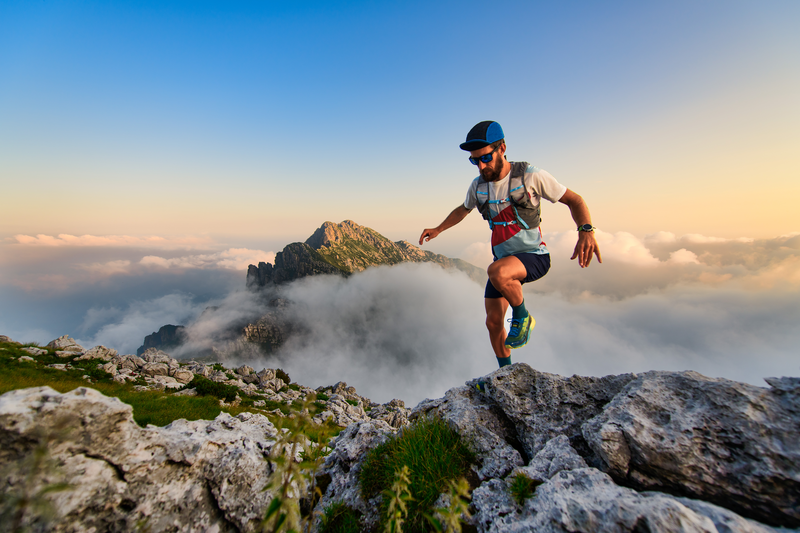
95% of researchers rate our articles as excellent or good
Learn more about the work of our research integrity team to safeguard the quality of each article we publish.
Find out more
ORIGINAL RESEARCH article
Front. Plant Sci. , 13 June 2023
Sec. Plant Abiotic Stress
Volume 14 - 2023 | https://doi.org/10.3389/fpls.2023.1144379
Brassinazole-resistant (BZR) transcription factors (TFs) are key players in brassinolides (BRs) signaling pathway, which is widely involved in regulating plant growth and development, as well as in plant responding to a variety stresses. Despite their critical roles, little is known about BZR TFs in wheat. In this study, we performed genome-wide analysis of BZR gene family from wheat genome, and 20 TaBZRs were identified. Based on the phylogenetic relationships of TaBZR and BZRs from rice and Arabidopsis, all BZR genes were clustered into four groups. The intron-exon structural patterns and conserved protein motifs of TaBZRs showed high group specificity. TaBZR5, 7, and 9 were significantly induced after salt, drought treatment, and stripe rust infection. However, TaBZR16, which was significantly upregulated under NaCl application, was not expressed during wheat-stripe rust fungus interaction. These results indicated that BZR genes in wheat play different roles in response to various stresses. The results of this study will lay a foundation for further in-depth functional studies of TaBZRs and will provide information for the breeding and genetic improvement of wheat against drought and salt stresses.
The brassinosteroids (BRs) are plant specific and are widely distributed among plants (Clouse and Sasse, 1998; Schaller, 2003; Agarwal et al., 2006). BRs play fundamental roles including regulating seed germination, cell growth, reproduction, senescence, and root growth (Nolan et al., 2020; Hu et al., 2021). Current studies have shown that BR mediates abiotic stresses in plants and play crucial role in resistance to temperature changes, salinity, drought, and organic pollutants (Vardhini and Anjum, 2015; Lee et al., 2018; Ahammed et al., 2020).
Brassinazole-resistant (BZR) transcription factors play important roles in the BR signaling pathway through regulating the expression of BR genes (Yin et al., 2005; Li et al., 2018; Yu et al., 2019). They positive regulate BR signaling and participate in a variety of stress response pathways (He et al., 2005; Miyaji et al., 2014; Singh et al., 2014; Li et al., 2017). In Arabidopsis thaliana, BZR1 and BRI1-EMS-SUPPRESSOR 1 (BES1) are well characterized members in the BZR family, with at least four other homologs designated as BES1/BZR1 Homolog1 (BEH1) to BEH4 (Yin et al., 2005; Sun et al., 2010). The N-terminal domain with DNA-binding activity of BZR protein is highly conserved (He et al., 2005; Yin et al., 2005). BZR proteins also contain glycosylation sites of the Glycogen synthase kinase 3 (GSK3), which consists of 22-24 amino acids. Besides, some BZR members also contain PEST (Proline-Glutamic acid-Serine-Threonine) motif to control protein stability (Yin et al., 2005).
