11936-0
Hanin, A. N., Parveez, G. K. A., Rasid, O. A., Masani, M. Y. A. (2020). Biolistic-mediated oil palm transformation with alfalfa glucanase (AGLU1) and rice chitinase (RCH10) genes for increasing oil palm resistance towards Ganoderma boninense. Ind. Crops Prod. 144, 112008. doi: 10.1016/j.indcrop.2019.112008
Hasan, Y., Turner, P. D. (1994). “Research at BAH LIAS research station on basal stem rot of oil palm,” in Proceedings of the 1st International Workshop on Perennial Crop Diseases caused by Ganoderma, UPM, Bangi, Selangor; Publisher: Palm Oil Research Institute of Malaysia, 28 November–3 December 1994.
Hasegawa, M., Mitsuhara, I., Seo, S., Imai, T., Koga, J., Okada, K., et al. (2010). Phytoalexin accumulation in the interaction between rice and the blast fungus. Mol. Plant Microbe Interact. 23, 1000–1011. doi: 10.1094/MPMI-23-8-1000
Hashim, K. B. (1991). “Results of four trials on Ganoderma basal stem rot of oil palm in golden hope estate,” in Proceedings of the Ganoderma Workshop, PORIM, Selangor, Malaysia, September 1990 (Bangi, Selangor: Palm Oil Research Institute of Malaysia). 67–80.
Hickey, L. T., Hafeez, A., Robinson, H., Jackson, S. A., Leal-Bertioli, S. C. M., Tester, M., et al. (2019). Breeding crops to feed 10 billion. Nat. Biotechnol. 37, 744–754. doi: 10.1038/s41587-019-0152-9
Ho, C. T., Khairudin, H. (1997). Usefulness of soil mounding treatments in prolonging productivity of prime-aged Ganoderma infected palms. Planter 73, 239–244.
Ho, P. Y., Namasivayam, P., Sundram, S., Ho, C. L. (2020). Expression of genes encoding manganese peroxidase and laccase of Ganoderma boninense in response to nitrogen sources, hydrogen peroxide and phytohormones. Genes 11, 1263. doi: 10.3390/genes11111263
Ho, C. L., Tan, Y. C., Yeoh, K. A., Ghazali, A. K., Yee, W. Y., Hoh, C. C. (2016). De novo transcriptome analyses of host-fungal interactions in oil palm (Elaeis guineensis jacq.). BMC Genom. 17, 1–19. doi: 10.1186/s12864-016-2368-0
Idris, A. S. (1999). Basal stem rot (BSR) of oil palm (Elaeis guineensis jacq.) in Malaysia: factors associated with variation in disease severity (London: University of London).
Idris, A. S. (2009). “Basal stem rot in Malaysia-biology, economic importance, epidemiology, detection and control,” in Proceeding of the International Workshop on Awareness, Detection and Control of Oil Palm Devastating Diseases, Kuala Lumpur Convention Centre, Malaysia, November 2009. 13–57.
Idris, A. S., Ariffin, D., Swinburne, T. R., Watt, T. A. (2000). “The identity of ganoderma species responsible for BSR disease of oil palm in Malaysia-pathogenicity test,” in MPOB Inf. Ser. MPOB TT No. 77b.
Idris, A. S., Ismail, S., Ariffin, D., Ahmad, H. (2002). “Control of Ganoderma-infected palm - development of pressure injection and field application,” in MPOB Inf. Ser. MPOB TT No. 131.
Idris, A. S., Ismail, S., Ariffin, D., Ahmad, H. (2004a). “Prolonging the productive life of Ganoderma infected palms with hexaconazole,” in MPOB Inf. Ser. MPOB TT No. 214.
Idris, A., Kushairi, A., Ismail, S., Ariffin, D. (2004b). Selection for partial resistance in oil palm progenies to Ganoderma basal stem rot. J. Oil Palm Res. 16, 12–18.
Idris, A. S., Maizatul, S. M. (2012a). “Stump treatment with dazomet for controlling Ganoderma disease in oil palm,” in MPOB Inf. Ser. MPOB TT No. 107.
Idris, A. S., Maizatul, S. M. (2012b). “Prolonging the productive life of Ganoderma infected oil palm with dazomet,” in MPOB Inf. Ser. MPOB TT No. 108.
Idris, A. S., Rajinder, S., Madihah, A. Z., Wahid, M. B. (2010). “Multiplex PCR-DNA kit for early detection and identification of Ganoderma species in oil palm,” in MPOB Inf. Ser. MPOB TT No. 73.
Jee, W. R., Chong, K. P. (2015). Ganoderma colonization in oil palm tissues and soil after treated with phenolic acids. Adv. Environ. Biol. 9, 7–12.
