- 1Guangdong Provincial Key Laboratory of Plant Adaptation and Molecular Design, Guangzhou Key Laboratory of Crop Gene Editing, Innovative Center of Molecular Genetics and Evolution, School of Life Sciences, Guangzhou University, Guangzhou, China
- 2Key Laboratory of Soybean Biology of Ministry of Education China, Northeast Agricultural University, Harbin, China
Soybean (Glycine max) is a major protein and oil crop. Soybean basic region/leucine zipper (bZIP) transcription factors are involved in many regulatory pathways, including yield, stress responses, environmental signaling, and carbon-nitrogen balance. Here, we discuss the members of the soybean bZIP family and their classification: 161 members have been identified and clustered into 13 groups. Our review of the transcriptional regulation and functions of soybean bZIP members provides important information for future study of bZIP transcription factors and genetic resources for soybean breeding.
Introduction
Transcription factors (TFs) can be grouped into different families according to their DNA-binding and multimerization domains. Basic region/leucine zipper (bZIP) TFs are characterized by a conserved bZIP domain composed of two motifs: a basic region responsible for binding to specific DNA sequences, and a leucine zipper motif required for dimerization (Hurst, 1995; Wingender et al., 2001; Jakoby et al., 2002). Plant bZIP TFs function in stress and hormone signaling, organ and tissue differentiation, photomorphogenesis, cell elongation, nitrogen/carbon balance, energy metabolism, flower development, seed development, pathogen defense, and gibberellin biosynthesis (Chern et al., 1996; Albani et al., 1997; Oyama et al., 1997; Fukazawa et al., 2000; Jakoby et al., 2002; Cluis et al., 2004; Corrêa et al., 2008; Weltmeier et al., 2009). The functions of plant bZIP proteins appear to be more complex and broader than those of other TFs (Wei et al., 2010).
Due to their crucial roles in numerous biological processes, bZIP TFs have been studied in many plant species: 78 bZIP genes have been identified in Arabidopsis thaliana (Dröge-Laser et al., 2018), 92 in rice (Oryza sativa) (Corrêa et al., 2008), 125 in maize (Zea mays) (Wei et al., 2012), 64 in cucumber (Cucumis sativus) (Yoshida et al., 2015a), and 69 in tomato (Solanum lycopersicum) (Li et al., 2015). Jakoby et al. (2002) classified Arabidopsis bZIP genes into 10 groups (A, B, C, D, E, F, G, H, I, and S) based on the similarity in the basic region and other conserved motifs. Dröge-Laser et al. (2018) reported four new members in Arabidopsis, AtbZIP76-AtbZIP79, and excluded AtbZIP73 as a pseudogene, and classified these 78 AtbZIPs into 13 groups (designated A-M). AtbZIPs are associated with a plethora of functions; most AtbZIPs in each group display group-specific properties (Jakoby et al., 2002; Dröge-Laser et al., 2018).
Soybean (Glycine max [L.] Merr) is an important food and industrial crop. Many bZIP genes have been found in soybean. Liao et al. (2008) identified 131 GmbZIP TFs and classified them into 10 groups (A, B, C, D, E, F, G, H, I, and S). Most GmbZIP proteins cluster with the AtbZIP proteins, whereas several GmbZIP members form a distinct S group. Wang et al. (2015) identified 138 GmbZIPs, and Zhang et al. (2018) identified and classified 160 GmbZIPs into 12 groups (A, B, C, D, E, F, G, H, I, J, K, and S). In soybean, 124 and 122 out of 160 GmbZIPs are involved in drought and flooding responses, respectively (Zhang et al., 2018), however, many GmbZIPs have been implicated in various biological processes besides abiotic stress responses. In this review, we analyze the cladistics and expression profiles of GmbZIP and AtbZIP genes, and focus on the well-studied GmbZIP genes, in order to summarize and predict the functions of GmbZIP TFs, and to provide perspectives for their further identification and use in soybean breeding.
Cladistic analysis of GmbZIP proteins
Soybean and Arabidopsis bZIP proteins have been recently updated (Sussmilch et al., 2015; Dröge-Laser et al., 2018; Zhang et al., 2018), we regenerated the cladistic tree, and the names of soybean bZIP proteins were based on Zhang et al. (2018) in this review. We aligned the full-length GmbZIP and AtbZIP amino acid sequences by MAFFT v7.505 with default parameters and then conducted a cladistic analysis using iQtree with model JTT+F+R5 and polishing using iTOL (https://itol.embl.de). The GmbZIPs and AtbZIPs classified into 13 groups, which is the same as Zhang et al. (2018) and Dröge-Laser et al. (2018) (Figure 1).
Expression patterns of GmbZIP genes
We obtained expression values (fragments per kilobase of exon per million mapped fragments (FPKMs)) of GmbZIP and AtbZIP genes in different tissues/organs (seeds, flowers, leaves, shoot apices, stems, and roots) from the websites at Phytozome12 (https://phytozome.jgi.doe.gov/pz/portal.html) (Schmutz et al., 2010), and AtGenExpress Plus-Extended Tissue Series in the Arabidopsis eFP Browser (http://bar.utoronto.ca/efp_arabidopsis/cgi-bin/efpWeb.cgi) with the Developmental Baseline as the parameter and other parameters remaining the default (Schmid et al., 2005) (Figure 1). Arabidopsis tissues/organs were selected for consisting with soybean tissues/organs. We removed GmbZIP88, AtbZIP18, AtbZIP23, AtbZIP36, AtbZIP62, AtbZIP70, AtbZIP75, and AtbZIP76 due to lack of expression in all tissues/organs. The expression data of the bZIP genes of two species were row scale normalized respectively after log2FPKM+1 by using TBtools (Chen et al., 2020a), and displayed in the heatmap by iTOL (Figure 1; Table S1).
Group A bZIPs regulate abiotic stress responses, plant development, and flowering
We further classified 13 Arabidopsis and 31 soybean members in group A, characterized as having conserved motifs containing phosphorylation sites, into four subgroups (Jakoby et al., 2002; Dröge-Laser et al., 2018). The first subgroup contained AtbZIP15 and AtbZIP35-AtbZIP38, which are involved in abscisic acid (ABA) and stress signaling (Choi et al., 2000; Uno et al., 2000). ABA is a plant hormone that regulates diverse processes including stomatal closure, osmotic stress response, and seed maturation and germination (Yoshida et al., 2015b). The members of this first subgroup contained abscisic acid responsive element (ABRE) binding factors (ABFs) that function at the core of ABA signaling (Banerjee and Roychoudhury, 2017). Under osmotic stress conditions (such as drought and high salinity), accumulating ABA is perceived by Pyrabactin resistance 1
(PYR1)/PYR1-LIKE (PYL)/Regulatory components of ABA receptor (RCAR) receptors that inhibit the phosphatase activities of protein phosphatase type 2Cs (PP2Cs). ABA-PYR1/PYL/RCAR-PP2C complexes activate (Sucrose non-fermenting-1) SNF1-related protein kinase 2s (SnRK2s), then SnRK2s directly phosphorylate ABFs to strongly enhance their transactivation properties by directly binding to ABRE cis-elements (Yoshida et al., 2015b). We identified four GmbZIP TFs in this subgroup, of which GmbZIP14, GmbZIP59, GmbZIP50, and GmbZIP33 were mainly expressed in roots and flowers (Figure 1). Because they clustered with AtbZIP35-AtbZIP 38 in the cladistic tree, they likely also participate in ABA and stress signaling. This idea is reinforced by the detailed analysis of GmbZIP14 (name in the reference is listed in Table 1), which is localized in the nucleus and is responsive to ABA, drought, high salinity, and low temperature. Overexpressing GmbZIP14 improves tolerance to high salt, low temperature, and drought in transgenic plants. Furthermore, some ABREs exist in the promoter region of GmbZIP14 targets, such as ABA insensitive 1 (ABI1), ABI2, RD29B, RAB18, KAT1, and KAT2, whose expression is also affected by ABA, drought, and high salinity (Gao et al., 2011).
The second subgroup contained ABI5/DPBF1/AtbZIP39, which has been extensively characterized in Arabidopsis and functions in ABA-dependent seed maturation and germination (Lopez-Molina et al., 2001; Skubacz et al., 2016). We classified 16 GmbZIP TFs into this subgroup, most of which were highly expressed in seeds (Figure 1), suggesting their potential functions in seed development and germination. In soybean, seed weight is one of the most important yield determinants (Smith and Camper, 1970). The GmbZIP TFs ABA-responsive element binding protein 3-1 (AREB3-1/GmbZIP35), AREB3-2/GmbZIP57, and GmbZIP92 (belonging to Group D) regulate distinct seed development processes by acting with LEAFY COTYLEDON1 (LEC1), a central TF of seed development that controls embryo morphogenesis, photosynthesis, and seed maturation (Jo et al., 2019). LEC1 alone and the LEC1-AREB3 module primarily regulate genes involved in embryo morphogenesis. LEC1-AREB3, LEC1-AREB3-GmbZIP92, and LEC1-AREB3-GmbZIP92-ABI3 modules regulate genes involved in photosynthesis. The LEC1-AREB3-GmbZIP92-ABI3 module also regulates seed maturation genes (Jo et al., 2020) (Figure 2C). Soybean FD-like 19 (GmFDL19, GmbZIP146) also classified in this subgroup, is highly induced by ABA, polyethylene glycol (PEG 6000), and high salinity. Overexpressing GmFDL19 in soybean enhances drought and salt tolerance at the seedling stage. Furthermore, GmFDL19/GmbZIP146 overexpression reduces Na+ ion accumulation and up-regulates the expression of several ABA- and stress-responsive genes (Li et al., 2017).