BZR1 is a positive regulator of the BR signaling pathway and cooperates with light signal transcription factors to regulate cell elongation and plant growth (Li et al., 2001; Sun et al., 2010). Moreover, BZR1/BES1 positively regulated freezing tolerance by binding to the promoter of genes involved in both CBF-dependent and CBF-independent pathways in Arabidopsis (Li et al., 2017). It was reported that AtBES1 played a negative role in drought responses (Chen and Yin, 2017). On the contrary, TaBZR2 positively regulates drought responses by activation of TaGST1 (Xiao-Yu et al., 2019). Furthermore, TaBZR2 also conferred resistance to wheat stripe rust by activation of chitinase Cht20.2 transcription (Bai et al., 2021).
Wheat (Triticum aestivum L.) is one of the most important food crops, and plays irreplaceable role in feeding the population of the world (Yin et al., 2018). The BZR family genes has been reported in sugar beet (Wang et al., 2019), Brassica rapa (Saha et al., 2015), Legume (Li et al., 2018), Nicotiana benthamiana (Chen et al., 2021), and Pyrus bretschneideri (Cao et al., 2020). However, the information of the evolutionary history and expression patterns of BZRs in wheat is limited. Consequently, in this study, a comprehensive genome-wide analysis was carried out to characterize the BZR family in wheat, and their expression profiles responses to different abiotic and biotic stresses were assessed. The present study will lay a foundation for future functional analysis of BZR genes in wheat, especially in response to abiotic stresses.
The wheat genomic data were downloaded from the International Wheat Genome Sequencing Consortium (IWGSC) RefSeq v1.1 (https://wheat-urgi.versailles.inra.fr/Seq-Repository/Assemblies/). The hidden Markov model file of the BZR protein conserved domain (PF05687.10) was downloaded from the Pfam database (http://pfam.xfam.org/search/). The known BZR protein sequences from rice (http://rice.plantbiology.msu.edu/index.shtml), Arabidopsis thaliana (http://www.arabidopsis.org/index.jsp), and the Markov file PF05687.10 were regarded as queries for BLASTp (e-value < 10-5) against the wheat database IWGSC.v1.1 (Han et al., 2019). The derived wheat BZRs (TaBZRs) sequences were then submitted to the online software Pfam (http://pfam.xfam.org/search/) to eliminate the sequences without intact BES1_N domain, which is conserved in BZR protein. The protein characteristics of TaBZRs, including amino acid length (aa), molecular weight (MW), theoretical isoelectric point (pI), instability index, aliphatic index, and hydrophilic coefficient, were analyzed by ExPASy Server10 (SIB Bioinformatics Resource Portal, https://prosite.expasy.org/PS50011).
The protein sequences of TaBZRs and known BZRs from rice and A. thaliana were collected to construct a Neighbor-joining tree with 1000 replicated-bootstrap in MEGA7 (Kumar et al., 2016). The produced phylogenetic tree was further modified and illustrated by the online tool Tree of Life (version 3.2.317, http://itol.embl.de/) (Zhu et al., 2019).
Gene structure annotations of TaBZRs were extracted from wheat genome annotation file. The conserved motifs of TaBZRs were analyzed by online tools MEME (v4.9.1) (Bailey et al., 2015) with the parameters setting as follows: any number of non-overlapping occurrences of each motif in each sequence; a maximum of 20 different motifs; and motif width ranging from 6 to 100 aa. The integrated image was drawn by TBtools software (Chen et al., 2020). Afterwards, the predicted motifs were analyzed by InterPro (http://www.ebi.ac.uk/interpro) (Hunter et al., 2009; Blum et al., 2021).
The upstream 1500bp regions of initiation codon (ATG) of TaBZR family genes were identified by searching wheat genome data, and were analyzed to predict the cis-regulatory elements using the online program PlantCARE (http://bioinformatics.psb.ugent.be/webtools/plantcare/html/).
Multiple RNA-seq data was downloaded from the NCBI SRA database, and was mapped to the wheat reference genome by Hisat2. Then, the transcripts were calculated by cufflinks. The values of fragments per kilobase of exon model per million mapped reads (FPKM) of TaBZRs were used to construct the heatmap by the R package “pheatmap”. The expression patterns of TaBZR genes under different abiotic stresses in leaf and root were detected by real-time quantitative PCR. The wheat seedlings with the first leaf fully expanded were treated with 100 mmol/L NaCl, 20% polyethylene glycol (PEG 6000), and 100 mmol/L ABA, respectively. For cold and heat treatment, wheat seedlings were put in illumination incubator setting at 4°C and 40°C, respectively. Leaf samples were collected at 0h, 6h, 12h, and 36h after treatment. The samples were immediately froze in liquid nitrogen, and then stored at -80°C for further use. Total RNA was extracted from wheat leaves and roots, and cDNA was synthesized by reverse transcriptase (Vazyme, Nanjing, China) according to the manufacturer’s instruction. Gene specific primers were designed using Primer Premier 5.0 and listed in Table S1. The procedure was carried out as follows: pre-denaturation at 94°C for 3 min, denaturation at 94°C for 10s, primer annealing/extension at 60°C for 30 s and collection of fluorescence signal at 72°C for 40s. The relative gene expression levels were calculated using the 2–ΔΔCt method (Livak and Schmittgen, 2001). All the experiments were performed in triplicates. Ta2291 was set as the reference gene to standardize the expression data (Paolacci et al., 2009).
