- 1College of Grassland Agriculture, Northwest A&F University, Yangling, Shaanxi, China
- 2College of Life Sciences, Guizhou University, Guiyang, Guizhou, China
- 3Institute of Soil and Water Conservation, Northwest A&F University, Yangling, Shaanxi, China
- 4School of Agriculture and Environment, Massey University, Palmerston North, New Zealand
Fertilization could influence ecosystem structure and functioning through species turnover (ST) and intraspecific trait variation (ITV), especially in nutrient limited ecosystems. To quantify the relative importance of ITV and ST in driving community functional structure and productivity changes under nitrogen (N) and phosphorous (P) addition in semiarid grasslands. In this regard, we conducted a four-year fertilizer addition experiment in a semiarid grassland on the Loess Plateau, China. We examined how fertilization affects species-level leaf and root trait plasticity to evaluate the ability of plants to manifest different levels of traits in response to different N and P addition. Also, we assessed how ITV or ST dominated community-weighted mean (CWM) traits and functional diversity variations and evaluated their effects on grassland productivity. The results showed that the patterns of plasticity varied greatly among different plant species, and leaf and root traits showed coordinated variations following fertilization. Increasing the level of N and P increased CWM_specific leaf area (CWM_SLA), CWM_leaf N concentration (CWM_LN) and CWM_maximum plant height (CWM_Hmax) and ITV predominate these CWM traits variations. As a results, increased CWM_Hmax, CWM_LN and CWM_SLA positively influenced grassland productivity. In contrast, functional divergence decreased with increasing N and P and showed negative relationships with grassland productivity. Our results emphasized that CWM traits and functional diversity contrastingly drive changes in grassland productivity under N and P addition.
1 Introduction
In China, the Loess Plateau is a key ecological conservation area, where 42.9% of it accounts for grasslands (Gang et al., 2018; Guo et al., 2022a). Grassland ecosystems play irreplaceable roles in human survival and development due to their ecological (e.g., nutrient cycling, soil and water conservation) and economic (e.g., providing the feed base for grazing livestock) functions (Han et al., 2011; Liu et al., 2021; Guo et al., 2022a). However, massive losses of nitrogen (N) and phosphorus (P) owing to long-term soil erosion have limited the restoration process and ecological functions of grasslands on the Loess Plateau(Liu et al., 2013; Fay et al., 2015; Yang et al., 2022a).
Both N and P, play critical roles in improving community functions and accelerating the grassland restoration process (Rowe et al., 2006; Vitousek et al., 2010; DeSiervo et al., 2023). In the past decades, some studies have looked into how fertilization affects species interaction and biodiversity to understand the ecological functions of grasslands under various nutrient levels in the Loess Plateau and to develop management practices to improve the restoration process (Wang et al., 2014; Chen et al., 2021; Yang et al., 2022b). However, most researchers primarily assessed the impact of various fertilization levels on grassland ecosystem functioning at the community level, while they did not consider their relationship with the species composition changes and the ecophysiological responses at the individual species level characterized by functional traits (Stevens, 2016; Zhang et al., 2018). Investigating the impacts exerted by plant species can further our understanding of the response of grassland ecosystem functions under nutrient addition. Because the response of ecosystems to anthropogenic disturbances and environmental changes starts with the different responses of individual plant species, and subsequently, it scales up to the community level(Lavorel and Garnier, 2002).
At species level, plants can improve their adaptability to environmental changes through trait plasticity (Turcotte and Levine, 2016). Trait plasticity is the ability to manifest different levels of specific traits, enabling plants to cope with various environmental constraints (Funk et al., 2017). Trait plasticity also determines species-specific responses to nutrient addition (Kichenin et al., 2013). However, the direction and magnitude of plasticity differ among species (Lin et al., 2020). Generally, aboveground (i.e. shoot) and belowground (i.e. root system) traits respond differently to environmental changes (Weemstra et al., 2016). While leaf traits are tightly related to photosynthetic function and plant production, root traits determine the nutrient acquisition strategies of plants (Funk et al., 2017; Caplan et al., 2019). Nevertheless, coordination between leaf and root traits plays pivotal roles in forming adaptation strategies to environmental changes (Faucon et al., 2017). Yet, few studies explored the coordination between leaf and root traits in response to nutrient addition in grassland ecosystems on the Loess Plateau.
At community level, environmental changes such as nutrient addition can influence community functional structure through intraspecific trait variation (ITV), species turnover (ST), or both depending on the intensity of changes. ITV represents contribution to the overall functional trait response to environmental changes. ST represents the change of species composition (Albert et al., 2010). Under low-intensity environmental changes, community-level traits may respond through ITV, with no noticeable changes in species compositions (Kichenin et al., 2013). While under high-intensity environmental changes, variation in community-level traits is attributed to ST (Kichenin et al., 2013). ITV and ST play essential roles in grassland ecosystems in shaping the community functional structure under nutrient addition (Read et al., 2017). However, their effects on the community functional structure vary with the studied traits and experimental duration (Zhou et al., 2018). Alterations in the community functional structure are usually determined by community-weighted mean traits (CWM, mass-ratio hypothesis) (Grime, 1998) and functional diversity (FD, diversity hypothesis) (Diaz et al., 2007; Mao et al., 2017). CWM traits focus on dominant species that predominantly determine the responses of community functional structure to nutrient addition (Grime, 1998). While FD describes the functional trait diversity within a species community, and often expresses by functional evenness (FEve), functional dispersion (FDis), functional divergence (FDiv), and Rao’s quadratic entropy (RaoQ) (Table S1) (Petchey and Gaston, 2006). Given the role of ITV and ST in ecosystem function, it is crucial to understand the relative contribution of both factors in grassland community functional structure under nutrient addition. Yet, few researchers have assessed if alterations in the community functional structure following nutrient addition are governed by ITV or ST in semiarid grasslands.
Several studies have shown that species functional traits predominantly regulate ecosystem functioning (Wellstein et al., 2011; Chen et al., 2023). Community-level functional traits better explain variations in ecosystem functioning than species based matrices (Roscher et al., 2012). When community functional structure is dominated by ST, the composition of community will shift towards species with dominant functional traits (Smith et al., 2009), leading to alterations in grassland productivity (Isbell et al., 2013). Given that, grassland productivity is a key indicator of grassland ecosystem functioning (Schaub et al., 2020).
Nutrient addition caused species loss and trait divergence, resulting in variation in community functional structure, further affecting grassland productivity (Niu et al., 2014). Hence, a critical factor in developing theoretical support for grassland management is to determine components of community functional structures that affect the variations in ecosystem functioning following nutrient addition. In view of this, a four-year fertilizer (i.e., N and P) addition experiment was conducted on a semiarid grassland to (1) quantify the responses of leaf and root trait plasticity (i.e., species-level) and community functional structures (i.e., community-level) to N and P addition (2) quantify the contribution of ST and ITV in driving the community functional structure in response to N and P addition, and (3) assess the effects of variations in community functional structure on grassland productivity under N and P addition.
2 Materials and methods
2.1 Study area
The study was conducted in a semiarid grassland in Shaanxi Province, China, with an altitude of 1010–1431 m, an average temperature of 8.8°C and average annual rainfall of 528.8 mm (Figure 1). The soil type is loamy loess soil, and the landform is a typical hilly-gullied landscape (Guo et al., 2022b). The soil total N and P of the study area are 0.60 and 0.58 g kg-1, respectively. For this research, a 600 m2 area of the grassland was fenced to avoid grazing disturbance (Table S2). There was a total of 13 species in the grassland community before N and P additions in 2017. The relative abundance of common species were Bothriochloa ischaemum (L.) Keng (27.30%), Artemisia sacrorum Ledeb. (11.70%), Lespedeza davurica (Laxmann) Schindler (11.70%), Stipa bungeana Trin. (12.67%), Potentilla tanacetifolia Willd. ex D.F.K.Schltdl. (16.36%), and Artemisia scoparia (Waldst. & Kit.) Pamp (5.39%). The coverage and aboveground biomass of the grassland community were 41% and 118.7 g m-2 before N and P additions in 2017 (Table S3).
2.2 Experimental design
The research was initiated in late August 2017. The experimental design was split-plot with twelve main plots (4 × 4 m) and four subplots (2 × 2 m). Each main plot was laid out in a randomized block design with three replicates. A 1.5-m buffer zone was allocated between adjacent blocks. Four main plots were randomly assigned to four levels of N, namely 0, 25, 50, and 100 kg N ha‐1 yr‐1(hereinafter referred to as N0, N25, N50, and N100, respectively). Moreover, four subplots per main plot were randomly assigned to four levels of P, namely 0, 20, 40, and 80 kg P2O5 ha‐1 yr-1 (hereinafter referred to as P0, P20, P40, and P80, respectively) (Figure S1). The lowest N addition level (N25) was applied according to the atmospheric N deposition level (about 21.76 kg N ha-1 yr-1) on the Loess Plateau (Liang et al., 2016). N50 and N100 treatments were about twice and four times of atmospheric N deposition level to estimate further consequences of N deposition. The P addition levels were applied according to the proper P addition treatments in the experiment of promoting grassland restoration based on our previous study. N and P fertilizers were applied in calcium ammonium nitrate and triple superphosphate, respectively. Fertilization was applied manually to each subplot every year at the beginning of the growing season before rainfall on June 4, 2017, May 21, 2018, June 13, 2019 and June 11, 2020, respectively. Two separate 1 × 1 m fixed quadrats were established randomly in each subplot for community survey (i.e., structure and function) and measuring functional traits.
