- 1College of Horticulture and Gardening, Yangtze University, Jingzhou, Hubei, China
- 2State Key Laboratory of Tea Plant Biology and Utilization, Anhui Agricultural University, Hefei, Anhui, China
- 3Department of Chemistry, Faculty of Science, University of Hradec Kralove, Hradec Kralove, Czechia
- 4ICAR-Central Citrus Research Institute, Nagpur, Maharashtra, India
- 5Botany and Microbiology Department, College of Science, King Saud University, Riyadh, Saudi Arabia
- 6Plant Production Department, College of Food and Agricultural Sciences, King Saud University, Riyadh, Saudi Arabia
The culturable endophytic fungus Serendipita indica has many beneficial effects on plants, but whether and how it affects physiological activities and phosphorus (P) acquisition of tea seedlings at low P levels is unclear. The objective of this study was to analyze the effects of inoculation with S. indica on growth, gas exchange, chlorophyll fluorescence, auxins, cytokinins, P levels, and expressions of two phosphate transporter (PT) genes in leaves of tea (Camellia sinensis L. cv. Fudingdabaicha) seedlings grown at 0.5 μM (P0.5) and 50 μM (P50) P levels. Sixteen weeks after the inoculation, S. indica colonized roots of tea seedlings, with root fungal colonization rates reaching 62.18% and 81.34% at P0.5 and P50 levels, respectively. Although plant growth behavior, leaf gas exchange, chlorophyll values, nitrogen balance index, and chlorophyll fluorescence parameters of tea seedlings were suppressed at P0.5 versus P50 levels, inoculation of S. indica mitigated the negative effects to some extent, along with more prominent promotion at P0.5 levels. S. indica inoculation significantly increased leaf P and indoleacetic acid concentrations at P0.5 and P50 levels and leaf isopentenyladenine, dihydrozeatin, and transzeatin concentrations at P0.5 levels, coupled with the reduction of indolebutyric acid at P50 levels. Inoculation of S. indica up-regulated the relative expression of leaf CsPT1 at P0.5 and P50 levels and CsPT4 at P0.5 levels. It is concluded that S. indica promoted P acquisition and growth in tea seedlings under P deficit conditions by increasing cytokinins and indoleacetic acid and CsPT1 and CsPT4 expression.
Introduction
Phosphorus (P) is an important mineral nutrient required for plant growth, and it is involved in many metabolic processes of plants, including carbon allocation, energy transfer, photosynthesis, and respiration (Taghinasab et al., 2018). Insufficient P concentrations in plants negatively affect their metabolisms, leading to the reduction of plant productivity (Taghinasab et al., 2018). Plants can absorb inorganic phosphate from the soil around the roots through phosphate transporters (PTs) (Liao et al., 2019). The P in the soil is easily fixed, which limits the uptake of P by the roots. The uptake of soil P by the roots cannot meet the needs of plant growth (Vance et al., 2003; Wu F et al., 2019). Plants can establish a symbiotic relationship with soil arbuscular mycorrhizal fungi (AMF) in roots (Zou et al., 2021; Wang et al., 2023), in which the mycorrhizal pathway helps plants to acquire P from the soil (Deng et al., 2022; Sun RT et al., 2022). However, as obligate symbiotic fungi, AMF is unable to reproduce rapidly in vitro without plant roots, thereby limiting its application on a large scale in the field.
Serendipita indica (Sav. Verma, Aj. Varma, Rexer, G. Kost & P. Franken) M. Weiß, Waller, A. Zuccaro & Selosse (earlier Piriformospora indica) is an endophytic fungus isolated from the rhizosphere of desert plants in India (Cao MA et al., 2022), and S. indica can be cultured in vitro without plant roots (Yang et al., 2020). The fungus has important benefits in P uptake and growth of host plants (Loha et al., 2018; Yang L et al., 2021; Cao MA et al., 2022; Liu et al., 2022). In addition, S. indica has many positive effects on promoting water absorption, enhancing stress resistance, and increasing crop yield (Saddique et al., 2018; Bakhshandeh et al., 2020; Tyagi et al., 2022). Similar to AMF, S. indica also promotes plant growth performance. Wang et al. (2022) found that S. indica changed the pH value and phosphatase activities of cadmium-contaminated soil to improve the photosynthesis of soybean. Similarly, Liu BH et al. (2021) found that S. indica promoted chlorophyll content, fluorescence parameters, and antioxidant enzyme activities to improve the growth performance of walnut plants under drought stress. S. indica could establish the symbiosis with the roots of Pleurotus orientalis, which thus improved root architecture, net CO2 assimilation, and light use efficiency (Wu C et al., 2019). In addition, S. indica promoted soil acidic, neutral, alkaline, and total phosphatase activities of trifoliate orange seedlings, thus improved P acquisition (Yang L et al., 2021). Field inoculation experiments also showed that S. indica could promote the accumulation of glucose, fructose and sucrose as well as P concentrations in Newhall Navel orange fruits (Cheng et al., 2022). It means that S. indica could bring benefits to plants in many ways.
Tea (Camellia sinensis L.), an important cash crop in the world, is mainly planted in acidic soil and is often limited by soil P deficiency (Zheng et al., 2012; Wang et al., 2015). Studies have found that AMF improved root system architecture of tea plants and up-regulated the expression of CsPT1 and CsPT4 to promote the absorption of P and plant growth (Shao et al., 2021). The inability of AMF to propagate on a large scale limits its use in the field, whereas S. indica presents AMF-like properties and can be cultured in vitro, thus having a strong potential for large-scale application in the field (Yang L et al., 2021; Cao MA et al., 2022). At present, it is not clear whether S. indica has a positive effect on plant growth and P acquisition of tea plants, and what the underlying mechanism of the effect is.
The present study tried to analyze the effects of S. indica inoculation on the growth, leaf gas exchange, chlorophyll fluorescence parameters, nitrogen balance index, phytohormone levels, leaf P concentrations, and PT gene expression of tea plants grown at two P levels, in order to uncover the mechanism of S. indica promoting P acquisition of tea plants and the potential application of S. indica in field tea cultivation.
Materials and methods
Fungal inoculums
S. indica was provided by Professor Tian, College of Life Sciences, Yangtze University (Jingzhou, China). Fungal mass of S. indica were inoculated into solid potato glucose medium and incubated at 23 °C for 3 weeks. After the mycelium covered the medium, a small amount of sterile water was added. Subsequently, the fungus on the surface of the medium was transferred to another triangular flask using a sterile glass rod, with 500 mL sterile water to obtain a spore suspension of S. indica. The spore suspension was mixed with distilled water at a ratio of 1: 20 as the next fungal inoculums, in which the number of spores was calculated using a spectrophotometer and the concentration was 2.81 × 109 CFU (colony forming units)/mL.
Plant culture
Seeds of C. sinensis L. cv. Fudingdabaicha were provided by the Tea Research Institute, Guizhou Academy of Agricultural Science (Guiyang, China). The seeds were disinfected with 75% ethanol for 10 minutes and washed with sterile water 3 times. Then the seeds were germinated in autoclaved (121°C, 0.12 MPa, 1 h) sand in an incubator with a diurnal temperature of 28°C/20°C. After 4 weeks, the tea seedlings having two leaves were transplanted into plastic pots with an upper diameter of 18 cm, a bottom diameter of 11 cm, and a height of 15 cm. The potted substrate was an autoclaved (121°C, 0.12 MPa, 2 h) mixture of the soil and sand, with a volume ratio of 3:1. The soil characteristics were pH 5.9, Olsen-P 10.4 mg/kg, available K 38.6 mg/kg, and soil organic carbon 8.0 mg/g. Each pot contained 1.2 kg of autoclaved substrates.
S. indica inoculations were performed at the time of transplanting, where S. indica-inoculated treatment was arranged as 50 mL of above spore suspension per pot. The treatment without S. indica inoculation was applied with an equal amount of autoclaved (121°C, 0.12 MPa, 2 h) spore suspension.
Two P levels including 0.5 μM and 50 μM P were arranged according to Shao et al. (2021). P concentrations were achieved by varying the KH2PO4 levels in the Hoagland solutions, and the details had been described in Shao et al. (2021). One week after S. indica inoculation, P treatments were performed, where each pot received 80 mL of designed Hoagland solutions, once every two days, for a total of six applications.
All potted seedlings were placed in a greenhouse with a photo flux density of 948 μmol/m2/s, diural-night temperature of 28°C/23°C, and relative air humidity of 67%. During the experiment, each pot received 50 mL distilled water per day on non-P-treated days. The position of the experimental pots was moved weekly to avoid differences in the surrounding environment. The experiment lasted for 16 weeks from May to September 2021.
Experimental design
This experiment consisted of two factors, including two P treatments (0.5 μM and 50 μM P) as well as two fungal inoculation treatments (S. indica inoculation and uninoculation). A total of four treatments were arranged, namely, (i) tea seedlings inoculated without S. indica under 0.5 μM P conditions (P0.5-Si), (ii) tea seedlings inoculated with S. indica under 0.5 μM P conditions (P0.5+Si), (iii) tea seedlings inoculated without S. indica under 50 μM P conditions (P50-Si), and (iv) tea seedlings inoculated with S. indica under 50 μM P conditions (P50+Si), respectively. Each treatment replicated eight times, in a total of 32 pots, in a completely randomized arrangement.
