- 1Commonwealth Scientific and Industrial Research Organisation (CSIRO) Agriculture and Food, Acton, ACT, Australia
- 2Commonwealth Scientific and Industrial Research Organisation (CSIRO) Microbiomes for One Systems Health Future Science Platform, Acton, ACT, Australia
- 3Commonwealth Scientific and Industrial Research Organisation (CSIRO) Advanced Engineering Biology Future Science Platform, Acton, ACT, Australia
In the search for new crop protection microbial biocontrol agents, isolates from the genus Streptomyces are commonly found with promising attributes. Streptomyces are natural soil dwellers and have evolved as plant symbionts producing specialised metabolites with antibiotic and antifungal activities. Streptomyces biocontrol strains can effectively suppress plant pathogens via direct antimicrobial activity, but also induce plant resistance through indirect biosynthetic pathways. The investigation of factors stimulating the production and release of Streptomyces bioactive compounds is commonly conducted in vitro, between Streptomyces sp. and a plant pathogen. However, recent research is starting to shed light on the behaviour of these biocontrol agents in planta, where the biotic and abiotic conditions share little similarity to those of controlled laboratory conditions. With a focus on specialised metabolites, this review details (i) the various methods by which Streptomyces biocontrol agents employ specialised metabolites as an additional line of defence against plant pathogens, (ii) the signals shared in the tripartite system of plant, pathogen and biocontrol agent, and (iii) an outlook on new approaches to expedite the identification and ecological understanding of these metabolites under a crop protection lens.
Introduction: Streptomyces-plant interactions and their role in crop protection
Microbial biocontrol offers an attractive ‘green’ alternative to widely used synthetic chemicals in the management of plant diseases and weeds, and there is a long history of bioprospecting for novel biocontrol strains (Barratt et al., 2018; Collinge et al., 2022). A microbial biological control agent (MBCA) is defined as a microorganism – or its natural products – used to combat pests, weeds, and diseases. MBCAs can function through one or more modes of action, (Collinge et al., 2022). These include competition for nutrients and space, parasitism, host-induced resistance, or antibiosis through the action of antimicrobial specialised metabolites, hydrolytic enzymes, or phytotoxins (Köhl et al., 2019; Boro et al., 2022). Differing modes of action can be delivered through individual microbes, with varying specificity and efficacy towards individual pathogens, or alternatively, brought together in assembled microbial consortia (Mukherjee et al., 2013; Fira et al., 2018; Mukhopadhyay and Kumar, 2020; Blake et al., 2021; Boro et al., 2022). The association of multiple MBCAs leads to combined modes of action that can act synergistically, providing more effective disease control than individual microbes (Bahkali et al., 2014; Minchev et al., 2021). Recent studies highlight that the strategic combination and deployment of multiple modes of action should be considered to overcome current gaps in the design of microbial inoculants for crop protection. Identifying the ecological function and chemical diversity of microbial specialised metabolites is essential to predict synergistic interactions in biocontrol consortia and during host microbiome assembly.
Members of the Streptomyces genus are of great interest as microbial inoculants due to their extensive secondary metabolism, producing over 70% of the natural products currently used in medicine and agriculture (Alam et al., 2022). In the field of plant health, Streptomyces spp. can exhibit beneficial functional traits through the production of specialised metabolites that enhance nutrient uptake, promote plant growth, alleviate abiotic stress, induce resistance, and prevent pathogen or pest invasion and establishment through nematicidal or insecticidal activities (Barka et al., 2016; Rey and Dumas, 2017; Newitt et al., 2019; O’Sullivan et al., 2021a; Park et al., 2023). Although predominantly associated with beneficial relationships, a small group of Streptomyces can produce phytotoxins and show pathogenic characteristics towards plants (Rey and Dumas, 2017; Alam et al., 2022). Several products containing Streptomyces microbial cells, or their purified metabolites, have been commercialised as biocontrol agents (Viaene et al., 2016; Rey and Dumas, 2017; Shi et al., 2020; Collinge et al., 2022). A non-exhaustive list of Streptomyces-derived specialised metabolites, which have been tested on live plants with a view to controlling plant disease, is shown in Table 1. The development of new biological control products is based on our ability to detect them within complex systems and confirm their bioactivity using sufficiently tailored screening methods. For example, many bioactivity screens and observable phenotypes will only detect the most abundant and/or most bioactive specialised metabolites. Compounding this issue, specialised metabolites produced by MBCAs in vitro are often much higher in concentration than levels produced in plant associations or in soil (reviewed in Köhl et al., 2019). Thus, better screening tools are required to place microbial specialised metabolite discovery and function into an ecological context.
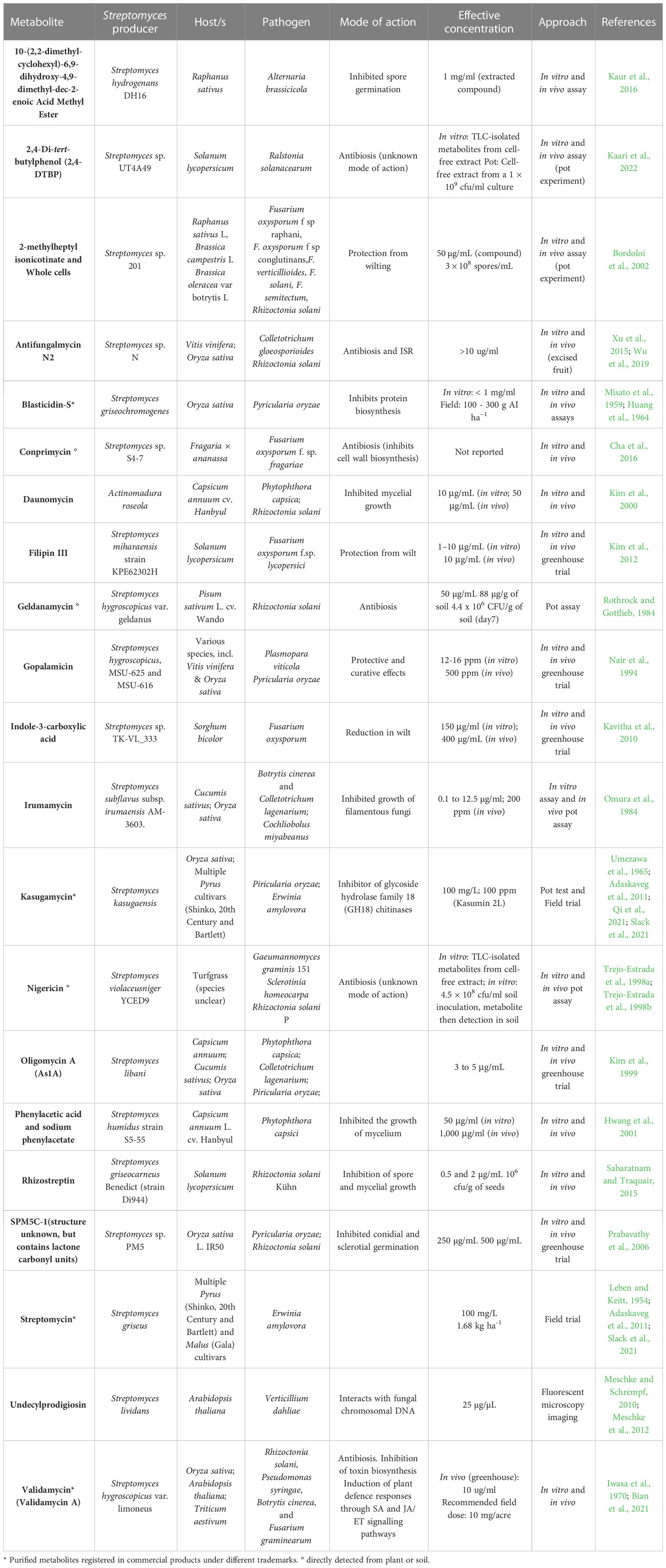
Table 1 Synthesis of well-characterised Streptomyces – pathogen – host interactions available in the literature.
In this review, we focus on the Streptomyces specialised metabolites that mediate host interactions with pathogens or host associated communities. We focus on the discrepancies between in vitro and in vivo analysis of Streptomyces biocontrol agents. This review brings together the disciplines of biocontrol and microbial ecology to compare metabolites produced by MBCAs and the range of novel specialised metabolites found in plant microbiomes which are yet to be characterised, and how these metabolites can be exploited for plant health and post-harvest applications. Finally, we highlight some of the challenges and new approaches used for in vivo metabolite characterisation such as the use of biosensors, and novel culturing techniques for the discovery, deployment, and manipulation of Streptomyces and their specialised metabolites for plant protection.
Exploitation of Streptomyces specialised metabolite potential
Recent reviews estimate that Streptomyces species have the potential to produce at least 150,000 more specialised metabolites than currently known (Barka et al., 2016; Donald et al., 2022; Lacey and Rutledge, 2022). This metabolic potential can be attributed to their impressive diversity of biosynthetic gene clusters (BGCs), the highest of all known bacteria (Gavriilidou et al., 2022). Biosynthetic gene clusters are defined as a group of genes located physically near or adjacent to one another in the genome, which encode the enzymes required for the synthesis of a particular specialised metabolite. The cluster may also include genes for regulation of the metabolite in question, its transport, or self-resistance (Medema et al., 2015; Kautsar et al., 2020).
Streptomyces populations residing in soils or associated with plants exist in a resource- and space-competitive environment. The capacity to produce diverse specialised metabolites reflects the versatility required to thrive in challenging environments (Brader et al., 2014; Viaene et al., 2016; Hoskisson and Fernández-Martínez, 2018). Furthermore, for those Streptomyces spp. that reside as endophytes (most of which originate from bulk and rhizosphere soils), larger repertoires of specialised metabolites may be required, both to outcompete other organisms in multiple ecological niches (e.g., soil and plant endosphere), and as signals mediating interactions with host and associated microbiome. Given the size of BGCs, the expression of the biosynthetic components and subsequent biosynthesis of the specialised metabolites are energy demanding processes. To compensate for this energy cost, the benefit of their biological activity must be substantial, and the process tightly regulated (Hoskisson and Fernández-Martínez, 2018). In addition, the bacterium must maintain physiological regulation of specialised metabolite production for survival, growth, and sporulation processes. A detailed review by Hoskisson and Fernández-Martínez (2018) reports the complex external and internal factors influencing the regulation of specialised metabolite production in Streptomyces and other Actinobacteria – including sensors, regulators, signalling molecules, and metabolite precursors.
Several studies have targeted the isolation of new Streptomyces species (spp.) and other MBCAs from plant compartments, with the aim of identifying novel microbial strains and specialised metabolites for use against plant diseases (Brader et al., 2014; Collinge et al., 2022; Wang et al., 2022). For example, endophytic Streptomyces isolates collected from the roots of healthy wheat plants in Western Australia suppressed wheat fungal diseases in planta and promoted expression of genes involved in plant defence (Coombs and Franco, 2003; Franco et al., 2007; Belt et al., 2021; O’Sullivan et al., 2021b). While metabolites were not examined in planta, fungal suppression was attributed to antifungal metabolites, the activity of which was detectable using in vitro assays and cell-free fermentation extracts. Endophyte culture collections such as these, among others (Matsumoto and Takahashi, 2017), provide a great experimental resource to study the potential plant colonisation of Streptomyces MBCAs across host species and genotypes, and for analysis of in planta metabolite production.