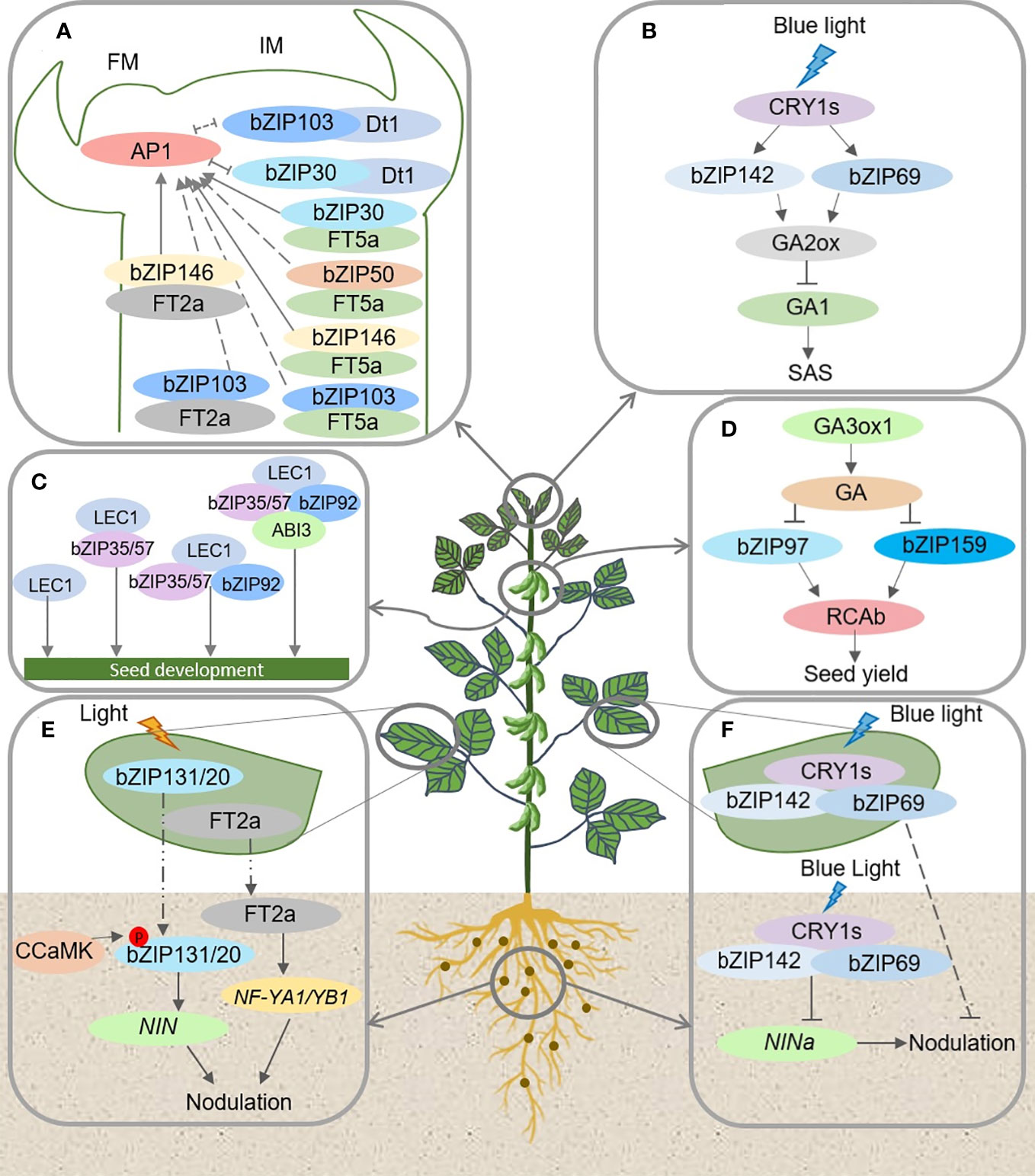
Figure 2 Regulation mechanisms of soybean bZIP TFs involved in selected signaling pathways. (A) The soybean bZIP TFs FDc1/bZIP30, FDL06/bZIP50, FDL12/bZIP103, and FDL19/bZIP146 function together with Dt1, FT2a, and/or FT5a in the regulation of flowering and stem growth habit. (B) STF1/bZIP142 and STF2/bZIP69, whose abundances are increased by light-activated CRY1s, directly upregulate the expression of genes encoding gibberellin 2 oxidases to deactivate gibberellin 1 and control the blue light-induced shade avoidance syndrome. (C) The soybean bZIP TFs ABA-responsive element binding protein 3-1 (AREB3-1/bZIP35), AREB3-2/bZIP57, and bZIP92 regulate distinct seed development processes by acting with LEC1. (D) bZIP97 and bZIP159 are involved in gibberellin biosynthesis, which is associated with soybean seed yield. (E) STF3/bZIP131-STF4/bZIP20-FT module integrates the light-induced shoot signal and the rhizobium-activated root signal, which coordinately promote nodule formation. (F) CRY1s interact with and activate STF1/bZIP142 and STF2/bZIP69 transcription in shoots and roots, which repress NINa expression, thereby inhibiting nodulation. FM: Floral meristem, IM: Inflorescence meristem, SAS: Shade avoidance syndrome. The solid arrows and the solid blunt ended arrows represent the stimulation and inhibition effect on downstream gene or substances, respectively. The dashed lines represent the stimulation remains to be further confirmed. –..– represent movement of proteins.
The third subgroup, containing FD (AtbZIP14) and FD PARALOG (FDP, AtbZIP27), is involved in control of the Arabidopsis floral transition (Abe et al., 2005). The AtbZIP TF FD promotes flowering with the florigen FLOWERING LOCUS T (FT) as a florigen activation complex (Abe et al., 2005; Wigge et al., 2005). TERMINAL FLOWER 1 (TFL1) competes with FT for FD binding and represses the transcription of floral meristem identity genes, such as LEAFY (LFY) and APETALA 1 (AP1) (Gustafson-Brown et al., 1994; Hanano and Goto, 2011). GmTFL1b (Dt1), the soybean ortholog of Arabidopsis TFL1, controls stem growth habit (Liu et al., 2010; Tian et al., 2010) and flowering time (Yue et al., 2021), which strongly influence soybean grain yield (Bernard, 1972; Heatherly and Smith, 2004; Cao et al., 2016). Soybean contains five FD and FDP homologs, which all belong to this subgroup (Sussmilch et al., 2015) (Figure 1). Dt1 interacts with GmFDc1/GmbZIP30 and binds to ACGT cis-elements in the promoter region of GmAP1a to repress its activity during plant height and flowering time regulation in soybean (Chen et al., 2020b; Yue et al., 2021). Overexpressing GmFDc1/GmbZIP30 leads to early flowering and fewer nodes, suggesting that GmFDc1/GmbZIP30 functions as a floral transition activator (Yue et al., 2021). GmFT5a interferes with Dt1 for GmFDc1 binding and enhances the positive effect of GmFDc1/GmbZIP30 on GmAP1 expression (Yue et al., 2021). GmFT2a and GmFT5a both induce flowering; however, GmFT5a plays an additional role in termination of shoot apical meristem growth shortly after floral induction (Takeshima et al., 2019). GmFT5a, but not GmFT2a, competes with Dt1 for GmFDc1/GmbZIP30 binding to more rapidly terminate stem growth (Takeshima et al., 2019; Yue et al., 2021) (Figure 2A). The functions of the other four FD and FDP homologs are not known, and can only be inferred from their gene expression patterns: GmbZIP9 is mainly expressed in shoot apices and seeds, GmbZIP48, GmbZIP30, GmbZIP4 and GmbZIP161 in shoot apices and stems. These distinct expression patterns indicate their functional differentiation during soybean selection and domestication. Furthermore, three other group A members are also involved in floral transition: GmFDL06/GmbZIP50, which interacts with GmFT5a; GmFDL12/GmbZIP103, which functions together with Dt1, GmFT2a, and GmFT5a; and GmFDL19/GmbZIP146, which associates with GmFT2a and GmFT5a (Nan et al., 2014; Takeshima et al., 2019). GmFDL19/GmbZIP146 overexpression in soybean causes early flowering, which may be mediated by upregulation of floral identity genes, such as Suppressor of overexpression of CO 1 (SOC1s), LFYs, and AP1s, the possible direct targets of GmFDL19/GmbZIP146 (Nan et al., 2014) (Figure 2A).
Group A Arabidopsis bZIPs could be divided into four subgroups; three of them have specific functions: ABA and stress signaling, seed maturation and germination, and flowering time and stem growth. Although only a few Group A soybean members are well studied, their functions are largely consistent with those of Arabidopsis members. These studies provide directions for studying other members in each subgroup.
Group B and K bZIPs function in endoplasmic reticulum stress pathways
Two bZIPs (AtbZIP17 and AtbZIP28) in group B and the only member in group K (AtbZIP60) function in two endoplasmic reticulum (ER) stress pathways (Iwata and Koizumi, 2005; Howell, 2013). ER stress occurs under adverse environmental conditions, and the ER stress response is implicated in seed development and pathogen response (Vitale and Ceriotti, 2004). AtbZIP17 and AtbZIP28 regulate the unfolded protein response pathway, and AtbZIP60 is involved in a plant-specific ER stress response signal transduction pathway (Iwata and Koizumi, 2005; Howell, 2013). There are two soybean members in group B, GmbZIP21 and GmbZIP147, whose genes are highly expressed in seeds. Their expression patterns provide clues about their analogous functions in the ER stress response; however, their functions are not clearly understood. The only soybean member in group K (GmbZIP15) is involved in the abiotic stress response, different from its Arabidopsis homolog AtbZIP60. GmbZIP15 transcription is suppressed under salt- and drought-stress conditions. Overexpressing GmbZIP15 in soybean results in hypersensitivity to abiotic stress compared with wild-type plants, which is associated with lower expression of stress-responsive genes, defective stomatal aperture regulation, and reduced antioxidant enzyme activities (Zhang et al., 2020). Considering the crucial functions of AtbZIP60 in the ER stress response, the functions of GmbZIP15 deserve more attention.