2.3 Community survey and trait measurements
Plant maximum plant height (Hmax), abundance, and coverage were measured in August 21, 2020 in each 1×1 m fixed quadrat. The aboveground material of all plant species within each quadrat was cut, oven-dried at 80°C, and weighed. The community productivity was estimated as the sum of the harvested aboveground biomass. Relative biomass of each species was the aboveground biomass of each species/community aboveground biomass.
Plant functional traits were evaluated using 2–3 fully mature individuals for each dominant species (i.e., those species account for at least 80% of community biomass) in each fixed quadrat. 10-15 mature and fully expanded sun-exposed leaves per individual were chosen for measuring leaf traits, and 10-15 fine roots (diameter< 2 mm) of the same individuals from which we collected leaves were chosen for measuring fine root traits. Functional traits of leaves and roots were measured according to standard protocols (Pérez-Harguindeguy et al., 2013). The leaf and root samples were placed in sealed plastic bags and stored in a chiller until they were weighed and scanned. We used a digital caliper to measure leaf thickness at the midpoint of leaves while avoiding the major veins. We then scanned the leaf and root samples using a portable scanner (CanoScan LiDE 120) and measured the leaf area using the ImageJ software (http://imagej.nih.gov/ij/). Each fine root image was processed using the WINRHIZO software (Regent Instruments Inc., Quebec City, Canada) to determine the root length and root surface area. All samples were oven-dried for 48 h (65°C) to determine the leaf and root dry mass. We then calculated the specific leaf area (SLA; cm2 g-1) as leaf area/leaf dry mass and the specific root length (SRL; cm g-1) as root length/root dry mass. Leaf tissue density (LTD; g cm-3) was calculated as leaf dry mass/leaf volume, and leaf volume was calculated as leaf area × leaf thickness. The specific root surface area (SRA; cm2 g-1) was calculated as root surface area/root dry mass. Finally, the leaves and fine roots of each species were ground and passed through a 0.25-mm sieve. The sieved samples were used to measure the leaf and root N and P concentrations (LN, LP, RN, and RP, respectively; g kg-1). LN and RN were measured with an auto-Kjeldahl instrument (Kjektec System 2300 Distilling Unit, Foss, Sweden). LP and RP were determined using the molybdenum–antimony colorimetric method.
2.4 Statistical analysis
Plant trait plasticity was evaluated using the plasticity index (PI). Under fertilization addition, a positive PI indicates that trait values are higher than that of the control treatment (Lin et al., 2020). The PI was calculated following Godoy et al. (2011):
where ‘Mean (fertilization)’ and ‘Mean (N0P0)’ denote the mean functional traits of species under nutrient addition and N0P0 treatments, respectively, ‘Max [Mean (fertilization), Mean (N0P0)]’ represents the maximum mean values of the assessed traits in each treatment.
To determine the community functional structure, The ‘dbFD’ function in the FD package was used to calculate the CWM traits and FD in R 4.1.3(R Development Core Team). The contribution of ST and ITV in explaining variations in functional diversity and CWM traits was determined based on the method proposed by Lepš et al. (2011), which is based on the sum of squares decomposition method. The total Sum of Squares (SS) was the total variation of ITV, ST and covariation. Detailed information on this method is provided in the supplementary file.
The effects of N and P addition on CWM traits and FD were analyzed using an analysis of variance (ANOVA). The least significant difference (LSD) criterion was used for post hoc multiple comparisons of significant differences between different N and P treatments. The ANOVA was performed using GenStat version 18.0 (VSN International Ltd., Rothamsted, UK).
A principal component analysis (PCA) was conducted to correlate functional traits and relative biomass using CANOCO 5.0 (ter Braak and Smilauer, 2012). In order to evaluate the effects of the changes of different CWM traits and different FD caused by different N and P addition on community productivity, redundancy analysis (RDA) analysis was conducted to evaluate the effect of the changes of different CWM traits and different FD on community productivity via the “vegan” package in R 4.1.3. The relative contribution of CWM traits and different FD to community productivity were estimated on the basis of hierarchical partitioning via the “rdacca.hp” package in R 4.1.3 (Lai et al., 2022). Eventually, SEM (structural equation model) was constructed using Amos 24.0 (Amos Development Co., Greene, Maine, USA) to evaluate the relative importance and hypothetical paths of the community functional structure exhibiting significant independent effects and regulating community productivity. Root mean square error of approximation (RMSEA) and a chi-square (χ2) test with the associated probability were adopted to evaluate model fitness. The model fit was deemed acceptable when 0 ≤ χ2/df ≤ 2, 0 ≤ RMSEA ≤ 0.1 and χ2 and RMSEA values were nonsignificant (p>0.05).
3 Results
3.1 Trait plasticity
It was noted that N addition resulted in significant impacts on all functional traits except for SLA, SRA, RN and RP (p< 0.05; Table S4). LP, LN: LP, LTD, RP and RN: RP were the only functional traits significantly impacted following P addition (p<0.05; Table S4).
The PI of LN was greater than zero in all treatments for B. ischaemum (except for N0P40), P. tanacetifolia, and A. scoparia. The PI of LP for A. scoparia was greater than zero in all treatments, while the PI of LP was negative for the other five species when they were treated with N addition only. The PI of LN: LP was only higher than zero for all species treated only with N. The PI of SLA was greater than zero for all six species in all treatments, except for A. sacrorum treated with P-only (Figure 2).
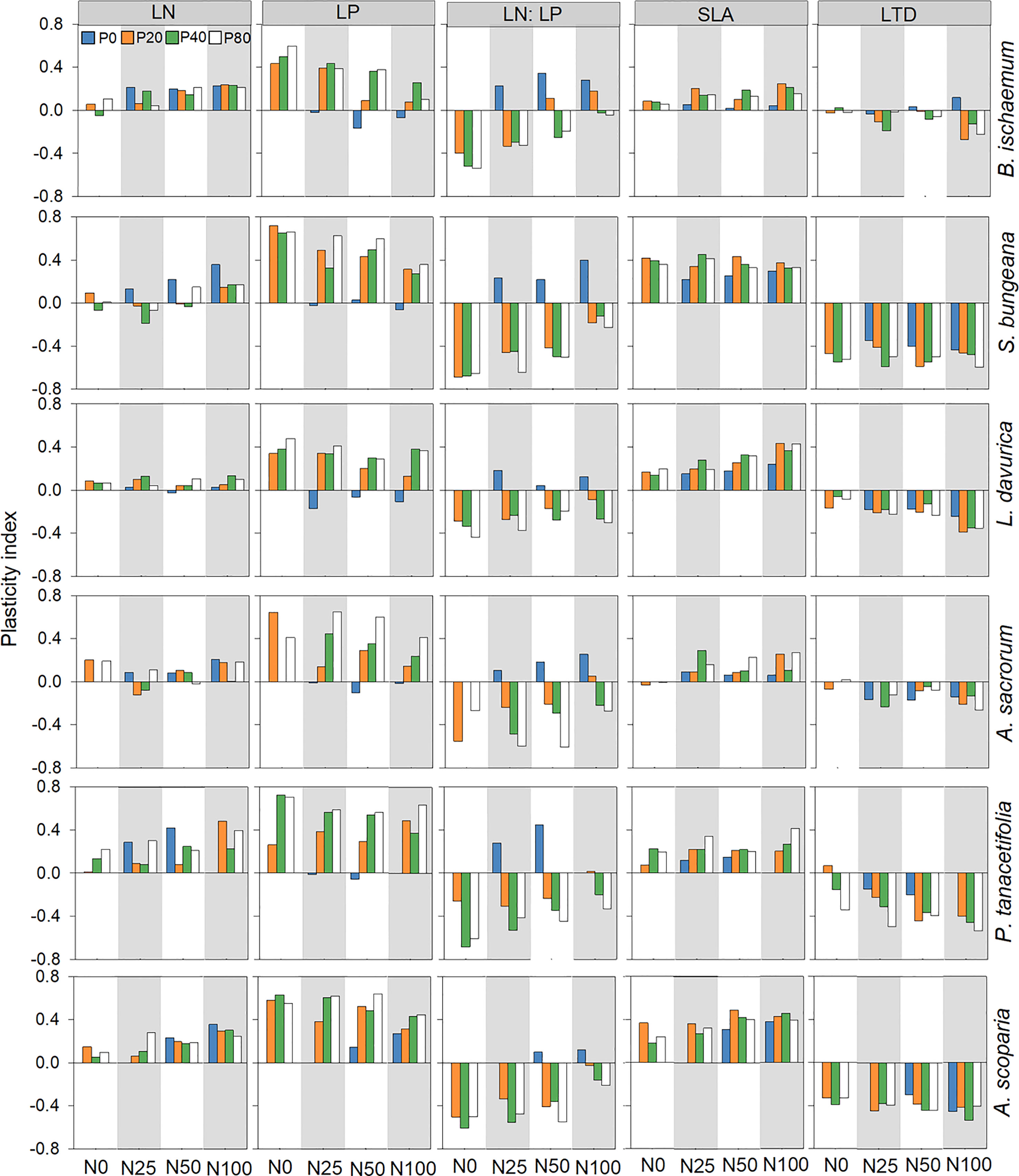
Figure 2 Leaf trait plasticity index of plant species following different levels of N and P addition. leaf P concentration (LP) Leaf N concentration (LN); leaf N:P ratio (LN: LP); specific leaf area (SLA); leaf tissue density (LTD).