Measurements of growth and root fungal colonization rate
Plant height, stem diameter, leaf number, shoot biomass, and root biomass were measured at plant harvest. The root fungal colonization was stained as per the protocol described by Yang L. et al. (2021), in which 1-cm root segments were incubated with 10% KOH solution at 90°C for 2 h, bleached with 10% hydrogen peroxide solution for 15 min, acidified with 0.2 mol/L HCl solution for 10 min, and stained with 0.05% trypan blue in lactophenol solution for 35 s. The fungal colonization of roots was observed under a microscope, and then the percentage of the number of fungus-colonized root segments versus the total number of observed root segments was set as the root fungal colonization rate.
Measurements of leaf gas exchange, nitrogen balance index, and chlorophyll fluorescence parameters
In the morning of a sunny day before harvest, leaf gas exchange parameters, including net photosynthetic rate (Pn), intercellular CO2 concentration (Ci), stomatal conductance (Gs), and transpiration rate (Tr), were determined by a Li-6400 photosynthetic apparatus (LI-COR Inc., Lincoln, NE, USA) according to the user’s guide. Then, leaves were measured by a portable Plant Polyphenol-Chlorophyll Instrument (Dualex, Force-A company of France, Orsay, France), and chlorophyll index (Chl) and nitrogen balance index (Nbi) were obtained.
Chlorophyll fluorescence parameters including Fv/Fm_Lss (the maximum efficiency of PSII), QY_max (the maximum mass quantum yield), QY_Lss (the minimum quantum yield) and NPQ_Lss (the non-photochemical quenching) were determined in leaves by a high-throughput plant fluorescence phenotype monitoring platform (RAP-FLUO) of Gufeng Optoelectronic Co., Ltd., Wuhan, Hubei, China.
Measurement of leaf P and endogenous hormones
A 0.3 g dry sample of leaves was digested with nitric acid/hydrogen peroxide (Wheal et al., 2011) and centrifuged at 300×g/min for 5 min. The supernantant was fixed and diluted to determine leaf P concentrations the inductively coupled plasma optical emission spectrometry (ICP-OES) (IRIS Advantage, Thermo, Waltham, MA, USA).
According to the protocol of Liu RC et al. (2021), indole acetic acid (IAA), indole butyric acid (IBA), dihydrozetin (DZ), isopentenyladenine (IP), and trans-zetin (TZ) were extracted from leaves and analyzed by the High Performance Liquid Chromatograph (LC-100, Shanghai Precision Instruments, Shanghai, China) (Chen et al., 2009) with a C18 column (250 mm × 4.6 mm × 5 μm), 0.8 mL/min flow rate through the extraction column, 35°C of the column temperature, 40 minutes of the detection time, and 254 nm of the detection wavelength.
Analysis of relative expression of two PT genes in leaves
The 0.1 g leaf fresh samples were ground in liquid nitrogen, and total RNA was extracted using the TaKaRa MiniBEST Plant RNA Extraction Kit. The primeScript™ RT kit and gDNA eraser were used for reverse transcription of RNA. We selected two high-affinity PT genes, including CsPT1 and CsPT4 as per the results of Xin et al. (2017). Their primers were designed based on the online Genbank (http://www.ncbi.nlm.nih.gov/genbank/) and shown in the Supplementary Table S1. The expression of CsPT1 and CsPT4 in leaves was measured by quantitative real-time fluorescence PCR (qRT-PCR), and the relative gene expression was calculated using the method described by Livak and Schmittgen (2001), using the GADPH as an internal reference gene (Xin et al., 2017). The data were normalized to the expression of tea plants uninoculated with S. indica grown at the P0.5 level.
Data analysis
Data were analyzed using the two-way analysis of variance (ANOVA) by SAS software (8.1v; SAS Institute Inc., Cary, NC, USA), and significant differences between treatments were compared with Duncan’s multiple range tests at P < 0.05.
Results
Changes in root fungal colonization rate
No fungal colonization was found in the roots of tea seedlings uninoculated with S. indica, while the roots of tea seedlings inoculated with S. indica were colonized to varying degrees (Figure 1A), with fungal colonization rates ranging from 62.18% at P0.5 levels to 81.34% at P50 levels (Table 1). The root fungal colonization rate under P50 conditions was 0.31-fold significantly higher than under P0.5 conditions. There was a significant interaction in root fungal colonization rate between S. indica inoculations and P treatment.
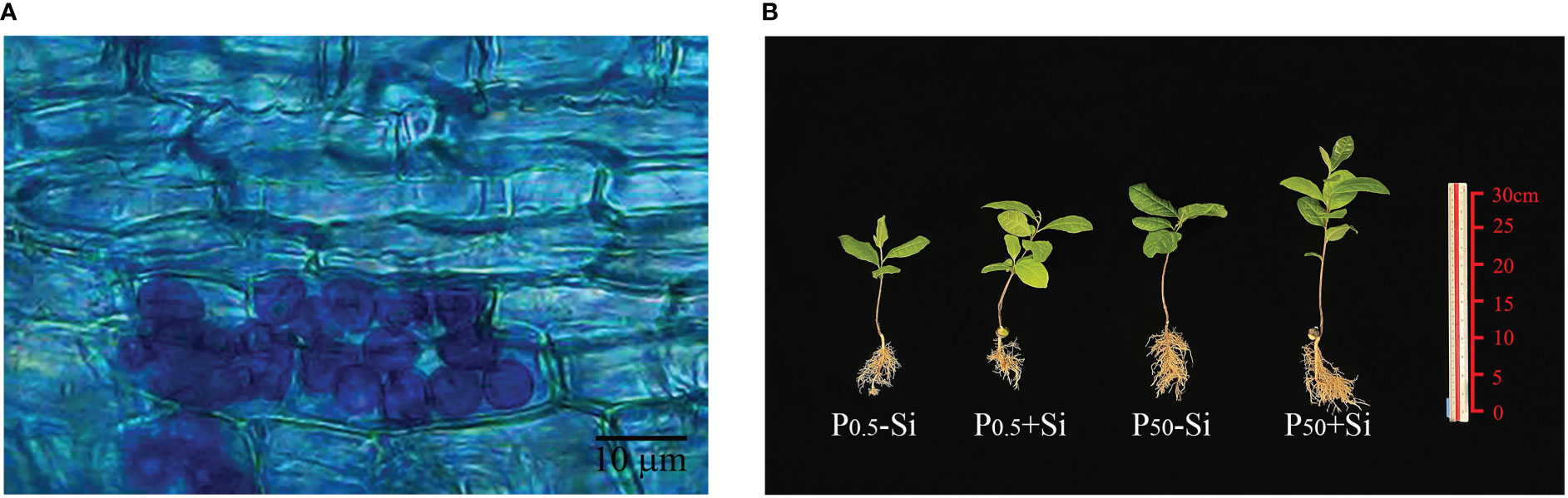
Figure 1 Root colonization of Serendipita indica (A) and plant growth responses (B) of tea seedlings inoculated with S. indica at P0.5 and P50 levels. The abbreviations are shown in Table 1.
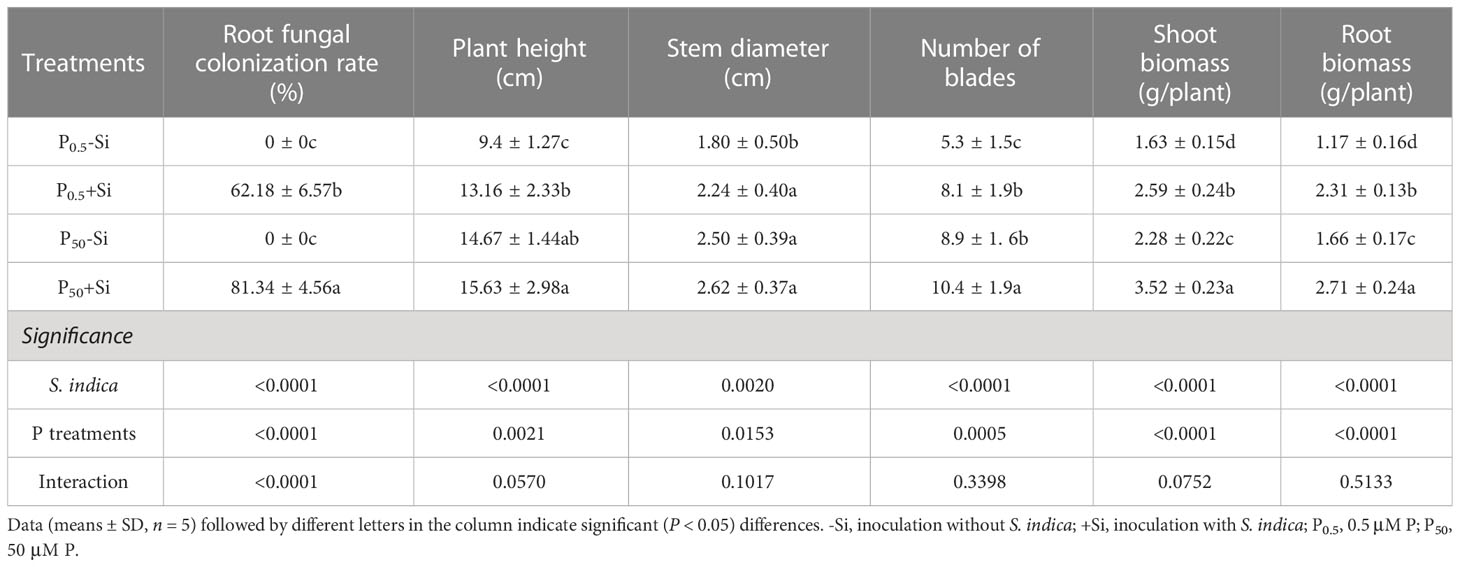
Table 1 Effects of inoculation with Serendipita indica on root fungal colonization and plant growth parameters of tea seedlings at P0.5 and P50 levels.