Group C and S bZIPs regulate stress responses and plant development
In Arabidopsis, four group C members preferentially heterodimerize with five group S1 members, which is defined as the ‘C/S1 bZIP network’ (Jakoby et al., 2002; Weltmeier et al., 2006). The C/S1 bZIP network is involved in metabolic readjustment during low-energy signaling, downstream of SnRK1 (Baena-González et al., 2007). SnRK1-C/S1 signaling is involved in pathogen defense, which is an energy-consuming process requiring metabolic readjustment. Likewise, C/S1 bZIPs, such as AtbZIP10 in Arabidopsis and AtbZIP63 orthologs in several plant species, are implicated in pathogen defense (Dröge-Laser et al., 1997; Kuhlmann et al., 2003; Kaminaka et al., 2006; Shen et al., 2016). We detected 8 and 28 soybean members in groups C and S, respectively, and most of them are expressed in roots and stems, similar to their Arabidopsis counterparts (Figure 1). Dröge-Laser et al. (1997) isolated a group C member, G/HBF-1/GmbZIP76, which activates pathogen defense by binding to the promoters of Chalcone Synthase 15 (CHS15), CHS7, and CHS1, which belong to the chalcone synthase (CHS) family that catalyzes the first and key regulatory step of flavonoid biosynthesis, the well-characterized defense substances (Dixon et al., 1983; Hahlbrock and Scheel, 1989; Wingender et al., 1990; Yoshida et al., 2008). In-vitro phosphorylation of G/HBF-1/GmbZIP76 enhances its binding to the CHS15 promoter. A cytosolic serine kinase that is stimulated by an avirulent strain of the soybean pathogen Pseudomonas syringae pv. glycinea was identified using recombinant G/HBF-1/GmbZIP76 as a substrate. Stimulation of G/HBF-1/GmbZIP76 kinase activity and G/HBF-1/GmbZIP76 phosphorylation are terminal events in a signal pathway to activate early transcription-dependent plant defense responses (Dröge-Laser et al., 1997). Genes encoding the Group C proteins GmbZIP53 and GmbZIP27 are differentially expressed in the resistant soybean cultivar PI561356 during Asian soybean rust (ASR) infection, which is caused by an obligate biotrophic pathogenic fungus Phakopsora pachyrhizi and results in yield losses of up to 80%, indicating their important roles in the response to ASR infection (Patil et al., 1997; Alves et al., 2015).
Moreover, some Arabidopsis C and S1 members are also involved in abiotic stress responses, such as AtbZIP1 and its partners AtbZIP53, AtbZIP10, and AtbZIP25 (Sun et al., 2012; Hartmann et al., 2015). In soybean, GmbZIP61, GmbZIP51, GmbZIP8, and GmbZIP32 in groups C and S positively regulate drought and salt stress responses; and GmbZIP53 positively regulates drought, salt, and low-temperature stress responses; GmbZIP152 positively regulate drought, salt, heavy metal, and Sclerotinia sclerotiorum stress responses (Liao et al., 2008; Xu et al., 2015; Xu et al., 2016; Yang et al., 2020; Chai et al., 2022). (GmbZIP61 overexpression in Arabidopsis improved salt tolerance by elevating the survival rate, rosette diameter, relative electrolyte leakage, and proline content after a 200 mM NaCl treatment. GmbZIP61 binds to the ACGT motif in promoters and influences the expression of many stress-related genes as well as the accumulation of proline, Na+, and K+ (Xu et al., 2016). GmbZIP51 is induced by multiple abiotic stresses. GmbZIP51 overexpression in Arabidopsis and soybean hairy roots improves tolerance to drought and salt stresses and enhances the expression of the stress-responsive genes GmMYB48, GmWD40, Dehydrins 15 (GmDHN15), Glutathione S-transferase 1 (GmGST1), and Late Embryogenesis Abundant (GmLEA) (Yang et al., 2020). GmbZIP8 is implicated in abiotic stress responses. GmbZIP8 is induced by ABA and salt stress. Promoter analysis indicated that the GmbZIP8 promoter contains cis-acting elements involved in defense and stress responses, such as the ABREs involved in the ABA response and the MYB binding site involved in the drought response. Arabidopsis plants heterologously expressing the GmbZIP8 promoter indicated that GmbZIP8 is strongly induced by ABA and weakly induced by salt (Xu et al., 2015). Liao et al. (2008) reported that GmbZIP32 and GmbZIP53 are differentially regulated by various treatments. GmbZIP32 is induced by drought, flooding, and salt stress, but moderately induced by ABA treatment; GmbZIP53 is slightly induced by drought and salt treatments. Transgenic Arabidopsis plants overexpressing GmbZIP32 or GmbZIP53 had reduced ABA sensitivity, but enhanced salt and low-temperature stress tolerance. GmbZIP152 is significantly induced by salt, drought, heavy metal, and S. sclerotiorum stresses in soybean. Overexpression of GmbZIP152 in Arabidopsis enhances the resistance to the abiotic and S. sclerotiorum stresses. ABA-, JA-, ETH-, and SA-induced biotic- and abiotic-related genes, such as Early Response to Dehydration 1 (GmERD1) and Pathogenesis-related 2 (GmPR2), might be the direct targets of GmbZIP152 (Chai et al., 2022).
The C/S1 bZIP network is also implicated in seed development. The Group C members in Arabidopsis (AtbZIP10 and AtbZIP25) control seed storage protein biosynthesis; AtbZIP53 activates seed maturation by interacting with AtbZIP10 and AtbZIP25 (Lara et al., 2003; Alonso et al., 2009). GmbZIP97 (Group S) and GmbZIP159 (Group C) are involved in gibberellin biosynthesis, which is associated with soybean hundred-seed weight and seed number. Knocking out Gibberellin 3-oxidase1 (GmGA3ox1), which encodes a key gibberellin biosynthesis enzyme, decreases the content of bioactive gibberellins in leaves while enhancing photosynthesis, thereby promoting seed yield by upregulating GmbZIP97 (Group S) and GmbZIP159 (Group C), and then GmbZIP97 and GmbZIP159 directly activate ribulose-1,5-bispho-sphate carboxylase-oxygenase (Rubisco) activases (GmRCAb). Further, GmRCAb induces the production of more Rubisco to increase photosynthesis and ensures sufficient energy transport from leaves to seeds (Hu et al., 2022) (Figure 2D).
Because many genes encoding Group S members are expressed in soybean roots, some of them are implicated in regulating root size and architecture, which are important for yield performance (Price et al., 2002; Manavalan et al., 2009). A major quantitative trait locus on chromosome 8 (the Satt315-I locus) controls tap root length, lateral root number, and shoot length in soybean. Eleven TF genes were identified within the confidence interval of this region, among them, the Group S member GmbZIP61 is highly expressed in the root pericycle and nodules. Pericycle cells form lateral root primordium, which determine lateral root number (Manavalan et al., 2015). GmbZIP43, closely related to GmbZIP61, is also highly expressed in roots, which suggests its similar function in regulating root architecture.
Arabidopsis Group C members always heterodimerize and work together with Group S1 members; however, this phenomenon has not been reported in soybean. Therefore, soybean C and S members may have similar biochemical properties, but further study at the molecular level is needed.
Group D members have diverse functions
Characterized by a short zipper domain, a conserved C terminus, and a more variable N terminus, Group D comprises the so-called ‘TGACG motif-binding factors’ (TGAs), which are further divided into five clades in Arabidopsis (Gatz, 2013). AtTGA1/AtbZIP47 and AtTGA4/AtbZIP57 in Clade I participate in root nitrate uptake, nitrate responses, apoplastic defenses, ER stress responses, salicylic acid biosynthesis, and pathogen defense (Miao et al., 1994; Wang and Fobert, 2013; Alvarez et al., 2014; Sun et al., 2018). The Arabidopsis Clade II factors AtTGA2/AtbZIP20, AtTGA5/AtbZIP26, and AtTGA6/AtbZIP45 play crucial roles in systemic acquired resistance and detoxification processes (Xiang et al., 1997; Müller and Berger, 2008; Fu and Dong, 2013). AtTGA3/AtbZIP22 in Clade III is involved in basal pathogen resistance and in mediating phytohormonal cross-talk between salicylic acid and cytokinin (Choi et al., 2010). Arabidopsis Clade IV members AtTGA9/AtbZIP21 and AtTGA10/AtbZIP65 regulate anther development (Murmu et al., 2010). The Arabidopsis Clade V member AtTGA8/PERIANTHIA (AtPAN/AtbZIP46) controls the formation of floral organ primordia (Chuang et al., 1999; Maier et al., 2011). The soybean members in each clade were expressed in distinct tissues (Figure 1), which suggests their differential roles in soybean. TGACG motif-binding factor 17 (GmTGA17, GmbZIP104), encoding a Clade IV protein, is strongly induced by drought and salt stress. Heterologous overexpression of GmTGA17 in Arabidopsis and homologous overexpression in soybean hairy roots enhanced drought and salt tolerance (Li et al., 2019). GmTGA17/GmbZIP104 is highly expressed in roots, stems, and flowers (Figure 1), which indicated its potential function in another development, similar to AtTGA9/AtbZIP21 and AtTGA10/AtbZIP65.
Group E and M bZIPs regulate pollen wall formation and biotic stress responses
We identified six Arabidopsis and four soybean bZIP TFs in Group E. Research on Arabidopsis Group E bZIP TFs is limited. Only one study reported that one member from Group E, AtbZIP34, has an essential role in pollen wall formation, and the atbzip34 mutants show pollen morphology and pollen germination defects. AtbZIP34 is involved in lipid metabolism, cellular transport, and intine biosynthesis by regulating the putative downstream gene MYB97 (Gibalová et al., 2009). The soybean bZIP TFs GmbZIP106 and GmbZIP114 are closely related to AtbZIP34. GmbZIP106 and GmbZIP114 are highly expressed in flowers, suggesting their involvement in floral organ development (Figure 1).
Two other soybean bZIP TF genes, GmbZIPE1/GmbZIP115 (Group M) and GmbZIPE2/GmbZIP45 (Group E), are differentially expressed during ASR infection in the resistant soybean cultivar PI561356, indicating that their proteins participate in the response to ASR infection (Alves et al., 2015), but their exact functions and regulation mechanisms need further study. All soybean Group M members are highly expressed in seeds, indicating that they have similar functions (Figure 1).
