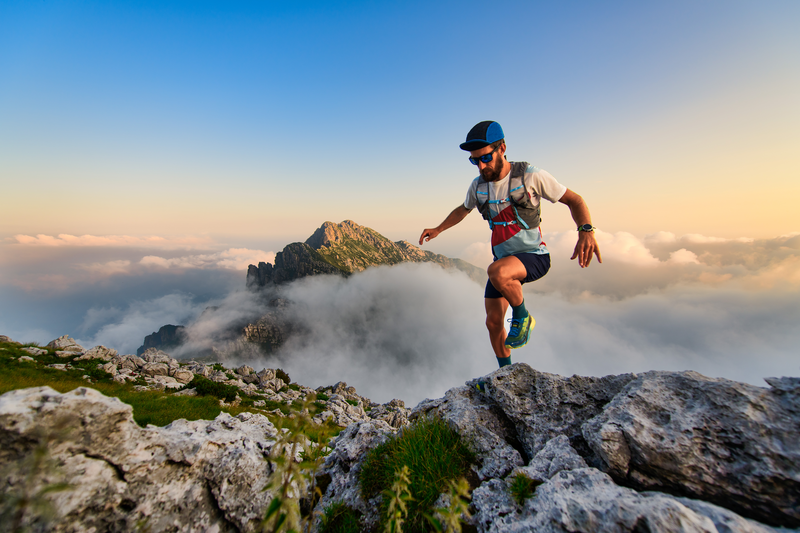
94% of researchers rate our articles as excellent or good
Learn more about the work of our research integrity team to safeguard the quality of each article we publish.
Find out more
EDITORIAL article
Front. Plant Sci. , 10 March 2023
Sec. Plant Bioinformatics
Volume 14 - 2023 | https://doi.org/10.3389/fpls.2023.1149469
This article is part of the Research Topic Harnessing Genebanks: High-Throughput Phenotyping and Genotyping of Crop Wild Relatives and Landraces View all 14 articles
Editorial on the Research Topic
Harnessing genebanks: High-throughput phenotyping and genotyping of crop wild relatives and landraces
Worldwide genebanks hold phenotypic and genetic novelty useful to increase yield, crop adaptability, and agrobiodiversity (Tanksley and McCouch, 1997) while buffering crop genetic erosion (Khoury et al., 2021). However, new strategies for genebank utilization must be empowered in order to meet increasing global food demand (McCouch, 2013; Bohra et al., 2021) with crop alternatives resilient to climate change, sustainable to the environment and the biodiversity, and profitable for communities (Scherer et al., 2020). Therefore, in order to contribute filling this gap on genebank mining, this Research Topic compiles recent developments able to speed up crop improvement processes by leveraging high-throughput phenotyping and genotyping of crop wild relatives (CWR) and landraces (Singh et al., 2022). As discussed in the next section, the amassed works innovate different steps of genebank characterization, utilization, and allelic deployment, including germplasm identification, conservation, pre-breeding screening for genepool diversity and associated markers, and introgression breeding.
Crop wild relatives are feasible sources for novel genetic and phenotypic variation (Bohra et al., 2021), as exemplified by Inagaki et al. The team demonstrated that a rice (Oryza sativa L.) near-isogenic line (NIL) that carries a genomic segment on chromosome seven from a Thai O. rufipogon CWR, narrowed by marker-assisted selection from a BC4F2 generation, is able to capture an optimum spectrum of plant shapes for sunlight receiving efficiency, which in turn maximizes vegetative growth and weed suppression. The historical crop-wild transition and genetic interchange during domestication also reinforces the value of wild genepools, as shown by Yuan et al. The team recovered loci responsible for seed coat color pattern during domestication of wild soybean [Glycine max (L.) Merr.] through whole-genome re-sequencing of 276 F10 recombinant inbred lines (RILs) derived from a cross between cultivated and wild soybean accessions. Phenotypic variations from CWR may also be retained in the offspring between cultivated and wild genepools, as demonstrated by Li et al. (2021) Specifically, they captured 31 agronomic-relevant QTL and allelic variation (e.g., for grain formation and length) from weedy rice (Oryza sativa f. spontanea) via whole-genome sequencing of a 199 F2 population obtained by crossing a weedy rice with low heterozygosity and a cultivated rice variety. These results by Inagaki et al. (2021), Yuan et al., and Li et al. (2021) exemplify the utilization of wild genetic resources to increase the genetic base of cultivated crops for sustainable and resilient agricultural production.