An underexplored component of the Streptomyces metabolome associated with plant interactions are its volatile organic compounds (VOCs). Often, these metabolites are studied in vitro using microbes grown in the absence of interacting organisms (reviewed in (Korpi et al., 2009; Meredith and Tfaily, 2022)). However, Streptomyces can be prolific producers of VOCs, with in vitro studies detecting 40 or more VOCs from individual strains (Li et al., 2010; Cordovez et al., 2015). Produced in small quantities in their ecological niches, Streptomyces VOCs can be exploited in post-harvest crop protection to reduce chemical residues on foods (Zhao et al., 2022). Commonly identified antifungal VOC specialised metabolites from Streptomyces spp. and other MBCAs include dimethyl disulfide and dimethyl trisulfide (Li et al., 2010; Kang et al., 2021). More recently β-caryophyllene, l-linalool, and 2-ethyl-5-methylpyrazine have been isolated from a range of Streptomyces spp. and can suppress fungal rot or spot diseases in fruits and nuts (Lyu et al., 2020; Boukaew et al., 2021; Gong et al., 2022). Streptomyces non-volatile specialised metabolites can also play an important role in the management of post-harvest disease in fruit. For example, the macrolides lucensomycin and 32,33-didehydroroflamycoin, or reveromycin polyketides isolated from fermentation broths of Streptomyces spp. have been effective in inhibiting fruit rot caused by the fungal pathogen Botrytis cinerea in grapes, tomatoes, and strawberries (Lyu et al., 2017; Kim et al., 2019; Kim et al., 2020; Nguyen et al., 2021). In addition, the antimicrobial polymer Epsilon-poly-L-lysine isolated from several Streptomyces spp. is widely used in the agricultural and pharmaceutical industries and has activity against post-harvest fungal diseases, such as grey mould (B. cinerea), blue mould (Penicillium expansum), and anthracnose (Colletotrichum gloeosporioides) in avocado, mango, and papaya fruits [(Bai et al., 2022); also reviewed in Wang et al. (2021)].
Challenges of traditional in vitro approaches for MBCA and specialised metabolite discovery
In our effort to better understand and exploit novel MBCAs and their specialised metabolites, culturable microbes of interest are typically characterised from collections, in isolation. This involves growing individual strains on nutrient medium under controlled conditions (Collinge et al., 2022). However, changes in nutrient medium can lead to the isolation and identification of different specialised metabolites with varying efficacy against targeted pathogens (Rateb et al., 2011; Rashad et al., 2015; Hemphill et al., 2017; Köhl et al., 2019; Pan et al., 2019). The nutrient concentration in conventional culture media is often vastly different from those found in soils and plant tissues and may inadvertently disadvantage the growth and activity of specific microbes of interest (Köhl et al., 2019). Nutrient stress or alteration studies, commonly referred to as OSMAC approaches (One Strain Many Compounds, reviewed in Pan et al., 2019), shed light on the effects of nutrient limitation in activating the production of specialised metabolites in Streptomyces spp. (reviewed in Hoskisson and Fernández-Martínez, 2018). For example, the depletion of sugar or other nutrients is commonly associated with the activation of microbial secondary metabolite production (e.g., antibiotics), as a means of sensing microbial competition in the environment and is an important survival mechanism (Hoskisson and Fernández-Martínez, 2018; Van Bergeijk et al., 2020). Apart from nutrient depletion, factors influencing the morphology of Streptomyces mycelia, or its exploratory growth mode are also emerging as triggers for differential BGC expression in vitro versus in planta (Dobson et al., 2008; Celler et al., 2012; Shepherdson and Elliot, 2022).
While in vitro studies have been fruitful in isolating potential Streptomyces MBCAs and identifying some of the signals that elicit specialised metabolite production, genome sequencing has revealed that this genus encodes far greater metabolic potential than was previously thought. Furthermore, our understanding of the ecological signals that trigger BGC expression is far from complete. In fact, the metabolic potential of Streptomyces may be underestimated by up to 90%, with the handful of compounds detected in laboratory grown cultures being a mere snapshot of their genomic potential (Rutledge and Challis, 2015; Barka et al., 2016; Rey and Dumas, 2017; Hoskisson and Fernández-Martínez, 2018; Van Bergeijk et al., 2020). Indeed, some individual strains possess more than 100 putative BGCs (Rutledge and Challis, 2015; Rey and Dumas, 2017; Moore et al., 2023). When the biotic or abiotic conditions required to activate BGC expression are unknown, the BGC is considered ‘silent’. Others are termed ‘cryptic’ if the specialised metabolite, activity, or phenotype cannot be linked to a predicted BGC. Recently, the structural characteristics and genomic clustering of BGCs have facilitated the development of several BGC predictive genome mining tools, such as AntiSMASH (Blin et al., 2021). However, more advances are required to identify conditions inducing BGC expression, the chemical structure of the resulting metabolites, and their function.
This large, underexplored world of Streptomyces metabolic potential leads us to question current approaches used for bioprospecting and MBCA discovery. To date, the screening of most available MBCAs and their specialised metabolites have been conducted in vitro, by culturing microbe and pathogen in isolation. These experiments restrict our ability to predict the behaviour of MBCAs in planta. As such, in vitro screening methods to identify biocontrol agents have overlooked strains which, when subsequently grown in planta, produce positive results. Recently, Besset-Manzoni and co-workers (2019) asked the question “Does in vitro selection of biocontrol agents guarantee success in planta?” Using a library of over 200 bacteria isolated from soil, the authors screened for in vitro growth inhibition against the wheat fungal pathogen Fusarium graminearum, using dual-culture agar plate assays and cell-free supernatants containing secreted compounds from liquid cultures. Low correlation between assays was observed, suggesting growth media affected the production of antifungal compound(s) and/or co-culturing bacteria and pathogen may be required to elicit antifungal metabolite production. A subset of bacterial strains evaluated for disease suppression on wheat indicated that most active strains in vitro were not necessarily the most effective in planta, and vice versa. With a focus on screening Streptomyces spp. and other Actinobacteria against the wheat pathogen Fusarium pseudograminearum, O’Sullivan independently arrived at similar outcomes (O’Sullivan et al., 2021b). These studies highlight not only a lack of translation from in vitro screens to in planta bioactivity, but also that conclusions made solely on in vitro screens may lead to promising biocontrol microbes being overlooked.
Bespoke in vitro approaches for specialised metabolite production
While in vitro workflows enable isolation of specialised metabolites from pure cultures, they are only suitable for MBCA that can be cultured, and lack any insight into the behaviour of the isolate in the environment (Figure 1). We can apply our knowledge of the plant and microbe relationships influencing Streptomyces secondary metabolism to tailor in vitro approaches better aligned to an ecological context. For example, Streptomyces co-cultivation with other microbes has proven to be quite useful. In 2017 Yu and coworkers studied capacity of Streptomyces spp., non-Streptomyces bacteria, and fungi to increase biocontrol activity of Streptomyces rimosus against the plant pathogen Fusarium oxysporum f. sp. cucumerinum through co-culturing (Yu et al., 2017). Co-cultivation with fungal species recorded the highest induction ability, indicating perception of fungal-derived signals may be required for the activation of antifungal BGCs and their compounds. Fungal cell wall chitin subunits and their derivates also induce expression of antibiotics in Streptomyces (Nazari et al., 2013). Further examples of beneficial in vitro microbial interactions are the co-culturing of Streptomyces coelicolor and the soil bacterium Myxococcus xanthus, leading to a 20-fold increase in production of a polyketide antibiotic (Pérez et al., 2011), and the co-culturing of multiple Streptomyces spp. to induce antibiotic production (Ueda et al., 2000).
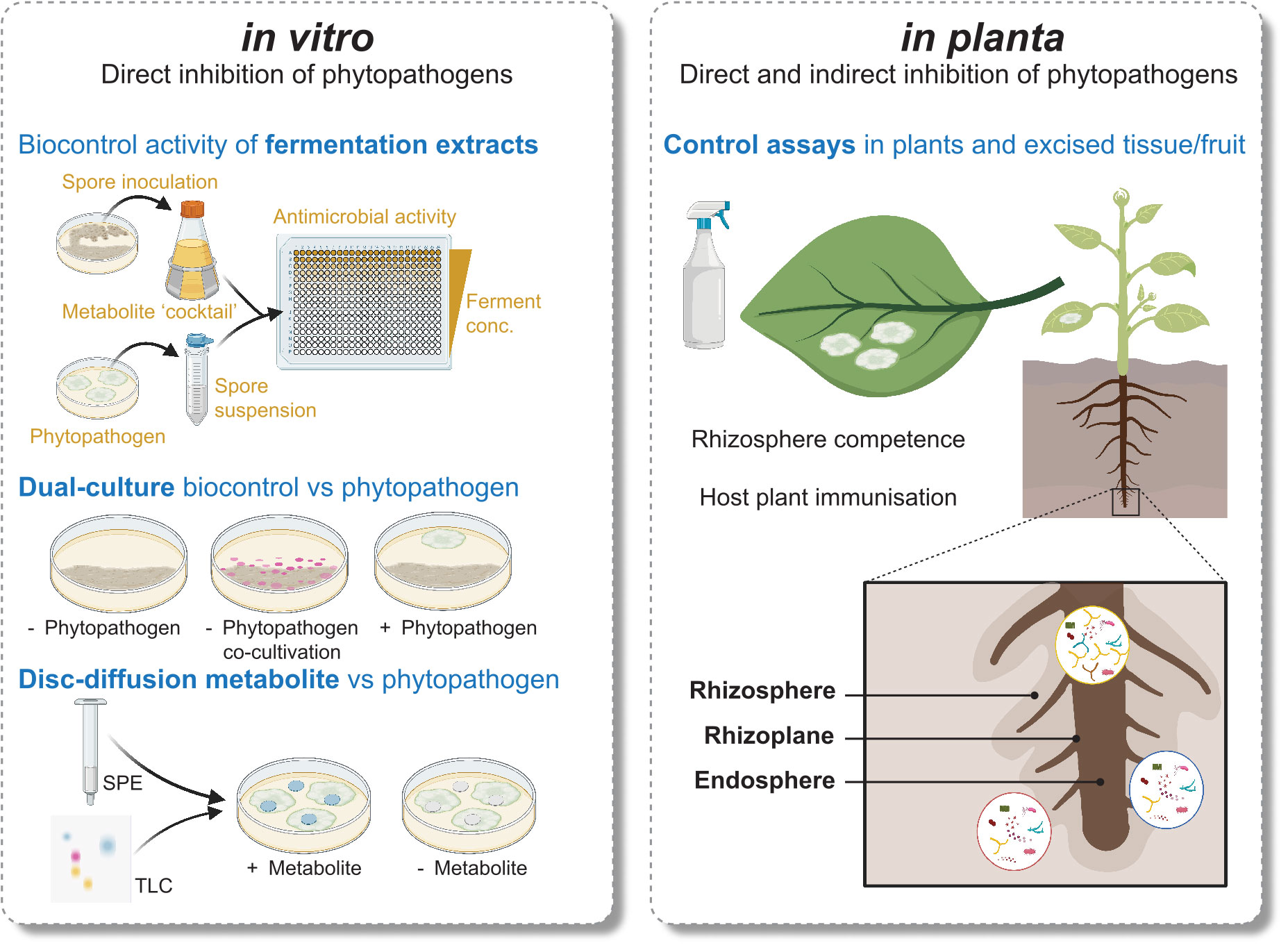
Figure 1 Comparison of several in vitro and in planta approaches used to discover and characterise Streptomyces MBCA and specialised metabolites.
Streptomyces-derived elicitors can also be used to modulate secondary metabolism and promote expression of cryptic BGCs. For example, the linear azole-containing peptide goadsporin - originally named after its ability to stimulate sporulation - isolated from the soil Streptomyces sp. strain TP-A0584 promotes specialised metabolite production in a broad range, but not all, Streptomyces spp. (Onaka et al., 2001; Arnison et al., 2013; Onaka, 2017). In another example, Yamanaka et al. (2005) identified the siderophore desferrioxamine E produced by Streptomyces griseus and other members of Actinobacteria, induces aerial mycelium formation, and antibiotic and pigment production. Co-cultivation and elicitation studies provide a mechanism for activating and deciphering the inter- and intradomain interaction signals that manipulate production of Streptomyces metabolites, and how these may play out in plant-associated environments.