The PI of RN was greater than zero for all species and treatment combinations except for B. ischaemum treated with N50P20, L. davurica treated with N0P80, N25P20, and N100P80, and A. scoparia treated with N0P40. Similarly, the PI of RP was greater than zero for most of the species and treatment combinations. However, the PI of RN: RP was generally higher than zero for all species treated only with N. The PI of SRL was greater than zero for all species and treatment combinations except for L. davurica treated with P0 and various levels of P in combination with N50 and P. tanacetifolia treated with N0P20 (Figure 3).
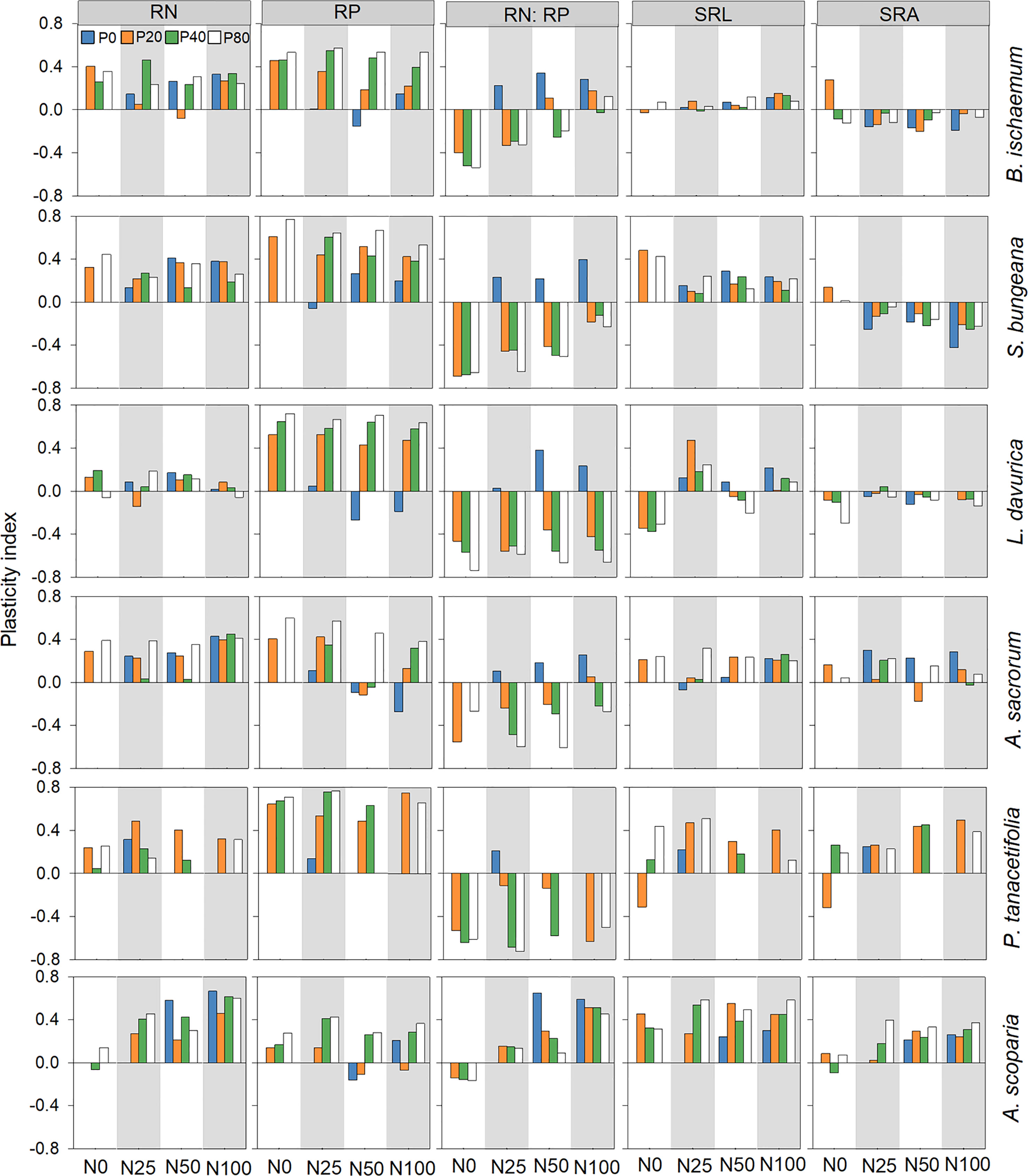
Figure 3 Root trait plasticity index of plant species following different levels of N and P addition. Root N concentration (RN); root P concentration (RP); root N:P ratio (RN: RP); specific root length (SRL); specific root surface area (SRA).
All six species showed substantial variations in PI. For instance, the PI of SLA of B. ischaemum was significantly lower than S. bungeana and A. scoparia (p<0.05; Figure 2; Table S5). It was also noted that the plasticity in LTD of S. bungeana exhibited significantly lower plasticity than other species for (p<0.05; Figure 2; Table S5), and the PI of SRL was significantly greater for S. bungeana and A. scoparia than B. ischaemum (p<0.05; Figure 3; Table S5).
3.2 Relative biomass and functional traits
The relative biomass of B. ischaemum was significantly higher in N25 and N50 treatments than that recorded for the N100 treatment, regardless of the level of P (p<0.05; Figure 4; Table S6). N and P interaction showed significant effects on the relative biomass of L. davurica, it was significantly lower in treatments involving N in combination with various P levels than in P-only treatments (p<0.05). In contrast, a combination of N and P resulted in significantly greater relative biomass in A. sacrorum compared to those treatments involving only one of these nutrient elements (p<0.05). The relative biomass of A. scoparia was significantly higher in treatments involving N100 with various P levels than in the P-only treatment (p<0.05; Figure 4; Table S6).
Different relationships between relative biomass and functional traits were recorded following different levels of N and P addition (Figure 5). The increase in the relative biomass was positively related to RP, Hmax and SLA in the N-only treatment (Figure 5A). The higher relative biomass was primarily due to an increase in LN: LP in the P-only treatment (Figure 5B). The higher relative biomass was correlated with high RP, SRL, and SRA in treatments involving N25 combined with P (Figure 5E). The increase in relative biomass was positively associated with RP, Hmax and SLA in N50 combined with P (Figure 5D). The higher relative biomass was mainly due to an increase in Hmax treated with various P levels in combination with N100 (Figure 5E).
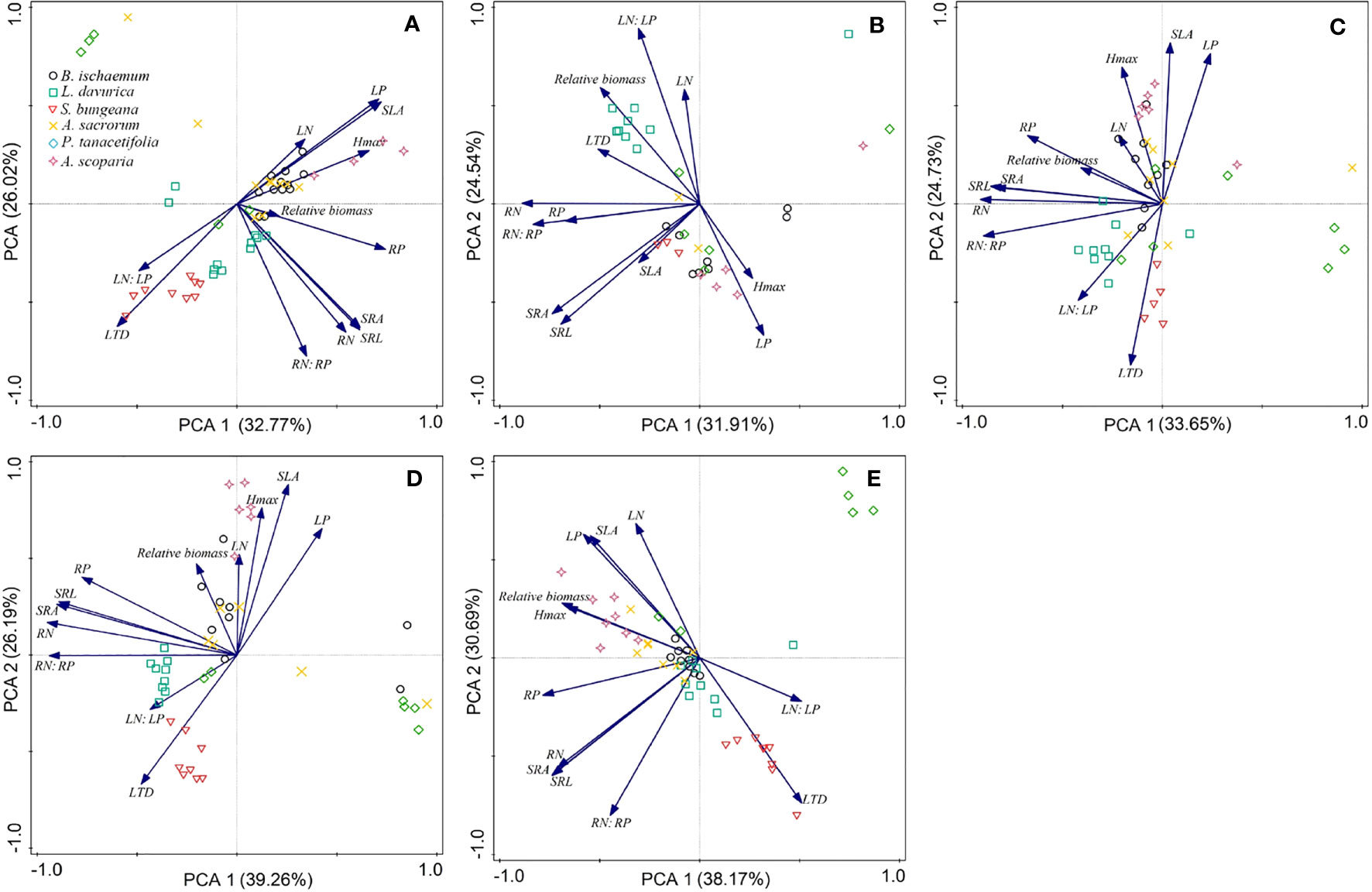
Figure 5 Principal components analysis (PCA) of functional traits and relative biomass of the six species after N and P addition. (A): only N; (B): only P; (C): N25 in combination with all levels of P; (D): N50 in combination with all levels of P; (E): N100 in combination with all levels of P.