Landraces, having been selected under subsistence agricultural environments, also hold a broad representation of useful natural variation (McCouch, 2004). For instance, Osterman et al. used LASSO models to link environmental variation with adaptive genetic diversity in red clover (Trifolium pratense L.), a perennial temperate forage legume, through genotyping 382 accessions from Scandinavia, including landraces and CWR, with 661 single nucleotide polymorphism (SNP) markers derived from seqSNP-targeted sequencing. The genomic landscape of genetic variation was also unveiled by Mulugeta et al. The authors used a diversity panel of durum wheat (Triticum durum Desf.) collected from the Ethiopian highlands, and identified 44 genomic regions associated with grain yield and related traits using last-generation (i.e., FarmCPU) genome-wide association study (GWAS) models that inputted 10,045 SNP markers derived from the 25k Illumina wheat SNP array. In order to further explore isolated pockets of cryptic agrobiodiversity in Ethiopia, Enyew et al. and Gebeyehu et al. respectively examined the patterns of genetic diversity in sorghum [Sorghum bicolor (L.) Moench] and noug (Guizotia abyssinica), an outcrossing edible oilseed crop. The former team genotyped a total of 359 sorghum individuals, comprising 24 landrace accessions, with 3,001 gene-based single nucleotide polymorphism (SNP) markers, and found that sorghum accessions with bent peduncles exhibited more genetic variation than those with erect peduncles (Enyew et al.). The latter team performed RNA-Seq based transcriptome sequencing of 30 noug genotypes (Gebeyehu et al.), and predictably captured greater genetic similarity among self-compatible genotypes compared to self-incompatible accessions. Finally, Nuraga et al. introduced enset (Ensete ventricosum), a multipurpose crop grown in southern Ethiopia for human food, animal feed, and fiber. They demonstrated that enset landraces were not genetically differentiated based on their use-value (i.e., medicinal vs. non-medicinal landraces) through genotyping 51 accessions with 15 simple sequence repeat (SSR) markers. Altogether, the works by Osterman et al. (2022), Enyew et al. (2022), Gebeyehu et al., and Nuraga et al. demonstrate the potential of landraces as sources of genetic innovation for agrobiodiversity.
The latter study by Nuraga et al. also illustrates how CWR and landraces are being used for exotic non-conventional purposes (Von Wettberg et al., 2020). Following Nuraga et al., medicinal applications were also addressed in the work by Cui el al., using Gardenia (Gardenia jasminoides Ellis), a Chinese perennial shrub from the Rubiaceae family with edible flowers and medicinal fruits. The authors conducted syntenic analyses with model species within the Rubiaceae family (i.e., Coffea arabica, C. canephora, and Ophiorrhiza pumila), and discovered 18 and 31 QTL associated with growth- and leaf-related traits, respectively, by genotyping 200 F1 hybrids with 4,249 genotyping by sequencing (GBS)-derived SNP markers. The couple of studies by Nuraga et al. and Cui et al. provide fundamental knowledge that enables further exploration of alternative uses of CWR and landraces (Wu et al., 2022).
Meanwhile, hybridization has played a key role in bidirectional adaptive introgression (Abbott et al., 2013) as well as hybrid breeding within crop-wild complexes (Cortés et al., 2022a). For example, Volk et al. (2022) explored the signatures of admixture from the cultivated apple [Malus domestica (Suckow) Borkh] into the progenitor species M. sieversii (Ledeb.) M. Roem., M. orientalis Uglitzk., and M. sylvestris (L.) Mill. (Cornille et al., 2014) by genotyping 463 accessions using the 20K apple SNP array. On the other hand, Conejo-Rodriguez et al. leveraged hybrid phenotyping for pre-breeding in beans (Phaseolus spp.). They proposed an innovative pipeline to determine parental phenomic proportions in hybrids, and validated it using interspecific crosses derived from common bean (P. vulgaris L.), tepary bean (P. acutifolius A. Gray), and its wild relative P. parvifolius Freytag. The latter two served as donors of heat and drought tolerance (tepary, Buitrago-Bitar et al., 2021), and resistance to common bacterial blight (CBB), respectively (Conejo-Rodriguez et al.). Both studies by Volk et al. (2022) and Conejo-Rodriguez et al. (2022) highlight introgression breeding as a still valid alternative to bridge historical barriers between crops and their wild genepools (Labroo et al., 2021).
An additional study harnessing modern phenomic ‘big data’ tools in diverse genepools was reported by Restrepo-Montoya et al. They utilized qualitative descriptors to curate and catalogue cotton (Gossypium spp.) germplasm encompassing an impressive dataset of 1,616 observations between 2011 and 2019 on 7,941 unique accessions and 50 species. The last two pre-breeding phenotyping efforts carried out by Conejo Rodriguez et al. and Restrepo-Montoya et al. enable designing and targeting downstream goals in breeding by defining trait families, categorizing germplasm diversity, and pinpointing exotic outlier accessions.
Although great advancement has been made in the field of phenotyping, matching the exponential achievement from the genomic arena still requires more innovative analytical tools. Candidate platforms should be capable to merge and interpret complex multi-dimensional datasets embracing diversity from CWR and landraces. To this end, the review by Tirnaz et al. (2022) envisioned novel avenues to utilize genebank resources, for instance via de novo domestication, genome editing, and speed breeding. This review further advocated for computational (e.g., machine learning) approaches suitable for speeding up the incorporation of exotic genepools into breeding programs to improve crop production, adaptability and sustainability (Tirnaz et al.).