Changes in plant growth behavior
Plant growth behavior including plant height, stem diameter, leaf number, shoot biomass, and root biomass was higher at P50 levels than at P0.5 levels (Figure 1B; Table 1). In addition, compared with S. indica uninoculation, S. indica inoculation significantly increased leaf number, shoot biomass, and root biomass by 17.00%, 54.39%, and 63.25% at P50 levels, respectively, along with no significant change in plant height and stem diameter (Table 1). Nevertheless, S. indica inoculation significantly elevated plant height, stem diameter, leaf number, shoot biomass, and root biomass by 40.00%, 24.44%, 52.16%, 58.90%, and 97.44% at P0.5 levels, respectively, compared with non-S. indica inoculation. Significant interaction did not appear on these growth variables.
Changes in leaf gas exchange
P0.5 treatment significantly inhibited leaf Pn, Gs, Ci, and Tr, regardless of S. indica inoculation or not, compared with P50 treatment (Table 2). However, S. indica inoculation significantly improved these gas exchange variables. At the P0.5 level, S. indica significantly increased leaf Pn and Tr by 25.13% and 78.26%, respectively; at the P50 level, S. indica significantly elevated leaf Pn, Gs, and Tr by 56.40%, 69.45% and 132.14%, respectively, compared with non-S. indica treatment. Significant interaction between S. indica inoculation and P treatments appeared on Pn, Gs, and Tr.
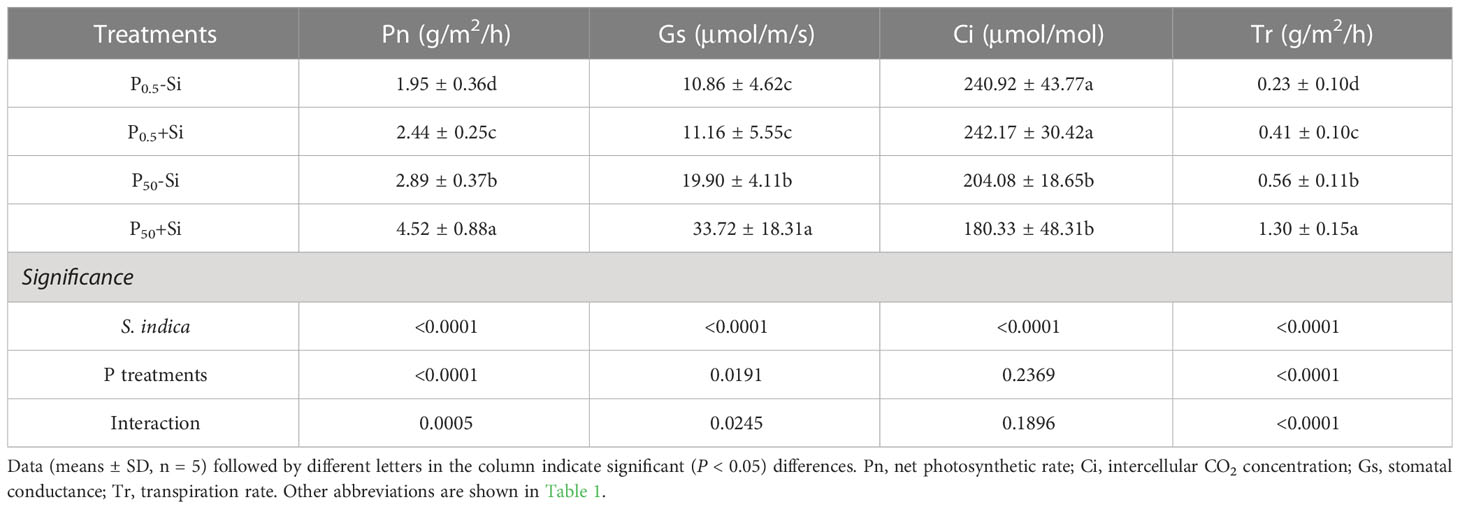
Table 2 Effects of inoculation with Serendipita indica on leaf gas exchange parameters of tea seedlings at P0.5 and P50 levels.
Changes in leaf Chl and Nbi
P0.5 treatment significantly inhibited leaf Chl and Nbi of inoculated and uninoculated tea plants, compared with P50 treatment: 47.59% and 98.03% lower in inoculated seedlings and 49.94% and 92.29% lower in uninoculated seedlings, respectively (Figures 2A, B). Compared with inoculation without S. indica, Nbi and Chl after S. indica inoculation significantly increased by 28.94% and 16.05% under P50 conditions and by 25.21% and 17.90% under P0.5 conditions, respectively. No significant interaction occurred in Chi and Nbi (Table 3).
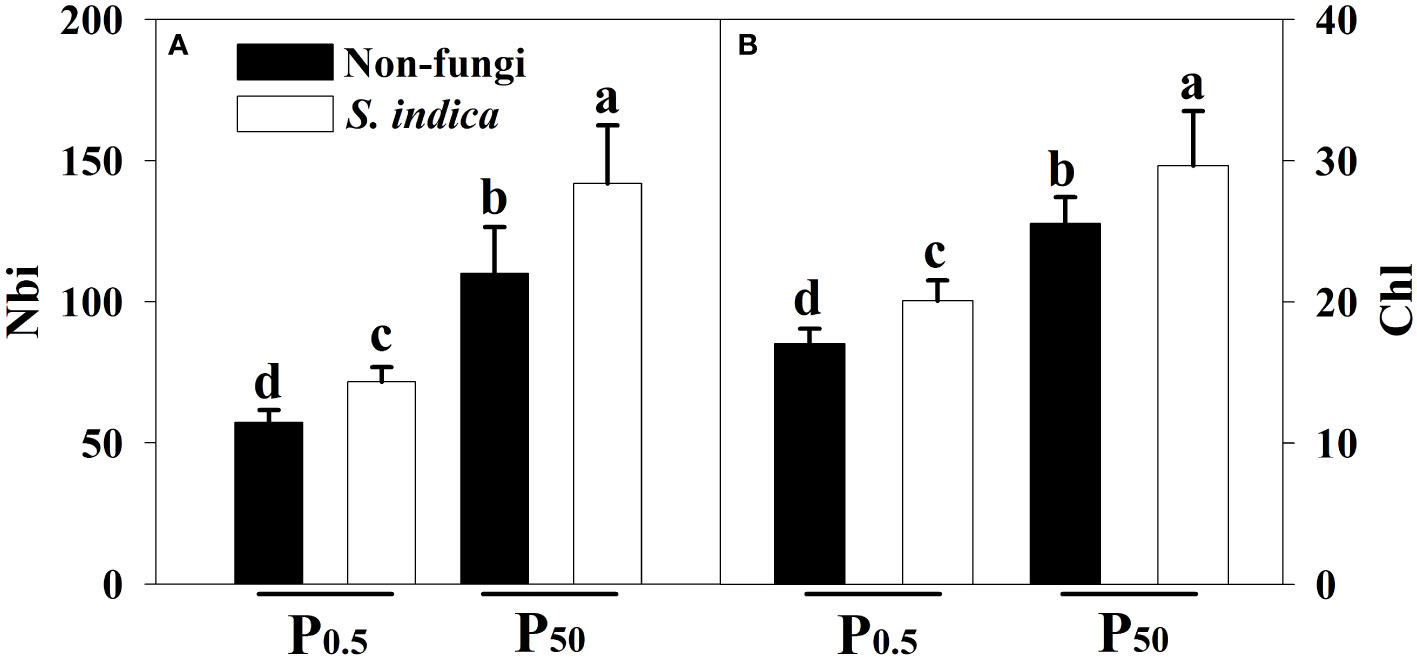
Figure 2 Effects of inoculation with Serendipita indica on nitrogen balance index (Nbi) (A) and leaf chlorophyll index (Chl) (B) of tea seedlings at P0.5 and P50 levels. Data (means ± SD, n = 5) are significantly (P < 0.05) different if followed by different letters above the bars. The abbreviations are shown in Table 1.