3.3 Community functional structure
Adding N and P led to divergent CWM values for leaf traits and Hmax (Figure 6). The results revealed that the effects of N addition were significant for CWM_LN, CWM_LP, CWM_LN: LP, and CWM_Hmax (p<0.05), while P addition significantly impacted all leaf traits (p<0.05). The interaction between N and P addition significantly impacted CWM_LN: LP, CWM_LTD and CWM_Hmax (p< 0.05). CWM LN increased significantly after treatment with N or P alone than N0P0. Increasing level of P addition significantly promoted CWM_LP. CWM_SLA promoted significantly under all P levels as compared with P0. However, the impact of P addition on CWM_SLA was more pronounced at the highest level of N. CWM_Hmax increased significantly with increasing the level of N, with P addition synergizing the positive impact of N (Figure 6).
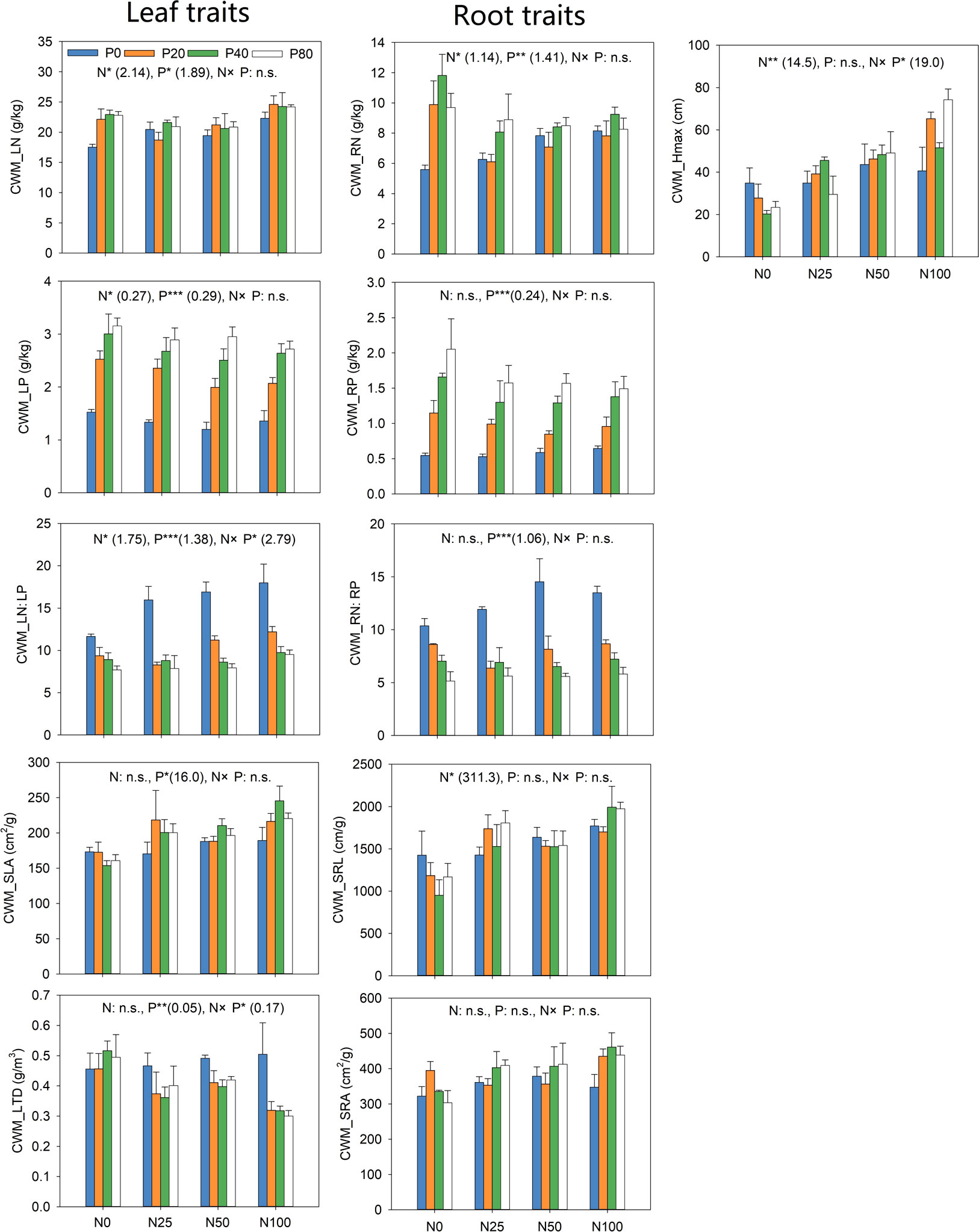
Figure 6 Response of community-weighted mean (CWM) traits to N and P addition. The numbers in brackets are the values of Least significant difference (LSD). *p<0.05; **p<0.01; ***p<0.001; n.s. not significant.
N addition only had significant effects on CWM_RN and CWM_SRL (p< 0.05). P addition significantly impacted CWM_RN, CWM_RP and CWM_RN: RP (p< 0.05). However, N addition only significantly decreased CWM_RN (Figure 6). All N levels promoted CWM_SRL significantly compared with N0. All P levels significantly enhanced CWM_RP. In contrast, increasing the level of P significantly reduced CWM_RN: RP (Figure 6).
N addition had a significant effect on FDiv (p<0.05; Figure 7), while the addition of P significantly impacted RaoQ and FDis (p<0.05). N and P interactions greatly affected FDiv (p<0.05). The greatest and lowest values for RaoQ and FDis at all P levels were recorded at N50 and N100, respectively. FDiv was generally lower in all N levels compared to the N0P0, with P addition exacerbating the reduction, especially at N100 (Figure 7).
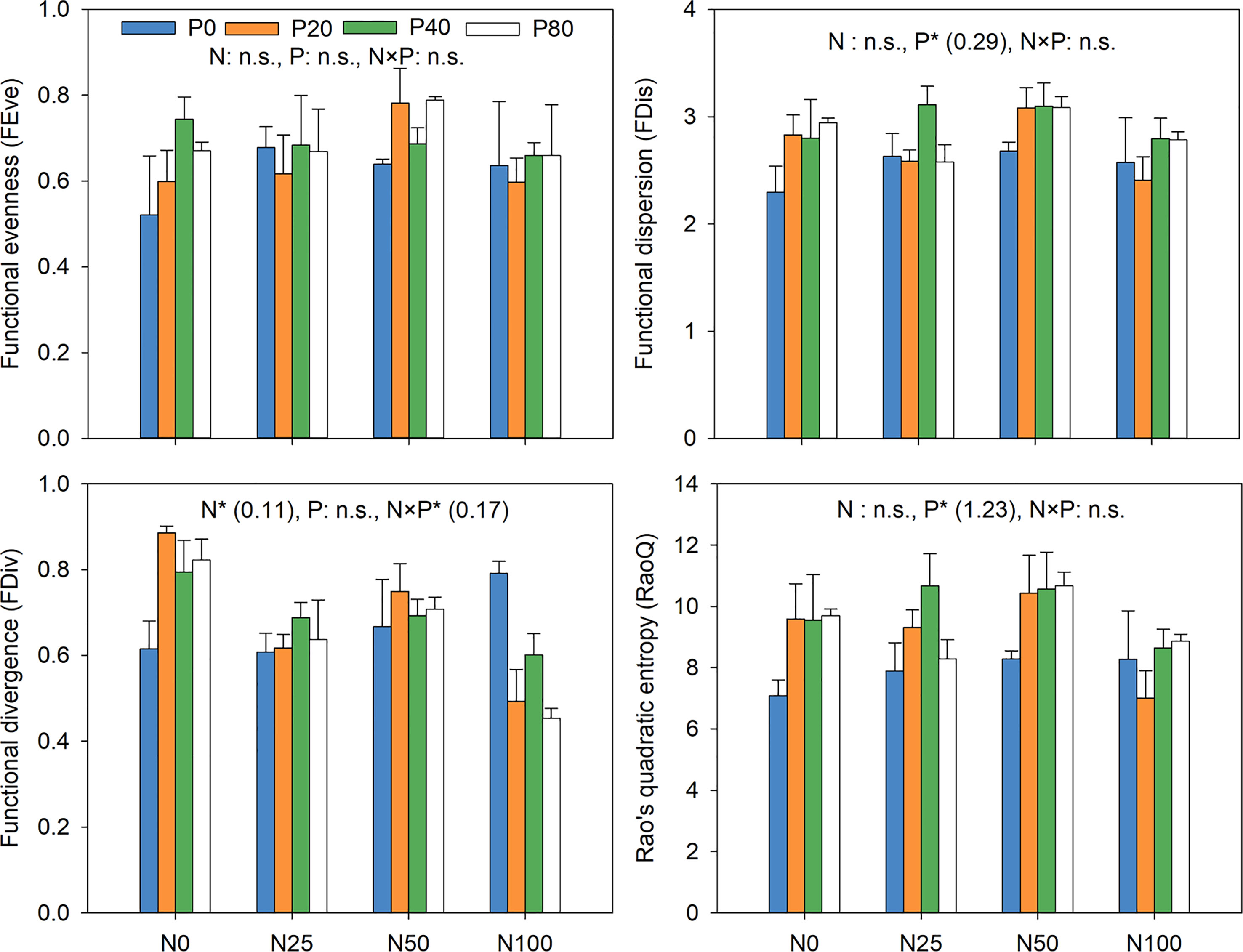
Figure 7 Response of functional diversity to N and P addition. Inset numbers in brackets show the values of Least significant difference (LSD). *p<0.05. n.s. not significant.