Crop wild relatives and landraces stored at genebanks harbor unique variation that may benefit food security, sustainability (Tanksley and McCouch, 1997) and resilient climate change adaptation (Renzi et al., 2022). Yet, their factual utilization has been hampered by poor characterizations, genetic incompatibilities, and polygenic variance (Coyne et al., 2020). Therefore, an improved roadmap to address these bottlenecks with modern technologies is a prerequisite for their effective utilization (Figure 1). A first pivotal research avenue worth considering is mining phenotypic and genetic variation hidden within CWR and landraces (Singh et al., 2022) through extended sampling targeting isolated pockets of cryptic diversity (Ramirez-Villegas et al., 2020), robust ecological data curation (Waldvogel et al., 2020b) [e.g., targeting specific abiotic stresses such as drought (Cortés and Blair, 2018) and heat tolerance (López-Hernández and Cortés)], dense linkage disequilibrium (LD) guided genomic characterizations (Blair et al., 2018), and geographic-wide agronomical (Osterman et al. 2022) and physiological (Conejo-Rodriguez et al.) trials across diverse germplasm and environments [recently referred to as enviromics (Costa-Neto and Fritsche-Neto, 2021; Crossa et al., 2021; Resende et al., 2021)].
Figure 1 Schematic roadmap to harness genebanks. Each box marks pivotal research avenues (with the corresponding recommendations within) worth considering for mining, unlocking and utilizing phenotypic and genetic novelty hidden within crop wild relatives (CWR) and landraces. Ultimately, achieving food security with resilient and sustainable agrifood systems capable to cope with climate change, biodiversity loss, and increased pollution would require nature-based solutions for climate-smart alimentary schemes inspired in underutilized plant species, CWR, and landraces.
Second, bridging crop–wild incompatibilities and unlocking novel diversity may rely on hybrid breeding via bridge genotypes (Conejo-Rodriguez et al. 2022), and introgression breeding (Migicovsky and Myles, 2017) from wild genepools into cultivars [e.g., Inagaki et al. (2021), Yuan et al. (2022), and Li et al. (2021)], and viceversa (Volk et al.). To overcome genotype incompatibility and embryo abortion issues, typically found in simpler backcrosses, pre-breeding schemes could be advanced into multiple alternating backcrosses between exotic donors and elite genepools (Burbano-Erazo et al., 2021), as in classical congruity backcrossing (Muñoz et al., 2003). Other promising alternatives to achieve a more optimal retention of the exotic phenotype include: embryo rescue, genomic-assisted backcrossing (Migicovsky and Myles, 2017), speed breeding (Watson et al., 2018; Alves et al., 2020; Bohra et al., 2021), tissue-specific gene editing [e.g., for exotic alleles (Martignago et al., 2019), de novo domestication (Lemmon et al., 2018), precocious flowering time, and compatibility factors (Fritsche et al., 2018)], and grafting with wild rootstocks [e.g., for biotic and abiotic stress tolerance (Warschefsky et al., 2016), adaptation to soil toxicity (Fernández-Paz et al., 2021), growth traits (Cañas-Gutiérrez et al., 2022), and yield (Reyes-Herrera et al., 2020)]. Merging these strategies could facilitate even quicker transitions from the wild genepools compared to classical inter-specific and crop-wild controlled pollination.
As a third step, major challenges to utilize CWR and landraces for improvement of complex polygenic adaptive traits (Renzi et al., 2022) can be tackled by linking last-generation experimental setups [e.g., diversifying selection (McCouch, 2004), introgression breeding (Muñoz et al., 2003; Burgarella et al., 2019), speed breeding (Watson et al., 2018; Alves et al., 2020; Kumar et al., 2020; Varshney et al., 2021b), de novo domestication (Fernie and Yan, 2019), and genome editing (Lemmon et al., 2018)] with modern analytical developments [e.g., last-generation genetic clustering (Enyew et al., 2022; Gebeyehu et al., 2022; Nuraga et al., 2022) and mapping Mulugeta et al. 2023, predictive breeding (Ahmar et al., 2021), genomic-informed selection (Desta and Ortiz, 2014; Crossa et al., 2017), and machine learning (Varshney, 2021; Tirnaz et al., 2022)]. Such trans-disciplinary approaches (Tirnaz et al., 2022) will enable better reconstructions of the genomic landscapes (Ellegren and Wolf, 2017; Barnaby et al., 2020; Tong and Nikoloski, 2021) of trait variation (Barnaby et al., 2022; Li et al.; Yuan et al.), and the selection signatures of past (Cortés et al., 2020; Waldvogel et al., 2020) and modern adaptation (Cortés et al., 2022b; Osterman et al., 2022). Retrieving complex polygenic interactions across the genomic architectures of quantitative (Boyle et al., 2017) and adaptive traits (Barrett and Hoekstra, 2011; Barghi et al., 2020) is a prerequisite to pinpoint exotic alleles enclosed within CWR and landraces (Cortés et al., 2012), and speed up the utilization of this natural variation as part of conservation and pre-breeding programs (Migicovsky and Myles, 2017).