Streptomyces in the plant-soil environment
The use of next-generation sequencing technologies to study microbial diversity has substantiated long-standing hypotheses that Streptomyces spp. dominate microbial communities across soil ecosystems, especially in the root microbiome (Goodfellow and Williams, 1983; Viaene et al., 2016; Matsumoto and Takahashi, 2017). While the relative abundance of Streptomyces differs among plant species and genotypes, studies generally agree on their strong enrichment in the root endosphere (within the root), compared to the rhizosphere (soil surrounding the root) and bulk soil (reviewed by Viaene et al., 2016). For example, Streptomyces are enriched in the root endosphere of Arabidopsis thaliana (Arabidopsis) and rice plants (Bulgarelli et al., 2012; Edwards et al., 2015). Furthermore, Singer et al. (2019) found a consistent enrichment of Streptomyces in the root endosphere and rhizoplane (the root surface) of four different switchgrass genotypes compared to rhizosphere and bulk soils.
Although edaphic factors are the main drivers of root microbiome assembly (Bulgarelli et al., 2013), several studies indicate that Streptomyces interactions are host-dependent (Bakker et al., 2014; Becklund et al., 2016). This suggests that host-derived signals can shape the plant-associated microbiome composition in a manner that favours Streptomyces diversity and richness. In line with this, Fitzpatrick et al. (2018) reported the differential capacity of angiosperm species to specifically recruit Streptomyces under drought-stress conditions. The high rates of Streptomyces colonisation under stressful environmental conditions could also be explained by the spore-forming ability of Actinobacteria, which provides greater resilience compared to less hardy bacterial lineages (Nessner Kavamura et al., 2013; Taketani et al., 2017). The production of antimicrobial compounds by Streptomyces that inhibit the growth of competing microbes is another leading hypothesis for their abundance in plants (Fitzpatrick et al., 2018). While the use of tagged strains and electron microscopy have further confirmed Streptomyces’ ability to colonise root structures, little is known about the host-selection mechanisms leading to Streptomyces root recruitment. For instance, studies combining DNA profiling and stable isotope labelling techniques to identify taxa that can metabolise photosynthates have demonstrated that Streptomyces struggle to compete for root exudates in the root microbiomes of Arabidopsis and wheat (Prudence et al., 2021; Worsley et al., 2021). Taken together, our (arguably restricted) understanding of the strategies for Streptomyces recruitment and the unknown factors driving root colonisation, suggests the presence of host-specific mechanisms and Streptomyces preference for host traits.
In planta approaches for biocontrol and specialised metabolite discovery
Culture-dependent MBCA research has left many knowledge gaps: How do MBCAs behave in planta? Are they producing the same metabolites as observed in pure culture, and if not, what else might they be producing? In vitro experiments suggest that Streptomyces secondary metabolite biosynthesis is increased by compounds such as phytohormones (Worsley et al., 2020) and chitin (Nazari et al., 2013), indicating that in planta production of antimicrobial compounds is influenced by plant host and nearby microbes. As Streptomyces secondary metabolite diversity seems linked to interactions with hosts and microbial communities, future research on the characterisation of Streptomyces silent BGCs and cryptic secondary metabolites should take place in response to host environments (Seipke et al., 2012; Nazari et al., 2013).
Recent advances in metabolomics, metatranscriptomics, and metagenomics has helped to bridge the knowledge gap, shedding light on the activity of individual biocontrol agents within soils, the rhizosphere, and the endosphere, including the metabolic potential of the entire microbial community associated with plants (Figure 2).
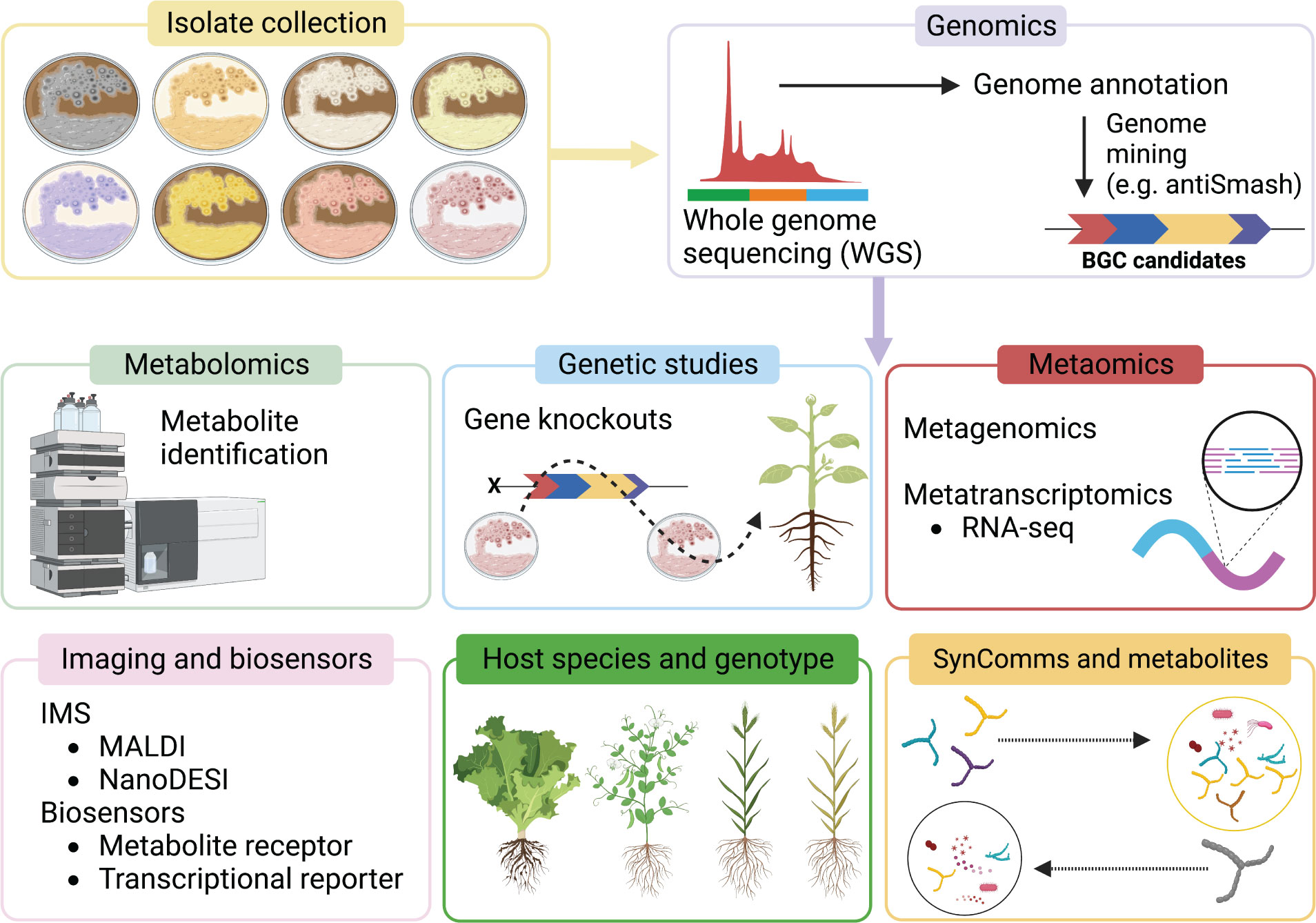
Figure 2 Schematic of current and emerging approaches used to identify and characterise Streptomyces MBCA and specialised metabolites. Tools such as genetic studies, metaomics, imaging mass spectrometry (IMS), biosensors, and new bioinformatics approaches are particularly informative components for functional characterisation.
Omics approaches reveal the true metabolic potential of plant and soil microbiomes
The past two decades have witnessed remarkable advances in the speed and accuracy of Omics technologies used to quantify and characterise an organism’s complement of biopolymers (DNA, RNA, and proteins) and metabolites. Further developments have expanded the scale of these Omics approaches to the community level, capturing an unprecedented view of the taxonomic and functional diversity of microbes that populate plants and their environments (Metaomics). Metagenomic surveys have been used to great effect in cataloguing the genetic potential encoded in complex microbial communities and in the identification of putative BGCs involved in the biosynthesis of specialised metabolites (Santana-Pereira et al., 2020; Van Goethem et al., 2021; Chen et al., 2018). Metatranscriptomics and metaproteomics have enhanced these insights with global snapshots of expression dynamics, providing community-level information on the magnitude, timing, and regulatory factors associated with BGC production (Anderson and Fernando, 2021; Edlund et al., 2018). Finally, metabolomics completes this picture by identifying the totality of metabolites present in complex environmental samples, and in so doing, resolving the link between the genetic potential of microbial consortia and the specialised metabolites they produce. In this way, Metaomic approaches have identified (and begun to close) the considerable gap between predicted and experimentally verified microbial specialised metabolites. For example, two similar studies by Crits-Christoph and colleagues used a combination of metagenomic and metatranscriptomic approaches to identify several hundred novel BGCs from understudied bacterial phyla in soil samples (Crits-Christoph et al., 2018; Crits-Christoph et al., 2022). This vast diversity of BGCs in bulk soils is corroborated by the works of Carrión and colleagues, who in 2019 combined multiple metaomics techniques to quantify the number of BGCs in root microbiomes grown in disease suppressive soil (Carrión et al., 2019). Out of the 700 BGCs that were detected in this study, only 10 could be confidently assigned with a metabolite product. Further metatranscriptomic investigation indicated that none of those ten well characterised BGCs were expressed in planta. Instead, a handful of uncharacterised BGC were correlated with disease suppression, one of which was confirmed via isolation of the bacterium harbouring the BGC. A mutant line, with deletions within the BGC failed to suppress disease like the wild type did. Such metaomic studies clearly show that we have only begun to scratch the surface when it comes to metabolic potential in soils and plant microbiomes, however technical hurdles remain to better understand this potential. Without sufficient sequencing read depth (bot RNA and DNA sequencing) BGCs can be missed, or have very low transcript abundance. And while identifying BGCs in genome sequence data is straightforward, assigning a metabolite product nevertheless requires experimental validation.
While bulk soil contains vast numbers of BGCs, producing almost entirely unknown metabolites from extremely diverse prokaryote taxa, plant microbiomes are considerably more streamlined, with Streptomyces being one of the major taxa. This was recently demonstrated by Dror and colleagues, who utilised a range of metabarcoding and metagenomics techniques to compare the abundance of BGCs from the roots of lettuce, tomato, and bulk soil (Dror et al., 2020). Focusing on those BGCs encoding polyketide synthase (PKS) and non-ribosomal peptide synthase genes (NRPS), the authors showed that Streptomyces spp. were responsible for many of the BGCs expressed in roots. The researchers were able to assign a putative metabolite product for a larger portion of those BGCs expressed and identify the same gene cluster from other root metagenomes. Using similar metabarcoding techniques, Aleti et al. investigated potato rhizosphere samples from Peru (Aleti et al., 2017), again finding Streptomyces comprising a substantial portion of the rhizosphere community, while encoding over one thousand putative PKS and NRPS gene clusters. Interestingly, in both studies, some of the most abundant and highly expressed gene clusters detected were encoded by Streptomyces spp., and the predicted metabolite products matched characterised specialised metabolites with known antibiotic properties. With respect to plant diseases, this supports the long-held hypothesis that Streptomyces spp. are ‘recruited’ by plants to exploit the strong antifungal and antibacterial metabolites they produce. The relatively high success in assigning putative metabolite products to common or abundant gene clusters in plant microbiomes observed here also suggests these gene clusters represent microbial functions of particular benefit to the host plant. Overall, these studies demonstrate that complementary metaomic approaches can be used to comprehensively detect and characterise the MBGC potential of plant and soil microbiomes, which greatly increase the scale and speed of research surveying for novel pharmaceutical and agricultural products, as well as unravelling the role MBGCs have in shaping plant-microbe ecology and evolution.
In planta analyses of potential MBCA specialised metabolites
In comparison to studies using sequencing-based techniques to detect the presence and/or transcription of genes encoding antifungal metabolites, there is a paucity of works in which active metabolites are measured in planta, or its biosynthesis confirmed via knockout mutants of the MBCA. Even by widening the focus of this review to include MBCAs of other bacterial genera, very few studies have been able to confirm whether active metabolites produced in vitro account for the biocontrol activity seen in planta. In contrast, more attention has been given to studying the other half of pathogen-MBCA interactions, through the analysis of toxins produced by fungal pathogens, such as those produced during head blight infections by Fusarium sp. (Palazzini et al., 2007; Palumbo et al., 2008; Palazzini et al., 2016).