3.4 Relative contribution of variability explained by ST versus ITV
Under N addition, ITV significantly contributed to the variation in CWM_SLA (16%) and CWM_LTD (12%). Both ST and ITV significantly contributed to variations of CWM_LN, CWM_LP, CWM_LN: LP, and CWM_Hmax under N addition, though these variations were primarily driven by ITV (16-29%). positive covariance effects between ST and ITV were found for CWM_SLA, CWM_LTD and CWM_Hmax. Under P addition, ST explained more variations in CWM_LN (15%), while the contribution of ITV to the variations in CWM_LP (60%), CWM_LN: LP (75%), CWM_SLA (9%) and CWM_LTD (6%) was more significant. Covariance effects between ITV and ST were only negative for CWM_LN and CWM_LN: LP under P addition. Under N and P interactive effects. Covariance effects between ST and ITV were positive for CWM_Hmax and all CWM leaf trait values (except for CWM_LP) under the interactive effects of N and P addition (Figure 8).
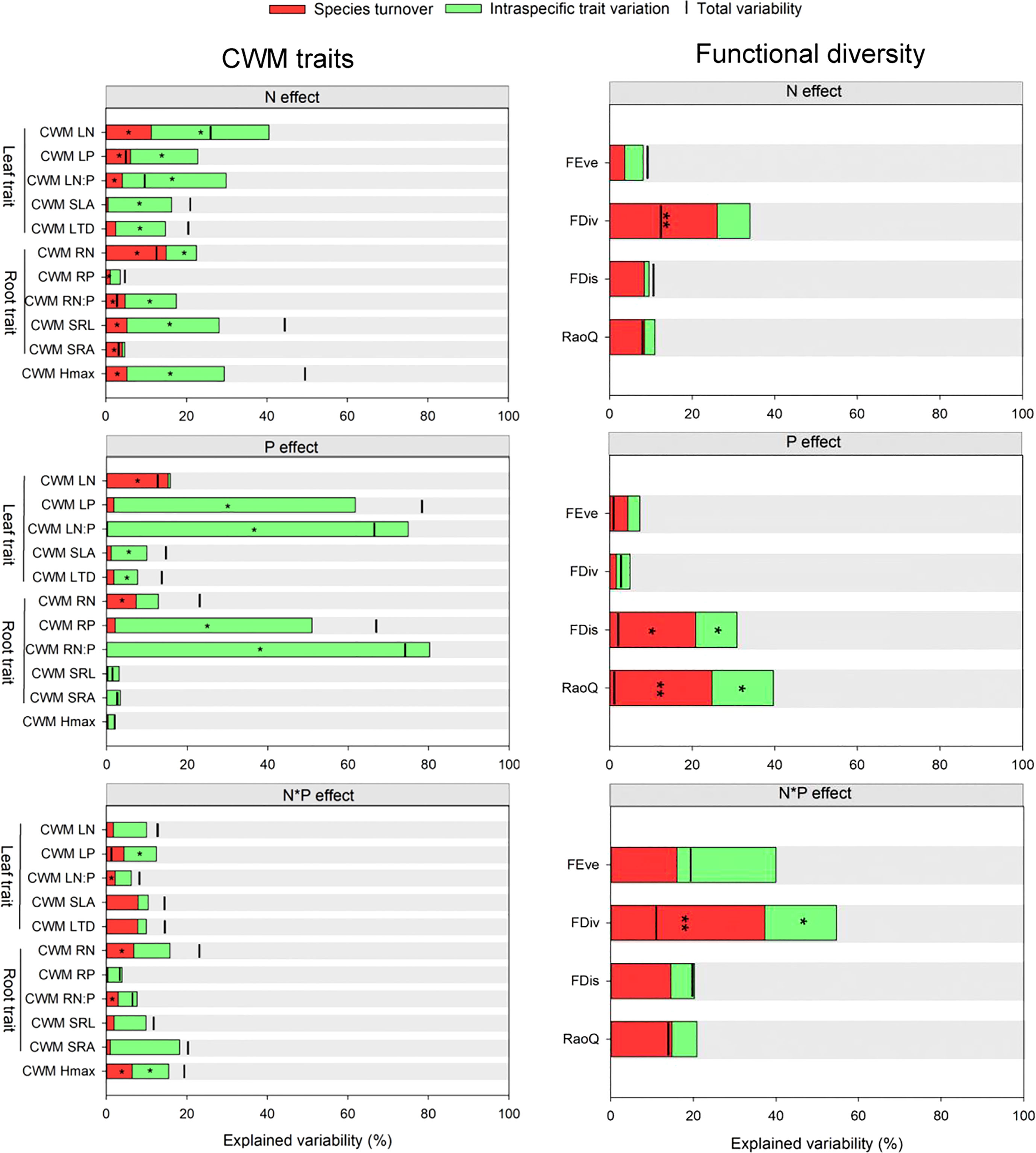
Figure 8 Variability in community-weighted mean (CWM) trait values based on intraspecific trait variation and species turnover. The distance between the bar and the top of the column represents covariation effects. Significant effect is indicated using * and ** at 5% and 1% level of probability.
ITV and ST had significant impacts on all CWM values for root traits except for CWM_RP and CWM_SRA under N addition. Under P addition, ITV contributed significantly to changes in CWM_RP (49%) and CWM_RN: RP (80%). Positive covariance effects between ITV and ST were only found for CWM_RP (Figure 8). ST had significant impacts on FDiv under N addition. ITV and ST significantly contributed to FDis and RaoQ under P addition, and ST primarily drove them. Under N and P addition interactive effects, ST significantly explained variability in FDiv (Figure 8).
3.5 Linkages between grassland productivity and community functional structure
Based on the hierarchical partitioning canonical analysis, CWM traits and FD indices explained 70.8% of variations in grassland productivity (Figure 9A). The variables which explained the highest part of the variability were CWM_Hmax (R2 = 0.232, p< 0.01), followed by CWM_SLA (R2 = 0.206, p< 0.01), FDiv (R2 = 0.148, p< 0.01) and CWM_LN (R2 = 0.049, p< 0.05). The model explained 74% of total variations in grassland productivity (Figure 9B). According to the model, both N and P additions significantly promoted CWM_Hmax and CWM_LN, and both traits positively affected grassland productivity. High grassland productivity was also positively associated with increasing CWM_SLA induced by N addition. However, N addition indirectly influenced grassland productivity through its negative effect on FDiv.