Fourth, novel approaches need to de defined in order to leverage CWR and landraces (Varshney et al., 2021b; Tirnaz et al.). After all, boosting crop adaptability, sustainability, and yield in the face of changing climate, biodiversity loss, and increased pollution requires multilateral trans-disciplinary efforts. Various actors, including producers, scientist and transfer officers, decision makers, funding bodies and marketers should converge to plan and implement innovative strategies capable to meet future global food demands, while facing enlarged threats from abiotic (Blair et al., 2016; Cortés and López-Hernández, 2021) and biotic (Guevara-Escudero et al., 2021) pressures. Some of this pioneering strategies may include (1) prospection of genotypes candidate for exotic alleles (Migicovsky and Myles, 2017), ancient cultivars (Atchison et al., 2016; Thapa et al., 2021), and novel crops (Von Wettberg et al., 2020; Cui et al., 2022; Nuraga et al.); (2) multidimensional ‘big data’ compilation and management from the scientific, climatic and marketing arenas (Tirnaz et al., 2022) to identify conservation priorities (Castaneda-Alvarez et al., 2016), novel markets and value chains (Smale and Jamora, 2020), and (3) innovation for the production, transformation, delivery, commercialization and utilization of CWR- and landrace-derived crop varieties (McCouch, 2013) and ecosystem services (Tyack et al., 2020).
Further studies and implementation practices are urgently required to better harness high-throughput phenotyping and genotyping of CWR and landraces at genebanks. Ultimately, CWR and landraces can be utilized as resources to effectively explore phenotypic and genetic variants associated with innovative phenotypes, and to transfer those traits into customized cultivars (Varshney et al., 2021a). Alternatively, CWR and landraces may themselves be adopted as novel food sources (Von Wettberg et al., 2020). For these strategies to succeed, holistic multilateral and trans-disciplinary agendas should also prioritize an open-access networks approach (Spindel and McCouch, 2016) for mobilizing crop biodiversity (McCouch et al., 2016). On balance, embracing food security with resilient and sustainable crop systems requires equalizing multidimensional access of communities, minorities and vulnerable systems, including farmers from developing countries, women in agriculture, early-career researchers, global South-South partners, and orphan crops. Only then, underutilized plants, CWR and landraces would play an active role as nature-based solutions for agrifood systems.
AC conceived the Research Topic with insights from JB. Both authors made substantial contributions in preparing, editing and reviewing the contents of the Research Topic on “Harnessing Genebanks: High-Throughput Phenotyping and Genotyping of CWR and Landraces”. AC wrote a first version of the editorial, later edited by JB. Both approved it for publication.
Vetenskapsrådet (grants 2016-00418 and 2022-04411), Kungliga Vetenskapsakademien (grant BS20170036), and the British Council (grant 527023146) are deeply acknowledged for funding AJC as PI during the conception and execution of this Research Topic. The Graduate Research School in Genomic Ecology (GENECO) from Lund University is also recognized for the mobility funding that assisted the synergistic meeting between AC and M.W. Blair in the spring of 2015 at Nashville, TN, United States. Fulbright U.S. Specialist Program is thanked for supporting M.W. Blair’s visit to AC in Rionegro (Antioquia, Colombia) in the summer of 2019. Both encounters ultimately laid the conceptual foundations of this Research Topic.
The authors express their profound acknowledgment to all contributors, including authors, reviewers and assisting editors, who made possible this successful Research Topic. Discussions with M.W. Blair, I. Cerón-Souza, C.H. Galeano, F. López-Hernández, C.I. Medina, A.A. Navas-Arboleda, D. Peláez, P.H. Reyes-Herrera, A.P. Tofińo-Rivera, M. Urban, and R. Yockteng where insightful for some of the perspectives on genebank utilization discussed here. Recognition is also given to M.J. Torres for assistance during the preparation of this Research Topic. Finally, we are as well in debt with the editorial board of Frontiers in Plant Science and its staff for encouraging, assisting and enabling this Research Topic on “Harnessing Genebanks: High-Throughput Phenotyping and Genotyping of Crop Wild Relatives and Landraces”.
The authors declare that the research was conducted in the absence of any commercial or financial relationships that could be construed as a potential conflict of interest.
All claims expressed in this article are solely those of the authors and do not necessarily represent those of their affiliated organizations, or those of the publisher, the editors and the reviewers. Any product that may be evaluated in this article, or claim that may be made by its manufacturer, is not guaranteed or endorsed by the publisher.