Kadarusman, Y. B., Herabadi, A. G. (2018). Improving sustainable development within Indonesian palm oil: the importance of the reward system. Sust. Dev. 26, 422–434. doi: 10.1002/sd.1715
Kanchiswamy, C. N. (2016). DNA-Free genome editing methods for targeted crop improvement. Plant Cell Rep. 35, 1469–1474. doi: 10.1007/s00299-016-1982-2
Kandan, A., Radjacommare, R., Ramanathan, A., Raguchander, T., Balasubramanian, P., Samiyappan, R. (2009). Molecular biology of Ganoderma pathogenicity and diagnosis in coconut seedlings. Folia Microbiol. 54, 147–152. doi: 10.1007/s12223-009-0022-9
Karmakar, S., Das, P., Panda, D., Xie, K., Baig, M. J., Molla, K. A. (2022). A detailed landscape of CRISPR-cas-mediated plant disease and pest management. Plant Sci. 323, 111376. doi: 10.1016/j.plantsci.2022.111376
Khairudin, H. (1990). Basal stem rot of oil palm: incidence, etiology and control (Selangor (Serdang: Universiti Pertanian Malaysia).
Khairunniza-Bejo, S., Vong, C. N. (2014). “Detection of basal stem rot (BSR) infected oil palm tree using laser scanning data,” in Second International Conference on Agricultural and Food Engineering; Agriculture and Agricultural Science Procedia, Kuala Lumpur, Malaysia (Amsterdam, The Netherlands: Elsevier B. V.), 2014 Dec 1–3. 156–164.
Khan, F. S., Goher, F., Zhang, D., Shi, P., Li, Z., Htwe, Y. M., et al. (2022). Is CRISPR/Cas9 a way forward to fast-track genetic improvement in commercial palms? prospects and limits. Front. Plant Sci. 13. doi: 10.3389/fpls.2022.1042828
Kurnia, J., Jangam, S., Akhtar, S. (2016). Advances in biofuel production from oil palm and palm oil processing wastes: a review. Biofuel Res. J. 3, 332–346. doi: 10.18331/BRJ2016.3.1.3
Lee, K. W., Omar, D., Cheng, G. L. E., Nasehi, A., Wong, M. Y. (2018). Characterization of phenazine and phenazine-1-carboxylic acid isolated from Pseudomonas aeruginosa UPMP3 and their antifungal activities against Ganoderma boninense. Pertanika J. Trop. Agric. Sci. 41, 1795–1809.
Lei, R., Li, Y., Li, L., Wang, J., Cui, Z., Ju, R., et al. (2022). A CRISPR/Cas12a-based portable platform for rapid detection of Leptosphaeria maculans in brassica crops. Front. Plant Sci. 13, 976510. doi: 10.3389/fpls.2022.976510
Liaghat, A., Mansor, S., Ehsani, R., Mohd Shafri, H. Z., Meon, A., Sankaran, S. (2013). Mid-infrared spectroscopy for early detection of basal stem rot disease in oil palm. Comp. Electron. Agric. 101, 48–54. doi: 10.1016/j.compag.2013.12.012
Liang, Z., Chen, K., Li, T., Zhang, Y., Wang, Y., Zhao, Q., et al. (2017). Efficient DNA-free genome editing of bread wheat using CRISPR/Cas9 ribonucleoprotein complexes. Nat. Commun. 8, 14261. doi: 10.1038/ncomms14261
Lim, P. H., Gansau, J. A., Chong, K. P. (2018). Streptomyces spp. as a potential biocontrol agent against Ganoderma boninense of basal stem rot in oil palm. J. Oil Palm Res. 30, 265–275. doi: 10.21894/jopr.2018.0024
Lim, K. H., Udin, W. (2010). “Management of Ganoderma in peat soil in Indonesia,” in Proceedings of the Second International Seminar on Oil Palm Diseases: Advances in Ganoderma Research and Management, Yogyakarta, Indonesia (Bangi, Selangor: Malaysian Palm Oil Board). 157.
Liu, H., Wang, H., Liao, X. L., Gao, B., Lu, X., Sun, D., et al. (2022). Mycoviral gene integration converts a plant pathogenic fungus into a biocontrol agent. Proc. Natl. Acad. Sci. U. S. A 119, e2214096119. doi: 10.1073/pnas.2214096119
Lotfi, R., Kalaji, H. M., Valizadeh, G. R., Khalilvand Behrozyar, E., Hemati, A., Gharavi-Kochebagh, P., et al. (2018). Effects of humic acid on photosynthetic efficiency of rapeseed plants growing under different watering conditions. Photosynthetica 56, 962–970. doi: 10.1007/s11099-017-0745-9
Lu, H. P., Liu, S. M., Xu, S. L., Chen, W. Y., Zhou, X., Tan, Y. Y., et al. (2017). CRISPR-s: an active interference element for a rapid and inexpensive selection of genome-edited, transgene-free rice plants. Plant Biotechnol. J. 15, 1371–1373. doi: 10.1111/pbi.12788
Madihah, A. Z., Idris, A. S., Rafidah, A. R. (2014). Polyclonal antibodies of Ganoderma boninense isolated from Malaysian oil palm for detection of basal stem rot disease. Afr. J. Biotechnol. 13, 3455–3463. doi: 10.5897/AJB2013.13604
Maluin, F. N., Hussein, M. Z., Yusof, N. A., Fakurazi, S., Idris, A. S., Hilmi, N. H. Z., et al. (2019b). Preparation of chitosan-hexaconazole nanoparticles as fungicide nanodelivery system for combating Ganoderma disease in oil palm. Molecules 24, 2498–2514. doi: 10.3390/molecules24132498
Maluin, F. N., Hussein, M. Z., Yusof, N. A., Fakurazi, S., Idris, A. S., Zainol Hilmi, N. H., et al. (2019a). Enhanced fungicidal efficacy on: Ganoderma boninense by simultaneous co-delivery of hexaconazole and dazomet from their chitosan nanoparticles. RSC Adv. 9, 27083–27095. doi: 10.1039/c9ra05417k
Mao, Y., Zhang, H., Xu, N., Zhang, B., Gou, F., Zhu, J. K. (2013). Application of the CRISPR–cas system for efficient genome engineering in plants. Mol. Plant 6, 2008–2011. doi: 10.1093/mp/sst121
Markom, M. A. B. (2009). Feasibility study of utilising electronic nose to detect BSR disease in oil palm plantation (Kangar (Perlis: Universiti Malaysia Perlis).