To identify the wheat BZR genes from the reference genome, we collected 12 known BZR genes from two model plants (six from rice and six from Arabidopsis). After removing the redundant sequences and conserved domain analysis, 20 BZR genes were identified in wheat (Table 1). Interestingly, from the results of conserved domain analysis from Pfam online tool, we found that five genes not only contained BES1_N domain, but also contained Glyco_hydro_14 domain (PF01373) in C-terminals (Table S1). TaBZRs were named according to their chromosome location. As shown in Table 1, TaBZRs were distributed on 15 chromosomes, which exhibits uneven distribution. The protein length was from 178 aa to 686 aa, of which most of the gene lengths were around 300 aa. Accordingly, the molecular weight of protein was between 19.27 KD to 75.47 KD. From Table 1, it is clearly that those genes having two conserved domains have the longer sequence and bigger molecular weight. The pI value was from 5.4 to 9.4, most of which were more than 8, suggesting that most of TaBZRs were basic proteins. Furthermore, the negative GRAVY value indicated that all of the TaBZRs were hydrophilic protein.
To study the phylogenetic relationships of TaBZRs, a Neighbor-joining tree was constructed. As shown in Figure 1, the wheat proteins have closer relationships with proteins from rice. The total of 32 BZR proteins were divided into four groups. All the BZRs in Arabidopsis were clustered into group III and IV, which have more members than group I and II. All of the proteins which have two conserved domains as mentioned above (Tables 1, S1) were clustered in Group I. In addition, the triplet genes in wheat genome have the closely relationships through the phylogenetic tree.
Figure 1 Phylogenetic analysis of protein sequences of BZRs from wheat, rice, and Arabidopsis. The predicted amino acid sequences were aligned by Clustalw2 sequence alignment program and a phylogenetic tree was constructed based on Neighbor-joining method with 1000 bootstrap replicates in MEGA 7 software. BZRs from rice and Arabidopsis were distinguished with black dot and black triangle, respectively.
The distribution and composition of conservative motifs in TaBZRs were predicted by MEME online tool. As shown in Figure 2A, only Motif 1 presents in all TaBZRs. After analysis in Pfam (Table S2), Motif 1 was belonged to BES1_N domain, which is a screening criteria in present study. In addition, Motif 4, 5, and 7 were belonged to Glyco_hydro_14 domain, and only present in Group I, which were consistent with the conserved domain analysis and phylogenetic tree analysis. Furthermore, Motif 2, 10, 18 were also specifically present in Group I. In Group III and Group IV, there are also group-specific motifs: Motif 9 and 16 present in Group III; Motif 13, 15, 17, and 6 present in Group IV. Motif 3 and 8 are present in both Group III and Group IV, which indicates the close relationships of these two groups.
Figure 2 Analysis of conserved motifs, gene structure, and domains in the BZR genes of wheat. (A) Conserved protein domains predicted in TaBZR proteins. The conserved motifs of TaBZRs were identified by MEME tool. Each motif was represented by a colored box. (B) Gene structures of TaBZR members. Exons and introns were represented by green boxes and black lines, respectively. UTR region was represented by green inverted triangle. Conserved domains were represented by yellow (BES1_N) and pink (Glyco_hydro_14).
To study the gene structures of TaBZRs, we analyzed the intron-exon structure by using annotations extracted from GFF3 file and observed that all the studied TaBZRs were interrupted by introns (Figure 2B). As shown in Figure 2B, except for the genes in Group I, which have 7 to 10 introns, the genes in other groups only have one intron. The obvious difference between Group I and other groups is due to Glyco_hydro_14 domain presented in Group I.