Group F bZIPs regulate zinc deficiency and salt stress responses
Arabidopsis Group F members AtbZIP19 and AtbZIP23 are essential for adaptation to zinc deficiency in Arabidopsis roots (Assuncão et al., 2010). AtbZIP24 is an important regulator of the salt stress response; transcriptional repression of AtbZIP24 improves salt tolerance in Arabidopsis (Yang et al., 2009). GmbZIP84 in this group, whose gene is also highly expressed in roots, may have similar functions as its Arabidopsis homologs (AtbZIP19, AtbZIP23, and AtbZIP24).
Group G bZIPs regulate abiotic stress responses
Group G proteins are characterized by a proline-rich N-terminal activation domain (Jakoby et al., 2002). G-BOX-BINDING FACTOR1 (AtGBF1), a well-known Arabidopsis Group G bZIP TF, regulates blue-light-dependent hypocotyl expansion, lateral root development, natural senescence, and salicylic acid-dependent pathogen defense (Weisshaar et al., 1991; Schindler et al., 1992; Smykowski et al., 2010; Maurya et al., 2015; Giri et al., 2017). We identified 14 soybean bZIPs in Group G, and Soybean G-box binding factor 1 (SGBF-1, GmbZIP82), GmbZIP6, GmbZIP10, and GmbZIP133 are homologs of Arabidopsis GBF1, and their genes are mainly expressed in flowers and leaves (Figure 1). SGBF-1/GmbZIP82 has been extensively studied and participates in abiotic stress responses. SGBF-1/GmbZIP82 binds directly to ABREs in cold-regulated gene promoters. SGBF-1/GmbZIP82 interacts with the C2H2-type zinc finger protein SCOF-1 to up-regulate COLD-REGULATED (AtCOR) expression and enhance cold tolerance in transgenic Arabidopsis (Kim et al., 2001). Another well-studied soybean Group G member is GmbZIP28, which is slightly induced by NaCl treatment. Transgenic Arabidopsis plants overexpressing GmbZIP28showed reduced ABA sensitivity, but increased salt and low-temperature tolerance. GmbZIP28 binds to GLM (GTGAGTCAT), ABRE (CCACGTGG), and PB-like (TGAAAA) cis-elements and may function in ABA signaling by upregulating ABI1 and ABI2, and has roles in stress tolerance by regulating various stress-responsive genes (Liao et al., 2008). Also in Group G, GmbZIP154, GmbZIP58, and GmbZIP130 are homologs of AtGBF2 and AtGBF3; and GmbZIP72, GmbZIP1, GmbZIP68, and GmbZIP132 are homologs of AtbZIP16 and AtbZIP68, but their functions are not clearly understood.
Group H bZIPs regulate environmental signaling and carbon-nitrogen balance
Group H contains only two Arabidopsis members, ELONGATED HYPOCOTYL5 (AtHY5, AtbZIP56) and HY5 HOMOLOG (AtHYH, AtbZIP64). AtHY5/AtbZIP56 promote photomorphogenesis downstream of phytochromes, cryptochromes, and UV-B photoreceptors, and regulates cell elongation, cell proliferation, chloroplast development, lateral root development, pigment accumulation, and nutrient assimilation (Gangappa and Botto, 2016). AtHYH/AtbZIP64 forms heterodimers with AtHY5/AtbZIP56 and enhances transcriptional activation; AtHYH/AtbZIP64 acts redundantly with AtHY5/AtbZIP56 to regulate hypocotyl growth, lateral root growth, pigment accumulation, and the expression of light-inducible genes (Holm et al., 2002; Gangappa and Botto, 2016). Four soybean bZIP TFs belong to this group. Soybean TGACG-motif-binding factor 1 (STF1, GmbZIP142) and STF2/GmbZIP69, mainly expressed in leaves, are homologs of AtHY5. STF3/GmbZIP131 and STF4/GmbZIP20, mainly expressed in leaves, are homologs of AtHYH (Figure 1).
STF1/GmbZIP142 plays a positive role in photomorphogenesis and phytohormone signaling (Song et al., 2008). The C terminus of STF1/GmbZIP142 complemented the Athy5 Arabidopsis mutant phenotype for hypocotyl length, root gravitropic response, and chlorophyll and anthocyanin content, indicating their analogous roles in Arabidopsis and soybean. STF1/GmbZIP142 interacts with three B-box zinc finger proteins STO homolog (GmSTH), and GmSTH2 and with Constitutively Photomorphogenic 1 (GmCOP1), which play important roles in light-dependent development and gene expression. The regulatory mechanisms that involve GmCOP1, STF1/GmbZIP142, and the B-box factors in soybean may be similar to those in Arabidopsis, including STF1/GmbZIP142, GmSTO, and GmSTH degradation in the dark via the GmCOP1-mediated ubiquitination pathway (Shin et al., 2016). In addition, both AtHY5/AtbZIP56 and STF1/GmbZIP142 have strong binding affinity to ACGT-containing elements, suggesting that these two proteins have similar functions and may regulate similar downstream genes (Song et al., 2008).
AtHY5/AtbZIP56 also participates in the shade avoidance response; AtHY5/AtbZIP56 and AtHYH/AtbZIP64 are induced by low red/far-red light ratios (Ciolfi et al., 2013). Soybean displays the classic shade avoidance syndrome, including exaggerated stem elongation, which leads to lodging and yield reduction under dense planting conditions. Two AtHY5/AtbZIP56 homologs in soybean, STF1/GmbZIP142 and STF2/GmbZIP69, whose abundances are increased by light-activated Cryptochrome Circadian Regulator 1 (GmCRY1s), directly upregulate the expression of genes encoding gibberellin 2 oxidases to deactivate gibberellin 1 and repress stem elongation and control the blue light-induced shade avoidance syndrome. GmCRY1b overexpression lines are promising lodging-resistant options for dense planting and intercropping conditions (Lyu et al., 2021) (Figure 2B).
In Arabidopsis, another important role of AtHY5/AtbZIP56 is to adjust the carbon-nitrogen balance. In leaves, AtHY5/AtbZIP56 activates the transcription of SWEET-facilitator genes to support sucrose export to roots (Chen et al., 2016). AtHY5/AtbZIP56 moves from shoot to root to activate its own expression to promote nitrate uptake by activating NITRATE TRANSPORTER2.1 (AtNRT2.1) expression (Chen et al., 2016). In roots, AtHY5/AtbZIP56 is involved in nitrogen signaling pathways by positively regulating NITRATE REDUCTASE2 (AtNIA2) and NITRITE REDUCTASE1 (AtNIR1) expression, and negatively regulating AtNRT1.1 and AMMONIUM TRANSPORTER1;2 (AtAMT1;2) expression (Yanagisawa, 2014; Huang et al., 2015). Different from Arabidopsis, legumes evolved a symbiotic relationship with rhizobia, who fix atmospheric nitrogen and provide nitrogen nutrients to their host plant, and the soybean AtHY5 homologs STF1/GmbZIP142 and STF2/GmbZIP69 are also involved in light-mediated symbiotic root nodulation (Wang et al., 2021). Specifically, the blue light receptor GmCRY1-STF1/GmbZIP142-STF2/GmbZIP69 module plays a pivotal role in integrating darkness/blue light and nodulation signals. Soybean perceives blue light by GmCRY1s, which activates STF1/GmbZIP142 and STF2/GmbZIP69 transcription in shoots and roots. Root GmCRY1s interact with and elevate the levels of STF1/GmbZIP142 and STF2/GmbZIP69, which repress Nodule Inception a (GmNINa) expression, thereby inhibiting nodulation (Ji et al., 2021) (Figure 2F). Wang et al. (2021) demonstrated that light-induced STF3/GmbZIP131, STF4/GmbZIP20, and GmFTs interdependently induce nodule organogenesis from shoots to roots. The rhizobium-activated calcium- and calmodulin-dependent protein kinase (CCaMK) phosphorylates STF3/GmbZIP131, triggering STF3/GmbZIP131-FT2a complex formation, which directly activates GmNIN and Nuclear factor Y (GmNF-YA1 and GmNF-YB1) expression. The GmCCaMK-STF3/GmbZIP131-STF4/GmbZIP20-FT module integrates the light-induced shoot signal and the rhizobium-activated root signal, which coordinately promote nodule formation (Figure 2E).
Group I bZIPs regulate plant development
We identified 12 Arabidopsis and 17 soybean members in Group I, who share a characteristic lysine residue in the basic domain that replaces the highly conserved arginine (Jakoby et al., 2002). The best-studied Arabidopsis member in group I is VIRE2-INTERACTING PROTEIN 1 (AtVIP1, AtbZIP51), which is involved in the Agrobacterium tumefaciens response, pathogen response, abiotic stress response, cell proliferation, and plant development (Van Leene et al., 2016). The most closely related soybean bZIP TFs are GmbZIP11, GmbZIP134, and GmbZIP78. GmbZIP11 and GmbZIP134 are highly expressed in leaves and stems; GmbZIP78 is highly expressed in seeds (Figure 1).
Group I members regulate plant development. AtbZIP29 regulates the cell number in leaves and root meristems by controlling cell wall organization, and DRINK ME (AtDKM, AtbZIP30) affects meristematic tissues and gynoecium development (Lozano-Sotomayor et al., 2016; Van Leene et al., 2016). Their homologous genes in soybean, GmbZIP85, GmbZIP139, and GmbZIP31, are highly expressed in flowers, which implies their similar roles as AtDKM, whereas GmbZIP124 and GmbZIP96 are highly expressed in roots and stems, which suggests similar functions as those of AtbZIP29. Another Group I member, AtbZIP18, controls pollen development by interacting with AtbZIP34, AtbZIP52, and AtbZIP61, and works redundantly with AtbZIP34 (Gibalová et al., 2017). AtbZIP18, AtbZIP52, AtbZIP34, and AtbZIP61 have one to four homologs in soybean; their unknown redundancy may make it difficult to study their functions. In Group I, AtbZIP59 interacts with lateral organ boundaries domain (LBD) TFs to regulate auxin-induced callus formation, which is required for plant regeneration (Xu et al., 2018). GmbZIP87, GmbZIP99, GmbZIP118, and GmbZIP125 are mainly expressed in roots and stems, but their exact functions are unknown.