Meor Yusoff, M. S., Khalid, M. A., Idris, A. S. (2009). Identification of basal stem rot disease in local palm oil by microfocus XRF. Nucl. Related Technol. 6, 282–287.
Mercière, M., Boulord, R., Carasco-Lacombe, C., Klopp, C., Lee, Y. P., Tan, J. S., et al. (2017). About Ganoderma boninense in oil palm plantations of Sumatra and peninsular Malaysia: ancient population expansion, extensive gene flow and large scale dispersion ability. Fungal Biol. 121, 529–540. doi: 10.1016/j.funbio.2017.01.001
Midot, F., Lau, S., Wong, W. C., Tung, H. J., Yap, M. L., Lo, M. L., et al. (2019). Genetic diversity and demographic history of Ganoderma boninense in oil palm plantations of Sarawak, Malaysia inferred from ITS regions. Microorganisms 7, 464–480. doi: 10.3390/microorganisms7100464
Miller, R. N. G., Holderness, M., Bridge, P. D., Chung, G. F., Zakaria, M. H. (1999). Genetic diversity of Ganoderma in oil palm plantings. Plant Pathol. 48, 595–603. doi: 10.1046/j.1365-3059.1999.00390.x
Mohan, D., Namasivayam, P., Saidi, N. B., Ho, C. L. (2022). Temporal expression of defense-related genes in Ganoderma-infected oil palm roots. Trees 36, 1029–1038. doi: 10.1007/s00468-022-02269-1
Mohd Rakib, M. R., Bong, C. F. J., Khairulmazmi, A., Idris, A. S. (2014). Genetic and morphological diversity of Ganoderma species isolated from infected oil palms (Elaeis guineensis). Int. J. Agric. Biol. 16, 691–699.
Mohd Zuhar, L., Madihah, A. Z., Ahmad, S. A., Zainal, Z., Idris, A. S., Shaharuddin, N. A. (2021). Identification of oil palm’s consistently upregulated genes during early infections of Ganoderma boninense via RNA-seq technology and real-time quantitative PCR. Plants 10, 2026–2042. doi: 10.3390/plants10102026
Moncalvo, J. M. (2000). Systematics of ganoderma. Eds. Flood, J., Bridge, P. D., Holderness, M. (Wallingford, UK: CABI Publishing), 23–45. doi: 10.1079/9780851993881.0023
Mu, K., Ren, X., Yang, H., Zhang, T., Yan, W., Yuan, F., et al. (2022). CRISPR-Cas12a-based diagnostics of wheat fungal diseases. J. Agric. Food Chem. 70, 7240–7247. doi: 10.1021/acs.jafc.1c08391
Murphy, D. J. (2017). Transgenic oil palm. Eds. Soh, A. C., Mayes, S., Roberts, J. A. (Boca Raton, FL: CRC Press), 363–373.
Musa, H., Nusaibah, S. A., Khairulmazmi, A. (2018). Assessment on Trichoderma spp. mixture as a potential biocontrol agent of Ganoderma boninense infected oil palm seedlings. J. Oil Palm Res. 30, 403–415. doi: 10.21894/jopr.2018.0035
Mustafa, I. F., Hussein, M. Z., Saifullah, B., Idris, A. S., Hilmi, N. H. Z., Fakurazi, S. (2018a). Synthesis of (Hexaconazolezinc hexaconazolezinc/aluminum-layered double hydroxide nanocomposite) fungicide nanodelivery system for controlling Ganoderma disease in oil palm. J. Agric. Food Chem. 66, 806–813. doi: 10.1021/acs.jafc.7b04222
Mustafa, I. F., Hussein, M. Z., Seman, I. A., Hilmi, N. H. Z., Fakurazi, S. (2018b). Synthesis of dazomet zinc/aluminum layered double hydroxide nanocomposite and its phytotoxicity effect on oil palm seed growth. ACS Sustain. Chem. Eng. 6, 16064–16072. doi: 10.1021/acssuschemeng.8b02584
Naher, L., Yusuf, U. K., Habib, S. H., Ky, H., Siddiquee, S. (2018). Mycoparasitism activity of Trichoderma harzianum associated with chitinase expression against Ganoderma boninense. Pak. J. Bot. 50, 1241–1245.