In order to study the response of TaBZR genes to various signaling factors, we used the 1.5 kb sequence upstream of its transcription starting position to find different cis-acting elements. A total of 36 cis-acting elements were predicted (Figure 3; Table S3). It is interesting that no cis-acting elements were predicted in the promoter regions of TaBZR4, TaBZR10, TaBZR11, TaBZR14, and TaBZR20, which were belonged to Group I and Group II. These cis-acting elements were clustered into four categories: biotic/abiotic stress (6), growth and development (6), hormones (12), and light response (12). Results showed that the promoter sequences of TaBZR genes have various cis-regulatory elements. In biological/abiotic stress group, the cis-acting element GC-motif and ARE were the most common ones. The largest variety is associated with light response elements, such as GATA-motif, I-box, and G-box, among which G-box is the most abundant cis-acting element related to optical response. The cis-acting element related to abscisic acid (ABRE), auxin (AuxRR), gibberellin (GARE-motif, P-box), salicylic acid (TCA-element), and methyl jasmonate reaction (TGACG-motif), CGTCA-motif, zein metabolism (O2-site), and auxin reaction (TGA-element) were found. Six cis-elements were involved in growth and development group, in which TATA-box were the most.
Figure 3 Cis-Acting elements in the promoters of TaBZRs. The upstream 1500bp regions of TaBZR family genes were analyzed to determine the cis-regulatory elements using the online program PlantCARE and the heatmap was generated by TBtools software.
The original RNA-seq data from multiple conditional transcriptome analysis were downloaded from NCBI SRA database and used to analyze the expression patterns of TaBZR genes (Figure 4; Table S4). Some TaBZRs has alternative splicing isoforms, so all the isoforms were included for transcriptome analysis in present study. From Figure 4, it was clear that the genes with different alternative splicing transcripts were almost un-expressed in the listed conditions. These results indicated that these genes might have been lost functions during evolution. On the contrary, TaBZR5, TaBZR7, and TaBZR9 were expressed at high levels in general in all abiotic treatments. Furthermore, the expression levels of these three genes were sharply up-regulated under heat-drought treatment (Figure 4C) and NaCl application in QM6 and Chinese Spring cultivar (Figure 4D), which indicated that these three genes play important roles in wheat against abiotic stresses especially salt and heat treatment. We also found that some genes play roles in wheat resistance to salt stress, including TaBZR1, 2, 3, 17, 18, 16, and 19, especially TaBZR16 were significantly induced by NaCl application. From the above results, we can conclude that many TaBZR geneshave specific functions in response to salt, heat, and drought stresses.
Figure 4 Expression patterns of TaBZRs under different abiotic treatments. The transcriptome raw data was downloaded from NCBI SRA database. The FPKM values were transformed by log2. The raw data of TaBZRs genes is provided in Table S3. (A) The expression patterns of TaBZRs in different tissues. (B) The expression patterns of TaBZRs after drought treatment. (C) The expression patterns of TaBZRs after drought and heat treatments. (D) The expression patterns of TaBZRs in two different cultivars after NaCl treatment.
The expression patterns of TaBZRs against stripe rust infection in different time points were analyzed (Figure 5; Table S5). Figure 5 shows the expression levels of TaBZRs under wheat stripe rust infection. Similar to the previous results, the genes with lower or none expression levels were still not induced by stripe rust, which solidified the above hypothesis of loss of function. The expression levels of TaBZR5, 7, 9 were sharply rose at the first day post stripe rust inoculation, which indicated that these three genes might play important roles during the earlier stage of the interaction between wheat and stripe rust. On the contrary, the expression of TaBZR11 was depressed at the first day, and was induced afterwards. And the expression level of TaBZR11 reached the highest at 9th day, which illustrated that TaBZR11 might have an effect at the later stage of the wheat-stripe rust interaction.
Figure 5 Expression patterns of TaBZRs after stripe rust fungus infection. The transcriptome raw data was downloaded from NCBI SRA database. The expression levels of TaBZR genes were provided in Table S5.
BZR transcription factors play an essential role in stress responses (Wang et al., 2002). According to the RNA-seq data, the genes (TaBZR5, TaBZR7, TaBZR9, and TaBZR16) with high expression levels were screened out, among which TaBZR5, TaBZR7, and TaBZR9 are triplet genes, which may have similar functions. Therefore, we further analyzed the expression patterns of TaBZR5 and TaBZR16 in five different abiotic stresses using qRT-PCR. The expression level of TaBZR5 was significantly up-regulated in various conditions except for PEG treatment (Figure 6). In addition, the expression level of TaBZR5 reached the peak at 6h after heat treatment, while the expression level achieved the top at 12h after cold and ABA treatment and at 36h under salt stress. However, TaBZR16 showed different expression patterns. The expression was obviously induced after PEG treatment, while the expression levels were not obviously up-regulated after spraying ABA. These results indicated that TaBZR5 and TaBZR16 may play different roles and in different pathways in wheat response to cold, PEG, heat, and ABA treatments. However, similar to TaBZR5, the expression level reached the peak at 36h after salt treatment, which indicated that both TaBZR5 and TaBZR16 possibly participated in salt treatment at the same time. In conclusion, the above results indicated that members of TaBZRs gene family have differentiated their functions to adapt to different stresses in wheat during the process of evolution.