Abbott, R., Albach, D., Ansell, S., Arntzen, J. W., Baird, S. J. E., Bierne, N., et al. (2013). Hybridization and speciation. J. Evolutionary Biol. 26, 229–246. doi: 10.1111/j.1420-9101.2012.02599.x
Ahmar, S., Ballesta, P., Ali, M., Mora-Poblete, F. (2021). Achievements and challenges of genomics-assisted breeding in forest trees: From marker-assisted selection to genome editing. Int. J. Mol. Sci. 22, 10583. doi: 10.3390/ijms221910583
Alves, F. C., Balmant, K. M., Resende, M. F. R., Kirst, M., Los Campos, G. (2020). Accelerating forest tree breeding by integrating genomic selection and greenhouse phenotyping. Plant Genome 13, e20048. doi: 10.1002/tpg2.20048
Atchison, G. W., Nevado, B., Eastwood, R. J., Contreras-Ortiz, N., Reynel, C., Madrinan, S., et al. (2016). Lost crops of the incas: Origins of domestication of the Andean pulse crop tarwi, Lupinus mutabilis. Am. J. Bot. 103, 1592–1606. doi: 10.3732/ajb.1600171
Barnaby, J. Y., Huggins, T. D., Lee, H., McClung, A. M., Pinson, S. R. M., Oh, M., et al. (2020). Vis/NIR hyperspectral imaging distinguishes sub-population, production environment, and physicochemical grain properties in rice. Sci. Rep. 10, 9284. doi: 10.1038/s41598-020-65999-7
Barnaby, J. Y., McClung, A. M., Edwards, J. D., Pinson, S. R. M., et al. (2022). Identification of quantitative trait loci for tillering, root, and shoot biomass at the maximum tillering stage in rice. Sci. Rep. 12, 13304. doi: 10.1038/s41598-022-17109-y
Barghi, N., Hermisson, J., SchlöTterer, C. (2020). Polygenic adaptation: A unifying framework to understand positive selection. Nat. Rev. Genet. 21, 769–781. doi: 10.1038/s41576-020-0250-z
Barrett, R. D. H., Hoekstra, H. E. (2011). Molecular spandrels: Tests of adaptation at the genetic level. Nat. Rev. Genet. 12, 767–780. doi: 10.1038/nrg3015
Blair, M. W., Cortes, A. J., Farmer, A. D., Huang, W., Ambachew, D., Penmetsa, R. V., et al. (2018). Uneven recombination rate and linkage disequilibrium across a reference snp map for common bean (Phaseolus vulgaris L.). PloS One 13, e0189597. doi: 10.1371/journal.pone.0189597
Blair, M. W., Cortés, A. J., This, D. (2016). Identification of an Erecta gene and its drought adaptation associations with wild and cultivated common bean. Plant Sci. 242, 250–259. doi: 10.1016/j.plantsci.2015.08.004
Bohra, A., Kilian, B., Sivasankar, S., Caccamo, M., Mba, C., Mccouch, S. R., et al. (2021). Reap the crop wild relatives for breeding future crops. Trends Biotechnol 40(4), 412-431. doi: 10.1016/j.tig.2021.08.002
Boyle, E. A., Li, Y. I., Pritchard, J. K. (2017). An expanded view of complex traits: From polygenic to omnigenic. Cell 169, 1177–1186. doi: 10.1016/j.cell.2017.05.038
Buitrago-Bitar, M. A., Cortés, A. J., López-Hernández, F., Londoño-Caicedo, J. M., Muñoz-Florez, J. E., Muñoz, L. C., et al. (2021). Allelic diversity at abiotic stress responsive genes in relationship to ecological drought indices for cultivated tepary bean, Phaseolus acutifolius A. Gray, and its wild relatives. Genes 12, 556. doi: 10.3390/genes12040556
Burbano-Erazo, E., León-Pacheco, R., Cordero-Cordero, C., López-Hernández, F., Cortés, A., Tofiño-Rivera, A. (2021). Multi-environment yield components in advanced common bean (Phaseolus vulgaris L.) × tepary bean (P. acutifolius A. Gray) interspecific lines for heat and drought tolerance. Agronomy 11, 1978. doi: 10.3390/agronomy11101978
Burgarella, C., Barnaud, A., Kane, N. A., Jankowski, F., Scarcelli, N., Billot, C., et al. (2019). Adaptive introgression: An untapped evolutionary mechanism for crop adaptation. Front. Plant Sci. 10, 4. doi: 10.3389/fpls.2019.00004
Cañas-Gutiérrez, G. P., Sepulveda-Ortega, S., López-Hernández, F., Navas-Arboleda, A. A., Cortés, A. J. (2022). Inheritance of yield components and morphological traits in avocado cv. Hass from “Criollo” “Elite trees” Via half-Sib seedling rootstocks. Front. Plant Sci. 13. doi: 10.3389/fpls.2022.843099