Conclusions and perspectives
Plant bZIP TFs regulate a variety of biological processes. The functions of some soybean bZIP TFs have been extensively studied (Table 1). Most soybean members in Groups B, K, D, E, M, and G regulate abiotic stress responses, which differs from the various functions of Arabidopsis members in these groups, such as ER stress response, root nitrate uptake, nitrate responses, apoplastic defenses, pathogen defense, floral organ development, pollen development, lateral root development, and natural senescence. However, the functions of soybean members in Groups A, C, S, and H are highly consistent with those of Arabidopsis members in these groups. Our knowledge of soybean members in Groups B, K, D, E, M, and G is limiteds, and reports on the functions of Group F, I, J, and N soybean members are even less clear. Therefore, detailed investigations of all soybean bZIP TF functions and molecular mechanisms are needed. The cladistic, transcriptional, and functional information we provided in each group will be useful for future studies.
Soybean is an important protein and oil crop. Soybean yield is determined by multiple traits, including flowering time, node number, internode length, effective branching number, pod number per plant, seed number per plant, and hundred-seed weight (Pedersen and Lauer, 2004; Lu et al., 2017; Lu et al., 2020). Soybean bZIP TFs regulate many important yield traits. For example, GmFDc1, GmFDL12, GmFDL19, and GmFDL06 (Group A) are transcription cofactors of Dt1, GmFT2a, and/or GmFT5a, which determine stem growth habit and/or flowering time of soybean (Nan et al., 2014; Takeshima et al., 2019; Li et al., 2021; Yue et al., 2021). The detailed functions and regulatory mechanisms of these Group A TFs remain unclear, but they appear to have enormous contributions to soybean yield. STF1 and STF2 (Group H) are involved in the shade avoidance syndrome, which corresponds to internode length of soybean main stems (Lyu et al., 2021). AREB3 (Group A) and GmbZIP92 (Group D) regulate seed development by acting with LEC1 (Jo et al., 2020). GmbZIP97 (Group S) and GmbZIP159 (Group C) are involved in the gibberellin-mediated seed development pathway (Hu et al., 2022). These bZIP TFs are optimal gene resources for soybean breeding. Crop yield is reduced when plants are exposed to extreme environmental conditions such as high salt, drought, cold, and heat, as well as to biotic stresses such as insects and pathogen invasion. Plants exhibit numerous adaptive and protective responses to various abiotic and biotic stimuli. Most of the soybean bZIP TFs are considered abiotic stress regulators; about 75.6% of soybean bZIP genes display transcriptional changes after abiotic stress treatment (Zhang et al., 2018). Among the well-studied soybean bZIP TFs, 11 regulate abiotic stress responses, and 5 are involved in biotic stress responses (Table 1). Most of the environmental-stress-related Arabidopsis bZIP TFs are distributed in Groups A, C, S, B, K, D, G, and F. Although the functional diversity and molecular mechanisms of these soybean members need further study, preliminary data provide a valuable basis for future study.
Biological nitrogen fixation is an alternative to nitrogen fertilizer; the ability of legumes to form a symbiosis with nitrogen-fixing rhizobia provides a distinct advantage (Ferguson et al., 2010). STF1, STF2, STF3, and STF4 (Group H) are orthologs of AtHY5 and AtHYH. STF1 and STF2 suppress soybean nodulation, while STF3 and STF4 play positive roles in light-induced nodulation responses in soybean (Ji et al., 2021; Wang et al., 2021). Further studies are needed to determine why these proteins regulate nodulation in different ways, and different strategies should be used to utilize these gene resources in soybean breeding.
The functions of some soybean bZIP TFs have been analyzed via physiological experiments and genetic engineering; however, the biochemical properties and regulation mechanisms of many members remain unclear. Because of the duplication of the soybean genome, soybean contains two to ten bZIP TF homologs, which makes it difficult to analyze their homodimers/heterodimers, binding sites, and knockout phenotypes. Advances in genomics and molecular biology have facilitated cloning of homologs and screening of TF binding sites, and clustered regularly interspaced short palindromic repeats (CRISPR)/CRISPR-associated protein 9 (Cas9) gene editing technologies have accelerated the generation of single and multiple mutants for studying genes and their functions, which will also facilitate the study of soybean bZIP TFs.
Author contributions
XL, LZ, and FK conceptualized the idea, LY and XP wrote the initial manuscript draft. All authors contributed to the article and approved the submitted version.
Funding
This work was supported by the National Natural Science Foundation of China (31901567); the Science and Technology Projects in Guangzhou, China (Grant 202002030180 to XL, Grant 2023A04J1503 to LY); the China Postdoctoral Science Foundation (2019M662843); and the Guangdong Provincial Key Laboratory of Plant Adaptation and Molecular Design.
Conflict of interest
The authors declare that the research was conducted in the absence of any commercial or financial relationships that could be construed as a potential conflict of interest.
Publisher’s note
All claims expressed in this article are solely those of the authors and do not necessarily represent those of their affiliated organizations, or those of the publisher, the editors and the reviewers. Any product that may be evaluated in this article, or claim that may be made by its manufacturer, is not guaranteed or endorsed by the publisher.
Supplementary material
The Supplementary Material for this article can be found online at: https://www.frontiersin.org/articles/10.3389/fpls.2023.1150363/full#supplementary-material
References
Abe, M., Kobayashi, Y., Yamamoto, S., Ichinoki, H., Notaguchi, M., Goto, K. (2005). FD, a bZIP protein mediating signals from the floral pathway integrator FT at the shoot apex. Science. 309, 1052–1057. doi: 10.1126/science.1115983
Albani, D., Hammond-Kosack, M. C., Smith, C., Conlan, S., Colot, V., Holdsworth, M., et al. (1997). The wheat transcriptional activator SPA: A seed-specific bZIP protein that recognizes the GCN4-like motif in the bifactorial endosperm box of prolamin genes. Plant Cell. 9, 171–184. doi: 10.1105/tpc.9.2.171
Alonso, R., Oñate-Sánchez, L., Weltmeier, F., Ehlert, A., Diaz, I., Dietrich, K., et al. (2009). A pivotal role of the basic leucine zipper transcription factor bZIP53 in the regulation of arabidopsis seed maturation gene expression based on heterodimerization and protein complex formation. Plant Cell. 21, 1747–1761. doi: 10.1105/tpc.108.062968
Alvarez, J. M., Riveras, E., Vidal, E. A., Gras, D. E., Contreras-López, O., Tamayo, K. P., et al. (2014). Systems approach identifies TGA1 and TGA4 transcription factors as important regulatory components of the nitrate response of arabidopsis thaliana roots. Plant J. 80, 1–13. doi: 10.1111/tpj.12618
Alves, M. S., Soares, Z. G., Vidigal, P. M. P., Barros, E. G., Poddanosqui, A. M. P., Aoyagi, L. N. (2015). Differential expression of four soybean bZIP genes during Phakopsora pachyrhizi infection. Funct. Integr. Genomics 15, 685–696.