Changes in leaf chlorophyll fluorescence parameters
P0.5 treatment significantly suppressed Fv/Fm_Lss, QY_max, and QY_Lss in uninoculated seedlings, compared to P50 treatment (Figures 3A–D). Similarly, inoculated plants showed lower Fv/Fm_Lss and QY_Lss while higher NPQ_Lss at the P0.5 level than at the P50 level. Compared with the uninoculated treatment, inoculation with S. indica showed a significant decrease in NPQ_Lss by 26.47% at P50, coupled with no significant changes in Fv/Fm_Lss, QY_max, and QY_Lss; Fv/Fm_Lss and QY_Lss increased significantly by 37.5% and 71.43% at P0.5 after inoculation with S. indica, but QY_ max and NPQ_Lss did not change significantly. A significant interaction occurred in Fv/Fm_Lss (Table 3).
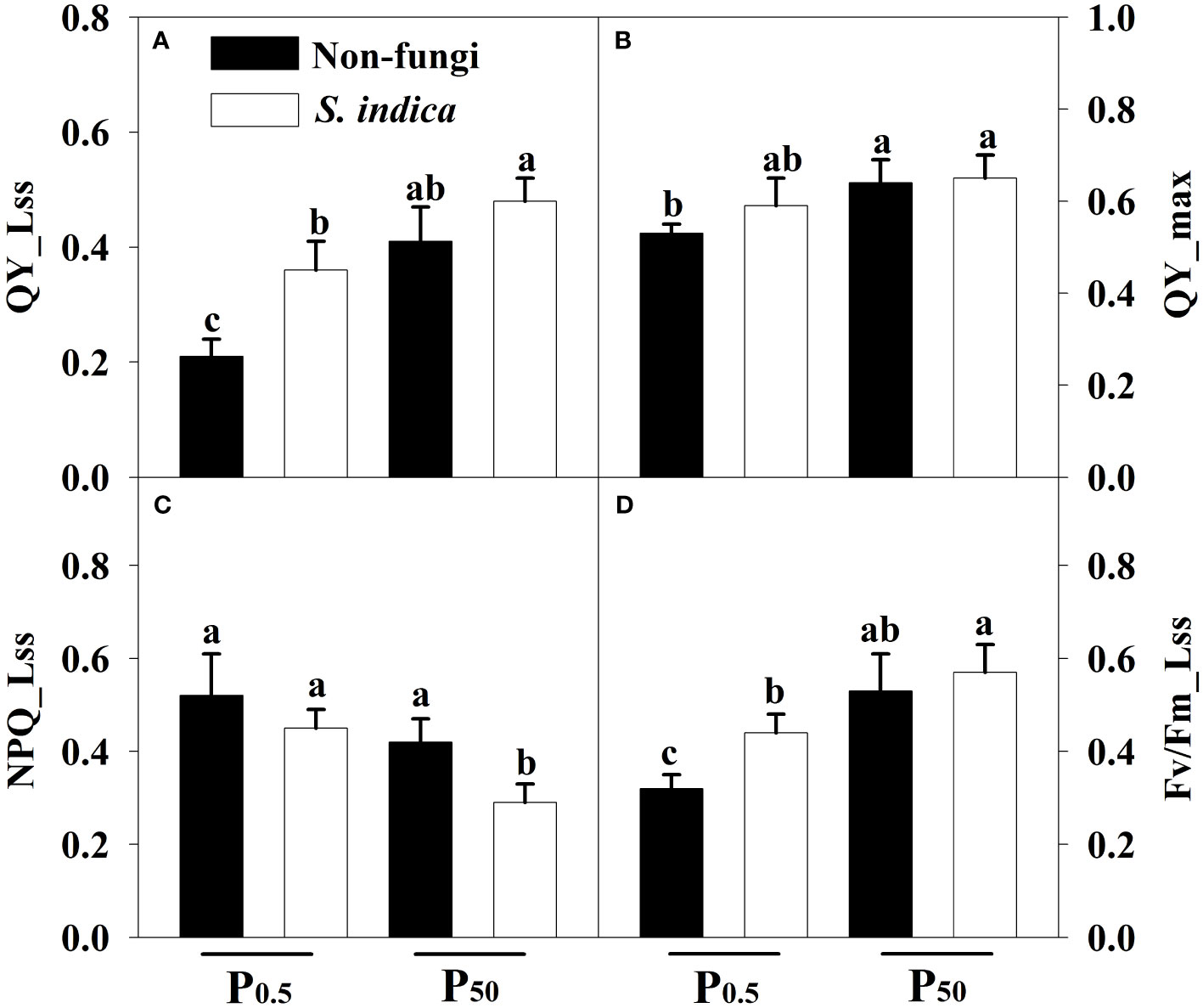
Figure 3 Effects of inoculation with Serendipita indica on leaf QY_Lss (A), QY_max (B), NPQ_Lss (C), and Fv/Fm_Lss (D) of tea seedlings at P0.5 and P50 levels. Data (means ± SD, n = 5) are significantly (P < 0.05) different if followed by different letters above the bars. Fv/Fm_Lss, the maximum efficiency of PSII; QY_max, the maximum mass quantum yield; QY_Lss, the minimum quantum yield; NPQ_Lss, the non-photochemical quenching. Other abbreviations are shown in Table 1.
Changes in leaf endogenious hormones
Compared with the P50 treatment, the P0.5 treatment significantly increased IAA levels in leaves of uninoculated seedlings by 18.57% and decreased leaf IBA and TZ levels by 5.22% and 13.38%, respectively, along with no significant difference in leaf IP and DZ levels (Figures 4A–E). Similarly, compared with the P50 treatment, the P0.5 treatment significantly increased leaf IP, DZ and IAA levels of inoculated seedlings by 13.94%, 4.19% and 12.99%, respectively, and decreased leaf TZ levels by 27.44%, coupled with no significant change in leaf IBA levels. At the P0.5 level, inoculation of S. indica significantly increased leaf IP, DZ, TZ, and IAA levels by 8.36%, 4.59%, 13.46%, and 4.82%, respectively, accompanied by no significant change in leaf IBA levels, as compared with uninoculation of S. indica. At the P50 level, inoculation of S. indica significantly increased leaf TZ and IAA levels by 27.53% and 10.00%, respectively, but significantly decreased leaf IP and IBA levels by 4.04% and 9.61%, with no significant effect on leaf DZ levels, as compared with uninoculation of S. indica. In addition, there was a significant interaction at the IP, TZ and IBA levels between S. indica inoculation and P treatments (Table 3).
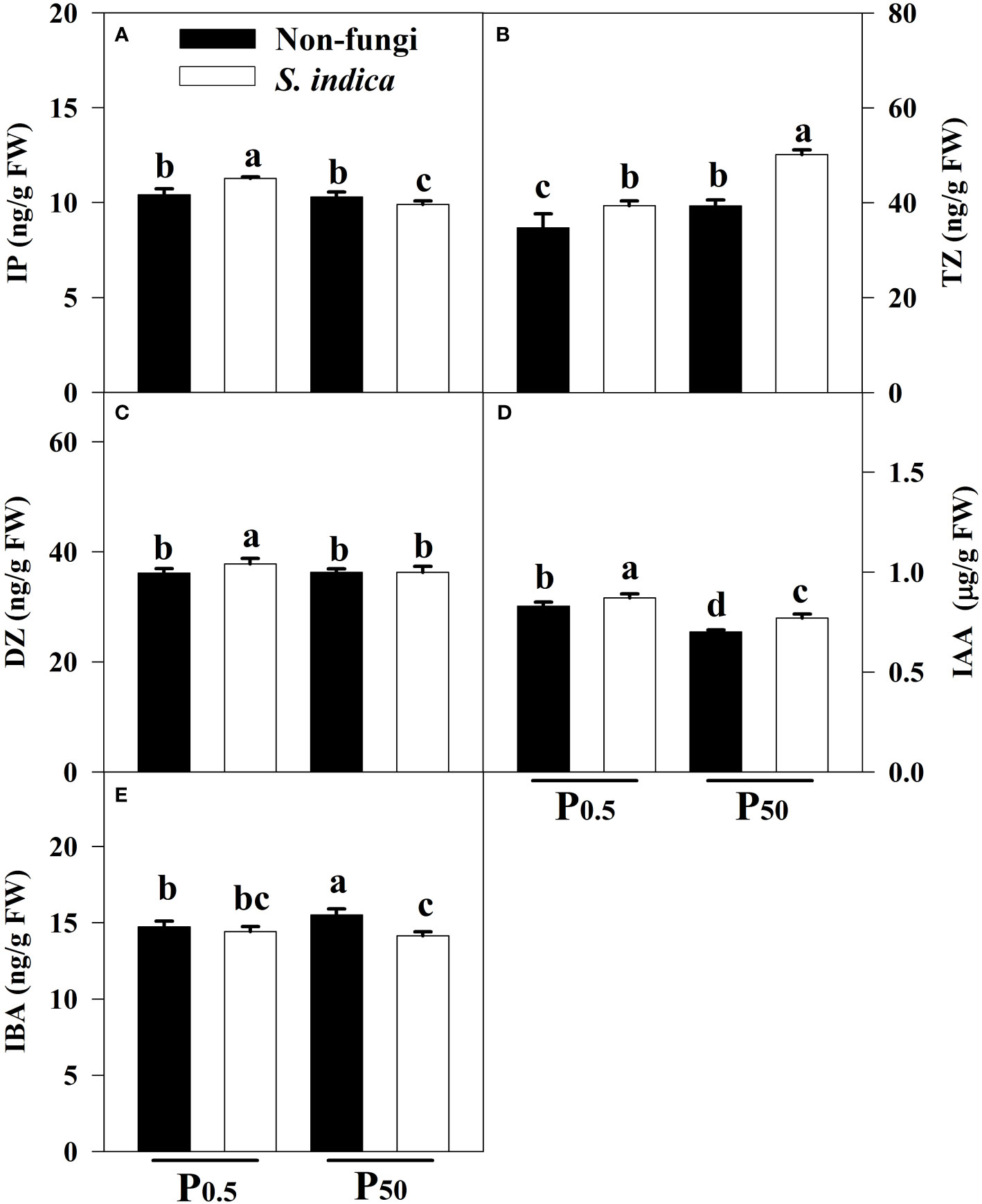
Figure 4 Effects of inoculation with Serendipita indica on leaf IP (A), TZ (B), DZ (C), IAA (D), and IBA (E) of tea seedlings at P0.5 and P50 levels. Data (means ± SD, n = 5) are significantly (P < 0.05) different if followed by different letters above the bars. IAA, indole acetic acid; IBA, indole butyric acid; DZ, dihydrozetin; IP, isopentenyladenine; TZ, trans-zetin. Other abbreviations are shown in Table 1.