Castaneda-Alvarez, N. P., Khoury, C. K., Achicanoy, H. A., Bernau, V., Dempewolf, H., Eastwood, R. J., et al. (2016). Global conservation priorities for crop wild relatives. Nat. Plants 2, 16022. doi: 10.1038/nplants.2016.22
Cornille, A., Giraud, T., Smulders, M. J. M., Roldán-Ruiz, I., Gladieux, P. (2014). The domestication and evolutionary ecology of apples. Trends Genet. 30, 57–65. doi: 10.1016/j.tig.2013.10.002
Cortés, A. J., López-Hernańndez, F. (2021). Harnessing crop wild diversity for climate change adaptation. Genes 12, 783. doi: 10.3390/genes12050783
Cortés, A. J., Blair, M. W. (2018). Genotyping by sequencing and genome – environment associations in wild common bean predict widespread divergent adaptation to drought. Front. Plant Sci. 9, 128. doi: 10.3389/fpls.2018.00128
Cortés, A. J., Cornille, A., Yockteng, R. (2022a). Evolutionary genetics of crop-wild complexes. Genes 13, 1. doi: 10.3390/genes13010001
Cortés, A. J., López-Hernández, F., Blair, M. W. (2022b). Genome–environment associations, an innovative tool for studying heritable evolutionary adaptation in orphan crops and wild relatives. Front. Genet. 13, 910386. doi: 10.3389/fgene.2022.910386
Cortés, A. J., López-Hernández, F., Osorio-Rodriguez, D. (2020). Predicting thermal adaptation by looking into populations’ genomic past. Front. Genet. 11, 564515. doi: 10.3389/fgene.2020.564515
Cortés, A. J., This, D., Chavarro, C., Madriñán, S., Blair, M. W. (2012). Nucleotide diversity patterns at the drought-related Dreb2 encoding genes in wild and cultivated common bean (Phaseolus vulgaris L.). Theor. Appl. Genet. 125, 1069–1085. doi: 10.1007/s00122-012-1896-5
Costa-Neto, G., Fritsche-Neto, R. (2021). Enviromics: Bridging different sources of data, building one framework. Crop Breed. Appl. Biotechnol. 21, e393521S393512. doi: 10.1590/1984-70332021v21sa25
Coyne, C. J., Kumar, S., Von Wettberg, E. J. B., Marques, E., Berger, J. D., Redden, R. J., et al. (2020). Potential and limits of exploitation of crop wild relatives for pea, lentil, and chickpea improvement. Legume Sci. 2 (2), e36. doi: 10.1002/leg3.36
Crossa, J., Fritsche-Neto, R., Montesinos-Lopez, O. A., Costa-Neto, G., Dreisigacker, S., Montesinos-Lopez, A., et al. (2021). The modern plant breeding triangle: Optimizing the use of genomics, phenomics, and enviromics data. Front. Plant Sci. 12. doi: 10.3389/fpls.2021.651480
Crossa, J., Perez-Rodriguez, P., Cuevas, J., Montesinos-Lopez, O., Jarquin, D., De Los Campos, G., et al. (2017). Genomic selection in plant breeding: Methods, models, and perspectives. Trends Plant Sci. 22, 961–975. doi: 10.1016/j.tplants.2017.08.011
Desta, Z. A., Ortiz, R. (2014). Genomic selection: Genome-wide prediction in plant improvement. Trends Plant Sci. 19, 592–601. doi: 10.1016/j.tplants.2014.05.006
Ellegren, H., Wolf, J. B. W. (2017). Parallelism in genomic landscapes of differentiation, conserved genomic features and the role of linked selection. J. Evol. Biol. 30, 1516–1518. doi: 10.1111/jeb.13113
Fernández-Paz, J., Cortés, A. J., Hernández-Varela, C. A., Mejía-De-Tafur, M. S., Rodriguez-Medina, C., Baligar, V. C. (2021). Rootstock-mediated genetic variance in cadmium uptake by juvenile cacao (Theobroma cacao L.) genotypes, and its effect on growth and physiology. Front. Plant Sci. 12. 10.3389/fpls.2021.777842
Fernie, A. R., Yan, J. (2019). De novo domestication: An alternative route toward new crops for the future. Mol. Plant 12, 615–631. doi: 10.1016/j.molp.2019.03.016
Fritsche, S., Klocko, A. L., Boron, A., Brunner, A. M., Thorlby, G. (2018). Strategies for engineering reproductive sterility in plantation forests. Front. Plant Sci. 9, 1671. doi: 10.3389/fpls.2018.01671
Guevara-Escudero, M., Osorio, A. N., Cortés, A. J. (2021). Integrative pre-breeding for biotic resistance in forest trees. Plants 10, 2022. doi: 10.3390/plants10102022
Inagaki, N., Asami, H., Hirabayashi, H., Uchino, A., Imaizumi, T., Ishimaru, K. (2021). A rice ancestral genetic resource conferring ideal plant shapes for vegetative growth and weed suppression. Front. Plant Sci. 12, 748531. doi: 10.3389/fpls.2021.748531