Several studies have been able to assign Streptomyces specific metabolites to disease suppressive activity in certain soils and plant species. In 2016, Cha and colleagues investigated soil that was suppressive to Fusarium wilt in strawberries (Cha et al., 2016). They isolated a strain of Streptomyces (sp. S4-7) from this soil, and through mutagenesis and chemistry studies, assigned the antifungal activity to a thiopeptide they named conprimycin. When introduced to strawberry plants challenged with Fusarium, wild type S4-7 provided the plant host with an increased resistance to Fusarium, while conprimycin deficient mutants did not. Interestingly, mutants deficient in ectoine production (a small molecule implicated in protein chaperone interactions and osmoprotectant roles) were also conprimycin deficient, and unable to control Fusarium wilt (Cha et al., 2016). This work mirrors earlier studies by Rothrock and Gottlieb (1984), who asserted that the benzoquinone geldanamycin, produced by Streptomyces sp. in Rhizoctonia resistant soils, was one of the primary active metabolites in the soil (Rothrock and Gottlieb, 1984). Also focusing on Rhizoctonia, works by Trejo-Estrada and colleagues detected nigericin and geldanamycin from Streptomyces sp. YCED9 in soil and thatch grass samples (Trejo-Estrada et al., 1998a). Geldanamycin was also identified as the active metabolite produced by Streptomyces melanosprofaciens EF-76 in a more recent study (Agbessi et al., 2003). Geldanamycin deficient mutants of S. melanosprofaciens EF-76 were unable to control Streptomyces scabies (the causal agent of potato scab) in field trials, while the wild type displayed biocontrol activity. Collectively, these studies show that specific gene clusters can account for disease resistant soils, as well as the relative ubiquitousness of geldanamycin in these systems, and highlight the power of gene knockouts to confirm MBCA modes of action.
Similar reverse genetic approaches have been used in planta to identify specialised metabolites from well-studied MBCAs. A combination of culture-independent and culture-dependent methods were utilised to identify a specific BGC in Pseudomonas sp. CH-C52 from soils suppressive to Rhizoctonia solani infections. Knockouts of this gene cluster resulted in Pseudomonas sp. CH-C52 that were ineffective at protecting sugar beet seedlings from the disease (Mendes et al., 2011). The metabolite in question, thanamycin (a member of the syringomycin family), was later identified using innovative methods, such as mass spectrometry imaging and mass spectral networking (Watrous et al., 2012; van der Voort et al., 2015). Again, in Pseudomonas, Chen et al. (2018) studied the microbiome of wheat heads, isolating the novel biocontrol Pseudomonas piscium, which protected the host from Fusarium graminearum infections. Using knockout mutants, the authors identified that antifungal activity was mediated by phenazine-1-carboxamide (Chen et al., 2018). In another effort to understand the biocontrol of F. graminearum, Crane et al. (2013) were able to quantify the antifungal lipopeptide iturin produced in planta by the commercial MBCA strain Bacillus amyloliquefaciens “TrigoCor”. While the population of B. amyloliquefaciens remained constant during the experimental period, the level of iturin on wheat heads decreased over time. This study perfectly illustrates the need to adopt analytical techniques within in planta studies, to confirm that the desired metabolites of MBCAs are present when and where required.
The research summarised above, discussing both untargeted analyses of microbiome secondary metabolism and focused studies on single bacteria, highlights the dichotomy currently present in biocontrol research. While plant microbiomes present a nearly inexhaustible wealth of novel metabolites that can be exploited to combat plant pathogens, MBCAs are typically first identified via in vitro methods, with little in planta analyses to understand their behaviour once the host plant has been colonised. These in vitro tests rely on the active metabolite(s) being synthesised in planta, an environment far removed from that of an agar plate, which means MBCA screenings are biased towards isolates that express gene clusters in both settings. Detecting MBCA-derived metabolites in planta is rarely conducted but is necessary to validate many assumptions within the field of research. While analytical chemistry techniques may not be sensitive enough to detect the metabolites in question, biosensor-based methods have the potential to detect low abundance metabolites in planta, and even provide spatial information.
Biosensors as direct or indirect reporters of MBCA and specialised metabolite activity
Biosensors are enzymes, antibodies, or microorganisms, which can detect specific molecules in a sample and report their presence as a quantifiable signal. As such, they can be engineered to respond to specialised metabolite activity (Liu et al., 2015). For example, under the control of a metabolically responsive promoter or a specific metabolite ligand-binding receptor, biosensors are often expressed in a microbial host that in the presence or absence of a target molecule in the microbes environment activates a signal, such as fluorescence. Biosensors with sufficient sensitivity and/or adequate abundance of the reporter protein can facilitate spatial and temporal detection of metabolites. Biosensors have been frequently applied to Streptomyces within in vitro settings to identify conditions that improve antibiotic production or in molecular approaches to manipulate BGC expression (Ostash et al., 2010; Guo et al., 2015; Sun et al., 2017; Kasey et al., 2018; Li et al., 2019). However, they are also useful tools to gain an understanding of microbial metabolites in ecological settings (Leveau and Lindow, 2002; Zhang and Keasling, 2011). For example, Hansen et al. (2021) developed a biosensor to detect the bacterial specialised metabolite 2,4-diacetylphloroglucinol (DAPG), involved in phytopathogen suppression, in a variety of grassland soils. Subsequently, they used the biosensor platform to investigate how interactions within the Pseudomonas genus specifically affected the production of DAPG and induced or suppressed plant-beneficial activities in other rhizobacteria (Hansen et al., 2022). Similarly, Masarra et al. (2015) used a Chromobacterium violaceum mutant that produces the pigment violacein in response to quorum sensing molecules as a ‘native’ biosensor to screen a library of 63 soil-isolated Streptomyces isolates for metabolites with quorum quenching activity. Finally, Chane et al. (2019) developed a biosensor to monitor quorum signal molecule detection or degradation in the Actinobacteria biocontrol Rhodococcus erythropolis in response to co-inoculation onto potato tubers with the soft rot pathogen Pectobacterium atrosepticum.
Biosensors can also be incorporated within a plant host to report on host detection of a MBCA or its metabolites. For example, in a study by Vergnes et al. (2020) a GUS reporter under the control of the Arabidopsis Pathogenesis-related1 (PR1) promoter was used to screen for Streptomyces species that could stimulate defence signalling pathways in transgenic Arabidopsis carrying the reporter. They found one Streptomyces strain (AgN23) that triggered jasmonic acid and salicylic acid dependent responses. In a separate study, Belt et al. (2021) described the use of a luciferase reporter under the control of the stress-responsive Glutathione S-transferase-7 (GSTF7) promoter, to screen a collection of Actinobacteria to identify candidates that could activate plant stress-responses. In this study, a Streptomyces strain (KB001) and its fermentation extract were identified, which helped protect Arabidopsis plants against two fungal pathogens, Sclerotinia sclerotiorum and Rhizoctonia solani. Similarly, a PR1:GUS gene reporter was used to verify the activation of salicylic acid signalling pathway in transgenic Arabidopsis plants using fermentation broth of Streptomyces strain JCK-6131 (Le et al., 2021). These studies reinforce the importance of studying MBCAs in a tripartite system, where there efficacy involves priming plant immunity, which is overlooked in simple in vitro systems.
These studies highlight how biosensors incorporated into plant hosts or microbes are important tools to study MBCAs in planta or the activity of their secreted specialised metabolites, and how they could be tailored towards real-time spatial or even cellular detection of biological responses. Much like the nascent technique of imaging mass spectrometry, the use of reporter lines in planta has the potential to provide information on how MBCA strains behave in the presence of the plant host and the wider microbiome, including many of the unanswered questions posed in Figure 3.
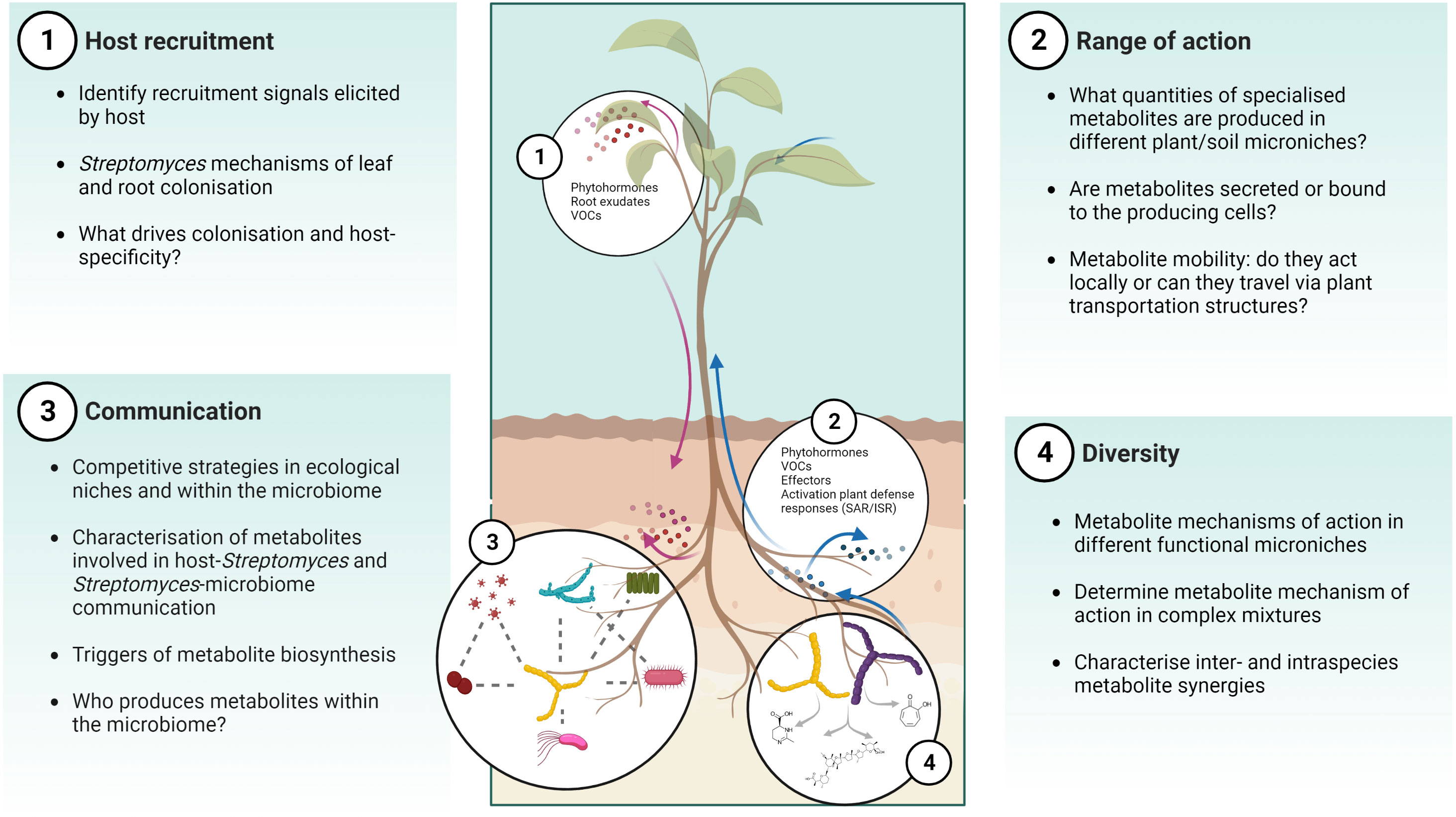
Figure 3 Challenges and opportunities for MBCA specialised metabolite identification and characterisation in vivo. There are several gaps in our understanding of the ecological function of Streptomyces and their specialised metabolites in the soil-plant environment, including host recruitment, range of action, communication, and diversity. Created with Biorender.