Changes in leaf P concentrations
P0.5 treatment significantly reduced leaf P concentrations by 57.33% in uninoculated plants and 38.53% in inoculated plants, respectively, compared with P50 treatment (Figure 5). Additionally, compared with non-S. indica inoculation, S. indica inoculation significantly increased leaf P concentrations by 27.97% in P50 and by 45.33% in P0.5, respectively. Significant interaction did not appear on leaf P concentrations (Table 3).
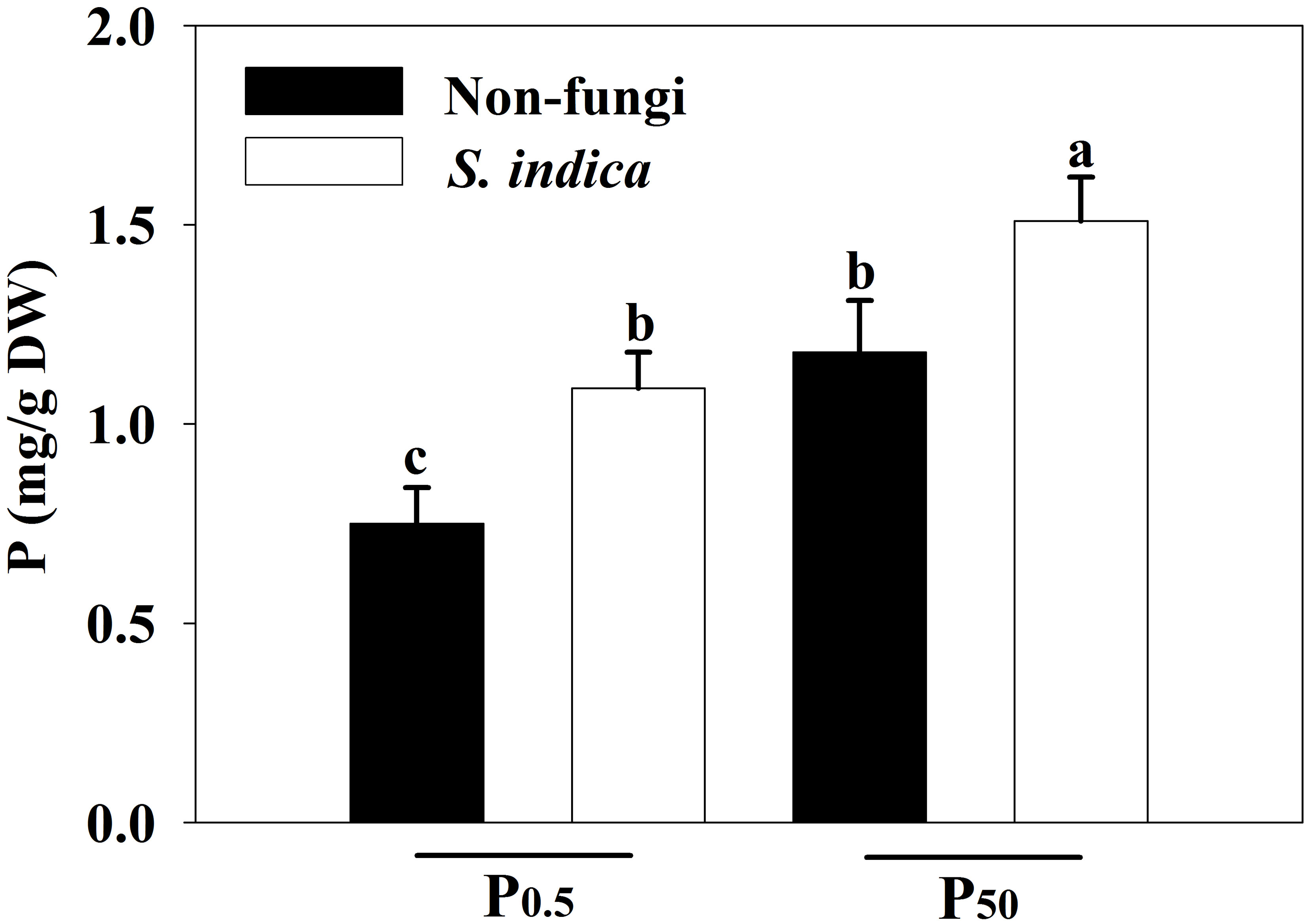
Figure 5 Effects of inoculation with Serendipita indica on leaf P concentrations of tea seedlings at P0.5 and P50 levels. Data (means ± SD, n = 5) are significantly (P < 0.05) different if followed by different letters above the bars. P, phosphorus. Other abbreviations are shown in Table 1.
Changes in leaf CsPTs expression
The expression of CsPT1 and CsPT4 genes in leaves of tea seedlings was regulated by soil P levels and S. indica (Figures 6A, B). Compared with the P50 treatment, the P0.5 treatment significantly induced the up-regulated expression of CsPT1, but suppressed the expression of CsPT4, irrespectively of S. indica inoculation or not. On the other hand, inoculation of S. indica significantly increased the expression of leaf CsPT1 and CsPT4 under P0.5 conditions by 0.16- and 0.17-fold, respectively. However, inoculation of S. indica under P50 conditions only up-regulated leaf CsPT1 expression by 0.31-fold, plus no significant change in CsPT4 expression. Significant interaction did not appear on the two CsPTs expression (Table 3).
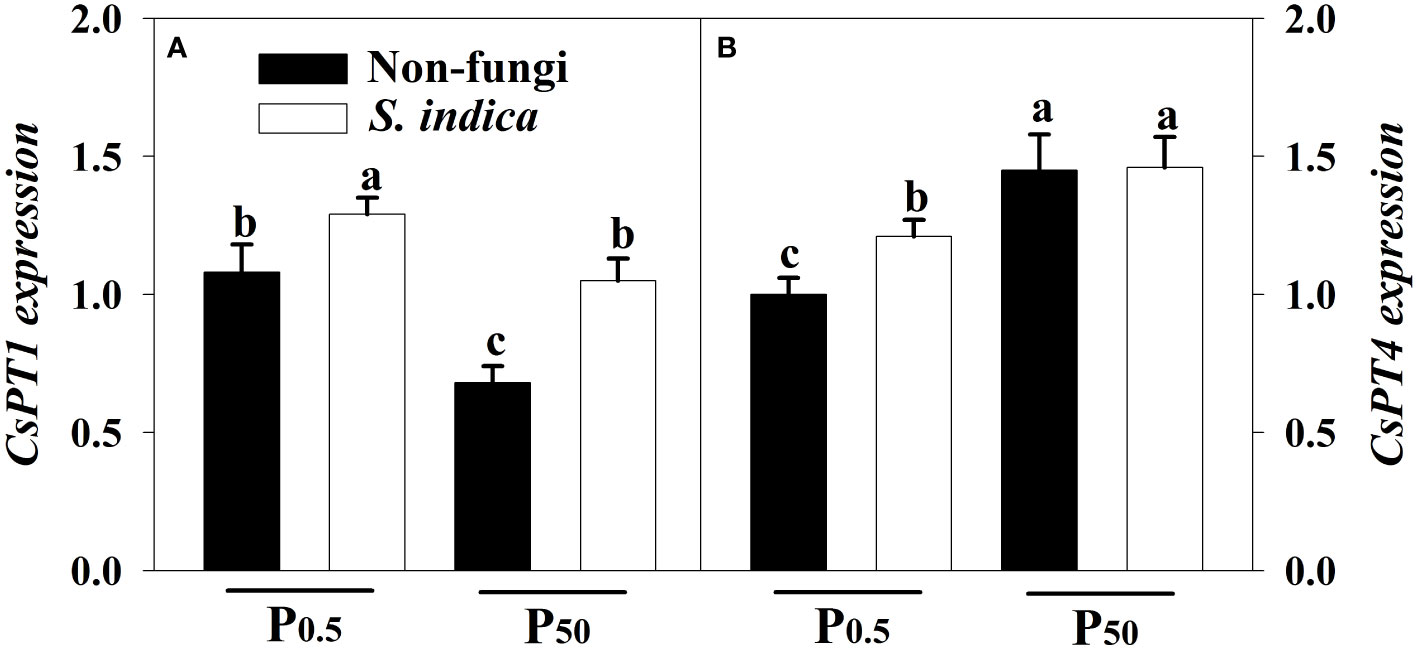
Figure 6 Effects of inoculation with Serendipita indica on leaf CsPT1 (A) and CsPT4 (B) expressions of tea seedlings at P0.5 and P50 levels. Data (means ± SD, n = 3) are significantly (P < 0.05) different if followed by different letters above the bars. The abbreviations are shown in Table 1.