Naidu, Y., Siddiqui, Y., Rafii, M. Y., Saud, H. M., Idris, A. S. (2017). Investigating the effect of white-rot hymenomycetes biodegradation on basal stem rot infected oil palm wood blocks: biochemical and anatomical characterization. Ind. Crops Prod. 108, 872–882. doi: 10.1016/j.indcrop.2017.08.064
Najihah, N. I., Hanafi, M. M., Idris, A. S., Hakim, M. A. (2015). Silicon treatment in oil palms confers resistance to basal stem rot disease caused by Ganoderma boninense. Crop Prot. 67, 151–159. doi: 10.1016/j.cropro.2014.10.004
Najmie, M. M. K., Khalid, K., Sidek, A. A., Jusoh, M. A. (2011). Density and ultrasonic characterization of oil palm trunk infected by Ganoderma boninense disease. Meas. Sci. Rev. 11, 160–164. doi: 10.2478/v10048-011-0026-x
Nelson, A. (2015) Half of Europe opts out of new GM crop scheme, guardian. Available at: https://www.theguardian.com/environment/2015/oct/01/half-of-europe-opts-out-of-new-gm-crop-scheme (Accessed December 20, 2022).
Ngando-Ebongue, G. F., Ajambang, W. N., Koona, P., Lalu Firman, B., Arondel, V. (2012). Oil palm. Ed. Gupta, S. K. (New York, USA: Springer Science), 165–200. doi: 10.1007/978-1-4614-0356-2_7
Nur Azura, A. B., Yusoff, M., Tan, G. Y. A., Jegadeesh, R., Appleton, D. R., Vikineswary, S. (2016). Streptomyces sanglieri which colonised and enhanced the growth of elaeis guineensis jacq. seedlings was antagonistic to Ganoderma boninense in in vitro studies. J. Ind. Microbiol. Biotechnol. 43, 485–493. doi: 10.1007/s10295-015-1724-4
Nurfahisza, A. R., Rafiqah, M. A., Masani, M. Y. A., Hanin, A. N., Rasid, O. A., Parveez, G. K. A., et al. (2014). Molecular analysis of transgenic oil palm to detect the presence of transgenes. J. Oil Palm Res. 26, 73–80.
Nusaibah, S. A., Saad, G., Tan, G. H. (2017). Antagonistic efficacy of Trichoderma harzianum and Bacillus cereus against Ganoderma disease of oil palm via dip, place and drench (DPD) artificial inoculation technique. Int. J. Agric. Biol. 19, 299–306. doi: 10.17957/IJAB/15.0280
Nusaibah, S. A., Siti Nor Akmar, A., Idris, A. S., Sariah, M., Mohamad Pauzi, Z. (2016). Involvement of metabolites in early defense mechanism of oil palm (Elaeis guineensis jacq.) against Ganoderma disease. Plant Physiol. Biochem. 109, 156–165. doi: 10.1016/j.plaphy.2016.09.014
Nusaibah, S. A., Siti Nor Akmar, A., Mohamad Pauzi, Z., Idris, A. S., Sariah, M. (2011). Detection of phytosterol in Ganoderma boninense infected oil palm seedlings through GC-MS analysis. J. Oil Palm Res. 23, 1069–1077.
Olaniyi, O. N., Szulczyk, K. R. (2020). Estimating the economic damage and treatment cost of basal stem rot striking the Malaysian oil palms. For. Policy Econ. 116, 102163. doi: 10.1016/j.forpol.2020.102163
Ommelna, B. G., Jennifer, A. N., Chong, K. P. (2012). The potential of chitosan in suppressing Ganoderma boninense infection in oil-palm seedlings. J. Sustain. Sci. Manage. 7, 186–192.
Ong, C. E., Goh, Y. K., Tan, S. Y., Goh, Y. K., Goh, K. J. (2018). A preliminary study on the effects of salicylic and jasmonic acids on Ganoderma boninense growth, mycelial hydrophobicity, and media pH under in vitro assays. Arch. Phytopathol. Plant Prot. 51, 122–127. doi: 10.1080/03235408.2018.1439870
Osterloh, K. R., Nusser, A. (2014). “X-Ray and neutron radiological methods to support the conservation of wooden artworks soaked with a polluting impregnant “Carbolineum”,” in Proceedings of the 11th European Conference on Non-Destructive Testing (Brussels, Belgium: European Federation), Prague, Czech Republic, 6–10 Oct 2014.