Outlook
The prevalence of Streptomyces spp. across soil environments and the relationships they form with plant hosts has attracted interest in their exploitation for crop production. As detailed within this review, they have a long history as MBCAs but their discovery and deployment in agricultural settings has been hindered by ill-suited or narrow-focused in vitro bioactivity screens, and the underexplored investigation of their metabolic potential in planta. Future work should address questions such as how, what, when, and where Streptomyces BGCs are expressed, how do we detect and isolate specialised metabolites, describe their functions in an ecological context, and determine which are most suitable for biocontrol applications (Figure 3).
To provide the best outcomes for Streptomyces biocontrol agents, future research should prioritise interactions involving Streptomyces, the plant host, a target pathogen, and where feasible, assess these dynamic interactions within the context of the host-associated microbiome. Focusing on this tripartite system would also expedite effective MBCA practices, such as their sensitivity to synthetic fungicides (Palazzini et al., 2018; Wachowska et al., 2020). Furthermore, while this review has primarily discussed specialised metabolites originating from Streptomyces species, modern analyses of tripartite systems are beginning to highlight how pathogens also alter microbiome structure and the plant-derived metabolome (Seybold et al., 2020; Dove et al., 2022; Jiang et al., 2023).
Despite advances in microbial metabolite identification and characterisation, there are still many challenges to their evaluation in planta. New omics tools such as metatranscriptomics and metametabolomics coupled with genetics tools such as gene knockout or gene editing studies provide a pathway to the discovery and dissection of MBCA modes of action in planta, of their specialised metabolites and other bioactive compounds, and the interplay between MBCAs and their host and microbiome (Figure 2). In planta mass spectrometry imaging could be a vital tool to uncover a plethora of novel specialised metabolites and better understand the host-Streptomyces-microbiome environment in real-time, and biosensors are promising tools tailored towards studying single metabolites in plant tissues.
Harnessing this new knowledge allows us to identify gaps in MBCA deployment and provides scope to build durability into agricultural systems. Synthetic microbial communities (SynComs), which are small microbial consortia with multiple beneficial microbial traits, could be used to tailor the design of MBCAs (de Souza et al., 2020; Verma et al., 2022). Incorporating the substantial metabolic potential from Streptomyces adapted to different environments and microniches could also improve durability. Beyond Streptomyces, other species of the phylum Actinobacteria may also offer new functionality with studies isolating rare Actinobacterial strains from root endospheres at higher frequency than rhizospheres or bulk soil (Matsumoto and Takahashi, 2017). Finally, metagenomic tools facilitate the identification of non-culturable Streptomyces and other biocontrols, paving the way for the analysis of their specialised metabolite repertoire, their functions, and the signals that elicit their expression in planta.
Author contributions
LD, MG, and LT conceived the review topic and outline. LD, MG, MR, SL, and LT wrote the review. All authors contributed to the article and approved the submitted version.
Funding
LD and SL are supported by the CSIRO Microbiomes for One Systems Health Future Science Platform and MR is supported by a CSIRO Research Office Postdoctoral Fellowship.
Acknowledgments
The authors thank Megan Outram for her helpful discussion.
Conflict of interest
The authors declare that the research was conducted in the absence of any commercial or financial relationships that could be construed as a potential conflict of interest.
Publisher’s note
All claims expressed in this article are solely those of the authors and do not necessarily represent those of their affiliated organizations, or those of the publisher, the editors and the reviewers. Any product that may be evaluated in this article, or claim that may be made by its manufacturer, is not guaranteed or endorsed by the publisher.
References
Adaskaveg, J. E., Förster, H., Wade, M. L. (2011). Effectiveness of kasugamycin against erwinia amylovora and its potential use for managing fire blight of pear. Plant Dis. 95 (4), 448–454. doi: 10.1094/pdis-09-10-0679
Agbessi, S., Beauséjour, J., Déry, C., Beaulieu, C. (2003). Antagonistic properties of two recombinant strains of streptomyces melanosporofaciens obtained by intraspecific protoplast fusion. Appl. Microbiol. Biotechnol. 62 (2-3), 233–238. doi: 10.1007/s00253-003-1256-0
Alam, K., Mazumder, A., Sikdar, S., Zhao, Y.-M., Hao, J., Song, C., et al. (2022). Streptomyces: the biofactory of secondary metabolites. Front. Microbiol. 13. doi: 10.3389/fmicb.2022.968053
Aleti, G., Nikolić, B., Brader, G., Pandey, R. V., Antonielli, L., Pfeiffer, S., et al. (2017). Secondary metabolite genes encoded by potato rhizosphere microbiomes in the Andean highlands are diverse and vary with sampling site and vegetation stage. Sci. Rep. 7 (1), 2330. doi: 10.1038/s41598-017-02314-x
Anderson, C. L., Fernando, S. C. (2021). Insights into rumen microbial biosynthetic gene cluster diversity through genome-resolved metagenomics. Commun. Biol. 4 (1). doi: 10.1038/s42003-021-02331-7
Arnison, P. G., Bibb, M. J., Bierbaum, G., Bowers, A. A., Bugni, T. S., Bulaj, G., et al. (2013). Ribosomally synthesized and post-translationally modified peptide natural products: overview and recommendations for a universal nomenclature. Nat. Prod. Rep. 30 (1), 108–160. doi: 10.1039/c2np20085f
Bahkali, A. H., Elsheshtawi, M., Mousa, R. A., Elgorban, A. M., Alzarqaa, A. A. (2014). Biological control of sclerotinia sclerotiorum in beans with antagonistic microorganisms under greenhouse conditions. Res. Crops 15 (4), 884–892. doi: 10.5958/2348-7542.2014.01426.0
Bai, J.-L., Wang, H.-H., Zhang, J.-M., Wu, Q.-P., Mo, S.-P., He, Y.-L., et al. (2022). Postharvest quality maintenance of wax apple and guava fruits by use of a fermented broth of an ϵ-poly-l-lysine-producing streptomyces strain. PloS One 17 (3), e0265457. doi: 10.1371/journal.pone.0265457
Bakker, M. G., Schlatter, D. C., Otto-Hanson, L., Kinkel, L. L. (2014). Diffuse symbioses: roles of plant-plant, plant-microbe and microbe-microbe interactions in structuring the soil microbiome. Mol. Ecol. 23 (6), 1571–1583. doi: 10.1111/mec.12571
Barka, E. A., Vatsa, P., Sanchez, L., Gaveau-Vaillant, N., Jacquard, C., Klenk, H.-P., et al. (2016). Taxonomy, physiology, and natural products of actinobacteria. Microbiol. Mol. Biol. Rev. 80 (1), 1–43. doi: 10.1128/MMBR.00019-15
Barratt, B. I. P., Moran, V. C., Bigler, F., Van Lenteren, J. C. (2018). The status of biological control and recommendations for improving uptake for the future. BioControl 63 (1), 155–167. doi: 10.1007/s10526-017-9831-y
Becklund, K., Powers, J., Kinkel, L. (2016). Tree species effects on pathogen-suppressive capacities of soil bacteria across two tropical dry forests in Costa Rica. Oecologia 182 (3), 789–802. doi: 10.1007/s00442-016-3702-6
Belt, K., Foley, R. C., O’Sullivan, C. A., Roper, M. M., Singh, K. B., Thatcher, L. F. (2021). A plant stress-responsive bioreporter coupled with transcriptomic analysis allows rapid screening for biocontrols of necrotrophic fungal pathogens. Front. Mol. Biosci. 8. doi: 10.3389/fmolb.2021.708530
Besset-Manzoni, Y., Joly, P., Brutel, A., Gerin, F., Soudière, O., Langin, T., et al. (2019). Does in vitro selection of biocontrol agents guarantee success in planta? a study case of wheat protection against fusarium seedling blight by soil bacteria. PloS One 14 (12), e0225655. doi: 10.1371/journal.pone.0225655
Bian, C., Duan, Y., Xiu, Q., Wang, J., Tao, X., Zhou, M. (2021). Mechanism of validamycin a inhibiting DON biosynthesis and synergizing with DMI fungicides against Fusarium graminearum. Mol. Plant Pathol. 22 (7), 769–785. doi: 10.1111/mpp.13060
Blake, C., Christensen, M. N., Kovács, Á. T. (2021). Molecular aspects of plant growth promotion and protection by bacillus subtilis. Mol. Plant-Microbe Interactions® 34 (1), 15–25. doi: 10.1094/mpmi-08-20-0225-cr
Blin, K., Shaw, S., Kloosterman, A. M., Charlop-Powers, Z., van Wezel, G. P., Medema, M. H., et al. (2021). antiSMASH 6.0: improving cluster detection and comparison capabilities. Nucleic Acids Res. 49 (W1), W29–W35. doi: 10.1093/nar/gkab335
Bordoloi, G. N., Kumari, B., Guha, A., Thakur, D., Bordoloi, M., Roy, M. K., et al. (2002). Potential of a novel antibiotic, 2-methylheptyl isonicotinate, as a biocontrol agent against fusarial wilt of crucifers. Pest Manage. Sci. 58 (3), 297–302. doi: 10.1002/ps.457
Boro, M., Sannyasi, S., Chettri, D., Verma, A. K. (2022). Microorganisms in biological control strategies to manage microbial plant pathogens: a review. Arch. Microbiol. 204 (11). doi: 10.1007/s00203-022-03279-w
Boukaew, S., Cheirsilp, B., Prasertsan, P., Yossan, S. (2021). Antifungal effect of volatile organic compounds produced by streptomyces salmonis PSRDC-09 against anthracnose pathogen colletotrichum gloeosporioides PSU-03 in postharvest chili fruit. J. Appl. Microbiol. 131 (3), 1452–1463. doi: 10.1111/jam.15037
Brader, G., Compant, S., Mitter, B., Trognitz, F., Sessitsch, A. (2014). Metabolic potential of endophytic bacteria. Curr. Opin. Biotechnol. 27, 30–37. doi: 10.1016/j.copbio.2013.09.012
Bulgarelli, D., Rott, M., Schlaeppi, K., Ver Loren Van Themaat, E., Ahmadinejad, N., Assenza, F., et al. (2012). Revealing structure and assembly cues for arabidopsis root-inhabiting bacterial microbiota. Nature 488 (7409), 91–95. doi: 10.1038/nature11336
Bulgarelli, D., Schlaeppi, K., Spaepen, S., Van Themaat, E. V. L., Schulze-Lefert, P. (2013). Structure and functions of the bacterial microbiota of plants. Annu. Rev. Plant Biol. 64 (1), 807–838. doi: 10.1146/annurev-arplant-050312-120106