Figure 6 qRT-PCR analysis of expressions of TaBZR5 and TaBZR16 under five different abiotic treatments. The treatments include Cold, NaCl, PEG, Heat, and ABA spraying. Then the wheat leaves were sampled at 0h, 6h, 12h, and 36h after treatments. The expression values were calculated by 2–ΔΔCt method. This experiment was carried out with three independent biological repetitions and three technical repetitions in each biological repetition.
BR is a plant-specific steroid hormone that manifests a variety of stress-resistance functions and various growth-regulating effects (Lee et al., 2018). Moreover, BR signal transduction system plays an important role in plant growth and development (Kir et al., 2015). BZR transcription factors play indispensable roles in the connections between BR and other signaling pathways (Lee et al., 2018; Cui et al., 2019). It was reported that PhBEH2 might an important hub for the crosstalk between signaling pathways in Petunia hybrida (Verhoef et al., 2013). However, limit genome-wide studies were performed on the BZR TF family in wheat. Identification and characterization of BZR TF family members in wheat are important starting points for exploring this gene family’s function in monocots. In this study, we used bioinformatics methods to analyze the BZR family in wheat and identified 20 TaBZRs (Table 1). Furthermore, the phylogenetic tree, gene structure, conserved motifs, and transcriptome data were analyzed. Our results will lay a foundation for further functional analysis of TaBZRs families.
It was reported that TaBZR2 (TaBZR9 in present study) acts as a positive regulator in drought responses by activating TaGST1 and mediates the crosstalk between BR and drought signaling pathways (Xiao-Yu et al., 2019). In present study, by analyzing transcriptome data, we found that TaBZR9 not only participated in response to drought stress, but also play important roles in wheat against salt treatment (Figure 4). Furthermore, recently, Bai et al. (2021) reported that TaBZR2 (TaBZR9 in present study) conferred broad-spectrum resistance to stripe rust fungus by activating a chitinase gene TaCht20.2 transcriptions. In present study, we also found that TaBZR5, 7, and 9 were up-regulated after stripe rust infection (Figure 5). Therefore, we can speculate that TaBZR9 might play as a hub in wheat response to various biotic and abiotic conditions, and should be given priority in further functional researches.
The expression levels of TaBZR11 were down-regulated at 6 hours after heat and drought treatments (Figure 4C), which is opposite with that of TaBZR9. These results indicated that TaBZR11 may play a negative role in regulating wheat against heat and drought stresses. It is interesting that TaBZR11 contains two conserved domains as mentioned before. While it is not clear whether BES1_N domain have the primary functions in these conditions. We also find out an interesting gene, TaBZR16, which was significantly upregulated under NaCl application (Figure 4), while not expressed during wheat-stripe rust fungus interaction (Figure 5). From this result, we can speculate that TaBZR16 specifically participated in wheat against NaCl treatment. Furthermore, it can be concluded that BZR genes in wheat play different roles in response to various stresses.
In this study, we performed a comprehensive genome-wide analysis of 20 wheat BZR gene characteristics, intron-exon structure, genomic distribution, conserved motifs, phylogeny, and expression analysis. At present, a large number of BZR genes in rice, maize, and Arabidopsis plants provide important information for the study of BZR genes in monocotyledonous wheat. The results of this study will lay a foundation for further in-depth functional studies of TaBZRs and will provide information for the breeding and genetic improvement of wheat against drought and salt stresses.
The original contributions presented in the study are included in the article/Supplementary Material. Further inquiries can be directed to the corresponding author.
YC designed this article; PZ, HY, and YL directed the data analysis and manuscript writing. All authors contributed to the article and approved the submitted version.
This work was supported by “Open Project Program of Engineering Research Center of Ecology and Agricultural Use of Wetland, Ministry of Education (KFT202103)”.
We thank Yongli Qiao (College of Life Sciences, Shanghai Normal University) for his guidance and wonderful suggestions for our manuscript.
The authors declare that the research was conducted in the absence of any commercial or financial relationships that could be construed as a potential conflict of interest.