Parveez, G. K. A., Kamil, N. N., Zawawi, N. Z., Ong Abdullah, M., Rasuddin, R., Loh, S. K., et al. (2022). Oil palm economic performance in Malaysia and R&D progress in 2021. J. Oil Palm Res. 34, 185–218. doi: 10.21894/jopr.2022.0036
Paterson, R. R. M. (2007). Ganoderma disease of oil palm - a white rot perspective necessary for integrated control. Crop Prot. 26, 1369–1376. doi: 10.1016/j.cropro.2006.11.009
Paudzai, F. A. M., Sundram, S., Yusof, M. T., Angel, L., Hashim, A. M., Abdullah, S. N. A. (2019). Induced systemic resistance and promotion of plant growth in oil palm seedlings by endophytic Trichoderma virens. J. Oil Palm Res. 31, 572–581. doi: 10.21894/jopr.2019.0031
Pawlowski, M. L., Bowen, C. R., Hill, C. B., Hartman, G. L. (2016). Responses of soybean genotypes to pathogen infection after the application of elicitors. Crop Prot. 87, 78–84. doi: 10.1016/j.cropro.2016.04.022
Pedras, M. S. C., Ahiahonu, P. W. K. (2005). Metabolism and detoxification of phytoalexins and analogs by phytopathogenic fungi. Phytochemistry 66, 391–411. doi: 10.1016/j.phytochem.2004.12.032
Peiris, R. H., Budman, H., Moresoli, C., Legge, R. L. (2011). Identification of humic acid-like and fulvic acid-like natural organic matter in river water using fluorescence spectroscopy. Water Sci. Technol. 63, 2427–2433. doi: 10.2166/wst.2011.439
Pieterse, C. M. J., Leon-Reyes, A., Ent, S. V. D., Wees, S. C. M. V. (2009). Networking by small molecule hormones in plant immunity. Nat. Chem. Biol. 5, 308–316. doi: 10.1038/nchembio.164
Pilotti, C. A. (2005). Stem rots of oil palm caused by Ganoderma boninense: pathogen biology and epidemiology. Mycopathologia 159, 129–137. doi: 10.1007/s11046-004-4435-3
Pilotti, C. A., Gorea, E. A., Bonneau, L. (2018). Basidiospores as sources of inoculum in the spread of Ganoderma boninense in oil palm plantations in Papua new Guinea. Plant Pathol. 67, 1841–1849. doi: 10.1111/ppa.12915
Pilotti, C. A., Killah, G., Rama, D., Gorea, E. A., Mudge, A. M. (2021). A preliminary study to identify and distinguish southern tropical populations of Ganoderma boninense from oil palm via mating assays, sequence data, and microsatellite markers. Mycologia 113, 574–585. doi: 10.1080/00275514.2020.1858687
Pilotti, C. A., Sanderson, F. R., Aitken, E. A. B. (2003). Genetic structure of a population of Ganoderma boninense on oil palm. Plant Pathol. 52, 455–463. doi: 10.1046/j.1365-3059.2003.00870.x
Purba, R. Y., Utomo, C., Sipayung, A. (1994). Ganoderma research on oil palm and its current research in the Indonesian oil palm research institute. Ed. Holderness, M. (Wallingford, UK: CABI Publishing).
Rahamah Bivi, M., Paiko, A. S., Khairulmazmi, A., Akhtar, M. S., Idris, A. S. (2016). Control of basal stem rot disease in oil palm by supplementation of calcium, copper, and salicylic acid. Plant Pathol. J. 32, 396–406. doi: 10.5423/PPJ.OA.03.2016.0052
Rahman, K. A., Othman, R. (2020). Influence of pH levels on disease development in oil palm seedling roots infected with Ganoderma boninense. Rhizosphere 13, 100181. doi: 10.1016/j.rhisph.2019.100181
Rees, R. W., Flood, J., Hasan, Y., Cooper, R. M. (2007). Effects of inoculum potential, shading and soil temperature on root infection of oil palm seedlings by the basal stem rot pathogen Ganoderma boninense. Plant Pathol. 56, 862–870. doi: 10.1111/j.1365-3059.2007.01621.x
Rees, R. W., Flood, J., Hasan, Y., Potter, U., Cooper, R. M. (2009). Basal stem rot of oil palm (Elaeis guineensis), mode of root infection and lower stem invasion by Ganoderma boninense. Plant Pathol. 58, 982–989. doi: 10.1111/j.1365-3059.2009.02100.x
Rolph, H., Wijesekara, R., Lardner, R., Abdullah, F., Kirk, P. M., Holderness, M., et al. (2000). Molecular variation in ganoderma isolates from oil palm, coconut and betelnut. Eds. Flood, J., Bridge, P. D., Holderness, M. (Wallingford, UK: CABI Publishing), 205–221. doi: 10.1079/9780851993881.0205
Rosli, R., Amiruddin, N., Ab Halim, M. A., Chan, P. L., Chan, K. L., Azizi, N., et al. (2018). Comparative genomic and transcriptomic analysis of selected fatty acid biosynthesis genes and CNL disease resistance genes in oil palm. PloS One 13, 1–17. doi: 10.1371/journal.pone.0194792
Rozlianah, F. S., Jualang, A. G., Chong, K. P. (2015). Fatty acids and phenols involved in resistance of oil palm to Ganoderma boninense. Adv. Environ. Biol. 9, 11–16.