Assuncão, A. G., Herrero, E., Lin, Y. F., Huettel, B., Talukdar, S., Smaczniak, C., et al. (2010). Arabidopsis thaliana transcription factors bZIP19 and bZIP23 regulate the adaptation to zinc deficiency. Proc. Natl. Acad. Sci. U.S.A. 107 (22), 10296–10301. doi: 10.1073/pnas.1004788107
Baena-González, E., Rolland, F., Thevelein, J. M., Sheen, J. (2007). A central integrator of transcription networks in plant stress and energy signalling. Nature 448, 938–942. doi: 10.1038/nature06069
Banerjee, A., Roychoudhury, A. (2017). Abscisic-acid-dependent basic leucine zipper (bZIP) transcription factors in plant abiotic stress. Protoplasma 254, 3–16. doi: 10.1007/s00709-015-0920-4
Bernard, R. L. (1972). Two genes affecting stem termination in soybeans. Crop Sci. 12, 235–239. doi: 10.2135/cropsci1972.0011183X001200020028x
Cao, D., Takeshima, R., Zhao, C., Liu, B., Jun, A., Kong, F. (2016). Molecular mechanisms of flowering under long days and stem growth habit in soybean. J. Exp. Bot. 8, 1873–1884. doi: 10.1093/jxb/erw394
Chai, M., Fan, R., Huang, Y., Jiang, X., Wai, M. H., Yang, Q., et al. (2022). GmbZIP152, a soybean bZIP transcription factor, confers multiple biotic and abiotic stress responses in plant. Int. J. Mol. Sci. 23, 10935. doi: 10.3390/ijms231810935
Chen, C., Chen, H., Zhang, Y., Thomas, H. R., Frank, M. H., He, Y., et al. (2020a). TBtools: an integrative toolkit developed for interactive analyses of big biological data. Mol. Plant 13 (8), 1194–1202. doi: 10.1016/j.molp.2020.06.009
Chen, L., Nan, H., Kong, L., Yue, L., Yang, H., Zhao, Q., et al. (2020b). Soybean AP1 homologs control flowering time and plant height. j. integr. Plant Biol. 62, 1–12. doi: 10.1111/jipb.12988
Chen, X., Yao, Q., Gao, X., Jiang, C., Harberd, N. P., Fu, X. (2016). Shoot-to-root mobile transcription factor HY5 coordinates plant carbon and nitrogen acquisition. Curr. Biol. 26, 640–646. doi: 10.1016/j.cub.2015.12.066
Chern, M. S., Eiben, H. G., Bustos, M. M. (1996). The developmentally regulated bZIP factor ROM1 modulates transcription from lectin and storage protein genes in bean embryos. Plant J. 10, 135–148. doi: 10.1046/j.1365-313X.1996.10010135.x
Choi, H., Hong, J., Ha, J., Kang, J., Kim, S. Y. (2000). ABFs, a family of ABA- responsive element binding factors. J. Biol. Chem. 275, 1723–1730. doi: 10.1074/jbc.275.3.1723
Choi, J., Huh, S. U., Kojima, M., Sakakibara, H., Paek, K. H., Hwang, I. (2010). The cytokinin-activated transcription factor ARR2 promotes plant immunity via TGA3/NPR1-dependent salicylic acid signaling in arabidopsis. Dev. Cell. 19, 284–295. doi: 10.1016/j.devcel.2010.07.011
Chuang, C. F., Running, M. P., Williams, R. W., Meyerowitz, E. M. (1999). The PERIANTHIA gene encodes a bZIP protein involved in the determination of floral organ number in arabidopsis thaliana. Genes Dev. 13, 334–344. doi: 10.1101/gad.13.3.334
Ciolfi, A., Sessa, G., Sassi, M., Possenti, M., Salvucci, S., Carabelli, M., et al. (2013). Dynamics of the shade-avoidance response in arabidopsis. Plant Physiol. 163, 331–353. doi: 10.1104/pp.113.221549
Cluis, C. P., Mouchel, C. F., Hardtke, C. S. (2004). The arabidopsis transcription factor HY5 integrates light and hormone signaling pathways. Plant J. 38, 332–347. doi: 10.1111/j.1365-313X.2004.02052.x
Corrêa, L. G. G., Riaño-Pachón, D. M., Schrago, C. G., Vicentini dos Santos, R., Mueller-Roeber, B., Vincentz, M. (2008). The role of bZIP transcription factors in green plant evolution: adaptive features emerging from four founder genes. PloS One 3 (8), e2944. doi: 10.1371/journal.pone.0002944
Dixon, R. A., Dey, P. M., Lamb, C. J. (1983). Phytoalexins: enzymology and molecular biology. Adv. Enzymol. Relat. Areas. Mol. Biol. 55, 1–136. doi: 10.1002/9780470123010.ch1
Dröge-Laser, W., Kaiser, A., Lindsay, W. P., Halkier, B. A., Loake, G. J., Doerner, P., et al. (1997). Rapid stimulation of a soybean protein-serine kinase that phosphorylates a novel bZIP DNA-binding protein, G/HBF-1, during the induction of early transcription-dependent defenses. EMBO J. 16, 726–738. doi: 10.1093/emboj/16.4.726
Dröge-Laser, W., Snoek, B. L., Snel, B., Weiste, C. (2018). The arabidopsis bZIP transcription factor family - an update. Curr. Opin. Plant Biol. 45, 36–49. doi: 10.1016/j.pbi.2018.05.001
Ferguson, B. J., Indrasumunar, A., Hayashi, S., Lin, M. H., Lin, Y. H., Reid, D. E. (2010). Molecular analysis of legume nodule development and autoregulation. J. Integr. Plant Biol. 52 (1), 61–76.
Fu, Z. Q., Dong, X. (2013). Systemic acquired resistance: turning local infection into global defense. Annu. Rev. Plant Biol. 64, 839–863. doi: 10.1146/annurev-arplant-042811-105606
Fukazawa, J., Sakai, T., Ishida, S., Yamaguchi, I., Kamiya, Y., Takahashi, Y. (2000). REPRESSION OF SHOOT GROWTH, a bZIP transcriptional activator, regulates cell elongation by controlling the level of gibberellins. Plant Cell. 12, 901–915. doi: 10.1105/tpc.12.6.901
Gangappa, S. N., Botto, J. F. (2016). The multifaceted roles of HY5 in plant growth and development. Mol. Plant 9, 1353–1365. doi: 10.1016/j.molp.2016.07.002
Gao, S. Q., Chen, M., Xu, Z. S., Zhao, C. P., Li, L., Xu, H., et al. (2011). The soybean GmbZIP1 transcription factor enhances multiple abiotic stress tolerances in transgenic plants. Plant Mol. Biol. 75, 537–553. doi: 10.1007/s11103-011-9738-4
Gatz, C. (2013). From pioneers to team players: TGA transcription factors provide a molecular link. Mol. Plant Microbe Interact. 26, 151–159. doi: 10.1094/MPMI-04-12-0078-IA
Gibalová, A., Reňák, D., Matczuk, K., Dupl’áková, N., Cháb, D., Twell, D., et al. (2009). AtbZIP34 is required for arabidopsis pollen wall patterning and the control of several metabolic pathways in developing pollen. Plant Mol. Biol. 70, 581–601. doi: 10.1007/s11103-009-9493-y
Gibalová, A., Steinbachová, L., Hafidh, S., Bláhová, V., Gadiou, Z., Michailidis, C., et al. (2017). Characterization of pollen-expressed bZIP protein interactions and the role of ATbZIP18 in the male gametophyte. Plant Reprod. 30, 1–17. doi: 10.1007/s00497-016-0295-5
Giri, M. K., Singh, N., Banday, Z. Z., Singh, V., Ram, H., Singh, D., et al. (2017). GBF1 differentially regulates CAT2 and PAD4 transcription to promote pathogen defense in arabidopsis thaliana. Plant J. 91, 802–815. doi: 10.1111/tpj.13608
Gustafson-Brown, C., Savidge, B., Yanofsky, M. F. (1994). Regulation of the arabidopsis floral homeotic gene APETALA1. Cell. 76, 131–143. doi: 10.1016/0092-8674(94)90178-3
Hahlbrock, K., Scheel, D. (1989). Physiology and molecular biology of phenylpropanoid metabolism. Annu. Rev. Plant Physiol. Plant Mol. Biol. 40, 347–369. doi: 10.1146/annurev.pp.40.060189.002023
Hanano, S., Goto, K. (2011). Arabidopsis TERMINAL FLOWER1 is involved in the regulation of flowering time and inflorescence development through transcriptional repression. Plant Cell. 23, 3172–3184. doi: 10.1105/tpc.111.088641
Hartmann, L., Pedrotti, L., Weiste, C., Fekete, A., Schierstaedt, J., Göttler, J., et al. (2015). Crosstalk between two bZIP signaling pathways orchestrates salt-induced metabolic reprogramming in arabidopsis roots. Plant Cell. 27, 2244–2260. doi: 10.1105/tpc.15.00163
Heatherly, L. G., Smith, J. R. (2004). Effect of soybean stem growth habit on height and node number after beginning bloom in the mid-southern USA. Crop Sci. 44, 1855–1858. doi: 10.2135/cropsci2004.1855
Holm, M., Ma, L. G., Qu, L. J., Deng, X. W. (2002). Two interacting bZIP proteins are direct targets of COP1-mediated control of light dependent gene expression in arabidopsis. Genes Dev. 16, 1247–1259. doi: 10.1101/gad.969702
Howell, S. H. (2013). Endoplasmic reticulum stress responses in plants. Annu. Rev. Plant Biol. 64, 477–499. doi: 10.1146/annurev-arplant-050312-120053
Hu, D., Li, X., Yang, Z., Liu, S., Hao, D., Chao, M., et al. (2022). Downregulation of a gibberellin 3b-hydroxylase enhances photosynthesis and increases seed yield in soybean. New Phytol. 235, 502–517. doi: 10.1111/nph.18153
Huang, L., Zhang, H., Zhang, H., Deng, X. W., Wei, N. (2015). HY5 regulates nitrite reductase 1 (NIR1) and ammonium transporter1;2 (AMT1;2) in arabidopsis seedlings. Plant Sci. 238, 330–339. doi: 10.1016/j.plantsci.2015.05.004
Iwata, Y., Koizumi, N. (2005). An arabidopsis transcription factor, AtbZIP60, regulates the endoplasmic reticulum stress response in a manner unique to plants. Proc. Natl. Acad. Sci. U.S.A. 102, 5280–5285. doi: 10.1073/pnas.0408941102
Jakoby, M., Weisshaar, B., Droge Laser, W., Vicente Carbajosa, J., Tiedemann, J., Kroj, T., et al. (2002). bZIP transcription factors in arabidopsis. Trends Plant Sci. 7, 106–111. doi: 10.1016/S1360-1385(01)02223-3
Ji, H., Xiao, R., Lyu, X., Chen, J., Zhang, X., Wang, Z., et al. (2021). Differential light-dependent regulation of soybean nodulation by papilionoid-specific HY5 homologs, curr. Biol. 32, 1–13.
Jo, L., Pelletier, J. M., Harada, J. J. (2019). Central role of the LEAFY COTYLEDON1 transcription factor in seed development. J. Integr. Plant Biol. 61, 564–580. doi: 10.1111/jipb.12806
Jo, L., Pelletier, J. M., Hsu, S. W., Badena, R., Goldberg, R. B., Harada, J. J. (2020). Combinatorial interactions of the LEC1 transcription factor specify diverse developmental programs during soybean seed development. Proc. Natl. Acad. Sci. U.S.A. 117 (2), 1223–1232. doi: 10.1073/pnas.1918441117
Kaminaka, H., Nake, C., Epple, P., Dittgen, J., Schutze, K., Chaban, C., et al. (2006). bZIP10-LSD1 antagonism modulates basal defense and cell death in arabidopsis following infection. EMBO J. 25, 4400–4411. doi: 10.1038/sj.emboj.7601312
Kim, J. C., Lee, S. H., Cheong, Y. H., Cheol-Min Yoo, C. M., Lee, S. I., Chun, H. J., et al. (2001). A novel cold-inducible zinc finger protein from soybean, SCOF-1, enhances cold tolerance in transgenic plants. Plant J. 25 (3), 247–259. doi: 10.1046/j.1365-313x.2001.00947.x
Kuhlmann, M., Horvay, K., Strathmann, A., Heinekamp, T., Fischer, U., Böttner, S., et al. (2003). The a-helical D1 domain of the tobacco bZIP transcription factor BZI-1 interacts with the ankyrin-repeat protein ANK1 and is important for BZI-1 function, both in auxin signaling and pathogen response. J. Biol. Chem. 278, 8786–8794. doi: 10.1074/jbc.M210292200
Lara, P., Onate-Sanchez, L., Abraham, Z., Ferrandiz, C., Diaz, I., Carbonero, P., et al. (2003). Synergistic activation of seed storage protein gene expression in arabidopsis by ABI3 and two bZIPs related to OPAQUE2. J. Biol. Chem. 278, 21003–21011. doi: 10.1074/jbc.M210538200
Li, B., Liu, Y., Cui, X. Y., Fu, J. D., Zhou, Y. B., Zheng, W. J. (2019). Genome-wide characterization and expression analysis of soybean tga transcription factors identified a novel tga gene involved in drought and salt tolerance. Front. Plant Sci. 10, 549.