Khoury, C. K., Brush, S., Costich, D. E., Curry, H. A., Haan, S., Engels, J. M. M., et al. (2021). Crop genetic erosion: Understanding and responding to loss of crop diversity. New Phytol. 233, 84–118. doi: 10.1111/nph.17733
Kumar, S., Hilario, E., Deng, C. H., Molloy, C. (2020). Turbocharging introgression breeding of perennial fruit crops: A case study on apple. Horticulture Res. 7, 47. doi: 10.1038/s41438-020-0270-z
Labroo, M. R., Studer, A. J., Rutkoski, J. E. (2021). Heterosis and hybrid crop breeding: A multidisciplinary review. Front. Genet. 12. doi: 10.3389/fgene.2021.643761
Lemmon, Z. H., Reem, N. T., Dalrymple, J., Soyk, S., Swartwood, K. E., Rodriguez-Leal, D., et al. (2018). Rapid improvement of domestication traits in an orphan crop by genome editing. Nat. Plants 4, 766–770. doi: 10.1038/s41477-018-0259-x
López-Hernández, F., Cortés, A. J. (2019). Last-generation genome–environment associations reveal the genetic basis of heat tolerance in common bean (Phaseolus vulgaris L.). Front. Genet. 10, 22. doi: 10.3389/fgene.2019.00954
Martignago, D., Rico-Medina, A., Blasco-Escamez, D., Fontanet-Manzaneque, J. B., Cano-Delgado, A. I. (2019). Drought resistance by engineering plant tissue-specific responses. Front. Plant Sci. 10, 1676. doi: 10.3389/fpls.2019.01676
McCouch, S. (2004). Diversifying selection in plant breeding. PloS Biol. 2, 1507–1512. doi: 10.1371/journal.pbio.0020347
McCouch, S. R., Wright, M. H., Tung, C. W., Maron, L. G., Mcnally, K. L., Fitzgerald, M., et al. (2016). Open access resources for genome-wide association mapping in rice. Nat. Commun. 7, 10532. doi: 10.1038/ncomms10532
Migicovsky, Z., Myles, S. (2017). Exploiting wild relatives for genomics-assisted breeding of perennial crops. Front. Plant Sci. 8, 460. doi: 10.3389/fpls.2017.00460
Muñoz, L. C., Blair, M. W., Duque, M. C., Tohme, J., Roca, W. (2003). Introgression in common bean X tepary bean interspecific congruity-backcross lines as measured by aflp markers. Crop Sci. 44, 637–645. doi: 10.2135/cropsci2004.6370
Ramirez-Villegas, J., Khoury, C. K., Achicanoy, H. A., Mendez, A. C., Diaz, M. V., Sosa, C. C., et al. (2020). A gap analysis modelling framework to prioritize collecting for ex situ conservation of crop landraces. Divers. Distrib 26, 730–742. doi: 10.1111/ddi.13046
Renzi, J. P., Coyne, C. J., Berger, J., Von Wettberg, E., Nelson, M., Ureta, S., et al. (2022). How could the use of crop wild relatives in breeding increase the adaptation of crops to marginal environments? Front. Plant Sci. 13. doi: 10.3389/fpls.2022.886162
Resende, R. T., Piepho, H. P., Rosa, G. J. M., Silva-Junior, O. B., Silva, F. F. E., Resende, M. D. V. D., et al. (2021). Enviromics in breeding: Applications and perspectives on envirotypic-assisted selection. Theor. Appl. Genet. 134, 95–112. doi: 10.1007/s00122-020-03684-z
Reyes-Herrera, P. H., Muñoz-Baena, L., Velásquez-Zapata, V., Patiño, L., Delgado-Paz, O. A., Díaz-Diez, C. A., et al. (2020). Inheritance of rootstock effects in avocado (Persea americana mill.) cv. hass. Front. Plant Sci. 11, 555071. doi: 10.3389/fpls.2020.555071
Scherer, L., Svenning, J. C., Huang, J., Seymour, C. L., Sandel, B., Mueller, N., et al. (2020). Global priorities of environmental issues to combat food insecurity and biodiversity loss. Sci. Total Environ. 730, 139096. doi: 10.1016/j.scitotenv.2020.139096
Singh, G., Gudi, S., Amandeep, Upadhyay, P., Shekhawat, P. K., Nayak, G., Goyal, L., et al. (2022). Unlocking the hidden variation from wild repository for accelerating genetic gain in legumes. Front. Plant Sci. 13. doi: 10.3389/fpls.2022.1035878
Smale, M., Jamora, N. (2020). Valuing genebanks. Food Secur. 12, 905–918. doi: 10.1007/s12571-020-01034-x
Spindel, J. E., McCouch, S. R. (2016). When more is better: How data sharing would accelerate genomic selection of crop plants. New Phytol. 212, 814–826. doi: 10.1111/nph.14174
Tanksley, S. D., McCouch, S. R. (1997). Seed banks and molecular maps: Unlocking genetic potential from the wild. Science 227, 1063–1066. doi: 10.1126/science.277.5329.1063