Sahebi, M., Hanafi, M. M., Wong, M. Y., Idris, A. S., Azizi, P., Jahromi, M. F., et al. (2015). Towards immunity of oil palm against Ganoderma fungus infection. Acta Physiol. Plant 37, 1–16. doi: 10.1007/s11738-015-1939-z
Said, N., Omar, D., Nasehi, A., Wong, M. Y. (2019). Pyraclostrobin suppressed Ganoderma basal stem rot (BSR), promoted plant growth and induced early expression of β-1,3-glucanase in oil palm (Elaeis guineensis). J. Oil Palm Res. 31, 248–261. doi: 10.21894/jopr.2019.0021
Sanderson, F. R., Pilloti, C. A. (1997). Ganoderma basal stem rot: an enigma or just time to rethink the problem. Planter 73, 489–493.
Santoso, H., Gunawan, T., Jatmiko, R. H., Darmosarkoro, W., Minasny, B. (2011). Mapping and identifying basal stem rot disease in oil palms in north Sumatra with QuickBird imagery. Precis Agric. 12, 233–248. doi: 10.1007/s11119-010-9172-7
Sariah, M., Zakaria, H. (2000). The use of soil amendments for the control of basal stem rot of oil-palm seedlings. Eds. Flood, J., Bridge, P. D., Holderness, M. (Wallingford, UK: CABI Publishing), 89–99. doi: 10.1079/9780851993881.0089
Shafri, H. Z. M., Anuar, M. I. (2008). “Hyperspectral signal analysis for detecting disease infection in oil palms,” in International Conference on Computer and Electrical Engineering, Phuket, Thailand (New York, U.S.: IEEE). 312–316.
Shafri, H. Z. M., Hamdan, N., Anuar, M. I. (2012). Detection of stressed oil palms from an airborne sensor using optimized spectral indices. Int. J. Remote. Sens. 33, 4293–4311. doi: 10.1080/01431161.2011.619208
Shcherbakova, L., Kartashov, M., Statsyuk, N., Pasechnik, T., Dzhavakhiya, V. (2020). Assessment of the sensitivity of some plant pathogenic fungi to 6-demethylmevinolin, a putative natural sensitizer able to help overcoming the fungicide resistance of plant pathogens. Antibiotics 9, 842–854. doi: 10.3390/antibiotics9120842
Sim, C. S. F., Cheow, Y. L., Ng, S. L., Ting, A. S. Y. (2019). Biocontrol activities of metal-tolerant endophytes against Ganoderma boninense in oil palm seedlings cultivated under metal stress. Biol. Control 132, 66–71. doi: 10.1016/j.biocontrol.2019.02.001
Singh, R., Ong-Abdullah, M., Low, E. T. L., Manaf, M. A. A., Rosli, R., Nookiah, R., et al. (2013). Oil palm genome sequence reveals divergence of interfertile species in old and new worlds. Nature 500, 335–339. doi: 10.1038/nature12309
Singh, B., Ryan, J. (2015). Managing fertilizers to enhance soil health (France: International Fertilizer Industry Association).
Soepena, H., Purba, R. Y., Pawirosukarto, S. (2000). A control strategy for basal stem rot (Ganoderma) on oil palm. Eds. Flood, J., Bridge, P. D., Holderness, M. (Wallingford, UK: CABI Publishing), 83–88. doi: 10.1079/9780851993881.0083
Soh, A. C. (2017). Breeding and genetics of the oil palm. Eds. Lai, O. M., Tan, C. P., Akoh, C. C. (Urbana, Il: AOCS Press), 31–58. doi: 10.1016/B978-0-9818936-9-3.50005-8
Soh, A. C. (2018). Applications and challenges of biotechnology in oil palm breeding. IOP Conf. Ser.: Earth Environ. Sci. 183, 12002. doi: 10.1088/1755-1315/183/1/012002
Soh, A. C., Mayes, S., Roberts, J., Barcelos, E., Amblard, P., Alvarado, A., et al. (2017). Elaeis oleifera × elaeis guineensis interspecific hybrid improvement. Eds. Soh, A. C., Mayes, S., Roberts, J. A. (Boca Raton, FL: CRC Press), 283–296. doi: 10.1016/B978-0-9818936-9-3.50005-8
Stichnothe, H., Schuchardt, F., Rahutomo, S. (2014). European Renewable energy directive: critical analysis of important default values and methods for calculating greenhouse gas (GHG) emissions of palm oil biodiesel. Int. J. Life Cycle Assess. 19, 1294–1304. doi: 10.1007/s11367-014-0738-x
Sujarit, K., Pathom-aree, W., Mori, M., Dobashi, K., Shiomi, K., Lumyong, S. (2020). Streptomyces palmae CMU-AB204T, an antifungal producing-actinomycete, as a potential biocontrol agent to protect palm oil producing trees from basal stem rot disease fungus, Ganoderma boninense. Biol. Control 148, 104307. doi: 10.1016/j.biocontrol.2020.104307
Sun, J., Qiu, C., Ding, Y., Wang, Y., Sun, L., Fan, K., et al. (2020). Fulvic acid ameliorates drought stress-induced damage in tea plants by regulating the ascorbate metabolism and flavonoids biosynthesis. BMC Genom. 21, 1–13. doi: 10.1186/s12864-020-06815-4
Sundram, S. (2013). The effects of Trichoderma in surface mulches supplemented with conidial drenches in the disease development of Ganoderma basal stem rot in oil palm. J. Oil Palm Res. 25, 314–325.