Li, Y., Chen, Q., Nan, H., Li, X., Lu, S., Zhao, X., et al. (2017). Overexpression of GmFDL19 enhances tolerance to drought and salt stresses in soybean. PloS One 12 (6), e0179554. doi: 10.1371/journal.pone.0179554
Li, X., Fang, C., Yang, Y., Lv, T., Su, T., Chen, L., et al. (2021). Overcoming the genetic compensation response of soybean florigens to improve adaptation and yield at low latitudes, curr. Biol. 31, 1–13. doi: 10.1016/j.cub.2021.12.041
Li, D., Fu, F., Zhang, H., Song, F. (2015). Genome-wide systematic characterization of the bZIP transcriptional factor family in tomato (Solanum lycopersicum l.). BMC Genom. 16, 771. doi: 10.1186/s12864-015-1990-6
Liao, Y., Zou, H. F., Wei, W., Hao, Y. J., Tian, A. G., Huang, J., et al. (2008). Soybean GmbZIP44, GmbZIP62 and GmbZIP78 genes function as negative regulator of ABA signaling and confer salt and freezing tolerance in transgenic arabidopsis. Planta. 228, 225–240. doi: 10.1007/s00425-008-0731-3
Liu, B., Watanabe, S., Uchiyama, T., Kong, F., Kanazawa, A., Xia, Z., et al. (2010). The soybean stem growth habit gene Dt1 is an ortholog of arabidopsis TERMINAL FLOWER1. Plant Physiol. 153, 198–210. doi: 10.1104/pp.109.150607
Lopez-Molina, L., Mongrand, S., Chua, N. H. (2001). A postgermination developmental arrest checkpoint is mediated by abscisic acid and requires the ABI5 transcription factor in arabidopsis. Proc. Natl. Acad. Sci. U.S.A. 98, 4782–4787. doi: 10.1073/pnas.081594298
Lozano-Sotomayor, P., Chávez Montes, R. A., Silvestre-Vañó, M., Herrera-Ubaldo, H., Greco, R., Pablo-Villa, J., et al. (2016). Altered expression of the bZIP transcription factor DRINK ME affects growth and reproductive development in arabidopsis thaliana. Plant J. 88, 437–451. doi: 10.1111/tpj.13264
Lu, S. J., Dong, L. D., Fang, C., Liu, S. L., Kong, L. P., Cheng, Q., et al. (2020). Stepwise selection on homeologous PRR genes controlling flowering and maturity during soybean domestication. Nat. Genet. 52, 428–436. doi: 10.1038/s41588-020-0604-7
Lu, S. J., Zhao, X. H., Hu, Y. L., Liu, S. L., Nan, H. Y., Li, X. M., et al. (2017). Natural variation at the soybean J locus improves adaptation to the tropics and enhances yield. Nat. Genet. 49, 773–779. doi: 10.1038/ng.3819
Lyu, X., Cheng, Q., Qin, C., Li, Y., Xu, X., Ji, R., et al. (2021). GmCRY1s modulate gibberellin metabolism to regulate soybean shade avoidance in response to reduced blue light. Mol. Plant 14, 298–314. doi: 10.1016/j.molp.2020.11.016
Maier, A. T., Stehling-sun, S., Offenburger, S., Lohmann, J. U. (2011). The bZIP transcription factor PERIANTHIA: a multifunctional hub for meristem control. Front. Plant Sci. 2, 1–17. doi: 10.3389/fpls.2011.00079
Manavalan, L. P., Guttikonda, S. K., Tran, L. P., Nguyen, H. T. (2009). Physiological and molecular approaches to improve drought resistance in soybean. Plant Cell Physiol. 50, 1260–1276. doi: 10.1093/pcp/pcp082
Manavalan, L. P., Prince, S. J., Musket, T. A., Chaky, J., Deshmukh, R., Vuong, T. D., et al. (2015). Identification of novel QTL governing root architectural traits in an interspecific soybean population. PloS One 10 (3), e0120490. doi: 10.1371/journal.pone.0120490
Maurya, J. P., Sethi, V., Gangappa, S. N., Gupta, N., Chattopadhyay, S. (2015). Interaction of MYC2 and GBF1 results in functional antagonism in blue light-mediated arabidopsis seedling development. Plant J. 83, 439–450. doi: 10.1111/tpj.12899
Miao, Z. H., Liu, X., Lam, E. (1994). TGA3 is a distinct member of the TGA family of bZIP transcription factors in arabidopsis thaliana. Plant Mol. Biol. 25, 1–11. doi: 10.1007/BF00024193
Müller, M. J., Berger, S. (2008). General detoxification and stress responses are mediated by oxidized lipids through TGA transcription factors in arabidopsis. Plant Cell. 20, 768–785. doi: 10.1105/tpc.107.054809
Murmu, J., Bush, M. J., DeLong, C., Li, S., Xu, M., Khan, M., et al. (2010). Arabidopsis basic leucine-zipper transcription factors TGA9 and TGA10 interact with floral glutaredoxins ROXY1 and ROXY2 and are redundantly required for anther development. Plant Physiol. 154, 1492–1504. doi: 10.1104/pp.110.159111
Nan, H., Cao, D., Zhang, D., Li, Y., Lu, S., Tang, L., et al. (2014). GmFT2a and GmFT5a redundantly and differentially regulate flowering through interaction with and upregulation of the bZIP transcription factor GmFDL19 in soybean. PloS One 9, e97669. doi: 10.1371/journal.pone.0097669
Oyama, T., Shimura, Y., Okada, K. (1997). The arabidopsis HY5 gene encodes a bZIP protein that regulates stimulus-induced development of root and hypocotyl. Genes Dev. 11, 2983–2995. doi: 10.1101/gad.11.22.2983
Patil, V. S., Wuike, R. V., Thakare, C. S., Chirame, B. B. (1997). Viability of uredospores of phakopsora pachyrhizi syd. at different storage conditions. J. Maharashtra Agric. Univ. 22, 260–261.
Pedersen, P., Lauer, J. G. (2004). Response of soybean yield components to management system and planting date. Agron. J. 96, 1372–1381. doi: 10.2134/agronj2004.1372
Price, A. H., Townend, J., Jones, M. P., Audebert, A., Courtois, B. (2002). Mapping QTLs associated with drought avoidance in upland rice grown in the Philippines and West Africa. Plant Mol. Biol. 48, 683–695. doi: 10.1023/A:1014805625790
Schindler, U., Menkens, A. E., Beckmann, H., Ecker, J. R., Cashmore, A. R. (1992). Heterodimerization between light-regulated and ubiquitously expressed arabidopsis GBF bZIP proteins. EMBO J. 11, 1261–1273. doi: 10.1002/j.1460-2075.1992.tb05170.x
Schmid, M., Davison, T. S., Henz, S. R., Pape, U. J., Demar, M., Vingron, M., et al. (2005). A gene expression map of arabidopsis thaliana development. Nat. Genet. 37 (5), 501–506. doi: 10.1038/ng1543
Schmutz, J., Cannon, S., Schlueter, J., Ma, J., Mitros, T., Nelson, W., et al. (2010). Genome sequence of the palaeopolyploid soybean. Nature 463, 178–183. doi: 10.1038/nature08670
Shen, L., Liu, Z., Yang, S., Yang, T., Liang, J., Wen, J., et al. (2016). Pepper CabZIP63 acts as a positive regulator during ralstonia solanacearum or high temperature-high humidity challenge in a positive feedback loop with CaWRKY40. J. Exp. Bot. 67, 2439–2451. doi: 10.1093/jxb/erw069
Shin, S. Y., Kim, S. H., Kim, H. J., Jeon, S. J., Sim, S. A., Ryu, G. R., et al. (2016). Isolation of three b-box zinc finger proteins that interact with STF1 and COP1 defines a HY5/COP1 interaction network involved in light control of development in soybean. BBRC. 478, 1080e1086. doi: 10.1016/j.bbrc.2016.08.069
Skubacz, A., Daszkowska-Golec, A., Szarejko, I. (2016). The role and regulation of ABI5 (ABA-insensitive 5) in plant development, abiotic stress responses and phytohormone crosstalk. Front. Plant Sci. 7, 1884. doi: 10.3389/fpls.2016.01884
Smith, T. J., Camper, H. M. (1970). Effects of seed size on soybean performance. Agro. J. 67, 681–684. doi: 10.2134/agronj1975.00021962006700050025x
Smykowski, A., Zimmermann, P., Zentgraf, U. (2010). G-Box binding factor1 reduces CATALASE2 expression and regulates the onset of leaf senescence in arabidopsis. Plant Physiol. 153, 1321–1331. doi: 10.1104/pp.110.157180
Song, Y. H., Yoo, C. M., Hong, A. P., Kim, S. H., Jeong, H. J., Kim, H. J., et al. (2008). DNA-Binding study identifies c-box and hybrid C/G-box or C/A-box motifs as high-affinity binding sites for STF1 and LONG HYPOCOTYL5 proteins. Plant Physiol. 146, 1862e1877. doi: 10.1104/pp.107.113217
Sun, T., Busta, L., Zhang, Q., Ding, P., Jetter, R., Zhang, Y. (2018). T: GACG-binding factor 1 (TGA1) and TGA4 regulate salicylic acid and pipecolic acid biosynthesis by modulating the expression of systemic acquired resistance deficient 1 (SARD1) and calmodulin-binding protein 60 g (CBP60 g). New Phytol. 217, 344–354. doi: 10.1111/nph.14780
Sun, X., Li, Y., Cai, H., Bai, X., Ji, W., Ding, X., et al. (2012). The arabidopsis AtbZIP1 transcription factor is a positive regulator of plant tolerance to salt, osmotic and drought stresses. J. Plant Res. 125, 429–438. doi: 10.1007/s10265-011-0448-4
Sussmilch, F. C., Berbel, A., Hecht, V., Vander Schoor, J. K., Ferrándiz, C., Madueño, F., et al. (2015). Pea VEGETATIVE2 is an FD homolog that is essential for flowering and compound inflorescence development. Plant Cell. 27 (4), 1046–1046. doi: 10.1105/tpc.115.136150
Takeshima, R., Nan, H., Harigai, K., Dong, L., Zhu, J., Lu, S., et al. (2019). Functional divergence between soybean FLOWERING LOCUS T orthologues FT2a and FT5a in post-flowering stem growth. J. Exp. Bot. 70, 3941–3953. doi: 10.1093/jxb/erz199
Tian, Z., Wang, X., Lee, R., Li, Y., Specht, J. E., Nelson, R. L., et al. (2010). Artificial selection for determinate growth habit in soybean. P. Natl. Acad. Sci. U.S.A. 107, 8563–8568. doi: 10.1073/pnas.1000088107
Uno, Y., Furihata, T., Abe, H., Yoshida, R., Shinozaki, K., Yamaguchi-Shinozake, K. (2000). Arabidopsis basic leucine zipper transcription factors involved in an abscisic acid-dependent signal transduction pathway under drought and high-salinity conditions. P. Natl. Acad. Sci. U.S.A. 97 (21), 11632–11637. doi: 10.1073/pnas.190309197
Van Leene, J., Blomme, J., Kulkarni, S. R., Cannoot, B., De Winne, N., Eeckhout, D., et al. (2016). Functional characterization of the arabidopsis transcription factor bZIP29 reveals its role in leaf and root development. J. Exp. Bot. 67, 5825–5840. doi: 10.1093/jxb/erw347
Vitale, A., Ceriotti, A. (2004). Protein quality control mechanisms and protein storage in the endoplasmic reticulum. a conflict of interests? Plant Physiol. 136, 3420–3426.