Thapa, R., Edwards, M., Blair, M. W. (2021). Relationship of cultivated grain amaranth species and wild relative accessions. Genes 12, 1849. doi: 10.3390/genes12121849
Tong, H., Nikoloski, Z. (2021). Machine learning approaches for crop improvement: Leveraging phenotypic and genotypic big data. J. Plant Physiol. 257, 153354. doi: 10.1016/j.jplph.2020.153354
Tyack, N., Dempewolf, H., Khoury, C. K. (2020). The potential of payment for ecosystem services for crop wild relative conservation. Plants 9, 1305. doi: 10.3390/plants9101305
Varshney, R. K. (2021). The plant genome special issue: Advances in genomic selection and application of machine learning in genomic prediction for crop improvement. Plant Genome 14(3), e20178. doi: 10.1002/tpg2.20178
Varshney, R. K., Barmukh, R., Roorkiwal, M., Qi, Y., Kholova, J., Tuberosa, R., et al. (2021a). Breeding custom-designed crops for improved drought adaptation. Advanced Genet. 2, e202100017. doi: 10.1002/ggn2.202100017
Varshney, R. K., Bohra, A., Roorkiwal, M., Barmukh, R., Cowling, W. A., Chitikineni, A., et al. (2021b). Fast-forward breeding for a food-secure world. Trends Genet. 37, 1124–1136. doi: 10.1016/j.tig.2021.08.002
Von Wettberg, E., Davis, T. M., Smýkal, P. (2020). Wild plants as source of new crops. Front. Plant Sci. 11. doi: 10.3389/fpls.2020.591554
Waldvogel, A. M., Feldmeyer, B., Rolshausen, G., Exposito-Alonso, M., Rellstab, C., Kofler, R., et al. (2020a). Evolutionary genomics can improve prediction of species’ responses to climate change. Evol. Lett. 4, 4–18. doi: 10.1002/evl3.154
Waldvogel, A. M., Schreiber, D., Pfenninger, M., Feldmeyer, B. (2020b). Climate change genomics calls for standardised data reporting. Front. Ecol. Evol. 8, 242. doi: 10.3389/fevo.2020.00242
Warschefsky, E. J., Klein, L. L., Frank, M. H., Chitwood, D. H., Londo, J. P., Von Wettberg, E. J. B., et al. (2016). Rootstocks: Diversity, domestication, and impacts on shoot phenotypes. Trends Plant Sci. 21, 418–437. doi: 10.1016/j.tplants.2015.11.008
Watson, A., Ghosh, S., Williams, M. J., Cuddy, W. S., Simmonds, J., Rey, M. D., et al. (2018). Speed breeding is a powerful tool to accelerate crop research and breeding. Nat. Plants 4, 23–29. doi: 10.1038/s41477-017-0083-8
Keywords: germplasm, ex situ conservation, food security, pre-breeding, exotic variation, elite varieties, polygenic variation, adaptation
Citation: Cortés AJ and Barnaby JY (2023) Editorial: Harnessing genebanks: High-throughput phenotyping and genotyping of crop wild relatives and landraces. Front. Plant Sci. 14:1149469. doi: 10.3389/fpls.2023.1149469
Received: 22 January 2023; Accepted: 26 January 2023;
Published: 10 March 2023.
Edited and Reviewed by:
Andrea Zuccolo, Kaust, Saudi ArabiaCopyright © 2023 Cortés and Barnaby. This is an open-access article distributed under the terms of the Creative Commons Attribution License (CC BY). The use, distribution or reproduction in other forums is permitted, provided the original author(s) and the copyright owner(s) are credited and that the original publication in this journal is cited, in accordance with accepted academic practice. No use, distribution or reproduction is permitted which does not comply with these terms.
*Correspondence: Andrés J. Cortés, YWNvcnRlc0BhZ3Jvc2F2aWEuY28=
†Secondary address: Andrés J. Cortés, Facultad de Ciencias Agrarias – Departamento de Ciencias Forestales, Universidad Nacional de Colombia – Sede Medellín, Medellín, Colombia
Disclaimer: All claims expressed in this article are solely those of the authors and do not necessarily represent those of their affiliated organizations, or those of the publisher, the editors and the reviewers. Any product that may be evaluated in this article or claim that may be made by its manufacturer is not guaranteed or endorsed by the publisher.
Research integrity at Frontiers
Learn more about the work of our research integrity team to safeguard the quality of each article we publish.