All claims expressed in this article are solely those of the authors and do not necessarily represent those of their affiliated organizations, or those of the publisher, the editors and the reviewers. Any product that may be evaluated in this article, or claim that may be made by its manufacturer, is not guaranteed or endorsed by the publisher.
The Supplementary Material for this article can be found online at: https://www.frontiersin.org/articles/10.3389/fpls.2023.1144379/full#supplementary-material
Supplementary Table 3 | Cis-Acting elements in the promoters of TaBZRs.
Agarwal, P. K., Agarwal, P., Reddy, M. K., Sopory, S. K. (2006). Role of DREB transcription factors in abiotic and biotic stress tolerance in plants. Plant Cell Rep. 25 (12), 1263–1274. doi: 10.1007/s00299-006-0204-8
Ahammed, G. J., Li, X., Liu, A., Chen, S. (2020). Brassinosteroids in plant tolerance to abiotic stress. J. Plant Growth Regul. 39, 1451–1464. doi: 10.1007/s00344-020-10098-0
Bai, X. X., Zhan, G. M., Tian, S. X., Peng, H., Cui, X. Y., Islam, M. A., et al. (2021). Transcription factor BZR2 activates chitinase Cht20.2 transcription to confer resistance to wheat stripe rust. Plant Physiol. 187 (4), 2749–2762. doi: 10.1093/plphys/kiab383
Bailey, T. L., Johnson, J., Grant, C. E., Noble, W. S. (2015). The MEME suite. Nucleic Acids Res. 43 (W1), W39–W49. doi: 10.1093/nar/gkv416
Blum, M., Chang, H. Y., Chuguransky, S., Grego, T., Kandasaamy, S., Mitchell, A., et al. (2021). The InterPro protein families and domains database: 20 years on. Nucleic Acids Res. 49 (D1), D344–D354. doi: 10.1093/nar/gkaa977
Cao, Y. P., Meng, D. D., Li, X. X., Wang, L. H., Cai, Y. P., Jiang, L. (2020). A Chinese white pear (Pyrus bretschneideri) BZR gene PbBZR1 act as a transcriptional repressor of lignin biosynthetic genes in fruits. Front. Plant Sci. 11. doi: 10.3389/fpls.2020.01087
Chen, C., Chen, H., Zhang, Y., Thomas, H. R., Frank, M. H., He, Y. H., et al. (2020). TBtools: an integrative toolkit developed for interactive analyses of big biological data. Mol. Plant 13 (8), 1194–1202. doi: 10.1016/j.molp.2020.06.009
Chen, X. W., Wu, X. Y., Qiu, S. Y., Zheng, H. Y., Lu, Y. W., Peng, J. J., et al. (2021). Genome-wide identification and expression profiling of the BZR transcription factor gene family in Nicotiana benthamiana. Int. J. Mol. Sci. 22 (19), 10379. doi: 10.3390/ijms221910379
Chen, J., Yin, Y. (2017). WRKY transcription factors are involved in brassinosteroid signaling and mediate the crosstalk between plant growth and drought tolerance. Plant Signal. Behav. 12 (11), e1365212. doi: 10.1080/15592324.2017.1365212
Clouse, S. D., Sasse, J. M. (1998). BRASSINOSTEROIDS: essential regulators of plant growth and development. Annu. Rev. Plant Physiol. Plant Mol. Biol. 49, 427–451. doi: 10.1146/annurev.arplant.49.1.427
Cui, X. Y., Gao, Y., Guo, J., Yu, T. F., Zheng, W. J., Liu, Y. W., et al. (2019). BES/BZR transcription factor TaBZR2 positively regulates drought responses by activation of TaGST1. Plant Physiol. 180 (1), 605–620. doi: 10.1104/pp.19.00100
Fang, Z. W., He, Y. Q., Liu, Y. K., Jiang, W. Q., Song, J. H., Wang, S. P., et al. (2020). Bioinformatic identification and analyses of the non-specific lipid transfer proteins in wheat. J. Integr. Agr. 19 (5), 1170–1185. doi: 10.1016/S2095-3119(19)62776-0
Han, Z., Liu, Y., Deng, X., Liu, D., Liu, Y., Hu, Y., et al. (2019). Genome-wide identification and expression analysis of expansin gene family in common wheat (Triticum aestivum L.). BMC Genomics 20 (1), 101. doi: 10.1186/s12864-019-5455-1
He, J. X., Gendron, J. M., Sun, Y., Gampala, S. S. L., Gendron, N., Catherine, Q. S., et al. (2005). BZR1 is a transcriptional repressor with dual roles in brassinosteroid homeostasis and growth responses. Science 307 (5715), 1634–1638. doi: 10.1126/science.1107580