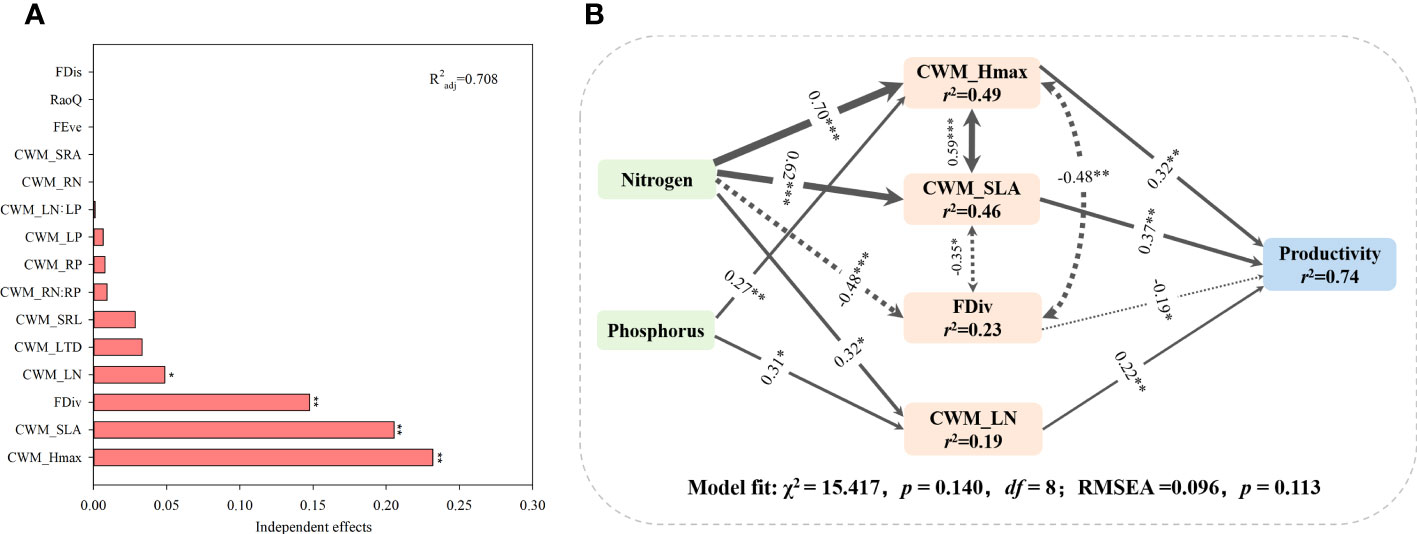
Figure 9 (A) Independent effects of functional diversity and CWM traits on productivity were evaluated on the basis of hierarchical partitioning. (B) Structural equation model (SEM) indicating the relationship between nutrient addition, CWM_Hmax, CWM_SLA, Fdiv, CWM_LN and community productivity (χ2 = 15.417, df =8, p = 0.140). Solid and dashed arrows represent significant positive and negative pathways at *p< 0.05; **p< 0.01; ***p< 0.001.
4 Discussion
4.1 Trait plasticity regulates species-specific responses to N and P addition
Plant response to N and P addition is manifested by the patterns of trait plasticity (Lin et al., 2020). This research revealed that the patterns of plasticity varied greatly among different plant traits and species (Figures 2, 3). For instance, the plasticity indices of LN, LP, RN, and RP were greater than zero under N and P addition, indicating that the addition of both elements promoted the nutrient absorption capacity of plants. This research also revealed that the plasticity indices of RN and RP were higher than those of LN and LP, which is possibly due to (1) competition between the leaf and root for nutrients, (2) the root system having the advantage of being close to the nutrient source, and (3) fine roots being the most sensitive plant part to nutrient addition (Kleyer and Minden, 2015; Kou et al., 2018). Moreover, the plasticity indices of LN: LP and RN: RP were higher than zero in the N-only treatment, primarily owing to greater plasticity recorded for LN and RN under N addition than LP and RP. RN, RP, and SRL mostly performed greater plasticity than zero, which indicated the enhancement of nutrient absorption capacity after fertilization, and the greater plasticity of RN and RP compared with SRL and SRA (Figure 3), indicated that root nutrient concentrations are more plastic than root morphological traits under nutrient addition (Kramer-Walter and Laughlin, 2017). These results indicate that functional traits’ response to nutrients is trait-specific, and overall plant adaptation strategies can be formed by the coordination between leaf and root traits (Faucon et al., 2017).
Functional traits determine plant resource use and growth strategies under different fertilization levels (Funk et al., 2017). In this regard, plant strategies for nutrient uptake can be categorized into acquisitive and conservative strategies (Seastedt et al., 2020), with acquisitive plant species generally benefiting from higher functional trait plasticity (e.g., SLA and SRL) (Wang et al., 2018). B. ischaemum exhibited lower trait plasticity (e.g., SLA, LTD, SRL and SRA), implying that this species was conservative species. Conservative perennial plant species have high resource utilization efficiency with low requirements; however, at higher levels of fertilization, they are at competitive disadvantages with acquisitive species (Gonzalez-Paleo and Ravetta, 2018). Thus the relative biomass of B. ischaemum increased and benefited from low fertilization levels, while decreased at higher levels of fertilization. While the relative biomass of L. davurica was higher in the P-only treatment and N25 combined with P, which was associated with higher RP, SRL, and SRA (Figure 4). This was likely owing to the positive impacts of P on the biological N2-fixation performance of this legume species (Chen et al., 2020). In comparison, in nutrient-rich environments, plants with acquisitive traits (e.g., greater SLA) usually grow faster and gain competitive advantages (Wang et al., 2018). As a result, A. scoparia and A. sacrorum performed greater relative biomass at high levels of N and P, indicating that these two species was acquisitive plant species characterized by higher functional trait plasticity and higher Hmax and SLA (Figure 4). We also noted that both A. scoparia and A. sacrorum gained greater SLA at higher levels of N and P.
4.2 Variation of community functional structure following fertilization were mainly from ITV
Unravelling the specific roles of ITV and ST in community functional composition shifts is essential for understanding the community’s response to environmental changes (Delpiano et al., 2020). It has been suggested that higher adaptation abilities of plant species in response to fertilizer addition positively correlate with relative contributions of ITV to variations in community-level mean trait values (Lü et al., 2018). In contrast, the relative contributions of ST to improving species tolerance in changing environments are of minor importance (Kichenin et al., 2013). Our research revealed that ITV significantly contributed to variations in most plant traits under N and P addition. For instance, RP and SLA increased significantly at the community level with N and P addition, and their variability was primarily explained by ITV. This suggested that these traits are more sensitive to N and P addition. However, there were a few traits whose variations under nutrient addition were primarily driven by ST. For instance, LN and RN increased significantly at the community level with P addition, and their variation was mainly attributed to ST.
A positive covariance effect between ITV and ST for CWM traits exhibited synergistic responses of these traits following nutrient addition, whereas negative covariations indicate opposite directions of ITV and ST in trait selection (Lepš et al., 2011). For instance, we recorded positive covariations between ITV and ST for CWM_Hmax under the interactive effects of N and P addition, suggesting that these effects reinforced each other, leading to an increase in Hmax at the community level following the addition of both nutrient elements. On the other hand, we recorded negative covariations between ITV and ST for CWM_LN and CWM_LN: LP under N and P addition, suggesting there were contrasting trends through ST and ITV, leading to weakening responses a decreasing trend of these leaf traits at the community level following nutrient addition (Siefert and Ritchie, 2016).
This research showed that ITV primarily affected these variations of community functional composition under four years nutrient addition experiments that the results were consistent with that ITV played a pivotal role in community functional composition variability during short-term nutrient addition, while ST became more prominent during long-term fertilization Zhou et al. (2018). The dominant effect of ITV also revealed that neglecting the role of ITV in community variability leads to a great underestimation of community functional composition responses to changing environments Lepš et al. (2011). However, factors such as functional traits and spatial scale play a role in the explanatory contribution of ITV and ST (Zhou et al., 2018).
4.3 CWM traits and functional diversity together effect diversity and productivity
Since grassland productivity is a prominent indicator of vigorous grassland ecosystems and grassland restoration(Schaub et al., 2020; Yang et al., 2022a), evaluating the impact of nutrient-induced variations in community functional structure can inform us of grassland ecosystem functioning. CWM traits and functional diversity have primarily been used to unravel the relationship between functional traits and grassland ecosystem functioning (Wang et al., 2020). We found that CWM traits and functional diversity play a pivotal contrasting role in explaining variations in grassland productivity under nutrient addition (Figure 9). On one hand, increasing CWM_SLA and CWM_Hmax after nutrient addition directly promoted community productivity. This is because CWM_SLA and CWM_Hmax are essential factors in determining the competitive ability of species for light (Zheng and Ma, 2018). La Pierre and Smith (2015) also demonstrated that nutrient addition promoted rapid plant growth and high community productivity typically through greater SLA and height. High CWM_SLA is generally associated with an enhanced photosynthetic rate and rapid biomass production (Funk et al., 2017; Mao et al., 2017). Plant height is a crucial factor determining plant competitive abilities (Siefert and Ritchie, 2016; Lin et al., 2020; Liu et al., 2021). Tall species can capture and incest more light energy into biomass production (DeMalach et al., 2017).
On the other hand, N addition led to a reduction in FDiv. Low FDiv values indicate a low degree of niche differentiation and, thus, high competition for resources such as light and nutrients (Mouchet et al., 2010). One possible explanation is that nutrient addition favours species with highly competitive abilities (i.e., species with higher Hmax and SLA), leading to excluding species with low competitive abilities (Turcotte and Levine, 2016; Read et al., 2017; Liu et al., 2021). The results of this research showed that CWM traits work in concert with functional diversity to influence grassland productivity; thus, it is essential to consider both trait-based metrics to understand the effect of environmental changes on grassland ecosystem functioning.
5 Conclusion
In this research, we conducted a four-year nutrient addition study to assess community functional structures and grassland productivity under N and P addition in a grassland ecosystem in the Loess Plateau. This study proved that aboveground (i.e., leaf) and belowground (i.e., root system) traits exhibit coordinated variations under N and P addition, with ITV predominantly driving the variations in community functional structures in response to nutrient addition. We also found that variations in CWM traits and functional diversity jointly affect the response of grassland productivity to nutrient addition. Future studies should involve further long-term studies and additional traits data under different environments in order to extend the finding of this research to broader regions.