Carrión, V. J., Perez-Jaramillo, J., Cordovez, V., Tracanna, V., De Hollander, M., Ruiz-Buck, D., et al. (2019). Pathogen-induced activation of disease-suppressive functions in the endophytic root microbiome. Science 366 (6465), 606–612. doi: 10.1126/science.aaw9285
Celler, K., Picioreanu, C., Van Loosdrecht, M. C. M., Van Wezel, G. P. (2012). Structured morphological modeling as a framework for rational strain design of streptomyces species. Antonie van Leeuwenhoek 102 (3), 409–423. doi: 10.1007/s10482-012-9760-9
Cha, J.-Y., Han, S., Hong, H.-J., Cho, H., Kim, D., Kwon, Y., et al. (2016). Microbial and biochemical basis of a fusarium wilt-suppressive soil. ISME J. 10 (1), 119–129. doi: 10.1038/ismej.2015.95
Chane, A., Barbey, C., Robert, M., Merieau, A., Konto-Ghiorghi, Y., Beury-Cirou, A., et al. (2019). Biocontrol of soft rot: confocal microscopy highlights virulent pectobacterial communication and its jamming by rhodococcal quorum-quenching. Mol. Plant-Microbe Interactions® 32 (7), 802–812. doi: 10.1094/mpmi-11-18-0314-r
Chen, Y., Wang, J., Yang, N., Wen, Z., Sun, X., Chai, Y., et al. (2018). Wheat microbiome bacteria can reduce virulence of a plant pathogenic fungus by altering histone acetylation. Nat. Commun. 9 (1), 3429. doi: 10.1038/s41467-018-05683-7
Collinge, D. B., Jensen, D. F., Rabiey, M., Sarrocco, S., Shaw, M. W., Shaw, R. H. (2022). Biological control of plant diseases – what has been achieved and what is the direction? Plant Pathol. 71 (5), 1024–1047. doi: 10.1111/ppa.13555
Coombs, J. T., Franco, C. M. M. (2003). Isolation and identification of actinobacteria from surface-sterilized wheat roots. Appl. Environ. Microbiol. 69 (9), 5603–5608. doi: 10.1128/AEM.69.9.5603-5608.2003
Cordovez, V., Carrion, V. J., Etalo, D. W., Mumm, R., Zhu, H., van Wezel, G. P., et al. (2015). Diversity and functions of volatile organic compounds produced by streptomyces from a disease-suppressive soil. Front. Microbiol. 6. doi: 10.3389/fmicb.2015.01081
Crane, J. M., Gibson, D. M., Vaughan, R. H., Bergstrom, G. C. (2013). Iturin levels on wheat spikes linked to biological control of fusarium head blight by Bacillus amyloliquefaciens. Phytopathology® 103 (2), 146–155. doi: 10.1094/phyto-07-12-0154-r
Crits-Christoph, A., Diamond, S., Al-Shayeb, B., Valentin-Alvarado, L., Banfield, J. F. (2022). A widely distributed genus of soil acidobacteria genomically enriched in biosynthetic gene clusters. ISME Commun. 2 (1), 70. doi: 10.1038/s43705-022-00140-5
Crits-Christoph, A., Diamond, S., Butterfield, C. N., Thomas, B. C., Banfield, J. F. (2018). Novel soil bacteria possess diverse genes for secondary metabolite biosynthesis. Nature 558 (7710), 440–444. doi: 10.1038/s41586-018-0207-y
de Souza, R. S. C., Armanhi, J. S. L., Arruda, P. (2020). From microbiome to traits: designing synthetic microbial communities for improved crop resiliency. Front. Plant Sci. 11. doi: 10.3389/fpls.2020.01179
Dobson, L. F., O’Cleirigh, C. C., O’Shea, D. G. (2008). The influence of morphology on geldanamycin production in submerged fermentations of streptomyces hygroscopicus var. geldanus. Appl. Microbiol. Biotechnol. 79 (5), 859–866. doi: 10.1007/s00253-008-1493-3
Donald, L., Pipite, A., Subramani, R., Owen, J., Keyzers, R. A., Taufa, T. (2022). Streptomyces: still the biggest producer of new natural secondary metabolites, a current perspective. Microbiol. Res. 13 (3), 418–465. doi: 10.3390/microbiolres13030031
Dove, N. C., Carrell, A. A., Engle, N. L., Klingeman, D. M., Rodriguez, M., Wahl, T., et al. (2022). Relationships between sphaerulina musiva infection and the populus microbiome and metabolome. mSystems 7 (4), e00120–e00122. doi: 10.1128/msystems.00120-22
Dror, B., Wang, Z., Brady, S. F., Jurkevitch, E., Cytryn, E. (2020). Elucidating the diversity and potential function of nonribosomal peptide and polyketide biosynthetic gene clusters in the root microbiome. mSystems 5 (6), e00866–e00820. doi: 10.1128/mSystems.00866-20
Edlund, A., Yang, Y., Yooseph, S., He, X., Shi, W., McLean, J. S. (2018). Uncovering complex microbiome activities via metatranscriptomics during 24 hours of oral biofilm assembly and maturation. Microbiome 6 (1). doi: 10.1186/s40168-018-0591-4
Edwards, J., Johnson, C., Santos-Medellín, C., Lurie, E., Podishetty, N. K., Bhatnagar, S., et al. (2015). Structure, variation, and assembly of the root-associated microbiomes of rice. Proc. Natl. Acad. Sci. 112 (8), E911–E920. doi: 10.1073/pnas.1414592112
Fira, D., Dimkić, I., Berić, T., Lozo, J., Stanković, S. (2018). Biological control of plant pathogens by bacillus species. J. Biotechnol. 285, 44–55. doi: 10.1016/j.jbiotec.2018.07.044
Fitzpatrick, C. R., Copeland, J., Wang, P. W., Guttman, D. S., Kotanen, P. M., Johnson, M. T. J. (2018). Assembly and ecological function of the root microbiome across angiosperm plant species. Proc. Natl. Acad. Sci. 115 (6), E1157–E1165. doi: 10.1073/pnas.1717617115
Franco, C., Michelsen, P., Percy, N., Conn, V., Listiana, E., Moll, S., et al. (2007). Actinobacterial endophytes for improved crop performance. Australas. Plant Pathol. 36 (6), 524. doi: 10.1071/ap07067
Gavriilidou, A., Kautsar, S. A., Zaburannyi, N., Krug, D., Müller, R., Medema, M. H., et al. (2022). Compendium of specialized metabolite biosynthetic diversity encoded in bacterial genomes. Nat. Microbiol. 7 (5), 726–735. doi: 10.1038/s41564-022-01110-2
Gong, Y., Liu, J.-Q., Xu, M.-J., Zhang, C.-M., Gao, J., Li, C.-G., et al. (2022). Antifungal volatile organic compounds from streptomyces setonii WY228 control black spot disease of sweet potato. Appl. Environ. Microbiol. 88 (6), e02317–e02321. doi: 10.1128/aem.02317-21
Goodfellow, M., Williams, S. T. (1983). Ecology of actinomycetes. Annu. Rev. Microbiol. 37 (1), 189–216. doi: 10.1146/annurev.mi.37.100183.001201
Guo, F., Xiang, S., Li, L., Wang, B., Rajasärkkä, J., Gröndahl-Yli-Hannuksela, K., et al. (2015). Targeted activation of silent natural product biosynthesis pathways by reporter-guided mutant selection. Metab. Eng. 28, 134–142. doi: 10.1016/j.ymben.2014.12.006
Hansen, M. L., He, Z., Wibowo, M., Jelsbak, L. (2021). A whole-cell biosensor for detection of 2,4-diacetylphloroglucinol (DAPG)-producing bacteria from grassland soil. Appl. Environ. Microbiol. 87 (3), e01400–e01420. doi: 10.1128/AEM.01400-20
Hansen, M. L., Wibowo, M., Jarmusch, S. A., Larsen, T. O., Jelsbak, L. (2022). Sequential interspecies interactions affect production of antimicrobial secondary metabolites in pseudomonas protegens DTU9.1. ISME J. 16 (12), 2680–2690. doi: 10.1038/s41396-022-01322-8
Hemphill, C. F. P., Sureechatchaiyan, P., Kassack, M. U., Orfali, R. S., Lin, W., Daletos, G., et al. (2017). OSMAC approach leads to new fusarielin metabolites from fusarium tricinctum. J. Antibiotics 70 (6), 726–732. doi: 10.1038/ja.2017.21
Hoskisson, P. A., Fernández-Martínez, L. T. (2018). Regulation of specialised metabolites in actinobacteria - expanding the paradigms. Environ. Microbiol. Rep. 10 (3), 231–238. doi: 10.1111/1758-2229.12629
Huang, K. T., Misato, T., Asuyama, H. (1964). Effect of blasticidin s on protein synthesis of piricularia oryzae. J. Antibiotics Ser. A 17 (2), 65–70. doi: 10.11554/antibioticsa.17.2_65
Hwang, B. K., Lim, S. W., Kim, B. S., Lee, J. Y., Moon, S. S. (2001). Isolation and in vivo and in vitro antifungal activity of phenylacetic acid and sodium phenylacetate from streptomyces humidus. Appl. Environ. Microbiol. 67 (8), 3739–3745. doi: 10.1128/AEM.67.8.3739-3745.2001
Iwasa, T., Yamamoto, H., Shibata, M. (1970). Studies on validamycins, new antibiotics. I. J. Antibiotics 23 (12), 595–602. doi: 10.7164/antibiotics.23.595
Jiang, H., Xu, X., Fang, Y., Ogunyemi, S. O., Ahmed, T., Li, X., et al. (2023). Metabarcoding reveals response of rice rhizosphere bacterial community to rice bacterial leaf blight. Microbiol. Res. 270, 127344. doi: 10.1016/j.micres.2023.127344
Kaari, M., Joseph, J., Manikkam, R., Sreenivasan, A., Venugopal, G. (2022). Biological control of streptomyces sp. UT4A49 to suppress tomato bacterial wilt disease and its metabolite profiling. J. King Saud Univ. - Sci. 34 (1), 101688. doi: 10.1016/j.jksus.2021.101688
Kang, S., Lumactud, R., Li, N., Bell, T. H., Kim, H.-S., Park, S.-Y., et al. (2021). Harnessing chemical ecology for environment-friendly crop protection. Phytopathology® 111 (10), 1697–1710. doi: 10.1094/phyto-01-21-0035-rvw
Kasey, C. M., Zerrad, M., Li, Y., Cropp, T. A., Williams, G. J. (2018). Development of transcription factor-based designer macrolide biosensors for metabolic engineering and synthetic biology. ACS Synthetic Biol. 7 (1), 227–239. doi: 10.1021/acssynbio.7b00287
Kaur, T., Kaur, A., Sharma, V., Manhas, R. K. (2016). Purification and characterization of a new antifungal compound 10-(2,2-dimethyl-cyclohexyl)-6,9-dihydroxy-4,9-dimethyl-dec-2-enoic acid methyl ester from streptomyces hydrogenans strain DH16. Front. Microbiol. 7. doi: 10.3389/fmicb.2016.01004
Kautsar, S. A., Blin, K., Shaw, S., Weber, T., Medema, M. H. (2020). BiG-FAM: the biosynthetic gene cluster families database. Nucleic Acids Res. 49 (D1), D490–D497. doi: 10.1093/nar/gkaa812
Kavitha, A., Prabhakar, P., Vijayalakshmi, M., Venkateswarlu, Y. (2010). Purification and biological evaluation of the metabolites produced by streptomyces sp. TK-VL_333. Res. Microbiol. 161 (5), 335–345. doi: 10.1016/j.resmic.2010.03.011
Kim, J. D., Han, J. W., Hwang, I. C., Lee, D., Kim, B. S. (2012). Identification and biocontrol efficacy of streptomyces miharaensis producing filipin III against fusarium wilt. J. Basic Microbiol. 52 (2), 150–159. doi: 10.1002/jobm.201100134
Kim, J. D., Kang, J. E., Kim, B. S. (2020). Postharvest disease control efficacy of the polyene macrolide lucensomycin produced by streptomyces plumbeus strain CA5 against gray mold on grapes. Postharvest Biol. Technol. 162, 111115. doi: 10.1016/j.postharvbio.2019.111115
Kim, B. S., Moon, S. S., Hwang, B. K. (1999). Isolation, identification, and antifungal activity of a macrolide antibiotic, oligomycin a, produced by streptomyces libani. Can. J. Bot. 77 (6), 850–858. doi: 10.1139/b99-044