Sundram, S., Chris, D., Sioban, O., Idris, A. S. (2006). Preliminary studies on the development of monoclonal antibodies against mycelia of Ganoderma boninense, the causal pathogen of basal stem rot of oil palm. Malaysian J. Microbiol. 2, 30–34. doi: 10.21161/mjm.210605
Sundram, S., Meon, S., Seman, I. A., Othman, R. (2011). Symbiotic interaction of endophytic bacteria with arbuscular mycorrhizal fungi and its antagonistic effect on Ganoderma boninense. J. Microbiol. 49, 551–557. doi: 10.1007/s12275-011-0489-3
Supramani, S., Rejab, N. A., Ilham, Z., Wan-Mohtar, W. A. A. Q. I., Ghosh, S. (2022). Basal stem rot of oil palm incited by Ganoderma species: a review. Eur. J. Plant Pathol. 1-20. doi: 10.1007/s10658-022-02546-2
Surendran, A., Siddiqui, Y., Manickam, S., Ali, A. (2018). Role of benzoic and salicylic acids in the immunization of oil palm seedlings-challenged by Ganoderma boninense. Ind. Crops Prod. 122, 358–365. doi: 10.1016/j.indcrop.2018.06.005
Susanto, A., Sudharto, P., Purba, R. (2005). Enhancing biological control of basal stem rot disease (Ganoderma boninense) in oil palm plantations. Mycopathologia 159, 153–157. doi: 10.1007/s11046-004-4438-0
Susanto, A., Suprianto, E., Purba, A. R., Prasetyo, A. E. (2009). “Oil palm breeding for tolerance to G. boninense,” in Disease Management Strategies in Plantations, Jogjakarta, Medan, Indonesia; Indonesian Oil Palm Research Institute. 4–8.
Su’ud, M. M., Loonis, P., Idris, A. S. (2007). Towards automatic recognition and grading of Ganoderma infection pattern using fuzzy systems. T. Eng. Comput. Technol. 19, 1–6.
Takeshi, Y., Yuki, I., Naoto, S. (2000). Oligosaccharide elicitors and their receptors for plant defense responses. Trends Glycosci. Glycotechnol. 12, 113–120. doi: 10.4052/tigg.12.113
Tan, J. S., Lee, Y. P., Syed Alwee, L. Y., Kulandaivelu, S. S. R., Mercière, M., Zaremski, A., et al. (2018). Fungal diseases affecting oil palm FELDA global ventures research and development, Malaysia. Ed. Camus, L. (Cambridge, UK: Burleigh Dodds Science Publishing), 23–42.
Tay, Z. H., Chong, K. P. (2016). The potential of papaya leaf extract in controlling Ganoderma boninense. IOP conf. Ser.: Earth Environ. Sci. 36, 12027. doi: 10.1088/1755-1315/36/1/012027
Tee, S. S., Tan, Y. C., Abdullah, F., Ong-Abdullah, M., Ho, C. L. (2013). Transcriptome of oil palm (Elaeis guineensis jacq.) roots treated with Ganoderma boninense. Tree Genet. Genom. 9, 377–386. doi: 10.1007/s11295-012-0559-7
Thakur, M., Sohal, B. S. (2013). Role of elicitors in inducing resistance in plants against pathogen infection: a review. ISRN Biochem. 2013, 1–10. doi: 10.1155/2013/762412
Tisné, S., Pomiès, V., Riou, V., Syahputra, I., Cochard, B., Denis, M. (2017). Identification of Ganoderma disease resistance loci using natural field infection of an oil palm multiparental population. G3-Genes Genom. Genet. 7, 1683–1692. doi: 10.1534/g3.117.041764
Turnbull, N., de Franqueville, H., Breton, F., Jeyen, S., Syahoutra, I., Cochard, B., et al. (2014). “Breeding methodology to select oil palm planting material partially resistant to Ganoderma boninense,” in 5th quadrennial international oil palm conference, Bali Nusa Dua Convention Center, Medan, Indonesia; Indonesian. 17–19.