Discussion
The symbiotic degree between plants and endophytic fungi is regulated by substrate P levels (Wu et al., 2015). In this study, the P0.5 treatment dramatically inhibited root colonization rate of S. indica in tea seedlings, compared to the P50 treatment, which may be due to the fact that under conditions of P deficiency, plants acquire P mainly through increased root-hair density (Shao et al., 2021), thus reducing the dependence on S. indica.
In the present study, we also found that leaf P concentrations were significantly higher under P50 conditions than under P0.5 conditions, and S. indica colonization promoted leaf P acquisition by tea seedlings under both P0.5 and P50 conditions, which is in agreement with the findings of Taghinasab et al. (2018) on wheat. S. indica improved plant growth behavior of tea seedlings to varying degrees, with a prominent positive effect appeared at the P0.5 level than at the P50 level. This suggests that the presence of endophytic fungi, such as S. indica, plays a crucial role in maintaining good plant growth under the condition of substrate P deficiency (Poveda et al., 2021). This is consistent with the results of previous studies in wheat (Taghinasab et al., 2018) and sunflower (Eliaspour et al., 2020).
P is an important macronutrient in plants that plays an important role in maintaining photosynthetic electron transfer efficiency as well as ribulose-1,5-bisphosphate carboxylase (Rubisco) activity (Nguyen et al., 2022). In the present study, we found that P50 promoted leaf Pn, Gs, and Tr in tea, compared with P0.5, showing that leaf gas exchange in tea seedlings is dependent on the level of substrate P. On the other hand, inoculation of S. indica also significantly increased leaf Pn and Tr at P0.5 and P50 levels. This is in agreement with the results of Sun YL et al. (2022) on alfalfa. Generally, P supply can accelerate leaf Pn and Tr (Pang et al., 2018). We also found that increased substrate P levels as well as S. indica inoculation significantly increased leaf Chi levels and Nbi, thus suggesting that the endophytic fungus stimulate photosynthetic activity by increasing Chl content as well as N levels to improve plant photosynthetic capacity (Liu et al., 2019). Hallasgo et al. (2020) also reported that inoculation of S. indica on 3-week-old tomato seedlings promoted N concentrations. These results demonstrated that S. indica improved nutrient uptake as well as Chl formation and gas exchange in tea seedlings (Mathur et al., 2018).
Chlorophyll fluorescence can reflect the absorption, utilization, transfer and dissipation processes of light energy by PSI and PSII (Cao YW et al., 2022). In this study, under S. indica-uninoculated conditions, compared with P0.5, Fv/Fm_Lss, QY_max, and QY_Lss increased significantly under P50 conditions, which is consistent with Yang YM et al. (2021) on soybean. It confirmed that chlorophyll fluorescence parameters are closely related to P levels. In addition, NPQ is an important indicator for plant adaptation to low P conditions (Yang YM et al., 2021). P0.5 significantly promoted NQP_Lss in S. indica-inoculated tea seedlings, compared with P50, further indicating that S. indica-inoculated plants have better ability to adapt to low P than uninoculated plants. Fv/Fm_Lss is an important indicator to determine whether plants are subject to photoinhibition (Chen et al., 2022). In this study, P0.5 treatment resulted in a photoinhibition in tea seedlings, whereas inoculation with S. indica significantly increased Fv/Fm_Lss at the P0.5 level, indicating that the photoinhibition of inoculated plants was somewhat alleviated and that photosynthetic organs could effectively convert absorbed light energy into biochemical energy, thus maintaining normal photosynthetic efficiency. Similar results were also reported by previous studies (Liu RC et al., 2021; Sun YL et al., 2022). Thus, inoculated tea seedlings with the endophytic fungus were able to slow down photoinhibition and promote light energy efficiency, thus maintaining efficient photochemical efficiency, especially at the P0.5 level. It reveals an important role of S. indica in tea seedlings growth under soil P deficit, but the exact mechanism is still unclear, and further deciphering how S. indica is involved in the host’s photochemical regulation of efficiency.
Plants regulate their growth and development through changing hormone levels to adapt to environments (Perez-Alonso et al., 2021), of which auxins and cytokinin are the main regulatory factors of plant growth and development (Bielach et al., 2017). In this study, two auxins, IAA and IBA, were measured and it was found that under uninoculated conditions, leaf IAA levels were significantly decreased under P50 conditions compared to P0.5, which is consistent with previous findings (Huang et al., 2022). While, IBA levels were significantly increased at P50 versus P0.5 levels. IBA is the storage form and source of IAA, which is more stable than IAA (Kunkel and Harper, 2018). Inoculated tea seedlings with S. indica recorded significantly higher leaf IAA levels and relatively lower IBA levels than uninoculated seedlings in P0.5 and P50, which is consistent with the results of Zhang et al. (2019) in trifoliate orange seedlings inoculated with Funneliformis mosseae. Such change may be due to the inoculation of S. indica to promote peroxidase activity, thus accelerating the conversion of IBA to IAA (Frick and Strader, 2018; Cheng et al., 2020). These results confirmed that the promotion of plant growth by symbiotic fungi under nutrient deficiency is mainly related to its regulation of IAA. Cytokinins usually act synergistically with auxins to promote plant growth and development (Kieber and Schaller, 2018).
In this study, P0.5 significantly decreased only leaf TZ levels of inoculated and uninoculated seedlings, compared to P50. Under P-deficient conditions, plants maintain their P uptake by increasing cis-zeatin (CZ) levels, which inhibits the synthesis of TZ, thus increasing the ratio of CZ/TZ to maintain its response to the cytokinin signaling pathway for facilitating plant P acquisition (Silva-Navas et al., 2019). In the present study, leaf IP, TZ, and DZ levels of P0.5-treated tea seedlingss were significantly increased after inoculation with S. indica, which is due to the combination of S. indica and low P promoting isopentenyl transferase (an important rate-limiting enzyme for cytokinin biosynthesis that catalyzes the breakdown of isopentenyl pyrophosphate and adenosine monophosphate to produce isopentenyl adenosine monophosphate as a precursor of cytokinins) activity (Liu et al., 2020), thus inducing cytokinin production in young tea seedlings.
In addition, S. indica can promote the acquisition of P in plants by inducing the expression of PT genes (Yang L et al., 2021; Cao MA et al., 2022). In tea, CsPT1 and CsPT4 belong to the Pht1 family and are mainly responsible for absorbing inorganic phosphate from soil (Xin et al., 2017). It was documented that an arbuscular mycorrhizal fungus, Claroideoglomus etunicatum, could induce the up-regulation of CsPT1 gene and promote P absorption in tea plants grown in both P0.5 and P50 (Shao et al., 2021). In this study, CsPT1 expression in young leaves was increased, while CsPT4 expression was decreased under the condition of P0.5 versus P50. Xin et al. (2017) observed that low P induced the up-regulated expression of CsPT4 in roots, along with higher expression of CsPT4 in old leaves than roots. It is necessary to comprehensively analyze the response pattern of CsPTs to low P in old leaves, young leaves, stems, and roots. The present study also indicated S. indica colonization led to up-regulation of CsPT1 expression under P0.5 and P50 conditions and CsPT4 expression under P0.5 conditions. This suggests that S. indica up-regulates the expression levels of CsPT1 and CsPT4 at low P levels, further revealing the importance of endophytic fungi under nutrient deficit. At the appropriate P level, S. indica only increased the expression level of CsPT1, and had no effect on the expression of CsPT4. In fact, in S. indica, a high-affinity phosphate transporter SiHPO80 could be up-regulated under the condition of appropriate P levels (Loha et al., 2018), which may compensate for the unresponsive expression of CsPT4 at the appropriate P level. Moreover, the expression of CsPT1 was up-regulated not only by S. indica but also by the arbuscular mycorrhizal fungus C. etunicatum, both at the P0.5 and P50 levels (Shao et al., 2021), which reveal that CsPT1 is up-regulated by beneficial fungi, but whether its expression is specifically induced remains to be further verified. In addition, S. indica also releases acid phosphatase into the environment (Kushwaha and Kumar, 2022), thus dissolving insoluble P in the soil for enhanced P acquisition of the host. It is suggested that S. indica can improve host P acquisition through a variety of ways, such as up-regulation of host PTs expression and self-release of acid phosphatase and SiHPO80 expression. However, it requires assessing how such multiple ways work at different P levels.