Hu, D., Wei, L., Liao, W. (2021). Brassinosteroids in plants: crosstalk with small-molecule compounds. Biomolecules 11 (12), 1800. doi: 10.3390/biom11121800
Hunter, S., Apweiler, R., Attwood, T. K., Bairoch, A., Bateman, A., Binns, D. (2009). InterPro: the integrative protein signature database. Nucleic Acids Res. 37 (Database issue), D211–D215. doi: 10.1093/nar/gkn785
Kir, G., Ye, H. X., Nelissen, H., Neelakandan, A. K., Kusnandar, A. S., Luo, A. D., et al. (2015). RNA Interference knockdown of BRASSINOSTEROID INSENSITIVE1 in maize reveals novel functions for brassinosteroid signaling in controlling plant architecture. Plant Physiol. 169 (1), 826–839. doi: 10.1104/pp.15.00367
Kumar, S., Stecher, G., Tamura, K. (2016). MEGA7: molecular evolutionary genetics analysis version 7.0 for bigger datasets. Mol. Biol. Evol. 33 (7), 1870–1874. doi: 10.1093/molbev/msw054
Lee, J., Shim, D., Moon, S., Kim, H., Bae, W., Kim, K. (2018). Genome-wide transcriptomic analysis of BR-deficient micro-tom reveals correlations between drought stress tolerance and brassinosteroid signaling in tomato. Plant Physiol. Biochem. 127, 553–560. doi: 10.1016/j.plaphy.2018.04.031
Li, Y., He, L., Li, J., Chen, J., Liu, C. (2018). Genome-wide identification, characterization, and expression profiling of the legume BZR transcription factor gene family. Front. Plant Sci. 9, 1332. doi: 10.3389/fpls.2018.01332
Li, Q. F., Lu, J., Yu, J. W., Zhang, C. Q., He, J. X., Liu, Q. Q. (2018). The brassinosteroid-regulated transcription factors BZR1/BES1 function as a coordinator in multisignal-regulated plant growth. Biochim. Biophys. Acta Gene Regul. Mech. 1861 (6), 561–571. doi: 10.1016/j.bbagrm.2018.04.003
Li, J. M., Nam, K. H., Vafeados, D., Chory, J. (2001). BIN2, a new brassinosteroid-insensitive locus in Arabidopsis. Plant Physiol. 127 (1), 14–22. doi: 10.1104/pp.127.1.14
Li, H., Ye, K. Y., Shi, Y. T., Cheng, J. K., Zhang, X. Y., Yang, S. H. (2017). BZR1 positively regulates freezing tolerance via CBF-dependent and CBF-independent pathways in Arabidopsis. Mol. Plant 10 (4), 545–559. doi: 10.1016/j.molp.2017.01.004
Livak, K. J., Schmittgen, T. D. (2001). Analysis of relative gene expression data using real-time quantitative PCR and the 2–ΔΔCT method. Methods 25 (4), 402–408. doi: 10.1006/meth.2001.1262
Miyaji, T., Yamagami, A., Kume, N., Sakuta, M., Osada, H., Asami, T., et al. (2014). Brassinosteroid-related transcription factor BIL1/BZR1 increases plant resistance to insect feeding. Biosci. Biotechnol. Biochem. 78 (6), 960–968. doi: 10.1080/09168451.2014.910093
Nolan, T. M., Vukašinović, N., Liu, D., Russinova, E., Yin, Y. (2020). Brassinosteroids: multidimensional regulators of plant growth, development, and stress responses. Plant Cell 32 (2), 295–318. doi: 10.1105/tpc.19.00335
Paolacci, A. R., Tanzarella, O. A., Porceddu, E., Ciaffi, M. (2009). Identification and validation of reference genes for quantitative RT-PCR normalization in wheat. BMC Mol. Biol. 10, 11. doi: 10.1186/1471-2199-10-11
Saha, G., Park, J. I., Jung, H. J., Ahmed, N. U., Kayum, M. A., Kang, J. G., et al. (2015). Molecular characterization of BZR transcription factor family and abiotic stress induced expression profiling in Brassica rapa. Plant Physiol. Biochem. 92, 92–104. doi: 10.1016/j.plaphy.2015.04.013
Schaller, H. (2003). The role of sterols in plant growth and development. Prog. Lipid Res. 42 (3), 163–175. doi: 10.1016/s0163-7827(02)00047-4
Singh, A. P., Fridman, Y., Friedlander-Shani, L., Tarkowska, D., Strnad, M., Savaldi-Goldstein, S. (2014). Activity of the brassinosteroid transcription factors brassinazole Resistant1 and brassinosteroid Insensitive1-ethyl methanesulfonate-Suppressor1/Brassinazole Resistant2 blocks developmental reprogramming in response to low phosphate availability. Plant Physiol. 166 (2), 678–688. doi: 10.1104/pp.114.245019