Data availability statement
The raw data supporting the conclusions of this article will be made available by the authors, without undue reservation.
Author contributions
YY, ZC, BX and ZW planned and designed the research and guided the entire process of the study; CD, WL and RZ conducted the experiments and finished the material collection, YY, HG and ZW analyzed data, wrote and edited the manuscript. All authors contributed to the article and approved the submitted version.
Funding
Financial support came from the National Natural Science Foundation of China Projects (Nos. 41977077, 41671289 and 42277464).
Conflict of interest
The authors declare that the research was conducted in the absence of any commercial or financial relationships that could be construed as a potential conflict of interest.
Publisher’s note
All claims expressed in this article are solely those of the authors and do not necessarily represent those of their affiliated organizations, or those of the publisher, the editors and the reviewers. Any product that may be evaluated in this article, or claim that may be made by its manufacturer, is not guaranteed or endorsed by the publisher.
Supplementary material
The Supplementary Material for this article can be found online at: https://www.frontiersin.org/articles/10.3389/fpls.2023.1145709/full#supplementary-material
References
Albert, C. H., Thuiller, W., Yoccoz, N. G., Douzet, R., Aubert, S., Lavorel, S. (2010). A multi-trait approach reveals the structure and the relative importance of intra-vs. interspecific variability Plant traits. Funct. Ecology. 24, 1192–1201. doi: 10.1111/j.1365-2435.2010.01727.x
Caplan, J. S., Meiners, S. J., Flores-Moreno, H., McCormack, M. L. (2019). Fine-root traits are linked to species dynamics in a successional plant community. Ecology. 100 (3), e02588. doi: 10.1002/ecy.2588
Chen, Z., Xiong, P., Zhou, J., Lai, S., Jian, C., Xu, W., et al. (2021). Effects of plant diversity on semiarid grassland stability depends on functional group composition and dynamics under N and P addition. Sci. total environment. 799, 149482. doi: 10.1016/j.scitotenv.2021.149482
Chen, Z., Xiong, P., Zhou, J., Yang, Q., Wang, Z., Xu, B. (2020). Grassland productivity and diversity changes in responses to N and P addition depend primarily on tall clonal and annual species in semiarid Loess Plateau. Ecol. Engineering. 145, 105727. doi: 10.1016/j.ecoleng.2020.105727
Chen, Z., Zhou, J., Lai, S., Jian, C., Chen, Y., Luo, Y., et al. (2023). Species differences in stoichiometric homeostasis affect grassland community stability under N and P addition. Environ. Sci. Pollut. Res. 30, 61913–61926. doi: 10.1007/s11356-023-26479-3
Delpiano, C. A., Prieto, I., Loayza, A. P., Carvajal, D. E., Squeo, F. A. (2020). Different responses of leaf and root traits to changes in soil nutrient availability do not converge into a community-level plant economics spectrum. Plant Soil. 450, 463–478. doi: 10.1007/s11104-020-04515-2
DeMalach, N., Zaady, E., Kadmon, R. (2017). Light asymmetry explains the effect of nutrient enrichment on grassland diversity. Ecol. Letters. 20 (1), 60–69. doi: 10.1111/ele.12706
DeSiervo, M. H., Sullivan, L. L., Kahan, L. M., Seabloom, E. W., Shoemaker, L. G. (2023). Disturbance alters transience but nutrients determine equilibria during grassland succession with multiple global change drivers. Ecol. Lett. 26 (7), 1132–1144. doi: 10.1111/ele.14229
Diaz, S., Lavorel, S., de Bello, F., Quetier, F., Grigulis, K., Robson, M. (2007). Incorporating plant functional diversity effects in ecosystem service assessments. Proc. Natl. Acad. Sci. United States America 104, 20684–20689. doi: 10.1073/pnas.0704716104
Faucon, M. P., Houben, D., Lambers, H. (2017). Plant functional traits: soil and ecosystem services. Trends Plant Science. 22 (5), 385–394. doi: 10.1016/j.tplants.2017.01.005
Fay, P. A., Prober, S. M., Harpole, W. S., Knops, J. M. H., Bakker, J. D., Borer, E. T., et al. (2015). Grassland productivity limited by multiple nutrients. Nat. Plants 1 (7), 15080. doi: 10.1038/nplants.2015.80
Funk, J. L., Larson, J. E., Ames, G. M., Butterfield, B. J., Cavender-Bares, J., Firn, J., et al. (2017). Revisiting the Holy Grail: using plant functional traits to understand ecological processes. Biol. Rev. 92 (2), 1156–1173. doi: 10.1111/brv.12275
Gang, C., Zhao, W., Zhao, T., Zhang, Y., Gao, X., Wen, Z. (2018). The impacts of land conversion and management measures on the grassland net primary productivity over the Loess Plateau, Northern China. Sci. Total Environment. 645, 827–836. doi: 10.1016/j.scitotenv.2018.07.161
Godoy, O., Valladares, F., Castro-Díez, P. (2011). Multispecies comparison reveals that invasive and native plants differ in their traits but not in their plasticity. Funct. Ecology. 25 (6), 1248–1259. doi: 10.1111/j.1365-2435.2011.01886.x
Gonzalez-Paleo, L., Ravetta, D. A. (2018). Relationship between photosynthetic rate, water use and leaf structure in desert annual and perennial forbs differing in their growth. Photosynthetica. 56 (4), 1177–1187. doi: 10.1007/s11099-018-0810-z
Grime, J. P. (1998). Benefits of plant diversity to ecosystems: immediate, filter and founder effects. J. Ecology. 86 (6), 902–910. doi: 10.1046/j.1365-2745.1998.00306.x
Guo, Q., Wen, Z., Ghanizadeh, H., Fan, Y., Zheng, C., Yang, X., et al. (2022a). Stochastic processes dominate assembly of soil fungal community in grazing excluded grasslands in northwestern China. J. Soils Sediments 23 (1), 156–171. doi: 10.1007/s11368-022-03315-8
Guo, Q., Wen, Z., Ghanizadeh, H., Zheng, C., Fan, Y., Yang, X., et al. (2022b). Shift in microbial communities mediated by vegetation-soil characteristics following subshrub encroachment in a semi-arid grassland. Ecol. Indicators. 137, 108768. doi: 10.1016/j.ecolind.2022.108768
Han, X., Tsunekawa, A., Tsubo, M., Li, S. (2011). Aboveground biomass response to increasing nitrogen deposition on grassland on the northern Loess Plateau of China. Acta Agriculturae Scandinavica Section B — Soil Plant Science. 61 (2), 112–121. doi: 10.1080/09064710903544201
Isbell, F., Reich, P. B., Tilman, D., Hobbie, S. E., Polasky, S., Binder, S. (2013). Nutrient enrichment, biodiversity loss, and consequent declines in ecosystem productivity. Proc. Natl. Acad. Sci. United States America. 110 (29), 11911–11916. doi: 10.1073/pnas.1310880110
Kichenin, E., Wardle, D. A., Peltzer, D. A., Morse, C. W., Freschet, G. T. (2013). Contrasting effects of plant inter- and intraspecific variation on community-level trait measures along an environmental gradient. Funct. Ecology. 27 (5), 1254–1261. doi: 10.1111/1365-2435.12116
Kleyer, M., Minden, V. (2015). Why functional ecology should consider all plant organs: An allocation-based perspective. Basic Appl. Ecol. 16 (1), 1–9. doi: 10.1016/j.baae.2014.11.002
Kou, L., Jiang, L., Fu, X., Dai, X., Wang, H., Li, S. (2018). Nitrogen deposition increases root production and turnover but slows root decomposition in Pinus elliottii plantations. New Phytologist. 218 (4), 1450–1461. doi: 10.1111/nph.15066
Kramer-Walter, K. R., Laughlin, D. C. (2017). Root nutrient concentration and biomass allocation are more plastic than morphological traits in response to nutrient limitation. Plant Soil. 416, 539–550. doi: 10.1007/s11104-017-3234-9
Lai, J., Zou, Y., Zhang, J., Peres-Neto, P. R. (2022). Generalizing hierarchical and variation partitioning in multiple regression and canonical analyses using the rdacca.hp R package. Methods Ecol. Evolution. 13 (4), 782–788. doi: 10.1111/2041-210X.13800
La Pierre, K. J., Smith, M. D. (2015). Functional trait expression of grassland species shift with short- and long-term nutrient additions. Plant Ecology. 216 (2), 307–318. doi: 10.1007/s11258-014-0438-4
Lavorel, S., Garnier, E. (2002). Predicting changes in community composition and ecosystem functioning from plant traits: revisiting the Holy Grail. Funct. Ecology. 16 (5), 545–556. doi: 10.1046/j.1365-2435.2002.00664.x
Lepš, J., de Bello, F., Šmilauer, P., Doležal, J. (2011). Community trait response to environment: disentangling species turnover vs intraspecific trait variability effects. Ecography 34 (5), 856–863. doi: 10.1111/j.1600-0587.2010.06904.x