Kim, B. S., Moon, S. S., Hwang, B. K. (2000). Structure elucidation and antifungal activity of an anthracycline antibiotic, daunomycin, isolated from actinomadura oseola. J. Agric. Food Chem. 48 (5), 1875–1881. doi: 10.1021/jf990402u
Kim, J. D., Park, M. Y., Jeon, B. J., Kim, B. S. (2019). Disease control efficacy of 32,33-didehydroroflamycoin produced by streptomyces rectiviolaceus strain DY46 against gray mold of tomato fruit. Sci. Rep. 9 (1), 13533. doi: 10.1038/s41598-019-49779-6
Köhl, J., Kolnaar, R., Ravensberg, W. J. (2019). Mode of action of microbial biological control agents against plant diseases: relevance beyond efficacy. Front. Plant Sci. 10. doi: 10.3389/fpls.2019.00845
Korpi, A., Järnberg, J., Pasanen, A.-L. (2009). Microbial volatile organic compounds. Crit. Rev. Toxicol. 39 (2), 139–193. doi: 10.1080/10408440802291497
Lacey, H. J., Rutledge, P. J. (2022). Recently dscovered secondary metabolites from streptomyces species. Molecules 27 (3), 887. doi: 10.3390/molecules27030887
Le, K. D., Kim, J., Nguyen, H. T., Yu, N. H., Park, A. R., Lee, C. W., et al. (2021). Streptomyces sp. JCK-6131 protects plants against bacterial and fungal diseases via two mechanisms. Front. Plant Sci. 12. doi: 10.3389/fpls.2021.726266
Leben, C., Keitt, G. W. (1954). Antibiotics and plant disease, effects of antibiotics in control of plant diseases. J. Agric. Food Chem. 2 (5), 234–239. doi: 10.1021/jf60025a003
Leveau, J. H. J., Lindow, S. E. (2002). Bioreporters in microbial ecology. Curr. Opin. Microbiol. 5 (3), 259–265. doi: 10.1016/S1369-5274(02)00321-1
Li, L., Liu, X., Jiang, W., Lu, Y. (2019). Recent advances in synthetic biology approaches to optimize production of bioactive natural products in actinobacteria. Front. Microbiol. 10. doi: 10.3389/fmicb.2019.02467
Li, Q., Ning, P., Zheng, L., Huang, J., Li, G., Hsiang, T. (2010). Fumigant activity of volatiles of streptomyces globisporus JK-1 against penicillium italicum on citrus microcarpa. Postharvest Biol. Technol. 58 (2), 157–165. doi: 10.1016/j.postharvbio.2010.06.003
Liu, D., Evans, T., Zhang, F. (2015). Applications and advances of metabolite biosensors for metabolic engineering. Metab. Eng. 31, 35–43. doi: 10.1016/j.ymben.2015.06.008
Lyu, A., Liu, H., Che, H., Yang, L., Zhang, J., Wu, M., et al. (2017). Reveromycins a and b from streptomyces sp. 3–10: antifungal activity against plant pathogenic fungi in vitro and in a strawberry food model system. Front. Microbiol. 8. doi: 10.3389/fmicb.2017.00550
Lyu, A., Yang, L., Wu, M., Zhang, J., Li, G. (2020). High efficacy of the volatile organic compounds of streptomyces yanglinensis 3-10 in suppression of aspergillus contamination on peanut kernels. Front. Microbiol. 11. doi: 10.3389/fmicb.2020.00142
Masarra, M. S., Khaled, M. A. A., Mohammad, M. A., Nadia, A. H. H. (2015). Characterization of the quorum quenching activity of streptomyces minutiscleroticus: a new approach for infection control. Afr. J. Microbiol. Res. 9 (8), 492–502. doi: 10.5897/ajmr2014.7316
Matsumoto, A., Takahashi, Y. (2017). Endophytic actinomycetes: promising source of novel bioactive compounds. J. Antibiotics 70 (5), 514–519. doi: 10.1038/ja.2017.20
Medema, M. H., Kottmann, R., Yilmaz, P., Cummings, M., Biggins, J. B., Blin, K., et al. (2015). Minimum information about a biosynthetic gene cluster. Nat. Chem. Biol. 11 (9), 625–631. doi: 10.1038/nchembio.1890
Mendes, R., Kruijt, M., de Bruijn, I., Dekkers, E., van der Voort, M., Schneider, J. H. M., et al. (2011). Deciphering the rhizosphere microbiome for disease-suppressive bacteria. Science 332 (6033), 1097–1100. doi: 10.1126/science.1203980
Meredith, L. K., Tfaily, M. M. (2022). Capturing the microbial volatilome: an oft overlooked ‘ome’. Trends Microbiol. 30 (7), 622–631. doi: 10.1016/j.tim.2021.12.004
Meschke, H., Schrempf, H. (2010). Streptomyces lividans inhibits the proliferation of the fungus verticillium dahliae on seeds and roots of Arabidopsis thaliana. Microb. Biotechnol. 3 (4), 428–443. doi: 10.1111/j.1751-7915.2010.00165.x
Meschke, H., Walter, S., Schrempf, H. (2012). Characterization and localization of prodiginines from streptomyces lividans suppressing Verticillium dahliae in the absence or presence of Arabidopsis thaliana. Environ. Microbiol. 14 (4), 940–952. doi: 10.1111/j.1462-2920.2011.02665.x
Minchev, Z., Kostenko, O., Soler, R., Pozo, M. J. (2021). Microbial consortia for effective biocontrol of root and foliar diseases in tomato. Front. Plant Sci. 12. doi: 10.3389/fpls.2021.756368
Misato, T., Ishii, I., Asakawa, M., Okimoto, Y., Fukunaga, K. (1959). Antibiotics as protectant fungicides against rice blast. Japanese J. Phytopathol. 24 (5), 302–306. doi: 10.3186/jjphytopath.24.302
Moore, S. J., Lai, H.-E., Li, J., Freemont, P. S. (2023). Streptomyces cell-free systems for natural product discovery and engineering. Natural Product Rep. 40(2), 228–236. doi: 10.1039/D2NP00057A
Mukherjee, P. K., Horwitz, B. A., Herrera-Estrella, A., Schmoll, M., Kenerley, C. M. (2013). Trichoderma research in the genome era. Annu. Rev. Phytopathol. 51 (1), 105–129. doi: 10.1146/annurev-phyto-082712-102353
Mukhopadhyay, R., Kumar, D. (2020). Trichoderma: a beneficial antifungal agent and insights into its mechanism of biocontrol potential. Egyptian J. Biol. Pest Control 30 (1), 133. doi: 10.1186/s41938-020-00333-x
Nair, M. G., Chandra, A., Thorogood, D. L., Ammermann, E., Walker, N., Kiehs, K. (1994). Gopalamicin, an antifungal macrodiolide produced by soil actinomycetes. J. Agric. Food Chem. 42 (10), 2308–2310. doi: 10.1021/jf00046a043
Nazari, B., Kobayashi, M., Saito, A., Hassaninasab, A., Miyashita, K., Fujii, T. (2013). Chitin-induced gene expression in secondary metabolic pathways of streptomyces coelicolor A3(2) grown in soil. Appl. Environ. Microbiol. 79 (2), 707–713. doi: 10.1128/AEM.02217-12
Nessner Kavamura, V., Taketani, R. G., Lançoni, M. D., Andreote, F. D., Mendes, R., Soares De Melo, I. (2013). Water regime influences bulk soil and rhizosphere of cereus jamacaru bacterial communities in the Brazilian caatinga biome. PloS One 8 (9), e73606. doi: 10.1371/journal.pone.0073606
Newitt, J., Prudence, S., Hutchings, M., Worsley, S. (2019). Biocontrol of cereal crop diseases using Streptomycetes. Pathogens 8 (2), 78. doi: 10.3390/pathogens8020078
Nguyen, H. T. T., Park, A. R., Hwang, I. M., Kim, J.-C. (2021). Identification and delineation of action mechanism of antifungal agents: reveromycin e and its new derivative isolated from streptomyces sp. JCK-6141. Postharvest Biol. Technol. 182, 111700. doi: 10.1016/j.postharvbio.2021.111700
Omura, S., Tanaka, Y., Takahashi, Y., Chia, I., Inoue, M., Iwai, Y. (1984). Irumamycin, an antifungal 20-membered macrolide produced by a streptomyces. taxonomy, fermentation and biological properties. J. Antibiotics 37 (12), 1572–1578. doi: 10.7164/antibiotics.37.1572
Onaka, H. (2017). Novel antibiotic screening methods to awaken silent or cryptic secondary metabolic pathways in actinomycetes. J. Antibiotics 70 (8), 865–870. doi: 10.1038/ja.2017.51
Onaka, H., Tabata, H., Igarashi, Y., Sato, Y., Furumai, T. (2001). Goadsporin, a chemical substance which promotes secondary metabolism and morphogenesis in streptomycetes. i. purification and characterization. J. Antibiotics 54 (12), 1036–1044. doi: 10.7164/antibiotics.54.1036
Ostash, B., Ostash, I., Zhu, L., Kharel, M. K., Luzhetskyy, A., Bechthold, A., et al. (2010). Properties of lanK-based regulatory circuit involved in landomycin biosynthesis in streptomyces cyanogenus S136. Russian J. Genet. 46 (5), 530–535. doi: 10.1134/s1022795410050030
O’Sullivan, C. A., Belt, K., Thatcher, L. F. (2021a). Tackling control of a cosmopolitan phytopathogen: sclerotinia. Front. Plant Sci. 12. doi: 10.3389/fpls.2021.707509
O’Sullivan, C. A., Roper, M. M., Myers, C. A., Thatcher, L. F. (2021b). Developing actinobacterial endophytes as biocontrol products for fusarium pseudograminearum in wheat. Front. Bioeng. Biotechnol. 9. doi: 10.3389/fbioe.2021.691770
Palazzini, J. M., Alberione, E., Torres, A., Donat, C., Köhl, J., Chulze, S. (2016). Biological control of fusarium graminearum sensu stricto, causal agent of fusarium head blight of wheat, using formulated antagonists under field conditions in Argentina. Biol. Control 94, 56–61. doi: 10.1016/j.biocontrol.2015.12.009
Palazzini, J. M., Ramirez, M. L., Torres, A. M., Chulze, S. N. (2007). Potential biocontrol agents for fusarium head blight and deoxynivalenol production in wheat. Crop Prot. 26 (11), 1702–1710. doi: 10.1016/j.cropro.2007.03.004
Palazzini, J. M., Torres, A. M., Chulze, S. N. (2018). Tolerance of triazole-based fungicides by biocontrol agents used to control fusarium head blight in wheat in Argentina. Lett. Appl. Microbiol. 66 (5), 434–438. doi: 10.1111/lam.12869
Palumbo, J. D., O’Keeffe, T. L., Abbas, H. K. (2008). Microbial interactions with mycotoxigenic fungi and mycotoxins. Toxin Rev. 27 (3-4), 261–285. doi: 10.1080/15569540802416301
Pan, R., Bai, X., Chen, J., Zhang, H., Wang, H. (2019). Exploring structural diversity of microbe secondary metabolites using OSMAC strategy: a literature review. Front. Microbiol. 10. doi: 10.3389/fmicb.2019.00294
Park, E.-J., Jang, H.-J., Park, J.-Y., Yang, Y. K., Kim, M. J., Park, C. S., et al. (2023). Efficacy evaluation of streptomyces nigrescens KA-1 against the root-knot nematode meloidogyne incognita. Biol. Control 179, 105150. doi: 10.1016/j.biocontrol.2023.105150
Pérez, J., Muñoz-Dorado, J., Braña, A. F., Shimkets, L. J., Sevillano, L., Santamaría, R. I. (2011). Myxococcus xanthus induces actinorhodin overproduction and aerial mycelium formation by streptomyces coelicolor. Microb. Biotechnol. 4 (2), 175–183. doi: 10.1111/j.1751-7915.2010.00208.x
Prabavathy, V. R., Mathivanan, N., Murugesan, K. (2006). Control of blast and sheath blight diseases of rice using antifungal metabolites produced by streptomyces sp. PM5. Biol. Control 39 (3), 313–319. doi: 10.1016/j.biocontrol.2006.07.011
Prudence, S. M., Newitt, J. T., Worsley, S. F., Macey, M. C., Murrell, J. C., Lehtovirta-Morley, L. E., et al. (2021). Soil, senescence and exudate utilisation: characterisation of the paragon var. spring bread wheat root microbiome. Environ. Microbiome 16 (1), 12. doi: 10.1186/s40793-021-00381-2