Turner, P. D. (1965). The incidence of Ganoderma disease of oil palms in Malaya and its relation to previous crop. Ann. Appl. Biol. 55, 417–423. doi: 10.1111/j.1744-7348.1965.tb07954.x
Vadamalai, G., Perera, A. A. F. L. K., Hanold, D., Rezaian, M. A., Randles, J. W. (2009). Detection of coconut cadang-cadang viroid sequences in oil and coconut palm by ribonuclease protection assay. Ann. Appl. Biol. 154, 117–125. doi: 10.1111/j.1744-7348.2008.00278.x
Varkkey, H., Tyson, A., Choiruzzad, S. A. B. (2018). Palm oil intensification and expansion in Indonesia and Malaysia: environmental and socio-political factors influencing policy. For. Policy Econ. 92, 148–159. doi: 10.1016/j.forpol.2018.05.002
Wakefield, E. M. (1920). Diseases of the oil palm in West Africa. Bull. Misc. Inform. 9, 306–308. doi: 10.2307/4107530
Wang, M., Gao, L., Dong, S., Sun, Y., Shen, Q., Guo, S. (2017). Role of silicon on plant–pathogen interactions. Front. Plant Sci. 8. doi: 10.3389/fpls.2017.00701
Wening, S., Rahmadi, H. Y., Arif, M., Supena, N., Siregar, H. A., Prasetyo, A. E., et al. (2016). “Construction of Ganoderma resistant oil palm planting material,” in 6th IOPRI-MPOB International Seminar: Current research and management of pests, Ganoderma, and pollination in oil palm for higher productivity, Medan, North Sumatera Indonesia, Indonesia (Medan, Indonesia: Indonesian Oil Palm Research Institute). 1–14.
Whalen, M. C. (2005). Host defence in a developmental context. Mol. Plant Pathol. 6, 347–360. doi: 10.1111/j.1364-3703.2005.00286.x
Widhalm, J. R., Dudareva, N. (2015). A familiar ring to it: biosynthesis of plant benzoic acids. Mol. Plant 8, 83–97. doi: 10.1016/j.molp.2014.12.001
Wong, W. C., Tung, H. J., Fadhilah, M. N., Midot, F., Lau, S., Melling, L., et al. (2021). Genetic diversity and gene flow amongst admixed populations of Ganoderma boninense, causal agent of basal stem rot in African oil palm (Elaeis guineensis jacq.) in Sarawak (Malaysia), peninsular Malaysia, and Sumatra (Indonesia). Mycologia 113, 902–917. doi: 10.1080/00275514.2021.1884815
Yeap, W. C., Norkhairunnisa, K. C. M., Norfadzilah, J., Muad, M. R., Appleton, D. R., Harikrishna, K. (2021). An efficient clustered regularly interspaced short palindromic repeat (CRISPR)/CRISPR-associated protein 9 mutagenesis system for oil palm (Elaeis guineensis). Front. Plant Sci. 12. doi: 10.3389/fpls.2021.773656
Yunus, A. M. M., Ho, C. L., Parveez, G. K. A. (2008). Construction of PHB and PHBV transformation vectors for bioplastics production in oil palm. J. Oil Palm Res. 2, 37–55.
Zakaria, L., Ali, N. S., Salleh, B., Zakaria, M. (2009). Molecular analysis of Ganoderma species from different hosts in peninsula Malaysia. J. Biol. Sci. 9, 12–20. doi: 10.3923/jbs.2009.12.20
Zakaria, L., Kulaveraasingham, H., Tan, S. G., Abdullah, F., Ho, Y. W. (2005). Random amplified polymorphic DNA (RAPD) and random amplified microsatellite (RAMS) of Ganoderma from infected oil palm and coconut stumps in Malaysia. Asian Pacific J. Mol. Biol. Biotechnol. 13, 23–34.
Zhang, L., Gao, M., Zhang, L., Li, B., Han, M., Alva, A. K., et al. (2013). Role of exogenous glycinebetaine and humic acid in mitigating drought stress-induced adverse effects in Malus robusta seedlings. Turk. J. Bot. 37, 920–929. doi: 10.3906/bot-1212-21
Zhang, Z., Mao, Y., Ha, S., Liu, W., Botella, J. R., Zhu, J. K. (2016). A multiplex CRISPR/Cas9 platform for fast and efficient editing of multiple genes in Arabidopsis. Plant Cell Rep. 35, 1519–1533. doi: 10.1007/s00299-015-1900-z
Keywords: control, diagnostics, Ganoderma boninense, oil palm, pathogenicity
Citation: Khoo YW and Chong KP (2023) Ganoderma boninense: general characteristics of pathogenicity and methods of control. Front. Plant Sci. 14:1156869. doi: 10.3389/fpls.2023.1156869
Received: 02 February 2023; Accepted: 23 June 2023;
Published: 10 July 2023.
Edited by:
Islam Hamim, Bangladesh Agricultural University, BangladeshReviewed by:
Chiew Foan Chin, University of Nottingham, MalaysiaCopyright © 2023 Khoo and Chong. This is an open-access article distributed under the terms of the Creative Commons Attribution License (CC BY). The use, distribution or reproduction in other forums is permitted, provided the original author(s) and the copyright owner(s) are credited and that the original publication in this journal is cited, in accordance with accepted academic practice. No use, distribution or reproduction is permitted which does not comply with these terms.
*Correspondence: Khim Phin Chong, Y2hvbmdrcEB1bXMuZWR1Lm15
Disclaimer: All claims expressed in this article are solely those of the authors and do not necessarily represent those of their affiliated organizations, or those of the publisher, the editors and the reviewers. Any product that may be evaluated in this article or claim that may be made by its manufacturer is not guaranteed or endorsed by the publisher.
Research integrity at Frontiers
Learn more about the work of our research integrity team to safeguard the quality of each article we publish.