Sun, Y., Fan, X. Y., Cao, D. M., Tang, W., He, K., Zhu, J. Y., et al. (2010). Integration of brassinosteroid signal transduction with the transcription network for plant growth regulation in Arabidopsis. Dev. Cell 19 (5), 765–777. doi: 10.1016/j.devcel.2010.10.010
Vardhini, B. V., Anjum, N. A. (2015). Brassinosteroids make plant life easier under abiotic stresses mainly by modulating major components of antioxidant defense system. Front. Environ. Sci. 2. doi: 10.3389/fenvs.2014.00067
Verhoef, N., Yokota, T., Shibata, K., Boer, G. J., Gerats, T., Vandenbussche, M., et al. (2013). Brassinosteroid biosynthesis and signalling in petunia hybrida. J. Exp. Bot. 64 (8), 2435–2448. doi: 10.1093/jxb/ert102
Wang, Z. Y., Nakano, T., Gendron, J., He, J., Chen, M., Vafeados, D., et al. (2002). Nuclear-localized BZR1 mediates brassinosteroid-induced growth and feedback suppression of brassinosteroid biosynthesis. Dev. Cell 2 (4), 505–513. doi: 10.1016/s1534-5807(02)00153-3
Wang, W., Sun, Y. Q., Li, G. L., Zhang, S. Y. (2019). Genome-wide identification, characterization, and expression patterns of the BZR transcription factor family in sugar beet (Beta vulgaris l.). BMC Plant Biol. 19 (1), 191. doi: 10.1186/s12870-019-1783-1
Yin, J. L., Fang, Z. W., Sun, C., Zhang, P., Zhang, X., Lu, C., et al. (2018). Rapid identification of a stripe rust resistant gene in a space-induced wheat mutant using specific locus amplified fragment (SLAF) sequencing. Sci. Rep. 8 (1), 3086. doi: 10.1038/s41598-018-21489-5
Yin, Y., Vafeados, D., Tao, Y., Yoshida, S., Asami, T., Chory, J. (2005). A new class of transcription factors mediates brassinosteroid-regulated gene expression in Arabidopsis. Cell 120 (2), 249–259. doi: 10.1016/j.cell.2004.11.044
Yu, H. Q., Sun, F. A., Feng, W. Q., Lu, F. Z., Li, W. C., Fu, F. L. (2019). The BES1/BZR1 transcription factors regulate growth, development and stress resistance in plants. Yi Chuan. 41 (3), 206–214. doi: 10.16288/j.yczz.18-253
Keywords: TaBZRs, bioinformatics, phylogenetic analysis, group specificity, stripe rust
Citation: Zhang P, Yan H, Liu Y and Chai Y (2023) Genome-wide identification and functional characterization of wheat Brassinazole-resistant transcription factors in response to abiotic stresses and stripe rust infection. Front. Plant Sci. 14:1144379. doi: 10.3389/fpls.2023.1144379
Received: 14 January 2023; Accepted: 10 May 2023;
Published: 13 June 2023.
Edited by:
Ligeng Ma, Capital Normal University, ChinaReviewed by:
Huan Luo, Chungnam National University, Republic of KoreaCopyright © 2023 Zhang, Yan, Liu and Chai. This is an open-access article distributed under the terms of the Creative Commons Attribution License (CC BY). The use, distribution or reproduction in other forums is permitted, provided the original author(s) and the copyright owner(s) are credited and that the original publication in this journal is cited, in accordance with accepted academic practice. No use, distribution or reproduction is permitted which does not comply with these terms.
*Correspondence: Yi Chai, Y2hhaXlpMTIzNDU2QDEyNi5jb20=
†These authors have contributed equally to this work
Disclaimer: All claims expressed in this article are solely those of the authors and do not necessarily represent those of their affiliated organizations, or those of the publisher, the editors and the reviewers. Any product that may be evaluated in this article or claim that may be made by its manufacturer is not guaranteed or endorsed by the publisher.
Research integrity at Frontiers
Learn more about the work of our research integrity team to safeguard the quality of each article we publish.