Conclusion
The symbiosis between S. indica and tea seedlings was regulated by soil P levels, and an appropriate soil P level was favorable for symbiotic formation in roots. At the P0.5 level, inoculation of S. indica promoted P acquisition and thus plant growth in tea seedlings by regulating the expression of CsPT1 and CsPT4 genes as well as increased chlorophyll, gas exchange, and endogenous IAA, IP, and DZ levels. Such results provide a new pathway for future inoculation of tea seedlings with a culturable endophytic fungus S. indica in the field. However, the mechanism on how S. indica promotes P acquisition of tea plants at low P levels needs to be further investigated.
Data availability statement
The original contributions presented in the study are included in the article/Supplementary Material. Further inquiries can be directed to the corresponding authors.
Author contributions
Conceptualization, Q-SW and TY; data curation, Z-YR and A-QL; methodology, Z-YR; resources, Q-SW; supervision, Q-SW and TY; writing—original draft, Z-YR; writing—review and editing, AH, KK, EA, and Q-SW. All authors contributed to the article and approved the submitted version.
Funding
This work was supported by the Open Fund of State Key Laboratory of Tea Plant Biology and Utilization (SKLTOF20220108). The authors would like to extend their sincere appreciation to the Researchers Supporting Project Number (RSP2023R356), King Saud University, Riyadh, Saudi Arabia.
Acknowledgments
The authors would like to extend their sincere appreciation to the Researchers Supporting Project Number (RSP2023R356), King Saud University, Riyadh, Saudi Arabia.
Conflict of interest
The authors declare that the research was conducted in the absence of any commercial or financial relationships that could be construed as a potential conflict of interest.
Publisher’s note
All claims expressed in this article are solely those of the authors and do not necessarily represent those of their affiliated organizations, or those of the publisher, the editors and the reviewers. Any product that may be evaluated in this article, or claim that may be made by its manufacturer, is not guaranteed or endorsed by the publisher.
Supplementary material
The Supplementary Material for this article can be found online at: https://www.frontiersin.org/articles/10.3389/fpls.2023.1146182/full#supplementary-material
References
Bakhshandeh, E., Pirdashti, H., Shahsavarpour Lendeh, K., Gilani, Z., Yaghoubi Khanghahi, M., Crecchio, C. (2020). Effects of plant growth promoting microorganisms inoculums on mineral nutrition, growth and productivity of rice (Oryza sativa l.). J. Plant Nutr. 43, 1643–1660. doi: 10.1080/01904167.2020.1739297
Bielach, A., Hrtyan, M., Tognetti, V. B. (2017). Plants under stress: Involvement of auxin and cytokinin. Int. J. Mol. Sci. 18, 1427. doi: 10.3390/ijms18071427
Cao, M. A., Liu, R. C., Xiao, Z. Y., Hashem, A., Allah, E. F. A., Alsayed, M. F., et al. (2022). Symbiotic fungi alter the acquisition of phosphorus in Camellia oleifera through regulating root architecture, plant phosphate transporter gene expressions and soil phosphatase activities. J. Fungi 8, 800. doi: 10.3390/jof8080800
Cao, Y. W., Pan, Y. H., Wang, M., Liu, T. H., Meng, X. H., Guo, S. W. (2022). The effects of different nitrogen forms on chlorophyll fluorescence and photosystem II in Lonicera japonica. J. Plant Growth Regul. doi: 10.1007/s00344-022-10873-1
Chen, Q., Qi, W. B., Reiter, R. J., Wei, W., Wang, B. M. (2009). Exogenously applied melatonin stimulates root growth and raises endogenous indoleacetic acid in roots of etiolated seedlings of Brassica juncea. J. Plant Physiol. 166, 324–328. doi: 10.1016/j.jplph.2008.06.002
Chen, P. Y., Yang, C., Zhang, Q. S., Zhou, S., Yang, Y. K., Luo, J. (2022). Effects of shading treatment on chlorophyll fluorescence characteristics of Calanthe reflexa leaves. J. Plateau Agric. 6, 585–592. doi: 10.19707/j.cnki.jpa.2022.06.010
Cheng, C. Z., Li, D., Qi, Q., Sun, X. L., Anue, M. R., David, B. M., et al. (2020). The root endophytic fungus Serendipita indica improves resistance of banana to fusarium oxysporum f. sp. cubense tropical race 4. Eur. J. Plant Pathol. 156, 87–100. doi: 10.1007/s10658-019-01863-3
Cheng, X. F., Xie, M. M., Li, Y. (2022). Effects of field inoculation with arbuscular mycorrhizal fungi and endophytic fungi on fruit quality and soil properties of newhall navel orange. Appl. Soil Ecol. 170, 104308. doi: 10.1016/j.apsoil.2021.104308
Deng, C., Sun, R.-T., Ma, Q., Yang, Q.-H., Zhou, N., Hashem, A., et al. (2022). Mycorrhizal effects on active components and associated gene expressions in leaves of Polygonum cuspidatum under P stress. Agronomy 12, 2970. doi: 10.3390/agronomy12122970
Eliaspour, S., Sharifi, R. S., Shirkhani, A. (2020). Evaluation of interaction between Piriformospora indica, animal manure and NPK fertilizer on quantitative and qualitative yield and absorption of elements in sunflower. Food Sci. Nutr. 8, 2789–2797. doi: 10.1002/fsn3.1571
Frick, E. M., Strader, L. C. (2018). Roles for IBA-derived auxin in plant development. J. Exp. Bot. 69, 169–177. doi: 10.1093/jxb/erx298
Hallasgo, A. M., Spangl, B., Steinkellner, S., Hage-Ahmed, K. (2020). The fungal endophyte Serendipita williamsii does not affect phosphorus status but carbon and nitrogen dynamics in arbuscular mycorrhizal tomato plants. J. Fungi 6, 233. doi: 10.3390/jof6040233
Huang, W. G., Jiang, W. D., Yao, Y. B., Song, X. X., Yuan, H. M., Ren, C. Y., et al. (2022). The effects of phosphorus deficiency on the morpho-physiology and expression of LusWRKYs in Linum usitatissimum l. Pak. J. Bot. 54, 95–103. doi: 10.30848/PJB2022-1(26
Kieber, J. J., Schaller, G. E. (2018). Cytokinin signaling in plant development. Development 145, 149344. doi: 10.1242/dev.149344
Kunkel, B. N., Harper, C. P. (2018). The roles of auxin during interactions between bacterial plant pathogens and their hosts. J. Exp. Bot. 69, 245–254. doi: 10.1093/jxb/erx447
Kushwaha, A. S., Kumar, M. (2022). An effective in-gel assay protocol for the assessment of acid phosphatase (ACPase) isoform expression in the fungus Serendipita indica. 3 Biotech. 12, 40. doi: 10.1007/s13205-021-03103-0
Liao, Y. Y., Li, J. L., Pan, R. L., Chiou, T. J. (2019). Structure-function analysis reveals amino acid residues of arabidopsis phosphate transporter AtPHT1;1 crucial for its activity. Front. Plant Sci. 10, 1158. doi: 10.3389/fpls.2019.01158
Liu, R. C., Gao, W. Q., Srivastava, A. K., Zou, Y. N., Kuča, K., Hashem, A., et al. (2021). Differential effects of exogenous glomalin-related soil proteins on plant growth of trifoliate orange through regulating auxin changes. Front. Plant Sci. 12, 745402. doi: 10.3389/fpls.2021.745402
Liu, B. H., Jing, D. W., Liu, F. C., Ma, H. L., Liu, X. H., Peng, L. (2021). Serendipita indica alleviates drought stress responses in walnut (Juglans regia l.) seedlings by stimulating osmotic adjustment and antioxidant defense system. Appl. Microbiol. Biot. 105, 8951–8968. doi: 10.1007/s00253-021-11653-9
Liu, M. H., Wang, Y. X., Li, Q., Xiao, W. F., Song, X. Z. (2019). Photosynthesis, ecological stoichiometry, and non-structural carbohydrate response to simulated nitrogen deposition and phosphorus addition in chinese fir forests. Forests 10, 1068. doi: 10.3390/f10121068
Liu, R. C., Yang, L., Zou, Y. N., Wu, Q. S. (2022). Root-associated endophytic fungi modulate endogenous auxin and cytokinin levels to improve plant biomass and root morphology of trifoliate orange. Hortic. Plant J. doi: 10.1016/j.hpj.2022.08.009
Liu, Y., Zhang, M., Meng, Z., Wang, B., Chen, M. (2020). Research progress on the roles of cytokinin in plant response to stress. Int. J. Mol. Sci. 21, 6574. doi: 10.3390/ijms21186574
Livak, K. J., Schmittgen, T. D. (2001). Analysis of relative gene expression data using real-time quantitative PCR and the 2–ΔΔCt method. Methods 25, 402–408. doi: 10.1006/meth.2001.1262