Liang, T., Tong, Y., Liu, X. (2016). High nitrogen deposition in an agricultural ecosystem of Shaanxi, China. Environ. Sci. pollut. R 23, 13210–13221. doi: 10.1007/s11356-016-6374-1
Lin, G., Zeng, D.-H., Mao, R. (2020). Traits and their plasticity determine responses of plant performance and community functional property to nitrogen enrichment in a boreal peatland. Plant Soil. 449 (1-2), 151–167. doi: 10.1007/s11104-020-04478-4
Liu, Z.-P., Shao, M.-A., Wang, Y.-Q. (2013). Spatial patterns of soil total nitrogen and soil total phosphorus across the entire Loess Plateau region of China. Geoderma. 197, 67–78. doi: 10.1016/j.geoderma.2012.12.011
Liu, J., Yang, X., Ghanizadeh, H., Guo, Q., Fan, Y., Zhang, B., et al. (2021). Long-term enclosure can benefit grassland community stability on the Loess Plateau of China. Sustainability. 13 (1), 213. doi: 10.3390/su13010213
Lü, X.-T., Hu, Y.-Y., Zhang, H.-Y., Wei, H., Hou, S.-L., Yang, G.-J., et al. (2018). Intraspecific variation drives community-level stoichiometric responses to nitrogen and water enrichment in a temperate steppe. Plant Soil. 423 (1-2), 307–315. doi: 10.1007/s11104-017-3519-z
Mao, W., Felton, A. J., Zhang, T. (2017). Linking changes to intraspecific trait diversity to community functional diversity and biomass in response to snow and nitrogen addition within an Inner Mongolian Grassland. Front. Plant Sci. 8, 339. doi: 10.3389/fpls.2017.00339
Mouchet, M. A., Villéger, S., Mason, N. W. H., Mouillot, D. (2010). Functional diversity measures: an overview of their redundancy and their ability to discriminate community assembly rules. Funct. Ecology. 24 (4), 867–876. doi: 10.1111/j.1365-2435.2010.01695.x
Niu, K., Choler, P., de Bello, F., Mirotchnick, N., Du, G., Sun, S. (2014). Fertilization decreases species diversity but increases functional diversity: A three-year experiment in a Tibetan alpine meadow. Agriculture Ecosyst. Environment. 182, 106–112. doi: 10.1016/j.agee.2013.07.015
Pérez-Harguindeguy, N., Díaz, S., Garnier, E., Lavorel, S., Poorter, H., Jaureguiberry, P., et al. (2013). New handbook for standardised measurement of plant functional traits worldwide. Aust. J. Botany. 61 (3), 167–234. doi: 10.1071/BT12225
Petchey, O. L., Gaston, K. J. (2006). Functional diversity: back to basics and looking forward. Ecol. Letters. 9 (6), 741–758. doi: 10.1111/j.1461-0248.2006.00924.x
Read, Q. D., Henning, J. A., Sanders, N. J. (2017). Intraspecific variation in traits reduces ability of trait-based models to predict community structure. J. Vegetation Science. 28 (5), 1070–1081. doi: 10.1111/jvs.12555
Roscher, C., Schumacher, J., Gubsch, M., Lipowsky, A., Weigelt, A., Buchmann, N., et al. (2012). Using plant functional traits to explain diversity-productivity relationships. PloS ONE 7, e36760. doi: 10.1371/journal.pone.0036760
Rowe, E. C., Healey, J. R., Edwards-Jones, G., Hills, J., Howells, M., Jones, D. L. (2006). Fertilizer application during primary succession changes the structure of plant and herbivore communities. Biol. Conserv. 131 (4), 510–522. doi: 10.1016/j.biocon.2006.02.023
Schaub, S., Finger, R., Leiber, F., Probst, S., Kreuzer, M., Weigelt, A., et al. (2020). Plant diversity effects on forage quality, yield and revenues of semi-natural grasslands. Nat. Commun. 11 (1), 768. doi: 10.1038/s41467-020-14541-4
Seastedt, T. R., White, C. T., Tucker, C., Beaury, E. M., Concilio, A. L., Gasarch, E. I., et al. (2020). Decadal dynamics of dry alpine meadows under nitrogen and phosphorus additions. Plant Ecology. 221 (8), 647–658. doi: 10.1007/s11258-020-01039-8
Siefert, A., Ritchie, M. E. (2016). Intraspecific trait variation drives functional responses of old-field plant communities to nutrient enrichment. Oecologia. 181 (1), 245–255. doi: 10.1007/s00442-016-3563-z
Smith, M. D., Knapp, A. K., Collins, S. L. (2009). A framework for assessing ecosystem dynamics in response to chronic resource alterations induced by global change. Ecology 90, 3279–3298. doi: 10.1890/08-1815.1
Stevens, C. J. (2016). How long do ecosystems take to recover from atmospheric nitrogen deposition? Biol. Conserv. 200, 160–167. doi: 10.1016/j.biocon.2016.06.005
ter Braak, C. J. F., Smilauer, P. (2012). Canoco reference manual and user’s guide: Software for ordination (version 5.0). Microcomputer Power (Ithaca, NY, USA).
Turcotte, M. M., Levine, J. M. (2016). Phenotypic plasticity and species coexistence. Trends Ecol. Evolution. 31 (10), 803–813. doi: 10.1016/j.tree.2016.07.013
Vitousek, P. M., Porder, S., Houlton, B. Z., Chadwick, O. A. (2010). Terrestrial phosphorus limitation: mechanisms, implications, and nitrogen-phosphorus interactions. Ecol. Applications. 20 (1), 5–15. doi: 10.1890/08-0127.1
Wang, W., Liu, H., Zhang, J., Li, Z., Wang, L., Wang, Z., et al. (2020). Effect of grazing types on community-weighted mean functional traits and ecosystem functions on inner Mongolian steppe, China. Sustainability 12 (17), 7169. doi: 10.3390/su12177169
Wang, F., Shi, G., Nicholas, O., Yao, B., Ji, M., Wang, W., et al. (2018). Ecosystem nitrogen retention is regulated by plant community trait interactions with nutrient status in an alpine meadow. J. Ecology. 106 (4), 1570–1581. doi: 10.1111/1365-2745.12924
Wang, C., Zhu, F., Zhao, X., Dong, K. (2014). The effects of N and P additions on microbial N transformations and biomass on saline-alkaline grassland of Loess Plateau of Northern China. Geoderma. 213, 419–425. doi: 10.1016/j.geoderma.2013.08.003
Weemstra, M., Mommer, L., Visser, E. J. W., van Ruijven, J., Kuyper, T. W., Mohren, G. M. J., et al. (2016). Towards a multidimensional root trait framework: a tree root review. New Phytologist. 211 (4), 1159–1169. doi: 10.1111/nph.14003
Wellstein, C., Schröder, B., Reineking, B., Zimmermann, N. E. (2011). Understanding species and community response to environmental change – A functional trait perspective. Agriculture Ecosyst. Environment. 145 (1), 1–4. doi: 10.1016/j.agee.2011.06.024
Yang, Y., Chen, Z., Xu, B., Wei, J., Zhu, X., Yao, H., et al. (2022b). Using trait-based methods to study the response of grassland to fertilization in the grassland in semiarid areas in the Loess Plateau of China. Plants-Basel. 11 (15), 2045. doi: 10.3390/plants11152045
Yang, X., Yan, X., Guo, Q., Ghanizadeh, H., Li, M., Tuo, H., et al. (2022a). Effects of different management practices on plant community and soil properties in a restored grassland. J. Soil Sci. Plant Nutr. 22 (3), 3811–3821. doi: 10.1007/s42729-022-00932-7
Zhang, W., Ren, C., Deng, J., Zhao, F., Yang, G., Tong, X., et al. (2018). Plant functional composition and species diversity affect soil C, N, and P during secondary succession of abandoned farmland on the Loess Plateau. Ecol. Engineering. 122, 91–99. doi: 10.1016/j.ecoleng.2018.07.031
Zheng, Z., Ma, P. (2018). Changes in above and belowground traits of a rhizome clonal plant explain its predominance under nitrogen addition. Plant Soil. 432 (1-2), 415–424. doi: 10.1007/s11104-018-3815-2
Keywords: leaf traits, root traits, community functional structure, fertilization, semiarid grassland
Citation: Yang Y, Chen Z, Xu B, Ghanizadeh H, Li W, Ding C, Zhou R and Wen Z (2023) Contrasting patterns of community-weighted mean traits and functional diversity in driving grassland productivity changes under N and P addition. Front. Plant Sci. 14:1145709. doi: 10.3389/fpls.2023.1145709
Received: 16 January 2023; Accepted: 28 July 2023;
Published: 15 August 2023.
Edited by:
Luís Silva, University of the Azores, PortugalReviewed by:
Z. Y. Yuan, Chinese Academy of Sciences (CAS), ChinaFurong Niu, University of Arizona, United States
Copyright © 2023 Yang, Chen, Xu, Ghanizadeh, Li, Ding, Zhou and Wen. This is an open-access article distributed under the terms of the Creative Commons Attribution License (CC BY). The use, distribution or reproduction in other forums is permitted, provided the original author(s) and the copyright owner(s) are credited and that the original publication in this journal is cited, in accordance with accepted academic practice. No use, distribution or reproduction is permitted which does not comply with these terms.
*Correspondence: Zhongming Wen, em13ZW5AbXMuaXN3Yy5hYy5jbg==