Qi, H., Jiang, X., Ding, Y., Liu, T., Yang, Q. (2021). Discovery of kasugamycin as a potent inhibitor of glycoside hydrolase family 18 chitinases. Front. Mol. Biosci. 8. doi: 10.3389/fmolb.2021.640356
Rashad, F. M., Fathy, H. M., El-Zayat, A. S., Elghonaimy, A. M. (2015). Isolation and characterization of multifunctional streptomyces species with antimicrobial, nematicidal and phytohormone activities from marine environments in Egypt. Microbiol. Res. 175, 34–47. doi: 10.1016/j.micres.2015.03.002
Rateb, M. E., Houssen, W. E., Harrison, W. T. A., Deng, H., Okoro, C. K., Asenjo, J. A., et al. (2011). Diverse metabolic profiles of a streptomyces strain isolated from a hyper-arid environment. J. Natural Products 74 (9), 1965–1971. doi: 10.1021/np200470u
Rey, T., Dumas, B. (2017). Plenty is no plague: streptomyces symbiosis with crops. Trends Plant Sci. 22 (1), 30–37. doi: 10.1016/j.tplants.2016.10.008
Rothrock, C. S., Gottlieb, D. (1984). Role of antibiosis in antagonism of streptomyces hygroscopicus var. geldanus to rhizoctonia solani in soil. Can. J. Microbiol. 30 (12), 1440–1447. doi: 10.1139/m84-230
Rutledge, P. J., Challis, G. L. (2015). Discovery of microbial natural products by activation of silent biosynthetic gene clusters. Nat. Rev. Microbiol. 13 (8), 509–523. doi: 10.1038/nrmicro3496
Sabaratnam, S., Traquair, J. A. (2015). Mechanism of antagonism by streptomyces griseocarneus (strain Di944) against fungal pathogens of greenhouse-grown tomato transplants. Can. J. Plant Pathol. 37 (2), 197–211. doi: 10.1080/07060661.2015.1039062
Santana-Pereira, A. L. R., Sandoval-Powers, M., Monsma, S., Zhou, J., Santos, S. R., Mead, D. A., et al. (2020). Discovery of novel biosynthetic gene cluster diversity from a soil metagenomic library. Front. Microbiol. 11. doi: 10.3389/fmicb.2020.585398
Seipke, R. F., Kaltenpoth, M., Hutchings, M. I. (2012). Streptomyces as symbionts: an emerging and widespread theme? FEMS Microbiol. Rev. 36 (4), 862–876. doi: 10.1111/j.1574-6976.2011.00313.x
Seybold, H., Demetrowitsch, T. J., Hassani, M. A., Szymczak, S., Reim, E., Haueisen, J., et al. (2020). A fungal pathogen induces systemic susceptibility and systemic shifts in wheat metabolome and microbiome composition. Nat. Commun. 11 (1), 1910. doi: 10.1038/s41467-020-15633-x
Shepherdson, E. M. F., Elliot, M. A. (2022). Cryptic specialized metabolites drive streptomyces exploration and provide a competitive advantage during growth with other microbes. Proc. Natl. Acad. Sci. 119 (40). doi: 10.1073/pnas.2211052119
Shi, L., Wu, Z., Zhang, Y., Zhang, Z., Fang, W., Wang, Y., et al. (2020). Herbicidal secondary metabolites from actinomycetes: structure diversity, modes of action, and their roles in the development of herbicides. J. Agric. Food Chem. 68 (1), 17–32. doi: 10.1021/acs.jafc.9b06126
Singer, E., Bonnette, J., Kenaley, S. C., Woyke, T., Juenger, T. E. (2019). Plant compartment and genetic variation drive microbiome composition in switchgrass roots. Environ. Microbiol. Rep. 11 (2), 185–195. doi: 10.1111/1758-2229.12727
Slack, S. M., Walters, K. J., Outwater, C. A., Sundin, G. W. (2021). Effect of kasugamycin, oxytetracycline, and streptomycin on in-orchard population dynamics of erwinia amylovora on apple flower stigmas. Plant Dis. 105 (6), 1843–1850. doi: 10.1094/pdis-07-20-1469-re
Sun, Y.-Q., Busche, T., Rückert, C., Paulus, C., Rebets, Y., Novakova, R., et al. (2017). Development of a biosensor concept to detect the production of cluster-specific secondary metabolites. ACS Synthetic Biol. 6 (6), 1026–1033. doi: 10.1021/acssynbio.6b00353
Taketani, R. G., Lançoni, M. D., Kavamura, V. N., Durrer, A., Andreote, F. D., Melo, I. S. (2017). Dry season constrains bacterial phylogenetic diversity in a semi-arid rhizosphere system. Microb. Ecol. 73 (1), 153–161. doi: 10.1007/s00248-016-0835-4
Trejo-Estrada, S. R., Paszczynski, A., Crawford, D. L. (1998a). Antibiotics and enzymes produced by the biocontrol agent streptomyces violaceusniger YCED-9. J. Ind. Microbiol. Biotechnol. 21 (1-2), 81–90. doi: 10.1038/sj.jim.2900549
Trejo-Estrada, S. R., Sepulveda, I. R., Crawford, D. L. (1998b). In vitro and in vivo antagonism of streptomyces violaceusniger YCED9 against fungal pathogens of turfgrass. World J. Microbiol. Biotechnol. 14 (6), 865–872. doi: 10.1023/A:1008877224089
Ueda, K., Kawai, S., Ogawa, H.-O., Kiyama, A., Kubota, T., Kawanobe, H., et al. (2000). Wide distribution of interspecific stimulatory events on antibiotic production and sporulation among streptomyces species. J. Antibiotics 53 (9), 979–982. doi: 10.7164/antibiotics.53.979
Umezawa, H., Okami, Y., Hashimoto, T., Suhara, Y., Hamada, M., Takeuchi, T. (1965). A new antibiotic, kasugamycin. J. Antibiot (Tokyo) 18, 101–103.
Van Bergeijk, D. A., Terlouw, B. R., Medema, M. H., Van Wezel, G. P. (2020). Ecology and genomics of actinobacteria: new concepts for natural product discovery. Nat. Rev. Microbiol. 18 (10), 546–558. doi: 10.1038/s41579-020-0379-y
van der Voort, M., Meijer, H., Schmidt, Y., Watrous, J., Dekkers, E., Mendes, R., et al. (2015). Genome mining and metabolic profiling of the rhizosphere bacterium pseudomonas sp. SH-C52 for antimicrobial compounds. Front. Microbiol. 6. doi: 10.3389/fmicb.2015.00693
Van Goethem, M. W., Osborn, A. R., Bowen, B. P., Andeer, P. F., Swenson, T. L., Clum, A., et al. (2021). Long-read metagenomics of soil communities reveals phylum-specific secondary metabolite dynamics. Commun. Biol. 4 (1), 1302. doi: 10.1038/s42003-021-02809-4
Vergnes, S., Gayrard, D., Veyssière, M., Toulotte, J., Martinez, Y., Dumont, V., et al. (2020). Phyllosphere colonization by a soil streptomyces sp. promotes plant defense responses against fungal infection. Mol. Plant-Microbe Interactions® 33 (2), 223–234. doi: 10.1094/mpmi-05-19-0142-r
Verma, H., Kant, C., Singh, S. K., White, J. F., Kumar, A., Droby, S. (2022). Biocontrol potential of microbial consortia: approaches in food security and disease management. Springer Int. Publishing, 187–203. doi: 10.1007/978-3-030-87512-1_7
Viaene, T., Langendries, S., Beirinckx, S., Maes, M., Goormachtig, S. (2016). Streptomyces as a plant’s best friend? FEMS Microbiol. Ecol. 92 (8). doi: 10.1093/femsec/fiw119
Wachowska, U., Kucharska, K., Pluskota, W., Czaplicki, S., Stuper-Szablewska, K. (2020). Bacteria associated with winter wheat degrade fusarium mycotoxins and triazole fungicide residues. Agronomy 10 (11), 1673. doi: 10.3390/agronomy10111673
Wang, B., Li, L., Lin, Y., Shen, D., Shao, X., Zhong, C., et al. (2022). Targeted isolation of biocontrol agents from plants through phytopathogen co-culture and pathogen enrichment. Phytopathol. Res. 4 (1), 19. doi: 10.1186/s42483-022-00124-2
Wang, L., Zhang, C., Zhang, J., Rao, Z., Xu, X., Mao, Z., et al. (2021). Epsilon-poly-L-lysine: recent advances in biomanufacturing and applications. Front. Bioeng. Biotechnol. 9. doi: 10.3389/fbioe.2021.748976
Watrous, J., Roach, P., Alexandrov, T., Heath, B. S., Yang, J. Y., Kersten, R. D., et al. (2012). Mass spectral molecular networking of living microbial colonies. Proc. Natl. Acad. Sci. 109 (26), E1743–E1752. doi: 10.1073/pnas.1203689109
Worsley, S. F., Macey, M. C., Prudence, S. M. M., Wilkinson, B., Murrell, J. C., Hutchings, M. I. (2021). Investigating the role of root exudates in recruiting streptomyces bacteria to the arabidopsis thaliana microbiome. Front. Mol. Biosci. 8. doi: 10.3389/fmolb.2021.686110
Worsley, S. F., Newitt, J., Rassbach, J., Batey, S. F. D., Holmes, N. A., Murrell, J. C., et al. (2020). Streptomyces endophytes promote host health and enhance growth across plant species. Appl. Environ. Microbiol. 86 (16), e01053–e01020. doi: 10.1128/AEM.01053-20
Wu, Z.-M., Yang, Y., Li, K.-T. (2019). Ntagonistic activity of a novel antifungalmycin N2 from streptomyces sp. N2 and its biocontrol efficacy against Rhizoctonia solani. FEMS Microbiol. Lett. 366 (3). doi: 10.1093/femsle/fnz018
Xu, B., Chen, W., Wu, Z.-M., Long, Y., Li, K.-T. (2015). A novel and effective streptomyces sp. n2 against various phytopathogenic fungi. Appl. Biochem. Biotechnol. 177 (6), 1338–1347. doi: 10.1007/s12010-015-1818-5
Yamanaka, K., Oikawa, H., Ogawa, H.-O., Hosono, K., Shinmachi, F., Takano, H., et al. (2005). Desferrioxamine e produced by streptomyces griseus stimulates growth and development of Streptomyces tanashiensis. Microbiology 151 (9), 2899–2905. doi: 10.1099/mic.0.28139-0
Yu, J., Liu, Q., Chen, C., Qi, X. (2017). Antifungal activity change of streptomyces rimosus MY02 mediated by confront culture with other microorganism. J. Basic Microbiol. 57 (3), 276–282. doi: 10.1002/jobm.201600498
Zhang, F., Keasling, J. (2011). Biosensors and their applications in microbial metabolic engineering. Trends Microbiol. 19 (7), 323–329. doi: 10.1016/j.tim.2011.05.003
Keywords: Streptomyces, biocontrol, crop protection, microbiome, specialised metabolites, Actinobacteria, natural products, biopesticide
Citation: Dow L, Gallart M, Ramarajan M, Law SR and Thatcher LF (2023) Streptomyces and their specialised metabolites for phytopathogen control – comparative in vitro and in planta metabolic approaches. Front. Plant Sci. 14:1151912. doi: 10.3389/fpls.2023.1151912
Received: 26 January 2023; Accepted: 30 May 2023;
Published: 14 June 2023.
Edited by:
Yasser Nehela, University of Florida, United StatesReviewed by:
Alessandro Passera, University of Milan, ItalyManoj Kumar Solanki, University of Silesia in Katowice, Poland
Manish Kumar Dubey, Chandigarh University, India
Copyright © 2023 Dow, Gallart, Ramarajan, Law and Thatcher. This is an open-access article distributed under the terms of the Creative Commons Attribution License (CC BY). The use, distribution or reproduction in other forums is permitted, provided the original author(s) and the copyright owner(s) are credited and that the original publication in this journal is cited, in accordance with accepted academic practice. No use, distribution or reproduction is permitted which does not comply with these terms.
*Correspondence: Louise F. Thatcher, TG91aXNlLlRoYXRjaGVyQGNzaXJvLmF1