Loha, A., Kashyap, A. K., Sharma, P. (2018). A putative cyclin, SiPHO80 from root endophytic fungus Serendipita indica regulates phosphate homeostasis, salinity and heavy metal toxicity tolerance. Biochem. Bioph. Res. Co. 507, 414–419. doi: 10.1016/j.bbrc.2018.11.053
Mathur, S., Sharma, M. P., Jajoo, A. (2018). Improved photosynthetic efficacy of maize (Zea mays) plants with arbuscular mycorrhizal fungi (AMF) under high temperature stress. J. Photoch. Photobio. B. 180, 149–154. doi: 10.1016/j.jphotobiol.2018.02.002
Nguyen, V., Palmer, L., Stangoulis, J. (2022). Higher photochemical quenching and better maintenance of carbon dioxide fixation are key traits for phosphorus use efficiency in the wheat breeding line, RAC875. Front. Plant Sci. 12, 816211. doi: 10.3389/fpls.2021.816211
Pang, J. Y., Zhao, H. X., Bansal, R., Bohuon, E., Lambers, H., Ryan, M. H., et al. (2018). Leaf transpiration plays a role in phosphorus acquisition among a large set of chickpea genotypes. Plant Cell Environ. 41, 2069–2079. doi: 10.1111/pce.13139
Perez-Alonso, M. M., Ortiz-Garcia, P., Moya-Cuevas, J., Lehmann, T., Sánchez-Parra, B., Björk, R. G., et al. (2021). Endogenous indole-3-acetamide levels contribute to the crosstalk between auxin and abscisic acid, and trigger plant stress responses in arabidopsis. J. Exp. Bot. 72, 459–475. doi: 10.1093/jxb/eraa485
Poveda, J., Eugui, D., Abril-Urias, P., Velasco, P. (2021). Endophytic fungi as direct plant growth promoters for sustainable agricultural production. Symbiosis 85, 1–19. doi: 10.1007/s13199-021-00789-x
Saddique, M. A., Ali, Z., Khan, A. S., Rana, I. A., Shamsi, I. H. (2018). Inoculation with the endophyte Piriformospora indica significantly affects mechanisms involved in osmotic stress in rice. Rice 11, 34. doi: 10.1186/s12284-018-0226-1
Shao, Y. D., Hu, X. C., Wu, Q. S. (2021). Mycorrhizas promote p acquisition of tea plants through changes in root morphology and p transporter gene expression. S. Afr. J. Bot. 137, 455–462. doi: 10.1016/j.sajb.2020.11.028
Silva-Navas, J., Conesa, C. M., Saez, A., Navarro-Neila, S., Garcia-Mina, J. M., Zamarreño, A. M., et al. (2019). Role of cis-zeatin in root responses to phosphate starvation. New Phytol. 224, 242–257. doi: 10.1111/nph.16020
Sun, Y. L., Wang, X. Z., Ma, C. H., Zhang, Q. B. (2022). Effects of nitrogen and phosphorus addition on agronomic characters, photosynthetic performance and anatomical structure of alfalfa in northern xinjiang, China. Agronomy 12, 1613. doi: 10.3390/agronomy12071613
Sun, R. T., Zhang, Z. Z., Liu, M. Y., Feng, X. C., Zhou, N., Feng, H. D., et al. (2022). Arbuscular mycorrhizal fungi and phosphorus supply accelerate main medicinal component production of Polygonum cuspidatum. Front. Microbiol 13, 1006140. doi: 10.3389/fmicb.2022.1006140
Taghinasab, M., Imani, J., Steffens, D., Glaeser, S. P., Kogel, K. H. (2018). The root endophytes Trametes versicolor and Piriformospora indica increase grain yield and p content in wheat. Plant Soil 426, 339–348. doi: 10.1007/s11104-018-3624-7
Tyagi, J., Chaudhary, P., Mishra, A., Khatwani, M., Dey, S., Varma, A. (2022). Role of endophytes in abiotic stress tolerance: With special emphasis on Serendipita indica. Int. J. Environ. Res. 16, 62. doi: 10.1007/s41742-022-00439-0
Vance, C. P., Uhde, S. C., Allan, D. L. (2003). Phosphorus acquisition and use: critical adaptations by plants for securing a nonrenewable resource. New Phytol. 157, 423–447. doi: 10.1046/j.1469-8137.2003.00695.x
Wang, X. H., Fan, X., Wang, W., Song, F. (2022). Use of Serendipita indica to improve soybean growth, physiological properties, and soil enzymatic activities under different cd concentrations. Chem. Biol. Technol. Agric. 9, 66. doi: 10.1186/s40538-022-00331-1
Wang, H. J., Gong, Z. M., Chen, X., Wang, Y. (2015). Status and distribution of soil nutrients in tea garden. Hubei Agric. Sci. 54, 3875–3879. doi: 10.14088/j.cnki.issn0439-8114.2015.16.011
Wang, Y., Zou, Y. N., Shu, B., Wu, Q. S. (2023). Deciphering molecular mechanisms regarding enhanced drought tolerance in plants by arbuscular mycorrhizal fungi. Sci Hortic 308, 111591. doi: 10.1016/j.scienta.2022.111591
Wheal, M. S., Fowles, T. O., Palmer, L. T. (2011). A cost-effective acid digestion method using closed polypropylene tubes for inductively coupled plasma optical emission spectrometry (ICP-OES) analysis of plant essential elements. Anal. Methods 3, 2854–2863. doi: 10.1039/C1AY05430A
Wu, F., Li, J. R., Chen, Y. L., Zhang, L. P., Zhang, Y., Wang, S., et al. (2019). Effects of phosphate solubilizing bacteria on the growth, photosynthesis, and nutrient uptake of Camellia oleifera Abel. Forests 10, 348. doi: 10.3390/f10040348
Wu, Q. S., Li, Y., Zou, Y. N., He, X. H. (2015). Arbuscular mycorrhiza mediates glomalin-related soil protein production and soil enzyme activities in the rhizosphere of trifoliate orange grown under different p levels. Mycorrhiza 25, 121–130. doi: 10.1007/s00572-014-0594-3
Wu, C., Wei, Q., Deng, J., Zhang, W. (2019). Changes in gas exchange, root growth, and biomass accumulation of Platycladus orientalis seedlings colonized by Serendipita indica. J. For. Res. 30, 1199–1207. doi: 10.1007/s11676-018-0712-8
Xin, H. H., Wang, W. D., Wang, M. L., Ma, Q. P., Gan, Y. D., Li, X. H. (2017). Molecular cloning, subcellular localization and expression analysis of CsPT4 gene in tea plant (Camellia sinensis). J. Tea Sci. 37, 493–502. doi: 10.13305/j.cnki.jts.2017.05.008
Yang, L., Cao, J. L., Zou, Y. N., Wu, Q. S., Kuča, K. (2020). Piriformospora indica: A root endophytic fungus and its roles in plants. Not. Bot. Horti Agrobo. 48, 1–13. doi: 10.15835/nbha48111761
Yang, Y. M., Zhu, X. H., Cui, R. F., Wang, R. Y., Li, H. Y., Wang, J. S., et al. (2021). Identification of soybean phosphorous efficiency QTLs and genes using chlorophyll fluorescence parameters through GWAS and RNA-seq. Planta 254, 110. doi: 10.1007/s00425-021-03760-8
Yang, L., Zou, Y. N., Tian, Z. H., Wu, Q. S., Kuča, K. (2021). Effects of beneficial endophytic fungal inoculants on plant growth and nutrient absorption of trifoliate orange seedlings. Sci. Hortic. 277, 109815. doi: 10.1016/j.scienta.2020.109815
Zhang, F., Wang, P., Zou, Y. N., Wu, Q. S., Kuča, K. (2019). Effects of mycorrhizal fungi on root-hair growth and hormone levels of taproot and lateral roots in trifoliate orange under drought stress. Arch. Agron. Soil Sci. 65, 1316–1330. doi: 10.1080/03650340.2018.1563780
Zheng, H. L., Qi, Y. P., Chen, R. B., Zhang, F. Z., Chen, L. S. (2012). Effects of phosphorus supply on the quality of green tea. Food Chem. 130, 908–914. doi: 10.1016/j.foodchem.2011.08.008
Keywords: auxin, endophytic fungi, cash crop, nutrient deficit, phytohormone, symbiosis
Citation: Rong Z-Y, Lei A-Q, Wu Q-S, Srivastava AK, Hashem A, Abd_Allah EF, Kuča K and Yang T (2023) Serendipita indica promotes P acquisition and growth in tea seedlings under P deficit conditions by increasing cytokinins and indoleacetic acid and phosphate transporter gene expression. Front. Plant Sci. 14:1146182. doi: 10.3389/fpls.2023.1146182
Received: 17 January 2023; Accepted: 13 February 2023;
Published: 17 March 2023.
Edited by:
Umakanta Ngangkham, Research Complex for NEH Region Manipur Centre (ICAR), IndiaReviewed by:
Hassan Etesami, University of Tehran, IranKarthikeyan Adhimoolam, Jeju National University, Republic of Korea
Copyright © 2023 Rong, Lei, Wu, Srivastava, Hashem, Abd_Allah, Kuča and Yang. This is an open-access article distributed under the terms of the Creative Commons Attribution License (CC BY). The use, distribution or reproduction in other forums is permitted, provided the original author(s) and the copyright owner(s) are credited and that the original publication in this journal is cited, in accordance with accepted academic practice. No use, distribution or reproduction is permitted which does not comply with these terms.
*Correspondence: Qiang-Sheng Wu, d3VxaWFuZ3NoQDE2My5jb20=; Tianyuan Yang, eWFuZ3RpYW55dWFuMjAwOEAxNjMuY29t