Wang, Z., Cheng, K., Wan, L., Yan, L., Jiang, H., Liu, S., et al. (2015). Genome-wide analysis of the basic leucine zipper (bZIP) transcription factor gene family in six legume genomes. BMC Genom. 16, 1053. doi: 10.1186/s12864-015-2258-x
Wang, L., Fobert, P. R. (2013). Arabidopsis clade I TGA factors regulate apoplastic defences against the bacterial pathogen pseudomonas syringae through endoplasmic reticulum- based processes. PloS One 8, e77378. doi: 10.1371/journal.pone.0077378
Wang, T., Guo, J., Peng, Y., Lyu, X., Liu, B., Sun, S., et al. (2021). Light-induced mobile factors from shoots regulate rhizobium-triggered soybean root nodulation. Science. 374, 65–71. doi: 10.1126/science.abh2890
Wei, K., Chen, J., Wang, Y., Chen, Y., Chen, S., Lin, Y., et al. (2012). Genome-wide analysis of bZIP-encoding genes in maize. DNA Res. 19, 463–476. doi: 10.1093/dnares/dss026
Wei, L. Q., Xu, W. Y., Deng, Z. Y., Su, Z., Xue, Y., Wang, T. (2010). Genome-scale analysis and comparison of gene expression profiles in developing and germinated pollen in oryza sativa. BMC Genom. 11, 338. doi: 10.1186/1471-2164-11-338
Weisshaar, B., Armstrong, G. A., Block, A., da Costa e Silva, O., Hahlbrock, K. (1991). Light-inducible and constitutively expressed DNA-binding proteins recognizing a plant promoter element with functional relevance in light responsiveness. EMBO J. 10, 1777–1786. doi: 10.1002/j.1460-2075.1991.tb07702.x
Weltmeier, F., Ehlert, A., Mayer, C. S., Kietrich, K., Wang, X., Schütze, K., et al. (2006). Combinatorial control of arabidopsis proline dehydrogenase transcription by specific heterodimerisation of bZIP transcription factors. EMBO J. 25, 3133–3143. doi: 10.1038/sj.emboj.7601206
Weltmeier, F., Rahmani, F., Ehlert, A., Dietrich, K., Schutze, K., Wang, X., et al. (2009). Expression patterns within the arabidopsis C/S1 bZIP transcription factor network: availability of heterodimerization partners controls gene expression during stress response and development. Plant Mol. Biol. 69, 107–119. doi: 10.1007/s11103-008-9410-9
Wigge, P. A., Kim, M. C., Jaeger, K. E., Busch, W., Schmid, M., Lohmann, J. U., et al. (2005). Integration of spatial and temporal information during floral induction in arabidopsis. Science. 309, 1056–1059. doi: 10.1126/science.1114358
Wingender, E., Chen, X., Fricke, E., Geffers, R., Hehl, R., Liebich, I., et al. (2001). The TRANSFAC system on gene expression regulation. Nucleic Acids Res. 29, 281–283. doi: 10.1093/nar/29.1.281
Wingender, R., Rohrig, H., Horicke, C., Schell, J. (1990). Cis-regulatory elements involved in ultraviolet light regulation and plant defense. Plant Cell. 2, 1019–1026.
Xiang, C., Miao, Z., Lam, E. (1997). DNA-Binding properties, genomic organization and expression pattern of TGA6, a new member of the TGA family of bZIP transcription factors in arabidopsis thaliana. Plant Mol. Biol. 34, 403–415. doi: 10.1023/A:1005873500238
Xu, Z., Ali, Z., Xu, L., He, X., Huang, H., Yi, J., et al. (2016). The nuclear protein GmbZIP110 has transcription activation activity and plays important roles in the response to salinity stress in soybean. Sci. Rep. 6, 20366. doi: 10.1038/srep20366
Xu, C., Cao, H., Zhang, Q., Wang, H., Xin, W., Xu, E., et al. (2018). Control of auxin-induced callus formation by bZIP59-LBD complex in arabidopsis regeneration. Nat. Plants. 4, 108–115. doi: 10.1038/s41477-017-0095-4
Xu, L., Xu, Z., Liu, X., Huang, Y., He, X., Ma, H., et al. (2015). The subcellular localization and ectopic expression analysis in arabidopsis of soybean GmbZIP60 gene. J. Plant Biochem. Biotechnol. 24 (1), 9–17. doi: 10.1007/s13562-013-0228-4
Yanagisawa, S. (2014). Transcription factors involved in controlling the expression of nitrate reductase genes in higher plants. Plant Sci. 229, 167–171. doi: 10.1016/j.plantsci.2014.09.006
Yang, O., Popova, O. V., Süthoff, U., Lüking, I., Dietz, K. J., Golldack, D. (2009). The arabidopsis basic leucine zipper transcription factor AtbZIP24 regulates complex transcriptional networks involved in abiotic stress resistance. Gene. 436, 45–55. doi: 10.1016/j.gene.2009.02.010
Yang, Y., Yu, T. F., Ma, J., Chen, J., Zhou, Y. B., Chen, M., et al. (2020). The soybean bZIP transcription factor gene GmbZIP2 confers drought and salt resistances in transgenic plants. Int. J. Mol. Sci. 21, 670. doi: 10.3390/ijms21020670
Yoshida, T., Fujita, Y., Maruyama, K., Mogami, J., Todaka, D., Shinozaki, K., et al. (2015a). Four arabidopsis AREB/ABF transcription factors function predominantly in gene expression downstream of SnRK2 kinases in abscisic acid signalling in response to osmotic stress. Plant Cell Environ. 38 (1), 35–49. doi: 10.1111/pce.12351
Yoshida, T., Mogami, J., Yamaguchi-Shinozaki, K. (2015b). Omics approaches toward defining the comprehensive abscisic acid signaling network in plants. Plant Cell Physiol. 56, 1043–1052. doi: 10.1093/pcp/pcv060
Yoshida, K., Wakamatsu, S., Sakuta, M. (2008). Characterization of SBZ1, a soybean bZIP protein that binds to the chalcone synthase gene promoter. Plant Biotechnol. 25, 131–140. doi: 10.5511/plantbiotechnology.25.131
Yue, L., Li, X., Fang, C., Chen, L., Yang, H., Yang, J., et al. (2021). FT5a interferes with the Dt1-AP1 feed-back loop to control flowering time and shoot determinacy in soybean. J. Integr. Plant Biol. 63, 1004–1020. doi: 10.1111/jipb.13070
Zhang, M., Liu, Y., Cai, H., Guo, M., Chai, M., She, Z., et al. (2020). The bZIP transcription factor GmbZIP15 negatively regulates salt- and drought-stress responses in soybean. Int. J. Mol. Sci. 21, 7778. doi: 10.3390/ijms21207778
Keywords: soybean, transcription factor, bZIP, function, expression patterns
Citation: Yue L, Pei X, Kong F, Zhao L and Lin X (2023) Divergence of functions and expression patterns of soybean bZIP transcription factors. Front. Plant Sci. 14:1150363. doi: 10.3389/fpls.2023.1150363
Received: 24 January 2023; Accepted: 24 March 2023;
Published: 14 April 2023.
Edited by:
Chuanen Zhou, Shandong University, ChinaCopyright © 2023 Yue, Pei, Kong, Zhao and Lin. This is an open-access article distributed under the terms of the Creative Commons Attribution License (CC BY). The use, distribution or reproduction in other forums is permitted, provided the original author(s) and the copyright owner(s) are credited and that the original publication in this journal is cited, in accordance with accepted academic practice. No use, distribution or reproduction is permitted which does not comply with these terms.
*Correspondence: Xiaoya Lin, eGlhb3lhbGluQGd6aHUuZWR1LmNu; Lin Zhao, emhhb2xpbm5lYXVAMTI2LmNvbQ==
†These authors have contributed equally to this work